- 1Institute of Animal Breeding and Genetics, Justus-Liebig-University Gießen, Gießen, Germany
- 2Chair of Animal Breeding, Technische Universität München, Freising-Weihenstephan, Germany
- 3School of Science and Technics of Animal Production, Faculty of Agricultural Sciences, University of Abomey-Calavi, Abomey-Calavi, Benin
The Dwarf Lagune and the Savannah Somba cattle in Benin are typical representatives of the endangered West African indigenous Shorthorn taurine. The Lagune was previously exported to African and European countries and bred as Dahomey cattle, whereas the Somba contributed to the formation of two indigenous hybrids known as Borgou and Pabli cattle. These breeds are affected by demographic, economic, and environmental pressures in local production systems. Considering current and historical genomic data, we applied a formal test of admixture, estimated admixture proportions, and computed genomic inbreeding coefficients to characterize the five breeds. Subsequently, we unraveled the most recent selection signatures using the cross-population extended haplotype homozygosity approach, based on the current and historical genotypes. Results from principal component analyses and high proportion of Lagune ancestry confirm the Lagune origin of the European Dahomey cattle. Moreover, the Dahomey cattle displayed neither indicine nor European taurine (EUT) background, but they shared on average 40% of autozygosity from common ancestors, dated approximately eight generations ago. The Lagune cattle presented inbreeding coefficients larger than 0.13; however, the Somba and the hybrids (Borgou and Pabli) were less inbred (≤0.08). We detected evidence of admixture in the Somba and Lagune cattle, but they exhibited a similar African taurine (AFT) ancestral proportion (≥96%) to historical populations, respectively. A moderate and stable AFT ancestral proportion (62%) was also inferred for less admixed hybrid cattle including the Pabli. In contrast, the current Borgou samples displayed a lower AFT ancestral proportion (47%) than historical samples (63%). Irrespective of the admixture proportions, the hybrid populations displayed more selection signatures related to economic traits (reproduction, growth, and milk) than the taurine. In contrast, the taurine, especially the Somba, presented several regions known to be associated with adaptive traits (immunity and feed efficiency). The identified subregion of bovine leukocyte antigen (BoLA) class IIb (including DSB and BOLA-DYA) in Somba cattle is interestingly uncommon in other African breeds, suggesting further investigations to understand its association with specific adaptation to endemic diseases in Benin. Overall, our study provides deeper insights into recent evolutionary processes in the Beninese indigenous cattle and their aptitude for conservation and genetic improvement.
Introduction
Western Africa represents a reservoir of the unique and diverse African animal genetic resources, due to a complex history including migration, dispersion, natural and artificial selection, and crossbreeding (Hanotte et al., 2002; Stock and Gifford-Gonzalez, 2013). The region is the exclusive current habitat for indigenous taurine cattle (Bos taurus) on the continent (Mwai et al., 2015). African humpless taurine cattle are the earliest known cattle populations on the continent according to historical and archaeological evidence (Blench and MacDonald, 1999). These animals share early common ancestors with European taurine (EUT) cattle dated to before the domestication process (Ho et al., 2008; Murray et al., 2010). They are subdivided into two subgroups, the Longhorn and the Shorthorn taurine. The origins of the two populations are still controversial, but it is scientifically accepted that they have been separately introduced into West Africa several millennia BC (Epstein, 1971; Payne and Hodges, 1997; Hanotte et al., 2002). The N’Dama is the unique reported Longhorn taurine breed, whereas the humpless Shorthorns include the Savannah breeds (Baoulé, Doayo, and Somba) and the Dwarf forest breeds (Dwarf Muturu, Liberian Dwarf, and Lagune), which are barely characterized across West African countries (Rege, 1999).
The Somba and Lagune represent indigenous African Savannah and Dwarf taurine in Benin, respectively. Both are characterized by stocky animals, resistant to diseases (especially trypanosome), and able to survive and produce in harsh environments (Dossa and Vanvanhossou, 2016; Ahozonlin et al., 2020). The Somba cattle are the typical ancestral residue of West African Savannah Shorthorns that migrated to Togo, Ghana, and the Ivory-coast (Rege, 1999). They have been preserved from admixture until recent past in the Atacora mountain area in Northwest Benin (Hall et al., 1995; Rege, 1999). The Lagune cattle are present not only in Southern Benin but also in the coastal areas and near lagoons in West and Central Africa, as indicated by their name (Rege et al., 1994). The Lagune are described as the smallest of the African taurine (AFT) cattle (93 cm of average height at withers) and acquired their Dwarf phenotype through adaptation to environmental constraints in their belt (Rege et al., 1994). According to previous studies, this breed is genetically different from other Shorthorn cattle breeds (Moazami Goudarzi et al., 2001; Gautier et al., 2009). Berthier et al. (2015) reported higher trypanotolerance with lesser anemic condition in Lagune animals in comparison to the Baoule. However, genomic regions affected by divergent selection and environmental adaptation of the Lagune and the Somba remain unknown.
The Lagune cattle from Benin had been exported during the early 20th century (around 1904) to different African countries such as Zaire (current Democratic Republic of Congo), Zambia, Gabon, as well as to Europe where they are known as Dahomey cattle (Rege et al., 1994; Porter et al., 2016), because Dahomey is the former name of the country of Benin. The European Dahomey cattle are presently kept and bred by a breeder association involving 77 farmers (Verband Europäisches Dahomey-Zwergrind, 2019) from four European countries including Germany, Austria, Czech Republic, and Switzerland (http://www.dahomey-zwergrind.com). To date, neither scientific study nor census addressed these animals. According to the breeder association, the current population is characterized by a small size (adult body weight between 150 and 300 kg and sacrum height from 80 to 105 cm), short horn, easy calving, and good temperament. These characteristics are similar to those of the original Lagune population in Benin and may indicate that the Dahomey population conserved its purity through the past decades. However, the European climate is different from the one encountered in Benin, i.e., less heat stress, but varying temperatures between winter and summer, and also varying sunlight duration. In addition, the new production environment of the Dahomey cattle implies the reduction of disease infections with potentially improved feeding and housing systems in opposition to the harsh production conditions in Benin (characterized by food and water scarcity and the risk for disease infections). Consequently, this geographical isolation may have altered frequencies for alleles and haplotypes associated with specific genetic features in the Dahomey population.
The livestock production systems in Benin like in the other African countries have experienced a drastic transformation in the last decades (Mwai et al., 2015; Houessou et al., 2019a). New challenges arise in African pastoral regions along with the increasing demand for animal products, food insecurity, and poverty. Indeed, demographic explosion increases the pressures on animal product markets in West Africa and accentuates the need to develop more productive breeds. Simultaneously, anthropogenic activities such as deforestation and urbanization associated with climate changes have shrunk feed and water resources and increased disease challenges, forcing herders to develop new breeding strategies such as herd mobility or feed supplementation (Houessou et al., 2019a; Ahozonlin and Dossa, 2020). In this context, locally adapted animals are required to cope with the various instabilities in production environments, but the indigenous Shorthorn cattle in Benin are increasingly threatened. The trypanotolerant taurine cattle (without any Zebu ancestry) reported in the region by MacHugh et al. (1997) and Hanotte et al. (2002) are progressively replaced by crossbreeds and trypanosusceptible Zebu cattle, including White Fulani, Sokoto Gudali, and Red Bororo (Dossa and Vanvanhossou, 2016; Houessou et al., 2019b; Ahozonlin and Dossa, 2020). In consequence, significant adoption of prophylactic measures is observed in Beninese herds dominated by crossbreed and Zebu cattle in comparison to other local herds (Houessou et al., 2020).
In regard to the increasing uncontrolled crossbreeding, two other indigenous hybrid cattle, the Borgou and Pabli, are also endangered in Benin. The Pabli originally reported in the region of Kerou (Northwest Benin) is scarcely described and is considered as extinct by absorption from crossbreeding with Borgou (Belemsaga et al., 2005; Egito et al., 2007). However, recent evaluations revealed the existence of a population with slight genetic differences in comparison to the Borgou (Scheper et al., 2020). The Borgou cattle mainly located in the Nord-Eastern and Central regions of Benin were described as an intermediate crossbreed between taurine and indicine cattle (Porter, 1991). The origin of Borgou is still under discussion, but it is assumed to be a product of an admixture between the taurine Somba and the White Fulani Zebu (Porter, 1991; Belemsaga et al., 2005). Flori et al. (2014) characterized the admixture in Borgou as an efficient short-term adaptation strategy to environmental conditions and disease pressures and identified different genomic regions involved in adaptive mechanisms. However, the Borgou cattle population is now highly affected by Zebu cattle influence due to admixture (Scheper et al., 2020). The increased crossbreeding with trypanosusceptible Zebu cattle over a short period questions adaptive features such as resistance to diseases in Borgou, Somba, and Lagune taurine populations. It is therefore urgent to gain more insight into the genetic composition of the current Beninese cattle population to ensure the sustainability of livestock production.
To understand the genetic architecture underlying adaptive and productive abilities of various breeds evolving in challenging environments, selection signature analyses have the potential to detect specific genomic footprints in terms of differences in marker allele frequencies or in haplotypic mosaicism (Qanbari and Simianer, 2014; Aliloo et al., 2020; Kim et al., 2020). According to Freedman et al. (2016), admixture and subsequent recombination break down parental haplotypes and expand mosaic regions through the genome. Thus, extensive admixture in local breeds may reduce the signal of strong homozygosity of extended haplotypes involved in adaptive processes. Moreover, the assessment of homozygous-by-descent (HBD) segments or runs of homozygosity (ROH) is valuable to describe a population and investigate demographic histories. HBD are chromosome segments inherited from an ancestor and may be exploited to estimate inbreeding (Leutenegger et al., 2003).
In this study, we aimed to genetically characterize the current endangered cattle breeds in Benin and evaluate the effects of admixture and environmental factors related to late changes in production systems. Specifically, we first assessed the admixture level in the different populations and compared them with historical samples. Second, through selection signature analysis, we investigated genomic regions and biological mechanisms involved or affected by recent natural or artificial selection and admixture in the Beninese cattle breeds. Subsequently, we investigated the genetic differentiation resulting from the isolation of the Dahomey cattle.
Materials and Methods
Sampling Design and Genotype Data
Hair samples were collected from 449 animals from the four local breeds (Borgou 181, Pabli-Kerou 58, Lagune 150, and Somba 60) in Beninese local farms in 2016 and 2017. The sampling locations were identified ensuring a large coverage of the main geographical distribution of the breeds in the country (Figure 1). In each herd, one animal representing a (pure) local breed was identified by the farmer and selected. To reduce the relatedness between the samples, one or two animals per village were sampled in 90% of the 298 investigated villages. In the remaining villages, additional animals (three to ten animals in total per village) were sampled for the assessment of further socioeconomic and ecological factors including transhumance and climate (see Supplementary Table S1A and Scheper et al. (2020) for more details). Furthermore, thirty Dahomey cattle were sampled in 2019 in 30 different herds across Europe (Germany 23, Austria 4, Switzerland 2, and Czech Republic 1).
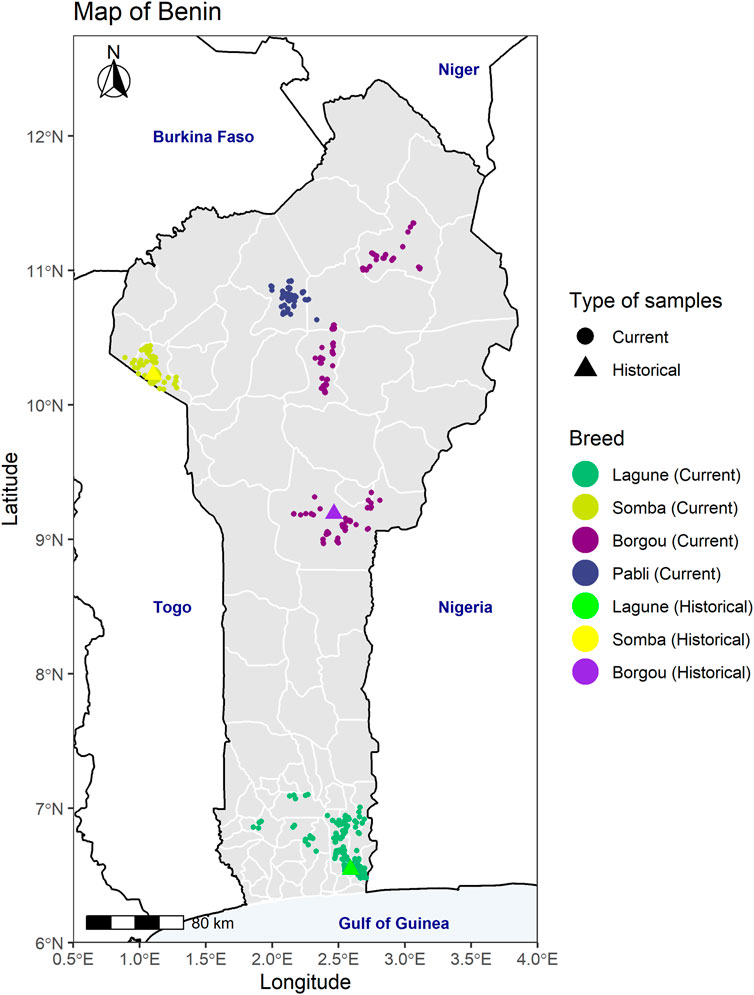
FIGURE 1. Map of Benin with the sampling locations of the different Beninese cattle populations investigated in the country. For the historical data, the geographical coordinates of each sample are not available. In consequence, the coordinates of the sampling regions as described by Moazami Goudarzi et al. (2001) were plotted: Porto-Novo for the Lagune, Boukombé for the Somba, and the department of Borgou for the Borgou samples.
The sampled animals were genotyped with the Illumina BovineSNP50 BeadChip. SNP variants with a call rate <95% and a minor allele frequency (MAF < 0.01) as well as animals with a high percentage of missing genotypes (call rate < 90%) were filtered out in PLINK (Purcell et al., 2007). The final genotype dataset including 40,109 SNP from 460 cattle was used for different analyses.
Description of the Current Beninese Cattle Population
Population Structure
The genetic structure of our samples was first assessed by applying the fastStructure software (Raj et al., 2014). The algorithm was run using the simple prior model and testing different numbers of populations (K) ranging from 2 to 10. Afterward, the optimal K was defined using the chooseK.py function. The identified optimal K was finally incorporated into the logistic prior model, to estimate individual genetic proportions describing the population structure, following the recommendations by Raj et al. (2014).
Second, we applied the unsupervised k-means clustering in the “adegenet” R package (Jombart, 2008; Jombart and Ahmed, 2011) to define homogeneous genetic clusters and to exclude potential outgroups animals from the breeds due to sampling. The find.clusters function was applied, considering the following parameters: maximum number of clusters (max.n.clust) = 100, number of principal components (n.pca) = 100, number of iterations in each run (n.iter) = 109, number of starting centroids in each run (n.start) = 30, and the default parameters for the remaining arguments (see package description for further details, Jombart, 2008; Jombart and Ahmed, 2011). Finally, the optimal number of genetic clusters was defined by choosing the k-value based on the Bayesian information criterion (BIC), as recommended by the authors (Jombart 2008; Jombart and Ahmed 2011).
Genome-Wide Inbreeding Coefficients
We utilized the “RzooRoH” package (Druet and Gautier, 2017; Bertrand et al., 2019) in R to identify HBD and infer the contribution of ancestors from different past generations to inbreeding in our current cattle populations. The software applies a hidden Markov model (HMM) to fit the individual genome as a succession of HBD and non-HBD segments, considering marker allele frequencies, genotyping error rates, and intermarker genetic distances (Druet et al., 2020). In a multiple HBD class model, HBD segments are assigned to K age-related classes associated with different rates (Rk). The class rates (Rk) are related to an expected length and exponential distribution of HBD segments. They are equivalent to twice the number of generations to the common ancestor. The proportion of the genome belonging to a specific HBD class is defined as “realized autozygosity” in the respective HBD class. Similarly, the genome-wide inbreeding coefficient is estimated as the cumulative fraction of the genome that is HBD in the current population with respect to an ancestral base population. As suggested by the authors of the “RzooRoH” package (Druet and Gautier, 2017; Bertrand et al., 2019), we applied a default “MixKR” model with 10 classes (9 HBD classes and 1 non-HBD class) and predefined rates (Rk) 2, 4, 8, 16, 32, 64, 128, 256, 512, and 512, respectively.
Comparison of Current Beninese Cattle Population With Historical Data
Extension of the Genotype Dataset With Historical Data
We contrast the genotype of the current Beninese cattle population with available historical genotypes, retrieved from the WIDDE database (Sempéré et al., 2015). In a first step, the genotype data of 133 animals from Beninese cattle breeds (Borgou 45, Lagune 44, and Somba 44) sampled in different locations (see Figure 1 and Supplementary Table S1B) between 1997 and 2000 (Moazami Goudarzi et al., 2001; Gautier et al., 2009) were merged to the genotype data of our new samples. The dataset with 42,802 SNP from 588 animals was submitted to quality control in PLINK (Purcell et al., 2007), with the parameters --geno 0.05, --mind 0.25, and --maf 0.01. After quality control, the final genotype dataset including 32,533 SNP from 586 cattle was used to apply principal component analysis and unsupervised k-means clustering. Second, further African Shorthorn taurine breed (Baoulé), African Longhorn taurine breed (N’Dama), African indicine breeds (Zebu White Fulani and Zebu Bororo), Asian indicine breeds (Gir, Brahman, Ongole, and Nellore), EUT breeds (Angus, Holstein, Charolais, Shorthorn, and Salers), and African hybrid (Kuri) were included. This extension resulted in 52,341 SNP from 997 animals. However, only 30,637 SNP from 997 animals passed the quality control procedure (with the parameters --geno 0.05, --mind 0.25, and --maf 0.01 in PLINK) and were considered in the principal component analysis, unsupervised k-means clustering, and admixture tests (see below for more details). Finally, 21 Gayal (Bos frontalis, Gao et al., 2017) samples were added to the previously extended dataset for the estimation of admixture proportion through the calculation of the f4-ratio. Likewise, we used the same filtering parameters (--geno 0.05, --mind 0.25, and --maf 0.01) in PLINK. The genotype data consisted of 52,364 SNP and 30,228 SNP from 1,018 animals before and after quality control, respectively. All the genotype data exploited in this study are fully described and available in a public repository (see the section “Data Availability Statement”). Before being merged with our samples, the historical genotype data were remapped with the current reference assembly ARS-UCD1.2/bosTau9 (GenBank Bioproject PRJNA391427) and flipped (with the --flip flag in PLINK) to correct for strand inconsistency (Purcell et al., 2007).
Principal Component Analyses and Clustering
The genetic divergence between the Beninese cattle populations and other AFT was assessed using the principal component analysis (PCA) in PLINK (Purcell et al., 2007) and using the unsupervised k-means clustering in “adegenet” (Jombart, 2008; Jombart and Ahmed, 2011) in R. The analyses were subsequently repeated considering EUT and indicine breeds in order to investigate potential introgression of these breeds in our samples, especially in Dahomey. The optimal number of genetic clusters for the unsupervised k-means clustering was defined using the same approach as described above.
Admixture and Estimation of Ancestral Proportion
We tested admixture and inferred admixture proportion in the current and historical Beninese indigenous cattle populations by means of the three-population test (F3) and the F4-ratio estimation in Admixtools, respectively (Patterson et al., 2012). The methods are based on f-statistics, corresponding to the average of F values over markers. The Admixtools software uses allele frequencies of the available samples to estimate unbiased f3 and f4 statistics (see Patterson et al., 2012, for more details). The three-population test is a formal test of admixture. Negative f3 (X; B, C) indicates that the allele frequencies in population X tend to be intermediate between B and C and indicates admixture in X populations from populations related to B and C. The f4 statistics were used to infer admixture proportions in the Beninese cattle populations (target populations) through the calculation of the F4-ratio or alpha (Eq. 3; Patterson et al., 2012). We considered the phylogeny model introduced by Flori et al. (2014) to estimate alpha, as AFT ancestral proportions in our target populations.
where X is the target population, A is the Salers population as EUT ancestral, O is the Gayal as outgroup population, B is Baoulé (BAO) as the Shorthorn AFT reference population, and C is Gir as the indicine reference population.
Detection of Regions Under Recent Selection—Gene Annotation and QTL Mapping
We investigated divergence in extended haplotype homozygosity between the current and historical Beninese cattle populations, in order to detect positive selection signatures or genomic footprints left by recent demographic events. Specifically, we compared DAH_cur, LA_cur, and LA_out with LA_hist; SO_cur with SO_hist; and BO_cur and Adm_cur with BO_hist. The cross-population–extended haplotype homozygosity (XP-EHH) approach (Sabeti et al., 2002) was implemented in the “Rehh” package in R (version 3.1.2; Gautier and Vitalis, 2012; Gautier et al., 2017). Prior to the analyses, the main genotype was phased and missing variants were imputed in Beagle 5.1 (Browning et al., 2018). Afterward, integrated site-specific extended haplotype homozygosity (iES) was calculated for each focal marker in the respective population with the “Rehh” package. The XP-EHH statistics were computed as the standardized log ratio of the iES of the two populations. One-sided p-values were estimated for XP-EHH to identify strong extended homozygosity in our current populations relative to the respective historical populations (as described above). The estimated p-values were subsequently adjusted (Benjamini and Hochberg, 1995) in R. Variants with adjusted p-values ≤ 0.05 were considered as significant. In addition, we used a conservative approach similar to those described in previous studies (Flori et al., 2014; Bertolini et al., 2020; Han et al., 2020) and defined as candidate regions under selection, sliding windows of 0.5 MB spanning at least three significant markers. The calc_candidate_regions function in “Rehh” was used to detect the candidate regions. Neighboring windows with significant SNP were merged to one candidate region. The peak of each candidate region, i.e., the SNP with the lowest adjusted p-values in a region, was considered as core SNP.
Genes located in the candidate regions for positive selection signatures were annotated from the Ensembl genome database (2020) (http://www.ensembl.org/biomart/martview/) and submitted to gene ontology (GO) enrichment analysis using the Gene Ontology web-tool (Ashburner et al., 2000; Gene Ontology Consortium, 2019). Fisher’s exact threshold of p-values < 0.01 was considered to identify overrepresented GO terms for biological processes and Reactome pathways. In addition, QTL that overlapped with the candidate regions under selection were mapped from the online data analysis tools of the cattle database (Hu et al., 2019) and summarized in major production and functional categories: milk, carcass quality, reproduction, body weight, conformation, feed intake, heat tolerance, and health traits (see Supplementary Table S2 for more details). Subsequently, for each population, we calculated the frequency of the QTL which is equal to the number of candidate regions overlapping with the given QTL per the total number of candidate regions.
Results
Population Structure and Inbreeding in the Current Beninese Cattle Population
Structure of the Current Population
The analyses with the fastStructure algorithm revealed four model components to explain the population structure of our samples. Similarly, the model complexity that maximizes marginal likelihood was equal to four. Considering the posterior mean of admixture proportion in the logistic model, we identified three components representing Somba, Lagune, and Dahomey samples, respectively (Supplementary Table S3). The fourth component was mainly made based on Borgou animals. However, some hybrid samples displayed genetic proportions across two or more components. This structure was similar to those obtained with adegenet (Figure 2). The unsupervised k-means clustering presented an optimal K (number of clusters, Supplementary Figure S1) equal to 6 and displayed the hybrid animals in different three clusters (Supplementary Figure S1 and Table 1). Consequently, a large Lagune group named LA_cur (n = 110) was separated from other Lagune samples called LA_out (n = 25). Forty-two Borgou and 56 Pabli animals (except two outgroups) formed a homogeneous cluster named Adm_cur (n = 98). The remaining Borgou animals were allocated to the BO_cur cluster. The Dahomey and the Somba correspond to the DAH_cur and SO_cur, respectively (Table 1). LA_cur, LA_out, DAH_cur, SO_cur, BO_cur, and Adm_cur were considered as populations for further analyses instead of original breed assignments.
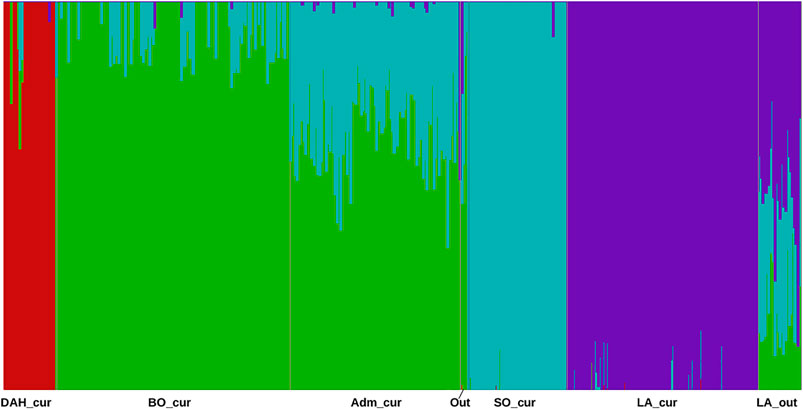
FIGURE 2. Barplot for admixture proportions inferred by fastStructure in the Beninese cattle populations. Dah_cur = current Dahomey; LA_cur = current pure Lagune; LA_out = admixed Lagune; SO_cur = current Somba; BO_cur = current Borgou highly admixed; Adm_cur = current moderately admixed animal including Pabli with some Borgou, Out = Outgroups (see Table 1 for more information).
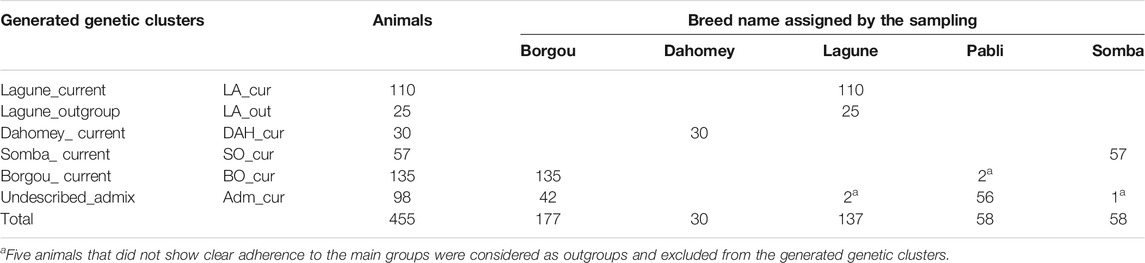
TABLE 1. Genetic clusters generated from unsupervised k-means clustering applied on the current Beninese cattle population.
Genomic Inbreeding Coefficients
The genome-wide contributions and genomic inbreeding coefficients with respect to different age-related HBD classes in the Beninese cattle populations are presented in Figures 3A,B, respectively. Overall, 40% of the genome of DAH_cur samples was HBD and was related only to HBD classes with Rk ≤ 16. We observed a major contribution of autozygosity from the HBD class with Rk = 8, accounting for 63.32% of the total HBD proportion in DAH_cur. The HBD classes with Rk = 4 and Rk = 16 contributed to 15.70 and 16.80% of the overall autozygosity, respectively. The estimated genomic inbreeding coefficients were 0.17 and 0.13 in LA_cur and LA_out, respectively, when considering all HBD classes (i.e., the most remote base population). The major contribution of autozygosity in LA_cur came from the ancient HBD class with Rk = 128 (40% of the HBD proportion). Recent HBD classes with Rk = 4 and Rk = 8 explained 13.78 and 7.80% of the total autozygosity in LA_cur, respectively, whereas 27.67 and 26.11% of the overall autozygosity in LA_out were derived from the two classes. Tracing back to the oldest ancestors, the fraction of the genome that was HBD in SO_cur was equal to 0.08 and was mainly originated from very ancient HBD classes with Rk = 256 (27% of the total HBD proportion) and Rk = 512 (33% of the total HBD proportion). The contribution of recent classes to autozygosity in SO_cur was lower (≤13% of the HBD loci). The estimated genomic inbreeding coefficients were 0.08 for BO_cur and 0.07 for Adm_cur. The HBD class with Rk = 256 was the main source of autozygosity, contributing to 77.94 and 73.09% of the total HBD proportions in BO_cur and Adm_cur, respectively.
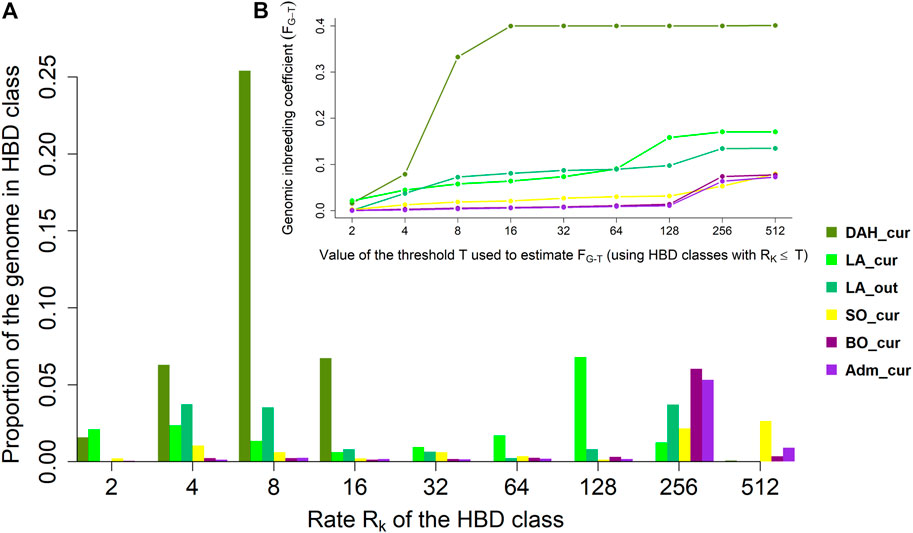
FIGURE 3. (A) Average realized autozygosity and (B) cumulative genomic inbreeding associated with different age-related homozygous-by-descent (HBD) classes in Beninese cattle populations. Dah_cur = current Dahomey; LA_cur = current pure Lagune; LA_out = admixed Lagune; SO_cur = current Somba; BO_cur = current Borgou highly admixed; Adm_cur = current moderately admixed animal including Pabli with some Borgou.
Comparison of Current Beninese Cattle Population With Historical Data
Principal Component Analyses and Clustering
The first and second principal components (PC) from PCA presented a clear separation of the Beninese breeds (Figure 4A). Within each breed, recent and historical samples were distinctly displayed along the second PC. The Dahomey cattle (DAH_cur) were projected next to LA_cur on PC1 but have a major contribution to PC2. Similar differentiations between current and historical samples were observed with the unsupervised k-means clustering, especially in Borgou and Lagune (BIC values suggested seven clusters, see Supplementary Figure S2A). Indeed, the unsupervised k-means clustering assigned the animals from Adm_cur, BO_cur, and BO_hist in three different clusters (clusters 2, 6, and 7), respectively (Supplementary Table S4A). Similarly, the current Lagune samples (LA_cur and LA_out) were grouped into cluster 4 and historical samples (LA_hist) into cluster 1. DAH_cur formed its own cluster (cluster 4). However, the current and historical Somba samples (SO_cur and SO_hist) were jointly grouped into cluster 5.
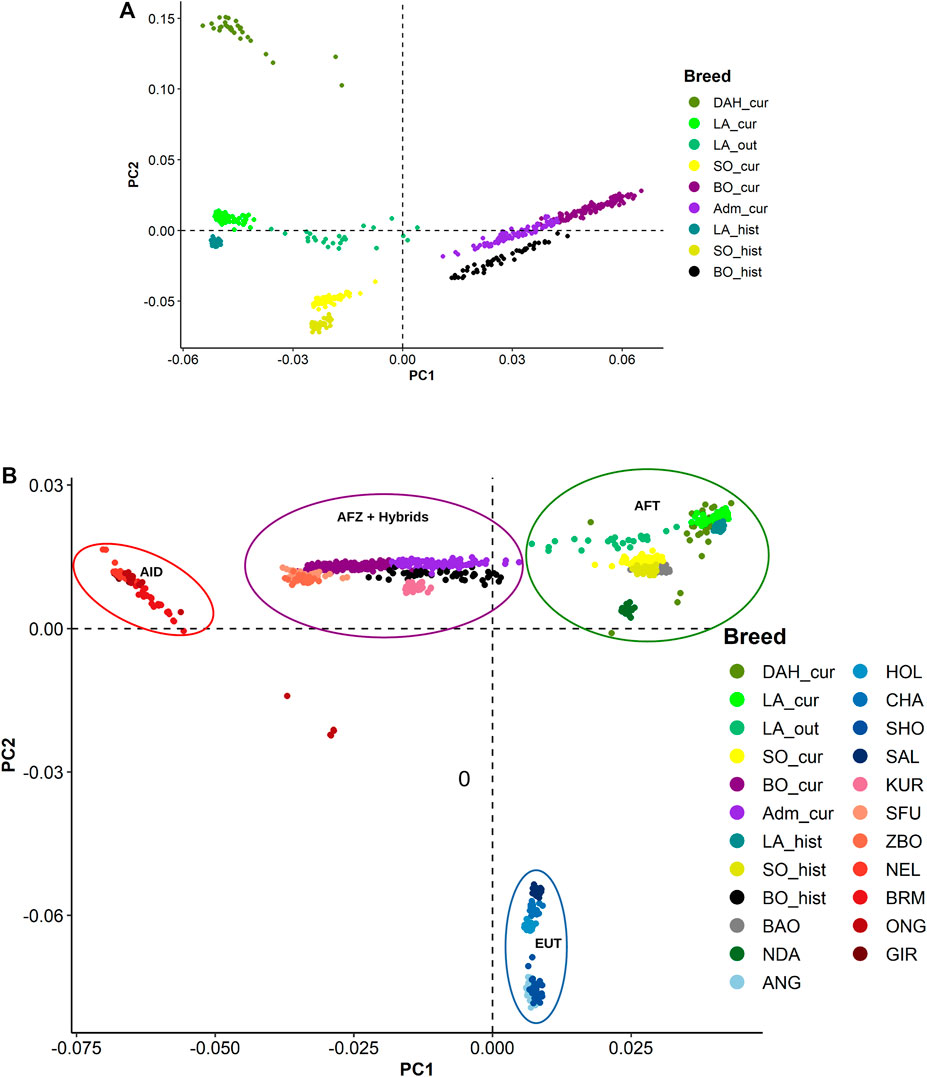
FIGURE 4. Principal components (PC) displaying genomic divergence: (A) in the different Beninese cattle populations; (B) in the Beninese cattle with additional African and European taurine (EUT) breeds, African and Asian indicine breeds, and African crossbreeds. Dah_cur = current Dahomey; LA_cur = current pure Lagune; LA_out = admixed Lagune; SO_cur = current Somba; BO_cur = current Borgou highly admixed; Adm_cur = current moderately admixed animal including Pabli with some Borgou; LA_hist = historical Lagune; SO_hist = historical Somba; BO_hist = historical Borgou; ANG = Angus; HOL = Holstein; CHA = Charolais; SHO = Shorthorn; BAO = Baoulé; NDA = N’Dama; GIR = Gir; SFU = Sudanese Fulani; ZBO = Zebu Bororo; BRM = Brahman; ONG = Ongole; NEL = Nellore; KUR = Kuri; SAL = Salers.
We repeated the diversity analyses on the extended dataset including additional AFT, EUT, AFZ, Asian indicine (AID), and hybrids. Considering the PCA, the taurine animals (AFT and EUT) were separated from indicine (AFZ and AID) and hybrids samples along the first component, whereas the second component (PC2) showed a separation between the AFT and EUT (Figure 4B). Interestingly, the Dahomey was aggregated with the Lagune far away from EUT. The AID (Gir, Brahman, Ongole, and Nellore) also were clearly separated from the AFZ (ZFU and ZBO) and hybrids. Eight clusters (low BIC at k = 8; Supplementary Figure S2B) were identified from the unsupervised k-means clustering. Current and historical Somba samples (SO_cur and SO_hist) formed one genetic group (cluster 2) with the other AFT (BAO and NDA). The Lagune samples (LA_cur, LA_out, and LA_hist) were grouped into cluster 2. Similarly, DAH_cur is displayed in one cluster (cluster 1). Moreover, the hybrids (Adm_cur, BO_cur, and BO_hist) were grouped with Kuri, ZFU, and ZBO in cluster 6. The other genetic groups consisted of the EUT and AID (Supplementary Table S4B).
Formal Test of Admixture and Inference of Ancestral Proportion
The formal test of admixture of three populations resulted in positive f3-ratios for DAH_cur, LA_cur, and SO_cur (Table 2). In contrast, we obtained negative statistics for LA_out, BO_cur and Adm_cur with the most significantly negative f3 value for BO_cur (f3 = −0.08; Z = −39.08). Considering the historical data, the f3-ratio test resulted in positive values for LA_hist but was negative for SO_hist and BO_hist.
The estimation of the ancestral AFT with the f4-ratio test revealed high proportions (alpha values superior to 0.97) in DAH_cur, LA_cur, and SO_cur, respectively (Table 2). In contrast, lower alpha values were found for LA_out, Adm_cur, and BO_cur. In comparison to the historical samples, we observed slight reductions of AFT ancestral proportions in Somba (0.96 for SO_cur against 0.97 for SO_hist) and in Adm_cur (0.62 against 0.63 for BO_hist). The current Borgou (BO_cur) displayed an important reduction of AFT ancestral proportions (alpha = 0.47) in comparison to historical samples (BO_hist). For LA_cur and LA_hist, the estimated AFT ancestral proportions were equal to 1.00, respectively.
Selection Signatures
Dahomey and Lagune
We detected no significant SNP presenting strong extended homozygosity in DAH_cur and LA_out relative to LA_hist. In contrast, the current Lagune population (LA_cur) displayed 19 significant SNP for positive selection (Figure 5A and Table 3). Among the SNP, 12 were positioned in a total of three candidate regions on BTA1, 18, and 21. The region 15.5–16 Mb on BTA21 contained the largest number of significant SNP (six SNP). The region 47.5–48 Mb on BTA18 included the most significant SNP rs110495745 (p_adjust = 8.40 × 10−04), which is positioned within the WDR87 gene. In total, 9 candidate genes were annotated within the three regions. GO enrichment analysis identified functional enriched terms such as cellular processes (Table 4). QTL associated with reproduction (67%) and conformation (67%) were predominant in the candidate regions (Figure 6).
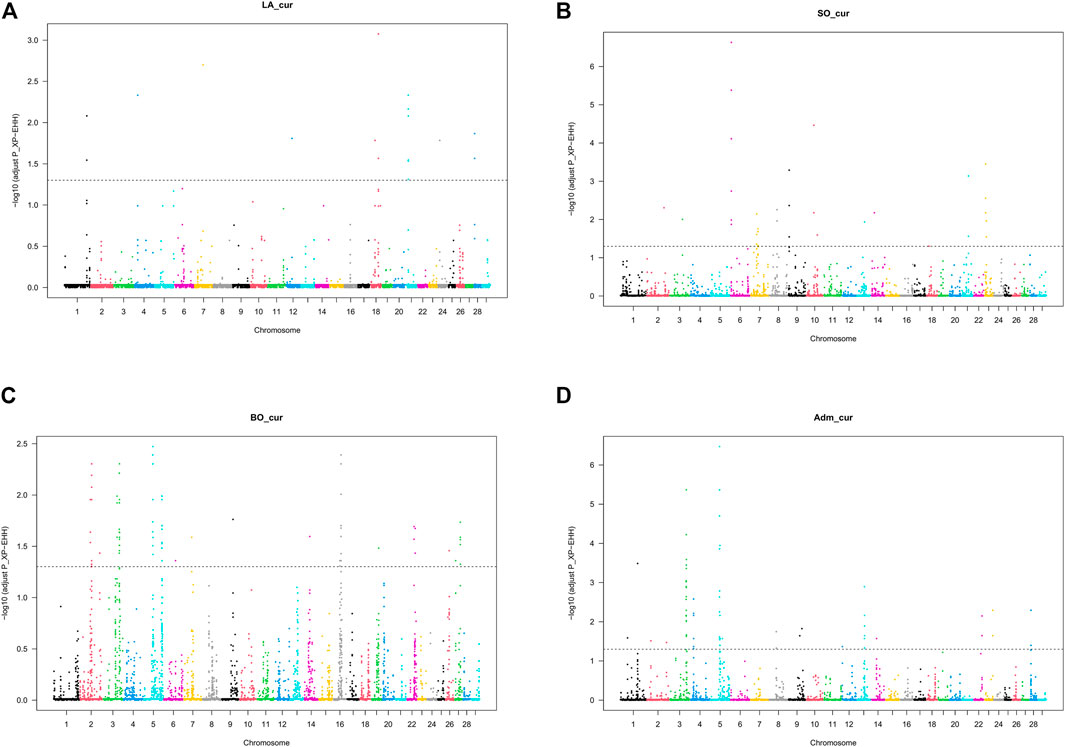
FIGURE 5. Manhattan plot for cross-population extended haplotype homozygosity (XP-EHH) test for selection signature in Beninese cattle populations: (A) LA_cur, current Lagune pure population, (B) SO_cur, current Somba population, (C) BO_cur, current Borgou highly admixed, and (D) Adm_cur, moderately admixed hybrids.
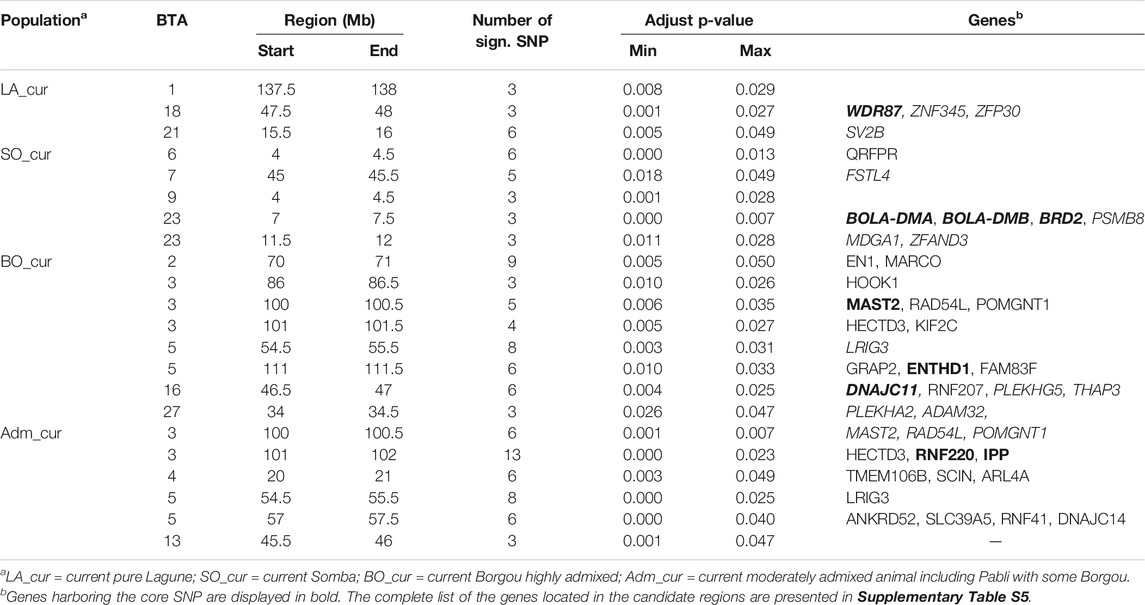
TABLE 3. Candidate regions harboring positive recent selection signatures in Beninese cattle populations.
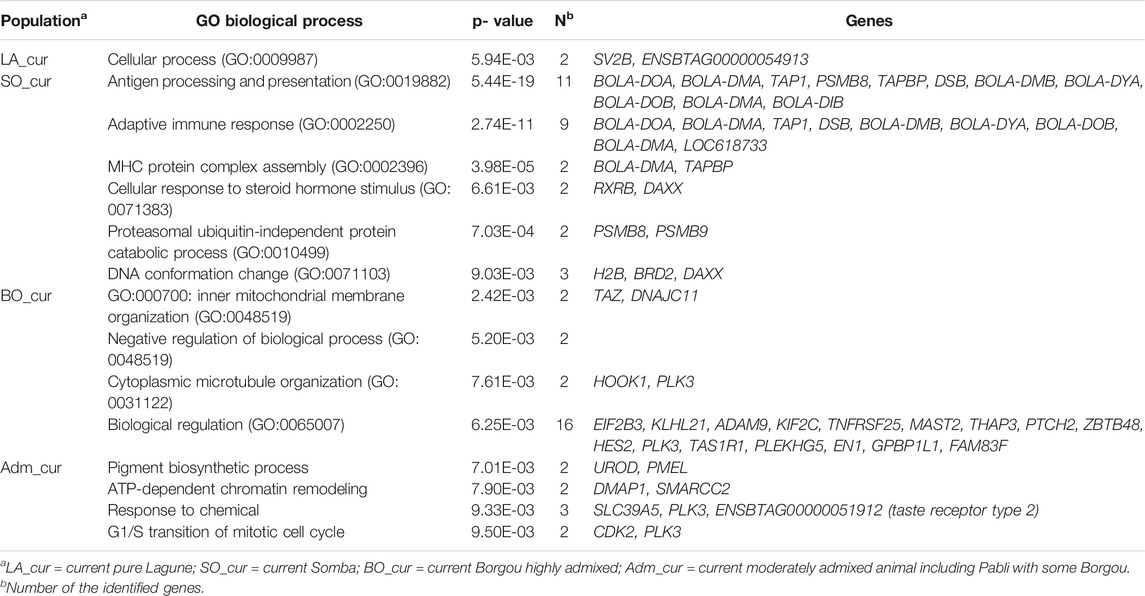
TABLE 4. Enriched gene ontology (GO) biological process for genes in candidate regions under positive selection in Beninese cattle populations.
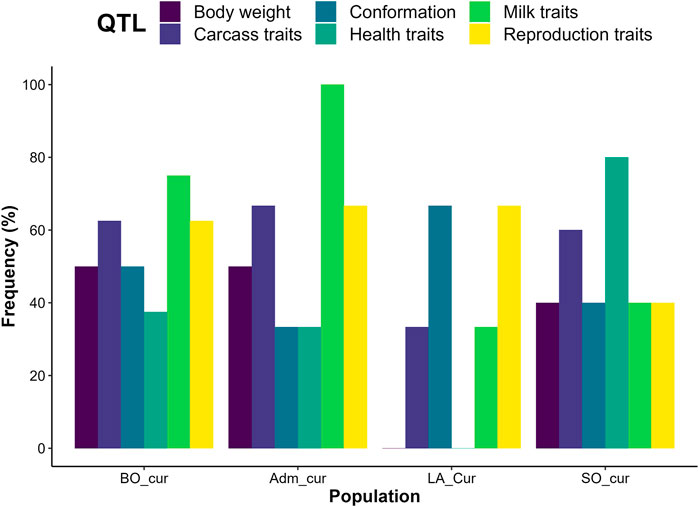
FIGURE 6. Proportion of candidate regions with quantitative trait loci (QTL) controlling varied trait categories. LA_cur = current pure Lagune; SO_cur = current Somba; BO_cur = current Borgou highly admixed; Adm_cur = current moderately admixed animal including Pabli with some Borgou.
Somba
The analysis of positive selection signatures in SO_cur relative to SO_hist detected 35 significant SNP (Figure 5B). Five candidate regions under recent selection in SO_cur were identified on BTA6, 7, 9, and 23, harboring in total 20 significant SNP. The region 4–4.5 Mb on BTA6 displayed the majority of the significant SNP (6 SNP) and the most significant SNP (rs42405104, p_adjust = 2.34 × 10−07). This SNP is not positioned in any gene. However, the second most significant SNP (p_adjust = 3.57 × 10−04) is positioned in the vicinity of three genes (BOLA-DMB, BOLA-DMA and BRD2) on BTA 23. In total, 33 genes were mapped in the candidate regions. GO enrichment analysis of the genes revealed highly significant GO biological processes such as antigen processing and adaptive immune response (Table 4). We observed that the majority of the candidate regions under positive selection in SO_cur overlapped with QTL affecting heath traits (80%) and carcass quality (60%, Figure 6).
Crossbreeds
We detected several genomic regions under recent selection in BO_cur and Adm_cur relative to BO_hist (Figures 5C,D). A total of 77 SNP displayed strong homozygosity in BO_cur, with adjusted p_values for XP-EHH below the significance threshold (p_adjust ≤ 0.05). Among these SNP, 44 were located in eight candidate regions on BTA2, 3, 5, 16, and 27 (Table 3). The regions that spanned from 70 to 71 Mb on BTA2 and from 54.5 to 55.5 Mb on BTA5 contained the largest number of significant SNP (9 and 8 significant SNP, respectively). The later region hosted the highest peak with the SNP rs41637710 (p_adjust = 0.003). This SNP was not in the vicinity of any gene. However, three significant SNP (rs110903828 on BTA27, rs41637109 on BTA16, and rs43361717 on BTA3) were positioned within the genes HTRA4, DNAJC11, and TMEM53, respectively. A total of 49 genes were mapped within the genomic candidate regions. GO enrichment analysis revealed several significant GO biological processes including cytoplasmic microtubule organization and inner mitochondrial membrane organization (Table 4). Moreover, several QTL affecting animal performances overlapped with the regions under positive selection in BO_cur. QTL associated with milk (75%), carcass (63%), and reproduction traits (63%) were the most represented (Figure 6).
In Adm_cur, we identified 69 significant SNP under positive selection. A subset of 42 SNP was located in six candidate regions on BTA3, 4, 5, and 13. Three candidate regions in Adm_cur overlapped with those detected in BO_cur (Table 3). Similarly, the SNP rs41637710 located on BTA5 (54.5–55.5 Mb) showed the lowest adjusted p-value (p_adjust = 3.38 × 10−07). The segment on BTA3 (101–102 Mb) hosted the largest number of extreme SNP (13 SNP). The candidate regions in Adm_cur included 63 genes. The genes DNAJC11, ENTHD1, and MAST2 hosted significant SNP, whereas others (IL23A, PAN2, and CNPY2) were mapped at a close distance to the significant SNP. GO enriched terms included biological processes such as pigment biosynthetic process and response to chemicals (Table 4). Like in BO_cur, the majority of QTL, located in the candidate regions of positive selection in Adm_cur, were related to milk (100%), carcass (67%), and reproduction traits (67%, Figure 6).
Discussion
Population Structure and Admixture Tests
The results of the population structure analyses are in line with the breed foundation of the different cattle populations in Benin and their divergence from other African and European cattle breeds (Mwai et al., 2015). First, we observed high proximity of the Somba and Baoulé populations as Savannah Shorthorns and their separation to the Dwarf (forest) Lagune Shorthorn (Rege et al., 1994). In addition, the divergence of the Shorthorn breeds from the Longhorn N’Dama illustrates the rich genetic diversity of West African indigenous cattle breeds and the necessity to unravel specific signatures in each population. Second, our study confirms the Lagune origin of the Dahomey cattle. We found neither indicine nor EUT background in the Dahomey population. The genetic purity of the Dahomey cattle is probably due to their promotion in a close production system organized by the Dahomey-Zwergrind breeder association aiming at the conservation of the breed (http://www.dahomey-zwergrind.com). The inference of the oldest common ancestor dated from eight generations ago (approximately 24 years ago). The high genomic inbreeding coefficient in Rk = 8 (4 generations equal to 16 years ago) in the Dahomey population may be related to the recent creation of the association in 2001. These results suggest that the founders of the current Dahomey population kept by farmers may have originated from a small number of Dahomey cattle four generations ago (http://www.dahomey-zwergrind.com). However, the low frequency of short ROH segments, resulting in low genomic inbreeding coefficients (<0.1) in very young classes (Rk ≤ 4), indicates a reduction of mating between related individuals in recent generations (Druet and Gautier, 2017). Exchange of breeding animals between the association members and consideration of Dahomey cattle currently held in different zoos across Germany and other European countries (Zootierliste, 2020: https://www.zootierliste.de) may contribute to control inbreeding and to increase genetic diversity in the population currently managed by the Dahomey–Zwergrind breeder association.
The results of the PCA analyses differentiated historical and current populations as well as populations affected by admixture. The identification of admixed animals (LA_out) from relatively pure Lagune is confirmed by the formal admixture test, the three-population test, and the estimation of admixture proportions. Our findings are in accordance with the increasing crossbreeding in Lagune cattle due to the extension of transhumance as reported by Scheper et al. (2020) and Ahozonlin and Dossa (2020). The large genomic inbreeding in the Lagune (LA_cur) is in line with the small number of the original populations formerly distributed in clusters across West African coastal and forest regions (Rege, 1999). Fortunately, the estimation of high AFT ancestral proportion in LA_cur suggests the existence of a relatively pure population, which may be valuable for the conservation of this indigenous taurine breed. The estimated alpha value of 1.00 may be related to the reference population considered. However, the Baoulé (BAO) is the closest shorthorn taurine with available historical genotype data, whereas the GIR is the purest indicine reference population as the majority of AFZ are admixed (Flori et al., 2014).
We found that Somba cattle are less affected than Lagune by Zebu introgression. Previous studies observed that its habitat in the hilly region of Atacora protected from Zebu introgression (Rege et al., 1994; Hall et al., 1995). In comparison to the location of the other local breeds (Northeastern and Southern Benin), the lower pressure of transhumance in Boukombe and lower economic and demographic pressures, resulting in less “modernization” of cattle management and crossbreeding, are some advantages (Houessou et al., 2019a; Scheper et al., 2020). However, the lower AFT ancestral proportion in SO_cur compared to SO_hist confirms the threat of admixture in Somba cattle mainly caused by entrustment practices (Hall et al., 1995; Dossa and Vanvanhossou, 2016; Vanvanhossou et al., 2021). In addition, the negative and positive f-statistics in the historical (SO_hist) and the current (SO_cur) Somba populations, respectively, indicate former introgression episodes followed by genetic drift (Patterson et al., 2012; Kim et al., 2020). We also observed that Somba cattle remain less inbred despite the reduction of population size and their shrinkage into the unique location of Boukombe (Dossa and Vanvanhossou, 2016).
The results from fastStructure corroborate the Somba background of the Beninese crossbreeds. In addition, the hybrid populations (BO_cur and Adm_cur) presented a relatively low genetic proximity to the AID (Gir, Brahman, Ongole, and Nellore), but they were clustered close to the AFZ (ZFU and ZBO). These results are in agreement with their origin as described by different authors (Atchy, 1976; Flori et al., 2014). The identification of different levels of admixture in the hybrid samples was confirmed by the f3 and f4 admixture tests. The lower AFT ancestral proportion in BO_cur compared to the historical population BO_hist confirms the increasing introgression of AFZ in smallholder Borgou herds as indicated by Scheper et al. (2020). BO_cur representing more than 75% of the current Borgou samples suggests an intensive admixture and a high risk of full replacement of the Borgou population by AFZ genotypes. We observed similar AFT ancestral proportions in the historical Borgou (Bo_hist) and Adm_cur. The AFT ancestral proportion in the later population, comprising the Pabli samples, indicates the existence of a residue of the Beninese indigenous crossbreeds. According to Pecaud (1912) (as reported by Atchy, 1976), the Pabli breed results from crossbreeding between Borgou and Somba around the year 1905. In addition, the region of Kerou hosting the Pabli cattle in Western Benin is also less affected by transhumance. The association between spatial indicine introgression and transhumance in the Beninese cattle population was described by Scheper et al. (2020). Finally, the existence of crossbreed populations with divergent admixture levels offers the opportunity to evaluate the impact of crossbreeding in terms of divergence in extended haplotype homozygosity profiles.
Selection Signatures
Previous studies (Lohmueller et al., 2010; Freedman et al., 2016) observed that admixture or further demographic events (population bottlenecks due to diseases) affect ancestral haplotypes and increase the occurrence of mosaics in the genome (Freedman et al., 2016; Aliloo et al., 2020). This may impede the distinction of genomic footprints left by neutral processes and natural selection. In consequence, we did not expect evidence for historical selections in the Beninese cattle population. Nevertheless, the selection is not dissociable from admixture in several African cattle breeds. Admixture is a historical practice in African cattle production and is considered as a quick means of animal upgrading (Flori et al., 2014). Researchers reported that the selection of the animal or the breed for crossbreeding is driven by farmer interests including desired productive (milk, meat, and reproductive) and adaptive features (left by natural selection) (Boutrais, 2007). In this context, the prevalence of specific genomic regions or functional traits within a population may reflect the production goals of the farmers. In addition, specific features in the genome of African cattle populations (including several populations with various crossbreeding histories) are commonly assessed with selection signature analyses (Gautier et al., 2009; Taye et al., 2018; Aliloo et al., 2020; Kim et al., 2020).
By contrasting the current and historical cattle populations in the Beninese taurine and crossbreeds, we focus on genomic footprints resulting from recent environmental pressures or herd management. Indeed, environmental and socioeconomic factors have induced diverse changes in cattle management practices in Benin. These include the adoption of cattle mobility in taurine or agropastoralist herds (former sedentary), the increase of herd mobility frequencies and amplitudes, the migration and settlement of several agropastoralists from Sahelian countries or Northern Benin into Southern Benin, and the extension of animal entrustment practices (from agropastoralists to traditional pastoralists) (Houessou et al., 2019a; Houessou et al., 2020; Vanvanhossou et al., 2021). Despite the limited period between our and the historical samples, we identified a few candidate regions, providing new insights into the evolutionary process in the indigenous breeds. In comparison to the taurine, we observed that the crossbreeds, especially BO_cur, displayed most of the candidate regions. These results are in line with increased Zebu introgression in BO_cur, leading to a higher admixture proportion than Adm_cur and more genetic divergence from the historical Borgou (BO_hist). Regarding the Somba (SO_cur), the identification of five candidate regions mainly associated with immunity features suggests the importance of disease pressures in its belt, as extensively discussed below.
Our approach to detect a strong signal of homozygosity considering temporal subpopulations within the same breed is similar to the one applied by Naderi et al. (2020), who contrasted the current German Holstein to one of its recent ancestors, the local dual-purpose German black pied cattle (DSN). In addition, we aimed to reduce the bias due to the uncertainty of the ancestral base population and applied the XP-EHH method to detect complete sweeps in contrast to the Integrated Haplotype Score (IHS) approach (Qanbari and Simianer, 2014). Indeed, the IHS method usually applied to investigate within-breed selection signatures relies on ancestral allele frequency which is inconsistently defined in different studies. For instance, Utsunomiya et al. (2013) derived ancestral alleles from common founders of Bovidae species, namely, Bos gaurus, Bos grunniens, and Bubalus bubalis. Kim et al. (2020) considered fixed alleles in African Buffalo as ancestral alleles to determine selection signatures in the African population, while Gautier et al. (2009) estimated ancestral alleles for West African cattle breeds based on alleles frequencies in indicines and African and European taurine samples. Finally, we expect to reduce false-positive results due to multiple tests, by considering adjusted p-values to define significant SNP and by defining only regions with at least three significant SNP as candidate regions. The respective candidate regions identified for each cattle population are discussed in the ongoing sections.
Lagune
We were not able to detect any candidate regions for DAH_cur. The Dahomey cattle may have acquired very few complete selective sweeps that are not detectable by the XP-EHH. This result reflects the breeding strategy with only focus on leisure and is in line with its high genomic inbreeding coefficient (http://www.dahomey-zwergrind.com). Further investigations including a complete sequencing of the Dahomey cattle and other methods of selection signature analyses may help untangle the genetic divergence between the Dahomey and the Lagune.
The admixed LA_out population was not considered for selection signature analysis because of the divergence in introgression levels as shown in the population structure analyses and admixture test. The LA_out group is the product of diverse admixed animals sampled as Lagune and therefore cannot represent any specific cattle population in Benin. On the contrary, LA_cur, as a relatively pure Lagune population, displayed three candidate regions. The region 15.5–16 Mb on BTA21 including the most significant SNP in LA_cur is identical to selection signatures reported in Holstein and North African cattle (Taye et al., 2017b; Ben-Jemaa et al., 2020). The SV2B gene in this region is associated with feed intake in cattle (Seabury et al., 2017). In contrast to the other populations investigated in this study, the candidate regions under selection in LA_cur encompassed relatively few genes functionally described in the literature. Nevertheless, the WDR87 gene, hosting the most significant SNP, is related to carcass traits in cattle (Lim et al., 2013). In addition, the majority of the genes are involved in cellular and regulation of RNA biosynthetic processes, which are responsible for feed efficiency and body weight (Olivieri et al., 2016). Similarly, QTL associated with body weight, milk and reproductive traits are predominant in the candidate regions.
Somba
With regard to the five candidate regions under selection in SO_cur, two were detected on BTA23. This chromosome is known to have two subregions (classes IIa and IIb) of the bovine leukocyte antigen (BoLA), also called bovine major histocompatibility complex (MHC), which is determinant in the development of acquired immune responses to diverse parasitic and viral diseases (Ellis and Ballingall, 1999; Takeshima and Aida, 2006). The selective region 7–7.5 Mb (BTA23) spanning several genes (e.g., DSB, BOLA-DYA, BOLA-DMB, and BOLA-DOA) overlaps with the subregion of BoLA class IIb (Takeshima and Aida, 2006). These BoLA class IIb genes are specific to ruminants but are less characterized, in contrast to genes in the BoLA class IIa (e.g., BoLA-DRB3 and BoLA-DQA3) (Takeshima and Aida, 2006). In addition, Ballingall and McKeever (2005) associated the rare polymorphism of BoLA class IIb genes to evolutionary processes under functional constraints. Selection signatures in this genomic region were only found in Angus and Brangus cattle (Goszczynski et al., 2018; Maiorano et al., 2018), whereas selection for adaptive immunity in African cattle breeds is usually identified within the BoLA class IIa region (Ballingall et al., 1997; Kim et al., 2017; Tijjani et al., 2019). Therefore, recent selection signatures in the specific BoLA class IIb genes may indicate a possible adaptation of the Somba cattle to endemic diseases, especially to anthrax. Indeed, the Somba cattle are affected by several recent episodes of anthrax outbreaks especially in the years 2007, 2009, 2012, and 2013 (Dossa and Vanvanhossou, 2016). Further studies are required to investigate the association between BoLA subregion IIb and resistance to disease in Somba and other African cattle breeds. In addition, other candidate genes include PSMB9 and HSD17B8 which are involved in meat and growth traits (Lee et al., 2012; Ma et al., 2015), FSTL4 associated with milk production (Sanchez et al., 2019) and ZFAND3 responsible for reproduction (Mohammadi et al., 2020). The predominance of candidate genes associated with immune response is confirmed by enriched terms such as antigen processing and presentation, and adaptive immune response. However, other biological processes include the regulation of cellular metabolic processes and intracellular protein transport mechanisms. The identified bovine QTL suggests selection on body weight, carcass, reproduction, and milk traits in the Somba cattle.
Crossbreeds
The regions of selection (54.5–55.5 Mb on BTA5, 100–100.5 Mb, and 101–101.5 Mb on BTA3) overlapping in the two hybrid populations are in line with their common indicine background. The significant SNP mapped in the region from 100 to 101.5 MB on BTA3 were positioned in various genes including the RNF220 gene. This gene has been previously identified under selection in West African cattle (Gautier et al., 2009). In addition, the region includes genomic footprints of signatures in South African and East African Shorthorn hybrids with indicine ancestry deficiency in the later breed (Bahbahani et al., 2015; Zwane et al., 2019). These findings suggest that the region may represent an ancient and stable footprint of selection in indigenous African hybrids. The RNF220 gene is involved in calving performance and milk yield (Abdel-Shafy et al., 2020; Purfield et al., 2020). In addition, the HECTD3 in this region is associated with cell cycle regulation and fat deposition, while PLK3 is related to gain and feed intake in cattle (Yu et al., 2009; Bahbahani et al., 2015; Zarek et al., 2017). We also detected in this region the PTCH2 and SLC6A9 which are involved in reproduction and Porphyria disease, respectively (Nezamzadeh et al., 2005; Basavaraja et al., 2021). The region 55–55.5 Mb on BTA5 previously displayed evidence of selective sweeps in the EUT Charolais as well as in a tropical crossbreed between Charolais and Zebu, namely, the Canchim (Xu et al., 2015; Urbinati et al., 2016; Naval-Sánchez et al., 2020). Moreover, the LRIG3 gene in this region is associated with body length in cattle and litter size in pigs (Xu et al., 2015; Metodiev et al., 2018).
Specific selection signatures detected in BO_cur include the selective sweep 46.5–47.5 Mb on BTA16. This region is of great interest. It presents several significant SNP and overlaps with genomic footprints detected in East African Zebu cattle as well as in a subpopulation of the German dual-purpose black and white cattle (Taye et al., 2018; Naderi et al., 2020). The region contains DNAJC11, a heat shock protein gene, involved in response to heat stress (Li et al., 2015). In addition, the candidate regions of selection (BTA 5:111.5–111.5 Mb and BTA 2:70–71 Mb) are known candidate regions under selection in different African, European and Asian cattle and sheep breeds (Hudson et al., 2014; Bomba et al., 2015; Wang et al., 2015; Bertolini et al., 2020). The region 111.5–111.5 Mb on BTA5 contributed to positive selection for natural virus resistance and to extensive admixture in West Sahelian African human populations (Cagliani et al., 2011; Pérez-Rivas et al., 2014; Triska et al., 2015). In addition, the segment BTA 2:70–71 Mb covered various candidate genes such as EN1, involved in growth traits in cattle (Buroker, 2014). Further regions under selection in BO_cur host candidate genes, significantly associated with different traits, including ADAM32, ADAM9, HTRA4, and KLHL21 with residual feed intake and immune responses, PLEKHA2 and TNFRSF25 with growth and carcass performances, HTRA4 with milk, and HOOK1 with heat stress (Fan et al., 2015; Tizioto et al., 2015; Seong et al., 2016; Blanco et al., 2017; Hardie et al., 2017; Hay and Roberts, 2018; Sengar et al., 2018; Skibiel et al., 2018; Oliveira et al., 2019; Braz et al., 2020; Brunes et al., 2021; Soares et al., 2021).
Regarding the selection signatures in Adm_cur, the region 20–21 Mb on BTA4 in Adm_cur is consistent with the region reported by Naderi et al. (2020), who identified selection signatures in close distance to the TMEM106B gene in the German black pied cattle. In addition, the ARL4A gene was reported in the context of selective sweeps in Australian Holstein (Larkin et al., 2012) and with regard to copy number variations in African Nguni cattle and Polish Holstein (Wang et al., 2015; Mielczarek et al., 2017). The latter gene is associated with milk production in dairy (Raschia et al., 2018; Khan et al., 2020). The SCIN gene, also mapped in the region, is involved in residual feed intake in cattle (Salleh et al., 2017). The remaining regions under selection in Adm_cur spanned other candidate genes including ANKRD52, RNF41, and MYL6 associated with height and carcass traits (Cai et al., 2019; Moravčíková et al., 2019; Feitosa et al., 2021), and COQ10A and RNF41 related to milk trait and calf mortality (Lázaro et al., 2021; Marín-Garzón et al., 2021). In addition, the SARNP gene is related to animal reproduction (Labrecque et al., 2014), while the DNAJC14 is involved in heat stress (Bahbahani et al., 2015; Rehman et al., 2020).
Overall, the common and specific candidate regions identified in the hybrid populations confirm selection signatures in African and European crossbreeds (Supplementary Table S6). They cover several candidate genes related to economic and functional traits. The enriched biological processes including inner mitochondrial membrane organization and ATP-dependent chromatin remodeling are related to carcass traits, milk production, and reproduction in cattle (Lu et al., 2016; Zhang and Xie, 2019; Shi et al., 2021). We also observed that the hybrids present more candidate regions related to heat response than the taurine populations, which is in line with the admixture with Zebu cattle known to tolerate high environmental heat loads (Taye et al., 2017a; Kim et al., 2020). Moreover, few genes in candidate regions of selection are involved in immune response and feed efficiency. The evidence of selection for adaptive traits in the hybrid populations (including BO_cur that is highly admixed) may be related to the fact that the AFZ introduced in the production environments of the West African taurine for decades have also developed various adaptive features (Atchy, 1976; Houessou et al., 2019b). Consequently, their crossbreeding with indigenous taurine cattle reduces the risk of diluting adaptive traits in local breeds while offering the opportunity to increase animal performances. Our findings are in line with those reported in other African cattle breeds (Aliloo et al., 2020; Kim et al., 2020) and suggest the ability to develop robust and productive breeds via crossbreeding. Nevertheless, the improvement of cattle breeding in West Africa requires the establishment of sustainable crossbreeding programs and the enhancement of genomic selection including genotype by environment interactions in the indigenous breeds. These will be achieved through the determination of suitable breeds and optimal proportions of admixture, considering social-ecological constraints (Wollny, 2003; Wurzinger et al., 2014).
Conclusion
In this study, we confirm that the Dahomey cattle currently bred in Europe are a subpopulation of the Beninese indigenous Lagune breed. The high genomic inbreeding in the Dahomey population is due to its current breeding system. The introduction of new animals from zoological parks can increase the diversity of the Dahomey population. Moreover, the Beninese taurine indigenous Lagune and Somba cattle still conserve a high proportion of AFT ancestry, in comparison to the historical population. The Borgou displays a risk of full genetic replacement by African Zebu. However, we observed the existence of a hybrid population relatively less affected by ongoing indicine introgression and comparable to the historical Borgou.
We found no evidence of the negative impact of admixture on the adaptive features in the cattle populations including the crossbreeds, as they all present several genomic footprints involved in immune response, feed efficiency, and heat stress. Moreover, specific candidate regions in the Somba cattle demonstrate selection pressures related to endemic diseases in the habitat areas of the breed. Overall, identified recent selection in Beninese indigenous cattle towards productive traits such as reproduction, milk, and carcass traits favor the improvement of the economic merits of the breeds.
Data Availability Statement
All the data supporting the results of this article are presented within the article or in the additional files. The raw genotypic data used in this study are openly accessible at http://dx.doi.org/10.22029/jlupub-73.
Author Contributions
SV, CS, LD, and SK conceived the project. SU collected the data, designed and applied the statistical analyses, and drafted the manuscript. TY and CS supported in statistical analyses and manuscript preparation. SK, RF, and LD reviewed and edited the manuscript. All authors read and approved the final manuscript.
Funding
This research was funded by the VolkswagenStiftung, Hannover, Germany (grant Az 94 829). We acknowledge funding of SV through the German Academic Exchange Service (DAAD) scholarship program: Research Grants-Doctoral Programmes in Germany.
Conflict of Interest
The authors declare that the research was conducted in the absence of any commercial or financial relationships that could be construed as a potential conflict of interest.
Publisher’s Note
All claims expressed in this article are solely those of the authors and do not necessarily represent those of their affiliated organizations, or those of the publisher, the editors, and the reviewers. Any product that may be evaluated in this article, or claim that may be made by its manufacturer, is not guaranteed or endorsed by the publisher.
Acknowledgments
The authors are thankful to the farmers in Benin and the Verband Europäisches Dahomey-Zwergrind for their valuable help and their collaboration during the collection of hair samples.
Supplementary Material
The Supplementary Material for this article can be found online at: https://www.frontiersin.org/articles/10.3389/fgene.2021.657282/full#supplementary-material
References
Abdel-Shafy, H., Awad, M. A. A., El-Regalaty, H., Ismael, A., El-Assal, S. E.-D., and Abou-Bakr, S. (2020). A Single-Step Genomic Evaluation for Milk Production in Egyptian Buffalo. Livestock Sci. 234, 103977. doi:10.1016/j.livsci.2020.103977
Ahozonlin, M. C., Dossa, L. H., Dahouda, M., and Gbangboche, A. B. (2020). Morphological Divergence in the West African Shorthorn Lagune Cattle Populations from Benin. Trop. Anim. Health Prod. 52, 803–814. doi:10.1007/s11250-019-02071-1
Ahozonlin, M. C., and Dossa, L. H. (2020). Diversity and Resilience to Socio-Ecological Changes of Smallholder Lagune Cattle Farming Systems of Benin. Sustainability 12, 7616. doi:10.3390/su12187616
Aliloo, H., Mrode, R., Okeyo, A. M., and Gibson, J. P. (2020). Ancestral Haplotype Mapping for GWAS and Detection of Signatures of Selection in Admixed Dairy Cattle of Kenya. Front. Genet. 11, 544. doi:10.3389/fgene.2020.00544
Ashburner, M., Ball, C. A., Blake, J. A., Botstein, D., Butler, H., Cherry, J. M., et al. (2000). Gene Ontology: Tool for the Unification of Biology. Nat. Genet. 25, 25–29. doi:10.1038/75556
Atchy, A. A. (1976). Contribution à l’étude de la transhumance en Republique populaire du Bénin. PhD Thesis. Senegal: Université de Dakar.
Bahbahani, H., Clifford, H., Wragg, D., Mbole-Kariuki, M. N., van Tassell, C., Sonstegard, T., et al. (2015). Signatures of Positive Selection in East African Shorthorn Zebu: A Genome-wide Single Nucleotide Polymorphism Analysis. Sci. Rep. 5, 11729. doi:10.1038/srep11729
Ballingall, K. T., Luyai, A., and McKeever, D. J. (1997). Analysis of Genetic Diversity at the DQA Loci in African Cattle: Evidence for a BoLA-DQA3 Locus. Immunogenetics 46, 237–244. doi:10.1007/s002510050268
Ballingall, K. T., and McKeever, D. J. (2005). Conservation of Promoter, Coding and Intronic Regions of the Non-classical MHC Class IIDYAgene Suggests Evolution under Functional Constraints. Anim. Genet. 36, 237–239. doi:10.1111/j.1365-2052.2005.01281.x
Basavaraja, R., Drum, J. N., Sapuleni, J., Bibi, L., Friedlander, G., Kumar, S., et al. (2021). Downregulated Luteolytic Pathways in the Transcriptome of Early Pregnancy Bovine Corpus Luteum Are Mimicked by Interferon-Tau In Vitro. BMC Genomics 22, 452. doi:10.1186/s12864-021-07747-3
Belemsaga, D., Lombo, Y., Thevenon, S., and Sylla, S. (2005). “Inventory Analysis of West African Cattle Breeds,” in Applications of Gene-Based Technologies for Improving Animal Production and Health in Developing Countries. Editors H. P. Makkar, and G. J. Viljoen (Berlin/Heidelberg: Springer-Verlag), 167–173.
Ben-Jemaa, S., Mastrangelo, S., Lee, S.-H., Lee, J. H., and Boussaha, M. (2020). Genome-Wide Scan for Selection Signatures Reveals Novel Insights into the Adaptive Capacity in Local North African Cattle. Sci. Rep. 10, 19466. doi:10.1038/s41598-020-76576-3
Benjamini, Y., and Hochberg, Y. (1995). Controlling the False Discovery Rate: A Practical and Powerful Approach to Multiple Testing. J. R. Stat. Soc. Ser. B (Methodological) 57, 289–300. doi:10.1111/j.2517-6161.1995.tb02031.x
Berthier, D., Peylhard, M., Dayo, G.-K., Flori, L., Sylla, S., Bolly, S., et al. (2015). A Comparison of Phenotypic Traits Related to Trypanotolerance in Five West African Cattle Breeds Highlights the Value of Shorthorn Taurine Breeds. PLoS ONE 10, e0126498. doi:10.1371/journal.pone.0126498
Bertolini, F., Schiavo, G., Bovo, S., Sardina, M. T., Mastrangelo, S., Dall’Olio, S., et al. (2020). Comparative Selection Signature Analyses Identify Genomic Footprints in Reggiana Cattle, The Traditional Breed of the Parmigiano-Reggiano Cheese Production System. Animal 14, 921–932. doi:10.1017/S1751731119003318
Bertrand, A. R., Kadri, N. K., Flori, L., Gautier, M., and Druet, T. (2019). RZooRoH: An R Package to Characterize Individual Genomic Autozygosity and Identify Homozygous‐by‐Descent Segments. Methods Ecol. Evol. 10, 860–866. doi:10.1111/2041-210X.13167
Blanco, F. C., Soria, M. A., Klepp, L. I., and Bigi, F. (2017). ERAP1 and PDE8A Are Downregulated in Cattle Protected against Bovine Tuberculosis. J. Mol. Microbiol. Biotechnol. 27, 237–245. doi:10.1159/000479183
Bomba, L., Nicolazzi, E. L., Milanesi, M., Negrini, R., Mancini, G., Biscarini, F., et al. (2015). Relative Extended Haplotype Homozygosity Signals across Breeds Reveal Dairy and Beef Specific Signatures of Selection. Genet. Sel. Evol. 47, 25. doi:10.1186/s12711-015-0113-9
Boutrais, J. (2007). The Fulani and Cattle Breeds: Crossbreeding and Heritage Strategies. Africa 77, 18–36. doi:10.3366/afr.2007.77.1.18
Braz, C. U., Rowan, T. N., Schnabel, R. D., and Decker, J. E. (2020). Extensive Genome-wide Association Analyses Identify Genotype-By-Environment Interactions of Growth Traits in Simmental Cattle. BioRxiv 11, 13335. doi:10.1101/2020.01.09.900902
Browning, B. L., Zhou, Y., and Browning, S. R. (2018). A One-Penny Imputed Genome from Next-Generation Reference Panels. Am. J. Hum. Genet. 103, 338–348. doi:10.1016/j.ajhg.2018.07.015
Brunes, L. C., Baldi, F., Lopes, F. B., Lôbo, R. B., Espigolan, R., Costa, M. F. O., et al. (2021). Weighted Single‐Step Genome‐Wide Association Study and Pathway Analyses for Feed Efficiency Traits in Nellore Cattle. J. Anim. Breed. Genet. 138, 23–44. doi:10.1111/jbg.12496
Cagliani, R., Riva, S., Fumagalli, M., Biasin, M., Caputo, S. L., Mazzotta, F., et al. (2011). A Positively Selected APOBEC3H Haplotype Is Associated with Natural Resistance to HIV-1 Infection. Evolution 65, 3311–3322. doi:10.1111/j.1558-5646.2011.01368.x
Cai, Z., Guldbrandtsen, B., Lund, M. S., and Sahana, G. (2019). Weighting Sequence Variants Based on Their Annotation Increases the Power of Genome-Wide Association Studies in Dairy Cattle. Genet. Sel. Evol. 51, 20. doi:10.1186/s12711-019-0463-9
Dossa, L. H., and Vanvanhossou, F. U. S. (2016). The Indigenous Somba Cattle of the Hilly Atacora Region in North-West Benin: Threats and Opportunities for its Sustainable Use. Trop. Anim. Health Prod. 48, 349–359. doi:10.1007/s11250-015-0958-5
Druet, T., Oleński, K., Flori, L., Bertrand, A. R., Olech, W., Tokarska, M., et al. (2020). Genomic Footprints of Recovery in the European Bison. J. Hered. 111, 194–203. doi:10.1093/jhered/esaa002
Druet, T., and Gautier, M. (2017). A Model-Based Approach to Characterize Individual Inbreeding at Both Global and Local Genomic Scales. Mol. Ecol. 26, 5820–5841. doi:10.1111/mec.14324
E. Buroker, N. (2014). Computational STAT4 rSNP Analysis, Transcriptional Factor Binding Sites and Disease. J. Bioinform. Diabetes 1, 18–53. doi:10.14302/issn.2374-9431.jbd-15-890
Egito, A. A., Paiva, S. R., Albuquerque, M. D. S. M., Mariante, A. S., Almeida, L. D., Castro, S. R., et al. (2007). Microsatellite Based Genetic Diversity and Relationships Among Ten Creole and Commercial Cattle Breeds Raised in Brazil. BMC Genet. 8, 83. doi:10.1186/1471-2156-8-83
Ellis, S. A., and Ballingall, K. T. (1999). Cattle MHC: Evolution in Action? Immunol. Rev. 167, 159–168. doi:10.1111/j.1600-065X.1999.tb01389.x
Ensembl genome database (2020). Available at: http://www.ensembl.org/biomart/martview/ (Accessed November 04, 2020).
Epstein, H. (1971). The Origin of the Domestic Animals of Africa. New York: Africana Publishing Corporation.
Fan, H., Wu, Y., Zhou, X., Xia, J., Zhang, W., Song, Y., et al. (2015). Pathway-Based Genome-Wide Association Studies for Two Meat Production Traits in Simmental Cattle. Sci. Rep. 5, 18389. doi:10.1038/srep18389
Feitosa, F. L. B., Pereira, A. S. C., Mueller, L. F., Fonseca, P. A. d. S., Braz, C. U., Amorin, S., et al. (2021). Genome-wide Association Study for Beef Fatty Acid Profile Using Haplotypes in Nellore Cattle. Livestock Sci. 245, 104396. doi:10.1016/j.livsci.2021.104396
Flori, L., Thevenon, S., Dayo, G.-K., Senou, M., Sylla, S., Berthier, D., et al. (2014). Adaptive Admixture in the West African Bovine Hybrid Zone: Insight from the Borgou Population. Mol. Ecol. 23, 3241–3257. doi:10.1111/mec.12816
Freedman, A. H., Schweizer, R. M., Ortega-Del Vecchyo, D., Han, E., Davis, B. W., Gronau, I., et al. (2016). Demographically-Based Evaluation of Genomic Regions under Selection in Domestic Dogs. Plos Genet. 12, e1005851. doi:10.1371/journal.pgen.1005851
Gao, Y., Gautier, M., Ding, X., Zhang, H., Wang, Y., Wang, X., et al. (2017). Species Composition and Environmental Adaptation of Indigenous Chinese Cattle. Sci. Rep. 7, 16196. doi:10.1038/s41598-017-16438-7
Gautier, M., Flori, L., Riebler, A., Jaffrézic, F., Laloé, D., Gut, I., et al. (2009). A Whole Genome Bayesian Scan for Adaptive Genetic Divergence in West African Cattle. BMC Genomics 10, 550. doi:10.1186/1471-2164-10-550
Gautier, M., Klassmann, A., and Vitalis, R. (2017). rehh2.0: A Reimplementation of the R Packagerehhto Detect Positive Selection from Haplotype Structure. Mol. Ecol. Resour. 17, 78–90. doi:10.1111/1755-0998.12634
Gautier, M., and Vitalis, R. (2012). Rehh: An R Package to Detect Footprints of Selection in Genome-Wide SNP Data from Haplotype Structure. Bioinformatics 28, 1176–1177. doi:10.1093/bioinformatics/bts115
Gene Ontology Consortium (2019). The Gene Ontology Resource: 20 Years and Still GOing strong. Nucleic Acids Res. 47, D330–D338. doi:10.1093/nar/gky1055
Goszczynski, D. E., Corbi-Botto, C. M., Durand, H. M., Rogberg-Muñoz, A., Munilla, S., Peral-Garcia, P., et al. (2018). Evidence of Positive Selection Towards Zebuine Haplotypes in the BoLA Region of Brangus Cattle. Animal 12, 215–223. doi:10.1017/S1751731117001380
Hall, S. J. G., Gnaho, L. K., and Meghen, C. (1995). Une enquête sur la race bovine Somba au Bénin. Rev. Elev. Med. Vet. Pays Trop. 48, 77–83. doi:10.19182/remvt.9492
Han, H., McGivney, B. A., Farries, G., Katz, L. M., MacHugh, D. E., Randhawa, I. A. S., et al. (2020). Selection in Australian Thoroughbred Horses Acts on a Locus Associated with Early Two-Year Old Speed. PLoS ONE 15, e0227212. doi:10.1371/journal.pone.0227212
Hanotte, O., Bradley, D. G., Ochieng, J. W., Verjee, Y., Hill, E. W., and Rege, J. E. O. (2002). African Pastoralism: Genetic Imprints of Origins and Migrations. Science 296, 336–339. doi:10.1126/science.1069878
Hardie, L. C., VandeHaar, M. J., Tempelman, R. J., Weigel, K. A., Armentano, L. E., Wiggans, G. R., et al. (2017). The Genetic and Biological Basis of Feed Efficiency in Mid-Lactation Holstein Dairy Cows. J. Dairy Sci. 100, 9061–9075. doi:10.3168/jds.2017-12604
Hay, E. H., and Roberts, A. (2018). Genome-Wide Association Study for Carcass Traits in a Composite Beef Cattle Breed. Livestock Sci. 213, 35–43. doi:10.1016/j.livsci.2018.04.018
Ho, S. Y. W., Larson, G., Edwards, C. J., Heupink, T. H., Lakin, K. E., Holland, P. W. H., et al. (2008). Correlating Bayesian Date Estimates with Climatic Events and Domestication Using a Bovine Case Study. Biol. Lett. 4, 370–374. doi:10.1098/rsbl.2008.0073
Houessou, S. O., Dossa, L. H., Assogba, C. A., Diogo, R. V. C., Vanvanhossou, S. F. U., and Schlecht, E. (2020). The Role of Cross-Border Transhumance in Influencing Resident Herders' Cattle Husbandry Practices and Use of Genetic Resources. Animal 14, 2378–2386. doi:10.1017/S1751731120001378
Houessou, S. O., Dossa, L. H., Diogo, R. V. C., Ahozonlin, M. C., Dahouda, M., and Schlecht, E. (2019b). Confronting Pastoralists' Knowledge of Cattle Breeds Raised in the Extensive Production Systems of Benin with Multivariate Analyses of Morphological Traits. PLoS ONE 14, e0222756. doi:10.1371/journal.pone.0222756
Houessou, S. O., Dossa, L. H., Diogo, R. V. C., Houinato, M., Buerkert, A., and Schlecht, E. (2019a). Change and Continuity in Traditional Cattle Farming Systems of West African Coast Countries: A Case Study from Benin. Agric. Syst. 168, 112–122. doi:10.1016/j.agsy.2018.11.003
Hu, Z.-L., Park, C. A., and Reecy, J. M. (2019). Building a Livestock Genetic and Genomic Information Knowledgebase through Integrative Developments of Animal QTLdb and CorrDB. Nucleic Acids Res. 47, D701–D710. doi:10.1093/nar/gky1084
Hudson, N. J., Porto-Neto, L. R., Kijas, J., McWilliam, S., Taft, R. J., and Reverter, A. (2014). Information Compression Exploits Patterns of Genome Composition to Discriminate Populations and Highlight Regions of Evolutionary Interest. BMC Bioinformatics 15, 66. doi:10.1186/1471-2105-15-66
Jombart, T. (2008). Adegenet: A R Package for the Multivariate Analysis of Genetic Markers. Bioinformatics 24, 1403–1405. doi:10.1093/bioinformatics/btn129
Jombart, T., and Ahmed, I. (2011). Adegenet 1.3-1: New Tools for the Analysis of Genome-Wide SNP Data. Bioinformatics 27, 3070–3071. doi:10.1093/bioinformatics/btr521
Khan, M. Z., Liu, L., Zhang, Z., Khan, A., Wang, D., Mi, S., et al. (2020). Folic Acid Supplementation Regulates Milk Production Variables, Metabolic Associated Genes and Pathways in Perinatal Holsteins. J. Anim. Physiol. Anim. Nutr. 104, 483–492. doi:10.1111/jpn.13313
Kim, J., Hanotte, O., Mwai, O. A., Dessie, T., Bashir, S., Diallo, B., et al. (2017). The Genome Landscape of Indigenous African Cattle. Genome Biol. 18, 34. doi:10.1186/s13059-017-1153-y
Kim, K., Kwon, T., Dessie, T., Yoo, D., Mwai, O. A., Jang, J., et al. (2020). The Mosaic Genome of Indigenous African Cattle as a Unique Genetic Resource for African Pastoralism. Nat. Genet. 52, 1099–1110. doi:10.1038/s41588-020-0694-2
Labrecque, R., Vigneault, C., Blondin, P., and Sirard, M.-A. (2014). Gene Expression Analysis of Bovine Oocytes at Optimal Coasting Time Combined with GnRH Antagonist during the No-FSH Period. Theriogenology 81, 1092–1100. doi:10.1016/j.theriogenology.2014.01.037
Larkin, D. M., Daetwyler, H. D., Hernandez, A. G., Wright, C. L., Hetrick, L. A., Boucek, L., et al. (2012). Whole-Genome Resequencing of Two Elite Sires for the Detection of Haplotypes under Selection in Dairy Cattle. Proc. Natl. Acad. Sci. 109, 7693–7698. doi:10.1073/pnas.1114546109
Lázaro, S. F., Tonhati, H., Oliveira, H. R., Silva, A. A., Nascimento, A. V., Santos, D. J. A., et al. (2021). Genomic Studies of Milk-Related Traits in Water Buffalo (Bubalus Bubalis) Based on Single-Step Genomic Best Linear Unbiased Prediction and Random Regression Models. J. Dairy Sci. 104, 5768–5793. doi:10.3168/jds.2020-19534
Lee, S. H., van der Werf, J., Lee, S. H., Lim, D. J., Park, E. W., Gondro, C., et al. (2012). Genome Wide QTL Mapping to Identify Candidate Genes for Carcass Traits in Hanwoo (Korean Cattle). Genes Genom 34, 43–49. doi:10.1007/s13258-011-0081-6
Leutenegger, A.-L., Prum, B., Génin, E., Verny, C., Lemainque, A., Clerget-Darpoux, F., et al. (2003). Estimation of the Inbreeding Coefficient through Use of Genomic Data. Am. J. Hum. Genet. 73, 516–523. doi:10.1086/378207
Li, L., Sun, Y., Wu, J., Li, X., Luo, M., and Wang, G. (2015). The Global Effect of Heat on Gene Expression in Cultured Bovine Mammary Epithelial Cells. Cell Stress and Chaperones 20, 381–389. doi:10.1007/s12192-014-0559-7
Lim, D., Lee, S.-H., Kim, N.-K., Cho, Y.-M., Chai, H.-H., Seong, H.-H., et al. (2013). Gene Co-Expression Analysis to Characterize Genes Related to Marbling Trait in Hanwoo (Korean) Cattle. Asian Australas. J. Anim. Sci. 26, 19–29. doi:10.5713/ajas.2012.12375
Lohmueller, K. E., Bustamante, C. D., and Clark, A. G. (2010). The Effect of Recent Admixture on Inference of Ancient Human Population History. Genetics 185, 611–622. doi:10.1534/genetics.109.113761
Lu, J., Argov-Argaman, N., Anggrek, J., Boeren, S., van Hooijdonk, T., Vervoort, J., et al. (2016). The Protein and Lipid Composition of the Membrane of Milk Fat Globules Depends on Their Size. J. Dairy Sci. 99, 4726–4738. doi:10.3168/jds.2015-10375
Ma, Y., Chen, N., Li, F., Fu, W., Han, Y., Chang, Y., et al. (2015). Bovine HSD17B8 Gene and its Relationship with Growth and Meat Quality Traits. Sci. Bull. 60, 1617–1621. doi:10.1007/s11434-015-0882-0
MacHugh, D. E., Shriver, M. D., Loftus, R. T., Cunningham, P., and Bradley, D. G. (1997). Microsatellite DNA Variation and the Evolution, Domestication and Phylogeography of Taurine and Zebu Cattle (Bos taurus and Bos indicus). Genetics 146, 1071–1086. doi:10.1093/genetics/146.3.1071
Maiorano, A. M., Lourenco, D. L., Tsuruta, S., Ospina, A. M. T., Stafuzza, N. B., Masuda, Y., et al. (2018). Assessing Genetic Architecture and Signatures of Selection of Dual Purpose Gir Cattle Populations Using Genomic Information. PLoS ONE 13, e0200694. doi:10.1371/journal.pone.0200694
Marín-Garzón, N. A., Magalhães, A. F. B., Schmidt, P. I., Serna, M., Fonseca, L. F. S., Salatta, B. M., et al. (2021). Genome-Wide Scan Reveals Genomic Regions and Candidate Genes Underlying Direct and Maternal Effects of Preweaning Calf Mortality in Nellore Cattle. Genomics 113, 1386–1395. doi:10.1016/j.ygeno.2021.02.021
Metodiev, S., Thekkoot, D. M., Young, J. M., Onteru, S., Rothschild, M. F., and Dekkers, J. C. M. (2018). A Whole-Genome Association Study for Litter Size and Litter Weight Traits in Pigs. Livestock Sci. 211, 87–97. doi:10.1016/j.livsci.2018.03.004
Mielczarek, M., Frąszczak, M., Giannico, R., Minozzi, G., Williams, J. L., Wojdak-Maksymiec, K., et al. (2017). Analysis of Copy Number Variations in Holstein-Friesian Cow Genomes Based on Whole-Genome Sequence Data. J. Dairy Sci. 100, 5515–5525. doi:10.3168/jds.2016-11987
Moazami Goudarzi, K., Belemsaga, D. M. A., Ceriotti, G., Laloë, D., Fagbohoum, F., Kouagou, N. T., et al. (2001). Caractérisation de la race bovine Somba à l'aide de marqueurs moléculaires. Rev. Elev. Med. Vet. Pays Trop. 54, 129. doi:10.19182/remvt.9791
Mohammadi, A., Alijani, S., Rafat, S. A., and Abdollahi-Arpanahi, R. (2020). Genome-Wide Association Study and Pathway Analysis for Female Fertility Traits in Iranian Holstein Cattle. Ann. Anim. Sci. 20, 825–851. doi:10.2478/aoas-2020-0031
Moravčíková, N., Kasarda, R., Vostrý, L., Krupová, Z., Krupa, E., Lehocká, K., et al. (2019). Analysis of Selection Signatures in the Beef Cattle Genome. Czech J. Anim. Sci. 64, 491–503. doi:10.17221/226/2019-CJAS
Murray, C., Huerta-Sanchez, E., Casey, F., and Bradley, D. G. (2010). Cattle Demographic History Modelled from Autosomal Sequence Variation. Phil. Trans. R. Soc. B 365, 2531–2539. doi:10.1098/rstb.2010.0103
Mwai, O., Hanotte, O., Kwon, Y.-J., and Cho, S. (2015). Invited Review - African Indigenous Cattle: Unique Genetic Resources in a Rapidly Changing World. Asian Australas. J. Anim. Sci. 28, 911–921. doi:10.5713/ajas.15.0002R
Naderi, S., Moradi, M. H., Farhadian, M., Yin, T., Jaeger, M., Scheper, C., et al. (2020). Assessing Selection Signatures within and between Selected Lines of Dual‐Purpose Black and White and German Holstein Cattle. Anim. Genet. 51, 391–408. doi:10.1111/age.12925
Naval-Sánchez, M., Porto-Neto, L. R., Cardoso, D. F., Hayes, B. J., Daetwyler, H. D., Kijas, J., et al. (2020). Selection Signatures in Tropical Cattle Are Enriched for Promoter and Coding Regions and Reveal Missense Mutations in the Damage Response Gene HELB. Genet. Sel. Evol. 52, 27. doi:10.1186/s12711-020-00546-6
Nezamzadeh, R., Seubert, A., Pohlenz, J., and Brenig, B. (2005). Identification of a Mutation in the Ovine Uroporphyrinogen Decarboxylase (UROD) Gene Associated with a Type of Porphyria. Anim. Genet. 36, 297–302. doi:10.1111/j.1365-2052.2005.01301.x
Oliveira, H. R., Lourenco, D. A. L., Masuda, Y., Misztal, I., Tsuruta, S., Jamrozik, J., et al. (2019). Single-Step Genome-Wide Association for Longitudinal Traits of Canadian Ayrshire, Holstein, and Jersey Dairy Cattle. J. Dairy Sci. 102, 9995–10011. doi:10.3168/jds.2019-16821
Olivieri, B. F., Mercadante, M. E. Z., Cyrillo, J. N. D. S. G., Branco, R. H., Bonilha, S. F. M., de Albuquerque, L. G., et al. (2016). Genomic Regions Associated with Feed Efficiency Indicator Traits in an Experimental Nellore Cattle Population. PLoS ONE 11, e0164390. doi:10.1371/journal.pone.0164390
Patterson, N., Moorjani, P., Luo, Y., Mallick, S., Rohland, N., Zhan, Y., et al. (2012). Ancient Admixture in Human History. Genetics 192, 1065–1093. doi:10.1534/genetics.112.145037
Payne, W. J. A., and Hodges, J. (1997). Tropical Cattle: Origins, Breeds and Breeding Policies. Oxford, United Kingdom: Blackwell Science.
Pecaud, M. G. (1912). L’élevage des Animaux Domestiques au Dahomey; Imp. du Gvt. Général de l'A.O.F: Gorée, Senegal, 157p.
Pérez-Rivas, L. G., Jerez, J. M., Carmona, R., de Luque, V., Vicioso, L., Claros, M. G., et al. (2014). A microRNA Signature Associated with Early Recurrence in Breast Cancer. PLoS ONE 9, e91884. doi:10.1371/journal.pone.0091884
Porter, V., Alderson, L., Hall, S. J. G., and Sponenberg, D. P. (2016). Mason's World Encyclopedia of Livestock Breeds and Breeding. Wallingford: CABI Publishing.
Porter, V. (1991). Cattle. A Handbook to the Breeds of the World. Facts on File. London: Christopher Helm.
Purcell, S., Neale, B., Todd-Brown, K., Thomas, L., Ferreira, M. A. R., Bender, D., et al. (2007). PLINK: A Tool Set for Whole-Genome Association and Population-Based Linkage Analyses. Am. J. Hum. Genet. 81, 559–575. doi:10.1086/519795
Purfield, D. C., Evans, R. D., and Berry, D. P. (2020). Breed- and Trait-Specific Associations Define the Genetic Architecture of Calving Performance Traits in Cattle. J. Anim. Sci. 98, 1–18. doi:10.1093/jas/skaa151
Qanbari, S., and Simianer, H. (2014). Mapping Signatures of Positive Selection in the Genome of Livestock. Livestock Sci. 166, 133–143. doi:10.1016/j.livsci.2014.05.003
Raj, A., Stephens, M., and Pritchard, J. K. (2014). fastSTRUCTURE: Variational Inference of Population Structure in Large SNP Data Sets. Genetics 197, 573–589. doi:10.1534/genetics.114.164350
Raschia, M. A., Nani, J. P., Maizon, D. O., Beribe, M. J., Amadio, A. F., and Poli, M. A. (2018). Single Nucleotide Polymorphisms in Candidate Genes Associated with Milk Yield in Argentinean Holstein and Holstein X Jersey Cows. J. Anim. Sci. Technol. 60, 31. doi:10.1186/s40781-018-0189-1
R. Blench, and K. MacDonald (Editors) (1999). The Origins and Development of African Livestock: Archaeology, Genetics, Linguistics and Ethnography (London, United Kingdom: Routledge).
Rege, J. E., Aboagye, G. S., and Tawah, C. L. (1994). Shorthorn Cattle of West and Central Africa. I. Origin, Distribution, Classification and Population Statistics. World Anim. Rev. 78, 2–13.
Rege, J. E. O. (1999). The State of African Cattle Genetic Resources I. Classification Framework and Identification of Threatened and Extinct Breeds. Anim. Genet. Resour. Inf. 25, 1–25. doi:10.1017/S1014233900003448
Rehman, S. U., Nadeem, A., Javed, M., Hassan, F.-U., Luo, X., Khalid, R. B., et al. (2020). Genomic Identification, Evolution and Sequence Analysis of the Heat-Shock Protein Gene Family in Buffalo. Genes 11, 1388. doi:10.3390/genes11111388
Salleh, M. S., Mazzoni, G., Höglund, J. K., Olijhoek, D. W., Lund, P., Løvendahl, P., et al. (2017). RNA-Seq Transcriptomics and Pathway Analyses Reveal Potential Regulatory Genes and Molecular Mechanisms in High- and Low-Residual Feed Intake in Nordic Dairy Cattle. BMC Genomics 18, 258. doi:10.1186/s12864-017-3622-9
Sanchez, M.-P., Ramayo-Caldas, Y., Wolf, V., Laithier, C., El Jabri, M., Michenet, A., et al. (2019). Sequence-Based GWAS, Network and Pathway Analyses Reveal Genes Co-Associated with Milk Cheese-Making Properties and Milk Composition in Montbéliarde Cows. Genet. Sel. Evol. 51, 34. doi:10.1186/s12711-019-0473-7
Scheper, C., Bohlouli, M., Brügemann, K., Weimann, C., Vanvanhossou, S. F. U., König, S., et al. (2020). The Role of Agro‐Ecological Factors and Transboundary Transhumance in Shaping the Genetic Diversity in Four Indigenous Cattle Populations of Benin. J. Anim. Breed. Genet. 137, 622–640. doi:10.1111/jbg.12495
Seabury, C. M., Oldeschulte, D. L., Saatchi, M., Beever, J. E., Decker, J. E., Halley, Y. A., et al. (2017). Genome-Wide Association Study for Feed Efficiency and Growth Traits in U.S. Beef Cattle. BMC Genomics 18, 386. doi:10.1186/s12864-017-3754-y
Sempéré, G., Moazami-Goudarzi, K., Eggen, A., Laloë, D., Gautier, M., and Flori, L. (2015). WIDDE: A Web-Interfaced Next Generation Database for Genetic Diversity Exploration, with a First Application in Cattle. BMC Genomics 16, 940. doi:10.1186/s12864-015-2181-1
Sengar, G. S., Deb, R., Singh, U., Raja, T. V., Kant, R., Sajjanar, B., et al. (2018). Differential Expression of microRNAs Associated with thermal Stress in Frieswal (Bos T X Bos I) Crossbred Dairy Cattle. Cell Stress and Chaperones 23, 155–170. doi:10.1007/s12192-017-0833-6
Seong, J., Yoon, H., and Kong, H. S. (2016). Identification of microRNA and Target Gene Associated with Marbling Score in Korean Cattle (Hanwoo). Genes Genom 38, 529–538. doi:10.1007/s13258-016-0401-y
Shi, Y., Zhao, P., Dang, Y., Li, S., Luo, L., Hu, B., et al. (2021). Functional Roles of the Chromatin Remodeler SMARCA5 in Mouse and Bovine Preimplantation Embryos†. Biol. Reprod. 105, 359–370. doi:10.1093/biolre/ioab081
Skibiel, A. L., Peñagaricano, F., Amorín, R., Ahmed, B. M., Dahl, G. E., and Laporta, J. (2018). In Utero Heat Stress Alters the Offspring Epigenome. Sci. Rep. 8, 14609. doi:10.1038/s41598-018-32975-1
Soares, R. A. N., Vargas, G., Duffield, T., Schenkel, F., and Squires, E. J. (2021). Genome-Wide Association Study and Functional Analyses for Clinical and Subclinical Ketosis in Holstein Cattle. J. Dairy Sci. 104, 10076–10089. doi:10.3168/jds.2020-20101
Stock, F., and Gifford-Gonzalez, D. (2013). Genetics and African Cattle Domestication. Afr. Archaeol. Rev. 30, 51–72. doi:10.1007/s10437-013-9131-6
Takeshima, S.-N., and Aida, Y. (2006). Structure, Function and Disease Susceptibility of the Bovine Major Histocompatibility Complex. Anim. Sci. J. 77, 138–150. doi:10.1111/j.1740-0929.2006.00332.x
Taye, M., Lee, W., Caetano-Anolles, K., Dessie, T., Cho, S., Jong Oh, S., et al. (2018). Exploring the Genomes of East African Indicine Cattle Breeds Reveals Signature of Selection for Tropical Environmental Adaptation Traits. Cogent Food Agric. 4, 1552552. doi:10.1080/23311932.2018.1552552
Taye, M., Lee, W., Caetano-Anolles, K., Dessie, T., Hanotte, O., Mwai, O. A., et al. (2017a). Whole Genome Detection of Signature of Positive Selection in African Cattle Reveals Selection for Thermotolerance. Anim. Sci. J. 88, 1889–1901. doi:10.1111/asj.12851
Taye, M., Lee, W., Jeon, S., Yoon, J., Dessie, T., Hanotte, O., et al. (2017b). Exploring Evidence of Positive Selection Signatures in Cattle Breeds Selected for Different Traits. Mamm. Genome 28, 528–541. doi:10.1007/s00335-017-9715-6
Tijjani, A., Utsunomiya, Y. T., Ezekwe, A. G., Nashiru, O., and Hanotte, O. (2019). Genome Sequence Analysis Reveals Selection Signatures in Endangered Trypanotolerant West African Muturu Cattle. Front. Genet. 10, 442. doi:10.3389/fgene.2019.00442
Tizioto, P. C., Coutinho, L. L., Decker, J. E., Schnabel, R. D., Rosa, K. O., Oliveira, P. S., et al. (2015). Global Liver Gene Expression Differences in Nelore Steers with Divergent Residual Feed Intake Phenotypes. BMC Genomics 16, 242. doi:10.1186/s12864-015-1464-x
Triska, P., Soares, P., Patin, E., Fernandes, V., Cerny, V., and Pereira, L. (2015). Extensive Admixture and Selective Pressure across the Sahel Belt. Genome Biol. Evol. 7, 3484–3495. doi:10.1093/gbe/evv236
Urbinati, I., Stafuzza, N. B., Oliveira, M. T., Chud, T. C. S., Higa, R. H., Regitano, L. C. D. A., et al. (2016). Selection Signatures in Canchim Beef Cattle. J. Anim. Sci Biotechnol 7, 29. doi:10.1186/s40104-016-0089-5
Utsunomiya, Y. T., Pérez O’Brien, A. M., Sonstegard, T. S., van Tassell, C. P., do Carmo, A. S., Mészáros, G., et al. (2013). Detecting Loci under Recent Positive Selection in Dairy and Beef Cattle by Combining Different Genome-Wide Scan Methods. PLoS ONE 8, e64280. doi:10.1371/journal.pone.0064280
Vanvanhossou, S. F. U., Koura, I. B., and Dossa, L. H. (2021). The Implications of Herd Entrustment Practice for the Sustainable Use of Cattle Genetic Resources in the (Agro)-Pastoral Systems of West Africa: A Case Study from Benin. Pastoralism 11, 8. doi:10.1186/s13570-020-00189-8
Verband Europäisches Dahomey-Zwergrind (2019). Tiere. Available at: http://www.dahomey-zwergrind.com/ (Accessed November 21, 2020).
Wang, M. D., Dzama, K., Hefer, C. A., and Muchadeyi, F. C. (2015). Genomic Population Structure and Prevalence of Copy Number Variations in South African Nguni Cattle. BMC Genomics 16, 894. doi:10.1186/s12864-015-2122-z
Wollny, C. B. A. (2003). The Need to Conserve Farm Animal Genetic Resources in Africa: Should Policy Makers Be Concerned? Ecol. Econ. 45, 341–351. doi:10.1016/S0921-8009(03)00089-2
Wurzinger, M., Mirkena, T., and Sölkner, J. (2014). Animal Breeding Strategies in Africa: Current Issues and the Way Forward. J. Anim. Breed. Genet. 131, 327–328. doi:10.1111/jbg.12116
Xu, L., Bickhart, D. M., Cole, J. B., Schroeder, S. G., Song, J., Tassell, C. P. V., et al. (2015). Genomic Signatures Reveal New Evidences for Selection of Important Traits in Domestic Cattle. Mol. Biol. Evol. 32, 711–725. doi:10.1093/molbev/msu333
Yu, S.-L., Lee, S.-M., Kang, M.-J., Jeong, H.-J., Sang, B.-C., Jeon, J.-T., et al. (2009). Identification of Differentially Expressed Genes Between Preadipocytes and Adipocytes Using Affymetrix Bovine Genome Array. J. Anim. Sci. Technol. 51, 443–452. doi:10.5187/JAST.2009.51.6.443
Zarek, C. M., Lindholm-Perry, A. K., Kuehn, L. A., and Freetly, H. C. (2017). Differential Expression of Genes Related to Gain and Intake in the Liver of Beef Cattle. BMC Res. Notes 10, 1. doi:10.1186/s13104-016-2345-3
Zhang, X., and Xie, J. (2019). Analysis of Proteins Associated with Quality Deterioration of Grouper Fillets Based on TMT Quantitative Proteomics during Refrigerated Storage. Molecules 24, 2641. doi:10.3390/molecules24142641
Zootierliste (2020). Dahomey-Zwergrind. Available at: https://www.zootierliste.de/ (Accessed December 21, 2020).
Keywords: Dahomey cattle, genomic inbreeding, admixture, haplotype, BoLA genes, adaptation
Citation: Vanvanhossou SFU, Yin T, Scheper C, Fries R, Dossa LH and König S (2021) Unraveling Admixture, Inbreeding, and Recent Selection Signatures in West African Indigenous Cattle Populations in Benin. Front. Genet. 12:657282. doi: 10.3389/fgene.2021.657282
Received: 22 January 2021; Accepted: 07 October 2021;
Published: 08 December 2021.
Edited by:
Olivier Hubert Hanotte, University of Nottingham, United KingdomReviewed by:
Sophie Thevenon, CIRAD, FranceAbdulfatai Tijjani, International Livestock Research Institute, Ethiopia
Copyright © 2021 Vanvanhossou, Yin, Scheper, Fries, Dossa and König. This is an open-access article distributed under the terms of the Creative Commons Attribution License (CC BY). The use, distribution or reproduction in other forums is permitted, provided the original author(s) and the copyright owner(s) are credited and that the original publication in this journal is cited, in accordance with accepted academic practice. No use, distribution or reproduction is permitted which does not comply with these terms.
*Correspondence: Sven König, U3Zlbi5Lb2VuaWdAYWdyYXIudW5pLWdpZXNzZW4uZGU=