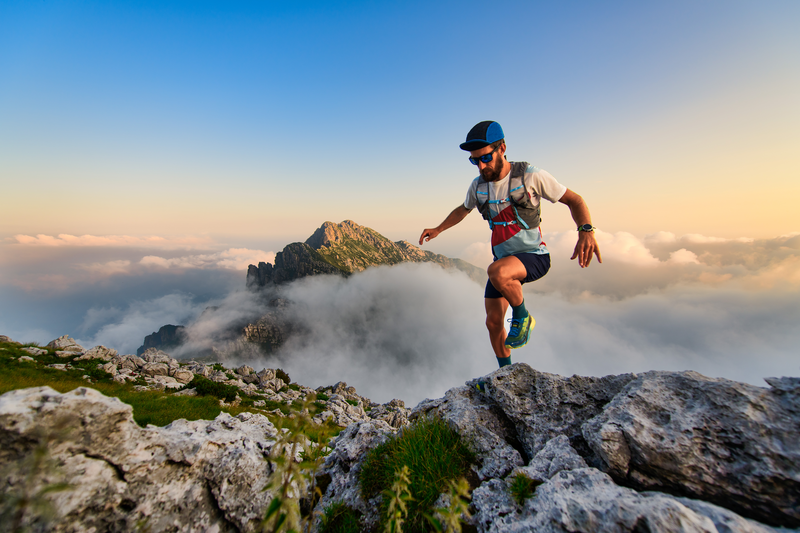
95% of researchers rate our articles as excellent or good
Learn more about the work of our research integrity team to safeguard the quality of each article we publish.
Find out more
CASE REPORT article
Front. Genet. , 19 October 2015
Sec. Genetics of Common and Rare Diseases
Volume 6 - 2015 | https://doi.org/10.3389/fgene.2015.00311
This article is part of the Research Topic Impact of nuclear genetic variants on mitochondrial pathophysiology View all 20 articles
Dominant optic neuropathies causing fiber loss in the optic nerve are among the most frequent inherited mitochondrial diseases. In most genetically resolved cases, the disease is associated to a mutation in OPA1, which encodes an inner mitochondrial dynamin involved in network fusion, cristae structure and mitochondrial genome maintenance. OPA1 cleavage is regulated by two m-AAA proteases, SPG7 and AFG3L2, which are, respectively involved in Spastic Paraplegia 7 and Spino-Cerebellar Ataxia 28. Here, we identified a novel mutation c.1402C>T in AFG3L2, modifying the arginine 468 in cysteine in an evolutionary highly conserved arginine-finger motif, in a family with optic atrophy and mild intellectual disability. Ophthalmic examinations disclosed a loss of retinal nerve fibers on the temporal and nasal sides of the optic disk and a red–green dyschromatopsia. Thus, our results suggest that neuro-ophthalmological symptom as optic atrophy might be associated with AFG3L2 mutations, and should prompt the screening of this gene in patients with isolated and syndromic inherited optic neuropathies.
Dominant optic atrophy (DOA) is a neurodegenerative disease that primarily targets the retinal ganglion cell (RGC), representing an important cause of early onset blindness. In most patients, the clinical presentation is restricted to visual impairment, but in about 20% of them, other symptoms are associated such as deafness, muscle weakness, and peripheral neuropathy (Yu-Wai-Man et al., 2010; Lenaers et al., 2012; Maresca et al., 2013). DOA is caused by mutations mostly in OPA1 (Alexander et al., 2000; Delettre et al., 2000; Ferre et al., 2015) and rarely in OPA3 (Reynier et al., 2004) or SPG7 (Klebe et al., 2012), all encoding mitochondrial inner membrane proteins. In some cases, DOA associated to deafness is caused by WFS1 mutations (Rendtorff et al., 2011), encoding an endoplasmic reticulum protein, regulating calcium homeostasis and consequently mitochondrial physiology. Nevertheless, the systematic screening by Sanger sequencing of exonic sequences in OPA1, OPA3, and WFS1 genes in our DOA cohort only allowed for about 50% of genetic diagnoses. We further excluded OPA1 deletion/duplication in all patients, by multiplex ligation-dependent probe amplification (SALSA MLPA KIT P229-B1 OPA1 – MRC-Holland), except one, who presented a full OPA1 deletion.
Thus, to deepen DOA genetic diagnosis, we performed whole exome sequencing (WES) on the proband from 12 unresolved DOA families. We report here the results obtained for one family, presenting an Optic Atrophy and mild intellectual disability (Figure 1A). Amongst the 60,000 variants identified by WES on the index patient III.4, no mutation was found in OPA1, OPA3, and WFS1 genes, that were covered by a minimal sequencing depth of 50x, 44x, and 40x, respectively. We also carefully excluded mutations in eleven genes (AIFM1, ATP1A3, c12orf65, CISD2, FXN, MTPAP, PRPS1, SFXN4, SPG7, TIMM8, and TSFM), for which mutations might cause clinical presentations related to our patients (e.g., Mohr-Tranebjaerg and CAPOS syndromes). Thus, we progressively filtered WES data. After excluding intronic, 5′UTR, 3′UTR and synonymous variants, we obtained 14,241 exonic and splicing variants. A total of 9,776 variants were selected with a number of call higher than 4 using the GATK, VarScan, SNVer, Lofreq, Platypus softwares. We discarded common variants reported in NHLBI Exome Sequencing Project, Exome Variant Server, NCBI, the 1000 genomes and ExAC ver0.2, with a frequency greater than 1%, leading to 145 left variants. Then 30 deleterious SNPs predicted by SIFT, PolyPhen-2, and Mutation Taster softwares were retained (see Supplementary Table S1). Finally, these variants were filtered for heterozygous mutations in gene encoding mitochondrial protein (MitoCarta), as to date all DOA genes are involved in mitochondrial physiology. We only found the c.1402C>T heterozygous mutation in AFG3L2 exon 11 (Figure 1B) that was never reported before in any database (see above for a list). It modifies an evolutionary highly conserved arginine 468 in cysteine (p.Arg468Cys) within an arginine-finger motif (RPGRxxR) that is found in the AAA domain of all AFG3L2 orthologs and SPG7 paralogs. The arginine 468 is also conserved in the prokaryote FtsH from E. coli (Figure 1C). In silico prediction of the p.Arg468Cys pathogenicity by Polyphen2, SIFT, Mutation taster, imutant, Pmut, MetaSVM, MetaLR, FathMM, and PhD-SNP programs gave the highest scores, supporting the pathogenicity of this mutation.
FIGURE 1. Identification of AFG3L2 mutation. (A) Pedigree showing the family with the affected members in black, and the segregation of the p.Arg468Cys amino-acid change in the two affected patients, knowing that DNA sample was not available for the other family members. (B) Electrophoregram presenting the AFG3L2 c.1402C>T heterozygous mutation. (C) Alignment of AFG3L2 orthologs (amino-acids 460 to 476) showing the evolutionary conserved positions around the Arginine 468, which is squared in red in the triple arginine motif (bold rectangles). (H.s. Homo sapiens; D.r. Danio rerio; X.l. Xenopus laevis; D.m. Drosophila melanogaster; A.t. Arabidopsis thaliana; E.c. Escherichia coli FtsH protein; SPG7: human paralog of AFG3L2).
This 48-year-old male complained of visual difficulties since 12 years of age including decrease in visual acuity, photophobia, and color vision impairment with very slow progression of visual loss. He otherwise had normal development without neuromuscular or other sensory involvement, but he had mild intellectual disability. His best corrected visual acuity was decreased to 0.3 and 0.4 in the right and left eyes, respectively. Fundus examination revealed bilateral moderate optic disk pallor (Figure 2A, top), and optical coherence tomography (OCT) disclosed a moderate overall decrease in the thickness of the retinal nerve fiber layer (Figure 2B, top). Color vision testing showed a red/green axis of dyschromatopsia and visual field indicated a decrease in light sensitivity without scotoma (not shown). He refused further neurological examination and MRI scanning.
FIGURE 2. Ophthalmological exploration of AFG3L2 patients. (A) Eye fundus (RE: right eye; LE: left eye) from patient II.4 (top) and patient III.4 (bottom) disclose temporal pallor of the optic disks. (B) Optical Coherence Tomography scanning and measurement of the retinal nerve fiber layers of the optic disks evidenced a drastic thickness reduction in the temporal and nasal quadrants of patient II.4 (top) and III.4 (bottom).
His son was born after a normal pregnancy and delivery was uneventful. Motor development was normal, he managed to walk unaided at 14 months. Learning difficulties were noticed in primary school. Cognitive evaluation disclosed a moderate intellectual disability (Wechsler Intelligence Scale III: Total Intellectual Quotient 47) and the patient benefited of a special educational program from the age of 8, and was admitted in an institution for patients with mental impairment from the age of 14. This patient had no clinical complaint especially no unsteadiness, no clumsiness, no cramping, no fatigability. Clinical examination was performed at the age of 18 and disclosed a discrete Kyphosis, while motor strength, sensory evaluation, osteotendinous reflexes and cranial motor nerve functions were normal. Oculomotor movements were normal. The patient did not exhibit any sign of stato-kinetic ataxia; his family never noticed any jerk, and clinical examination did not disclose myoclonias. Audiological assessment including tonal and vocal audiometry was normal.
Ophthalmological investigations disclosed a best corrected visual acuity of 0.5 on both eyes, with difficulties to fixate his gaze. Fundus examination revealed bilateral severe optic disk pallor (Figure 2A, bottom) and OCT examination disclosed a marked overall decrease in the thickness of the retinal nerve fiber layer (Figure 2B, bottom). His brain MRI disclosed reduced size of the optic tract (Figure 3A, left) and atrophy of the optic chiasma (Figure 3A, right), without any sign of cerebellar atrophy (Figure 3B, left and right). MRI spectroscopy was normal.
FIGURE 3. Brain MRI of patient III.4 at 18 years of age. (A) Left: Frontal section, T2 sequence, showing atrophy of the optic nerve (arrow) and Right: reduced size of the chiasma (arrow). Magnification of the optic track (Left) and chiasma (Right) regions are shown underneath the full picture. (B) Left: Sagittal section, T1 sequence, showing a normal aspect of the brain, especially of the vermis (arrow); Right: axial section, T2 sequence, showing a normal aspect of the posterior fossa, especially the cerebellar hemisphere (arrow).
Both patients denied to do further examination, therefore skin biopsy and brain MRI of the father were not available. The other family members were not compliant with clinical and genetic analysis.
The AFG3L2 and SPG7 m-AAA proteases have crucial multiple functions in the homeostasis of mitochondrial protein synthesis, assembly and degradation. On the one hand, homo and hetero-polymers of these proteases have broad functional targets, as for example proteins involved in the assembly of ribosomes and respiratory chain complexes, the degradation of misfolded proteins and the maintenance of mitochondrial nucleoids. On the other hand, they have also highly specific targets as the proteolytic cleavage of MRLP32 and OPA1 (Martinelli and Rugarli, 2010). The latter cleavage in OPA1 amino-terminal region is required for the physiological equilibrium between pro-fusion and pro-fission isoforms of this large GTPase (Ehses et al., 2009). In this respect, AFG3L2 down-regulation affects respiratory capacity and calcium uptake, and induces mitochondrial network fragmentation (Maltecca et al., 2012), a cellular phenotype that we also found in cell lines with altered OPA1 expression (Olichon et al., 2007; Chevrollier et al., 2008; Dayanithi et al., 2010). The possible consequences of AFG3L2 mutation might be related to the pathophysiology of DOA, a disease which is caused by OPA1 haplo-insufficiency or eventually by a dominant negative process (Olichon et al., 2007). Thus, the p.Arg468Cys mutation might affect AFG3L2 specific interaction with OPA1 long isoform and consequently its processing, leading to a bias in OPA1 physiological maturation and pro-fusion activity.
To date, three genes have been identified as responsible for non-syndromic DOA: OPA1, OPA3, and SPG7 (Alexander et al., 2000; Delettre et al., 2000; Reynier et al., 2004; Klebe et al., 2012), encoding proteins embedded in the inner mitochondrial membrane (Olichon et al., 2002; Atorino et al., 2003; Grau et al., 2013), and involved directly or indirectly in mitochondrial fusion and fission (Olichon et al., 2003; Ishihara et al., 2006; Ryu et al., 2010). OPA1 mutations are by far the most frequently found in patients with non-syndromic DOA, and to date more than 250 mutations have been identified in this gene (Ferre et al., 2015). Three and one dominant mutations were respectively identified in OPA3 and SPG7 genes. In virtually all cases, patients show optic disk atrophy with a predominant loss of the temporal retinal nerve fiber bundle revealed by OCT examination (Klebe et al., 2012; Lenaers et al., 2012). Nevertheless, it should be stressed that DOA is frequently associated to secondary symptoms, most often neurosensory deafness (Amati-Bonneau et al., 2005), but also external ophthalmoplegia, myopathy, peripheral neuropathy, ataxia, and spastic paraplegia for OPA1 patients (Yu-Wai-Man et al., 2010), and cataract, postural tremor, extrapyramidal signs, ataxia, areflexia, and neurosensory deafness for OPA3 patients (Reynier et al., 2004; Ayrignac et al., 2012; Grau et al., 2013). Concerning SPG7, most recessive patients with primary spastic paraplegia also present optic atrophy, whereas in the single family reported with a dominant SPG7 mutation, patients had isolated optic atrophy (Klebe et al., 2012).
Importantly, combined optic nerve atrophy and encephalopathy were not previously reported in families with dominant inheritance, whereas this is encountered in syndromic recessive forms of optic atrophy (Metodiev et al., 2014). Thus the clinical presentation that we report here is somehow particular in associating DOA to mild intellectual disability.
Mutations in AFG3L2 are responsible for the SCA28 and SPAX5 forms of spinocerebellar ataxia (Di Bella et al., 2010; Pierson et al., 2011). In SCA28, most cases are caused by heterozygous mutations in exon 15 and 16, with a single exception (c.1295A>C, p.N432T) in exon 10 (Cagnoli et al., 2010; Di Bella et al., 2010), whereas in SPAX5, two families were reported with homozygous mutations in exon 15 (c.1847A>G, p.Y616C and c.1875G>A, p.M625I; Pierson et al., 2011; Muona et al., 2015), (Figure 4). Functional studies of the encoded m-AAA protease with mutation in exons 15 or 16 revealed an abnormal C-terminus M41 peptidase domain compromising the stability and proteolytic activity of the m-AAA complex, possibly through a dominant negative process. By contrast, the exon 10 mutation modifies the structure of the pore channel entry, in which substrates are pulled to be degraded (Di Bella et al., 2010). In addition, two AFG3L2 gene deletions have been reported in SCA28 patients, one of the entire gene (Myers et al., 2014), and one including exon 14 to exon 16 (Smets et al., 2014), as well as one frame shift mutation in exon 15, deleting the last 144 amino acids (Musova et al., 2014), (Figure 4). Thus AFG3L2 haplo-insufficiency is one of the possible causes of SCA28. Very recently, a novel c.346A>G, p.G116R mutation in the N-terminal domain of AFG3L2 protein was reported in a patient presenting spontaneous myoclonus at proximal limb associated to pyramidal signs. As for the cases we report here, this young patient did not present cerebellar ataxia, oculomotor abnormalities or sensory deficits (Mancini et al., 2015), although we cannot exclude that they will develop these symptoms later in life.
FIGURE 4. Localization of AFG3L2 mutations in the gene and protein. The structures of AFG3L2 gene (17 exons) and the protein (domains and amino-acid positions) are described on the top and bottom of the figure, respectively, with all the pathogenic mutations and associated clinical presentation reported to date. In black: SCA28, in blue: SPAX5, in green: Myoclony and pyramidal signs, in red: present case.
In both SCA28 and SPAX5 presentations, the patients have a typical cerebellar syndrome with ataxia, dysarthria, and oculomotor apraxia, variously associated with pyramidal and muscular signs, eventually including ptosis and external ophthalmoplegia (Cagnoli et al., 2010; Di Bella et al., 2010; Pierson et al., 2011). In none of them, optic atrophy, cognitive deficit or intellectual disability has ever been reported. Yet, optic atrophy is found associated with spastic paraplegia linked to recessive mutations in SPG7, the paralog of AFG3L2 encoding another component of the m-AAA protease involved in OPA1 proteolytic maturation (Casari et al., 1998; Arnoldi et al., 2008). In addition, genetic analysis of a large family with isolated optic atrophy disclosed the first dominant SPG7 mutation, c.1232A>C, p.D411A, affecting the AAA domain (Klebe et al., 2012), 56 amino-acids upstream of the conserved RPGR motif, in which we found the AFG3L2 mutation.
Both patients described in this study, who harbored a novel AFG3L2 mutation, had typical signs of hereditary optic atrophy, without abnormal gait or signs of cerebellar impairment. Indeed, they complained of bilateral, symmetrical visual loss starting in infancy and they showed optic disk pallor with thinned retinal nerve fiber layer. Dyschromatopsia was also present in one of the patient. This is in contrast with the SCA28 and SPAX5 presentations in which optic atrophy has never been reported. Yet, it is possible that asymptomatic optic atrophy exists in SCA28 and SPAX5 patients since decreased visual acuity can be absent in moderate forms of optic atrophy, suggesting that future patients with AFG3L2 mutations should have systematic fundus and OCT examinations. Although the cases we report here do not present the spinocerebellar ataxia typical of SCA28 patients, we cannot rule out the possibility that they will develop this symptom later in their life time.
Finally, both patients showed mild mental retardation, a finding which has not yet been associated to DOA, SCA28, or SPAX5. Whether cognitive disturbances is related to the AFG3L2 mutation itself, or to an independent phenomenon related to another dominant locus segregating separately from AFG3L2 was not determined. Therefore, a fortuitous association of DOA and mental deficiency in these patients cannot be ruled out. Nevertheless, we suggest that genetically unresolved DOA patients with or without intellectual disability could be screened for the presence of an AFG3L2 mutation. In this respect, finding novel AFG3L2 mutations related to DOA or disclosing optic atrophy in SCA28 patients would strongly reinforce the data that we presented here.
Dominant Optic Atrophy is among the most frequent mitochondrial disorder and probably the most frequent form of inherited optic neuropathy (Lenaers et al., 2012) and essentially associated to an OPA1 mutation. Nevertheless exonic sequencing of this gene and OPA3 and SPG7, the other known DOA genes, only leads to some 50% of positive results. Therefore, identification of additional genes involved in DOA is required to help genetic diagnosis. In this respect, the identification of a dominant mutation in SPG7 and eventually in AFG3L2 in DOA patients suggests that m-AAA proteases could be involved in a pathway responsible of retinal ganglion neuron degeneration, and contribute to a better understanding of the etiology and genotype – phenotype correlations of inherited diseases affecting the optic nerve. Although probably very uncommon, the possible involvement of AFG3L2 and SPG7 in optic neuropathies should prompt the screening of these genes in isolated and syndromic DOA patients, negative for OPA1 and OPA3 mutation. They also should favor neuro-ophthalmological examinations in SCA28 and SPAX5 patients with spinocerebellar ataxia.
All authors contributed to the conception and the design of the study, to the analysis of data, to the writing and approval of the manuscript to be published, and agree to be accountable for all the aspects of the work. AR, SM, CG, and CPH contributed to the clinical investigations. MJ, SS, and GL contributed to the genetic analysis.
The authors declare that the research was conducted in the absence of any commercial or financial relationships that could be construed as a potential conflict of interest.
We are indebted to INSERM, CNRS, the University of Montpellier, the University of Angers, the CHU d’Angers, the region Pays de la Loire, Angers Loire Metropole, the Fondation pour les Maladies Rares and Fondation pour la Recherche Médicale, and the following patient associations: Retina France, UNADEV, Ouvrir Les Yeux for their financial the support. Drs. B. Wissinger and C. de Layon are acknowledged for constructive discussions.
Informed written consent was obtained from all patients to perform genetic studies.
The Department of Ophthalmology of the University-Hospital of Montpellier has the authorization # 11018S from the French Ministry of Health to perform biomedical research in the field of physiology, pathophysiology, epidemiology, and genetics in ophthalmology.
The Supplementary Material for this article can be found online at: http://journal.frontiersin.org/article/10.3389/fgene.2015.00311
TABLE S1 | Listing of the 30 damaging single nucleotide polymorphisms identified in the whole exome sequencing of the patient III.4.
Alexander, C., Votruba, M., Pesch, U. E., Thiselton, D. L., Mayer, S., Moore, A., et al. (2000). OPA1, encoding a dynamin-related GTPase, is mutated in autosomal dominant optic atrophy linked to chromosome 3q28. Nat. Genet. 26, 211–215. doi: 10.1038/79944
Amati-Bonneau, P., Guichet, A., Olichon, A., Chevrollier, A., Viala, F., Miot, S., et al. (2005). OPA1 R445H mutation in optic atrophy associated with sensorineural deafness. Ann. Neurol. 58, 958–963. doi: 10.1002/ana.20681
Arnoldi, A., Tonelli, A., Crippa, F., Villani, G., Pacelli, C., Sironi, M., et al. (2008). A clinical, genetic, and biochemical characterization of SPG7 mutations in a large cohort of patients with hereditary spastic paraplegia. Hum. Mutat. 29, 522–531. doi: 10.1002/humu.20682
Atorino, L., Silvestri, L., Koppen, M., Cassina, L., Ballabio, A., Marconi, R., et al. (2003). Loss of m-AAA protease in mitochondria causes complex I deficiency and increased sensitivity to oxidative stress in hereditary spastic paraplegia. J. Cell Biol. 163, 777–787. doi: 10.1083/jcb.200304112
Ayrignac, X., Liauzun, C., Lenaers, G., Renard, D., Amati-Bonneau, P., de Seze, J., et al. (2012). OPA3 –related autosomal dominant optic atrophy and cataract with ataxia and areflexia. Eur. Neurol. 68, 108–110. doi: 10.1159/000339310
Cagnoli, C., Stevanin, G., Brussino, A., Barberis, M., Mancini, C., Margolis, R. L., et al. (2010). Missense mutations in the AFG3L2 proteolytic domain account for approximately 1.5% of European autosomal dominant cerebellar ataxias. Hum. Mutat. 31, 1117–1124. doi: 10.1002/humu.21342
Casari, G., De Fusco, M., Ciarmatori, S., Zeviani, M., Mora, M., Fernandez, P., et al. (1998). Spastic paraplegia and OXPHOS impairment caused by mutations in paraplegin, a nuclear-encoded mitochondrial metalloprotease. Cell 93, 973–983. doi: 10.1016/S0092-8674(00)81203-9
Chevrollier, A., Guillet, V., Loiseau, D., Gueguen, N., de Crescenzo, M. A., Verny, C., et al. (2008). Hereditary optic neuropathies share a common mitochondrial coupling defect. Ann. Neurol. 63, 794–798. doi: 10.1002/ana.21385
Dayanithi, G., Chen-Kuo-Chang, M., Viero, C., Hamel, C., Muller, A., and Lenaers, G. (2010). Characterization of Ca2+ signalling in postnatal mouse retinal ganglion cells: involvement of OPA1 in Ca2+ clearance. Ophthalmic Genet. 31, 53–65. doi: 10.3109/13816811003698117
Delettre, C., Lenaers, G., Griffoin, J. M., Gigarel, N., Lorenzo, C., Belenguer, P., et al. (2000). Nuclear gene OPA1, encoding a mitochondrial dynamin-related protein, is mutated in dominant optic atrophy. Nat. Genet. 26, 207–210. doi: 10.1038/79936
Di Bella, D., Lazzaro, F., Brusco, A., Plumari, M., Battaglia, G., Pastore, A., et al. (2010). Mutations in the mitochondrial protease gene AFG3L2 cause dominant hereditary ataxia SCA28. Nat. Genet. 42, 313–321. doi: 10.1038/ng.544
Ehses, S., Raschke, I., Mancuso, G., Bernacchia, A., Geimer, S., Tondera, D., et al. (2009). Regulation of OPA1 processing and mitochondrial fusion by m-AAA protease isoenzymes and OMA1. J. Cell Biol. 187, 1023–1036. doi: 10.1083/jcb.200906084
Ferre, M., Caignard, A., Milea, D., Leruez, S., Cassereau, J., Chevrollier, A., et al. (2015). Improved locus-specific database for OPA1 mutations allows inclusion of advanced clinical data. Hum. Mutat. 36, 20–25. doi: 10.1002/humu.22703
Grau, T., Burbulla, L. F., Engl, G., Delettre, C., Delprat, B., Oexle, K., et al. (2013). A novel heterozygous OPA3 mutation located in the mitochondrial target sequence results in altered steady-state levels and fragmented mitochondrial network. J. Med. Genet. 50, 848–858. doi: 10.1136/jmedgenet-2013-101774
Ishihara, N., Fujita, Y., Oka, T., and Mihara, K. (2006). Regulation of mitochondrial morphology through proteolytic cleavage of OPA1. EMBO J. 25, 2966–2977. doi: 10.1038/sj.emboj.7601184
Klebe, S., Depienne, C., Gerber, S., Challe, G., Anheim, M., Charles, P., et al. (2012). Spastic paraplegia gene 7 in patients with spasticity and/or optic neuropathy. Brain 135, 2980–2993. doi: 10.1093/brain/aws240
Lenaers, G., Hamel, C., Delettre, C., Amati-Bonneau, P., Procaccio, V., Bonneau, D., et al. (2012). Dominant optic atrophy. Orphanet J. Rare Dis. 7, 46. doi: 10.1186/1750-1172-7-46
Maltecca, F., De Stefani, D., Cassina, L., Consolato, F., Wasilewski, M., Scorrano, L., et al. (2012). Respiratory dysfunction by AFG3L2 deficiency causes decreased mitochondrial calcium uptake via organellar network fragmentation. Hum. Mol. Genet. 21, 3858–3870. doi: 10.1093/hmg/dds214
Mancini, C., Orsi, L., Guo, Y., Li, J., Chen, Y., Wang, F., et al. (2015). An atypical form of AOA2 with myoclonus associated with mutations in SETX and AFG3L2. BMC Med. Genet. 16:16. doi: 10.1186/s12881-015-0159-0
Maresca, A., la Morgia, C., Caporali, L., Valentino, M. L., and Carelli, V. (2013). The optic nerve: a “mito-window” on mitochondrial neurodegeneration. Mol. Cell. Neurosci. 55, 62–76. doi: 10.1016/j.mcn.2012.08.004
Martinelli, P., and Rugarli, E. I. (2010). Emerging roles of mitochondrial proteases in neurodegeneration. Biochim. Biophys. Acta 1797, 1–10. doi: 10.1016/j.bbabio.2009.07.013
Metodiev, M. D., Gerber, S., Hubert, L., Delahodde, A., Chretien, D., Gerard, X., et al. (2014). Mutations in the tricarboxylic acid cycle enzyme, aconitase 2, cause either isolated or syndromic optic neuropathy with encephalopathy and cerebellar atrophy. J. Med. Genet. 51, 834–838. doi: 10.1136/jmedgenet-2014-102532
Muona, M., Berkovic, S. F., Dibbens, L. M., Oliver, K. L., Maljevic, S., Bayly, M. A., et al. (2015). A recurrent de novo mutation in KCNC1 causes progressive myoclonus epilepsy. Nat. Genet. 47, 39–46. doi: 10.1038/ng.3144
Musova, Z., Kaiserova, M., Kriegova, E., Fillerova, R., Vasovcak, P., Santava, A., et al. (2014). A novel frameshift mutation in the AFG3L2 gene in a patient with spinocerebellar ataxia. Cerebellum 13, 331–337. doi: 10.1007/s12311-013-0538-z
Myers, K. A., Warman Chardon, J., Huang, L., and Boycott, K. M. (2014). Deletion of AFG3L2 associated with spinocerebellar ataxia type 28 in the context of multiple genomic anomalies. Am. J. Med. Genet. A 164A, 3209–3212. doi: 10.1002/ajmg.a.36771
Olichon, A., Baricault, L., Gas, N., Guillou, E., Valette, A., Belenguer, P., et al. (2003). Loss of OPA1 perturbates the mitochondrial inner membrane structure and integrity, leading to cytochrome c release and apoptosis. J. Biol. Chem. 278, 7743–7746. doi: 10.1074/jbc.C200677200
Olichon, A., Emorine, L. J., Descoins, E., Pelloquin, L., Brichese, L., Gas, N., et al. (2002). The human dynamin-related protein OPA1 is anchored to the mitochondrial inner membrane facing the inter-membrane space. FEBS Lett. 523, 171–176. doi: 10.1016/S0014-5793(02)02985-X
Olichon, A., Landes, T., Arnaune-Pelloquin, L., Emorine, L. J., Mils, V., Guichet, A., et al. (2007). Effects of OPA1 mutations on mitochondrial morphology and apoptosis: relevance to ADOA pathogenesis. J. Cell. Physiol. 211, 423–430. doi: 10.1002/jcp.20950
Pierson, T. M., Adams, D., Bonn, F., Martinelli, P., Cherukuri, P. F., Teer, J. K., et al. (2011). Whole-exome sequencing identifies homozygous AFG3L2 mutations in a spastic ataxia-neuropathy syndrome linked to mitochondrial m-AAA proteases. PLoS Genet. 7:e1002325. doi: 10.1371/journal.pgen.1002325
Rendtorff, N. D., Lodahl, M., Boulahbel, H., Johansen, I. R., Pandya, A., Welch, K. O., et al. (2011). Identification of p.A684V missense mutation in the WFS1 gene as a frequent cause of autosomal dominant optic atrophy and hearing impairment. Am. J. Med. Genet. A 155A, 1298–1313. doi: 10.1002/ajmg.a.33970
Reynier, P., Amati-Bonneau, P., Verny, C., Olichon, A., Simard, G., Guichet, A., et al. (2004). OPA3 gene mutations responsible for autosomal dominant optic atrophy and cataract. J. Med. Genet. 41, e110. doi: 10.1136/jmg.2003.016576
Ryu, S. W., Jeong, H. J., Choi, M., Karbowski, M., and Choi, C. (2010). Optic atrophy 3 as a protein of the mitochondrial outer membrane induces mitochondrial fragmentation. Cell. Mol. Life Sci. 67, 2839–2850. doi: 10.1007/s00018-010-0365-z
Smets, K., Deconinck, T., Baets, J., Sieben, A., Martin, J. J., Smouts, I., et al. (2014). Partial deletion of AFG3L2 causing spinocerebellar ataxia type 28. Neurology 82, 2092–2100. doi: 10.1212/WNL.0000000000000491
Keywords: optic nerve, dominant mutation, AFG3L2, OPA1, retinal ganglion neurons
Citation: Charif M, Roubertie A, Salime S, Mamouni S, Goizet C, Hamel CP and Lenaers G (2015) A novel mutation of AFG3L2 might cause dominant optic atrophy in patients with mild intellectual disability. Front. Genet. 6:311. doi: 10.3389/fgene.2015.00311
Received: 25 March 2015; Accepted: 28 September 2015;
Published: 19 October 2015.
Edited by:
Enrico Baruffini, University of Parma, ItalyReviewed by:
Alfredo Brusco, University of Torino, ItalyCopyright © 2015 Charif, Roubertie, Salime, Mamouni, Goizet, Hamel and Lenaers. This is an open-access article distributed under the terms of the Creative Commons Attribution License (CC BY). The use, distribution or reproduction in other forums is permitted, provided the original author(s) or licensor are credited and that the original publication in this journal is cited, in accordance with accepted academic practice. No use, distribution or reproduction is permitted which does not comply with these terms.
*Correspondence: Guy Lenaers, Z3V5LmxlbmFlcnNAaW5zZXJtLmZy
Disclaimer: All claims expressed in this article are solely those of the authors and do not necessarily represent those of their affiliated organizations, or those of the publisher, the editors and the reviewers. Any product that may be evaluated in this article or claim that may be made by its manufacturer is not guaranteed or endorsed by the publisher.
Research integrity at Frontiers
Learn more about the work of our research integrity team to safeguard the quality of each article we publish.