- 1Department of Genetic Engineering and Biotechnology, School of Life Sciences, Shahjalal University of Science and Technology, Sylhet, Bangladesh
- 2Louvain Institute of Biomolecular Science and Technology, Université catholique de Louvain, Louvain-la-Neuve, Belgium
- 3Department of Dermatology, Sylhet Women’s Medical College, Sylhet, Bangladesh
Aquaporins (AQPs) are integral membrane proteins and found in all living organisms from bacteria to human. AQPs mainly involved in the transmembrane diffusion of water as well as various small solutes in a bidirectional manner are widely distributed in various human tissues. Human contains 13 AQPs (AQP0–AQP12) which are divided into three sub-classes namely orthodox aquaporin (AQP0, 1, 2, 4, 5, 6, and 8), aquaglyceroporin (AQP3, 7, 9, and 10) and super or unorthodox aquaporin (AQP11 and 12) based on their pore selectivity. Human AQPs are functionally diverse, which are involved in wide variety of non-infectious diseases including cancer, renal dysfunction, neurological disorder, epilepsy, skin disease, metabolic syndrome, and even cardiac diseases. However, the association of AQPs with infectious diseases has not been fully evaluated. Several studies have unveiled that AQPs can be regulated by microbial and parasitic infections that suggest their involvement in microbial pathogenesis, inflammation-associated responses and AQP-mediated cell water homeostasis. This review mainly aims to shed light on the involvement of AQPs in infectious and non-infectious diseases and potential AQPs-target modulators. Furthermore, AQP structures, tissue-specific distributions and their physiological relevance, functional diversity and regulations have been discussed. Altogether, this review would be useful for further investigation of AQPs as a potential therapeutic target for treatment of infectious as well as non-infectious diseases.
Introduction
Aquaporins (AQPs) are channel-forming integral membrane proteins and found in all living organisms from bacteria to human (Agre et al., 1998; Azad et al., 2011b, 2016, 2018, 2021) and even in chlorella virus (Gazzarrini et al., 2006). AQPs mainly facilitate the transmembrane diffusion of water as well as various small solutes and are involved in cellular trafficking and many physiological processes (Hedfalk et al., 2006; Törnroth-Horsefield et al., 2006; Azad et al., 2008; Törnroth-Horsefield et al., 2010; Janosi and Ceccarelli, 2013; Kaptan et al., 2015; Pelagalli et al., 2016; Canessa Fortuna et al., 2019). Functionally diverged human AQPs are involved in wide variety of non-infectious diseases including cancer, renal dysfunction, neurological disorder, epilepsy, skin disease, metabolic syndrome, and even cardiac diseases (Verkman et al., 2008a,b, 2014; Verkerk et al., 2019). Growing data suggest their possible involvement in cell volume regulating events associated with various non-infectious diseases (Verkman et al., 2014; Pelagalli et al., 2016; Meli et al., 2018). Consequently, AQPs have become a potential drug target in clinical medicine (Verkman, 2012; Verkman et al., 2014; Esteva-Font et al., 2016; Méndez-Giménez et al., 2018; Abir-Awan et al., 2019).
Many studies and several reviews have been done on the involvement of AQPs and their regulations in non-infectious diseases. However, limited studies have been done involving AQPs in infectious diseases although studies on plant-pathogens interactions involving AQPs have been advanced (Wang R. et al., 2018; Zhang et al., 2019; Li et al., 2020; Xu and Zwiazek, 2020). An infectious disease, an illness caused by bacteria, virus, fungi or parasite, is one of the foremost reasons of morbidity and mortality in individuals worldwide. The pathogen can spread and shed in different parts of the body as shown in Figure 1 and develop systematic or localized infection. Furthermore, dysbiosis of human microbiota imparts significant metabolic and immunologic perturbations on the host leading to many local and systemic diseases (Liang et al., 2018). Both acute and chronic inflammatory processes induced by microbial infections involve disbursement of huge metabolic energy, loss of function, tissue damage and destruction, vascular leakage and hemorrhages (Akıncı et al., 2013; Molinas et al., 2016; Meli et al., 2018). Infection-induced cell migration, production, and accumulation of effector molecules to the infected sites change the cell morphology, volume and movement, which are associated with alteration of cellular and tissue homeostasis (Medzhitov, 2008). The inability of cells to regulate fluid movement through the biological membranes results in imbalanced homeostasis and leads to severe alteration of cell physiology (Meli et al., 2018). Cells regulate their shapes and volume, and control homeostasis by utilizing water, the most abundant molecule in the body. Therefore, AQPs might have crucial roles for controlling cellular volume and homeostasis in infectious diseases.
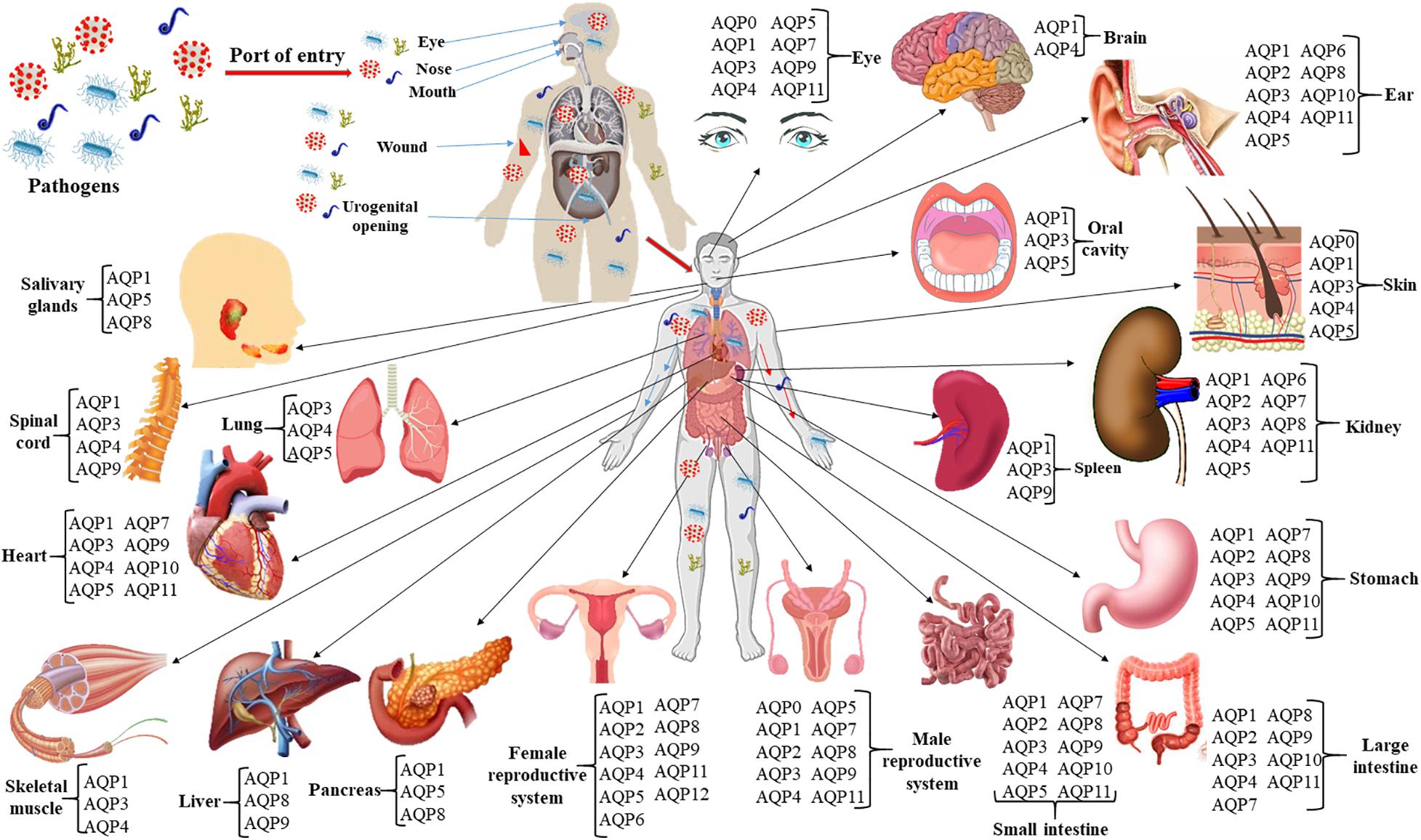
Figure 1. Infection route, spreading and shedding at different organs of the body and tissue-specific distribution of AQPs. The roles of AQPs in non-infectious diseases such as different cancers and tumors, cerebral edema and ischemic stroke, obesity, renal and skin diseases, and cataracts have been widely studied. At least 10 AQPs of different organs are associated with bacterial, viral, and parasitic infections. Expressions of AQPs in infectious diseases are summarized in Table 2 of the current review.
Based on the recent studies so far done on the involvement of AQPs in infections, we herein discuss the potential roles of AQPs in infectious diseases, infection-induced inflammatory process, and in maintenance of infection-mediated loss of cellular homeostasis or tissue damage. Furthermore, the structural and functional diversity of human AQPs and their roles in non-infectious diseases, and finally AQPs as the potential drug targets are also discussed. Discussions on the roles of AQPs in infectious and non-infectious diseases in the same review will stimulate researchers to focus and explore AQPs in infectious diseases. To the best of our knowledge, it is the first review to focus on the involvement of AQPs in infectious diseases.
Structural Properties of Human Aquaporins
Although the amino acid sequences differ substantially, the structure of AQPs is highly conserved having a common tetrameric arrangement; each subunit behaves as a functional channel (Murata et al., 2000; Gomes et al., 2009; Schenk et al., 2010; Azad et al., 2016, 2018). However, a fifth pore is formed in the center of the tetramer. Each monomer is constituted of six transmembrane (TM) α-helices (H1–H6) with five connecting loops (loops LA–LE) and cytoplasmic N- and C-termini and form an individual pore that specifies the transport activity (Figure 2). There are two main constrictions in the channel. The first constriction is formed by two highly conserved Asn-Pro-Ala (NPA) motifs on loops B and E that is involved in proton exclusion (Sui et al., 2001; Tajkhorshid et al., 2002). Both NPA motifs protrude into the membrane from opposite side and form the seventh pseudo TM helix. The second constriction, called the aromatic/arginine (ar/R) selectivity filter, is formed by four residues from helix H2 and H5, and loop E (LE1 and LE2) (Fu et al., 2000; Heymann and Engel, 2000; Sui et al., 2001; Tajkhorshid et al., 2002; Kitchen et al., 2019). Substitutions at this ar/R selectivity filter are thought to determine the broad spectrum of substrate conductance (Beitz et al., 2006; Azad et al., 2012). While all AQPs share the same structural core architecture, there are some distinct structural variations in loops and the N- and C-termini suggesting their functional and/or regulatory roles (Gonen and Walz, 2006; Azad et al., 2021).
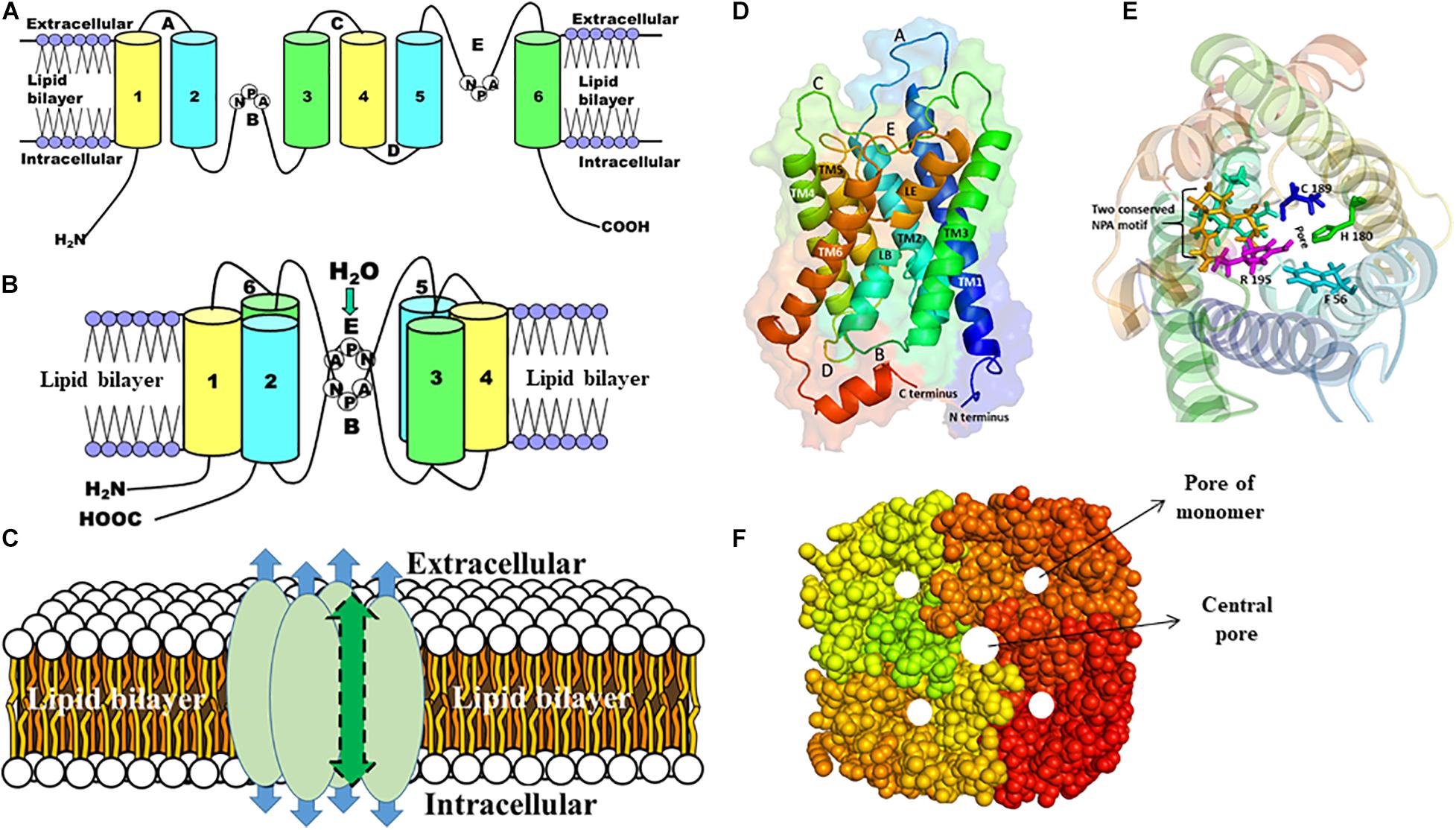
Figure 2. The structure of AQP monomer and homotetramers. A schematic representation of the general structures of AQP is shown (A–C). (A) Each AQP monomer has six transmembrane domains (1–6) spanning the plasma membrane, which are connected with five loops (A–E). (B) Two conserved NPA motifs in loops B and E are juxtaposed oppositely to form the channel through which molecules are passed. (C) Each AQP monomer contains independent pore (shown as blue arrow) and the monomers are assembled as tetramers to form a central pore (shown as green arrow). (D) Side view of the structure of the human AQP1 monomer, which shows six transmembrane α-helices (TM1-6) including pseudo TM (LE and LB) that are connected with five different loops (A–E). (E) The top view of the human AQP1 is shown. The residues (F56, H180, C189, and Arg195) in the ar/R constriction and two NPA motifs (yellow and cyan) are shown in sticks. (F) The top view of the AQP homotetramers with filled amino acid residues is shown. The pore of each monomer and the central pore of the homotetramers are shown as white circles.
Tissue-Specific Distribution and Physiological Relevance of Human Aquaporins
The complete tissue-specific distribution of AQPs throughout the human body and their physiological relevance are summarized (Figure 1 and Table 1). Several AQP isoforms (AQP1, 3, 4, 5, 7, 8, 9, and 11) are expressed in the brain and nervous system, however, AQP1 and AQP4 show relatively higher expression in the brain (Filippidis et al., 2016; Shchepareva and Zakharova, 2020). AQP1 is expressed in epithelial cells of the choroid plexus and plays a role in cerebrospinal fluid (CSF) formation (Brown et al., 2004). AQP1 is also present in primary sensory neurons and is proposed to be involved in pain perception (Borsani, 2010; Zhang and Verkman, 2010). AQP4 is found at the border between parenchyma and fluid-filled compartments and, more specifically, in astrocyte cell membranes, ependymal cells, and osmosensory areas of the hypothalamus (Jung et al., 1994; Xu et al., 2017). Eight (AQP0, 1, 3, 4, 5, 7, 9, and 11) of the 13 human AQPs are found in the eye (Figure 1; Tran et al., 2013; Schey et al., 2014). These AQPs perform various physiological roles such as maintenance of transparent cornea and lens, corneal wound healing, maintaining tear film osmolarity and retinal homeostasis (Schey et al., 2014). Nine AQPs (AQP1, 2, 3, 4, 5, 6, 8, 10, and 11) have been reported in human ear which are involved in neuronal signal transduction, regulating cell movement and lipid metabolism as well as immunological functions (Jung et al., 2017). Six AQPs are found in the skin, which are distributed to epidermis (AQP1, 3, 7, and 10), dermis (AQP1, 3, and 5), and hypodermis (AQP7) (Patel et al., 2017). Skin AQPs are associated with skin hydration, cell proliferation and differentiation, migration, immunity, and wound healing (Boury-Jamot et al., 2009; Sebastian et al., 2015; Bollag et al., 2020). Specifically, AQP3 is involved in epidermal cell proliferation and migration; AQP1 controls water movement between blood and dermis to maintain hydration; AQP5, found in both epical and basolateral membranes of the sweat gland is involved in water secretion; AQP7 plays important role to release glycerol from adipocytes for energy production and AQP9 facilitates the transport of glycerol, urea, and H2O2 for defense mechanism (Patel et al., 2017).
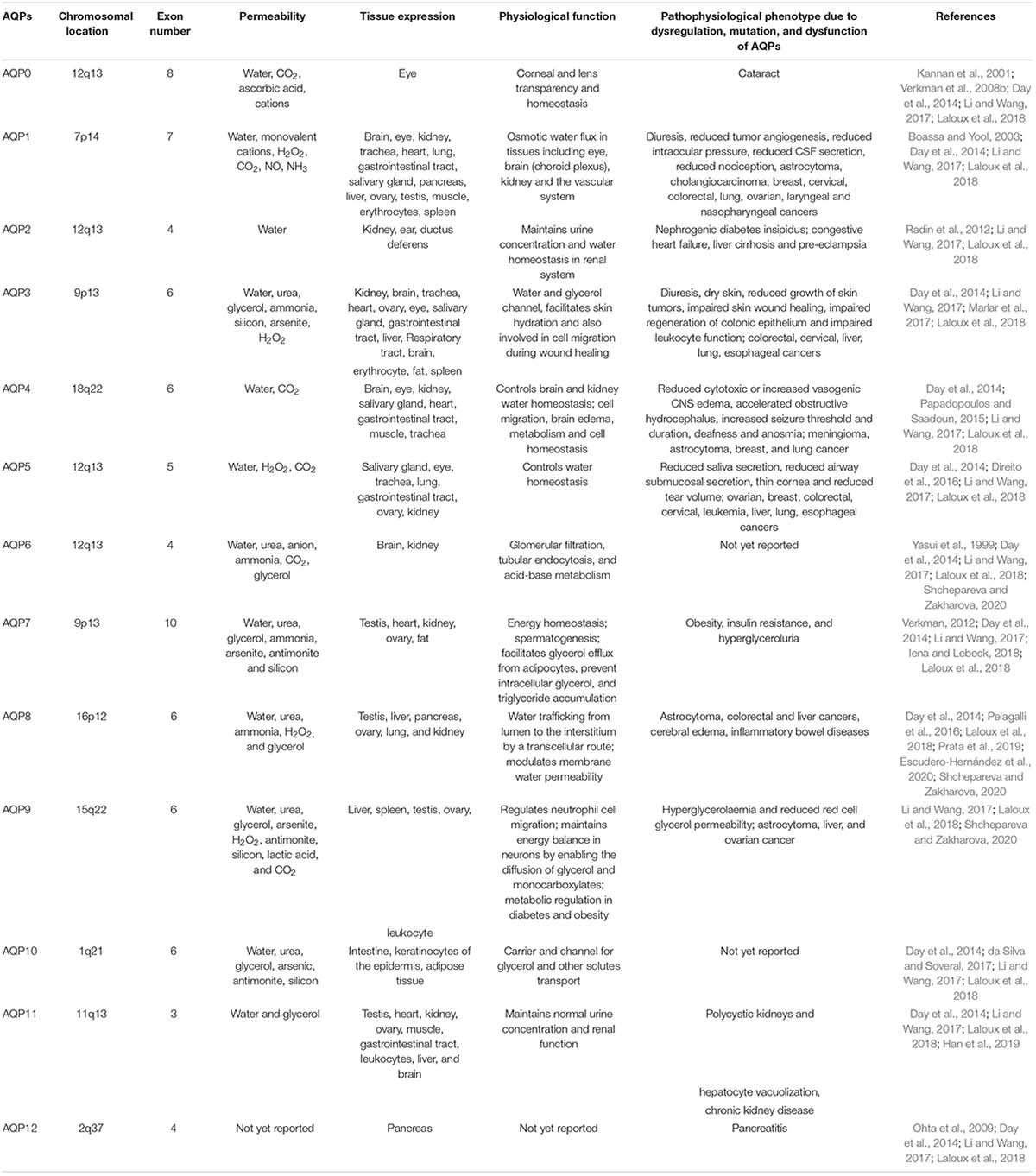
Table 1. Human AQPs major characteristics from chromosomal location to physiological relevance with the pathophysiological phenotypes due to their dysregulation, mutation, and dysfunction.
Nine AQPs (AQP1, 2, 3, 4, 5, 6, 7, 8, and 11) are expressed in kidneys to maintain the body water homeostasis, tissue development together with metamorphosis of various substances. Kidney AQPs play important roles in both the short- and long-term regulation of water balance (He and Yang, 2019; Su et al., 2020). AQP1 is one of the most abundant AQPs in the kidney, which is involved in water reabsorption in various segment of the kidney such as loop of Henle, apical and basolateral membranes of proximal tubules and vasa recta (Su et al., 2020). The remaining kidney AQPs are found in collecting duct and proximal tubule (Matsuzaki et al., 2017). Eight AQPs namely AQP1, 3, 4, 5, 7, 9, 10, and 11 are found in cardiovascular system (Figure 1). These AQP homologs located to the heart, endothelial cells, and vascular smooth muscle participate in the transportation of water, glycerol, and lactic acid, which play pivotal roles in cardiac physiology (Butler et al., 2006; Verkerk et al., 2019; Montiel et al., 2020). Ten AQPs (AQP0, 1, 2, 3, 4, 5, 7, 8, 9, and 11) expressed in male reproductive system (Figure 1) act as water, glycerol and ion channel during various process involved such as spermatogenesis, sperm osmoadaptation, and folliculogenesis (Day et al., 2014; Carrageta et al., 2020). These AQPs are localized in various sections of the male reproductive system including epididymis, testis, efferent ducts, prostate, seminiferous tubules, seminal vesicles, germ cells, sertoli cells, spermatids, spermatozoa, and vas deferens (Carrageta et al., 2020). AQP1, 2, 3, 4, 5, 6, 7, 8, 9, 11, and 12 have been shown to be expressed in different segments of the female reproductive system such as vagina, cervix, uterus, oviduct, ovary, oocyte, embryo, amnion, and chorion (Kordowitzki et al., 2020). Various types of biological process of the female reproductive system are conducted through AQPs like vaginal lubrication, sperm movement and implantation, and follicular development (Kordowitzki et al., 2020). In human digestive system, eleven AQP isoforms are found except AQP6 and 12 (Zhu et al., 2016; Liao et al., 2020). The most prolific AQP in the digestive system is AQP1 which is found in all organs including small and large intestine, salivary gland, oral cavity, esophagus, stomach, liver, gall bladder, bile duct, and pancreas (Zhu et al., 2016; Liao et al., 2020). Their extensive distribution indicates their wide range of biological functions like water and glycerol transportation, saliva excretion, adipose absorption, pancreatic excretion, cellular proliferation, migration, invasion, apoptosis, gastric acid secretion, and cellular signaling (Liao et al., 2020). AQP1, 3, and 4 are expressed in skeletal muscle (Figure 1). The localization of AQP1 and AQP4 within the muscle tissue suggests their possible function as water channel during muscular contraction (Au et al., 2004; Frigeri et al., 2004; Mobasheri et al., 2004). AQP1 and AQP3 identified within the nucleus pulposus cells of the human intervertebral disk might be involved in cell swelling during mechanistic load (Frigeri et al., 2004; Rutkovskiy et al., 2013). AQP9 is found in osteoclast cells, astrocytes, and catecholaminergic neurons; however, it is not essential for osteoclast function or differentiation under normal physiological conditions (Rutkovskiy et al., 2013; Halsey et al., 2018).
Functional Diversity and Regulation of Human Aquaporins
Aquaporins facilitate the transport of water and other small solutes such as glycerol, nitrate, urea, ammonia, H2O2, CO2, O2, arsenic, antimony, silicon, sodium ion, and aluminum malate (Table 1, Liu et al., 2002; Carbrey and Agre, 2009; Garneau et al., 2015; Byrt et al., 2017; Wang et al., 2017); some are physiologically important and some are toxic heavy metals (Bienert and Chaumont, 2011; Mukhopadhyay et al., 2014; Perez Di Giorgio et al., 2014; Finn and Cerdà, 2015). While AQPs play important physiological roles in humans, their mutations, malfunction or dysfunction, and dysregulation generate a lot of pathophysiological phenotypes leading to many severe diseases (Table 1). The transcellular water movement is strongly organized by the amount and activity of AQPs present in cellular membranes, and the movement of water molecules is occurred based on the osmotic gradient (Chaumont and Tyerman, 2014). All thirteen AQPs reported in human (AQP0–12) to date, are usually classified into three groups: orthodox AQPs (AQP0, 1, 2, 4, 5, 6, and 8), aquaglyceroporins (AQP3, 7, 9, and 10), and the unorthodox AQPs also called superaquaporins (AQP11 and 12) (Soto et al., 2012; Abascal et al., 2014; Finn et al., 2014; Finn and Cerdà, 2015). It has been reported that AQP3, 8, 9, and 11 are capable of facilitating the diffusion of H2O2 (Miller et al., 2010; Hara-Chikuma et al., 2012a; Bienert and Chaumont, 2014; Medraño-Fernandez et al., 2016; Watanabe et al., 2016; Bestetti et al., 2020; Hara-Chikuma et al., 2020), and the AQP-facilitated H2O2 transport is involved in psoriasis development and teleost spermatozoon motility (Chauvigné et al., 2015; Hara-Chikuma et al., 2015; Thiagarajah et al., 2017). AQP1, 3, 6, 7, 8, 9, and 10 are permeable to ammonia (Litman et al., 2009; Finn and Cerdà, 2015). The ammonia transporting mechanism is not yet fully resolved but some experimental data show that ammonia is transported in its neutral form, NH3. Aquaglyceroporins (AQP3, 7, 9, and 10) and AQP6 are also reported to transport the urea, which might be involved in energy metabolism (Litman et al., 2009). Human AQPs have been shown to facilitate diffusion of arsenite at neutral pH. Arsenite is used as a chemotherapeutic agent for acute promyelocytic leukemia and diseases caused by protozoan parasites because of its toxic properties to the cells (Rosen and Tamás, 2010). AQP3, 7, 9, and 10 have also been reported to facilitate the transport of antimonite (Liu et al., 2002). The overexpression of AQP has been shown to increase the uptake of antimonite and hypersensitivity of leukemia as well as lung adenocarcinoma cell lines (Bhattacharjee et al., 2004; Mukhopadhyay et al., 2014). Several studies reported that AQP0, 1, 4, 5, and 6 are involved in CO2 transport (Table 1). Recently, AQP1 has been shown to facilitate O2 transport by the spectrophotometric assay using yeast spheroplasts (Zwiazek et al., 2017). AQP1 is also reported to transport the nitric oxide (NO) (Herrera et al., 2006). In Xenopus oocytes, human AQP1 led to a PKA-activated and/or cGMP-activated ion permeability by its phosphorylation (Yool et al., 1996). Additionally, AQP6 acts as an anion channel, which is activated by low pH or Hg2+ (Hazama et al., 2002). The ion channel activity of AQPs could play a vital role for several pathophysiological processes including tumor progression (Saadoun et al., 2005a). It is speculated that the central pore of AQP tetramer could be the channel for transporting gases and ions (Hub and de Groot, 2006; Hub and de Groot, 2008). However, gases or ions permeation activities of AQPs are still controversial and required to be further investigated to figure out the physiological relevance of these new putative substrates. Two orthodox AQPs (AQP1 and 5) and four aquaglyceroporins (AQP3, 7, 9, and 10) expressed in the skin facilitate the transport of water and some other small solutes such as glycerol, which play critical roles in regulating numerous skin parameters (Boury-Jamot et al., 2006, 2009; Patel et al., 2017).
Aquaporins are synthesized and inserted in the endoplasmic reticulum membrane and finally localized to the target membrane via the secretory Sec61 translocon present in all domains of life (Pitonzo and Skach, 2006; Azad et al., 2011a). Their activity needs to be properly regulated in the target membrane to keep the nutrient homeostasis in cells (Chaumont and Tyerman, 2014). Presence of several AQPs in human suggests their multiple functions in cellular and organs levels. Some AQPs are present in intracellular vesicles, but can relocate later in the PM. For instance, AQP2 and AQP8 relocate to the PM from intracellular vesicles in renal collecting ducts and rat hepatocytes in response to vasopressin and cAMP, respectively (Marples et al., 1995; Nielsen et al., 1995). Some AQPs have intracellular functions, i.e., AQP8 and 9 were detected in the mitochondrial membrane (Amiry-Moghaddam et al., 2005; Calamita et al., 2005; Molinas et al., 2012). AQP trafficking is very dynamic process that at first targets the PM and removes it from the membrane for degradation or recycling in the endosome. Interestingly, the mislocalization of AQPs could lead to some human congenital disorders i.e., nephrogenic diabetes insipidus (NDI) is caused by AQP2 mislocalization (Bichet et al., 2012). The localization of AQP4, the predominant AQP isoform in the brain, relies on two C-terminus motifs namely a tyrosine motif (YxxΦ; Φ, V/L/I/F) and a dileucine-like motif. Upon mutation of any of the two motifs to alanine, AQP4 was relocated to the apical membrane instead of the basolateral membrane (Matter et al., 1992; Hunziker and Fumey, 1994). Phosphorylation is one of the important regulatory mechanisms that is involved in both gating and trafficking of AQPs (Li and Wang, 2017; Santoni, 2017; Takano et al., 2017). The precise relocalization of AQP2 to the PM from intracellular vesicles under vasopressin treatment involves the phosphorylation of the C-terminus Ser256 (Fushimi et al., 1997; van Balkom et al., 2002). However, the phosphorylation of Ser261 was found in vesicle-localized AQP2, which need to be dephosphorylated for PM relocation (Hoffert et al., 2007; Lu et al., 2008; Tamma et al., 2011). On the other hand, AQPs recycling is an important process for cells, and AQPs have been shown to be ubiquitinated to control its degradation (Leitch et al., 2001). For example, AQP2 is ubiquitinated at Lys270, which triggers its internalization in kidney collecting duct cells (Kamsteeg et al., 2006).
Roles of Aquaporins in Infectious Diseases
Pathogenic bacteria, fungi, virus, and parasites can cause systemic infection and spread to different organs of the body (Figure 1). Sepsis, the most common cause of death, appears as a clinical syndrome following a local infection accompanied with an appropriate inflammatory response and becomes amplified with detrimental effects on the whole body leading to dysfunction of numerous organs (Keel, 2014). Sepsis displayed by two or more systemic inflammatory response syndrome (SIRS) increases the risk of secondary infection. However, the immune system becomes stimulated in response to both local and systemic infection, which may influence the expression and function of AQPs to maintain cellular and tissue homeostasis (Table 2).
Bacterial Infections and AQPs
Bacteremia or septicemia occurs when bacteria spread in the bloodstream following a bacterial infection elsewhere in the body, such as the skin or lungs and so on. This is very precarious as the bacteria including their toxins can be carried through the bloodstream to the entire body. Bacteria are a prominent source of both endotoxins and exotoxins, which lead to severe sepsis and death (Opal, 2007; Ramachandran, 2014).
Bacterial endotoxins such as lipopolysaccharide (LPS) serve as an important stimulator to regulate the expression of human AQPs (Rump and Adamzik, 2018). Vassiliou et al. (2013) reported that expression of AQP1 is increased twofold in leukocytes in response to sepsis in patients of intensive care unit (ICU) (Table 2). They showed in vitro that LPS stimulates polymorphonuclear granulocytes (PMNs) for increased expression of AQP1, and the LPS-stimulated PMNs transiently increased cell volume upon hypotonic treatment. LPS exposure to cell lines also increased the expression of AQP1 but decreased that of AQP3 and AQP5 (Rump et al., 2013; Li et al., 2015). However, another study showed that LPS decreased the expression of AQP5, but not AQP3 and AQP4 in human primary bronchial epithelial cells (Shen et al., 2012). In LPS induced-endotoxemia, expression of AQP1 in the kidney and heart might have a protective role because mice deficient in AQP1 revealed predisposition to endotoxemia-induced acute kidney injury (AKI) (Wang et al., 2008) and cardiac hypertrophy (Madonna et al., 2012; Montiel et al., 2014), respectively (Table 2). However, upon exposure of LPS, expression of AQP1 and AQP5 is decreased in lung cells but that of AQP8 and AQP9 remains unaffected (Fisher et al., 2012; Ma and Liu, 2013; Tao et al., 2016; Kang et al., 2017). In animal models, several studies showed that LPS-induced endotoxemia down-regulated AQP2 expression in kidney (Grinevich et al., 2004; Chagnon et al., 2008; Olesen et al., 2009). However, pretreatment of model animals with continuous erythropoietin receptor activator or propofol or α-lipoic acid prevents AQP2 down-regulation and protects against endotoxemia-induced AKI (Chagnon et al., 2008; Cui et al., 2011; Rodrigues et al., 2012; Suh et al., 2015). While LPS-induced TNF-α down-regulates AQP8 in rat liver (Lehmann et al., 2008), systemic bacterial LPS stimulates up-regulation of AQP9 in rat brain (Wang et al., 2009). The LPS-induced TNF-α-mediated down-regulation of AQP8 in hepatocytes has been proposed as a potential molecular mechanism for pathogenesis of sepsis-associated cholestasis (Lehmann et al., 2008; Marinelli et al., 2011). The up-regulation of AQP9 expression is suggested to exert important roles in water transport associated with the pathophysiology of brain edema induced by LPS injection (Wang et al., 2009). Furthermore, the up-regulation of AQP4 in brain in response to LPS may aggravate the brain edema (Du et al., 2014). AQPs are supposed to play important role in septic encephalopathy associated with brain edema (Davies, 2002). It has been shown that AQP9 is up-regulated in blood leukocytes in response to intravenous bacterial LPS (Talwar et al., 2006). The expression of this homolog is increased in patients SIRS compared to healthy individuals (Matsushima et al., 2014), and in infective endocarditis patients (Thuny et al., 2012). Furthermore, Pseudomonas aeruginosa produces quorum sensing molecules and induce increased expression, distribution and reorganization of AQP9 in macrophages by changing the cell volume accompanied water fluxes across cell membrane through AQP9 (Holm et al., 2015, 2016) (Table 2). These events affect cell migration and phagocytosis which may have influence on the immunity, outcome of infection, inflammation, and thus disease development (Holm et al., 2015, 2016).
Expression of several AQPs is changed during diarrhea caused by enteropathogenic and enterohemorrhagic Escherichia coli, their LPS and enterotoxin of Vibrio cholerae (Flach et al., 2007; Zhu et al., 2016). Bacteria introduced into the body through oral ingestion of contaminated water or food colonize in the intestinal epithelial cells and employ different effector proteins in the host and alter the normal function of cells and cause diarrhea. Along with several other onset mechanisms of diarrhea such as reduction of absorptive surface area due to the effacement of microvilli (Donnenberg et al., 1993), altered ion channel activity (Guttman et al., 2006) or tight junction disruption resulting in loss of barrier function (Dean et al., 2006), AQPs are thought to contribute to the development of diarrhea after pathogen invasion (Guttman et al., 2007). At least six AQPs (AQP1, 3, 4, 5, 8, and 9) are localized in the apical and basolateral membranes of the human colon (Liao et al., 2020), which might be involved in the extraction of water and electrolyte from solid waste back into the body and thus dehydrate feces (Ma and Verkman, 1999). Inhibition, reduced expression and mislocalization of AQPs in the human colon might have roles in onset of diarrhea. Down-regulation of AQP3 in the duodenum is associated with acute diarrhea caused by V. cholera (Flach et al., 2007). In rat, the inhibition of AQP3 by HgCl2 and CuSO4 induced colon diarrhea keeping its expression static both in mRNA and protein levels (Ikarashi et al., 2012) (Table 2). In the same model, cholera toxin reduced the expression of mucosal AQP8 in the small intestine to cause diarrhea (Flach et al., 2004). Alteration in AQPs localization might be important in the onset of diarrhea. A mouse model infected with Citrobacter rodentium demonstrated that the AQP2 and AQP3 mislocalized within cytoplasm of colonocytes rather than their normal location in the cell membranes, and the infected mice eventually showed phenotypic diarrhea (Guttman et al., 2007). In piglet model, enterotoxigenic E. coli and LPS reduced expression of mucosal AQP8 in the jejunum and developed diarrhea (Loos et al., 2012; Hou et al., 2013). Several studies showed that some substances such as emodin, berberine, and MgSO4 may change and regulate water transport and absorption activity of AQPs involving the cAMP-dependent/p-CREB signaling pathway (Ikarashi et al., 2011; Zhang et al., 2012b; Liu et al., 2014; Zheng et al., 2014).
Helicobacter pylori regarded as the class I carcinogen in the human stomach and are responsible for the development of gastric carcinomas in the distal portion of the stomach (Konturek et al., 2009; Shin et al., 2010). Gastric carcinogenesis might be initiated from combination of events including increased DNA damage, decreased DNA repair activity and genetic instability in gastric cells due to mutation in mitochondrial DNA following H. pylori infection (Machado et al., 2010). H. pylori strains produce various toxins including cytotoxin-associated gene A (CagA) and vacuolating cytotoxin (VacA) to promote carcinogenesis (Jang et al., 2010). AQPs have shown its potential roles in tumor cell migration and proliferation (Hu and Verkman, 2006). A study showed that AQP3 and 5 expressed in higher levels in gastric carcinomas than the normal mucosa were associated with lymph node metastasis and lymphovascular invasion (Shen et al., 2010). Another independent study revealed that higher expression of AQP3 correlated with H. pylori infection status in gastric cancer tissues in comparison with the normal mucosa (Wang G. et al., 2012). This study further showed that H. pylori infection increased expression of AQP3 in gastric mucosa in a Sprague Dawley rat model. Recently, Wen et al. (2018) proposed that the up-regulation of AQP3 in gastric carcinoma caused by H. pylori was associated with the activation of reactive oxygen species (ROS) pathway (Table 2).
Although there are no enough studies associating infectious colitis with AQPs, a study showed a significant reduction in the expression of AQP7 and 8 both in protein and mRNA levels (Hardin et al., 2004). The down regulation of AQPs in infectious colitis may be associated with the microbiome of the host (Wang et al., 2019). Mice deficient in AQP4 alleviates experimental colitis, and it has been shown that there is significant difference in the microbiome of AQP4-knockout mice compared to the WT mice (Wang et al., 2019). The perturbation in the microbiome is further connected with the levels of inflammatory molecules such as IL-6, IL-10, and TNF. AQPs may modulate intestinal inflammation through the regulation of the abundance of intestinal microbiota (Wang et al., 2019).
Viral Infection and AQPs
As mentioned earlier, AQPs play important roles in cell volume, cell migration, organelle physiology through regulating cellular and tissue water homeostasis (Verkman, 2005; Verkman et al., 2008a; Karlsson et al., 2013a,b; Holm et al., 2015). Several studies have been done showing the involvement of AQPs with the viral infections (Table 2).
Very recently, it has been shown that human papilloma virus (HPV) infection affects the expression and functionality of AQP8, and lead to male sub-fertility (Pellavio et al., 2020). AQP8 expressed both in the PM and intragranular membranes of sperm cells has been suggested to facilitate the transport of water and H2O2, and the AQP8-mediated H2O2 permeability might be involved in ROS scavenging and thus detoxification (Laforenza et al., 2016; Pellavio et al., 2020). However, the direct interaction between the capsid protein L1 of the HPV and AQP8 alters the water and H2O2 permeability (Pellavio et al., 2020). The HPV is a common causative agent of cancer (Tornesello and Buonaguro, 2020). The HPV infection status and the overexpression of AQP1 and 3 are associated with poor outcome of cervical cancer (Table 2); however, their overexpression levels are not independent risk factors which are related with the prognosis of cervical carcinoma (Chen et al., 2014). These AQPs might have influenced the prognosis of cervical cancer by promoting HPV invasion, tumor growth and lymphatic metastasis (Chen et al., 2014).
In experimental mice model, Herpes simplex virus (HSV) decreased AQP4 in the acute phase of infection but increased its expression along with AQP1 in the long-term infection (Martinez Torres et al., 2007) (Table 2). As the AQP4 is widely expressed in the brain-blood interfaces (Manley et al., 2000), it might have regulated the pathophysiology of the acute and chronic HSV encephalitis (HSVE), and the modulation of AQP4 could be a potential target for treatment of HSVE (Martinez Torres et al., 2007). AQP4 antibody and concomitant HSV-1 and HSV-1 infections have been associated with myeloradiculitis and encephalopathy; consequently AQP4 antibody test could be routinely used for HSV infection-mediated encephalopathy or neuromyelitis optica and autoimmune AQP4 channelopathy (Marin Collazo et al., 2018; Peng et al., 2018). Immunohistochemistry revealed that the neuronal cell population that conveyed HSV-1 infection through the anterior commissure in a rat model was positive of AQP9 (Jennische et al., 2015). This study reports that the CSF samples from HSVE patients showed higher levels of AQP9 compared to controls, suggesting AQP9 to be involved in viral spreading and pathogenesis of HSVE (Jennische et al., 2015; Bello-Morales et al., 2020).
Crimean–Congo hemorrhagic fever virus (CCHFV), a highly pathogenic arthropod-borne agent, causes infectious disease with multiple organ failure and severe hemorrhage in vascular system in humans. Vascular permeability plays a pivotal role in the development of this disease which is regulated by AQPs. A study showed that infection by Hazara virus, a model for CCHFV, reduced cellular and prenuclear AQP6 distribution and changed the cell volume (Molinas et al., 2016) (Table 2). The viral infection down-regulated the expression of AQP6 at mRNA levels in human cells. However, overexpression of AQP8 in human cells displayed protective roles by decreasing the viral infectivity (Molinas et al., 2016). Recently, it has been reported that Epstein-Barr virus (EBV)-associated nasopharyngeal carcinoma (EBVaNPC) and lymphoma (EBVaL) are concomitant with the single-nucleotide polymorphism locus of AQP3 (rs2231231) (Wang J. et al., 2018). This study reveals that the homozygous genotype is commonly detected in patients with EBVaNPC and EBVaL. Neurological complications with the phenotype of neuromyelitis optica spectrum disorder (NMOSD) have been described in two patients with dengue fever following infection with the Dengue virus (Lana-Peixoto et al., 2018). Both patients showing brainstem symptoms or isolated unilateral optic neuritis were positive for serum AQP4 antibody (Lana-Peixoto et al., 2018), and AQP4 antibodies have been proposed as a pathogenic and diagnostic biomarker for NMOSD (Berger et al., 2017). A very recent case report concludes that AQP4 antibody positive NMOSD co-exists with varicella-zoster virus radiculomyelitis (Eguchi et al., 2020). It is reported that decrease of AQP4 is concomitant with astrocyte dysfunction for pathogenesis of cortical degeneration in HIV-associated neurocognitive disorders (Xing et al., 2017); however, increase of this AQP homolog is associated with HIV dementia (St Hillaire et al., 2005).
A study in mouse model infected with Rotavirus demonstrates that the down-regulation of AQP1, 4, and 8 are associated with Rotavirus diarrhea, a major worldwide cause of infantile gastroenteritis (Cao M. et al., 2014). Likewise, pulmonary adenoviral infections in mice down-regulated AQP1 and AQP5 in lung epithelia cells (Towne et al., 2000). However, higher expression of AQP1 at both mRNA and protein levels was reported in cirrhotic liver tissues in patients infected with Hepatitis B virus compared to normal tissues (Xian et al., 2009). Interestingly, Chlorella virus MT325 has been reported to have an AQP gene, aqpv1 which functions as an aquaglyceroporin in Xenopus oocytes (Gazzarrini et al., 2006). Co-expression of this viral AQP with its potassium channel in the Xenopus oocytes synergistically increases the water transport that could have pathophysiological relevance. However, no further investigation has been done with this viral AQP to confirm the pathophysiological relevance and other viral AQP is not yet reported.
Parasitic Infection and AQPs
Organisms known as parasites depend on a host for feeding and reproduction include members of numerous taxa mainly protozoa, helminths, and arthropods. In a global context, the most important human protozoan parasites including Leishmania and Plasmodium are transmitted by bloodsucking arthropods, and Toxoplasma is soil or food-born. Parasites harm their hosts by causing serious disease or even death (Ni et al., 2017; Walochnik et al., 2017).
Plasmodium parasite causing malaria disease in human infects the erythrocyte by passing through the multiple hepatocyte cells in the liver. In the liver stage, the parasite is matured to its infectious form merozoite and released to the blood stream to cause the symptoms of malaria. The obligatory liver stage is the critical part of this parasite invasion and AQP plays positive role in the nutrient transfer to the parasite from the host. During invasion in hepatocyte and blood cells, a parasitophorous vacuole membrane (PVM) derived from the host is formed around the parasite and act as an interface between the host cell and the parasite (Sherling and Ooij, 2016; Nyboer et al., 2018). The PVM is a prerequisite for Plasmodium growth and development. A study showed AQP3 localized in the PVM, facilitated the transport of water and glycerol to Plasmodium, and identified it as essential to parasite development in hepatoma cells (Posfai et al., 2018). Several studies revealed that AQP3 is recruited to the PVM in liver-stage P. berghei schizonts and blood-stage P. falciparum and P. vivax schizonts, and is thought to facilitate the transport of water or nutrients between the parasites and the host cell (Bietz et al., 2009; Posfai et al., 2018, 2020). These studies discussed that a significantly induced AQP3 expression was observed in the infected hepatocytes compared to the uninfected ones (Table 2). However, deletion of AQP3 or treatment with AQP3 inhibitor can reduce the pathogen burden in the liver and blood stage of infection (Bietz et al., 2009; Posfai et al., 2018, 2020). Therefore, AQP3 might have played important role in Plasmodium infection and its modulation would facilitate disease control efforts. Although human and rats have AQP3, the mice lack this AQP homolog but possess AQP9 that plays role as an aquaglyceroporin. AQP9-deficient mice missed the function of glycerol channel in their erythrocytes, and infected with P. berghei survived longer compared to the WT mice (Liu et al., 2007). This study suggests that the transport pathway through AQP9 may contribute to the virulence of intraerythrocytic stages of malarial infection (Table 2). However, AQP4 has been suggested to play protective roles in murine cerebral malaria (Promeneur et al., 2007). This study showed that mice infected with P. berghei and showing cerebral malaria displayed a reduction of brain AQP4 at transcript and protein levels. In comparison with the WT mice, AQP4-knockout mice showed earlier appearance and more severe signs of cerebral malaria with greater brain edema. Another independent study revealed that AQP4 has association with the immunoregulation of the liver granuloma formation during schistosomiasis developed by a parasite Schistosoma japonicum in mice (Zhang et al., 2015) (Table 2). S. japonicum-infected AQP4-null mice with schistosomiasis exhibited greater granulomatous response with increased accumulation of eosinophils, macrophages and Th2 but reduced Th1 and T regulatory cells generation. Upregulation of Th2 and Th17 cells and downregulation of Th1 and T regulatory cells are the hallmarks of granuloma formation in schistosomiasis (Baumgart et al., 2006; Turner et al., 2011; Wen et al., 2011).
Involvement of Aquaporins in Non-Infectious Diseases
The AQPs are involved in a wide range of human non-infectious diseases including cancer, cerebral edema and ischemic stroke, renal dysfunction, glaucoma, epilepsy, and obesity (Table 1). Defects in AQP genes may lead to several human diseases like hereditary NDI (Deen et al., 1994) and congenital cataracts (Verkman and Mitra, 2000).
AQPs in Cancer
Aquaporins play a key role in cancer pathogenesis and several tumor-related processes including tumor edema, tumor cell migration/invasion, tumor proliferation and angiogenesis (Saadoun et al., 2002a,b, 2005a,b; Hara-Chikuma and Verkman, 2006; Auguste et al., 2007; Warth et al., 2007). About twenty types of tumors have been shown to be associated with the expression of AQPs (Khajah and Luqmani, 2016). Astrocytomas are infiltrating brain tumors that arise from astrocytes, which are histologically classified as grades II, III, or IV, with the most malignant grade IV also termed as the glioblastoma. In human astrocytomas, the expression levels of AQP1, 4, and 9 are largely increased (Saadoun et al., 2002a,b; Nico et al., 2009; El Hindy et al., 2013; Jelen et al., 2013), while the level of AQP1 was shown to be either increased (Mazal et al., 2005) or decreased (Aishima et al., 2007) in cholangiocarcinoma. In comparison with the normal tissues, AQP1, 3, and 5 are up-regulated in colorectal (Moon et al., 2003; Yoshida et al., 2013; Shi et al., 2014), cervical (Zhang et al., 2012a), and breast cancers (Kang et al., 2015; Qin et al., 2016) but AQP8 is down-regulated in the former case (Wang W. et al., 2012). Higher levels of AQP3 and 5, and lower levels of AQP8 and 9 are reported in liver cancer (Jablonski et al., 2007; Guo et al., 2013). Several studies show that the overexpression of AQP1, 3, 4, and 5 is associated with lung cancer (Hoque et al., 2006; Machida et al., 2011; Xie et al., 2012). Similarly, increased level of AQP5 has been reported in chronic myelogenous leukemia (Chae et al., 2008), esophageal cancer (Liu et al., 2013), and pancreatic cancer (Burghardt et al., 2003). During tumor proliferation, AQP5 interacts with the Ras-MAPK pathway and cyclin D1/CDK4 complexes in colon cancer and with the EGFR/ERK1/2/p38 MAPK signaling cascade in lung cancer, resulting in enhanced proliferation, differentiation and survival (Kang et al., 2008; Zhang et al., 2010).
AQPs in Cerebral Edema and Ischemic Stroke
Cerebral edema plays a central role in the pathophysiology of many neurological disorders, including ischemic injury, traumatic brain injury, brain tumors leading to elevated intracranial pressure, decreased cerebral blood flow, ischemia, cerebral herniation, and death (Zador et al., 2009; Filippidis et al., 2016). AQP4 expressed in perivascular astrocyte end-feet plays a homeostatic role in water exchange between brain parenchyma and CSF in the ventricular and subarachnoid compartments (Amiry-Moghaddam et al., 2003). In the ischemic stroke mouse model, the presence of AQP4 was shown to aggravate post-ischemic cytotoxic edema as measured by post-ischemic hemispheric enlargement, while AQP4-null mice showed an opposite effect with an improved neurological outcome (Manley et al., 2000; Papadopoulos and Verkman, 2007). In the acute water intoxication model, AQP4-knockout mice had markedly reduced mortality from hyponatremia compared to WT mice. The protection attributed by the absence of AQP4 may be linked to reduced blood-brain barrier water permeability and a reduced rate of water flux into the brain parenchyma (Papadopoulos and Verkman, 2007). As a bidirectional water channel, AQP4 facilitates brain water accumulation in cytotoxic edema and clearance of excess brain water in vasogenic and interstitial edema (Verkman, 2012). In a mouse model of transient cerebral ischemia, AQP4 expression was rapidly up-regulated in perivascular end-feet, reaching the first peak after 1 hour (h) coinciding with early cerebral swelling in the core and border of the lesion, and the second peak in the penumbra after 48 h correlating with the degree of brain swelling (Ribeiro Mde et al., 2006). This finding suggests that AQP4 could be the major water channel involved in water movements after transient cerebral ischemia.
AQPs in Renal Diseases
In the kidney, eight AQPs are expressed in different segments and various cells to maintain normal urine concentration and renal function (Noda et al., 2010; Li et al., 2017). Among them, AQP2 that is crucial for urine concentration function is regulated by transcriptional factors and post-transcriptional modifications for its expression and function (He and Yang, 2019). Mutation or functional loss of AQP2 leads to NDI, a rare disease characterized by polyuria and polydipsia (Kotnik et al., 2007). The significant up-regulation of AQP5 in the kidney tissue of diabetic nephropathy (DN) patients leads to polyuria (Afkarian et al., 2016).
Chronic kidney disease (CKD) is a multifactorial disorder playing an important role in diabetes, hypertension, dyslipidemia and proteinuria (Tsai et al., 2016). A cohort study among 620 patients with CKD revealed that AQP11 rs2276415 variant is associated with CKD progression (Han et al., 2019). AQP1 is expressed in the epithelia lining 71% renal cysts in human autosomal dominant polycystic kidney disease (ADPKD), 44% of which are derived from the proximal tubules (Bachinsky et al., 1995). Two-thirds of the cysts express either AQP1 or renal collecting duct water channel AQP2 (Devuyst, 1998). It is reported that the overexpression of AQP1 inhibited renal cyst development by restraining Wnt/β-catenin signaling in an orthologous ADPKD mice model (Wang W. et al., 2015). On the other hand, the deletion of AQP1 promoted cyst development in embryonic kidney with polycystic kidney diseases (PKD) in mice. Analysis of the renal phenotype of AQP11-null mice showed that their kidneys are large, anemic and polycystic, which is similar with PKD in human. The cysts are absent in the medulla but abundant in the cortex where AQP11 is highly expressed (Li et al., 2017). The expression of AQP6 becomes changed with the development of renal cell carcinoma and oncocytoma (Yusenko et al., 2009; Tan et al., 2010).
AQPs in Obesity
Obesity is defined as the enlargement and inflammation of adipose tissues, which is one of the most important metabolic disorders of this century and its deposition in internal organs is a major risk for the development of diabetes, dyslipidemia, hypertension, atherosclerosis, cardiovascular, and neurodegenerative diseases commonly recognized as metabolic syndrome (Zimmet et al., 2001; Flegal et al., 2012). AQPs play an important role in adipose tissue biology as well as the onset of obesity. AQP7, an aquaglyceroporin that can release glycerol from adipocytes to tissue interstitium (Hara-Chikuma et al., 2005), has been identified in human and mice adipose tissues (Kuriyama et al., 1997; Ishibashi et al., 1998; Rojek et al., 2008) and adipocytes (Kishida et al., 2000; Miranda et al., 2010). Higher expression of AQP7 was observed in obese insulin-resistant mice and adipose tissues of type 2 diabetic rats compared to their control groups, suggesting that dysregulation of AQP7 might be associated with increased input of glycerol for hepatic gluconeogenesis and increased glucose level in type 2 diabetes (Kishida et al., 2000; Lee et al., 2005; Rojek et al., 2008). AQP7-null mice showed accumulation of cellular glycerol, triacylglycerols (TAG) and glycerol kinase up-regulation, which leads to development of progressive adipocyte hypertrophy and early obesity onset (Hara-Chikuma et al., 2005; Hibuse et al., 2005). However, although AQP7-null mice in some other studies did not confirm obesity development, all these studies confirmed the association of AQP7 with glycerol metabolism (Matsumura et al., 2007; Skowronski et al., 2007). The down- and up-regulation of AQP7 gene by feeding and fasting concomitant with glycerol production from endogenous TAG and lipolysis, respectively, are inversely related with plasma insulin levels (Kishida et al., 2000; da Silva and Soveral, 2017). In obese individuals, down-regulation of AQP7 in subcutaneous fat is associated with fat accumulation and adipocyte hypertrophy, however, it’s up-regulation in visceral fat is correlated with lipolysis (Rodríguez et al., 2011; Madeira et al., 2015). However, AQP7-null mice still show measurable glycerol secretion, indicating the presence of other glycerol transporters rather than AQP7 (Kishida et al., 2000). Indeed, among the other aquaglyceroporins, AQP3, 9, and 10 are found in the PM of adipocytes in subcutaneous and visceral adipose tissue, and additionally AQP3 in intragranular membranes (da Silva and Soveral, 2017). However, further research is necessary to investigate whether they are associated with obesity or not.
AQPs in Cataracts
Natural congenital autosomal dominant cataracts are clinically diverse and genetically heterogeneous due to AQP0 mutations in humans (Bateman et al., 2000; Berry et al., 2000; Geyer et al., 2006) and mice [Cataract Fraser (CatFr) (Shiels and Bassnett, 1996); cataract lens opacity (Catlop) (Shiels and Bassnett, 1996); cataract Tohoku (CatTohm) (Okamura et al., 2003)]. Knockout of AQP0 in mouse also resulted in cataract (Shiels et al., 2001).
AQPs in Skin Diseases
Skin AQPs aforementioned are expressed in deep to superficial including hypodermis, dermis and epidermis, and their mutation, dysregulation, malfunction or dysfunction lead numerous skin diseases (Patel et al., 2017). Although it is not yet confirmed, an inflammatory skin disease, erythema toxicum neonatorum characterized with papules or pustules only in infants is supposed to be associated with increased levels of AQP1 in epidermis, dermis, and blood vessels (Marchini et al., 2003; Patel et al., 2017). A recent study with keratinocyte cell lines has hypothesized that down-regulation of AQP1, 3, and 9 might be concomitant with the aging of the skin induced by long-term exposure to blue light (Avola et al., 2018).
Down-regulation and/or mislocalization of AQP3, the most abundant and largely studied aquaglyceroporin in the skin, are associated with psoriasis (Lee et al., 2012; Patel et al., 2017; Bollag et al., 2020) and vitiligo (Kim and Lee, 2010; Esmat et al., 2018). However, although some studies have reported increased mRNA levels of AQP3 in proliferating keratinocytes in psoriasis (Bowcock et al., 2001; Swindell et al., 2014), its expression is down-regulated with later differentiation of keratinocytes (Zheng and Bollinger Bollag, 2003). Another study reported that AQP3-null mice showed reduced psoriatic lesion development and epidermal hyperplasia, and suggested that AQP3 levels remained unchanged in psoriasis (Hara-Chikuma et al., 2015). Therefore, it is still controversial whether AQP3 is up- or down-regulated in psoriasis (Patel et al., 2017). Controversial observations are also reported on non-melanoma skin cancers (NMSC), basal and squamous cell carcinoma (SCC) involving AQP3. While a study with immunohistochemical approach revealed the down-regulation of AQP3 in NMSC and SCC (Seleit et al., 2015), another study observed up-regulation of this protein in both diseases (Ishimoto et al., 2012). The later observation was supported by the study showing AQP3-null mice resistant to tumor formation in the model of SCC development (Hara-Chikuma and Verkman, 2008). The up-regulation of AQP3 both in mRNA and protein levels has been demonstrated in atopic dermatitis lesions or eczema in human patients and mouse models (Olsson et al., 2006; Nakahigashi et al., 2011). The down-regulation of AQP3 is supposed to be associated with xeroderma observed in diabetes because streptozotocin-induced diabetic mice showed decreased levels of AQP3 with reduced dermal water content (Ikarashi et al., 2017; Bollag et al., 2020). Recent studies demonstrated that down-regulation of AQP3 might be involved in some other skin diseases such as bullous pemphigus (Korany et al., 2019) and symmetrical acrokeratoderma (Rong et al., 2019); however, it’s up-regulation might be involved in scleroderma/systemic sclerosis (Luo et al., 2016).
Although limited studies have been done on the association of other AQPs with skin disorder, in patients with palmoplantar keratoderma, a skin disease characterized by hyperkeratosis in the soles and palms, mutations in AQP5 have been identified (Blaydon et al., 2013; Cao X. et al., 2014; Krøigård et al., 2016). A study shows that dendritic cells deficient in AQP7 reduce their antigen presentation (Hara-Chikuma et al., 2012b). The pathophysiologic phenotypes in the skin due to loss of AQP9 and 10 or their malfunction or dysfunction are not yet known, it is supposed that these AQP homologs might have shared similar functions as AQP3 (Patel et al., 2017).
Aquaporins as Potential Drug and Diagnostic Targets
Aquaporins that facilitate the transport of water, the essential molecule of life, and some other physiologically important small molecules, and even ions, are expressed in all the organs of the human body (Figure 1 and Table 1). Discussion aforementioned reveals that the dysregulation, mutation, dysfunction and malfunction of AQPs have been associated as a key event with different life-threatening infectious and non-infectious diseases. Consequently, it has been essential to modulate the function or expression of AQPs during numerous infectious and non-infectious pathologies including different types of cancers, edema, obesity, brain injury, glaucoma, type 2 diabetes, NDI, AKI, CDK, DN, skin diseases, sepsis and sepsis-associated cholestasis, endotoximia and endotoximia-induced AKI, infective endocarditis, diarrhea, NMOSD, malaria, schistosomiasis, leishmaniasis, and several other conditions. Therefore, AQPs have been explored as an important drug and diagnostic target. Numerous studies have proposed many AQP-specific modulators and/or inhibitors including heavy metal-based inhibitors, cysteine inhibitors, small molecule inhibitors to modulate or inhibit the function and expression of human AQPs and parasite AQPs (Fadiel et al., 2009; Verkman et al., 2014; Wang J. et al., 2015; Esteva-Font et al., 2016; Méndez-Giménez et al., 2018; Abir-Awan et al., 2019; Hara-Chikuma et al., 2020).
Mercury inhibits the water transport function in AQPs with Cys 189, such as AQP1 (Esteva-Font et al., 2016). Similarly, heavy metal-based AQPs inhibitors such as silver- and gold-containing compounds were investigated as potential anticancer and anti-diabetic drugs in animal or human cell lines (Delporte et al., 2009; Madeira et al., 2014; Méndez-Giménez et al., 2018). Mercury and these heavy metals-based compounds interact with the cysteine in the vicinity of the highly conserved NPA motifs and inhibit the AQP permeability by causing blockage of the pore or conformational changes of the channel (Preston et al., 1993; Niemietz and Tyerman, 2002; Martins et al., 2012). However, mercury and heavy metals-based compounds cause irreversible cytotoxicity in human cells, and therefore, small molecule inhibitors/modulators without heavy metals to inhibit/modulate the function and expression of AQPs may have clinical implications against several diseases including cancers, diabetes and others (Best et al., 2009; Wang J. et al., 2015; Méndez-Giménez et al., 2018; Abir-Awan et al., 2019). The currently available AQP inhibitors, their structures, and the AQPs inhibited by them are listed in a very recent study (Abir-Awan et al., 2019). Acetazolamide, carbonic anhydrase inhibitor, used in glaucoma to reduce aqueous humor production, have been shown as irreversible inhibitor of AQP1 and 4 which protected tumor from cytotoxic edema and promoted tumor metastasis in glioma (Gao et al., 2006; Wang J. et al., 2015). Tetraethylammonium inhibits human AQP1, 2 and 4 expressed in Xenopus oocytes (Abir-Awan et al., 2019). Besides, anti-epileptic drugs such as topiramate, zonisamide, and lamotrigine are thought as possible inhibitors of AQP1, 4, and 5 although there is no conclusive evidence as safe AQP inhibitors (Shank and Maryanoff, 2008; Yang et al., 2008). AQP4-target inhibitor TGN-020 and aquaglyceroporins AQP3, AQP7 and AQP9-targeted inhibitors phloretin and compounds DFP00173 and Z433927330 have been identified (Abir-Awan et al., 2019; Sonntag et al., 2019). Auphen, an inhibitor of AQP3 that localize in the PVM between the host cell and the Plasmodium parasite reduces P. vivax liver hypnozoite and schizont burden, and inhibits P. vivax asexual blood-stage growth, and thus suggest that the AQP3 may be targeted for malaria treatment (Posfai et al., 2020). However, while some molecules are inhibitory in one study have no activity in other study due to variability within the experimental methods (Abir-Awan et al., 2019). Moreover, targeting the mechanism of calmodulin-mediated cell-surface localization of AQP4 instead of directly targeting the channel activity offers a new alternative smart approach for anti-edematous therapy (Kitchen et al., 2020).
It is certainly stimulating that the AQP gene (AQP1-cDNA) transfer has been developed and is under clinical trial (Baum et al., 2012). However, more studies are necessary to verify the efficacy and safety of AQP gene transfer. The recent discovery of new monoclonal antibody prevented macrophage-dependent liver injury by inhibition of AQP3-mediated H2O2 transport (Hara-Chikuma et al., 2020). Although the anti-AQP4 IgG poses no effect on water transport, it is under clinical trials for neuromyelitis optica treatments (Levy, 2017). However, AQP4-IgG possess diagnostics values in patients with NMOSD (Holmøy et al., 2020). In DN, AQP2 and 5 in urine can be used as potential novel biomarkers with sensitivity and early appearance (Wu et al., 2013; Lu et al., 2016; Han et al., 2019). Furthermore, AQP6 has been proposed as a biomarker for renal cancer diagnosis (Yusenko et al., 2009; Tan et al., 2010). In addition, AQP3, 5, 8, and 9 might be potential biomarker for several cancers of the digestive system namely colorectal, gastric, esophageal, and hepatocellular cancers (Nagaraju et al., 2016). However, there are still a lot of challenges in the way to develop AQPs-target modulators. Therefore, more studies with optimum high-throughput analysis are necessary to identify AQP modulators which are clinically urgent. Furthermore, AQPs as the potential diagnostic targets deserve additional studies.
Again, while Plasmodium spp. Toxoplasma gondii express one and two AQP homologs, respectively, Trypanosoma cruzi and Leishmania spp. encode up to five AQPs (Von Bülow and Beitz, 2015). In parasites, these AQPs are involved in multiple physiological processes such as regulation of osmotic pressure, nutrient uptake, and metabolic product efflux and/or host-parasite interactions (Von Bülow and Beitz, 2015; Ni et al., 2017; Ma et al., 2019). Furthermore, AQPs are the most abundant proteins in some parasites (Castro-Borges et al., 2011). Consequently, AQPs in parasites have become an important drug target (Ni et al., 2017). Although limited studies have been done on drug-like inhibitors for apicomplexan AQPs (Song et al., 2012; Meier et al., 2018), PbAQP-null P. berhei showed conspicuously reduced growth, virulence and progression through the liver stage (Promeneur et al., 2007; Promeneur et al., 2018). The TbAQP2 of three AQPs from Trypanosoma brucei plays an important role to uptake pantamidine, an anti-trypanosomal drug (Uzcategui et al., 2004; Von Bülow and Beitz, 2015; Song et al., 2016). AQPs in Leishmania major (LmAQP1) and Schistosoma mansoni (SmAQP) serve as channels for metalloids such as As and Sb from the anti-parasitic drugs (Gourbal et al., 2004; Faghiri and Skelly, 2009; Mukhopadhyay and Beitz, 2010; Mukhopadhyay et al., 2011). Furthermore, the SmAQP facilitates the transport of glycolytic-end product lactate to escape from toxicity (Faghiri et al., 2010; Meier et al., 2018). Substitution of Arg in the selectivity filter of LmAQP1 to Ala or Lys minimized the transport of As and Sb in cells, and thus increased the resistance to As and Sb in comparison with cells expressing the WT LmAQP1 (Figarella et al., 2007). Another study shows that the side chain of Ala163 in LmAQP1 may play role in drug resistance due to steric hindrance effect, and substitution of Thr164 to Cys imparts the mercury sensitivity by blocking the channel by HgCl2 (Mukhopadhyay et al., 2011). Very recently, it has been shown that RNA silencing treatment significantly reduced mRNA of TcAQP1 in adult Toxocara canis, a neglected parasitic nematode, keeping the phenotypic characteristics unchanged (Ma et al., 2019). The TcAQP1 has been suggested as a channel for drug uptake as the TcAQP1-knockout T. canis compromised the nematocidal activity of albendazole in vitro. These studies collectively suggest that parasite AQPs might be important candidate for therapeutic targets and drug entry routes. However, with human AQPs, researchers have to find out the specific effectors of pathogen AQPs with high affinity considering large number of studies. The physiological role of AQP has been extensively investigated only in E. coli and in lesser extent in Pseudomonas aeruginosa, Streptococcus mutans, and Lactobacillus plantarum (Tong et al., 2019). Therefore, to target the bacterial AQPs, the physiological role of this protein in bacteria should be established because the genomes of all bacteria have no AQP gene (Tong et al., 2019).
Conclusion and Future Perspectives
Humans and pathogens always struggle each other to survive, and for this, both parties use a wide range of different strategies and learn from the counterpart to evolve in close connection. The host–pathogen interaction is a dynamic, ever evolving process. Intriguingly, increasing studies aforementioned support the notion that AQPs are important players in the host–pathogen interaction. This review will further increase the understanding about the involvement of AQPs in infectious and non-infectious diseases. Several physiologically relevant substrates get access to cells via AQPs that can largely influence the cellular behavior, which will ultimately determine either the disease development or cell defense mechanisms. Furthermore, AQPs are the key players for maintenance cellular and tissue homeostasis during inflammation, the common event in disease development. Dysregulation, dysfunction and malfunction of AQPs during the disease development are therefore assuming an increasing translational value in pathophysiology with promising medical applications. The regulatory mechanisms of AQPs in infectious and non-infectious diseases seem to be tissue- and AQP-specific (Tables 1, 2 and Figure 1). Therefore, intensive research should be focused on the functional regulation of AQPs during infectious and non-infectious diseases. Investigation on the regulation and functional roles of AQPs would not only provide novel insights on the diagnosis and prognosis of diseases, but also facilitate the development of potential therapeutics. Furthermore, intensive knowledge of AQP physiology would be one of the key ways to overcome the limitation of currently available AQP pharmacological modulators/inhibitors. The tissue-specific distribution of AQPs might suggest the necessity of tissue-specific or cell-specific discovery of AQP modulators. However, although the involvement of AQP in different life-threatening diseases including infectious diseases is quite clear, research on AQP-specific modulators are not advanced enough. The translation of AQP research into the drug development would open a new window to treat the life-threatening diseases. For translating the AQP research to solve the real life problems, researchers have to focus the future research for better understanding the mechanistic relationship between modulation of AQP function and a reduction in a specific disease. Additionally, targeting a fundamental cellular process such as altering the subcellular localization of an AQP, rather than trying to block the substrate accessible pore, would be a broadly applicable strategy for future AQP-based drug development (Kitchen et al., 2020). Besides, their broad range of substrate transport capacity, their additional involvement in interactome, signaling and trafficking indicates that researchers need more research for better understanding of AQP mystery. Furthermore, it is a challenge for researchers to establish the speculation of gases and ions permeation through the central pore of the AQP tetramer in addition to the physiological roles of these substances in the human body.
A mentionable limitation of this review is that several research outcomes were discussed from model animal studies for mimicking human physiology due to small numbers of studies with humans and human cells. Elucidation of mechanistic expression and functional regulation of AQPs in human studies might be helpful for developing tissue- and AQP-specific novel therapeutics.
Author Contributions
AA and JA: concept and design. AA, TR, JA, AH, TE, and PC: partial manuscript writing. TR, JA, AH, and AA: figures and tables preparation. AA: data interpretation, compilation, supervision, and editing of the whole manuscript. All authors: contributed to the article and approved the submitted version.
Funding
This work was partially supported by grants in aid from the Research Centre, Shahjalal University of Science and Technology, Sylhet, Bangladesh (No. LS/2018/3/14).
Conflict of Interest
The authors declare that the research was conducted in the absence of any commercial or financial relationships that could be construed as a potential conflict of interest.
References
Abascal, F., Irisarri, I., and Zardoya, R. (2014). Diversity and evolution of membrane intrinsic proteins. Biochim. Biophys. Acta 1840, 1468–1481. doi: 10.1016/j.bbagen.2013.12.001
Abir-Awan, M., Kitchen, P., Salman, M. M., Conner, M. T., Conner, A. C., and Bill, R. M. (2019). Inhibitors of mammalian aquaporin water channels. Int. J. Mol. Sci. 20:1589. doi: 10.3390/ijms20071589
Afkarian, M., Zelnick, L. R., Hall, Y. N., Heagerty, P. J., Tuttle, K., Weiss, N. S., et al. (2016). Clinical manifestations of kidney disease among us adults with diabetes, 1988-2014. JAMA 316, 602–610. doi: 10.1001/jama.2016.10924
Agre, P., Bonhivers, M., and Borgnia, M. J. (1998). The aquaporins, blueprints for cellular plumbing systems. J. Biol. Chem. 273, 14659–14662. doi: 10.1074/jbc.273.24.14659
Aishima, S., Kuroda, Y., Nishihara, Y., Taguchi, K., Iguchi, T., Taketomi, A., et al. (2007). Down-regulation of aquaporin-1 in intrahepatic cholangiocarcinoma is related to tumor progression and mucin expression. Hum. Pathol. 38, 1819–1825. doi: 10.1016/j.humpath.2007.04.016
Akıncı, E., Bodur, H., and Leblebicioglu, H. (2013). Pathogenesis of crimean-congo hemorrhagic fever. Vector Borne Zoonotic Dis. 13, 429–437. doi: 10.1089/vbz.2012.1061
Amiry-Moghaddam, M., Lindland, H., Zelenin, S., Roberg, B. A., Gundersen, B. B., et al. (2005). Brain mitochondria contain aquaporin water channels: evidence for the expression of a short AQP9 isoform in the inner mitochondrial membrane. FASEB J. 19, 1459–1467. doi: 10.1096/fj.04-3515com
Amiry-Moghaddam, M., Otsuka, T., Hurn, P. D., Traystman, R. J., Haug, F. M., Froehner, S. C., et al. (2003). An alpha-syntrophin-dependent pool of AQP4 in astroglial end-feet confers bidirectional water flow between blood and brain. Proc. Natl. Acad. Sci. U.S.A. 100, 2106–2111. doi: 10.1073/pnas.0437946100
Au, C. G., Cooper, S. T., Lo, H. P., Compton, A. G., Yang, N., Wintour, E. M., et al. (2004). Expression of aquaporin 1 in human cardiac and skeletal muscle. J. Mol. Cell Cardiol. 36, 655–662. doi: 10.1016/j.yjmcc.2004.01.009
Auguste, K. I., Jin, S., Uchida, K., Yan, D., Manley, G. T., Papadopoulos, M. C., et al. (2007). Greatly impaired migration of implanted aquaporin-4-deficient astroglial cells in mouse brain toward a site of injury. FASEB J. 21, 108–116. doi: 10.1096/fj.06-6848com
Avola, R., Graziano, A. C. E., Pannuzzo, G., and Cardile, V. (2018). Blue light induces down-regulation of aquaporin 1, 3, and 9 in human keratinocytes. Cells 7:197. doi: 10.3390/cells7110197
Azad, A. K., Ahmed, J., Alum, M. A., Hasan, M. M., Ishikawa, T., and Sawa, Y. (2018). Prediction of arsenic and antimony transporter major intrinsic proteins from the genomes of crop plants. Int. J. Biol.Macromol. 107, 2630–2642. doi: 10.1016/j.ijbiomac.2017.10.153
Azad, A. K., Ahmed, J., Alum, M. A., Hasan, M. M., Ishikawa, T., Sawa, Y., et al. (2016). Genome-wide characterization of major intrinsic proteins in four grass plants and their non-aqua transport selectivity profiles with comparative perspective. PLoS One 11:e0157735. doi: 10.1371/journal.pone.0157735
Azad, A. K., Ahmed, J., Hakim, A., Hasan, M. M., Alum, M. A., Hasan, M., et al. (2021). Genome-wide characterization deciphers distinct properties of aquaporins in six Phytophthora species. Curr. Bioinform. 16:1. doi: 10.2174/1574893616666210204150332
Azad, A. K., Jahan, M. A., Hasan, M. M., Ishikawa, T., Sawa, Y., and Shibata, H. (2011a). Molecular cloning and sequence and 3D models analysis of the Sec61α subunit of protein translocation complex from Penicillium ochrochloron. BMB Rep. 44, 719–724. doi: 10.5483/BMBRep.2011.44.11.719
Azad, A. K., Katsuhara, M., Sawa, Y., Ishikawa, T., and Shibata, H. (2008). Characterization of four plasma membrane aquaporins in tulip petals: a putative homolog is regulated by phosphorylation. Plant Cell Physiol. 49, 1196–1208. doi: 10.1093/pcp/pcn095
Azad, A. K., Sato, R., Ohtani, K., Sawa, Y., Ishikawa, T., and Shibata, H. (2011b). Functional characterization and hyperosmotic regulation of aquaporin in Synechocystis sp. PCC 6803. Plant Sci. 180, 375–382. doi: 10.1016/j.plantsci.2010.10.010
Azad, A. K., Yoshikawa, N., Ishikawa, T., Sawa, Y., and Shibata, H. (2012). Substitution of a single amino acid residue in the aromatic/arginine selectivity filter alters the transport profiles of tonoplast aquaporin homologs. Biochim. Biophys. Acta 1818, 1–11. doi: 10.1016/j.bbamem.2011.09.014
Bachinsky, D. R., Sabolic, I., Emmanouel, D. S., Jefferson, D. M., Carone, F. A., Brown, D., et al. (1995). Water channel expression in human ADPKD kidneys. Am. J. Physiol. 268:F398. doi: 10.1152/ajprenal.1995.268.3.F398
Bateman, J. B., Johannes, M., Flodman, P., Geyer, D. D., Clancy, K. P., Heinzmann, C., et al. (2000). A new locus for autosomal dominant cataract on chromosome 12q13. Investig. Ophthalmol. Vis. Sci. 41, 2665–2670.
Baum, B. J., Alevizos, I., Zheng, C., Cotrim, A. P., Liu, S., Mccullagh, L., et al. (2012). Early responses to adenoviral-mediated transfer of the aquaporin-1 cDNA for radiation-induced salivary hypofunction. Proc. Natl. Acad. Sci. U.S.A. 109, 19403–19407. doi: 10.1073/pnas.1210662109
Baumgart, M., Tompkins, F., Leng, J., and Hesse, M. (2006). Naturally occurring CD4+Foxp3+ regulatory T cells are an essential, IL-10-independent part of the immunoregulatory network in Schistosoma mansoni egg-induced inflammation. J. Immunol. 176, 5374–5387. doi: 10.4049/jimmunol.176.9.5374
Beitz, E., Wu, B., Holm, L. M., Schultz, J. E., and Zeuthen, T. (2006). Point mutations in the aromatic/arginine region in aquaporin 1 allow passage of urea, glycerol, ammonia, and protons. Proc. Natl. Acad. Sci. U.S.A. 103, 269–274. doi: 10.1073/pnas.0507225103
Bello-Morales, R., Andreu, S., and López-Guerrero, J. A. (2020). The role of herpes simplex virus Type 1 infection in demyelination of the central nervous system. Int. J. Mol. Sci. 21:5026. doi: 10.1186/s12883-016-0779-9
Berger, B., Hottenrott, T., Rauer, S., and Stich, O. (2017). Screening for onconeural antibodies in neuromyelitis optica spectrum disorders. BMC Neurol. 17:5. doi: 10.1186/s12883-016-0779-9
Berry, V., Francis, P., Kaushal, S., Moore, A., and Bhattacharya, S. (2000). Missense mutations in MIP underlie autosomal dominant ‘polymorphic’ and lamellar cataracts linked to 12q. Nat. Genet. 25, 15–17. doi: 10.1038/75538
Best, L., Brown, P. D., Yates, A. P., Perret, J., Virreira, M., Beauwens, R., et al. (2009). Contrasting effects of glycerol and urea transport on rat pancreatic beta-cell function. Cell Physiol. Biochem. 23, 255–264. doi: 10.1159/000218172
Bestetti, S., Galli, M., Sorrentino, I., Pinton, P., Rimessi, A., Sitia, R., et al. (2020). Human aquaporin-11 guarantees efficient transport of H2O2 across the endoplasmic reticulum membrane. Redox Biol. 28, 101326–101326. doi: 10.1016/j.redox.2019.101326
Bhattacharjee, H., Carbrey, J., Rosen, B. P., and Mukhopadhyay, R. (2004). Drug uptake and pharmacological modulation of drug sensitivity in leukemia by AQP9. Biochem. Bioph. Res. Commun. 322, 836–841. doi: 10.1016/j.bbrc.2004.08.002
Bichet, D. G., El Tarazi, A., Matar, J., Lussier, Y., Arthus, M.-F., Lonergan, M., et al. (2012). Aquaporin-2: new mutations responsible for autosomal-recessive nephrogenic diabetes insipidus-update and epidemiology. Clin. Kid. J. 5, 195–202. doi: 10.1093/ckj/sfs029
Bienert, G. P., and Chaumont, F. (2011). “Plant aquaporins: roles in water homeostasis, nutrition, and signaling processes,” in Transporters and Pumps in Plant Signaling. Signaling and Communication in Plants, eds M. Geisler and K. Venema, (Berlin.: Springer), 7. doi: 10.1007/978-3-642-14369-4_1
Bienert, G. P., and Chaumont, F. (2014). Aquaporin-facilitated transmembrane diffusion of hydrogen peroxide. Biochim. Biophys Acta 1840, 1596–1604. doi: 10.1016/j.bbagen.2013.09.017
Bietz, S., Montilla, I., Külzer, S., Przyborski, J. M., and Lingelbach, K. (2009). Recruitment of human aquaporin 3 to internal membranes in the Plasmodium falciparum infected erythrocyte. Mol. Biochem. Parasitol. 167, 48–53. doi: 10.1016/j.molbiopara.2009.04.006
Blaydon, D. C., Lind, L. K., Plagnol, V., Linton, K. J., Smith, F. J., Wilson, N. J., et al. (2013). Mutations in AQP5, encoding a water-channel protein, cause autosomal-dominant diffuse nonepidermolytic palmoplantar keratoderma. Am. J. Hum. Genet. 93, 330–335. doi: 10.1016/j.ajhg.2013.06.008
Boassa, D., and Yool, A. J. (2003). Single amino acids in the carboxyl terminal domain of aquaporin-1 contribute to cGMP-dependent ion channel activation. BMC Physiol. 3:12. doi: 10.1186/1472-6793-3-12
Bollag, W. B., Aitkens, L., White, J., and Hyndman, K. A. (2020). Aquaporin-3 in the epidermis: more than skin deep. Am. J. Physiol. Cell Physiol. 318, C1144–C1153. doi: 10.1152/ajpcell.00075.2020
Borsani, E. (2010). Aquaporins in sensory and pain transmission. Curr. Neuropharmacol. 8, 122–127. doi: 10.2174/157015910791233187
Boury-Jamot, M., Daraspe, J., Bonté, F., Perrier, E., Schnebert, S., Dumas, M., et al. (2009). Skin aquaporins: function in hydration, wound healing, and skin epidermis homeostasis. Handb. Exp. Pharmacol. 190, 205–217. doi: 10.1007/978-3-540-79885-9_10
Boury-Jamot, M., Sougrat, R., Tailhardat, M., Le Varlet, B., Bonté, F., Dumas, M., et al. (2006). Expression and function of aquaporins in human skin: is aquaporin-3 just a glycerol transporter? Biochim. Biophys. Acta 1758, 1034–1042. doi: 10.1016/j.bbamem.2006.06.013
Bowcock, A. M., Shannon, W., Du, F., Duncan, J., Cao, K., Aftergut, K., et al. (2001). Insights into psoriasis and other inflammatory diseases from large-scale gene expression studies. Hum. Mol. Genet. 10, 1793–1805. doi: 10.1093/hmg/10.17.1793
Brown, P. D., Davies, S. L., Speake, T., and Millar, I. D. (2004). Molecular mechanisms of cerebrospinal fluid production. Neuroscience 129, 957–970. doi: 10.1016/j.neuroscience.2004.07.003
Burghardt, B., Elkaer, M. L., Kwon, T. H., Rácz, G. Z., Varga, G., Steward, M. C., et al. (2003). Distribution of aquaporin water channels AQP1 and AQP5 in the ductal system of the human pancreas. Gut 52, 1008–1016. doi: 10.1136/gut.52.7.1008
Butler, T. L., Au, C. G., Yang, B., Egan, J. R., Tan, Y. M., Hardeman, et al. (2006). Cardiac aquaporin expression in humans, rats, and mice. Am. J. Physiol. Heart Circ. Physiol. 291, H705–H713. doi: 10.1152/ajpheart.00090.2006
Byrt, C. S., Zhao, M., Kourghi, M., Bose, J., Henderson, S. W., Qiu, J., et al. (2017). Non-selective cation channel activity of aquaporin AtPIP2;1 regulated by Ca(2+) and pH. Plant Cell Environ. 40, 802–815. doi: 10.1111/pce.12832
Calamita, G., Ferri, D., Gena, P., Liquori, G. E., Cavalier, A., and Thomas, D. (2005). The inner mitochondrial membrane has aquaporin-8 water channels and is highly permeable to water. J. Biol. Chem. 280, 17149–17153. doi: 10.1074/jbc.C400595200
Canessa Fortuna, A., Zerbetto, De Palma, G., Aliperti Car, L., Armentia, L., Vitali, V., et al. (2019). Gating in plant plasma membrane aquaporins: the involvement of leucine in the formation of a pore constriction in the closed state. FEBS J. 286, 3473–3487. doi: 10.1111/febs.14922
Cao, M., Yang, M., Ou, Z., Li, D., Geng, L., Chen, P., et al. (2014). Involvement of aquaporins in a mouse model of rotavirus diarrhea. Virol. Sin. 29, 211–217. doi: 10.1007/s12250-014-3469-z
Cao, X., Yin, J., Wang, H., Zhao, J., Zhang, J., Dai, L., et al. (2014). Mutation in AQP5, encoding aquaporin 5, causes palmoplantar keratoderma Bothnia type. J. Invest. Dermatol. 134, 284–287. doi: 10.1038/jid.2013.302
Carbrey, J. M., and Agre, P. (2009). Discovery of the aquaporins and development of the field. Handb. Exp. Pharmacol. 190, 3–28. doi: 10.1007/978-3-540-79885-9_1
Carrageta, D. F., Bernardino, R. L., Soveral, G., Calamita, G., Alves, M. G., and Oliveira, P. F. (2020). Aquaporins and male (in)fertility: expression and role throughout the male reproductive tract. Arch. Biochem. Biophys. 679:108222. doi: 10.1016/j.abb.2019.108222
Castro-Borges, W., Simpson, D. M., Dowle, A., Curwen, R. S., Thomas-Oates, J., Beynon, R. J., et al. (2011). Abundance of tegument surface proteins in the human blood fluke Schistosoma mansoni determined by QconCAT proteomics. J. Proteomics 74, 1519–1533. doi: 10.1016/j.jprot.2011.06.011
Chae, Y. K., Kang, S. K., Kim, M. S., Woo, J., Lee, J., Chang, S., et al. (2008). Human AQP5 plays a role in the progression of chronic myelogenous leukemia (CML). PLoS One 3:e2594. doi: 10.1371/journal.pone.0002594
Chagnon, F., Vaidya, V. S., Plante, G. E., Bonventre, J. V., Bernard, A., Guindi, C., et al. (2008). Modulation of aquaporin-2/vasopressin2 receptor kidney expression and tubular injury after endotoxin (lipopolysaccharide) challenge. Crit. Care Med. 36, 3054–3061. doi: 10.1097/CCM.0b013e318186a938
Chaumont, F., and Tyerman, S. D. (2014). Aquaporins: highly regulated channels controlling plant water relations. Plant Physiol. 164, 1600–1618. doi: 10.1104/pp.113.233791
Chauvigné, F., Boj, M., Finn, R. N., and Cerdà, J. (2015). Mitochondrial aquaporin-8-mediated hydrogen peroxide transport is essential for teleost spermatozoon motility. Sci. Rep. 5:7789. doi: 10.1038/srep07789
Chen, R., Shi, Y., Amiduo, R., Tuokan, T., and Suzuk, L. (2014). Expression and prognostic value of aquaporin 1, 3 in cervical carcinoma in women of Uygur ethnicity from Xinjiang, China. PLoS One 9:e98576. doi: 10.1371/journal.pone.0098576
Cui, W. Y., Tian, A. Y., and Bai, T. (2011). Protective effects of propofol on endotoxemia-induced acute kidney injury in rats. Clin. Exp. Pharmacol. Physiol. 38, 747–754. doi: 10.1111/j.1440-1681.2011.05584.x
da Silva, I. V., and Soveral, G. (2017). Aquaporins in obesity. Adv. Exp. Med. Biol. 969, 227–238. doi: 10.1007/978-94-024-1057-0_15
Davies, D. C. (2002). Blood-brain barrier breakdown in septic encephalopathy and brain tumours. J. Anat. 200, 639–646. doi: 10.1046/j.1469-7580.2002.00065.x
Day, R. E., Kitchen, P., Owen, D. S., Bland, C., Marshall, L., Conner, A. C., et al. (2014). Human aquaporins: regulators of transcellular water flow. Biochim. Biophys. Acta 1840, 1492–1506. doi: 10.1016/j.bbagen.2013.09.033
Dean, P., Maresca, M., Schüller, S., Phillips, A. D., and Kenny, B. (2006). Potent diarrheagenic mechanism mediated by the cooperative action of three enteropathogenic Escherichia coli-injected effector proteins. Proc. Natl. Acad. Sci. U.S.A. 103, 1876–1881. doi: 10.1073/pnas.0509451103
Deen, P. M., Verdijk, M. A., Knoers, N. V., Wieringa, B., Monnens, L. A., Van Os, C. H., et al. (1994). Requirement of human renal water channel aquaporin-2 for vasopressin-dependent concentration of urine. Science 264, 92–95. doi: 10.1126/science.8140421
Delporte, C., Virreira, M., Crutzen, R., Louchami, K., Sener, A., Malaisse, W. J., et al. (2009). Functional role of aquaglyceroporin 7 expression in the pancreatic beta-cell line BRIN-BD11. J. Cell Physiol. 221, 424–429. doi: 10.1002/jcp.21872
Devuyst, O. (1998). The expression of water channels AQP1 and AQP2 in a large series of ADPKD kidneys. Nephron 78, 116–117. doi: 10.1159/000044893
Direito, I., Madeira, A., Brito, M. A., and Soveral, G. (2016). Aquaporin-5: from structure to function and dysfunction in cancer. Cell Mol. Life Sci. 73, 1623–1640. doi: 10.1007/s00018-016-2142-0
Donnenberg, M. S., Tacket, C. O., James, S. P., Losonsky, G., Nataro, J. P., Wasserman, S. S., et al. (1993). Role of the eaeA gene in experimental enteropathogenic Escherichia coli infection. J. Clin. Investig. 92, 1412–1417. doi: 10.1172/JCI116717
Du, Y., Meng, Y., Lv, X., Guo, L., Wang, X., Su, Z., et al. (2014). Dexamethasone attenuates LPS-induced changes in expression of urea transporter and aquaporin proteins, ameliorating brain endotoxemia in mice. Int. J. clin. 7, 8443–8452.
Eguchi, H., Takeshige, H., Nakajima, S., Kanou, M., Nakajima, A., Fuse, A., et al. (2020). Herpes zoster radiculomyelitis with aquaporin-4 antibodies: a case report and literature review. Front. Neurol. 11:585303. doi: 10.3389/fneur.2020.585303
El Hindy, N., Bankfalvi, A., Herring, A., Adamzik, M., Lambertz, N., Zhu, Y., et al. (2013). Correlation of aquaporin-1 water channel protein expression with tumor angiogenesis in human astrocytoma. Anticancer Res. 33, 609–613.
Escudero-Hernández, C., Münch, A., Østvik, A. E., Granlund, A. V. B., and Koch, S. (2020). The water channel aquaporin 8 is a critical regulator of intestinal fluid homeostasis in collagenous colitis. J. Crohns. Colitis 14, 962–973. doi: 10.1093/ecco-jcc/jjaa020
Esmat, S., Zeid, O. A., Abdel Halim, D. M., Fawzy, M. T., Abdel Latif, M., Lazarova, V., et al. (2018). Tissue expression of aquaporin 3 in different sites of vitiligo: an immunohistochemical study. J. Eur. Acad. Dermatol. Venereol. 32, e455–e456. doi: 10.1111/jdv.15049
Esteva-Font, C., Jin, B.-J., Lee, S., Phuan, P.-W., Anderson, M. O., and Verkman, A. S. (2016). Experimental evaluation of proposed small-molecule inhibitors of water channel aquaporin-1. Mol. Pharmacol. 89, 686–693. doi: 10.1124/mol.116.103929
Fadiel, A., Isokpehi, R. D., Stambouli, N., Hamza, A., Benammar-Elgaaied, A., and Scalise, T. J. (2009). Protozoan parasite aquaporins. Expert Rev. Proteomic 6, 199–211. doi: 10.1586/epr.09.10
Faghiri, Z., Camargo, S. M. R., Huggel, K., Forster, I. C., Ndegwa, D., Verrey, F., et al. (2010). The tegument of the human parasitic worm Schistosoma mansoni as an excretory organ: the surface aquaporin SmAQP is a lactate transporter. PLoS one 5:e10451. doi: 10.1371/journal.pone.0010451
Faghiri, Z., and Skelly, P. J. (2009). The role of tegumental aquaporin from the human parasitic worm, Schistosoma mansoni, in osmoregulation and drug uptake. FASEB J. 23, 2780–2789. doi: 10.1096/fj.09-130757
Figarella, K., Uzcategui, N. L., Zhou, Y., Lefurgey, A., Ouellette, M., Bhattacharjee, H., et al. (2007). Biochemical characterization of Leishmania major aquaglyceroporin LmAQP1: possible role in volume regulation and osmotaxis. Mol. Microbiol. 65, 1006–1017. doi: 10.1111/j.1365-2958.2007.05845.x
Filippidis, A. S., Carozza, R. B., and Rekate, H. L. (2016). Aquaporins in brain edema and neuropathological conditions. Int. J. Mol. Sci. 18:55. doi: 10.3390/ijms18010055
Finn, R. N., and Cerdà, J. (2015). Evolution and functional diversity of aquaporins. Biol. Bull. 229, 6–23. doi: 10.1086/BBLv229n1p6
Finn, R. N., Chauvigné, F., Hlidberg, J. B., Cutler, C. P., and Cerdá, J. (2014), The lineage-specific evolution of aquaporin gene clusters facilitated tetrapod terrestrial adaptation. PLoS One 9:e113686. doi: 10.1371/journal.pone.0113686
Fisher, B. J., Kraskauskas, D., Martin, E. J., Farkas, D., Wegelin, J. A., Brophy, D., et al. (2012). Mechanisms of attenuation of abdominal sepsis induced acute lung injury by ascorbic acid. Am. J. Physiol. Lung Cell Mol. Physiol. 303, L20–L32. doi: 10.1152/ajplung.00300.2011
Flach, C. F., Qadri, F., Bhuiyan, T. R., Alam, N. H., Jennische, E., Holmgren, J., et al. (2007). Differential expression of intestinal membrane transporters in cholera patients. FEBS Lett. 581, 3183–3188. doi: 10.1016/j.febslet.2007.06.001
Flach, C. F., Lange, S., Jennische, E., and Lönnroth, I. (2004). Cholera toxin induces expression of ion channels and carriers in rat small intestinal mucosa. FEBS Lett. 561, 122–126. doi: 10.1016/S0014-5793(04)00139-5
Flegal, K. M., Carroll, M. D., Kit, B. K., and Ogden, C. L. (2012). Prevalence of obesity and trends in the distribution of body mass index among US adults, 1999-2010. JAMA 307, 491–497. doi: 10.1001/jama.2012.39
Frigeri, A., Nicchia, G. P., Balena, R., Nico, B., and Svelto, M. (2004). Aquaporins in skeletal muscle: reassessment of the functional role of aquaporin-4. FASEB J. 18, 905–907. doi: 10.1096/fj.03-0987fje
Fu, D., Libson, A., Miercke, L. J., Weitzman, C., Nollert, P., Krucinski, J., et al. (2000). Structure of a glycerol-conducting channel and the basis for its selectivity. Science 290, 481–486. doi: 10.1126/science.290.5491.481
Fushimi, K., Sasaki, S., and Marumo, F. (1997). Phosphorylation of serine 256 is required for cAMP-dependent regulatory exocytosis of the aquaporin-2 water channel. J. Biol. Chem. 272, 14800–14804. doi: 10.1074/jbc.272.23.14800
Gao, J., Wang, X., Chang, Y., Zhang, J., Song, Q., Yu, H., et al. (2006). Acetazolamide inhibits osmotic water permeability by interaction with aquaporin-1. Anal. Biochem. 350, 165–170. doi: 10.1016/j.ab.2006.01.003
Garneau, A. P., Carpentier, G. A., Marcoux, A.-A., Frenette-Cotton, R., Simard, C. F., Rémus-Borel, W., et al. (2015). Aquaporins mediate silicon transport in humans. PLoS One 10:e0136149. doi: 10.1371/journal.pone.0136149
Gazzarrini, S., Kang, M., Epimashko, S., Van Etten, J. L., Dainty, J., Thiel, G., et al. (2006). Chlorella virus MT325 encodes water and potassium channels that interact synergistically. Proc. Natl. Acad. Sci. U.S.A. 103, 5355–5360. doi: 10.1073/pnas.0600848103
Geyer, D. D., Spence, M. A., Johannes, M., Flodman, P., Clancy, K. P., Berry, R., et al. (2006). Novel single-base deletional mutation in major intrinsic protein (MIP) in autosomal dominant cataract. Am. J. Ophthalmol. 141, 761–763. doi: 10.1016/j.ajo.2005.11.008
Gomes, D., Agasse, A., Thiébaud, P., Delrot, S., Gerós, H., and Chaumont, F. (2009). Aquaporins are multifunctional water and solute transporters highly divergent in living organisms. Biochim. Biophys. Acta 1788, 1213–1228. doi: 10.1016/j.bbamem.2009.03.009
Gonen, T., and Walz, T. (2006). The structure of aquaporins. Q. Rev. Biophys. 39, 361–396. doi: 10.1017/S0033583506004458
Gourbal, B., Sonuc, N., Bhattacharjee, H., Legare, D., Sundar, S., Ouellette, M., et al. (2004). Drug uptake and modulation of drug resistance in Leishmania by an aquaglyceroporin. J. Biol. Chem. 279, 31010–31017. doi: 10.1074/jbc.M403959200
Grinevich, V., Knepper, M. A., Verbalis, J., Reyes, I., and Aguilera, G. (2004). Acute endotoxemia in rats induces down-regulation of V2 vasopressin receptors and aquaporin-2 content in the kidney medulla. Kidney Int. 65, 54–62. doi: 10.1111/j.1523-1755.2004.00378.x
Guo, X., Sun, T., Yang, M., Li, Z., Li, Z., and Gao, Y. (2013). Prognostic value of combined aquaporin 3 and aquaporin 5 overexpression in hepatocellular carcinoma. Biomed. Res. Int. 2013:206525. doi: 10.1155/2013/206525
Guttman, J. A., Li, Y., Wickham, M. E., Deng, W., Vogl, A. W., and Finlay, B. B. (2006). Attaching and effacing pathogen-induced tight junction disruption in vivo. Cell Microbiol. 8, 634–645. doi: 10.1111/j.1462-5822.2005.00656.x
Guttman, J. A., Samji, F. N., Li, Y., Deng, W., Lin, A., and Finlay, B. B. (2007). Aquaporins contribute to diarrhoea caused by attaching and effacing bacterial pathogens. Cell Microbiol. 9, 131–141. doi: 10.1111/j.1462-5822.2006.00773.x
Halsey, A. M., Conner, A. C., Bill, R. M., Logan, A., and Ahmed, Z. (2018). Aquaporins and their regulation after spinal cord injury. Cells 7:174. doi: 10.3390/cells7100174
Han, B., Wu, X., Huang, P. P., Zhu, F. X., and Liu, S. (2019). Aquaporin 11 rs2276415 variant and progression of chronic kidney disease. Nephrol. Dial. Transplant. 34, 970–973. doi: 10.1093/ndt/gfy219
Hara-Chikuma, M., Chikuma, S., Sugiyama, Y., Kabashima, K., Verkman, A. S., Inoue, S., et al. (2012a). Chemokine-dependent T cell migration requires aquaporin-3-mediated hydrogen peroxide uptake. J. Exp. Med. 209, 1743–1752. doi: 10.1084/jem.20112398
Hara-Chikuma, M., Satooka, H., Watanabe, S., Honda, T., Miyachi, Y., Watanabe, T., et al. (2015). Aquaporin-3-mediated hydrogen peroxide transport is required for NF-κB signalling in keratinocytes and development of psoriasis. Nat. Commun. 6:7454. doi: 10.1038/ncomms8454
Hara-Chikuma, M., Sohara, E., Rai, T., Ikawa, M., Okabe, M., Sasaki, S., et al. (2005). Progressive adipocyte hypertrophy in aquaporin-7-deficient mice: adipocyte glycerol permeability as a novel regulator of fat accumulation. J. Biol. Chem. 280, 15493–15496. doi: 10.1074/jbc.C500028200
Hara-Chikuma, M., Sugiyama, Y., Kabashima, K., Sohara, E., Uchida, S., Sasaki, S., et al. (2012b). Involvement of aquaporin-7 in the cutaneous primary immune response through modulation of antigen uptake and migration in dendritic cells. FASEB J. 26, 211–218. doi: 10.1096/fj.11-186627
Hara-Chikuma, M., Tanaka, M., Verkman, A. S., and Yasui, M. (2020). Inhibition of aquaporin-3 in macrophages by a monoclonal antibody as potential therapy for liver injury. Nat. Commun. 11:5666. doi: 10.1038/s41467-020-19491-5
Hara-Chikuma, M., and Verkman, A. S. (2006). Aquaporin-1 facilitates epithelial cell migration in kidney proximal tubule. J. Am. Soc. Nephrol. 17, 39–45. doi: 10.1681/ASN.2005080846
Hara-Chikuma, M., and Verkman, A. S. (2008). Prevention of skin tumorigenesis and impairment of epidermal cell proliferation by targeted aquaporin-3 gene disruption. Mol. Cell Biol. 28, 326–332. doi: 10.1128/MCB.01482-07
Hardin, J. A., Wallace, L. E., Wong, J. F., O’loughlin, E. V., Urbanski, S. J., Gall, D. G., et al. (2004). Aquaporin expression is downregulated in a murine model of colitis and in patients with ulcerative colitis, Crohn’s disease and infectious colitis. Cell Tissue Res. 318, 313–323. doi: 10.1007/s00441-004-0932-4
Hazama, A., Kozono, D., Guggino, W. B., Agre, P., and Yasui, M. (2002). Ion permeation of AQP6 water channel protein. Single channel recordings after Hg2+ activation. J. Biol. Chem. 277, 29224–29230. doi: 10.1074/jbc.M204258200
He, J., and Yang, B. (2019). Aquaporins in renal diseases. Int. J. Mol. Sci. 20:366. doi: 10.3390/ijms20020366
Hedfalk, K., Törnroth-Horsefield, S., Nyblom, M., Johanson, U., Kjellbom, P., and Neutze, R. (2006). Aquaporin gating. Curr. Opin. Struct. Biol. 16, 447–456. doi: 10.1016/j.sbi.2006.06.009
Herrera, M., Hong, N. J., and Garvin, J. L. (2006). Aquaporin-1 transports NO across cell membranes. Hypertension 48, 157–164. doi: 10.1161/01.HYP.0000223652.29338.77
Heymann, J. B., and Engel, A. (2000). Structural clues in the sequences of the aquaporins. J. Mol. Biol. 295, 1039–1053. doi: 10.1006/jmbi.1999.3413
Hibuse, T., Maeda, N., Funahashi, T., Yamamoto, K., Nagasawa, A., Mizunoya, W., et al. (2005). Aquaporin 7 deficiency is associated with development of obesity through activation of adipose glycerol kinase. Proc. Natl. Acad Sci. U.S.A. 102, 10993–10998. doi: 10.1073/pnas.0503291102
Hoffert, J. D., Nielsen, J., Yu, M. J., Pisitkun, T., Schleicher, S. M., Nielsen, S., et al. (2007). Dynamics of aquaporin-2 serine-261 phosphorylation in response to short-term vasopressin treatment in collecting duct. Am. J. Physiol. Renal Physiol. 292, F691–F700. doi: 10.1152/ajprenal.00284.2006
Holm, A., Karlsson, T., and Vikström, E. (2015). Pseudomonas aeruginosa lasI/rhlI quorum sensing genes promote phagocytosis and aquaporin 9 redistribution to the leading and trailing regions in macrophages. Front. Microbiol. 6:915. doi: 10.3389/fmicb.2015.00915
Holm, A., Magnusson, K. E., and Vikström, E. (2016). Pseudomonas aeruginosa N-3-oxo-dodecanoyl-homoserine lactone elicits changes in cell volume, morphology, and AQP9 characteristics in macrophages. Front. Cell Infect. Microbiol. 6:32. doi: 10.3389/fcimb.2016.00032
Holmøy, T., Høglund, R. A., Illes, Z., Myhr, K.-M., and Torkildsen, Ø (2020). Recent progress in maintenance treatment of neuromyelitis optica spectrum disorder. J. Neurol. [Epub ahead of print] doi: 10.1007/s00415-020-10235-5
Hoque, M. O., Soria, J. C., Woo, J., Lee, T., Lee, J., Jang, S. J., et al. (2006). Aquaporin 1 is overexpressed in lung cancer and stimulates NIH-3T3 cell proliferation and anchorage-independent growth. Am. J. Pathol. 168, 1345–1353. doi: 10.2353/ajpath.2006.050596
Hou, Y., Wang, L., Yi, D., Ding, B., Yang, Z., Li, J., et al. (2013). N-acetylcysteine reduces inflammation in the small intestine by regulating redox, EGF and TLR4 signaling. Amino Acids 45, 513–522. doi: 10.1007/s00726-012-1295-x
Hu, J., and Verkman, A. S. (2006). Increased migration and metastatic potential of tumor cells expressing aquaporin water channels. FASEB J. 20, 1892–1894. doi: 10.1096/fj.06-5930fje
Hub, J. S., and de Groot, B. L. (2006). Does CO2 permeate through aquaporin-1? Biophys. J. 91, 842–848. doi: 10.1529/biophysj.106.081406
Hub, J. S., and de Groot, B. L. (2008). Mechanism of selectivity in aquaporins and aquaglyceroporins. Proc. Natl. Acad. Sci. U.S.A. 105, 1198–1203. doi: 10.1073/pnas.0707662104
Hunziker, W., and Fumey, C. (1994). A di-leucine motif mediates endocytosis and basolateral sorting of macrophage IgG Fc receptors in MDCK cells. EMBO J. 13, 2963–2969. doi: 10.1002/j.1460-2075.1994.tb06594.x
Iena, F. M., and Lebeck, J. (2018). Implications of aquaglyceroporin 7 in energy metabolism. Int. J. Mol. Sci. 19:154. doi: 10.3390/ijms19010154
Ikarashi, N., Kon, R., Iizasa, T., Suzuki, N., Hiruma, R., Suenaga, K., et al. (2012). Inhibition of aquaporin-3 water channel in the colon induces diarrhea. Biol. Pharm. Bull. 35, 957–962. doi: 10.1248/bpb.35.957
Ikarashi, N., Kon, R., Kaneko, M., Mizukami, N., Kusunoki, Y., and Sugiyama, K. (2017). Relationship between aging-related skin dryness and aquaporins. Int. J. Mol. Sci. 18:1559. doi: 10.3390/ijms18071559
Ikarashi, N., Ushiki, T., Mochizuki, T., Toda, T., Kudo, T., Baba, K., et al. (2011). Effects of magnesium sulphate administration on aquaporin 3 in rat gastrointestinal tract. Biol. Pharm. Bull. 34, 238–242. doi: 10.1248/bpb.34.238
Ishibashi, K., Yamauchi, K., Kageyama, Y., Saito-Ohara, F., Ikeuchi, T., Marumo, F., et al. (1998). Molecular characterization of human Aquaporin-7 gene and its chromosomal mapping. Biochim. Biophys. Acta 1399, 62–66. doi: 10.1016/S0167-4781(98)00094-3
Ishimoto, S., Wada, K., Usami, Y., Tanaka, N., Aikawa, T., Okura, M., et al. (2012). Differential expression of aquaporin 5 and aquaporin 3 in squamous cell carcinoma and adenoid cystic carcinoma. Int. J. Oncol. 41, 67–75. doi: 10.3892/ijo.2012.1445
Jablonski, E. M., Mattocks, M. A., Sokolov, E., Koniaris, L. G., Hughes, F. M. Jr., Fausto, N., et al. (2007). Decreased aquaporin expression leads to increased resistance to apoptosis in hepatocellular carcinoma. Cancer Lett. 250, 36–46. doi: 10.1016/j.canlet.2006.09.013
Jang, S., Jones, K. R., Olsen, C. H., Joo, Y. M., Yoo, Y.-J., Chung, I.-S., et al. (2010). Epidemiological link between gastric disease and polymorphisms in VacA and CagA. J. Clin. Microbiol. 48, 559–567. doi: 10.1128/JCM.01501-09
Janosi, L., and Ceccarelli, M. (2013). The gating mechanism of the human aquaporin 5 revealed by molecular dynamics simulations. PLoS One 8:e59897. doi: 10.1371/journal.pone.0059897
Jelen, S., Parm Ulhøi, B., Larsen, A., Frøkiær, J., Nielsen, S., and Rützler, M. (2013). AQP9 expression in glioblastoma multiforme tumors is limited to a small population of astrocytic cells and CD15(+)/CalB(+) leukocytes. PLoS One 8:e75764. doi: 10.1371/journal.pone.0075764
Jennische, E., Eriksson, C. E., Lange, S., Trybala, E., and Bergström, T. (2015). The anterior commissure is a pathway for contralateral spread of herpes simplex virus type 1 after olfactory tract infection. J. Neurovirol. 21, 129–147. doi: 10.1007/s13365-014-0312-0
Jung, J. S., Bhat, R. V., Preston, G. M., Guggino, W. B., Baraban, J. M., and Agre, P. (1994). Molecular characterization of an aquaporin cDNA from brain: candidate osmoreceptor and regulator of water balance. Proc. Natl. Acad. Sci. U.S.A. 91, 13052–13056. doi: 10.1073/pnas.91.26.13052
Jung, S. Y., Kim, S. S., Kim, Y. I., Kim, S. H., and Yeo, S. G. (2017). A review: expression of aquaporins in otitis media. Int. J. Mol. Sci. 18:2164. doi: 10.3390/ijms18102164
Kamsteeg, E. J., Hendriks, G., Boone, M., Konings, I. B., Oorschot, V., Van Der Sluijs, P., et al. (2006). Short-chain ubiquitination mediates the regulated endocytosis of the aquaporin-2 water channel. Proc. Natl. Acad. Sci. U.S.A. 103, 18344–18349. doi: 10.1073/pnas.0604073103
Kang, S., Chae, Y. S., Lee, S. J., Kang, B. W., Kim, J. G., et al. (2015). Aquaporin 3 expression predicts survival in patients with HER2-positive early breast cancer. Anticancer Res. 35, 2775–2782.
Kang, S. K., Chae, Y. K., Woo, J., Kim, M. S., Park, J. C., Lee, J., et al. (2008). Role of human aquaporin 5 in colorectal carcinogenesis. Am. J. Pathol. 173, 518–525. doi: 10.2353/ajpath.2008.071198
Kang, X., Lu, X.-G., Zhan, L.-B., Liang, Z.-K., Guo, W.-X., Ma, Q., et al. (2017). Dai-Huang-Fu-Zi-Tang alleviates pulmonary and intestinal injury with severe acute pancreatitis via regulating aquaporins in rats. BMC Compl. Altern. Med. 17:288. doi: 10.1186/s12906-017-1789-x
Kannan, R., Stolz, A., Ji, Q., Prasad, P. D., and Ganapathy, V. (2001). Vitamin C transport in human lens epithelial cells: evidence for the presence of SVCT2. Exp. Eye Res. 73, 159–165. doi: 10.1006/exer.2001.1024
Kaptan, S., Assentoft, M., Schneider, H. P., Fenton, R. A., Deitmer, J. W., and Macaulay, N. (2015). H95 Is a pH-Dependent gate in Aquaporin 4. Structure 23, 2309–2318. doi: 10.1016/j.str.2015.08.020
Karlsson, T., Bolshakova, A., Magalhães, M. A. O., Loitto, V. M., and Magnusson, K.-E. (2013a). Fluxes of water through aquaporin 9 weaken membrane-cytoskeleton anchorage and promote formation of membrane protrusions. PLoS One 8:e59901. doi: 10.1371/journal.pone.0059901
Karlsson, T., Lagerholm, B. C., Vikström, E., Loitto, V. M., and Magnusson, K. E. (2013b). Water fluxes through aquaporin-9 prime epithelial cells for rapid wound healing. Biochem. Biophys. Res. Commun. 430, 993–998. doi: 10.1016/j.bbrc.2012.11.125
Keel, M. J. B. (2014). “Systemic infections and sepsis,” in General Trauma Care and Related Aspects. European Manual of Medicine, eds H. J. Oestern, O. Trentz, and S. Uranues, (Berlin: Springer).
Khajah, M., and Luqmani, Y. (2016). Role of Aquaporins in Breast Cancer Progression and Metastasis. Tumor Metastasis, InTechOpen, Chapter 4, 59–83. doi: 10.5772/64446
Kim, N. H., and Lee, A. Y. (2010). Reduced aquaporin3 expression and survival of keratinocytes in the depigmented epidermis of vitiligo. J. Invest. Dermatol. 130, 2231–2239. doi: 10.1038/jid.2010.99
Kishida, K., Kuriyama, H., Funahashi, T., Shimomura, I., Kihara, S., Ouchi, N., et al. (2000). Aquaporin adipose, a putative glycerol channel in adipocytes. J. Biol. Chem. 275, 20896–20902. doi: 10.1074/jbc.M001119200
Kitchen, P., Salman, M. M., Halsey, A. M., Clarke-Bland, C., Macdonald, J. A., Ishida, H., et al. (2020). Targeting Aquaporin-4 subcellular localization to treat central nervous system edema. Cell 181, 784.e19–799.e19. doi: 10.1016/j.cell.2020.03.037
Kitchen, P., Salman, M. M., Pickel, S. U., Jennings, J., Törnroth-Horsefield, S., Conner, M. T., et al. (2019). Water channel pore size determines exclusion properties but not solute selectivity. Sci. Rep. 9:20369. doi: 10.1038/s41598-019-56814-z
Konturek, P. C., Konturek, S. J., and Brzozowski, T. (2009). Helicobacter pylori infection in gastric cancerogenesis. J. Physiol. Pharmacol. 60, 3–21.
Korany, M. M., Amer, M. A., Rashed, L. A., and Saleh, M. A. (2019). Downregulation of aquaporin 3 in bullous pemphigoid patients. Arch. Dermatol. Res. 311, 93–97. doi: 10.1007/s00403-018-1884-y
Kordowitzki, P., Kranc, W., Bryl, R., Kempisty, B., Skowronska, A., and Skowronski, M. T. (2020). The relevance of aquaporins for the physiology, pathology, and aging of the female reproductive system in mammals. Cells 9:2570. doi: 10.3390/cells9122570
Kotnik, P., Battelino, T., Debeljak, M., Podkrajsek, K. T., Waldhauser, F., Frøkiaer, J., et al. (2007). Correlation between AVPR2 mutations and urinary AQP2 excretion in patients with nephrogenic diabetes insipidus. J. Pediatr. Endocrinol. Metab. 20, 483–489. doi: 10.1515/JPEM.2007.20.4.483
Krøigård, A. B., Hetland, L. E., Clemmensen, O., Blaydon, D. C., Hertz, J. M., and Bygum, A. (2016). The first Danish family reported with an AQP5 mutation presenting diffuse non-epidermolytic palmoplantar keratoderma of Bothnian type, hyperhidrosis and frequent Corynebacterium infections: a case report. BMC Dermatol. 16:7. doi: 10.1186/s12895-016-0044-3
Kuriyama, H., Kawamoto, S., Ishida, N., Ohno, I., Mita, S., Matsuzawa, Y., et al. (1997). Molecular cloning and expression of a novel human aquaporin from adipose tissue with glycerol permeability. Biochem. Biophys. Res. Commun. 241, 53–58. doi: 10.1006/bbrc.1997.7769
Laforenza, U., Pellavio, G., Marchetti, A. L., Omes, C., Todaro, F., and Gastaldi, G. (2016). Aquaporin-mediated water and hydrogen peroxide transport is involved in normal human spermatozoa functioning. Int. J. Mol. Sci. 18:66. doi: 10.3390/ijms18010066
Laloux, T., Junqueira, B., Maistriaux, L. C., Ahmed, J., Jurkiewicz, A., and Chaumont, F. (2018). Plant and mammal aquaporins: same but different. Int. J. Mol. Sci. 19:521. doi: 10.3390/ijms19020521
Lana-Peixoto, M. A., Pedrosa, D., Talim, N., Amaral, J., Horta, A., and Kleinpaul, R. (2018). Neuromyelitis optica spectrum disorder associated with dengue virus infection. J. Neuroimmunol. 318, 53–55. doi: 10.1016/j.jneuroim.2018.02.003
Lee, D. H., Park, D. B., Lee, Y. K., An, C. S., Oh, Y. S., Kang, J. S., et al. (2005). The effects of thiazolidinedione treatment on the regulations of aquaglyceroporins and glycerol kinase in OLETF rats. Metabolism 54, 1282–1289. doi: 10.1016/j.metabol.2005.04.015
Lee, Y., Je, Y. J., Lee, S. S., Li, Z. J., Choi, D. K., Kwon, Y. B., et al. (2012). Changes in transepidermal water loss and skin hydration according to expression of aquaporin-3 in psoriasis. Ann. Dermatol. 24, 168–174. doi: 10.5021/ad.2012.24.2.168
Lehmann, G. L., Carreras, F. I., Soria, L. R., Gradilone, S. A., and Marinelli, R. A. (2008). LPS induces the TNF-alpha-mediated downregulation of rat liver aquaporin-8: role in sepsis-associated cholestasis. Am. J. Physiol. Gastrointest. Liver Physiol. 294, G567–G575. doi: 10.1152/ajpgi.00232.2007
Leitch, V., Agre, P., and King, L. S. (2001). Altered ubiquitination and stability of aquaporin-1 in hypertonic stress. Proc. Natl. Acad Sci. U.S.A. 98, 2894–2898. doi: 10.1073/pnas.041616498
Levy, M. (2017). Highly Soluble Aquaporin-4 Extracellular Loop Peptide Immunization for the Treatment of Optic Neuritis. U.S. Patent US20170080063A. Baltimore, MD: The Johns Hopkins University
Li, C., and Wang, W. (2017). Molecular biology of aquaporins. Adv. Exp. Med. Biol. 969, 1–34. doi: 10.1007/978-94-024-1057-0_1
Li, F.-X., Huang, L.-Z., Dong, C., Wang, J.-P., Wu, H.-J., and Shuang, S.-M. (2015). Down-regulation of aquaporin3 expression by lipopolysaccharide via p38/c-Jun N-terminal kinase signalling pathway in HT-29 human colon epithelial cells. World J. Gastroenterol. 21, 4547–4554. doi: 10.3748/wjg.v21.i15.4547
Li, G., Chen, T., Zhang, Z., Li, B., and Tian, S. (2020). Roles of aquaporins in plant-pathogen interaction. Plants 9:1134. doi: 10.3390/plants9091134
Li, Y., Wang, W., Jiang, T., and Yang, B. (2017). Aquaporins in urinary system. Adv. Exp. Med. Biol. 969, 131–148. doi: 10.1007/978-94-024-1057-0_9
Liang, D., Leung, R. K.-K., Guan, W., and Au, W. W. (2018). Involvement of gut microbiome in human health and disease: brief overview, knowledge gaps and research opportunities. Gut Pathog. 10:3. doi: 10.1186/s13099-018-0230-4
Liao, S., Gan, L., Lv, L., and Mei, Z. (2020). The regulatory roles of aquaporins in the digestive system. Genes Dis. (in press). doi: 10.1016/j.gendis.2019.12.011
Litman, T., Søgaard, R., and Zeuthen, T. (2009). Ammonia and urea permeability of mammalian aquaporins. Handb. Exp. Pharmacol. 190, 327–358. doi: 10.1007/978-3-540-79885-9_17
Liu, C., Zheng, Y., Xu, W., Wang, H., and Lin, N. (2014). Rhubarb tannins extract inhibits the expression of aquaporins 2 and 3 in magnesium sulphate-induced diarrhoea model. Biomed. Res. Int. 2014:619465. doi: 10.1155/2014/619465
Liu, S., Zhang, S., Jiang, H., Yang, Y., and Jiang, Y. (2013). Co-expression of AQP3 and AQP5 in esophageal squamous cell carcinoma correlates with aggressive tumor progression and poor prognosis. Med. Oncol. 30:636. doi: 10.1007/s12032-013-0636-2
Liu, Y., Promeneur, D., Rojek, A., Kumar, N., Frøkiaer, J., Nielsen, S., et al. (2007). Aquaporin 9 is the major pathway for glycerol uptake by mouse erythrocytes, with implications for malarial virulence. Proc. Natl. Acad. Sci. U.S.A. 104, 12560–12564. doi: 10.1073/pnas.0705313104
Liu, Z., Shen, J., Carbrey, J. M., Mukhopadhyay, R., Agre, P., and Rosen, B. P. (2002). Arsenite transport by mammalian aquaglyceroporins AQP7 and AQP9. Proc. Natl. Acad. Sci. U.S.A. 99, 6053–6058. doi: 10.1073/pnas.092131899
Loos, M., Geens, M., Schauvliege, S., Gasthuys, F., Van Der Meulen, J., Dubreuil, J. D., et al. (2012). Role of heat-stable enterotoxins in the induction of early immune responses in piglets after infection with enterotoxigenic Escherichia coli. PLoS One 7:e41041. doi: 10.1371/journal.pone.0041041
Lu, H. J., Matsuzaki, T., Bouley, R., Hasler, U., Qin, Q. H., and Brown, D. (2008). The phosphorylation state of serine 256 is dominant over that of serine 261 in the regulation of AQP2 trafficking in renal epithelial cells. Am. J. Physiol. Renal Physiol. 295, F290–F294. doi: 10.1152/ajprenal.00072.2008
Lu, Y., Chen, L., Zhao, B., Xiao, Z., Meng, T., Zhou, Q., et al. (2016). Urine AQP5 is a potential novel biomarker of diabetic nephropathy. J. Diabetes Complic. 30, 819–825. doi: 10.1016/j.jdiacomp.2016.03.026
Luo, J., Liu, X., Liu, J., Jiang, M., Luo, M., and Zhao, J. (2016). Activation of TGF-β1 by AQP3-Mediated H(2)O(2) transport into fibroblasts of a bleomycin-induced mouse model of scleroderma. J. Invest. Dermatol. 136, 2372–2379. doi: 10.1016/j.jid.2016.07.014
Ma, G., Jiang, A., Luo, Y., Luo, Y., Huang, H., and Zhou, R. (2019). Aquaporin 1 is located on the intestinal basolateral membrane in Toxocara canis and might play a role in drug uptake. Parasit. Vectors 12:243. doi: 10.1186/s13071-019-3500-1
Ma, T., and Liu, Z. (2013). Functions of aquaporin 1 and α-epithelial Na+ channel in rat acute lung injury induced by acute ischemic kidney injury. Int. Urol. Nephrol. 45, 1187–1196. doi: 10.1007/s11255-012-0355-1
Ma, T., and Verkman, A. S. (1999). Aquaporin water channels in gastrointestinal physiology. J. Physiol. 517(Pt 2), 317–326. doi: 10.1111/j.1469-7793.1999.0317t.x
Machado, A. M. D., Figueiredo, C., Seruca, R., and Rasmussen, L. J. (2010). Helicobacter pylori infection generates genetic instability in gastric cells. Biochim. Biophys. Acta. Rev. Cancer 1806, 58–65. doi: 10.1016/j.bbcan.2010.01.007
Machida, Y., Ueda, Y., Shimasaki, M., Sato, K., Sagawa, M., Katsuda, S., et al. (2011). Relationship of aquaporin 1, 3, and 5 expression in lung cancer cells to cellular differentiation, invasive growth, and metastasis potential. Hum. Pathol. 42, 669–678. doi: 10.1016/j.humpath.2010.07.022
Madeira, A., De Almeida, A., De Graaf, C., Camps, M., Zorzano, A., Moura, T. F., et al. (2014). A gold coordination compound as a chemical probe to unravel aquaporin-7 function. Chembiochem 15, 1487–1494. doi: 10.1002/cbic.201402103
Madeira, A., Moura, T. F., and Soveral, G. (2015). Aquaglyceroporins: implications in adipose biology and obesity. Cell. Mol. Life Sci. 72, 759–771. doi: 10.1007/s00018-014-1773-2
Madonna, R., Jiang, J., and Geng, Y. J. (2012). Attenuated expression of gelsolin in association with induction of aquaporin-1 and nitric oxide synthase in dysfunctional hearts of aging mice exposed to endotoxin. Int. J. Immunopathol. Pharmacol. 25, 911–922. doi: 10.1177/039463201202500409
Manley, G. T., Fujimura, M., Ma, T., Noshita, N., Filiz, F., Bollen, A. W., et al. (2000). Aquaporin-4 deletion in mice reduces brain edema after acute water intoxication and ischemic stroke. Nat. Med. 6, 159–163. doi: 10.1038/72256
Marchini, G., Ståbi, B., Kankes, K., Lonne-Rahm, S., Østergaard, M., and Nielsen, S. (2003). AQP1 and AQP3, psoriasin, and nitric oxide synthases 1-3 are inflammatory mediators in erythema toxicum neonatorum. Pediatr. Dermatol. 20, 377–384. doi: 10.1046/j.1525-1470.2003.20501.x
Marin Collazo, I. V., Howe, C. L., Lucchinetti, C. F., and Tobin, W. O. (2018). Neuromyelitis optica and herpes simplex virus 2: a viral trigger for aquaporin-4 autoimmunity? Neurologist 23, 92–93. doi: 10.1097/NRL.0000000000000172
Marinelli, R. A., Lehmann, G. L., Soria, L. R., and Marchissio, M. J. (2011). Hepatocyte aquaporins in bile formation and cholestasis. Front. Biosci. 16:2642–2652. doi: 10.2741/3877
Marlar, S., Jensen, H. H., Login, F. H., and Nejsum, L. N. (2017). Aquaporin-3 in cancer. Int. J. Mol. Sci. 18:2106. doi: 10.3390/ijms18102106
Marples, D., Knepper, M. A., Christensen, E. I., and Nielsen, S. (1995). Redistribution of aquaporin-2 water channels induced by vasopressin in rat kidney inner medullary collecting duct. Am. J. Physiol. 269, C655–C664. doi: 10.1152/ajpcell.1995.269.3.C655
Martinez Torres, F. J., Völcker, D., Dörner, N., Lenhard, T., Nielsen, S., Haas, J., et al. (2007). Aquaporin 4 regulation during acute and long-term experimental Herpes simplex virus encephalitis. J. Neurovirol. 13, 38–46. doi: 10.1080/13550280601145340
Martins, A. P., Marrone, A., Ciancetta, A., Galán Cobo, A., Echevarría, M., Moura, T. F., et al. (2012). Targeting aquaporin function: potent inhibition of aquaglyceroporin-3 by a gold-based compound. PLoS One 7:e37435. doi: 10.1371/journal.pone.0037435
Matsumura, K., Chang, B. H., Fujimiya, M., Chen, W., Kulkarni, R. N., Eguchi, Y., et al. (2007). Aquaporin 7 is a beta-cell protein and regulator of intraislet glycerol content and glycerol kinase activity, beta-cell mass, and insulin production and secretion. Mol. Cell Biol. 27, 6026–6037. doi: 10.1128/MCB.00384-07
Matsushima, A., Ogura, H., Koh, T., Shimazu, T., and Sugimoto, H. (2014). Enhanced expression of aquaporin 9 in activated polymorphonuclear leukocytes in patients with systemic inflammatory response syndrome. Shock 42, 322–326. doi: 10.1097/SHK.0000000000000218
Matsuzaki, T., Yaguchi, T., Shimizu, K., Kita, A., Ishibashi, K., and Takata, K. (2017). The distribution and function of aquaporins in the kidney: resolved and unresolved questions. Anat. Sci. Int. 92, 187–199. doi: 10.1007/s12565-016-0325-2
Matter, K., Hunziker, W., and Mellman, I. (1992). Basolateral sorting of LDL receptor in MDCK cells: the cytoplasmic domain contains two tyrosine-dependent targeting determinants. Cell 71, 741–753. doi: 10.1016/0092-8674(92)90551-M
Mazal, P. R., Susani, M., Wrba, F., and Haitel, A. (2005). Diagnostic significance of aquaporin-1 in liver tumors. Hum. Pathol. 36, 1226–1231. doi: 10.1016/j.humpath.2005.09.002
Medraño-Fernandez, I., Bestetti, S., Bertolotti, M., Bienert, G. P., Bottino, C., Laforenza, U., et al. (2016). Stress regulates aquaporin-8 permeability to impact cell growth and survival. Antioxid. Redox Signal. 24, 1031–1044. doi: 10.1089/ars.2016.6636
Medzhitov, R. (2008). Origin and physiological roles of inflammation. Nature 454, 428–435. doi: 10.1038/nature07201
Meier, A., Erler, H., and Beitz, E. (2018). Targeting channels and transporters in protozoan parasite infections. Front. Chem. 6:88. doi: 10.3389/fchem.2018.00088
Meli, R., Pirozzi, C., and Pelagalli, A. (2018). New perspectives on the potential role of aquaporins (AQPs) in the physiology of inflammation. Front. Physiol. 9:101. doi: 10.3389/fphys.2018.00101
Méndez-Giménez, L., Ezquerro, S., Da Silva, I. V., Soveral, G., Frühbeck, G., and Rodríguez, A. (2018). Pancreatic Aquaporin-7: a novel target for anti-diabetic drugs? Front. Chem. 6:99. doi: 10.3389/fchem.2018.00099
Miller, E. W., Dickinson, B. C., and Chang, C. J. (2010). Aquaporin-3 mediates hydrogen peroxide uptake to regulate downstream intracellular signaling. Proc. Natl. Acad. Sci. U.S.A. 107, 15681–15686. doi: 10.1073/pnas.1005776107
Miranda, M., Escoté, X., Ceperuelo-Mallafré, V., Alcaide, M. J., Simón, I., Vilarrasa, N., et al. (2010). Paired subcutaneous and visceral adipose tissue aquaporin-7 expression in human obesity and type 2 diabetes: differences and similarities between depots. J. Clin. Endocrinol. Metab. 95, 3470–3479. doi: 10.1210/jc.2009-2655
Mobasheri, A., Trujillo, E., Bell, S., Carter, S. D., Clegg, P. D., MartıìN-Vasallo, P., et al. (2004). Aquaporin water channels AQP1 and AQP3, are expressed in equine articular chondrocytes. Vet. J. 168, 143–150. doi: 10.1016/j.tvjl.2003.08.001
Molinas, A., Mirazimi, A., Holm, A., Loitto, V. M., Magnusson, K. E., and Vikström, E. (2016). Protective role of host aquaporin 6 against Hazara virus, a model for Crimean-Congo hemorrhagic fever virus infection. FEMS Microbiol. Lett. 363:fnw058. doi: 10.1093/femsle/fnw058
Molinas, S. M., Trumper, L., and Marinelli, R. A. (2012). Mitochondrial aquaporin-8 in renal proximal tubule cells: evidence for a role in the response to metabolic acidosis. Am. J. Physiol. Renal Physiol. 303, F458–F466. doi: 10.1152/ajprenal.00226.2012
Montiel, V., Bella, R., Michel, L. Y. M., Esfahani, H., De Mulder, D., Robinson, E. L., et al. (2020). Inhibition of aquaporin-1 prevents myocardial remodeling by blocking the transmembrane transport of hydrogen peroxide. Sci. Transl. Med. 12:eaay2176. doi: 10.1126/scitranslmed.aay2176
Montiel, V., Leon Gomez, E., Bouzin, C., Esfahani, H., Romero Perez, M., Lobysheva, I., et al. (2014). Genetic deletion of aquaporin-1 results in microcardia and low blood pressure in mouse with intact nitric oxide-dependent relaxation, but enhanced prostanoids-dependent relaxation. Pflugers Arch. 466, 237–251. doi: 10.1007/s00424-013-1325-x
Moon, C., Soria, J.-C., Jang, S. J., Lee, J., Hoque, M. O., Sibony, M., et al. (2003). Involvement of aquaporins in colorectal carcinogenesis. Oncogene 22, 6699–6703. doi: 10.1038/sj.onc.1206762
Mukhopadhyay, R., and Beitz, E. (2010). Metalloid transport by aquaglyceroporins: consequences in the treatment of human diseases. Adv. Exp. Med. Biol. 679, 57–69. doi: 10.1007/978-1-4419-6315-4_5
Mukhopadhyay, R., Bhattacharjee, H., and Rosen, B. P. (2014). Aquaglyceroporins: generalized metalloid channels. Biochim. Biophys. Acta 1840, 1583–1591. doi: 10.1016/j.bbagen.2013.11.021
Mukhopadhyay, R., Mandal, G., Atluri, V. S., Figarella, K., Uzcategui, N. L., Zhou, Y., et al. (2011). The role of alanine 163 in solute permeability of Leishmania major aquaglyceroporin LmAQP1. Mol. Biochem. Parasitol. 175, 83–90. doi: 10.1016/j.molbiopara.2010.09.007
Murata, K., Mitsuoka, K., Hirai, T., Walz, T., Agre, P., and Heymann (2000). Structural determinants of water permeation through aquaporin-1. Nature 407, 599–605. doi: 10.1038/35036519s
Nagaraju, G. P., Basha, R., Rajitha, B., Alese, O. B., Alam, A., and Pattnaik, S. (2016). Aquaporins: their role in gastrointestinal malignancies. Cancer Lett. 373, 12–18. doi: 10.1016/j.canlet.2016.01.003
Nakahigashi, K., Kabashima, K., Ikoma, A., Verkman, A. S., Miyachi, Y., and Hara-Chikuma, M. (2011). Upregulation of Aquaporin-3 is involved in keratinocyte proliferation and epidermal hyperplasia. J. Invest. Dermatol. 131, 865–873. doi: 10.1038/jid.2010.395
Ni, Z. X., Cui, J. M., Zhang, N. Z., and Fu, B. Q. (2017). Structural and evolutionary divergence of aquaporins in parasites (Review). Mol. Med. Rep. 15, 3943–3948. doi: 10.3892/mmr.2017.6505
Nico, B., Mangieri, D., Tamma, R., Longo, V., Annese, T., Crivellato, E., et al. (2009). Aquaporin-4 contributes to the resolution of peritumoural brain oedema in human glioblastoma multiforme after combined chemotherapy and radiotherapy. Eur. J. Cancer 45, 3315–3325. doi: 10.1016/j.ejca.2009.09.023
Nielsen, S., Chou, C. L., Marples, D., Christensen, E. I., Kishore, B. K., and Knepper, M. A. (1995). Vasopressin increases water permeability of kidney collecting duct by inducing translocation of aquaporin-CD water channels to plasma membrane. Proc. Natl. Acad. Sci. U.S.A. 92, 1013–1017. doi: 10.1073/pnas.92.4.1013
Niemietz, C. M., and Tyerman, S. D. (2002). New potent inhibitors of aquaporins: silver and gold compounds inhibit aquaporins of plant and human origin. FEBS Lett. 531, 443–447. doi: 10.1016/S0014-5793(02)03581-0
Noda, Y., Sohara, E., Ohta, E., and Sasaki, S. (2010). Aquaporins in kidney pathophysiology. Nat. Rev. Nephrol. 6, 168–178. doi: 10.1038/nrneph.2009.231
Nyboer, B., Heiss, K., Mueller, A. K., and Ingmundson, A. (2018). The Plasmodium liver-stage parasitophorous vacuole: a front-line of communication between parasite and host. Int. J. Med. Microbiol. 308, 107–117. doi: 10.1016/j.ijmm.2017.09.008
Ohta, E., Itoh, T., Nemoto, T., Kumagai, J., Ko, S. B., Ishibashi, K., et al. (2009). Pancreas-specific aquaporin 12 null mice showed increased susceptibility to caerulein-induced acute pancreatitis. Am. J. Physiol. Cell. Physiol. 297, C1368–C1378. doi: 10.1152/ajpcell.00117.2009
Okamura, T., Miyoshi, I., Takahashi, K., Mototani, Y., Ishigaki, S., Kon, Y., et al. (2003). Bilateral congenital cataracts result from a gain-of-function mutation in the gene for aquaporin-0 in mice. Genomics 81, 361–368. doi: 10.1016/S0888-7543(03)00029-6
Olesen, E. T., De Seigneux, S., Wang, G., Lütken, S. C., Frøkiaer, J., Kwon, T. H., et al. (2009). Rapid and segmental specific dysregulation of AQP2, S256-pAQP2 and renal sodium transporters in rats with LPS-induced endotoxaemia. Nephrol. Dial. Transplant. 24, 2338–2349. doi: 10.1093/ndt/gfp011
Olsson, M., Broberg, A., Jernås, M., Carlsson, L., Rudemo, M., Suurküla, M., et al. (2006). Increased expression of aquaporin 3 in atopic eczema. Allergy 61, 1132–1137. doi: 10.1111/j.1398-9995.2006.01151.x
Opal, S. M. (2007). The host response to endotoxin, antilipopolysaccharide strategies, and the management of severe sepsis. Int. J. Med. Microbiol. 297, 365–377. doi: 10.1016/j.ijmm.2007.03.006
Papadopoulos, M. C., and Saadoun, S. (2015). Key roles of aquaporins in tumor biology. Biochim. Biophys. Acta Biomembr. 1848, 2576–2583. doi: 10.1016/j.bbamem.2014.09.001
Papadopoulos, M. C., and Verkman, A. S. (2007). Aquaporin-4 and brain edema. Pediatr. Nephrol. 22, 778–784. doi: 10.1007/s00467-006-0411-0
Patel, R., Kevin Heard, L., Chen, X., and Bollag, W. B. (2017). Aquaporins in the skin. Adv. Exp. Med. Biol. 969, 173–191. doi: 10.1007/978-94-024-1057-0_11
Pelagalli, A., Squillacioti, C., Mirabella, N., and Meli, R. (2016). Aquaporins in health and disease: an overview focusing on the gut of different species. Int. J. Mol. Sci. 17:1213. doi: 10.3390/ijms17081213
Pellavio, G., Todaro, F., Alberizzi, P., Scotti, C., Gastaldi, G., Lolicato, M., et al. (2020). HPV infection affects human sperm functionality by inhibition of aquaporin-8. Cells 9:1241. doi: 10.3390/cells9051241
Peng, A., Cai, J., Lai, W., Zhang, L., Qiu, X., Zhu, X., et al. (2018). Autoimmune Aquaporin-4 channelopathy presented with psychiatric symptoms: a case report. Neuropsychiatry 8, 801–807. doi: 10.4172/Neuropsychiatry.1000406
Perez Di Giorgio, J., Soto, G., Alleva, K., Jozefkowicz, C., Amodeo, G., et al. (2014). Prediction of aquaporin function by integrating evolutionary and functional analyses. J. Membr. Biol. 247, 107–125. doi: 10.1007/s00232-013-9618-8
Pitonzo, D., and Skach, W. R. (2006). Molecular mechanisms of aquaporin biogenesis by the endoplasmic reticulum Sec61 translocon. Biochim. Biophys. Acta 1758, 976–988. doi: 10.1016/j.bbamem.2006.04.021
Posfai, D., Maher, S. P., Roesch, C., Vantaux, A., Sylvester, K., Péneau, J., et al. (2020). Plasmodium vivax liver and blood stages recruit the druggable host membrane channel Aquaporin-3. Cell Chem. Biol. 27, 719.e5–727.e5. doi: 10.1016/j.chembiol.2020.03.009
Posfai, D., Sylvester, K., Reddy, A., Ganley, J. G., Wirth, J., Cullen, Q. E., et al. (2018). Plasmodium parasite exploits host aquaporin-3 during liver stage malaria infection. PLoS Pathog. 14:e1007057. doi: 10.1371/journal.ppat.1007057
Prata, C., Hrelia, S., and Fiorentini, D. (2019). Peroxiporins in cancer. Int. J. Mol. Sci. 20:1371. doi: 10.3390/ijms20061371
Preston, G. M., Jung, J. S., Guggino, W. B., and Agre, P. (1993). The mercury-sensitive residue at cysteine 189 in the CHIP28 water channel. J. Biol. Chem. 268, 17–20. doi: 10.1016/S0021-9258(18)54108-9
Promeneur, D., Liu, Y., Maciel, J., Agre, P., King, L. S., and Kumar, N. (2007). Aquaglyceroporin PbAQP during intraerythrocytic development of the malaria parasite Plasmodium berghei. Proc. Natl. Acad. Sci. U.S.A. 104, 2211–2216. doi: 10.1073/pnas.0610843104
Promeneur, D., Lunde, L. K., Amiry-Moghaddam, M., and Agre, P. (2013). Protective role of brain water channel AQP4 in murine cerebral malaria. Proc. Natl. Acad. Sci. U.S.A. 110, 1035–1040. doi: 10.1073/pnas.1220566110
Promeneur, D., Mlambo, G., Agre, P., and Coppens, I. (2018). Aquaglyceroporin PbAQP is required for efficient progression through the liver stage of Plasmodium infection. Sci. Rep. 8:655. doi: 10.1038/s41598-017-18987-3
Qin, F., Zhang, H., Shao, Y., Liu, X., Yang, L., Huang, Y., et al. (2016). Expression of aquaporin1, a water channel protein, in cytoplasm is negatively correlated with prognosis of breast cancer patients. Oncotarget 7, 8143–8154. doi: 10.18632/oncotarget.6994
Radin, M. J., Yu, M.-J., Stoedkilde, L., Miller, R. L., Hoffert, J. D., Frokiaer, J., et al. (2012). Aquaporin-2 regulation in health and disease. Vet. Clin. Pathol. 41, 455–470. doi: 10.1111/j.1939-165x.2012.00488.x
Ramachandran, G. (2014). Gram-positive and gram-negative bacterial toxins in sepsis: a brief review. Virulence 5, 213–218. doi: 10.4161/viru.27024
Ribeiro Mde, C., Hirt, L., Bogousslavsky, J., Regli, L., and Badaut, J. (2006). Time course of aquaporin expression after transient focal cerebral ischemia in mice. J. Neurosci. Res. 83, 1231–1240. doi: 10.1002/jnr.20819
Rodrigues, C. E., Sanches, T. R., Volpini, R. A., Shimizu, M. H., Kuriki, P. S., Camara, N. O., et al. (2012). Effects of continuous erythropoietin receptor activator in sepsis-induced acute kidney injury and multi-organ dysfunction. PLoS One 7:e29893. doi: 10.1371/journal.pone.0029893
Rodríguez, A., Catalán, V., Gómez-Ambrosi, J., García-Navarro, S., Rotellar, F., Valentí, V., et al. (2011). Insulin- and leptin-mediated control of aquaglyceroporins in human adipocytes and hepatocytes is mediated via the PI3K/Akt/mTOR signaling cascade. J. Clin. Endocrinol. Metab. 96, E586–E597. doi: 10.1210/jc.2010-1408
Rojek, A., Praetorius, J., Frøkiaer, J., Nielsen, S., and Fenton, R. A. (2008). A current view of the mammalian aquaglyceroporins. Annu. Rev. Physiol. 70, 301–327. doi: 10.1146/annurev.physiol.70.113006.100452
Rong, M. L., Zhang, Z. W., Luo, Y., Shi, G., and Fan, Y. M. (2019). Epidermal aquaporin-3 downexpression in symmetrical acrokeratoderma. J. Eur. Acad. Dermatol. Venereol. 33, e208–e209. doi: 10.1111/jdv.15476
Rosen, B. P., and Tamás, M. J. (2010). “Arsenic transport in prokaryotes and eukaryotic microbes,” in MIPs and Their Role in the Exchange of Metalloids. Advances in Experimental Medicine and Biology, eds T. P. Jahn and G. P. Bienert, (New York, NY: Springer), 679. doi: 10.1007/978-1-4419-6315-4_4
Rump, K., and Adamzik, M. (2018). Function of aquaporins in sepsis: a systematic review. Cell Biosci. 8:10. doi: 10.1186/s13578-018-0211-9
Rump, K., Brendt, P., Frey, U. H., Schäfer, S. T., Siffert, W., Peters, J., et al. (2013). Aquaporin 1 and 5 expression evoked by the β2 adrenoreceptor agonist terbutaline and lipopolysaccharide in mice and in the human monocytic cell line THP-1 is differentially regulated. Shock 40, 430–436. doi: 10.1097/SHK.0000000000000035
Rutkovskiy, A., Valen, G., and Vaage, J. (2013). Cardiac aquaporins. Basic Res. Cardiol. 108:393. doi: 10.1007/s00395-013-0393-6
Saadoun, S., Papadopoulos, M. C., Davies, D. C., Bell, B. A., and Krishna, S. (2002a). Increased aquaporin 1 water channel expression in human brain tumours. Br. J. Cancer 87, 621–623. doi: 10.1038/sj.bjc.6600512
Saadoun, S., Papadopoulos, M. C., Davies, D. C., Krishna, S., and Bell, B. A. (2002b). Aquaporin-4 expression is increased in oedematous human brain tumours. J. Neurol. Neurosurg. Psychiatry 72, 262–265. doi: 10.1136/jnnp.72.2.262
Saadoun, S., Papadopoulos, M. C., Hara-Chikuma, M., and Verkman, A. S. (2005a). Impairment of angiogenesis and cell migration by targeted aquaporin-1 gene disruption. Nature 434, 786–792. doi: 10.1038/nature03460
Saadoun, S., Papadopoulos, M. C., Watanabe, H., Yan, D., Manley, G. T., and Verkman, A. S. (2005b). Involvement of aquaporin-4 in astroglial cell migration and glial scar formation. J. Cell Sci. 118, 5691–5698. doi: 10.1242/jcs.02680
Santoni, V. (2017). “Plant aquaporin posttranslational regulation,” in Plant Aquaporins: From Transport to Signaling, eds F. Chaumont and S. D. Tyerman, (Cham: Springer International Publishing), 83–105. doi: 10.1007/978-3-319-49395-4_4
Schenk, A. D., Hite, R. K., Engel, A., Fujiyoshi, Y., and Walz, T. (2010). Electron crystallography and aquaporins. Methods Enzymol. 483, 91–119. doi: 10.1016/S0076-6879(10)83005-8
Schey, K. L., Wang, Z., Wenke, J. L., and Qi, Y. (2014). Aquaporins in the eye: expression, function, and roles in ocular disease. Biochim. Biophys. Acta 1840, 1513–1523. doi: 10.1016/j.bbagen.2013.10.037
Sebastian, R., Chau, E., Fillmore, P., Matthews, J., Price, L. A., Sidhaye, V., et al. (2015). Epidermal aquaporin-3 is increased in the cutaneous burn wound. Burns 41, 843–847. doi: 10.1016/j.burns.2014.10.033
Seleit, I., Bakry, O. A., Al Sharaky, D., and Ragheb, E. (2015). Evaluation of Aquaporin-3 role in nonmelanoma skin cancer: an immunohistochemical study. Ultrastruct. Pathol. 39, 306–317. doi: 10.3109/01913123.2015.1022241
Shank, R. P., and Maryanoff, B. E. (2008). Molecular pharmacodynamics, clinical therapeutics, and pharmacokinetics of topiramate. CNS Neurosci. Ther. 14, 120–142. doi: 10.1111/j.1527-3458.2008.00041.x
Shchepareva, M. E., and Zakharova, M. N. (2020). Functional role of aquaporins in the nervous system under normal and pathological conditions. Neurochem. J. 14, 1–8. doi: 10.1134/S1819712420010171
Shen, L., Zhu, Z., Huang, Y., Shu, Y., Sun, M., and Xu, H. (2010). Expression profile of multiple aquaporins in human gastric carcinoma and its clinical significance. Biomed. Pharmacother. 64, 313–318.
Shen, Y., Wang, X., Wang, Y., Wang, X., Chen, Z., Jin, M., et al. (2012). Lipopolysaccharide decreases aquaporin 5, but not aquaporin 3 or aquaporin 4, expression in human primary bronchial epithelial cells. Respirology 17, 1144–1149.
Sherling, E., and Ooij, C. (2016). Host cell remodeling by pathogens: the exomembrane system in Plasmodium-infected erythrocytesa. FEMS Microbiol. Rev. 40, 701–721.
Shi, X., Wu, S., Yang, Y., Tang, L., Wang, Y., Dong, J., et al. (2014). AQP5 silencing suppresses p38 MAPK signaling and improves drug resistance in colon cancer cells. Tumour. Biol. 35, 7035–7045.
Shiels, A., and Bassnett, S. (1996). Mutations in the founder of the MIP gene family underlie cataract development in the mouse. Nat. Genet. 12, 212–215.
Shiels, A., Bassnett, S., Varadaraj, K., Mathias, R., Al-Ghoul, K., Kuszak, J., et al. (2001). Optical dysfunction of the crystalline lens in aquaporin-0-deficient mice. Physiol. Genomics 7, 179–186.
Shin, C. M., Kim, N., Yang, H. J., Cho, S. I., Lee, H. S., Kim, J. S., et al. (2010). Stomach cancer risk in gastric cancer relatives: interaction between Helicobacter pylori infection and family history of gastric cancer for the risk of stomach cancer. J. Clin. Gastroenterol. 44, e34–e39.
Skowronski, M. T., Lebeck, J., Rojek, A., Praetorius, J., Füchtbauer, E. M., Frøkiaer, J., et al. (2007). AQP7 is localized in capillaries of adipose tissue, cardiac and striated muscle: implications in glycerol metabolism. Am. J. Physiol. Renal. Physiol. 292, F956–F965.
Song, J., Almasalmeh, A., Krenc, D., and Beitz, E. (2012). Molar concentrations of sorbitol and polyethylene glycol inhibit the Plasmodium aquaglyceroporin but not that of E. coli: involvement of the channel vestibules. Biochim. Biophys. Acta Biomembr. 1818, 1218–1224.
Song, J., Baker, N., Rothert, M., Henke, B., Jeacock, L., Horn, D., et al. (2016). Pentamidine is not a permeant but a nanomolar inhibitor of the Trypanosoma brucei Aquaglyceroporin-2. PLoS Pathog. 12:e1005436. doi: 10.1371/journal.ppat.1005436
Sonntag, Y., Gena, P., Maggio, A., Singh, T., Artner, I., Oklinski, M. K., et al. (2019). Identification and characterization of potent and selective aquaporin-3 and aquaporin-7 inhibitors. J. Biol. Chem. 294, 7377–7387.
Soto, G., Alleva, K., Amodeo, G., Muschietti, J., and Ayub, N. D. (2012). New insight into the evolution of aquaporins from flowering plants and vertebrates: orthologous identification and functional transfer is possible. Gene 503, 165–176.
St Hillaire, C., Vargas, D., Pardo, C. A., Gincel, D., Mann, J., Rothstein, J. D., et al. (2005). Aquaporin 4 is increased in association with human immunodeficiency virus dementia: implications for disease pathogenesis. J. Neurovirol. 11, 535–543.
Su, W., Cao, R., Zhang, X. Y., and Guan, Y. (2020). Aquaporins in the kidney: physiology and pathophysiology. Am. J. Physiol. Renal Physiol. 318, F193–F203.
Suh, S. H., Lee, K. E., Kim, I. J., Kim, O., Kim, C. S., Choi, J. S., et al. (2015). Alpha-lipoic acid attenuates lipopolysaccharide-induced kidney injury. Clin. Exp. Nephrol. 19, 82–91.
Sui, H., Han, B. G., Lee, J. K., Walian, P., and Jap, B. K. (2001). Structural basis of water-specific transport through the AQP1 water channel. Nature 414, 872–878.
Swindell, W. R., Xing, X., Voorhees, J. J., Elder, J. T., Johnston, A., and Gudjonsson, J. E. (2014). Integrative RNA-seq and microarray data analysis reveals GC content and gene length biases in the psoriasis transcriptome. Physiol. Genomics 46, 533–546.
Tajkhorshid, E., Nollert, P., Jensen, M., Miercke, L. J., O’connell, J., Stroud, R. M., et al. (2002). Control of the selectivity of the aquaporin water channel family by global orientational tuning. Science 296, 525–530.
Takano, J., Yoshinari, A., and Luu, D. T. (2017). “Plant aquaporin trafficking,” in Plant Aquaporins: From Transport to Signaling, eds F. Chaumont and S. D. Tyerman, (Cham: Springer International Publishing), 47–81.
Talwar, S., Munson, P. J., Barb, J., Fiuza, C., Cintron, A. P., Logun, C., et al. (2006). Gene expression profiles of peripheral blood leukocytes after endotoxin challenge in humans. Physiol. Genomics 25, 203–215.
Tamma, G., Robben, J. H., Trimpert, C., Boone, M., and Deen, P. M. (2011). Regulation of AQP2 localization by S256 and S261 phosphorylation and ubiquitination. Am. J. Physiol. Cell Physiol. 300, C636–C646.
Tan, M. H., Wong, C. F., Tan, H. L., Yang, X. J., Ditlev, J., Matsuda, D., et al. (2010). Genomic expression and single-nucleotide polymorphism profiling discriminates chromophobe renal cell carcinoma and oncocytoma. BMC Cancer 10:196. doi: 10.1186/1471-2407-10-196
Tao, B., Liu, L., Wang, N., Wang, W., Jiang, J., and Zhang, J. (2016). Effects of hydrogen-rich saline on aquaporin 1, 5 in septic rat lungs. J. Surg. Res. 202, 291–298.
Thiagarajah, J. R., Chang, J., Goettel, J. A., Verkman, A. S., and Lencer, W. I. (2017). Aquaporin-3 mediates hydrogen peroxide-dependent responses to environmental stress in colonic epithelia. Proc. Natl. Acad. Sci. U.S.A. 114, 568–573.
Thuny, F., Textoris, J., Amara, A. B., Filali, A. E., Capo, C., Habib, G., et al. (2012). The gene expression analysis of blood reveals S100A11 and AQP9 as potential biomarkers of infective endocarditis. PLoS One 7:e31490. doi: 10.1371/journal.pone.0031490
Tornesello, M. L., and Buonaguro, F. M. (2020). Human papillomavirus and cancers. Cancers 12:3772. doi: 10.3390/cancers12123772
Törnroth-Horsefield, S., Hedfalk, K., Fischer, G., Lindkvist-Petersson, K., and Neutze, R. (2010). Structural insights into eukaryotic aquaporin regulation. FEBS Lett. 584, 2580–2588.
Törnroth-Horsefield, S., Wang, Y., Hedfalk, K., Johanson, U., Karlsson, M., Tajkhorshid, E., et al. (2006). Structural mechanism of plant aquaporin gating. Nature 439, 688–694.
Towne, J. E., Harrod, K. S., Krane, C. M., and Menon, A. G. (2000). Decreased expression of aquaporin (AQP)1 and AQP5 in mouse lung after acute viral infection. Am. J. Respir. Cell Mol. Biol. 22, 34–44.
Tran, T. L., Bek, T., Holm, L., La Cour, M., Nielsen, S., Prause, J. U., et al. (2013). Aquaporins 6-12 in the human eye. Acta Ophthalmol. 91, 557–563.
Tsai, W. C., Wu, H. Y., Peng, Y. S., Ko, M. J., Wu, M. S., Hung, K. Y., et al. (2016). Risk factors for development and progression of chronic kidney disease: a systematic review and exploratory meta-analysis. Medicine 95:e3013.
Turner, J. D., Jenkins, G. R., Hogg, K. G., Aynsley, S. A., Paveley, R. A., Cook, P. C., et al. (2011). CD4+CD25+ regulatory cells contribute to the regulation of colonic Th2 granulomatous pathology caused by schistosome infection. PLoS Negl. Trop. Dis. 5:e1269. doi: 10.1371/journal.pntd.0001269
Uzcategui, N. L., Szallies, A., Pavlovic-Djuranovic, S., Palmada, M., Figarella, K., Boehmer, C., et al. (2004). Cloning, heterologous expression, and characterization of three aquaglyceroporins from Trypanosoma brucei. J. Biol. Chem. 279, 42669–42676.
van Balkom, B. W., Savelkoul, P. J., Markovich, D., Hofman, E., Nielsen, S., Van Der Sluijs, P., et al. (2002). The role of putative phosphorylation sites in the targeting and shuttling of the aquaporin-2 water channel. J. Biol. Chem. 277, 41473–41479.
Vassiliou, A. G., Maniatis, N. A., Orfanos, S. E., Mastora, Z., Jahaj, E., Paparountas, T., et al. (2013). Induced expression and functional effects of aquaporin-1 in human leukocytes in sepsis. Crit. Care 17:R199.
Verkerk, A. O., Lodder, E. M., and Wilders, R. (2019). Aquaporin channels in the heart-physiology and pathophysiology. Int. J. Mol. Sci. 20:2039.
Verkman, A. S. (2005). More than just water channels: unexpected cellular roles of aquaporins. J. Cell Sci. 118, 3225–3232.
Verkman, A. S., Anderson, M. O., and Papadopoulos, M. C. (2014). Aquaporins: important but elusive drug targets. Nat. Rev. Drug Discov. 13, 259–277.
Verkman, A. S., Hara-Chikuma, M., and Papadopoulos, M. C. (2008a). Aquaporins–new players in cancer biology. J. Mol. Med. 86, 523–529.
Verkman, A. S., and Mitra, A. K. (2000). Structure and function of aquaporin water channels. Am. J. Physiol. Renal Physiol. 278, F13–F28.
Verkman, A. S., Ruiz-Ederra, J., and Levin, M. H. (2008b). Functions of aquaporins in the eye. Prog. Retin. Eye Res. 27, 420–433.
Von Bülow, J., and Beitz, E. (2015). Number and regulation of protozoan aquaporins reflect environmental complexity. Biol. Bull. 229, 38–46.
Walochnik, J., Auer, H., and Joachim, A. (2017). “Parasitic infections in and animals humans,” in Comparative Medicine, ed. E. Jensen-Jarolim, (Berlin: Springer), 178–188.
Wang, G., Gao, F., Zhang, W., Chen, J., Wang, T., Zhang, G., et al. (2012). Involvement of Aquaporin 3 in Helicobacter pylori-related gastric diseases. PLoS One 7:e49104. doi: 10.1371/journal.pone.0049104
Wang, H., Jin, R., Tian, P., and Zhuo, Z. (2009). Enhanced expression of aquaporin-9 in rat brain edema induced by bacterial lipopolysaccharides. J. Huazhong Univ. Sci. Technol. 29, 150–155.
Wang, J., Feng, L., Zhu, Z., Zheng, M., Wang, D., Chen, Z., et al. (2015). Aquaporins as diagnostic and therapeutic targets in cancer: how far we are? J. Transl. Med. 13:96.
Wang, J., Liu, W., Zhao, Z., Zhang, Y., and Luo, B. (2018). Association of the Aquaporin 3 gene polymorphism (rs2231231) with epstein-barr virus-associated cancers in China. Intervirology 61, 72–78.
Wang, L., Tang, H., Wang, C., Hu, Y., Wang, S., and Shen, L. (2019). Aquaporin 4 deficiency alleviates experimental colitis in mice. FASEB J. 33, 8935–8944.
Wang, R., Wang, M., Chen, K., Wang, S., Mur, L. A. J., and Guo, S. (2018). Exploring the roles of aquaporins in plant?microbe interactions. Cells 7:267.
Wang, W., Li, C., Summer, S., Falk, S., Wang, W., Ljubanovic, D., et al. (2008). Role of AQP1 in endotoxemia-induced acute kidney injury. Am. J. Physiol. Renal Physiol. 294, F1473–F1480.
Wang, W., Li, F., Sun, Y., Lei, L., Zhou, H., Lei, T., et al. (2015). Aquaporin-1 retards renal cyst development in polycystic kidney disease by inhibition of Wnt signaling. FASEB J. 29, 1551–1563.
Wang, W., Li, Q., Yang, T., Bai, G., Li, D., Li, Q., et al. (2012). Expression of AQP5 and AQP8 in human colorectal carcinoma and their clinical significance. World J. Surg. Oncol. 10:242.
Wang, Y., Li, R., Li, D., Jia, X., Zhou, D., Li, J., et al. (2017). NIP1;2 is a plasma membrane-localized transporter mediating aluminum uptake, translocation, and tolerance in Arabidopsis. Proc. Natl. Acad. Sci. U.S.A. 114, 5047–5052.
Warth, A., Simon, P., Capper, D., Goeppert, B., Tabatabai, G., Herzog, H., et al. (2007). Expression pattern of the water channel aquaporin-4 in human gliomas is associated with blood-brain barrier disturbance but not with patient survival. J. Neurosci. Res. 85, 1336–1346.
Watanabe, S., Moniaga, C. S., Nielsen, S., and Hara-Chikuma, M. (2016). Aquaporin-9 facilitates membrane transport of hydrogen peroxide in mammalian cells. Biochem. Biophys. Res. Commun. 471, 191–197.
Wen, J., Wang, Y., Gao, C., Zhang, G., You, Q., Zhang, W., et al. (2018). Helicobacter pylori infection promotes Aquaporin 3 expression via the ROS-HIF-1α-AQP3-ROS loop in stomach mucosa: a potential novel mechanism for cancer pathogenesis. Oncogene 37, 3549–3561.
Wen, X., He, L., Chi, Y., Zhou, S., Hoellwarth, J., Zhang, C., et al. (2011). Dynamics of Th17 cells and their role in Schistosoma japonicum infection in C57BL/6 mice. PLoS Negl. Trop. Dis. 5:e1399. doi: 10.1371/journal.pntd.0001399
Wu, H., Chen, L., Zhang, X., Zhou, Q., Li, J. M., Berger, S., et al. (2013). Aqp5 is a new transcriptional target of Dot1a and a regulator of Aqp2. PLoS One 8:e53342. doi: 10.1371/journal.pone.0053342
Xian, Z. H., Cong, W. M., Wang, Y. H., Wang, B., and Wu, M. C. (2009). Expression and localization of aquaporin-1 in human cirrhotic liver. Pathol. Res. Pract. 205, 774–780.
Xie, Y., Wen, X., Jiang, Z., Fu, H. Q., Han, H., and Dai, L. (2012). Aquaporin 1 and aquaporin 4 are involved in invasion of lung cancer cells. Clin. Lab. 58, 75–80.
Xing, H. Q., Zhang, Y., Izumo, K., Arishima, S., Kubota, R., Ye, X., et al. (2017). Decrease of aquaporin-4 and excitatory amino acid transporter-2 indicate astrocyte dysfunction for pathogenesis of cortical degeneration in HIV-associated neurocognitive disorders. Neuropathology 37, 25–34.
Xu, H., and Zwiazek, J. J. (2020). Fungal aquaporins in ectomycorrhizal root water transport. Front. Plant Sci. 11:302. doi: 10.3389/fpls.2020.00302
Xu, M., Xiao, M., Li, S., and Yang, B. (2017). Aquaporins in nervous system. Adv Exp Med Biol. 969, 81–103.
Yang, B., Zhang, H., and Verkman, A. S. (2008). Lack of aquaporin-4 water transport inhibition by antiepileptics and arylsulfonamides. Bioorg. Med. Chem. 16, 7489–7493.
Yasui, M., Kwon, T.-H., Knepper, M. A., Nielsen, S., and Agre, P. (1999). Aquaporin-6: an intracellular vesicle water channel protein in renal epithelia. Proc. Natl. Acad. Sci. U.S.A. 96, 5808–5813.
Yool, A. J., Stamer, W. D., and Regan, J. W. (1996). Forskolin stimulation of water and cation permeability in aquaporin 1 water channels. Science 273, 1216–1218.
Yoshida, T., Hojo, S., Sekine, S., Sawada, S., Okumura, T., and Nagata, T. (2013). Expression of aquaporin-1 is a poor prognostic factor for stage II and III colon cancer. Mol. Clin. Oncol. 1, 953–958.
Yusenko, M. V., Zubakov, D., and Kovacs, G. (2009). Gene expression profiling of chromophobe renal cell carcinomas and renal oncocytomas by Affymetrix GeneChip using pooled and individual tumours. Int. J. Biol. Sci. 5, 517–527.
Zador, Z., Stiver, S., Wang, V., and Manley, G. T. (2009). Role of aquaporin-4 in cerebral edema and stroke. Handb. Exp. Pharmacol 190, 159–170.
Zhang, H., and Verkman, A. S. (2010). Aquaporin-1 tunes pain perception by interaction with Na(v)1.8 Na+ channels in dorsal root ganglion neurons. J. Biol. Chem. 285, 5896–5906.
Zhang, L., Chen, L., and Dong, H. (2019). Plant aquaporins in infection by and immunity against pathogens - a critical review. Front. Plant Sci. 10:632. doi: 10.3389/fpls.2019.00632
Zhang, T., Zhao, C., Chen, D., and Zhou, Z. (2012a). Overexpression of AQP5 in cervical cancer: correlation with clinicopathological features and prognosis. Med. Oncol. 29, 1998–2004.
Zhang, W., Zhu, J., Song, X., Xu, Z., Xue, X., Chen, X., et al. (2015). An association of Aquaporin-4 with the immunoregulation of liver pathology in mice infected with Schistosoma japonicum. Parasit. Vectors 8:37.
Zhang, Y., Wang, X., Sha, S., Liang, S., Zhao, L., Liu, L., et al. (2012b). Berberine increases the expression of NHE3 and AQP4 in sennosideA-induced diarrhoea model. Fitoterapia 83, 1014–1022.
Zhang, Z., Chen, Z., Song, Y., Zhang, P., Hu, J., and Bai, C. (2010). Expression of aquaporin 5 increases proliferation and metastasis potential of lung cancer. J. Pathol. 221, 210–220.
Zheng, X., and Bollinger Bollag, W. (2003). Aquaporin 3 colocates with phospholipase d2 in caveolin-rich membrane microdomains and is downregulated upon keratinocyte differentiation. J. Invest. Dermatol. 121, 1487–1495.
Zheng, Y. F., Liu, C. F., Lai, W. F., Xiang, Q., Li, Z. F., Wang, H., et al. (2014). The laxative effect of emodin is attributable to increased aquaporin 3 expression in the colon of mice and HT-29 cells. Fitoterapia 96, 25–32.
Zhu, C., Chen, Z., and Jiang, Z. (2016). Expression, distribution and role of aquaporin water channels in human and animal stomach and intestines. Int. J. Mol. Sci. 17:1399.
Zimmet, P., Alberti, K. G., and Shaw, J. (2001). Global and societal implications of the diabetes epidemic. Nature 414, 782–787.
Keywords: human aquaporins, aquaporins and infectious diseases, water homeostasis, functional regulation, drug targets
Citation: Azad AK, Raihan T, Ahmed J, Hakim A, Emon TH and Chowdhury PA (2021) Human Aquaporins: Functional Diversity and Potential Roles in Infectious and Non-infectious Diseases. Front. Genet. 12:654865. doi: 10.3389/fgene.2021.654865
Received: 17 January 2021; Accepted: 22 February 2021;
Published: 16 March 2021.
Edited by:
Mahbuba Rahman, Sidra Medicine, QatarReviewed by:
Kenichi Ishibashi, Meiji Pharmaceutical University, JapanMonsur Ali, McMaster University, Canada
Md Nurul Islam, Intact Genomics, Inc, United States
Copyright © 2021 Azad, Raihan, Ahmed, Hakim, Emon and Chowdhury. This is an open-access article distributed under the terms of the Creative Commons Attribution License (CC BY). The use, distribution or reproduction in other forums is permitted, provided the original author(s) and the copyright owner(s) are credited and that the original publication in this journal is cited, in accordance with accepted academic practice. No use, distribution or reproduction is permitted which does not comply with these terms.
*Correspondence: Abul Kalam Azad, ZGFrYXphZC1idGNAc3VzdC5lZHU=
†These authors have contributed equally to this work