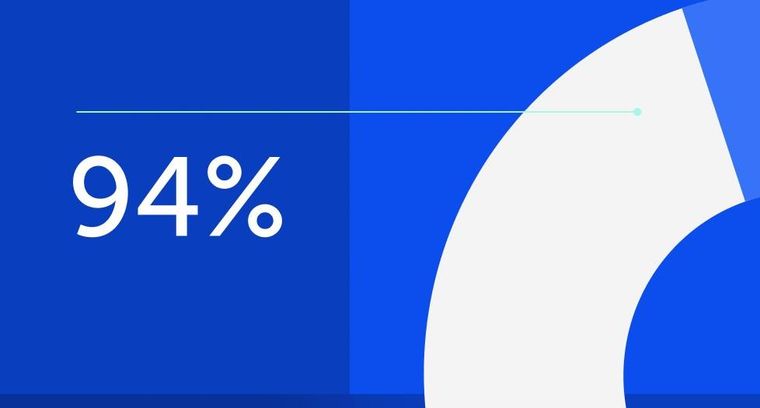
94% of researchers rate our articles as excellent or good
Learn more about the work of our research integrity team to safeguard the quality of each article we publish.
Find out more
ORIGINAL RESEARCH article
Front. Genet., 08 March 2021
Sec. Evolutionary and Population Genetics
Volume 12 - 2021 | https://doi.org/10.3389/fgene.2021.632232
Edible bird’s nest (EBN) is a popular delicacy in the Asian Pacific region originating from Indonesia, Malaysia, Thailand and Vietnam, which consist of various potential medicine value in Traditional Chinese Medicine (TCM). Thailand is one of the main exporters of EBN. However, the genetic information of EBN, a key part of molecular biology, has yet to be reported in Thailand. It is necessary to explore the genetic information of EBN in Thailand based on a quick and simple method to help protect the rights and interests of consumers. This research aimed to systematically evaluate different methods of extracting EBN DNA to improve the efficiency of the analysis of cytochrome b (Cytb) and NADH dehydrogenase subunit 2 (ND2) gene sequences, the establishment of phylogenetic trees, and the genetic information of EBN in Thailand. Additionally, we aimed to develop a quick and simple method for identifying EBN from different species based on the genetic information and amplification-refractory mutation system PCR (ARMS-PCR). By comparing the four methods [cetyltrimethylammonium bromide (CTAB), sodium dodecyl sulfate (SDS), kit and guanidinium isothiocyanate methods] for EBN extraction, we found that the guanidinium isothiocyanate method was the optimal extraction method. Phylogenetic trees generated on the basis of Cytb and ND2 gene analyses showed that 26 samples of house EBN and 4 samples of cave EBN came from Aerodramus fuciphagus and Aerodramus maximus, respectively. In addition, to distinguish different samples from different species of Apodiformes, we designed 4 polymerase chain reaction (PCR) amplification primers based on the ND2 gene sequences of A. fuciphagus and A. maximus. The ARMS-PCR results showed band lengths for A. fuciphagus EBN of 533, 402, and 201 bp, while those for A. maximus EBN were 463, 317, and 201 bp. Collectively, the results showed that ARMS-PCR is a fast and simple method for the genetic identification of EBN based on designing specific original identification primers.
Edible bird’s nest (EBN), produced by swiftlets of the Aerodramus genus (mainly Aerodramus fuciphagus and Aerodramus maximus) is constructed from viscous, sticky secretions of mucin glycoprotein from a pair of sublingual glands beneath the tongue of swiftlets (Looi et al., 2017). EBN has been esteemed as a nutritious food since the Yuan dynasty in China, which consist of various potential medicine value in Traditional Chinese Medicine (TCM). It is widely distributed in Indonesia, Malaysia, Thailand, Huaiji, and other Southeast Asian regions along the Pacific. Generally, there are two classification of EBN: house nests (found in swiftlet houses) and cave nests (found in natural caves) (Liu et al., 2020). With the development of the house nest industry and newly discovered properties of EBN, including neuroprotection and pro-conceptive effects, the consumption of EBN is gradually increasing (Careena et al., 2018; Albishtue et al., 2019). However, the EBN market value is estimated to range from $1000 to $50 000 per kg according to the swiftlet species (Liu et al., 2020). To increase profits, some unethical suppliers introduce cheaper adulterants into EBN, including high-protein and high-carbohydrate substances (Huang et al., 2019).
With the increasing occurrence of adulteration, there has been widespread interest in developing simple, sensitive and accurate techniques to check for the quality and authenticity of EBN, including physical and chemical techniques. For example, thermal analysis methods, gel electrophoresis technology, Fourier transform infrared spectroscopy (FTIR), Raman spectroscopy and the analysis of EBN fiber microstructure using arrays are applicable with the goal of the rapid authentication of EBN (Yang et al., 2014; Shim et al., 2017; Guo et al., 2018; Dai et al., 2020). Separation techniques have also been widely used, such as gas chromatography and liquid chromatography, which can analyze the composition of amino acids, saccharides, peptides, the polyunsaturated fatty acids, saturated fatty acids and mono-unsaturated fatty acids (Chua et al., 2015; Wong et al., 2018; Lee et al., 2020). At present, molecular biology is the most precise method for checking the quality of EBN. The loop-mediated isothermal amplification (LAMP) assay and polymerase chain reaction-restriction fragment length polymorphism (PCR-RFLP) analysis could be applicable for authenticating EBN (Lee et al., 2019; Liu et al., 2020). However, these techniques of genetic identification require expensive instrumentation and tedious sample preparation. On the other hand, the analysis of genetic information is generally carried out on the basis of sample-specific genes (Lee et al., 2017). Although, previous research have showed that the EBN samples in Indonesia derived from A. fuciphagus while the EBN samples in Huaiji derived from Apus nipalensis (Lin et al., 2009). EBNs from different producing areas show difference in genetic information. Thailand is one of the main exporters of EBN. However, the genetic information of EBN in Thailand has yet to be reported. Thus, it is urgent to develop a reliable and systematic method to clarify genetic information on EBN in Thailand.
Recently, many research suggested amplification-refractory mutation system PCR (ARMS-PCR) is the most rapid, reliable and cost-efficient genotyping method to identify genetic information (Alyethodi et al., 2018; Chao et al., 2018; Jin et al., 2020). Compared with PCR-RFLP, ARMS-PCR can detect the single nucleotide polymorphisms (SNPs) by four primers using only one PCR and needn’t restriction enzymes, probes and expensive equipment (Jin et al., 2020). Therefore, we hypothesized that ARMS-PCR was a reliable and simple method to identify genetic information on EBN in Thailand.
A total of 33 samples were purchased from Thailand, Indonesia and China by Guangzhou Tongkang Pharmaceutical Co., Ltd. (Table 1). Morphological identification was performed by Professor Xiaoping Lai and Professor Geng Li (Table 2). All samples were deposited in the Laboratory Animal Center of Guangzhou University of Chinese Medicine for future use. Cetyltrimethylammonium bromide (CTAB), Tris-base, Triton X-100, dichloromethane, isopropanol and Polyvinylpyrrolidone (PVP) were from Sigma-Aldrich Co., Ltd. (St. Louis, MO, United States). Sodium dodecyl sulfate (SDS) and ethylenediamine tetraacetic acid (EDTA) were from Biosharp Co., Ltd. (Hefei, Anhui, China). Buccal swab DNA extraction kit was from TIANGEN BIOTECH Co., Ltd. (Beijing, China). Guanidine isothiocyanate was from Solarbio Co., Ltd. (Beijing, China). Tris-HCl buffer and Tris-saturated phenol were from Leagene Biotechnology Co., Ltd. (Beijing, China). Dithiothreitol (DDT) was from Beyotime Co., Ltd. (Shanghai, China). Isoamylol and ethanol were from Sinopharm Chemical Reagent Co., Ltd. (Ningbo, China).
All samples were ground in a mortar by freezing using liquid nitrogen. Then EBN powder was obtained and deposited at −80°C for further experiments. To develop an efficient and rapid DNA extraction protocol, four different methods for DNA extraction from EBN were evaluated. Total genomic DNA from the EBN samples was extracted using the CTAB method, SDS method, a buccal swab DNA extraction kit (kit method) and the guanidine isothiocyanate method (Attitalla, 2011; Kampmann et al., 2016; Green and Sambrook, 2018; Xia et al., 2019).
First, 25 mg of EBN powder from five samples such as N5, N6, ID1, CNH2, and CNH3, respectively, was dissolved at 65°C in 200 μL CTAB buffer (10 mM Tris-HCl, 0.8 mM EDTA, 0.49 M NaCl, 2% CTAB, 2% DTT, and 3% PVP) for 1 h. Then, an equal volume of phenol (Tris-saturated phenol, dichloromethane, isopropanol = 25:24:1, pH 8.0) was added to the supernatant. After centrifugation (10,000 r/min) for 10 min, the supernatant was collected, and an equal volume of dichloromethane/isoamylol (24:1) was added. Then, 5 M NaCl (1/20 of the volume) and absolute ethanol (2 times the supernatant volume) were added. The solution was sealed in an airtight manner and placed at −20°C for 30 min, followed by centrifugation at 7,000 r/min at 4°C for 5 min. The sediment was collected and washed with the following sequence: 70% 200 μL ethanol and 100% 200 μL ethanol. After air drying at room temperature, 30 μL of TE buffer (10 mM Tris-HCl and 1 mM EDTA, pH 8.0) was added to the samples, followed by incubation for 5 min, and the samples were then stored at −20°C.
25 mg EBN powder (N5, N6, ID1, CNH2, and CNH3) was dissolved in 500 μL SDS buffer (10 M NaOH, 0.1 M EDTA, 0.1 M Tris-HCl, 0.5 M NaCl, 10% SDS, 2% DTT, and 3% PVP) and 200 μL TE buffer at 55°C for 1 h. Then, an equal volume of Tris-saturated phenol was added to the supernatant, followed by centrifugation (12,000 r/min) for 10 min. The supernatant was collected, and an equal volume of dichloromethane/isoamylol (24:1), 0.1 volume of 3 M sodium acetate and 2.5 times the volume of 100% ethanol were added successively. Then, the solution was sealed in an airtight manner and placed at −20°C for 120 min, followed by centrifugation at 12,000 r/min for 5 min. The sediment was collected and washed with 1 mL 70% ethanol. Finally, 30 μL TE buffer was added to the samples, followed by incubation for 5 min and storage at −20°C.
25 mg EBN powder (N5, N6, ID1, CNH2, and CNH3) was dissolved in 400 μL GA buffer and 20 μL protease K at 56°C for 1 h. Then, 400 μL GB buffer was added to the samples, which were placed at 70°C for 10 min. When the temperature of the solution dropped to room temperature, 200 μL of 100% ethanol was added. The solution and flocculent precipitate were removed from the adsorption column and centrifuged (12,000 r/min) for 30 s. Then, the sediment was washed in the following sequence: 500 μL GD buffer, followed by 600 μL PW buffer. After centrifugation at 12,000 r/min for 3 s, 30 μL TE buffer was added to the sediment, followed by incubation for 5 min and storage at −20°C.
25 mg EBN powder (D1–6, Z1–3, X1–3, N2–15, N17–19, N21, ID1, CNH2, and CNH3) was dissolved in 200 μL TE buffer and 400 μL guanidine isothiocyanate buffer (5 M guanidine isothiocyanate, 0.1 M EDTA, 0.1 M Tris-HCl, 1.3% Triton X-100, and 3% PVP) at 55°C for 1 h. Then, 300 μL of saturated phenol and 300 μL of dichloromethane/isoamylol (24:1) were added to the solution. After centrifugation (13,000 r/min) for 10 min, the supernatant was collected, and an equal volume of dichloromethane was added. After centrifugation (13,000 r/min) for 10 min, isoamylol (0.8 volume) was added to the supernatant, which was then centrifuged (12,000 r/min) for 10 min. The sediment was collected and washed with 1 mL of 70% ethanol. Finally, the samples were dissolved in 25 μL TE buffer and stored at −20°C.
The purity and concentration of DNA play a vital role in PCR, which is an important method of molecular analysis. Therefore, we sought to screen the best method of DNA extraction among common methods including the CTAB method, SDS method, kit method and guanidine isothiocyanate method according to the DNA mass concentration and A260/280 ratio. The DNA concentration and the ratio of the absorbance at 260 and 280 nm (A260/280 ratio) were evaluated on the same day as DNA extraction using a NanoDrop Lite spectrophotometer (Thermo Fisher Scientific, Waltham, MA, United States) (Sales et al., 2020). The A260/280 ratio was used to evaluate the purity of the DNA.
The inhibition of PCR amplification was detected for the EBN DNA obtained by the four different extraction methods. The cytochrome b (Cytb) gene and NADH dehydrogenase subunit 2 (ND2) gene were amplified by PCR with primers designed by Primer Premier 5.0 (Table 3). The amplification reaction system had a volume of 25 μL, including 1 μL of each primer (10 μM), 2 μL (100 ng) of genomic DNA, and 12.5 μL of 2× Master Mix (blue) buffer (Beyotime, Shanghai, China). The reaction mixture was placed into the PCR amplification instrument, and PCR amplification, was performed according to a previously reported procedure (Liu et al., 2020). The PCR conditions started with the initial denaturation at 94°C for 5 min, 40 cycles of 94°C for 30 s, 58°C for 90 s, and 72°C for 60 s, followed by a final extension at 72°C for 4 min. In addition, the size of the amplicons as shown in Table 3.
The PCR products were detected by performing agarose gel electrophoresis in 0.5× TBE buffer for 25 min at 180 V. Then, the gels were placed on a gel imager to evaluate the effect of PCR amplification. The PCR solution was sent to Beijing Qingke Biotechnology Co., Ltd. for bi-directional DNA sequencing.
Low-mass sequences and primer regions were removed using GeneStudio v.2.2.0.0, and images of the peaks obtained by sequencing were spliced and proofread. Then, an online search in the NCBI database1 was performed to obtain the sequences that were the most similar to those of the samples. The Cytb sequences of A. fuciphagus, A. maximus, Aerodramus fuciphagus germani and Amazilia tzacatl were downloaded from NCBI and combined with the sample sequences to form dataset 1. In addition, the ND2 sequences of A. fuciphagus, A. maximus, A. fuciphagus germani, and A. tzacatl were downloaded from NCBI and combined with the sample sequences to form dataset 2. After alignment using MAFFT v7.308, gBlocks was used to identify conserved fragments in the datasets (Katoh and Standley, 2013). Then, to ensure the accuracy of the analysis, the displacement of each dataset was calculated with DAMBE v7.0 (Xia, 2018). In addition, the X-value of each base substitution model was calculated with jModelTest v2.17, and the optimum base substitution model was selected based on the Akaike information criterion (AIC) standard (Darriba et al., 2012). Finally, the evolutionary distance among the samples were determined with MEGA-X based on kimura 2-parameter model (K2P). Maximum likelihood (ML) trees were constructed using MEGA-X, the reliability of the topology was evaluated using bootstrap values derived from 1,000 repetitions. The ML trees were visualized on the ITOL server2.
Traditional methods of genetic identification present several disadvantages, such as their time-consuming nature and the complicated steps involved. Thus, we designed primers according to the sequence of ND2 for quickly identifying EBNs produced by A. fuciphagus or A. maximus. Four pairs of primers were designed with Primer Premier 5.0 according to the ND2 sequence of A. fuciphagus downloaded at NCBI3. Then, the samples were amplified in an amplification reaction system of 20 μL, including 0.5 μL of each primer, 7 μL (350 ng) genomic DNA, 1 μL MgCl2, 2 μL dNTPs, 0.5 μL Taq DNA polymerase, and 7.5 μL of 2× Master Mix Buffer. The solution was placed in the PCR amplification instrument, and PCR amplification was performed. The PCR conditions started with the initial denaturation at 95°C for 5 min, 40 cycles of 95°C for 30 s, 65°C for 30 s, and 72°C for 60 s, followed by a final extension at 72°C for 10 min. Furthermore, the PCR products were evaluated by agarose gel electrophoresis in 0.5× TBE buffer for 50 min at 100 V. Then, the gels were placed in a gel imager to detect the results.
The results were analyzed with SPSS 22.0 software (SPSS, Inc., Chicago, IL, United States) and expressed as the mean ± standard deviation. The data were analyzed by one-way analysis of variance (ANOVA) and Least-Signification Difference (LSD). P < 0.05 was considered to indicate significant differences.
As shown in Table 4, the DNA mass concentration and A260/280 ratio obtained following extraction via the guanidine isothiocyanate method were 51.381 ± 29.011 ng/μL and 1.654 ± 0.153, respectively. This revealed that the guanidine isothiocyanate method resulted in less protein and RNA contamination and was the most stable method for extracting EBN DNA. In addition, the results obtained from the four methods showed that all EBN DNA samples obtained via the guanidine isothiocyanate method were amplified successfully with a clear single band (Figure 1). These results suggest that the guanidine isothiocyanate method is suitable for extracting EBN DNA.
Table 4. Concentration and purity of extracted edible bird’s nest DNA determined with the cetyltrimethylammonium bromide, sodium dodecyl sulfate, buccal swab DNA extraction kit and guanidine isothiocyanate methods.
Figure 1. The Polymerase chain reaction (PCR) products obtained from the Cetyltrimethylammonium bromide (CTAB), Sodium dodecyl sulfate (SDS), buccal swab DNA extraction kit (Kit method) and guanidine isothiocyanate methods were checked on agarose gels. (A) PCR products from the CTAB method. (B) PCR products from SDS method. (C) PCR products from the kit method. (D) PCR products from the guanidine isothiocyanate method. Lanes 1–3: Porcine skin, lanes 4–6: N5, lanes 7–9: N6, lanes 10–12: ID1, lanes 13–15: CNH2, lanes 16–18: CNH3, lanes 19–21: agar, lanes 22–24: vermicelli.
According to the higher copy number and faster evolutionary mutation rate of mitochondrial DNA (mtDNA) in EBN, the Cytb and ND2 genes are commonly used for genetic identification. Therefore, we explored a more suitable gene for ARMS-PCR analysis via Basic Local Alignment Search Tool (BLAST) and K2P evolutionary distance analysis. First, we evenly cut the sequences of the Cytb and ND2 gene fragments from all samples by using MEGA-X. Then, we obtained gene sequences of Cytb and ND2 with lengths of 356 and 726 bp, respectively. The BLAST results showed that the samples mainly came from two species, A. fuciphagus and A. maximus. According to the gene sequences of Cytb and ND2, samples Z1–Z3, D1–D6, X1–X3, N2–N8, N12, N14–15, N17–N21, and ID1 showed 97–100% similarity to A. fuciphagus, while samples N9, N11, N13, and N19 showed 97–100% similarity to A. maximus (Tables 5, 6). Subsequently, the results of K2P evolutionary distance analysis showed that the evolutionary distance between the Cytb sequences of the samples ranged from 0.003 to 0.050 (Figure 2). The distance between the ND2 sequences of the samples ranged from 0.001 to 2.135 (Figure 3). Samples Z1–Z3, D1–D6, X1–X3, N2–N8, N12, N14–15, N17–N21, and ID1 exhibited evolutionary distances ranging from 0 to 0.023, while samples N9, N11, N13, and N19 exhibited large evolutionary distances from other samples, which was consistent with the BLAST results.
Table 6. Basic local alignment search tool analysis results for NADH dehydrogenase subunit 2 (ND2) gene sequences.
Figure 2. Kimura 2-parameter model distance of edible bird’s nest samples according to cytochrome b (Cytb) gene sequences.
Figure 3. Kimura 2-parameter model distance of edible bird’s nest samples according to NADH dehydrogenase subunit 2 (ND2) gene sequences.
Furthermore, based on the Cytb and ND2 gene sequences, we constructed two ML trees to evaluate the evolutionary relationships among the EBNs. As shown in Figure 4, samples N9, N11, N13, and N19 were grouped with A. maximus at a confidence level of 98%, indicating that these samples almost certainly came from A. maximus. Samples N15 and N21 were grouped with A. fuciphagus and A. fuciphagus germani at a 53% confidence level. As shown in Figure 5, samples N9, N11, N13, and N19 were grouped with A. maximus at a confidence level of 66%. Samples Z1–Z3, D1–D6, X1–X3, N2–N8, N12, N14–15, N17–N21, and ID1 were grouped with A. fuciphagus and A. fuciphagus germani at a 71% confidence level, which indicates that these samples came from either A. fuciphagus or A. fuciphagus germani. The above results suggest that ND2, which contains more mutation sites than Cytb, is a more suitable gene for ARMS-PCR analysis to distinguish the genetic information of EBN.
Figure 4. Phylogenetic tree of edible bird’s nest samples based on the maximum likelihood tree analysis of cytochrome b (Cytb) sequences.
Figure 5. Phylogenetic tree of edible bird’s nest samples based on the maximum likelihood tree analysis of NADH dehydrogenase subunit 2 (ND2) sequences.
As shown in Figure 6, samples D1–3, Z1–3, X1–3, N5–8, and N14 were shown to belong to A. fuciphagus. The results suggested that the A. fuciphagus sequence could be digested into three fragments of 533, 402, and 201 bp, whereas the sequence of A. maximus did not follow the same pattern (Figure 6). In addition, compared with sample N5, samples N9, N11, N13, and N19 were identified to belong to A. maximus according to the visualization of three bands of 463, 317, and 201 bp (Figure 7).
Figure 6. Amplification-refractory mutation system polymerase chain reaction (ARMS-PCR) profile. Analysis of genomic DNA from Aerodramus fuciphagus with the Af/1-553 bp-F/R primers. Lane M: 2,000 bp marker; lane 1 to lane 4: N5–N8; lane 5: N9; lane 6: N14; lane 7: N11; lane 8: N13; lane 9: N19; lanes 10–12: D1–D3; lanes 13–15: Z1–Z3; lanes 16–18: X1–X3. Samples N9, N11, N13, and N19 are from A. maximus in Thailand.
Figure 7. Amplification-refractory mutation system polymerase chain reaction (ARMS-PCR) profile. Analysis of genomic DNA from A. maximus with the Am-ND2-463 bp-F/R, Am-ND2-A-317 bp-F and Am-ND2-G-201 bp-R primers. Lane M: 2000 bp marker; lane 1: N9; lane 2: N11; lane 3: N5; lane 4: N13; lane 5: N19; lane 6: N6. Samples N5 and N6 are from A. fuciphagus in Thailand.
Since EBN is considered a natural food product produced from swiftlet’s saliva which consist of various potential medicine value in Traditional Chinese Medicine (TCM). There is an urgent need to develop stable and efficient identification methods for EBN genetic identification. At present, sample-specific EBN genes that can be amplified and replicated by PCR are viable targets for the assessment of food quality. However, the efficient extraction and purification of DNA are vital for successful amplification (Vázquez-Novelle et al., 2005). Thus, we primarily assessed four different methods of DNA extraction. The guanidine isothiocyanate method is a rapid quantitative extraction method that does not rely on labor-intensive standard methodology (Lippke et al., 1987). Several reports have shown that the guanidine isothiocyanate method can extract proteins and RNA with significantly equivalent results (Monteiro et al., 2016; Siala et al., 2017; Joy et al., 2018). Our results also showed that the guanidine isothiocyanate method produced a clear single band in a shorter time than the other three methods (Figure 1). Therefore, we further distinguished the genetic information of EBN from Thailand by using the guanidine isothiocyanate method for DNA extraction.
Bioinformatics helps us understand complex biological problems by investigating similarities and differences that exist in polynucleic acids at the sequence level by using alignment algorithms, including dynamic programming and BLAST. BLAST is not only a sequence similarity search program but is also one of the most widely used bioinformatics research tools (Johnson et al., 2008). As shown by the BLAST, K2P distance and ML tree results, both the Cytb and ND2 genes can be used to genetically distinguish the source of EBNs (Figures 2–5 and Tables 5, 6). Furthermore, we identified 26 samples of house EBN in Thailand (Z1–Z3, D1–D6, X1–X3, N2–N8, N12, N14–15, N17–N21, and ID1) and 4 samples of cave EBN in Thailand (N9, N11, N13, and N19) belonging to A. fuciphagus and A. maximus, respectively. In accordance with the present results, previous studies have demonstrated that swiftlets producing white nest and living in swiftlet houses in Thailand should be considered members of a single panmictic population due to high gene flow between colonies and large population sizes (Aowphol et al., 2008). However, the above results based on the sequencing of Cytb and ND2 gene fragments could accurately genetically distinguish the EBNs from Thailand. This method is time consuming, has a high cost and cannot be generalized for application in the EBN market to identify EBN. On the other hand, the market value of different species differs greatly. The market values of A. germani, A. fuciphagus, A. maximus, and Apus EBNs are $46,000 per kg, $24,000 per kg, $15,000 per kg, and $10,000 per kg, respectively (Liu et al., 2020). Thus, there is an urgent need to develop simple, sensitive, accurate techniques to check the quality of EBNs.
ARMS-PCR is a simple, reliable and non-isotopic method that uses a set of 4 primers in a single PCR run to allow genotyping solely by the inspection of reaction mixtures after agarose gel electrophoresis (Newton et al., 1989; Rubio et al., 2008). The basis of ARMS-PCR is that each inner primer specifically matches one of the alleles associated with a SNP and includes a mismatch at position -2 from the 3′ terminus (Sauer et al., 2000). Recently, ARMS-PCR has been widely used to detect polymorphisms in genes such as NFKB1 ATTG, BRAF, and EGFR (T790M) (Chao et al., 2018; Li et al., 2019a, b). Thus, we further developed a novel method to distinguish the genetic information of EBN based on ARMS-PCR by using the ND2 gene, which exhibits more mutation sites than Cytb. First, we designed 2 primers located on either side of a SNP site and 2 primers with different upstream and downstream distances from the SNP site according to the ND2 gene sequences of A. fuciphagus and A. maximus. Then, we could rapidly identify EBNs derived from A. fuciphagus or A. maximus according to the size of the bands obtained from agarose gel electrophoresis. Consistent with the above results, samples D1–3, Z1–3, X1–3, N5–8, and N14 were found to belong to A. fuciphagus, for which three bands of 533, 402, and 201 bp were obtained upon agarose gel electrophoresis (Figure 6). Samples N9, N11, N13, and N19 were shown to belong to A. maximus, according to the visualization of three bands of 463, 317, and 201 bp (Figure 7). These results indicate that ARMS-PCR, a rapid, reliable and sensitive method for the genetic identification of EBNs between A. maximus and A. fuciphagus.
The purpose of the current study was to develop a rapid, reliable and sensitive method for replenishing different genetic information of EBN in Thailand to identify EBN from different species. The first major finding was that the guanidine isothiocyanate method is suitable for extracting EBN DNA. The second major finding was that 26 samples of house EBN in Thailand and 4 samples of cave EBN in Thailand belonged to A. fuciphagus and A. maximus, respectively. Finally, we found that ARMS-PCR was a rapid, reliable, and sensitive method for the genetic identification of EBN.
The datasets generated for this study can be found in online repositories. The names of the repository/repositories and accession number(s) can be found in the article/Supplementary Material.
LY and DL designed the research. YF, PL, and KL performed the study. DL and WZ analyzed the data. YF and DL wrote the manuscript. GL and XL revised the manuscript. MW, YL, and YW provided technical support. All authors contributed to the article and approved the submitted version.
This work was supported by the projects of the National Natural Science Foundation of China (81173498), the Guangdong Provincial Science and Technology Planning Project (2017A050506045), Dapeng New District Industrial Development Fund Support Project (KY20180107), and Special Innovation Project of Guangdong Provincial Education Department (2018KTSCX036).
YL was employed by the company Guangzhou Tongkang Pharmaceutical Co., Ltd.
The remaining authors declare that the research was conducted in the absence of any commercial or financial relationships that could be construed as a potential conflict of interest.
The Supplementary Material for this article can be found online at: https://www.frontiersin.org/articles/10.3389/fgene.2021.632232/full#supplementary-material
Albishtue, A. A., Yimer, N., Zakaria, M. Z. A., Haron, A. W., Babji, A. S., Abubakar, A. A., et al. (2019). Effects of EBN on embryo implantation, plasma concentrations of reproductive hormones, and uterine expressions of genes of PCNA, steroids, growth factors and their receptors in rats. Theriogenology 126, 310–319. doi: 10.1016/j.theriogenology.2018.12.026
Alyethodi, R. R., Singh, U., Kumar, S., Alex, R., Deb, R., Sengar, G. S., et al. (2018). T-ARMS PCR genotyping of SNP rs445709131 using thermostable strand displacement polymerase. BMC Res. Notes 11:132. doi: 10.1186/s13104-018-3236-6
Aowphol, A., Voris, H. K., Feldheim, K. A., Harnyuttanakorn, P., and Thirakhupt, K. (2008). Genetic homogeneity among colonies of the white-nest swiftlet (Aerodramus fuciphagus) in Thailand. Zool. Sci. 25, 372–380. doi: 10.2108/zsj.25.372
Attitalla, I. H. (2011). Modified CTAB method for high quality genomic DNA extraction from medicinal plants. Pak. J. Biol. Sci. 14, 998–999.
Careena, S., Sani, D., Tan, S. N., Lim, C. W., Hassan, S., Norhafizah, M., et al. (2018). Effect of Edible Bird’s Nest Extract on Lipopolysaccharide-Induced Impairment of Learning and Memory in Wistar Rats. Evid. Based Complem. Alternat. Med. 2018:9318789. doi: 10.1155/2018/9318789
Chao, J., Chai, L., Yin, X., Zhou, Z., Xu, S., Zhu, W., et al. (2018). Application of Single-Tube Tri-Primer ARMS-PCR to Detect the NFKB1 ATTG Insertion/Deletion Polymorphism. Genet. Test Mol. Biomarkers 22, 443–447. doi: 10.1089/gtmb.2018.0034
Chua, Y. G., Chan, S. H., Bloodworth, B. C., Li, S. F., and Leong, L. P. (2015). Identification of edible Bird’s nest with amino acid and monosaccharide analysis. J. Agric. Food Chem. 63, 279–289. doi: 10.1021/jf503157n
Dai, Y., Cao, J., Wang, Y., Chen, Y., and Jiang, L. (2020). A comprehensive review of edible bird’s nest. Food Res. Internat. 2020:109875. doi: 10.1016/j.foodres.2020.109875
Darriba, D., Taboada, G. L., Doallo, R., and Posada, D. (2012). jModelTest 2: more models, new heuristics and parallel computing. Nat. Methods 9:772. doi: 10.1038/nmeth.2109
Green, M. R., and Sambrook, J. (2018). A Single-Step Method for the Simultaneous Preparation of DNA, RNA, and Protein from Cells and Tissues. Cold Spring Harb. Protoc. 2018:rot093500. doi: 10.1101/pdb.prot093500
Guo, L., Wu, Y., Liu, M., Ge, Y., and Chen, Y. (2018). Rapid authentication of edible bird’s nest by FTIR spectroscopy combined with chemometrics. J. Sci. Food Agric. 98, 3057–3065. doi: 10.1002/jsfa.8805
Huang, X., Li, Z., Zou, X., Shi, J., Elrasheid Tahir, H., Xu, Y., et al. (2019). A low cost smart system to analyze different types of edible Bird’s nest adulteration based on colorimetric sensor array. J. Food Drug. Anal. 27, 876–886. doi: 10.1016/j.jfda.2019.06.004
Jin, C., Li, Z., Zheng, X., Shen, K., Chao, J., Dong, Y., et al. (2020). Development and validation of T-ARMS-PCR to detect CYP2C19∗17 allele. J. Clin. Lab Anal. 34:e23005. doi: 10.1002/jcla.23005
Johnson, M., Zaretskaya, I., Raytselis, Y., Merezhuk, Y., McGinnis, S., and Madden, T. L. (2008). NCBI BLAST: a better web interface. Nucleic Acids Res. 36, W5–W9. doi: 10.1093/nar/gkn201
Joy, A. P., Ayre, D. C., Chute, I. C., Beauregard, A. P., Wajnberg, G., Ghosh, A., et al. (2018). Proteome profiling of extracellular vesicles captured with the affinity peptide Vn96: comparison of Laemmli and TRIzol© protein-extraction methods. J. Extracell. Vesicles 7:1438727. doi: 10.1080/20013078.2018.1438727
Kampmann, M. L., Buchard, A., Børsting, C., and Morling, N. (2016). High-throughput sequencing of forensic genetic samples using punches of FTA cards with buccal swabs. Biotechniques 61, 149–151. doi: 10.2144/000114453
Katoh, K., and Standley, D. M. (2013). MAFFT multiple sequence alignment software version 7: improvements in performance and usability. Mol. Biol. Evol. 30, 772–780. doi: 10.1093/molbev/mst010
Lee, M. S., Huang, J. Y., Lien, Y. Y., and Sheu, S. C. (2019). The rapid and sensitive detection of edible bird’s nest (Aerodramus fuciphagus) in processed food by a loop-mediated isothermal amplification (LAMP) assay. J. Food Drug Anal. 27, 154–163. doi: 10.1016/j.jfda.2018.08.003
Lee, T., Lee, C., Nurul, A. A., Supparmaniam, K., Wong, S., Znati, M., et al. (2020). Characterization of Polar and Non-Polar Compounds of House Edible Bird’s Nest (EBN) from Johor. Malaysia. Chem. Biodivers 17:e1900419. doi: 10.1002/cbdv.201900419
Lee, T. H., Wani, W. A., Koay, Y. S., Kavita, S., Tan, E. T. T., and Shreaz, S. (2017). Recent advances in the identification and authentication methods of edible bird’s nest. Food Res. Int. 100(Pt 1), 14–27. doi: 10.1016/j.foodres.2017.07.036
Li, X., Li, E., Du, J., Wang, J., and Zheng, B. (2019a). BRAF mutation analysis by ARMS-PCR refines thyroid nodule management. Clin. Endocrinol. 91, 834–841. doi: 10.1111/cen.14079
Li, Y., Xu, Y., Wu, X., He, C., Liu, Q., and Wang, F. (2019b). Comprehensive analysis of EGFR T790M detection by ddPCR and ARMS-PCR and the effect of mutant abundance on the efficacy of osimertinib in NSCLC patients. J. Thorac. Dis. 11, 3004–3014. doi: 10.21037/jtd.2019.07.42
Lin, J.-R., Zhou, H., Lai, X.-P., Hou, Y., Xian, X.-M., Chen, J.-N., et al. (2009). Genetic identification of edible birds’ nest based on mitochondrial DNA sequences. Food Res. Int. 42, 1053–1061. doi: 10.1016/j.foodres.2009.04.014
Lippke, J. A., Strzempko, M. N., Raia, F. F., Simon, S. L., and French, C. K. (1987). Isolation of intact high-molecular-weight DNA by using guanidine isothiocyanate. Appl. Environ. Microbiol. 53, 2588–2589. doi: 10.1128/aem.53.10.2588-2589.1987
Liu, K., Wu, M., Lin, X., Lonan, P., Chen, S., Wu, Y., et al. (2020). Molecular analysis of edible bird’s nest and rapid authentication of Aerodramus fuciphagus from its subspecies by PCR-RFLP based on the cytb gene. Anal. Methods 12, 2710–2717. doi: 10.1039/c9ay02548k
Looi, Q. H., Amin, H., Aini, I., Zuki, M., and Omar, A. R. (2017). De novo transcriptome analysis shows differential expression of genes in salivary glands of edible bird’s nest producing swiftlets. BMC Genom. 18:504. doi: 10.1186/s12864-017-3861-9
Monteiro, M. B., Santos-Bezerra, D. P., Thieme, K., Passarelli, M., Machado, U. F., Lin, C. J., et al. (2016). Optimization of total RNA isolation from human urinary sediment. Clin. Chim. Acta 462, 158–161. doi: 10.1016/j.cca.2016.09.018
Newton, C. R., Graham, A., Heptinstall, L. E., Powell, S. J., Summers, C., Kalsheker, N., et al. (1989). Analysis of any point mutation in DNA. The amplification refractory mutation system (ARMS). Nucleic Acids Res. 17, 2503–2516. doi: 10.1093/nar/17.7.2503
Rubio, M., Caranta, C., and Palloix, A. (2008). Functional markers for selection of potyvirus resistance alleles at the pvr2-eIF4E locus in pepper using tetra-primer ARMS-PCR. Genome 51, 767–771. doi: 10.1139/g08-056
Sales, K., Miranda, D. E. O., da Silva, F. J., Otranto, D., Figueredo, L. A., and Dantas-Torres, F. (2020). Evaluation of different storage times and preservation methods on phlebotomine sand fly DNA concentration and purity. Parasit. Vectors 13:399. doi: 10.1186/s13071-020-04270-4
Sauer, S., Lechner, D., Berlin, K., Lehrach, H., Escary, J. L., Fox, N., et al. (2000). A novel procedure for efficient genotyping of single nucleotide polymorphisms. Nucleic Acids Res. 28:E13. doi: 10.1093/nar/28.5.e13
Shim, E. K., Chandra, G. F., and Lee, S. Y. (2017). Thermal analysis methods for the rapid identification and authentication of swiftlet (Aerodramus fuciphagus) edible bird’s nest - A mucin glycoprotein. Food Res. Int. 95, 9–18. doi: 10.1016/j.foodres.2017.02.018
Siala, M., Barbana, A., Smaoui, S., Hachicha, S., Marouane, C., Kammoun, S., et al. (2017). Screening and Detecting Salmonella in Different Food Matrices in Southern Tunisia Using a Combined Enrichment/Real-Time PCR Method: Correlation with Conventional Culture Method. Front. Microbiol. 8:2416. doi: 10.3389/fmicb.2017.02416
Vázquez-Novelle, M. D., Pazos, A. J., Abad, M., Sánchez, J. L., and Pérez-Parallé, M. L. (2005). Eight-hour PCR-based procedure for the detection of Salmonella in raw oysters. FEMS Microbiol. Lett. 243, 279–283. doi: 10.1016/j.femsle.2004.12.016
Wong, Z. C. F., Chan, G. K. L., Wu, K. Q. Y., Poon, K. K. M., Chen, Y., Dong, T. T. X., et al. (2018). Complete digestion of edible bird’s nest releases free N-acetylneuraminic acid and small peptides: an efficient method to improve functional properties. Food Funct. 9, 5139–5149. doi: 10.1039/c8fo00991k
Xia, X. (2018). DAMBE7: New and Improved Tools for Data Analysis in Molecular Biology and Evolution. Mol. Biol. Evol. 35, 1550–1552. doi: 10.1093/molbev/msy073
Xia, Y., Chen, F., Du, Y., Liu, C., Bu, G., Xin, Y., et al. (2019). A modified SDS-based DNA extraction method from raw soybean. Biosci. Rep. 39:bsr20182271. doi: 10.1042/bsr20182271
Keywords: edible bird’s nest, ARMS-PCR, ND2, genetic information, guanidinium isothiocyanate, Aerodramus fuciphagus, Aerodramus maximus
Citation: Lv D, Fan Y, Zhong W, Lonan P, Liu K, Wu M, Wu Y, Liang Y, Lai X, Li G and Yu L (2021) Genetic Identification of Edible Bird’s Nest in Thailand Based on ARMS-PCR. Front. Genet. 12:632232. doi: 10.3389/fgene.2021.632232
Received: 24 November 2020; Accepted: 12 February 2021;
Published: 08 March 2021.
Edited by:
Alison G. Nazareno, Federal University of Minas Gerais, BrazilReviewed by:
Ting Hun Lee, University of Technology Malaysia, MalaysiaCopyright © 2021 Lv, Fan, Zhong, Lonan, Liu, Wu, Wu, Liang, Lai, Li and Yu. This is an open-access article distributed under the terms of the Creative Commons Attribution License (CC BY). The use, distribution or reproduction in other forums is permitted, provided the original author(s) and the copyright owner(s) are credited and that the original publication in this journal is cited, in accordance with accepted academic practice. No use, distribution or reproduction is permitted which does not comply with these terms.
*Correspondence: Xiaoping Lai, THhwODhAZ3p1Y20uZWR1LmNu; Geng Li, bGdAZ3p1Y20uZWR1LmNu; Liangwen Yu, ZmlzaGVybWFuQGd6dWNtLmVkdS5jbg==
Disclaimer: All claims expressed in this article are solely those of the authors and do not necessarily represent those of their affiliated organizations, or those of the publisher, the editors and the reviewers. Any product that may be evaluated in this article or claim that may be made by its manufacturer is not guaranteed or endorsed by the publisher.
Research integrity at Frontiers
Learn more about the work of our research integrity team to safeguard the quality of each article we publish.