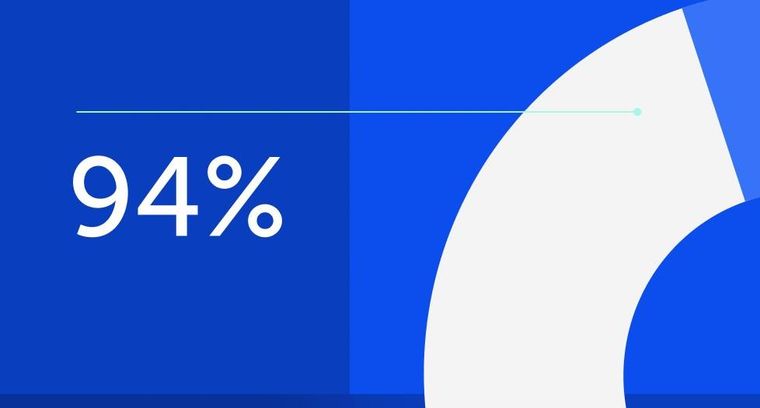
94% of researchers rate our articles as excellent or good
Learn more about the work of our research integrity team to safeguard the quality of each article we publish.
Find out more
ORIGINAL RESEARCH article
Front. Genet., 27 September 2021
Sec. Livestock Genomics
Volume 12 - 2021 | https://doi.org/10.3389/fgene.2021.670390
This article is part of the Research TopicPhenotypic Characterization, Genetics and Genomics of Livestock in Low Input SystemsView all 16 articles
The genomes of crossbred (admixed) individuals are a mosaic of ancestral haplotypes formed by recombination in each generation. The proportion of these ancestral haplotypes in certain genomic regions can be responsible for either susceptibility or tolerance against pathogens, and for performances in production traits. Using a medium-density genomic marker panel from the Illumina Bovine SNP50 BeadChip, we estimated individual admixture proportions for Baoulé x Zebu crossbred cattle in Burkina Faso, which were tested for trypanosome infection by direct ELISA from blood samples. Furthermore, we calculated local ancestry deviation from average for each SNP across 29 autosomes to identify potential regions under selection in the trypanotolerant Baoulé cattle and their crossbreds. We identified significant deviation from the local average ancestry (above 5 and 10% genome-wide thresholds) on chromosomes 8 and 19 in the positive animals, while the negative ones showed higher deviation on chromosomes 6, 19, 21, and 22. Some candidate genes on chromosome 6 (PDGFRA) and chromosome 19 (CDC6) have been found associated to trypanotolerance in West African taurines. Screening for FST outliers in trypanosome positive/negative animals we detected seven variants putatively under selection. Finally, we identified a minimum set of highly ancestry informative markers for routine admixture testing. The results of this study contribute to a better understanding of the genetic basis of trypanotolerance in Baoulé cattle and their crossbreeds. Furthermore, we provide a small informative marker set to monitor admixture in this valuable indigenous breed. As such, our results are important for conserving the genetic uniqueness and trypanotolerance of Baoulé cattle, as well as for the improvement of Baoulé and Zebu crossbreds in specific community-based breeding programs.
The Bos taurus taurus and Bos taurus indicus subspecies are the two most important cattle types in West Africa (Belemsaga et al., 2005; Okeyo Mwai et al., 2015). These animals have been raised in complex social and political processes, and they have adapted to harsh environmental conditions over the centuries (Dueppen, 2012). The adaptive traits include the tolerance to diseases and drought, ability to walk long distances, and capacity to survive on poor pastures (Okeyo Mwai et al., 2015). Yet, this valuable diversity is increasingly threatened by genetic dilution due to changes in production systems, livestock herders’ preferences for specific breeds and/or traits, market conditions and other opportunities (Hanotte et al., 2010). The taurine cattle, more specifically the Baoulé cattle have existed in the tsetse fly (Glossina spp.) challenged zones for long and therefore acquired trypanotolerance, an immunology phenomenon that has a genetic basis (Naessens et al., 2002; Agyemang, 2005). These animals have a capacity to rid themselves of trypanosome parasites and maintain low parasitemia. Thus, trypanotolerant animals have been introduced in other tsetse affected countries of Africa to make use of their genetic advantage in purebred populations or crossed to other types like Zebu. Several studies revealed admixture among the taurine and Zebu subspecies (Hanotte, 2002; Freeman et al., 2004; Flori et al., 2014) as the result of the continuous genetic flow that occurs every year during seasonal cross-border livestock movements from the drier Sahelian zones in the north to the more humid zones in the south of West and East Africa.
The south-western region of Burkina Faso is the original habitat of Baoulé cattle. In this area, production systems are mixed crop-livestock and agroforestry, with the Lobi ethnic group concentrating on subsistence crop production while the transhumant people tend to keep their lifestyle of pastoral livestock production. The cattle population in this region is estimated at 343,000 heads, representing about 4% of the estimated national stock of 9 million according to the Ministry of Animal Resources (Ministere des ressources animales, 2014). The livestock system is extensive in all studied departments (Zoma et al., 2020), with 7 to 100 cattle per farmers. The indigenous Baoulé cattle, despite its small size and lower growth rate, is well adapted to the local environment of West Africa. It has gained cultural importance due to its social roles and tolerance to trypanosomiasis (Zoma et al., 2020). However, the continued crossbreeding with Zebu cattle because of its large size threatens the integrity of the Baoulé breed (Yougbaré et al., 2020). Recently, community-based breeding programs have been implemented in the south-western region of Burkina Faso to conserve and improve the local genetic resources of the indigenous Baoulé cattle as well as Baoulé x Zebu advanced crosses (Ouédraogo et al., 2020; Zoma et al., 2020).
Since the advent of high-throughput single nucleotide polymorphism (SNP) genotyping, inferring selection signatures from differences in local admixture levels has received considerable attention in human genetics (Tang et al., 2007; Jin et al., 2012; Bhatia et al., 2014). Similar studies in livestock investigated local ancestry levels of New World Creole cattle (Gautier and Naves, 2011; Flori et al., 2014; Pitt et al., 2018) and selection signatures in dairy cattle in East Africa, resulting from admixture of European breeds (Kim and Rothschild, 2014), as well as in East African short horn Zebu (Bahbahani et al., 2015).
The genomic ancestry proportions between trypano-susceptible indicine Zebu and the trypano-tolerant taurine Baoulé cattle in Burkina Faso were assessed based on microsatellites and 155 SNPs in 23 candidate regions (Smetko et al., 2015). In this study, we followed up and extended on the previous work using dense genomic marker data. Our study aimed to estimate the individual local ancestry proportions for each SNP to identify potential regions under selection in Baoulé x Zebu crossbreds tested positive or negative for trypanosomosis and finding a small set of ancestry informative SNP for routine admixture testing. Estimating the proportional contributions of ancestral populations in admixed (crossbred) individuals is important to clarify the population structure, historical background, and pattern of admixture along the genome of admixed individuals.
This study was carried out in the province of Poni in the South-western administrative region of Burkina Faso. We selected three study sites with different management and breeding systems of Baoulé cattle and Baoulé x shorthorn Zebu crossbreds, including 27 villages from the Bouroum-Bouroum, Kampti and Loropéni departments (Figure 1). In the Bouroum-Bouroum department, we worked with 55 sedentary farmers of the ethnic group of Lobi, who keep purebred Baoulé and are the owners of these animals. In Kampti, we included 18 farms with mostly Baoulé x Zebu crossbreds and some pure Baoulé owned by the Mossi ethnic group, but herded by the transhumant Fulani people. Finally, in Loropéni 15 farms were included mainly with crossbred animals kept by Lobi and Djan breeders. As all samples were collected within close geographic distances (< 50 km) in the tsetse infested province of Poni, it is reasonable to assume that all individuals were exposed to the same trypanosome infection challenge.
A total of 737 blood samples, including 387 Baoulé and Baoulé x Zebu crossbreds from Bouroum-Bouroum, and 350 crossbreeds from Kampti and Loropéni were collected in EDTA tubes during the health monitoring activities of the “Characterization and Sustainable Utilization of Local Cattle Breeds” (LoCaBreed) project. DNA extraction from EDTA blood samples was performed with the MasterPureTM DNA Purification Kit for Blood Version II (Biozym Scientific, Oldendorf, Germany) following the manufacturer’s protocol. The trypanosomosis status was recorded by indirect ELISA test to diagnose positive or negative trypanosome infection in the blood samples (Desquesnes et al., 2003) resulting in a total of 377 positive and 360 negative animals (Table 1).
The genotyping of the 737 DNA samples with the Illumina Bovine SNP50 BeadChip was performed at Neogen (Lincoln, United States). Additional genotypes from 30 purebred Zebu and 35 crossbreds (Pérez et al., 2014) were included in the study to represent the two ancestral populations (Baoulé and Zebu) and to increase the number of crossbreds, summing to a total of 802 animals. Stringent quality filtering of the data was performed with PLINK 1.9 (Purcell et al., 2007; Chang et al., 2015). Specifically, the dataset was controlled to exclude non-autosomal SNPs, and SNPs with a minor allele frequency (MAF) lower than 0.05, a call rate less than 90% and those that deviated from Hardy Weinberg equilibrium with Fisher’s exact test with P-value 1 × 10 − 6. After quality control, 28,034 SNPs and 776 animals were available for subsequent analyses.
Unsupervised global ancestry estimation was performed with the full set of quality controlled SNPs using ADMIXTURE software (Alexander et al., 2009) with the number of ancestral populations (Baoulé and Zebu) fixed at two (K = 2). The admixture bar plots for ancestry proportions were created in R with the barplot function (The R Development Core Team, 2020). We calculated the frequencies of the admixture levels for all animals in Excel and plotted them in categories of 0.1 steps. We assigned 30 purebred Baoulé (global admixture levels ≥ 0.999 Baoulé) and 30 purebred Zebu (global admixture levels ≥ 0.987) as reference populations to investigate local admixture levels in 716 animals that were considered as potential crossbreds based on the sampling information. Animals found to be purebred (global admixture levels ≥ 0.995 Baoulé) were removed from the pool of crossbreds.
Local Ancestry in Mixed Populations (LAMP) is a program for estimating locus-specific ancestries in admixed individuals, using allele frequencies of the reference populations (Sankararaman et al., 2008). We applied the LAMP-ANC mode implemented in LAMP and provided the estimated allele frequencies files for Baoulé and Zebu as the purebred ancestral populations. LAMP-ANC is a modification of the LAMP mode and shows higher accuracy allowing triple mixing to be estimated, while LAMP cannot determine frequencies for more than two ancestral populations (Sankararaman et al., 2008). The following parameters were set: admixture proportions (alpha) = 0.8 for Baoulé and ≥ 0.2 for Zebu based on the global ancestry estimation using ADMIXTURE program, number of generations since admixture (g) = 2 and recombination rate (r) = 10–8. We estimated the local ancestry proportion, as well as the “delta ancestry” with R in trypanosome positive and negative trypanosomosis animals following Khayatzadeh et al. (2016) using a custom script (see section “Data Availability”). The “delta ancestry” reflects the extreme fluctuations in ancestry differences across the genome, which are calculated by subtracting the genome-wide ancestry from locus-specific ancestry for each ancestry component. Such extreme fluctuations in ancestry differences are unlikely to have occurred by random genetic drift and potentially exhibit a selection signature in the admixed individuals (Tang et al., 2007). To identify significant deviations from the genome-wide average ancestry, we performed permutation tests (Doerge and Churchill, 1996) of the local ancestry proportions over the whole genome of admixed animals as proposed and carried out by Tang et al. (2007) in an admixed human population (Puerto Ricans) and replicated by Gautier and Naves (2011), Flori et al. (2014) in African Taurine, and Khayatzadeh et al. (2016) in composite cattle breed (Swiss Fleckvieh) to find significant thresholds for the deviations of local genetic ancestries from global ancestries. Separating animals with positive and negative trypanosomosis status, for each animal we concatenated the local ancestry estimations of all 29 autosomes and then permuted the circularized genome by cutting at a random location and rearranging the two resulting pieces of the genome for each individual independently. This type of permutation largely preserves the extent of Linkage Disequilibrium (LD), assuming that it is homogeneously distributed over the whole genome. We implemented 1,000 permutations. The distributions of maximum and minimum over all permutations were then used to define the 5 and 10% genome-wide thresholds levels that indicated significant deviation of the observed local ancestries from the genome-wide average ancestry (Tang et al., 2007; Gautier and Naves, 2011; Khayatzadeh et al., 2016).
We applied BayeScan 2.1 (Foll and Gaggiotti, 2008) to identify FST outlier loci putatively under selection between the trypanosome positive (n = 244) and negative (n = 266) crossbred animals using a cut-off at p < 0.05 corrected for a false discovery rate [FDR; (Benjamini and Hochberg, 1995)]. BayeScan uses a Reversible Jump Markov Chain Monte Carlo (RJ-MCMC) algorithm to obtain posterior distributions, with 100,000 iterations and a Burn-in length of 50,000 iterations. The regions within ± 0.5 Mb of the most significant SNPs were searched for any potential associated genes based on the ARS UCD1.2 Bos Taurus Genome Assembly on the NCBI database.
We aimed to identify SNPs with the highest FST differentiation between the 30 pure Baoulé and 30 pure Zebu. We re-filtered the original dataset for MAF < 10%, individual and genotype missingness < 10%, respectively. The FST values were calculated in PLINK following Weir and Cockerham (1984). With these we were able to provide a set of top 200 FST markers, which were then used as a starting point to manually remove markers less than 5 Mb to each other—preference given to higher FST markers. Based on this, we selected the top 15, 25, 50, and 100 SNPs, and extracted these for the crossbred animals and repeated the global admixture analysis (K = 2). We used the cor function in R to calculate the Pearson correlation coefficient (Pearson’s r) for pairwise determining the linear association between admixture levels (ancestry proportion) estimated based on the different sets of ancestry informative SNPs [all SNPs (35,952 SNPs) versus the top 100, 50, 25, 15 SNPs]. Significance of the Pearson’s r was assessed with the P-value from the Pearson Correlation Coefficient Calculator (Social Science Statistics, 2021).
The individual admixture proportions using the full set of SNPs were estimated for all pure and admixed animals and are presented in Figure 2. The distribution of the global admixture proportions for the 802 animals is presented in Figure 3. Notably, we detected 91 cattle with a Baoulé ancestry > 0.995 among the presumed crossbreds, which we excluded from the subsequent analysis of local ancestry in admixed animals.
Figure 2. Global Admixture in Baoulé—Zebu crossbred cattle with the quality-filtered set of 28,034 single nucleotide polymorphisms (SNPs).
Figure 3. Distribution of the global admixture proportions for 802 animals with the full set of 31,612 single nucleotide polymorphisms (SNPs).
The average ancestry estimation for every single SNP was performed across 29 autosomes for trypanosome positive and negative Baoulé x Zebu crossbreds, respectively. The permutation tests over all chromosomes indicated significant local ancestry deviation from the average (above the 5 and 10% genome-wide thresholds) in chromosomes 8 and 19 for trypanosome positive crossbreds (Figure 4), and in chromosomes 6, 19, 21, and 22 for trypanosome negative animals, respectively (Figure 5). The local admixture proportions for these chromosomes are presented in Figure 6 and for all other chromosomes in Supplementary Figures 1, 2. We further visualized the deviations from the average ancestry in the respective chromosomes and identified regions of higher delta ancestry (wide peaks) on chromosome 8 between 35–50 Mb and in chromosome 21 between 20–35 Mb and 40–50 Mb, respectively (Figure 7). These genomic regions might harbor candidate genes associated to tolerance or susceptibility of trypanosomosis.
Figure 4. Local ancestry deviations based on the permutation threshold for the 244 positive crossbreds animals. Orange and blue lines signify the 5 and 10% genome-wide threshold.
Figure 5. Local ancestry deviations based on the permutation threshold for the 266 negative crossbreds animals. Orange and blue lines signify the 5 and 10% genome-wide threshold.
Figure 6. Individual admixture proportions across chromosomes 6, 8, 19, 21, and 22 for the 244 trypanosomose positive and 266 negative crossbreds as determined by LAMP.
Figure 7. The delta ancestry across chromosomes 6, 8, 19, 21, and 22 for the 244 positive and 266 negative crossbreds trypanosomosis status. The red line shows the deviation.
We screened the genomes of the Baoule and Zebu crossbred animals for outlier SNPs with high FST values and disregarded the pure-bred Baulé and Zebu. Among these crossbred animals we grouped them in trypanosome positive and negative animals to avoid detection of breed differences unrelated to trypanosome tolerance. We detected seven variants with a FDR corrected threshold of p < 0.05 (Figure 8). The seven outlier SNPs with the highest levels of FST values were found in chromosomes 2, 3, 5, 20, 21, and 23, and are presented in Table 2, together with their neighboring genes. The positions of the SNPs were not located in regions with higher delta ancestry.
Figure 8. FST outliers between trypanosome positive and negative crossbreds. The vertical line shows the FDR corrected cut-off (p < 0.05); the outlier SNP names and positions are provided in Table 2.
To reliably detect hybrids even with a small set of SNPs applicable for routine genetic monitoring, we selected the most ancestry informative markers resulting in the highest differentiation between Baoulé and Zebu cattle. The 100 SNPs with the highest divergence presented FST values ranging between 0.98 and 0.79 (Supplementary Table 1). We estimated admixture levels of the crossbred individuals using the top 15, 25, 50, and 100 SNPs (Supplementary Figure 5). The Pearson correlation coefficients r between the levels of admixture obtained with the full dataset of 35,952 SNPs and each of the sets of top SNPs were generally high and ranged between 0.949 (allSNPs/top15) and 0.990 (allSNPs/top100) (Table 3). All Pearson correlation coefficients were statistically significant (p < 0.001).
Table 3. Pearson’s correlation coefficient matrix displaying r2 values between the levels of admixture using the most ancestry informative markers compared to the full data set of 35,952 SNPs (allSNPs).
The high amounts of global admixture observed in the taurine cattle population in the three studied departments of Burkina Faso indicated mixed genetic backgrounds of the cattle in Bouroum-Bouroum, Kampti and Loropeni (Figures 2, 3). The observed admixture levels within the departments are likely due to unrestricted mating among cattle of different genetic backgrounds. Long-distance migrations within and across countries, utilization of communal pastures, exchange of breeding animals, and uncontrolled mating facilitate constant gene flow. Houessou et al. (2019) explained this situation by lack of selection and high levels of gene flow due to cyclical cross-border cattle herd movements known as “transhumance” and extensive commercial transactions of cattle in the West African region.
The uncontrolled mating in extensive production systems, which are typically practiced in West Africa, can lead to the introgression of Zebu genes in the small taurine cattle population, which represents a threat to their genetic integrity (Dossa and Vanvanhossou, 2016), and might lead to a potential dilution of their trypanotolerance (Traoré et al., 2015; Albert et al., 2019). The increasing importance of Zebu in the South-western region of Burkina Faso might endanger Baoulé cattle in the long term. As the North, which is the preferred area for Zebu cattle, is hit by drought, increasing numbers of Zebu cattle breeders looking for pasture are moving to the South-west where the climate is quite favorable and grass is still abundant. Thus, suitable management is required for the sustainable use of local breeds, and recently community-based breeding programs (CBBP) for Baoulé cattle and their crossbreds have been implemented (Ouédraogo et al., 2020). Within the CBBP, Zoma et al. (2020) identified four distinct types of cattle production systems sedentary Lobi farms, sedentary crossbreed farms, semi-transhumant Fulani Zebu farms, and transhumant Fulani Zebu farms. The admixture between Zebus and Baoulé cattle observed in this study could be due to differences in the production systems. Furthermore, notable size differences between purebred and crossbred Baoulé were confirmed (Yougbaré et al., 2020) and breeders prefer to have large animals like Zebu cattle. As shown in Figure 2, we identified several purebred Baoulé cattle that had been considered as admixed based on the sampling information. These animals originated from the populations of Loropeni and Bouroum-Bouroum where the farmers have a preference for breeding purebred Baoulé (Zoma et al., 2020).
In a recently admixed population, ancestral populations have been mixing for a relatively small number of generations, resulting in a new population with different proportions of their genome derived from the original parental populations (Khayatzadeh et al., 2016). Local ancestry analysis of admixed populations has been successfully used to detect recent selection in admixed Swiss Fleckvieh cattle (Khayatzadeh et al., 2016), as well as selection for Zebu introgressed regions in Colombian creole taurine cattle (Pitt et al., 2018). In our study, we applied this approach to identify significantly different local admixture levels and detected five chromosomes with higher deviation from the average ancestries, with an excess of Baoulé ancestry, which might account for a higher tolerance to trypanosomiasis. Similarly, Decker et al. (2014) investigated the population structure of domesticated cattle and calculated Asian indicine (B. t. indicus), Eurasian taurine, and African taurine (both B. t. taurus) ancestry proportions.
We applied an approach of significance testing and performed a permutation test of circularizing the genome by concatenating the SNPs of all autosomes in a single string, cutting this string once and rearranging the two resulting segments, as proposed by Tang et al. (2007). The permutation approach removes not only the effects of selection, but also the local effects of genetic drift; the threshold is considered non-conservative. Nevertheless, based on simulations (Tang et al., 2007) outliers are unlikely to be due to genetic drift. Therefore, this procedure is considered robust to find significant signals for selection while accounting for confounding effects of demographic history of the admixed cattle.
We found regions deviating from the average ancestry with a higher amount of Baoulé proportions on chromosomes 6, 8, and 19 in trypanosome negative individuals. A previous study (Noyes et al., 2011) identified VAV1, PIK3R5, RAC1, VAV2, GAB2, and INPP5D genes in chromosome 8 to be genes under selection in Muturu and N’Dama cattle breeds in response to trypanosomes infection. Surprisingly, we also found higher Baoulé ancestry in chromosome 8 (35–50 Mb) also in trypanosome positive cattle, which could indicate that these regions harbor beneficial Baoulé haplotypes, which are not connected to trypanosomosis tolerance. These regions might harbor genes of general importance for adaptation to the environment. Some canadidate genes on chromosome 6 at 71373513-71421283 (PDGFRA) and chromosome 19 at 41185975-41196948 (CDC6) for trypanotolerance in West African taurines have been found on these chromosomes (Tijjani, 2019) overlapping with the regions identified in our study. Furthermore, Smetko et al. (2015) identified chromosomes 7 and 22 as regions with the highest Baoulé ancestry proportion, similar to our results.
Identifying recent positive selection signatures in domesticated animals can provide information on beneficial mutations and their underlying biological pathways for economically important traits. Global FST values are one useful method to detect selection signatures across breeds (Biswas and Akey, 2006). The seven outlier SNPs, which we identified between trypanosome positive and negative crossbreds, were on chromosome 2, 3, 5, 20, 21, and 23. The chromosomes BTA 2, 3, 5, and 23 have previously been identified harboring common candidate genes in Muturu and N’Dama breeds linked to trypanotolerance in West African taurine population as well as selected candidate genes in Muturu cattle only (Tijjani et al., 2019). Functional annotation and enrichment analyses based on Reactome pathways in PANTHER ver 13.1 (Thomas et al., 2003) confirmed their relevance in response to trypanosome infection pathways. In our study, we identified other genes (Table 2) such as LOC100138101, LMO4, LTRM2, ISL1, PTCHD4, and HIVEP1 as genes potentially responsible for trypanotolerance.
From previous studies genes such as TICAM1, ARHGAP15, SLC40A1, GFM1, and INHBA have been proposed as candidate genes for trypanotolerance on chromosomes 2, 3, and 5 (Dayo et al., 2009; Noyes et al., 2011). Bahbahani et al. (2018) identified the genes LTA4H, IL7, IL15, FCN, LTA4H, and NFAM1 as potential targets of natural selection related to immunity in Sheko cattle, which are a mixture of Asian zebu and African taurine ancestry and considered a trypanotolerant breed with high potential for milk production.
The indigenous cattle breeds are disappearing because of indiscriminate crossbreeding by individual farmers, and schemes for genetic improvement developed without concern for preserving locally adapted breeds (Belemsaga et al., 2005). Many breeding programs or genetic improvement strategies in developing countries failed due to the lack of involvement of farmers in the different steps of implementation (Wurzinger et al., 2011). In many developing countries, livestock crossbreeding has been implemented with poor or no pedigree recording. Thus, ancestry informative markers would provide a great opportunity to estimate the level of admixture in a cost-effective way. Sölkner et al. (2010) proposed that individual admixture levels were estimated more accurately based on the genomic data using panels of pure reference animals, compared to estimation based on pedigree. (Getachew et al., 2017) indicated that the Ovine 50KSNP array is a powerful tool to identify small sets of AIMs for admixture studies in crossbred sheep populations in Ethiopia.
The minimum set of the 25 highest differentiating SNPs (Supplementary Table 1) can be used to develop an efficient competitive allele-specific PCR (KASPTM, LGC Group, United States) genotyping assay. Such an easy and fast genotyping array can be implemented at any laboratory equipped with Real-Time PCR machine and can be used for routine monitoring of hybridization in Baoulé cattle. Getachew et al. (2017) identified a total of 74 SNPs from the Ovine 50K SNP data as AIMs. The SNPs were selected based on their FST values showing the highest levels of allele frequency differentiation between the two parental breeds similar to our methodology. These AIMs provided close estimation with pedigree information. Correlation coefficient between breed level based on admixture estimates from 25 SNP data obtained in this study (r = 0.99; Table 3) was higher compared to the correlation value of 0.96 obtained from ∼500 AIMs suggested to predict breed composition in cattle (Frkonja et al., 2012) or the correlation values in the range of 0.89 to 0.96 reported for different human populations in prediction of admixture levels (Halder et al., 2008). Other studies (Judge et al., 2017) recommended at least 300 informative SNPs identified based on similar diversity parameters to be used for genomic-based breed composition prediction. However, as the purpose of our AIM set was to differentiate between only two ancestral breeds (Baoulé and Zebu) the number of 25 highest differentiating SNPs was sufficient to detect admixed individuals (Table 3 and Supplementary Figure 5). The existence of such a 25 SNP set allows their genotyping locally in Burkina Faso, providing a sustainable and low-cost solution to monitor admixture rates in these populations. We will further validate the 25 most AIMs in a larger group of confirmed crossbreds in Burkina Faso. Understanding the relationship between genetic admixture and performances is crucial for the success for local cattle breed conservation and crossbreeding programs. Ideally, a combination of pedigree and genomic information is used in breeding programs. Applying small sets of AIMs is a cost-effective option to estimate the levels of admixture in situations where pedigree recording is difficult like in Burkina Faso.
In this study, we identified local ancestry proportions in genomic regions potentially related to trypanotolerance in front of a global admixture background. Based on a 10% genome-wide threshold exploring extreme deviations from the average distribution of delta ancestry, the chromosomes 6, 8, 19, 21, and 22 contained higher ancestral proportions of Baoulé cattle. Furthermore, we identified genes such as LOC100138101, LMO4, LTRM2, ISL1, PTCHD4, and HIVEP1 as genes potentially responsible for trypanotolerance. Identification of genomic regions harboring genes related to trypanotolerance is a strong argument for conservation not only of Baoulé cattle, but all trypanotolerant breeds. The subsequent integration of these regions to genomes of non-trypanotolerant breeds via admixture provides a sustainable and effective use of these breeds, despite their lower production characteristics. As such, our study contributes to a better understanding of the genetic mechanism underlying trypanotolerance and will allow building a suitable breeding strategy for Baoulé cattle and their crossbreds in the south-western region of Burkina Faso.
The results indicate that the Bovine 50KSNP array is a powerful tool to identify small sets of AIMs as a cost-effective option to estimate the levels of admixture in situations where pedigree recording is difficult like in Burkina Faso. The minimum set of the 25 highest differentiating SNPs can be used to develop an efficient competitive allele-specific PCR assay.
Quality controlled Bovine 50k SNP chip data, including 31,612 SNPs of the 802 animals included in this study, were uploaded to DRYAD. The dataset has been assigned a unique identifier (doi: 10.5061/dryad.547d7wm7f).
Ethical review and approval was not required for the animal study because Samples were collected during the official health monitoring activities of the APPEAR Project “Characterization and Sustainable Utilization of Local Cattle Breeds in Burkina Faso” approved by the Ministry of Agriculture and Irrigation Development (Ministère de l’Agriculture et des Aménagements Hydro-agricoles), Burkina Faso.
JS conceived the original idea of the study and together with GM, PB, BY, and NK further developed the idea and decided on the set of analysis. BY and PB did the statistical analysis and wrote the text. BY, DO, BZ, AS, SO-K, SM, HT, and ATr collected the data for the analysis and together with JS, GM, PB, NK, ATa, PO-W, MW, ATr, and OM critically reviewed the text. All authors approved the final version of the manuscript.
This study was funded by Austrian Partnership in High Education and Research for Development (APPEAR) through project 120 LoCaBreed: Local cattle breed of Burkina Faso, characterization and sustainable use.
NK was employed by company SUISAG, Switzerland.
The remaining authors declare that the research was conducted in the absence of any commercial or financial relationships that could be construed as a potential conflict of interest.
All claims expressed in this article are solely those of the authors and do not necessarily represent those of their affiliated organizations, or those of the publisher, the editors and the reviewers. Any product that may be evaluated in this article, or claim that may be made by its manufacturer, is not guaranteed or endorsed by the publisher.
The Supplementary Material for this article can be found online at: https://www.frontiersin.org/articles/10.3389/fgene.2021.670390/full#supplementary-material
Supplementary Figure 1 | The local ancestry estimation plot for 29 autosomes chromosomes for 266 trypanosome negative, in Baoulé X Zebu crossbred cattle, excluding CHR 6, 8, 19, 21, and 22 which are presented in Figure 6.
Supplementary Figure 2 | The local ancestry estimation plot for 29 autosomes chromosomes for 244 trypanosome positive, in Baoulé X Zebu crossbred cattle, excluding CHR 6, 8, 19, 21, and 22 which are presented in Figure 6.
Supplementary Figure 3 | The delta ancestry for 29 autosomes chromosomes for 244 trypanosome positive, in Baoulé X Zebu crossbred cattle excluding CHR 6, 8, 19, 21, and 22, which are presented in Figure 7.
Supplementary Figure 4 | The delta ancestry for 29 autosomes for 266 trypanosome negative Baoulé X Zebu crossbred cattle excluding CHR 6, 8, 19, 21, and 22 which are presented in Figure 7.
Supplementary Figure 5 | Admixture graphs of the top 100, 50, 25, and 15 ancestry informative SNPs.
Supplementary Table 1 | The 100 highest differentiating SNPs according the FST value.
Agyemang, K. (2005). Trypanotolerant Livestock in the Context of Trypanosomiasis Intervention Strategies. Rome: Food & Agriculture Org.
Albert, S., Moustapha, G., Stéphane, A. R. T., and Amadou, T. (2019). Is the introgression of Lobi/Baoul cattle by zebuine genes in Burkina Faso Lobi cattle threatened? Afr. J. Biotechnol. 18, 77–85. doi: 10.5897/AJB2018.16467
Alexander, D. H., Novembre, J., and Lange, K. (2009). Fast model-based estimation of ancestry in unrelated individuals. Genome Res. 19, 1655–1664. doi: 10.1101/gr.094052.109
Bahbahani, H., Afana, A., and Wragg, D. (2018). Signatures génomiques de l’introgression adaptative et de l’adaptation environnementale chez les bovins Sheko du sud-ouest de l’Éthiopie. PLoS One 13:e0202479. doi: 10.1371/journal.pone.0202479
Bahbahani, H., Clifford, H., Wragg, D., and Mbole-Kariuki, M. N. (2015). Signatures of positive selection in East African Shorthorn Zebu: A genome-wide single nucleotide polymorphism analysis. Sci. Rep. 5:11729. doi: 10.1038/srep11729
Belemsaga, D. M. A., Lombo, Y., Thevenon, S., and Sylla, S. (2005). “Inventory Analysis of West African Cattle Breeds,” in Applications of Gene-Based Technologies for Improving Animal Production and Health in Developing Countries, eds H. P. S. Makkar and G. J. Viljoen (Berlin: Springer-Verlag), 167–173. doi: 10.1007/1-4020-3312-5_15
Benjamini, Y., and Hochberg, Y. (1995). Controlling the False Discovery Rate: A Practical and Powerful Approach to Multiple Testing. J. R. Stat. Soc. Series B. Meth. 57, 289–300. doi: 10.1111/j.2517-6161.1995.tb02031.x
Bhatia, G., Tandon, A., Patterson, N., and Aldrich, M. C. (2014). Genome-wide Scan of 29,141 African Americans Finds No Evidence of Directional Selection since Admixture. Am. J. Med. Genet. 95, 437–444. doi: 10.1016/j.ajhg.2014.08.011
Biswas, S., and Akey, J. M. (2006). Genomic insights into positive selection. Trends Genet. 22, 437–446. doi: 10.1016/j.tig.2006.06.005
Chang, C. C., Chow, C. C., Tellier, L. C., and Vattikuti, S. (2015). Second-generation PLINK: rising to the challenge of larger and richer datasets. GigaScience 4:7.
Dayo, G.-K., Thevenon, S., Berthier, D., Moazami-Goudarzi, K., Denis, C., Cuny, G., et al. (2009). Detection of selection signatures within candidate regions underlying trypanotolerance in outbred cattle populations. Mol. Ecol. 18, 1801–1813. doi: 10.1111/j.1365-294X.2009.04141.x
Decker, J. E., McKay, S. D., Rolf, M. M., and Kim, J. (2014). Worldwide Patterns of Ancestry, Divergence, and Admixture in Domesticated Cattle. PLoS Genet. 10:e1004254. doi: 10.1371/journal.pgen.1004254
Desquesnes, M., Bengaly, Z., and Dia, M. L. (2003). Evaluation de la persistance des anticorps détectés par Elisa-indirect Trypanosoma vivax après traitement trypanocide chez des. Rev. Elev. Med. Vet. Pays. Trop. 56, 141–144. doi: 10.19182/remvt.9855
Doerge, R. W., and Churchill, G. A. (1996). Permutation Tests for Multiple Loci Affecting a Quantitative Character. Genetics 142, 285–294. doi: 10.1093/genetics/142.1.285
Dossa, L. H., and Vanvanhossou, F. U. S. (2016). The indigenous Somba cattle of the hilly Atacora region in North-West Benin: threats and opportunities for its sustainable use. Trop. Anim. Health Prod. 48, 349–359. doi: 10.1007/s11250-015-0958-5
Dueppen, S. A. (2012). Cattle in the West African savanna: evidence from 1st millennium CE Kirikongo, Burkina Faso. J. Archaeol. Sci. Rep. 39, 92–101. doi: 10.1016/j.jas.2011.09.005
Flori, L., Thevenon, S., Dayo, G.-K., and Senou, M. (2014). Adaptive admixture in the West African bovine hybrid zone: insight from the Borgou population. Mol. Ecol. 23, 3241–3257. doi: 10.1111/mec.12816
Foll, M., and Gaggiotti, O. (2008). A Genome-Scan Method to Identify Selected Loci Appropriate for Both Dominant and Codominant Markers: A Bayesian Perspective. Genetics 180, 977–993. doi: 10.1534/genetics.108.092221
Freeman, A. R., Meghen, C. M., MacHUGH, D. E., and Loftus, R. T. (2004). Admixture and diversity in West African cattle populations. Mol. Ecol. 13, 3477–3487. doi: 10.1111/j.1365-294x.2004.02311.x
Frkonja, A., Gredler, B., Schnyder, U., Curik, I., and Sölkner, J. (2012). Prediction of breed composition in an admixed cattle population. Anim. Genet. 43, 696–703. doi: 10.1111/j.1365-2052.2012.02345.x
Gautier, M., and Naves, M. (2011). Footprints of selection in the ancestral admixture of a New World Creole cattle breed. Mol. Ecol. 20, 3128–3143. doi: 10.1111/j.1365-294x.2011.05163.x
Getachew, T., Huson, H. J., Wurzinger, M., and Burgstaller, J. (2017). Identifying highly informative genetic markers for quantification of ancestry proportions in crossbred sheep populations: implications for choosing optimum levels of admixture BMC. Genet 18:80. doi: 10.1186/s12863-017-0526-2
Halder, I., Shriver, M., Thomas, M., Fernandez, J. R., and Frudakis, T. (2008). A panel of ancestry informative markers for estimating individual biogeographical ancestry and admixture from four continents: utility and applications. Hum. Mutat. 29, 648–658. doi: 10.1002/humu.20695
Hanotte, O. (2002). African Pastoralism: Genetic Imprints of Origins and Migrations. Science 296, 336–339. doi: 10.1126/science.1069878
Hanotte, O., Dessie, T., and Kemp, S. (2010). Time to Tap Africa’s Livestock Genomes. Science 328, 1640–1641. doi: 10.1126/science.1186254
Houessou, S. O., Dossa, L. H., Diogo, R. V. C., and Ahozonlin, M. C. (2019). Confronting pastoralists’ knowledge of cattle breeds raised in the extensive production systems of Benin with multivariate analyses of morphological traits. PLoS One 14:e0222756. doi: 10.1371/journal.pone.0222756
Jin, W., Xu, S., Wang, H., and Yu, Y. (2012). Genome-wide detection of natural selection in African Americans pre- and post-admixture. Genome Res. 22, 519–527. doi: 10.1101/gr.124784.111
Judge, M. M., Kelleher, M. M., Kearney, J. F., Sleator, R. D., and Berry, D. P. (2017). Ultra-low-density genotype panels for breed assignment of Angus and Hereford cattle. Animal 11, 938–947. doi: 10.1017/S1751731116002457
Khayatzadeh, N., Mészáros, G., Utsunomiya, Y. T., and Garcia, J. F. (2016). Locus-specific ancestry to detect recent response to selection in admixed Swiss Fleckvieh cattle. Anim. Genet. 47, 637–646. doi: 10.1111/age.12470
Kim, E.-S., and Rothschild, M. F. (2014). Genomic adaptation of admixed dairy cattle in East Africa. Front. Genet. 5:443. doi: 10.3389/fgene.2014.00443
Ministere des ressources animales (2014). Annuaire des statistiques d’élevage 2014 (Burkina Faso). Ouagadougou: MINISTERE DES RESSOURCES ANIMALES.
Naessens, J., Teale, A. J., and Sileghem, M. (2002). Identification of mechanisms of natural resistance to African trypanosomiasis in cattle. Vet. Immunol. Immunopathol. 87, 187–194. doi: 10.1016/s0165-2427(02)00070-3
Noyes, H., Brass, A., Obara, I., and Anderson, S. (2011). Genetic and expression analysis of cattle identifies candidate genes in pathways responding to Trypanosoma congolense infection. PNAS 108, 9304–9309. doi: 10.1073/pnas.1013486108
Okeyo Mwai, A., Hanotte, O. H., Kwon, Y.-J., and Cho, S. (2015). African indigenous cattle: Unique genetic resources in a rapidly changing world. Asian-Australas. J. Anim. Sci. 2015:0002R. doi: 10.5713/ajas.15.0002R
Ouédraogo, D., Soudré, A., Ouédraogo-Koné, S., and Zoma, B. L. (2020). Breeding objectives and practices in three local cattle breed production systems in Burkina Faso with implication for the design of breeding programs. J. Livsci. 232:103910. doi: 10.1016/j.livsci.2019.103910
Pérez, O. A. M., Mészáros, G., Utsunomiya, Y. T., and Sonstegard, T. S. (2014). Linkage disequilibrium levels in Bos indicus and Bos taurus cattle using medium and high density SNP chip data and different minor allele frequency distributions. J. Livsci. 166, 121–132. doi: 10.1016/j.livsci.2014.05.007
Pitt, D., Bruford, M. W., Barbato, M., and Orozco-terWengel, P. (2018). Demography and rapid local adaptation shape Creole cattle genome diversity in the tropics. Evolut. Applicat. 12, 105–122. doi: 10.1111/eva.12641
Purcell, S., Neale, B., Todd-Brown, K., and Thomas, L. (2007). PLINK: A Tool Set for Whole-Genome Association and Population-Based Linkage Analyses. Am. J. Med. Genet. 81, 559–575. doi: 10.1086/519795
Sankararaman, S., Sridhar, S., Kimmel, G., and Halperin, E. (2008). Estimating Local Ancestry in Admixed Populations. Am. J. Med. Genet. 82, 290–303. doi: 10.1016/j.ajhg.2007.09.022
Smetko, A., Soudre, A., Silbermayr, K., and Müller, S. (2015). Trypanosomosis: potential driver of selection in African cattle. Front. Genet. 6:137. doi: 10.3389/fgene.2015.00137
Sölkner, J., Frkonja, A., Raadsma, H. W., and Jonas, E. (2010). Estimation of Individual Levels of Admixture in Crossbred Populations from SNP Chip Data: Examples with Sheep and Cattle Populations. Interbull Bull. 10, 62–66.
Tang, H., Choudhry, S., Mei, R., and Morgan, M. (2007). Recent Genetic Selection in the Ancestral Admixture of Puerto Ricans. Am. J. Med. Genet. 81, 626–633. doi: 10.1086/520769
The R Development Core Team (2020). A Language and Environment for Statistical Computing. Geneva: R Core Team.
Thomas, P. D., Kejariwal, A., Campbell, M. J., Mi, H., Diemer, K., Guo, N., et al. (2003). PANTHER: a browsable database of gene products organized by biological function, using curated protein family and subfamily classification. Nucleic Acids Res. 31, 334–341. doi: 10.1093/nar/gkg115
Tijjani, A. (2019). Genome diversity and adaptation of African Taurine and Zebu cattle. nottingham: eprints.
Tijjani, A., Utsunomiya, Y. T., Ezekwe, A. G., Nashiru, O., and Hanotte, O. (2019). Genome sequence analysis reveals selection signatures in endangered trypanotolerant West African muturu cattle. Front. Genet. 10:442. doi: 10.3389/fgene.2019.00442
Traoré, A., Koudandé, D. O., Fernández, I., and Soudré, A. (2015). Geographical assessment of body measurements and qualitative traits in West African cattle. Trop. Anim. Health Prod. 47, 1505–1513. doi: 10.1007/s11250-015-0891-7
Weir, B. S., and Cockerham, C. C., 1984. Estimating F-statistics for the analysis of population structure. Evolution 38, 1358–1370. doi: 10.2307/2408641
Wurzinger, M., Sölkner, J., and Iñiguez, L. (2011). Important aspects and limitations in considering community-based breeding programs for low-input smallholder livestock systems. Small. Rumin. Res. 98, 170–175. doi: 10.1016/j.smallrumres.2011.03.035
Yougbaré, B., Soudré, A., Ouédraogo, D., and Zoma, B. L. (2020). Morphometric characterization of purebred and crossbred Baoulé cattle in Burkina Faso. Acta Agric. Scand. A Anim. Sci. 0, 1–10. doi: 10.1080/09064702.2020.1825785
Keywords: admixture, local ancestry deviation, selection signature, SNP, Fst, cattle, Burkina Faso
Citation: Yougbaré B, Ouédraogo D, Tapsoba ASR, Soudré A, Zoma BL, Orozco-terWengel P, Moumouni S, Ouédraogo-Koné S, Wurzinger M, Tamboura HH, Traoré A, Mwai OA, Sölkner J, Khayatzadeh N, Mészáros G and Burger PA (2021) Local Ancestry to Identify Selection in Response to Trypanosome Infection in Baoulé x Zebu Crossbred Cattle in Burkina Faso. Front. Genet. 12:670390. doi: 10.3389/fgene.2021.670390
Received: 21 February 2021; Accepted: 09 August 2021;
Published: 27 September 2021.
Edited by:
Mohammed Ali Al Abri, Sultan Qaboos University, OmanReviewed by:
Pablo Fonseca, University of Guelph, CanadaCopyright © 2021 Yougbaré, Ouédraogo, Tapsoba, Soudré, Zoma, Orozco-terWengel, Moumouni, Ouédraogo-Koné, Wurzinger, Tamboura, Traoré, Mwai, Sölkner, Khayatzadeh, Mészáros and Burger. This is an open-access article distributed under the terms of the Creative Commons Attribution License (CC BY). The use, distribution or reproduction in other forums is permitted, provided the original author(s) and the copyright owner(s) are credited and that the original publication in this journal is cited, in accordance with accepted academic practice. No use, distribution or reproduction is permitted which does not comply with these terms.
*Correspondence: Negar Khayatzadeh, a2gubmVnYXJAZ21haWwuY29t
Disclaimer: All claims expressed in this article are solely those of the authors and do not necessarily represent those of their affiliated organizations, or those of the publisher, the editors and the reviewers. Any product that may be evaluated in this article or claim that may be made by its manufacturer is not guaranteed or endorsed by the publisher.
Research integrity at Frontiers
Learn more about the work of our research integrity team to safeguard the quality of each article we publish.