- Molecular Developmental Biology, Institute of Molecular Biosciences and Cluster of Excellence Frankfurt Macromolecular Complexes, Department of Biosciences, J. W. Goethe University, Frankfurt, Germany
Quercetin is a flavonoid that is ubiquitously found in vegetables and fruits. Like other flavonoids, it is active in balancing cellular reactive oxygen species (ROS) levels and has a cyto-protective function. Previously, a link between ROS balancing, aging, and the activity of O-methyltransferases was reported in different organisms including the aging model Podospora anserina. Here we describe a role of the S-adenosylmethionine-dependent O-methyltransferase PaMTH1 in quercetin-induced lifespan extension. We found that effects of quercetin treatment depend on the methylation state of the flavonoid. Specifically, we observed that quercetin treatment increases the lifespan of the wild type but not of the PaMth1 deletion mutant. The lifespan increasing effect is not associated with effects of quercetin on mitochondrial respiration or ROS levels but linked to the induction of the PaMth1 gene. Overall, our data demonstrate a novel role of O-methyltransferase in quercetin-induced longevity and identify the underlying pathway as part of a network of longevity assurance pathways with the perspective to intervene into mechanisms of biological aging.
Introduction
One of the oldest dreams of the human species is the dream of a long and healthy life. In search of the “fountain of youth,” diverse strategies for interventions into aging processes have been suggested. Among others, these are regular exercise and different types of diets. For instance, caloric restriction is well known in different species to lead to longevity and better health in old age (Fontana et al., 2010). Another one is a mediterranean type of diet rich in fruits, vegetables, and olive oil (Chrysohoou et al., 2004; Tyrovolas and Panagiotakos, 2010; Bach-Faig et al., 2011).
A main goal of current aging research is to identify specific active components in complex diets and to elucidate the mechanisms by which they lead to healthy aging. As part of this goal, components of nutrients, which are considered to promote healthy life, are investigated. Polyphenols are such substances, which represent one of the largest and ubiquitous groups of secondary metabolites found in fruits and vegetables. With more than 6000 known members, flavonoids are the largest subgroup of polyphenols (Manach et al., 2005). It is known that flavonoids are excellent scavengers of reactive oxygen species (ROS) (Bors et al., 1994). Apart from this function as antioxidants, flavonoids can also act as pro-oxidants at least under some circumstances as in the presence of a high concentrations of free transition metal ions (Canada et al., 1990; Decker, 1997; Croft, 1998; Knab and Osiewacz, 2010) or high doses of flavonoids (Yen et al., 2003; Wätjen et al., 2005). In general, flavonoids affect the abundance of ROS, molecules that, in low abundance, are essential for cell signaling to control healthy development. At high concentrations, due to their reactivity, ROS lead to damage of all kinds of cellular components and to degenerative processes like disease and aging. Such negative effects of ROS have been conceptualized in the “free radical theory of aging” (FRAT) and the “mitochondrial free radical theory of aging” (MFRAT) (Harman, 1956, 1972). These theories paved the road for aging research for decades. For instance, an increased ROS scavenging was found to increase organismic lifespan of Drosophila melanogaster (Agarwal and Sohal, 1994; Sohal et al., 1995), Caenorhabditis elegans (Larsen, 1993), Podospora anserina (Zintel et al., 2011), and to increase chronological lifespan of Saccharomyces cerevisiae (Harris et al., 2003).
A link between the anti-oxidative capacity, aging, and lifespan control was also demonstrated in studies with quercetin, the major representative of flavonoids. In studies with different model systems, a ROS scavenging potential of quercetin was observed (Kampkötter et al., 2007, 2008; Nabavi et al., 2012). For instance, the anti-oxidative capacity of quercetin can be linked to lifespan extension in C. elegans, S. cerevisiae, and D. melanogaster (Belinha et al., 2007; Kampkötter et al., 2008; Saul et al., 2008; Pietsch et al., 2009). Furthermore, Niklas et al. (2012) first reported an increased longevity in human cell cultures by quercetin treatment. Beside the anti-oxidative capacity, studies with cell cultures and rats identified a pro-oxidative effect of quercetin (Laughton et al., 1989; Metodiewa et al., 1999; Robaszkiewicz et al., 2007). In vitro studies demonstrated that the anti-oxidative as well as the pro-oxidative function of quercetin is attributed to the characteristic catechol group containing two vicinal hydroxyl residues (3′- or 4′-hydroxyl residues) (Heijnen et al., 2002). O-Methylation (from now on termed methylation) of these residues can prevent both functions (Cao et al., 1997; Lemanska et al., 2004; Duenas et al., 2010). In humans and rodents, it was shown that this kind of methylation is catalyzed by catechol-O-methyltransferases, a specific group of O-methyltransferases (Zhu et al., 1994, 2010; Chen et al., 2011).
Also in P. anserina, an aging model with a short lifespan that is accessible to genetic and molecular manipulation (Scheckhuber and Osiewacz, 2008; Wiemer et al., 2016) a protein, which accumulates during aging in total protein extracts, has been identified. This protein turned out to be an O-methyltransferase (PaMTH1) with significant homology to the catechol-O-methyltransferases of humans, rodents, and other species (Averbeck et al., 2000). In vitro studies revealed that flavonoids are potential substrates of PaMTH1. An activity assay with PaMTH1 recognized myricetin, quercetin, and other flavonoids with vicinal hydroxyl residues as potential substrates (Kunstmann and Osiewacz, 2008). NMR spectroscopy confirmed that PaMTH1 has the ability to methylate myricetin and that PaMTH1 is active as a dimer (Chatterjee et al., 2015). Furthermore, in vivo studies revealed an age-related PaMTH1 accumulation not only in total cell extracts but also in mitochondria (Averbeck et al., 2000; Groebe et al., 2007; Kunstmann and Osiewacz, 2008).
A connection of oxidative effects and methyltransferase function was demonstrated in P. anserina. Overexpression of PaMth1 was shown to lead to a reduced protein carbonylation suggesting that the methylation of PaMTH1 substrates prevents the incomplete oxidation and thereby the formation of ROS (Kunstmann and Osiewacz, 2008). This protective role of PaMTH1 was confirmed in investigations with a PaMth1 deletion mutant, which showed a decreased oxidative stress resistance (Kunstmann and Osiewacz, 2009). Such a link between O-methyltransferase activity and aging was also obtained in other organisms. For instance, in rats a link between an O-methyltransferase and age-related repair of damaged proteins was demonstrated (Shirasawa et al., 1995). In humans an increase of the abundance and activity of an O-methyltransferase during aging was reported (Tunbridge et al., 2007). It appears that methylation of substrates by O-methyltransferase is part of evolutionary conserved pathways which affect organismal aging. However, the detailed underlying mechanisms are still poorly elucidated.
In a series of recent studies, we started to investigate the impact of natural compounds on P. anserina aging. Among others, we studied the polyphenols gossypol (Warnsmann et al., 2017), curcumin (Warnsmann and Osiewacz, 2016), and here we investigated quercetin. We report novel details about the impact of quercetin on lifespan in P. anserina. We demonstrate that quercetin supplementation leads to an increased abundance of the PaMTH1 O-methyltransferase and that quercetin-induced lifespan extension requires the methylation of this flavonoid by PaMTH1. Furthermore, we reveal effects on respiration and ROS levels attributed to unmethylated quercetin which are not necessary for lifespan control.
Materials and Methods
P. anserina Strains and Cultivation
In this study, the P. anserina wild-type (WT) strain “s” (Rizet, 1953) and the previously generated strains ΔPaMth1 (Kunstmann and Osiewacz, 2009) and PaMth1_OEx (Kunstmann and Osiewacz, 2008) were used. All transgenic strains are in the genetic background of the WT strain “s.” Strains were grown on standard cornmeal agar (BMM) at 27°C under constant light (Osiewacz et al., 2013). For spore germination, standard cornmeal agar (BMM) with 60 mM ammonium acetate was used and incubated at 27°C in the dark for 2 days (d). All strains of this study were derived from monokaryotic ascospores (Osiewacz et al., 2013).
Quercetin and Isorhamnetin Supplementation
After spore germination, all subsequent cultivating steps were performed on M2 medium (Osiewacz et al., 2013) with a supplementation by 300 μM of quercetin (Sigma, Q4951) or 300 μM isorhamnetin (Roth, 6528.1). Additionally, as a control, M2 medium was supplemented with 0.15% dimethylsulfoxide (DMSO; Roth, 4720.1).
Growth Rate and Lifespan Determination
Determination of the lifespan and the growth rate of P. anserina cultures derived from monokaryotic ascospores were performed on M2 medium with or without supplements at 27°C and constant light as previously described (Osiewacz et al., 2013). The lifespan of P. anserina is defined as the time period in days (d) of linear hyphal growth while the growth rate is defined as the measured growth (cm) per time period (d).
Isolation of Mitochondria
P. anserina strains were grown on cellophane foil covered solid M2 medium containing different supplements for 2 d at 27°C and constant light. Mycelial pieces were transferred to CM-liquid medium containing different supplements and grown at 27°C and constant light for additional 2 d. Mitochondria of P. anserina cultures was isolated as previously described by differential centrifugation for measurement of mitochondrial oxygen consumption and by discontinuous sucrose gradient (20–36–50%) ultracentrifugation for blue-native polyacrylamide gels (BN-PAGE) analysis (Osiewacz et al., 2013).
Mitochondrial Oxygen Consumption
Determination of mitochondrial oxygen consumption was performed at 27°C by high-resolution respirometry (Oxygraph-2k series C and G, OROBOROS Instruments, Innsbruck, Austria). 200 μg freshly prepared mitochondria was injected into 2 ml air saturated oxygen buffer (0.3 M sucrose, 10 mM KH2PO4, 5 mM MgCl2, 1 mM EDTA, 10 mM KCl, and 0.1% BSA; pH 7.2). To promote the ADP-limited complex I-dependent state 4 respiration (state 4) 10 mM pyruvate (Sigma-Aldrich, P2256) and 2 mM malate (Sigma-Aldrich, M1000) were added. Subsequently, 1.5 mM ADP (Sigma-Aldrich, A5285) was added to determine complex I-dependent state 3 respiration (state 3). Data were analyzed using the manufacturer’s software DatLab 6.
Blue-Native Polyacrylamide Gels (BN-PAGE)
Blue-native polyacrylamide gels was performed as previously described (Wittig et al., 2006). For sample preparation, 100 μg of mitochondrial protein extracts were solubilized using a digitonin (Sigma-Aldrich, D141) protein ratio of 3:1 (w/w). Linear gradient gels (4–13%) overlaid with 3.5% stacking gels were used for separation of the solubilized mitochondrial protein extracts. Respiratory chain components were visualized by Coomassie blue staining and assigned as described previously (Krause et al., 2004).
Determination of Superoxide Release
Qualitative determination of superoxide release from mycelia was performed by monitoring the reduction of nitroblue tetrazolium (NBT, Sigma-Aldrich, N6876) using a modified protocol of Munkres (1990). Briefly, P. anserina strains were cultivated for 4 d on M2 agar medium with the different supplements in the dark at 27°C. The plates were floated with 5 ml staining solution for superoxide (contains 5 mM MOPS pH 7.6, 2.5 mM NBT) and incubated for 30 min in the dark at 27°C. The solution was decanted, the plates were incubated for additional 3 h in the dark, and at 27°C to obtain the desired staining intensity.
Hydrogen Peroxide Release Measurements
Qualitative determination of hydrogen peroxide release from mycelia was performed by monitoring the oxidation of diaminobenzidine (DAB, Sigma-Aldrich, D-8001) according to a modified protocol of Munkres (1990). P. anserina strains were cultivated for 4 d on M2 agar medium with the different supplements in the dark and 27°C. The plates were floated with 5 ml staining solution for hydrogen peroxide (contains 100 mM Tris/HCl pH 6.9, 2.5 mM DAB; dissolved at 60°C for 10 min) and incubated for 30 min in the dark and 27°C. The solution was decanted, the plates were incubated for additional 3 h in the dark, and 27°C to obtain the desired staining intensity. Quantitative measurement of hydrogen peroxide was performed as previously described (Kowald et al., 2012).
Isolation of Total Protein Extract
Isolation of total protein extract was performed as previously described (Osiewacz et al., 2013). Like for mitochondria isolation, appropriate supplements were added to M2 agar and to CM-liquid media.
“In-Gel” SOD Activity Assay
“In-gel” SOD activity assays were performed as described (Osiewacz et al., 2013).
“In-Gel” Peroxidase and Catalase Activity Assay
“In-gel” peroxidase and catalase activity assays were performed as described in a previously published protocol (Wayne and Diaz, 1986).
Western Blot Analysis
Separation of 100 μg total protein extract by SDS–PAGE and following transfer of proteins to PVDF membranes (Immobilon-FL, Millipore) was performed according to standard protocols (Knuppertz et al., 2014). Blocking and antibody incubation of blotted PVDF membranes were performed in accordance to the Odyssey “Western Blot Analysis” handbook (LI-COR). Primary antibodies were raised against a PaSOD2-specific synthetic peptide ([Ac]-CERFLGTSEATKL[OH]; New England Peptide; 1:2000 dilution) corresponding to AA 225–236 and against a PaMTH1-specific synthetic peptide (PFNEETADRVSAYC-KLH; Sigma; 1:2000 dilution with total protein extract and 1:1000 dilution with mitochondrial protein extract). Additionally, following commercial available antibodies were used: a polyclonal rat MnSOD antibody (Biomal Stressgen, SOD-111, dilution: 1:2000) was used to detect P. anserina mitochondrial superoxide dismutase (SOD) (PaSOD3) and a polyclonal Cu/ZnSOD antibody (Biomol Stressgen, SOD-100; 1:2000 dilution) was used for detection of the cytoplasmic SOD (PaSOD1). Subsequently, secondary antibodies with the infrared dye IR Dye 800 (dilution: 1:15,000, Li-COR Biosciences) or IR Dye 680 LT (dilution: 1:20,000, Li-COR Biosciences) were used. For detection and densitometric quantification, the “Odyssey Infrared Imaging System” (Li-COR Biosciences) was used.
Statistical Analysis
For statistical analysis of lifespans, the IBM SPSS statistics 19 software package was used and Kaplan–Meier survival estimates generated. Significances were determined with pairwise comparison with three independent statistical tests [Breslow (generalized Wilcoxon), Log Rank (Mantel–Cox), and the Tarone–Ware]. In the figure legends only the P-value calculated with the Breslow test is given, all other values are in the supplements (Supplementary Table S1). For all other statistical analysis, the two-tailed Student’s t-test was used. The minimum level of statistical significance was set at P ≤ 0.05. ∗P ≤ 0.05, ∗∗P ≤ 0.01, ∗∗∗P ≤ 0.001.
Results
Quercetin Leads to an Increased Lifespan of P. anserina
During the course of investigations to elucidate the mechanistic effects of natural compounds on organismal aging and lifespan control, we focused our interest on the polyphenol quercetin. We used the fungal aging model P. anserina (Scheckhuber and Osiewacz, 2008) which is a good model system for these kind of studies (Warnsmann and Osiewacz, 2016; Warnsmann et al., 2017). First, we analyzed the effect of increasing quercetin concentrations on the growth rate of the fungus and found a concentration-dependent growth rate decrease. A concentration of 5 mM was found to reduce the growth rate by 50% whereas 300 μM lead to a 10% decrease (Figure 1A). These results demonstrate that exogenous quercetin is taken up by P. anserina filamentous cells (hyphae) and that the effects of the polyphenol are concentration dependent.
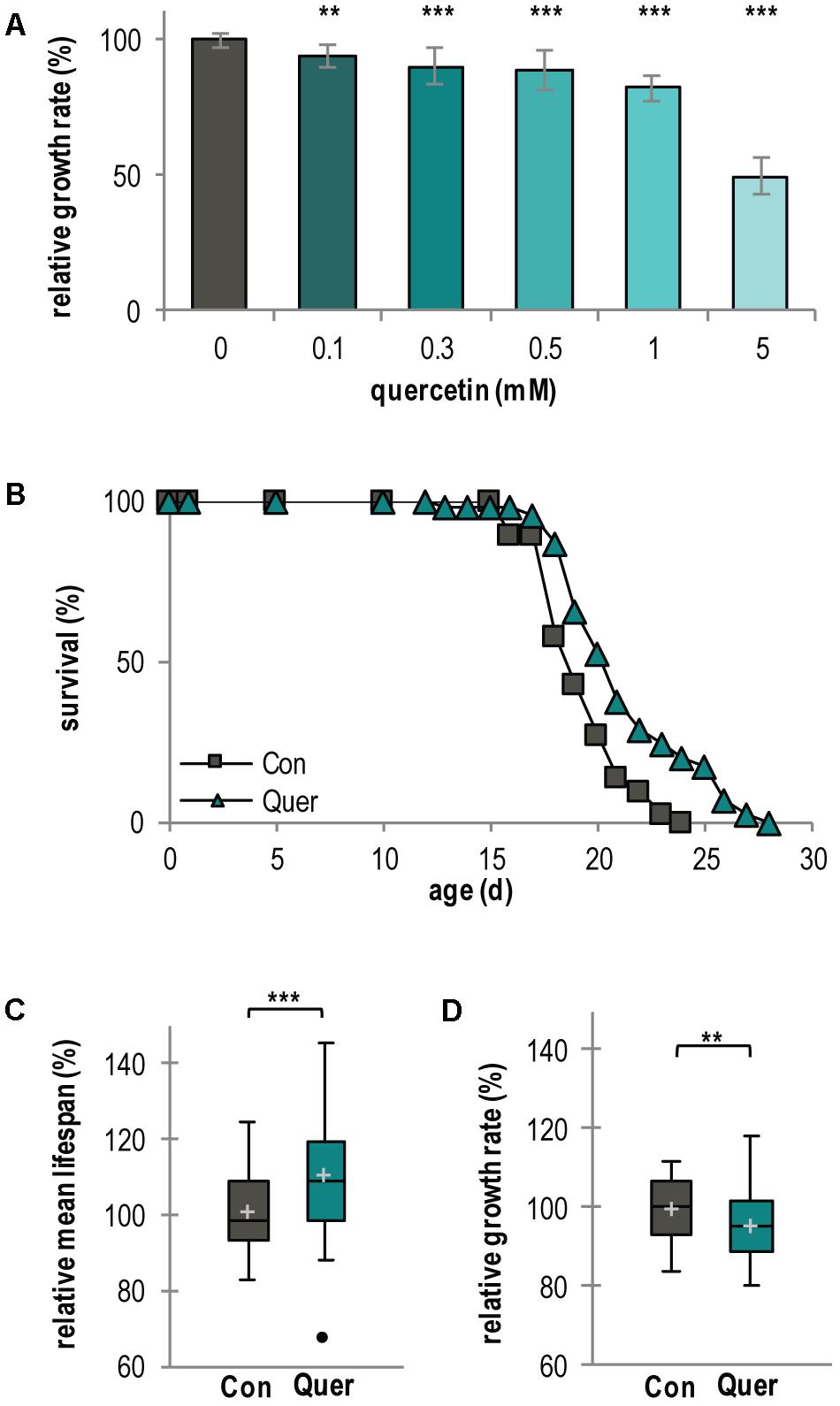
FIGURE 1. Lifespan of P. anserina WT is increased by quercetin treatment. (A) Growth rate of P. anserina WT strains on M2 medium containing increasing concentrations of quercetin as indicated (n = 10 for each concentration). (B) Survival curve of P. anserina WT cultures grown on standard M2 medium containing the solvent DMSO as control (Con; total n = 45) or quercetin (Quer; total n = 46; P = 0.00085). (C) The mean lifespan and (D) the mean growth rates of P. anserina WT cultures treated with quercetin are shown as relative values to the DMSO control. Error bars correspond to the standard deviation. The P-values of the survival curves were determined by SPSS with three different tests and for mean lifespan and growth rate by two-tailed Student’s t-test. ∗∗P < 0.01, ∗∗∗P < 0.001.
Next, we investigated the effect of quercetin on lifespan in some more detail. We choose 300 μM quercetin and as control medium containing 0.15% of the solvent DMSO, a concentration that was also used in all further investigations. Under these conditions, quercetin moderately affects growth while DMSO does neither influences lifespan nor the growth rate (Supplementary Figures S1A–C). Treatment of the P. anserina WT resulted in a mean lifespan increase of 10.2% (21 d vs. 19 d) and a maximal lifespan extension of 16.6% (28 d vs. 24 d) (Figures 1B,C and Supplementary Figure S2A). As expected, the growth rate is only slightly reduced (Figure 1D and Supplementary Figure S2B).
Mitochondrial Respiration and Respiratory Complexes Are Increased by Quercetin
Since a link between lifespan, mitochondrial function, and quality control in P. anserina and other fungi is well documented (Francis et al., 2007; Scheckhuber et al., 2011; Adam et al., 2012; Kurashima et al., 2013; Fischer et al., 2015) and since we found that other polyphenols (i.e., curcumin and gossypol) affect mitochondrial respiration in P. anserina (Warnsmann and Osiewacz, 2016; Warnsmann et al., 2017), we next analyzed the effect of quercetin on mitochondrial function. First, we measured the oxygen consumption rate (OCR) of isolated mitochondria by high-resolution respirometry. The complex I-dependent OCR was determined using mitochondria isolated from WT cultures treated with quercetin and DMSO, respectively. Compared to the DMSO control, quercetin significantly increased both state 4 (ADP-limiting conditions) and state 3 OCR (addition of ADP) (Figure 2A).
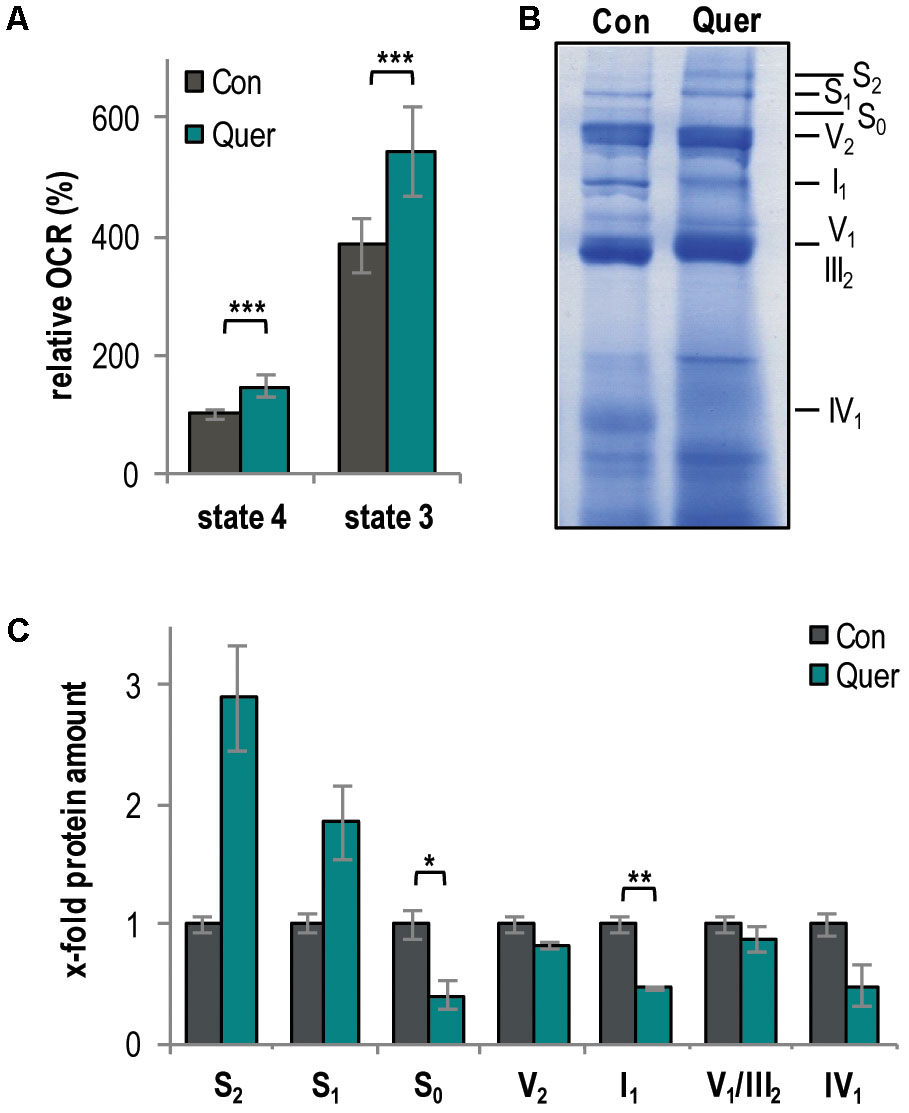
FIGURE 2. Quercetin increases respiration and the abundance of respiratory supercomplexes in the P. anserina WT. (A) Complex I-dependent oxygen consumption rate (OCR) of mitochondria from WT cultures treated with quercetin (Quer, n = 4 biological replicates, each with four to seven technical replicates) compared to mitochondria from cultures treated with DMSO (Con; n = 4 biological replicates, each with three to five technical replicates). State 4 OCR of DMSO-treated mitochondria is set to 100%. (B) Representative BN-PAGE analysis of isolated mitochondria from WT cultures treated with quercetin (Quer) compared to DMSO (Con)-treated cultures (n = 3 biological replicates for each treatment). The CI1CIII2CIV0-2 (S0-2) supercomplexes, dimeric complexes III and V (III2 and V2) as well as monomeric complexes I1, IV1, and V1 were visualized by Coomassie staining. (C) Densitometric quantification of relative protein levels of ETC complexes and supercomplexes in mitochondrial extracts from quercetin-treated cultures compared to DMSO-treated cultures. Optical densities of the different complexes were normalized to the total Coomassie staining of the corresponding lane. The mean abundance in the DMSO control was set to 1. Error bars correspond to standard deviation. The P-values were determined by two-tailed Student’s t-test. ∗P < 0.05, ∗∗P < 0.01, ∗∗∗P < 0.001.
An increased respiration often results from the increased abundance of mitochondrial respiratory supercomplexes (mtRSCs) (Schägger and Pfeiffer, 2000; Bianchi et al., 2004; Genova and Lenaz, 2013). Therefore, we next analyzed the composition of the mitochondrial respiratory chain by BN-PAGE analysis and found changes. In particular, quercetin supplementation increased the abundance of the mtRSCs S1 and S2 and reduced the abundance of the mtRSC S0 and of monomeric complexes I and IV (Figures 2B,C).
Superoxide Anion Release Is Increased by Quercetin
The catechol group of quercetin contains two vicinal hydroxyl residues, which can lead to ROS production (Knab and Osiewacz, 2010). In addition, an altered respiration should also affect ROS production. Therefore, we next analyzed the production and detoxification of ROS, respectively. First, we histochemically measured the release of superoxide anions and hydrogen peroxide (Munkres, 1990) from WT cultures treated with DMSO and quercetin. Changes in the release of the superoxide anion and of hydrogen peroxide by P. anserina cultures are indirect measures of the ROS level in different cellular compartments including the cytoplasm and mitochondria. We observed an increase in superoxide anion (increased precipitate specifically at the growth front) and a decrease in hydrogen peroxide release (slight decrease of precipitate in the whole colony) (Figure 3A). The hydrogen peroxide release was subsequently confirmed by a quantitative photometric analysis. Consistently, we observed a significant decrease of hydrogen peroxide in cultures treated with quercetin compared to DMSO (Figure 3B).
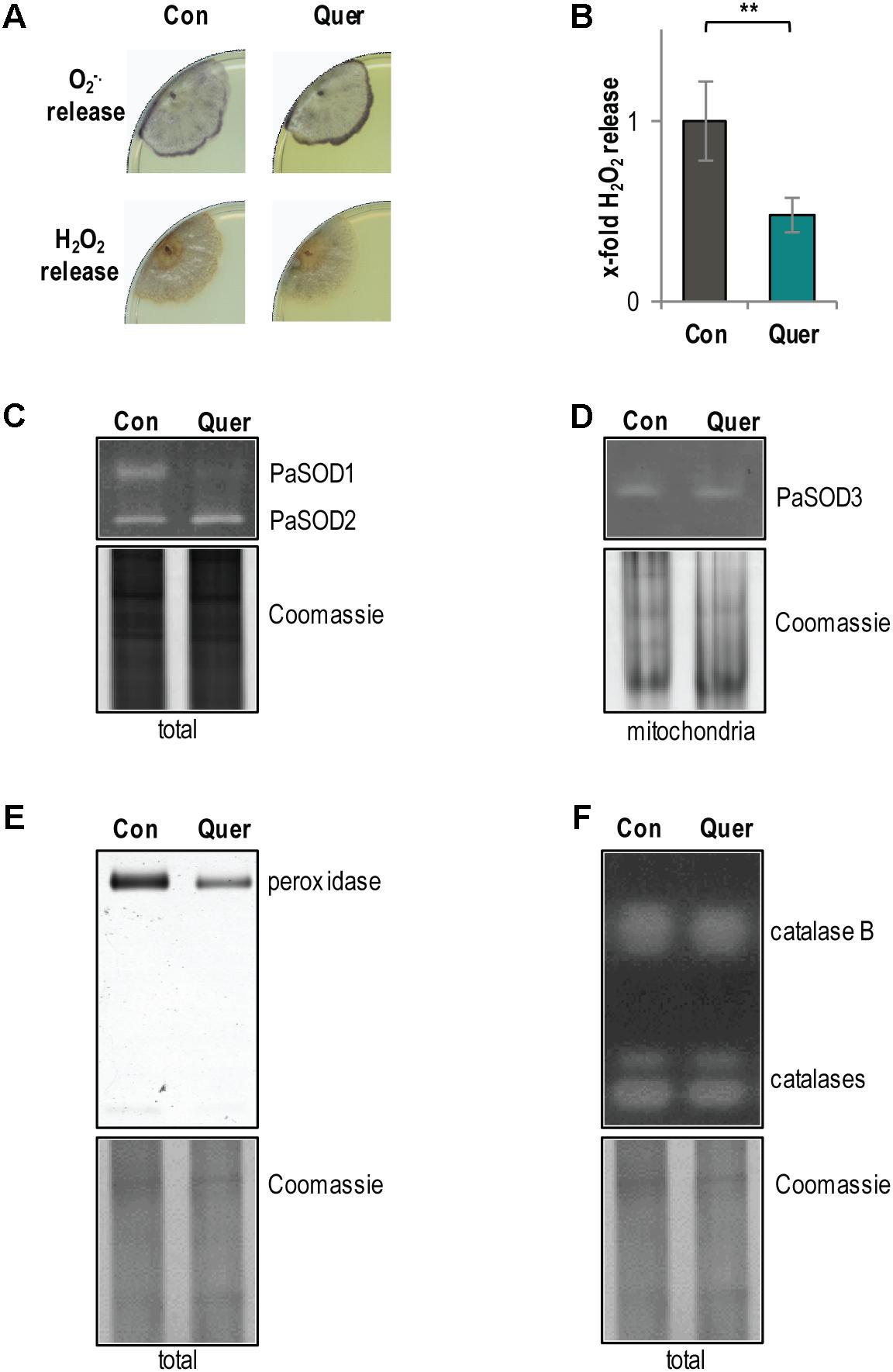
FIGURE 3. ROS metabolism of the P. anserina WT is affected by quercetin. (A) Qualitative determination of superoxide anion release by a histochemical NBT staining and hydrogen peroxide release by a histochemical DAB staining in P. anserina WT cultures (n = 4) treated with quercetin (Quer) or DMSO (Con). (B) Quantitative measurement of H2O2 release from P. anserina WT cultures treated with quercetin (Quer; n = 5) compared with DMSO (Con; n = 5) treatment. The mean release of the DMSO-treated WT was set to 1. Error bars correspond to the standard deviation and P-value was determined by two-tailed Student’s t-test. ∗∗P < 0.01. (C) Representative “in-gel” superoxide dismutase (SOD) (PaSOD1 and PaSOD2) activity staining from total protein extract of P. anserina WT cultures treated with quercetin (Quer; n = 4) or DMSO (Con; n = 4). (D) Representative “in-gel” SOD (PaSOD3) activity staining from mitochondrial protein extract of P. anserina WT cultures treated with quercetin (Quer; n = 3) or DMSO (Con; n = 3). (E) Representative “in-gel” peroxidase and (F) catalase activity staining from total protein extracts of P. anserina WT cultures treated with quercetin (Quer; n = 4) or DMSO (Con; n = 4).
The observed increase in superoxide anion release may be caused by an increased production or a reduced detoxification of superoxide anions. Accordingly, we analyzed the activity of superoxide anion and hydrogen peroxide converting enzymes, respectively. Activity of the three known SOD isoforms of P. anserina was investigated by “in-gel” staining. Compared to the DMSO control, we found a strong decrease in cytosolic PaSOD1 activity in quercetin-treated WT cultures (Figure 3C). The activity of the secreted PaSOD2 (Zintel et al., 2010) (Figure 3C) and of the mitochondrial PaSOD3 (Zintel et al., 2010) (Figure 3D) did not change. Consistent with previous findings showing that PaSOD1, PaSOD2, and PaSOD3 are regulated post-translationally (Borghouts et al., 2002; Wiemer and Osiewacz, 2014), we found no significant differences in the protein amount of all three isoforms upon quercetin treatment (Supplementary Figure S3). In addition, compared to the DMSO control, the activity of the hydrogen peroxide scavenging enzyme peroxidase was reduced after quercetin treatment (Figure 3E), whereas the activity of catalases, another class of hydrogen peroxide scavenging enzymes, was not affected (Figure 3F). Overall, these data indicate that the ROS detoxification system is impaired by quercetin treatment what certainly contributes to an increased superoxide anion abundance in the P. anserina WT.
The PaMTH1 Level in P. anserina Is Increased by Quercetin
Since it is known that methylation of vicinal hydroxyl groups from polyphenols affects the generation of ROS (Cao et al., 1997; Lemanska et al., 2004; Duenas et al., 2010; Knab and Osiewacz, 2010) and since previous “in vitro” studies revealed that quercetin is a potential substrate of the PaMTH1 O-methyltransferase (Kunstmann and Osiewacz, 2008), we next analyzed whether quercetin impacts the abundance of PaMTH1. Indeed, compared to the DMSO control, we found a 1.6-fold increase of the PaMTH1 abundance in total protein extract of the WT upon quercetin treatment (Figures 4A,B). Moreover, previous studies demonstrated an increased abundance and a translocation of PaMTH1 from the cytosol to mitochondria during aging (Averbeck et al., 2000; Groebe et al., 2007; Kunstmann and Osiewacz, 2008). Therefore, we analyzed the abundance of PaMTH1 in mitochondrial protein extracts from quercetin-treated WT cultures. Compared to the DMSO control, we found a 3.2-fold increase in mitochondria of treated cultures (Figures 4C,D). These results indicate that quercetin stimulates the PaMTH1 and its translocation into mitochondria.
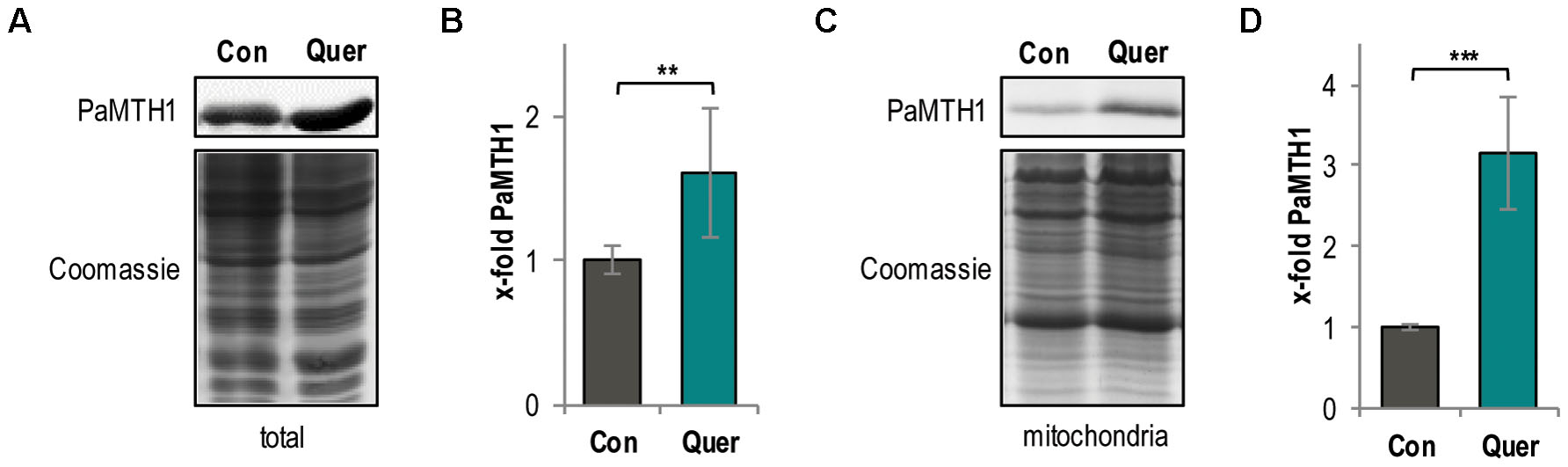
FIGURE 4. Total methyltransferase level is increased by quercetin treatment in the P. anserina WT. (A) Representative western blot analysis of total protein extract of P. anserina WT cultures treated with DMSO (Con; n = 10 biological replicates) and quercetin (Quer; n = 10 biological replicates), respectively. (B) Quantification of PaMTH1 protein level normalized to the Coomassie-stained gel. Protein level in the DMSO control was set to 1. (C) Representative western blot analysis of mitochondrial protein extract of P. anserina WT cultures treated with DMSO (Con; n = 6 biological replicates) or quercetin (Quer; n = 6 biological replicates). (D) Quantification of PaMTH1 protein abundance normalized to the Coomassie-stained gel. Protein level in the DMSO control was set to 1. Error bars correspond to the standard deviation and P-values were determined by two-tailed Student’s t-test. ∗∗P < 0.01, ∗∗∗P < 0.001.
PaMTH1 Is Required for Quercetin-Induced Longevity
The quercetin-induced increase of PaMTH1 abundance suggests a role of PaMTH1 in quercetin-induced longevity. To address this possibility experimentally, we investigated the effect of quercetin in a PaMth1 deletion mutant (ΔPaMth1) and a PaMth1 overexpression strain (PaMth1_OEx). First, we determined the lifespan of both mutants. Strikingly, in comparison to the WT (Figure 1B), the lifespan of ΔPaMth1 is not affected by quercetin treatment (Figure 5A). Most interestingly, the mean lifespan increasing effect of quercetin in the WT (Figure 1C) is not observed in the deletion mutant (Figure 5B, Table 1, and Supplementary Figure S2C). Also, this mutant exhibited no difference in relative growth rate upon quercetin treatment (Figure 5C and Supplementary Figure S2D). In contrast, in PaMth1 overexpressing strains lifespan is increased by quercetin (Figure 5D). Interestingly, quercetin has a significantly stronger effect on mean lifespan of PaMth1_OEx than in the WT. In the overexpression mutant, the relative mean lifespan is increased by 13.4% (23 d vs. 20 d) (Figure 5E, Table 1, and Supplementary Figure S2E), while an increase of only 10.2% is observed in the WT (Figure 1C, Table 1). As in the WT, the growth rate of the PaMth1 overexpression mutant is slightly reduced (Figure 5F and Supplementary Figure S2F). Compared to the WT treated with quercetin we found a stronger increase in the amount of PaMTH1 in the overexpression mutant (Figures 5G,H). Therefore, we assume that in the PaMth1 overexpression mutant quercetin methylation is higher than the WT suggesting that the level of quercetin methylation regulated lifespan extension.
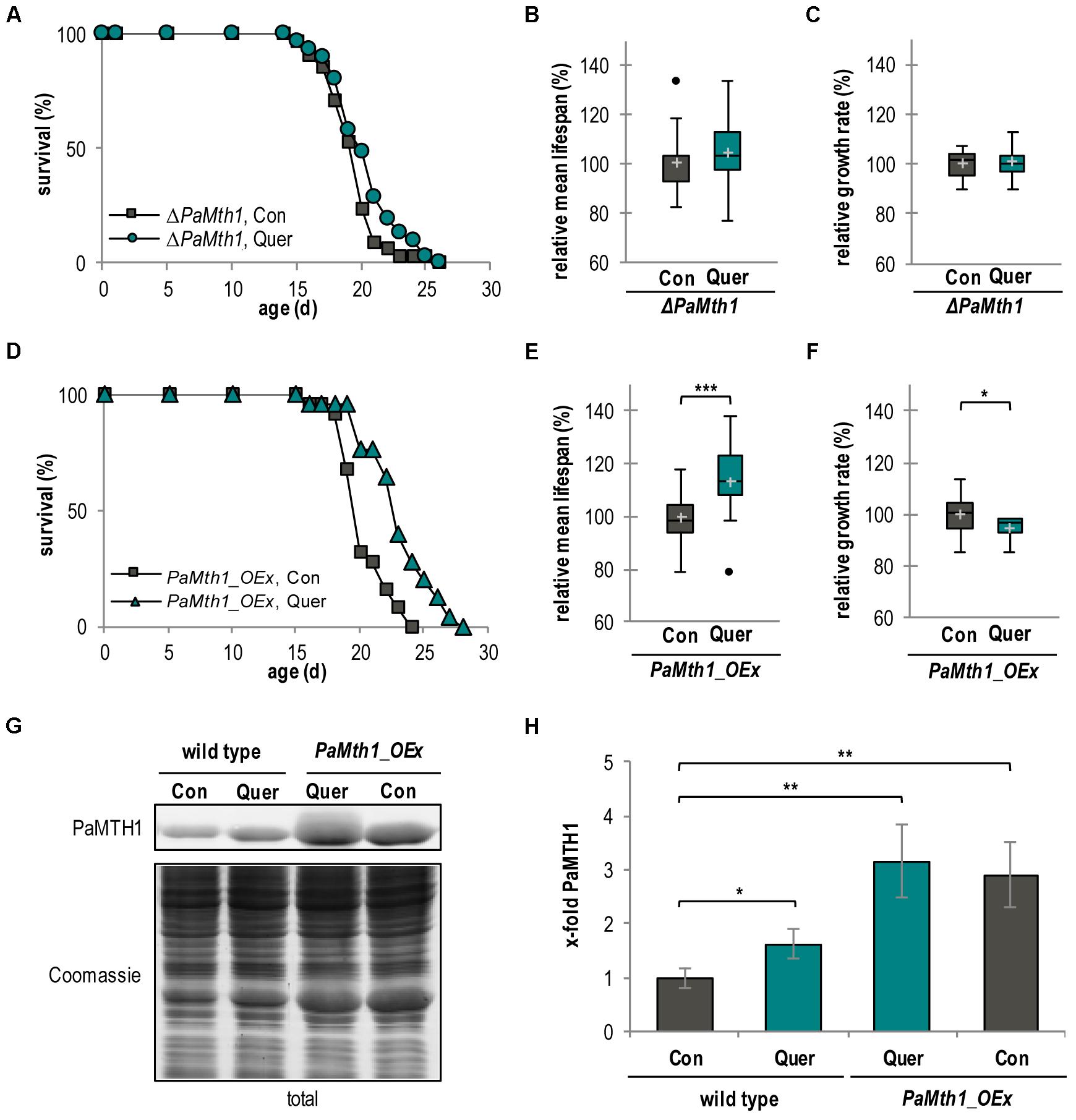
FIGURE 5. Lifespan extension by quercetin is dependent of O-methyltransferase activity. (A) Lifespan of ΔPaMth1 cultures grown on standard M2 medium containing DMSO (Con; n = 34) or quercetin (Quer; n = 31; P = 0.13878). (B) Relative mean lifespan of cultures from (A). Data shown as relative values compared to the DMSO control, which was set to 100%, ±standard derivation. (C) Relative mean growth rate of cultures from (A). Data shown as relative values compared to the DMSO control, which was set to 100%, ±standard derivation. (D) Lifespan of P. anserina Mth1_OEx cultures grown on standard M2 medium containing DMSO (Con; n = 25) or quercetin (Quer; n = 25; P = 0.00012). (E) Relative mean lifespan of cultures from (D). Data are shown as relative values compared to the DMSO control, which was set to 100%, ±standard derivation. (F) Relative mean growth rate of cultures from (D). Data are indicated as relative values compared to the DMSO control, which was set to 100%, ±standard derivation. (G) Representative western blot analysis of total protein extract of P. anserina WT (n = 4) and PaMth1_OEx cultures (OEx; n = 4) treated with DMSO (Con) or quercetin (Quer). (H) Quantification of PaMTH1 protein level normalized to the Coomassie-stained gel. Protein level in WT cultures treated with DMSO was set to 1. Error bars correspond to the standard deviation and P-values were determined by two-tailed Student’s t-test. ∗P < 0.05; ∗∗P < 0.01, ∗∗∗P < 0.001.
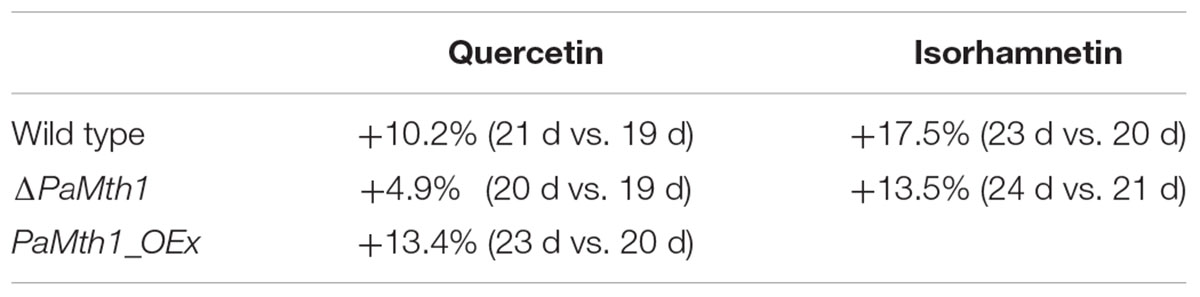
TABLE 1. Overview about the relative change in mean lifespan by quercetin and isorhamnetin in different P. anserina strains.
Overall, our data suggest that PaMTH1 is required for quercetin-induced lifespan extension and provides new mechanistic support of earlier findings about a role of PaMTH1 in lifespan control (Kunstmann and Osiewacz, 2008, 2009; Chatterjee et al., 2015).
PaMTH1 Affects Mitochondrial Respiration and ROS Abundance
To experimentally analyze the impact of quercetin and PaMTH1 on aging and lifespan control in some more detail, we next investigated the effect of the methylation of quercetin on respiration in PaMth1 mutants. Unexpectedly, we found that, in comparison to the WT, quercetin treatment leads to a higher increase of the OCR in both analyzed respiration states in the PaMth1 deletion mutant (Figure 6A and Table 2). In concordance, the OCR of the overexpression mutant is less affected compared to the WT (Figure 6B and Table 2).
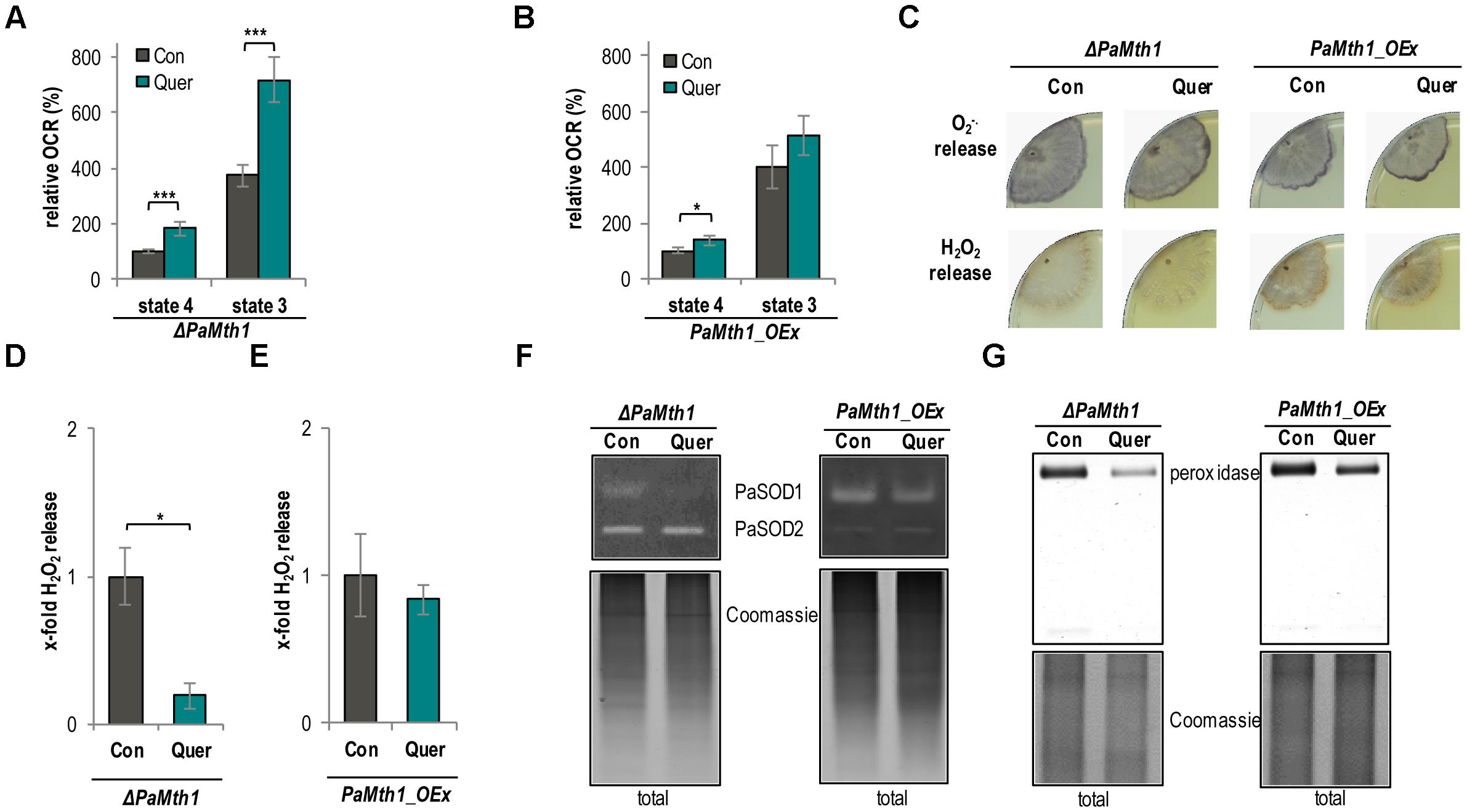
FIGURE 6. Mitochondrial function and ROS metabolism are affected depending on the methylation state of quercetin. (A) Complex I-dependent OCR of mitochondria from ΔPaMth1 cultures treated with quercetin (Quer, n = 3 biological replicates, each with six technical replicates) compared to mitochondria from cultures treated with DMSO (Con; n = 3 biological replicates, each with five technical replicates). State 4 OCR of DMSO-treated mitochondria was set to 100%. (B) Complex I-dependent OCR of mitochondria from PaMth1_OEx cultures treated with quercetin (Quer, n = 3 biological replicates, each with five to six technical replicates) compared to mitochondria from cultures treated with DMSO (Con; n = 3 biological replicates, each with five technical replicates). State 4 OCR of DMSO-treated mitochondria was set to 100%. (C) Qualitative determination of superoxide anion release by a histochemical NBT staining and hydrogen peroxide release by a histochemical DAB staining in ΔPaMth1 and PaMth1_OEx cultures (n = 4) treated with quercetin (Quer) or DMSO (Con). (D) Quantification of H2O2 release measurement from P. anserina ΔPaMth1 cultures treated with quercetin (Quer; n = 4) compared to DMSO (Con; n = 4) treatment. The mean release of the DMSO-treated cultures was set to 1. (E) Quantification of H2O2 release measurement from P. anserina PaMth1_OEx cultures treated with quercetin (Quer; n = 4) compared to DMSO (Con; n = 4) treatment. The mean release of the DMSO-treated cultures was set to 1. (F) Representative “in-gel” SOD (PaSOD1 and PaSOD2) activity staining and (G) “in-gel” peroxidase activity staining from total protein extract of ΔPaMth1 and PaMth1_OEx cultures treated with quercetin (Quer; n = 4) or DMSO (Con; n = 4). Error bars correspond to the standard deviation and P-values were determined by two-tailed Student’s t-test. ∗P < 0.05, ∗∗P < 0.01, ∗∗∗P < 0.001.
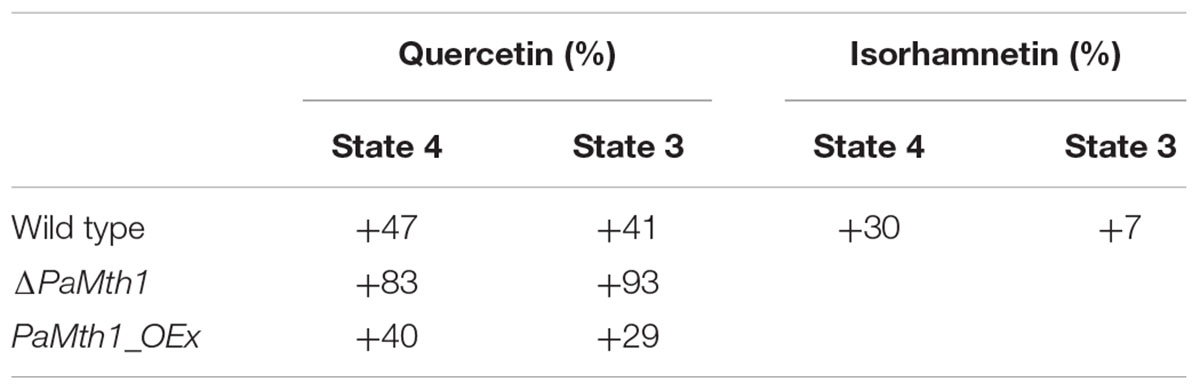
TABLE 2. Overview about the relative changes in the respiration states of P. anserina WT and mutants with the different supplementations compared to the respective DMSO control.
Next, we analyzed whether or not ROS levels are influenced by the methylation of quercetin. We histochemically examined the superoxide anion and hydrogen peroxid release of both PaMth1 mutants. Compared to the WT we found a higher release of superoxide anions in the deletion mutant. In the overexpression mutant the release is hardly affected (Figure 6C). In contrast, hydrogen peroxide is lowered in the PaMth1 deletion strain and rather unaffected in the overexpression strain (Figure 6C). We quantified hydrogen peroxid release and observed a significant lower release in the deletion mutant and no change in the overexpression strain (Figures 6D,E) suggesting a methylation-dependent effect of quercetin on the activity of the superoxide scavenging enzyme PaSOD1. This conclusion is supported by a strongly decreased PaSOD1 activity in ΔPaMth1, and a slightly reduced activity in PaMth1_OEx after quercetin treatment (Figure 6F). Like in the WT, these activity changes do not result from differences in the amount of PaSOD1 but rather from post-translational activation (Supplementary Figure S4). The lowered hydrogen peroxide release seems to affect the activity of the hydrogen peroxide scavenging peroxidase. Quercetin treatment leads to a strongly reduced peroxidase activity in ΔPaMth1 and a slight decrease in PaMth1_OEx (Figure 6G).
Isorhamnetin Increases the Lifespan of P. anserina
To confirm the impact of methylation of quercetin on aging and lifespan control we next investigated the impact of isorhamnetin, a methylated metabolite of quercetin, on the lifespan of P. anserina. Compared to quercetin the amount of PaMTH1 is not affected by isorhamnetin (Figures 7A,B). Similar to quercetin (Figure 1B), isorhamnetin increases the lifespan of the WT (Figure 7C). Compared to the DMSO control, the mean lifespan is significantly increased by 17.5% (23 d vs. 20 d; Figure 7D and Supplementary Figure S5A). Strikingly, this mean lifespan increase is significantly more pronounced by treatment with isorhamnetin (+17.5%) than with quercetin (+10.2%; Table 1) suggesting that lifespan extension is dependent on the methylation state of quercetin. This is confirmed by data obtained from the PaMth1 deletion mutant. Unlike quercetin, for which methylation depends on endogenous PaMTH1, isorhamnetin is independent of this enzyme. Consistently, lifespan increase is observed in the absence of the enzyme (Figure 7E). Compared to the DMSO control, the mean lifespan is increased by 13.5% (24 d vs. 21 d) (Figure 7F, Table 1, and Supplementary Figure S5B).
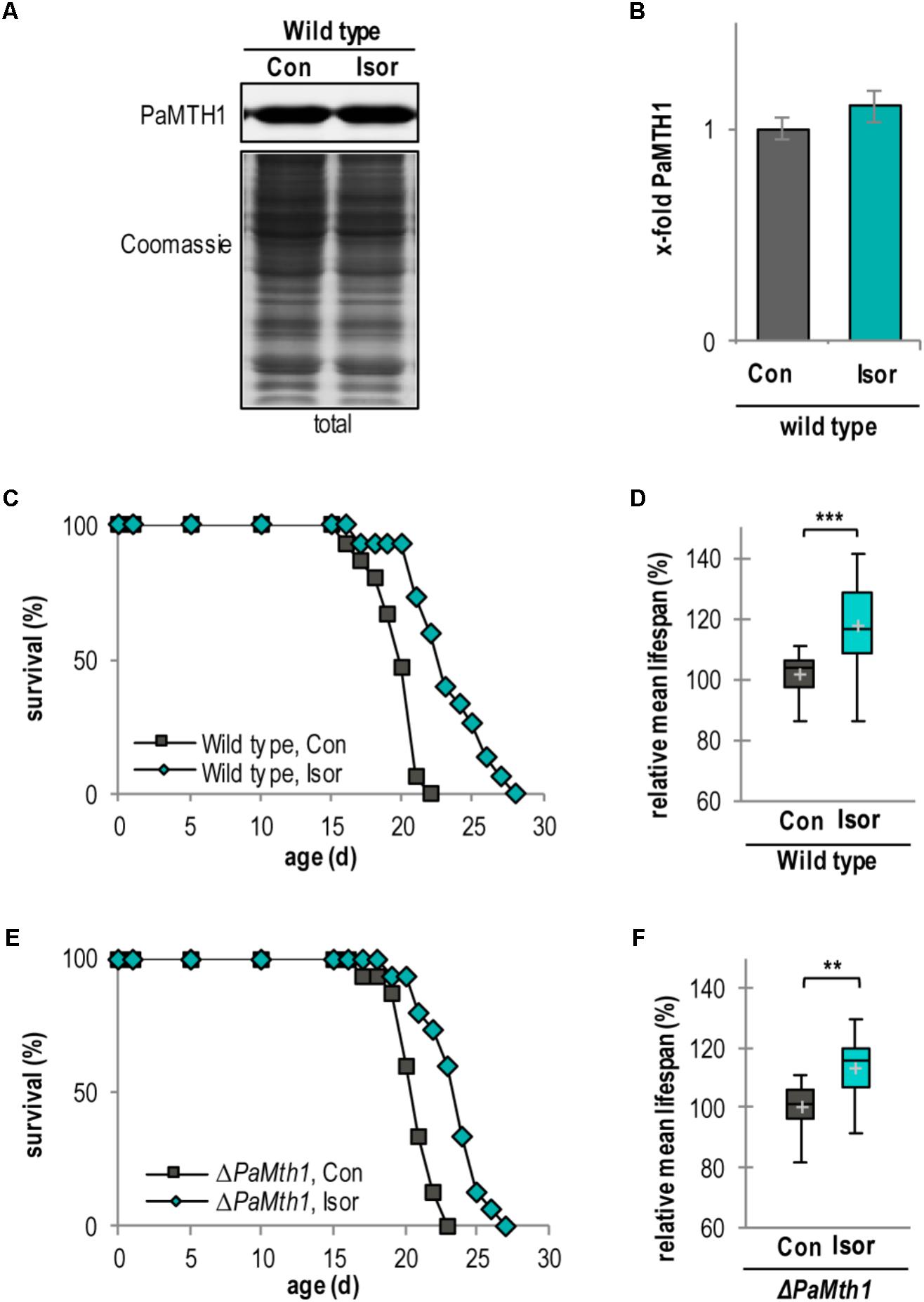
FIGURE 7. Isorhamnetin increases lifespan of the WT and PaMth1 deletion mutant. (A) Representative western blot analysis of total protein extract of P. anserina WT cultures treated with DMSO (Con; n = 4) and isorhamnetin (Isor; n = 4), respectively. (B) Quantification of PaMTH1 protein level normalized to the Coomassie-stained gel. Protein level in the DMSO control was set to 1. Protein level in the DMSO control was set to 1. (C) Lifespan of P. anserina WT cultures grown on standard M2 medium containing DMSO (Con; total n = 15) or isorhamnetin (Isor; total n = 15; P = 0.00016). (D) Lifespan of ΔPaMth1 cultures grown on standard M2 medium containing DMSO (Con; total n = 15) or isorhamnetin (Isor; total n = 15; P = 0.00045). (E) Relative mean lifespan of cultures from (A). Data shown as relative values compared to the DMSO control, which was set to 100%. (F) Relative mean lifespan of cultures from (B). Data shown as relative values compared to the DMSO control, which was set to 100%. Error bars correspond to the standard deviation and P-values of the survival curves were determined by SPSS with three different tests and for mean lifespan by two-tailed Student’s t-test. ∗∗P < 0.01, ∗∗∗P < 0.001.
Next, we analyzed the impact of isorhamnetin on respiration, the respiratory chain, and ROS abundance. Treatment with isorhamnetin did not show any effect on complex I-dependent OCR of WT mitochondria (Figure 8A). This unchanged respiration suggested that the composition of the respiratory chain is unchanged as well. Indeed, isorhamnetin did not change the composition of the respiratory chain as seen in the BN-PAGE (Figure 8B). Quantification of protein bands revealed that the amount of respiratory chain complexes and mtRSCs was unchanged (Figure 8C). Also, the analysis of the ROS metabolism provides no differences in strains treated with isorhamnetin or DMSO. In detail, the release of superoxide anions and hydrogen peroxide (Figures 8D,E) as well as the activity of PaSOD1 (Figure 8F) and of peroxidase is not affected (Figure 8G). These data support the findings from investigations with the PaMth1 mutants that methylated quercetin affects lifespan and not the OCR and the ROS abundance.
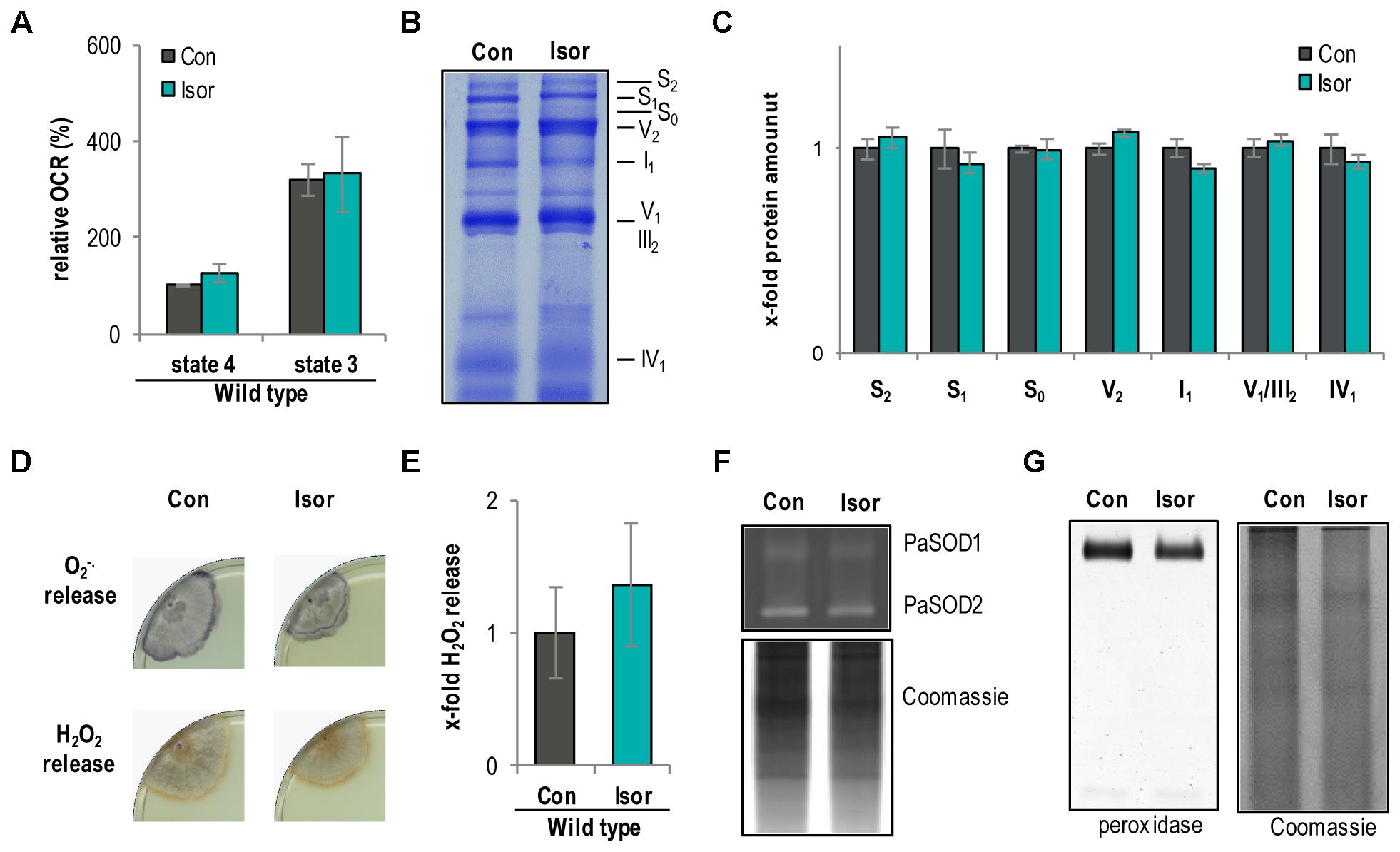
FIGURE 8. Isorhamnetin do not affect respiration and ROS metabolism of the P. anserina WT. (A) Complex I-dependent OCR of mitochondria from WT cultures treated with isorhamnetin (Isor, n = 4 biological replicates, each with three to six technical replicates) compared to mitochondria from DMSO-treated cultures (Con; n = 3 biological replicates, each with five technical replicates). State 4 OCR of DMSO-treated mitochondria was set to 100%. (B) Representative BN-PAGE analysis of isolated mitochondria from WT cultures treated with isorhamnetin (Isor) compared to DMSO (Con) treated. The CI1CIII2CIV0-2 (S0-2) supercomplexes, dimeric complexes III and V (III2 and V2) as well as monomeric complexes I1, IV1, and V1 are visualized by Coomassie staining. (C) Densitometric quantification of relative protein levels of ETC complexes and supercomplexes in mitochondrial extracts from isorhamnetin-treated cultures compared to DMSO-treated cultures. Optical densities of the different complexes were normalized to the total Coomassie staining of the corresponding lane. The mean abundance in the DMSO control was set to 1. (D) Qualitative determination of superoxide anion and hydrogen peroxide release in P. anserina WT cultures (n = 4) treated with isorhamnetin (Isor) or DMSO (Con) by a histochemical staining protocol using NBT and DAB. (E) Quantification of H2O2 release measurement from P. anserina PaMth1_OEx cultures treated with quercetin (Quer; n = 4) compared to DMSO (Con; n = 4) treatment. The mean release of the DMSO-treated cultures was set to 1. (F) Representative “in-gel” SOD (PaSOD1 and PaSOD2) activity staining, (G) representative “in-gel” peroxidase activity staining from total protein extract of P. anserina WT cultures treated with isorhamnetin (Isor) or DMSO (Con).
Discussion
In our effort to unravel mechanistic insights about the role of natural compounds used to intervene into aging processes, we found that supplementation of the growth medium with 300 μM quercetin leads to an increase in mean and maximal lifespan of P. anserina. These data are in line with previously published findings in C. elegans, S. cerevisiae, and D. melanogaster (Belinha et al., 2007; Kampkötter et al., 2008; Saul et al., 2008; Pietsch et al., 2009). Similar to the 10% lifespan extension in P. anserina, Surco-Laos et al. (2011) also found a lifespan extension by 10% in C. elegans after treatment with quercetin. Most strikingly, in P. anserina this effect is linked to an increased abundance of PaMTH1, an enzyme that previously was found to accumulate in total cell extracts and in mitochondria during aging (Averbeck et al., 2000; Groebe et al., 2007; Kunstmann and Osiewacz, 2008). An in-vitro analysis revealed an S-adenosylmethionine-dependent O-methyltransferase activity of PaMTH1 which methylates vicinal hydroxyl groups in polyphenols like myricetin and quercetin (Kunstmann and Osiewacz, 2008; Chatterjee et al., 2015). Overexpression of PaMth1 leads to an increased lifespan while deletion of the gene resulted in a decreased resistance against oxidative stress and a shortened lifespan (Kunstmann and Osiewacz, 2008, 2009).
In the current study we show for the first time that the S-adenosylmethionine-dependent O-methyltransferase PaMTH1 is required for quercetin-induced lifespan extension. Particularly, data from investigations of PaMth1 mutants revealed a methylation-dependent quercetin effect on lifespan. Following supplementation of the growth medium with the methylated quercetin derivative isorhamnetin supports the conclusion that effects of quercetin treatment are depending on the methylation of this flavonoid. While quercetin does not affect lifespan of the PaMth1 deletion mutant, isorhamnetin leads to lifespan extension. In the WT, isorhamnetin has a significantly greater effect than quercetin. However, although not statistically different, there is a marginal difference found in the PaMth1 deletion mutant. We cannot completely exclude that this difference results from the activity of other O-methyltransferases or that unmethylated quercetin also has some minor effect on lifespan. Regardless, the PaMTH1 O-methyltransferase, which in a previous in vitro assay was shown to effectively methylate quercetin (Kunstmann and Osiewacz, 2008; Knab and Osiewacz, 2010), is responsible for the observed lifespan extension of our study. Furthermore, the association of quercetin, PaMTH1, and methylation is supported by data from C. elegans showing that transcript-levels of two different O-methyltransferases are upregulated upon treatment with quercetin or the polyphenol tannic acid (Pietsch et al., 2012). Also, previous studies with C. elegans showed that methylated derivatives of quercetin or epicatechin have a more pronounced effect on lifespan than the unmethylated versions (Surco-Laos et al., 2011, 2012). However, the C. elegans study did not experimentally link the effect of an O-methyltransferase-dependent methylation, the dietary application of quercetin and lifespan.
Apart from the methylation-dependent effects of quercetin, our study shows that not all quercetin effects are caused by methylated derivatives and linked to the lifespan-extending effect of quercetin. In particular, we found that the effect on OCR and the pro-oxidative effects, the decreased PaSOD1 activity and the increased superoxide release, are based on the unmethylated quercetin without a link to lifespan extension. In concordance with the role of O-methylation in the prevention of pro-oxidative effects (Cao et al., 1997; Lemanska et al., 2004; Duenas et al., 2010), we found that isorhamnetin has no pro-oxidative capacity like quercetin. From these data we conclude that unmethylated quercetin leads to a decline of components of the cellular ROS scavenging system (e.g., PaSOD1) as seen in the WT and the PaMth1 deletion mutant.
Despite the increase of superoxide anion release, we obtained an enhanced OCR resulting from an increase in mtRSCs upon quercetin treatment of the WT. Normally, an increase in mtRSCs is known to improve electron flow (Schägger and Pfeiffer, 2000; Bianchi et al., 2003, 2004) and thereby reduces the generation of superoxide anion (Lenaz et al., 2010; Ghelli et al., 2013; Maranzana et al., 2013). Hence, the increase of OCR and mtRSCs (S1 and S2) abundance is a possible kind of compensation mechanism to prevent a superoxide anion generation in addition to the generation through unmethylated hydroxyl residues of quercetin.
Our observation that methylation-dependent effects of quercetin may also apply to higher eukaryotes including humans are supported by different studies in pigs, rodents, and humans. Previous investigations revealed that after oral application of quercetin mostly methylated quercetin metabolites accumulated in blood plasma and in different organs (Zhu et al., 1994; Manach et al., 1998, 2005; Ader et al., 2000; Boyle et al., 2000; Cermak et al., 2003; Hubbard et al., 2003). For instance in humans, after oral application of quercetin, 21% of this flavonoid circulating in the plasma was present as the methylated metabolite isorhamnetin (Day et al., 2001). In rats, this percentage (86%) was even higher (Morand et al., 1998; Manach et al., 1999). In line with our findings and with findings from other organisms, Niklas et al. (2012) exhibited an increased longevity in human cell cultures by quercetin treatment. In contrast to our study, this lifespan increasing effect was not linked to methylated derivative of quercetin. This role of a methyltransferase is now an important issue with perspectives for the use of quercetin in therapies to intervene into human aging. For instance, one approach could be to induce a more efficient cellular methylation capacity of quercetin via the induction of genes coding for specific O-methyltransferases. Another possible strategy is a direct diet with isorhamnetin, a strategy that currently is a very costly treatment. Investigations with model systems like P. anserina may help to unravel more mechanistic details about effective triggers in this field of research and to develop realistic interventions into aging processes.
Author Contributions
HO conceived and supervised this study. VW and SH performed the experiments. VW, SH, and HO analyzed the data. HO and VW wrote the manuscript. All authors read the final version of the manuscript.
Funding
This work was supported by grants of the Deutsche Forschungsgemeinschaft through SFB 1177 and by the LOEWE excellence initiative (project: Integrated Fungal Research) of the state of Hessen (Germany) to HO.
Conflict of Interest Statement
The authors declare that the research was conducted in the absence of any commercial or financial relationships that could be construed as a potential conflict of interest.
Supplementary Material
The Supplementary Material for this article can be found online at: https://www.frontiersin.org/articles/10.3389/fgene.2018.00160/full#supplementary-material
References
Adam, C., Picard, M., Dequard-Chablat, M., Sellem, C. H., Hermann-Le Denmat, S., and Contamine, V. (2012). Biological roles of the Podospora anserina mitochondrial Lon protease and the importance of its N-domain. PLoS One 7:e38138. doi: 10.1371/journal.pone.0038138
Ader, P., Wessmann, A., and Wolffram, S. (2000). Bioavailability and metabolism of the flavonol quercetin in the pig. Free Radic. Biol. Med. 28, 1056–1067. doi: 10.1016/S0891-5849(00)00195-7
Agarwal, S., and Sohal, R. S. (1994). DNA oxidative damage and life expectancy in houseflies. Proc. Natl. Acad. Sci. U.S.A. 91, 12332–12335. doi: 10.1073/pnas.91.25.12332
Averbeck, N. B., Jensen, O. N., Mann, M., Schägger, H., and Osiewacz, H. D. (2000). Identification and characterization of PaMTH1, a putative O-methyltransferase accumulating during senescence of Podospora anserina cultures. Curr. Genet. 37, 200–208. doi: 10.1007/s002940050520
Bach-Faig, A., Berry, E. M., Lairon, D., Reguant, J., Trichopoulou, A., Dernini, S., et al. (2011). Mediterranean diet pyramid today. Science and cultural updates. Public Health Nutr. 14, 2274–2284. doi: 10.1017/S1368980011002515
Belinha, I., Amorim, M. A., Rodrigues, P., de Freitas, V., Moradas-Ferreira, P., Mateus, N., et al. (2007). Quercetin increases oxidative stress resistance and longevity in Saccharomyces cerevisiae. J. Agric. Food Chem. 55, 2446–2451. doi: 10.1021/jf063302e
Bianchi, C., Fato, R., Genova, M. L., Parenti Castelli, G., and Lenaz, G. (2003). Structural and functional organization of Complex I in the mitochondrial respiratory chain. Biofactors 18, 3–9. doi: 10.1002/biof.5520180202
Bianchi, C., Genova, M. L., Parenti Castelli, G., and Lenaz, G. (2004). The mitochondrial respiratory chain is partially organized in a supercomplex assembly: kinetic evidence using flux control analysis. J. Biol. Chem. 279, 36562–36569. doi: 10.1074/jbc.M405135200
Borghouts, C., Scheckhuber, C. Q., Werner, A., and Osiewacz, H. D. (2002). Respiration, copper availability and SOD activity in P. anserina strains with different lifespan. Biogerontology 3, 143–153. doi: 10.1023/A:1015696404723
Bors, W., Michel, C., and Saran, M. (1994). Flavonoid antioxidants: rate constants for reactions with oxygen radicals. Methods Enzymol. 234, 420–429. doi: 10.1016/0076-6879(94)34112-5
Boyle, S. P., Dobson, V. L., Duthie, S. J., Hinselwood, D. C., Kyle, J. A., and Collins, A. R. (2000). Bioavailability and efficiency of rutin as an antioxidant: a human supplementation study. Eur. J. Clin. Nutr. 54, 774–782. doi: 10.1038/sj.ejcn.1601090
Canada, A. T., Giannella, E., Nguyen, T. D., and Mason, R. P. (1990). The production of reactive oxygen species by dietary flavonols. Free Radic. Biol. Med. 9, 441–449. doi: 10.1016/0891-5849(90)90022-B
Cao, G., Sofic, E., and Prior, R. L. (1997). Antioxidant and prooxidant behavior of flavonoids: structure-activity relationships. Free Radic. Biol. Med. 22, 749–760. doi: 10.1016/S0891-5849(96)00351-6
Cermak, R., Landgraf, S., and Wolffram, S. (2003). The bioavailability of quercetin in pigs depends on the glycoside moiety and on dietary factors. J. Nutr. 133, 2802–2807. doi: 10.1093/jn/133.9.2802
Chatterjee, D., Kudlinzki, D., Linhard, V., Saxena, K., Schieborr, U., Gande, S. L., et al. (2015). Structure and biophysical characterization of the S-Adenosylmethionine-dependent O-Methyltransferase PaMTH1, a putative enzyme accumulating during senescence of Podospora anserina. J. Biol. Chem. 290, 16415–16430. doi: 10.1074/jbc.M115.660829
Chen, Z., Chen, M., Pan, H., Sun, S., Li, L., Zeng, S., et al. (2011). Role of catechol-O-methyltransferase in the disposition of luteolin in rats. Drug Metab. Dispos. 39, 667–674. doi: 10.1124/dmd.110.037333
Chrysohoou, C., Panagiotakos, D. B., Pitsavos, C., Das, U. N., and Stefanadis, C. (2004). Adherence to the Mediterranean diet attenuates inflammation and coagulation process in healthy adults: the ATTICA Study. J. Am. Coll. Cardiol. 44, 152–158. doi: 10.1016/j.jacc.2004.03.039
Croft, K. D. (1998). The chemistry and biological effects of flavonoids and phenolic acids. Ann. N. Y. Acad. Sci. 854, 435–442. doi: 10.1111/j.1749-6632.1998.tb09922.x
Day, A. J., Mellon, F., Barron, D., Sarrazin, G., Morgan, M. R., and Williamson, G. (2001). Human metabolism of dietary flavonoids: identification of plasma metabolites of quercetin. Free Radic. Res. 35, 941–952. doi: 10.1080/10715760100301441
Decker, E. A. (1997). Phenolics: prooxidants or antioxidants? Nutr. Rev. 55(11 Pt 1), 396–398. doi: 10.1111/j.1753-4887.1997.tb01580.x
Duenas, M., González-Manzano, S., González-Paramás, A., and Santos-Buelga, C. (2010). Antioxidant evaluation of O-methylated metabolites of catechin, epicatechin and quercetin. J. Pharm. Biomed. Anal. 51, 443–449. doi: 10.1016/j.jpba.2009.04.007
Fischer, F., Filippis, C., and Osiewacz, H. D. (2015). RCF1-dependent respiratory supercomplexes are integral for lifespan-maintenance in a fungal ageing model. Sci. Rep. 5:12697. doi: 10.1038/srep12697
Fontana, L., Partridge, L., and Longo, V. D. (2010). Extending healthy life span–from yeast to humans. Science 328, 321–326. doi: 10.1126/science.1172539
Francis, B. R., White, K. H., and Thorsness, P. E. (2007). Mutations in the Atp1p and Atp3p subunits of yeast ATP synthase differentially affect respiration and fermentation in Saccharomyces cerevisiae. J. Bioenerg. Biomembr. 39, 127–144. doi: 10.1007/s10863-007-9071-4
Genova, M. L., and Lenaz, G. (2013). A critical appraisal of the role of respiratory supercomplexes in mitochondria. Biol. Chem. 394, 631–639. doi: 10.1515/hsz-2012-0317
Ghelli, A., Tropeano, C. V., Calvaruso, M. A., Marchesini, A., Iommarini, L., Porcelli, A. M., et al. (2013). The cytochrome b p.278Y > C mutation causative of a multisystem disorder enhances superoxide production and alters supramolecular interactions of respiratory chain complexes. Hum. Mol. Genet. 22, 2141–2151. doi: 10.1093/hmg/ddt067
Groebe, K., Krause, F., Kunstmann, B., Unterluggauer, H., Reifschneider, N. H., Scheckhuber, C. Q., et al. (2007). Differential proteomic profiling of mitochondria from Podospora anserina, rat and human reveals distinct patterns of age-related oxidative changes. Exp. Gerontol. 42, 887–898. doi: 10.1016/j.exger.2007.07.001
Harman, D. (1956). Aging: a theory based on free radical and radiation chemistry. J. Gerontol. 11, 298–300. doi: 10.1093/geronj/11.3.298
Harman, D. (1972). The biologic clock: the mitochondria? J. Am. Geriatr. Soc. 20, 145–147. doi: 10.1111/j.1532-5415.1972.tb00787.x
Harris, N., Costa, V., MacLean, M., Mollapour, M., Moradas-Ferreira, P., and Piper, P. W. (2003). Mnsod overexpression extends the yeast chronological (G(0)) life span but acts independently of Sir2p histone deacetylase to shorten the replicative life span of dividing cells. Free Radic. Biol. Med. 34, 1599–1606. doi: 10.1016/S0891-5849(03)00210-7
Heijnen, C. G., Haenen, G. R., Oostveen, R. M., Stalpers, E. M., and Bast, A. (2002). Protection of flavonoids against lipid peroxidation: the structure activity relationship revisited. Free Radic. Res. 36, 575–581. doi: 10.1080/10715760290025951
Hubbard, G. P., Wolffram, S., Lovegrove, J. A., and Gibbins, J. M. (2003). The role of polyphenolic compounds in the diet as inhibitors of platelet function. Proc. Nutr. Soc. 62, 469–478. doi: 10.1079/PNS2003253
Kampkötter, A., Nkwonkam, C. G., Zurawski, R. F., Timpel, C., Chovolou, Y., Wätjen, W., et al. (2007). Investigations of protective effects of the flavonoids quercetin and rutin on stress resistance in the model organism Caenorhabditis elegans. Toxicology 234, 113–123. doi: 10.1016/j.tox.2007.02.006
Kampkötter, A., Timpel, C., Zurawski, R. F., Ruhl, S., Chovolou, Y., Proksch, P., et al. (2008). Increase of stress resistance and lifespan of Caenorhabditis elegans by quercetin. Comp. Biochem. Physiol. B Biochem. Mol. Biol. 149, 314–323. doi: 10.1016/j.cbpb.2007.10.004
Knab, B., and Osiewacz, H. D. (2010). Methylation of polyphenols with vicinal hydroxyl groups: a protection pathway increasing organismal lifespan. Cell Cycle 9, 3387–3388. doi: 10.4161/cc.9.17.13016
Knuppertz, L., Hamann, A., Pampaloni, F., Stelzer, E., and Osiewacz, H. D. (2014). Identification of autophagy as a longevity-assurance mechanism in the aging model Podospora anserina. Autophagy 10, 822–834. doi: 10.4161/auto.28148
Kowald, A., Hamann, A., Zintel, S., Ullrich, S., Klipp, E., and Osiewacz, H. D. (2012). A systems biological analysis links ROS metabolism to mitochondrial protein quality control. Mech. Ageing Dev. 133, 331–337. doi: 10.1016/j.mad.2012.03.008
Krause, F., Scheckhuber, C. Q., Werner, A., Rexroth, S., Reifschneider, N. H., Dencher, N. A., et al. (2004). Supramolecular organization of cytochrome c oxidase- and alternative oxidase-dependent respiratory chains in the filamentous fungus Podospora anserina. J. Biol. Chem. 279, 26453–26461. doi: 10.1074/jbc.M402756200
Kunstmann, B., and Osiewacz, H. D. (2008). Over-expression of an S-adenosylmethionine-dependent methyltransferase leads to an extended lifespan of Podospora anserina without impairments in vital functions. Aging Cell 7, 651–662. doi: 10.1111/j.1474-9726.2008.00412.x
Kunstmann, B., and Osiewacz, H. D. (2009). The S-adenosylmethionine dependent O-methyltransferase PaMTH1: a longevity assurance factor protecting Podospora anserina against oxidative stress. Aging 1, 328–334. doi: 10.18632/aging.100029
Kurashima, K., Chae, M., Inoue, H., Hatakeyama, S., and Tanaka, S. (2013). A uvs-5 strain is deficient for a mitofusin gene homologue, fzo1, involved in maintenance of long life span in Neurospora crassa. Eukaryot. Cell 12, 233–243. doi: 10.1128/EC.00226-12
Larsen, P. L. (1993). Aging and resistance to oxidative damage in Caenorhabditis elegans. Proc. Natl. Acad. Sci. U.S.A. 90, 8905–8909. doi: 10.1073/pnas.90.19.8905
Laughton, M. J., Halliwell, B., Evans, P. J., and Hoult, J. R. (1989). Antioxidant and pro-oxidant actions of the plant phenolics quercetin, gossypol and myricetin. Effects on lipid peroxidation, hydroxyl radical generation and bleomycin-dependent damage to DNA. Biochem. Pharmacol. 38, 2859–2865. doi: 10.1016/0006-2952(89)90442-5
Lemanska, K., van der Woude, H., Szymusiak, H., Boersma, M. G., Gliszczynska-Swiglo, A., Rietjens, I. M., et al. (2004). The effect of catechol O-methylation on radical scavenging characteristics of quercetin and luteolin–a mechanistic insight. Free Radic. Res. 38, 639–647. doi: 10.1080/10715760410001694062
Lenaz, G., Baracca, A., Barbero, G., Bergamini, C., Dalmonte, M. E., Del Sole, M., et al. (2010). Mitochondrial respiratory chain super-complex I-III in physiology and pathology. Biochim. Biophys. Acta 1797, 633–640. doi: 10.1016/j.bbabio.2010.01.025
Manach, C., Morand, C., Crespy, V., Demigné, C., Texier, O., Régérat, F., et al. (1998). Quercetin is recovered in human plasma as conjugated derivatives which retain antioxidant properties. FEBS Lett. 426, 331–336. doi: 10.1016/S0014-5793(98)00367-6
Manach, C., Texier, O., Morand, C., Crespy, V., Regerat, F., Demigne, C., et al. (1999). Comparison of the bioavailability of quercetin and catechin in rats. Free Radic. Biol. Med. 27, 1259–1266. doi: 10.1016/S0891-5849(99)00159-8
Manach, C., Williamson, G., Morand, C., Scalbert, A., and Rémésy, C. (2005). Bioavailability and bioefficacy of polyphenols in humans. I. Review of 97 bioavailability studies. Am. J. Clin. Nutr. 81(Suppl. 1), 230S–242S.
Maranzana, E., Barbero, G., Falasca, A. I., Lenaz, G., and Genova, M. L. (2013). Mitochondrial respiratory supercomplex association limits production of reactive oxygen species from complex I. Antioxid. Redox Signal. 19, 1469–1480. doi: 10.1089/ars.2012.4845
Metodiewa, D., Jaiswal, A. K., Cenas, N., Dickancaité, E., and Segura-Aguilar, J. (1999). Quercetin may act as a cytotoxic prooxidant after its metabolic activation to semiquinone and quinoidal product. Free Radic. Biol. Med. 26, 107–116. doi: 10.1016/S0891-5849(98)00167-1
Morand, C., Crespy, V., Manach, C., Besson, C., Demigne, C., and Remesy, C. (1998). Plasma metabolites of quercetin and their antioxidant properties. Am. J. Physiol. 275(1 Pt 2), R212–R219. doi: 10.1152/ajpregu.1998.275.1.R212
Munkres, K. D. (1990). Histochemical detection of superoxide radicals and hydrogen peroxide by Age-1 mutants of Neurospora. Fungal Genet. Newsl. 37, 24–25. doi: 10.4148/1941-4765.1477
Nabavi, S. F., Nabavi, S. M., Mirzaei, M., and Moghaddam, A. H. (2012). Protective effect of quercetin against sodium fluoride induced oxidative stress in rat’s heart. Food Funct. 3, 437–441. doi: 10.1039/c2fo10264a
Niklas, J., Nonnenmacher, Y., Rose, T., Sandig, V., and Heinzle, E. (2012). Quercetin treatment changes fluxes in the primary metabolism and increases culture longevity and recombinant alpha(1)-antitrypsin production in human AGE1.HN cells. Appl. Microbiol. Biotechnol. 94, 57–67. doi: 10.1007/s00253-011-3811-4
Osiewacz, H. D., Hamann, A., and Zintel, S. (2013). Assessing organismal aging in the filamentous fungus Podospora anserina. Methods Mol. Biol. 965, 439–462. doi: 10.1007/978-1-62703-239-1_29
Pietsch, K., Saul, N., Menzel, R., Stürzenbaum, S. R., and Steinberg, C. E. (2009). Quercetin mediated lifespan extension in Caenorhabditis elegans is modulated by age-1, daf-2, sek-1 and unc-43. Biogerontology 10, 565–578. doi: 10.1007/s10522-008-9199-6
Pietsch, K., Saul, N., Swain, S. C., Menzel, R., Steinberg, C. E., and Stürzenbaum, S. R. (2012). Meta-analysis of global transcriptomics suggests that conserved genetic pathways are responsible for quercetin and tannic acid mediated longevity in C. elegans. Front. Genet. 3:48. doi: 10.3389/fgene.2012.00048
Rizet, G. (1953). Impossibility of obtaining uninterrupted and unlimited multiplication of the ascomycete Podospora anserina. C. R. Hebd. Seances Acad. Sci. 237, 838–840.
Robaszkiewicz, A., Balcerczyk, A., and Bartosz, G. (2007). Antioxidative and prooxidative effects of quercetin on A549 cells. Cell Biol. Int. 31, 1245–1250. doi: 10.1016/j.cellbi.2007.04.009
Saul, N., Pietsch, K., Menzel, R., and Steinberg, C. E. (2008). Quercetin-mediated longevity in Caenorhabditis elegans: is DAF-16 involved? Mech. Ageing Dev. 129, 611–613. doi: 10.1016/j.mad.2008.07.001
Schägger, H., and Pfeiffer, K. (2000). Supercomplexes in the respiratory chains of yeast and mammalian mitochondria. EMBO J. 19, 1777–1783. doi: 10.1093/emboj/19.8.1777
Scheckhuber, C. Q., Houthoofd, K., Weil, A. C., Werner, A., De Vreese, A., Vanfleteren, J. R., et al. (2011). Alternative oxidase dependent respiration leads to an increased mitochondrial content in two long-lived mutants of the aging model Podospora anserina. PLoS One 6:e16620. doi: 10.1371/journal.pone.0016620
Scheckhuber, C. Q., and Osiewacz, H. D. (2008). Podospora anserina: a model organism to study mechanisms of healthy ageing. Mol. Genet. Genomics 280, 365–374. doi: 10.1007/s00438-008-0378-6
Shirasawa, T., Endoh, R., Zeng, Y. X., Sakamoto, K., and Mori, H. (1995). Protein L-isoaspartyl methyltransferase: developmentally regulated gene expression and protein localization in the central nervous system of aged rat. Neurosci. Lett. 188, 37–40. doi: 10.1016/0304-3940(95)11389-E
Sohal, R. S., Agarwal, A., Agarwal, S., and Orr, W. C. (1995). Simultaneous overexpression of copper- and zinc-containing superoxide dismutase and catalase retards age-related oxidative damage and increases metabolic potential in Drosophila melanogaster. J. Biol. Chem. 270, 15671–15674. doi: 10.1074/jbc.270.26.15671
Surco-Laos, F., Cabello, J., Gómez-Orte, E., González-Manzano, S., Gonzalez-Paramás, A. M., Santos-Buelga, C., et al. (2011). Effects of O-methylated metabolites of quercetin on oxidative stress, thermotolerance, lifespan and bioavailability on Caenorhabditis elegans. Food Funct. 2, 445–456. doi: 10.1039/c1fo10049a
Surco-Laos, F., Dueñas, M., González-Manzano, S., Cabello, J., Santos-Buelga, C., and González-Paramás, A. M. (2012). Influence of catechins and their methylated metabolites on lifespan and resistance to oxidative and thermal stress of Caenorhabditis elegans and epicatechin uptake. Food Res. Int. 46, 514–521. doi: 10.1016/j.foodres.2011.10.014
Tunbridge, E. M., Weickert, C. S., Kleinman, J. E., Herman, M. M., Chen, J., Kolachana, B. S., et al. (2007). Catechol-o-methyltransferase enzyme activity and protein expression in human prefrontal cortex across the postnatal lifespan. Cereb. Cortex 17, 1206–1212. doi: 10.1093/cercor/bhl032
Tyrovolas, S., and Panagiotakos, D. B. (2010). The role of Mediterranean type of diet on the development of cancer and cardiovascular disease, in the elderly: a systematic review. Maturitas 65, 122–130. doi: 10.1016/j.maturitas.2009.07.003
Warnsmann, V., Meyer, N., Hamann, A., Kögel, D., and Osiewacz, H. D. (2017). A novel role of the mitochondrial permeability transition pore in (-)-gossypol-induced mitochondrial dysfunction. Mech. Ageing Dev. 170, 45–58. doi: 10.1016/j.mad.2017.06.004
Warnsmann, V., and Osiewacz, H. D. (2016). Curcumin treatment affects mitochondrial respiration, induces autophagy and increases lifespan of the fungal aging model Podospora anserina. Endocytobiosis Cell Res. 27, 20–26.
Wätjen, W., Michels, G., Steffan, B., Niering, P., Chovolou, Y., Kampkotter, A., et al. (2005). Low concentrations of flavonoids are protective in rat H4IIE cells whereas high concentrations cause DNA damage and apoptosis. J. Nutr. 135, 525–531. doi: 10.1093/jn/135.3.525
Wayne, L. G., and Diaz, G. A. (1986). A double staining method for differentiating between two classes of mycobacterial catalase in polyacrylamide electrophoresis gels. Anal. Biochem. 157, 89–92. doi: 10.1016/0003-2697(86)90200-9
Wiemer, M., Grimm, C., and Osiewacz, H. D. (2016). “Molecular control of fungal senescence and longevity,” in The Mycota, ed. K. Esser (Heidelberg: Springer VErlag), 155–181. doi: 10.1007/978-3-319-25844-7_8
Wiemer, M., and Osiewacz, H. D. (2014). Effect of paraquat-induced oxidative stress on gene expression and aging of the filamentous ascomycete Podospora anserina. Microb. Cell 1, 225–240. doi: 10.15698/mic2014.07.155
Wittig, I., Braun, H. P., and Schägger, H. (2006). Blue native PAGE. Nat. Protoc. 1, 418–428. doi: 10.1038/nprot.2006.62
Yen, G. C., Duh, P. D., Tsai, H. L., and Huang, S. L. (2003). Pro-oxidative properties of flavonoids in human lymphocytes. Biosci. Biotechnol. Biochem. 67, 1215–1222. doi: 10.1271/bbb.67.1215
Zhu, B. T., Ezell, E. L., and Liehr, J. G. (1994). Catechol-O-methyltransferase-catalyzed rapid O-methylation of mutagenic flavonoids. Metabolic inactivation as a possible reason for their lack of carcinogenicity in vivo. J. Biol. Chem. 269, 292–299.
Zhu, B. T., Wu, K. Y., Wang, P., Cai, M. X., and Conney, A. H. (2010). O-methylation of catechol estrogens by human placental catechol-O-methyltransferase: interindividual differences in sensitivity to heat inactivation and to inhibition by dietary polyphenols. Drug Metab. Dispos. 38, 1892–1899. doi: 10.1124/dmd.110.033548
Zintel, S., Bernhardt, D., Rogowska-Wrzesinska, A., and Osiewacz, H. D. (2011). PaCATB, a secreted catalase protecting Podospora anserina against exogenous oxidative stress. Aging 3, 768–781. doi: 10.18632/aging.100360
Keywords: Podospora anserina, lifespan, quercetin, methyltransferase, isorhamnetin
Citation: Warnsmann V, Hainbuch S and Osiewacz HD (2018) Quercetin-Induced Lifespan Extension in Podospora anserina Requires Methylation of the Flavonoid by the O-Methyltransferase PaMTH1. Front. Genet. 9:160. doi: 10.3389/fgene.2018.00160
Received: 05 January 2018; Accepted: 17 April 2018;
Published: 04 May 2018.
Edited by:
S. Michal Jazwinski, Tulane University, United StatesReviewed by:
Alexander DeLuna, Centro de Investigación y de Estudios Avanzados del Instituto Politécnico Nacional (CINVESTAV), MexicoMark A. McCormick, Buck Institute for Research on Aging, United States
Rasika Vartak, Arizona State University, United States
Copyright © 2018 Warnsmann, Hainbuch and Osiewacz. This is an open-access article distributed under the terms of the Creative Commons Attribution License (CC BY). The use, distribution or reproduction in other forums is permitted, provided the original author(s) and the copyright owner are credited and that the original publication in this journal is cited, in accordance with accepted academic practice. No use, distribution or reproduction is permitted which does not comply with these terms.
*Correspondence: Heinz D. Osiewacz, Osiewacz@bio.uni-frankfurt.de