- School of Public Health, Health Science Center, Xi’an Jiaotong University, Xi’an, China
Objective: This study aims to identify novel candidate genes associated with osteonecrosis of the femoral head (ONFH).
Methods: A transcriptome-wide association study (TWAS) was performed by integrating the genome-wide association study dataset of osteonecrosis (ON) in the UK Biobank with pre-computed mRNA expression reference weights of muscle skeleton (MS) and blood. The ON-associated genes identified by TWAS were further subjected to gene ontology (GO) analysis by the DAVID tool. Finally, a trans-omics comparative analysis of TWAS and genome-wide mRNA expression profiling was conducted to identify the common genes and the GO terms shared by both DNA-level TWAS and mRNA-level expression profile for ONFH.
Results: TWAS totally identified 564 genes that were with PTWAS value <0.05 for MS and blood, such as CBX1 (PTWAS = 0.0001 for MS), SRPK2 (PTWAS = 0.0002 for blood), and MYO5A (PTWAS = 0.0005 for blood). After comparing the genes detected by TWAS with the differentially expressed genes identified by mRNA expression profiling, we detected 59 overlapped genes, such as STEAP4 [PTWAS = 0.0270, FC (fold change)mRNA = 7.03], RABEP1 (PTWAS = 0.010, FCmRNA = 2.22), and MORC3 (PTWAS = 0.0053, FCmRNA = 2.92). The GO analysis of TWAS-identified genes discovered 53 GO terms for ON. Further comparing the GO results of TWAS and mRNA expression profiling identified four overlapped GO terms, including cysteine-type endopeptidase activity (PTWAS = 0.0006, PmRNA = 0.0227), extracellular space (PTWAS = 0.0342, PmRNA = 0.0012), protein binding (PTWAS = 0.0112, PmRNA = 0.0106), and ATP binding (PTWAS = 0.0464, PmRNA = 0.0033).
Conclusion: Several ONFH-associated genes and GO terms were identified by integrating TWAS and mRNA expression profiling. It provides novel clues to reveal the pathogenesis of ONFH.
Introduction
Osteonecrosis (ON) is a common orthopedic disorder, the pathological features of which is the death of bone cells owing to the decrease of blood flow (Assouline-Dayan et al., 2002). Although ON can occur at different skeletal sites, such as the hips, jaw, knees, shoulders, and ankles, the femoral head is the mostly affected one. There are 20,000 to 30,000 new cases of osteonecrosis of the femoral head (ONFH) in the United States every year, of which about 5–10% end up with total hip replacement (Malizos et al., 2007).
Osteonecrosis is a complex multifactorial disease which is affected by both genetic elements and environmental factors (Baek et al., 2017). The risk factors for developing ON include serious trauma, corticosteroid medications, immunosuppressive therapy, autoimmune diseases, and chronic alcohol intake (Mont et al., 2000; Gladman et al., 2001). During the past decades, a number of genetic factors for ON have been conducted, linking specific genes or susceptibility loci to the pathogenesis of ON (Hadjigeorgiou et al., 2008; Karol et al., 2015; Sun et al., 2015; Zhou et al., 2015). For example, a meta-analysis study reported that vascular endothelial growth factors, endothelial nitric oxide synthase, and ATP-binding cassette subfamily B member 1 transporter (ABCB1) polymorphisms were associated with the risk of ONFH (Zhou et al., 2015). Another study observed that glucocorticoid-associated ON was associated with the genetic locus near the glutamate receptor gene (Karol et al., 2015). The R192Q and rs662 polymorphisms in paraoxonase-1 were also reported to increase the susceptibility of ONFH (Hadjigeorgiou et al., 2007; Li et al., 2017). Hypofibrinolysis conferred by the 4G/4G plasminogen activator inhibitor-1 gene variant is a major predisposing factor for avascular ON in renal transplant patients (Ferrari et al., 2002). The genetic polymorphisms in ABCB1 gene (C3435T), apolipo-protein B (ApoB) gene (C7623T), and cAMP-response element binding protein-binding protein (CBP) gene (rs3751845) increased and were helpful for predicting the risk of steroid-induced ONFH (Kuribayashi et al., 2008). However, previous studies mostly focused on single or several gene defects associated with ONFH, and a few large-scale genetic studies of ONFH have been conducted. The genetic mechanism of ON remains elusive now.
Genome-wide association studies (GWAS) is a powerful approach for identifying the susceptibility genes of complex diseases or traits. However, a great number of genetic variants affect complex traits by regulating gene expression and then changing the abundance of one or multiple proteins (Lappalainen et al., 2013; Zhang et al., 2015). For instance, the non-coding regulatory loci, such as expression quantitative trait loci and methylation quantitative traits loci (Grubert et al., 2015), can affect the risk of diseases through regulating the expression levels of disease-related genes. The GWAS-identified genetic loci are mostly located in the non-coding regulatory regions of genome. These causal genetic variants within non-coding regulatory regions are commonly indistinguishable from the neighboring markers and are likely to be missed in previous GWAS (Zhang and Lupski, 2015). In recent years, transcriptome-wide association study (TWAS) has been proposed, which is capable of identifying disease-associated genes at the mRNA expression level (Gusev et al., 2016a). TWAS has been applied to the genetic studies of multiple complex human diseases and presents good performance for disease gene mapping (Gusev et al., 2016b; Thériault et al., 2017). For instance, Gusev et al. (2016b) performed a TWAS of schizophrenia through integrating a GWAS dataset of schizophrenia and mRNA expression references from the brain, blood, and adipose tissues. Finally, they identified 157 schizophrenia-associated genes, of which 35 were novel. Wu et al. (2018) performed a TWAS to evaluate the associations between genetically predicted gene expression level and breast cancer risk and identified 48 candidate genes for breast cancer.
In this study, using the latest GWAS dataset of ON obtained from the UK Biobank, we first conducted a TWAS to scan candidate genes for ON. The ON-associated genes identified by TWAS were further subjected to gene ontology (GO) enrichment analysis by DAVID tool. To validate the TWAS results of ON, we also compared the TWAS results with the mRNA expression profiles of ONFH to identify common genes and GO terms shared by TWAS and mRNA expression profiling.
Materials and Methods
GWAS Summary Dataset of ON
The GWAS summary dataset of ON was driven from the UK Biobank database1 (Bycroft et al., 2018; Canela-Xandri et al., 2018). Briefly, the UK Biobank genetic dataset contains genome-wide genotype data for 452,264 participants, including 603 osteonecrosis patients, as defined by the International Classification of Diseases, Tenth Revision, (ICD-10) code “M87.” DNA was extracted from frozen-stored blood samples and performed for genotyping using the marker content of the UK Biobank Axiom array. The samples were imputed by a new version of the program referred to as IMPUTE4.2 Principal component analysis was applied to account for the population structure in both sample and marker-based quality control. The GWAS summary data contain 623,94 genotyped variants that passed quality control, 9,113,133 imputed variants that passed quality control, all 30,798,054 imputed variants available for downloading, and 9,113,133 imputed variants that passed quality control with a P different than 0 [for detailed information of the subjects, genotyping, imputation, and quality control, refer to the published studies (Bycroft et al., 2018; Canela-Xandri et al., 2018)].
Gene Expression Profile of BMSCs
The mRNA expression profiling data of bone marrow mesenchymal stem cells (BMSCs) of ONFH patients was used here (Wang et al., 2018). Briefly, three patients with steroid-induced ONFH and three control subjects were enrolled from the Department of Orthopedic. ONFH was diagnosed based on preoperative radiographs and magnetic resonance images. Arraystar Human lncRNA microarray V3 (GPL16956), covering 26,109 mRNAs, was used for microarray analysis. Unpaired Student’s t-test was performed to evaluate the differences between the two groups. False discovery rate controlling was used to correct the P-value with Benjamini–Hochberg algorithm in R 3.4.1 suite (Lucent Technologies). Differentially expressed mRNAs were identified at | fold change (FC)| > 2.0 and Benjamini–Hochberg-corrected P values < 0.05. A total of 838 up-regulated mRNAs and 1,937 down-regulated mRNAs were identified in the ONFH group [for a detailed description of samples, experimental design, statistical analysis, and quality control, refer to the previous study (Wang et al., 2018)].
TWAS of ON
TWAS of ON was performed using the FUSION software3 through integrating the UK Biobank ON GWAS summary data and pre-computed gene expression reference weights of peripheral blood, whole blood, and muscle skeleton (Gusev and Ko, 2016). Briefly, the gene expression weights of a certain tissue were first calculated using the prediction models implemented in FUSION. For a given gene, Bayesian sparse linear mixed model (Zhou et al., 2013b) was firstly used to compute SNP expression weights in the 1-Mb cis locus: let w denote weights, Z denote ON Z scores, and L denotes SNP correlation (LD) matrix. The formula “ZTWAS = w′Z/(w′Lw)1/2” was then used to estimate the association between predicted expression and ON (Gusev and Ko, 2016). Finally, we got the gene–disease association by performing the expression imputation on chromosome one by one. In this study, the gene expression reference weight panels of peripheral blood (n = 1,247), whole blood (n = 1,264), and muscle skeleton (n = 361) were downloaded from the FUSION website.4 P value was calculated by FUSION for each gene.
Gene Ontology Enrichment Analysis
The ON-associated genes identified by TWAS were further analyzed by the Database for Annotation, Visualization, and Integrated Discovery (DAVID)5 for GO enrichment (Huang da et al., 2009). The differently expressed mRNA of ONFH was also subjected to GO enrichment analysis. Finally, the GO enrichment results of TWAS and mRNA expression profile were compared to identify common GO terms for ONFH.
Ethics
Our research data was downloaded from an online public database and does not involve ethical issues.
Results
TWAS Results of ON
Transcriptome-wide association study of ON identified 154 genes with P value < 0.05 in MS, such as STPG1 (PTWAS = 0.0015), CTSS (PTWAS = 0.0022), and THEM4 (PTWAS = 0.01). The total 154 significant genes were presented in Supplementary Table 1. We also identified 128 genes with P values < 0.05 in peripheral blood (Supplementary Table 2) and 279 genes with P values < 0.05 in whole blood (Supplementary Table 3), such as GLT25D2 (PTWAS = 0.0078), VAMP4 (PTWAS = 0.0080), USP24 (PTWAS = 0.0022), and LAPTM5 (PTWAS = 0.0027). The top 10 significant genes identified by TWAS for ON are shown in Table 1.
Common Genes Shared by TWAS and mRNA Expression Profiling
We further compared the genes identified by TWAS of ON with the differently expressed genes identified by mRNA expression profiling of ONFH. We detected 59 common genes shared by TWAS and mRNA expression profiling (Supplementary Table 4), such as RGPD8 (PTWAS = 0.0060, FCmRNA = 2.97), RABEP1 (PTWAS = 0.0099, FCmRNA = 2.22), STEAP4 (PTWAS = 0.0273, FCmRNA = 7.03), KDM5A (PTWAS = 0.0202, FCmRNA = 2.17), KLHL24 (PTWAS = 0.0347, FCmRNA = 2.36), and PDLIM1 (PTWAS = 0.0473, FCmRNA = 2.14). The top 10 common genes shared by TWAS and mRNA expression profiling are presented in Table 2.
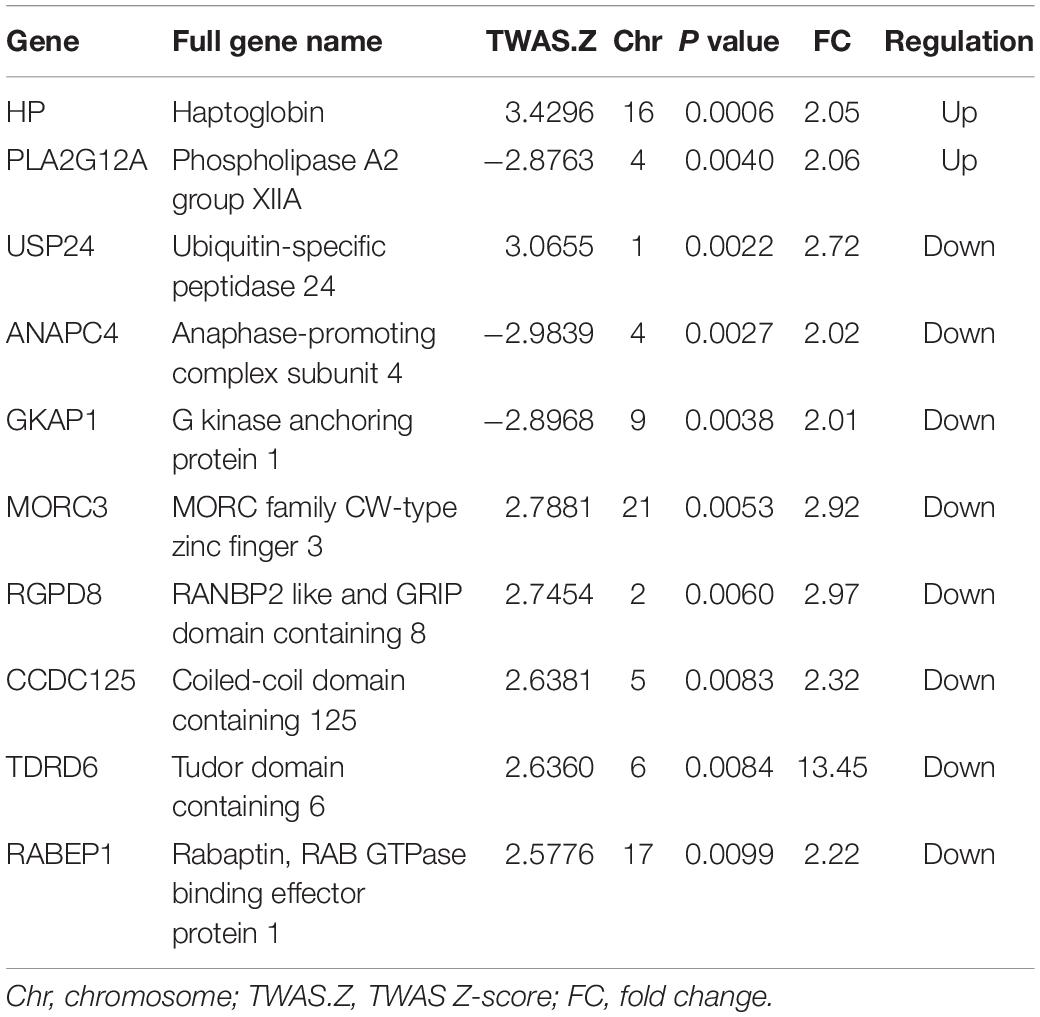
Table 2. Common genes detected by both transcriptome-wide association study (TWAS) and mRNA expression profiles of bone marrow mesenchymal stem cells.
GO Enrichment Analysis Results
Gene ontology enrichment analysis of the genes identified by TWAS detected 53 GO terms with P value < 0.05 for ON, such as mitochondrial matrix (P value = 0.0027), RNA catabolic process (P value = 2.91 × 10–4), and membrane (P value = 0.0096). Further comparing the GO enrichment analysis results of TWAS and mRNA expression profiling detected four common GO terms, including cysteine-type endopeptidase activity (PTWAS = 0.0006, PmRNA = 0.0227), extracellular space (PTWAS = 0.0342, PmRNA = 0.0012), protein binding (PTWAS = 0.0112, PmRNA = 0.0106), and ATP binding (PTWAS = 0.0464, PmRNA = 0.0033).
Discussion
Limited efforts have been paid to explore the genetic mechanism of ONFH by now. The genes implicated in the development of ON remain largely unknown. In this study, we conducted a genome-wide integrative analysis of TWAS and mRNA expression profiling by identifying multiple ONFH-associated genes, such as STEAP4, RABEP1, and MORC3.
STEAP4 encodes a protein that belongs to the six transmembrane epithelial antigens of prostate (STEAP) family and resides in the Golgi apparatus. Previous studies demonstrated that STEAP4 is involved in responses to inflammatory and glucose metabolism (Wellen et al., 2007; ten Freyhaus et al., 2012; Kim et al., 2015). In addition, there is a study which identified that STEAP4 links to inflammation and colon cancer as a critical regulator of mitochondrial dysfunction (Xue et al., 2017). There has been no study report about STEAP4 in ON. However, one study indicated that STEAP4 had a critical role in cellular iron uptake and utilization in osteoclasts and was indispensable for osteoclast development and function (Zhou et al., 2013a). Impaired blood supply to the bone is associated with ON. Therefore, our result suggested that STEAP4 may be a regulator for iron uptake and utilization to link blood circulation and ON.
MORC3 encodes MORC family CW-type zinc finger protein 3, which localizes to the nuclear matrix. A previous study conducted by Jadhav et al. (2016) showed that MORC3 mutant mice exhibit bone cell differentiation. Furthermore, the localization of morc3 protein in (MUT±) osteoclasts and (MUT±) mice in the nuclear membrane to the cytoplasm, the localization of MORC3 protein in MORC3 (mut±) osteoclasts, and MORC3 (mut±) mice transferring from the nuclear membrane to the cytoplasm displayed increased osteoblast differentiation and altered gene expression (Jadhav et al., 2016). Another study demonstrated that MORC3 mutant mice exhibited reduced cortical thickness and area, followed by changed hematopoietic stem cell niche and bone cell differentiation (Hong et al., 2017).
RABEP1 encodes Rab GTPase-binding effector protein 1. It belongs to rabaptin protein family. It has been demonstrated that hypoxia was implicated in the development of bone diseases, including ON (Liu et al., 2015; Yin et al., 2020). A recent study investigated the role of hypoxia and hypoxia-inducible factor 1α (HIF-1α) in fibrodysplasia ossificans progressiva (FOP). They found that HIF-1α could increase the duration and intensity of BMP signaling through RABEP1-mediated retention of ACVR1 in hypoxic connective tissue progenitor cells from FOP patients (Wang et al., 2016). In addition, RABEP1 was identified as one of the novel candidate genes influencing spinal volumetric bone mineral density in rats (Alam et al., 2010).
Gene ontology enrichment analysis detected several GO terms, such as mitochondrial matrix, ATP binding, positive regulation of cell matrix adhesion, and RNA catabolic process. The mitochondrial matrix is the structural basis of energy metabolism and oxidative stress (Cadenas, 2018). A study indicated that steroid-associated mitochondrial injury and redox failure are important elements in the pathogenesis of ON (Tsuchiya et al., 2018). ATP binding is closely related to mitochondrial matrix. ABCB1 polymorphism contributes to the risk of ONFH (Zhou et al., 2015; Zhang et al., 2017). The positive regulation of cell matrix adhesion was identified to be implicated in the regulation of hypoxia (Zhang et al., 2018), which is one of the factors that cause apoptosis of bone cells (Seamon et al., 2012). In addition, RNA catabolic process is another identified GO enrichment term. Previous studies have demonstrated that RNA stability provides a rapid level of regulation that can have major effects in maintaining global inflammation (Herman and Autieri, 2018; Nyati et al., 2020).
To the best of our knowledge, this is the first TWAS of ON and identified multiple candidate genes whose imputed mRNA expression levels were associated with ON. To enhance the reliability and persuasiveness of our study, we further compared the TWAS results with the mRNA expression profiling of ONFH. We identified multiple common genes and GO terms shared by both DNA-level TWAS and mRNA expression profiling for ON. Despite that the power of TWAS is great, there are also two limitations that should be noted. Firstly, although TWAS is not confounded by reverse causality (disease→ expression independent of SNP), it is well nigh impossible to draw the instances of pleiotropy in statistics (where a SNP or linked SNPs affect ON and expression independently) and truly causal susceptibility genes. Secondly, there is some heterogeneity between GWAS data and gene expression profile. In detail, the GWAS data originate from European ON participants defined by ICD codes, while the gene expression data originate steroid-induced ONFH of Chinese ancestry. There has been lack of data set from the same ancestry and samples. Therefore, one should be careful in applying our study results, and further studies are needed to confirm our findings.
In summary, we conducted a genome-wide integrative analysis of TWAS and mRNA expression profiling of ON. We identified multiple candidate genes and their biological terms associated with ON. Our results provide novel clues for clarifying the pathogenesis of ON.
Data Availability Statement
The original contributions presented in the study are included in the article/Supplementary Material, further inquiries can be directed to the corresponding author.
Ethics Statement
Ethical review and approval was not required for the study on human participants in accordance with the local legislation and institutional requirements. The patients/participants provided their written informed consent to participate in this study.
Author Contributions
MM and PL carried out the rSNP analysis and drafted the manuscript. LL, SC, BC, CL, ST, WL, YW, XG, and CW participated in its design and helped to draft the manuscript. All authors read and approved the final manuscript.
Funding
This work was supported by the National Natural Scientific Foundation of China (82073495).
Conflict of Interest
The authors declare that the research was conducted in the absence of any commercial or financial relationships that could be construed as a potential conflict of interest.
Supplementary Material
The Supplementary Material for this article can be found online at: https://www.frontiersin.org/articles/10.3389/fgene.2021.663080/full#supplementary-material
Footnotes
- ^ http://geneatlas.roslin.ed.ac.uk/
- ^ https://jmarchini.org/software/
- ^ http://gusevlab.org/projects/fusion/
- ^ http://gusevlab.org/projects/fusion/
- ^ https://david.ncifcrf.gov/
References
Alam, I., Sun, Q., Koller, D. L., Liu, L., Liu, Y., Edenberg, H. J., et al. (2010). Genes influencing spinal bone mineral density in inbred F344, LEW, COP, and DA rats. Funct. Integr. Genom. 10, 63–72. doi: 10.1007/s10142-009-0147-6
Assouline-Dayan, Y., Chang, C., Greenspan, A., Shoenfeld, Y., and Gershwin, M. E. (2002). Pathogenesis and natural history of osteonecrosis. Semin. Arthritis Rheum 32, 94–124.
Baek, S. H., Kim, K. I., Yoon, K. S., Kim, T. H., and Kim, S. Y. (2017). Genome-wide association scans for idiopathic osteonecrosis of the femoral head in a Korean population. Mol. Med. Rep. 15, 750–758. doi: 10.3892/mmr.2016.6036
Bycroft, C., Freeman, C., Petkova, D., Band, G., Elliott, L. T., Sharp, K., et al. (2018). The UK Biobank resource with deep phenotyping and genomic data. Nature 562, 203–209. doi: 10.1038/s41586-018-0579-z
Cadenas, S. (2018). Mitochondrial uncoupling, ROS generation and cardioprotection. Biochim. Biophys. Acta Bioenergy 1859, 940–950. doi: 10.1016/j.bbabio.2018.05.019
Canela-Xandri, O., Rawlik, K., and Tenesa, A. (2018). An atlas of genetic associations in UK Biobank. Nat. Genet. 50, 1593–1599. doi: 10.1038/s41588-018-0248-z
Ferrari, P., Schroeder, V., Anderson, S., Kocovic, L., Vogt, B., Schiesser, D., et al. (2002). Association of plasminogen activator inhibitor-1 genotype with avascular osteonecrosis in steroid-treated renal allograft recipients. Transplantation 74, 1147–1152. doi: 10.1097/00007890-200210270-00016
Gladman, D. D., Urowitz, M. B., Chaudhry-Ahluwalia, V., Hallet, D. C., and Cook, R. J. (2001). Predictive factors for symptomatic osteonecrosis in patients with systemic lupus erythematosus. J. Rheum. 28, 761–765.
Grubert, F., Zaugg, J. B., Kasowski, M., Ursu, O., Spacek, D. V., Martin, A. R., et al. (2015). Genetic control of chromatin states in humans involves local and distal chromosomal interactions. Cell 162, 1051–1065. doi: 10.1016/j.cell.2015.07.048
Gusev, A., and Ko, A. (2016). Integrative approaches for large-scale transcriptome-wide association studies. Nat. Genet. 48, 245–252.
Gusev, A., Ko, A., Shi, H., Bhatia, G., Chung, W., Penninx, B. W., et al. (2016a). Integrative approaches for large-scale transcriptome-wide association studies. Nat. Genet. 48, 245–252. doi: 10.1038/ng.3506
Gusev, A., Mancuso, N., Finucane, H. K., Reshef, Y., Song, L., Safi, A., et al. (2016b). Transcriptome-wide association study of schizophrenia and chromatin activity yields mechanistic disease insights. bioRxiv [Preprint]. doi: 10.1101/067355
Hadjigeorgiou, G., Dardiotis, E., Dardioti, M., Karantanas, A., Dimitroulias, A., and Malizos, K. (2008). Genetic association studies in osteonecrosis of the femoral head: mini review of the literature. Skeletal. Radiol. 37, 1–7. doi: 10.1007/s00256-007-0395-2
Hadjigeorgiou, G. M., Malizos, K., Dardiotis, E., Aggelakis, K., Dardioti, M., Zibis, A., et al. (2007). Paraoxonase 1 gene polymorphisms in patients with osteonecrosis of the femoral head with and without cerebral white matter lesions. J. Orthop. Res. 25, 1087–1093. doi: 10.1002/jor.20393
Herman, A. B., and Autieri, M. V. (2018). Cardiovascular disease, inflammation, and mRNA stability. Aging (Albany N.Y.) 10, 3046–3047. doi: 10.18632/aging.101619
Hong, G., Qiu, H., Wang, C., Jadhav, G., Wang, H., Tickner, J., et al. (2017). The emerging role of MORC family proteins in cancer development and bone homeostasis. J. Cell Physiol. 232, 928–934. doi: 10.1002/jcp.25665
Huang da, W., Sherman, B. T., and Lempicki, R. A. (2009). Bioinformatics enrichment tools: paths toward the comprehensive functional analysis of large gene lists. Nucleic Acids Res. 37, 1–13. doi: 10.1093/nar/gkn923
Jadhav, G., Teguh, D., Kenny, J., Tickner, J., and Xu, J. (2016). Morc3 mutant mice exhibit reduced cortical area and thickness, accompanied by altered haematopoietic stem cells niche and bone cell differentiation. Sci. Rep. 6:25964. doi: 10.1038/srep25964
Karol, S. E., Yang, W., Van Driest, S. L., Chang, T. Y., Kaste, S., Bowton, E., et al. (2015). Genetics of glucocorticoid-associated osteonecrosis in children with acute lymphoblastic leukemia. Blood 126, 1770–1776. doi: 10.1182/blood-2015-05-643601
Kim, H. Y., Park, S. Y., Lee, M. H., Rho, J. H., Oh, Y. J., Jung, H. U., et al. (2015). Hepatic STAMP2 alleviates high fat diet-induced hepatic steatosis and insulin resistance. J. Hepatol. 63, 477–485. doi: 10.1016/j.jhep.2015.01.025
Kuribayashi, M., Fujioka, M., Takahashi, K. A., Arai, Y., Hirata, T., Nakajima, S., et al. (2008). Combination analysis of three polymorphisms for predicting the risk for steroid-induced osteonecrosis of the femoral head. J. Orthop. Sci. 13, 297–303. doi: 10.1007/s00776-008-1244-4
Lappalainen, T., Sammeth, M., Friedlander, M. R., t Hoen, P. A., Monlong, J., Rivas, M. A., et al. (2013). Transcriptome and genome sequencing uncovers functional variation in humans. Nature 501, 506–511. doi: 10.1038/nature12531
Li, J. M., Li, Y., and Wang, L. (2017). The genetic association between PON1 polymorphisms and osteonecrosis of femoral head: a case-control study. Med. (Baltimore) 96:e8198. doi: 10.1097/md.0000000000008198
Liu, Y., Jiang, W., Liu, S., Su, X., and Zhou, S. (2015). Combined effect of tnf-α polymorphisms and hypoxia on steroid-induced osteonecrosis of femoral head. Int. J. Clin. Exp. Pathol. 8, 3215–3219.
Malizos, K. N., Karantanas, A. H., Varitimidis, S. E., Dailiana, Z. H., Bargiotas, K., and Maris, T. (2007). Osteonecrosis of the femoral head: etiology, imaging and treatment. Eur. J. Radiol. 63, 16–28. doi: 10.1016/j.ejrad.2007.03.019
Mont, M. A., Payman, R. K., Laporte, D. M., Petri, M., Jones, L. C., and Hungerford, D. S. (2000). Atraumatic osteonecrosis of the humeral head. J. Rheum. 27, 1766–1773.
Nyati, K. K., Zaman, M. M., Sharma, P., and Kishimoto, T. (2020). Arid5a, an RNA-binding protein in immune regulation: RNA stability, inflammation, and autoimmunity. Trends Immunol. 41, 255–268. doi: 10.1016/j.it.2020.01.004
Seamon, J., Keller, T., Saleh, J., and Cui, Q. (2012). The pathogenesis of nontraumatic osteonecrosis. Arthritis 2012, 601763–601763. doi: 10.1155/2012/601763
Sun, J., Wen, X., Jin, F., Li, Y., Hu, J., and Sun, Y. (2015). Bioinformatics analyses of differentially expressed genes associated with bisphosphonate-related osteonecrosis of the jaw in patients with multiple myeloma. Onco Targets Ther. 8, 2681–2688. doi: 10.2147/ott.s88463
ten Freyhaus, H., Calay, E. S., Yalcin, A., Vallerie, S. N., Yang, L., Calay, Z. Z., et al. (2012). Stamp2 controls macrophage inflammation through nicotinamide adenine dinucleotide phosphate homeostasis and protects against atherosclerosis. Cell Metab. 16, 81–89. doi: 10.1016/j.cmet.2012.05.009
Thériault, S., Gaudreault, N., Lamontagne, M., Messika-Zeitoun, D., Clavel, M.-A., Capoulade, R., et al. (2017). A transcriptome-wide association study identifies PALMD as a susceptibility gene for calcific aortic valve stenosis. bioRxiv [Preprint] doi: 10.1101/184945
Tsuchiya, M., Ichiseki, T., Ueda, S., Ueda, Y., Shimazaki, M., Kaneuji, A., et al. (2018). Mitochondrial stress and redox failure in steroid-associated osteonecrosis. Int. J. Med. Sci. 15, 205–209. doi: 10.7150/ijms.22525
Wang, H. T., Lindborg, C., Lounev, V., Kim, J. H., McCarrick-Walmsley, R., Xu, M. Q., et al. (2016). Cellular hypoxia promotes heterotopic ossification by amplifying BMP signaling. J. Bone Miner. Res. 31, 1652–1665. doi: 10.1002/jbmr.2848
Wang, Q., Yang, Q., Chen, G., Du, Z., Ren, M., Wang, A., et al. (2018). LncRNA expression profiling of BMSCs in osteonecrosis of the femoral head associated with increased adipogenic and decreased osteogenic differentiation. Sci. Rep. 8:9127. doi: 10.1038/s41598-018-27501-2
Wellen, K. E., Fucho, R., Gregor, M. F., Furuhashi, M., Morgan, C., Lindstad, T., et al. (2007). Coordinated regulation of nutrient and inflammatory responses by STAMP2 is essential for metabolic homeostasis. Cell 129, 537–548. doi: 10.1016/j.cell.2007.02.049
Wu, L., Shi, W., Long, J., and Guo, X. (2018). A transcriptome-wide association study of 229,000 women identifies new candidate susceptibility genes for breast cancer. Nat. Genet. 50, 968–978. doi: 10.1038/s41588-018-0132-x
Xue, X., Bredell, B. X., Anderson, E. R., Martin, A., Mays, C., Nagao-Kitamoto, H., et al. (2017). Quantitative proteomics identifies STEAP4 as a critical regulator of mitochondrial dysfunction linking inflammation and colon cancer. Proc. Natl. Acad. Sci.U.S.A. 114, E9608–E9617. doi: 10.1073/pnas.1712946114
Yin, B. H., Chen, H. C., Zhang, W., Li, T. Z., Gao, Q. M., and Liu, J. W. (2020). Effects of hypoxia environment on osteonecrosis of the femoral head in Sprague-Dawley rats. J. Bone Miner. Metab. 38, 780–793. doi: 10.1007/s00774-020-01114-0
Zhang, F., and Lupski, J. R. (2015). Non-coding genetic variants in human disease. Hum. Mol. Genet. 24, R102–R110. doi: 10.1093/hmg/ddv259
Zhang, K., Kong, X., Feng, G., Xiang, W., Chen, L., Yang, F., et al. (2018). Investigation of hypoxia networks in ovarian cancer via bioinformatics analysis. J. Ovarian Res. 11:16. doi: 10.1186/s13048-018-0388-x
Zhang, X., Joehanes, R., and Chen, B. H. (2015). Identification of common genetic variants controlling transcript isoform variation in human whole blood. Nat Genet 47, 345–352. doi: 10.1038/ng.3220
Zhang, Y., Xie, H., Zhao, D., Wang, B., Yang, L., and Meng, Q. (2017). Association of ABCB1 C3435T polymorphism with the susceptibility to osteonecrosis of the femoral head: a meta-analysis. Med. (Baltimore) 96:e6049. doi: 10.1097/md.0000000000006049
Zhou, J., Ye, S., Fujiwara, T., Manolagas, S. C., and Zhao, H. (2013a). Steap4 plays a critical role in osteoclastogenesis in vitro by regulating cellular iron/reactive oxygen species (ROS) levels and cAMP response element-binding protein (CREB) activation. J. Biol. Chem. 288, 30064–30074. doi: 10.1074/jbc.M113.478750
Zhou, X., Carbonetto, P., and Stephens, M. (2013b). Polygenic modeling with bayesian sparse linear mixed models. PLos Genet. 9:e1003264. doi: 10.1371/journal.pgen.1003264
Keywords: genome wide association study (GWAS), mRNA, disease-associated genes, osteonecrosis, transcriptome-wide association study (TWAS)
Citation: Ma M, Li P, Liu L, Cheng S, Cheng B, Liang C, Tan S, Li W, Wen Y, Guo X and Wu C (2021) Integrating Transcriptome-Wide Association Study and mRNA Expression Profiling Identifies Novel Genes Associated With Osteonecrosis of the Femoral Head. Front. Genet. 12:663080. doi: 10.3389/fgene.2021.663080
Received: 02 February 2021; Accepted: 29 April 2021;
Published: 07 June 2021.
Edited by:
Jordi Pérez-Tur, Superior Council of Scientific Investigations (CSIC), SpainReviewed by:
Yan Gong, University of Florida, United StatesFang Fang Yu, Zhengzhou University, China
Copyright © 2021 Ma, Li, Liu, Cheng, Cheng, Liang, Tan, Li, Wen, Guo and Wu. This is an open-access article distributed under the terms of the Creative Commons Attribution License (CC BY). The use, distribution or reproduction in other forums is permitted, provided the original author(s) and the copyright owner(s) are credited and that the original publication in this journal is cited, in accordance with accepted academic practice. No use, distribution or reproduction is permitted which does not comply with these terms.
*Correspondence: Cuiyan Wu, d3VjdWl5YW5AeGp0dS5lZHUuY24=
†These authors have contributed equally to this work