- 1Simon F.S. Li Marine Science Laboratory, State Key Laboratory of Agrobiotechnology, School of Life Sciences, The Chinese University of Hong Kong, Hong Kong, China
- 2Department of Biology, Queen’s University, Kingston, ON, Canada
- 3Department of Cell and Systems Biology, University of Toronto, Toronto, ON, Canada
Insects are arguably the most successful group of animals in the world in terms of both species numbers and diverse habitats. The sesquiterpenoids juvenile hormone, methyl farnesoate, and farnesoic acid are well known to regulate metamorphosis, reproduction, sexual dimorphism, eusociality, and defense in insects. Nevertheless, different insects have evolved with different sesquiterpenoid biosynthetic pathway as well as products. On the other hand, non-coding RNAs such as microRNAs have been implicated in regulation of many important biological processes, and have recently been explored in the regulation of sesquiterpenoid production. In this review, we summarize the latest findings on the diversity of sesquiterpenoids reported in different groups of insects, as well as the recent advancements in the understanding of regulation of sesquiterpenoid production by microRNAs.
Diverse Biosynthetic Pathways and Types of Insect Sesquiterpenoids
In insects and crustaceans, sesquiterpenoid hormones including farnesoic acid (FA), methyl farnesoate (MF) and juvenile hormone (JH) regulate the development, metamorphosis and reproduction (Cheong et al., 2015). The beginning step in the biosynthesis of the sesquiterpenoids starts from acetyl-CoA which goes through the universal eukaryotic mevalonate (MVA) pathway to synthesize farnesyl pyrophosphate (FPP) (Tobe and Bendena, 1999; Belles et al., 2005; Hui et al., 2010, 2013). In the presence of FPP pyrophosphatase, FPP is then converted to farnesol and can further generate farnesal with the catalyzation by farnesol dehydrogenase. Farnesoic acid (FA) will then be generated via further dehydrogenation with farnesal dehydrogenase in different insects. A summary of the sesquiterpenoid biosynthetic pathway is shown in Figure 1.
Despite all insects utilizing a common biosynthetic pathway in the production of FA, diverse pathways have evolved in the downstream process of sesquiterpenoids production. For insects in the order blattodea, coleoptera, diptera, and orthoptera, esterification of FA occurs in the corpora allata (CA), which will form MF catalyzed by a SAM-dependent juvenile hormone acid O-methyltransferase (JHAMT) (Shinoda and Itoyama, 2003). In insects such as cockroaches (Huang et al., 2015), honeybees (Bomtorin et al., 2014), locusts (Marchal et al., 2011), and pea aphids (Daimon and Shinoda, 2013), MF is oxidized by epoxidase CYP15A1 in formation of JH-III (Figure 1). Direct applications of FA on fruit flies increased the biosynthesis of MF and JH-III in both larval and adult stages, while JHB3 biosynthesis is inhibited in larvae (Bendena et al., 2011). Moreover, diverse biosynthetic pathways for production of JH-III have also been identified in other insects (Figure 1). For instance, in the coleopterans such as beetles, CYP15A1 can first oxidize FA to form JH-III acid, followed by methylation with JHAMT resulting in the formation of JH-III (Minakuchi et al., 2015; Jiang et al., 2017); while in lepidopterans, the conversion of FA to JH-III acid is performed with another epoxidase CYP15C1 followed by subsequent methylation by JHAMT (Daimon et al., 2012; Figure 1). Furthermore, different sesquiterpenoid products have also been identified in various types of insects (Figure 1 and Table 1). In the dipterans including flies, JH-III bisepoxide (JHB3) has been identified (Richard et al., 1989). In the hemipterans like the stinkbugs, JH-III skipped bisepoxide (JHSB3) is formed (Kotaki et al., 2009); and in the lepidopterans such as moths, specific JH homologs including JH-I, JH-II, JH-0, and 4-methyl JH-I are produced (Belles et al., 2005; Figure 1 and Table 1). It is worth mentioning that JH-I is found in the male accessory glands of the cecropia moth, and whether it performs the suspected hormonal function remains unknown (Paroulek and Sláma, 2014; De Loof and Schoofs, 2019).
Diverse Roles of Sesquiterpenoids in Insects
Regulation of Metamorphosis
A special feature of insects is that they have evolved with distinct modes of metamorphosis, including hemimetaboly (incomplete) and holometaboly (complete) (Sehnal et al., 1996). These biological events are collectively controlled by sesquiterpenoids that inhibit metamorphosis, and ecdysteroids such as 20-hydroxyecysone (20E) that trigger metamorphosis (Konopova et al., 2011; Liu et al., 2018; Niwa and Niwa, 2014a,b). In general, sesquiterpenoid inhibits ecdysteroids action, and when their biosynthesis in the CA is suppressed via the inhibition of JHAMT and 3-hydroxy-3-methylglutaryl Coenzyme-A reductase (HMGR), metamorphosis can then occur (Cheong et al., 2015; Liu et al., 2018; Qu et al., 2018). An overview is shown in Figure 2.
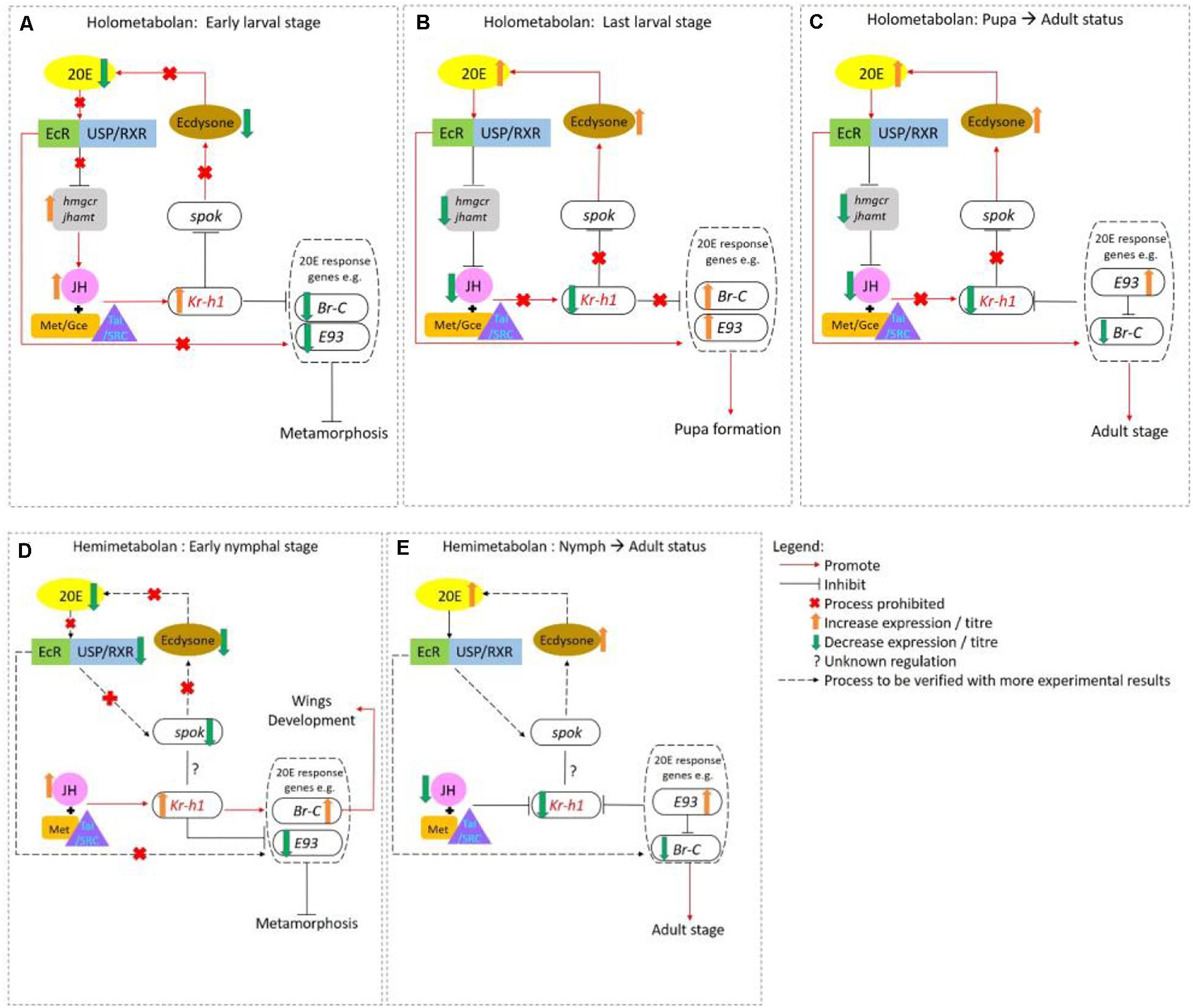
Figure 2. Interaction of sesquiterpenoid juvenile hormone (JH) and ecdysteroid during metamorphosis in holometabolans (A–C) and hemimetabolans (D,E). (A) In early larval stages of holometabolous insects, JH-Met/Tai receptor complex activates the transcription of primary JH-early responsive gene Kr-h1 which prevents immature larvae from precocious larval development by inhibiting Br-C, E93, and Spok expression. (B) When JH levels drastically drop in the last larval stage, 20E acts through EcR/USP to activate the transcription of 20E-early responsive genes such as Br-C, E93, E74, E75, Ftz-f1 and initiate larval-pupal transition. (C) At the end of the pupal stage, the Br-C levels decline again which upregulates the expression of E93 that drives the pupa-adult transition. In hemimetabolous insect, JH titer remains high from hatching until the last nymphal stage. (D) During the early nymphal, high Kr-h1 expression level is maintained by JH which inhibits metamorphosis by repressing E93 expression. (E) In the last nymphal stage, the JH titers fall followed by the Kr-h1 expression level. For details, please refer to main text and Truman, 2019
In the best studied holometabolous insect, the fly Drosophila melanogaster, sesquiterpenoids exert status quo function to prevent metamorphosis in the early larval stage (Cheong et al., 2015; Qu et al., 2018). Sesquiterpenoids JH-III, JHB3, and their immediate precursor MF can all bind to the C-terminal of the intracellular receptor Methoprene-tolerant (Met) or its paralog named Germ-cell expressed (Gce) in Drosophila, which encodes a transcription factor of the bHLH-PAS family (Ashok et al., 1988; Jindra et al., 2015; Wen et al., 2015). The binding affinities of sesquiterpenoids to Gce are differ with a rank order of JH-III > JHB3 > MF which is in line with their developmental potency (Bittova et al., 2019). After the binding of JH with Met or Gce in formation of a functional complex, another bHLH-PAS protein that acts as the steroid receptor co-activator [Taiman (Tai)] in D. melanogaster or SRC in other insect species is recruited, which together binds to the specific JH response element (JHRE) on the promoter region of Krüppel homolog 1 (Kr-h1) to activate transcription (Kayukawa et al., 2012; Qu et al., 2018). Previous studies have demonstrated that Kr-h1 can transduce the JH signal to repress 20E primary responsive genes, including ecdysone receptor (EcR), Broad-complex (Br-C), ecdysone-inducible proteins E75 and E93, which subsequently inhibit 20E biosynthesis in the prothoracic gland (Kayukawa et al., 2016; Liu et al., 2018); and can also inhibit the expression of steroidogenic enzyme gene Spok by binding to the Kr-h1 binding site (KBS) and turn on the methylation which in turns also leads to the suppression of ecdysone biosynthesis (Song and Zhou, 2019; Zhang T. et al., 2018; Figures 2, 3).
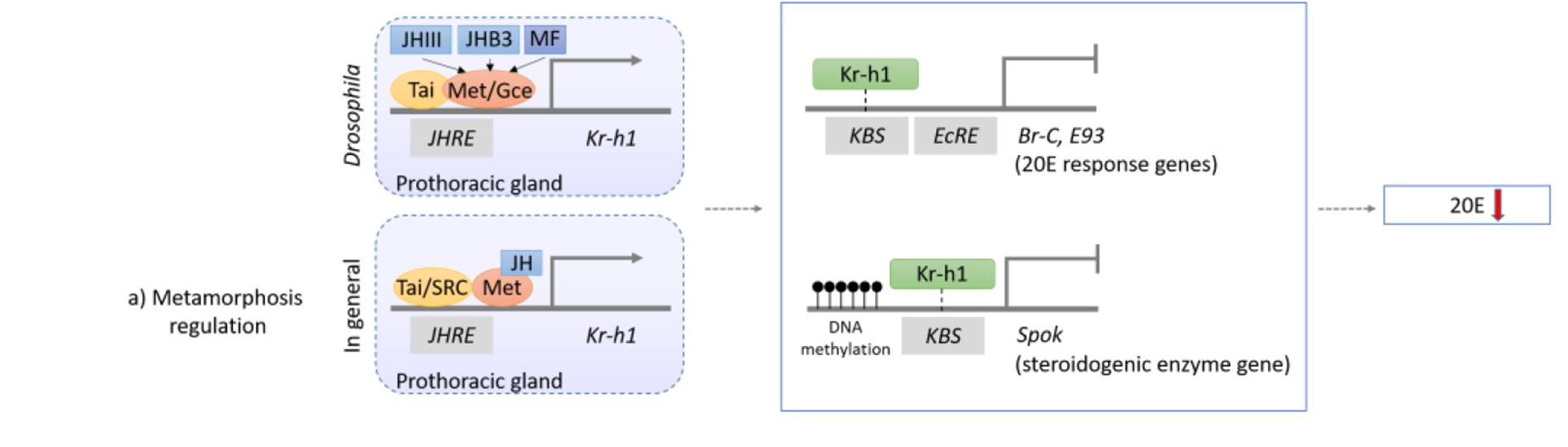
Figure 3. Schematic diagram showing the mechanism of sesquiterpenoids in metamorphosis regulation in Drosophila and other insects. In fly Drosophila, JH-III, JHB3, and MF will bind to the JH receptor Met or Gce, while in other insects, JH-III will bind to Met in other insects (for details, please refer to text). The complex will then further dimerize with Tai and bind to specific JHRE to initiate the expression of Kr-h1. Kr-h1 protein will then bind to the KBS to inhibit expressions of 20E response genes (Br-C and E93), and will also bind to KBS and initiates DNA methylation of a steroidogenic enzyme gene Spok, which will all result in the lower titer of 20E and inhibition of metamorphosis.
In other holometabolous insects including beetle Tribolium castaneum, moths Bombyx mori and Helicoverpa armigera, as well as hemimetabolous insects including cockroach Blattella germanica, planthopper Nilaparvata lugens, and stinkbug Pyrrhocoris apterus and Rhodnius prolixus, Kr-h1 has also exhibited anti-metamorphic effects (Minakuchi et al., 2009; Konopova et al., 2011; Lozano and Belles, 2011; Kayukawa et al., 2017; Li et al., 2018; Zhang W. N. et al., 2018).
During the larval-pupal transition in Drosophila, 20E binds to EcR proteins and Ultraspiracle (Usp) to form a heterodimer (Riddiford et al., 2000), and this complex will further trigger the transcription of 20E primary-response genes including Br-C, E74, E75, and E93. These downstream genes have been identified with essential functions in molting. For instances, E93 enables the larval tissues to execute apoptosis and promotes the formation of adult tissues (Ureña et al., 2016); and the Gce/Tai (but not Met/Tai) complex activates E75A functions in preimaginal molts (Dubrovsky et al., 2011). In beetle T. castaneum, Met has also proven to bind JH with high affinity via the highly conserved hydrophobic pocket within its PAS-B domain (Charles et al., 2011). In lepidopteran, USP can also bind JH (Dubrovsky, 2005). In moth Manduca, JP29 isolated from epidermis has also been suggested as another potential JH receptor, which has found to be highly specific to JH binding but with low affinity (Truman and Riddiford, 2002).
Regulation of Reproduction
Apart from repressing metamorphosis in insects, sesquiterpenoids also play an important role in stimulating reproduction in adult insects, including processes such as vitellogenesis, oogenesis and polyploidization (Wyatt and Davey, 1996). In female Drosophila, sesquiterpenoids have long been known to regulate the oogenesis and vitellogenesis (Postlethwait and Weiser, 1973; Swevers et al., 2005; Riddiford, 2012). The titer of JH is promoted with expression of ecdysis triggering hormone (ETH) binding to its receptor (ETHR) whose synthesis is governed by 20E (Meiselman et al., 2017; Roy et al., 2018).
Similar but diverse mechanisms have also been discovered in other insects. In the beetle T. castaneum, JH-mediated Met and Kr-h1 promote vitellogenin (Vg) synthesis in the fat body (Parthasarathy et al., 2010; Figure 4Ai), and Met can also trigger insulin-like peptides (ILPs) ILP2 and ILP3 by AKT pathway to phosphorylate the fork head transcription factor (FOXO) and induce Vg expression (Sheng et al., 2011; Figure 4Aii). In mosquito Aedes aegypti, expression of Kr-h1 triggered by Met together with Cycle and steroid receptor coactivator SRC/FISC after adult emergence supported that sesquiterpenoid is essential for previtellogenic development (Zhu et al., 2010; Shin et al., 2012). In migratory locust Locusta migratoria, JH together with Met/SRC complex are found to be pivotal in maintaining Vg expression and oocyte development (Song et al., 2014), and can promote cell polyploidization by regulating the expression of cyclin-dependent kinase 6 (Cdk6) and adenovirus E2 factor-1 (E2f1) (Wu et al., 2016; Wu Z. et al., 2018; Figure 4Aiii). JH activates Na+/K+-ATPase for the induction of patency in vitellogenic follicular epithelium, where Vg can then reach the surface of maturing oocyte (Jing et al., 2018). In the stinkbug P. apterus, nevertheless, Vg synthesis is mainly regulated by JH signaling genes Met and Tai independent of Kr-h1 (Smykal et al., 2014).
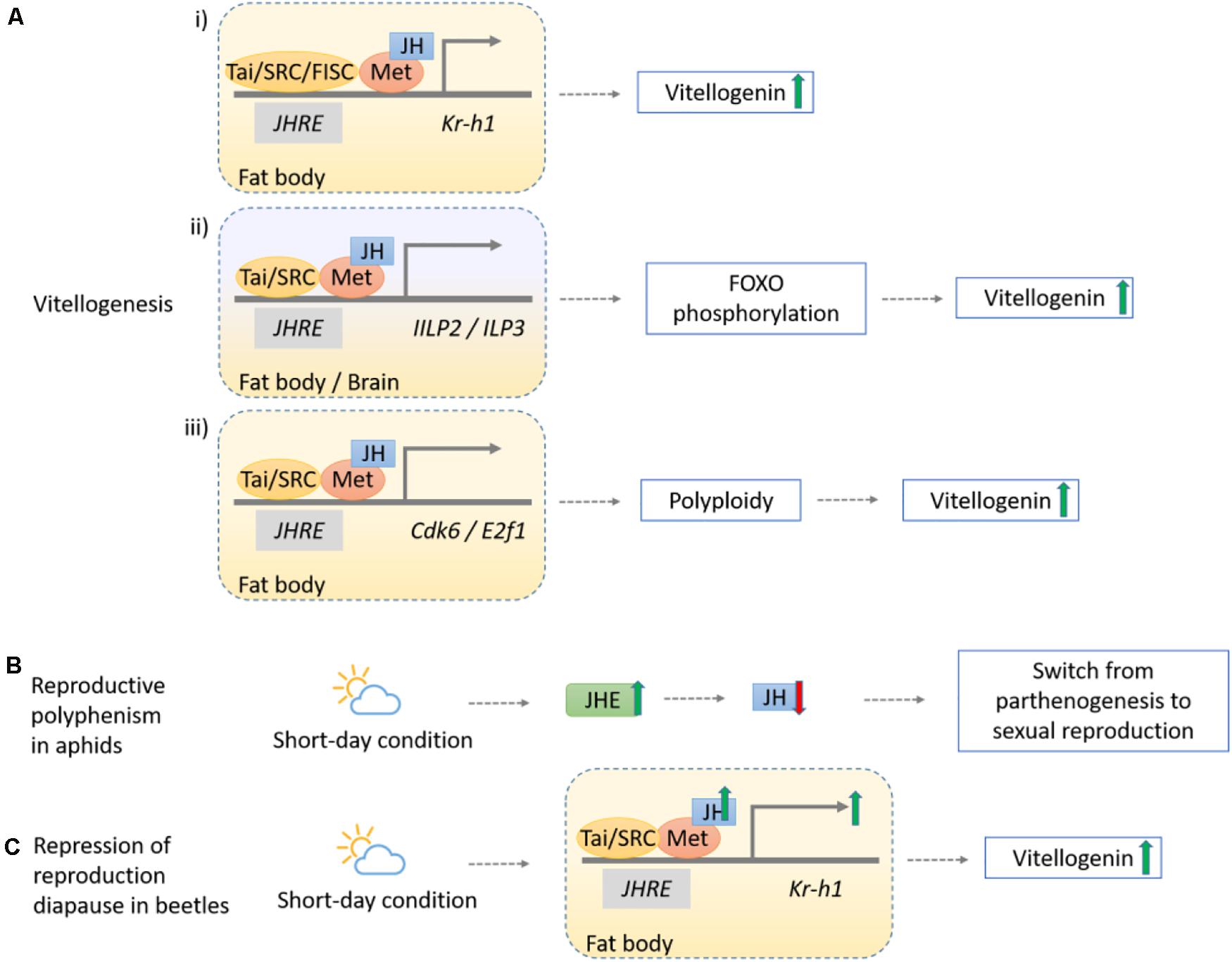
Figure 4. Schematic diagram showing the mechanisms of sesquiterpenoids in regulation of insect reproduction. (Ai) The JH-Met-Tai/SRC complex upregulates Kr-h1 to increase Vg synthesis level, as observed in T. castaneum, A. aegypti (with an additional complex FISC), L. migratoria but not in P. apterus. (Aii) The JH-Met-Tai complex initiates transcriptions of ILP2 and ILP3, which phosphorylates the fork head transcription factor (FOXO) through ILP signaling pathway and induces Vg expression in T. castaneum. (Aiii) The JH-Met-Tai/SRC complex promotes expression of core mediators in cell cycle progression, Cdk6 and E2f1, to facilitate vitellogenesis in L. migratoria. (B) Reproductive polyphenism in aphid A. pisum occurs during the short-day condition given the increased JHE activity, and the lowering of JH result in the switch from parthenogenesis to sexual reproduction. (C) Repression of reproduction diapause in beetles C. bowringi initiates in short-day condition where the upregulation of the JH-Met-Kr-h1 pathway genes expression increases Vg synthesis.
In addition, sesquiterpenoids can mediate insect reproduction under different light conditions. In aphids, reproductive polyphenism alternates their reproductive modes from parthenogenesis to sexual reproduction given different photoperiodic duration. In Acyrthosiphon pisum, enhanced sesquiterpenoid degradation by juvenile hormone esterase (JHE) accounts for the lower JH titer during short-day conditions that produces sexual morphs, in contrast to the higher JH titer in parthenogenetic morphs during long-day conditions (Ishikawa et al., 2012; Figure 4B). In beetle Colaphellus bowringi, high sesquiterpenoid titer upregulates expression of vitellogenin receptor (VgR) via JH-Met-Kr-h1 signaling and promotes Vg synthesis and ovary development during short-day period, while low JH titer initiates reproductive diapause and promotes lipid storage in the fat body instead of Vg synthesis during the long-day period (Liu et al., 2016, 2019; Figure 4C).
Sexual Dimorphism and Dimorphic Behavior
Sexual dimorphism is commonly observed in insects. Nevertheless, the extreme sexually dimorphic traits of juvenile-like females without pupation and ephemeral winged males after a pupal stage in scale insects have raised questions as to how these features could arise. By transcriptomic and qRT-PCR analyses of post-embryonic stages of Ericerus pela, lower Met, Tai, and Kr-h1 expression levels are found in pupal and adult males as compared to females. Together with a surge in Br-C expression in male prepupal stage, the sex-specific regulation lead to the complete metamorphosis in males but not in females (Yang et al., 2015; Figure 5A). In another scale insect Planococcus kraunhiae, qRT-PCR analysis on a daily sampling of different development stages reveal that expression levels of Kr-h1 are higher in male-biased embryos and early nymphs, and lower during prepupal and after pupal stages (Vea et al., 2016). However, elevation of JH or Met, Tai, and Kr-h1 gene expressions as observed in E. pela is not found in the adult P. kraunhiae females.
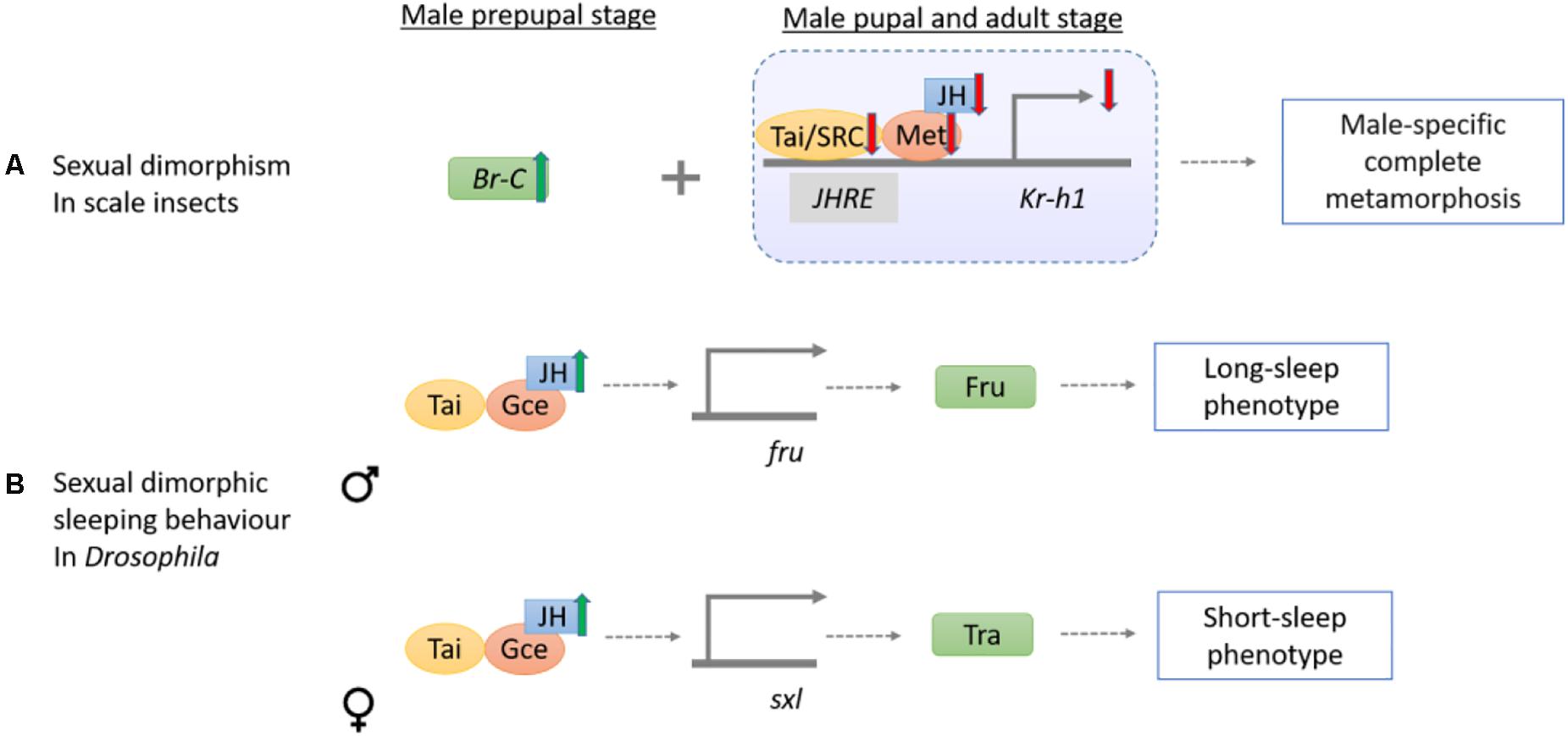
Figure 5. Schematic diagram showing the mechanisms of sesquiterpenoids in regulation of insect sexual dimorphism and dimorphic behavior. (A) In scale insects, sexual dimorphism of winged male adults is linked to the signaling of JH, Met, Tai/SRC, and Kr-h1, whereas they are increased in early developmental stages but decreased during the pupal and adult stages. (B) The sexual dimorphic sleeping behavior in Drosophila is maintained by the JH-Gce signal on the fru and sxl genes in male and female, respectively. fru then encodes Fru for inducing long-sleep pattern in male while sxl induces Tra for short-sleep phenotype in female.
In Drosophila, JH can also control sexual dimorphic behaviors including locomotory and sleeping activities (Belgacem and Martin, 2007; Wu B. et al., 2018; Figure 5B). In the presence of JH by overexpression of JHAMT, longer sleep in males and shorter sleep in females are observed (Wu B. et al., 2018). Interestingly, gce mutant male flies sleep less while female sleep more but mutation in the Met dose not exhibit a similar result (Wu B. et al., 2018). The binary switch gene sex-lethal (Sxl) can impose female development via promoting expression of fruitless (fru), doublesex (dsx), and transformer (tra). Male development occurs when sxl is turned off (Kappes et al., 2011). In the jhamt and gce mutant, Fru, sxl, and tra transcript level were almost halved. Decreasing sleep time occurred when fru in male flies and when female tra was expressed in Fru neurons of males, suggesting JH-Gce signaling can potentially act as a regulatory pathway in sexually dimorphic sleep pattern (Wu B. et al., 2018).
Eusociality
Some insects such as ants, bees, termites and wasps are well known for their eusociality in which they live cooperatively in a colony and only some individuals are reproductive. Such processes have also been linked to JH.
Across ant species, the effects of JH act with different eusocial complexity (Figure 6A). For ants with simple, queenless societies, e.g., Streblognathus and Diacamma, low JH titer is recorded in the gamergates with high individual ranks within the hierarchy, and elevated JH level result in a loss of the reproductive status of the alpha workers (Sommer et al., 1993; Cuvillier-Hot et al., 2004; Brent et al., 2006). For species that have secondarily revert to queenless, simple societies, e.g., Dinoponera quadriceps, JH application can increase the regressed ovaries in queenless ants (Norman et al., 2019). For ants with complex society such as Pogonomyrmex rugosus, JH analogs (methoprene) stimulate the production of queens and upregulate Vg gene expression. The effect of JH in ants is interpreted as mimicking the effect of hibernation (Libbrecht et al., 2013), where low temperature or the associated photoperiod changes up-regulate the insulin/insulin-like growth factor signaling pathway (IIS) genes in queens. No direct result has proven the relationship of IIS and JH in ants to date, and yet, the production of JH in the CA is affected by the release of neuropeptides regulated by IIS in Drosophila (Tu et al., 2005). JH may also directly or indirectly regulate of caste polyethism via changing the division of labor and maternal effects. Elevated JH titer can alter the behavior of workers of Acromyrmex octospinosus leaf-cutting ants by making them more active, threat responsive, and less interested in intranida works such as taking care of larva and fungal cultivation (Norman and Hughes, 2016). During the maternal stage of Pogonomyrmex harvest ants, additional JH also resulted in a 50% increase in worker body size and significantly reduced in total number of progeny reared (Cahan et al., 2011).
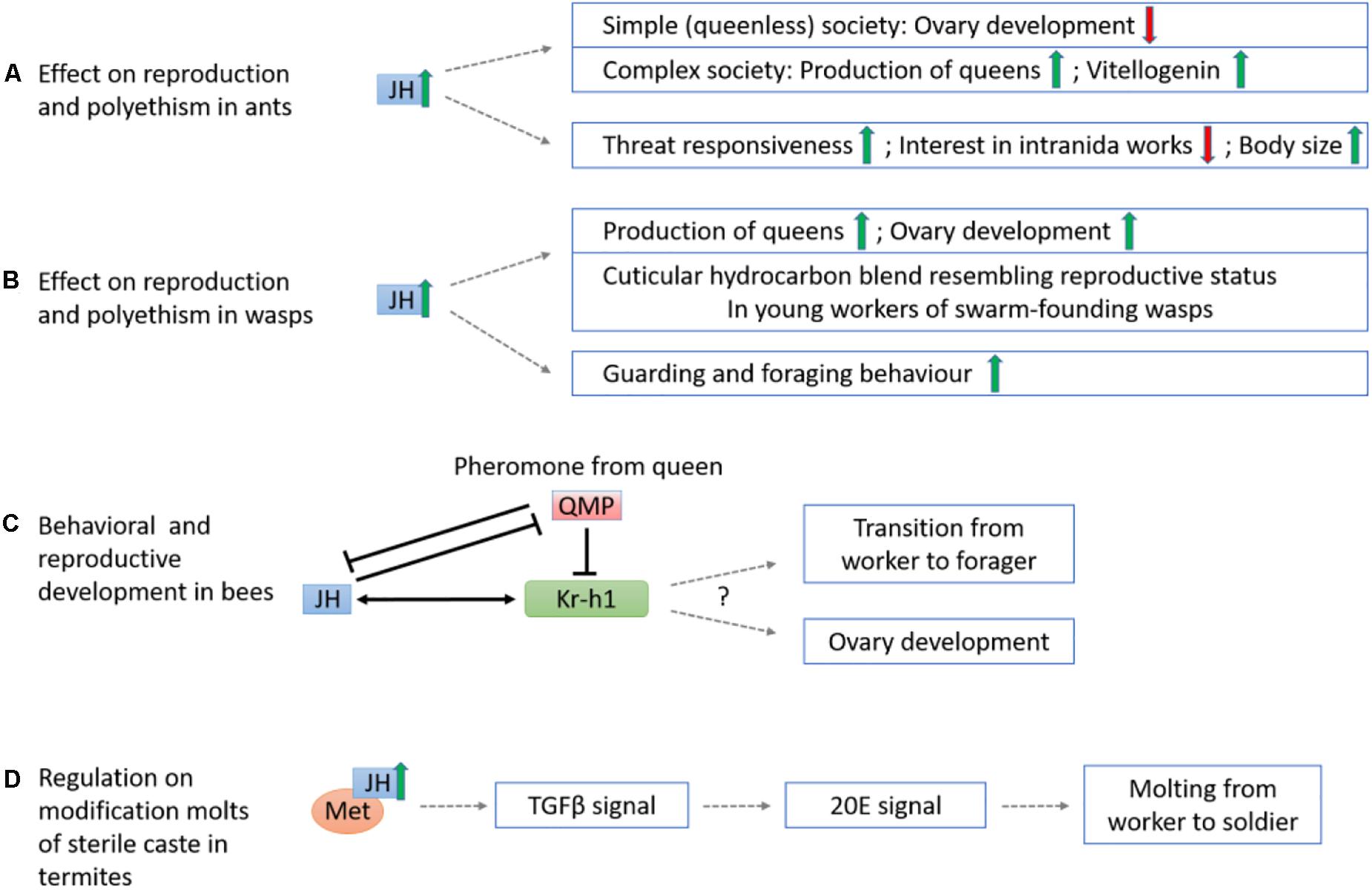
Figure 6. Schematic diagram showing the mechanisms of sesquiterpenoids in regulation of insect eusociality. (A) The effect of JH on reproduction and polyethism in ants. The elevation of JH represses ovary development in simple, queenless societies but promotes queen production and vitellogenin expression in complex societies. JH also induces threat responsiveness and reduces interest in intraida works in ants, and may also increase their body size. (B) The effect of JH on reproduction and polyethism in wasps. JH generally stimulates the production of queens and ovary development, and mediates cuticular hydrocarbon blend resembling reproductive status in young workers of swarm-founding wasps. Besides, JH triggers the guarding and foraging behavior. (C) The queen releases QMP which downregulates JH and Kr-h1 and thus inhibits ovary development of workers and the transition from in-hive worker to forager in bees. (D) The increase of JH in JH-Met signaling pathway induces TGFβ and 20E signals that promote the modification molts from worker to soldier in the sterile caste of termite Z. nevadensis.
Similarly, JH also appears to have different effects on wasp species with various eusociality (Figure 6B). Previous studies indicated JH could modulate age polyethism and promote the production of foragers in highly eusocial species such as Polybiine wasps (O’Donnell and Jeanne, 1993; O’Donnell, 1998), and could mediate both age polyethism (Shorter and Tibbetts, 2009) and reproductive division of labor in primitively eusocial species such as Polistes. Application of JH analog methoprene promotes the onset of guarding behavior, the number of foraging females, and stimulates the production of queens (Barth et al., 1975; Röseler et al., 1980, 1984, 1985; Lozano et al., 2015; Giray et al., 2005). Nevertheless, in other primitive eusocial species such as Ropalidia marginata that has both post-imaginal regulation of reproductive division of labor and age polyethism, JH could only accelerate ovarian development but not age polyethism (Agrahari and Gadagkar, 2003). For caste-flexible swarm-founding wasp Synoeca surinama, JH functions as gonadotropin and directly modifies the cuticular hydrocarbon blend of young workers to resemble that of a reproductive one but does not necessarily link to dominance behavior (Kelstrup et al., 2014).
It is worth also noting that the response to JH could be different among members of the same colony. In Polistes canadensis, the effect of JH on ovaries are different between queens and workers as a potential trophic advantage of the queens over the workers (Giray et al., 2005), while in Polistes dominulus where queens nest cooperatively with other queens, JH has a stronger effect on the dominance, fertility, and aggressiveness of large queens (Tibbetts and Izzo, 2009; Tibbetts et al., 2011, 2018). In species Polistes metricus with non-cooperative nest-founding queen pattern, JH leads to an increase of fertility for all individuals, but among the cooperative workers, large workers increase their fertility in response to JH more while small workers do not (Tibbetts and Sheehan, 2012).
In honeybees Apis mellifera, repression of ovary development, of in-hive workers, were induced by the downregulation of Kr-h1 expression controlled by the queen’s release of mandibular pheromone (QMP) (Grozinger and Robinson, 2007; Figure 6C). In methoprene (JH analog)-treated workers, Kr-h1 expression is no longer repressed by QMP suggesting an antagonistic relationship between sesquiterpenoids and QMP. In addition, the transition of working to foraging behavior were also found to link to a higher JH titer and Kr-h1 level (Grozinger and Robinson, 2007). On the other hand, in the bumblebee Bombus terrestris, similar to the honeybee mentioned above, QMP reduces Kr-h1 level but the difference in Kr-h1 expression between the working and foraging bees are not significant (Shpigler et al., 2010). However, among a group of queenless workers, the dominant individuals have a higher Kr-h1 expression with active ovaries whereas subordinate individuals have a downregulated Kr-h1 expression level with undeveloped ovaries (Shpigler et al., 2010). These studies highlighted the possible roles of sesquiterpenoids in the eusociality in bees.
In termites, eusociality is maintained through differentiation into reproductive caste and sterile soldier caste, in which a higher JH titer induces differentiation of workers via an intermediate presoldier stage to become sterile soldiers (Roisin, 1996). Transcriptomic and RNA interference (RNAi) analyses in three molting stages (worker, presoldier and soldier) of termite Zootermopsis nevadensis show that the JH-Met and transforming growth factor beta (TGFβ) pathways are involved in the ecdysteroid synthesis for molting in soldier formation (Masuoka et al., 2018; Figure 6D). However, suppression on Kr-h1 via RNAi has no effect on JH analog induced molting, demonstrating that the molting effect mainly depends on JH-Met induced pathways (Masuoka et al., 2018). This in turn also suggested that JH may alternatively promotes molting instead of solely inhibiting metamorphosis.
Defense
Terpenes in plants have been the major focus on the understanding the plant defense against the insects, and the role of sesquiterpenoids in insect defense has also been documented in a much lesser extent when comparing to the aforementioned roles. In blister beetles, sesquiterpenoid cantharidin is produced and released as a defensive toxin during disturbance (Carrel et al., 1993). Transcriptomic analyses on Mylabris cichorii identified that the mevalonate pathway in synthesis of JH is correlated with the cantharidin biosynthesis (Huang et al., 2016). In another blister beetle Epicauta chinensis, RNAi knockdown of CYP15A1 and JH epoxide hydrolase (JHEH) result in inhibition of cantharidin biosynthesis, suggesting degradation of JH-III is essential in producing potential precursors of cantharidin (Jiang et al., 2017; Figure 7).

Figure 7. Schematic diagram showing the potential involvement of juvenile hormone in regulation of insect defensive toxin production. The metabolism of JH-III through its degradation pathway by JHE and JHEH is essential for the biosynthesis of the defensive toxin cantharidin in blister beetles.
MicroRNA Regulations on Sesquiterpenoids
Non-coding RNAs such as microRNAs (miRNAs) have been implicated in regulation of many important biological processes (Lucas and Raikhel, 2013; Wang et al., 2014; Yang et al., 2014; Cao et al., 2017; Qu et al., 2018). In canonical miRNA biogenesis pathway in insects (Figure 8), primary miRNA transcript (pri-miRNA) is first transcribed from miRNA gene by RNA polymerase II, followed by processing by Drosha with the help of partner Pasha to generate the precursor miRNA (pre-miRNA) (Denli et al., 2004; Kim et al., 2009). Transported from nucleus to cytoplasm with the help of Exportin-5 and RAN-GTP, pre-miRNA is further processed by Dicer and Loquacious to produce miRNA/miRNA∗ duplex, which will be loaded into the Argonaute (Ago) by HSP70/HSP90 chaperone machinery to form mature RNA-induced silencing complex (RISC) after strand selection (Bartel, 2004; Kim et al., 2009; Iwasaki et al., 2010). Recently, miRNAs have been explored in the regulation of sesquiterpenoids. In Blattella germanica, silencing the expression of Dicer-1 shows that miRNAs regulation is related to metamorphosis (Gomez-Orte and Belles, 2009), and treatment of methoprene on Drosophila S2 cells also reveal the differential expression of miR-34, miR-100, miR-125, and let-7 (Sempere et al., 2003).
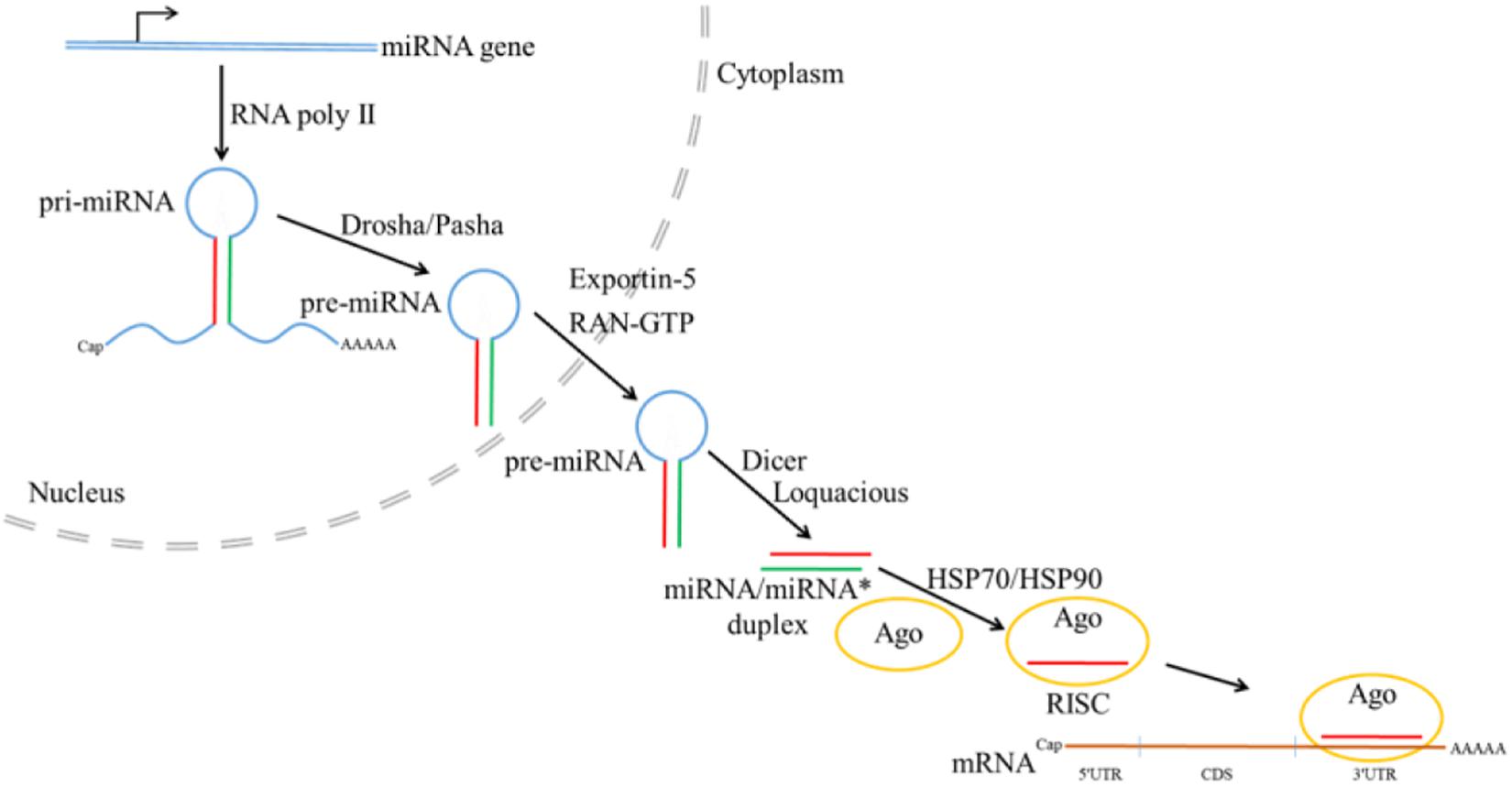
Figure 8. Canonical microRNA biogenesis pathway in Drosophila (the figure is summarized from Bartel, 2004; Denli et al., 2004; Kim et al., 2009; Iwasaki et al., 2010; Cao et al., 2017; Qu et al., 2018).
In many insects, miRNAs have also been found to potentially regulate different sesquiterpenoid pathway genes (Table 2). For instances, in mosquito A. aegypti, four JH biosynthetic enzyme genes including 3-hydroxy-3-methylglutaryl-coenzyme A reductase (HMGR), diphosphomevalonate decarboxylase (PP-MevD), aldehyde dehydrogenase (ALDH), and farnesyl-pyrophosphate synthase (FPPS) were in silico predicted to be potentially regulated by miRNAs (Nouzova et al., 2018). In addition, in the adult female mosquito, mosquito specific miR-1890 targets JH-controlled chymotrypsin-like SP, JHA15 that involve in the regulation of blood digestion, ovary development and egg deposition (Lucas et al., 2015).
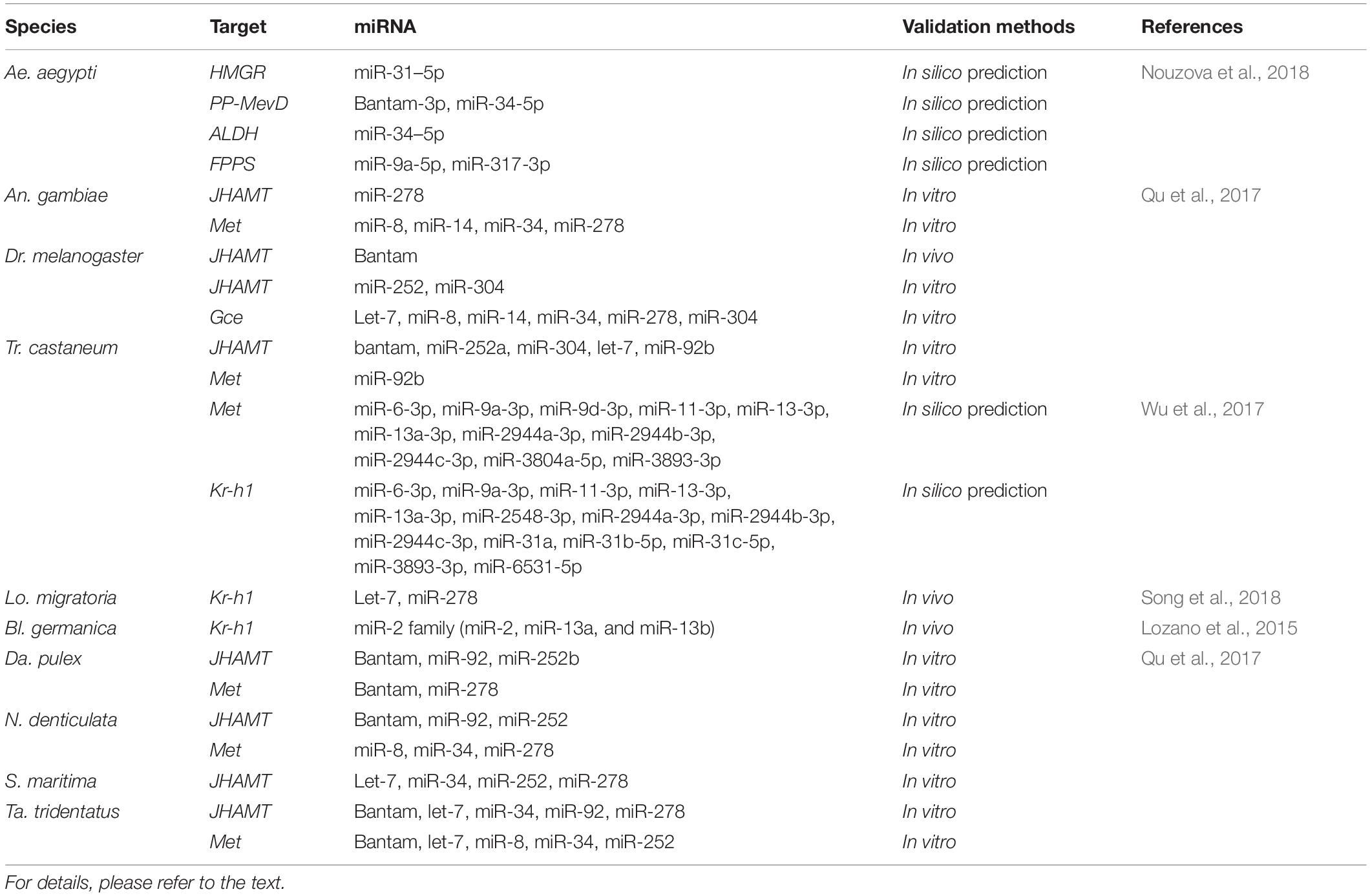
Table 2. Published studies of potential microRNA regulators on insect sesquiterpenoid pathway genes.
In T. castaneum, developmental defects and lethality are observed after knocking down Dcr-1 and Ago-1, and in silico prediction showed that putative JH receptor Met and JH-inducible transcription factor Kr-h1 were targeted by 11 miRNAs and 14 miRNAs respectively (Wu et al., 2017).
In L. migratoria, Ago-1-dependent miRNAs are involved in oogenesis (Song et al., 2013), with let-7 and miR-278 caused decrease of yolk protein precursors results in defects of ovarian development and oocyte maturation through Kr-h1 (Song et al., 2018), and application of miR-2/13/71 agomiR leads to inhibition of oocyte maturation and ovarian growth whilst the expression level of this miRNA cluster could be decreased to achieve vitellogenesis and oogenesis (Song et al., 2019).
In B. germanica, expression of Dicer-1 whose depletion causes sterile females, is negatively related to JH levels, indicating the important roles of miRNAs and interaction between miRNAs and JH in oogenesis (Tanaka and Piulachs, 2012). Specifically, treatment with miR-2-inhibitor on last instar resulted metamorphic defects, and treatment with miR-2 mimic on the Dicer-1-depleted juvenile can complete metamorphosis from nymph to adults (Lozano et al., 2015).
In order to strengthen ability of adaptation, brown planthoppers, Nilaparvata lugens, shows polyphenism with two phenotypes, long-winged and short-winged morphs. miR-34, whose expression level can be upregulated or downregulated by JH and 20E, respectively, can target insulin receptor-1 to be involved in the modulation of wing polyphenism (Ye et al., 2019).
In H. armigera, 20E and JH are involved in the control of climbing behaviors of single nucleopolyhedrovirus (HaSNPV) infected larvae. Methoprene treatment decreases expression of Br-C Z2 and increases expression of these miRNAs miR-8 and miR-429 which could target Br-C Z2 (Zhang S. et al., 2018), implying the miRNA-mediated crosstalk between 20E and JH.
In Drosophila, miRNA bantam has been found to interact with JHAMT both in silico, in vitro, and in vivo (Qu et al., 2017). The overexpression of microRNA bantam in the brain decreases expression levels of JHAMT.; The knockdown of bantam increases the expression level of JHAMT (Qu et al., 2017; Figure 9). Hormonal measurement in bantam mutants demonstrates decreased sesquiterpenoid levels and male genital defects. bantam mutant phenotypes can be rescued by exogenous sesquiterpenoid application (Qu et al., 2017). In other arthropods including other insects, crustaceans, myriapod and chelicerate, the roles of bantam and other miRNAs on JHAMT and Met have also been tested both in silico and in vitro, revealing a conserved system of miRNAs in regulation of sesquiterpenoids established in the arthropod ancestor (Qu et al., 2017; Table 2). A list summarizing the latest knowledge on miRNA regulation of sesquiterpenoid pathway genes are shown in Table 2.
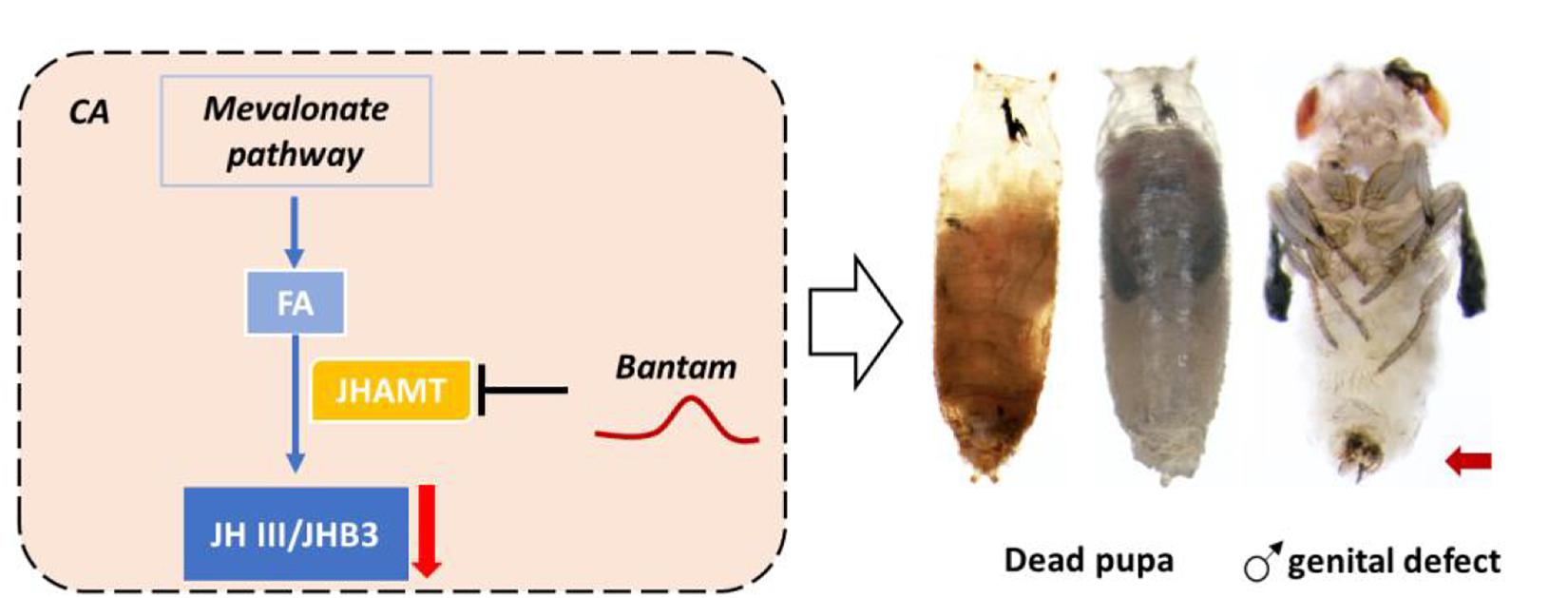
Figure 9. MicroRNA bantam regulates JH titer via targeting JHAMT in D. melanogaster. Up-regulation of bantam repressed expression of JHAMT and reduced the titer of JH III and JHB3, which resulted in dead pupa and male genital defects.
Author Contributions
SSKT, SL, CL, and JH wrote the first draft of the manuscript. All authors proofread the final version of the manuscript.
Funding
This work was supported by the Hong Kong Research Grant Council (RGC) General Research Fund (GRF) (14100919, 14100420). SSKT, SL, and CL were supported by studentships by the Chinese University of Hong Kong.
Conflict of Interest
The authors declare that the research was conducted in the absence of any commercial or financial relationships that could be construed as a potential conflict of interest.
References
Agrahari, M., and Gadagkar, R. (2003). Juvenile hormone accelerates ovarian development and does not affect age polyethism in the primitively eusocial wasp, Ropalidia marginata. J. Insect Physiol. 49, 217–222. doi: 10.1016/s0022-1910(02)00268-8
Ashok, M., Turner, C., and Wilson, T. G. (1988). Insect juvenile hormone resistance gene homology with the bHLH-PAS family of transcriptional regulators. Proc. Natl. Acad. Sci. U.S.A. 95, 2761–2766. doi: 10.1073/pnas.95.6.2761
Bartel, D. P. (2004). MicroRNAs: genomics, biogenesis, mechanism, and function. Cell 116, 281–297. doi: 10.1016/s0092-8674(04)00045-5
Barth, R. H., Lester, L. J., Sroka, P., Kessler, T., and Hearn, R. (1975). Juvenile HORMONE PROMOTES DOMINANCE BEHAVIOR AND OVARIAN DEVELOPMENT IN SOCIAL WASPs (Polistes annularis). Experientia 31, 691–692.
Belgacem, Y. H., and Martin, J. R. (2007). Hmgcr in the corpus allatum controls sexual dimorphism of locomotor activity and body size via the insulin pathway in Drosophila. PLoS One 2:e187. doi: 10.1371/journal.pone.0000187
Belles, X., Martin, D., and Piulachs, M. D. (2005). The mevalonate pathway and the synthesis of juvenile hormone in insects. Annu. Rev. Entomol. 50, 181–199. doi: 10.1146/annurev.ento.50.071803.130356
Bendena, W. G., Zhang, J., Burtenshaw, S. M., and Tobe, S. S. (2011). Evidence for differential biosynthesis of juvenile hormone (and related) sesquiterpenoids in Drosophila melanogaster. Gen. Comp. Endocrinol. 172, 56–61. doi: 10.1016/j.ygcen.2011.02.014
Bergot, B. J., Baker, F. C., Cerf, D. C., Jamieson, G., and Schooley, D. A. (1981). Juvenile Hormone Biochemistry, eds G. E. Pratt and G. T. Brooks (Amsterdam: Elsevier), 33–45.
Bergot, B. J., Jamieson, G. C., Ratcliff, M. A., and Schooley, D. A. (1980). JH zero: new naturally occurring insect juvenile hormone from developing embryos of the tobacco hornworm. Science 210, 336–338. doi: 10.1126/science.210.4467.336
Bittova, L., Jedlicka, P., Dracinsky, M., Kirubakaran, P., Vondrasek, J., Hanus, R., et al. (2019). Exquisite ligand stereoselectivity of a Drosophila juvenile hormone receptor contrasts with its broad agonist repertoire. J. Biol. Chem. 294, 410–423. doi: 10.1074/jbc.RA118.005992
Bomtorin, A. D., Mackert, A., Rosa, G. C., Moda, L. M., Martins, J. R., Bitondi, M. M. G., et al. (2014). Juvenile hormone biosynthesis gene expression in the corpora allata of honey bee (Apis mellifera L.) female castes. PLoS One 9:e86923. doi: 10.1371/journal.pone.0086923
Brent, C., Peeters, C., Dietemann, V., Crewe, R., and Vargo, E. (2006). Hormonal correlates of reproductive status in the queenless ponerine ant, Streblognathus peetersi. J. Comp. Physiol. A Neuroethol. Sens. Neural Behav. Physiol. 192, 315–320.
Cahan, S. H., Graves, C. J., and Brent, C. S. (2011). Intergenerational effect of juvenile hormone on offspring in Pogonomyrmex harvester ants. J. Comp. Physiol. B 181, 991–999. doi: 10.1007/s00360-011-0587-x
Cao, J. Q., Tong, W. S., Yu, H. Y., Tobe, S. S., Bendena, W. G., and Hui, J. H. L. (2017). The role of microRNAs in Drosophila regulation of insulin-like peptides and ecdysteroid signaling: Where are we now? Adv. Insect Physiol. 53, 55–85. doi: 10.1016/bs.aiip.2017.02.002
Carrel, J. E., McCairel, M. H., Slagle, A. J., Doom, J. P., Brill, J., and McCormick, J. P. (1993). Cantharidin production in a blister beetle. Experientia 49, 171–174. doi: 10.1007/BF01989424
Charles, J. P., Iwema, T., Epa, V. C., Takaki, K., Rynes, J., and Jindra, M. (2011). Ligand-binding properties of a juvenile hormone receptor, Methoprene-tolerant. Proc. Natl. Acad. Sci. U.S.A. 108, 21128–21133. doi: 10.1073/pnas.1116123109
Cheong, S. P., Huang, J., Bendena, W. G., Tobe, S. S., and Hui, J. H. (2015). Evolution of Ecdysis and metamorphosis in arthropods: the rise of regulation of juvenile hormone. Integr. Comp. Biol. 55, 878–890. doi: 10.1093/icb/icv066
Cuvillier-Hot, V., Lenoir, A., and Peeters, C. (2004). Reproductive monopoly enforced by sterile police workers in a queenless ant. Behav. Ecol. 15, 970–975.
Daimon, T., and Shinoda, T. (2013). Function, diversity, and application of insect juvenile hormone epoxidases (CYP15). Biotechnol. Appl. Biochem. 60, 82–91. doi: 10.1002/bab.1058
Daimon, T., Kozaki, T., Niwa, R., Kobayashi, I., Furuta, K., Namiki, T., et al. (2012). Precocious metamorphosis in the juvenile hormone-deficient mutant of the silkworm, Bombyx mori. PLoS Genet. 8:e1002486. doi: 10.1371/journal.pgen.1002486
De Loof, A., and Schoofs, L. (2019). Mode of action of Farnesol, the “Noble Unknown” in particular in Ca2+ homeostasis, and its juvenile hormone-esters in evolutionary retrospect. Front. Neurosci. 13:141. doi: 10.3389/fnins.2019.00141
Denli, A. M., Tops, B. B., Plasterk, R. H., Ketting, R. F., and Hannon, G. J. (2004). Processing of primary microRNAs by the Microprocessor complex. Nature 432, 231–235. doi: 10.1038/nature03049
Dubrovsky, E. B. (2005). Hormonal cross talk in insect development. Trends Endocrinol. Metab. 16, 6–11. doi: 10.1016/j.tem.2004.11.003
Dubrovsky, E. B., Dubrovskaya, V. A., Bernardo, T., Otte, V., DiFilippo, R., and Bryan, H. (2011). The Drosophila FTZ-F1 nuclear receptor mediates juvenile hormone activation of E75A gene expression through an intracellular pathway. J. Biol. Chem. 286, 33689–33700. doi: 10.1074/jbc.M111.273458
Giray, T., Giovanetti, M., and West-Eberhard, M. J. (2005). Juvenile hormone, reproduction, and worker behavior in the neotropical social wasp Polistes Canadensis. Proc. Natl. Acad. Sci. U.S.A. 102, 3330–3335. doi: 10.1073/pnas.0409560102
Gomez-Orte, E., and Belles, X. (2009). MicroRNA-dependent metamorphosis in hemimetabolan insects. Proc. Natl. Acad. Sci. U.S.A. 106, 21678–21682. doi: 10.1073/pnas.0907391106
Grozinger, C. M., and Robinson, G. E. (2007). Endocrine modulation of a pheromone-responsive gene in the honey bee brain. J. Comp. Physiol. A Neuroethol. Sens. Neural. Behav. Physiol. 193, 461–470. doi: 10.1007/s00359-006-0202-x
Huang, J., Marchal, E., Hult, E. R., and Tobe, S. S. (2015). Characterization of the juvenile hormone pathway in the viviparous cockroach, Diploptera punctate. PLoS One 10:e0117291. doi: 10.1371/journal.pone.0117291
Huang, Y., Wang, Z., Zha, S., Wang, Y., Jiang, W., Liao, Y., et al. (2016). De novo transcriptome and expression profile analysis to reveal genes and pathways potentially involved in cantharidin biosynthesis in the blister beetle Mylabris cichorii. PLoS One 11:e0146953. doi: 10.1371/journal.pone.0146953
Hui, J. H., Benena, W. G., and Tobe, S. S. (2013). “Future perspectives for research on the biosynthesis of juvenile hormones and related sesquiterpenoids in Arthropod endocrinology and ecotoxicology,” in Juvenile Hormone and Juvenoids: Moldeling Biological Effects and Environmental, ed. J. Devillers (New York, NY: CRC Press), 15–30.
Hui, J. H. L., Hayward, A., Bendena, W. G., Takahashi, T., and Tobe, S. S. (2010). Evolution and functional divergence of enzymes involved in sesquiterpenoid hormone biosynthesis in crustaceans and insects. Peptides 31, 451–455. doi: 10.1016/j.peptides.2009.10.003
Ishikawa, A., Ogawa, K., Gotoh, H., Walsh, T. K., Tagu, D., Brisson, J. A., et al. (2012). Juvenile hormone titre and related gene expression during the change of reproductive modes in the pea aphid. Insect Mol. Biol. 21, 49–60. doi: 10.1111/j.1365-2583.2011.01111.x
Iwasaki, S., Kobayashi, M., Yoda, M., Sakaguchi, Y., Katsuma, S., Suzuki, T., et al. (2010). Hsc70/Hsp90 chaperone machinery mediates ATP-dependent RISC loading of small RNA duplexes. Mol. Cell 39, 292–299. doi: 10.1016/j.molcel.2010.05.015
Jiang, M., Lu, S., and Zhang, Y. (2017). Characterization of juvenile hormone related genes regulating cantharidin biosynthesis in Epicauta chinensis. Sci. Rep. 7:2308. doi: 10.1038/s41598-017-02393-w
Jindra, M., Uhlirova, M., Charles, J. P., Smykal, V., and Hill, R. J. (2015). Genetic evidence for function of the bHLH-PAS Protein Gce/Met as a juvenile hormone receptor. PLoS Genet. 11:e1005394. doi: 10.1371/journal.pgen.1005394
Jing, Y.-P., An, H., Zhang, S., Wang, N., and Zhou, S. (2018). Protein kinase C mediates juvenile hormone-dependent phosphorylation of Na+/K+-ATPase to induce ovarian follicular patency for yolk protein uptake. J. Biol. Chem. 293, 20112–20122. doi: 10.1074/jbc.RA118.005692
Judy, K. J., Schooley, D. A., Dunham, L. L., Hall, M. S., Bergot, B. J., and Siddall, J. B. (1973). Isolation, structure, and absolute configuration of a new natural insect juvenile hormone from Manduca sexta. Proc. Natl. Acad. Sci. U.S.A. 70, 1509–1513. doi: 10.1073/pnas.70.5.1509
Kappes, G., Deshpande, G., Mulvey, B. B., Horabin, J. I., and Schedl, P. (2011). The Drosophila Myc gene, diminutive, is a positive regulator of the Sex-lethal establishment promoter, Sxl-Pe. Proc. Natl. Acad. Sci. U.S.A. 108, 1543–1548. doi: 10.1073/pnas.1017006108
Kayukawa, T., Jouraku, A., Ito, Y., and Shinoda, T. (2017). Molecular mechanism underlying juvenile hormone-mediated repression of precocious larval–adult metamorphosis. Proc. Natl. Acad. Sci. U.S.A. 114, 1057–1062. doi: 10.1073/pnas.1615423114
Kayukawa, T., Minakuchi, C., Namiki, T., Togawa, T., Yoshiyama, M., Kamimura, M., et al. (2012). Transcriptional regulation of juvenile hormone-mediated induction of Krüppel homolog 1, a repressor of insect metamorphosis. Proc. Natl. Acad. Sci. U.S.A. 109, 11729–11734. doi: 10.1073/pnas.1204951109
Kayukawa, T., Nagamine, K., Ito, Y., Nishita, Y., Ishikawa, Y., and Shinoda, T. (2016). Krüppel homolog 1 inhibits insect metamorphosis via direct transcriptional repression of broad-complex, a Pupal Specifier Gene. J. Biol. Chem. 291, 1751–1762. doi: 10.1074/jbc.M115.686121
Kelstrup, H. C., Hartfelder, K., Nascimento, F. S., and Riddiford, L. M. (2014). The role of juvenile hormone in dominance behavior, reproduction and cuticular pheromone signaling in the caste-flexible epiponine wasp, Synoeca surinama Front. Zool. 11:78. doi: 10.1186/s12983-014-0078-5
Kim, V. N., Han, J., and Siomi, M. C. (2009). Biogenesis of small RNAs in animals. Nat. Rev. Mol. Cell Biol. 10, 126–139. doi: 10.1038/nrm2632
Konopova, B., Smykal, V., and Jindra, M. (2011). Common and distinct roles of juvenile hormone signaling genes in metamorphosis of holometabolous and hemimetabolous insects. PLoS One 6:e28728. doi: 10.1371/journal.pone.0028728
Kotaki, T., Shinada, T., Kaihara, K., Ohfune, Y., and Numata, H. (2009). Structure determination of a new juvenile hormone from a Heteropteran insect. Org. Lett. 11, 5234–5237. doi: 10.1021/ol902161x
Li, K. L., Yuan, S. Y., Nanda, S., Wang, W. X., Lai, F. X., Fu, Q., et al. (2018). The Roles of E93 and Kr-h1 in Metamorphosis of Nilaparvata lugens. Front. Physiol. 9:1677. doi: 10.3389/fphys.2018.01677
Libbrecht, R., Corona, M., Wende, F., Azevedo, D. O., Serrao, J. E., and Keller, L. (2013). Interplay between insulin signaling, juvenile hormone, and vitellogenin regulates maternal effects on polyphenism in ants. Proc. Natl. Acad. Sci. U.S.A. 110, 11050–11055. doi: 10.1073/pnas.1221781110
Liu, S., Li, K., Gao, Y., Chen, W., Ge, W., Feng, Q., et al. (2018). Antagonistic actions of juvenile hormone and 20-hydroxyecdysone within the ring gland determine developmental transitions in Drosophila. Proc. Natl. Acad. Sci. U.S.A. 115, 139–144. doi: 10.1073/pnas.1716897115
Liu, W., Guo, S., Sun, D., Zhu, L., Zhu, F., Lei, C. L., et al. (2019). Molecular characterization and juvenile hormone-regulated transcription of the vitellogenin receptor in the cabbage beetle Colaphellus bowringi. Comp. Biochem. Physiol. A. Mol. Integr. Physiol. 229, 69–75. doi: 10.1016/j.cbpa.2018.12.004
Liu, W., Li, Y., Zhu, L., Zhu, F., Lei, C. L., and Wang, X. P. (2016). Juvenile hormone facilitates the antagonism between adult reproduction and diapause through the methoprene-tolerant gene in the female Colaphellus bowringi. Insect Biochem. Mol. Biol. 74, 50–60. doi: 10.1016/j.ibmb.2016.05.004
Lozano, J., and Belles, X. (2011). Conserved repressive function of Krüppel homolog 1 on insect metamorphosis in hemimetabolous and holometabolous species. Sci. Rep. 1:163. doi: 10.1038/srep00163
Lozano, J., Montañez, R., and Belles, X. (2015). MiR-2 family regulates insect metamorphosis by controlling the juvenile hormone signaling pathway. Proc. Natl. Acad. Sci. U.S.A. 112, 3740–3745. doi: 10.1073/pnas.1418522112
Lucas, K., and Raikhel, A. S. (2013). Insect microRNAs: biogenesis, expression profiling and biological functions. Insect Biochem. Mol. Biol. 43, 24–38. doi: 10.1016/j.ibmb.2012.10.009
Lucas, K. J., Zhao, B., Roy, S., Gervaise, A. L., and Raikhel, A. S. (2015). Mosquito-specific microRNA-1890 targets the juvenile hormone-regulated serine protease JHA15 in the female mosquito gut. RNA Biol. 12, 1383–1390. doi: 10.1080/15476286.2015.1101525
Marchal, E., Zhang, J., Badisco, L., Verlinden, H., Hult, E. F., Van Wielendaele, P., et al. (2011). Final steps in juvenile hormone biosynthesis in the desert locust, Schistocerca gregaria. Insect Biochem. Mol. Biol. 41, 219–227. doi: 10.1016/j.ibmb.2010.12.007
Masuoka, Y., Yaguchi, H., Toga, K., Shigenobu, S., and Maekawa, K. (2018). TGFβ signaling related genes are involved in hormonal mediation during termite soldier differentiation. PLoS Genet. 14:e1007338. doi: 10.1371/journal.pgen.1007338
Meiselman, M., Lee, S. S., Tran, R., Dai, H., Ding, Y., Rivera-Perez, C., et al. (2017). Endocrine network essential for reproductive success in Drosophila melanogaster. Proc. Natl. Acad. Sci. U.S.A. 114, E3849–E3858. doi: 10.1073/pnas.1620760114
Meyer, A. S., Schneiderman, H. A., Hanzmann, E., and Ko, J. H. (1968). The two juvenile hormones from the cecropia silk moth. Proc. Natl. Acad. Sci. U.S.A. 60, 853–860. doi: 10.1073/pnas.60.3.853
Minakuchi, C., Ishii, F., Washidu, Y., Ichikawa, A., Tanaka, T., Miura, K., et al. (2015). Expressional and functional analysis of CYP15A1, a juvenile hormone epoxidase, in the red flour beetle Tribolium castaneum. J. Insect Physiol. 80, 61–70. doi: 10.1016/j.jinsphys.2015.04.008
Minakuchi, C., Namiki, T., and Shinoda, T. (2009). Krüppel homolog 1, an early juvenile hormone-response gene downstream of Methoprene-tolerant, mediates its anti-metamorphic action in the red flour beetle Tribolium castaneum. Dev. Biol. 325, 341–350. doi: 10.1016/j.ydbio.2008.10.016
Niwa, R., and Niwa, Y. S. (2014a). Enzymes for ecdysteroid biosynthesis: their biological functions in insects and beyond. Biosci. Biotechnol. Biochem. 78, 1283–1292. doi: 10.1080/09168451.2014.942250
Niwa, Y. S., and Niwa, R. (2014b). Neural control of steroid hormone biosynthesis during development in the fruit fly Drosophila melanogaster. Genes Genet. Syst. 89, 27–34. doi: 10.1266/ggs.89.27
Norman, V. C., and Hughes, W. (2016). Behavioural effects of juvenile hormone and their influence on division of labour in leaf-cutting ant societies. J. Exp. Biol. 219, 8–11. doi: 10.1242/jeb.132803
Norman, V. C., Pamminger, T., Nascimento, F., and Hughes, W. (2019). The role of juvenile hormone in regulating reproductive physiology and dominance in Dinoponera quadriceps ants. PeerJ 7:e6512. doi: 10.7717/peerj.6512
Nouzova, M., Etebari, K., Noriega, F. G., and Asgari, S. (2018). A comparative analysis of corpora allata-corpora cardiaca microRNA repertoires revealed significant changes during mosquito metamorphosis. Insect Biochem. Mol. Biol. 96, 10–18. doi: 10.1016/j.ibmb.2018.03.007
O’Donnell, S. (1998). Reproductive caste determination in eusocial wasps (Hymenoptera : Vespidae). Annu. Rev. Entomol. 43, 323–346. doi: 10.1146/annurev.ento.43.1.323
O’Donnell, S., and Jeanne, R. L. (1993). Methoprene accelerates age polyethism in workers of a social wasp Polybia occidentalis. Physiol. Entomol. 18, 189–194.
Paroulek, M., and Sláma, K. (2014). Production of the sesquiterpenoid, juvenile hormone-1 (JH-I), and of vitamin E in the accessory sexual (colleterial) glands of adult male moths, Hyalophora cecropia (Linnaeus, 1758), (Lepidoptera: Saturniidae). Life Exc. Biol. 2, 102–124. doi: 10.9784/LEB2(2)Paroulek.01
Parthasarathy, R., Sun, Z., Bai, H., and Palli, S. R. (2010). Juvenile hormone regulation of vitellogenin synthesis in the red flour beetle, Tribolium castaneum. Insect Biochem. Mol. Biol. 40, 405–414. doi: 10.1016/j.ibmb.2010.03.006
Postlethwait, J. H., and Weiser, K. (1973). Vitellogenesis induced by Juvenile Hormone in the Female Sterile Mutant apterous-four in Drosophila melanogaster. Nat. New Biol. 244, 284–285. doi: 10.1038/newbio244284a0
Qu, Z., Bendena, W. G., Nong, W., Siggens, K. W., Noriega, F. G., Kai, Z. P., et al. (2017). MicroRNAs regulate the sesquiterpenoid hormonal pathway in Drosophila and other arthropods. Proc. Biol. Sci. 284:20171827. doi: 10.1098/rspb.2017.1827
Qu, Z., Bendena, W. G., Tobe, S. S., and Hui, J. H. L. (2018). Juvenile hormone and sesquiterpenoids in arthropods: biosynthesis, signaling, and role of MicroRNA. J. Steroid Biochem. 184, 69–76. doi: 10.1016/j.jsbmb.2018.01.013
Richard, D. S., Applebaum, S. W., Sliter, T. J., Baker, F. C., Schooley, D. A., Reuter, C. C., et al. (1989). Juvenile hormone bisepoxide biosynthesis in vitro by the ring gland of Drosophila melanogaster: a putative juvenile hormone in the higher Diptera. Proc. Natl. Acad. Sci. U.S.A. 86, 1421–1425.
Riddiford, L. M. (2012). How does juvenile hormone control insect metamorphosis and reproduction? Gen. Comp. Endocrinol. 179, 477–484. doi: 10.1016/j.ygcen.2012.06.001
Riddiford, L. M., Cherbas, P., and Truman, J. W. (2000). Ecdysone receptors and their biological actions. Vitam. Horm. 60, 1–73.
Roisin, Y. (1996). Castes in humivorous and litter-dwelling neotropical nasute termites (Isoptera, Termitidae). Ins. Soc. 43, 375–389. doi: 10.1007/BF01258410
Röller, H., Dahm, K. H., Sweely, C. C., and Trost, B. M. (1967). The structure of the juvenile hormone. Angew. Chem. Int. Ed. 6, 179–180.
Röseler, P. F., Röseler, I., and Strambi, A. (1980). The activity of corpora allata in dominant and subordinated females of the wasp Polistes gallicus. Insectes Soc. 27, 97–107.
Röseler, P. F., Röseler, I., and Strambi, A. (1985). Role of ovaries and ecdysteroids in dominance hierarchy establishment among foundresses of the primitively social wasp, Polistes gallicus. Behav. Ecol. Sociobiol. 18, 9–13.
Röseler, P. F., Röseler, I., Strambi, A., and Augier, R. (1984). Influence of insect hormones on the establishment of dominance hierarchies among foundresses of the paper wasp, Polistes gallicus. Behav. Ecol. Sociobiol. 15, 133–142. doi: 10.1007/BF00299381
Roy, S., Saha, T. T., Zou, Z., and Raikhel, A. S. (2018). Regulatory pathways controlling female insect reproduction. Annu. Rev. Entomol. 63, 489–511. doi: 10.1146/annurev-ento-020117-043258
Sehnal, F., Svacha, P., and Zrzavy, J. (1996). “Evolution of insect metamorphosis,” in Metamorphosis. Postembryonic reprogramming of gene expression in amphibian and insect cells, eds L. I. Gilbert, J. R. Tata, and B. G. Atkinson (San Diego, CA: Academic Press.), 3–58.
Sempere, L. F., Sokol, N. S., Dubrovsky, E. B., Berger, E. M., and Ambros, V. (2003). Temporal regulation of microRNA expression in Drosophila melanogaster mediated by hormonal signals and broad-Complex gene activity. Dev. Biol. 259, 9–18.
Sheng, Z., Xu, J., Bai, H., Zhu, F., and Palli, S. R. (2011). Juvenile hormone regulates vitellogenin gene expression through insulin-like peptide signaling pathway in the red flour beetle, Tribolium castaneum. J. Biol. Chem. 286, 41924–41936. doi: 10.1074/jbc.M111.269845
Shin, S. W., Zou, Z., Shah, T. T., and Raikhel, A. S. (2012). bHLH-PAS heterodimer of methoprene-tolerant and cycle mediates circadian expression of juvenile hormone-induced mosquito genes. Proc. Natl. Acad. Sci. U.S.A. 109, 16576–16581. doi: 10.1073/pnas.1214209109
Shinoda, T., and Itoyama, K. (2003). Juvenile hormone acid methyltransferase: a key regulatory enzyme for insect metamorphosis. Proc. Natl. Acad. Sci. U.S.A. 100, 11986–11991. doi: 10.1073/pnas.2134232100
Shorter, J. R., and Tibbetts, E. A. (2009). The effect of juvenile hormone on temporal polyethism in the paper wasp Polistes dominulus. Insect. Soc. 56, 7–13. doi: 10.1007/s00040-008-1026-1
Shpigler, H., Patch, H. M., Cohen, M., Fan, Y., Grozinger, C. M., and Bloch, G. (2010). The transcription factor Krüppel homolog 1 is linked to hormone mediated social organization in bees. BMC Evol. Biol. 10:120. doi: 10.1186/1471-2148-10-120
Smykal, V., Bajgar, A., Provaznik, J., Fexova, S., Buricova, M., Takaki, K., et al. (2014). Juvenile hormone signaling during reproduction and development of the linden bug, Pyrrhocoris apterus. Insect Biochem. Mol. Biol. 45, 69–76. doi: 10.1016/j.ibmb.2013.12.003
Sommer, K., Hölldobler, B., and Rembold, H. (1993). Behavioral and physiological aspects of reproductive control in a Diacamma species from Malaysia (Formicidae, Ponerinae). Ethology 94, 162–170. doi: 10.1111/j.1439-0310.1993.tb00556.x
Song, J., Guo, W., Jiang, F., Kang, L., and Zhou, S. (2013). Argonaute 1 is indispensable for juvenile hormone mediated oogenesis in the migratory locust, Locusta migratoria. Insect Biochem. Mol. Biol. 43, 879–887. doi: 10.1016/j.ibmb.2013.06.004
Song, J., Li, W., Zhao, H., Gao, L., Fan, Y., and Zhou, S. (2018). The microRNAs let-7 and miR-278 regulate insect metamorphosis and oogenesis by targeting the juvenile hormone early-response gene Krüppel-homolog 1. Development 145:dev170670. doi: 10.1242/dev.170670
Song, J., Li, W., Zhao, H., and Zhou, S. (2019). Clustered miR-2, miR-13a, miR-13b and miR-71 coordinately target Notch gene to regulate oogenesis of the migratory locust Locusta migratoria. Insect Biochem. Mol. Biol. 106, 39–46. doi: 10.1016/j.ibmb.2018.11.004
Song, J., Wu, Z., Wang, Z., Deng, S., and Zhou, S. (2014). Krüppel-homolog 1 mediates juvenile hormone action to promote vitellogenesis and oocyte maturation in the migratory locust. Insect Biochem. Mol. Biol. 52, 94–101. doi: 10.1016/j.ibmb.2014.07.001
Song, J., and Zhou, S. (2019). Post-transcriptional regulation of insect metamorphosis and oogenesis. Cell. Mol. Life Sci. 77, 1893–1909. doi: 10.1007/s00018-019-03361-5
Swevers, L., Raikhel, A., Sappington, T., Shirk, P., and Iatrou, K. (2005). “Vitellogenesis and post-vitellogenic maturation of the insect ovarian follicle,” in Comprehensive Insect Physiology, Biochemistry, Pharmacology and Molecular Biology, Vol. 3, eds L. Gilbert, S. Gill, and K. Iatrou (Amsterdam: Elsevier), 87–155. doi: 10.1016/B0-44-451924-6/00093-4
Tanaka, E. D., and Piulachs, M. D. (2012). Dicer-1 is a key enzyme in the regulation of oogenesis in panoistic ovaries. Biol. Cell 104, 452–461. doi: 10.1111/boc.201100044
Tibbetts, E. A., and Izzo, A. S. (2009). Endocrine mediated phenotypic plasticity- Condition-dependent effects of JH on dominance and fertility of wasp queens. Horm. Behav. 56, 527–531. doi: 10.1016/j.yhbeh.2009.09.003
Tibbetts, E. A., and Sheehan, M. J. (2012). The effect of juvenile hormone on Polistes wasp fertility varies with cooperative behavior. Horm. Behav. 61, 559–564. doi: 10.1016/j.yhbeh.2012.02.002
Tibbetts, E. A., Levy, S., and Donajkowski, K. (2011). Reproductive plasticity in Polistes paper wasp workers and the evolutionary origins of sociality. J. Insect Physiol. 57, 995–999. doi: 10.1016/j.jinsphys.2011.04.016
Tibbetts, E. A., Fearon, M. L., Wong, E., Huang, Z. Y., and Tinghitella, R. M. (2018). Rapid juvenile hormone downregulation in subordinate wasp queens facilitates stable cooperation. Proc. Biol. Sci. 285:20172645. doi: 10.1098/rspb.2017.2645
Tobe, S. S., and Bendena, W. G. (1999). The regulation of juvenile hormone production in arthropods: functional and evolutionary perspectives. Ann. N.Y. Acad. Sci. 300–310. doi: 10.1111/j.1749-6632.1999.tb07901.x
Truman, J. W. (2019). The evolution of insect metamorphosis. Curr. Biol. 29, R1252–R1268. doi: 10.1016/j.cub.2019.10.009
Truman, J. W., and Riddiford, L. M. (2002). Endocrine insights into the evolution of metamorphosis in insects. Annu. Rev. Entomol. 47, 467–500. doi: 10.1146/annurev.ento.47.091201.145230
Tu, M. P., Yin, C. M., and Tatar, M. (2005). Mutations in insulin signaling pathway alter juvenile hormone synthesis in Drosophila melanogaster. Gen. Comp. Endocrinol. 142, 347–356. doi: 10.1016/j.ygcen.2005.02.009
Ureña, E., Chafino, S., Manjón, C., Franch-Marro, X., and Martín, D. (2016). The Occurrence of the Holometabolous Pupal Stage Requires the Interaction between E93, Krüppel-Homolog 1 and Broad-Complex. PLoS Genet. 12:e1006020. doi: 10.1371/journal.pgen.1006020
Vea, I. M., Tanaka, S., Shiotsuki, T., Jouraku, A., Tanaka, T., and Minakuchi, C. (2016). Differential juvenile hormone variations in scale insect extreme sexual dimorphism. PLoS One 11:e0149459. doi: 10.1371/journal.pone.0149459
Wang, C., Feng, T., Wan, Q., Kong, Y., and Yuan, L. (2014). miR-124 controls Drosophila behavior and is required for neural development. Int. J. Dev. Neurosci. 38, 105–112. doi: 10.1016/j.ijdevneu.2014.08.006
Wen, D., Rivera-Perez, C., Abdou, M., Jia, Q., He, Q., Liu, X., et al. (2015). Methyl farnesoate plays a dual role in regulating drosophila metamorphosis. PLoS Genet. 13:e1005038. doi: 10.1371/journal.pgen.1005038
Wu, B., Ma, L., Zhang, E., Du, J., Liu, S., Price, J., et al. (2018). Sexual dimorphism of sleep regulated by juvenile hormone signaling in Drosophila. PLoS Genet. 14:e1007318. doi: 10.1371/journal.pgen.1007318
Wu, W., Xiong, W., Li, C., Zhai, M., Li, Y., Ma, F., et al. (2017). MicroRNA-dependent regulation of metamorphosis and identification of microRNAs in the red flour beetle, Tribolium castaneum. Genomics 109, 362–373. doi: 10.1016/j.ygeno.2017.06.001
Wu, Z., Guo, W., Xie, Y., and Zhou, S. (2016). Juvenile hormone activates the transcription of cell-division-cycle 6 (Cdc6) for Polyploidy-dependent Insect Vitellogenesis and Oogenesis. J. Biol. Chem. 291, 5418–5427. doi: 10.1074/jbc.M115.698936
Wu, Z., Guo, W., Yang, L., He, Q., and Zhou, S. (2018). Juvenile hormone promotes locust fat body cell polyploidization and vitellogenesis by activating the transcription of Cdk6 and E2f1. Insect Biochem. Mol. Biol. 102, 1–10.
Wyatt, G. R., and Davey, K. G. (1996). Cellular and molecular actions of juvenile hormone. II. Roles of juvenile hormone in adult insects. Adv. Insect Physiol. 26, 1–155.
Yang, M., Wei, Y., Jiang, F., Wang, Y., Guo, X., He, J., et al. (2014). MicroRNA-133 inhibits behavioral aggregation by controlling dopamine synthesis in locusts. PLoS Genet. 10:e1004206. doi: 10.1371/journal.pgen.1004206
Yang, P., Chen, X. M., Liu, W. W., Feng, Y., and Sun, T. (2015). Transcriptome analysis of sexually dimorphic Chinese white wax scale insects reveals key differences in developmental programs and transcription factor expression. Sci. Rep. 5:8141. doi: 10.1038/srep08141
Ye, X., Xu, L., Li, X., He, K., Hua, H., Cao, Z., et al. (2019). miR-34 modulates wing polyphenism in planthopper. PLoS Genet. 15:e1008235. doi: 10.1371/journal.pgen.1008235
Zhang, S., An, S., Hoover, K., Li, Z., Li, X., Liu, X., et al. (2018). Host miRNAs are involved in hormonal regulation of HaSNPV-triggered climbing behaviour in Helicoverpa armigera. Mol. Ecol. 27, 459–475. doi: 10.1111/mec.14457
Zhang, T., Song, W., Zheng, L., Qian, W., Wei, L., Yang, Y., et al. (2018). Krüppel homolog 1 represses insect ecdysone biosynthesis by directly inhibiting the transcription of steroidogenic enzymes. Proc. Natl. Acad. Sci. U.S.A. 115, 3960–3965. doi: 10.1073/pnas.1800435115
Zhang, W. N., Ma, L., Liu, C., Chen, L., Xiao, H. J., and Liang, G. M. (2018). Dissecting the role of Krüppel homolog 1 in the metamorphosis and female reproduction of the cotton bollworm, Helicoverpa armigera. Insect Mol. Biol. 27, 492–504. doi: 10.1111/imb.12389
Keywords: insect, sesquiterpenoid, juvenile hormone, microRNA, evolution
Citation: Tsang SSK, Law STS, Li C, Qu Z, Bendena WG, Tobe SS and Hui JHL (2020) Diversity of Insect Sesquiterpenoid Regulation. Front. Genet. 11:1027. doi: 10.3389/fgene.2020.01027
Received: 29 May 2020; Accepted: 11 August 2020;
Published: 10 September 2020.
Edited by:
Zhongxia Wu, Henan University, ChinaReviewed by:
Wen Liu, Huazhong Agricultural University, ChinaDeng Huimin, South China Normal University, China
Copyright © 2020 Tsang, Law, Li, Qu, Bendena, Tobe and Hui. This is an open-access article distributed under the terms of the Creative Commons Attribution License (CC BY). The use, distribution or reproduction in other forums is permitted, provided the original author(s) and the copyright owner(s) are credited and that the original publication in this journal is cited, in accordance with accepted academic practice. No use, distribution or reproduction is permitted which does not comply with these terms.
*Correspondence: Jerome H. L. Hui, jeromehui@cuhk.edu.hk
†These authors have contributed equally to this work