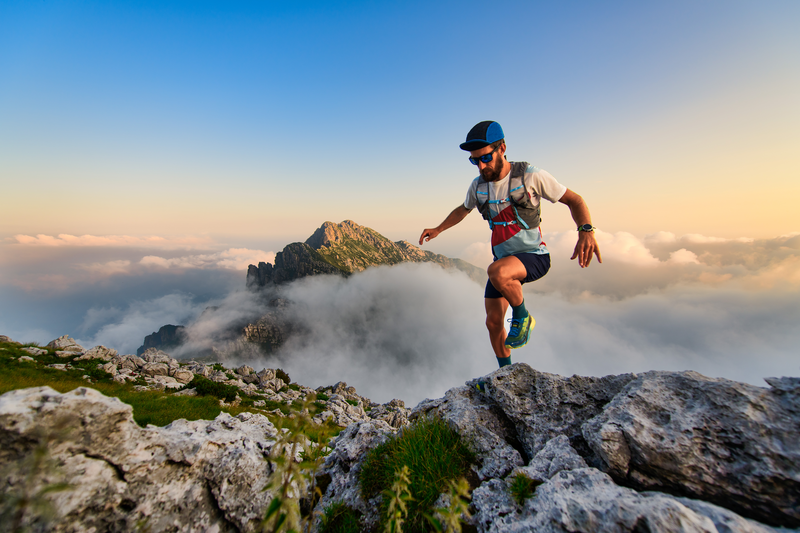
94% of researchers rate our articles as excellent or good
Learn more about the work of our research integrity team to safeguard the quality of each article we publish.
Find out more
REVIEW article
Front. Future Transp. , 26 April 2021
Sec. Transportation Systems Modeling
Volume 2 - 2021 | https://doi.org/10.3389/ffutr.2021.626935
The recent growth and adoption of unmanned aerial vehicles (UAVs) is due to their low development cost, high aerial mobility, advanced battery technology, rotors, gyroscopes, GPS, cameras, sensors, and wide range of applications. The UAV offers new possibilities for business in civil and noncivil applications such as parcel delivery, aerial mapping, agriculture, wildlife conservation, surveillance, and search and rescue. However, considering the exponential growth of various types of aerial vehicles, it is clear that advanced air traffic management services are urgently needed to control and handle the ever-increasing air traffics. In this paper, we first present the two major low-altitude UAV traffic management systems in the United States and Europe and compare them thoroughly. We present the global vision and advancement of UAV traffic management system. To understand the complexity of future air transportation system, we discuss the necessary elements required for an urban air traffic management system. We provide key strategic elements for an advanced urban traffic management through connectivity, open source, safety enhancement, and automation to maximize its impacts on the innovative future air traffic and mobility system. In the end, we discuss the challenging security issues in urban traffic management system.
The rapid development of unmanned aerial vehicles (UAVs), along with their low development cost, high aerial mobility, and wide range of applications, offers new possibilities for business in civil and noncivil applications. In recent times, UAVs are also commonly known as drones, aerial vehicles, flying cars, etc.; however, NASA has termed the commonly known drones as UAVs. UAVs are flying robots that can fly autonomously or remotely operated by human pilots. Some of the applications of UAVs are parcel delivery, aerial mapping, agriculture, wildlife conservation, aerial photography, surveillance, rescue operations, etc. The UAV has been known for decades, and the first mass-produced military drone was Radioplane OQ-2 during World War II in United States (O’Donnell, 1999). The recent growth and adoption of UAVs is primarily due to the advances in battery technology, endurance, rotors, gyroscopes, GPS, cameras, and sensors, as well as public access. The production of small UAVs (sUAVs) has created technology that is modern, inexpensive, and easy to use with access to all by providing several applications. It has become a valuable resource for technical usages and a common recreational consumer object. The UAVs can be used in dangerous scenarios where a human presence is very difficult or almost impossible. However, the UAVs cannot attain full applicability due to some hurdles such as complex environments, limited sensor capability, communication problem, and energy issues. While attempts have been made to resolve these shortcomings, there is a need for a more efficient and effective system in terms of advanced sensor perception capability, efficient autonavigation ability, etc. Moreover, air traffic management (ATM), which controls and manages commercial aircrafts, cannot handle UAVs at uncontrolled airspaces. NASA developed a management control framework for noncommercial low-level aircraft in 2013 to ensure the secure incorporation of aircraft into the airspace. Since 2016, NASA has led several UAS traffic management (UTM) research and development projects along with different industry members under the Testing Capability Level (TCL) program. Many successful experiments and demonstrations for UAV mission planning, long-distance implementation, and connectivity beyond the visual line of sight (BVLOS) maneuvers show the steady growth of the UAV traffic management industry. However, the popularity of UAVs application in the commercial and public space provides drawbacks as well. The UAVs can be used as spy drones or can be used to carry harmful weapons. In order to prevent such malicious actions and to fulfill the requirements of urban air traffic management services, there is an establishment of a regulatory system for the worldwide deployment of UAVs. The Federal Aviation Administration (FAA) in the US and European Aviation Safety Agency (EASA) in Europe are two major and active regulatory authorities who are working effortlessly to generate regulations and opportunities for commercialized UAVs. Similarly, there are many other countries, such as Korea, Japan, China, Australia, United Kingdom, and Singapore, that have their own regulatory bodies and government institution for UAV and UTM regulation control at low levels. The UAS Service Suppliers (USS) are a major component of the UTM system, which has huge market expansion opportunities across the globe. Some of the basic requirements of UTM are identification, airspace management, flight operation and management, flight permission, obstacle information, advisory warning, weather information, blackbox features, etc.
Considering the exponential growth in the use of aerial vehicles, it is clear that there is an imminent need for air space management service to control and handle such air vehicles at a very low altitude. The large volume of aircraft makes the future air transportation system more complex. Thus, innovative, multifaceted, multidimensional air traffic management is required for smooth operations of UAVs and other air vehicles. The main contributions of this paper are as follows:
• In this paper, we present two major low-altitude UAV traffic management systems in the United States and Europe. We compare them by presenting similarities and differences between them.
• We present the global vision and advancement of UTM systems around the world and discuss the existing research trends based on UAVs and UTM.
• We present the requirements for advanced urban traffic management for sustainable future air transportation through automation to maximize the impacts on the innovative air traffic and mobility system.
• We also discussed important security challenges and issues in the UTM system.
The remainder of the paper is organized as follows. Background describes the brief background on the history and evolution of UAVs. Urban Aircraft Traffic Management System presents the air traffic management system and airspace concept design and describes the US and EU vision of UTM. In Global UTM Vision, we present the global vision of UTM system from different countries around the globe. Advanced Future Urban Air Traffic Management presents the seven components for sustainable development of the advanced UTM system and design of the advanced UTM system for the future air transportation system. Safety and Security Issues in Air Mobility Traffic Management discusses the security issues in air mobility traffic management, and finally, the paper is concluded in Conclusions. For ease of reading and understanding, a list of acronyms is presented in Table 1.
UAVs have been known for decades; they have been used by the military for warfare and can carry ballistic or nonballistic payloads, flying hundreds of miles (US Department of Defense, 2005). The first known application of UAVs dates back to 1849 in the form of air balloons equipped with bombs during the war between Austrians and Italians. Reginald Denny developed the first mass-produced military application remote-controlled aircraft called Radioplane OQ-2 during World War II (O’Donnell, 1999). Since the 1990s, small and micro-UAVs came into existence as the drone became popular. Today, the UAVs are used beyond the military operations with hundreds of applications for commercial and civilian purposes (e.g., recreational drones). Since the past decade, the UAVs have evolved technologically with major advancements in operational flexibility, battery capacity, remote functionality, size, weight, and cost reduction. The UAVs offer new business opportunities in both commercial and noncommercial applications, low-cost solutions, high aerial mobility, and extensive use cases such as logistics, aerial mapping, smart agriculture, wildlife conservation, cinema, documentaries, aerial surveillance, and search and rescue. Approximately 14.2 million drones are expected to fly in global airspace and the UAVs market is anticipated to reach about $2.83 billion by 2030 (Butterworth Hayes and Mahon, 2020), and commercial applications of UAVs in the field of smart cities, transport, agriculture, security, telecommunication, media, and entertainment have an estimated value of over $127 billion according to a PwC report (PwC, 2016).
The UAVs are powerful flying vehicles that can be operated autonomously or controlled by a pilot using advanced remote control system and apply aerodynamic forces and vertical propulsion system for vertical takeoff and landing (VTOL). The autonomous UAVs fly based on onboard sensor equipment such as inertial measurement unit (IMU) that generates acceleration based on accelerometers and measures shifts in rotating attitudes using one or more gyroscopes or magnetometer for direction drift adjustment. The unmanned aircraft systems (UAS) include a UAV, a ground controller, pilot, and a communication network between the remote controller and the UAV. The UAS can be semiautonomous or autonomous based on different sensors, control signals, and positioning systems. The various sensors, such as vision-based sensors and ultrasound sensors, installed on the UAV can be used for obstacle detection and collision avoidance utilizing artificial intelligence algorithms that calculate positions, altitudes, speeds, and battery. Many research and development teams around the world take initiatives and work on the UTM platform testing with different industrial partners under diverse testing capability plan.
The increasing volume of UAVs and evolving technical capabilities introduced several regulatory challenges and issues in many countries. In 2011, the International Civil Aviation Organization (ICAO) prepared international standardization for regulating certain UAV operations and published a statement under UAS (CIR328) that provides guidance materials for global UAV operation in harmonized, safe, and efficient way (ICAO, 2011). The European Commission introduced EU laws, rules, and regulation for UAVs (including all types of UAVs) in the European sky for harmonious operations. For UAVs of weight 150 kg or beyond, the flights and use cases are approved based on a case-by-case condition, which are subject to EU-level regulation. In the US, the FAA is the federal authority responsible for making rules and regulatory system for UAVs, handles aviation operation in all airspace, and maintains operating conditions to ensure transparency of operators and fostering efficient and fair access to airspace for all operators, including manned/unmanned aircraft. In the US, commercial or recreational UAVs should fly under the applicable UAV policy and regulations defined by 14 CFR Part 107 (FAA, 2016). Australia was the first country to implement drone legislation introducing Civil Aviation Safety Regulation (CASR) (CASR Section 101) in 2002 (Library of Congress, 2016). Australian drone legislation categorizes drones based on weight and purpose of their application (i.e., recreational or commercial). Approval is not required for recreational drones as long as certain safety guidelines are followed. The operator must obtain authorization from Civil Aviation Safety Authority (CASA) in the form of a Remote Pilot's License to fly drones weighing more than 2 kg commercially. Similarly in the UK, the primary documents for aviation regulation, i.e., Articles 241, 94, and 95 of the Air Navigation Order (ANO) 2016, regulate drones weighing less than 20 kg for recreational use. If the drones need to fly below the minimum altitude from the buildings and public, then they require permission from Civil Aviation Authority (CAA). Likewise, many countries around the globe have their own legislative proposals, rules, and regulations for UAV operation in their sky, and the scope of information and documents necessary under the rules of the countries differs, but most of them are common with European and US regulations. Some of the general rules and regulations (Fontanella et al., 2017) for flying UAVs in urban areas are given in Table 2. The UAV pilots or professional operators who fly UAV over 12 kg are required to get a remote pilot license/certificate before they fly for profit. However, the UAV recreational operators who fly within visual line of sight (VLOS) as a hobby, considering the UAV policy and rules under 14 CFR Parts 101 (e) and US-DOT released Part 107 regulations (FAA, 2016), do not require remote pilot certificates. A detailed UAV guideline for UAV operation and UAV operators regarding safety rules, restrictions, and standards are discussed in Sunil et al. (2016).
Watts et al. classified the aerial vehicles based on their platforms (e.g., commercial, military, or civilian) as well as the UAV characteristics (e.g., capabilities, length, size, maximum altitude, speed, payload, weight, and flight time) (Watts et al., 2012). These days, there is a considerable attempt to develop and produce flying aircrafts, varying from large to small-scaled and lightweight air vehicles for secret missions. Such activities have led to the production of various types of air vehicles with different shapes and modes of operation. There are several research works in academia and military community on the classification and types of the drones such as those discussed in Weibel and Hansman (2004), Brooke-Holland (2013), and Hassanalian and Abdelkefi (2017). Although there is no common agreement on UAV or civil aircraft classifications, these categories are consistent with the categorization used by both the academic community and the military community. The basic drone classifications are personalized aerial vehicle (PAV), unmanned aerial vehicle (UAV), small unmanned aerial vehicle (sUAV), micro aerial vehicle (MAV), nano air vehicle (NAV), and pico air vehicle (pAV). Table 3 shows the classification of various types of aerial vehicles based on the abovementioned characteristics (Fontanella et al., 2017).
The PAV is the largest flying vehicle in this classification. Its wingspan is up to 61 m for fixed wings, which is almost the wing size of commercial airplanes. Its weight is above 140 kg and up to 15,000 kg. These types of PAVs are mostly used by military or logistics to carry cargo from one location to another. The UAV spectrum consists of varieties of unmanned aircraft systems with varying wingspan, weight, and flight ranges. The medium and average UAV weights between 5 and 150 kg, and their flight range is from 10 to 250 km. The UAVs vary widely in their model, design, and configuration and can be horizontal takeoff and landing (HTOL), VTOL, or hybrid. The lightweight small UAVs (sUAVs) weigh from 2 to 5 kg and their flight range is from 10 to 15 km and wingspan range is from 1 to 2 m. Similarly, in recent decades, the requirement for intelligence missions has grown due to the production of a smaller drone like micro air vehicle (Mcmichael and Francis, 1997). MAVs weigh from 50 g to 2 kg and their flight range is less than 10 km and wingspan range is from 15 cm to 1 m. The MAV can have fixed wings, flapping wings, VTOL, rotary wings, etc. DARPA launched even smaller light aircraft called NAV (Mcmichael and Francis, 1997; Petricca et al., 2011). NAV is a lightweight vehicle in between 3 and 50 g having a wingspan of 2.5–15 cm with flight range less than 1 km and is commonly used for civilian leisure activities. The pAV is an extremely small drone that has comparable size with that of coins or insects. It weighs around 0.5g–3 g with wingspan from 0.25 to 2.5 cm. The pAV prototypes use flapping, rotary, or quadrotor wings that show impressive flight performances such as hovering, turning, and accelerating (Wood et al., 2012).
Airspace management avoids common intervention by all airspace users, enables detection of air defense, and secures the operation of all air traffic. The ICAO is responsible for planning and development of international air transportation system for safe aircraft operation. The ICAO classified the airspace based on separation, clearance, traffic information, and flight rules. Most countries follow the ICAO airspace classification (from Class A to Class G); however, some countries may use selected classes only while others might further change the rules and regulations for the sake of their national airspace safety and security (International Civil Aviation Organization (ICAO), 1990; Encyclopedia, 2011). In the case of UAVs, the low-level aircraft flights are planned in advance and are instantaneous. The air space is divided into two categories: controlled airspace and uncontrolled airspace. The controlled airspace is a general concept that encompasses different airspace classification with specified altitude ranges under which the air traffic control (ATC) service is given. The controlled airspace is further classified into five classes. They are Class A, Class B, Class C, Class D, and Class E. On the other hand, the uncontrolled airspace is Class G airspace that is a part of general airspace where the ATC has no responsibility or authority to control this airspace. In the US, National Airspace System (NAS) in cooperation with FAA has launched effective airspace and air traffic control system. FAA is responsible for regulating and dividing the airspace into classes and is further categorized based on its nature of operation, density, complexity, safety requirements, etc. The NAS categorizes the airspace into regulatory airspace and nonregulatory airspace (National Airspace System, 2021). The summary of airspace is given in Table 4. The Class F airspace is an uncontrolled airspace where flight operation can be conducted under Instrument Flight Rules (IFR) or Visual Flight Rules (VFR), but it is not used in most countries. Therefore, we have not included Class F in Table 4. Class G ranges from the bottom of the overlying Class E airspace to the top. Class E airspace is a controlled airspace, where the aircraft operating under IFR is required to obtain ATC clearance, while in Class G, aircraft operation can be conducted under both IFR and VFR without requiring ATC clearance. ATC does not have the right to control Class G air traffic, and pilots do not require the ATC permission to fly, but pilots should understand certain VFR applicable rules in Class G (NexGen, 2018).
A large number of UAVs use the Class G airspace because it is designated as uncontrolled airspace and least restrictive airspace. In this paper, we will focus on the uncontrolled airspace Class G where the ATM is not accountable for airspace management. The FAA does not have the responsibility or authority to control this airspace; however, it does not mean this airspace is unregulated. FAA might still apply some regulations for UAV operations. Class G airspace covers the surface to 700 or 1,200 ft. above ground level (AGL) or 14,500 ft mean sea level (MSL) depends on the base of the overlaying Class E airspace. Astral Aerial solutions, which is a subsidiary of the African cargo airline (Bazzolo, 2017), suggested a general airspace management for UAVs. The general UAV airspace is divided into three segments to separate the PAV, UAV, and sUAV operations considering the substantial operation and performance variations between these types of aircraft as shown in Figure 1. Generally, above 6,500 ft (i.e., 1,981 m) is assigned to commercial airspace where the manned or commercial aircraft are operated and are controlled by the ATM system. We will not discuss this airspace as it is out of the scope of this paper. According to Sunil et al. (2015), the PAVs/CAVs cover the airspace regions between 1,650 and 6,500 ft accounting that the PAVs/CAVs emphasize on the cruise control phase of the flight; i.e., they have fixed source and destination for human transportation or local cargo deliveries. The UAVs airspace region extends from 1,100 to 1,650 ft, while the sUAV can operate at a very low altitude below 400 ft. In addition, there is a no-fly buffer zone in between 400 and 500 ft to ensure enough space between UAVs and other manned aircraft. This provides safe operation for the manned aircraft that do not fly below 500 ft.
The volume of UAVs will increase exponentially with the production of inexpensive UAVs and lenient rules for UAVs. Moreover, there is an increase of commercial delivery operators and licensed UAS operators, and hobbyists can fly UAVs within VLOS in Class G airspace. The operation of these aircraft at a low altitude poses a number of issues and threats across uncontrolled and controlled airspaces. A functional, realistic, versatile, scalable, equitable, and implementable airspace architecture for UAV operations needs to be established that involves the simultaneous execution of different flight-missions and vehicle types (e.g., piloted, autonomous, VTOL, PAV, and UAV). Moreover, the planning and location of vertiport hubs take into account environmental and public considerations such as noise, pollution, and privacy. Sunil et al. (2015) discussed four airspace concept designs; they are the full-mix, layered, zones, and tubes concepts. Figure 2 shows a comparison of four airspace concepts in order of increasing structure including top view. a) Full mix concept is an unstructured airspace where the aircrafts move freely with four degrees of freedom from source to destination. The aircrafts fly with optimum velocities, fuel consumption, and flight altitudes. However, the air traffic is subjected to physical constraints such as weather conditions, and topography static and dynamic obstacles. b) In the case of layers concept, it has three degrees of freedom and airspace is divided into vertical altitude layers with each layer permitting specific heading velocity and horizontal route with an exception for ascending and descending of aircraft. It is anticipated to lower the risk of collision by reducing relative velocities between aircraft flying at the same altitude layer. This concept provides increased safety but at the cost of fuel efficiency and energy consumption. c) In the zones concept, the airspace is divided into radial or circular zones, which is similar to the ring roads used in urban cities. The aircraft can move in a clockwise and anticlockwise circular direction with inbound and outbound radial traffic for entering and exiting the radial zones. It has two degrees of freedom, i.e., altitude and speed. d) The tubes concept is expected to deliver optimal airspace construction. It provides preplanned and collision-free fixed routes with increased traffic movement predictability. All flights within the same layer or tube are expected to travel at the same altitude, direction, and speed at recommended space-time routes; thus, it has zero degrees of freedom. Tubes at the very same horizontal level never intersect except at nodes (Sunil et al., 2016). The speed of the aircraft increases with the increasing altitude of the layers. In future air transportation system, one of the concepts or hybrid concepts can be adopted for efficient and collision-free air traffic management.
FIGURE 2. Different airspace concepts in order of increasing structure: (A) Full Mix, (B) Layered, (C) Zone and (D) Tubes.
UAVs operation does not involve direct interaction with the existing ATM system. Thus, responsible authorities around the globe, such as the FAA, SESAR, etc., are concentrated on developing UAS regulatory framework and operation rules for effective and fair access of very low-level (VLL) airspace intended for all types of aircraft operators. An innovative drone traffic management system for low-altitude airspace similar to the ATM (for controlled air space) can provide new solutions and visions for drone operations. Moreover, it should provide a shared intention through public–private collaborations by using technologies to enable new markets while guaranteeing the safety, security, standards, and fairness of the NAS. The distinction between the UTM concept and the UTM system should be discussed before moving further deep into UTM. The UTM concept is a complicated framework in which many participants contribute to guarantee the required level of UAV operations in terms of standards as well as safe separation distance between UAVs and other ATM aircraft at a VLL to provide efficient air traffic flow, while the UTM system is a comprehensive technological implementation that involves software and requires equipment to operate the UAVs in the UTM system.
The low-altitude air traffic management system in the US is called UAS traffic management (UTM), while in Europe it is called Urban Space (U-space). A conceptual framework for the UTM known as Concept of Operation (ConOps) has been studied in the US, Europe, and other parts of the world. This ConOps addresses VLL dense airspace and provides air traffic management system where the manned/unmanned aircraft can fly collaboratively. The ConOps is a type of guideline explaining the features from the operator’s point of view of a proposed system that provides qualitative and quantitative descriptions of how to operate the system, how to coordinate VLL airspace, and what laws and guidelines should be imposed to allow secure UAV incorporating other airspace users. The ConOps provides UTM functionalities to assist the UAV operators to accomplish their tasks by maintaining safety, security, and privacy. It provides fair use of airspace for small as well as large UAV. Hereafter, we will discuss the US and EU vision of UAV traffic management systems in detail.
In 2013, the FAA in collaboration with NASA’s research team created a vision for a conceptual framework for UTM. The FAA in coordination with NASA and UAS community is responsible for UTM policy development and implementation. In 2015, the FAA and NASA formed a UTM Research Transition Team (RTT) that acts as the interorganizational platform to jointly explore concepts, generate experiments, and demonstrate a potential future for UTM system to empower low-altitude UAS operations on a large scale. They work together on conceptual design and prototype modeling such as airspace design, air traffic management, conflict avoidance, and dynamic geofencing, to enable an efficient UTM ecosystem. In 2017, the FAA’s UTM ecosystem implemented the Low Altitude Authorization and Notification Capability (LAANC) for sUAV so that UAS operators can access the controlled airspace near the airport locations through real-time validation of airspace authorizations below permitted altitudes. The FAA has approved many private companies to serve as UTM service supplier for LAANC. One of the authorized service suppliers of LAANC for UTM and U-space is Airmap (Airmap, 2020), which provides automatic airspace authorization as a service to UAVs that require access to the controlled airspace.
The ConOps emphasizes the UTM operations in any airspace, which are below 400 ft AGL. The goals of this ConOps are to provide a framework and identify the relevant operational and technical criteria for UTM ecosystem development. It defines the basic contextual and functional aspects related to UTM services that will help to create strategies through the various players and stakeholders engaged in UTM implementation. The FAA and NASA have released two volumes or versions of UTM ConOps (Kopardekar et al., 2016). The first version (Ver.1) was released in May 2018, which focused on collaborative efforts among FAA, NASA, industry partners, government, and nongovernment organizations. This document presents the concepts, use case developments, Technical Capability Levels (TCLs), rules, and responsibility of the related entities they have learned so far. Version 2 (i.e., Ver.2.0) is a continual progress of the previous UTM Ver.1.0, which was released in March 2020. Ver.2.0 is the expanded document that includes advanced and complex operations within different airspace classes, updated architecture, advanced UTM services, updated participation prerequisites, remote ID (RID) requirements, efficient contingency management notification, etc. The future version might include the continued maturation of UTM concept and implementation into NAS.
The UTM ConOps document envisioned the following points:
• The document describing the conceptual UTM framework and related UTM participants, including functional criteria for delivery of a large number of air traffic services
• Tasks and duties of various UTM participants and entities dealing with UTM services
• Advance application scenarios and demonstration of UTM services
The UTM architecture is broadly divided into two major portions, i.e., FAA development and deployment and industry development and deployment as shown in Figure 3. As discussed before, the FAA is a regulatory and operational entity for flights and provides operators with airspace restriction data originating from the FAA (e.g., airspace restrictions and maps with facilities). When required, the FAA communicates with UTM for information-sharing activities. the FAA has access to information at any time through the Flight Information Management System (FIMS). The FIMS is a core part of the entire UTM ecosystem that provides an essential traffic management framework to all UTM members. The FIMS is a data-sharing interface between UTM members. Conversely, the industry development is responsible for maintaining the UAS infrastructure, various services, and components that act as a part of UTM. The operators/airspace users are accountable for overall UTM operations such as UAV flight plans, intent information sharing, and safe operation of the system based on accessible information. The pilot is responsible for safe UAS flight, obeying airspace constraints and flight restrictions, avoiding obstacles on detection, no fly during hazardous weather, and tracking flight locations. UAS Service Suppliers (USS) offer services to operators to facilitate secure and effective use of airspace and satisfy the operating requirements of UTM such as flight planning, critical message distribution, tracking, and nonconflict of aircraft. The USS should follow an authentication scheme to ensure cybersecurity and serve as a coordination channel between distributed UTM players. USS stores log information in a database for analysis, regulation, and operator transparency purposes. The FAA has approved more than nine companies, including Kitty Hawk, Airmap, Delair, etc., as USS. USSs can leverage Supplementary Data Service Providers (SDSPs) for critical or advanced services through the USS network such as geographical and obstruction information, monitoring, and information on flight restrictions.
NASA led most of the UTM R&D initiatives. It has collaborated closely with numerous industry partners to test its UTM technology in the framework of its TCL program. The UTM RTT WGs goal is to create products in accordance with the NASA’s TCL architecture and evaluation schedule. The TCL is broken down into four phases from TCL1 to TCL4. As the research progresses, each phase increases in complexity and with specific technical goals that help demonstrate the system in terms of architecture, services provided, supported operations, etc. TCLs are designed based on risk-oriented criteria such as population density on the ground, air traffic density, and operating frequency of UAS. Each TCL capability is based on increasing functionality, application types, geographic locations, and use cases reflecting specific risks as shown in Figure 4.
• TCL1: In August 2015, TCL1 was tested and completed successfully with VLOS constraints at FAA testing sites. TCL1 addressed UAV operations for remote population and low traffic density for rural applications such as agriculture, infrastructure monitoring, and firefight applications. TCL1 reflects the low complexity level and low-risk UAS operational principle. The UAS operators manage airspace and readjust flight plan if a dispute or air conflict is reported in TCL1.
• TCL2: In October 2016, the TCL2 test was completed with BVLOS constraint above the sparse population at the Nevada test site. TCL2 test shows the long-range operation on moderate-low traffic density at rural or industrial site. It is capable of multiple BVLOS operations and used for tracking, contingency management, and operational procedures.
• TCL3: In May 2018, TCL3 was completed with manned/unmanned BVLOS safety operations like package delivery. It includes cooperative as well as uncooperative UAV monitoring abilities over moderate traffic density with suburban applications.
• TCL4: the TCL4 test was completed in August 2019, which involved UAV operations in dense population and high traffic density location. TCL4 is an advanced capability level leveraging results from TCL3. TCL4 tests expanded BVLOS with a prototype for large-scale contingency management and flight information for the entire UTM architecture. It comprises geofencing and detecting and locating collision avoidance for dense urban BVLOS operation. It involves advanced applications such as autonomous UAV, delivery, V2V communication, Internet, and newsgathering.
In 2016, the European Commission (EC) commenced an initiative aimed at guaranteeing the safe and secure integration of UAVs into the European airspace. The EU and EUROCONTROL founded the Single European Sky ATM Research Joint Undertaking (SESAR JU), which has 19 members and 100 companies including airport operators, regulators, military, research groups, and scientific community, collaborating in the EU and beyond (SESAR Joint Undertaking, 2020). In 2017, SESAR JU developed a vision for a conceptual framework for UAV integration and traffic management called Urban Space (U-space). In June 2018, SESAR JU under SESAR2020 exploratory research project and EU Horizon 2020 partly funded a research and development (R&D) project called Concept of Operation for EuRopean UTM systems (CORUS). CORUS is responsible for working on U-space ConOps. Initially, SESAR JU published a U-space Blueprint starting the vision and gradual deployment progress of U-space services that require complicated operations like advanced automation and communication in dense air traffic (SESAR Joint undertaking, 2017). The U-space is a collection of services and techniques that focus on a high degree of integration and process automation to enable safe, effective, stable, and secure accessibility for huge numbers of UAVs in European airspace. It offers an effective platform for facilitating regular UAV operations and encompasses all forms of activities including at and around manned airports. Moreover, U-space offers an operating structure to enable routine UAV operations and a simple and effective interface to manned aircraft, ATM service providers, and authoritative organizations. It ensures safe UAV operations in all conditions and in all airspace types focusing on VLL airspace.
The CORUS is a preliminary research project that is responsible for writing ConOps for U-space (i.e., European version of UTM). Similar to the US-UTM ConOps, U-space ConOps emphasizes on a VLL (Class G airspace) airspace, i.e., under 150 m AGL. In the case of U-space, the VLL is divided into three airspace types or volumes based on delivered services viz. X, Y, and Z. The X volume allows BVLOS operation, but no conflict resolution service is offered. However, access to Y and Z volume offers preflight conflict resolution and might have additional entry criteria like specific permission constraints or technical capabilities. The U-space includes all sizes of UAVs (comprising PAVs and CAVs), fully autonomous UAS, VLL operation near the airports, UAS controlled by multiple pilots, static and dynamic obstacles, etc. The CORUS R&D project released three versions of ConOps. The first version (Ver.1), which was an iterative development of ConOps, was released in April 2019. The second version (Ver.2) is a continuation and enhancement based on the previous version’s comments and was released in March 2019. Similarly, the final version (Ver.3) is the collection of annexes that provides further details of ConOps. Its use case was released in September 2019.
The U-space ConOps document envisioned the following points:
• U-space-enhanced overview that provides a detailed summary of U-space ConOps
• U-space functional concept that provides a reference manual for U-space operation and its ecosystem
• Collection of annexes of U-space ConOps
Several stakeholders and SESAR members are investing and contributing to creating a common U-space architecture that will contribute intelligently to the accomplishment of conceptual design and implementation. The U-space is flexible enough to be employed in all types of airspace besides VLL urban airspace and is able to integrate new concepts and technologies to sustain the airspace management in the future. U-space should integrate manned/unmanned airspace when attempting to establish a long-term solution (SESAR Joint undertaking, 2017). In U-space, the CAA is the major certified authority that administrates the geographical airspace in a given area. The functionality of operators and the role of FIMS in U-space are similar to the UTM as shown in Figure 5. The UAV collects the data based on several sensors and transmits to the U-space Service Providers (USSP) and makes onboard judgments with very little or no pilot intervention. The USSP is similar to UTM’s USS that provides access Services to UAV Operators in uncontrolled VLL airspaces. Furthermore, U-space’s Supplemental Data Service Provider (SDSP) is also similar to UTM’s SDSP that provides access to supplementary information related to meteorological conditions, land environment, cellular coverage, navigation, terrain information, etc. The U-space services based on the components used in the architecture are Service-to-Service Providers (SSP), Supplemental Data Services (SDS), and Services to UAV Operators (SUO). The SSP is the service provided between two service providers, i.e., between Aeronautical Information Management Providers (AIMP) to USSP or service provided from authority to USSP. One difference between the UTM and U-space architecture is the web-based U-space portal (SESAR, 2019) for easy access to the U-space components and reference materials for future U-space architects.
The SESAR R&D conducted the U-space programs in tandem with progressive and gradual deployment. U-space levels are built in an improved way using phases where technology is implemented as it matures. The CORUS ConOps defines the gradual growth of U-space through U1, U2, U3, and U4 based on increasing services, enabling technologies, automation, etc., which form the basis for the gradual deployment of services. Each new U-space phase corresponds to an implementation consisting of new service groups, associated capabilities that include the upgraded version of the existing services in the previous phases, and improving the safety of the system as shown in Figure 6. The four phases are as follows:
• U1 (U-Space Foundation Services): In 2019, U1 deployment phase started to deliver the foundation services that identify UAVs and operators and inform the operators regarding the restricted locations. The U1 allowed UAV operations in low traffic density locations with manned aerial vehicles. The basic U1 capabilities involve e-registration, e-identification, and geoawareness (European Commission SESAR, 2020).
• U2 (U-Space Initial Services): U2 provides an initial set of services for safe and secure management for the BVLOS operations. It establishes a connected and dispersed architecture for USSP and provides an initial level of connectivity and interface with ATM and manned aircraft. With the evolution of U2, several UAVs low-level airspace operations will increase including operation in controlled airspace. Its capabilities include geofencing, crisis management, tactical deconfliction, UAV information management, and ATC interface procedure. It is expected to be completed in 2022.
• U3 (U-Space Advanced Services): U3 is an advanced service based on the expertise obtained from U2, while enhancing and improving the new type of applications and services in an extreme dense and complex airspace environment. It will include airspace capability management and assistance for conflict detection such as automatic detection and avoidance (DAA), reliable communications system like V2X features, and improved interface with ATM and manned aircraft that results in a substantial increase of operations in all airspace environments (SESAR, 2020). The U3 capabilities will include urban air transportation, strategic deconfliction, cooperative ATC interface procedure, dynamic capacity management, dynamic geofencing, etc. The U-space advanced service is envisioned to be available in the year 2027.
• U4 (U-Space Full Services): The aim of U4 is to incorporate U-space with ATM completely by 2035+. U4 provides a full operational capacity of U-space with optimized aircraft interfaces and is enabled by a very high degree of computerization, networking, and automation for the UAV and U-space infrastructure. The U4 represents the SESAR vision for designing and implementing U-space services as a testing ground to facilitate progressive adoption in manned aircraft environment.
The comparison between the US and EU vision on UAS traffic management is given in Table 5. The US-UTM is not based on open-source architecture, so it will take some time to be accepted globally. The UTM concept focuses on FAA rules and US scenarios only and the core of the UTM; i.e., USS interacts with FIMS under the constraint of FAA. On the other hand, the EU U-space provides a web-based portal for easy access via accessible gateways for the architects to access, which helps them to constantly enhance and develop the U-space in the future consistently. This guarantees the most efficient way of ensuring coherence, completeness, and consistency of the materials developed by the numerous projects. There are various EU-based subprojects to enhance the U-space concept and operation. U-space can be easily accepted in all the EU countries due to similar operation conditions and scenarios. Although it might take some years for U-space to reach its full-service level, there is a rapid development in the R&D of the U-space and it might be accepted by other countries, which have similar airspace and UAV rules and regulations resembling the EU countries. However, both the existing UTM and U-space architectures do not connect with edge-cloud computing to reduce latency while accessing the system because latency plays an important role in air traffic management. It is not clear if both architectures will operate in a distributed manner in the future due to the risk of a single point of failure in centralization-based architecture.
Each individual company and major drone industries around the globe are trying to make their own UTM systems to control the drones in VLL airspaces. Since UTM has a very huge market value, all the drone industries from different countries around the world are competing to make a new and efficient UTM system. However, we will focus on the more general and a high-level UTM system where all the stakeholders and governments are working towards the R&D and implementation of the UTM. Most of the UAV traffic management systems have similar functions, operation, and goals as mentioned in US-UTM and EU-U-space.
In Korea, the Ministry of Land, Infrastructure, and Transport (MOLIT) is undertaking research towards national ConOps, while at the same time collaborating with NASA for secure national standardization, testing, and verification of drone traffic management. The Korean version of the drone traffic management system is called the K-drone system, which supports safe flights of multiple drones, such as drone flight monitoring and collision prevention. The MOLIT is also working towards Urban Air Mobility (UAM) for 3D low-altitude air traffic systems like drone taxi as a solution to urban ground traffic congestion. The MOLIT plans to announce the city air traffic roadmap with the goal of commercializing the first drone taxi and other services in 2025. Moreover, the Korea Institute of Aviation Safety Technology (KIAST) is also working on a research project called low-altitude UTM development and demonstration test for safe and efficient operation of UAS as well as to build VLL UTM system.
Similarly, a team of researchers from Air Traffic Management Research Institute (ATMRI) at Nanyang Technological University, Singapore (NTU Singapore), recently completed a second-stage safe and secure UAV operation on densely populated sky. ATMRI is a collaborative research facility operated by NTU and Civil Aviation Authority Singapore (CAAS) that plans to carry out R&D for UAV traffic management solution for Singapore Urban environment. In contrast to other countries, Singapore is focusing on implementing fully connected multiplatform UAVs (both VLOS and BVLOS) based on a cellular connection (LTE) flying under the altitude of 200 ft (60 m) for urban city.
In Japan, NEC, NTT DATA, NTT DoCoMo, Hitachi, Rakuten, KDDI, Zenrin, and Japan Meteorological Association collaboratively developed an integrated UAS traffic management prototype called UTM core. It consists of three management functions such as flight plan, airspace information, and flight status. They successfully demonstrated safe and secure UAV operation at the Fukushima robot test field. Moreover, there is a consortium called Japan Unmanned System Traffic & Radio Management (JUTM) that takes control of the activities related to air, ground, and water unmanned vehicles.
In September 2015, a 5-year project called the EU-China Civil Aviation Cooperation Project (EU-China APP) between EASA and Civil Aviation Administration of China (CAAC) was launched. The objective of this project is to develop civil UAS Operation Management System (UOMS). The UOMS is a unified system that serves as a Chinese approach to provide UAV traffic services. The UOMS collaborates with the General Aviation Flight Service (GAFS), communicates with other control frameworks within UAS, and interacts with the ATM.
The Australian Government including Commonwealth and State/Territory in cooperation with industry partners is in process of developing a UTM architecture. It would promote a combination of centralized government services and industry services to encourage equal and sustainable airspace access, support evolving drone requirements, and mitigate a wide range of risks and hazards. This needs the coordination of regulatory requirements to avoid discrepancy or repetition in regulatory rules and implementation of regulation through FIMS. The Australian UTM architecture is similar to US-UTM with slight modifications such as inclusion of government system elements and CASA (Emerging Aviation Technologies, 2020).
Meanwhile, the Global UTM Association (GUTMA) (Library of Congress, 2016), which is a global nonprofit consortium of UTM stakeholders, has introduced an advanced VLL global UTM architecture that acts as a guideline for global stakeholders to develop and nurture interoperable standard for UTM system. Its goal is to promote and enable rapid deployment of globally interoperable UTM services by using open-source software models and application interfaces, since the preference is not to provide one solution fitting all but several innovative and competitive advanced solutions. More than 22 countries around the globe including FAA have signed UTM service providers’ contract to present a foundation for the national UTM system.
The main objective of UTM is to integrate humans, information technologies, and services supported by aerial and land-based communications. UTM should be an open-source cloud-based architecture that is interoperable with manned/unmanned airspace. The interoperability protocol should ensure the communication timeliness, integrity of critical information, and seamless exchange of information between different entities to operate in a harmonious way. The UTM database can be designed on a superfast speed and fault-tolerant and distributed architecture. For designing future UTM system, globally accepted open-source UTM architecture similar to GUTMA architecture is suitable for sustainable, scalable, and evolving UTM system.
The existing UTM system is in its initial stages and cannot manage excessive traffic generated by a high density of UAVs. Considering the future transportation, UTM must be able to move logistics and human transportation from congested highways to clear airspaces providing facilities to Urban Air Mobility (UAM). Thus, the sky will be filled with different types of UAVs and passenger/logistic vehicles like air taxis and cargo air vehicles. The existing UTM’s scope is relevant to VLL airspaces that support BVLOS in certain levels; however, future UTM will focus on higher airspace levels including PAVs and CAVs. The seamless BVLOS operation in both VLL and higher altitudes is essential for achieving manned/unmanned airspace integration and coordination between them. The current UTM considers the semiautonomous UAVs and their communications are limited. Hence, it is necessary to develop a sustainable and advanced future UTM system that is interoperable, open source, and compliant with regulatory frameworks and fully autonomous.
The sustainable and advanced UTM systems require development strategies for managing huge numbers of manned/unmanned air vehicles. Enhanced, rapid, and cheaper air transportation for both logistics and humans would minimize cost, reduce congestion, and offer new business possibilities. The future UTM should be global and ideally based on open-source software and can be accessed by all the operators and pilots. In future UTM, the decision-making is greatly dependent on information collected from the different entities. A proper design and management of the future UTM before full-scale deployment is of utmost importance. The advanced future UTM should be based on seven major components for global and sustainable implementation. The seven components are shown in Figure 7 and are discussed below.
In the existing UTM, remote ID (RID) is currently in development, while in advanced future UTM, RID is very essential for sharing the aircraft e-registration, e-identity, digital authorization, and location information with other manned/unmanned aircraft, authorities, and UTM. This information helps in traffic monitoring, routing, collision prevention, and smooth autonomous operation of various aircraft. It helps to secure the digital identity and access management and monitor both the participating and nonparticipating aircraft. The present key challenges for remote ID and monitoring are regulation, standardization, and practical adoption.
In both controlled and uncontrolled airspace with mixed manned/unmanned aircraft, the UAVs should be installed with a collaborative advanced detect and avoid (aDAA) system (such as real-time avoidance devices and onboard equipment) for collision avoidance and ensure safe execution of aircraft. The aDAA should use 360-degree radial computer vision-based detection technology that allows dependable and safe BVLOS operations. The real-time DAA can be achieved using multiple communication technologies such as broadcasting location, V2X communication, and satellite, optical, and wireless communication. Extremely reliable UAVs with DAA system need a small separation distance between them as they can locate approaching aircraft and detect and avoid them efficiently with an accurate navigating system. For safe operation, spatial and temporal separation between UAVs should be considered based on Aircraft Safety Bounds (ASB) as shown in Figure 8 (DLR, 2017; Cho and Yoon, 2019). ABS is an elliptical or polygonal shape that encircles each aircraft based on its type, size, speed, technical capabilities, application, etc. It facilitates the strategic planning, clearance, and control of several individual aircraft within a future air traffic framework that would also take into account several requirement parameters like geofences, obstacles, etc. The Aircraft Safety Bounds are given as
where priority,
where
Communication technology plays a vital role in the UTM system for the seamless operation of air vehicles, even in BVLOS flights. Real-time communication, wide coverage area, secure networking, and very low latency are crucial factors that need to be guaranteed for smooth communication between different types of air vehicles within advanced UTM environment. The existing 4G/LTE still does not guarantee full network coverage as the cellular networks are optimized and designed only for terrestrial mobile users. The cell association patterns in high altitudes are quite different from the land. There are issues with propagation at high altitudes that results in higher interference in downlinks due to the likelihood of LoS propagation of interfering Base Stations (BS) (Lin et al., 2018). Moreover, as the aircraft fly in the sky at higher altitudes, the BS directional antenna and the main lobes should point towards the aircraft for minimum intercell interference (Euler et al., 2019). These issues can be satisfied by using 5G and 6G technology (Bajracharya et al., 2018). The 5G network connectivity in advanced UTM system can provide efficient communication between the manned/unmanned aircraft and UTM services as well as ground and marine vehicles. The 6G network envisions a heterogeneous architecture that integrates terrestrial infrastructures with nonterrestrial communications (Deebak and Al-Turjman, 2020; Giordani and Zorzi, 2020) such as low-orbit satellites that can help in efficient Communications, Navigation, Surveillance, and Information (CNSI). It aims to provide real-time communication with other services such as weather reporting, real-time data analysis, aircraft monitoring and tracking, airspace access, authorization, and edge and cloud computing services.
The increasing number of manned/unmanned aircraft sharing the global airspace has generated a need for advanced future technologies. It requires a new process to provide a more autonomous, integrated, and interactive airspace integration operation. It is important to create a more advanced, flexible, automated, and integrated traffic management system that combines existing solutions with global airspace. The interoperability provides fair airspace access to all. UTM must integrate the manned airspace to ensure safe, scalable, and long-term development of the airspace management based on shared information and exchanged situational data between manned/unmanned airspace. We need a new and diverse technology as well as resources across the UAV industry to leverage diversity for a more efficient, safer, more integrated system. The safe, secure, and scalable manned/unmanned airspace integration can be done by utilizing new technologies such as artificial intelligence, machine learning, and blockchain that can support interoperability and sustainability and optimize the capacity of the airspace.
According to Elliott and Stewart (2012), the autonomous UAVs can be divided into six levels with increasing intelligence and automation level. The fully autonomous UAV can decide its own mission, takes its own decisions during the flight, and carries out its own strategic planning without any human intervention. From level zero to level 2 in Figure 9, the pilot is in command for flight management and other operations while in level 3, the pilot is the fallback option in case of UAV failures or if any issue arises. Level 4 and level 5 do not require pilot for the flight management operation. Level 5 is fully autonomous while level 4 requires a system mission commander with aviation knowledge to execute command authority with respect to a particular operational design domain (European Cockpit Association AISBL, 2020). NASA and EASA have tested level 3 and level 4 autonomous UAVs as shown in Figure 9. In future, there will be a large number of urban air vehicles with medium to fully autonomous capabilities. Those air vehicles will have aDAA sensors to overcome various obstacles efficiently. Hence, the UTM system should be also fully autonomous during urban air vehicle communications, conflict avoidance, and flight management; i.e., the pilots and operators should have very less or no interaction between air vehicles and the UTM system. Sometimes during emergencies the flight routes need to be reorganized or takeoff holds should be applied reliably and precisely. In such situations, the UTM should support on-demand mobility requests based on priority. Thus, the system should support full flight automation including takeoff, landing, flight planning, automatic collision avoidance, automated authorizations, and sensor control without human intervention. However, in case of a lost connection or GPS failure, the air vehicles might enter unscheduled or restricted airspace. In such situations, alternative solutions may be utilizing redundant route planning or the aircraft should be embedded with safety control equipment that could lower the risk of link loss. In certain cases, minimum human intervention can be considered for backup operation only. The communication system, such as 5G and beyond 5G, is the main backbone for autonomous operation of the UAV and UTM system. The autonomous UTM ecosystem can be achieved by utilizing technologies such as machine learning techniques and cybersecurity features.
FIGURE 9. Automation level of UAV (Giordani and Zorzi, 2020).
The advanced UTM systems in an extremely complex future airspace environment will evolve with heavy air traffics. New regulation standards, policy, regulatory agendas, and new operating rules need to be enforced that take into account emergent technical solutions to guarantee harmony and integration. The UTM regulation should be in accordance with the UAV operation regulations and standards. The roles of the different actors should be set out explicitly. Furthermore, the risk-based approach must be accompanied by suitable methods for UAV activity and airspace. With new and standard regulation, the advanced UTM achieves appropriate level of functionality, reliable fault detection, and alert system reducing risks, preserving stability, and providing guaranteed QoS. The FAA plays a huge role in establishing new operating rules and creating regulation and performance requirements.
The Joint Authorities for Rulemaking on Unmanned Systems (JARUS), which is a group of experts from aviation authorities and safety organization from more than 61 countries including members from EASA, EUROCONTROL, FAA, etc., provides safety and operational requirements for integration and certification of drones/UAVs into airspace. The JARUS categorized the UAS safety operation into three categories based on unavoidable risks linked with drones/UAVs operations (JARUS, 2019). Category A (or open category) represents the UAV operation category with low unmitigated risks and applies minimum regulatory restrictions. The registration and self-certification may require compulsory airworthiness. Category B (or specific category) represents the category that lies between Category A and Category C. In this category, the UAV operations need to be assessed by the authority independently and an acceptable risk level is guaranteed by a risk assessment of the operation. Category C (or certified category) represents the UAV operations with full regulation complying high level of unmitigated risks. Category C requires airworthiness certificate, aircraft manuals, pilot license, design standards, product authorization, and other relevant certificates. The airworthiness certificate is a way of mitigating risks associated with UAV operations.
Safety in air mobility refers to the protection of air vehicles from collisions or unintentional accidents, while security in air mobility refers to the protection or defense of air vehicles from unintentional cyber-attacks, and privacy refers to the protection of the pilots or air vehicles private information against hackers or cyber-attacks.
Advanced UTM is accountable for controlling airspace and traffic flows at a tactical and strategic level for emergency management. The air traffic management mechanism is strategically responsible for the planning and segmentation of available airspace in order to allow optimal use. At the tactical level, the UTM is responsible for the observation, monitoring, and surveillance of the airspace. The embedded data management system collects all available traffic information (position, heading, and speed), weather, and geofencing information and sends alerts to aircraft as necessary, in order to provide tactical or situational awareness. The simplified accident-incident model for advanced UTM airspace collision risk in VLL is given in Figure 10 (SESAR Joint Undertaking-CORUS, 2019). Each orange box leads to a greater incident collision predecessor or a predecessor closer to a crash occurrence. In each connection between a pair of predecessors or between near collisions, a fatal accident can be stopped by collision avoidance barrier or multiple barriers. The barriers are intended to stop a series of events leading to a higher severity level of risk and, ultimately, to a collision.
In the case of commercial aviation, the general safety and security threats due to misuse of aircraft have been carefully inspected numerous times such as aircraft hijacking, bombing, and other illegal activities. In the case of UAVs, they pose risks and cause threats to the safety and security of the human and property similar to autonomous vehicles (Kim and Shrestha, 2020a; Kim and Shrestha, 2020b). The UAV pilots may endanger the civil aircraft by flying near commercial aircrafts, at high altitudes, breaking the air law, although there is no terrorist intent (Dourado, 2016). On the other hand, the safety and security of air vehicles are also very important. UAVs often face security risks of different sorts, such as sending malicious messages to UAVs, tampering of ECUs by hackers, and trying to reverse engineer their microcontrollers, applications, etc. The attack to UAV or UTM system is a serious challenge and poses a serious threat. Some of the security threats and countermeasures to the UTM ecosystems are as follows:
• Jamming: The attackers can generate jamming signals in the same radio frequency to interfere the communication between the operators and the UAVs or between UAVs and UTM causing crashes and fatalities (Krishna and Murphy, 2017). One solution to jamming attacks is to increase the signal-to-noise ratio, but there is a limitation on the transmitter side to increase the transmitting power as well as limitation to reduce noise at the receiver.
• Hijacking: The adversaries can hijack the radio or communication links between the UAVs and ground controller by de-authenticating the management frames that disconnect the link between them. As a result, the UAV will be under the control of the hijacker and can crash the UAV or cause severe casualties. As a solution, an efficient detection mechanism can be employed in association with encrypted transmitted frames or by restricting MAC address and hiding SSID in case of Wi-Fi-based communication.
• Spoofing: The attacker can masquerade as other UAVs by falsifying the information and then take control over the UAVs. A common example of spoofing or forgery attack in UAV is GPS spoofing. Defense mechanisms like jamming detection and multiple antenna defense mechanism may be deployed to avoid GPS spoofing attacks (Borio and Gioia, 2015). In UAVs, the ADS-B is used for broadcasting aircraft information (such as speed, location, angle, etc.), collision avoidance, navigation, and weather reporting during autopilot. The broadcasted information by the ADS-B to the neighboring aircraft is not encrypted; as a result, the attackers can inject the false data, which can in turn cause collisions with other aircrafts.
• Eavesdropping: In UTM ecosystem, if the intruder takes UAV ground controller’s secret keys, the whole system will be compromised. The adversaries can eavesdrop and acquire the transmitted data from the UAVs through the open communication channel. The authors in Cheon et al. (2018) implemented a new homomorphic encryption for UAV’s ground controllers called Linearly Homomorphic Authenticated Encryption (LinHAE) for safe autonomous flights by using linear operation among ciphertexts with a fast real-time controller encryption, assessment, and validation mechanism. LinHAE offers security against forgery and eavesdropping attacks.
• Denial of Service (DoS): The attackers send multiple requests to the controller triggering network congestion resulting in loss of connectivity and services to the UAV. In Hooper et al. (2016), authors acted as adversaries and used a Telnet program to send multiple requests to be the controller. It disrupts the connection between the UAV and its controller; as a result, the UAV crashed. Multilevel defense measures should be put in place to minimize network security risks. Moreover, secure cryptography and key mechanisms should be provided to UAVs to withstand such threats.
• Physical attack: Besides cyber-attacks on UAVs, there is another security issue: there might be physical attacks on UAVs by the attacker. The attacker might have access to the ground controller, or they can apprehend the flying UAV that is under their reach or capability levels. The attackers might dismantle the detained UAV to obtain internal information such as sensitive sensors’ data through various interfaces like USB or they might use software attacks. Sometimes the attacker attacks the security of the control system and manipulates the sensory data and navigation data by injecting false information. In order to prevent such physical attacks, additional sensors need to be installed to detect intruders, self-destroy technique should be employed if there is a serious threat to the device to prevent sensitive information or advanced cryptographic mechanism, and superior secure key management should be installed (Namuduri et al., 2017).
In this paper, we provide an in-depth review of existing urban traffic management systems for UAVs flying at low altitudes. We also present different types of UAVs based on their size, weight, and flying capabilities. We discussed the urban air space management and four different types of airspace concepts in order of increasing structure for UAVs flying at different altitudes. There are several previous research studies on UTM and U-space; however, there are no research articles that compare both UTM and U-space architectures in detail. In this paper, we discussed the UTM and U-space vision along with their architecture, ConOps, and capability levels and compared them side by side in more detail. We discussed the existing UTM systems around the globe, their activities, and progress in implementing the UTM. We also presented the global UTM vision and discussed on the adoption of open-source UTM system for global acceptance. The UTM, U-space, and other similar UTM architectures serve the same objective, i.e., to design and implement safe, secure, and global UTM architecture so that UAVs and PAVs have equal access to airspace and can fly without collision in urban cities by integrating commercial aircraft systems. Considering the complexity of future urban air transportation system, we discussed seven key elements that make a big global impact on complex future air transportation system. The key strategic elements such as connectivity, interoperability, open source, safety enhancement, and automation maximize their impacts on the innovative future air traffic and mobility system. We discussed on design and management of the future UTM before full-scale deployment in the coming years. We also discussed in detail the safety and cybersecurity issues faced by the future UTM and presented tentative solutions to tackle those issues. In conclusion, this paper provides a synopsis of various types of existing UTM architectures, their future advancements, challenging issues, and solutions in UAV and UTM systems.
RS and SK contributed to study conception and review and prepared the original draft. RS contributed to literature survey, research, data, and figures. SK contributed to supervision and valuable comments. IO provided the current status of Korean UAV and UTM regulations and perspective roadmap of future UTM and its communication. All authors gave final approval for the publication of the article.
This work was supported by the Technology Innovation Program (or Alchemist Project, 20012377, developing smart off the ground mobility) funded by the Ministry of Trade, Industry & Energy (MOTIE, Korea).
Author, IO was employed by the company Soomvi.
The remaining authors declare that the research was conducted in the absence of any commercial or financial relationships that could be construed as a potential conflict of interest.
The authors would like to thank the Industry and Technology Department of Incheon Metropolitan City for supporting the PAV and CAV convergence R&D center at Yonsei Global Campus through ITP (Incheon Techno-Park).
Airmap (2020). Automated airspace authorization is here. Available at: https://www.airmap.com/skyguide-airmap-deploy-low-altitude-authorization-notification-capability-laanc-geneva-lugano-switzerland/ (Accessed October 15, 2020).
Bajracharya, R., Shrestha, R., Ali, R., Musaddiq, A., and Kim, S. W. (2018). LWA in 5G: state-of-the-art architecture, opportunities, and research challenges. IEEE Commun. Mag. 56 (10), 134–141. doi:10.1109/mcom.2018.1701177
Bazzolo, G. (2017). “Africa search its own UAS Traffic Management systems,” beBee Producer. Available at: https://www.bebee.com/producer/@www-qudron-com/africa-search-its-own-uas-traffic-management-systems (Accessed October 15, 2020).
Borio, D., and Gioia, C. (2015). “Real-time jamming detection using the sum-of-squares paradigm,” in 2015 international conference on localization and GNSS, Gothenburg, Sweden, June 22–24, 2015 (ICL-GNSS), 1–6.
Brooke-Holland, L. (2013). Unmanned aerial vehicles (drones): an introduction. United Kingdom: International Affairs and Defence.
Butterworth Hayes, P., and Mahon, T. (2020). The market for UAV traffic management services. Hove, United Kingdom: Unmanned Publications Ltd.
Cheon, J. H., Han, K., Hong, S. M., Kim, H. J., Kim, J., Kim, S., et al. (2018). Toward a secure drone system: flying with real-time homomorphic authenticated encryption. IEEE Access 6, 24325–24339. doi:10.1109/access.2018.2819189
Cho, J., and Yoon, Y. (2019). “Extraction and interpretation of geometrical and topological properties of urban airspace for UAS operations,” in ATM seminar 2019, the 13th the USA/Europe ATM R&D Seminar, Vienna, Austria, June, 2019.
Deebak, B. D., and Al-Turjman, F. (2020). “Unmanned aerial vehicles in smart cities,” in Drone of IoT in 6G wireless communications: technology, challenges, and future aspects BT. Editor F. Al-Turjman (Cham, Switzerland: Springer International Publishing), 153–165.
DLR (2017). Integrating UAS into the future aviation system. Braunschweig: Institute of Flight Guidance.
Dourado, E. (2016). Do consumer drones endanger the national airspace? Evidence from wildlife strike data. Mercatus center, George Mason UniversityAvailable at: https://www.mercatus.org/publications/technology-and-innovation/do-consumer-drones-endanger-national-airspace-evidence#:∼:text=Our analysis has been based,of 2kg UAS flight time. (Accessed October 10, 2020).
Elliott, B., and Stewart, L. J. (2012). “Automation and autonomy in unmanned aircraft systems,” in Introduction to unmanned aircraft systems. Editors E. S. R. K. Barnhart, S. B. Hottman, and D. M. Marshall (Cleveland, United States: CRC Press), 99–122.
Encylopedia (2011). Airspace class. WikipediaAvailable at: https://en.wikipedia.org/wiki/Airspace_class (Accessed November 4, 2020).
Euler, S., Maattanen, H., Lin, X., Zou, Z., Bergström, M., and Sedin, J. (2019). “Mobility support for cellular connected unmanned aerial vehicles: performance and analysis,” in 2019 IEEE wireless communications and networking conference (WCNC), Marrakesh, Morocco, April 15–18, 2019 (New York, United States: IEEE), 1–6.
European Cockpit Association AISBL (2020). Unmanned aircraft systems and the concepts of automation and autonomy. ECA, 1–7. Available at: https://www.eurocockpit.be/sites/default/files/2020-04/Automation_Autonomy_ECA_Briefing_Paper_20_0423_F_1.pdf (Acceseed January 25, 2021).
European Comission SESAR (2020). Concept of operations for European UTM systems Ver.1. Bruxelles: EUROCONTROL.
FAA (2016). Summary of small unmanned aircraft rule (PART 107). FAA News. Available at: https://www.faa.gov/uas/media/Part_107_Summary.pdf (Accessed October 22, 2020).
Fontanella, R., Vetrella, A. R., Fasano, G., Accardo, A., Lo Moriello, R. S., Angrisani, L., et al. (2017). “A standardized approach to derive system specifications for drones operating in the future UTM scenario,” in 2017 5th IEEE international conference on models and technologies for intelligent transportation systems (MT-ITS). Naples, Italy, June 26–28, 2017 (New York, NY: IEEE), 250–255.
Giordani, M., and Zorzi, M. (2020). “Non-terrestrial networks in the 6G era: challenges and opportunities,” in IEEE Network (Early Access), 12–19. doi:10.1109/MNET.011.2000493
Hassanalian, M., and Abdelkefi, A. (2017). Classifications, applications, and design challenges of drones: a review. Prog. Aerosp. Sci. 91, 99–131. doi:10.1016/j.paerosci.2017.04.003
Hooper, M., Tian, Y., Zhou, R., Cao, B., Lauf, A. P., Watkins, L., et al. (2016). “Securing commercial WiFi-based UAVs from common security attacks,” in MILCOM 2016 - 2016 IEEE military communications conference, Maryland, United States, November, 2016 (New York, NY: IEEE) 1213–1218.
ICAO (2011). Unmanned aircraft systems (UAS), circular 328 AN/190. TrafikstyrelsenAvailable at: https://perma.cc/J5EM-TSAY (Accessed October 28, 2020).
International Civil Aviaiton Organization (ICAO) (1990). Aircraft operations, maintenance and general aviation. Available at: https://web.archive.org/web/20140407130450/http://www.icao.int/safety/ism/ICAO Annexes/Forms/AllItems.aspx (Accessed November 04, 2020).
Kim, S., and Shrestha, R. (2020a). “Automotive cyber security: introduction, challenges, and standardization,” in V2X current security issues, standards, challenges, use cases, and future trends BT. Editors S. Kim, and R. Shrestha (Singapore: Springer), 183–212.
Kim, S., and Shrestha, R. (2020b). “Automotive cyber security: introduction, challenges, and standardization,” in Security and privacy in intelligent autonomous vehicles. Editors S. Kim, and R. Shrestha (Singapore: Springer), 35–66.
Kopardekar, P., Rios, J., Prevot, T., Johnson, M., and Jung, J. (2016). “Unmanned aircraft system traffic management (UTM) concept of operations,” in 16th AIAA aviation technology, integration, and operations conference, Washington, DC, June 13–17, 2016, 1–16.
Krishna, C. G. L., and Murphy, R. R. (2017). “A review on cybersecurity vulnerabilities for unmanned aerial vehicles,” in IEEE international symposium on safety, security and rescue robotics (SSRR), Shanghai, China, March, 2017, 194–199.
Lin, X., Yajnanarayana, V., Muruganathan, S. D., Gao, S., Asplund, H., Maattanen, H. L., et al. (2018). The sky is not the limit: LTE for unmanned aerial vehicles. IEEE Commun. Mag. 56 (4), 204–210. doi:10.1109/mcom.2018.1700643
Mcmichael, J., and Francis, C. M. S. (1997). Micro air vehicles-toward a new dimension in flight. in WWW 1997. Available at: http://www.fas.org/irp/program/collect/docs/mav_auvsi.htm (Accessed October 10, 2020).
Namuduri, K., Chaumette, S., and Kim, J. H. (2017). UAV networks and communications. (Cambridge, United Kingdom: Cambridge University Press).
National Airspace System (2021). National airspace system categories and types. Available at: https://www.cfinotebook.net/notebook/national-airspace-system/national-airspace-system (Accessed October 26, 2020).
NexGen (2018). Unmanned aircraft system (UAS) traffic management (UTM)- concepts of operations version 1.0. US: Federal Aviation Administration (FAA).
O’Donnell, S. (1999). A short history of unmanned aerial vehicles (UAV). Available at: https://consortiq.com/short-history-unmanned-aerial-vehicles-uavs/ (Accessed July 12, 2020).
Petricca, L., Ohlckers, P., and Grinde, C. (2011). Micro- and nano-air vehicles: state of the art. Int. J. Aerosp. Eng. 2011, 214549. doi:10.1155/2011/214549
PwC (2016). Global market for commercial applications of drone technology valued at over 127bn. Price Waterhouse and Coopers & Lybrand (PwC)Available at: https://pwc.blogs.com/press_room/2016/05/global-market-for-commercial-applications-of-drone-technology-valued-at-over-127bn.html (Accessed December 15, 2020).
SESAR (2019). The European ATM portal. eATM PORTAL European ATM Master PlanAvailable at: https://www.eatmportal.eu/working/signin (Accessed September 02, 2020).
SESAR (2020). European ATM master plan- digitalising Europe’s aviation infrastructure. EU: European Union.
SESAR Joint Undertaking (2017). U-space blueprint. Luxembourg: Publications Office of the European Union, 1–12.
SESAR Joint Undertaking-CORUS (2019). Initial contingencies and constraints. EU: European Commission.
SESAR Joint Undertaking (2020). Supporting safe and secure drone operations in Europe: a preliminary summary of SESAR U-space research and innovation results (2017–2019). EU: Publications Office of the European Union.
Sunil, E., Hoekstra, J., Ellerbroek, J., Bussink, F., Nieuwenhuisen, D., Vidosavljevic, A., et al. (2015). “Metropolis: relating airspace structure and capacity for extreme traffic densities,” in ATM seminar 2015. 11th USA/EUROPE air traffic management R&D seminar, June 23–26, 2015, Lisbon, Portugal.
Sunil, E., Ellerbroek, J., Hoekstra, J., Vidosavljevic, A., Arntzen, M., Bussink, F., et al. (2016). Analysis of airspace structure and capacity for decentralized separation using fast-time simulations. J. Guid. Control Dyn. 40 (1), 38–51. doi:10.2514/1.G000528
US Department of Defense (2005). Unmanned aircraft systems roadmap 2005–2030. US: Department of Defence (DoD).
Watts, A. C., Ambrosia, V. G., and Hinkley, E. . (2012). Unmanned aircraft systems in remote sensing and scientific research: classification and considerations of use. Remote Sens. 4, 1671–1692. doi:10.3390/rs4061671
Weibel, R. E., and Hansman, R. J. (2004). Safety considerations for operation of different classes of UAVs in the NAS in 4th aviation technology. Integration and Operations Forum. American Institute of Aeronautics and Astronautics (AIAA), 1–6.
Keywords: UAV, UTM, future transportation, urban air transportation, operation concept
Citation: Shrestha R, Oh I and Kim S (2021) A Survey on Operation Concept, Advancements, and Challenging Issues of Urban Air Traffic Management. Front. Future Transp. 2:626935. doi: 10.3389/ffutr.2021.626935
Received: 07 November 2020; Accepted: 05 February 2021;
Published: 26 April 2021.
Edited by:
Xianfeng Yang, The University of Utah, United StatesReviewed by:
Constantinos Antoniou, Technical University of Munich, GermanyCopyright © 2021 Shrestha, Oh and Kim. This is an open-access article distributed under the terms of the Creative Commons Attribution License (CC BY). The use, distribution or reproduction in other forums is permitted, provided the original author(s) and the copyright owner(s) are credited and that the original publication in this journal is cited, in accordance with accepted academic practice. No use, distribution or reproduction is permitted which does not comply with these terms.
*Correspondence: Shiho Kim, c2hpaG9AeW9uc2VpLmFjLmty
Disclaimer: All claims expressed in this article are solely those of the authors and do not necessarily represent those of their affiliated organizations, or those of the publisher, the editors and the reviewers. Any product that may be evaluated in this article or claim that may be made by its manufacturer is not guaranteed or endorsed by the publisher.
Research integrity at Frontiers
Learn more about the work of our research integrity team to safeguard the quality of each article we publish.