- 1Laboratório de Genética Médica e Evolução, Departamento de Genética, Universidade Federal do Rio Grande do Sul, Porto Alegre, Brazil
- 2Laboratório de Biologia Molecular, Centro de Ciências da Saúde, Universidade do Vale do Rio dos Sinos, São Leopoldo, Brazil
- 3Laboratório de Ecologia de Mamíferos, Centro de Ciências da Saúde, Universidade do Vale do Rio dos Sinos, São Leopoldo, Brazil
Elasmobranchs, the group of cartilaginous fishes that include sharks and rays, are especially vulnerable to overfishing due to low fecundity and late sexual maturation. A significant number of elasmobranch species are currently overexploited or threatened by fisheries activities. Additionally, several recent reports have indicated that there has been a reduction in regional elasmobranch population sizes. Brazil is an important player in elasmobranch fisheries and one of the largest importers of shark meat. However, carcasses entering the shark meat market have usually had their fins and head removed, which poses a challenge to reliable species identification based on the morphology of captured individuals. This is further complicated by the fact that the internal Brazilian market trades several different elasmobranch species under a common popular name: “cação.” The use of such imprecise nomenclature, even among governmental agencies, is problematic for both controlling the negative effects of shark consumption and informing the consumer about the origins of the product. In this study, we used DNA barcoding (mtDNA, COI gene) to identify, at the species level, “cação” samples available in local markets from Southern Brazil. We collected 63 samples traded as “cação,” which we found to correspond to 20 different species. These included two teleost species: Xiphias gladius (n = 1) and Genidens barbus (n = 6), and 18 species from seven elasmobranch orders (Carcharhiniformes, n = 42; Squaliformes, n = 3; Squatiniformes, n = 2; Rhinopristiformes, n = 4; Myliobatiformes, n = 3; Rajiformes, n = 1; and Torpediniformes, n = 1). The most common species in our sample were Prionace glauca (n = 15) and Sphyrna lewini (n = 14), while all other species were represented by four samples or less. Considering IUCN criteria, 47% of the elasmobranch species found are threatened at the global level, while 53% are threatened and 47% are critically endangered in Brazil. These results underline that labeling the meat of any shark species as “cação” is problematic for monitoring catch allocations from the fishing industry and discourages consumer engagement in conservationist practices through informed decision-making.
Introduction
Elasmobranch (subclass Elasmobranchii) is a group of cartilaginous fishes that include sharks (superorder Selachii) and rays (superorder Batoidea). Even though elasmobranchs comprise less than 1% of the world fisheries catch (Food and Agriculture Organization of United Nations, 2014, 2016), these species have biological characteristics that make them particularly vulnerable to overfishing, such as a low fecundity and late sexual maturation (Bornatowski et al., 2014b). Indeed, several recent reports have indicated that there has been a reduction of elasmobranch populations, resulting in demographic collapse at a regional scale (Baum et al., 2003; Barausse et al., 2014). The overfishing of sharks is especially problematic because these top predators play a key role in marine ecosystems, and, therefore, their population dynamics may affect all local marine diversity (van der Elst, 1979; Heithaus et al., 2008; Gallagher et al., 2012; Pauly et al., 2013; Worm et al., 2013; Bornatowski et al., 2014a). In 1999, FAO (Food and Agriculture Organization) launched an international plan for the conservation and management of sharks and rays, recognizing the high vulnerability of these organisms (Vannuccini, 1999). However, despite this initiative, a significant number of elasmobranch species has remained overexploited or threatened by fisheries activities (Camhi et al., 2009; Cosandey-Godin and Morgan, 2011), which is illustrated by the 42% global increase in the shark meat trade from 2000 to 2011 (Food and Agriculture Organization of United Nations, 2015).
While shark fins are considered to be one of the most valuable products in the ocean (Gallagher and Hammerschlag, 2011), shark meat often attains only 20–60% of the price of tuna and mackerel meat (Bonfil, 1994). As a result, captured individuals usually have their fins removed for the shark fin market, the head is discarded, and the remaining central body part (“cigar”) is then sold for the shark meat market with no special care (Kotas et al., 2008; Ward-Paige et al., 2012). From a taxonomic point of view, the removal of the head and fins represents a challenge to reliable species identification based on morphological features, allowing shark carcasses to be traded fraudulently (Holmes et al., 2009).
Brazil is among the six countries that have the highest capture rate for elasmobranchs (Lack and Sant, 2006), even though a thorough assessment of the impact of industrial fishing is made difficult by inaccurate records (Barreto et al., 2017). Southern Brazil is a region of high elasmobranch diversity (Lucifora et al., 2011), and has a large extractive marine fishing industry, with approximately 160 thousand metric tons of fish caught annually (MPA. Boletim estatístico da pesca e aquicultura, 2011). The two southernmost states, Santa Catarina (SC) and Rio Grande do Sul (RS), are responsible for 98% of the catches (MPA. Boletim estatístico da pesca e aquicultura, 2011). In addition, Brazil is a major player in the meat trade market, acting as the world's largest importer of shark meat in 2011 (Food and Agriculture Organization of United Nations, 2015). Internally, the Brazilian shark meat market trades several different elasmobranch species under the popular name “cação” (or other related popular terms such as “caçonete” and “anjo”), which is used to label several species (Figure S1). For example, Neto (2013) found 21 different species traded under the common name “cação”, including hammerhead sharks (Sphyrna spp.), the blue shark (Prionace glauca), the tiger shark (Galeocerdo cuvier), the bull shark (Carcharhinus leucas), the Galapagos shark (C. galapagensis), and the blacktip shark (C. limbatus). Consumers value “cação” meat for its low cost and for being a “thornless fish” (Bornatowski et al., 2007). However, most consumers are not aware that “cação” is a synonym for sharks (or rays), and others believe that “cação” represents “a specific race of sharks” or even “a race of small sharks” (Bornatowski et al., 2015). Supermarkets, fisheries, and restaurants often omit any other information when selling “cação” meat. Indeed, the use of this term is so widespread that even Brazilian regulatory agencies categorize all elasmobranch species as “cação” without any species-specific information (MPA. Boletim estatístico da pesca e aquicultura, 2011).
The imprecise nomenclature of elasmobranchs makes it difficult to mitigate the negative effects of human shark consumption, as it becomes more difficult to inform the consumer if the product comes from a threatened species or from an illegal species trade. Since shark carcasses are sliced before being sold, it is virtually impossible to obtain accurate species diagnosis based on morphological traits for marketed elasmobranchs (Bornatowski et al., 2015). Therefore, there is an increasing need for fast, reliable, and cheap testing for determining the taxonomic identity of commercialized fishes (Rasmussen and Morrissey, 2008). A precise identification of marketed species also assures that the correct information is presented to the consumer, motivating him or her to take part in honest and regulated trade (Moretti et al., 2003; Martinez et al., 2005).
DNA barcoding uses a small fragment from a DNA sequence located within a standardized region of the genome to allow precise species identification (Hebert et al., 2003). In animals, the standard DNA barcode comes from a stretch of 650 base pairs (bp) from the 5′ end of the mitochondrial gene Cytochrome Oxidase Subunit I (COI or Cox 1) (Meyer and Paulay, 2005; Hajibabaei et al., 2007). This technique has been widely used in a range of studies of species identification (e.g., Meyer and Paulay, 2005; Lowenstein et al., 2010; Carvalho et al., 2011; Rodrigues-Filho et al., 2012; Galimberti et al., 2013). Whilst DNA barcoding is a valuable tool for species identification, especially when the entire organism cannot be accessed for morphology, there are important limitations concerning its accuracy, which depend on the reference database available and on the degree of genetic difference among species (see Frézal and Leblois, 2008 for a review on the pros and cons of DNA barcoding). The aim of this study is to use DNA barcodes to identify, at the species level, samples of “cação” (or similarly labeled) meat available in local markets in Southern Brazil. Finally, we discuss the implications of these findings in the context of elasmobranch conservation in Brazil.
Materials and Methods
Sample Collection
We studied samples sold under general names such as “cação,” “caçonete,” and “filé anjo,” which usually refer to elasmobranch species. Between 2008-2013 and in 2016 we acquired filet samples from local fish markets and supermarkets in different cities from the RS and SC states in Southern Brazil (Figure 1,Table 1). We also included in the analysis samples from Sphyrna lewini (n = 4), Pseudobatos horkelii (n = 2), Rhizoprionodon lalandii (n = 1), Narcine brasiliensis (n = 1), Zapteryx brevirostris (n = 2), and Gymnura altavela (n = 1), collected from fishing vessels and morphologically identified according to Figueiredo (1977), to serve as controls for the DNA barcode identification. These samples are identified as E__ in Table 1. All samples were stored in 95% ethanol at −20°C.
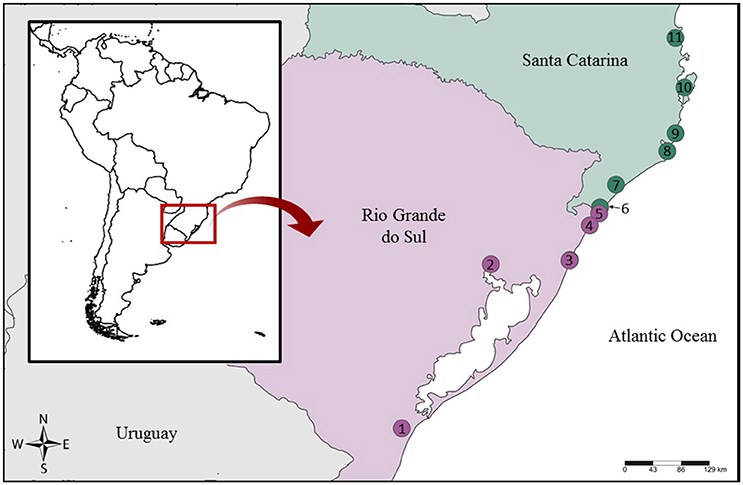
Figure 1. Sampling locations in Southern Brazil. 1, Rio Grande; 2, Porto Alegre; 3, Tramandaí + Imbé; 4, Arroio do Sal; 5, Torres; 6, Passo de Torres; 7, Araranguá; 8, Laguna; 9, Imbituba; 10, Florianópolis; 11, Itajaí.
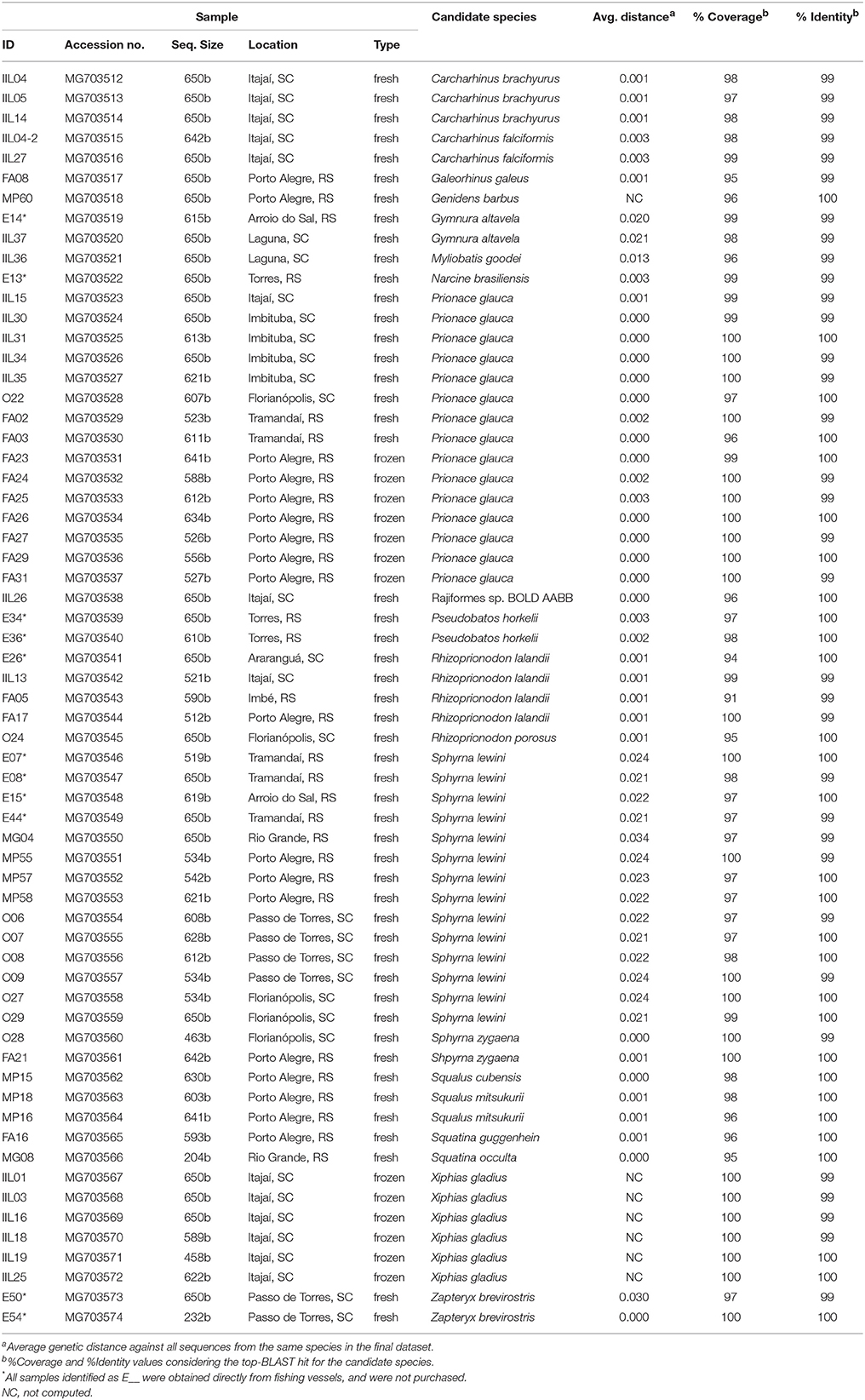
Table 1. Sample information, species identification, average genetic distance, and results from the BLAST search.
Laboratory Procedures
DNA extraction started from a small portion (~100 mg) of the tissue. For most samples we used the Wizard® Genomic DNA Purification Kit (Promega) modified to include an initial digestion step with 200 μg proteinase k (Aljanabi and Martinez, 1997). For the remaining samples, we used a protocol based on the CTAB method (Doyle, 1987). We used the COI primers FishF2 (5′ TCG ACT AAT CAT AAA GAT ATC GGC AC 3′) and FishR2 (5′ ACT TCA GGG TGA CCG AAG AAT CAG AA 3′) (Ward et al., 2005). Amplification reactions were prepared with 0.4 μM of each dNTP, 1.5 mM MgCl2, 0.5 μM of each primer, 1 U Taq Polymerase, and ~40 ng of genomic DNA. Cycling conditions included an initial denaturing step of 94°C for 5′, followed by 10 cycles of 94°C for 1′, 55°C (−0.5°C/cycle) for 1′, and 72°C for 1′30″, and 30 additional cycles of 94°C for 1′, 50°C for 1′, and 72°C for 1′30″, with a final extension step of 72°C for 5′. The amplification products were visualized on a 1% agarose gel stained with GelRed™ (Biotium). PCR products were purified enzymatically using 0.33U SAP (Shrimp Alkaline Phosphatase) and 3.33U ExoI (Exonuclease I). PCR products were sequenced by the Sanger method in Macrogen Inc. (Seoul, South Korea) and Ludwig Biotec (Porto Alegre, Brazil). DNA sequencing was performed on both strands using the primers mentioned above.
Data Analysis
The consensus sequence for each sample was assembled and trimmed in Geneious 9.1 (www.geneious.com). The reliability of each consensus sequence was assessed by a thorough visual inspection of the chromatograms used in the assemblies to check for sequencing errors and artifacts. Low quality regions in the chromatograms, identified as a stretch of five or more contiguous bases having high background noise and uneven spacing, were trimmed and removed before sequence assembly. Because the assembly algorithm gives more weight to better quality reads, cases of sequence heterogeneity between strands are resolved in favor of the best quality read or, if both reads had similar quality for that position, marking it as an ambiguous base (N, R, Y, etc.). The consensus sequence was then used as a query for comparison with the NCBI database (http://www.ncbi.nlm.nih.gov/) using the Basic Local Alignment Search Tool—Nucleotide (BLASTn). In all cases, BLAST matched COI sequences from elasmobranchs (or, in some cases, from teleosts) with good coverage and identity (see Results), suggesting that we generated authentic COI sequences from our samples. We recorded the species representing the top BLAST hit for each query. Following this, we built a dataset of 2,877 COI sequences deposited in the GenBank including all species of all genera represented in the list of top BLAST hits. For example, if the top BLAST hit for a given sample was S. lewini, we included all sequences from all Sphyrna species (including eventual “Sphyrna sp.” entries) in the dataset. We then picked at random 2–8 sequences for each species, which were aligned with the consensus sequences from the samples generated in this study using MAFFT 7.0 (Katoh and Standley, 2013), leading to a final dataset of 323 COI sequences for 147 species (including undescribed or unknown species). As a final quality control step, we checked the dataset for nonsense mutations and alignment gaps, as both could indicate the presence of nuclear mitochondrial translocations (Numts) (Triant and DeWoody, 2007). The final alignment file can be downloaded as Supplementary Material (File S1). The best substitution model (HKY+G+I) for this final dataset was estimated in jModelTest 2 based on the corrected Akaike Information Criterion (AICc) (Darriba et al., 2012). Pairwise genetic distances was estimated in PAUP* 4.0 (Swofford, 2002) based on most likely substitution model and its associated parameters [Lset base = (0.3624 0.2434 0.0914) nst = 2 tratio = 6.1561 rates = gamma shape = 0.8490 ncat = 4 pinvar = 0.4860]. For this final dataset, we inferred the maximum likelihood (ML) tree in RAxML 8.2.10 (Stamatakis, 2014). Node credibility was assessed based on 1,000 bootstrap replicates.
Results
In total, 63 samples were collected, amplified, sequenced, and compared to GenBank sequences (Table 1). High quality sequences ranged between 204 and 650 bases. There was no sequence heterogeneity between strands involving high quality bases from two or more reads. Overall, our analysis suggests the presence of 20 different species among the samples. Seven samples were identified as belonging to two Actinopterigii (ray-finned fishes) species: Xiphias gladius (Perciformes, swordfish; n = 6), and Genidens barbus (Siluriformes, white sea catfish; n = 1). The remaining samples may represent 18 elasmobranch species from three shark orders (Carcharhiniformes, Squaliformes, and Squatiniformes; n = 42, 3, 2, respectively) and four ray orders (Rhinopristiformes, Myliobatiformes, Rajiformes, and Torpediniformes; n = 4, 3, 1, 1, respectively). Three ray species (P. horkelii, Z. brevirostris, and N. brasiliensis) were only found in samples from fishing vessels (i.e., they were not purchased in the market).
Based on COI sequences, all but one elasmobranch samples were identified at the species level, representing 17 formally described species. One sample was associated with an undescribed or unsequenced species (which occurs in GenBank as “Rajiformes sp. BOLD: AABB1882”). The most common species found among market samples were Prionace glauca (blue shark, n = 15) and S. lewini (scalloped hammerhead shark, n = 14). All other species were far less common, including R. lalandii (Brazilian sharpnose shark, n = 4), Carcharhinus brachyurus (copper shark, n = 3), Carcharhinus falciformis (silky shark, n = 2), Sphyrna zygaena (smooth hammerhead shark, n = 2), Squalus mitsukurii (shortspine spurdog, n = 2), Galeorhinus galeus (school shark, n = 1), Rhizoprionodon porosus (Caribbean sharpnose shark, n = 1), Squalus cubensis (Cuban dogfish, n = 1), Squatina occulta (hidden angel shark, n = 1), and Squatina guggenheim (spiny angel shark, n = 1). All ray species identified in the study occurred once or twice among the samples: G. altavela (spiny butterfly ray, n = 2), P. horkelii (Brazilian guitarfish, n = 2), Z. brevirostris (shortnose guitarfish, n = 2), Myliobatis goodei (southern eagle ray, n = 1), and N. brasiliensis (Brazilian electric ray, n = 1).
The average genetic distance between each sample and representatives of its most likely candidate species (determined by its clustering in the ML tree) was always lower than 3.50%, and usually lower than 1% (Table 1). The ML tree showed cohesive clusters of conspecific sequences (Figure 2). The few exceptions, which had bootstrap support values lower than 90, included S. mitsukurii, C. brachyurus, S. guggenheim, and S. occulta (Figure 2). In all cases, however, the estimated genetic distance between our samples and reference sequences were used to indicate the most likely candidate species (shown in Table 1). An interesting case is sample MP16, whose top-hit in BLAST was Squalus montalbani, but clustered with S. mitsukurii in the ML tree (Figure 2). However, both MP16 and MP18 showed a much smaller distance from S. mitsukurii (0.0009) than to any other closely related species (0.0027 vs. S. cf. megalops; 0.0043 vs. S. montalbani; 0.0058 vs. S. chloroculus; and 0.0088 vs. S. cf. mitsukurii). Similarly, IIL04, IIL05, and IIL14 were much closer to C. brachyurus (0.0014) than to C. brevipinna (0.0150), MG08 was closer to S. occulta (0.0000) than to S. guggenheim (0.0071), while FA16 was closer to S. guggenheim (0.0008) than to S. occulta (0.0064). The complete distance matrix can be downloaded as Supplementary Material (File S2).
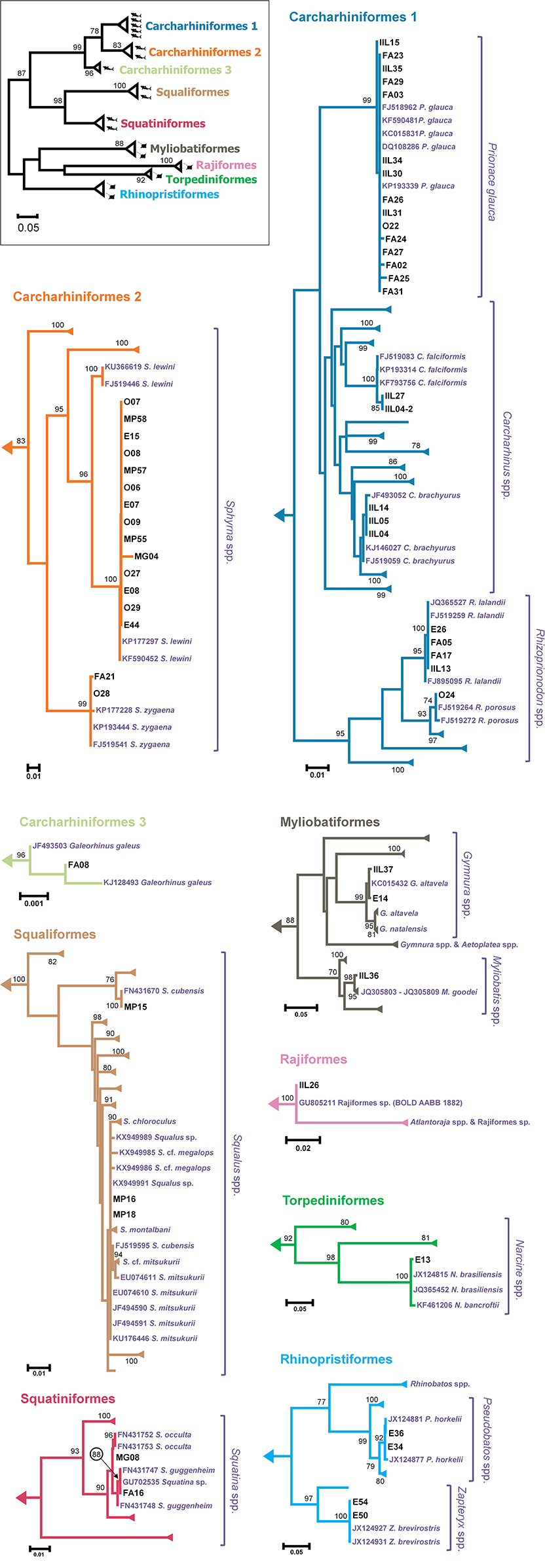
Figure 2. ML tree based on HKY+G+I distance. The miniature on the upper left side shows major groups, displayed in more detail in individual panels. The number of shark and ray symbols represent the number of different species identified in the study for each group. Please note that this is an unrooted tree. Most entries were collapsed and the names were omitted for clarity. Samples from the present study are labeled according to Table 1. The most likely candidate species, together with other closely related species are shown in red. The numbers above the branches represent bootstrap percentage based on 1,000 replicates. Bootstrap values <70 were omitted. Please note the different scale among panels. The full ML tree is available as Supplementary Material (File S3).
Discussion
We found 18 Elasmobranchii and two Actinopterigii species among the samples acquired in Southern Brazilian fish markets as “cação,” “caçonete,” or “filé anjo.” This represents 17% of all elasmobranch species registered for Southern Brazil and 13% of the species described for Brazil (Bornatowski et al., 2009). Other studies, based on other molecular markers, that aimed at species identification of shark filets from Northern Brazil have also shown the great number of species being trade without any taxonomic control (Rodrigues-Filho et al., 2009; Palmeira et al., 2013). Unfortunately, our DNA data does not allow us to conclude that these samples represent individuals captured in Southern Brazil. For example, most individuals included in the final dataset did not have location information. Additionally, even if this was available, it is unclear whether COI would have enough resolution to allow unambiguous recognition of regional stocks for these species (Antoniou and Magoulas, 2014). However, the fact that the vast majority of samples collected in this study were purchased fresh is a strong indication that these specimens may have been captured off Southern Brazil or in nearby areas.
The use of the COI DNA barcode allowed us to identify all samples at the specific level even though some cases deserve further discussion. The best match for IIL26 was an undescribed or unsequenced species, Rajiformes sp. BOLD:AAB1882 (Coverage = 96%, Identity = 100%). The sample MG08 resulted in a short DNA sequence, whose top-result in BLAST was against S. occulta (Coverage = 95%, Identity = 100%), but showed an inconclusive clustering with any Squatina species in the ML tree (Figure 2). Nevertheless, as occurred for other samples (FA16, IIL04, IIL05, IIL14, MP16, MP18), comparing the genetic distance among alternative candidate species allowed the identification of the most likely candidate for each sample. In the case of the samples associated to Squatina, species identification was corroborated by the fact that both S. occulta and S. guggenheim occur off Southern Brazil (Vaz and Vaz and De Carvalho, 2013) and that both samples were acquired as fresh filets, likely indicating a local catch. With a single exception, the species associated with the top-BLAST result also resulted in the lowest average genetic distance. The exception was MG16, whose top-BLAST result was Squalus montalbani (Coverage = 100%, Identity = 99%), but whose lowest average genetic distance was against S. mitsukurii, which also represented the second and third top-BLAST results (Coverage = 96%, Identity = 100%). The low genetic distance among Squalus species and the lack of a clear structure in the ML tree (Figure 2) may indicate that DNA barcoding for this genus may be more complicated than for other genera, and may require other genetic markers. From the taxonomic point of view, it is difficult to discriminate among Squalus species (Haddad and Gadig, 2005), which may be due to a shallow diversification time that is reflected in the low genetic distances among several species. The inherently difficult taxonomy of the genus may favor misnomers in reference databases. In this regard, S. cubensis presents a likely example of database confusion. There are two COI sequences for this species in GenBank. However, while the entry FJ519595 is close to S. mitsukurii (~0.2% genetic distance) the other, FN431670, is distantly related to it (~7.2% genetic distance) and associated with sample MP15 (Figure 2). These issues reinforce the importance of database curation and maintenance, with rigorous taxonomic criteria for the deposition of reference sequences (Ekrem et al., 2007; Teletchea, 2009; Dudgeon et al., 2012). It also highlights that in some cases it may be important to analyze additional genetic markers for a more accurate species identification (Mendonça et al., 2009; Moftah et al., 2011; Pérez-Jiménez et al., 2013).
The most abundant shark species in our samples were Prionace glauca and S. lewini (23.8 and 22.2%, respectively). P. glauca is distributed globally and its capture volume has been estimated at approximately 20 million individuals per year (Mendonça et al., 2012). Despite its endangered status (Table 2), P. glauca is the most fished shark in the world, representing 56% of the total catch of pelagic sharks, especially by industrial fisheries in which the target species are tuna or swordfish (Rose, 1996; Dulvy et al., 2003; Camhi et al., 2009). After the increasing market demand for shark fins and the high prices paid for them, these animals began to be targeted for the removal of these parts, with the carcasses being sold worldwide (Domingues, 2011). Indeed, we identified P. glauca in all samples acquired as frozen filets, which may reflect that these individuals were captured in other parts of the world, such as Taiwan and subsequently imported to Brazil (Figure S1). However, we also found P. glauca among fresh samples, which more likely indicates local capture. On the other hand, S. lewini was the most abundant species among fresh samples, which may indicate a higher local impact on this species. Several authors have raised concerns of predatory fishing for this species off Brazil due to the high commercial value of its fins (Amorim et al., 2011). This results in fishing pressures occurring over all phases and life cycles of these animals, including neonates (Mader et al., 2007) both on the continental shelf and in oceanic waters (Kotas, 2004; Kotas et al., 2005; Vooren and Klippel, 2005).
Regarding their conservation status, IUCN estimates that 47% of the elasmobranch species found in this study are considered threatened at the global level, 53% are threatened at the national level, and 47% are critically endangered at the national level (Table 2). It is difficult, however, to present a more regional picture, given that the red list for both Rio Grande do Sul and Santa Catarina states include only 59 and 23.5% of the species identified in this study, even though there are records for most of these species off these Brazilian states (Gadig, 2001). The conservation status for R. lalandii, S. mitsukurii, S. cubensis, M. goodei, and N. brasiliensis is unknown due to data deficiency (DD). In the worst-case scenario, ~50% of the species identified in this study would be threatened to some extent.
Our sampling was restricted to the south of Brazil due to a limited budget, but it would be important to perform similar studies in other Brazilian regions to provide a better picture of the shark fishing and trade in the country. It should be noted, however, that the Southern coast of Brazil is a hotspot for shark diversity, with high species richness, high endemism, and functional richness (Lucifora et al., 2011). Another future direction would be investigating how much of the shark meat market involves individuals fished locally.
Finally, an important issue in the conservation of these species is how local human populations will engage in more sustainable consumption practices. In this sense, labeling the meat of any shark species as “cação” may impose major barriers to conservation measures for this group, allowing the inadvertent consumption of protected species (Jacquet and Pauly, 2008). Indeed, Bornatowski et al. (2015), who interviewed fish meat consumers in Southern Brazil, reported that 61% of respondents claimed that they have never tried shark meat, even though they ate “cação.” In addition, 69% of respondents said they did not know that at least 25% of all elasmobranchs are threatened. Given these answers, it is evident that a significant portion of the population buying these products is not aware of the impact of their consumption habits, or of the current conservation status of elasmobranch species. Another issue for consumers is mislabeling of shark products, a common outcome of DNA barcode assessments of seafood products (Barbuto et al., 2010; Filonzi et al., 2010). This is illustrated by the presence of the two teleost species detected among our sample (Table 1). Therefore, it becomes essential and an ethical responsibility for the industry to label their products correctly and allow informed decision-making by the consumers. We suggest that all meat being sold as “cação” should be accompanied by the species common name, followed by its scientific name, and, whenever possible, the species threat categories according to the IUCN Red List. While fishing legislation may also have a positive impact on natural populations by suspending the capture and marketing of endangered elasmobranchs, environmental education measures focusing on the fishing community and on consumers will be fundamental for the effective protection of these species.
Author Contributions
FA-S, CS, LO, VV, and NF designed the study; FA-S, CS, CC, PF, and RM executed experimental procedures; FA-S, CS, PF, LG, LO, VV, and NF performed data analysis and interpretation; FA-S, CS, VV, and NF wrote the paper.
Funding
This research was supported by Conselho Nacional de Desenvolvimento Científico e Tecnológico (CNPq), Fundação Grupo Boticário de Proteção à Natureza (Projeto 0921_20112), Programa de Pós-Graduação em Biologia Animal (PPGBAN-UFRGS), and Programa de Pós-Graduação em Genética e Biologia Molecular (PPGBM-UFRGS) and Programa de Pós-Graduação em Biologia (UNISINOS).
Conflict of Interest Statement
The authors declare that the research was conducted in the absence of any commercial or financial relationships that could be construed as a potential conflict of interest.
Acknowledgments
The authors would like to thank Walter de Nisa e Castro Neto (Pró-Squalus), Maria João Veloso da Costa Ramos Pereira (UFRGS), Clarissa Fleck (McGill University), Andrea Thomaz (University of Michigan), Daniel Lyons (University of Michigan), Bonnie Armour (University of Adelaide), and two reviewers for contributions in earlier versions of this manuscript.
Supplementary Material
The Supplementary Material for this article can be found online at: https://www.frontiersin.org/articles/10.3389/fgene.2018.00138/full#supplementary-material
References
Aljanabi, S. M., and Martinez, I. (1997). Universal and rapid salt-extraction of high quality genomic DNA for PCR-based techniques. Nucleic Acids Res. 25, 4692–4693. doi: 10.1093/nar/25.22.4692
Amorim, A. F., Della-Fina, N., and Piva-Silva, N. (2011). Hammerheads sharks, Sphyrna lewini and S. zygaena caught by longliners off Southern Brasil 2007- 2008. Collect. Vol. Sci. Pap. ICCAT 66, 2121–2133.
Antoniou, A., and Magoulas, A. (2014). “Application of mitochondrial DNA in stock identification,” in Stock Identification Methods: Applications in Fishery Science, eds S. X. Cadrin, L. A. Kerr, and S. Mariani (Waltham, MA: Academic Press). 257–295.
Barausse, A., Correale, V., Curkovic, A., Finotto, L., Riginella, E., Visentin, E., et al. (2014). The role of fisheries and the environment in driving the decline of elasmobranchs in the northern Adriatic Sea. ICES J. Mar. Sci. 71, 1593–1603. doi: 10.1093/icesjms/fst222
Barbuto, M., Galimberti, A., Ferri, E., Labra, M., Malandra, R., Galli, P., et al. (2010). DNA barcoding reveals fraudulent substitutions in shark seafood products: the Italian case of “palombo” (Mustelus spp.). Food Res. Int. 43, 376–381. doi: 10.1016/j.foodres.2009.10.009
Barreto, R. R., Bornatowski, H., Motta, F. S., Santander-Neto, J., Vianna, G. M. S., and Lessa, R. (2017). Rethinking use and trade of pelagic sharks from Brazil. Mar. Policy 85, 114–122. doi: 10.1016/j.marpol.2017.08.016
Baum, J. K., Myers, R. A., Kehler, D. G., Worm, B., Harley, S. J., and Doherty, P. A. (2003). Collapse and conservation of shark populations in the Northwest Atlantic. Science 299, 389–392. doi: 10.1126/science.1079777
Bornatowski, H., Abilhoa, V., and Charvet-Almeida, P. (2009). Elasmobranchs of the Paraná Coast, southern Brazil, south-western Atlantic. Mar. Biodivers. Rec. 2:e158. doi: 10.1017/S1755267209990868
Bornatowski, H., Braga, R. R., Kalinowski, C., and Vitule, J. R. S. (2015). “Buying a pig in a poke”: the problem of elasmobranch meat consumption in Southern Brazil. Ethnobiol. Lett. 6, 196–202. doi: 10.14237/ebl.6.1.2015.451
Bornatowski, H., Braga, R. R., and Vitule, J. R. S. (2014a). Threats to sharks in a developing country: the need for effective simple conservation measures. Nat. Conserv. 12, 11–18. doi: 10.4322/natcon.2014.003
Bornatowski, H., Costa, L., Robert, M. C., and Pina, J. V. (2007). Hábitos alimentares de tubarões-martelo jovens, Sphyrna zygaena (Carcharhiniformes: Sphyrnidae), no litoral sul do Brasil. Biota Neotrop. 7, 213–216. doi: 10.1590/S1676-06032007000100025
Bornatowski, H., Navia, A. F., Braga, R. R., Abilhoa, V., and Corrêa, M. F. M. (2014b). Ecological importance of sharks and rays in a structural foodweb analysis in southern Brazil. ICES J. Mar. Sci. 71, 1586–1592. doi: 10.1093/icesjms/fsu025
Camhi, M. D., Valenti, S. V., Fordham, S. V., Fowler, S. L., and Gibson, C. (2009). The Conservation Status of Pelagic Sharks and Rays: Report of the IUCN Shark Specialist Group Pelagic Shark Red List Workshop. Newbury: IUCN Species Survival Commission Shark Specialist Group, 78.
Carvalho, D. C., Neto, D. A., Brasil, B. S., and Oliveira, D. A. (2011). DNA barcoding unveils a high rate of mislabeling in a commercial freshwater catfish from Brazil. Mitochondrial DNA 22, 97–105. doi: 10.3109/19401736.2011.588219
Cosandey-Godin, A., and Morgan, A. (2011). Fisheries Bycatch of Sharks: Options for Mitigation. Washington, DC: Ocean Science Division; Pew Environment Group.
Darriba, D., Taboada, G. L., Doallo, R., and Posada, D. (2012). jModelTest 2: more models, new heuristics and parallel computing. Nat. Methods 9, 772. doi: 10.1038/nmeth.2109
Domingues, R. R. (2011). Identificação Molecular, Biologia e Pesca de Tubarões do Gênero Carcharhinus (Chondrichthyes – Carcharhiniformes): Uma Contribuição Para a Gestão de Pesca do Estado de São Paulo, Brasil. Master's thesis, Instituto de Pesca, São Paulo.
Doyle, J. J. (1987). A rapid DNA isolation procedure for small quantities of fresh leaf tissue. Phytochem. Bull. 19, 11–15.
Dudgeon, C. L., Blower, D. C., Broderick, D., Giles, J. L., Holmes, B. J., Kashiwagi, T., et al. (2012). A review of the application of molecular genetics for fisheries management and conservation of sharks and rays. J. Fish Biol. 80, 1789–1843. doi: 10.1111/j.1095-8649.2012.03265.x
Dulvy, N. K., Sadovy, Y., and Reynolds, J. D. (2003). Extinction vulnerability in marine populations. Fish Fish. 4, 25–64. doi: 10.1046/j.1467-2979.2003.00105.x
Ekrem, T., Willassen, E., and Stur, E. (2007). A comprehensive DNA sequence library is essential for identification with DNA barcodes. Mol. Phylogenet. Evol. 43, 530–542. doi: 10.1016/j.ympev.2006.11.021
Food and Agriculture Organization of United Nations (FAO) (2014). The State of World Fisheries and Aquaculture 2014. Rome: FAO.
Food and Agriculture Organization of United Nations (FAO) (2015). State of the Global Market for Shark Products. Rome: FAO.
Food and Agriculture Organization of United Nations (FAO) (2016). The State of World Fisheries and Aquaculture 2016. Contributing to Food Security and Nutrition for All. Rome: FAO.
Figueiredo, J. L. (1977). Manual de Peixes Marinhos do Sudeste do Brasil–I. Introdução Cações, Raias e Quimeras. São Paulo: Museu de Zoologia; Universidade de São Paulo.
Filonzi, L., Chiesa, S., Vaghi, M., and Marzano, F. N. (2010). Molecular barcoding reveals mislabelling of commercial fish products in Italy. Food Res. Int. 43, 1383–1388. doi: 10.1016/j.foodres.2010.04.016
Frézal, L., and Leblois, R. (2008). Four years of DNA barcoding: current advances and prospects. Infect. Genet. Evol. 8, 727–736. doi: 10.1016/j.meegid.2008.05.005
Gadig, O. B. F. (2001). Tubarões da Costa Brasileira. Ph.D. thesis, Instituto de Biociências, Rio Claro.
Galimberti, A., De Mattia, F., Losa, A., Bruni, I., Federici, S., Casiraghi, M., et al. (2013). DNA barcoding as a new tool for food traceability. Food Res. Int. 50, 55–63. doi: 10.1016/j.foodres.2012.09.036
Gallagher, A. J., and Hammerschlag, N. (2011). Global shark currency: the distribution, frequency, and economic value of shark ecotourism. Curr. Issues Tour. 14, 797–812. doi: 10.1080/13683500.2011.585227
Gallagher, A. J., Kyne, P. M., and Hammerschlag, N. (2012). Ecological risk assessment and its application to elasmobranch conservation and management. J. Fish Biol. 80, 1727–1748. doi: 10.1111/j.1095-8649.2012.03235.x
Haddad, V. Jr., and Gadig, O. B. F. (2005). The spiny dogfish (‘cação-bagre’): description of an envenoming in a fisherman, with taxonomic and toxinologic comments on the Squalus gender. Toxicon 46, 108–110. doi: 10.1016/j.toxicon.2005.03.002
Hajibabaei, M., Singer, G. A., Hebert, P. D., and Hickey, D. A. (2007). DNA barcoding: how it complements taxonomy, molecular phylogenetics and population genetics. Trends Genet. 23, 167–172. doi: 10.1016/j.tig.2007.02.001
Hebert, P. D., Cywinska, A., and Ball, S. L. (2003). Biological identifications through DNA barcodes. Proc. R. Soc. Lond. B Biol. Sci. 270, 313–321. doi: 10.1098/rspb.2002.2218
Heithaus, M. R., Frid, A., Wirsing, A. J., and Worm, B. (2008). Predicting ecological consequences of marine top predator declines. Trends Ecol. Evol. 23, 202–210. doi: 10.1016/j.tree.2008.01.003
Holmes, B. H., Steinke, D., and Ward, R. D. (2009). Identification of shark and ray fins using DNA barcoding. Fish. Res. 95, 280–288. doi: 10.1016/j.fishres.2008.09.036
Instituto Chico Mendes de Preservação da Biodiversidade (2016). Brazil Red Book of Threatened Species of Fauna. Available online at: http://www.icmbio.gov.br/portal/images/stories/comunicacao/publicacoes/publicacoes-diversas/dcom_sumario_executivo_livro_vermelho_da_fauna_brasileira_ameacada_de_extincao_2016.pdf (Accessed April 11, 2018).
IUCN (2017). The IUCN Red List of Threatened Species. Available online at: http://www.iucnredlist.org/ (Accessed October 01, 2016).
Jacquet, J. L., and Pauly, D. (2008). Trade secrets: renaming and mislabeling of seafood. Mar. Policy 32, 309–318. doi: 10.1016/j.marpol.2007.06.007
Katoh, K., and Standley, D. M. (2013). MAFFT multiple sequence alignment software version 7: improvements in performance and usability. Mol. Biol. Evol. 30, 772–780. doi: 10.1093/molbev/mst010
Kotas, J. E. (2004). Dinâmica de Populações e pesca do Tubarão-martelo Sphyrna lewini (Griffith & Smith, 1834), Capturado no mar Territorial e zona Econômica Exclusiva do Sudeste-sul do Brasil. Doctoral thesis, Universidade de São Paulo, São Paulo.
Kotas, J. E., Petrere, M. Jr., Azevedo, V. D., and Santos, S. D. (2005). A Pesca de Emalhe e de Espinhel-de-Superfície na Região Sudeste-Sul do Brasil. Instituto Oceanográfico-USP.
Kotas, J. E., Petrere Junior, M., Fiedler, F., Mastrochirico, V., and Sales, G. (2008). A pesca de emalhe-de-superficie de Santa Catarina direcionada à captura dos tubarões-martelo, Sphyrna lewini (Griffith & Smith 1834) e Sphyrna zygaena (Linnaeus 1758). Atlântica 30, 113–128. doi: 10.5088/atlântica.v30i2.1511
Lack, M., and Sant, G. (2006). World Shark Catch, Production and Trade 1990-2003. Department of the Environment and Heritage.
Lowenstein, J. H., Burger, J., Jeitner, C. W., Amato, G., Kolokotronis, S. O., and Gochfeld, M. (2010). DNA barcodes reveal species-specific mercury levels in tuna sushi that pose a health risk to consumers. Biol. Lett. 6, 692–695. doi: 10.1098/rsbl.2010.0156
Lucifora, L. O., García, V. B., and Worm, B. (2011). Global diversity hotspots and conservation priorities for sharks. PLoS ONE 6:e19356. doi: 10.1371/journal.pone.0019356
Mader, A., Sander, M., Casa, G. E. Jr., Altenhofen, R. J., and dos Anjos, C. S. (2007). Evidências de sobrepesca do tubarão martelo (Sphyrna spp.) no Rio Grande do Sul, Brasil. Biodivers. Pampeana 5.
Martinez, I., James, D., and Loréal, H. (2005). Application of Modern Analytical Techniques to Ensure Seafood Safety and Authenticity. FAO Fisheries Technical Paper. Food and Agriculture Organization of United Nations, Rome.
Mendonça, F. F., Hashimoto, D. T., Porto-Foresti, F., Oliveira, C., Gadig, O. B. F., and Foresti, F. (2009). Identification of the shark species Rhizoprionodon lalandii and R. porosus (Elasmobranchii, Carcharhinidae) by multiplex PCR and PCR-RFLP techniques. Mol. Ecol. Resour. 9, 771–773. doi: 10.1111/j.1755-0998.2009.02524.x
Mendonça, F. F., Ussami, L. H., Hashimoto, D. T., Pereira, L. H., Porto-Foresti, F., Oliveira, C., et al. (2012). Identification and characterization of polymorphic microsatellite loci in the blue shark Prionace glauca, and cross-amplification in other shark species. J. Fish Biol. 80, 2643–2646. doi: 10.1111/j.1095-8649.2012.03291.x
Meyer, C. P., and Paulay, G. (2005). DNA barcoding: error rates based on comprehensive sampling. PLoS Biol. 3:e422. doi: 10.1371/journal.pbio.0030422
Moftah, M., Aziz, S. H., Elramah, S., and Favereaux, A. (2011). Classification of sharks in the Egyptian Mediterranean waters using morphological and DNA barcoding approaches. PLoS ONE 6:e27001. doi: 10.1371/journal.pone.0027001
Moretti, V. M., Turchini, G. M., Bellagamba, F., and Caprino, F. (2003). Traceability issues in fishery and aquaculture products. Vet. Res. Commun. 27, 497–505. doi: 10.1023/B:VERC.0000014207.01900.5c
MPA. Boletim estatístico da pesca e aquicultura (2011). Relatório do Ministério da Pesca e Aquicultura, 98. Available online at: http://www.icmbio.gov.br/cepsul/images/stories/biblioteca/download/estatistica/est_2011_bol__bra.pdf
Neto, D. A. P. (2013). Detecção de Adulteração de Espécies em Pescado e Derivados por Meio da Técnica de DNA Barcoding, Master's thesis, Universidade Federal de Minas Gerais, Belo Horizonte.
Palmeira, C. A. M., Rodrigues-Filho, L. F., Sales, J. P. L., Vallinoto, M., Schneider, H., and Sampaio, I. (2013). Commercialization of a critically endangered species (largetooth sawfish, Pristis perotteti) in fish markets of northern Brazil: authenticity by DNA analysis. Food Control 34, 249–252. doi: 10.1016/j.foodcont.2013.04.017
Pauly, D., Hilborn, R., and Branch, T. A. (2013). Fisheries: does catch reflect abundance? Nature 494, 303–306. doi: 10.1038/494303a
Pérez-Jiménez, J. C., Rocha-Olivares, A., and Sosa-Nishizaki, O. (2013). Morphological and molecular differentiation of smooth-hound sharks (Genus Mustelus, Family Triakidae) from the Gulf of California. J. Appl. Ichthyol. 29, 268–270. doi: 10.1111/jai.12042
Portaria (2014). MMA N°443, de 17 de Dezembro de 2014. Available online at: http://www.icmbio.gov.,br/cepsul/images/stories/legislacao/Portaria/2014/p_m ma_443_2014_lista_esp%C3%A9cies_amea%C3%A7adas_extin%C3%A7%C3 %A3o.pdf (Accessed September 03, 16)
Rasmussen, R. S., and Morrissey, M. T. (2008). DNA-based methods for the identification of commercial fish and seafood species. Compr. Rev. Food Sci. Food Saf. 7, 280–295. doi: 10.1111/j.1541-4337.2008.00046.x
Rodrigues-Filho, L. F., Pinhal, D., Sodré, D., and Vallinoto, M. (2012). “Shark DNA forensics: Applications and impacts on genetic diversity,” in Analysis of Genetic Variation in Animals, ed M. Caliskan (Rijeka: InTech), 269–286.
Rodrigues-Filho, L. F., Rocha, T. C., Rêgo, P. S., Schneider, H., Sampaio, I., and Vallinoto, M. (2009). Identification and phylogenetic inferences on stocks of sharks affected by the fishing industry off the Northern coast of Brazil. Genet. Mol. Biol. 32, 405–413. doi: 10.1590/S1415-47572009005000039
Rose, D. A. (1996). An Overview of World Trade in Sharks and Other Cartilaginous Fishes. Traffic International.
Stamatakis, A. (2014). RAxML version 8: a tool for phylogenetic analysis and post-analysis of large phylogenies. Bioinformatics 30, 1312–1313. doi: 10.1093/bioinformatics/btu033
Swofford, D. L. (2002). PAUP*. Phylogenetic Analysis Using Parsimony (*and Other Methods). Version 4. Sunderland, MA: Sinauer Associates.
Teletchea, F. (2009). Molecular identification methods of fish species: reassessment and possible applications. Rev. Fish Biol. Fish. 19:265. doi: 10.1007/s11160-009-9107-4
Triant, D. A., and DeWoody, J. A. (2007). The occurrence, detection, and avoidance of mitochondrial DNA translocations in mammalian systematics and phylogeography. J. Mammal. 88, 908–920. doi: 10.1644/06-MAMM-A-204R1.1
van der Elst, R. P. (1979). A proliferation of small sharks in the shore-based Natal sport fishery. Environ. Biol. Fish. 4, 349–362. doi: 10.1007/BF00005524
Vannuccini, S. (1999). Shark utilization, Marketing and Trade. No. 389. Food & Agriculture Org. Rome: FAO.
Vaz, D. F., and De Carvalho, M. R. (2013). Morphological and taxonomic revision of species of Squatina from the Southwestern Atlantic Ocean (Chondrichthyes: Squatiniformes: Squatinidae). Zootaxa 3695, 1–81. doi: 10.11646/zootaxa.3695.1.1
Vooren, C. M., and Klippel, S. (2005). Ações Para a Conservação de Tubarões e Raias no Sul do Brasil. Porto Alegre: Igaré.
Ward-Paige, C. A., Keith, D. M., Worm, B., and Lotze, H. K. (2012). Recovery potential and conservation options for elasmobranchs. J. Fish Biol. 80, 1844–1869. doi: 10.1111/j.1095-8649.2012.03246.x
Ward, R. D., Zemlak, T. S., Innes, B. H., Last, P. R., and Hebert, P. D. (2005). DNA barcoding Australia's fish species. Philos. Trans. R. Soc. Lond. B. Biol. Sci. 360, 1847–1857. doi: 10.1098/rstb.2005.1716
Keywords: cação, elasmobranch, cytochrome oxidase-1, shark fisheries, wildlife DNA forensics
Citation: Almerón-Souza F, Sperb C, Castilho CL, Figueiredo PICC, Gonçalves LT, Machado R, Oliveira LR, Valiati VH and Fagundes NJR (2018) Molecular Identification of Shark Meat From Local Markets in Southern Brazil Based on DNA Barcoding: Evidence for Mislabeling and Trade of Endangered Species. Front. Genet. 9:138. doi: 10.3389/fgene.2018.00138
Received: 01 November 2017; Accepted: 03 April 2018;
Published: 27 April 2018.
Edited by:
Rodrigo A. Torres, Universidade Federal de Pernambuco, BrazilReviewed by:
Henrik R. Nilsson, University of Gothenburg, SwedenReinaldo A. De Brito, Universidade Federal de São Carlos, Brazil
Copyright © 2018 Almerón-Souza, Sperb, Castilho, Figueiredo, Gonçalves, Machado, Oliveira, Valiati and Fagundes. This is an open-access article distributed under the terms of the Creative Commons Attribution License (CC BY). The use, distribution or reproduction in other forums is permitted, provided the original author(s) and the copyright owner are credited and that the original publication in this journal is cited, in accordance with accepted academic practice. No use, distribution or reproduction is permitted which does not comply with these terms.
*Correspondence: Victor H. Valiati, dmFsaWF0aUB1bmlzaW5vcy5icg==
Nelson J. R. Fagundes, bmVsc29uLmZhZ3VuZGVzQHVmcmdzLmJy
†These authors have contributed equally to this work.