- 1Department of Medical Biology and Genetics, School of Medicine, University of Rijeka, Rijeka, Croatia
- 2Laboratory for Radiobiology and Molecular Genetics, Vinča Institute of Nuclear Sciences, University of Belgrade, Belgrade, Serbia
- 3Department of Gynaecology and Obstetrics, Clinical Hospital Centre Rijeka, University of Rijeka, Rijeka, Croatia
Background: Down syndrome (DS) is one of the most common chromosomal abnormalities associated with congenital heart defects (CHD), with approximately 40 to 60% of cases showing cardiac defects. This study assessed (i) the association between maternal LINE-1 methylation and the occurrence of CHDs in children with DS and (ii) the impact of endogenous maternal factors (MTHFR C677T polymorphism and maternal age) and exogenous maternal factors (cigarette smoking, alcohol intake, medication use, body mass index and dietary habits such as folate intake) on maternal LINE-1 methylation and on the occurrence of CHD in children with DS.
Patients and Methods: The study included 90 mothers of children with DS of maternal origin (49% DS-CHD+ mothers/51% DS-CHD− mothers). LINE-1 DNA methylation was analyzed in peripheral blood lymphocytes by quantification of LINE-1 methylation using the MethyLight method. MTHFR C677T polymorphism genotyping was performed using PCR-RFLP.
Results: LINE-1 methylation was not significantly different between DS-CHD+ and DS-CHD− mothers (P = 0.997). Combination of MTHFR C677T genotype/diet and BMI were significant independent predictors of LINE-1 DNA methylation in DS-CHD+ mothers (β −0.40, P = 0.01 and β −0.32, P = 0.03, respectively). In the analyzed multivariate model (model P = 0.028), these two factors explained around 72% of the variance in LINE-1 DNA methylation in mothers of children with DS and CHD. The group with the highest BMI (≥30 kg/m2) had significantly lower LINE-1 methylation than the group with normal BMI (Bonferroni post hoc P = 0.03) and the overweight group (Bonferroni post hoc P = 0.04). The lowest LINE-1 DNA methylation values were found in DS-CHD+ mothers with the CT+TT genotype and a low-folate diet; the values were significantly lower than the values in mothers with the CC genotype and a folate-rich diet (Bonferroni post hoc P = 0.04).
Conclusion: Association between maternal LINE-1 methylation and CHD in children with DS was not found. Study showed that the MTHFR genotype/diet combination and BMI were significantly associated with LINE-1 methylation in mothers of children with DS-CHD+. These results highlight the need for a multifactorial approach to assess the roles of endogenous and exogenous maternal factors in maternal LINE-1 DNA methylation and the consequent pathologies in children. More extensive studies in a larger sample may help elucidate these relationships.
Introduction
Congenital heart defects (CHDs) are the most common birth defects in humans, with a prevalence of 0.8% (Dolk et al., 2011; Van Der Linde et al., 2011). The etiology of most CHDs is unknown but is thought to involve multiple genetic, epigenetic, environmental, and lifestyle factors (Botto et al., 2003; Pierpont et al., 2007; Dolk et al., 2011; Van Der Linde et al., 2011; Sun et al., 2015). Only about 15 to 20% of CHDs can be attributed to known causes, with 5 to 10% of cases with CHDs showing chromosomal abnormalities (Botto and Correa, 2003; Dolk et al., 2011). Trisomy 21 (OMIM 190685), which results in Down syndrome (DS), shows the highest association with major heart abnormalities, which are present in approximately 40 to 60% of individuals with DS. Such CHDs typically involve septal defects such as atrial septal defects, ventricular septal defects, and complete atrioventricular canal defects (Freeman et al., 2008; Marder et al., 2015). In addition to the direct effects of the chromosomal abnormality, maternal genotype, diet, and lifestyle factors, along with environmental exposures, may be involved in the development of heart anomalies in individuals with DS. Foremost among these maternal risk factors are folic acid deficiency and genetic variations of folate pathway genes, such as the methylenetetrahydrofolate reductase gene (MTHFR) (Brandalize et al., 2009; Hobbs et al., 2010; Coppedè, 2015; Asim et al., 2017). Altered maternal DNA methylation is suggested to be an underlying mechanism in the development of birth defects, including CHDs (Blom et al., 2006; Chowdhury et al., 2011; Barua and Junaid, 2015; Serra-Juhé et al., 2015; Spearman, 2017). Some risk factors have been proposed to modulate DNA methylation, including aging, body mass index (BMI), cigarette smoking, alcohol intake, folate deficiency, MTHFR polymorphisms, and hyperhomocysteinemia patterns (Chowdhury et al., 2011; Flom et al., 2011; Terry et al., 2011; Zacho et al., 2011; Delgado-Cruzata et al., 2015; Marques-Rocha et al., 2016; Mendelson et al., 2017; Wahl et al., 2017; Liu et al., 2018).
During the last decade, quantitative measurement of the methylation status of long interspersed nucleotide element-1 (LINE-1) in white blood cells (WBCs) has been used as a surrogate measure of global DNA methylation and as a potential biomarker in a variety of diseases (Weisenberger et al., 2005; Chowdhury et al., 2011). Maternal LINE-1 hypomethylation has been linked to an increased risk of non-syndromic CHD, particularly septal defects (Chowdhury et al., 2011). We previously found significantly lower levels of LINE-1 methylation in the mothers of children with DS than in the mothers of healthy children (Božović et al., 2015). However, the relationship between LINE-1 methylation and DS-associated CHD has not yet been investigated.
Thus, the aim of the present study was to assess (i) the association between maternal LINE-1 methylation and the occurrence of CHD in children with DS and (ii) the association of endogenous maternal factors (MTHFR C677T polymorphism and maternal age), and exogenous maternal factors (cigarette smoking, alcohol intake, medication use, body mass index, and dietary habits, such as folate intake) with LINE-1 methylation in mothers of children with DS and CHD.
Materials and Methods
Study Participants
The study population consisted of 90 mothers of children with maternally derived full trisomy 21. All participants were the same ethnicity (Caucasian); 49% (44/90) had children with DS and CHD (DS-CHD+ mothers), and 51% (46/90) had children with DS without CHD (DS-CHD− mothers). There was a septal defect in 82% (36/44) of the children with DS-CHD+, a patent foramen ovale in 11% (5/44), patent ductus arteriosus in 5% (2/44), and persistent truncus arteriosus in 2% (1/44). Information about CHD was obtained from each child’s medical records. Maternal blood samples were collected in collaboration with DS associations in larger cities in Croatia (Rijeka, Zagreb, Pula, Zadar, Split, Karlovac, Čakovec, and Osijek). The Ethics Committee of the School of Medicine, University of Rijeka, reviewed and approved all study protocols (reference number: 2170-24-01-13-04). Written informed consent was provided by all participants prior to participation in the study in accordance with the Declaration of Helsinki. Before the sampling, the mothers were asked to complete a specially created questionnaire that asked about demographic data, weight and height, intake of folate-rich foods, cigarette smoking, alcohol intake, and medication use. The questionnaire was adapted from a food frequency questionnaire that has been validated for Croatian women (Colić et al., 2009).
Genetic Analysis
Genomic DNA was extracted from peripheral blood leukocytes using the QIAamp DNA Blood FlexiGene DNA Kit (Qiagen, Hilden, Germany). Quantification of genomic DNA was performed using a spectrophotometer (BioMateTM3, Thermo Electron Corporation, United States). The parental origin of trisomy 21 was determined as described previously (Vraneković et al., 2012). The MTHFR C677T polymorphism was evaluated using polymerase chain reaction–restriction fragment length polymorphism (PCR-RFLP) (Coppedè et al., 2006). Using the EpiTect Bisulfite Kit (Qiagen, Hilden, Germany), 500 ng of genomic DNA was subjected to sodium bisulfite modification and resuspended in 30 μl of TE buffer. Bisulfite-treated DNA was diluted 10×, and 8.18 μl was used for each real-time PCR reaction. All samples were stored at −20°C until analysis.
LINE-1 DNA methylation was analyzed by quantifying LINE-1 methylation using previously developed and validated MethyLight methodology, which precision and reproducibility have been well described (Eads et al., 2000; Weisenberger et al., 2005; Chowdhury et al., 2011; Božović et al., 2015). The PCR primers and probes for LINE-1 and for Alu sequences (ALU-C4) (Applied Biosystems, Forest City, CA, United States) were designed/validated (Weisenberger et al., 2005) and described previously (Weisenberger et al., 2005; Božović et al., 2015). The LINE-1 primers lack CpG sites and the specific TaqMan MGB probes (corresponding to the methylated and unmethylated LINE-1 sequence after bisulphite treatment) were used in real-time PCR (Applied Biosystems, Forest City, CA, United States). An Alu-based real-time PCR control reaction was performed in parallel with each LINE-1 real-time PCR reaction to normalize DNA input, as previously described (Weisenberger et al., 2005; Božović et al., 2015). PCR reactions were performed with a final reaction volume of 25 μl in sealed 96-well plates on the ABI 7500 Real-Time PCR System (Applied Biosystems). The PCR reactions and cycle conditions for the LINE-1 and ALU-C4 assays were described previously (Božović et al., 2015). Real-time PCR was performed in duplicate for each sample. The standard curve was established using EpiTect Methylated Control DNA and EpiTect Unmethylated Control DNA (Qiagen), as described previously (Božović et al., 2015). After PCR amplification, the data were read using SDS 1.4.0 software (Applied Biosystems). The percentage of methylated reference (PMR) and the percentage of unmethylated reference (PUR) were calculated, and the final percentage of LINE-1 DNA methylation was calculated according to the formula PMR/(PMR+PUR) × 100 (Eads et al., 2000; Weisenberger et al., 2005; Božović et al., 2015). After all runs we randomly repeated runs for 10% of all samples and we have complete reproducibility of the results.
The Influence of Endogenous and Exogenous Factors on Maternal LINE1 DNA Methylation
The analysis included maternal age and BMI as continuous variables and folate supplement intake, cigarette smoking, alcohol intake, and medication use as categorical variables. BMI was also studied as a categorical variable (“WHO BMI”), and four groups were defined according to WHO classification: underweight, normal, overweight, and obese. Because the impact of folic acid intake is modified by genes that code for enzymes involved in folate metabolism, the analysis also included the variable “MTHFR C677T genotype/diet,” which was used to indicate the combination of the MTHFR C677T polymorphism and dietary folate intake (rich or poor) (Friso et al., 2002; Castro et al., 2004). A folate-rich diet was defined as the consumption of at least three folate-rich foods (green leafy vegetables, legumes, veal liver, fruit, corn flakes, muesli) at least 2–3 times each week; lower intake was considered a low-folate diet. Mothers who consumed folic acid daily from 4 weeks before conception until 8 weeks after conception were considered to be periconceptional folic acid users. The questionnaire asked about cigarette smoking, alcohol intake, and medication use both (i) during the first 6 weeks of pregnancy and (ii) currently. Mothers who consumed one glass of wine or one glass of beer or one small strong alcoholic drink at least one time per week were defined as alcohol consumers. Those who smoked cigarettes daily or occasionally were classified as smokers.
Statistical Analysis
Differences in the frequencies between the investigated groups of participants were estimated by the chi-square test. Continuous variables with skewed distribution between groups were compared using the Mann–Whitney U-test. Normally distributed continuous variables were compared using analysis of variance (ANOVA) with the Bonferroni post hoc test and are reported as means and standard deviations (SDs). Logistic regression was used to determine the odds ratio and 95% confidence interval for the association between MTHFR C677T polymorphism and the occurrence of CHD in children with DS. Multivariate regression analysis was used to estimate the effects of the endogenous and exogenous factors included in the model on LINE1 DNA methylation (MTHFR C677T polymorphism, age, BMI, dietary intake of folate, intake of folate supplements, smoking, alcohol intake, and medication use), and the results are presented as regression coefficient (beta) values plus the standard error (SE). Statistical significance was considered for P < 0.05. Data analysis was performed using Statistica for Windows 10.0 (StatSoft, Tulsa, OK, United States).
Results
Table 1 shows the characteristics of the study participants. DS-CHD+ mothers were significantly younger than DS-CHD− mothers (P = 0.003). The frequency of mothers who smoked cigarettes during the first 6 weeks of pregnancy was significantly higher in DS-CHD+ mothers than in DS-CHD− mothers (P = 0.005).
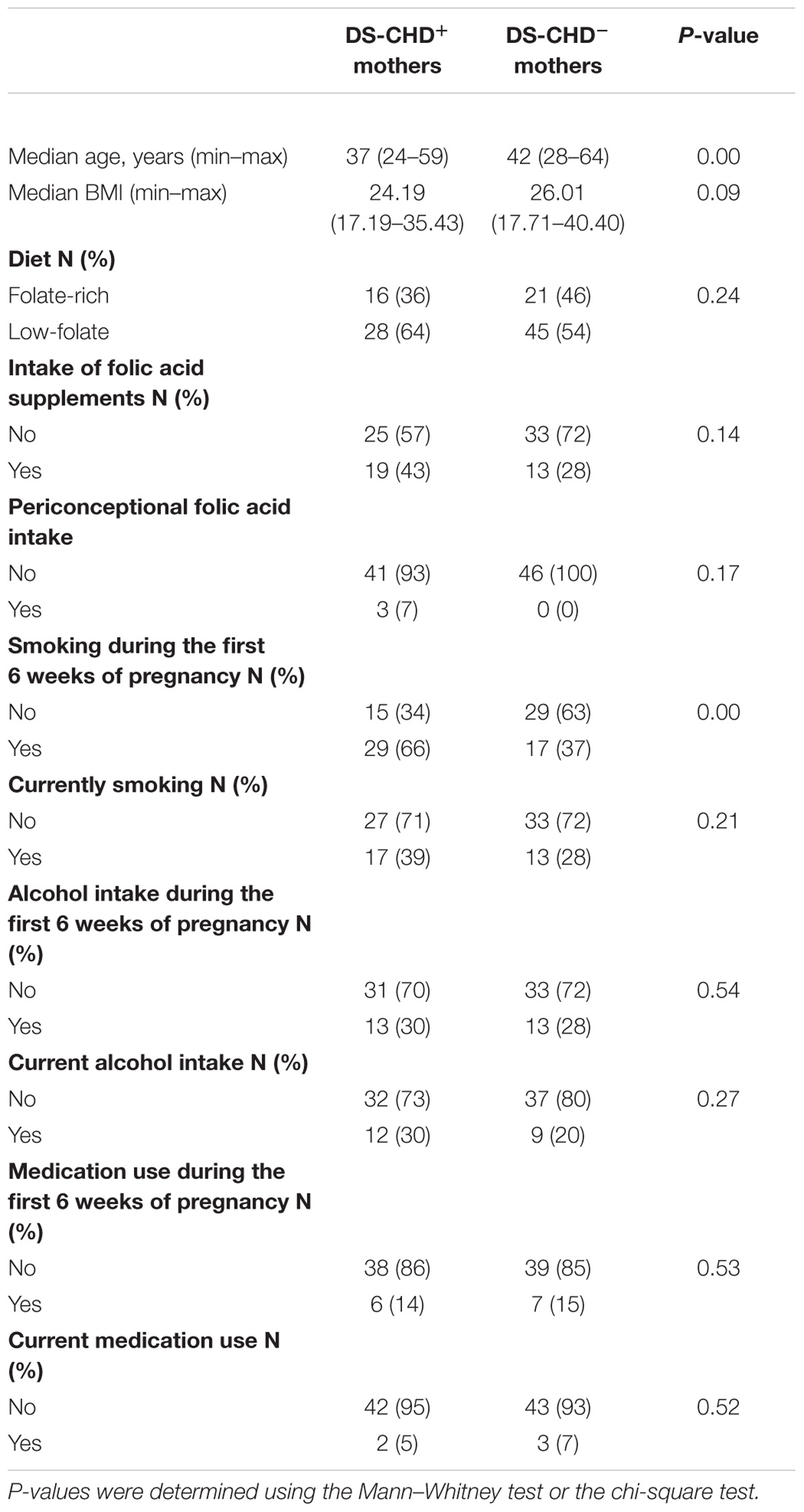
Table 1. Characteristics of mothers of children with Down syndrome (DS) with or without congenital heart defects (CHDs).
Supplementary Table S1 shows the allele and genotype frequencies of the MTHFR C677T polymorphism in DS-CHD+ and DS-CHD− mothers. There were no significant differences between the two groups.
Maternal LINE1 DNA Methylation
There was no significant difference in LINE-1 methylation between DS-CHD+ mothers (median: 95.30%; min–max: 88.68–99.90%) and DS-CHD− mothers (median: 95.51%; min–max: 79.13–99.73%) (P = 0.997). The difference in LINE-1 methylation between these groups remained non-significant after adjusting for two factors that were significantly different between these groups, namely maternal age and smoking during the first 6 weeks of pregnancy (adjusted OR = 1.03, 95% CI: 0.876–1.173, P = 0.853).
We have shown in Table 2 the values of LINE-1 methylation in DS-CHD+ mothers according to WHO BMI categories. Those in the highest WHO BMI category (≥30 kg/m2) had significantly lower LINE-1 methylation than those in either the normal WHO BMI category (Bonferroni post hoc P = 0.03) or the overweight WHO BMI category (Bonferroni post hoc P = 0.04). Table 3 lists the values for LINE-1 methylation in DS-CHD+ mothers according to MTHFR C677T genotype/diet combinations. The lowest LINE-1 DNA methylation values were observed in mothers with the CT+TT genotype and a low-folate diet. We performed multivariate regression analysis in order to evaluate the independent effect of the investigated parameters on LINE-1 DNA methylation in DS-CHD+ mothers. Among the investigated predictors (Table 4), only the MTHFR C677T genotype/diet combination and BMI were significantly independently associated with LINE-1 DNA methylation in DS-CHD+ mothers (β -0.40, P = 0.01 and β-0.32, P = 0.03, respectively).
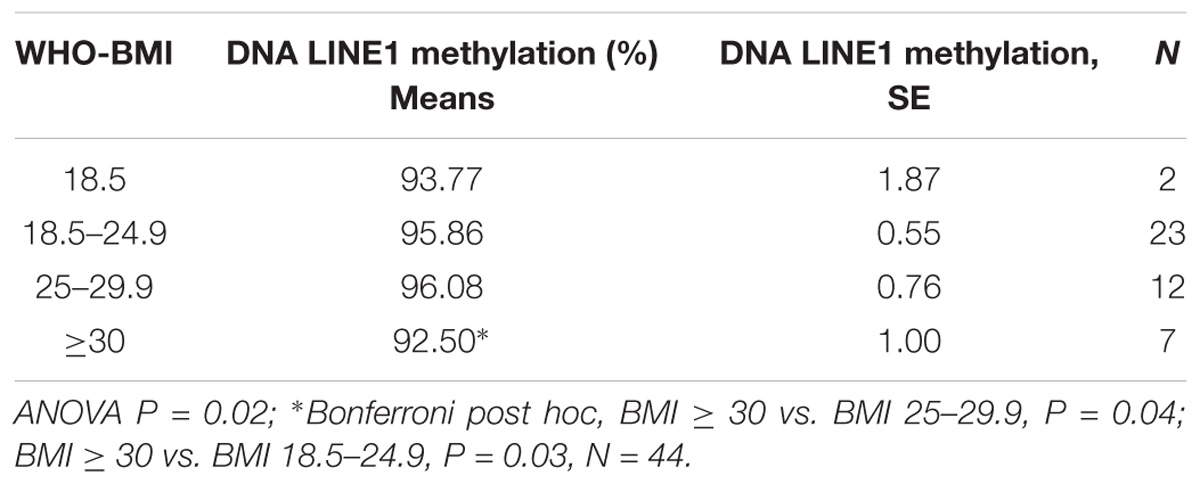
Table 2. LINE-1 methylation in mothers of children with DS with congenital heart defects (CHDs) according to World Health Organization (WHO) body mass index (BMI) category.
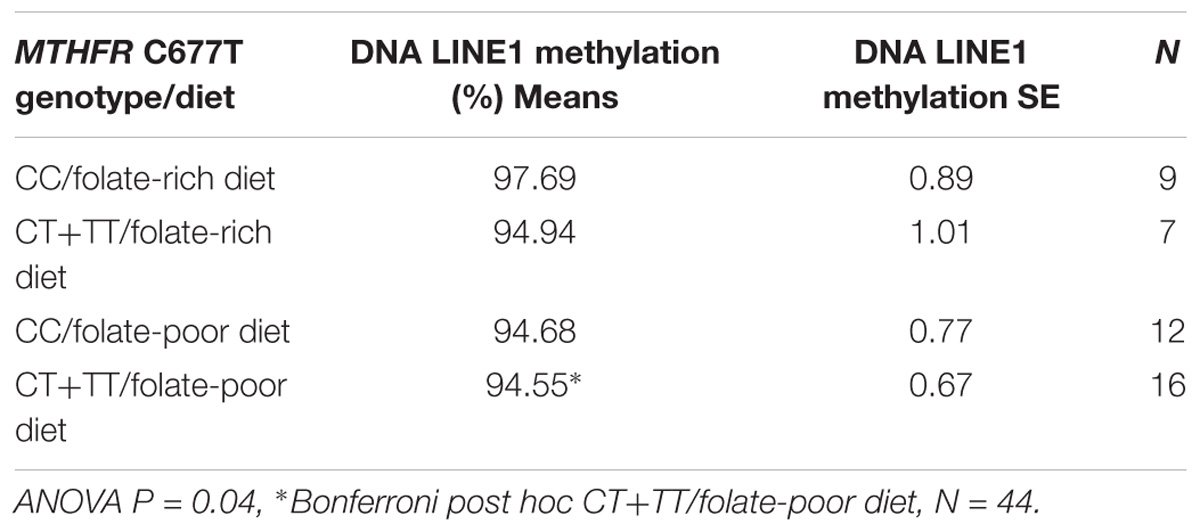
Table 3. LINE-1 methylation in mothers of children with DS with congenital heart defects (CHDs) according to the MTHFR C677T genotype/diet combination.
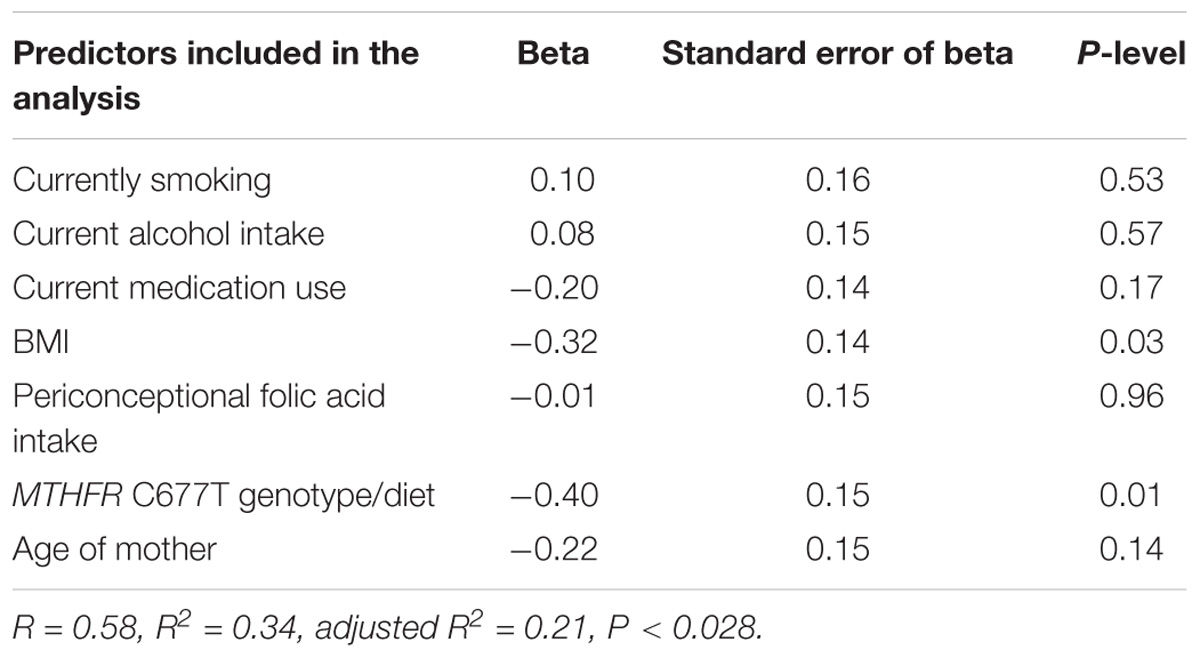
Table 4. Multivariate analysis of predictors that influence LINE-1 methylation in mothers of children with DS with congenital heart defects (CHDs).
In addition, we determined the LINE-1 methylation values in mothers of children with DS and septal defects. We found that the MTHFR C677T genotype/diet combination significantly influenced LINE-1 DNA methylation (Table 5). There were no statistically significant associations with LINE-1 methylation identified in DS-CHD− mothers (Supplementary Tables S2–S4).
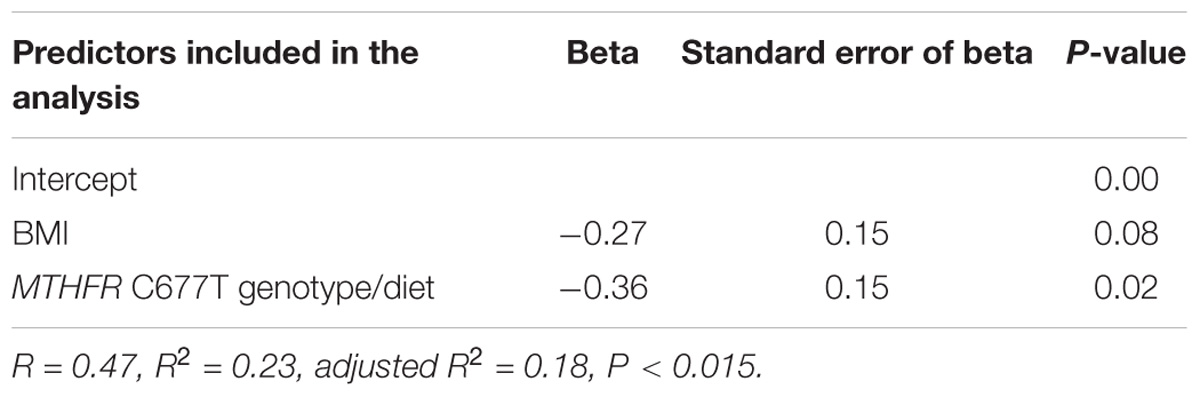
Table 5. Multivariate analysis of predictors that influence LINE-1 methylation in mothers of children with DS with septal defects.
Discussion
To the best of our knowledge, this is the first study to investigate the impact of endogenous maternal factors (MTHFR C677T polymorphism and maternal age) and exogenous maternal factors (cigarette smoking, alcohol intake, medication use, body mass index, dietary habits such as folate intake) on LINE-1 methylation in the mothers of children with DS regarding the presence of DS-associated CHDs, particularly regarding the presence of septal defect. The molecular mechanisms that underlie the epigenetic regulation of gene transcription are independent of DNA sequence, but they do depend on environmental stimuli, such as periconceptional maternal supplementation, diet, and the in utero environment (Barua and Junaid, 2015; Toriyama et al., 2017). The morphological processes that accompany embryonic heart development remain largely unknown, but multiple genetic, epigenetic, environmental, and lifestyle factors likely influence this process (Pierpont et al., 2007; Van Der Linde et al., 2011; Eriksson, 2016; Grunert et al., 2016; Toriyama et al., 2017).
We found that BMI and the MTHFR genotype/diet combination were significantly associated with variations in LINE-1 DNA methylation in DS-CHD+ mothers. Lower LINE1 DNA methylation values were significantly associated with the genotype containing the MTHFR 677T allele in combination with a low folate diet as well as with higher BMI, which is in accordance with previous studies (Friso et al., 2002; Castro et al., 2004; Piyathilake et al., 2011; Cai et al., 2014). Notably, it was reported previously that higher BMI is associated with lower LINE-1 methylation values in women of childbearing age (Piyathilake et al., 2011). The association between a maternal BMI that is higher than recommended by WHO and the occurrence of CHD in their offspring is well documented (Stothard et al., 2009; Piyathilake et al., 2011; Block et al., 2013; Cai et al., 2014). It is in line with our finding of significant influence of BMI only in the DS-CHD+ mothers. The mechanism by which BMI influences the development of CHD is not well understood, but it is thought that obesity is linked to lower concentrations of folate in the blood as well as with undiagnosed diabetes, both of which are maternal risk factors for CHD development (Hötzel, 1986; Becerra et al., 1990; Towner et al., 1995; Casanueva et al., 2000; Stothard et al., 2009; Hobbs et al., 2010). Likewise, increasing evidence suggests that folate metabolism and the resulting epigenetic modifications may contribute to the occurrence of CHD in individuals with DS (Brandalize et al., 2009; Elsayed et al., 2014; Coppedè, 2015).
DNA synthesis and methylation, processes that are folate-dependent, increase during pregnancy (Oommen et al., 2005). Folate pathway genes have been extensively investigated in regard to their association with CHD (Van Beynum et al., 2006, 2007; Wang et al., 2013; Elsayed et al., 2014). The MTHFR enzyme plays a key role in the regulation of folate availability in DNA synthesis and methylation. The C677T is one of the most important functional polymorphisms of the MTHFR gene (Frosst et al., 1995). Numerous studies have investigated the association between the MTHFR C677T polymorphism and the risk of CHDs, but the results have been inconsistent (Hobbs et al., 2005; Zhu et al., 2006; Brandalize et al., 2009; Božović et al., 2011). Meta-analyses showed that maternal MTHFR C677T polymorphism may contribute to the risk of CHDs (Wang et al., 2013; Xuan et al., 2014; Yang et al., 2018). Research on the relationship between the maternal MTHFR genotype and the development of CHD in children with DS has also yielded conflicting results (Brandalize et al., 2009; Hobbs et al., 2010; Božović et al., 2011; Elsayed et al., 2014; Coppedè, 2015). There has not yet been a meta-analysis, but almost all studies have shown that the MTHFR C677T genotype may be a maternal risk factor for CHD in children with DS, particularly if the mothers did not consume folic acid during the periconceptional period (Brandalize et al., 2009; Elsayed et al., 2014).
It is well established that increased folate intake can neutralize the impact of the MTHFR C677T polymorphism and restore normal enzyme activity (Guenther et al., 1999; Kluijtmans et al., 2003). Thus, a number of studies suggest that periconceptional maternal folic acid use has a protective effect on the occurrence of CHD in offspring, particularly for septal defects (Czeizel et al., 2001; Botto et al., 2003; Van Beynum et al., 2010). In our study, the periconceptional use of folate was not significantly associated with the level of LINE-1 methylation by itself. However, dietary folate intake in combination with the MTHFR C677T genotype showed a significant association with LINE-1 methylation levels. Moreover, our results revealed that the values of LINE-1 DNA methylation in DS-CHD+ mothers, as well as in the mothers of children with DS and septal defects, were clearly stratified according to the MTHFR C677T genotype/diet combination: the lowest values were observed in mothers with the CT+TT genotype and a low-folate diet, and the highest levels were observed in mothers with the CC genotype and a folate-rich diet. Chowdhury et al. also reported significant LINE-1 hypomethylation in women with children affected by septal defects (Chowdhury et al., 2011).
We found that the MTHFR C677T genotype/low folate diet combination was significantly associated with LINE-1 hypomethylation in mothers with children with DS who developed septal defects, and our previous study showed that significant LINE-1 hypomethylation (compared to controls) in the mothers of children with DS was itself significantly associated with the MTHFR C677T genotype/diet combination (Božović et al., 2015). Since the maternal environment potentially affects the fetus during pregnancy (Dimasuay et al., 2016), it is important that the analysis include as many factors as possible that could influence fetal development. In the present study, the MTHFR C677T genotype/diet combination and BMI showed a univariate association with LINE-1 DNA methylation in DS-CHD+ mothers. In addition, these parameters were independently associated with of LINE-1 DNA methylation in a multivariate analysis that included other maternal risk factors, such as smoking, periconceptional folic acid intake, medication and alcohol use, and age. In the multivariate model (model P < 0.028), these two factors explained around 72% of the variance in LINE-1 DNA methylation in DS-CHD+ mothers. Factors like cigarette smoking, alcohol intake, and age showed no associations with LINE-1 methylation status, which is in accordance with other studies (Terry et al., 2011; Zhang et al., 2011; Jones et al., 2015). Medication use was not significantly associated with LINE-1 DNA methylation, although only 6% of the participants were taking medications, and these belonged to several different therapeutic groups. Thus, we do not currently have enough data to discuss whether certain medications influence LINE-1 DNA methylation.
This study has several limitations. Notably, approximately 1/3 of fetuses with trisomy 21 are lost during early pregnancy (Savva et al., 2006). Thus, the true prenatal incidence of CHD in fetuses with trisomy 21 is unknown, and we can only speculate that those lost during early pregnancy may be more affected by CHDs than those who are born alive. Several studies have indicated that the increase of the prevalence of cardiac anomalies with decreasing fetal gestational age contributes to higher numbers of miscarriages (Gerlis, 1985; Tomek et al., 2009). Tomek et al. reported that the spectrum of CHDs that are diagnosed prenatally differs significantly from the spectrum of CHDs diagnosed postnatally in that there is a markedly higher proportion of additional abnormalities associated with those who are diagnosed prenatally (Tomek et al., 2009). Thus, we would have a clearer picture of the impact of maternal LINE-1 DNA methylation on DS-associated CHDs if we could analyze the maternal LINE-1 DNA methylation values during pregnancy (and thus during organogenesis) for all conceived fetuses with trisomy 21, since during this time, altered maternal DNA methylation would exert the greatest effects. It is possible that increases in cellular proliferation and carbon metabolism during pregnancy, as well as an increased demand for methyl groups during embryonic development, could have an even greater influence on maternal LINE-1 DNA methylation and contribute to the development of CHD in offspring. Given the rare prevalence of DS-associated CHD, the resources and sample size required to conduct such a study, and the difficulty in enrolling women before conception and monitoring them until the completion of their pregnancy, it would be very challenging to conduct this type of study. Also, analysis of other class of repetitive elements, such as ALU, might give additional information on the global DNA methylation in mothers of children with DS with regard to presence of CHD. It was suggested that Alu might be more informative in background where the LINE-1 hypomethylation might be influenced by genomic instability, carcinogenesis or aging (Erichsen et al., 2018).
In conclusion, we have not found the association of maternal LINE-1 methylation with CHD in children with DS. Yet, we have found significant association of the MTHFR genotype/diet combination and BMI with LINE-1 methylation in mothers of children with DS-CHD and in mothers of children with DS and septal defects. These results strongly support the need for a multifactorial approach in analysis of endogenous and exogenous maternal factors that could affect or are associated with maternal LINE-1 DNA methylation and the consequent pathologies in children. More extensive studies in a larger sample may help to validate these results while functional studies are inevitable to elucidate the causality and mechanisms of action of proposed factors.
Datasets Are Available on Request
The raw data supporting the conclusions of this manuscript will be made available by the authors, without undue reservation, to any qualified researcher.
Author Contributions
IBB, AS, MŽ, and JV processed the experimental data, performed the analysis, and drafted the manuscript. BB-M, AS, and MŽ were involved in planning and supervised the work. VM-S aided in collecting the patients. All authors discussed the results and commented on the manuscript.
Funding
This study was supported by grant (No. 13.06.1.2.38) from the University of Rijeka, Rijeka, Croatia.
Conflict of Interest Statement
The authors declare that the research was conducted in the absence of any commercial or financial relationships that could be construed as a potential conflict of interest.
Acknowledgments
The authors would like to thank all participants in this study.
Supplementary Material
The Supplementary Material for this article can be found online at: https://www.frontiersin.org/articles/10.3389/fgene.2019.00041/full#supplementary-material
Abbreviations
BMI, body mass index; CHD, congenital heart defects; DS, Down syndrome; LINE-1, long interspersed nucleotide element-1; MTHFR, methylenetetrahydrofolate reductase; PCR, polymerase chain reaction; PMR, percent of methylated reference; PUR, percent of unmethylated reference; WBC, white blood cells.
References
Asim, A, Agarwal, S., Panigrahi, I., Saiyed, N., and Bakshi, S. (2017). MTHFR promoter hypermethylation may lead to congenital heart defects in down syndrome. Intract. Rare Dis. Res. 6, 295–298. doi: 10.5582/irdr.2017.01068
Barua, S., and Junaid, M. A. (2015). Lifestyle, pregnancy and epigenetic effects. Epigenomics 7, 85–102. doi: 10.2217/epi.14.71
Becerra, J. E., Khoury, M. J., Cordero, J. F., and Erickson, J. D. (1990). Diabetes mellitus during pregnancy and the risks for specific birth defects: a population-based case-control study. Pediatrics 85, 1–9.
Block, S. R., Watkins, S. M., Salemi, J. L., Rutkowski, R., Tanner, J. P., Correia, J. A., et al. (2013). Maternal pre-pregnancy body mass index and risk of selected birth defects: evidence of a dose-response relationship. Paediatr. Perinat. Epidemiol. 27, 521–531. doi: 10.1111/ppe.12084
Blom, H. J., Shaw, G. M., Den Heijer, M., and Finnell, R. H. (2006). Neural tube defects and folate: case far from closed. Nat. Rev. Neurosci. 7, 724–731. doi: 10.1038/nrn1986
Botto, L. D., and Correa, A. (2003). Decreasing the burden of congenital heart anomalies: an epidemiologic evaluation of risk factors and survival. Prog. Pediatr. Cardiol. 18, 111–121. doi: 10.1016/S1058-9813(03)00084-5
Botto, L. D., Mulinare, J., and Erickson, J. D. (2003). Do multivitamin or folic acid supplements reduce the risk for congenital heart defects? Evidence and Gaps. Am. J. Med. Genet. A 121A, 95–101. doi: 10.1002/ajmg.a.20132
Božović, I. B., Stankovic, A., Zivković, M., Vraneković, J., Kapović, M., and Brajenović-Milić, B. (2015). Altered LINE-1 methylation in mothers of children with down syndrome. PLoS One 10:e0127423. doi: 10.1371/journal.pone.0127423
Božović, I. B., Vraneković, J., Čizmarević, N. S., Mahulja-Stamenković, V., Prpić, I., and Brajenović-Milić, B. (2011). MTHFR C677T and A1298C polymorphisms as a risk factor for congenital heart defects in down syndrome. Pediatr. Int. 53, 546–550. doi: 10.1111/j.1442-200X.2010.03310.x
Brandalize, A. P. C., Bandinelli, E., Dos Santos, P. A., Roisenberg, I., and Schüler-Faccini, L. (2009). Evaluation of C677T and A1298C polymorphisms of the MTHFR gene as maternal risk factors for down syndrome and congenital heart defects. Am. J. Med. Genet. Part A 149, 2080–2087. doi: 10.1002/ajmg.a.32989
Cai, G., Sun, X., Zhang, L., and Hong, Q. (2014). Association between maternal body mass index and congenital heart defects in offspring: a systematic review. Am. J. Obstet. Gynecol. 211, 91–117. doi: 10.1016/j.ajog.2014.03.028
Casanueva, E., Drijanski, A., Fernandez-Gaxiola, A. C., Meza, C., and Pfeffer, F. (2000). Folate deficiency is associated with obesity and anemia in mexican urban women. Nutr. Res. 20, 1389–1394. doi: 10.1016/S0271-5317(00)80020-2
Castro, R., Rivera, I., and Ravasco, P. (2004). 5, 10- methylenetetrahydrofolate reductase (MTHFR) 677C–>T and 1298A–C mutations are associated with DNA hypomethylation. J. Med. Genet. 41, 454–458. doi: 10.1136/jmg.2003.017244
Chowdhury, S., Cleves, M. A., MacLeod, S. L., James, S. L., Zhao, W., and Hobbs, C. H. (2011). Maternal DNA hypomethylation and congenital heart defects. Birth Defects Res Part A – Clin. Mol. Teratol. 91, 69–76. doi: 10.1002/bdra.20761
Colić B. I., Satalić, Z., Pedisić, Ž., Zizić, V., and Linarić, I. (2009). Validation of the folate food frequency questionnaire in vegetarians. Int. J. Food Sci. Nutr. 60(Suppl. 5), 88–95. doi: 10.1080/09637480802459384
Coppedè, F. (2015). The genetics of folate metabolism and maternal risk of birth of a child with down syndrome and associated congenital heart defects. Front. Genet. 6:223. doi: 10.3389/fgene.2015.00223
Coppedè, F., Marini, G., Bargagna, S., Stuppia, L., Minichilli, F., and Fontana, I. (2006). Folate gene polymorphisms and the risk of down syndrome pregnancies in young italian women. Am. J. Med. Genet. 140, 1083–1091. doi: 10.1002/ajmg.a.31217
Czeizel, A. E., Rockenbauer, M., Siffel, C., and Varga, E. (2001). Description and mission evaluation of the hungarian case-control surveillance of congenital abnormalities, 1980-1996. Teratology 63, 176–185. doi: 10.1002/tera.1032
Delgado-Cruzata, L., Zhang, W., McDonald, J. A., Tsai, W. Y., Valdovinos, C., and Falci, L., et al. (2015). Dietary modifications, weight loss, and changes in metabolic markers affect global DNA methylation in hispanic, african american, and afro-caribbean breast cancer survivors. J. Nutr. 145, 783–790. doi: 10.3945/jn.114.202853
Dimasuay, K. G., Boeuf, P., Powell, T. L., and Jansson, T. (2016). Placental responses to changes in the maternal environment determine fetal growth. Front. Physiol. 7:12. doi: 10.3389/fphys.2016.00012
Dolk, H., Loane, M., and Garne, E. (2011). Congenital heart defects in europe: prevalence and perinatal mortality, 2000 to 2005. Circulation 123, 841–849. doi: 10.1161/CIRCULATIONAHA.110.958405
Eads, C. A., Danenberg, K. D., Kawakami, K., Saltz, L. B., Blake, C., and Shibata, D., et al. (2000). Methy light: a high-throughput assay to measure DNA methylation. Nucleic Acids Res. doi: 10.1093/nar/28.8.e32
Elsayed, G. M., Elsayed, S. M., and Ezz-Elarab, S. S. (2014). Maternal MTHFR C677T genotype and septal defects in offspring with down syndrome: a pilot study. Egypt. J. Med. Hum Genet. 15, 39–44. doi: 10.1016/j.ejmhg.2013.09.003
Erichsen, L., Beermann, A., Arauzo-Bravo, M. J., Hassan, M., Dkhil, M. A., Al-Quraishy, S., et al. (2018). Genome-wide hypomethylation of LINE-1 and Alu retroelements in cell-free DNA of blood is an epigenetic biomarker of human aging. Saudi J. Biol. Sci. 25, 1220–1226. doi: 10.1016/j.sjbs.2018.02.005
Eriksson, J. G. (2016). Developmental origins of health and disease – From a small body size at birth to epigenetics. Ann. Med. 48, 456–467. doi: 10.1080/07853890.2016.1193786
Flom, J. D., Ferris, J. S., Liao, Y., Tehranifar, P., Richards, C. B., Cho, Y. H., et al. (2011). Prenatal smoke exposure and genomic DNA methylation in a multiethnic birth cohort. Cancer Epidemiol. Biomark. Prev. 20, 2518–2523. doi: 10.1158/1055-9965.EPI-11-0553
Freeman, S. B., Bean, L. H., Allen, E. G., Tinker, S. W., Locke, A. E., Druschel, C., et al. (2008). Ethnicity, sex, and the incidence of congenital heart defects: a report from the national down syndrome project. Genet. Med. 10, 173–180. doi: 10.1097/GIM.0b013e3181634867
Friso, S., Choi, S.-W., Girelli, D., Mason, J. B., Dolnikowski, G. G., Bagley, P. J., et al. (2002). A Common mutation in the 5,10-methylenetetrahydrofolate reductase gene affects genomic DNA methylation through an interaction with folate status. Proc. Nat. Acad. Sci. U.S.A. 99, 5606–5611. doi: 10.1073/pnas.062066299
Frosst, P., Blom, H. J., Milos, R., Goyette, P., Sheppard, C. A., Matthews, R. G., et al. (1995). A Candidate genetic risk factor for vascular disease: a common mutation in methylenetetrahydrofolate reductase. Nat. Genet. 10, 111–113. doi: 10.1038/ng0595-111
Gerlis, L. M. (1985). Cardiac malformations in spontaneous abortions. Int. J. Cardiol. 7, 29–43. doi: 10.1016/0167-5273(85)90170-6
Grunert, M, Dorn, C., Cui, H., Dunkel, I., Schulz, K., Schoenhals, S., et al. (2016). Comparative DNA methylation and gene expression analysis identifies novel genes for structural congenital heart diseases. Cardiovasc. Res. 112, 464–477. doi: 10.1093/cvr/cvw195
Guenther, B. D., Sheppard, C. A., and Tran, P. (1999). The structure and properties of methylenetetrahydrofolate reductase from Escherichia coli suggest how folate ameliorates human hyperhomocysteinemia. Nat. Struct. Biol. 6, 359–365. doi: 10.1038/7594
Hobbs, C. A., Cleves, M. A., Karim, M. A., Zhao, W., and MacLeod, S. L. (2010). Maternal folate-related gene environment interactions and congenital heart defects. Obstetr. Gynecol. 116, 316–322. doi: 10.1097/AOG.0b013e3181e80979
Hobbs, C. A., Cleves, M. A., Melnyk, S., Zhao, W., and James, S. J. (2005). Congenital heart defects and abnormal maternal biomarkers of methionine and homocysteine metabolism. Am. J. Clin. Nutr. 81, 147–153. doi: 10.1093/ajcn/81.1.147
Hötzel, D. (1986). Suboptimal nutritional status in obesity (selected nutrients). Bibl. Nutr. Dieta 37, 36–41.
Jones, M. J., Goodman, S. J., and Kobor, M. S. (2015). DNA methylation and healthy human aging. Aging Cell 14, 924–932. doi: 10.1111/acel.12349
Kluijtmans, L. A. J., Young, I. S., Boreham, C. A., Murray, L., McMaster, D., and McNulty, H. (2003). Genetic and nutritional factors contributing to hyperhomocysteinemia in young adults. Blood 101, 2483–2488. doi: 10.1182/blood.V101.7.2483
Liu, C., Marioni, R. E., Hedman, A. K., Pfeiffer, L., Tsai, P. C., and Reynolds, L. M., et al. (2018). A DNA methylation biomarker of alcohol consumption. Mol. Psychiatry 23, 422–433. doi: 10.1038/mp.2016.192
Marder, L., Tulloh, R., and Pascall, E. (2015). Cardiac problems in down syndrome. Paediatr. Child Health (U.K.) 25, 23–29. doi: 10.1016/j.paed.2014.07.011
Marques-Rocha, J., Milagro, F., Mansego, M. L., Mourão, D. M., Martínez, J. M., and Bressan, J. (2016). LINE-1 methylation is positively associated with healthier lifestyle but inversely related to body fat mass in healthy young individuals. Epigenetics 49–60. doi: 10.1080/15592294.2015.1135286
Mendelson, M. M., Marioni, R. E., Joehanes, R., Liu, C., Hedman, A. K., Aslibekyan, S., et al. (2017). Association of body mass index with DNA methylation and gene expression in blood cells and relations to cardiometabolic disease: a mendelian randomization approach. PLoS Med. 14:e1002215. doi: 10.1371/journal.pmed.1002215
Oommen, A. M., Griffin, J. B., Sarath, G., and Zempleni, J. (2005). Roles for nutrients in epigenetic events. J. Nutr. Biochem. 16, 74–77. doi: 10.1016/j.jnutbio.2004.08.004
Pierpont, M. E., Basson, C. T., Benson, D. W., Gelb, B. D., Giglia, T. M., Goldmuntz, E., et al. (2007). Genetic basis for congenital heart defects: current knowledge a scientific statement from the american heart association congenital cardiac defects committee, council on cardiovascular. Circulation 115, 3015–3038. doi: 10.1161/CIRCULATIONAHA.106.183056
Piyathilake, C., Badiga, S., Johanning, G., Alvarez, G., and Partridge, E. (2011). Predictors and health consequences of epigenetic changes associated with excess body weight in women of child-bearing age. Cancer Epidemiol. Biomarkers. Prev. 20:719 doi: 10.1158/1055-9965.EPI-11-0094
Savva, G. M., Morris, J. K., Mutton, D. E., and Alberman, E. (2006). Maternal age-specific fetal loss rates in down syndrome pregnancies. Prenat. Diagn. 26, 499–504. doi: 10.1002/pd.1443
Serra-Juhé, C., Cuscó, I., Homs, A., Flores, R., Torán, N., and Pérez-Jurado, L. A. (2015). DNA methylation abnormalities in congenital heart disease. Epigenetics 10, 167–177. doi: 10.1080/15592294.2014.998536
Spearman, A. D. (2017). Epigenetics for the pediatric cardiologist. Congenit. Heart Dis. 12, 828–833. doi: 10.1111/chd.12543
Stothard, K. J., Tennant, P. W. G., Bell, R., and Rankin, J. (2009). Maternal overweight and obesity and the risk of congenital anomalies: a systematic review and meta-analysis. JAMA 301, 636–650. doi: 10.1001/jama.2009.113
Sun, R. R., Liu, M., Lu, L., and Zheng, Y., and Zhang, P. (2015). Congenital heart disease: causes, diagnosis, symptoms, and treatments. Cell Biochem. Biophys. doi: 10.1007/s12013-015-0551-6
Terry, M. B., Delgado-Cruzata, L., Vin-Raviv, N., Wu, H. C., and Santella, R. M. (2011). DNA methylation in white blood cells: association with risk factors in epidemiologic studies. Epigenetics 6, 828–837. doi: 10.4161/epi.6.7.16500
Tomek, V., Marek, J., Jičínská, H., and Škovránek, J. (2009). Fetal cardiology in the czech republic: current management of prenatally diagnosed congenital heart diseases and arrhythmias. Phys Res. 58 (Suppl. 2), S159–S166. doi: 10.1016/j.arcped.2004.03.115
Toriyama, M., Toriyama, M., Wallingford, J. B., and Finnell, R. H. (2017). Folate-dependent methylation of septins governs ciliogenesis during neural tube closure. FASEB J. 31, 3622–3635. doi: 10.1096/fj.201700092R
Towner, D., Kjos, S. L., and Leung, B. (1995). Congenital malformation in pregnancies complicated by NIDDM: increased risk from poor maternal metabolic control but not from exposure to sulfonylurea drugs. Diabetes Care 18, 1446–1451. doi: 10.2337/diacare.18.11.1446
Van Beynum, I. M., den Heijer M., Blom H. J., and Kapusta, L. (2007). The MTHFR 677C->T polymorphism and the risk of congenital heart defects: a literature review and meta-analysis. QJM 100, 743–753. doi: 10.1093/qjmed/hcm094
Van Beynum, I. M., Kapusta, L., Bakker, M. K., Den Heijer, M., Blom, H. J., and De Walle, H. E. K. (2010). Protective effect of periconceptional folic acid supplements on the risk of congenital heart defects: a registry-based case-control study in the northern Netherlands. Eur. Heart. J. 31, 464–471. doi: 10.1093/eurheartj/ehp479
Van Beynum, I. M., Kapusta, L., and den Heijer, M. (2006). Maternal MTHFR C > T is a risk factor for congenital heart defects: effect modification by periconceptional folate supplementation. Eur. Heart. J. 27, 981–987. doi: 10.1093/eurheartj/ehi815
Van Der Linde, D., Konings, E. E. M., Slager, M. A., Witsenburg, M., Helbing, W. A., Takkenberg, J. J. M., et al. (2011). Birth prevalence of congenital heart disease worldwide: a systematic review and meta-analysis. J. Am. Coll. Cardiol. 58, 2241–2247. doi: 10.1016/j.jacc.2011.08.025
Vraneković, J., Božović, I. B., Grubić, Z., Wagner, J., Pavlinić, D., Dahoun, S., et al. (2012). Down syndrome: parental origin, recombination, and maternal age. Genet. Test. Mol. Biomark. 16, 70–73. doi: 10.1089/gtmb.2011.0066
Wahl, S. A., Drong, B., Lehne, M. L., Scott, W. R., Kunze, S., and Tsai, P. C., et al. (2017). Epigenome-wide association study of body mass index, and the adverse outcomes of adiposity. Nature 541, 81–86. doi: 10.1038/nature20784
Wang, W., Wang, Y., Gong, F., Zhu, W., and Fu, S. (2013). MTHFR C677T polymorphism and risk of congenital heart defects: evidence from 29 case-control and TDT studies. PLoS One 8:e0058041. doi: 10.1371/journal.pone.0058041
Weisenberger, D. J., Campan, M., Kim, M., Woods, C., Fiala, E., et al. (2005). Analysis of repetitive element DNA methylation by methylight. Nucleic. Acids. Res. 33, 6823–6836. doi: 10.1093/nar/gki987
Xuan, C., Li, H., Zhao, J. X., Wang, H. W., Wang, Y., Ning, C. N., et al. (2014). Association between MTHFR polymorphisms and congenital heart disease: a meta-analysis based on 9,329 cases and 15,076 controls. Sci. Rep. 4:7311. doi: 10.1038/srep07311
Yang, H. L., Yang, L. Y., Yu, C. H., and Shiao, S. P. K. (2018). Meta-prediction of MTHFR gene polymorphism and air pollution on the risks of congenital heart defects worldwide: a transgenerational analysis. Int. J. Environ. Res. Public Health 15:E1660. doi: 10.3390/ijerph15081660
Zacho, J., Yazdanyar, S., Bojesen S. E., Tybjærg-Hansen, A., and Nordestgaard, B. G. (2011). Hyperhomocysteinemia, methylenetetrahydrofolate reductase c.677C>T polymorphism and risk of cancer: cross-sectional and prospective studies and meta-analyses of 75,000 cases and 93,000 controls. Int. J. Cancer. 128, 644–652. doi: 10.1002/ijc.25375
Zhang, F. F., Cardarelli, R., and Carroll, J. (2011). Significant differences in LINE1 genomic DNA methylation by gender and race/ethnicity in peripheral blood. Epigenetics 6, 623–629. doi: 10.4161/epi.6.5.15335
Keywords: DNA methylation, LINE-1, congenital heart defects, Down syndrome, maternal risk
Citation: Babić Božović I, Stanković A, Živković M, Vraneković J, Mahulja-Stamenković V and Brajenović-Milić B (2019) Maternal LINE-1 DNA Methylation and Congenital Heart Defects in Down Syndrome. Front. Genet. 10:41. doi: 10.3389/fgene.2019.00041
Received: 20 July 2018; Accepted: 21 January 2019;
Published: 06 February 2019.
Edited by:
Yulan Liang, University of Maryland, Baltimore, United StatesReviewed by:
Minmin Liu, Van Andel Institute, United StatesLouise Esther Docherty, The University of Edinburgh, United Kingdom
Copyright © 2019 Babić Božović, Stanković, Živković, Vraneković, Mahulja-Stamenković and Brajenović-Milić. This is an open-access article distributed under the terms of the Creative Commons Attribution License (CC BY). The use, distribution or reproduction in other forums is permitted, provided the original author(s) and the copyright owner(s) are credited and that the original publication in this journal is cited, in accordance with accepted academic practice. No use, distribution or reproduction is permitted which does not comply with these terms.
*Correspondence: Bojana Brajenović-Milić, bojanabm@uniri.hr