- 1Department of Reproductive Medicine, Changzheng Hospital, Second Military Medical University, Shanghai, China
- 2Department of Physiology, Second Military Medical University, Shanghai, China
- 3School of Medicine, International Peace Maternity and Child Health Hospital, Shanghai Jiao Tong University, Shanghai, China
- 4Shanghai Key Laboratory for Assisted Reproduction and Reproductive Genetics, Shanghai, China
Preeclampsia (PE) is a pregnancy-related disease defined as onset of hypertension and proteinuria after the 20th week of pregnancy, which causes most maternal and perinatal morbidity and mortality. Although placental dysfunction is considered as the main cause of PE, the exact pathogenesis of PE is not yet fully understood. Long non-coding RNAs (lncRNAs) are implicated in a broad range of physiological and pathological processes, including the occurrence of PE. In this study, we investigated the expression and functions of HIF-1α pathway–related lncRNA-HEIPP (high expression in PE placenta) in the pathogenesis of PE. The expression of lncRNA-HEIPP in the placenta from women who underwent PE was screened by lncRNA microarray and then verified using real-time polymerase chain reaction. Then, the methylation profile of the lncRNA-HEIPP promoter and the enrichment of H3K4me3 binding were assessed by bisulfite pyrosequencing and chromatin immunoprecipitation (ChIP)–quantitative polymerase chain reaction (qPCR) assay, respectively. It was found that the level of lncRNA-HEIPP in the PE placenta was significantly higher than that in normal placenta and was increased in HTR-8/SVneo human trophoblast cells upon hypoxia treatment. Moreover, we reported that H3K4me3 manifested significantly higher promoter occupancy on lncRNA-HEIPP promoter in HTR-8/SVneo cells upon hypoxia treatment and found that the downregulation of lncRNA-HEIPP promoted trophoblast invasion. Our findings suggested that the hypoxia-induced expression of lncRNA-HEIPP mediated by H3K4me3 modification in trophoblast may contribute to the pathogenesis of PE.
Introduction
Preeclampsia (PE) is a common complication of human pregnancy defined as the occurrence of hypertension and significant proteinuria after the 20th week of gestation, occurring in approximately 3 to 5% of all pregnancies and resulting in about 50,000 maternal deaths annually worldwide (Gormley et al., 2017). Preeclampsia is also the leading cause of fetal morbidity and mortality (Mayrink et al., 2018). It is characterized by maternal arterial pressure >140/90 mmHg and proteinuria >0.3 g/24 h after 20 weeks’ gestation (Armaly et al., 2018). Current studies suggested that the pathogenesis of PE is closely related to immune system and genetic dysfunctions (Hosseini et al., 2018; Yong et al., 2018). However, the dysfunction of placenta plays a central role in the pathogenesis of PE (Aouache et al., 2018; Gutierrez et al., 2019).
Recent studies have shown that abnormal trophoblast differentiation, migration, and apoptosis often cause decreased invasion of trophoblast cells and remodeling of uterine spiral artery, resulting in reduced uteroplacental perfusion (Cotechini et al., 2014; Chen and Khalil, 2017; Brosens et al., 2019). This further leads to hypoxia and inflammatory changes in the placenta, which will eventually lead to PE (Harati-Sadegh et al., 2018; Tong and Giussani, 2019). Recent studies have shown that the important phenotypes of PE, such as hypertension and proteinuria, are closely related with hypoxia inducible factor-1α (HIF-1α) (Wang et al., 2017; Alici Davutoglu et al., 2018). HIF-1α is a transcription factor that controls the expression of hypoxia-induced factors such as vascular endothelial growth factor (VEGF), platelet-derived growth factor, and so on (Kuzmanov et al., 2012; Zhang et al., 2019), which are considered to play important roles during the pathogenesis of PE. Recently, it has been found that long non-coding RNA (lncRNA) could regulate the invasion and apoptosis of trophoblast cell lines (Song et al., 2017; Xu et al., 2018), suggesting their potential roles in PE occurrence. However, whether hypoxia and HIF-1α regulate the expression of specific lncRNAs during PE has not been investigated yet. So, the objective of the current study is to investigate the differentially expressed lncRNAs in human placenta between normal pregnancy and PE and dissect the mechanisms underlying the regulation of lncRNAs by hypoxia as well as their functions in trophoblast cells migration.
Materials and Methods
Patient Samples
All patients provided written informed consent, and this study was approved by the Human Research Ethics Committee of the Shanghai Changzheng Hospital at the Second Military Medical University. Thirty PE patients (mean age of 33.20 ± 0.46 years) and 30 control patients (mean age of 32.60 ± 0.47 years) who received a cesarean section were included in this study. Preeclampsia was clinically diagnosed before cesarean. The main criteria for PE diagnosis were as follows: systolic blood pressure ≥140 mm Hg or diastolic blood pressure ≥90 mm Hg on two occasions at least 4 h apar, and proteinuria >0.3 g/24 h. The control pregnancy was defined as no medical complications and proteinuria, and maternal blood pressure <140/90 mm Hg. Clinical characteristics and detailed information of all patients enrolled in this study are summarized in Table 1. The original demographic data of enrolled patients can be found in Supplementary Table 1. The placenta specimen was resected from the middle of villous lobule, avoiding any visible blood clot or calcification. To minimize blood contamination, each piece of tissue was intensively washed with ice-cold phosphate-buffered saline and then immediately snap-frozen in liquid nitrogen after resection. Of the 30 pairs of placenta samples, four pairs were randomly selected for lncRNA and mRNA microarray analysis, and the other samples were used for the following verification.
RNA Extraction
Total RNA was extracted from snap-frozen placenta tissues obtained from PE placenta patients and control patients using TRIzol Reagent (Invitrogen, Carlsbad, CA, United States) according to the manufacturer’s instruction. The RNA integrity and concentration were evaluated using the NanoDrop ND-1000 spectrophotometer and 2100 RNA Nano 6000 Assay Kit (Agilent Technologies, Santa Clara, CA, United States).
RNA Microarray and Computational Analysis
RNA with RNA integrity number greater than 6.5 purified from total RNA following the removal of rRNA was amplified and transcribed into fluorescent cDNA along the entire length of the transcripts without 3′ bias utilizing random priming method, and cDNA was labeled and hybridized to the Human LncRNA Array V2.0 (8 × 60, Arraystar). In addition, 30,215 coding transcripts were detected using the microarray as well. The microarray was performed by KangCheng Bio-tech (Shanghai, China). The arrays were scanned using the Agilent Scanner G2505B (Agilent Technologies), and the acquired array images were analyzed using Agilent Feature Extraction software (version 11.5.1; Agilent Technologies). Quantile normalization and subsequent data processing were performed using GeneSpring GX v11.5.1 software package (Agilent Technologies).
Gene Ontology Pathway Analysis
To investigate the roles and associated pathways of differentially expressed lncRNAs and mRNAs, Gene Ontology (GO) pathway analysis was performed to annotate the transcripts with terms under the biological process, cellular component, and molecular function ontologies. GO annotations of microarray genes were downloaded from NCBI1, UniProt2, and the GO3. A Fisher exact test was performed in order to locate the significant enrichment pathway. The resulting P-values were adjusted using the Benjamini–Hochberg false discovery rate (BH FDR) algorithm. Pathway categories with a FDR < 0.05 were reported.
Quantitative Reverse Transcription Real-Time Polymerase Chain Reaction
Total RNA (2 μg) was reverse transcribed with random hexamers using the Reverse Transcription System Kit (Promega Corporation, Madison, WI). LncRNA expression in placenta tissues was quantified using a standard quantitative reverse transcription real-time PCR (qRT-PCR) on the StepOne Plus system (Applied Biosystems, Foster City, CA, United States), in a total reaction volume of 20 μL, including 10 μL SYBR Premix Ex Taq (2x), 1 μL of PCR Forward Primer (10 uM), 1 μL of PCR Reverse Primer (10 uM), 2 μL of cDNA, and 6 μL of double-distilled water. Primer sets are listed in Supplementary Table 2. The qRT-PCR was performed with an initial denaturation step of 10 min at 95°C; 95°C (15 s), 60°C (30 s), 72°C (30 s) for a total 40 cycles; and a final extension step at 72°C for 5 min. All experiments were performed in triplicate. All samples were normalized to GAPDH. The geometric mean in each triplicate was used to calculate the relative lncRNAs concentrations (ΔCt = Ct of lncRNAs − Ct of GAPDH). The fold change of expression was calculated using the 2–Δ Δ Ct method.
Cell Line and Hypoxia Treatment
The immortalized EVT cell line HTR-8/SVneo was used. The cells were maintained in RPMI-1640 supplemented with 5% fetal calf serum, 1% L-glutamine 200 mM, and 1% penicillin–streptomycin (all from Invitrogen) under an atmosphere of 5% CO2 at 37°C. To establish the hypoxia model, the cells were exposed to 94%N2/1% O2/5% CO2 for 2, 24, 48, and 72 h. The control group was cultured under normal air condition for the corresponding period.
HIF-1α Overexpression in HTR-8/SVneo Cells
The coding sequence of human HIF-1α was amplified by PCR from human genomic DNA libraries (GENECHEM Company, Shanghai, China) and was subcloned into the pEZ-MO2 plasmid via homologous recombination according to manufacturer’s protocol (Hieff Clone® Plus One Step Cloning Kit, YEASEN, Shanghai, China) to construct pEZ-MO2–HIF-1α overexpression. The sequence of the construct was confirmed by DNA sequencing at Sangon Biotech Company (Shanghai, China). The HTR-8/SVneo cells at 70% confluence were transiently transfected with pEZ-MO2-HIF-1α plasmid for 48 h using Lipofectamine 3000 (Invitrogen) according to manufacturer’s instructions. The empty pEZ-MO2 plasmid was also transfected as negative control (NC).
Small Interfering RNA Transfection
lncRNA-HEIPP in HTR-8/SVneo cells were knocked down by transfection of preannealed stealth small interfering RNA (siRNA) duplexes designed online by Thermo Fisher’s BLOCK-iTTM RNAi Designer4, using Lipofectamine RNAiMAX transfection reagent (Invitrogen). Silencer® Negative Control #1 siRNA (si-NC, AM4611, Ambion) was transfected as an NC. The efficiency of knockdown was determined by RT-PCR.
Cell Counting Kit-8 Cell Proliferation Assay
Cell viability was assessed using the Cell Counting Kit-8 (Dojindo Laboratories, Kumamoto, Japan) according to the manufacturer’s protocol. Cell proliferation curves were plotted using the absorbance at each time point (0, 12, 24, and 48 h). All experiments were performed in triplicate.
Matrigel Invasion Assay
The invasion of HTR-8/SVneo cells across Matrigel was objectively evaluated in an invasion chamber according to the manufacturer’s protocol (Millipore). The cells transfected with si-NC or si-HEIPP (10 and 30 μL) were plated in the upper chambers of 24-well plates at a density of 1.0 × 104 cells/well in RPMI-1640 medium containing 5% fetal bovine serum; the membrane pore of the Transwell chamber was 8 μm in diameter. The membranes were coated with Matrigel (BD, United States) to form matrix barriers at the concentration of 200 μg/mL. A 700-μL aliquot of RPMI-1640 medium containing 10% FBS was immediately placed in the lower well of the chamber as a chemoattractant. After incubation for 48 h at 37°C, cells were completely removed from the upper surface of the filter using a sterile cotton swab, and cells that had migrated to the lower surface were fixed and stained with 0.1% crystal violet. Finally, the cell migration ability was determined by counting the number of stained cells on the membranes in 10 randomly selected, non-overlapping fields at 20× magnification under microscope. The migration index was calculated as the ratio of the percentage of cell migration in the various treatments to that of the vehicle. Each experiment was performed in triplicate and repeated three times.
Bisulfite Pyrosequencing
Bisulfite pyrosequencing was used to quantity DNA methylation at two sites in the DMR of the lncRNA-HEIPP gene. Two micrograms of DNA was subjected to bisulfate conversion using the EpiTect Bisulfite Kit (59104) (Qiagen Ltd., GmbH, Hilden, Germany). Pyrosequencing primers were designed using PyroMark Assay Design 2.0 (Qiagen Ltd., GmbH, Hilden, Germany) and listed in Supplementary Table 2. One hundred nanograms of bisulfite-converted DNA was PCR amplified in a 50-μL reaction volume containing 5 × PCR buffer (KAPA Ltd., United States), 10 mM of each dNTP (KAPA Ltd., United States), 50 mM forward and reverse primers (BGI Inc., Shanghai, China), and 1 U DNA polymerase (HotStart Taq, KAPA Ltd). The PCR cycling parameters were 95°C for 3 min; 40 cycles of 95°C for 30 s, a variable annealing temperature (Ta) for 30 s, and 72°C for 1 min; and extension at 72°C for 7 min. A 25-μL aliquot of each PCR product was subjected to pyrosequencing using the PyroMark Q96 ID Pyrosequencer (Qiagen Ltd.) following the manufacturer’s recommended protocols. The degree of methylation at each CpG site was determined using Pyro Q-CpG Quantification software. All samples were analyzed in triplicate.
ChIP Assay
The HTR-8 trophoblast cell line was used for ChIP-based analysis for enrichment of H3K4me3 on lncRNA-HEIPP promoter. ChIP assays were conducted according to the manufacturer’s instruction (Millipore, 17-10086). The chromatin extracted from cells was sheared, subjected to immunoprecipitation with H3K4me3 (Abcam) or immunoglobulin G (IgG) (Millipore) antibodies, reverse cross linked, and subjected to quantitative ChIP- PCR (qChIP). The qChIP was performed on sheared DNA with following primers: SP1: F: 5′ ATTTTGCTTCC TATCCCT 3′, R:5′ ACCGACAATCTCCTCAGT 3′, SP2: F: 5′ GA CTGAGGAGATTGTCGGT 3, R: 5′ CTTATGATGGTCTGGGT GA 3′, SP3: F: 5′ TGGAGGAGGGAGATGACA 3′, R: 5′ TGGA GGAGGGAGATGACA 3′, SP4: F: 5′ CATGCCCTCTCAGC CTAAC 3′, R: 5′ AGTCCCCCCAAGAAAAACA 3′. The four pairs of primers amplified −324 to −1,288 bp region upstream from the transcriptional starting site of lncRNA-HEIPP. The results were normalized to input and expressed as a percentage of the fold difference. IgG was used as an NC. Data were obtained from at least three independent experiments.
Statistical Analysis
All data are expressed as mean ± SD (standard deviation), and all statistical analyses were performed using the SPSS statistical software package (SPSS, Inc., Chicago, IL, United States). All the data were tested for homogeneity of variance by Bartlett test before analyzing the significance. Comparisons were made using Student t-test and analysis of variance. P < 0.05 was considered statistically significant.
Results
Differential Expression of lncRNAs in Placenta Between PE and Normal Patients
The demographic data of PE women and normal pregnant women are summarized in Table 1. There was no significant difference in maternal age between normal pregnancies and pregnancies with PE (P > 0.05). The systolic and diastolic blood pressure was significantly higher in pregnant women with PE (P < 0.001). The average proteinuria is 2.62 ± 0.20 g/24 h, which means that the cases enrolled this study were severe PE patients (proteinuria >2.0 g/24 h). Women from the PE group delivered earlier than normal pregnant women mostly due to the medical termination of the pregnancy, which is the major cause of the lower infant birth weight in the PE group, as there was no intrauterine growth restriction patient in the PE group enrolled in this study.
As the transcriptome of the placenta is dynamically changed during advancing gestation (Deyssenroth et al., 2017), to ensure the identical or similar baselines between the two disease groups, we selected four pairs of PE patients and controls with matched gestational age within 2 weeks (Supplementary Table 1). We aligned lncRNA array data into RefSeq_NR, UCSC, and Ensembl database and compared the lncRNA expression levels between four human PE placenta (P) and four normal samples (N). The expression profiles of lncRNAs were shown by calculating the log-fold change (P/N). Among these lncRNAs, 405 were consistently upregulated, and 344 lncRNAs were consistently downregulated (Figure 1A). The clustering analysis also showed the differential expression patterns of mRNAs between PE placentas and normal samples (Figure 1B). The complete dataset regarding the significantly upregulated and downregulated lncRNA or mRNA was deposit on the public repository5.
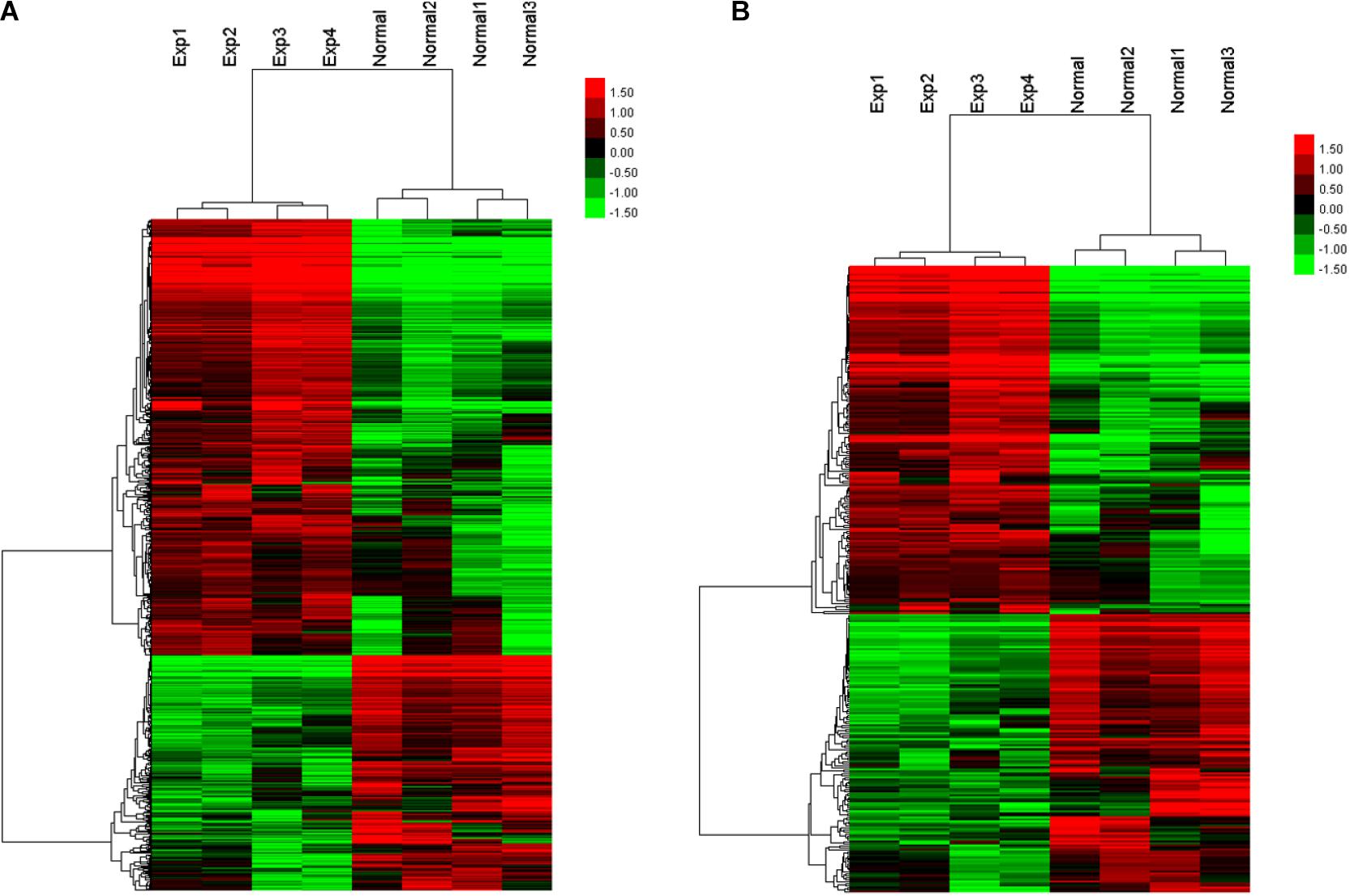
Figure 1. Heatmap and hierarchical clustering of lncRNA expression (A) and mRNA expression (B) between the preeclampsia placenta (Exp) and normal samples (Normal).
GO Pathway Analysis
GO pathway analysis was performed to determine the lncRNA and mRNA enrichment in biological processes, cellular components, and molecular functions. HIF-1α pathway is known to play an important role in the pathogenesis of PE and identified as one of the most significant enrichment signaling pathways under our experimental settings (Figure 2A and Supplementary Table 3). We found that a number of lncRNAs and molecules are closely related with HIF-1α pathway (Figure 2B), and the top 10 lncRNAs (AF064860.7, AC027612.3, RP11-244N9.4, RP11-574M7.1, RP11-80I15.4, RP11-939C17.2, XLOC_013276, AC023085.1, AC009236.1, and RP11-184D12.1) showing mostly dramatic different expression between PE and normal placentas were selected for further validation (Supplementary Table 4).
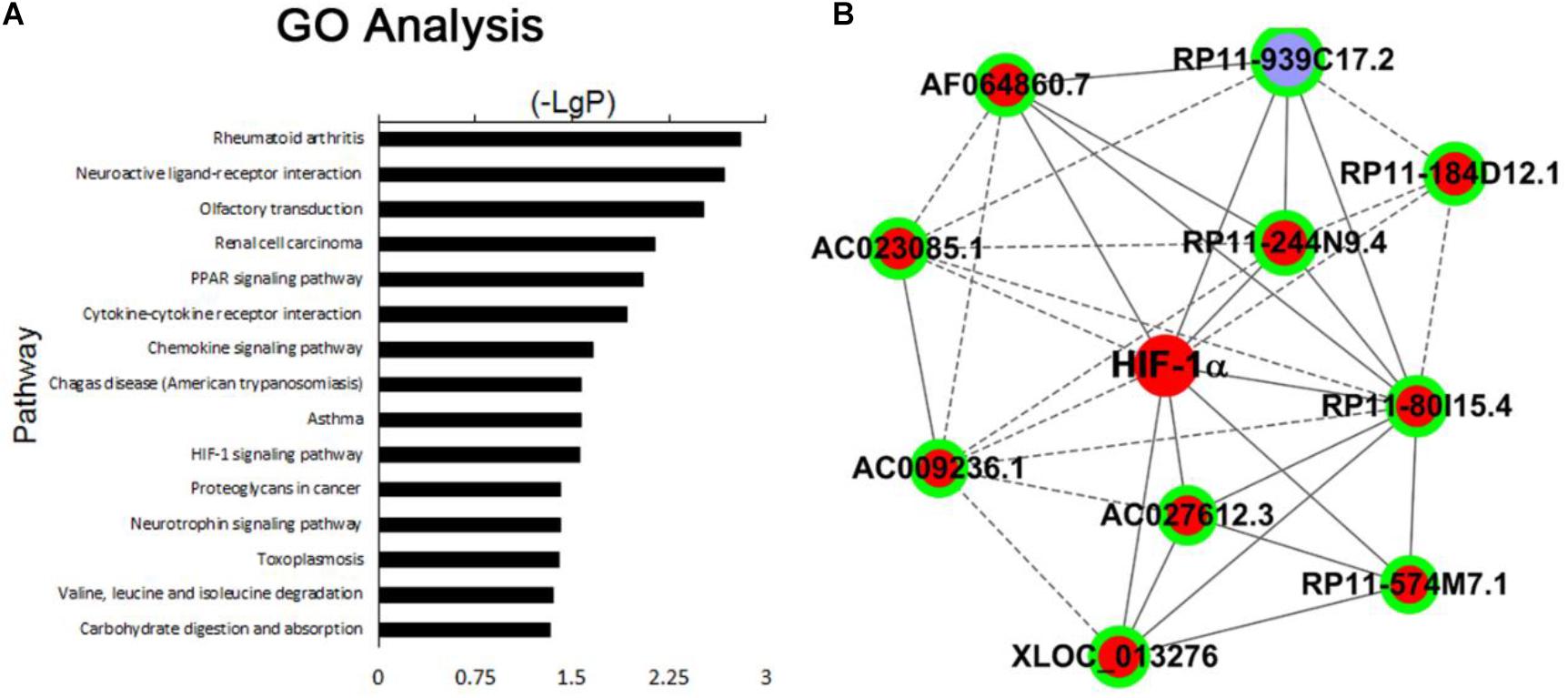
Figure 2. (A) The top 15 signaling pathways that were most significantly enriched in PE placenta compared with normal placenta. (B) Top 10 lncRNAs related with HIF-1α pathway: AF064860.7, AC027612.3, RP11-244N9.4, RP11-574M7.1, RP11-80I15.4, RP11-939C17.2, XLOC_013276, AC023085.1, AC009236.1, and RP11-184D12.1.
Validation of Differentially Expressed lncRNAs
To further validate the preliminary data from microarray, we performed qRT-PCR to determine the expression of the ten candidate lncRNAs related with HIF-1α pathway in 30 PE placenta tissues and 30 normal placenta tissues. We found that 6 of the 10 lncRNAs manifested significant upregulation in PE samples compared with normal samples (Figure 3A), which are consistent with the array results. Among the six lncRNAs, lncRNA-AF064860.7 showed the most significant differences between PE samples and normal samples, so that we named it lncRNA-HEIPP (high expression in PE placenta).
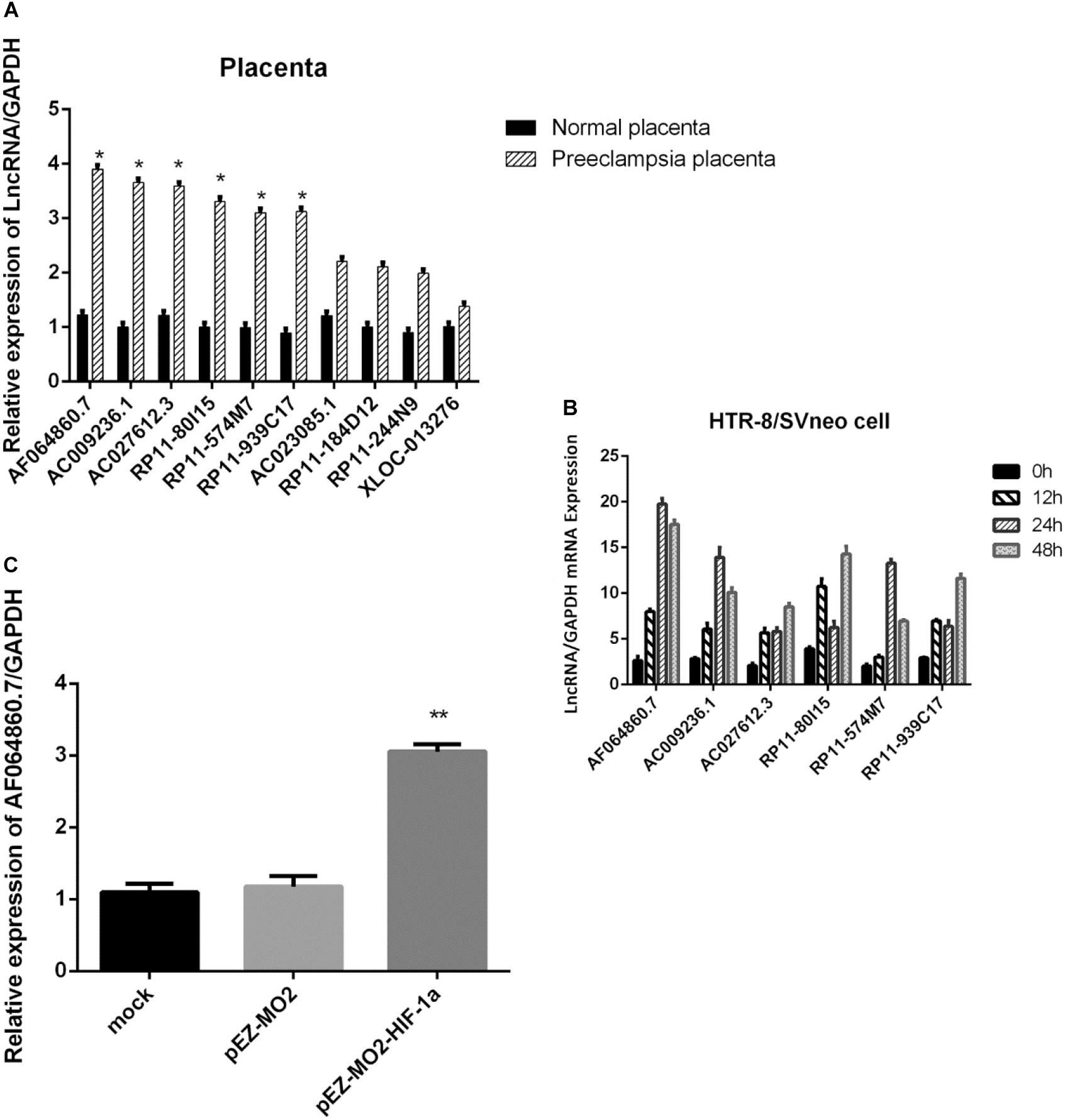
Figure 3. (A) Verification of 10 lncRNAs related with HIF-1a pathway in 30 preeclampsia placenta tissues and 30 normal placenta tissues using qPCR. (B) Time-dependent changes of the six candidate lncRNA expression upon hypoxia treatment in HTR-8/SVneo cells. (C) The overexpression of HIF-1α increased the expression of lncRNA-AF064860.7 (lncRNA-HEIPP) in HTR-8/SVneo cells. *p < 0.05, **p < 0.01.
In the HTR-8/SVneo human trophoblast cell lines, we found that hypoxia could induce the expression of lncRNA-HEIPP, which reached the highest level at 24 h upon hypoxia treatment (Figure 3B). Although the other five candidate lncRNAs also showed time-dependent changes of expression upon hypoxia treatment, the increasing trend and fold change were less significant than that in lncRNA-HEIPP. Moreover, the overexpression of HIF-1α increased the expression of lncRNA-HEIPP, further suggesting the interaction between HIF-1α pathway and lncRNA-HEIPP (Figure 3C).
Hypoxia Did Not Change the Methylation Profile of lncRNA-HEIPP Promoter in Placental Trophoblast Cells
As the epigenetic regulatory elements such as DNA methylation and H3K4me3 occupancy were critical for the gene expression regulation in placental development and trophoblast invasion (Apicella et al., 2019; Kwak et al., 2019), we analyzed the epigenetic regulatory elements of lncRNA-HEIPP in the placenta in PE versus controls. Specifically, compared to the normal oxygen condition, the DNA methylation levels of lncRNA-HEIPP promoter in HTR-8/SVneo human trophoblast cells increased along with the extension of hypoxia time, which reached the highest level at 24 h upon hypoxia treatment (Figure 4A). However, the increase of methylation level was not significant at each time point (Figure 4B).
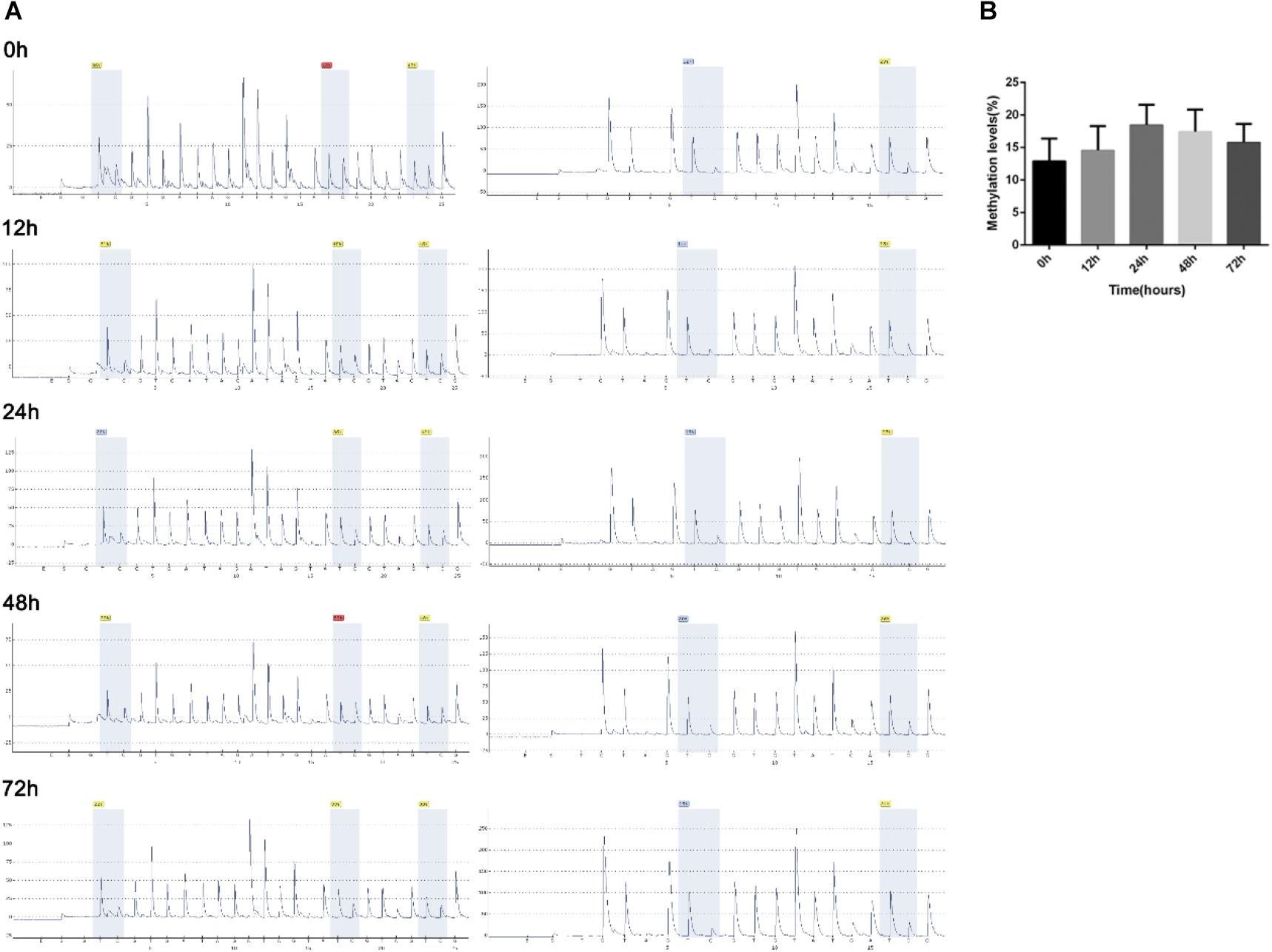
Figure 4. Methylation levels of lncRNA-HEIPP promoter in HTR-8/SVneo human trophoblast cells that underwent hypoxia. (A) The degree of methylation at each CpG site of lncRNA-HEIPP promoter between normoxia (left panel) and hypoxia (right panel) treatment at different time point. (B) The statistical graph showing the changes of methylation level of lncRNA-HEIPP promoter in HTR-8/SVneo human trophoblast cells that underwent hypoxia at different time point. n = 3, and all samples were analyzed in triplicate.
Binding of H3K4me3 in lncRNA-HEIPP Promoter Was Increased by Hypoxia Treatment
Enrichment of histone trimethylation at lysine 4 (H3K4me3) at lncRNA-HEIPP DMRs/promoters in HTR-8/SVneo human trophoblast cells that underwent hypoxia or normoxia was assessed using ChIP-qPCR. It was shown that the binding of H3K4me3 on lncRNA-HEIPP promoter was specifically enriched compared with the binding of IgG (Figure 5A). As shown in Figure 5B, the protein expression of H3K4me3 was not affected by hypoxia treatment. However, H3K4me3 manifested significantly higher promoter occupancy on lncRNA-HEIPP promoter in HTR-8/SVneo cells that underwent hypoxia for 24 h compared to those that underwent normoxia (Figure 5C), suggesting the histone modification, instead of the DNA methylation might be the epigenetic pathway that causes the increased expression of lncRNA-HEIPP in the placenta during PE undergone hypoxia.
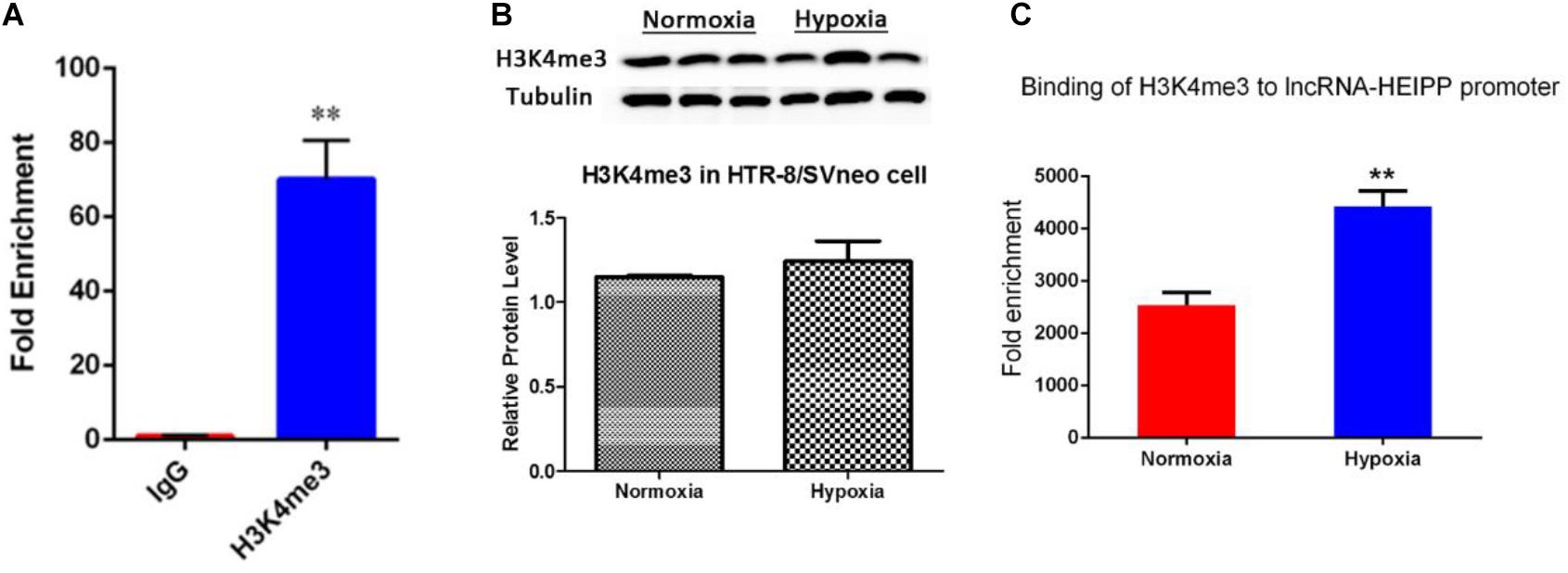
Figure 5. (A) ChIP analysis detected the enrichment of H3K4me3 binding on lncRNA-HEIPP promoter. (B) The protein expression of H3K4me3 in HTR-8/SVneo cells that underwent hypoxia and normoxia. (C) The changes of H3K4me3 occupancy on lncRNA-HEIPP promoter in HTR-8/SVneo cells that underwent hypoxia compared to those that underwent normoxia, **p < 0.01, n = 3.
Downregulation of lncRNA-HEIPP Promoted Trophoblast Invasion
Next, we assessed the biological functions of lncRNA-HEIPP in trophoblast cells. We knocked down the expression of lncRNA-HEIPP in HTR-8/SVneo human trophoblast cell line using HEIPP siRNA (si-HEIPP) and found that the proliferation of HTR8/SVneo cells was not affected (data not shown). However, the invasion of HTR8/SVneo cells was significantly promoted, compared to the cells treated with NC siRNA (Figure 6).
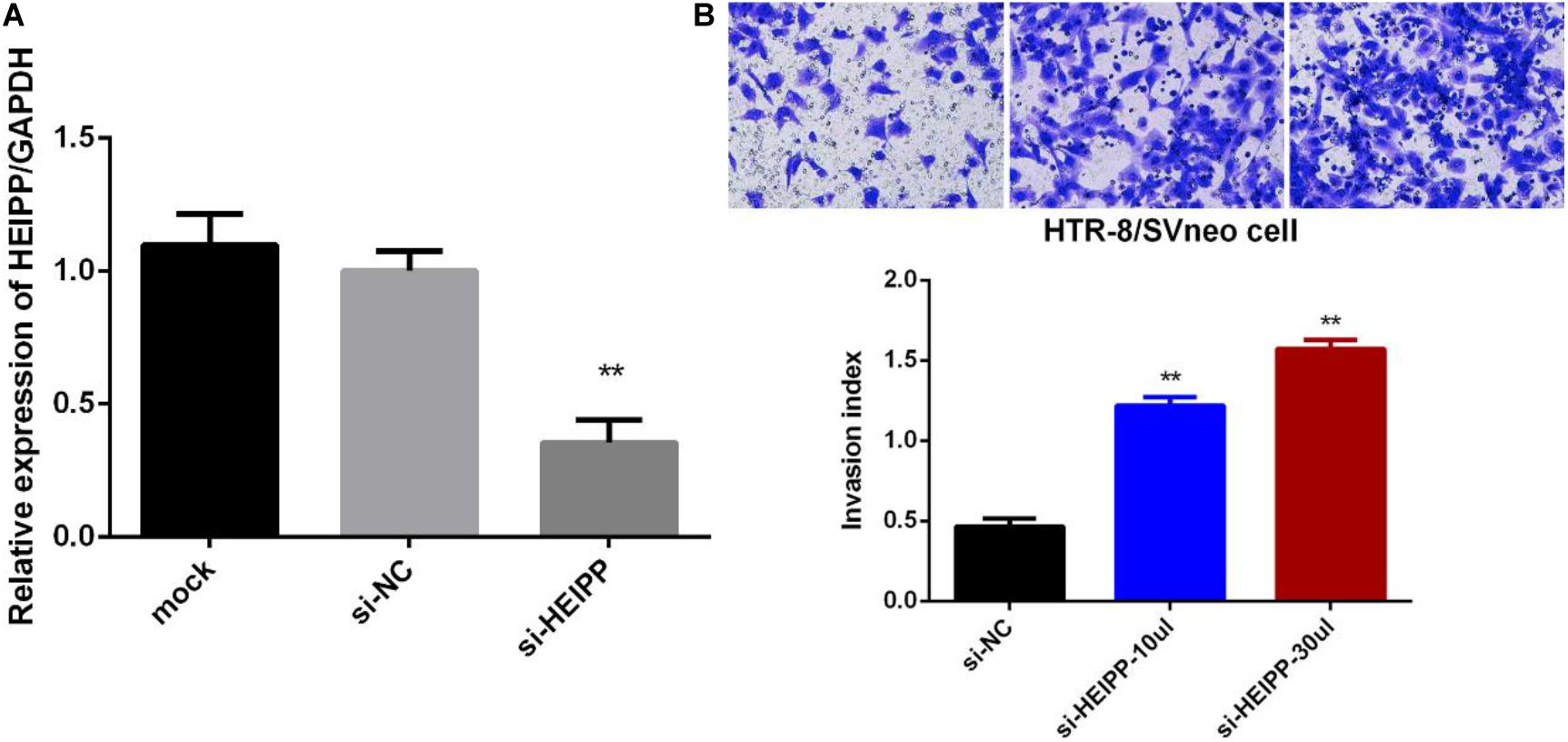
Figure 6. (A) Transfection of si-HEIPP significantly knocked down the expression of lncRNA-HEIPP. (B) Downregulation of lncRNA-HEIPP promoted HTR8/SVneo trophoblast cells invasion, **p < 0.01.
Discussion
Preeclampsia is a unique disease during pregnancy. It is a placental syndrome characterized by high blood pressure, edema, and proteinuria after 20 weeks of pregnancy (Gormley et al., 2017; Mayrink et al., 2018). Preeclampsia is the leading cause of perinatal and maternal death. At present, the PE incidence rate is 5–8% throughout the world and is 9.4% in China (Boriboonhirunsarn et al., 2017). Current studies strongly suggested that placenta play central roles in the pathogenesis of PE (Kawasaki et al., 2019). The proliferation and invasion of placental trophoblast cells are the key to successful pregnancy and embryonic development (Knofler and Pollheimer, 2013). The earlier study found that abnormal trophoblast differentiation, migration, and apoptosis often caused the decrease in the invasion of trophoblast cells and the failure of uterine spiral artery recasting, resulting in reduced uteroplacental perfusion and subsequently leading to PE (Kadyrov et al., 2006). However, the factors that affect placental trophoblast cells and the pathogenesis of PE have not been fully elucidated.
In recent years, epigenetic regulation has been revealed to play important roles in the embryonic development and the pathogenesis in many diseases. LncRNAs are a class of transcripts whose lengths exceed 200 nt. Initially, lncRNAs were thought to be transcribed noise. However, an increasing number of studies have reported that these lncRNAs have a series of important functions and participate in the development of many diseases, such as cancer (Liu et al., 2016), cardiovascular illnesses (Leung et al., 2013; Wang et al., 2016), and neurological illnesses (Jiang et al., 2016). Recently, some lncRNAs have been shown to be associated with PE (He et al., 2013; Chen et al., 2015; Zhang et al., 2015). However, their related pathways and regulation were not fully revealed yet. In our current study, we performed lncRNA microarray and found that 1,051 lncRNAs were upregulated, and 344 lncRNAs were downregulated in PE placenta compared with normal placenta, which is comparable to the microarray results from previous study that 738 differently expressed lncRNAs in the placentas of PE in comparison with normal pregnancies (He et al., 2013), but is much less than another study in which 15,646 upregulated and 13,178 downregulated lncRNAs were identified in the placenta tissues of early-onset PE patients (Long et al., 2016). However, in the latter study, the tissues were collected from early-onset PE patients, and the controls were preterm birth, and the biased factor, i.e., preterm labor, may partially explain the huge differences in the numbers of differentially expressed lncRNAs between studies.
The decreased trophoblast proliferation, migration, invasion, and stimulated apoptosis constitute the pivotal reasons leading to PE (Fu et al., 2013). Previous studies revealed different lncRNAs that affect cellular functions of trophoblast cells (Cao et al., 2017; Song et al., 2017; Jiao et al., 2018; Li et al., 2018). However, the hypoxia and HIF-1α–induced pathways play central roles in the pathogenesis of PE (Korkes et al., 2017; Harati-Sadegh et al., 2018; Tong and Giussani, 2019), whereas no HIF-1α–related lncRNAs have yet been revealed in the previous studies. In the current study, we first showed that lncRNAs related with HIF-1α pathway were significantly increased in PE placenta compared to normal placenta, among which lncRNA-HEIPP manifested most significant and time-dependent upregulation upon hypoxia treatment. In tumors, it was found that microRNAs and lncRNAs are important in enabling the key hypoxia-regulated processes (Ferdin et al., 2013; Choudhry et al., 2016). In placenta, some microRNAs, such as microRNA-218 and microRNA-18b, were shown to be induced by hypoxia and HIF-1a in PE patients (Fang et al., 2017; Wang et al., 2017). To our knowledge, lncRNA-HEIPP is the first lncRNA that was found to be upregulated by HIF-1a in placental tissues of PE patients. Moreover, Wang et al. (2018) also found that some lncRNAs, such as NONHSAT116812 and NONHSAT145880, were also confirmed in plasma specimens, making them to be potentially utilized as indicators for PE. Whether lncRNA-HEIPP can also be detected in plasma and used as novel indicators for PE warranted further confirmation.
In recent years, epigenetic regulation of placental gene expression has been considered to play an important role in human trophoblast differentiation (Gamage et al., 2018) and contribute to the onset of PE (Chelbi and Vaiman, 2008; Leavey et al., 2018). The studies showed higher expression levels of death domain-associated protein 6 (DAXX), MMP9, and BCL2 in placenta from PE tissue, and this increased expression was well correlated to promoter demethylation (Wang et al., 2010; Novakovic et al., 2017; Mohammadpour-Gharehbagh et al., 2019). However, a significant number of the observed methylation changes were not associated with corresponding changes in gene expression, and vice versa (Gamage et al., 2018; Leavey et al., 2018), indicating that alternate methods of epigenetic regulation will need to be explored in PE. Hypoxia was shown to alter the epigenetic profile in cultured human placental trophoblasts (Yuen et al., 2013). In this study, the DNA methylation levels of lncRNA-HEIPP promoter manifested increase upon hypoxia treatment, but the increases showed no significance at each time point after hypoxia. However, it was shown that the H3K4me3 occupancy of lncRNA-HEIPP promoter was significantly enriched in the PE placenta compared to the normal placenta. H3K4me3 is a histone modification marker that usually activates the gene transcription upon its binding to the promoter regions (Sati et al., 2012; Zhang et al., 2018; Fanucchi et al., 2019). These results suggested that the histone modification, instead of the DNA methylation, might be the epigenetic pathway that causes the increased expression of lncRNA-HEIPP in the placenta during PE.
Furthermore, we demonstrated that lncRNA-HEIPP knockdown promoted the invasion of trophoblasts. LncRNAs seem to contribute to PE via different pathways. LncRNA PRNCR1 promoted the progression of eclampsia by regulating the MAPK signal pathway (Jiao et al., 2018), while LncRNA CCAT1 promotes the progression of PE by regulating CDK4 (Li et al., 2018). As hypoxia usually induced the activation of JAK/STAT3 signaling pathway to promote trophoblast cell viability and angiogenesis in PE (Xu et al., 2017), whether the cellular functions of lncRNA-HEIPP were also mediated by JAK/STAT3 signaling pathway needs to be further investigated.
Conclusion
Our findings suggest that HIF-1a–related lncRNA-HEIPP is upregulated in the placenta from women who underwent PE mediated by the enriched binding of H3K4me3 in lncRNA-HEIPP promoter. LncRNA-HEIPP hampers the invasion of trophoblast cells and contributes to the pathogenesis of PE. Our results demonstrated that HIF-1a pathway–related lncRNA-HEIPP expression profile is a potentially new biomarker, and the manipulation of lncRNA-HEIPP may provide theoretical basis for prevention and treatment of PE.
Data Availability Statement
The microarray data has been uploaded to the GEO dataset and the accession number is GSE160888.
Ethics Statement
The studies involving human participants were reviewed and approved by Human Research Ethics Committee of the Shanghai Changzheng Hospital at the Second Military Medical University. The patients/participants provided their written informed consent to participate in this study.
Author Contributions
NS, HC, and YM: validation, formal analysis, and investigation. YM: writing – original draft. WP: methodology and investigation. XW and QZ: validation. LG: conceptualization, writing – review and editing, project administration, and funding acquisition. WL: conceptualization, supervision, and funding acquisition. All authors contributed to the article and approved the submitted version.
Funding
This work was supported by the National Key Research and Development Project Nos. 2018YFC1002802 (WL) and 2017YFC1001404 (LG and WL), National Natural Science Foundation of China Nos. 81901482 (NS), 81622020 and 81771608 (LG), and 81873821 (WL), Program of Shanghai Academic/Technology Research Leader No. 18XD1405100 (LG), “Dawn” Program of Shanghai Education Commission No. 17SG36 (LG), Program of Shanghai Subject Chief Scientist No. 2017BR017 (LG), and the Program for Professor of Special Appointment (Eastern Scholar) at Shanghai Institutions of Higher Learning (LG).
Conflict of Interest
The authors declare that the research was conducted in the absence of any commercial or financial relationships that could be construed as a potential conflict of interest.
Supplementary Material
The Supplementary Material for this article can be found online at: https://www.frontiersin.org/articles/10.3389/fgene.2020.559478/full#supplementary-material
Footnotes
- ^ http://www.ncbi.nlm.nih.gov/
- ^ http://www.uniprot.org/
- ^ http://www.geneontology.org/
- ^ http://rnaidesigner.thermofisher.com/rnaiexpress/rnaiDesign.jsp
- ^ http://doi.org/10.5281/zenodo.4039476
References
Alici Davutoglu, E., Akkaya Firat, A., Ozel, A., Yilmaz, N., Uzun, I., Temel Yuksel, I., et al. (2018). Evaluation of maternal serum hypoxia inducible factor-1alpha, progranulin and syndecan-1 levels in pregnancies with early- and late-onset preeclampsia. J. Maternal-fetal Neonatal Med. 31, 1976–1982. doi: 10.1080/14767058.2017.1333098
Aouache, R., Biquard, L., Vaiman, D., and Miralles, F. (2018). Oxidative stress in preeclampsia and placental diseases. Int. J. Mol. Sci. 19:1496. doi: 10.3390/ijms19051496
Apicella, C., Ruano, C. S. M., Mehats, C., Miralles, F., and Vaiman, D. (2019). The role of epigenetics in placental development and the etiology of preeclampsia. Int. J. Mol. Sci. 20:2837. doi: 10.3390/ijms20112837
Armaly, Z., Jadaon, J. E., Jabbour, A., and Abassi, Z. A. (2018). Preeclampsia: novel mechanisms and potential therapeutic approaches. Front. Physiol. 9:973. doi: 10.3389/fphys.2018.00973
Boriboonhirunsarn, D., Pradyachaipimol, A., and Viriyapak, B. (2017). Incidence of superimposed preeclampsia among pregnant Asian women with chronic hypertension. Hypertens. Pregnancy 36, 226–231. doi: 10.1080/10641955.2017.1311340
Brosens, I., Puttemans, P., and Benagiano, G. (2019). Placental bed research: i. the placental bed: from spiral arteries remodeling to the great obstetrical syndromes. Am. J. Obstetr. Gynecol. 221, 437–456. doi: 10.1016/j.ajog.2019.05.044
Cao, C., Li, J., Li, J., Liu, L., Cheng, X., and Jia, R. (2017). Long non-coding RNA Uc.187 is upregulated in preeclampsia and modulates proliferation, apoptosis, and invasion of HTR-8/SVneo trophoblast cells. J. Cell. Biochem. 118, 1462–1470. doi: 10.1002/jcb.25805
Chelbi, S. T., and Vaiman, D. (2008). Genetic and epigenetic factors contribute to the onset of preeclampsia. Mol. Cell. Endocrinol. 282, 120–129. doi: 10.1016/j.mce.2007.11.022
Chen, H., Meng, T., Liu, X., Sun, M., Tong, C., Liu, J., et al. (2015). Long non-coding RNA MALAT-1 is downregulated in preeclampsia and regulates proliferation, apoptosis, migration and invasion of JEG-3 trophoblast cells. Int. J. Clin. Exp. Pathol. 8, 12718–12727.
Chen, J., and Khalil, R. A. (2017). Matrix metalloproteinases in normal pregnancy and preeclampsia. Prog. Mol. Biol. Transl. Sci. 148, 87–165. doi: 10.1016/bs.pmbts.2017.04.001
Choudhry, H., Harris, A. L., and McIntyre, A. (2016). The tumour hypoxia induced non-coding transcriptome. Mol. Aspects Med. 4, 35–53. doi: 10.1016/j.mam.2016.01.003
Cotechini, T., Komisarenko, M., Sperou, A., Macdonald-Goodfellow, S., Adams, M. A., and Graham, C. H. (2014). Inflammation in rat pregnancy inhibits spiral artery remodeling leading to fetal growth restriction and features of preeclampsia. J. Exp. Med. 211, 165–179. doi: 10.1084/jem.20130295
Deyssenroth, M. A., Peng, S., Hao, K., Lambertini, L., Marsit, C. J., and Chen, J. (2017). Whole-transcriptome analysis delineates the human placenta gene network and its associations with fetal growth. BMC Genom. 18:520. doi: 10.1186/s12864-017-3878-0
Fang, M., Du, H., Han, B., Xia, G., Shi, X., Zhang, F., et al. (2017). Hypoxia-inducible microRNA-218 inhibits trophoblast invasion by targeting LASP1: implications for preeclampsia development. Int. J. Biochem. Cell Biol. 87, 95–103. doi: 10.1016/j.biocel.2017.04.005
Fanucchi, S., Fok, E. T., Dalla, E., Shibayama, Y., Borner, K., Chang, E. Y., et al. (2019). Immune genes are primed for robust transcription by proximal long noncoding RNAs located in nuclear compartments. Nat. Genet. 51, 138–150. doi: 10.1038/s41588-018-0298-2
Ferdin, J., Nishida, N., Wu, X., Nicoloso, M. S., Shah, M. Y., Devlin, C., et al. (2013). HINCUTs in cancer: hypoxia-induced noncoding ultraconserved transcripts. Cell Death. Differ. 20, 1675–1687. doi: 10.1038/cdd.2013.119
Fu, G., Ye, G., Nadeem, L., Ji, L., Manchanda, T., Wang, Y., et al. (2013). MicroRNA-376c impairs transforming growth factor-beta and nodal signaling to promote trophoblast cell proliferation and invasion. Hypertension 61, 864–872. doi: 10.1161/hypertensionaha.111.203489
Gamage, T., Schierding, W., Hurley, D., Tsai, P., Ludgate, J. L., Bhoothpur, C., et al. (2018). The role of DNA methylation in human trophoblast differentiation. Epigenetics 13, 1154–1173. doi: 10.1080/15592294.2018.1549462
Gormley, M., Ona, K., Kapidzic, M., Garrido-Gomez, T., Zdravkovic, T., and Fisher, S. J. (2017). Preeclampsia: novel insights from global RNA profiling of trophoblast subpopulations. Am. J. Obstet. Gynecol. 217, 200.e1–200.e17.
Gutierrez, J. A., Gomez, I., Chiarello, D. I., Salsoso, R., Klein, A. D., Guzman-Gutierrez, E., et al. (2019). Role of proteases in dysfunctional placental vascular remodelling in preeclampsia. Biochimica et Biophysica Acta. Mol. Basis Dis. 1866:165448. doi: 10.1016/j.bbadis.2019.04.004
Harati-Sadegh, M., Kohan, L., Teimoori, B., Mehrabani, M., and Salimi, S. (2018). The association of the placental Hypoxia-inducible factor1-alpha polymorphisms and HIF1-alpha mRNA expression with preeclampsia. Placenta 67, 31–37. doi: 10.1016/j.placenta.2018.05.005
He, X., He, Y., Xi, B., Zheng, J., Zeng, X., Cai, Q., et al. (2013). LncRNAs expression in preeclampsia placenta reveals the potential role of LncRNAs contributing to preeclampsia pathogenesis. PLoS One 8:e81437. doi: 10.1371/journal.pone.0081437
Hosseini, A., Dolati, S., Hashemi, V., Abdollahpour-Alitappeh, M., and Yousefi, M. (2018). Regulatory T and T helper 17 cells: their roles in preeclampsia. J. Cell. Physiol. 233, 6561–6573. doi: 10.1002/jcp.26604
Jiang, Q., Shan, K., Qun-Wang, X., Zhou, R. M., Yang, H., Liu, C., et al. (2016). Long non-coding RNA-MIAT promotes neurovascular remodeling in the eye and brain. Oncotarget 7, 49688–49698. doi: 10.18632/oncotarget.10434
Jiao, S., Wang, S. Y., and Huang, Y. (2018). LncRNA PRNCR1 promoted the progression of eclampsia by regulating the MAPK signal pathway. Eur. Rev. Med. Pharmacol. Sci. 22, 3635–3642.
Kadyrov, M., Kingdom, J. C., and Huppertz, B. (2006). Divergent trophoblast invasion and apoptosis in placental bed spiral arteries from pregnancies complicated by maternal anemia and early-onset preeclampsia/intrauterine growth restriction. Am. J. Obstet. Gynecol. 194, 557–563. doi: 10.1016/j.ajog.2005.07.035
Kawasaki, K., Kondoh, E., Chigusa, Y., Kawamura, Y., Mogami, H., Takeda, S., et al. (2019). Metabolomic profiles of placenta in preeclampsia. Hypertension 73, 671–679. doi: 10.1161/hypertensionaha.118.12389
Knofler, M., and Pollheimer, J. (2013). Human placental trophoblast invasion and differentiation: a particular focus on Wnt signaling. Front. Genet. 4:190. doi: 10.3389/fgene.2013.00190
Korkes, H. A., De Oliveira, L., Sass, N., Salahuddin, S., Karumanchi, S. A., and Rajakumar, A. (2017). Relationship between hypoxia and downstream pathogenic pathways in preeclampsia. Hypertens. Pregnancy 36, 145–150. doi: 10.1080/10641955.2016.1259627
Kuzmanov, A., Wielockx, B., Rezaei, M., Kettelhake, A., and Breier, G. (2012). Overexpression of factor inhibiting HIF-1 enhances vessel maturation and tumor growth via platelet-derived growth factor-C. Int. J. Cancer 131, E603–E613.
Kwak, Y. T., Muralimanoharan, S., Gogate, A. A., and Mendelson, C. R. (2019). Human trophoblast differentiation is associated with profound gene regulatory and epigenetic changes. Endocrinology 160, 2189–2203. doi: 10.1210/en.2019-00144
Leavey, K., Wilson, S. L., Bainbridge, S. A., Robinson, W. P., and Cox, B. J. (2018). Epigenetic regulation of placental gene expression in transcriptional subtypes of preeclampsia. Clin. Epigenet. 10:28.
Leung, A., Trac, C., Jin, W., Lanting, L., Akbany, A., Saetrom, P., et al. (2013). Novel long noncoding RNAs are regulated by angiotensin II in vascular smooth muscle cells. Circ. Res. 113, 266–278. doi: 10.1161/circresaha.112.300849
Li, J. L., Li, R., Gao, Y., Guo, W. C., Shi, P. X., and Li, M. (2018). LncRNA CCAT1 promotes the progression of preeclampsia by regulating CDK4. Eur. Rev. Med. Pharmacol. Sci. 22, 1216–1223.
Liu, D., Yu, X., Wang, S., Dai, E., Jiang, L., Wang, J., et al. (2016). The gain and loss of long noncoding RNA associated-competing endogenous RNAs in prostate cancer. Oncotarget 7, 57228–57238. doi: 10.18632/oncotarget.11128
Long, W., Rui, C., Song, X., Dai, X., Xue, X., Lu, Y., et al. (2016). Distinct expression profiles of lncRNAs between early-onset preeclampsia and preterm controls. Clin. Chimica Acta; Int. J. Clin. Chem. 463, 193–199. doi: 10.1016/j.cca.2016.10.036
Mayrink, J., Costa, M. L., and Cecatti, J. G. (2018). Preeclampsia in 2018: revisiting concepts, physiopathology, and prediction. Sci. World J. 2018:6268276.
Mohammadpour-Gharehbagh, A., Jahantigh, D., Eskandari, M., Sadegh, M. H., Nematollahi, M. H., Rezaei, M., et al. (2019). Genetic and epigenetic analysis of the BAX and BCL2 in the placenta of pregnant women complicated by preeclampsia. Apoptosis : Int. J. Program. Cell Death 24, 301–311. doi: 10.1007/s10495-018-1501-8
Novakovic, B., Evain-Brion, D., Murthi, P., Fournier, T., and Saffery, R. (2017). Variable DAXX gene methylation is a common feature of placental trophoblast differentiation, preeclampsia, and response to hypoxia. FASEB J. 31, 2380–2392. doi: 10.1096/fj.201601189rr
Sati, S., Ghosh, S., Jain, V., Scaria, V., and Sengupta, S. (2012). Genome-wide analysis reveals distinct patterns of epigenetic features in long non-coding RNA loci. Nucleic Acids Res. 40, 10018–10031. doi: 10.1093/nar/gks776
Song, X., Rui, C., Meng, L., Zhang, R., Shen, R., Ding, H., et al. (2017). Long non-coding RNA RPAIN regulates the invasion and apoptosis of trophoblast cell lines via complement protein C1q. Oncotarget 8, 7637–7646. doi: 10.18632/oncotarget.13826
Tong, W., and Giussani, D. A. (2019). Preeclampsia link to gestational hypoxia. J. Dev. Origins Health Dis. 10, 322–333. doi: 10.1017/s204017441900014x
Wang, S., Wang, X., Weng, Z., Zhang, S., Ning, H., and Li, B. (2017). Expression and role of microRNA 18b and hypoxia inducible factor-1alpha in placental tissues of preeclampsia patients. Exp. Therapeutic Med. 14, 4554–4560.
Wang, X., Chen, Y., Du, L., Li, X., Li, X., and Chen, D. (2018). Evaluation of circulating placenta-related long noncoding RNAs as potential biomarkers for preeclampsia. Exp. Therapeutic Med. 15, 4309–4317.
Wang, Y. N., Shan, K., Yao, M. D., Yao, J., Wang, J. J., Li, X., et al. (2016). Long Noncoding RNA-GAS5: a novel regulator of hypertension-induced vascular remodeling. Hypertension 68, 736–748. doi: 10.1161/hypertensionaha.116.07259
Wang, Z., Lu, S., Liu, C., Zhao, B., Pei, K., Tian, L., et al. (2010). Expressional and epigenetic alterations of placental matrix metalloproteinase 9 in preeclampsia. Gynecol. Endocrinol. 26, 96–102. doi: 10.3109/09513590903184100
Xu, C., Li, X., Guo, P., and Wang, J. (2017). Hypoxia-Induced activation of JAK/STAT3 signaling pathway promotes trophoblast cell viability and angiogenesis in preeclampsia. Med. Sci. Monitor : Int. Med. J. Exp. Clin. Res. 23, 4909–4917. doi: 10.12659/msm.905418
Xu, Y., Lian, Y., Zhang, Y., Huang, S., Zuo, Q., Yang, N., et al. (2018). The long non-coding RNA PVT1 represses ANGPTL4 transcription through binding with EZH2 in trophoblast cell. J. Cell Mol. Med. 22, 1272–1282.
Yong, H. E. J., Murthi, P., Brennecke, S. P., and Moses, E. K. (2018). Genetic approaches in preeclampsia. Methods Mol. Biol. 1710, 53–72. doi: 10.1007/978-1-4939-7498-6_5
Yuen, R. K., Chen, B., Blair, J. D., Robinson, W. P., and Nelson, D. M. (2013). Hypoxia alters the epigenetic profile in cultured human placental trophoblasts. Epigenetics 8, 192–202. doi: 10.4161/epi.23400
Zhang, E., He, X., Zhang, C., Su, J., Lu, X., Si, X., et al. (2018). A novel long noncoding RNA HOXC-AS3 mediates tumorigenesis of gastric cancer by binding to YBX1. Genome Biol. 19:154.
Zhang, Y., Zhao, H. J., Xia, X. R., Diao, F. Y., Ma, X., Wang, J., et al. (2019). Hypoxia-induced and HIF1alpha-VEGF-mediated tight junction dysfunction in choriocarcinoma cells: implications for preeclampsia. Clinica Chimica Acta; Int. J. Clin. Chem. 489, 203–211. doi: 10.1016/j.cca.2017.12.010
Keywords: lncRNA, placenta, preeclampsia, hypoxia, H3K4me3
Citation: Sun N, Chen H, Ma Y, Pang W, Wang X, Zhang Q, Gao L and Li W (2020) H3K4me3-Mediated Upregulation of LncRNA-HEIPP in Preeclampsia Placenta Affects Invasion of Trophoblast Cells. Front. Genet. 11:559478. doi: 10.3389/fgene.2020.559478
Received: 06 May 2020; Accepted: 16 November 2020;
Published: 11 December 2020.
Edited by:
Geng Chen, East China Normal University, ChinaReviewed by:
Guodong Fu, University of Toronto, CanadaTian Fuju, Shanghai Jiao Tong University School of Medicine, China
Copyright © 2020 Sun, Chen, Ma, Pang, Wang, Zhang, Gao and Li. This is an open-access article distributed under the terms of the Creative Commons Attribution License (CC BY). The use, distribution or reproduction in other forums is permitted, provided the original author(s) and the copyright owner(s) are credited and that the original publication in this journal is cited, in accordance with accepted academic practice. No use, distribution or reproduction is permitted which does not comply with these terms.
*Correspondence: Lu Gao, bHUuZ2FvQHNtbXUuZWR1LmNu; Wen Li, bGl3ZW5Ac21tdS5lZHUuY24=
†These authors have contributed equally to this work