- 1Center for Fisheries, Aquaculture, and Aquatic Sciences, School of Biological Sciences, Southern Illinois University, Carbondale, IL, United States
- 2Department of Soil and Water Sciences, University of Florida, Gainesville, FL, United States
Rivers and their flooded alluvial plains integrate physical, biological, and human processes at the scale of continents. Despite their ecological and economic values, these complex ecosystems are poorly understood and highly modified by humans. A primary problem is that most research in fluvial ecosystems has been conducted in small streams and then scaled up to rivers. Furthermore, the point where a stream transitions into a river is not well understood. Although many conceptual models exist, the role that large river–floodplain complexes play within these frameworks is lacking. These models focus on flooding as a temporary reset to river ecosystems, but floodplains and rivers may continue to interact long after floodwaters recede. We revisit the concept of the riverscape, a unique mosaic of perennially interacting wetland and channel habitats that have unique ecological properties during both non-flood and flooding periods relative to the small tributary streams within the riverscape network. This strong bidirectional interaction within low-lying alluvial plains may define large rivers. To determine whether a riverscape is indeed a useful unit of study for river ecology, conservation, and restoration, baseline conditions with measurable, comparable metrics, such as primary and secondary production need to be established. Responses of these metrics to multiple stressors and restoration such as levee setbacks, wetland mitigation, and dam removals will inform both basic models of riverscape function and future management actions. Because humans currently affect nearly all aspects of the environmental structure and function of riverscapes, human perceptions of riverscape value and threat need to be considered as a fundamental component of riverscape ecology.
Introduction
Despite the proximity of rivers and their associated alluvial plains to just about every human on Earth, they continue to be one of the world's least understood coupled ecosystems (Thorp et al., 2023). This is puzzling given the important roles that complex river landscapes play for people. Rivers serve urban and agricultural needs, with human water uses including irrigation, hydropower, and manufacturing placing severe stress on flows globally (Jägermeyr et al., 2017). Rivers convey waste away from human settlements, but at the cost of reducing water quality within rivers and adjacent wetlands. This problem is especially severe given that up to 80% of global wastewater is untreated, because the infrastructure and energy cost of water treatment is prohibitive for most countries (Koncagül et al., 2021). The economic value of rivers and their wetlands is hard to define, with considerable variability in valuations (Wilson and Carpenter, 1999; De Groot et al., 2012). Fisheries services of the impounded Upper Mississippi River alone are estimated at USD$ 1.3 billion (Schramm, 2017). It is clear that, without access to flowing surface waters, human welfare will suffer and economic activity will decline; therefore, maintaining river flow regimes is critical (Jorda-Capdevila and Rodriguez-Labajos, 2017). That said, rivers are typically treated with what seems to be malicious intent. Humans have transformed river landscapes in many ways with little regard for their impact on river biodiversity and ecosystem functions and services, including maintaining connectivity to floodplain ecosystems (Tockner and Stanford, 2002; Morrison et al., 2023). Across the world, river channels have been isolated by levees with human development occurring on floodplains, which typically makes the risks and damage from flooding worse (Knox et al., 2022). Flooding, which is a natural process contributing to river ecosystem structure and function (Humphries et al., 2014), is considered by many humans as a costly, dangerous, and maligned threat to be controlled and mitigated. Rivers and their floodplains clearly have a complicated relationship with humanity, requiring sound science to guide education, capabilities to make decisions, and the ability to implement policies that positively affect conservation, management, and restoration, and ultimately human health, welfare, and economic gain (Bayley, 1995).
Search for a conceptual river model
Relative to small streams, large rivers are not well understood. The sheer complexity of rivers makes them difficult to define. In fact, no real consensus exists about what makes up a river relative to a smaller stream, because no topological system defining stream or river order is perfect. Although a river may be defined as a Strahler-type fifth-order or larger stream, the discharge, length, watershed size, permanence, and many other characteristics of two streams joining with each other to make a river downstream vary tremendously. Some rivers may be quite large in base flow while others convey far less water than a stream in other watersheds. Although many rivers are naturally permanent, others have permanent flow created by human damming, wastewater discharges, or other engineering. Rivers that are intermittent, many of which still have subsurface flow when their beds are dry, are important components of regional ecosystems that need perennial protection and management (Datry et al., 2014; Acuna et al., 2017; Messager et al., 2021). Over long timescales, dry riverbeds are actually dynamic, geomorphic, and ecological places even though they defy the short-term perceptions of humans. Although floodplains are typically described as being separate from the river channel, in actuality, floodplains are a natural part of the river landscape, being connected hydrologically and biologically even when the river is constrained within its banks or trapped between levees (Roni et al., 2019).
River science has yet to find a unifying theory that reliably predicts ecological processes and structure, especially for large rivers and their floodplains (Palmer and Ruhi, 2019). This is important not only from a basic science perspective but also for setting expectations for applied assessments of river health and restoration projects. Perhaps the closest source of agreement among river scientists is that streams and rivers are shaped by the physical and biological characteristics of their watersheds rather than rivers carving out their own valleys (Hynes, 1975). A river integrates all the activities occurring in its watershed. Over the past several decades, freshwater scientists have searched for generalities about stream and river ecosystem productivity and community structure. The River Continuum Concept predicted that the upstream–downstream processes of organic matter production and transport shape productivity and community structure (Vannote et al., 1980). This was followed by the flood pulse hypothesis that considered how spates of lateral connectivity to floodplains affect river ecosystems (Junk et al., 1989). In rivers that are separated longitudinally by either natural or human-made barriers, repeating areas of flow variation both within the channel and onto floodplains may be key (Ward and Stanford, 1995). River flow can be considered as waves with different frequencies and amplitudes that shape river ecosystems (River Wave Concept) (Humphries et al., 2014). The Stream Biome Concept predicts that the biogeographical, continental-scale context by which a stream network is nested influences its ecosystem structure and function (Dodds et al., 2015). Given the fractal nature of river networks, repeating units of erosion and deposition that increase in spatial scale downstream hint that predictable ecological patterns exist (i.e., the River Ecosystem Synthesis, Thorp et al., 2006). As a further refinement, the network position hypothesis posits that ecosystems within-river segments are driven by the patterns of complexities of the river network in which they are contained (Brown and Swan, 2010). Thus, the current state of river science holds that ecological processes in rivers depend to some degree not only on location along the fluvial network (i.e., the Network Position Hypothesis; Melles et al., 2012) but also on disequilibrium dynamics such as the frequency of drying and flooding that occur along the river channel, its floodplain, and groundwater sources (Junk et al., 1989; Ward and Stanford, 1995). Rivers continue to have unique qualities that defy our ability to accurately and precisely categorize them into patches that can be termed functional process zones (FPZs) via the river ecosystem synthesis (Thorp et al., 2006). A primary goal for river science is to identify and bound the micro- and macro-scale constraints and emergent properties within FPZs that allow us to understand how rivers work and can be protected or restored as unique units. River research must identify the important metrics of these FPZs, which can be challenging in these large and dynamic ecosystems (Thorp et al., 2023).
Finding the right scales in time and space by which river networks can be categorized is important not only for testing general ecological hypotheses but also for defining units for river management (e.g., providing a desired function), conservation (e.g., protecting a function), and restoration (e.g., reviving a historical function) (Figure 1). Protecting entire river networks from their headwater streams to their deltas in lakes or oceans is an ideal goal but realistically impossible given the sheer geographical extent of many of these ecosystems, often stretching across continents and certainly conflicting with people. Ecologists have generally accepted that ecosystems are hierarchical in nature, where broader factors such as an entire lake ecosystem constrains the specific communities within it. Each community constrains its component populations through food web interactions and other biotic processes. Scaling up in the other direction, characteristics of the component populations and community “parts” translate to a categorical set of manageable emergent properties of the lake ecosystem such as the lake's ability to store or emit carbon. However, rivers are different than lakes and other ecosystems in that classical nested hierarchical relationships do not apply (Melles et al., 2012). Following the directional network from upstream to downstream, the downstream river is influenced by the smaller streams that feed it. However, the river typically does not constrain its component streams, or its entire watershed for that matter, meaning that few feedback mechanisms exist to reduce variability in responses of rivers to upstream effects. As a notable exception, barriers within rivers such as natural or human-made dams or pollutants that make the river impassable to organisms may constrain upstream processes (Turner, 2022). Identifying predictable, repeatable ecological and management units for rivers is a difficult challenge. Each river is a unique entity driven by a set of characteristics ranging from continental/geological scales to the unique zoogeographic and floral composition of its locale (Frissell et al., 1986; Dodds et al., 2015).
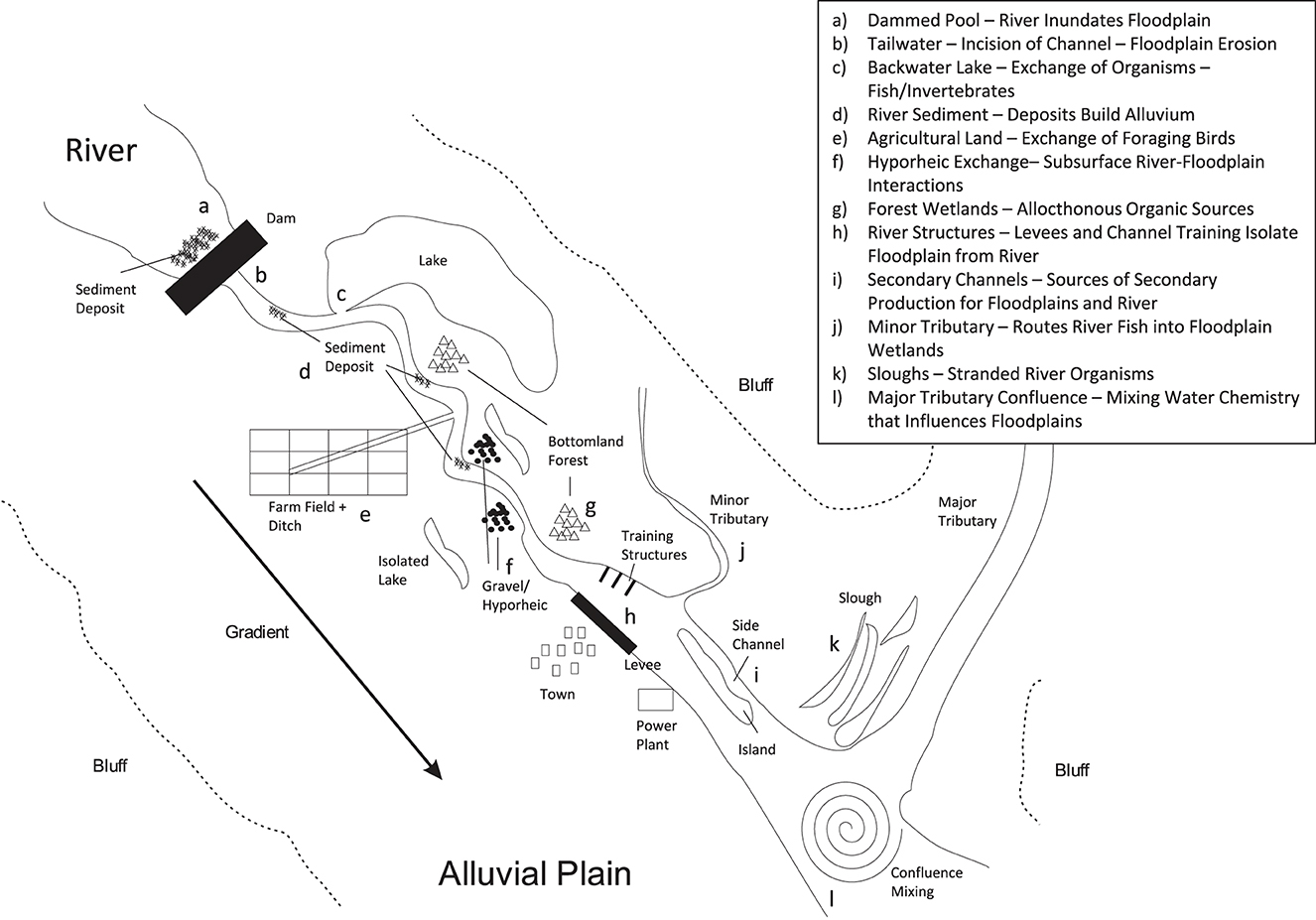
Figure 1. Complex mosaic of a riverscape below the channel's bankfull stage, where the alluvial plain and river channel continue to interact between flooding events. Examples of bidirectional interactions: (a) Above dams, the floodplain is perennially inundated by the river channel. (b) Below the dam within the tailwater, sediment-starved water in the river channel erodes the surrounding floodplain. (c) Backwater lakes within floodplains receive dispersing/migrating main channel fishes. Plankton from the lake drifts into the main channel. (d) Sedimentary materials collect within inside bends to build extra-channel areas. (e) Irrigated agricultural areas drained by ditches allow materials, nutrients, and organisms to exchange across the riverscape. (f) Subsurface flow of water, materials, chemicals, and hyporheic organisms occur between the river and the alluvial plain. (g) Bottomland forests exchange organisms and the source of perennial organic matter for river. (h) Levees and channel-training structures confine the river and starve the floodplain of river-derived materials. (i) Secondary/braided channels within the river landscape produce invertebrates and fish that are consumed by floodplain organisms such as river otters. (j) Minor tributaries convey fish and other organisms between floodplains and the river. (k) Slough areas adjacent to river channels strand river organisms and contribute to wetland production. (l) Major tributaries contribute nutrients, organic matter, and sediments that build alluvial plains downstream.
One issue with all river models searching for constrained, manageable units or FPZs throughout river networks is a strong focus on within-river processes, with the alluvial floodplain or riparian corridor considered as a temporally isolated source of materials rather than a co-equal component that is shaped by the river's behavior both during flooding and also during the remainder of the year (Tockner et al., 2000). In other words, the floodplain is considered as an independent, constraining driver of river structure and function. Perhaps this approach by ecologists is because of Hynes (1975) idea that the floodplain makes the river, which is true in a broadly physical sense as factors such as drainage, bedload, and slope affect the river's flow and geomorphology. However, from an ecological perspective, it may be more instructive to consider a bidirectional interaction. Biological components, scaling across the river and its floodplain mosaic from biogeochemistry to entire organisms, interact in complex ways during dry and wet periods (Ward and Tockner, 2001). Regardless of whether one central, all-encompassing hypothesis will ever be arrived at, understanding how rivers work and setting expectations for their care is going to require continued collaborative research among biologists, hydrologists, and geographers that embraces some key commonalities described in this review and an understanding of the complex roles of the floodplain, especially when humans are often in direct competition with the river for this important resource.
A key role of river scientists is to improve our understanding of the critical connections between rivers and floodplains and better convey what defines a river. Perhaps a river occurs when a network of streams combines into a broad, measurable landscape of flowing surface water and hyporheic wetted conditions that extends between bluffs (Figure 1). For a river, lateral processes of its alluvial landscape become significant in driving patterns of biodiversity and ecosystem production during both dry and flooding periods. Quantifying an ecological shift from a constrained stream with its ecosystem driven by upstream flow and temporary spates of flooding to a river ecosystem that is dominated by its complex alluvial landscape throughout the year is less the point than determining opportunities for conservation and management that bridges river and wetland ecology. This is not a new notion. Leopold and Marchand (1968) coined the term riverscape as a contraction of the terms “river” and “landscape” to identify a river's uniqueness in an aesthetic sense. This spatially explicit approach has been adopted by landscape ecologists working in river restoration (Wiens, 2002) and can be used to quantitatively consider perennial, bidirectional physical and biological river–wetland exchanges of surface and subsurface water, microbes, meiofauna, plankton, labile nutrients, decaying organic matter, and living macroorganisms such as dispersing insect larvae, circulating plankton, migrating fish, and flying birds, bats, and insects. A riverscape is defined henceforth as the complex mosaic of conditions created by river channels and their floodplains, with varying upstream influence (Torgersen et al., 2022). Coastal riverscapes also are influenced by downstream factors such as tidal intrusion and coastal wetlands. Riverscapes might be unique from streams in that they have proportionally higher local driving factors such as connected wetlands, significant groundwater, and high local autotrophic production driven by their wetlands while being influenced by intense, temporally variable spates of flooding and, in the case of rivers, feeding into deltas, estuaries, or embayments, and saltwater or lakewater inundation. In the Danube River's riverscape, Tockner et al. (1999) quantified the exchanges of organic materials and nutrients between the floodplain and the main channel, showing gradual changes in connectivity.
Meeting human needs while maintaining the multiple ecological functions of riverscapes requires sound science to help guide decision making by policymakers, river engineers, and natural resource managers. Because riverscapes rarely are confined within one policy jurisdiction as they cross states and countries upstream–downstream as well as laterally across floodplains (Tripp et al., 2019), their global importance needs to be clearly understood. This will require determining how local and regional processes and human actions within jurisdictions throughout the riverscape network contribute to the global commons, with implications not only for global biodiversity but also for life support for all humans on Earth. The goal of this review is to provide a non-exhaustive list of research priorities for riverscapes that may improve our understanding of basic ecological processes within rivers and also set expectations for ecological integrity and function by which human actions can be assessed and mitigated.
A general approach
Whether or not generalities can be applied universally to the study of riverscapes and their care by humans, the application of scientific principles that we do understand combined with brute force empirical approaches and an embrace of human social sciences are needed to tackle the global freshwater crisis humans are currently facing (Tickner et al., 2020). One of the most important goals of scientists should be to identify the key emergent properties of these complex human–ecological coupled systems as the world's climate and human water demands collide in the coming century (Figure 2). Biodiversity and the ecosystem production on which it depends are clearly two contenders (Palmer and Ruhi, 2019), but many other processes such as nutrient abatement, atmospheric gas exchange, and global hydrological cycling are critical services that riverscapes provide that are still not well understood. For example, the nutrient transport of rivers can be quantified in a simple way and then linked to complex outcomes such as algal blooms within rivers and in the estuarine and coastal ecosystems in which they spill.
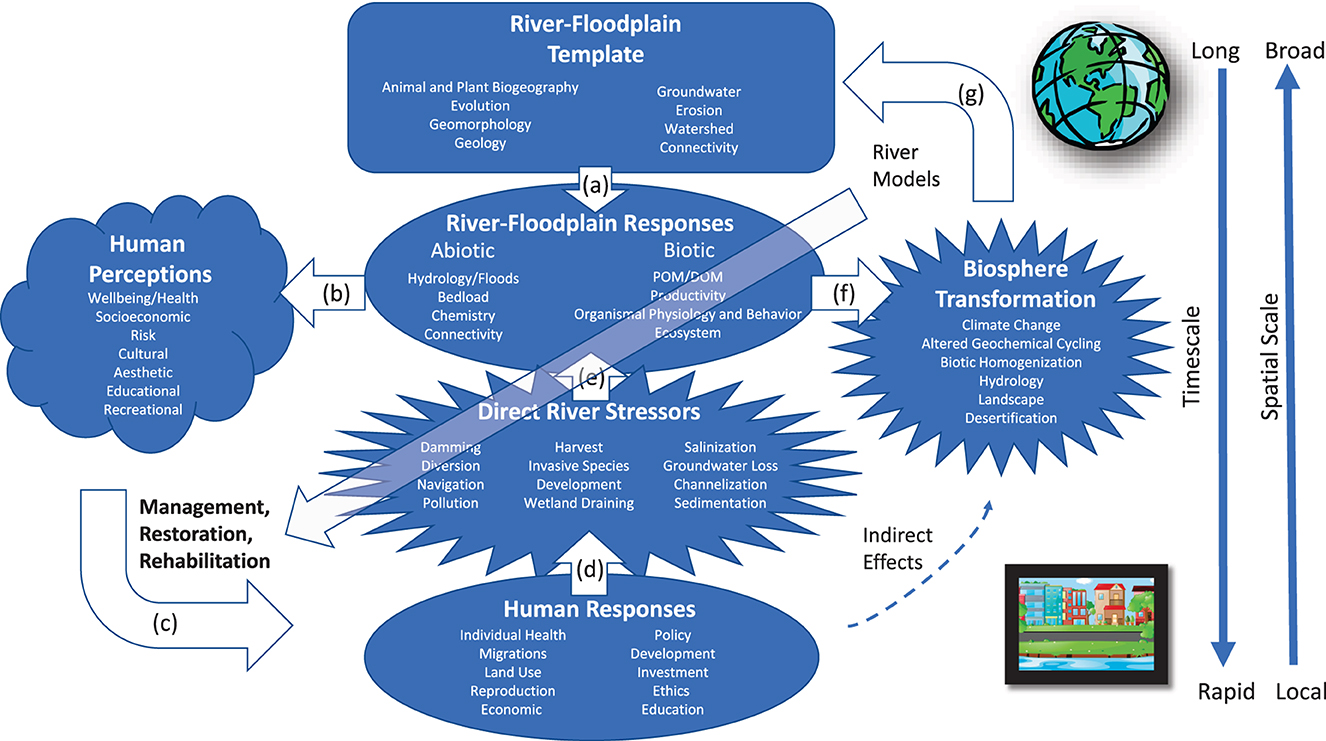
Figure 2. Framework for research in riverscape ecology. (a) The river and its floodplain ecosystems (i.e., riverscape) are driven by long-term (i.e., scale of centuries and continents) processes that affect contemporary ecological responses. (b) The condition and physical behavior of the riverscape (e.g., flooding intensity and fish production) influence human perceptions. (c) Human perceptions of the river lead to patterns of use, abuse, and mitigation. (d) Positive and negative human responses such as dam building and wetland restoration either increase or decrease the effects of multiple stressors, typically at short time scales. (e) Stressors and their alleviation directly feedback to riverscape responses. (f) Responses of the riverscape to the changing riverscape template and human effects contribute to patterns of biosphere transformation, which then (g) feeds back to the riverscape template. Humans indirectly affect rivers through biosphere transformation that further changes the riverscape template. River models (upper right-hand corner) typically focus on interactions among the riverscape template, the riverscape ecological responses, and its contribution to the biosphere. Human actions typically focus on perceptions (bottom left-hand corner). Conceptual models of the riverscape function need to be better linked to human responses and activities (diagonal arrow).
Because of the complexity of riverscapes, setting reference baseline conditions is challenging (Figure 2). Without perfect controls, understanding and classifying rivers requires a set of clear expectations for sorting through natural and human-induced effects. Ideally, identifying intact model riverscapes with sufficient similarities can be used to set expectations (Urbanic et al., 2021). Historical data from a variety of sources such as archaeological sites and museum collections can allow scientists to reconstruct past conditions to some degree (Barak et al., 2016). Cultural recollections can also be useful (Knopp et al., 2022). The biological traits of native communities may be used to set reference expectations for biodiversity (Verberk et al., 2013; Lima et al., 2017; Alahuhta et al., 2019). Historical geomorphological changes can provide predictions for future physical changes (Grabowski et al., 2014). Returning riverscapes to historical conditions may be particularly challenging or impossible given the rapid changes occurring in underlying baseline biological and physical processes (Death et al., 2015). The very basis of energy for river food webs may be changing. As atmospheric carbon enrichment of the planet continues to increase, the composition and quantity of organic matter in rivers is likely going to change (Kominoski and Rosemond, 2012). This may reduce food quality for higher trophic levels as the stoichiometric content of nitrogen and other more limiting nutrients declines relative to increasing carbon. Given changes in geomorphology, climate, and even basal resources, the riverscapes of the past may no longer be attainable.
Riverscape responses to changes in baseline conditions and human-induced stressors can be measured in many ways. Although much work has been conducted on estimating primary production and organic matter processing in riverscapes (Palmer and Ruhi, 2019), secondary production estimates in flowing water and the floodplain and associated quantitative energy flow webs that can be constructed with them are some of the most comprehensive ways to examine ecosystem structure and function (Benke and Huryn, 2017; Whiles and Patrick, 2022) (Figure 2). While the number of community secondary production studies in streams has increased over the last decade, there is still a major gap in the literature for large riverscapes. Patrick et al. (2019) assembled a database of 152 community secondary production estimates for streams and found only four studies of systems that were of eighth order or higher (~3% of all studies). This is, of course, related to the logistical challenges of sampling larger systems across multiple habitat types (e.g., main channel vs. oxbow). Nonetheless, creative sampling and analytical approaches may help address this gap, as was employed in a secondary production and energy flow study of the Colorado River (Cross et al., 2013). Others, such as Benke and Wallace (2015) have simply focused on the most productive habitats in larger systems, such as snags, rather than trying to examine the whole riverscape. Further studies of secondary production and energy flow dynamics in riverscapes, particularly those that examine energetic linkages between the channel and floodplain, will greatly enhance our understanding of these complex systems, and thus our ability to manage and restore them.
A contemporary riverscape is perceived by humans through the river's physical and biological services and threats (Flotemersch and Aho, 2021), which feeds back to human actions that either lead to improvements or more likely increase damage (Rohde et al., 2006) (Figure 2). For example, flood events cause humans to build levees. Levees actually increase flood damage when they inevitably fail, which prompts pressure to build bigger levees (Knox et al., 2022). Social research that informs managers about ways to quantify and evaluate human responses to the costs and benefits of functioning riverscapes is critical (Jorda-Capdevila and Rodriguez-Labajos, 2017; Basak et al., 2021). There are many ways to assign value to riverscapes, with money being one of the primary endpoints (Balasubramanian, 2019). Much of the biological value of riverscapes is below the surface and difficult for people to see; rivers are often inaccessible for recreational or cultural use, and ecosystem benefits such as nutrient abatement are hard to perceive until the problem is nearly unfixable. Thus, science must inform the public about the hidden values of riverscapes to break negative cycles of use and abuse.
So many human-caused stressors are affecting riverscapes simultaneously that it is impossible to consider the effect of each in isolation (Ormerod et al., 2010; Perujo et al., 2021) (Figure 2). Dams are one of the largest causes of stress, given their ubiquity (Belletti et al., 2020). However, a review of global flow regimes revealed that the effects of dams in large rivers were less influential than expected (Brown et al., 2023). Invasive species, pollutants, sedimentation, and many other stressors are involved and interacting, and when coupled with the changing baselines caused by climate change, the task of mitigating them is onerous (Ormerod et al., 2010). Using biological indicators coupled with mechanistic empirical research will allow investigators to assess contemporary conditions and, importantly, to assess how riverscapes respond to regulations and other actions that remove the stressors. For example, quantifying how indicators respond following a major restoration project such as dam removal will help scientists understand their utility for long-term assessments of riverscape condition (Stanley and Doyle, 2003; Bellmore et al., 2017). Unfortunately, assessments of restorations are rare (Bellmore et al., 2017), and despite the billions of dollars spent on restoration projects globally, there is no consensus on what constitutes successful riverscape restoration (Palmer and Ruhi, 2019).
Research directions
Effective riverscape research needs to be placed in the broad context of factors affecting baseline reference conditions while recognizing complex interactions with humans (Brierley and Fryirs, 2022) and setting realistic expectations for the risks and benefits set by the geomorphic and biotic template (Brierley and Fryirs, 2022) (Figure 1). This involves creating and sustaining collaborations among physical, social, and biological scientists to identify the most efficacious paths for maintaining riverscape function in the face of direct anthropogenic factors as well as changing climate (Torgersen et al., 2022). One particularly good example of this approach is the management of the Upper Mississippi River (UMR) ecosystem, where long-term government support for maintaining ecological integrity is a result of shared stewardship among stakeholders (Garvey et al., 2010; Sparks, 2010; Bouska et al., 2019). The Habitat Rehabilitation and Enhancement Project (HREP) in the UMR is directed by these stakeholders to create large restorations. One notable HREP example is the Emiquon Reserve Program in the Illinois UMR where a large floodplain wetland has been restored for native fishes, vegetation, and waterfowl (Lemke et al., 2017). Continuous, long-term datasets in the UMR that have been collected for >3 decades allow scientists to tease apart the relative impacts of baseline creep vs. human actions such as large restoration projects like HREP (Lemke et al., 2017) on the river ecosystem. Similar long-term assessments occur across the world. That said, river scientists appear to be siloed across continents (Wei and Wu, 2022), and advances in this area would benefit from more global collaboration involving sharing experiences and approaches within unique riverscapes.
Considerable metrics exist for quantifying riverscape condition and testing hypotheses about structure and function (Table 1). Scientists working with multiple interacting stressors focus on developing assessment tools such as biological indices that should correlate with reference expectations (Statzner and Beche, 2010; Noges et al., 2016; Wu et al., 2017; Lemm et al., 2019). Ecosystem expectations and roles of large riverscapes, including expected patterns of carbon fixation, organic matter processing, biomass production, metabolism, nutrient transformation, and gas exchange are still unknown relative to those in lakes, small streams, and wetlands (Palmer and Ruhi, 2019; Battin et al., 2023; McInerney et al., 2023). Linking physical processes, organisms, and ecosystem responses should improve the development of effective indicators (McInerney et al., 2023) as well as inform researchers about how riverscapes contribute to global processes such as climate change and respond to local conditions. Using direct estimates of whole-ecosystem secondary production as a directly comparable indicator of riverscape function is another important approach (Cross et al., 2013). Taking advantage of rare, large-scale flooding events can provide unique learning opportunities (Phelps et al., 2015; Rantala et al., 2016). A massive flood in 2011 forced the US government to inundate a 55,000-ha floodplain of the Mississippi River that had been disconnected for more than 70 years, showing that this agriculturally dominated former wetland was still able to mitigate a nitrogen flux from the river (Rantala et al., 2016). Linking data from remote sensing with physical characteristics (e.g., soil type, chlorophyll a) of the riverscape will allow scientists to link patch dynamics within riverscape networks to processes occurring at continental and global scales (Piégay et al., 2020).
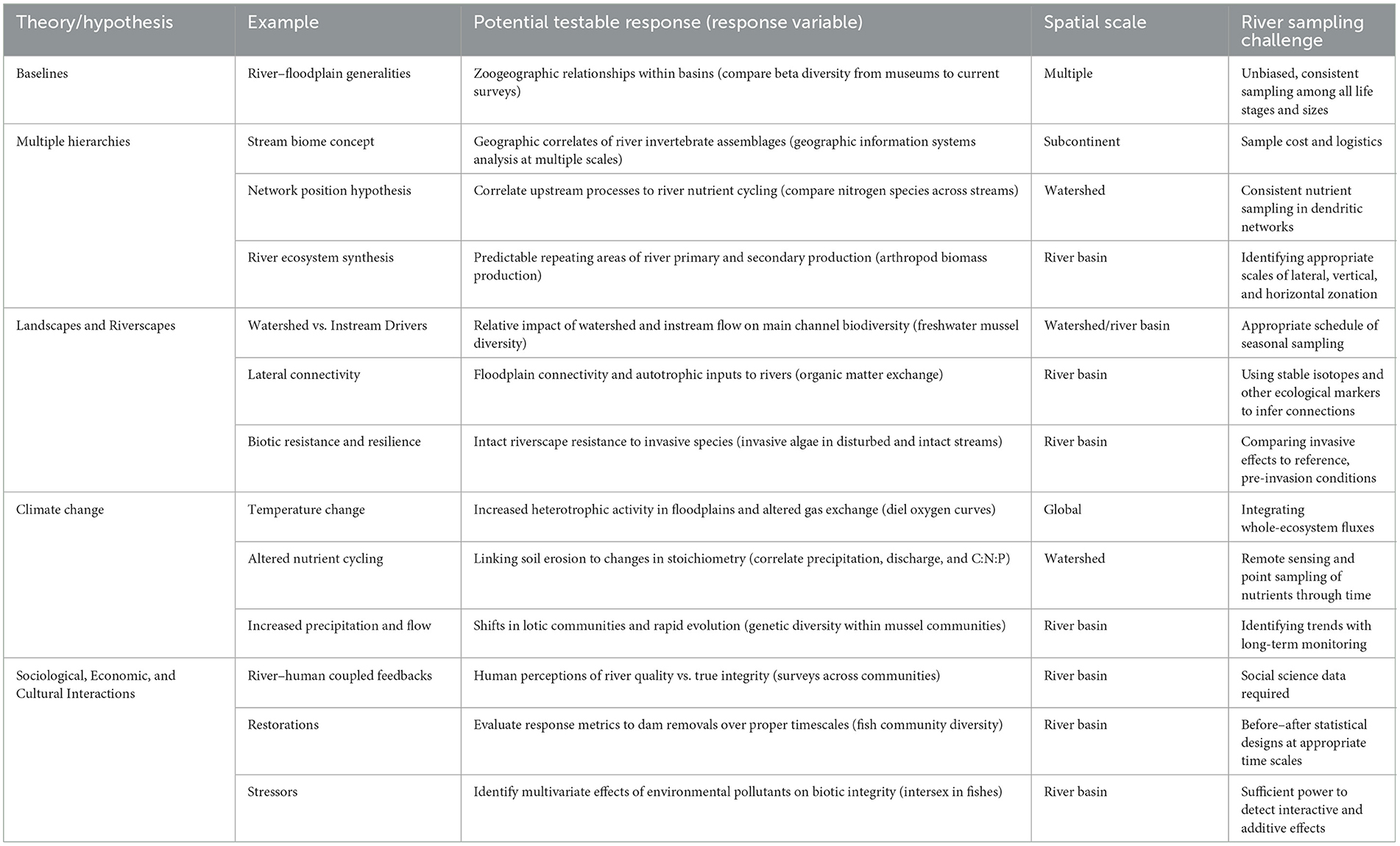
Table 1. Potential research directions for riverscape ecology as a function of identifying and setting appropriate baselines, developing guiding conceptual, hierarchical models, finding appropriate spatial scales of study and conservation, forecasting responses to environmental change, and understanding human responses.
River policy and management need to avoid treating river channels and their floodplains as occasionally connected entities that may require different management and conservation actions. Many floodplains are isolated from rivers to manage wildlife such as waterfowl or promote production of crops such as rice. Although this is among one of many uses of riverscapes, baseline patches for setting conservation or restoration goals will require facilitating bidirectional floodplain connectivity within the riverscape (Rood et al., 2005; Rohde et al., 2006; Roni et al., 2019). When an entire floodplain cannot be reconnected, setbacks of levees might be an option. As riverscape restorations occur, it is critical that spatially explicit assessments are done in ways that inform broader science rather than focusing on local responses of targeted organisms (Roni et al., 2019). Understanding mechanisms that foster novel predictions within the context of the riverscape in its network will lead to new, testable hypotheses and models. Assessments need to be well funded, long-term, and appropriately designed rather than be considered an afterthought.
Conclusions
Nearly a half billion humans on Earth do not have access at any time to a reliable source of freshwater (Kattel, 2019). Given seasonal variability in water scarcity, ~4 billion people suffer from water scarcity at least during some of the year (Koncagül et al., 2021). As the human global population climbs, pressure on surface and groundwater sources associated with riverscapes will increase substantially. Riverscape science will be required to provide information for action, with pressure to compromise between human and ecological needs as cycles of drought and flooding become more severe across much of the planet (Kattel, 2019). This is not just a problem of thirst in poorer countries. Increasing flood risks and a lack of water supplies suppress development and economic activity. As Bayley (1995) noted nearly three decades ago, rather than complaining about the heat as the house burns, riverscape scientists need to develop a consensus about research approaches in river–floodplain ecology, network effectively, and use lessons learned from current restoration and conservation projects to guide policy and actions at large spatial scales.
Author contributions
JG: Conceptualization, Writing – original draft. MW: Conceptualization, Writing – original draft.
Funding
The author(s) declare that no financial support was received for the research, authorship, and/or publication of this article.
Conflict of interest
The authors declare that the research was conducted in the absence of any commercial or financial relationships that could be construed as a potential conflict of interest.
The authors JG and MW declared that they were an editorial board member of Frontiers, at the time of submission. This had no impact on the peer review process and the final decision.
Publisher's note
All claims expressed in this article are solely those of the authors and do not necessarily represent those of their affiliated organizations, or those of the publisher, the editors and the reviewers. Any product that may be evaluated in this article, or claim that may be made by its manufacturer, is not guaranteed or endorsed by the publisher.
References
Acuna, V., Hunter, M., and Ruhi, A. (2017). Managing temporary streams and rivers as unique rather than second-class ecosystems. Biol. Conserv. 211, 12–19. doi: 10.1016/j.biocon.2016.12.025
Alahuhta, J., Eros, T., Kärnä, O. M., Soininen, J., Wang, J., and Heino, J. (2019). Understanding environmental change through the lens of trait-based, functional, and phylogenetic biodiversity in freshwater ecosystems. Environ. Rev. 27, 263–273. doi: 10.1139/er-2018-0071
Balasubramanian, M. (2019). Economic value of regulating ecosystem services: a comprehensive at the global level review. Environ. Monitor. Assessm. 191, 10. doi: 10.1007/s10661-019-7758-8
Barak, R. S., Hipp, A. L., Cavender-Bares, J., Pearse, W. D., Hotchkiss, S. C., and Lynch, E. A. (2016). Taking the long view: integrating recorded, archeological, paleoecological, and evolutionary data into ecological restoration. Int. J. Plant Sci. 177, 90–102. doi: 10.1086/683394
Basak, S. M., Hossain, M. S., Tusznio, J., and Grodzinska-Jurczak, M. (2021). Social benefits of river restoration from ecosystem services perspective: a systematic review. Environ. Sci. Policy 124, 90–100. doi: 10.1016/j.envsci.2021.06.005
Battin, T. J., Lauerwald, R., Bernhardt, E. S., Bertuzzo, E., Gener, L. G., Hall, R. O., et al. (2023). River ecosystem metabolism and carbon biogeochemistry in a changing world. Nature 613, 449–459. doi: 10.1038/s41586-022-05500-8
Bayley, P. B. (1995). Understanding large river floodplain ecosystems. Bioscience 45, 153–158. doi: 10.2307/1312554
Belletti, B., Garcia de Leaniz, C., Jones, J., Bizzi, S., Börger, L., Segura, G., et al. (2020). More than one million barriers fragment Europe's rivers. Nature 588, 436. doi: 10.1038/s41586-020-3005-2
Bellmore, R. J., Duda, J. J., Craig, L. S., Greene, S. L., Torgersen, C. E., Collins, M. J., et al. (2017). Status and trends of dam removal research in the United States. Wiley Interdis. Rev. Water 4, e1164. doi: 10.1002/wat2.1164
Benke, A. C., and Huryn, A. D. (2017). Secondary Production and Quantitative Food Webs. Cambridge, MA: Academic Press.
Benke, A. C., and Wallace, J. B. (2015). High secondary production in a coastal plain river is dominated by snag invertebrates and fuelled mainly by amorphous detritus. Freshwater Biol. 60, 236–255. doi: 10.1111/fwb.12460
Bouska, K. L., Houser, J. N., Jager, N. R. D., Van Appledorn, M., and Rogala, J. T. (2019). Applying concepts of general resilience to large river ecosystems: a case study from the upper Mississippi and Illinois rivers. Ecol. Ind. 101, 1094–1110. doi: 10.1016/j.ecolind.2019.02.002
Brierley, G., and Fryirs, K. (2022). Truths of the riverscape: moving beyond command-and-control to geomorphologically informed nature-based river management. Geosci. Lett. 9, 1–5. doi: 10.1186/s40562-022-00223-0
Brown, B. C., Fullerton, A. H., Kopp, D., Tromboni, F., Shogren, A. J., Webb, J. A., et al. (2023). The music of rivers: the mathematics of waves reveals global structure and drivers of streamflow regime. Water Res. Res. 59, e2023WR034484. doi: 10.1029/2023WR034484
Brown, B. L., and Swan, C. M. (2010). Dendritic network structure constrains metacommunity properties in riverine ecosystems. J. Anim. Ecol. 79, 571–580. doi: 10.1111/j.1365-2656.2010.01668.x
Cross, W. F., Baxter, C. V., Rosi-Marshall, E. J., Hall Jr, R. O., Kennedy, T. A., Donner, K. C., et al. (2013). Food-web dynamics in a large river discontinuum. Ecol. Monograph. 83, 311–337. doi: 10.1890/12-1727.1
Datry, T., Larned, S. T., and Tockner, K. (2014). Intermittent rivers: a challenge for freshwater ecology. Bioscience 64, 229–235. doi: 10.1093/biosci/bit027
De Groot, R., Brander, L., Van Der Ploeg, S., Costanza, R., Bernard, F., Braat, L., et al. (2012). Global estimates of the value of ecosystems and their services in monetary units. Ecosyst. Serv. 1, 50–61. doi: 10.1016/j.ecoser.2012.07.005
Death, R. G., Fuller, I. C., and Macklin, M. G. (2015). Resetting the river template: the potential for climate-related extreme floods to transform river geomorphology and ecology. Freshwater Biol. 60, 2477–2496. doi: 10.1111/fwb.12639
Dodds, W. K., Gido, K., Whiles, M. R., Daniels, M. D., and Grudzinski, B. P. (2015). The stream biome gradient concept: factors controlling lotic systems across broad biogeographic scales. Freshwater Sci. 34, 1–19. doi: 10.1086/679756
Flotemersch, J., and Aho, K. (2021). Factors influencing perceptions of aquatic ecosystems. Ambio 50, 425–435. doi: 10.1007/s13280-020-01358-0
Frissell, C. A., Liss, W. J., Warren, C. E., and Hurley, M. D. (1986). A hierarchical framework for stream habitat classification - viewing streams in a watershed context. Environ. Manage. 10, 199–214. doi: 10.1007/BF01867358
Garvey, J., Ickes, B., and Zigler, S. (2010). Challenges in merging fisheries research and management: the upper Mississippi river experience. Hydrobiologia 640, 125–144. doi: 10.1007/s10750-009-0061-x
Grabowski, R. C., Surian, N., and Gurnell, A. M. (2014). Characterizing geomorphological change to support sustainable river restoration and management. Wiley Interdiscip. Rev. Water 1, 483–512. doi: 10.1002/wat2.1037
Humphries, P., Keckeis, H., and Finlayson, B. (2014). The river wave concept: integrating river ecosystem models. Bioscience 64, 870–882. doi: 10.1093/biosci/biu130
Hynes, H. B. N. (1975). The stream and its valley. SIL Proc. 19, 1–15. doi: 10.1080/03680770.1974.11896033
Jägermeyr, J., Pastor, A., Biemans, H., and Gerten, D. (2017). Reconciling irrigated food production with environmental flows for sustainable development goals implementation. Nat. Commun. 8, 15900. doi: 10.1038/ncomms15900
Jorda-Capdevila, D., and Rodriguez-Labajos, B. (2017). Socioeconomic value(s) of restoring environmental flows: systematic review and guidance for assessment. River Res. Appl. 33, 305–320. doi: 10.1002/rra.3074
Junk, W., Bayley, P. B., and Sparks, R. E. (1989). “The flood pulse concept in river-floodplain systems,” in Proceedings of the International Large River Symposium (LARS), ed D. P. Dodge (Canberra, ACT: Canadian Special Publication of Fisheries and Aquatic Sciences), 106.
Kattel, G. R. (2019). State of future water regimes in the world's river basins: balancing the water between society and nature. Critic. Rev. Environ. Sci. Technol. 49, 1107–1133. doi: 10.1080/10643389.2019.1579621
Knopp, J. A., Levenstein, B., Watson, A., Ivanova, I., and Lento, J. (2022). Systematic review of documented indigenous knowledge of freshwater biodiversity in the circumpolar arctic. Freshwater Biol. 67, 194–209. doi: 10.1111/fwb.13570
Knox, R. L., Wohl, E. E., and Morrison, R. R. (2022). Levees don't protect, they disconnect: a critical review of how artificial levees impact floodplain functions. Sci. Total Environ. 837, 155773. doi: 10.1016/j.scitotenv.2022.155773
Kominoski, J. S., and Rosemond, A. D. (2012). Conservation from the bottom up: forecasting effects of global change on dynamics of organic matter and management needs for river networks. Freshwater Sci. 31, 51–68. doi: 10.1899/10-160.1
Koncagül, E., Tran, M., and Connor, R. (2021). The United Development Report 2021: Valuing Water; Facts and Figures. UNESCO World Water Assessment Programme, Perugia, Italy. Available online at: https://digitallibrary.un.org/record/3905488
Lemke, M. J., Walk, J. W., Lemke, A. M., Sparks, R. E., and Blodgett, K. D. (2017). Introduction: the ecology of a river floodplain and the Emiquon preserve. Hydrobiologia 804, 1–17. doi: 10.1007/s10750-017-3335-8
Lemm, J. U., Feld, C. K., and Birk, S. (2019). Diagnosing the causes of river deterioration using stressor-specific metrics. Sci. Total Environ. 651, 1105–1113. doi: 10.1016/j.scitotenv.2018.09.157
Leopold, L. B., and Marchand, M. O. (1968). On quantitative inventory of riverscape. Water Resour. Res. 4, 709. doi: 10.1029/WR004i004p00709
Lima, A. C., Wrona, F. J., and Soares, A. (2017). Fish traits as an alternative tool for the assessment of impacted rivers. Rev. Fish Biol. Fisheries 27, 31–42. doi: 10.1007/s11160-016-9446-x
McInerney, P. J., Giling, D. P., Wolfenden, B., and Sengupta, A. (2023). A synthesis of floodplain aquatic ecosystem metabolism and carbon flux using causal criteria analysis. Limnol. Oceanography 68, 97–109. doi: 10.1002/lno.12253
Melles, S. J., Jones, N. E., and Schmidt, B. (2012). Review of theoretical developments in stream ecology and their influence on stream classification and conservation planning. Freshwater Biol. 57, 415–434. doi: 10.1111/j.1365-2427.2011.02716.x
Messager, M. L., Lehner, B., Cockburn, C., Lamouroux, N., Pella, H., Snelder, T., et al. (2021). Global prevalence of non-perennial rivers and streams. Nature 594, 391–397. doi: 10.1038/s41586-021-03565-5
Morrison, R. R., Simonson, K., McManamay, R. A., and Carver, D. (2023). Degradation of floodplain integrity within the contiguous United States. Commun. Earth Enviro. 4, 1–4. doi: 10.1038/s43247-023-00877-4
Noges, P., Argillier, C., Borja, Á., Garmendia, J. M., Hanganu, J., Kodeš, V., et al. (2016). Quantified biotic and abiotic responses to multiple stress in freshwater, marine and ground waters. Sci. Total Environ. 540, 43–52. doi: 10.1016/j.scitotenv.2015.06.045
Ormerod, S. J., Dobson, M., Hildrew, A. G., and Townsend, C. R. (2010). Multiple stressors in freshwater ecosystems. Freshwater Biol. 55, 1–4. doi: 10.1111/j.1365-2427.2009.02395.x
Palmer, M., and Ruhi, A. (2019). Linkages between flow regime, biota, and ecosystem processes: implications for river restoration. Science 365, 1264. doi: 10.1126/science.aaw2087
Patrick, C. J., McGarvey, D. J., Larson, J. H., Cross, W. F., Allen, D. C., Benke, A. C., et al. (2019). Precipitation and temperature drive continental-scale patterns in stream invertebrate production. Sci. Adv. 5, eaav2348. doi: 10.1126/sciadv.aav2348
Perujo, N., Van den Brink, P. J., Segner, H., Mantyka-Pringle, C., Sabater, S., Birk, S., et al. (2021). A guideline to frame stressor effects in freshwater ecosystems. Sci. Total Environ. 777, 146112. doi: 10.1016/j.scitotenv.2021.146112
Phelps, Q. E., Tripp, S. J., Herzog, D. P., and Garvey, J. E. (2015). Temporary connectivity: the relative benefits of large river floodplain inundation in the lower Mississippi River. Restoration Ecol. 23, 53–56. doi: 10.1111/rec.12119
Piégay, H., Arnaud, F., Belletti, B., Bertrand, M., Bizzi, S., Carbonneau, P., et al. (2020). Remotely sensed rivers in the anthropocene: state of the art and prospects. Earth Surface Proc. Landf. 45, 157–188. doi: 10.1002/esp.4787
Rantala, H., Glover, D., Garvey, J., Phelps, Q., Tripp, S., Herzog, D., et al. (2016). Fish assemblage and ecosystem metabolism responses to reconnection of the bird's point-New Madrid floodway during the 2011 Mississippi River flood. River Res. Appl. 32, 1018–1029. doi: 10.1002/rra.2932
Rohde, S., Hostmann, M., Peter, A., and Ewald, K. C. (2006). Room for rivers: an integrative search strategy for floodplain restoration. Landscape Urb. Plan. 78, 50–70. doi: 10.1016/j.landurbplan.2005.05.006
Roni, P., Hall, J. E., Drenner, S. M., and Arterburn, D. (2019). Monitoring the effectiveness of floodplain habitat restoration: a review of methods and recommendations for future monitoring. Wiley Interdisc. Rev. Water 6, 1355. doi: 10.1002/wat2.1355
Rood, S. B., Samuelson, G. M., Braatne, J. H., Gourley, C. R., Hughes, F. M., and Mahoney, J. M. (2005). Managing river flows to restore floodplain forests. Front. Ecol. Environ. 3, 193–201. doi: 10.1890/1540-9295(2005)003(0193:MRFTRF)2.0.CO;2
Schramm, H. L. (2017). The fishery resources of the Mississippi river: a model for conservation and management. Fisheries 42, 574–585. doi: 10.1080/03632415.2017.1377554
Sparks, R. E. (2010). Forty years of science and management on the Upper Mississippi River: an analysis of the past and a view of the future. Hydrobiologia 640, 3–15. doi: 10.1007/s10750-009-0069-2
Stanley, E. H., and Doyle, M. W. (2003). Trading off: the ecological effects of dam removal. Front. Ecol. Environ. 1, 15–22. doi: 10.1890/1540-9295(2003)001(0015:TOTEEO)2.0.CO;2
Statzner, B., and Beche, L. A. (2010). Can biological invertebrate traits resolve effects of multiple stressors on running water ecosystems? Freshwater Biol. 55, 80–119. doi: 10.1111/j.1365-2427.2009.02369.x
Thorp, J. H., Thoms, M. C., and Delong, M. D. (2006). The riverine ecosystem synthesis: biocomplexity in river networks across space and time. River Res. Appl. 22, 123–147. doi: 10.1002/rra.901
Thorp, J. H., Thoms, M. C., Delong, M. D., and Maasri, A. (2023). The ecological nature of whole river macrosystems: new perspectives from the riverine ecosystem synthesis. Front. Ecol. Evol. 11, 1184433. doi: 10.3389/fevo.2023.1184433
Tickner, D., Opperman, J. J., Abell, R., Acreman, M., Arthington, A. H., Bunn, S. E., et al. (2020). Bending the curve of global freshwater biodiversity loss: an emergency recovery plan. BioScience 70, 330–342. doi: 10.1093/biosci/biaa002
Tockner, K., Malard, F., and Ward, J. V. (2000). An extension of the flood pulse concept. Hydrol. Proc. 14, 2861–2883. doi: 10.1002/1099-1085(200011/12)14:16/17<2861::AID-HYP124>3.0.CO;2-F
Tockner, K., Pennetzdorfer, D., Reiner, N., Schiemer, F., and Ward, J. V. (1999). Hydrological connectivity, and the exchange of organic matter and nutrients in a dynamic river-floodplain system (Danube, Austria). Freshwater Biol. 41, 521–535. doi: 10.1046/j.1365-2427.1999.00399.x
Tockner, K., and Stanford, J. A. (2002). Riverine flood plains: present state and future trends. Environ. Conserv. 29, 308–330. doi: 10.1017/S037689290200022X
Torgersen, C. E., Le Pichon, C., Fullerton, A. H., Dugdale, S. J., Duda, J. J., Giovannini, F., et al. (2022). Riverscape approaches in practice: perspectives and applications. Biol. Rev. 97, 481–504. doi: 10.1111/brv.12810
Tripp, S. J., Phelps, Q. E., Hupfeld, R. N., Herzog, D. P., Ostendorf, D. E., Moore, T. L., et al. (2019). Sturgeon and paddlefish migration: evidence to support the need for interjurisdictional management. Fisheries 44, 183–193. doi: 10.1002/fsh.10215
Turner, A. M. (2022). Chemical fragmentation of stream systems and fish species richness: The upstream effects of water pollution. Freshwater Sci. 41, 615–625. doi: 10.1086/722102
Urbanic, G., Mihaljevic, Z., Petkovska, V., and Urbanic, M. P. (2021). Back to ecology: reference conditions as a basis for assessment, restoration and sustainable management of large rivers. Water 13, 2596. doi: 10.3390/w13182596
Vannote, R. L., Minshall, G. W., Cummins, K. W., Sedell, J. R., and Cushing, C. E. (1980). River continuum concept. Can. J. Fish. Aquatic Sci. 37, 130–137. doi: 10.1139/f80-017
Verberk, W., van Noordwijk, C. G. E., and Hildrew, A. G. (2013). Delivering on a promise: integrating species traits to transform descriptive community ecology into a predictive science. Freshwater Sci. 32, 531–547. doi: 10.1899/12-092.1
Ward, J. V., and Stanford, J. A. (1995). The serial discontinuity concept - extending the model to floodplain rivers. Reg. Rivers Res. Manage. 10, 159–168. doi: 10.1002/rrr.3450100211
Ward, J. V., and Tockner, K. (2001). Biodiversity: towards a unifying theme for river ecology. Freshwater Biol. 46, 807–819. doi: 10.1046/j.1365-2427.2001.00713.x
Wei, Y., and Wu, S. (2022). The gulf of cross-disciplinary research collaborations on global river basins is not narrowed. Ambio 51, 1994–2006. doi: 10.1007/s13280-022-01716-0
Whiles, M. R., and Patrick, C. J. (2022). Secondary Production in Streams, Vol. 2. Oxford: University Press.
Wiens, J. A. (2002). Riverine landscapes: taking landscape ecology into the water. Freshwater Biol. 47, 501–515. doi: 10.1046/j.1365-2427.2002.00887.x
Wilson, M. A., and Carpenter, S. R. (1999). Economic valuation of freshwater ecosystem services in the United States: 1971-1997. Ecol. Appl. 9, 772–783. doi: 10.1890/1051-0761(1999)009(0772:EVOFES)2.0.CO;2
Keywords: river, riverscape, ecosystem, conservation, flooding, floodplain, wetland
Citation: Garvey JE and Whiles MR (2023) Incorporating the riverscape into models of river–floodplain function. Front. Freshw. Sci. 1:1265423. doi: 10.3389/ffwsc.2023.1265423
Received: 22 July 2023; Accepted: 23 October 2023;
Published: 30 November 2023.
Edited by:
Georg H. Niedrist, University of Innsbruck, AustriaReviewed by:
Francesca Pilotto, Norwegian Institute for Nature Research (NINA), NorwayLeandro E. Miranda, US Geological Survey and Mississippi State University, United States
Copyright © 2023 Garvey and Whiles. This is an open-access article distributed under the terms of the Creative Commons Attribution License (CC BY). The use, distribution or reproduction in other forums is permitted, provided the original author(s) and the copyright owner(s) are credited and that the original publication in this journal is cited, in accordance with accepted academic practice. No use, distribution or reproduction is permitted which does not comply with these terms.
*Correspondence: James E. Garvey, amdhcnZleSYjeDAwMDQwO3NpdS5lZHU=