- 1Department of Obstetrics and Gynecology, Hokkaido University School of Medicine, Hokkaido University, Sapporo, Japan
- 2State Key Laboratory of Oncology in South China, Department of Gynecology, Sun Yat-sen University Cancer Center, Guangzhou, China
- 3Department of Pathology and Laboratory Medicine, University of Tennessee Health Science Center, Memphis, TN, United States
- 4Center for Cancer Research, University of Tennessee Health Science Center, Memphis, TN, United States
- 5Division of Gynecologic Oncology, National Hospital Organization, Hokkaido Cancer Center (NHO), Sapporo, Japan
The nuclear paraspeckle assembly transcript 1 (NEAT1, a long non-coding RNA) is frequently overexpressed in human tumors, and higher NEAT1 expression is correlated with worse survival in cancer patients. NEAT1 drives tumor initiation and progression by modulating the expression of genes involved in the regulation of tumor cell growth, migration, invasion, metastasis, epithelial-to-mesenchymal transition, stem cell-like phenotype, chemoresistance and radioresistance, indicating the potential for NEAT1 to be a novel diagnostic biomarker and therapeutic target. Mechanistically, NEAT1 functions as a scaffold RNA molecule by interacting with EZH2 (a subunit of the polycomb repressive complex) to influence the expression of downstream effectors of EZH2, it also acts as a microRNA (miRNA) sponge to suppress the interactions between miRNAs and target mRNAs, and affects the expression of miR-129 by promoting the DNA methylation of the miR-129 promoter region. Knockdown of NEAT1 via small interfering RNA or short hairpin RNA inhibits the malignant behavior of tumor cells. In this review, we highlight the latest insights into the expression pattern, biological roles and mechanisms underlying the function and regulation of NEAT1 in tumors, and especially focus on its clinical implication as a new diagnostic biomarker and an attractive therapeutic target for cancers.
Introduction
Although most of the genome (around 80%) is actively transcribed into RNA, < 2% is actually translated into functional proteins (ENCODE Project Consortium, 2012), suggesting that the dominant fraction of the transcriptome consists of non-coding RNAs (ncRNAs). NcRNAs can be classified into small ncRNAs (< 200 nt) or long ncRNAs (>200 nt). One class of small ncRNAs, microRNAs (miRNAs), bind to the 3′-untranslated region of the target gene mRNA, thereby repressing target mRNA translation or inducing mRNA degradation. In contrast to well-studied miRNAs, the function and mechanism of long ncRNAs (lncRNAs) in human cancers are poorly characterized.
Categories of lncRNAs
According to genomic location, lncRNAs can be further subdivided into exon or intron sense-overlapping lncRNAs, intergenic lncRNAs, antisense lncRNAs, bidirectional lncRNAs and enhancer lncRNAs (Esteller, 2011; Thum and Condorelli, 2015; Figure 1).
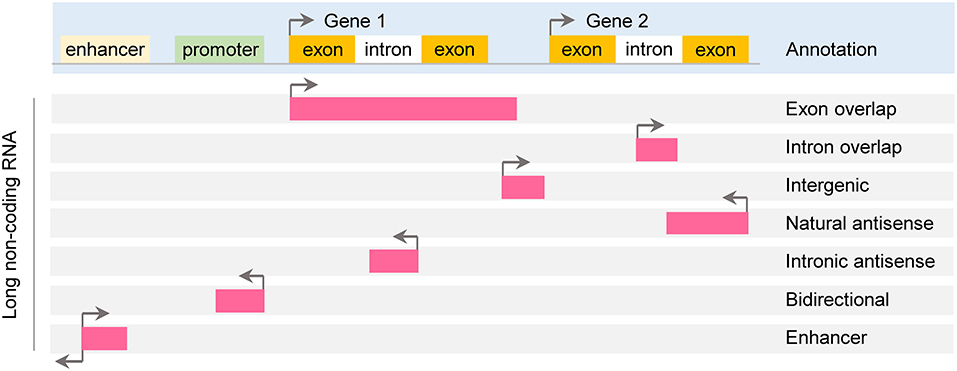
Figure 1. Classification of long noncoding RNA. Long non-coding RNAs (lncRNAs) are roughly classified based on their position relative to protein-coding genes: exon or intron sense-overlapping lncRNAs, intergenic lncRNAs, antisense lncRNAs, bidirectional lncRNAs and enhancer lncRNAs.
Functions and Mechanisms of lncRNAs
The function of lncRNA depends on their subcellular location (Chen L. L., 2016). Up to 30% of lncRNAs are found exclusively in the nucleus, ~15% are found exclusively in the cytoplasm, while the remaining lncRNAs are present in both nucleus and cytoplasm (Kapranov et al., 2007). In the nucleus, lncRNAs are important transcriptional and epigenetic modulators of nuclear functions, whereas cytoplasmic lncRNAs target mRNA transcripts and modulate mRNA stability and translation (Mercer and Mattick, 2013).
LncRNAs could form complex secondary and tertiary structures, which are proposed to provide multiple binding sites for other molecules (Liu et al., 2017b). LncRNAs regulates the expression of target genes by interacting with other molecules such as protein, DNA and RNA (Figure 2). Some lncRNAs can guide site-specific recruitment of transcriptional activators or suppressors to genomic sites and regulate gene expression (Mercer et al., 2009; Tripathi et al., 2010; Kotake et al., 2011; Wang and Chang, 2011; Li Y. et al., 2016; Xie et al., 2016). A number of lncRNAs may act as scaffolding proteins by recruiting chromatin remodeling complexes, including the polycomb repressive complex 1 (PRC1) and PRC2, to silence target-specific genes (Mercer et al., 2009; Tripathi et al., 2010; Kotake et al., 2011; Wang and Chang, 2011; Li Y. et al., 2016; Xie et al., 2016). Furthermore, lncRNAs can scaffold HBXIP and LSD1 to form a complex that activates transcription of c-Myc target genes (Mercer et al., 2009; Tripathi et al., 2010; Kotake et al., 2011; Wang and Chang, 2011; Li Y. et al., 2016; Xie et al., 2016). lncRNAs can bind to transcription factors as decoys to sequester them away from their targets, thereby affecting gene transcription (Mercer et al., 2009; Tripathi et al., 2010; Kotake et al., 2011; Wang and Chang, 2011; Li Y. et al., 2016; Xie et al., 2016). LncRNAs may serve as molecular sponges by harboring binding sites for miRNAs and sequester them away from their mRNA targets (Mercer et al., 2009; Tripathi et al., 2010; Kotake et al., 2011; Wang and Chang, 2011; Li Y. et al., 2016; Xie et al., 2016). Certain LncRNAs can regulate RNA splicing either by interacting with splicing factors or by binding the splicing junctions of pre-mRNA (Mercer et al., 2009; Tripathi et al., 2010; Kotake et al., 2011; Wang and Chang, 2011; Li Y. et al., 2016; Xie et al., 2016).
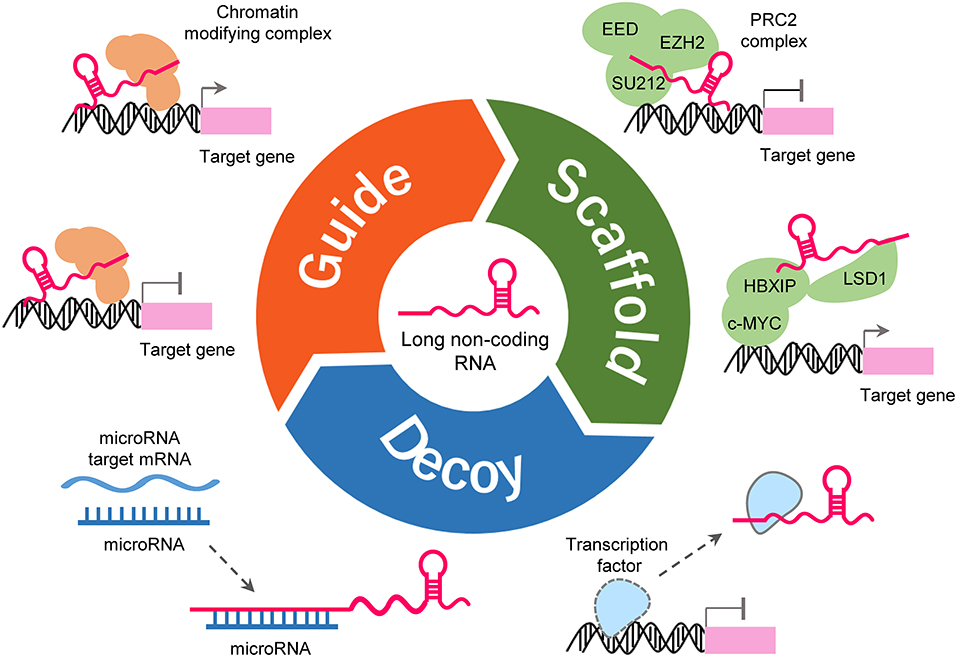
Figure 2. Functions and mechanisms of lncRNA. LncRNA can guide transcription factors to specific genomic locations for the regulation of gene expression (upper-left). LncRNA works as a scaffold to facilitate the assembling of chromatin remodeling complexes (upper-right). LncRNA can also serve as a sponge to titrate miRNAs out from their mRNA targets (lower-left). LncRNA can bind to transcription factors or other proteins as a decoy and sequester them away from chromatin (lower-right).
The Roles of lncRNAs in Cancer
Increasing number of studies have shown that lncRNAs are either up- or down-regulated in cancers. Some lncRNAs are expressed in a cell type-specific manner during differentiation and in certain cancers, whereas several lncRNAs such as MALAT1 are widely overexpressed in various cancers (Prensner and Chinnaiyan, 2011; Wang Y. et al., 2016). Their dysregulation is associated with tumorigenesis, cancer progression, metastasis and prognosis of various tumors (Schmitt and Chang, 2016), suggesting that lncRNAs may have roles as potential biomarkers for cancer. Actually, lncRNAs have been identified as prognostic and diagnostic biomarkers for lymph node metastasis and distant metastasis in early-stage cancer (Chen J. et al., 2016). Furthermore, extracellular lncRNAs can be stable and detectable in bodily fluids (such as blood and urine), thus the levels of circulating lncRNAs can be considered as promising non-invasive biomarkers over conventional biomarkers (Shi et al., 2016).
Through the mechanisms mentioned in Figure 2, lncRNAs exert their oncogenic or tumor suppressive functions in human tumors and they are key regulators of pathways involving all hallmarks of cancer (Prensner and Chinnaiyan, 2011; Schmitt and Chang, 2016; Wang Y. et al., 2016). Due to the massive involvement of lncRNAs in the mechanistic, functional and translational aspects of cancer biology, they have been explored as therapeutic targets. For example, aberrant expression of the lncRNA H19 occurs in ovarian cancer and other types of cancers (Yoshimura et al., 2018). The toxin vector DTA-H19 is a plasmid that expresses the diphtheria toxin A chain under the control of the H19 gene regulatory sequences (Mizrahi et al., 2009). The intra-tumoral injection of DTA-H19 caused significant inhibition of tumor growth in ovarian cancer xenograft models (Mizrahi et al., 2009). The safety, tolerability, and efficacy of DTA-H19 have been verified in a phase 1/2a clinical trial for the treatment of H19-overexpressing bladder cancer (Sidi et al., 2008).
Recent studies have demonstrated a complicated interplay and cross-regulation among different species of non-coding RNAs (Deng et al., 2016). LncRNAs and miRNAs can directly interact with and regulate each other through at least four mechanisms (Deng et al., 2016): (i) miRNA can decrease the abundance of lncRNA by reducing its stability (Yoon et al., 2012); (ii) lncRNAs can serve as sponges or decoys for miRNAs to decrease the available levels of miRNAs (Cesana et al., 2011); (iii) lncRNAs may compete with miRNAs for binding to mRNAs (Faghihi et al., 2010); and (iv) lncRNAs generate miRNAs from their exons and introns (Cesana et al., 2011).
NEAT1, a Novel Player in Tumor
Nuclear paraspeckle assembly transcript 1 (NEAT1, a lncRNA) is transcribed from the familial tumor syndrome multiple endocrine neoplasia (MEN) type 1 loci on chromosome 11q13.1 and encodes two transcriptional variants, NEAT1-1 (3756 bp) and NEAT1-2 (22,743 bp) (Bond and Fox, 2009; Figure 3A). NEAT1 is enriched in the nucleus but also found in the cytoplasm (van Heesch et al., 2014). NEAT1 appears to be dispensable for normal embryonic development and adult life, as mice lacking NEAT1 develop normally (Nakagawa et al., 2011). However, another study reported that genetic ablation of NEAT1 resulted in aberrant mammary gland morphogenesis and lactation defects (Standaert et al., 2014). Whether the loss of NEAT1 is compatible with normal cell viability and normal development should be further evaluated.
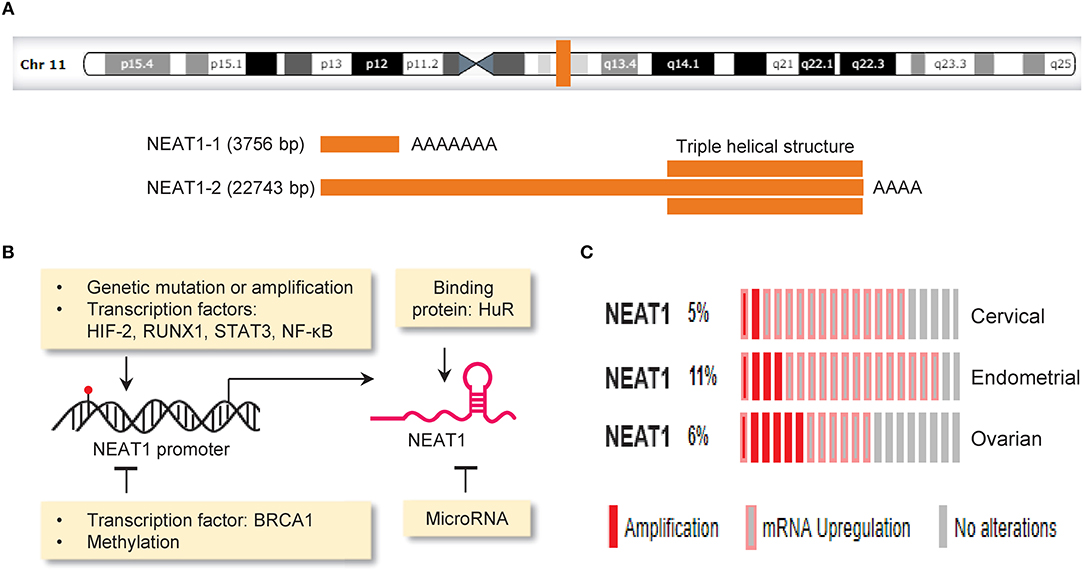
Figure 3. Mechanisms driving aberrant NEAT1 expression in cancer. (A) Genomic locus of the lncRNA NEAT1 on chromosome 11q13.1 (upper panel).The structure of NEAT1-1 and NEAT1-2 bottom panel). Two isoforms of NEAT1 (3,756 bp NEAT1-1 and 22,743 bp NEAT1-2) are transcribed from the same locus. NEAT1-2 possesses a unique triple helical structure at the 3′ end. (B) The expression of NEAT1 can be regulated by gene mutation (red), copy-number changes, transcription factors, DNA methylation, miRNA and RNA-binding protein. (C) Genetic alterations (amplification and RNA upregulation) of NEAT1 in samples of cervical, endometrial and ovarian cancer. The Cancer Genome Atlas (TCGA) datasets were retrieved in the cBioPortal database (www.cbioportal.org). Unaltered cases (gray) were not shown.
NEAT1 displays typical characteristics of cancer drivers, because it is responsible for tumor initiation and progression, and its frequent dysregulation in cancers correlates with clinical features such as metastasis, recurrence rate and patient survival (Lanzós et al., 2017).
Increasing evidence suggested that NEAT1 is overexpressed in many solid tumors, including non-small cell lung cancer (Pan et al., 2015; Sun et al., 2016), ovarian cancer (Kim et al., 2010; Chen Z. J. et al., 2016), cervical cancer (Han et al., 2017), hepatocellular carcinoma (Guo et al., 2015; Liu Z. et al., 2017), colorectal cancer (Li et al., 2015; Peng et al., 2017), gastric cancer (Ma et al., 2016), esophageal squamous cell carcinoma (Chen et al., 2015), endometrial cancer (Li Z. et al., 2016; Wang J. et al., 2017), cholangiocarcinoma (Zhang C. et al., 2018), laryngeal squamous cell cancer (Wang P. et al., 2016), pancreatic cancer (Huang et al., 2017), thyroid carcinoma (Li J. H et al., 2017), oral squamous cell carcinoma (Huang et al., 2018), nasopharyngeal carcinoma (Cheng and Guo, 2017; Liu F. et al., 2018), osteosarcoma (Wang H. et al., 2017; Hu et al., 2018), breast cancer (Zhang et al., 2017; Zhao et al., 2017), glioma (Zhen et al., 2016) and renal cell carcinoma (Liu et al., 2017a; Ning et al., 2017). However, its expression was reduced in acute promyelocytic leukemia (Zeng et al., 2014), indicating that the role of NEAT1 may vary with cancer types.
The overexpression of NEAT1 was significantly associated with poor overall survival in non-small cell lung cancer (Pan et al., 2015), ovarian cancer (Chen Z. J. et al., 2016), cervical cancer (Han et al., 2017), colorectal cancer (Li et al., 2015; Peng et al., 2017), hepatocellular carcinoma (Liu Z. et al., 2017), esophageal squamous cell carcinoma (Chen et al., 2015), pancreatic cancer (Huang et al., 2017), oral squamous cell carcinoma (Huang et al., 2018), breast cancer (Zhao et al., 2017), gastric cancer (Fu et al., 2016), breast cancer (Zhang et al., 2017), and renal cell carcinoma (Ning et al., 2017). Moreover, higher expression levels of NEAT1 were positively correlated with cancer stage and metastasis in endometrial cancer (Li Z. et al., 2016), ovarian cancer (Chen Z. J. et al., 2016), non-small cell lung cancer (Pan et al., 2015; Sun et al., 2017), hepatocellular carcinoma (Sun et al., 2016), esophageal squamous cell carcinoma (Chen et al., 2015), laryngeal squamous cell cancer (Wang P. et al., 2016), breast cancer (Zhao et al., 2017), gastric cancer (Fu et al., 2016), and osteosarcoma (Hu et al., 2018).
Mechanisms of NEAT1 Dysregulation in Cancer
The expression of NEAT1 in cancer cells is controlled by the following mechanisms: genetic alterations (such as copy number gain and gene mutation), transcription factors, DNA methylation, miRNAs and RNA-binding protein (Figure 3B).
The Cancer Genome Atlas (TCGA, a large-scale cancer genomics project) has revealed molecular alterations such as gene mutations, copy-number changes, upregulation or downregulation of mRNA and lncRNA across diverse tumor types. A comprehensive study analyzing lncRNA alterations in the TCGA datasets covering 5,860 tumor samples from 13 cancer types revealed that on average 13.16% of lncRNAs underwent copy number gains and 13.53% of lncRNAs underwent copy number loss (Yan et al., 2015). Here, we used the TCGA cervical, endometrial and ovarian cancer datasets to annotate genetic alterations of NEAT1 in these gynecological cancers via the cBioPortal online application (http://www.cbioportal.org). Consistent with previous observations (Kim et al., 2010; Chen Z. J. et al., 2016; Li Z. et al., 2016; Han et al., 2017; Wang J. et al., 2017), we found that genetic alterations occur in 5–11% of cervical, endometrial and ovarian cancers, and the predominant alterations were amplification and RNA upregulation (Figure 3C). The presence of copy number gain in NEAT1 gene might provide a possible explanation for high NEAT1 expression in these cancers. In addition, deep sequencing analysis of breast cancers has indicated mutations in the NEAT1 promoter region (Rheinbay et al., 2017). Another group also found an elevation of mutations in the NEAT1 promoter in renal cell carcinoma, and these mutations were associated with increased NEAT1 expression and unfavorable patient survival (Li S. et al., 2017). Although the functional impact of these mutations is unknown, mutations that occur in the NEAT1 promoter might affect the binding of a protein to the NEAT1 promoter and alter its expression (Rheinbay et al., 2017).
Several studies reported that transcription factors such as hypoxia-inducible factor (HIF)-2 and RUNX1 bind to the locus of NEAT1 and induce its expression in breast cancer (Choudhry et al., 2015; Barutcu et al., 2016). STAT3 and NF-κB, two downstream effectors of EGFR signaling, could bind to and activate the NEAT1 promoter in glioblastoma (Chen et al., 2018). On the other hand, BRCA1 inhibits NEAT1 expression through binding to its genomic binding site upstream of the NEAT1 gene in breast cancer (Lo et al., 2016). At the post-transcriptional level, NEAT1 is physically associated with and stabilized by an RNA-binding protein HuR (Chai et al., 2016).
Epigenetic mechanisms such as aberrant DNA methylation and miRNA dysregulation account for the aberrant expression of lncRNAs in tumors (Choudhry et al., 2015; Yan et al., 2015). The expression of NEAT1 was increased by the treatment with 5-AZA in hepatocellular carcinoma cells, indicating that DNA methylation is an important determinant of NEAT1 expression (Fang et al., 2017). Numerous miRNAs that directly target lncRNAs have identified in tumor cells (Braconi et al., 2011), and miRNAs that directly interact with NEAT1 will be discussed below. How epigenetic mechanisms (such as histone modifications) contribute to the transcriptional control of NEAT1 expression warrants further investigation.
NEAT1 Controls Cancer Initiation and Progression
NEAT1 inhibits cell cycle arrest and apoptosis, but promotes migration, invasion, metastasis, epithelial-to-mesenchymal transition (EMT), stem cell-like phenotype, chemoresistance and radioresistance, through at least three different molecular mechanisms (Figure 4 and Table 1): (i) NEAT1 functions as a scaffold RNA molecule by interacting with EZH2 (a subunit of the polycomb repressive complex) to influence the expression of downstream effectors of EZH2, (ii) NEAT1 acts as miRNA sponges to antagonize the interactions between multiple tumor suppressor miRNAs and target mRNAs, and (iii) NEAT1 suppresses the expression of miR-129 by promoting the DNA methylation of the miR-129 promoter region.
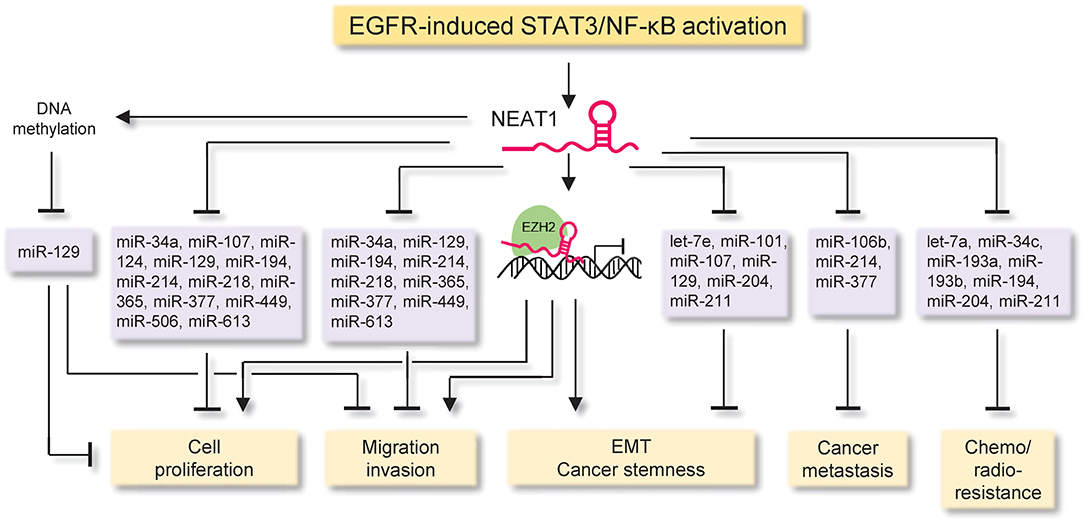
Figure 4. The proposed mechanisms of NEAT1 action in cancer. The diagram illustrates signaling events upstream and downstream of NEAT1 in cancer. The EGFR-STAT3/NF-κB signaling pathway is a critical triggering mechanism upstream of NEAT1, which inhibits cell cycle arrest and apoptosis, but promotes migration, invasion, metastasis, epithelial-to-mesenchymal transition, stem cell-like phenotype, chemoresistance and radioresistance, through at least three major mechanisms: (i) NEAT1 functions as a scaffold RNA molecule by interacting with EZH2 (a subunit of the polycomb repressive complex) to influence the expression of downstream effectors of EZH2, (ii) NEAT1 acts as a miRNA sponge to antagonize the interactions between multiple tumor suppressor miRNAs and target mRNAs, and (iii) NEAT1 suppresses the expression of miR-129 by promoting the DNA methylation of the miR-129 promoter.
In gastric cancer, laryngeal squamous cell cancer, pancreatic cancer, oral squamous cell carcinoma, nasopharyngeal carcinoma and breast cancer, knockdown of NEAT1 via small interfering RNA (siRNA) inhibited cell proliferation (Ma et al., 2016; Wang P. et al., 2016; Cheng and Guo, 2017; Huang et al., 2017, 2018; Qian et al., 2017). Similarly, the inhibition of NEAT1 via siRNA reduced cancer cell proliferation, migration and invasion in esophageal squamous cell carcinoma, endometrial cancer, glioma, hepatocellular carcinoma and renal cell carcinoma (Chen et al., 2015; Li Z. et al., 2016; Zhen et al., 2016; Ning et al., 2017; Wang Z. et al., 2017). Furthermore, short hairpin RNA (shRNA)-mediated silencing of NEAT1 significantly impaired cell proliferation, migration and invasion in cholangiocarcinoma (Zhang C. et al., 2018). Conversely, forced expression of NEAT1 enhanced cell growth, migration and invasion in endometrial cancer, papillary thyroid cancer, nasopharyngeal carcinoma, ovarian cancer, non-small cell lung cancer and breast cancer (Li Z. et al., 2016; Sun et al., 2016; Cheng and Guo, 2017; Ding et al., 2017; Wang J. et al., 2017; Zhao et al., 2017; Zhang H. et al., 2018).
EMT is a complex process in which epithelial cells acquire the characteristics of invasive mesenchymal cells and has been shown to contribute to tumorigenesis, invasion, metastasis, resistance to conventional chemotherapy, radiotherapy and small-molecule-targeted therapy (Dong et al., 2016; Nieto et al., 2016; Huo et al., 2017). Cancer stem cells (CSCs) are a class of pluripotent cells that possess a capacity for self-renewal and are resistant to chemotherapy and radiotherapy (Ayob and Ramasamy, 2018). Previous studies have established a link between EMT and CSC formation (Mani et al., 2008; Dong et al., 2014). NEAT1 overexpression promotes EMT and invasion in breast cancer, renal cell carcinoma, renal cell carcinoma, hepatoblastoma and nasopharyngeal carcinoma (Lu et al., 2016; Fu et al., 2017; Liu et al., 2017a; Li W. et al., 2017; Ning et al., 2017; Zhang et al., 2017; Zheng et al., 2018). Importantly, NEAT1 was found to be overexpressed in CD133+ glioma stem cells and knockdown of NEAT1 by siRNA reduced the ability of these cells to form colonies in soft agar (Yang et al., 2017). Glioma stem cells transfected with NEAT1 shRNA exhibited weaker proliferation, migration and invasion than that of those cells transfected with control shRNA (Gong et al., 2016). In sphere-forming cells generated from certain non-small cell lung cancer cell lines, downregulation of NEAT1 resulted in a significant decrease in the expression of CSC markers (CD133, CD44, ABCG2, Sox2, Nanog, and Oct-4) (Jiang et al., 2018). Not surprisingly, NEAT1 silencing by siRNA sensitized tumor cells to anti-cancer drugs such as sorafenib (Liu et al., 2017a), cisplatin (Hu et al., 2018; Liu F. et al., 2018), dexamethasone (Wu and Wang, 2018), and paclitaxel (An et al., 2017). shRNA-mediated reduction of NEAT1 enhanced the in vitro radiosensitivity of cancer cell lines to radiation therapy (Lu et al., 2016; Han et al., 2017).
NEAT1-1 and NEAT1-2 appear to have distinct roles in regulating the phenotypes of cancer cells. For example, in colorectal cancer cell lines, knockdown of NEAT1-1 could inhibit cell invasion and proliferation, whereas knockdown of NEAT1-2 promoted cell growth (Wu et al., 2015). The same study showed that expression of NEAT1-1 was significantly higher in liver metastatic lesions compared with adjacent normal colorectal tissues and primary colorectal cancer tissues (Wu et al., 2015). These findings suggested that NAT1-1 may act as a carcinogenic factor, while NEAT1-2 may be a tumor suppressor in colorectal cancer. It appears that the expression of NEAT1 isoforms is regulated in a cell type-specific manner. For example, in adult mouse tissues, NEAT1-1 is expressed in a broad range of cell types (Nakagawa et al., 2011). However, NEAT1-2 expression is largely restricted to the epithelial layers of digestive tissues (Nakagawa et al., 2011). The compositions of NEAT1 isoforms may vary in different cancer types. These dynamic changes in NEAT1 isoform expression may affect the main function of NEAT1 as an oncogene or a tumor suppressor. Therefore, elucidating the exact contribution of NEAT1 isoforms to the development of human tumors would be an exciting direction for future studies.
Mechanistically, NEAT1 recruits EZH2 to form the PRC2 complex and mediate the expression of EZH2 target genes, thereby promoting tumor cell growth and invasion in glioblastoma and cholangiocarcinoma (Chen et al., 2018; Zhang C. et al., 2018; Figure 4 and Table 1).
Additionally, by sponging a set of miRNAs, such as let-7a (Liu F. et al., 2018), let-7e (Gong et al., 2016), miR-34a (Ding et al., 2017; Liu et al., 2017a), miR-34c (Hu et al., 2018), miR-101 (Qian et al., 2017; Wang Y. et al., 2017), miR-106b (Sun et al., 2018), miR-107 (Wang P. et al., 2016; Yang et al., 2017), miR-124 (Chai et al., 2016; Cheng and Guo, 2017; Liu X. et al., 2018), miR-193a (Wu and Wang, 2018), miR-193b (Han et al., 2017), miR-194 (An et al., 2017; Wang H. et al., 2017), miR-204 (Lu et al., 2016), miR-211 (Li X. et al., 2017), miR-214 (Li J. H et al., 2017; Wang J. et al., 2017), miR-218 (Zhao et al., 2017), miR-365 (Huang et al., 2018), miR-377 (Sun et al., 2016), miR-449 (Zhen et al., 2016), miR-506 (Huang et al., 2017), and miR-613 (Wang Z. et al., 2017), NEAT1 could abolish miRNAs-mediated suppression of their target genes, therefore promoting tumor cell growth, migration, invasion, metastasis, EMT, stem cell-like phenotype, chemoresistance and radioresistance (Figure 4 and Table 1).
Changes in miRNA expression through altered DNA methylation affect tumor progression. NEAT1 was shown to silence the expression of miR-129 by increasing the DNA methylation level in its promoter region in breast cancer cells (Lo et al., 2016; Table 1).
Of note, NEAT1 overexpression induced the activity of the Wnt/β-catenin signaling, either by inhibiting the expression of miR-214 (Wang J. et al., 2017) or by interacting with EZH2 to reduce the expression of negative regulators of WNT/β-catenin signaling such as ICAT, GSK3B and Axin2 (Chen et al., 2018 Table 1).
NEAT1 as a Potential Diagnostic Biomarker of Cancer
Cancer type- or subtype-specific expression patterns and the sensitive and inexpensive quantitative detection methods for ncRNAs make lncRNAs suitable as hopeful biomarkers for cancer diagnosis and prognosis (Brunner et al., 2012; Bijnsdorp et al., 2017). LncRNAs can exhibit different expression patterns between subtypes of the same cancer (Su et al., 2014). Several lncRNAs (such as PCA3, PCGEM1 and PCAT-1) are highly specific to prostate cancer (Crea et al., 2014) and have been used to identify primary tumors.
Although lncRNAs are commonly expressed at lower levels than protein-coding mRNAs, some lncRNAs (including NEAT1) exhibit moderate to high levels of expression in clinical samples. Moreover, lncRNAs can be detected in body fluids such as serum, plasma, urine and saliva of cancer patients (Chandra Gupta and Nandan Tripathi, 2017). The diagnostic value of NEAT1 was validated in a cohort of colorectal cancer patients, because the whole-blood NEAT1 expression was significantly increased in cancer patients than persons without cancer (Wang Y. et al., 2017). Therefore, NEAT1 may be possibly used as a biomarker for the existence of primary cancer. The same study also reported that the whole-blood expression of NEAT1-1 was significantly higher in colorectal cancer patients with distant metastasis than in those without metastasis (Wang Y. et al., 2017). Thus, the whole-blood NEAT1 expression might serve as a biomarker for prediction of distant metastasis.
NEAT1 as a Potential Therapeutic Target in Cancer
Given the importance of the lncRNA-mediated networks that broadly affect cancer imitation and progression, lncRNAs are of interest for developing novel therapeutics. LncRNAs can be targeted by multiple approaches (Parasramka et al., 2016; Worku et al., 2017; Arun et al., 2018; Figure 5): (i) CRISPR/Cas-9 system can be used to knockout lncRNA; (ii) lncRNA transcript can be knocked down using lncRNA-specific siRNA and antisense oligonucleotide (ASO); (iii) small synthetic molecules/peptides/aptamers can be designed to block and antagonize the binding of lncRNAs with their binding partners (such as protein, DNA, RNA, or other interacting complexes); (iv) selective PRC1/2 inhibitors such as GSK343 (Ihira et al., 2017) can be used to inhibit the activity of lncRNA that function through chromatin remodeling; (v) miRNA regulates the expression of lncRNA, thus restoration or blocking of a specific miRNA using miRNA mimics or anti-miRNA inhibitor (such as locked nucleic acid and miRNA sponge) can indirectly alter the expression of lncRNA; (vi) synthetic lncRNA mimics could be used to restore the expression of tumor suppressor lncRNA; and (vii) approaches to target lncRNAs can be used in combination with other therapies such as chemotherapy and radiotherapy to enhance their effectiveness. For example, targeted depletion of NEAT1 by siRNA (Li J. H et al., 2017) or shRNA (Zhang C. et al., 2018; Zhang H. et al., 2018) displayed a significant therapeutic effect in vivo. In vivo studies also confirmed that knockdown of NEAT1 sensitized tumor cells to cisplatin/paclitaxel- or radio-induced tumor regression (An et al., 2017; Han et al., 2017; Hu et al., 2018).
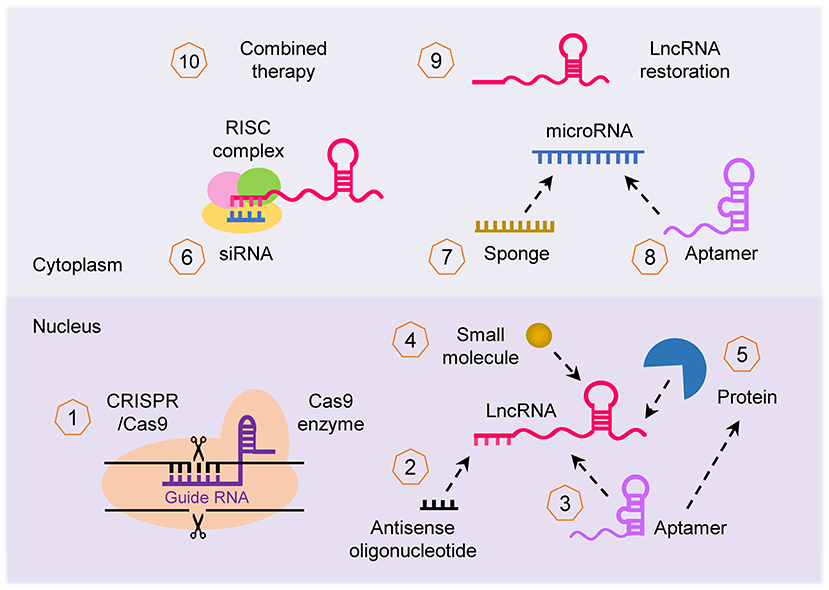
Figure 5. Therapeutic strategies to target lncRNA. Several approaches can be applied to target lncRNA in cancer for therapeutic purposes: (1) inhibition of lncRNA loci using the CRISPR/Cas-9 system, (2) knockdown of lncRNA transcript using antisense oligonucleotide, (3, 4) interfering with the binding of lncRNA with their binding partner (protein, DNA and RNA) with small synthetic molecule, peptide and aptamer, (5) repression of lncRNA that function through chromatin remodeling using selective PRC1/2 inhibitors, (6) silencing of lncRNA using specific siRNA, (7, 8) indirect targeting of lncRNA through modulating the function of miRNAs via miRNA mimics or anti-miRNA inhibitors such as aptamer, (9) restoring the expression of tumor suppressor lncRNA with synthetic lncRNA mimics, and (10) lncRNA can be applied for enhancing the effectiveness of other forms of therapy (such as chemotherapy and radiotherapy).
Apart from these strategies, targeting the upstream signaling pathways of NEAT1 or its binding partners would be another method to modulate NEAT1 expression or function in malignant tumors. Despite their potential side effects, blocking of NF-κB and STAT3 activity may represent a good approach to combat NEAT1-overexpressing tumors (Grivennikov and Karin, 2010; Liby et al., 2010). In addition, hypoxia is frequently observed in solid tumors and HIF-1/-2 has been potential targets for developing novel cancer therapeutics. A number of small molecule inhibitors of HIF-2 have been developed and might be used to downregulate the expression of NEAT1 (Yu et al., 2017). HuR is overexpressed in a wide variety of cancer types and stabilizes a large subset of mRNAs, which encode proteins implicated in tumor cell proliferation, survival, angiogenesis, invasion and metastasis (Kotta-Loizou et al., 2016). High-throughput screening methods have been established to identify small molecular molecules against HuR (Meisner et al., 2007). Thus, HuR inhibition may offer new conceptual routes to treat cancers expressing high levels of NEAT1.
Perspectives and Challenges
The current research on NEAT1 is still at a very early stage, but growing evidence has clearly identified NEAT1 as an attractive biomarker of cancer and an ideal candidate for lncRNA therapeutics. Although the broad implications of NEAT1 in cancers have been described, several key challenges associated with NEAT1 still exist: (i) The exact mechanisms for NEAT1-mediated carcinogenesis and metastasis remain elusive, although the interactions between PRC2 complex and miRNAs appears to be at least involved in these processes. High-throughput technologies such as ChIRP, PAR-CLIP and iCLIP have been used to reveal NEAT1-DNA or NEAT1-protein interactions (Hafner et al., 2010; Zhao et al., 2010; Chu et al., 2011; Tollervey et al., 2011; Yoon et al., 2014). (ii) The precise mechanisms by which the genetic and epigenetic factors contribute to NEAT1 dysregulation, as well as the downstream signaling pathways of NEAT1, are still far from clear. (iii) The NEAT1 loci might be targeted for therapeutic purposes using CRISPR/Cas-9 genome editing technology. However, a challenge is the safety evaluation of CRISPR/Cas-9-based therapeutics, such as the risk of disease occurrence due to unwanted mutations, the immune response to the delivery system and other toxic side effects. Taken together, NEAT1 has the potential to provide a new biomarker for the diagnosis and monitoring of cancers and will need to be taken into consideration in the development of NEAT1-targeting therapeutics (possibly in combination with chemotherapy and radiotherapy).
Author Contributions
PD and HW provided direction. PD, YX, and HW wrote the manuscript. JY, SH, NK, and YT made significant revisions to the manuscript. All authors read and approved the final manuscript.
Funding
This work was supported by a grant from JSPS Grant-in-Aid for Scientific Research (C) (16K11123 and 18K09278), the Science and Technology Planning Project of Guangdong Province, China (2014A020212124) and an NIH/NCI grant 1R21CA216585-01A1 to JY.
Conflict of Interest Statement
The authors declare that the research was conducted in the absence of any commercial or financial relationships that could be construed as a potential conflict of interest.
References
An, J., Lv, W., and Zhang, Y. (2017). LncRNA NEAT1 contributes to paclitaxel resistance of ovarian cancer cells by regulating ZEB1 expression via miR-194. Onco. Targets. Ther. 10, 5377–5390. doi: 10.2147/OTT.S147586
Arun, G., Diermeier, S. D., and Spector, D. L. (2018). Therapeutic targeting of long non-coding RNAs in cancer. Trends Mol. Med. 24, 257–277. doi: 10.1016/j.molmed.2018.01.001
Ayob, A. Z., and Ramasamy, T. S. (2018). Cancer stem cells as key drivers of tumour progression. J. Biomed. Sci. 25:20. doi: 10.1186/s12929-018-0426-4
Barutcu, A. R., Hong, D., Lajoie, B. R., McCord, R. P., van Wijnen, A. J., Lian, J. B., et al. (2016). RUNX1 contributes to higher-order chromatin organization and gene regulation in breast cancer cells. Biochim. Biophys. Acta 1859, 1389–1397. doi: 10.1016/j.bbagrm.2016.08.003
Bijnsdorp, I. V., van Royen, M. E., Verhaegh, G. W., and Martens-Uzunova, E. S. (2017). The non-coding transcriptome of prostate cancer: implications for clinical practice. Mol. Diagn. Ther. 21, 385–400. doi: 10.1007/s40291-017-0271-2
Bond, C. S., and Fox, A. H. (2009). Paraspeckles: nuclear bodies built on long noncoding RNA. J. Cell Biol. 186, 637–644. doi: 10.1083/jcb.200906113
Braconi, C., Kogure, T., Valeri, N., Huang, N., Nuovo, G., Costinean, S., et al. (2011). microRNA-29 can regulate expression of the long non-coding RNA gene MEG3 in hepatocellular cancer. Oncogene 30, 4750–4756. doi: 10.1038/onc.2011.193
Brunner, A. L., Beck, A. H., Edris, B., Sweeney, R. T., Zhu, S. X., Li, R., et al. (2012). Transcriptional profiling of long non-coding RNAs and novel transcribed regions across a diverse panel of archived human cancers. Genome Biol. 13:R75. doi: 10.1186/gb-2012-13-8-r75
Cesana, M., Cacchiarelli, D., Legnini, I., Santini, T., Sthandier, O., Chinappi, M., et al. (2011). A long noncoding RNA controls muscle differentiation by functioning as a competing endogenous RNA. Cell 147, 358–369. doi: 10.1016/j.cell.2011.09.028
Chai, Y., Liu, J., Zhang, Z., and Liu, L. (2016). HuR-regulated lncRNA NEAT1 stability in tumorigenesis and progression of ovarian cancer. Cancer Med. 5, 1588–1598. doi: 10.1002/cam4.710
Chandra Gupta, S., and Nandan Tripathi, Y. (2017). Potential of long non-coding RNAs in cancer patients: from biomarkers to therapeutic targets. Int. J. Cancer 140, 1955–1967. doi: 10.1002/ijc.30546
Chen, J., Chen, Y., Gu, L., Li, X., Gao, Y., Lyu, X., et al. (2016). LncRNAs act as prognostic and diagnostic biomarkers in renal cell carcinoma: a systematic review and meta-analysis. Oncotarget 7, 74325–74336. doi: 10.18632/oncotarget.11101
Chen, L. L. (2016). Linking long noncoding RNA localization and function. Trends Biochem. Sci. 41, 761–772. doi: 10.1016/j.tibs.2016.07.003
Chen, Q., Cai, J., Wang, Q., Wang, Y., Liu, M., Yang, J., et al. (2018). Long noncoding RNA NEAT1, regulated by the EGFR pathway, contributes to glioblastoma progression through the WNT/β-Catenin Pathway by scaffolding EZH2. Clin. Cancer Res. 24, 684–695. doi: 10.1158/1078-0432.CCR-17-0605
Chen, X., Kong, J., Ma, Z., Gao, S., and Feng, X. (2015). Up regulation of the long non-coding RNA NEAT1 promotes esophageal squamous cell carcinoma cell progression and correlates with poor prognosis. Am. J. Cancer Res. 5, 2808–2815.
Chen, Z. J., Zhang, Z., Xie, B. B., and Zhang, H. Y. (2016). Clinical significance of up-regulated lncRNA NEAT1 in prognosis of ovarian cancer. Eur. Rev. Med. Pharmacol. Sci. 20, 3373–3377.
Cheng, N., and Guo, Y. (2017). Long noncoding RNA NEAT1 promotes nasopharyngeal carcinoma progression through regulation of miR-124/NF-κB pathway. Onco. Targets. Ther. 10, 5843–5853. doi: 10.2147/OTT.S151800
Choudhry, H., Albukhari, A., Morotti, M., Haider, S., Moralli, D., Smythies, J., et al. (2015). Tumor hypoxia induces nuclear paraspeckle formation through HIF-2α dependent transcriptional activation of NEAT1 leading to cancer cell survival. Oncogene 34, 4482–4490. doi: 10.1038/onc.2014.378
Chu, C., Qu, K., Zhong, F. L., Artandi, S. E., and Chang, H. Y. (2011). Genomic maps of long noncoding RNA occupancy reveal principles of RNA-chromatin interactions. Mol. Cell 44, 667–678. doi: 10.1016/j.molcel.2011.08.027
Crea, F., Watahiki, A., Quagliata, L., Xue, H., Pikor, L., Parolia, A., et al. (2014). Identification of a long non-coding RNA as a novel biomarker and potential therapeutic target for metastatic prostate cancer. Oncotarget 5, 764–774. doi: 10.18632/oncotarget.1769
Deng, K., Wang, H., Guo, X., and Xia, J. (2016). The cross talk between long, non-coding RNAs and microRNAs in gastric cancer. Acta Biochim. Biophys. Sin. (Shanghai). 48, 111–116. doi: 10.1093/abbs/gmv120
Ding, N., Wu, H., Tao, T., and Peng, E. (2017). NEAT1 regulates cell proliferation and apoptosis of ovarian cancer by miR-34a-5p/BCL2. Onco. Targets. Ther. 10, 4905–4915. doi: 10.2147/OTT.S142446
Dong, P., Konno, Y., Watari, H., Hosaka, M., Noguchi, M., and Sakuragi, N. (2014). The impact of microRNA-mediated PI3K/AKT signaling on epithelial-mesenchymal transition and cancer stemness in endometrial cancer. J. Transl. Med. 12:231. doi: 10.1186/s12967-014-0231-0
Dong, P., Xiong, Y., Watari, H., Hanley, S. J., Konno, Y., Ihira, K., et al. (2016). Suppression of iASPP-dependent aggressiveness in cervical cancer through reversal of methylation silencing of microRNA-124. Sci. Rep. 6:35480. doi: 10.1038/srep35480
ENCODE Project Consortium (2012). An integrated encyclopedia of DNA elements in the human genome. Nature 489, 57–74. doi: 10.1038/nature11247
Esteller, M. (2011). Non-coding RNAs in human disease. Nat. Rev. Genet. 12, 861–874. doi: 10.1038/nrg3074
Faghihi, M. A., Zhang, M., Huang, J., Modarresi, F., Van der Brug, M. P., Nalls, M. A., et al. (2010). Evidence for natural antisense transcript-mediated inhibition of microRNA function. Genome Biol. 11:R56. doi: 10.1186/gb-2010-11-5-r56
Fang, L., Sun, J., Pan, Z., Song, Y., Zhong, L., Zhang, Y., et al. (2017). Long non-coding RNA NEAT1 promotes hepatocellular carcinoma cell proliferation through the regulation of miR-129-5p-VCP-IκB. Am. J. Physiol. Gastrointest. Liver Physiol. 313, G150–G156. doi: 10.1152/ajpgi.00426.2016
Fu, J. W., Kong, Y., and Sun, X. (2016). Long noncoding RNA NEAT1 is an unfavorable prognostic factor and regulates migration and invasion in gastric cancer. J. Cancer Res. Clin. Oncol. 142, 1571–1579. doi: 10.1007/s00432-016-2152-1
Fu, M. C., Yuan, L. Q., Zhang, T., Yan, X. M., Zhou, Y., Xia, H. L., et al. (2017). Nuclear paraspeckle assembly transcript 1 promotes the metastasis and epithelial-mesenchymal transition of hepatoblastoma cells by inhibiting miR-129-5p. Oncol. Lett. 14, 5773–5778. doi: 10.3892/ol.2017.6995
Gong, W., Zheng, J., Liu, X., Ma, J., Liu, Y., and Xue, Y. (2016). Knockdown of NEAT1 restrained the malignant progression of glioma stem cells by activating microRNA let-7e. Oncotarget 7, 62208–62223. doi: 10.18632/oncotarget.11403
Grivennikov, S. I., and Karin, M. (2010). Dangerous liaisons: STAT3 and NF-kappaB collaboration and crosstalk in cancer. Cytokine Growth Factor Rev. 21, 11–19. doi: 10.1016/j.cytogfr.2009.11.005
Guo, S., Chen, W., Luo, Y., Ren, F., Zhong, T., Rong, M., et al. (2015). Clinical implication of long non-coding RNA NEAT1 expression in hepatocellular carcinoma patients. Int. J. Clin. Exp. Pathol. 8, 5395–5402.
Hafner, M., Landthaler, M., Burger, L., Khorshid, M., Hausser, J., Berninger, P., et al. (2010). Transcriptome-wide identification of RNA-binding protein and microRNA target sites by PAR-CLIP. Cell 141, 129–141. doi: 10.1016/j.cell.2010.03.009
Han, D., Wang, J., and Cheng, G. (2017). LncRNA NEAT1 enhances the radio-resistance of cervical cancer via miR-193b-3p/CCND1 axis. Oncotarget 9, 2395–2409. doi: 10.18632/oncotarget.23416
Hu, Y., Yang, Q., Wang, L., Wang, S., Sun, F., Xu, D., et al. (2018). Knockdown of the oncogene lncRNA NEAT1 restores the availability of miR-34c and improves the sensitivity to cisplatin in osteosarcoma. Biosci. Rep. 38:BSR20180375. doi: 10.1042/BSR20180375
Huang, B., Liu, C., Wu, Q., Zhang, J., Min, Q., Sheng, T., et al. (2017). Long non-coding RNA NEAT1 facilitates pancreatic cancer progression through negative modulation of miR-506-3p. Biochem. Biophys. Res. Commun. 482, 828–834. doi: 10.1016/j.bbrc.2016.11.120
Huang, G., He, X., and Wei, X. L. (2018). lncRNA NEAT1 promotes cell proliferation and invasion by regulating miR-365/RGS20 in oral squamous cell carcinoma. Oncol. Rep. 39, 1948–1956. doi: 10.3892/or.2018.6283
Huo, W., Zhao, G., Yin, J., Ouyang, X., Wang, Y., Yang, C., et al. (2017). Lentiviral CRISPR/Cas9 vector mediated miR-21 gene editing inhibits the epithelial to mesenchymal transition in ovarian cancer cells. J. Cancer 8, 57–64. doi: 10.7150/jca.16723
Ihira, K., Dong, P., Xiong, Y., Watari, H., Konno, Y., Hanley, S. J., et al. (2017). EZH2 inhibition suppresses endometrial cancer progression via miR-361/Twist axis. Oncotarget 8, 13509–13520. doi: 10.18632/oncotarget.14586
Jiang, P., Chen, A., Wu, X., Zhou, M., Ul Haq, I., Mariyam, Z., et al. (2018). NEAT1 acts as an inducer of cancer stem cell-like phenotypes in NSCLC by inhibiting EGCG-upregulated CTR1. J. Cell. Physiol. 233, 4852–4863. doi: 10.1002/jcp.26288
Kapranov, P., Cheng, J., Dike, S., Nix, D. A., Duttagupta, R., Willingham, A. T., et al. (2007). RNA maps reveal new RNA classes and a possible function for pervasive transcription. Science 316, 1484–1488. doi: 10.1126/science.1138341
Kim, Y. S., Hwan, J. D., Bae, S., Bae, D. H., and Shick, W. A. (2010). Identification of differentially expressed genes using an annealing control primer system in stage III serous ovarian carcinoma. BMC Cancer 10:576. doi: 10.1186/1471-2407-10-576
Kotake, Y., Nakagawa, T., Kitagawa, K., Suzuki, S., Liu, N., Kitagawa, M., et al. (2011). Long non-coding RNA ANRIL is required for the PRC2 recruitment to and silencing of p15(INK4B) tumor suppressor gene. Oncogene 30, 1956–1962. doi: 10.1038/onc.2010.568
Kotta-Loizou, I., Vasilopoulos, S. N., Coutts, R. H., and Theocharis, S. (2016). Current evidence and future perspectives on HuR and breast cancer development, prognosis, and treatment. Neoplasia 18, 674–688. doi: 10.1016/j.neo.2016.09.002
Lanzós, A., Carlevaro-Fita, J., Mularoni, L., Reverter, F., Palumbo, E., Guigó, R., et al. (2017). Discovery of cancer driver long noncoding RNAs across 1112 tumour genomes: new candidates and distinguishing features. Sci. Rep. 7:41544. doi: 10.1038/srep41544
Li, J. H., Zhang, S. Q., Qiu, X. G., Zhang, S. J., Zheng, S. H., and Zhang, D. H. (2017). Long non-coding RNA NEAT1 promotes malignant progression of thyroid carcinoma by regulating miRNA-214. Int. J. Oncol. 50, 708–716. doi: 10.3892/ijo.2016.3803
Li, S., Shuch, B. M., and Gerstein, M. B. (2017). Whole-genome analysis of papillary kidney cancer finds significant noncoding alterations. PLoS Genet. 13:e1006685. doi: 10.1371/journal.pgen.1006685
Li, W., Zhang, Z., Liu, X., Cheng, X., Zhang, Y., Han, X., et al. (2017). The FOXN3-NEAT1-SIN3A repressor complex promotes progression of hormonally responsive breast cancer. J. Clin. Invest. 127, 3421–3440. doi: 10.1172/JCI94233
Li, X., Wang, S., Li, Z., Long, X., Guo, Z., Zhang, G., et al. (2017). The lncRNA NEAT1 facilitates cell growth and invasion via the miR-211/HMGA2 axis in breast cancer. Int. J. Biol. Macromol. 105(Pt 1), 346–353. doi: 10.1016/j.ijbiomac.2017.07.053
Li, Y., Li, Y., Chen, W., He, F., Tan, Z., Zheng, J., et al. (2015). NEAT expression is associated with tumor recurrence and unfavorable prognosis in colorectal cancer. Oncotarget 6, 27641–27650. doi: 10.18632/oncotarget.4737
Li, Y., Wang, Z., Shi, H., Li, H., Li, L., Fang, R., et al. (2016). HBXIP and LSD1 scaffolded by lncRNA hotair mediate transcriptional activation by c-Myc. Cancer Res. 76, 293–304. doi: 10.1158/0008-5472.CAN-14-3607
Li, Z., Wei, D., Yang, C., Sun, H., Lu, T., and Kuang, D. (2016). Overexpression of long noncoding RNA, NEAT1 promotes cell proliferation, invasion and migration in endometrial endometrioid adenocarcinoma. Biomed. Pharmacother. 84, 244–251. doi: 10.1016/j.biopha.2016.09.008
Liby, K. T., Royce, D. B., Risingsong, R., Williams, C. R., Maitra, A., Hruban, R. H., et al. (2010). Synthetic triterpenoids prolong survival in a transgenic mouse model of pancreatic cancer. Cancer Prev. Res(Phila). 3, 1427–1434. doi: 10.1158/1940-6207.CAPR-10-0197
Liu, F., Chen, N., Gong, Y., Xiao, R., Wang, W., and Pan, Z. (2017a). The long non-coding RNA NEAT1 enhances epithelial-to-mesenchymal transition and chemoresistance via the miR-34a/c-Met axis in renal cell carcinoma. Oncotarget 8, 62927–62938. doi: 10.18632/oncotarget.17757
Liu, F., Somarowthu, S., and Pyle, A. M. (2017b). Visualizing the secondary and tertiary architectural domains of lncRNA RepA. Nat. Chem. Biol. 13, 282–289. doi: 10.1038/nchembio.2272
Liu, F., Tai, Y., and Ma, J. (2018). LncRNA NEAT1/let-7a-5p axis regulates the cisplatin resistance in nasopharyngeal carcinoma by targeting Rsf-1 and modulating the Ras-MAPK pathway. Cancer Biol. Ther. 19, 534–542. doi: 10.1080/15384047.2018.1450119
Liu, X., Liang, Y., Song, R., Yang, G., Han, J., Lan, Y., et al. (2018). Long non-coding RNA NEAT1-modulated abnormal lipolysis via ATGL drives hepatocellular carcinoma proliferation. Mol. Cancer 17:90. doi: 10.1186/s12943-018-0838-5
Liu, Z., Chang, Q., Yang, F., Liu, B., Yao, H. W., Bai, Z. G., et al. (2017). Long non-coding RNA NEAT1 overexpression is associated with unfavorable prognosis in patients with hepatocellular carcinoma after hepatectomy: a Chinese population-based study. Eur. J. Surg. Oncol. 43, 1697–1703. doi: 10.1016/j.ejso.2017.06.013
Lo, P. K., Zhang, Y., Wolfson, B., Gernapudi, R., Yao, Y., Duru, N., et al. (2016). Dysregulation of the BRCA1/long non-coding RNA NEAT1 signaling axis contributes to breast tumorigenesis. Oncotarget 7, 65067–65089. doi: 10.18632/oncotarget.11364
Lu, Y., Li, T., Wei, G., Liu, L., Chen, Q., Xu, L., et al. (2016). The long non-coding RNA NEAT1 regulates epithelial to mesenchymal transition and radioresistance in through miR-204/ZEB1 axis in nasopharyngeal carcinoma. Tumour Biol. 37, 11733–11741. doi: 10.1007/s13277-015-4773-4
Ma, Y., Liu, L., Yan, F., Wei, W., Deng, J., and Sun, J. (2016). Enhanced expression of long non-coding RNA NEAT1 is associated with the progression of gastric adenocarcinomas. World J. Surg. Oncol. 14:41. doi: 10.1186/s12957-016-0799-3
Mani, S. A., Guo, W., Liao, M. J., Eaton, E. N., Ayyanan, A., Zhou, A. Y., et al. (2008). The epithelial-mesenchymal transition generates cells with properties of stem cells. Cell 133, 704–715. doi: 10.1016/j.cell.2008.03.027
Meisner, N. C., Hintersteiner, M., Mueller, K., Bauer, R., Seifert, J. M., Naegeli, H. U., et al. (2007). Identification and mechanistic characterization of low-molecular-weight inhibitors for HuR. Nat. Chem. Biol. 3, 508–515. doi: 10.1038/nchembio.2007.14
Mercer, T. R., Dinger, M. E., and Mattick, J. S. (2009). Long non-coding RNAs:insights into functions. Nat. Rev. Genet. 10, 155–159. doi: 10.1038/nrg2521
Mercer, T. R., and Mattick, J. S. (2013). Structure and function of long noncoding RNAs in epigenetic regulation. Nat. Struct. Mol. Biol. 20, 300–307. doi: 10.1038/nsmb.2480
Mizrahi, A., Czerniak, A., Levy, T., Amiur, S., Gallula, J., Matouk, I., et al. (2009). Development of targeted therapy for ovarian cancer mediated by a plasmid expressing diphtheria toxin under the control of H19 regulatory sequences. J. Transl. Med. 7:69. doi: 10.1186/1479-5876-7-69
Nakagawa, S., Naganuma, T., Shioi, G., and Hirose, T. (2011). Paraspeckles are subpopulation-specific nuclear bodies that are not essential in mice. J. Cell Biol. 193, 31–39. doi: 10.1083/jcb.201011110
Nieto, M. A., Huang, R. Y., Jackson, R. A., and Thiery, J. P. (2016). EMT: 2016. Cell 166, 21–45. doi: 10.1016/j.cell.2016.06.028
Ning, L., Li, Z., Wei, D., Chen, H., and Yang, C. (2017). LncRNA, NEAT1 is a prognosis biomarker and regulates cancer progression via epithelial-mesenchymal transition in clear cell renal cell carcinoma. Cancer Biomark. 19, 75–83. doi: 10.3233/CBM-160376
Pan, L. J., Zhong, T. F., Tang, R. X., Li, P., Dang, Y. W., Huang, S. N., et al. (2015). Upregulation and clinicopathological significance of long non-coding NEAT1 RNA in NSCLC tissues. Asian Pac. J. Cancer Prev. 16, 2851–2855. doi: 10.7314/APJCP.2015.16.7.2851
Parasramka, M. A., Maji, S., Matsuda, A., Yan, I. K., and Patel, T. (2016). Long non-coding RNAs as novel targets for therapy in hepatocellular carcinoma. Pharmacol. Ther. 161, 67–78. doi: 10.1016/j.pharmthera.2016.03.004
Peng, W., Wang, Z., and Fan, H. (2017). LncRNA NEAT1 impacts cell proliferation and apoptosis of colorectal cancer via regulation of Akt signaling. Pathol. Oncol. Res. 23, 651–656. doi: 10.1007/s12253-016-0172-4
Prensner, J. R., and Chinnaiyan, A. M. (2011). The emergence of lncRNAs in cancer biology. Cancer Discov. 1, 391–407. doi: 10.1158/2159-8290.CD-11-0209
Qian, K., Liu, G., Tang, Z., Hu, Y., Fang, Y., Chen, Z., et al. (2017). The long non-coding RNA NEAT1 interacted with miR-101 modulates breast cancer growth by targeting EZH2. Arch. Biochem. Biophys. 615, 1–9. doi: 10.1016/j.abb.2016.12.011
Rheinbay, E., Parasuraman, P., Grimsby, J., Tiao, G., Engreitz, J. M., Kim, J., et al. (2017). Recurrent and functional regulatory mutations in breast cancer. Nature 547, 55–60. doi: 10.1038/nature22992
Schmitt, A. M., and Chang, H. Y. (2016). Long noncoding RNAs in cancer pathways. Cancer Cell 29, 452–463. doi: 10.1016/j.ccell.2016.03.010
Shi, T., Gao, G., and Cao, Y. (2016). Long noncoding RNAs as novel biomarkers have a promising future in cancer diagnostics. Dis. Markers 2016:9085195. doi: 10.1155/2016/9085195
Sidi, A. A., Ohana, P., Benjamin, S., Shalev, M., Ransom, J. H., Lamm, D., et al. (2008). Phase I/II marker lesion study of intravesical BC-819 DNA plasmid in H19 over expressing superficial bladder cancer refractory to bacillus Calmette-Guerin. J. Urol. 180, 2379–2383. doi: 10.1016/j.juro.2008.08.006
Standaert, L., Adriaens, C., Radaelli, E., Van Keymeulen, A., Blanpain, C., Hirose, T., et al. (2014). The long noncoding RNA Neat1 is required for mammary gland development and lactation. RNA 20, 1844–1849. doi: 10.1261/rna.047332.114
Su, X., Malouf, G. G., Chen, Y., Zhang, J., Yao, H., Valero, V., et al. (2014). Comprehensive analysis of long non-coding RNAs in human breast cancer clinical subtypes. Oncotarget 5, 9864–9876. doi: 10.18632/oncotarget.2454
Sun, C., Li, S., Zhang, F., Xi, Y., Wang, L., Bi, Y., et al. (2016). Long non-coding RNA NEAT1 promotes non-small cell lung cancer progression through regulation of miR-377-3p-E2F3 pathway. Oncotarget 7, 51784–51814. doi: 10.18632/oncotarget.10108
Sun, S. J., Lin, Q., Ma, J. X., Shi, W. W., Yang, B., and Li, F. (2017). Long non-coding RNA NEAT1 acts as oncogene in NSCLC by regulating the Wnt signaling pathway. Eur. Rev. Med. Pharmacol. Sci. 21, 504–510.
Sun, W., Lan, X., Zhang, H., Wang, Z., Dong, W., He, L., et al. (2018). NEAT1_2 functions as a competing endogenous RNA to regulate ATAD2 expression by sponging microRNA-106b-5p in papillary thyroid cancer. Cell Death Dis. 9:380. doi: 10.1038/s41419-018-0418-z
Thum, T., and Condorelli, G. (2015). Long noncoding RNAs and microRNAs in cardiovascular pathophysiology.Circ. Res. 116, 751–762. doi: 10.1161/CIRCRESAHA.116.303549
Tollervey, J. R., Curk, T., Rogelj, B., Briese, M., Cereda, M., Kayikci, M., et al. (2011). Characterizing the RNA targets and position-dependent splicing regulation by TDP-43. Nat. Neurosci. 14, 452–458. doi: 10.1038/nn.2778
Tripathi, V., Ellis, J. D., Shen, Z., Song, D. Y., Pan, Q., Watt, A. T., et al. (2010). The nuclear-retained noncoding RNA MALAT1 regulates alternative splicing by modulating SR splicing factor phosphorylation. Mol. Cell 39, 925–938. doi: 10.1016/j.molcel.2010.08.011
van Heesch, S., van Iterson, M., Jacobi, J., Boymans, S., Essers, P. B., de Bruijn, E., et al. (2014). Extensive localization of long noncoding RNAs to the cytosol and mono- and polyribosomal complexes. Genome Biol. 15:R6. doi: 10.1186/gb-2014-15-1-r6
Wang, H., Yu, Y., Fan, S., and Luo, L. (2017). Knockdown of long non-coding RNA NEAT1 inhibits proliferation and invasion and induces apoptosis of osteosarcoma by inhibiting miR-194 expression. Yonsei Med. J. 58, 1092–1100. doi: 10.3349/ymj.2017.58.6.1092
Wang, J., Zhao, X., Guo, Z., Ma, X., Song, Y., and Guo, Y. (2017). Regulation of NEAT1/miR-214-3p on the growth, migration and invasion of endometrial carcinoma cells. Arch. Gynecol. Obstet. 295, 1469–1475. doi: 10.1007/s00404-017-4365-1
Wang, K. C., and Chang, H. Y. (2011). Molecular mechanisms of long noncoding RNAs. Mol. Cell 43, 904–914. doi: 10.1016/j.molcel.2011.08.018
Wang, P., Wu, T., Zhou, H., Jin, Q., He, G., Yu, H., et al. (2016). Long noncoding RNA NEAT1 promotes laryngeal squamous cell cancer through regulating miR-107/CDK6 pathway. J. Exp. Clin. Cancer Res. 35:22. doi: 10.1186/s13046-016-0297-z
Wang, Y., Wang, C., Chen, C., Wu, F., Shen, P., Zhang, P., et al. (2017). Long non-coding RNA NEAT1 regulates epithelial membrane protein 2 expression to repress nasopharyngeal carcinoma migration and irradiation-resistance through miR-101-3p as a competing endogenous RNA mechanism. Oncotarget 8, 70156–70171. doi: 10.18632/oncotarget.19596
Wang, Y., Xue, D., Li, Y., Pan, X., Zhang, X., Kuang, B., et al. (2016). The long noncoding RNA MALAT-1 is a novel biomarker in various cancers: a meta-analysis based on the GEO database and literature. J. Cancer 7, 991–1001. doi: 10.7150/jca.14663
Wang, Z., Zou, Q., Song, M., and Chen, J. (2017). NEAT1 promotes cell proliferation and invasion in hepatocellular carcinoma by negative regulating miR-613 expression. Biomed. Pharmacother. 94, 612–618. doi: 10.1016/j.biopha.2017.07.111
Worku, T., Bhattarai, D., Ayers, D., Wang, K., Wang, C., Rehman, Z. U., et al. (2017). Long non-coding RNAs: the new horizon of gene regulation in ovarian cancer. Cell. Physiol. Biochem. 44, 948–966. doi: 10.1159/000485395
Wu, Y., and Wang, H. (2018). LncRNA NEAT1 promotes dexamethasone resistance in multiple myeloma by targeting miR-193a/MCL1 pathway. J. Biochem. Mol. Toxicol. 32:e22008. doi: 10.1002/jbt.22008
Wu, Y., Yang, L., Zhao, J., Li, C., Nie, J., Liu, F., et al. (2015). Nuclear-enriched abundant transcript 1 as a diagnostic and prognostic biomarker in colorectal cancer. Mol. Cancer 14, 191. doi: 10.1186/s12943-015-0455-5
Xie, S. S., Jin, J., Xu, X., Zhuo, W., and Zhou, T. H. (2016). Emerging roles of non-coding RNAs in gastric cancer, Pathogenesis and clinical implications. World J. Gastroenterol. 22, 1213–1223. doi: 10.3748/wjg.v22.i3.1213
Yan, X., Hu, Z., Feng, Y., Hu, X., Yuan, J., Zhao, S. D., et al. (2015). Comprehensive genomic characterization of long non-coding RNAs across human cancers. Cancer Cell 28, 529–540. doi: 10.1016/j.ccell.2015.09.006
Yang, X., Xiao, Z., Du, X., Huang, L., and Du, G. (2017). Silencing of the long non-coding RNA NEAT1 suppresses glioma stem-like properties through modulation of the miR-107/CDK6 pathway. Oncol. Rep. 37, 555–562.
Yoon, J. H., Abdelmohsen, K., Srikantan, S., Yang, X., Martindale, J. L., De, S., et al. (2012). LincRNA-p21 suppresses target mRNA translation. Mol. Cell 47, 648–655. doi: 10.1016/j.molcel.2012.06.027
Yoon, J. H., De, S., Srikantan, S., Abdelmohsen, K., Grammatikakis, I., Kim, J., et al. (2014). PAR-CLIP analysis uncovers AUF1 impact on target RNA fate and genome integrity. Nat. Commun. 5:5248. doi: 10.1038/ncomms6248
Yoshimura, H., Matsuda, Y., Yamamoto, M., Kamiya, S., and Ishiwata, T. (2018). Expression and role of long non-coding RNA H19 in carcinogenesis. Front. Biosci (Landmark Ed). 23, 614–625. doi: 10.2741/4608
Yu, T., Tang, B., and Sun, X. (2017). Development of inhibitors targeting hypoxia-inducible factor 1 and 2 for cancer therapy. Yonsei Med. J. 58, 489–496. doi: 10.3349/ymj.2017.58.3.489
Zeng, C., Xu, Y., Xu, L., Yu, X., Cheng, J., Yang, L., et al. (2014). Inhibition of long non-coding RNA NEAT1 impairs myeloid differentiation in acute promyelocytic leukemia cells. BMC Cancer 14:693. doi: 10.1186/1471-2407-14-693
Zhang, C., Li, J. Y., Tian, F. Z., Zhao, G., Hu, H., Ma, Y. F., et al. (2018). Long noncoding RNA NEAT1 promotes growth and metastasis of cholangiocarcinoma cells. Oncol. Res. 26, 879–888. doi: 10.3727/096504017X15024935181289
Zhang, H., Cai, Y., Zheng, L., Zhang, Z., Lin, X., and Jiang, N. (2018). Long noncoding RNA NEAT1 regulate papillary thyroid cancer progression by modulating miR-129-5p/KLK7 expression. J. Cell. Physiol. 233, 6638–6648. doi: 10.1002/jcp.26425
Zhang, M., Wu, W. B., Wang, Z. W., and Wang, X. H. (2017). lncRNA NEAT1 is closely related with progression of breast cancer via promoting proliferation and EMT. Eur. Rev. Med. Pharmacol. Sci. 21, 1020–1026.
Zhao, D., Zhang, Y., Wang, N., and Yu, N. (2017). NEAT1 negatively regulates miR-218 expression and promotes breast cancer progression. Cancer Biomark. 20, 247–254. doi: 10.3233/CBM-170027
Zhao, J., Ohsumi, T. K., Kung, J. T., Ogawa, Y., Grau, D. J., Sarma, K., et al. (2010). Genome-wide identification of polycomb-associated RNAs by RIP-seq. Mol. Cell 40, 939–953. doi: 10.1016/j.molcel.2010.12.011
Zhen, L., Yun-Hui, L., Hong-Yu, D., Jun, M., and Yi-Long, Y. (2016). Long noncoding RNA NEAT1 promotes glioma pathogenesis by regulating miR-449b-5p/c-Met axis. Tumour Biol. 37, 673–683. doi: 10.1007/s13277-015-3843-y
Keywords: NEAT1, nuclear paraspeckle assembly transcript 1, long non-coding RNA, cancer diagnosis, cancer treatment, EMT, microRNA
Citation: Dong P, Xiong Y, Yue J, Hanley SJB, Kobayashi N, Todo Y and Watari H (2018) Long Non-coding RNA NEAT1: A Novel Target for Diagnosis and Therapy in Human Tumors. Front. Genet. 9:471. doi: 10.3389/fgene.2018.00471
Received: 09 August 2018; Accepted: 24 September 2018;
Published: 15 October 2018.
Edited by:
Ge Shan, University of Science and Technology of China, ChinaReviewed by:
Jernej Ule, University College London, United KingdomPeter G. Zaphiropoulos, Karolinska Institutet (KI), Sweden
Copyright © 2018 Dong, Xiong, Yue, Hanley, Kobayashi, Todo and Watari. This is an open-access article distributed under the terms of the Creative Commons Attribution License (CC BY). The use, distribution or reproduction in other forums is permitted, provided the original author(s) and the copyright owner(s) are credited and that the original publication in this journal is cited, in accordance with accepted academic practice. No use, distribution or reproduction is permitted which does not comply with these terms.
*Correspondence: Peixin Dong, dpx1cn@gmail.com
Hidemichi Watari, watarih@med.hokudai.ac.jp
†These authors have contributed equally to this work