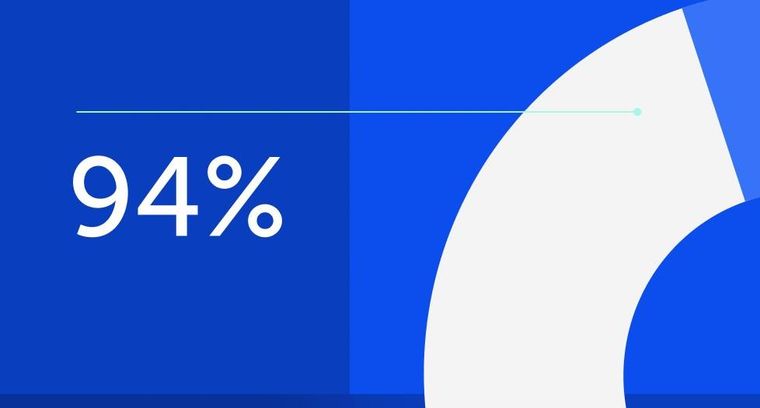
94% of researchers rate our articles as excellent or good
Learn more about the work of our research integrity team to safeguard the quality of each article we publish.
Find out more
ORIGINAL RESEARCH article
Front. Genet., 16 June 2021
Sec. Toxicogenomics
Volume 12 - 2021 | https://doi.org/10.3389/fgene.2021.664946
This article is part of the Research TopicGene-environment interactions: impact of inorganic and organic toxicants on redox status, DNA stability and epigenetic profileView all 5 articles
Prenatal exposure to perfluoroalkyl substances (PFAS), bisphenol A (BPA), lead (Pb), total mercury (THg), and methylmercury (MeHg) can affect fetal development. Factors influencing placental transfer rate of these toxins are poorly investigated. Whether prenatal exposure to pollutants has an effect on birth weight is incompletely understood. We therefore aimed (1) to determine placental transfer rates of PFAS, BPA, Pb, THg, and MeHg, (2) to analyze relationships between fetal exposure and birth outcome and (3) to analyze gene variants as mediators of placental transfer rates and birth outcome. Two hundred healthy pregnant women and their newborns participated in the study. BPA, 16 PFAS, THg, MeHg, and Pb were determined using HPLCMS/MS (BPA, PFAS), HPLC-CV-ICPMS (MeHg), CV-AFS (THg), and GF-AAS (Pb). Questionnaires and medical records were used to survey exposure sources and birth outcome. 20 single nucleotide polymorphisms and two deletion polymorphisms were determined by real-time PCR from both maternal and newborn blood. Genotype-phenotype associations were analyzed by categorical regression and logistic regression analysis. Specific gene variants were associated with altered placental transfer of PFAS (ALAD Lys59Asn, ABCG2 Gln141Lys), THg (UGT Tyr85Asp, GSTT1del, ABCC1 rs246221) and Pb (GSTP1 Ala114Val). A certain combination of three gene polymorphisms (ABCC1 rs246221, GCLM rs41303970, HFE His63Asp) was over-represented in newborns small for gestational age. 36% of Austrian and 75% of Slovakian mothers had levels exceeding the HBM guidance value I (2 μg/L) of the German HBM Commission for PFOA. 13% of newborns and 39% of women had Ery-Pb levels above 24 μg/kg, an approximation for the BMDL01 of 12 μg/L set by the European Food Safety Authority (EFSA). Our findings point to the need to minimize perinatal exposures to protect fetal health, especially those genetically predisposed to increased transplacental exposure.
The objective of the transnational human biomonitoring (HBM) project ‘‘UmMuKi1 : chemicals in mothers and their newborns in the Bratislava-Vienna region,” was to compare the exposure situation of pregnant women and its potential effect on birth weight in greater urban areas. Vienna (approximately 1,900,000 inhabitants), Austria, and Bratislava (approximately 420,000 inhabitants), Slovakia, are two Central European cities at approximately 50 km distance.
A strong focus in the UmMuKi study was on Perfluoroalkyl substances (PFAS) that are covered by International Conventions such as the Stockholm Convention on Persistent Organic Pollutants. Other substances of very high concern (SVHC) recognized under the European REACH2 Regulation are Bisphenol A (BPA) and lead (Pb). Mercury is individually addressed in the UN Minamata Convention which has entered into force in 2017.
Two PFAS, perfluorooctane acid (PFOA) and perfluorooctansulfonic acid (PFOS) are already restricted within the European Union to minimize exposures. Because the compounds are so persistent, it can be assumed that exposures will decrease slowly. For many other PFAS, the knowledge about their current uses and hazards is still very limited or missing entirely. Recently, 4730 PFAS related substances were identified by OECD3. Scientists, regulators, and civil society organizations are calling for effective and efficient assessment and management of PFAS not regulated so far (Ritscher et al., 2018). In addition, PFOS/PFOA are substituted by other PFAS and therefore exposure to these PFAS will increase within the next years and decades.
Previous data indicated low to moderate exposure to Pb and Hg in the Bratislava-Vienna region (e.g., Palkovicova et al., 2008; Gundacker et al., 2010a; Borošová et al., 2014). Exposure to PFAS, however, has not been investigated in this region before 2012. Also for BPA the information was scarce (Hohenblum et al., 2012).
Pregnant women and their newborns constitute a specifically vulnerable population. PFAS, BPA, Pb and Hg are known to be transferred via the placenta to the fetus with the potential to cause placental dysfunction and adversely affect fetal health (Esteban-Vasallo et al., 2012; Gundacker and Hengstschläger, 2012; Troisi et al., 2014; Govarts et al., 2018; Mao et al., 2020). The placental transfer rate, the ratio of umbilical cord to maternal blood concentrations, is a very informative marker of placental toxicokinetics as it indicates the permeability of the placenta to toxicants. Placental transfer rates vary over a wide range within a population as well as between populations (e.g., Stern and Smith, 2003), regardless of the level of exposure. It is reasonable to assume that genetic factors could explain this variability. In genotype-phenotype studies, very often only the maternal genotype is analyzed. From a genetic point of view, a distinction must be made between the contribution of the maternal genotype and the infant genotype to placental transfer capacities. The maternal genes determine the amount of xenobiotics present in maternal blood that come into contact with the placenta. The fetal genotype, however, determines the (transport) phenotype of the placental barrier, since all cell layers involved (syncytiotrophoblast, cytotrophoblast, placental endothelial cells) develop from embryonic cells (Gundacker et al., 2016).
Also the search for genetic variants associated with adverse birth outcomes is ongoing (e.g., Stalman et al., 2018). Small for gestational age (SGA), defined as < 10th growth percentile for gestational week, is among the leading causes of neonatal, infant, and childhood morbidity, mortality, and neurodevelopmental impairment, moreover it can adversely affect health during adult life (e.g., Gillman, 2005). The relationships between prenatal PFAS, BPA, Pb, and Hg exposures and fetal growth are complex and the findings very often inconsistent (Zhu et al., 2010; Nishioka et al., 2014; Murcia et al., 2016; Govarts et al., 2018; Lee et al., 2018; Kamai et al., 2019; Punshon et al., 2019). It was therefore indicated to analyze the potential relationships between adverse birth outcome (i.e., SGA), placental transfer rates of PFAS, BPA, Hg, and Pb, and functional gene sequence variants.
Based on previous studies that were showing certain gene variants to be associated with exposure levels of Hg, Pb, and other toxic metals (Gundacker et al., 2009, 2010b; Kayaalti et al., 2010; Goodrich et al., 2011), we selected a set of common functional polymorphisms in genes encoding for metallothioneins (MTs), Glutathione S-transferases (GSTs), glutamyl-cysteine ligase (GCL), UDP glucuronyltransferases (UGT), Vitamin D receptor (VDR), delta-aminolevulinic acid dehydratase (ALAD), Hemochromatosis (HFE), and ABC transporters (MDR1, MRP1, MRP2, BSEP, BCRP) to detect genotype-phenotype relationships in the perinatal period.
To conclude, the UmMuki study aims were to compare the regional exposure levels and sources of PFAS, BPA, Pb, total Hg, and methyl-Hg (MeHg), to analyze the influence of gene variants on placental transfer rates and birth outcome, and to assess the potential health risks deriving from the exposures to 16 PFAS (PFOS, PFOA, PFNA, PFHxS, PFDA, PFUnDA, PFBA, PFPeA, PFHxA PFHpA, PFDoA, PFTrDA, PFTeDA, PFBS, PFHptS, PFDS), BPA, total Hg (THg), methyl mercury (MeHg), and Pb.
Two hundred and thirty-four healthy pregnant women were recruited at the University clinic in Bratislava and at the Semmelweisklinik in Vienna, respectively, between 2010 and 2012. The study was approved by the ethic committee of the City of Vienna (EK 09-191-1109) and of the University clinic in Bratislava (EK-62-2010). All women, who met the inclusion criteria (i.e., healthy single term pregnancy, age of 18–45 years, signed informed consent), were enrolled in the study. Women having allergies (hay fever and allergic asthma) and hypothyreodism were not excluded. Thirty four women were not eligible because of gestational or sampling complications. Finally, 100 mother-child-pairs in Bratislava and 100 mother-child-pairs in Vienna participated in the study (Table 1). Study participants were interviewed via questionnaires by trained personal at the antenatal units. The recruiting interview was followed by another during gestational week (GW) 36–38, when maternal blood collection took place, and a third within 1–5 days after birth. The questionnaire items are depicted in Figure 1. Clinical data including pregnancy outcome (gestational age, birth weight, birth length, head circumference) were taken from medical records. The classification into small for gestational age (SGA) and large for gestational age (LGA) was made according to the growth percentiles depending on the week of gestation in which birth took place (SGA: birth weight < 10th growth percentile, LGA: Birth weight > 90th percentile). The group in between (10–90th perc.) was designated as AGA (adequate for gestational age).
Blood was sampled with the VACUETTE® Safety collection system. 3 × 7 ml of maternal venous whole blood and mucosal swab samples from the oral cavity, were taken within GW 36–38 of pregnancy. 2–3 × 7 ml of cord blood were collected within 1 h after childbirth. One 7 ml replicate of maternal/cord whole blood was centrifuged for 10 min at 3,000 rpm immediately after collection to separate erythrocytes from blood serum. Newborn whole blood (1 ml) was sampled before postnatal day 5. All samples were stored at –20°C until further treatment. After thawing, 1–1.5 g of erythrocytes were digested with a mixture of 4 ml 69 vol% HNO3 (Roth, supra quality) and 0.75–1 ml 30 vol% H2O2 (Merck). All samples were digested in Teflon vessels in a microwave oven (mls 1,200 mega, Germany). After cooling, each Teflon vessel was rinsed twice with 2 ml H2O, and the digestion solution was transferred into a polyethylene (PE) tube and filled up with millipore water to a volume of 10 ml.
For MeHg analyses, a 0.25 ml aliquot of whole blood samples of women and newborns were weighed into 15 ml PE vials. After addition of 2.5 mL of 0.1% v/v 2-mercaptoethanol in 1 M hydrochloric acid, the samples were extracted for 15 min in an ultrasonic bath. After filtration (0.45 μm membrane filters) the samples were analyzed with High Performance Liquid Chromatography-Cold Vapor-Inductively Coupled Plasma Mass Spectrometry (HPLC-CV-ICPMS).
DNA of 200 mother-child-pairs was genotyped for 20 single-nucleotide polymorphisms (SNPs) (Table 2) and two deletion polymorphisms (GSTT1, GSTM1) in candidate genes that have been linked to metabolism/detoxification of Hg, Pb, PFOS and PFOA: MT1a, MT4, GCLM, GCLC, GSTT1, GSTM1, UGT, VDR, ALAD, HFE, ABCB1, ABCC1, ABCC2, ABCG2, and ABCB11. All genotype frequencies (besides the a priori dichotomized GSTM1 and GSTT1 data, which could not be tested) were at Hardy Weinberg equilibrium.
Table 2. Nomenclature of examined polymorphisms and variant allele frequencies in 200 mother-child pairs.
Genomic DNA was extracted from maternal buccal swab samples, from frozen maternal peripheral blood and from frozen cord blood using the NucleoSpin Blood DNA extraction kit (Machery-Nagel). Genotyping was performed using commercially available TaqMan Assays (Life Technologies). PCR for each SNP were performed in a single reaction tube for wild-type and variant allele simultaneously in a StepOne Real-Time PCR System (Life Technologies). The 10 μl reaction consisted of ABsolute QPCR ROX Mix (Thermo Fischer), 25 ng of genomic DNA, 500 nM of each primer and of the corresponding pair for the TaqMan probes: 100 nM of the 5°C, followed by 45 cycles of 15 s at 95°C and 1 min at 60°C. Alleles were discriminated with StepOne Software v2.0. GSTM1 and GSTT1 deletions were analyzed using TaqMan gene expression assays (Life Technologies) in the single tube with the primers and 5−/− (homozygous deleted genotype) and + /? (homozygous intact or heterozygous genotype), which implies that the differences between homozygous intact and heterozygous genotype could not be investigated. The MT4a polymorphism was investigated by analysis of the PCR products including the SNP positions in multiplex fashion using standard homogeneous MassEXTENDED and iPLEX protocol. The samples were acquired and analyzed using mass spectrometer MassARRAY Compact Analyzer (Sequenom).
All study participants (N = 400) were genotyped. In accordance with the fact that the ancestral allele is not always the major allele (Table 2), we do not use this nomenclature in the following, but use the terms major and minor (or variant) allele. For computation, the genotype data of children and women were dichotomized to codes 1 (homozygous major allele) and 2 (heterozygous and homozygous minor allele). According to the differing methods of genotyping, the GSTM1 and GSTT1 genotypes were coded as 1 (wild type and heterozygous) and 2 (homozygous deleted). We determined 23% of children and 18% of women (GSTT1) and 53% of children and 52% of women (GSTM1) to be homozygous carriers of the deletion polymorphisms. The combined infant-maternal genotypes were coded in two categories (1: both women and newborns are homozygous carriers of the major allele, 2: all other genotypes).
A variant allele frequency score was generated for three gene variants associated with SGA, ranging from homozygous carriers of the major allele (score 1) to a maximum presence of variant alleles (score 12) as shown in Figure 5.
Due to budgetary reasons PFAS, BPA, and MeHg were analyzed in subsets (PFAS: 42 mother-newborn-pairs, BPA and MeHg: 40 mother-newborn-pairs). PFAS, BPA, and MeHg concentrations were analyzed in the accredited HBM laboratory of the Environment Agency Austria. THg and Pb were analyzed in the laboratory at the Medical University of Vienna.
Levels of free BPA in 40 blood samples were measured in 2012 at the accredited HBM laboratory of the Environmental Agency by HPLC-MS. Special efforts have been undertaken to avoid potential artifacts and to prevent false-positive detection of free BPA. Among these Bisphenol A free sampling material was chosen and analyzed previously to detect potential contamination sources. Blood samples were spiked with an isotopically labeled surrogate standard and extracted by SPE. The samples were analyzed with the same LC-MS/MS system as PFAS. For chromatographic separation a Luna C18 100 × 2 mm, 5 μm column (Phenomenex) was used with a gradient formed by water modified with hydrogen carbonate (pH 7) and acetonitrile. The analytical run was 9 min. External calibration was performed on eight levels ranging from 0.5 to 100 ng/mL. Sample extracts were measured once undiluted and once diluted (1/4 v/v). Positive findings were corrected with the recovery rate of the surrogate standard.
The levels of 19 PFAS were measured in randomly selected maternal and cord blood serum samples after preparation by solid phase extraction (SPE) using an Agilent Technologies 1290 Infinity Series (Agilent Technologies, Santa Clara, CA, United States) as HPLC system and an AB Applied Biosystems MDS SCIEX 4000 QTRAP LC/MS/MS System (AB Sciex Technologies, Framingham, MA, United States) as MS detector system. The detection was performed through specific mass transitions in electrospray ionization (ESI) negative mode and the quantification in multiple reaction monitoring (MRM) mode. Two analytical columns were used to ensure reliable separation of the target analyte peaks from the matrix (Luna C18 100 × 2 mm, 5 μm, and Kinetex PFP, 100 × 2.1 mm, 2,6 μm, both from Phenomenex, Aschaffenburg, Germany). Injection volume in both cases was 10 μL. The separation of measured PFAS was conducted by a gradient elution method using water modified with 10 mM ammonium acetate and methanol (Luna) or acetonitrile (Kinetex). Analytical runs were 25 min. and 60 min, respectively. External calibration was performed on 13 levels ranging from 0.1 to 25 ng/mL. Each sample was measured once with each chromatographic method. Analytical results are given as their means corrected by the blanks and recoveries of the corresponding internal standard. The limits of quantification (LOQs) were determined according to DIN 32645 (DIN 2008).
There was insufficient volume (<0.5 ml) of some blood samples to process them further, which explains the slightly lower number of samples for CordEry-THg, MatEry-THg, and MatEry-Pb (Table 3). Pb concentrations were analyzed by graphite furnace technique using the Hitachi Z-8200 AAS. Analyses of THg were conducted by using the mercur plus CV-AFS (Analytik-Jena, Germany). Quality control was assured by measuring blank test solutions and reference materials at each analysis run (Seronorm Trace Elements Human Whole Blood L-2, 210205). THg concentration of reference material (LOT 1003129: 16.1 ± 1.3 μg/L) and Pb content of reference material (LOT 1003192: 338 ± 21 μg/L) were in accordance with the certified levels for THg (16.0 ± 3.2 μg/L) and Pb (336 ± 18 μg/L). The limit of detection (LOD) was 0.07 μg/L (THg) and 1.7 μg/L (Pb). All metal contents were measured in duplicate (RSD < 10%) by the working curve method.
Table 3. Concentrations of Bisphenol A (BPA), Perfluoralkyl substances (PFAS), total mercury (THg), methyl mercury (MeHg), and lead (Pb) in maternal and newborn blood samples.
A subsample of 80 whole blood specimen (20 mother-child pairs in Bratislava and Vienna, respectively) was analyzed for MeHg. The selection was made on the basis of THg levels, i.e., the twenty samples with highest (>80th percentile) and lowest (<20th percentile) concentrations were chosen. We analyzed MeHg by HPLC-CV-ICPMS with a mobile phase (60 mM ammonium acetate, 0.1% v/v mercaptoethanol, 5% v/v methanol at pH 6.8), within 5 min on Atlantis dC18 (4.6 × 20 mm) chromatographic guard column at 20°C. Post column reduction was performed with 1% w/w sodium borohydride and 1 M hydrochloric acid followed by ICPMS detection at m/z 202. For quality assurance, analysis of extraction blanks, reference material NIST 955c-3 (Toxic metals in caprine blood), sample duplicates and spiked samples was performed. Recovery of MeHg for reference material NIST 955c-3 was 98 ± 7% (N = 4) and for spiked samples 95 ± 5% (N = 4). The relative standard deviation of duplicate samples was 4 ± 2.5% (N = 7). The LOQ and LOD for MeHg were 0.7 μg/L, respectively, 0.1 μg/L. MeHg concentrations < LOQ were replaced with the half LOQ.
Placental transfer rates were calculated by dividing the cord blood/serum concentrations by the maternal blood/serum concentrations for BPA, sumPFAS, PFOA, PFOS, PFNA, PFDA, PFUnDA, PFHxS, THg, MeHg, and Pb (Figure 3A). A ratio of 1 means equal concentrations in maternal blood and cord blood.
Bivariate associations between questionnaire data and medical record data with placental transfer rates, birth outcome (SGA vs. AGA), and genotypes were determined. Data from LGA babies (as N < 5) were excluded from these analyses. Non-parametric tests were used for placental transfer rates of BPA, PFAS, THg, MeHg, and Pb. Group differences and quota were analyzed with Mann-Whitney test, Kruskal-Wallis test and Chi-Square test. Spearman correlation was used in correlation analyses. In order to not increase the risk for false-negative findings, we applied P < 0.1 (and close to it) as the inclusion criteria into the further multivariate statistics using categorical regression analysis (CATREG) for metric outcome variables (placental transfer rates) and logistic regression for the nominal variable birth outcome (SGA-AGA).
All variables associated with placental transfer rates in bivariate analyses (P ≤ 0.11) were included into CATREG models (crude models). The CATREG analysis provides the Pratt-coefficient, which ranks the independent variables to their relative importance within a model. The non-significant and/or unimportant factors in this order were stepwise eliminated from the regression model using P > 0.1 and Pratt-Coefficient < 0.05 as elimination criteria (final models). For numerical predictors of birth weight, their significance and importance for binary outcomes (SGA, AGA) were assessed by their logistic regression coefficients. For this purpose, predictors were removed stepwise from the full model (containing all predictors) until further removal significantly lowered the prediction quality (AIC). Statistics were calculated with IBM SPSS Version 26 and R 4.0.0. The tests were performed as two-sided and P < 0.05 was set as the significance level.
As Table 1 shows, the study groups in Bratislava and Vienna differed in terms of eating habits, education and housing. Only some of these factors can explain differences in exposure to PFAS, Hg and Pb as outlined below.
BPA was detected in 27% of cord serum and 35% of maternal serum samples (Table 3), mostly from Bratislava (Figure 2B). BPA levels of women and newborns were significantly associated (r = 0.548, P < 0.001). In this low-exposed subgroup no correlation was found with known exposure factors such as consumption of canned food, occurrence or removal of dental inlays of synthetic origin, floors at home (e.g., PVC or laminate flooring). BPA data were excluded from all further statistical tests because of their limited information.
Figure 2. Median levels of (A) PFAS and (B) BPA, Pb, THg and MeHg in cord blood and maternal blood specimens. Site-specific differences are marked with asterisks. Kruskal Wallis-test *P < 0.05 and ***P < 0.001.
PFOA and PFOS contributed most to the PFAS content in serum (Table 3) with some significant differences in exposure between Bratislava and Vienna (Figure 2A). Site-specific sources of exposure could not be identified. PFAS concentrations in women and newborns correlated significantly (Supplementary Table 1). In CATREG analyses, MatS-PFAS concentrations and gestational length were significant predictors of CordS-PFAS concentrations. The main modulators of MatS-PFAS concentrations were study site and parity (Supplementary Table 2).
THg and MeHg as well as Pb blood levels of women and newborns correlated well (Supplementary Table 3). Significantly higher Pb concentrations were observed for study participants from Vienna in comparison to those in Bratislava. This was also true for THg and MeHg with regard to the blood levels of newborns (Figure 2B).
Fish consumption and maternal age were significant predictors of CordBl-THg, while fish consumption and number of amalgam fillings were the significant factors determining MatEry-THg (Supplementary Table 5). We observed higher cord blood mercury levels in boys than in girls (2.4 vs. 1.9 μg/kg; P = 0.035) that, however, were not attributable to enhanced fish consumption by women giving birth to boys (data not shown). The significant determinants of maternal Pb levels were study site, age of residential building, i.e., living in buildings constructed before or after 1945, and maternal age (Supplementary Table 5).
Placental transfer rates were toxicant-specific in the following order: MeHg > THg > PFOA > Pb > BPA > sumPFAS > PFNA > PFOS > PFHxS > PFDA > PFUnDA (Figure 3A). Most transfer rates of PFAS are significantly correlated (Figure 3B). An exception is PFHxS, whose transfer rate -within the PFAS–only correlates with that of PFUnDA. The correlation matrix also shows that the transfer rates of PFAS, Pb, THg and MeHg are, with few exceptions, not related to each other.
Figure 3. (A) Placental transfer rates (i.e., umbilical cord blood concentrations to maternal blood concentrations) of BPA (N = 14), sumPFAS (N = 42), PFOA (N = 41), PFOS (N = 39), PFNA (N = 39), PFDA (N = 39), PFUnDA (N = 30), PFHxS (N = 34), THg (N = 172), MeHg (N = 32), Pb (N = 198). (B) Correlation heatmap of placental transfer rates. *P < 0.05 and **P < 0.01 from Spearman correlation analyses.
In multivariate analysis, maternal education, and two gene variants (ALAD: rs1800435; ABCG2: rs2231142) when present in both mother and child, remained the most significant predictors of placental PFAS transfer (Tables 4, 5). Placental transfer of THg was reduced when pregnant women had amalgam fillings, ate pork meat and carried the GSTT1 deletion polymorphism. The infant genetic variants that modulate THg transfer to the fetus are UGT2B15 (rs1902023) and ABCC1 (rs246221) (Table 6). Maternal education, maternal smoking habits and a common GSTP1 polymorphism (rs1138272), when the mother carried the gene variant, remained significant predictors of placental Pb transfer (Table 7).
Birth weight, birth length and head circumference were significantly associated with gestational length (Figure 4A), with boys being more likely to benefit from a longer gestation period than girls (Figure 4B). 11% of newborns were SGA at each study site (Figure 4C). Although males were numerically overrepresented in the SGA group (Figure 4C), there was no statistically significant difference between neonatal sex and birth outcome (Chi-Square test P = 0.434).
Figure 4. (A) Correlations between neonatal anthropometry and gestational length ***P < 0.001 (Spearman correlation) (B) as exemplified for birth weight and gestational length in subgroups of girls (r = 0.583, N = 97) and boys (r = 0.417, N = 103). (C) Birth outcome (i.e., SGA, AGA, and LGA) is almost the same at both study sites (Chi-square test P = 0.998). (D) Birth weight increases with gestational length independent of birth outcome (SGA: r = 0.711, N = 22; AGA: r = 0.551, N = 172; LGA: r = 0.515, N = 4). (E) Relationship between residential area and birth outcome (Chi-Square test P = 0.019). Three study participants did not provide information on residential area (Table 1), resulting in smaller numbers of SGA cases (N = 21) and AGA cases (N = 171) here.
Birth weight always increased with gestational length independent of birth outcome (Figure 4D). The further factors associated with birth outcome (SGA-AGA) in bivariate statistics were: residential area (Figure 4E), and five gene variants related to maternal genotype (ABCC1, rs246221), infant genotype (VDR, rs1544410) or the combined maternal and infant genotypes (GCLM, rs41303970; HFE, rs1799945; ABCC2, rs717620) (Table 8). The logistic regression confirmed these genotypes to be associated with birth outcome (Table 9), of which ABCC1, GCLM, and HFE variant genotypes were over-represented in the SGA group when mothers and/or children carried the variant alleles; vice versa AGA newborns were more often homozygous for the major allele (Figure 5). A VDR variant genotype (rs1544410), gestational length and residential density (i.e., living in urban areas) were significantly associated with AGA (Table 9).
Figure 5. The distribution of ABCC1, GCLM, and HFE alleles in AGA and SGA cases. Chi-Square test P < 0.05.
We did not find the birth outcome associated with PFAS, THg, MeHg and Pb levels of mother-newborn-pairs nor with placental transfer rates (P > 0.05, respectively). Regarding neonatal anthropometry, weak but statistically significant associations (P < 0.05) were observed between CordBl-Hg and birth length (r = 0.152, N = 189) and between CordBl-Pb and head circumference (r = 0.154, N = 163). Several PFAS showed a significant positive correlation with gestational length (CordS-sumPFAS: r = 0.332; CordS-PFOA: r = 0.306; CordS-PFHxS: r = 0.342; P < 0.05, N = 42, respectively); this was the case also for placental transfer of PFNA (r = 0.355, P = 0.026, N = 39).
BPA is listed as substance of very high concern for reproductive toxicity (classified as reprotoxic chemical of the category 1B, CLP) and as endocrine disrupter for human health by the European Chemicals Agency ECHA4. BPA exposure of Austrian women and newborns is comparably low, which is confirmed by a previous Austrian study, where 16% of samples had BPA urine levels in a detectable range (Hohenblum et al., 2012); this, however, at a quite high LOQ of 0.6 μg/L. We have no explanation for the higher BPA concentrations in mother-child pairs from Bratislava. Known exposure pathways such as consumption of canned food, dental inlays of synthetic origin, flooring at home (e.g., PVC or laminate flooring) had no influence on BPA levels in this population.
PFAS levels particularly PFOA and PFOS differ substantially between Bratislava and Vienna. The sources for the site-specific exposures, however, are yet unclear. Maternal blood PFOA levels were elevated in Bratislava, not only compared to Vienna but also with regard to other studies (EFSA, 2018). Thirty six percent of Austrian and 75% of Slovakian mothers had levels exceeding the HBM guidance value I (2 μg/L) of the German HBM Commission for PFOA (Apel et al., 2017). In the updated scientific opinion (EFSA, 2020) with a PFAS mixture risk assessment, a lowest BMDL10 of 17.5 μg/L was derived for the sum of PFOA, PFNA, PFHxS and PFOS, based on the inverse association between serum levels of the sum of these four PFAS in 1-year-old children and their antibody titres against diphtheria. According to the European Food Safety Authority (EFSA) assessment, this BMDL10 in 1-year-old children corresponds to a maternal serum level of 6.9 ng/mL at a maternal age of 35 years and assuming 12 months of breastfeeding. Accordingly, maternal serum levels < 6.9 μg/L shall protect breast-fed children. In the present study, 31% of the women had serum levels > 6.9 μg/L for exposure to the sum of the four PFAS; the maximum sum value was 21.6 μg/L.
Pregnant women in Vienna ate more fish during pregnancy compared to women in Bratislava, which well explains their higher THg levels. With single exceptions the MeHg levels of newborn blood lay below 5 μg/L, the HBM-I value (alert level) set by the German HBM commission for total mercury (Schulz et al., 2012). None of the samples exceeded the HBM-II concentration of 15 μg/L (intervention level). MeHg, however, clearly contributes to THg levels in women and newborns (Table 3), which is in line with previous findings (e.g., Ask et al., 2002).
The German HBM commission suspended the HBM values in 2009 for Pb for several reasons, among them the adverse effects on neurodevelopment of children at blood levels below 100 μg/L and the possibility that Pb-induced effects persist into adulthood (Wilhelm et al., 2010). Similarly, the European Food Safety Authority (EFSA, 2010) says there is no threshold for critical Pb-induced health effects. Since 2012 the US CDC5 recommends the use of a reference blood Pb level of 50 μg/L to identify children, who require case management. In the UmMuKi study, the maximum CordEry-Pb concentration was 182 μg/kg (which is about 90 μg/L in whole blood). Five women and three newborns from Vienna had blood Pb levels above 100 μg/kg (about 50 μg/L) and 13% of newborns and 39% of women had levels beyond 24 μg/kg, a proxy for the EFSA BMDL01 of 12 μg/L based on developmental neurotoxicity (EFSA, 2012). A likely cause for the higher Pb exposure in Vienna is the large fraction of participants living in residential buildings constructed before 1945 (47% in Vienna vs. 10% in Bratislava). Such old buildings are still equipped with legacy lead plumbing, and tap water is a comparatively popular drinking water source in Vienna. The dissemination of effective countermeasures (e.g., draining the nightly stagnation water) would help mitigating lead exposure from tap water. The study site explains most of the variance in the models on Pb exposures (Supplementary Table 5). This implies that there are further so far unidentified sources of Pb exposure in the Bratislava-Vienna region.
PFAS, BPA and Pb concentrations were consistently lower in cord blood than in maternal blood indicating low transfer capacity of the human placenta for these substances. Overall, the correlation matrix on placental transfer rates speaks for different transfer mechanisms of THg and MeHg in comparison to Pb and PFAS. In-vitro findings from placental cell models showing that L-type amino acid transporters are involved in MeHg uptake (Straka et al., 2016; Balthasar et al., 2017) and that MRP1 is mediating its efflux (Granitzer et al., 2020) may explain why MeHg is transported so efficiently across the human placenta into fetal blood. However, our overall knowledge of the mechanisms responsible for toxicant-specific uptake, metabolism and export at the placental barrier, in particular of PFAS and Pb, is poor.
We found maternal education and two missense mutations associated with placental PFAS transfer rates. Apart from the general relationship between maternal education and neonatal health (Mensch et al., 2019), it remains unclear what aspect of maternal education—a surrogate variable that indicates socioeconomic, health and nutritional status—can contribute to placental transfer of PFAS. The amino acid substitution in the ALAD gene (Lys59Asn) leads to a more electronegative charge of the variant enzyme, which could alter binding affinities (Onalaja and Claudio, 2000). The missense mutation of ABCG2 (Gln141Lys) is associated with reduced ABCG2 expression (Slomka et al., 2020). The placental passage of PFOS and PFOA, however, has been shown to not depend on ABCG2 in a human placental perfusion model (Kummu et al., 2015).
Placental transfer of THg was reduced by the number of maternal amalgam fillings as well as the maternal consumption of pork. Amalgam fillings release inorganic mercury (IHg) (Clarkson, 2002). In endothelial cells treated with IHg or MeHg in the same concentrations, it was shown that IHg (HgII) is taken up into the cytoplasm to higher amounts than MeHg (Liu et al., 2020). In a study from China, pork meat was found to contain much more IHg than MeHg (Li et al., 2010). To our knowledge it has not been shown yet whether IHg prevents the uptake of MeHg into (placental) cells.
UGT2B15 is a drug-metabolizing phase II UDP glucuronosyltransferase (UGT) that metabolizes steroid hormones and is expressed in the human placenta (Lévesque et al., 1997). The missense mutation Tyr85Asp has been shown to be associated with drug clearance (e.g., of oxazepam; He et al., 2009), kinetics of androstane-3alpha, 17beta-diol and dihydrotestosterone (Lévesque et al., 1997), and estradiol levels in female breast cancer patients (Sparks et al., 2004). Hg is a well-known endocrine disruptor (e.g., Tan et al., 2009), however, it remains speculative how the investigated UGT2B15 genotype of infants might have changed placental Hg transfer.
The GST enzyme family protects cells from oxidative stress and toxic chemicals by detoxifying electrophilic compounds. The homozygous GSTT1 deletion, a well-studied polymorphism, impairs the enzyme’s catalytic activity, which increases sensitivity to oxidative stress and toxic substances (Hayes et al., 2005). The GSTT1 homozygous deletion was found associated with decreased urine THg (Goodrich et al., 2011) and higher levels of THg in hair (Gundacker et al., 2007). In the present study and other studies (Custodio et al., 2004, 2005; Lee et al., 2010; Barcelos et al., 2013, 2015), GSTT1 deletion had no effect on blood levels of THg or MeHg. We observed, however, a higher rate of transmission of THg via the placenta when the mother carried the GSTT1 deletion indicating an increased risk of fetal Hg exposure. Further research is necessary to determine the effect of cellular GSTT1 depletion on placental Hg kinetics.
MRP1 (ABCC1) is a multitasking ABC transporter, one of its central functions being to maintain the cellular redox status by the efflux of glutathione (GSH) conjugates (GS-X) and oxidized GSH (GS-SG) (Cole, 2014). Although ABCC1 gene variants were shown to be associated with Hg toxicokinetics (Llop et al., 2014; Engström et al., 2016), it has not yet been clarified whether Hg is transported by MRP1. In a recent work we confirmed Hg as a MRP1 substrate as well as that MRP1 function is essential for the viability of placental cells exposed to MeHg (Granitzer et al., 2020). In the present study, a non-synonymous ABCC1 SNP (rs246221), i.e., the presence of the C-allele in infants, was associated with a higher placental transfer rate of THg. Recent data that we gained in another study group confirm this association (unpublished data Gundacker). To our knowledge, however, it has not yet been researched how this nsSNP affects MRP1 expression and function. The SNP also could be linked to other functional gene variants not present in our panel.
Maternal education and maternal smoking were associated with enhanced Pb transfer across the human placenta. It is known that tobacco smoking deregulates ion transport and expression of antioxidant enzymes and metallothionein in the human placenta (Ronco et al., 2006; Votavova et al., 2011). In which way smoking habits might affect transport of Pb toward fetal blood remains unknown. GSTP1, a cytosolic GST, is highly expressed in the human placenta (Raijmakers et al., 2001). Pb exposure can induce GSTP1 activity in rats (Wright et al., 1998). A common missense polymorphism (Ala114Val) modifies the shape of the active site and thus substrate affinity, resulting in lower catalytic efficiency of GSTP1 (Zhong et al., 2006; Goodrich and Basu, 2012). The variant, however, has been rarely investigated with regard to Pb exposure levels and if so, no associations have been found (Gundacker et al., 2009). Also in the present study, the minor genotype 114Val was not associated with Pb levels of mother-newborn-pairs but with lowered Pb transfer across the placenta when the mother carried the T allele. This may indicate a crucial role of GSTP1 in Pb transfer across the placenta as Pb is likely to be transported by MRPs as a GSH conjugate (Ballatori et al., 2009). The formation of the Pb-GSH conjugate requires the presence of a GST catalyzing this conjugation reaction.
The here observed positive correlations between gestational length and sumPFAS, PFOA, PFHxS levels indicate accumulation with gestational age. PFOS, PFOA, and PFNA were shown to accumulate in placenta across gestation (Mamsen et al., 2019), which may harm placental functions (Szilagyi et al., 2020). A pooled analysis of 7 European birth cohorts found that prenatal exposure to PFOA (median cord serum level: 0.55 μg/L) may contribute to the prevalence of SGA (Govarts et al., 2018).
In the present study, gestational length was associated with birth outcome (SGA, AGA, LGA) confirming gestational age as a strong contributor to birth weight (Clausson et al., 2000; Donahue et al., 2010). Furthermore, AGA babies were over-represented in urban areas of Bratislava and Vienna. Although rurality can be associated with low birth weight and SGA (Strutz et al., 2012), also population-dense urban areas have been shown to have elevated rates of adverse birth outcomes (Kent et al., 2013). Low maternal education increases the risk for preterm birth and SGA (Ruiz et al., 2015). In our study group, women with basic education were more likely to live in rural areas (29%) than women with a university degree (10%) (Chi-Square test P = 0.041), which might explain the over-representation of AGA in the urban areas we have investigated. It remains to be clarified which (other) urban-specific factors in Bratislava and Vienna have contributed to birth outcome.
Three gene variants ABCC1 (rs246221; maternal genotype), GCLM (rs41303970; combined maternal-infant genotype) and HFE (rs1799945; combined maternal-infant genotype) were found to be associated with SGA. Interestingly, all three proteins are related to conditions of oxidative stress. ABCC1 has an important role in maintaining cellular redox status (Cole, 2014) also in placental cells (Granitzer et al., 2020), GCLM is the rate-limiting enzyme in the synthesis of the major cellular anti-oxidant GSH (Liu, 2013), and HFE malfunction is associated with systemic iron overload (hemochromatosis) promoting oxidative stress (De Souza et al., 2015). When combining these ABCC1, GCLM, and HFE genotypes, we found a significant over-representation of the major alleles in AGA as well as a significant over-representation of the variant alleles in SGA babies. Despite the small sample numbers we were able to analyze, the adverse effect of these gene variants on birth weight seems plausible. Oxidative stress conditions are known to be associated with lower birth weight and SGA (Weber et al., 2014). Moreover, the presence of the HFE H63D variant allele has been shown to predict a reduction of 110 g in birth weight (when infants carry the variant) and of 52 g (when mothers carry the variant) in a Pb-exposed population (Cantonwine et al., 2010).
Vitamin D deficiency during pregnancy can affect fetal growth as indicated in animal experiments (Liu et al., 2013; Wilson et al., 2015) and human studies (Harvey et al., 2014; Knabl et al., 2017). The possible mechanisms linking Vitamin D to fetal growth include calcium metabolism, bone growth, and placental functions such as human chorionic gonadotropin secretion, placental sex steroid production, and glucose transport (Knabl et al., 2017). Vitamin D activity is mediated by its receptor VDR. Among the most frequently examined VDR gene variants (FokI, rs2228570; ApaI rs7975232; Bsml rs1544410; TaqI, rs 731236), ApaI, BsmI, and TaqI genotype are in linkage disequilibrium in Caucasian populations (Knabl et al., 2017). In several studies these VDR SNPs increased the risk of preterm birth (Rosenfeld et al., 2017; Dutra et al., 2019). As determined in a meta-analysis, the here investigated VDR polymorphism (syn. BsmI, i.e., the A allele) protected from preterm delivery (Barchitta et al., 2018), which may explain why we found this VDR variant (infants carry the A allele) associated with AGA. More research on the possible protective role of this and other VDR gene variants on birth outcome is required.
Our study has several strengths. We described in a first attempt the exposure situation of pregnant women and newborns for a variety of substances of high concern including 16 PFAS compounds. We also performed genotyping in both pregnant women and newborns to obtain information on the contribution of the maternal, the infant, and the combined maternal-infant genotype to placental transfer rates of PFAS, Hg, and Pb, and to SGA prevalence. In most cases, the infant genotype (alone and in combination with the maternal genotype) explained placental transfer rates and SGA prevalence better than the maternal genotype alone. This at least suggests that the infant genotype has a large influence on placental transfer rates of the here examined toxins. Further studies are necessary to clarify these relationships, also by taking into account the paternal genotype.
Considering the low to moderate exposure in the Bratislava-Vienna region, the here reported findings might not be applicable to situations of higher exposure. As small sample numbers increase the risk of false-negative findings, one further limitation of the study is that BPA, PFAS, and MeHg levels could have been measured only in a limited number of pregnant women and newborns.
Fetal exposures to toxic substances and endocrine disrupters can adversely affect lifetime health. In agreement with other European studies, our results show the need to minimize perinatal exposures. Especially exposures to PFAS and Pb should be investigated at more regular intervals. With regard to Pb, because there is little recent data on exposure in Europe and the existing guidelines provide limited guidance. In case of PFAS it is necessary to evaluate the effectiveness of regulation measures as well as to avoid regrettable substitutions.
Despite of close geographical proximity, the exposure situation in Bratislava and Vienna was different. Efforts are required to identify the exposure sources to PFOA and BPA in Bratislava as well as to Pb and PFOS in Vienna. Our findings were the basis for information folders disseminated to pregnant women at clinics in Vienna and Bratislava as well as to the interested public. Follow-up studies are necessary in order to evaluate the effectiveness of such preventive measures.
We found gene sequence variants associated with placental transfer rate and with SGA prevalence. Basic research to investigate placental transfer rates of priority substances in connection with genetic factors is of utmost importance in order to define and protect genetically predisposed risk groups.
The datasets for this article are not publicly available due to concerns regarding participant/patient anonymity. Requests to access the datasets should be directed to the corresponding author.
The studies involving human participants were reviewed and approved by ethic committee of the City of Vienna (EK 09-191-1109) Ethic Committee of the University clinic in Bratislava (EK-62-2010). Written informed consent to participate in this study was obtained from the study participants or the participants’ legal guardians/relatives.
MU, MG, and CG: funding acquisition and writing–original draft. MU, CG, KG-R, and MG: study concept and design. KH and PR: recruitment of study participants and sampling (University Hospital Bratislava). KG-R, TR, and IT: recruitment of study participants and sampling (Semmelweis Klinik Vienna). ZD, MG, RK, VP, TR, IT, and WR: data collection. TR, IT, CG, SS, BK-V, and SW: analytics. CG, IO, MH, ZD, and MG: statistics and data interpretation. MG, MH, KG-R, KH, and SS: resources. CG, MH, MU, ZD, RK, IO, VP, and KG-R: writing–review and editing. All authors approved the final version of the manuscript.
The UmMuKi project (SK-AT N00055) received significant financial support from the European Transnational Cooperation (ETC) Programme Austria-Slovakia 2007–2013, funded by the European Regional Development Fund (ERDF). We thank the Austrian Federal Ministry of Agriculture, Forestry, Environment and Water Management, the Environment Agency Vienna, and MedGene, Bratislava, for taking over the national co-financing.
The authors declare that the research was conducted in the absence of any commercial or financial relationships that could be construed as a potential conflict of interest.
We wish to thank the study participants and the medical staff at the clinics, Lukas Damjanovic, Matthias Scheinast, Ulla Radler and Peter Chiba for technical support, Lucia Behalova for organizational support, and Martin Forsthuber for comments on the manuscript.
The Supplementary Material for this article can be found online at: https://www.frontiersin.org/articles/10.3389/fgene.2021.664946/full#supplementary-material
Supplementary Table 1 | Correlation matrix of PFAS levels (sum parameter§ and single compounds) in 42 cord serum and maternal serum samples each.
Supplementary Table 2 | Correlation matrix of THg, MeHg and Pb levels.
Supplementary Table 3 | Factors associated with PFAS§ levels in CATREG analysis.
Supplementary Table 4 | Associations of exposure factors with THg levels in CATREG analysis.
Supplementary Table 5 | Factors associated with Pb levels in CATREG analysis.
Apel, P., Angerer, J., Wilhelm, M., and Kolossa-Gehring, M. (2017). New HBM values for emerging substances, inventory of reference and HBM values in force, and working principles of the German Human Biomonitoring Commission. Int. J. Hyg. Environ. Health 220, 152–166. doi: 10.1016/j.ijheh.2016.09.007
Ask, K., Akesson, A., Berglund, M., and Vahter, M. (2002). Inorganic mercury and methylmercury in placentas of Swedish women. Environ. Health Perspect. 110, 523–526. doi: 10.1289/ehp.02110523
Ballatori, N., Krance, S. M., Marchan, R., and Hammond, C. L. (2009). Plasma membrane glutathione transporters and their roles in cell physiology and pathophysiology. Mol. Aspects Med. 30, 13–28. doi: 10.1016/j.mam.2008.08.004
Balthasar, C., Stangl, H., Widhalm, R., Granitzer, S., Hengstschläger, M., and Gundacker, C. (2017). Methylmercury uptake into BeWo Cells Depends on LAT2-4F2hc, a system L Amino acid transporter. Int. J. Mol. Sci. 18:1730. doi: 10.3390/ijms18081730
Barcelos, G. R., Souza, M. F., Oliveira, A., Lengert, A., Oliveira, M. T., Camargo, R. B., et al. (2015). Effects of genetic polymorphisms on antioxidant status and concentrations of the metals in the blood of riverside Amazonian communities co-exposed to Hg and Pb. Environ. Res. 138, 224–232. doi: 10.1016/j.envres.2015.02.017
Barcelos, G. R. M., Grotto, D., de Marco, K. C., Valentini, J., Lengert, A. V. H., Oliveira, A. ÁS. D., et al. (2013). Polymorphisms in glutathione-related genes modify mercury concentrations and antioxidant status in subjects environmentally exposed to methylmercury. Sci. Total Environ. 463–464, 319–325. doi: 10.1016/j.scitotenv.2013.06.029
Barchitta, M., Maugeri, A., La Rosa, M. C., Magnano San Lio, R., Favara, G., Panella, M., et al. (2018). Single Nucleotide polymorphisms in vitamin D receptor gene affect birth weight and the risk of preterm birth: results from the “Mamma & Bambino” Cohort and A Meta-Analysis. Nutrients 10:1172. doi: 10.3390/nu10091172
Borošová, D., Slotová, K., and Fabiánová, E. (2014). Mercury content in hairs of mother-child pairs in Slovakia as a biomarker of environmental exposure. Acta Chim. Slov. 7, 119–122. doi: 10.2478/acs-2014-0020
Cantonwine, D., Hu, H., Téllez-Rojo, M. M., Sánchez, B. N., Lamadrid-Figueroa, H., Ettinger, A. S., et al. (2010). HFE gene variants modify the association between maternal lead burden and infant birthweight: a prospective birth cohort study in Mexico City, Mexico. Environ. Health 9, 43–43. doi: 10.1186/1476-069X-9-43
Clarkson, T. W. (2002). The three modern faces of mercury. Environ. Health Perspect. 110, 11–23. doi: 10.1289/ehp.02110s111
Clausson, B., Lichtenstein, P., and Cnattingius, S. (2000). Genetic influence on birthweight and gestational length determined by studies in offspring of twins. BJOG 107, 375–381. doi: 10.1111/j.1471-0528.2000.tb13234.x
Cole, S. P. C. (2014). Multidrug resistance protein 1 (MRP1, ABCC1), a “multitasking” ATP-binding cassette (ABC) transporter. J. Biol. Chem. 289, 30880–30888. doi: 10.1074/jbc.R114.609248
Custodio, H. M., Broberg, K., Wennberg, M., Jansson, J. H., Vessby, B., Hallmans, G., et al. (2004). Polymorphisms in glutathione-related genes affect methylmercury retention. Arch. Environ. Health 59, 588–595. doi: 10.1080/00039890409603438
Custodio, H. M., Harari, R., Gerhardsson, L., Skerfving, S., and Broberg, K. (2005). Genetic influences on the retention of inorganic mercury. Arch. Environ. Occup. Health 60, 17–23. doi: 10.3200/aeoh.60.1.17-23
De Souza, G. F., Ribeiro, H. L. Jr., De Sousa, J. C., Heredia, F. F., De Freitas, R. M., Martins, M. R., et al. (2015). HFE gene mutation and oxidative damage biomarkers in patients with myelodysplastic syndromes and its relation to transfusional iron overload: an observational cross-sectional study. BMJ Open 5:e006048. doi: 10.1136/bmjopen-2014-006048
Donahue, S. M. A., Kleinman, K. P., Gillman, M. W., and Oken, E. (2010). Trends in birth weight and gestational length among singleton term births in the United States: 1990-2005. Obstet. Gynecol. 115, 357–364. doi: 10.1097/AOG.0b013e3181cbd5f5
Dutra, L. V., Affonso-Kaufman, F. A., Cafeo, F. R., Kassai, M. S., Barbosa, C. P., Santos Figueiredo, F. W., et al. (2019). Association between vitamin D plasma concentrations and VDR gene variants and the risk of premature birth. BMC Pregnancy Childbirth 20:3. doi: 10.1186/s12884-019-2671-2
EFSA (2012). Lead dietary exposure in the European population. EFSA J. 10:2831. doi: 10.2903/j.efsa.2012.2831. Available online at: www.efsa.europa.eu/efsajournal.
EFSA (2018). Risk to human health related to the presence of perfluorooctane sulfonic acid and perfluorooctanoic acid in food. EFSA J. 16:5194. doi: 10.2903/j.efsa.2018.5194
EFSA (2020). Risk to human health related to the presence of perfluoroalkyl substances in food. EFSA J. 18:e06223.
Engström, K., Love, T. M., Watson, G. E., Zareba, G., Yeates, A., Wahlberg, K., et al. (2016). Polymorphisms in ATP-binding cassette transporters associated with maternal methylmercury disposition and infant neurodevelopment in mother-infant pairs in the Seychelles child development study. Environ. Int. 94, 224–229. doi: 10.1016/j.envint.2016.05.027
Esteban-Vasallo, M. D., Aragonés, N., Pollan, M., López-Abente, G., and Perez-Gomez, B. (2012). Mercury, cadmium, and lead levels in human placenta: a systematic review. Environ. Health Perspect. 120, 1369–1377. doi: 10.1289/ehp.1204952
Gillman, M. W. (2005). Developmental origins of health and disease. N. Engl. J. Med. 353, 1848–1850. doi: 10.1056/NEJMe058187
Goodrich, J. M., and Basu, N. (2012). Variants of glutathione s-transferase pi 1 exhibit differential enzymatic activity and inhibition by heavy metals. Toxicol. Vitro 26, 630–635. doi: 10.1016/j.tiv.2012.02.005
Goodrich, J. M., Wang, Y., Gillespie, B., Werner, R., Franzblau, A., and Basu, N. (2011). Glutathione enzyme and selenoprotein polymorphisms associate with mercury biomarker levels in Michigan dental professionals. Toxicol. Appl. Pharmacol. 257, 301–308. doi: 10.1016/j.taap.2011.09.014
Govarts, E., Iszatt, N., Trnovec, T., de Cock, M., Eggesbø, M., Palkovicova Murinova, L., et al. (2018). Prenatal exposure to endocrine disrupting chemicals and risk of being born small for gestational age: Pooled analysis of seven European birth cohorts. Environ. Int. 115, 267–278. doi: 10.1016/j.envint.2018.03.017
Granitzer, S., Ellinger, I., Khan, R., Gelles, K., Widhalm, R., Hengstschläger, M., et al. (2020). In vitro function and in situ localization of Multidrug Resistance-associated Protein (MRP)1 (ABCC1) suggest a protective role against methyl mercury-induced oxidative stress in the human placenta. Arch. Toxicol. 94, 3799–3817. doi: 10.1007/s00204-020-02900-5
Gundacker, C., Fröhlich, S., Graf-Rohrmeister, K., Eibenberger, B., Jessenig, V., Gicic, D., et al. (2010a). Perinatal lead and mercury exposure in Austria. Sci. Total Environ. 408, 5744–5749. doi: 10.1016/j.scitotenv.2010.07.079
Gundacker, C., Gencik, M., and Hengstschläger, M. (2010b). The relevance of the individual genetic background for the toxicokinetics of two significant neurodevelopmental toxicants: Mercury and lead. Mutat. Res. 705, 130–140. doi: 10.1016/j.mrrev.2010.06.003
Gundacker, C., and Hengstschläger, M. (2012). The role of the placenta in fetal exposure to heavy metals. Wien. Med. Wochenschr. 162, 201–206. doi: 10.1007/s10354-012-0074-3
Gundacker, C., Komarnicki, G., Jagiello, P., Gencikova, A., Dahmen, N., Wittmann, K. J., et al. (2007). Glutathione-S-transferase polymorphism, metallothionein expression, and mercury levels among students in Austria. Sci. Total Environ. 385, 37–47. doi: 10.1016/j.scitotenv.2007.07.033
Gundacker, C., Neesen, J., Straka, E., Ellinger, I., Dolznig, H., and Hengstschläger, M. (2016). Genetics of the human placenta: implications for toxicokinetics. Arch. Toxicol. 90, 2563–2581. doi: 10.1007/s00204-016-1816-6
Gundacker, C., Wittmann, K. J., Kukuckova, M., Komarnicki, G., Hikkel, I., and Gencik, M. (2009). Genetic background of lead and mercury metabolism in a group of medical students in Austria. Environ. Res. 109, 786–796. doi: 10.1016/j.envres.2009.05.003
Harvey, N. C., Holroyd, C., Ntani, G., Javaid, K., Cooper, P., Moon, R., et al. (2014). Vitamin D supplementation in pregnancy: a systematic review. Health Technol. Assess. 18:450. doi: 10.3310/hta18450
Hayes, J. D., Flanagan, J. U., and Jowsey, I. R. (2005). Glutathione transferases. Annu. Rev. Pharmacol. Toxicol. 45, 51–88. doi: 10.1146/annurev.pharmtox.45.120403.095857
He, X., Hesse, L. M., Hazarika, S., Masse, G., Harmatz, J. S., Greenblatt, D. J., et al. (2009). Evidence for oxazepam as an in vivo probe of UGT2B15: oxazepam clearance is reduced by UGT2B15 D85Y polymorphism but unaffected by UGT2B17 deletion. Br. J. Clin. Pharmacol. 68, 721–730. doi: 10.1111/j.1365-2125.2009.03519.x
Hohenblum, P., Steinbichl, P., Raffesberg, W., Weiss, S., Moche, W., Vallant, B., et al. (2012). Pollution gets personal! A first population-based human biomonitoring study in Austria. Int. J. Hyg. Environ. Health 215, 176–179. doi: 10.1016/j.ijheh.2011.08.015
Kamai, E. M., McElrath, T. F., and Ferguson, K. K. (2019). Fetal growth in environmental epidemiology: mechanisms, limitations, and a review of associations with biomarkers of non-persistent chemical exposures during pregnancy. Environ. Health 18:43. doi: 10.1186/s12940-019-0480-8
Kayaalti, Z., Mergen, G., and Söylemezoğlu, T. (2010). Effect of metallothionein core promoter region polymorphism on cadmium, zinc and copper levels in autopsy kidney tissues from a Turkish population. Toxicol. Appl. Pharmacol. 245, 252–255. doi: 10.1016/j.taap.2010.03.007
Kent, S. T., McClure, L. A., Zaitchik, B. F., and Gohlke, J. M. (2013). Area-level risk factors for adverse birth outcomes: trends in urban and rural settings. BMC Pregnancy Childbirth 13:129. doi: 10.1186/1471-2393-13-129
Knabl, J., Vattai, A., Ye, Y., Jueckstock, J., Hutter, S., Kainer, F., et al. (2017). Role of placental VDR expression and function in common late pregnancy disorders. Int. J. Mol. Sci. 18:2340. doi: 10.3390/ijms18112340
Kummu, M., Sieppi, E., Koponen, J., Laatio, L., Vähäkangas, K., Kiviranta, H., et al. (2015). Organic anion transporter 4 (OAT 4) modifies placental transfer of perfluorinated alkyl acids PFOS and PFOA in human placental ex vivo perfusion system. Placenta 36, 1185–1191. doi: 10.1016/j.placenta.2015.07.119
Lee, B.-E., Hong, Y.-C., Park, H., Ha, M., Koo, B. S., Chang, N., et al. (2010). Interaction between GSTM1/GSTT1 polymorphism and blood mercury on birth weight. Environ. Health Perspect. 118, 437–443. doi: 10.1289/ehp.0900731
Lee, Y. M., Hong, Y.-C., Ha, M., Kim, Y., Park, H., Kim, H. S., et al. (2018). Prenatal Bisphenol-A exposure affects fetal length growth by maternal glutathione transferase polymorphisms, and neonatal exposure affects child volume growth by sex: From multiregional prospective birth cohort MOCEH study. Sci. Total Environ. 612, 1433–1441. doi: 10.1016/j.scitotenv.2017.08.317
Lévesque, E., Beaulieu, M., Green, M. D., Tephly, T. R., Bélanger, A., and Hum, D. W. (1997). Isolation and characterization of UGT2B15(Y85): a UDP-glucuronosyltransferase encoded by a polymorphic gene. Pharmacogenetics 7, 317–325. doi: 10.1097/00008571-199708000-00007
Li, P., Feng, X., and Qiu, G. (2010). Methylmercury exposure and health effects from rice and fish consumption: a review. Int. J. Environ. Res. Public Health 7, 2666–2691. doi: 10.3390/ijerph7062666
Liu, N. Q., Ouyang, Y., Bulut, Y., Lagishetty, V., Chan, S. Y., Hollis, B. W., et al. (2013). Dietary vitamin D restriction in pregnant female mice is associated with maternal hypertension and altered placental and fetal development. Endocrinology 154, 2270–2280. doi: 10.1210/en.2012-2270
Liu, S., Tsui, M. T.-K., Lee, E., Fowler, J., and Jia, Z. (2020). Uptake, efflux, and toxicity of inorganic and methyl mercury in the endothelial cells (EA.hy926). Scient. Rep. 10:9023. doi: 10.1038/s41598-020-66444-5
Llop, S., Engström, K., Ballester, F., Franforte, E., Alhamdow, A., Pisa, F., et al. (2014). Polymorphisms in ABC transporter genes and concentrations of mercury in newborns–evidence from two Mediterranean birth cohorts. PLoS One 9:e97172. doi: 10.1371/journal.pone.0097172
Mamsen, L. S., Björvang, R. D., Mucs, D., Vinnars, M.-T., Papadogiannakis, N., Lindh, C. H., et al. (2019). Concentrations of perfluoroalkyl substances (PFASs) in human embryonic and fetal organs from first, second, and third trimester pregnancies. Environ. Int. 124, 482–492. doi: 10.1016/j.envint.2019.01.010
Mao, J., Jain, A., Denslow, N. D., Nouri, M.-Z., Chen, S., Wang, T., et al. (2020). Bisphenol A and bisphenol S disruptions of the mouse placenta and potential effects on the placenta–brain axis. Proc. Natl. Acad. Sci. 117:4642. doi: 10.1073/pnas.1919563117
Mensch, B. S., Chuang, E. K., Melnikas, A. J., and Psaki, S. R. (2019). Evidence for causal links between education and maternal and child health: systematic review. Trop. Med. Int. Health 24, 504–522. doi: 10.1111/tmi.13218
Murcia, M., Ballester, F., Enning, A. M., Iñiguez, C., Valvi, D., Basterrechea, M., et al. (2016). Prenatal mercury exposure and birth outcomes. Environ. Res. 151, 11–20. doi: 10.1016/j.envres.2016.07.003
Nishioka, E., Yokoyama, K., Matsukawa, T., Vigeh, M., Hirayama, S., Ueno, T., et al. (2014). Evidence that birth weight is decreased by maternal lead levels below 5 μg/dl in male newborns. Reprod. Toxicol. 47, 21–26. doi: 10.1016/j.reprotox.2014.05.007
Onalaja, A. O., and Claudio, L. (2000). Genetic susceptibility to lead poisoning. Environ. Health Perspect. 108, 23–28. doi: 10.1289/ehp.00108s123
Palkovicova, L., Ursinyova, M., Masanova, V., Yu, Z., and Hertz-Picciotto, I. (2008). Maternal amalgam dental fillings as the source of mercury exposure in developing fetus and newborn. J. Expo. Sci. Environ. Epidemiol. 18, 326–331. doi: 10.1038/sj.jes.7500606
Punshon, T., Li, Z., Jackson, B. P., Parks, W. T., Romano, M., Conway, D., et al. (2019). Placental metal concentrations in relation to placental growth, efficiency and birth weight. Environ. Int. 126, 533–542. doi: 10.1016/j.envint.2019.01.063
Raijmakers, M. T. M., Steegers, E. A. P., and Peters, W. H. M. (2001). Glutathione S-transferases and thiol concentrations in embryonic and early fetal tissues. Hum. Reprod. 16, 2445–2450. doi: 10.1093/humrep/16.11.2445
Ritscher, A., Wang, Z., Scheringer, M., Boucher, J. M., Ahrens, L., Berger, U., et al. (2018). Zürich Statement on future actions on Per- and Polyfluoroalkyl substances (PFASs). Environ. Health Perspect. 126:84502. doi: 10.1289/ehp4158
Ronco, A. M., Garrido, F., and Llanos, M. N. (2006). Smoking specifically induces metallothionein-2 isoform in human placenta at term. Toxicology 223, 46–53. doi: 10.1016/j.tox.2006.03.002
Rosenfeld, T., Salem, H., Altarescu, G., Grisaru-Granovsky, S., Tevet, A., and Birk, R. (2017). Maternal-fetal vitamin D receptor polymorphisms significantly associated with preterm birth. Arch. Gynecol. Obstet. 296, 215–222. doi: 10.1007/s00404-017-4412-y
Ruiz, M., Goldblatt, P., Morrison, J., Kukla, L., Švancara, J., Riitta-Järvelin, M., et al. (2015). Mother’s education and the risk of preterm and small for gestational age birth: a DRIVERS meta-analysis of 12 European cohorts. J. Epidemiol. Community Health 69, 826–833. doi: 10.1136/jech-2014-205387
Schulz, C., Wilhelm, M., Heudorf, U., and Kolossa-Gehring, M. (2012). Reprint of “Update of the reference and HBM values derived by the german human biomonitoring commission”. Int. J. Hyg. Environ. Health 215, 150–158. doi: 10.1016/j.ijheh.2012.01.003
Slomka, M., Sobalska-Kwapis, M., Korycka-Machała, M., Dziadek, J., Bartosz, G., and Strapagiel, D. (2020). Comprehensive analysis of ABCG2 genetic variation in the polish population and Its inter-population comparison. Genes 11:1144. doi: 10.3390/genes11101144
Sparks, R., Ulrich, C. M., Bigler, J., Tworoger, S. S., Yasui, Y., Rajan, K. B., et al. (2004). UDP-glucuronosyltransferase and sulfotransferase polymorphisms, sex hormone concentrations, and tumor receptor status in breast cancer patients. Breast Cancer Res. 6:R488. doi: 10.1186/bcr818
Stalman, S. E., Solanky, N., Ishida, M., Alemán-Charlet, C., Abu-Amero, S., Alders, M., et al. (2018). Genetic analyses in small-for-gestational-age newborns. J. Clin. Endocrinol. Metab. 103, 917–925. doi: 10.1210/jc.2017-01843
Stern, A. H., and Smith, A. E. (2003). An assessment of the cord blood:maternal blood methylmercury ratio: implications for risk assessment. Environ. Health Perspect. 111:6187. doi: 10.1289/ehp.6187
Straka, E., Ellinger, I., Balthasar, C., Scheinast, M., Schatz, J., Szattler, T., et al. (2016). Mercury toxicokinetics of the healthy human term placenta involve amino acid transporters and ABC transporters. Toxicology 340, 34–42. doi: 10.1016/j.tox.2015.12.005
Strutz, K. L., Dozier, A. M., van Wijngaarden, E., and Glantz, J. C. (2012). Birth outcomes across three rural-urban typologies in the finger lakes region of New York. J. Rural Health 28, 162–173. doi: 10.1111/j.1748-0361.2011.00392.x
Szilagyi, J. T., Avula, V., and Fry, R. C. (2020). Perfluoroalkyl substances (PFAS) and their effects on the placenta, pregnancy, and child development: a potential mechanistic role for Placental Peroxisome Proliferator–Activated Receptors (PPARs). Curr. Environ. Health Rep. 7, 222–230. doi: 10.1007/s40572-020-00279-0
Tan, S. W., Meiller, J. C., and Mahaffey, K. R. (2009). The endocrine effects of mercury in humans and wildlife. Crit. Rev. Toxicol. 39, 228–269. doi: 10.1080/10408440802233259
Troisi, J., Mikelson, C., Richards, S., Symes, S., Adair, D., Zullo, F., et al. (2014). Placental concentrations of bisphenol A and birth weight from births in the Southeastern U.S. Placenta 35, 947–952. doi: 10.1016/j.placenta.2014.08.091
Votavova, H., Dostalova Merkerova, M., Fejglova, K., Vasikova, A., Krejcik, Z., Pastorkova, A., et al. (2011). Transcriptome alterations in maternal and fetal cells induced by tobacco smoke. Placenta 32, 763–770. doi: 10.1016/j.placenta.2011.06.022
Weber, D., Stuetz, W., Bernhard, W., Franz, A., Raith, M., Grune, T., et al. (2014). Oxidative stress markers and micronutrients in maternal and cord blood in relation to neonatal outcome. Eur. J. Clin. Nutr. 68, 215–222. doi: 10.1038/ejcn.2013.263
Wilhelm, M., Heinzow, B., Angerer, J., and Schulz, C. (2010). Reassessment of critical lead effects by the german human biomonitoring commission results in suspension of the human biomonitoring values (HBM I and HBM II) for lead in blood of children and adults. Int. J. Hyg. Environ. Health 213, 265–269. doi: 10.1016/j.ijheh.2010.04.002
Wilson, R. L., Buckberry, S., Spronk, F., Laurence, J. A., Leemaqz, S., O’Leary, S., et al. (2015). Vitamin D receptor gene ablation in the conceptus has limited effects on placental morphology, function and pregnancy outcome. PLoS One 10:e0131287. doi: 10.1371/journal.pone.0131287
Wright, L. S., Kornguth, S. E., Oberley, T. D., and Siegel, F. L. (1998). Effects of lead on glutathione s-transferase expression in rat kidney: A dose-response study. Toxicol. Sci. 46, 254–259. doi: 10.1006/toxs.1998.2543
Zhong, S.-L., Zhou, S.-F., Chen, X., Chan, S. Y., Chan, E., Ng, K.-Y., et al. (2006). Relationship between genotype and enzyme activity of glutathione S-transferases M1 and P1 in Chinese. Eur. J. Pharm. Sci. 28, 77–85. doi: 10.1016/j.ejps.2006.01.002
Keywords: placental transfer, birth outcome, perfluoroalkyl substances (PFAS), Bisphenol a (BPA), Mercury (Hg), lead (Pb), genotype-phenotype
Citation: Gundacker C, Graf-Rohrmeister K, Gencik M, Hengstschläger M, Holoman K, Rosa P, Kroismayr R, Offenthaler I, Plichta V, Reischer T, Teufl I, Raffesberg W, Scharf S, Köhler-Vallant B, Delissen Z, Weiß S and Uhl M (2021) Gene Variants Determine Placental Transfer of Perfluoroalkyl Substances (PFAS), Mercury (Hg) and Lead (Pb), and Birth Outcome: Findings From the UmMuKi Bratislava-Vienna Study. Front. Genet. 12:664946. doi: 10.3389/fgene.2021.664946
Received: 06 February 2021; Accepted: 17 May 2021;
Published: 16 June 2021.
Edited by:
José Rueff, New University of Lisbon, PortugalReviewed by:
Ola Hermanson, Karolinska Institutet (KI), SwedenCopyright © 2021 Gundacker, Graf-Rohrmeister, Gencik, Hengstschläger, Holoman, Rosa, Kroismayr, Offenthaler, Plichta, Reischer, Teufl, Raffesberg, Scharf, Köhler-Vallant, Delissen, Weiß and Uhl. This is an open-access article distributed under the terms of the Creative Commons Attribution License (CC BY). The use, distribution or reproduction in other forums is permitted, provided the original author(s) and the copyright owner(s) are credited and that the original publication in this journal is cited, in accordance with accepted academic practice. No use, distribution or reproduction is permitted which does not comply with these terms.
*Correspondence: Claudia Gundacker, Y2xhdWRpYS5ndW5kYWNrZXJAbWVkdW5pd2llbi5hYy5hdA==
†These authors have contributed equally to this work and share first authorship
Disclaimer: All claims expressed in this article are solely those of the authors and do not necessarily represent those of their affiliated organizations, or those of the publisher, the editors and the reviewers. Any product that may be evaluated in this article or claim that may be made by its manufacturer is not guaranteed or endorsed by the publisher.
Research integrity at Frontiers
Learn more about the work of our research integrity team to safeguard the quality of each article we publish.