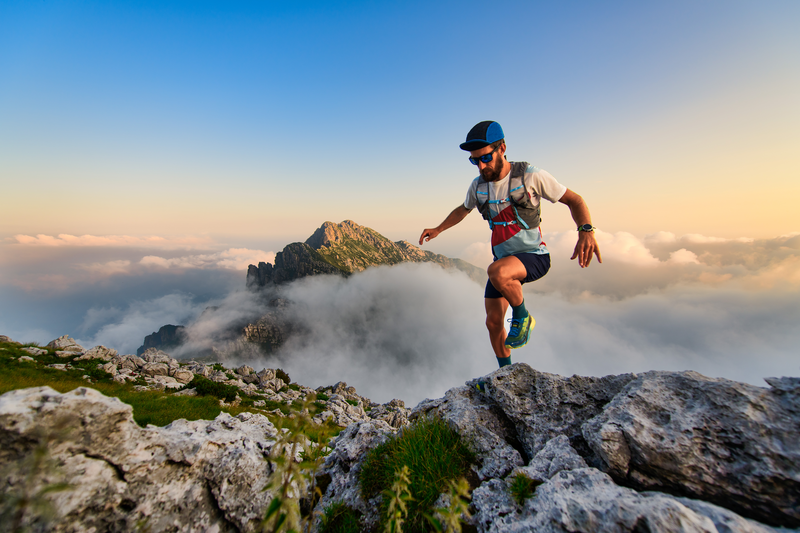
94% of researchers rate our articles as excellent or good
Learn more about the work of our research integrity team to safeguard the quality of each article we publish.
Find out more
CASE REPORT article
Front. Genet. , 18 April 2018
Sec. Genetics of Common and Rare Diseases
Volume 9 - 2018 | https://doi.org/10.3389/fgene.2018.00110
This article is part of the Research Topic Applying Next Generation Sequencing and Transgenic Models to Rare Disease Research View all 11 articles
Alström syndrome (AS) is a rare autosomal recessive disorder that shares clinical features with other ciliopathy-related diseases. Genetic mutation analysis is often required in making differential diagnosis but usually costly in time and effort using conventional Sanger sequencing. Herein we describe a Taiwanese patient presenting cone-rod dystrophy and early-onset obesity that progressed to diabetes mellitus with marked insulin resistance during adolescence. Whole exome sequencing of the patient's genomic DNA identified a novel frameshift mutation in exons 15 (c.10290_10291delTA, p.Lys3431Serfs*10) and a rare mutation in 16 (c.10823_10824delAG, p.Arg3609Alafs*6) of ALMS1 gene. The compound heterozygous mutations were predicted to render truncated proteins. This report highlighted the clinical utility of exome sequencing and extended the knowledge of mutation spectrum in AS patients.
Alström syndrome (AS; OMIM 203800) is a rare autosomal recessive disorder characterized by early-onset blindness due to cone-rod dystrophy, juvenile obesity followed by marked insulin resistance and type 2 diabetes mellitus, and progressive sensorineural hearing impairment that usually takes place within the first year of life (Marshall et al., 2007a). Moreover, a certain portion of AS patients also presents liver, kidney, neurological, cardiac, and pulmonary diseases (Marshall et al., 2005). No disease specific treatment is as yet available, whereas early morbidity and mortality are expected in affected patients.
The causative gene of AS has been recently ascribed to ALMS1, which harbors 23 exons and encodes a 461-kDa protein (ALMS1, centrosome and basal body associated protein) involved in ciliary functions (Hearn et al., 2002). Although the multi-organ pathogenesis of AS has not been fully delineated, it is suggested that truncated or dysfunctional proteins disrupt cell cycle regulation and intracellular trafficking (Girard and Petrovsky, 2011). Clinical diagnosis is based on observation of cardinal features. However, varied age of onset and evolutional severity of manifestations may be due to the allelic and expressive heterogeneity of AS and thus defer the diagnosis particularly in patients with only part of clinical features that are also shared by other ciliopathies, such as Bardet-Biedl syndrome (Marshall et al., 2007a). Under such a circumstance, confirmatory mutation analysis of ALMS1 is required and usually laborious by conventional Sanger sequencing, given the size and mutation spectrum of ALMS1 gene along with other disease associated genes involved in the ciliopathies (Marshall et al., 2007b). This hardship can be resolved by the application of next generation sequencing techniques, which are now available for the identification of genetic variants comprehensively (Bamshad et al., 2011). Here, we reported a Taiwanese patient with AS features and identified a novel mutations in ALMS1 through whole exome sequencing (WES).
A 16-year-old Taiwanese boy, born uneventfully to Taiwanese parents, measured 147 cm in height and 55 kg in weight with a body mass index 25.2 kg/m2. He presented hyperphagia and rapid weight gain at the age of 5 months when he measured 10 kg (>97th %) and 69 cm (>75th %). Meanwhile, he received diagnosis of bilateral nystagmus that progressed to nearly blindness at the age of 4. Funduscopic examinations revealed retinitis pigmentosa. He was hospitalized at the age of 11 because of diabetic ketoacidosis and acute pancreatitis. Examinations also revealed hypertriglyceridemia, severe fatty liver, and renal insufficiency. The initial metabolic survey found that marked insulin resistance and pancreatic insufficiency with the homeostatic model assessment for insulin resistance (HOMA-IR) index was 83.5 [a value greater than 2.6 may indicate insulin resistance in adolescents (Burrows et al., 2015)] and HOMA-ß 78.2% [a value greater than 100% may indicate reserved insulin secretion (Matthews et al., 1988)]. He was started on insulin therapy since then and the daily requirement progressively exceeded 260 IU/day with the presence of remarkable acanthosis nigricans within 5 years' time. Updated endocrine investigation showed an optimal increase of C-peptide secretion in response to intravenous glucagon stimulation. Echocardiography revealed a borderline left ventricular dilatation. The intellectual and hearing ability were both unaffected.
Informed written consent was acquired from the patient and his parents, as the entire procedure of this study was approved by the Institutional Review Board of the National Cheng Kung University Hospital (B-BR-104-063). The proband's genomic DNA was extracted from peripheral blood collected in EDTA-containing tubes. SureSelect QXT All human exon V6 (Agilent), which targeted 60 Mb of the exonic regions, was applied to construct the exome library that was then sequenced on Illumina NextSeq500 platform. We aligned the sequence reads to human genome reference Hg19 using Novoalign (www.novocraft.com) and identified single nucleotide variants and small insertions and deletions using Genome Analysis Toolkit 3.4 (GATK; www.broadinstitute.org/gatk). The sequence variants were annotated with SeattleSeqAnnotation (snp.gs.washington.edu/SeattleSeqAnnotation138) and novel variants were filtered against 1000 Genomes, dbSNP, and Genome Aggregation Database (gnomad.broadinstitute.org). Sanger sequencing was finally used to confirm the mutation.
With the average coverage of 40.1X on targeted regions, we identified 28,967 novel genetic variants in the exome sequencing. The summary of variants identified was listed in Table 1. Two pathological mutations in ALMS1 were identified: chr2:73786171delTA (c.10290_10291delTA) in exon 15 (Figure 1A, upper panel) and chr2:73799829delAG (c.10823_10824delAG) in exon 16 (Figure 1A, lower panel). These mutations were further confirmed by Sanger sequencing (Figure 1B) and the results showed that c.10290_10291delTA (p.Lys3431Serfs*10) was maternally inherited and c.10823_10824delAG (p.Arg3609Alafs*6) was paternally inherited. These mutations resided in a conserved stretch of amino acids and led to truncated proteins lacking the ALMS motif (Figures 2A,B).
Figure 1. Pedigree and mutation analysis of the family. (A) Alignment of exome sequences to Hg19 showing 2-base pair deletion in exon 15 and exon 16 of ALMS1. (B) The proband (II-1) carried compound heterozygous mutations: c.10290_10291delTA inherited from his mother (I-2) and c.10831_10832delAG from his father (I-1). WT, wild type.
Figure 2. (A) Mutation spectrum of ALMS1 and domains structure of ALMS1. The frameshift mutations identified in the proband in this study are in bold. (B) Conserved amino acid sequences of ALMS1 (amino acid 3431 and 3609) and the predicted truncated ALMS1 caused by the frameshift mutation identified in this proband.
In this report, we described a Taiwanese patient presenting clinical features compatible with AS, except the absence of hearing impairment. Using WES, we identified compound heterozygous mutations in the exons 15 and 16. Both affected alleles were frameshift mutations that were predicted to cause premature stop codon downstream and render truncated ALMS1 protein.
The ALMS1 protein consists of 4,169 amino acids and contains several domains including a potential signal peptide at residues 211–223, a leucine zipper at residues 2480–2501, and an ALMS motif at residues 4035–4167 (Collin et al., 2002; Hearn et al., 2002). The exact molecular role of these motifs is not completely elucidated, although truncated ALMS1 proteins have exhibited perturbed effects on intracellular localization, microtubular organization, cell cycle regulation (Hearn et al., 2002, 2005; Girard and Petrovsky, 2011). In our patient, truncated proteins, if transcribed from the observed mutations p.Lys3431Serfs*10 and p.Arg3609Alafs*6, are presumed to lack the ALMS motif that is essential in co-localization with centrioles and basal bodies (Knorz et al., 2010). Given that ALMS1 is ubiquitous expressed in human tissues, low abundance or functionality of transcripts may contribute to the multi-organ pathogenesis in AS, such as metabolic and neurosensory disorders (Collin et al., 2002; Hearn et al., 2002; Girard and Petrovsky, 2011). However, phenotypic variability exists in the same ALMS1 mutated spots, even within the same family (Titomanlio et al., 2004). Our patient did not present typically sensorineural hearing impairment, which can be explained by the prior assumption that genotype-phenotype correlation of ALMS1 mutations may be modified by other modifier genes or environmental factors (Collin et al., 2002; Marshall et al., 2007b).
A wide array of nonsense and frameshift mutations has been identified in coding regions of ALMS1 gene with potential hotspots preferentially located in exons 8, 10, and 16 (Figure 2A) (Marshall et al., 2007b; Ozantürk et al., 2015). Ethnicity remains as a strong contributor to the distribution of genetic variants. However, the skewed clustering of mutations may be affected by genetic founder effect and consanguinity. In East Asian descents, most reported mutations were disperse within the aforementioned cluster of exons but distinctive in location and structure (Liang et al., 2013; Marshall et al., 2015). Only a few variants have been reported more than once in East Asian populations, such as c.11116_111134del recurring in Taiwanese families (Marshall et al., 2007b; Lee et al., 2009). The rare variant c.10823_10824delAG has been exclusively reported in East Asian descents with an estimated allele frequency of 2.53 × 10−5 in the up-to-date Genome Aggregation Database1. Therefore, we assumed a genetic founder effect on this rare pathological variant. Obtaining genetic diagnosis of clinical patients in under-genotyped populations can expand our knowledge about this disease entity and relevant functionality of ALMS1 gene. From practical perspective, screening for these mutations in suspicious cases will be a feasible diagnostic step if there are sufficient data regarding the prevalence of ALMS1 mutations in the local population. Otherwise whole gene sequencing is usually required to fully capture the genotypes.
Technological advancement in high throughput sequencing has met clinical needs and thus hastened the identification of novel pathological variants in ALMS1 gene (Bamshad et al., 2011; Ozantürk et al., 2015). As compared to conventional Sanger sequencing, WES provides an effective and efficient alternative method that aids genetic diagnosis especially in cases with overlapping features among a certain number of differential diagnoses enlisted within the spectrum of ciliopathy. Moreover, the clinical utility would be significantly promoted within the near future as the cost of WES is expected to drop below that of Sanger sequencing, when reading a sizable coding region of target gene is required. Our report highlights the value of WES in providing genetic diagnosis in rare diseases.
M-CT and P-CC conceived the study; M-CT, Ye-YC, and Yu-YC collected clinical information; H-WY, TL, and P-CC conducted the bioinformatics analysis; M-CT drafted the manuscript and P-CC supervised the entire study. All the authors approved the final version of manuscript.
This study was supported by the research grant from National Cheng Kung University Hospital (NCKUH-10507026).
The authors declare that the research was conducted in the absence of any commercial or financial relationships that could be construed as a potential conflict of interest.
1. ^The Genome Aggregation Database. Available online at: http://gnomad.broadinstitute.org/about (Accessed January 18, 2018).
Bamshad, M. J., Ng, S. B., Bigham, A. W., Tabor, H. K., Emond, M. J., Nickerson, D. A., et al. (2011). Exome sequencing as a tool for Mendelian disease gene discovery. Nat. Rev. Genet. 12, 745–755. doi: 10.1038/nrg3031
Burrows, R., Correa-Burrows, P., Reyes, M., Blanco, E., Albala, C., and Gahagan, S. (2015). Healthy chilean adolescents with HOMA-IR ≥ 2.6 have increased cardiometabolic risk: association with genetic, biological, and environmental factors. J. Diabetes Res. 2015:783296. doi: 10.1155/2015/783296
Collin, G. B., Marshall, J. D., Ikeda, A., So, W. V., Russell-Eggitt, I., Maffei, P., et al. (2002). Mutations in ALMS1 cause obesity, type 2 diabetes and neurosensory degeneration in Alstrom syndrome. Nat. Genet. 31, 74–78 doi: 10.1038/ng867
Girard, D., and Petrovsky, N. (2011). Alstrom syndrome: insights into the pathogenesis of metabolic disorders. Nat. Rev. Endocrinol. 7, 77–88. doi: 10.1038/nrendo.2010.210
Hearn, T., Renforth, G. L., Spalluto, C., Hanley, N. A., Piper, K., Brickwood, S., et al. (2002). Mutation of ALMS1, a large gene with a tandem repeat encoding 47 amino acids, causes Alstrom syndrome. Nat. Genet. 31, 79–83. doi: 10.1038/ng874
Hearn, T., Spalluto, C., Phillips, V. J., Renforth, G. L., Copin, N., Hanley, N. A., et al. (2005). Subcellular localization of ALMS1 supports involvement of centrosome and basal body dysfunction in the pathogenesis of obesity, insulin resistance, and type 2 diabetes. Diabetes 54, 1581–1587. doi: 10.2337/diabetes.54.5.1581
Knorz, V. J., Spalluto, C., Lessard, M., Purvis, T. L., Adigun, F. F., Collin, G. B., et al. (2010). Centriolar association of ALMS1 and likely centrosomal functions of the ALMS motif-containing proteins C10orf90 and KIAA1731. Mol. Biol. Cell 21, 3617–3629. doi: 10.1091/mbc.E10-03-0246
Lee, N. C., Marshall, J. D., Collin, G. B., Naggert, J. K., Chien, Y. H., Tsai, W. Y., et al. (2009). Caloric restriction in Alstrom syndrome prevents hyperinsulinemia. Am. J. Med. Genet. A 149A, 666–668. doi: 10.1002/ajmg.a.32730
Liang, X., Li, H., Li, H., Xu, F., Dong, F., and Sui, R. (2013). Novel ALMS1 mutations in Chinese patients with Alstrom syndrome. Mol. Vis. 19, 1885–1891. Available online at: http://www.molvis.org/molvis/v19/1885
Marshall, J. D., Beck, S., Maffei, P., and Naggert, J. K. (2007a). Alstrom syndrome. Eur. J. Hum. Genet. 15, 1193–1202. doi: 10.1038/sj.ejhg.5201933
Marshall, J. D., Bronson, R. T., Collin, G. B., Nordstrom, A. D., Maffei, P., Paisey, R. B., et al. (2005). New Alstrom syndrome phenotypes based on the evaluation of 182 cases. Arch. Intern. Med. 165, 675–683. doi: 10.1001/archinte.165.6.675
Marshall, J. D., Hinman, E. G., Collin, G. B., Beck, S., Cerqueira, R., Maffei, P., et al. (2007b). Spectrum of ALMS1 variants and evaluation of genotype-phenotype correlations in Alstrom syndrome. Hum. Mutat. 28, 1114–1123. doi: 10.1002/humu.20577
Marshall, J. D., Muller, J., Collin, G. B., Milan, G., Kingsmore, S. F., Dinwiddie, D., et al. (2015). Alstrom Syndrome: mutation spectrum of ALMS1. Hum. Mutat. 36, 660–668. doi: 10.1002/humu.22796
Matthews, D. R., Hosker, J. P., Rudenski, A. S., Naylor, B. A., Treacher, D. F., and Turner, R. C. (1988). Homeostasis model assessment: insulin resistance and beta-cell function from fasting plasma glucose and insulin concentrations in man. Diabetologia 28, 412–419. doi: 10.1007/BF00280883
Ozantürk, A., Marshall, J. D., Collin, G. B., Düzenli, S., Marshall, R. P., Candan, S., et al. (2015). The phenotypic and molecular genetic spectrum of Alström syndrome in 44 Turkish kindreds and a literature review of Alström syndrome in Turkey. J. Hum. Genet. 60, 1–9. doi: 10.1038/jhg.2014.85
Keywords: Alström syndrome, ALMS1 gene, ciliopathy, whole exome sequencing, childhood obesity, retinitis pigmentosa
Citation: Tsai M-C, Yu H-W, Liu T, Chou Y-Y, Chiou Y-Y and Chen P-C (2018) Rare Compound Heterozygous Frameshift Mutations in ALMS1 Gene Identified Through Exome Sequencing in a Taiwanese Patient With Alström Syndrome. Front. Genet. 9:110. doi: 10.3389/fgene.2018.00110
Received: 14 December 2017; Accepted: 21 March 2018;
Published: 18 April 2018.
Edited by:
Arvin Gouw, Rare Genomics Institute, United StatesReviewed by:
Thomy J. L. de Ravel, University Hospitals Leuven, BelgiumCopyright © 2018 Tsai, Yu, Liu, Chou, Chiou and Chen. This is an open-access article distributed under the terms of the Creative Commons Attribution License (CC BY). The use, distribution or reproduction in other forums is permitted, provided the original author(s) and the copyright owner are credited and that the original publication in this journal is cited, in accordance with accepted academic practice. No use, distribution or reproduction is permitted which does not comply with these terms.
*Correspondence: Peng-Chieh Chen, cGVuZ2NoaWNAbWFpbC5uY2t1LmVkdS50dw==
Disclaimer: All claims expressed in this article are solely those of the authors and do not necessarily represent those of their affiliated organizations, or those of the publisher, the editors and the reviewers. Any product that may be evaluated in this article or claim that may be made by its manufacturer is not guaranteed or endorsed by the publisher.
Research integrity at Frontiers
Learn more about the work of our research integrity team to safeguard the quality of each article we publish.