- 1Department of Otorhinolaryngology—Head and Neck Surgery, University of Maryland School of Medicine, Baltimore, MD, United States
- 2The Institute of Biochemistry and Biotechnology, University of Veterinary and Animal Sciences, Lahore, Pakistan
- 3Department of Biotechnology, Virtual University of Pakistan, Lahore, Pakistan
Otitis media (OM) is an infective and inflammatory disorder known to be a major cause of hearing impairment across all age groups. Both acute and chronic OM result in substantial healthcare utilization related to antibiotic prescription and surgical procedures necessary for its management. Although several studies provided evidence of genetics playing a significant role in the susceptibility to OM, we had limited knowledge about the genes associated with OM until recently. Here we have summarized the known genetic factors that confer susceptibility to various forms of OM in mice and in humans and their genetic load, along with associated cellular signaling pathways. Spotlighted in this review are fucosyltransferase (FUT) enzymes, which have been implicated in the pathogenesis of OM. A comprehensive understanding of the functions of OM-associated genes may provide potential opportunities for its diagnosis and treatment.
Introduction
Otitis media (OM) is defined as an infective and inflammatory disorder of the middle ear. While OM is associated with significant heterogeneity in clinical presentation, the broad types include acute otitis media (AOM), chronic suppurative otitis media (CSOM), and chronic otitis media with effusion (OME). Previous studies have shown that the pooled incidence of AOM is about 11% worldwide, with 51% of cases occurring in children under the age of five (Monasta et al., 2012). The recurrence of AOM may lead to OME, which has a worldwide incidence rate of up to 5% (Monasta et al., 2012). Both AOM and OM continue to be associated with healthcare utilization in the form of antibiotic therapy, physician and emergency room visits, and common surgical procedures such as tympanostomy tubes, although the advent of effective antimicrobial therapy has led to a substantial reduction in the burden of CSOM (Thomas et al., 2004). A smaller number of studies have also described an association between middle ear infections and speech and language deficits, emphasizing the role of OM in childhood development (Roberts et al., 2004).
The most common bacteria isolated from the middle ear of patients with AOM include Streptococcus pneumoniae and Haemophilus influenzae, although Moraxella catarrhalis, Streptococcus pyogenes, and Staphylococcus aureus are less frequently observed. In contrast, Pseudomonas aeruginosa and S. aureus are the most frequently observed pathogens in CSOM (Giebink and Canafax, 1991).
OM is a multifactorial disorder that may be attributed to a combination of etiologic factors including immunologic, genetic, environmental, and anatomic characteristics. Seasonal microbial susceptibility and Eustachian tube dysfunction are the commonly observed causes (Swanson and Hoecker, 1996; Fireman, 1997). It is well-known that viruses from the respiratory airways also play a crucial role in the pathogenesis of AOM (Nokso-Koivisto et al., 2015). Further, exposure to tobacco smoke, the use of a pacifier, and daycare attendance are among the risk factors for OM, while breastfeeding and pneumococcal vaccines have protective effects (Swanson and Hoecker, 1996; Lubianca Neto et al., 2006; Abrahams and Labbok, 2011; Norhayati et al., 2017).
Beyond environmental factors, genetic background also confers susceptibility to OM, although the disease mechanism is not fully understood. Several OM-associated genes, identified through studies in humans and in animal models, are known to play fundamental roles in diverse biological processes including (1) the development of the middle ear cleft and the Eustachian tube, (2) immune response, (3) bacterial adhesion and viral infection rate, (4) regulation of extracellular matrix, and (5) clearance of pathogens (see Tables 1, 2 for specific studies). In this review, we summarized the genomic variants and factors that have been reported in patients with various forms of OM. Early genetic association studies, mouse, mouse-to-man, human candidate, and genome-wide association studies that correlate OM and genetic variations are also briefly discussed. However, we particularly focused on the recent findings of the associations of A2ML1 and FUT enzymes with OM and offered our perspective on the potential disease mechanism that intuitively can lead to OM in individuals harboring variants of FUT2.
Early Studies
The genetic contribution to OM susceptibility became evident in the 1980s after several studies showed that the prevalence of OM was disproportionately high in some ethnicities (native Americans and Australian aborigines) and relatively low in individuals of African ancestry (Clements, 1968; Bhutta, 2015). A surveillance study on ear and nasopharyngeal carriage was conducted among remote Australian aboriginal communities in 2013 and found that 50% of young children (mean age 13 months) had OME, 37% had AOM, and 12% had CSOM (Leach et al., 2016). Today, CSOM continues to be strongly implicated in the prevalence of hearing and learning disorders in Australian aboriginal communities (Morris, 1998).
One of the earliest genetic studies on OM, conducted in 1983, analyzed the blood groups (ABO) in a cohort of 610 children with chronic otitis media with effusion (COME) and concluded that blood group “A” was a genetic risk factor for OM based on their observation of its higher prevalence in children with COME as compared to non-affected children (Mortensen et al., 1983). Later studies have shown that human leukocyte antigen (HLA) 2 and HLA3 are strongly associated with AOM, while patients with COME have a lower frequency of HLA2 (Kalm et al., 1991, 1994). The heritability and genetic components of time with and the number of episodes with OME and AOM during the first 2 years of life were also investigated in a twin and a triplet study in 1999 and found a strong association between the duration or the number of episodes of OM and genetic makeup (Casselbrant et al., 1999).
The contribution of genetics to OM susceptibility is supported by studies reporting a higher incidence of OM in children with chromosomal abnormalities. For example, the prevalence of OME in children with Down syndrome approaches 38% (Austeng et al., 2013). Genes present on chromosome 21 in combination with craniofacial defects such as midfacial hypoplasia, short palate, and Eustachian tube dysfunction (Shibahara and Sando, 1989) and defects of the immune system (Ram and Chinen, 2011) observed in children with Down syndrome may contribute to their increased risk of OM. Ets1 gene, encoding a proto-oncogene, has been recently associated with craniofacial abnormalities and OM in a mouse study (see section Mouse and Mouse-to-Man Studies) (Carpinelli et al., 2015). In humans, the ETS2 gene that also belongs to the proto-oncogene gene family is present on chromosome 21 and may contribute to OM susceptibility in Down syndrome.
Several studies conducted on cohorts with Turner syndrome, a genetic disorder of partial or complete loss of chromosome X in females, described a highly variable (ranging from 9.1 to 91%) incidence of AOM (Sculerati et al., 1990; Bois et al., 2018). While the karyotype analysis did not reveal any significantly high-risk subgroup, females with Turner syndrome also have greater prevalence and longer duration of middle ear pathologies (Gawron et al., 2008; Bois et al., 2018). These findings implicate some of the X chromosome genes in middle ear development, function, or health.
Mouse and Mouse-to-Man Studies
The development and the phenotyping of transgenic and knockout mouse models in the last 30 years have significantly helped to identify several genes and genetic variations that confer susceptibility to OM in mice. Most of these mouse models spontaneously develop OM; studying their ear morphology and function provided insights into the disease pathophysiology at a molecular level. For instance, Eriksson et al. (2006) showed that plasminogen (Plg)-deficient mice spontaneously develop chronic OM by 18 weeks of age. Plasmin, the active serine proteinase enzyme form of PLG, is mainly involved in the dissociation of fibrin clots and promotes the degradation of the extracellular matrix (Ayon-Nunez et al., 2018). Plasmin plays a critical role in several cellular processes, including wound healing, immunity, tissue remodeling, inflammation, and cell migration (Tefs et al., 2006). Recent studies have shown that certain bacteria possess plasminogen-binding adhesions on their cell surface to exploit the fibrinolytic system, facilitating bacterial uptake and invasion (Raymond and Djordjevic, 2015; Ayon-Nunez et al., 2018).
The role of transcription factors in OM pathology became apparent through the studies of mutant mice lacking Eya4, Evi1, Tgif, Ets1, and Fli1 genes (Hardisty-Hughes et al., 2006; Parkinson et al., 2006; Depreux et al., 2008; Tateossian et al., 2013; Carpinelli et al., 2015). Mice lacking Eya4 have Eustachian tube dysfunction, leading to an increased incidence of OME and hearing impairment (Depreux et al., 2008). Variants in Evi1 in Junbo mice have been shown to cause susceptibility to CSOM. Junbo mice accumulate middle ear effusions and develop hypoxia, inflammation, and thickening of the mucoperiosteum (Parkinson et al., 2006; Bhutta et al., 2014). Later studies have shown that the loss of BPIFA1, one of the most abundant secretory proteins in the upper respiratory tract (Musa et al., 2012), exacerbates the severity of OM in Junbo mice. While Bpifa1 mutant mice did not show any OM susceptibility, the deletion of Bpifa1 in mice carrying Evi1 Junbo variant leads to the thickening of the middle ear mucosa and an increase of collagen deposition (Mulay et al., 2018). Loss of Tgif1, which encodes for TGIF1, results in OME accompanied by the thickening of the middle ear epithelial lining, an increase of goblet cell population, elevated levels of TNF-α and IL-1β in ear fluids, and conductive hearing loss in mice (Tateossian et al., 2013). Similarly, haploinsufficiency for Ets1 and Fli1 in mice results in craniofacial abnormalities, including a smaller middle ear cavity and fusion of ossicles to the walls of the middle ear (Carpinelli et al., 2015). Furthermore, Fli1± and Ets1± double-mutant mice have hearing impairment and their middle ear mucosa is infiltrated by proinflammatory cells, leading to OM (Carpinelli et al., 2015).
Hardisty et al. (2003) showed that Jeff mutant mice carrying a Fbxo11 variant have craniofacial abnormalities, elevated hearing thresholds, and middle ear effusion. Defects in the bulla cavitation were observed in Fbxo11 mutant mice, which ultimately result in middle ear adhesions and soft tissue mineralization of the bony anatomy (Del-Pozo et al., 2019). Using N-ethyl-N-nitrosourea mutagenesis, Crompton et al. (2017) showed that the pathogenic variant, p.Leu972Pro, also known as edison variant, in the Nischarin (Nisch) gene leads to mild craniofacial defects, spontaneous OM by 20 weeks, and progressive hearing loss. Recent studies have reported the association of TGIF1 and NISCH loci as potential risk areas for OM in humans (Bhutta et al., 2017), thus supporting the relevance of knowledge obtained from mouse models to the pathophysiology of OM in humans.
Finally, Eda and Edar transcription factors mutant mice (EdaTa and EdardlJ/dlJ) also developed chronic rhinitis and OM (Azar et al., 2016). In these mutants, the nasopharyngeal glandular epithelium fails to develop, which leads to the loss of lysozyme secretion, the reduction of mucociliary clearance, and the overgrowth of commensal bacteria. The spread of nasal S. aureus in EdaTa mice and of Escherichia coli in EdardlJ/dlJ mice into the middle ear bulla potentially triggers inflammation and OM (Azar et al., 2016). A non-exhaustive list of the most common loci associated with OM in mouse is presented in Table 1.
Human Candidate Gene-Based and Genome-Wide Association Studies
While early candidate gene-based OM studies have been done mostly on Caucasian patients with recurrent AOM and chronic OME (see section Early Studies), more recent genetic studies have been focusing on ethnic groups or communities for which marriages within the families are relatively common (e.g., indigenous Filipino community or Pakistani families) (Santos-Cortez et al., 2015, 2018). These studies have unveiled several novel genes and variants that confer susceptibility to familial OM (see section OM Susceptibility, Inbreeding, and Whole-Exome Sequencing): A2ML1 and FUT2 (Santos-Cortez et al., 2015, 2018).
Candidate Gene-Based Studies
Many variants in the genome have been associated with infectious diseases (Klebanov, 2018). In some instances, the clinical features and the biological mechanisms – such as immune response, inflammation, bacterial adhesion, viral infection, and mucociliary clearance – involved in those infectious diseases are compatible with the mechanisms involved during an episode of OM, thus marking the genes known for these disorders as prime candidates for OM susceptibility and recurrence. Candidate gene-based studies on OM have mainly involved genes associated with innate immunity and inflammation (Sale et al., 2011). Those studies have been performed on cohorts from all over the world including US, Finland, Australia, Netherlands, Greece, and Belgium and have identified over 100 alleles that confer susceptibility to various forms of OM (see Table 2 for the partial list of these alleles, genes, and associated clinical features). As evident from this non-exhaustive list, the identified genes belong to several different signaling cascades and developmental processes, including (a) immune response and inflammation (MBL2, TLR2, TLR4, CD14, FCGR2A, TGFB1, and PAI1) (Nuytinck et al., 2006; Wiertsema et al., 2006a, b; Emonts et al., 2007; Lee Y.C. et al., 2008; Ilia et al., 2014; Hafren et al., 2015), (b) cytokines (IL6, IL10, IL1A, IL1B, TNFA, and IFNG) (Patel et al., 2006; Alper et al., 2009; Revai et al., 2009; Ilia et al., 2014), (c) tissue clearance (SFPTA, SFTPA1, SFTPD, SLC11A1, MUC2, MUC5AC, and MUC5B) (Ramet et al., 2001; Sale et al., 2011; Rye et al., 2013; MacArthur et al., 2014), (d) transcriptional modulation (SMAD2 and SMAD4) (Rye et al., 2011), (e) chemosensitivity (CX3CR1) (Nokso-Koivisto et al., 2014), (f) protein modification (CPT1A and FBXO11) (Rye et al., 2011, 2012), and (g) channel activity (SCN1B) (Sale et al., 2011). Some of these genes, such as FBXO11, have been replicated in several independent studies, which further strengthen their role in susceptibility to OM (Segade et al., 2006; Rye et al., 2011).
Genome-Wide Association Studies
Several genome-wide association studies (GWAS) have been performed to identify new common (frequency of 75% or greater) low-risk markers (OR < 1.5) associated with OM subtypes. The findings of five salient GWAS are summarized here. In the Western Australian Pregnancy Cohort (Raine) study, a cohort of 416 patients prone to OM and 1,075 normal subjects was analyzed for 2,524,817 SNPs. Although the initial analysis revealed some association, no SNP reached GWAS significance (P < 10–8) nor could be replicated both in the Australian or US cohorts (Rye et al., 2012; Allen et al., 2014). Intriguingly, the GWAS of the Minnesota and Pittsburg cohorts identified a SNP (rs10497394 on chromosome 2) that showed a significant association (GWAS discovery P = 1.30 × 10–5, independent otitis media population Pmeta = 1.52 × 10–8) with susceptibility to either chronic OME or recurrent AOM (Allen et al., 2013). Finally, in a Finnish cohort (829 affected children and 2,118 randomly selected controls), the variants rs16974263 (GWAS discovery P = 1.77 × 10–7, sub-phenotype analysis Pmeta = 2.92 × 10–8), rs268662 (P = 1.564 × 10–6), and rs4150992 (P = 3.37 × 10–6) were the most significant variants associated with COME (Einarsdottir et al., 2016). In van Ingen et al. (2016) performed GWAS on a cohort of AOM children of European descent and reported a statistically significant association at 6q25.3 locus (rs2932989, Pmeta = 2.15 × 10–9). This study further demonstrated that the associated variants are correlated with the methylation status (cg05678571, p = 1.43 × 10–6) and expression levels (p = 9.3 × 10–5) of the FNDC1 gene. Also, an independent GWAS study on more than 200,000 individuals of European ancestry reported 14 genomic regions, including FUT2 (p-value: 3.51 × 10–30), TBX1 (1.17 × 10–19), HLA-DRB1 (rs4329147, 9.55 × 10–12), ABO (3.67 × 10–11), EFEMP1 (1.47 × 10–10), AUTS2 (3.75 × 10–9), CDHR3 (5.40 × 10–9), BSN (1.56 × 10–8), and PLG (3.78 × 10–8), that were significantly associated with childhood ear infection (Tian et al., 2017), further highlighting the contribution of genetic factors responsible for OM in humans.
OM Susceptibility, Inbreeding, and Whole-Exome Sequencing
A2ML1
In a large consanguineous indigenous Filipino pedigree with a high frequency of OM, Santos-Cortez et al. (2015) showed, by whole-exome and Sanger sequencing, that an 8 bp duplication in the A2ML1 gene (LOD score of 7.5) was associated with susceptibility to OM. The same duplication was found in a heterozygous or a homozygous fashion in three individuals (European American and Hispanic) of another cohort of 123 children prone to OM and absent in non-otitis-prone children and more than 62,000 next-generation sequences. The authors identified seven additional heterozygous A2ML1 variants in patients of European American and Hispanic American origin with OM. A2ML1 encodes alpha-2-macroglobulin-like protein 1 (A2ML1), a protein that traps proteinases and cleaves them. A follow-up study identified 16 additional A2ML1 variants in OM subjects in indigenous Filipino and Pakistani families and US probands (Larson et al., 2019). Based on the expression in the murine mucosal epithelium of the middle ear, it has been speculated that A2ML1 may have a protective function by regulating the proteases present in the middle ear cavity and may also regulate the desquamation of epidermis (Galliano et al., 2006). Recently, by 16S rRNA sequencing of the microbiota of the middle ear of an indigenous Filipino community prone to OM and segregating A2ML1 variants, although not having a statistically significant difference between the cases and the controls, a taxonomic analysis revealed the relative abundance of the phyla Fusobacteria and Bacteroidetes and the genus Fusobacterium in A2ML1 carriers compared to non-carriers (Santos-Cortez et al., 2016).
FUT2
In the same large consanguineous indigenous Filipino pedigree with high frequency of OM, partly due to variants in A2ML1, a subset of individuals were wild type for A2ML1 but were prone to OM (Santos-Cortez et al., 2015). Further genetic analysis determined that the FUT2 variant (p.Arg202∗, LOD score of 4.0) confer susceptibility to OM in those individuals (Santos-Cortez et al., 2018). Screening of DNA samples from 609 additional multi-ethnic families and simplex case subjects with OM by direct Sanger sequencing, linkage analysis, Fisher exact, and transmission disequilibrium tests revealed several other FUT2 variants (p.Arg138Cys, p.Trp154∗, and p.Ala104Val) that confer susceptibility to OM (Santos-Cortez et al., 2018).
FUT enzymes are involved in the protein glycosylation pathway. FUTs transfer an L-fucose sugar derived from GDP-fucose (donor substrate) to a protein (acceptor substrate). The FUT family contains 13 members (FUT1–FUT13), and many of the FUTs are essential for the synthesis of blood group antigens. FUTs are single-pass type II membrane proteins, resident to the trans-Golgi, while the catalytic domain of FUT proteins resides in the lumen of the Golgi. In humans, FUT1 and FUT2 encode galactoside 2-L-fucosyltransferase, while FUT3 encodes galactoside 3(4)-L-fucosyltransferase. FUT1 and FUT2 transfer L-fucose onto a β-D-galactosyl-(1→4)-N-acetyl-β-D-glucosaminyl derivative and create the oligosaccharide FuC-alpha [(1,2)Gal-beta-], also known as H antigen, which is a soluble precursor essential for the final step in the soluble A and B antigen synthesis pathway. FUT3 transfers L-fucose onto a β-D-galactosyl-(1→4)-N-acetyl-β-D-glucosaminyl derivative, or onto H antigen, in order to create blood group Lewis antigens.
FUT1 and FUT2 are differentially expressed in various cell types. For instance, FUT1 expression is restricted to cells of mesodermal origin (for example, erythrocytes), and FUT2 expression is being restricted to cells of endodermal origin (such as the middle ear mucosal cells). Therefore, A and B antigens will be expressed at the surface of red blood cells under the control of FUT1, while A and B antigens will be expressed at the surface of mucosal cells under the control of FUT2. Genetic variations in FUT1 and FUT2 naturally exist. Some FUT1 and FUT2 variants lead to non-functional enzymes, while certain variations in FUT2 can also lead to a reduction of its expression (Santos-Cortez et al., 2018). For instance, the FUT1 p.Tyr154Cys variant ablates the functional activity of the catalytic domain, resulting in the absence of A, B, or H antigens at the surface of erythrocytes (also known as the Bombay phenotype). Similarly, the FUT2 p.Trp154∗ variant causes absence of A, B, or H antigens at the surface of mucosal cells (a.k.a. non-secretor status) (Domino et al., 2001a, b). The p.Trp154∗ variant of FUT2 is also responsible for the non-secretor phenotype in European and African populations (47 and 42%, respectively).
A, B, and H and Lewis antigens are known to serve as an energy source while also regulating the adhesion of bacteria to the cell surface (Ewald and Sumner, 2018; Figure 1). Intuitively, the different blood group antigens and their quantity at the surface of the cells of the mucosal epithelium of the middle ear would have an impact on the microbiota present in the middle ear cavity, and various blood types have been associated with OM (Wiesen et al., 2019). For instance, studies in a Finnish cohort reported a protective effect of blood type O against recurrent AOM, whereas blood type A was associated with increased risk for chronic OME (Wiesen et al., 2019). When tested in vitro, the four FUT2 variants associated with OM (p.Ala104Val, p.Arg138Cys, p.Trp154∗, and p.Arg202∗) reduced the A antigen levels, while the two nonsense variants also reduced the FUT2 protein levels. Moreover, Fut2 is transiently upregulated in the murine middle ear after inoculation with the non-typeable H. influenza (Santos-Cortez et al., 2018). It is speculated that the OM-associated FUT2 variants are modifying the middle ear microbiome through the regulation of A antigen levels in the middle ear mucosa, thus conferring susceptibility to OM (Santos-Cortez et al., 2018).
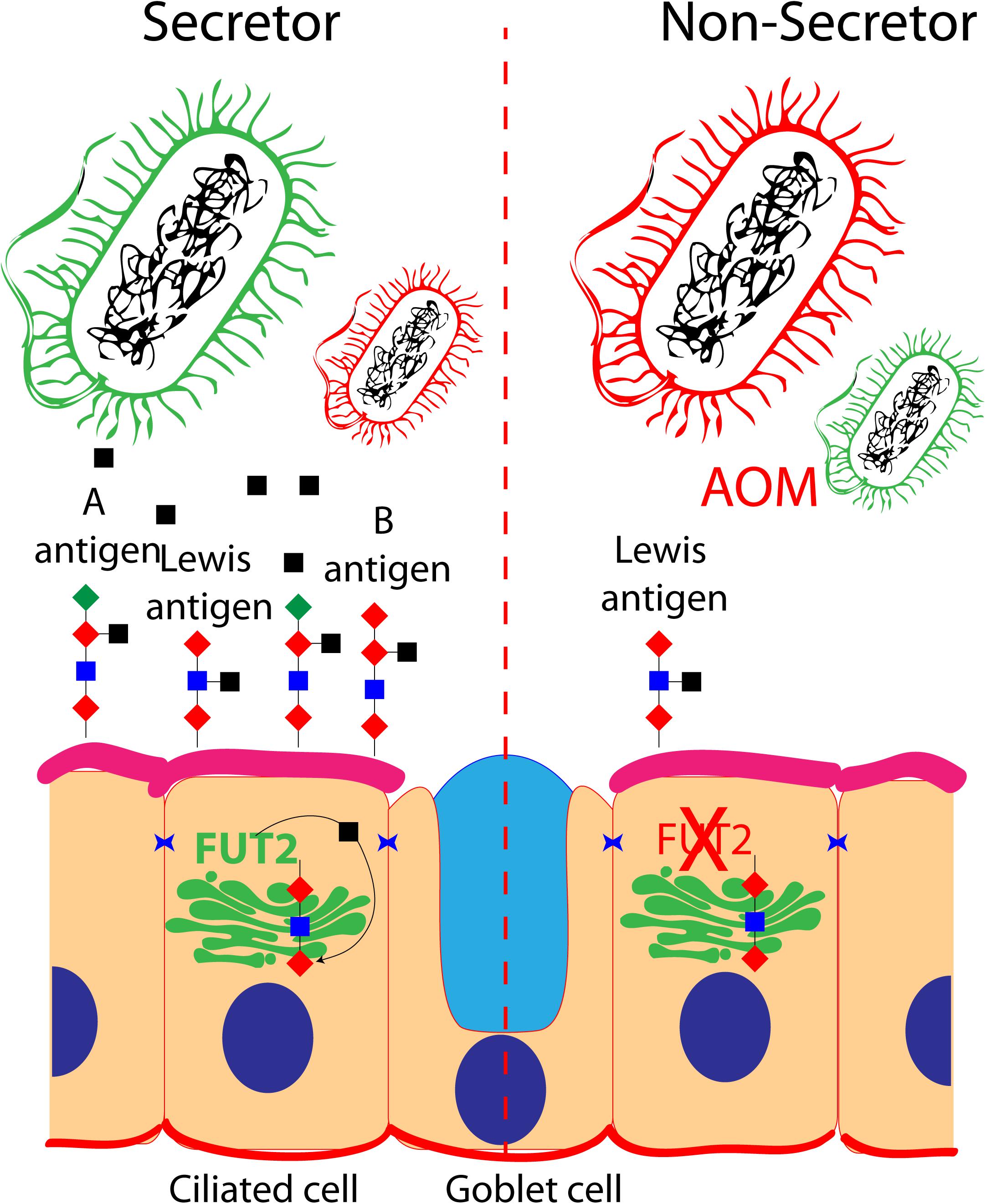
Figure 1. Role of FUT2 in the regulation of blood group and Lewis antigens at the surface of ciliated cells of the middle ear mucosa: secretor and non-secretor status are illustrated. The imbalance of optimal bacteria (green) and pathogenic bacteria (red) is shown in a non-secretor individual that is prone to acute otitis media.
While the microbial richness, structure, and composition differences were not statistically significant between the control individuals and the individuals prone to OM in the indigenous Filipino community segregating FUT2 p.Arg202∗ variant, the individuals from a Colorado cohort prone to OM and carrier for the FUT2 p.Trp154∗ variant had a relatively high abundance of Lactobacillales and Gamma-proteobacteria in their middle ears (Santos-Cortez et al., 2018). Further studies in animal models are necessary to fully understand the FUT2-associated OM mechanism.
Conclusion
The current genetic and molecular data revealed the association of OM with deficits in each of the following mechanisms: (1) development of the middle ear cavity and Eustachian tube, (2) immune response, (3) bacterial adhesion and viral infection, (4) regulation of the extracellular matrix, and (5) clearance of the middle ear.
In Figure 2, we have attempted to build a network that encompasses most of the known human proteins that have been associated with OM and our hypothesis about the potential impact on the microbiome of the middle ear cavity when these proteins are dysfunctional due to OM-associated genetic variants. Briefly, ABO and FUT2 are localized in the Golgi apparatus of the cells of the middle ear mucosa; these two proteins together create blood group Lewis antigens, potential sources of energy for microbiome. These antigens also provide an adhesion platform for the microbiota. Similarly, goblet cells secrete MUC5AC, MUC5B, MUC2 SFPTA, SFPTA1, and SFPTD to form mucus and surfactants in the middle ear cavity. Bacteria are present in these secretions and are cleared from the middle ear cavity by ciliated cells in order to maintain a healthy microbiome. Cells from the middle ear also secrete interleukins, chemokines, interferons, and necrosis and growth factors in order to recruit immune cells in the extracellular matrix. These cells fight infection and eliminate dead cells. During the inflammatory stage, the extracellular matrix is remodeled by proteases to allow immune cell infiltration. Those proteases are regulated and inhibited by A2ML1 and PAI1. A pathogenic genetic variation in any of those genes would eventually lead to middle ear infection and OM.
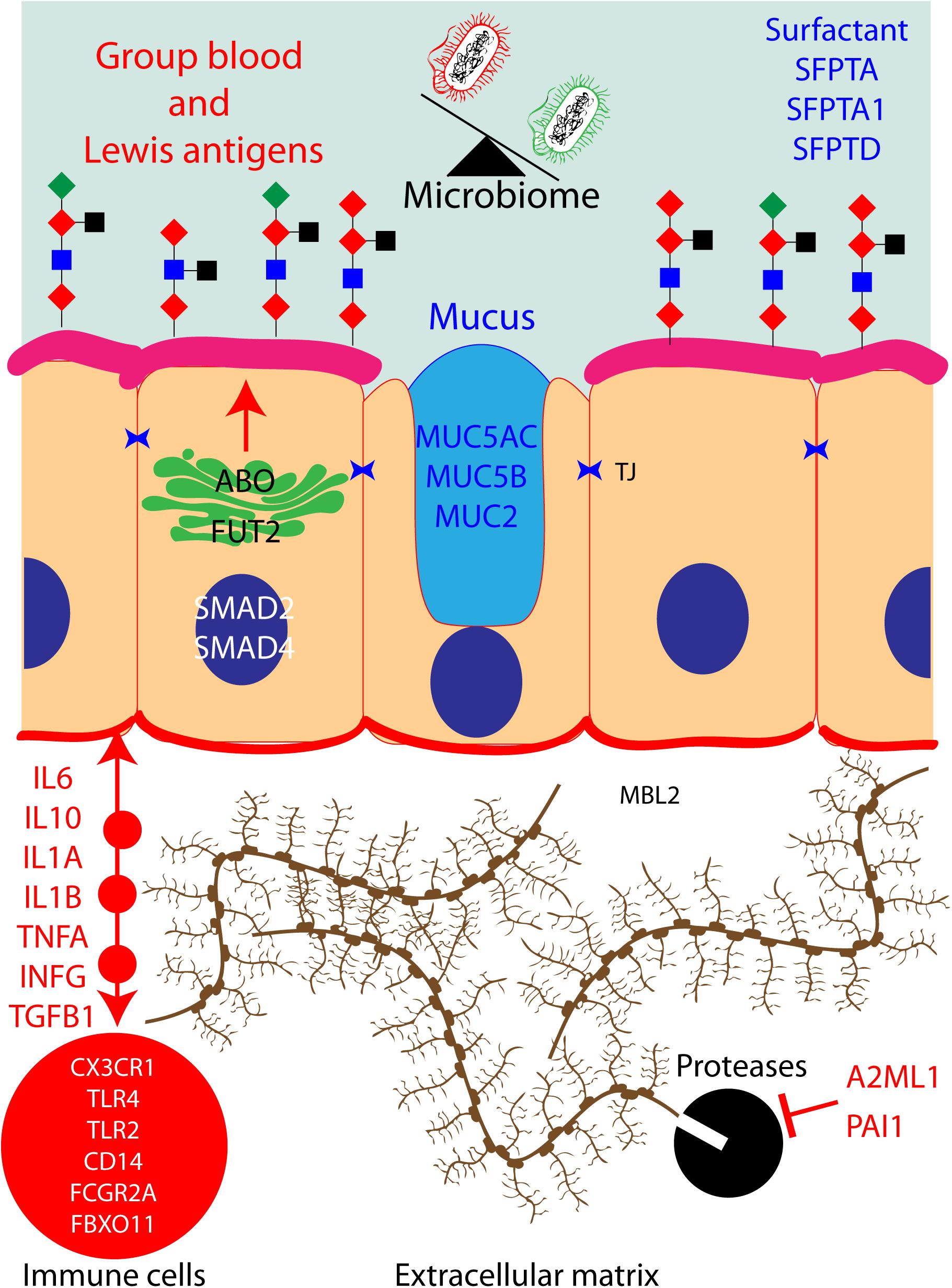
Figure 2. Protein pathways contributing to otitis media (OM) in human: hypothetical network of human proteins in the middle ear mucosa that have been associated with OM. When these proteins are dysfunctional due to OM-associated genetic variants, they could potentially have an impact on the microbiota of the middle ear cavity.
Future studies of the enrichment of certain microbiota in individuals with specific genetic variants may eventually help in identifying patients before chronic OM sets in or in devising a patient-specific treatment paradigm in the future.
Author Contributions
AG, SA, AI, IA, SR, and ZA wrote the draft and finalized it.
Funding
Research in the Ahmed Laboratory was supported by the National Institutes of Health/NIDCD R01DC012564 and R01DC016295.
Conflict of Interest
The authors declare that the research was conducted in the absence of any commercial or financial relationships that could be construed as a potential conflict of interest.
Acknowledgments
We thank Ms. Bernadine Monari, Dimitria Gomes, and Dr. Elodie Richard for their critiques of the manuscript.
References
Abrahams, S. W., and Labbok, M. H. (2011). Breastfeeding and otitis media: a review of recent evidence. Curr. Allergy Asthma Rep. 11, 508–512. doi: 10.1007/s11882-011-0218-3
Allen, E. K., Chen, W. M., Weeks, D. E., Chen, F., Hou, X., Mattos, J. L., et al. (2013). A genome-wide association study of chronic otitis media with effusion and recurrent otitis media identifies a novel susceptibility locus on chromosome 2. J. Assoc. Res. Otolaryngol. 14, 791–800. doi: 10.1007/s10162-013-0411-2
Allen, E. K., Manichaikul, A., Chen, W. M., Rich, S. S., Daly, K. A., Sale, M. M., et al. (2014). Evaluation of replication of variants associated with genetic risk of otitis media. PLoS One 9:e104212. doi: 10.1371/journal.pone.0104212
Alper, C. M., Winther, B., Hendley, J. O., and Doyle, W. J. (2009). Cytokine polymorphisms predict the frequency of otitis media as a complication of rhinovirus and RSV infections in children. Eur. Arch. Otorhinolaryngol. 266, 199–205. doi: 10.1007/s00405-008-0729-2
Austeng, M. E., Akre, H., Øverland, B., Abdelnoor, M., Falkenberg, E. S., and Kværner, K. J. (2013). Otitis media with effusion in children with in Down syndrome. Int. J. Pediatr. Otorhinolaryngol. 77, 1329–1332. doi: 10.1016/j.ijporl.2013.05.027
Ayon-Nunez, D. A., Fragoso, G., Bobes, R. J., and Laclette, J. (2018). Plasminogen-binding proteins as an evasion mechanism of the host’s innate immunity in infectious diseases∗. Biosci. Rep. 38,
Azar, A., Piccinelli, C., Brown, H., Headon, D., and Cheeseman, M. (2016). Ectodysplasin signalling deficiency in mouse models of hypohidrotic ectodermal dysplasia leads to middle ear and nasal pathology. Hum. Mol. Genet. 25, 3564–3577. doi: 10.1093/hmg/ddw202
Bhutta, M. F. (2015). Evolution and otitis media: a review, and a model to explain high prevalence in indigenous populations. Hum. Biol. 87, 92–108. doi: 10.13110/humanbiology.87.2.0092
Bhutta, M. F., Cheeseman, M. T., and Brown, S. D. (2014). Myringotomy in the Junbo mouse model of chronic otitis media alleviates inflammation and cellular hypoxia. Laryngoscope 124, E377–E383. doi: 10.1002/lary.24698
Bhutta, M. F., Lambie, J., Hobson, L., Goel, A., Hafrén, L., Einarsdottir, E., et al. (2017). A mouse-to-man candidate gene study identifies association of chronic otitis media with the loci TGIF1 and FBXO11. Sci. Rep. 7:12496. doi: 10.1038/s41598-017-12784-8
Bois, E., Nassar, M., Zenaty, D., Léger, J., Van Den Abbeele, T., and Teissier, N. (2018). Otologic disorders in Turner syndrome. Eur. Ann. Otorhinolaryngol. Head Neck Dis. 135, 21–24. doi: 10.1016/j.anorl.2017.08.006
Carpinelli, M. R., Kruse, E. A., Arhatari, B. D., Debrincat, M. A., Ogier, J. M., Bories, J. C., et al. (2015). Mice haploinsufficient for Ets1 and Fli1 display middle ear abnormalities and model aspects of jacobsen syndrome. Am. J. Pathol. 185, 1867–1876. doi: 10.1016/j.ajpath.2015.03.026
Casselbrant, M. L., Mandel, E. M., Fall, P. A., Rockette, H. E., Kurs-Lasky, M., Bluestone, C. D., et al. (1999). The heritability of otitis media: a twin and triplet study. JAMA 282, 2125–2130.
Cheeseman, M. T., Tyrer, H. E., Williams, D., Hough, T. A., Pathak, P., Romero, M. R., et al. (2011). HIF-VEGF pathways are critical for chronic otitis media in Junbo and Jeff mouse mutants. PLoS Genet. 7:e1002336. doi: 10.1371/journal.pgen.1002336
Chen, J., Ingham, N., Clare, S., Raisen, C., Vancollie, V. E., Ismail, O., et al. (2013). Mcph1-deficient mice reveal a role for MCPH1 in otitis media. PLoS One 8:e58156. doi: 10.1371/journal.pone.0058156
Clements, D. A. (1968). Otitis media and hearing loss in a small aboriginal community. Med. J. Aust. 1, 665–667. doi: 10.5694/j.1326-5377.1968.tb28789.x
Crompton, M., Purnell, T., Tyrer, H. E., Parker, A., Ball, G., Hardisty-Hughes, R. E., et al. (2017). A mutation in Nischarin causes otitis media via LIMK1 and NF-kappaB pathways. PLoS Genet. 13:e1006969. doi: 10.1371/journal.pgen.1006969
Del-Pozo, J., MacIntyre, N., Azar, A., Glover, J., Milne, E., and Cheeseman, M. (2019). Chronic otitis media is initiated by a bulla cavitation defect in the FBXO11 mouse model∗. Dis. Model Mech. 12, doi: 10.1242/dmm.038315
Depreux, F. F., Darrow, K., Conner, D. A., Eavey, R. D., Liberman, M. C., Seidman, C. E., et al. (2008). Eya4-deficient mice are a model for heritable otitis media. J. Clin. Invest. 118, 651–658. doi: 10.1172/JCI32899
Domino, S. E., Zhang, L., and Lowe, J. B. (2001b). Molecular cloning, genomic mapping, and expression of two secretor blood group alpha (1,2)fucosyltransferase genes differentially regulated in mouse uterine epithelium and gastrointestinal tract. J. Biol. Chem. 276, 23748–23756. doi: 10.1074/jbc.m100735200
Domino, S. E., Zhang, L., Gillespie, P. J., Saunders, T. L., and Lowe, J. B. (2001a). Deficiency of reproductive tract alpha(1,2)fucosylated glycans and normal fertility in mice with targeted deletions of the FUT1 or FUT2 alpha(1,2)fucosyltransferase locus. Mol. Cell Biol. 21, 8336–8345. doi: 10.1128/mcb.21.24.8336-8345.2001
Einarsdottir, E., Hafrén, L., Leinonen, E., Bhutta, M. F., Kentala, E., Kere, J., et al. (2016). Genome-wide association analysis reveals variants on chromosome 19 that contribute to childhood risk of chronic otitis media with effusion. Sci. Rep. 6:33240. doi: 10.1038/srep33240
Emonts, M., Veenhoven, R. H., Wiertsema, S. P., Houwing-Duistermaat, J. J., Walraven, V., de Groot, R., et al. (2007). Genetic polymorphisms in immunoresponse genes TNFA, IL6, IL10, and TLR4 are associated with recurrent acute otitis media. Pediatrics 120, 814–823. doi: 10.1542/peds.2007-0524
Eriksson, P. O., Li, J., Ny, T., and Hellström, S. (2006). Spontaneous development of otitis media in plasminogen-deficient mice. Int. J. Med. Microbiol. 296, 501–509. doi: 10.1016/j.ijmm.2006.04.002
Ewald, D. R., and Sumner, S. C. J. (2018). Human microbiota, blood group antigens, and disease. Wiley Interdiscip. Rev. Syst. Biol. Med. 10:e1413. doi: 10.1002/wsbm.1413
Fireman, P. (1997). Otitis media and eustachian tube dysfunction: connection to allergic rhinitis. J. Allergy Clin. Immunol. 99, S787–S797.
Galliano, M. F., Toulza, E., Gallinaro, H., Jonca, N., Ishida-Yamamoto, A., Serre, G., et al. (2006). A novel protease inhibitor of the alpha2-macroglobulin family expressed in the human epidermis. J. Biol. Chem. 281, 5780–5789. doi: 10.1074/jbc.m508017200
Gawron, W., Wikiera, B., Rostkowska-Nadolska, B., Orendorz-Fra̧czkowska, K., and Noczyńska, A. (2008). Evaluation of hearing organ in patients with Turner syndrome. Int. J. Pediatr. Otorhinolaryngol. 72, 575–579. doi: 10.1016/j.ijporl.2008.01.021
Gentile, D. A., Doyle, W. J., Zeevi, A., Howe-Adams, J., Kapadia, S., Trecki, J., et al. (2003). Cytokine gene polymorphisms moderate illness severity in infants with respiratory syncytial virus infection. Hum. Immunol. 64, 338–344. doi: 10.1016/s0198-8859(02)00827-3
Gessner, B. D., Gillingham, M. B., Wood, T., and Koeller, D. M. (2013). Association of a genetic variant of carnitine palmitoyltransferase 1A with infections in Alaska Native children. J. Pediatr. 163, 1716–1721. doi: 10.1016/j.jpeds.2013.07.010
Giebink, G. S., and Canafax, D. M. (1991). Antimicrobial treatment of otitis media. Semin. Respir. Infect. 6, 85–93.
Hafren, L., Einarsdottir, E., Kentala, E., Hammarén-Malmi, S., Bhutta, M. F., MacArthur, C. J., et al. (2015). Predisposition to childhood otitis media and genetic polymorphisms within the toll-like receptor 4 (TLR4) locus. PLoS One 10:e0132551. doi: 10.1371/journal.pone.0132551
Han, F., Yu, H., Li, P., Zhang, J., Tian, C., Li, H., et al. (2012). Mutation in Phex gene predisposes BALB/c-Phex(Hyp-Duk)/Y mice to otitis media. PLoS One 7:e43010. doi: 10.1371/journal.pone.0043010
Hardisty, R. E., Erven, A., Logan, K., Morse, S., Guionaud, S., Sancho-Oliver, S., et al. (2003). The deaf mouse mutant Jeff (Jf) is a single gene model of otitis media. J. Assoc. Res. Otolaryngol. 4, 130–138. doi: 10.1007/s10162-002-3015-9
Hardisty-Hughes, R. E., Tateossian, H., Morse, S. A., Romero, M. R., Middleton, A., Tymowska-Lalanne, Z., et al. (2006). A mutation in the F-box gene, Fbxo11, causes otitis media in the Jeff mouse. Hum. Mol. Genet. 15, 3273–3279. doi: 10.1093/hmg/ddl403
Hernandez, M., Leichtle, A., Pak, K., Ebmeyer, J., Euteneuer, S., Obonyo, M., et al. (2008). Myeloid differentiation primary response gene 88 is required for the resolution of otitis media. J. Infect. Dis. 198, 1862–1869. doi: 10.1086/593213
Ilia, S., Goulielmos, G. N., Samonis, G., and Galanakis, E. (2014). Polymorphisms in IL-6, IL-10, TNF-alpha, IFN-gamma and TGF-beta1 genes and susceptibility to acute otitis media in early infancy. Pediatr. Infect. Dis. J. 33, 518–521. doi: 10.1097/INF.0000000000000229
Joki-Erkkila, V. P., Puhakka, H., and Hurme, M. (2002). Cytokine gene polymorphism in recurrent acute otitis media. Arch. Otolaryngol. Head Neck Surg. 128, 17–20.
Kalm, O., Johnson, U., and Prellner, K. (1994). HLA frequency in patients with chronic secretory otitis media. Int. J. Pediatr. Otorhinolaryngol. 30, 151–157. doi: 10.1016/0165-5876(94)90198-8
Kalm, O., Johnson, U., Prellner, K., and Ninn, K. (1991). HLA frequency in patients with recurrent acute otitis media. Arch. Otolaryngol. Head Neck Surg. 117, 1296–1299. doi: 10.1001/archotol.1991.01870230112019
Kerschner, J. E., Hong, W., Taylor, S. R., Kerschner, J. A., Khampang, P., Wrege, K. C., et al. (2013). A novel model of spontaneous otitis media with effusion (OME) in the Oxgr1 knock-out mouse. Int. J. Pediatr. Otorhinolaryngol. 77, 79–84. doi: 10.1016/j.ijporl.2012.09.037
Klebanov, N. (2018). Genetic predisposition to infectious disease. Cureus 10:e3210. doi: 10.7759/cureus.3210
Larson, E. D., Magno, J. P. M., Steritz, M. J., Llanes, E. G. D. V., Cardwell, J., Pedro, M., et al. (2019). A2ML1 and otitis media: novel variants, differential expression, and relevant pathways. Hum. Mutat. 40, 1156–1171. doi: 10.1002/humu.23769
Leach, A. J., Wigger, C., Beissbarth, J., Woltring, D., Andrews, R., Chatfield, M. D., et al. (2016). General health, otitis media, nasopharyngeal carriage and middle ear microbiology in Northern Territory Aboriginal children vaccinated during consecutive periods of 10-valent or 13-valent pneumococcal conjugate vaccines. Int. J. Pediatr. Otorhinolaryngol. 86, 224–232. doi: 10.1016/j.ijporl.2016.05.011
Lee, H. Y., Takeshita, T., Shimada, J., Akopyan, A., Woo, J. I., Pan, H., et al. (2008). Induction of beta defensin 2 by NTHi requires TLR2 mediated MyD88 and IRAK-TRAF6-p38MAPK signaling pathway in human middle ear epithelial cells. BMC Infect. Dis. 8:87. doi: 10.1186/1471-2334-8-87
Lee, Y. C., Kim, C., Shim, J. S., Byun, J. Y., Park, M. S., Cha, C. I., et al. (2008). Toll-like receptors 2 and 4 and their mutations in patients with otitis media and middle ear effusion. Clin. Exp. Otorhinolaryngol. 1, 189–195. doi: 10.3342/ceo.2008.1.4.189
Leichtle, A., Hernandez, M., Ebmeyer, J., Yamasaki, K., Lai, Y., Radek, K., et al. (2010). CC chemokine ligand 3 overcomes the bacteriocidal and phagocytic defect of macrophages and hastens recovery from experimental otitis media in TNF-/- mice. J. Immunol. 184, 3087–3097. doi: 10.4049/jimmunol.0901167
Li, Q., Li, Y. X., Stahl, G. L., Thurman, J. M., He, Y., and Tong, H. H. (2011). Essential role of factor B of the alternative complement pathway in complement activation and opsonophagocytosis during acute pneumococcal otitis media in mice. Infect. Immun. 79, 2578–2585. doi: 10.1128/IAI.00168-11
Li, X., Xu, L., Li, J., Li, B., Bai, X., Strauss, J. F. I. I. I., et al. (2014). Otitis media in sperm-associated antigen 6 (Spag6)-deficient mice. PLoS One 9:e112879. doi: 10.1371/journal.pone.0112879
Lubianca Neto, J. F., Hemb, L., and Silva, D. B. (2006). Systematic literature review of modifiable risk factors for recurrent acute otitis media in childhood. J. Pediatr. 82, 87–96. doi: 10.2223/jped.1453
MacArthur, C. J., Wilmot, B., Wang, L., Schuller, M., Lighthall, J., and Trune, D. (2014). Genetic susceptibility to chronic otitis media with effusion: candidate gene single nucleotide polymorphisms. Laryngoscope 124, 1229–1235. doi: 10.1002/lary.24349
Maguire, S., Estabel, J., Ingham, N., Pearson, S., Ryder, E., Carragher, D. M., et al. (2014). Targeting of Slc25a21 is associated with orofacial defects and otitis media due to disrupted expression of a neighbouring gene. PLoS One 9:e91807. doi: 10.1371/journal.pone.0091807
Mitchell, C. R., Kempton, J. B., Scott-Tyler, B., and Trune, D. R. (1997). Otitis media incidence and impact on the auditory brain stem response in lipopolysaccharide-nonresponsive C3H/HeJ mice. Otolaryngol. Head Neck Surg. 117, 459–464. doi: 10.1016/s0194-5998(97)70014-7
Monasta, L., Ronfani, L., Marchetti, F., Montico, M., Vecchi Brumatti, L., Bavcar, A., et al. (2012). Burden of disease caused by otitis media: systematic review and global estimates. PLoS One 7:e36226.
Morris, P. S. (1998). A systematic review of clinical research addressing the prevalence, aetiology, diagnosis, prognosis and therapy of otitis media in Australian Aboriginal children. J. Paediatr. Child Health 34, 487–497. doi: 10.1046/j.1440-1754.1998.00299.x
Mortensen, E. H., Lildholdt, T., Gammelgård, N. P., and Christensen, P. H. (1983). Distribution of ABO blood groups in secretory otitis media and cholesteatoma. Clin. Otolaryngol. Allied Sci. 8, 263–265. doi: 10.1111/j.1365-2273.1983.tb01439.x
Mulay, A., Hood, D. W., Williams, D., Russell, C., Brown, S. D. M., Bingle, L., et al. (2018). Loss of the homeostatic protein BPIFA1, leads to exacerbation of otitis media severity in the Junbo mouse model. Sci. Rep. 8:3128. doi: 10.1038/s41598-018-21166-7
Musa, M., Wilson, K., Sun, L., Mulay, A., Bingle, L., Marriott, H. M., et al. (2012). Differential localisation of BPIFA1 (SPLUNC1) and BPIFB1 (LPLUNC1) in the nasal and oral cavities of mice. Cell Tissue Res. 350, 455–464. doi: 10.1007/s00441-012-1490-9
Nakamura, Y., Komori, M., Yamakawa, K., Hamajima, Y., Suzuki, M., Kim, Y., et al. (2013). Math1, retinoic acid, and TNF-alpha synergistically promote the differentiation of mucous cells in mouse middle ear epithelial cells in vitro. Pediatr. Res. 74, 259–265. doi: 10.1038/pr.2013.103
Nokso-Koivisto, J., Chonmaitree, T., Jennings, K., Matalon, R., Block, S., and Patel, J. A. (2014). Polymorphisms of immunity genes and susceptibility to otitis media in children. PLoS One 9:e93930. doi: 10.1371/journal.pone.0093930
Nokso-Koivisto, J., Marom, T., and Chonmaitree, T. (2015). Importance of viruses in acute otitis media. Curr. Opin. Pediatr. 27, 110–115. doi: 10.1097/MOP.0000000000000184
Norhayati, M. N., Ho, J. J., and Azman, M. Y. (2017). Influenza vaccines for preventing acute otitis media in infants and children. Cochrane Database Syst. Rev. 10:CD010089. doi: 10.1002/14651858.CD010089.pub3
Nuytinck, L., De Meester, E., and Van Thielen, M. (2006). Govaerts Role of mannose-binding lectin (MBL2) genotyping in predicting the risk of recurrent otitis media (rOM). Adv. Exp. Med. Biol. 586, 281–290. doi: 10.1007/0-387-34134-x_19
Parkinson, N., Hardisty-Hughes, R. E., Tateossian, H., Tsai, H. T., Brooker, D., Morse, S., et al. (2006). Mutation at the Evi1 locus in Junbo mice causes susceptibility to otitis media. PLoS Genet. 2:e149. doi: 10.1371/journal.pgen.0020149
Patel, J. A., Nair, S., Revai, K., Grady, J., Saeed, K., Matalon, R., et al. (2006). Association of proinflammatory cytokine gene polymorphisms with susceptibility to otitis media. Pediatrics 118, 2273–2279. doi: 10.1542/peds.2006-0764
Ram, G., and Chinen, J. (2011). Infections and immunodeficiency in Down syndrome. Clin. Exp. Immunol. 164, 9–16. doi: 10.1111/j.1365-2249.2011.04335.x
Ramet, M., Löfgren, J., Alho, O. P., and Hallman, M. (2001). Surfactant protein-A gene locus associated with recurrent otitis media. J. Pediatr. 138, 266–268. doi: 10.1067/mpd.2001.110133
Raymond, B. B., and Djordjevic, S. (2015). Exploitation of plasmin(ogen) by bacterial pathogens of veterinary significance. Vet. Microbiol. 178, 1–13. doi: 10.1016/j.vetmic.2015.04.008
Revai, K., Patel, J. A., Grady, J. J., Nair, S., Matalon, R., and Chonmaitree, T. (2009). Association between cytokine gene polymorphisms and risk for upper respiratory tract infection and acute otitis media. Clin. Infect. Dis. 49, 257–261. doi: 10.1086/599833
Roberts, J. E., Rosenfeld, R. M., and Zeisel, S. A. (2004). Otitis media and speech and language: a meta-analysis of prospective studies. Pediatrics 113(3 Pt 1), e238–e248. doi: 10.1542/peds.113.3.e238
Rye, M. S., Warrington, N. M., Scaman, E. S., Vijayasekaran, S., Coates, H. L., Anderson, D., et al. (2012). Genome-wide association study to identify the genetic determinants of otitis media susceptibility in childhood. PLoS One 7:e48215. doi: 10.1371/journal.pone.0048215
Rye, M. S., Wiertsema, S. P., Scaman, E. S., Oommen, J., Sun, W., Francis, R. W., et al. (2011). FBXO11, a regulator of the TGFbeta pathway, is associated with severe otitis media in Western Australian children. Genes Immun. 12, 352–359. doi: 10.1038/gene.2011.2
Rye, M. S., Wiertsema, S. P., Scaman, E. S., Thornton, R., Francis, R. W., Vijayasekaran, S., et al. (2013). Genetic and functional evidence for a role for SLC11A1 in susceptibility to otitis media in early childhood in a Western Australian population. Infect. Genet. Evol. 16, 411–418. doi: 10.1016/j.meegid.2013.03.023
Sale, M. M., Chen, W. M., Weeks, D. E., Mychaleckyj, J. C., Hou, X., Marion, M., et al. (2011). Evaluation of 15 functional candidate genes for association with chronic otitis media with effusion and/or recurrent otitis media (COME/ROM). PLoS One 6:e22297. doi: 10.1371/journal.pone.0022297
Santos-Cortez, R. L. P., Chiong, C. M., Frank, D. N., Ryan, A. F., Giese, A. P. J., Bootpetch Roberts, T., et al. (2018). FUT2 variants confer susceptibility to familial otitis media. Am. J. Hum. Genet. 103, 679–690. doi: 10.1016/j.ajhg.2018.09.010
Santos-Cortez, R. L., Chiong, C. M., Reyes-Quintos, M. R., Tantoco, M. L., Wang, X., Acharya, A., et al. (2015). Rare A2ML1 variants confer susceptibility to otitis media. Nat. Genet. 47, 917–920. doi: 10.1038/ng.3347
Santos-Cortez, R. L., Hutchinson, D. S., Ajami, N. J., Reyes-Quintos, M. R., Tantoco, M. L., Labra, P. J., et al. (2016). Middle ear microbiome differences in indigenous Filipinos with chronic otitis media due to a duplication in the A2ML1 gene. Infect. Dis. Poverty 5, 97.
Sculerati, N., Ledesma-Medina, J., Finegold, D. N., and Stool, S. E. (1990). Otitis media and hearing loss in Turner syndrome. Arch. Otolaryngol. Head Neck Surg. 116, 704–707.
Segade, F., Daly, K. A., Allred, D., Hicks, P. J., Cox, M., Brown, M., et al. (2006). Association of the FBXO11 gene with chronic otitis media with effusion and recurrent otitis media: the Minnesota COME/ROM family study. Arch. Otolaryngol. Head Neck Surg. 132, 729–733.
Shibahara, Y., and Sando, I. (1989). Congenital anomalies of the eustachian tube in Down syndrome. Histopathologic case report. Ann. Otol. Rhinol. Laryngol. 98(7 Pt 1), 543–547. doi: 10.1177/000348948909800709
Shimada, J., Moon, S. K., Lee, H. Y., Takeshita, T., Pan, H., Woo, J. I., et al. (2008). Lysozyme M deficiency leads to an increased susceptibility to Streptococcus pneumoniae-induced otitis media. BMC Infect. Dis. 8:134. doi: 10.1186/1471-2334-8-134
Shin, S. G., Koh, S. H., Woo, C. H., and Lim, J. H. (2014). PAI-1 inhibits development of chronic otitis media and tympanosclerosis in a mouse model of otitis media. Acta Otolaryngol. 134, 1231–1238. doi: 10.3109/00016489.2014.940554
Suzukawa, K., Tomlin, J., Pak, K., Chavez, E., Kurabi, A., Baird, A., et al. (2014). A mouse model of otitis media identifies HB-EGF as a mediator of inflammation-induced mucosal proliferation. PLoS One 9:e102739. doi: 10.1371/journal.pone.0102739
Swanson, J. A., and Hoecker, J. L. (1996). Concise review for primary-care physicians. Mayo. Clin Proc. 71, 179–183.
Tateossian, H., Morse, S., Parker, A., Mburu, P., Warr, N., Acevedo-Arozena, A., et al. (2013). Otitis media in the Tgif knockout mouse implicates TGFbeta signalling in chronic middle ear inflammatory disease. Hum. Mol. Genet. 22, 2553–2565. doi: 10.1093/hmg/ddt103
Tefs, K., Gueorguieva, M., Klammt, J., Allen, C. M., Aktas, D., Anlar, F. Y., et al. (2006). Molecular and clinical spectrum of type I plasminogen deficiency: a series of 50 patients. Blood 108, 3021–3026. doi: 10.1182/blood-2006-04-017350
Thomas, S. H., Meyers, A. D., Allen, G. C., and Thrasher, R. D. (2004). Otitis media with effusion. Pediatrics 113, 1412–1429.
Tian, C., Harris, B. S., and Johnson, K. R. (2016). Ectopic mineralization and conductive hearing loss in enpp1asj mutant mice, a new model for otitis media and tympanosclerosis. PLoS One 11:e0168159. doi: 10.1371/journal.pone.0168159
Tian, C., Hromatka, B. S., Kiefer, A. K., Eriksson, N., Noble, S. M., Tung, J. Y., et al. (2017). Genome-wide association and HLA region fine-mapping studies identify susceptibility loci for multiple common infections. Nat. Commun. 8:599. doi: 10.1038/s41467-017-00257-5
Tian, C., Yu, H., Yang, B., Han, F., Zheng, Y., Bartels, C. F., et al. (2012). Otitis media in a new mouse model for CHARGE syndrome with a deletion in the Chd7 gene. PLoS One 7:e34944. doi: 10.1371/journal.pone.0034944
Ubell, M. L., Khampang, A., and Kerschner, J. E. (2010). Mucin gene polymorphisms in otitis media patients. Laryngoscope 120, 132–138. doi: 10.1002/lary.20688
van Ingen, G., Li, J., Goedegebure, A., Pandey, R., Li, Y. R., March, M. E., et al. (2016). Genome-wide association study for acute otitis media in children identifies FNDC1 as disease contributing gene. Nat. Commun. 7:12792. doi: 10.1038/ncomms12792
Wiertsema, S. P., Hogenkamp, A., Wiertsema, S. P., Harthoorn, L. F., Loonstra, R., Hartog, A., et al. (2006a). Association of CD14 promoter polymorphism with otitis media and pneumococcal vaccine responses. Clin. Vaccine Immunol. 13, 892–897. doi: 10.1128/cvi.00100-06
Wiertsema, S. P., Veenhoven, R. H., Walraven, V., Uiterwaal, C. S., Schilder, A. G., Rijkers, G. T., et al. (2006b). Pneumococcal vaccine efficacy for mucosal pneumococcal infections depends on Fcgamma receptor IIa polymorphism. Vaccine 24, 792–797. doi: 10.1016/j.vaccine.2005.08.029
Wiesen, B. M., Hafrén, L., Einarsdottir, E., Kere, J., Mattila, P. S., Santos-Cortez, R. L., et al. (2019). ABO genotype and blood type are associated with otitis media. Genet. Test Mol. Biomarkers 23, 823–827. doi: 10.1089/gtmb.2019.0135
Woo, J. I., Pan, H., Oh, S., Lim, D. J., and Moon, S. K. (2010). Spiral ligament fibrocyte-derived MCP-1/CCL2 contributes to inner ear inflammation secondary to nontypeable H. influenzae-induced otitis media. BMC Infect. Dis. 10:314. doi: 10.1186/1471-2334-10-314
Yang, B., Tian, C., Zhang, Z. G., Han, F. C., Azem, R., Yu, H., et al. (2011). Sh3pxd2b mice are a model for craniofacial dysmorphology and otitis media. PLoS One 6:e22622. doi: 10.1371/journal.pone.0022622
Yao, W., Frie, M., Pan, J., Pak, K., Webster, N., Wasserman, S. I., et al. (2014). C-Jun N-terminal kinase (JNK) isoforms play differing roles in otitis media. BMC Immunol. 15:46. doi: 10.1186/s12865-014-0046-z
Keywords: otitis media (OM), omic, genetic, FUT, fucosyltransferase, A2ML1
Citation: Giese APJ, Ali S, Isaiah A, Aziz I, Riazuddin S and Ahmed ZM (2020) Genomics of Otitis Media (OM): Molecular Genetics Approaches to Characterize Disease Pathophysiology. Front. Genet. 11:313. doi: 10.3389/fgene.2020.00313
Received: 05 September 2019; Accepted: 16 March 2020;
Published: 23 April 2020.
Edited by:
Regie Santos-Cortez, University of Colorado, United StatesReviewed by:
Lena Hafren, University of Helsinki, FinlandGijs Van Ingen, Erasmus Medical Center, Netherlands
Copyright © 2020 Giese, Ali, Isaiah, Aziz, Riazuddin and Ahmed. This is an open-access article distributed under the terms of the Creative Commons Attribution License (CC BY). The use, distribution or reproduction in other forums is permitted, provided the original author(s) and the copyright owner(s) are credited and that the original publication in this journal is cited, in accordance with accepted academic practice. No use, distribution or reproduction is permitted which does not comply with these terms.
*Correspondence: Arnaud P. J. Giese, agiese@som.umaryland.edu; Zubair M. Ahmed, zmahmed@som.umaryland.edu
†These authors have contributed equally to this work