- 1Center for Discovery and Innovation, Hackensack Meridian Health, Nutley, NJ, United States
- 2Institute for Biodiversity and Ecosystem Dynamics, University of Amsterdam, Amsterdam, Netherlands
- 3Department of Medical Microbiology, Ege University Faculty of Medicine, Izmir, Turkey
- 4Division of Mycology, Faculty of Medicine, Çukurova University, Adana, Turkey
- 5Hackensack Meridian School of Medicine, Nutley, NJ, United States
- 6Laboratorio de Micologia Medica (LIM 53), Instituto de Medicina Tropical, Universidade de São Paulo, São Paulo, Brazil
- 7Laboratório Central (LIM 03), Hospital das Clínicas da Faculdade de Medicina da Universidade de São Paulo, São Paulo, Brazil
- 8Department of Medicine, Division of Infectious Diseases, Escola Paulista de Medicina, Universidade Federal de São Paulo, São Paulo, Brazil
- 9Georgetown University Lombardi Comprehensive Cancer Center, Washington, DC, United States
Fluconazole-resistant Candida parapsilosis (FLZR-CP) outbreaks are a growing public health concern and have been reported in numerous countries. Patients infected with FLZR-CP isolates show fluconazole therapeutic failure and have a significantly increased mortality rate. Because fluconazole is the most widely used antifungal agent in most regions with outbreaks, it is paramount to restore its antifungal activity. Milbemycin oxim (MOX), a well-known canine endectocide, is a potent efflux pump inhibitor that significantly potentiates the activity of fluconazole against FLZR C. glabrata and C. albicans. However, the FLZ-MOX combination has not been tested against FLZR-CP isolates, nor is it known whether MOX may also potentiate the activity of echinocandins, a different class of antifungal drugs. Furthermore, the extent of involvement of efflux pumps CDR1 and MDR1 and ergosterol biosynthesis enzyme ERG11 and their link with gain-of-function (GOF) mutations in their transcription regulators (TAC1, MRR1, and UPC2) are poorly characterized among FLZR-CP isolates. We analyzed 25 C. parapsilosis isolates collected from outbreaks in Turkey and Brazil by determining the expression levels of CDR1, MDR1, and ERG11, examining the presence of potential GOF mutations in their transcriptional regulators, and assessing the antifungal activity of FLZ-MOX and micafungin-MOX against FLZR and multidrug-resistant (MDR) C. parapsilosis isolates. ERG11 was found to be universally induced by fluconazole in all isolates, while expression of MDR1 was unchanged. Whereas mutations in MRR1 and UPC2 were not detected, CDR1 was overexpressed in three Brazilian FLZR-CP isolates, which also carried a novel TAC1L518F mutation. Of these three isolates, one showed increased basal expression of CDR1, while the other two overexpressed CDR1 only in the presence of fluconazole. Interestingly, MOX showed promising antifungal activity against FLZR isolates, reducing the FLZ MIC 8- to 32-fold. However, the MOX and micafungin combination did not exert activity against an MDR C. parapsilosis isolate. Collectively, our study documents that the mechanisms underpinning FLZR are region specific, where ERG11 mutations were the sole mechanism of FLZR in Turkish FLZR-CP isolates, while simultaneous overexpression of CDR1 was observed in some Brazilian counterparts. Moreover, MOX and fluconazole showed potent synergistic activity, while the MOX-micafungin combination showed no synergy.
Introduction
As commensals inhabiting various mucosal surfaces in humans, species belonging to the genus Candida are responsible for approximately 1.5 billion superficial and almost 1.5 million systemic infections annually worldwide and therefore pose a serious threat to public health (Brown et al., 2012). Although the advent of antifungal drugs has improved the clinical outcomes of afflicted patients, a shift in epidemiology and the emergence of drug-resistant fungi, such as C. glabrata and C. auris, have challenged the efficacy of the limited number of antifungal drugs available to treat candidiasis (Ahangarkani et al., 2020; Arastehfar et al., 2020a). Recently, severe clonal outbreaks due to fluconazole-resistant (FLZR) C. parapsilosis have been reported in numerous countries (Choi et al., 2018; Thomaz et al., 2018; Singh et al., 2019; Martini et al., 2020; Arastehfar et al., 2020a; Arastehfar et al., 2020b; Arastehfar et al., 2021; Corzo-Leon et al., 2021; Fekkar et al., 2021), which is a matter of serious concern because azole-naïve patients infected with FLZR C. parapsilosis show clinical failure to azoles, complicating the treatment, prolonging the duration of hospitalization, and increasing hospital-related costs. Most importantly, analyses of cohorts from Turkey and Brazil have revealed that patients infected with FLZR C. parapsilosis isolates have a significantly higher rate of mortality than patients infected with susceptible isolates (Arastehfar et al., 2020b; Arastehfar et al., 2021; Thomaz et al., 2021). Alarmingly, FLZR C. parapsilosis isolates continue to expand in clinical settings and replace their susceptible counterparts even when strict infection control strategies are applied (Thomaz et al., 2021). Thomaz et al. (2021) showed that FLZR C. parapsilosis from the hands of healthcare workers and the hospital environment share 100% genetic similarity with isolates obtained from the bloodstream of afflicted patients. Moreover, the recent emergence of genetically related multidrug-resistant C. parapsilosis exhibiting resistance to both frontline-used antifungal drugs, azoles and echinocandins, presents a new clinical challenge (Arastehfar et al., 2020c). Therefore, the severity of drug-resistant C. parapsilosis clonal outbreaks can be even higher in countries where this species is considered the second leading cause of candidemia, including South American (Nucci et al., 2013), South African (Govender et al., 2016), some European (Siopi et al., 2020), Mediterranean (Arikan-Akdagli et al., 2019), and South/East Asian countries (Kakeya et al., 2019; Guo et al., 2021).
Azole resistance in C. parapsilosis is mainly driven by drug target mutations in the ERG11 gene, including Y132F, K143R, Y132F+K143R, Y132F+G307A, and G458S (Grossman et al., 2015; Choi et al., 2018; Thomaz et al., 2018; Arastehfar et al., 2020b; Arastehfar et al., 2020c; Arastehfar et al., 2021; Corzo-Leon et al., 2021). Of note, FLZR C. parapsilosis isolates collected from different countries show either a narrow range of mutations in ERG11, such as Y132F in Brazil (Thomaz et al., 2018, 2021), South Korea (Choi et al., 2018), France (Fekkar et al., 2021), and Mexico (Corzo-Leon et al., 2021), or a high degree of variability of mutations, such as all of the aforementioned mutations found in Turkish isolates (Arastehfar et al., 2020a; Arastehfar et al., 2021). Although poorly studied in C. parapsilosis, other mechanisms underpinning azole resistance have been well characterized in C. albicans, including overexpression of the drug target ERG11 as well as of efflux pumps, namely, ATP-binding cassette (ABC) transporters (such as CDR1) and the major facilitator superfamily (such as MDR1) (Arastehfar et al., 2020b). The overexpression of CDR1, MDR1, and ERG11 typically involves gain-of-function (GOF) mutations in their transcriptional regulators, i.e., TAC1 (PDR1 ortholog in C. glabrata), MRR1, and UPC2, respectively (Arastehfar et al., 2020a). Interestingly, analysis of a comprehensive collection of clinical FLZR C. albicans isolates has shown that the overexpression of CDR1 and ERG11 and occasionally MDR1 almost always occur in combination with ERG11 mutations (Flowers et al., 2015). Moreover, it has been suggested that the overexpression of efflux pumps due to such GOF mutations is a virulence factor in C. glabrata (Ferrari et al., 2009).
Azoles are one of the most widely used antifungals, especially in developing countries (Arastehfar et al., 2019), and are most severely affected by FLZR C. parapsilosis. Given the extensive involvement of ABC transporters in azole resistance, some in vitro studies have taken advantage of ABC transporter inhibitors in combination with fluconazole, which have shown promising efficacy when tested in vitro and in vivo (Silva et al., 2013; Iyer et al., 2020). For instance, oxindole (Iyer et al., 2020) and milbemycin derivatives (Silva et al., 2013) have shown prominent efficacy against C. auris (Iyer et al., 2020) and C. albicans and C. glabrata (Silva et al., 2013), respectively. Milbemycin derivatives are particularly promising because they exert a broader activity and have a long half-life and low cytotoxicity (Silva et al., 2013). Moreover, the function of milbemycin extends beyond the inhibition of ABC transporters, as is an approved endectocide in canines, and it exerts fungicidal activity on its own through generation of reactive oxygen species (ROS) (Silva et al., 2013). Expectedly, milbemycin derivatives potentiated fluconazole efficacy when tested in mice infected with FLZR C. glabrata and C. albicans isolates, resulting in fungal burdens decreasing to the same levels as those infected with susceptible counterparts (Silva et al., 2013). Interestingly, milbemycin derivatives were also found to have a favorable safety profile, tolerability, and efficacy when tested in humans, although with a limited number of patients (Cotreau et al., 2003). However, the efficacy of milbemycin against FLZR C. parapsilosis has not been investigated.
Herein, we analyzed a comprehensive collection of FLZR and FLZS C. parapsilosis isolates obtained from outbreaks in Turkey and Brazil to investigate (a) the involvement of efflux pumps and ERG11 overexpression in azole resistance, (b) the identification of GOF mutations potentially driving this overexpression, and (c) the efficacy of milbemycin in combination with fluconazole against FLZR C. parapsilosis isolates.
Materials and methods
Candida parapsilosis strain collection
This study included 26 C. parapsilsis isolates collected from outbreaks in Turkish and Brazilian hospitals, including 21 FLZR, 4 fluconazole susceptible (FLZS), and one MDR isolate resistant to both fluconazole and echinocandins (Table 1). FLZR and FLZS isolates from both centers were included. Each single isolate represents a single patient. The single MDR C. parapsilosis was included to explore the efficacy of micafungin in combination with milbemycin oxim (MOX).
RNA extraction and gene expression analysis
Candida parapsilosis cultures grown overnight (150 rpm and 37°C) were washed with PBS once, and after adjusting the cultures to OD600 0.5, the cell suspensions were inoculated in fresh YPD and incubated for another 6 hours (250 rpm and 37°C). Subsequently, C. parapsilosis cells were washed twice with PBS, incubated in RPMI 1640 containing one dilution below the minimum inhibitory concentration (MIC) of fluconazole (105 cells/ml), incubated at 37°C and 250 rpm for 90 minutes, collected (13,000 rpm for 5 minutes) and stored at –80°C. RNA samples were extracted using a home-brew method described elsewhere (Pekmezovic et al., 2021) and were subjected to DNase treatment (QIAGEN) per the manufacturer’s suggestion. DNase-treated RNA samples were further purified using an RNeasy mini-Kit (QIAGEN) per the manufacturer’s suggestion.
qPCR was performed using the primers listed in Supplementary Table 1 designed for the current study. Only primers with high levels of reproducibility (≥99%) and efficiency (≥92) were used in our final qPCR assays. qPCR was performed with a One-Step TB Green PrimeScript RT-PCR Kit II (Perfect Real Time, TaKaRa, Shiga, Japan) using the universal program mentioned in Supplementary Table 1. qPCRs containing 40 ng of RNA samples, 0.4 µM of primers, 0.8 µL of enzyme (combination of both reverse transcriptase and Taq polymerase), and 10 µL of buffer in a final volume of 20 µL were subjected to an Mx3005P qPCR System (Agilent Technologies, Santa Clara, USA).
Experiments were carried out in two biological and at least two technical replicates, and gene expression data were normalized against ACT1 gene (Supplementary Table 1). Fold changes were determined using normalized data of C. parapsilosis cells treated with fluconazole relative to untreated initial inoculums of each sample using 2-ΔΔCT as described previously (Livak and Schmittgen, 2001). Overexpression was defined as a fold change ≥2 relative to the untreated cells. Basal expression values for each untreated samples were calculated using the following formula: 2-ΔCt, where ΔCt refers to the Ct gene of target-Ct ACT1. The Ct values of target and reference genes of each replicate were normalized against the average Ct values of target and reference genes from untreated samples. Microsoft Excel was used for gene expression analysis.
Sequencing
TAC1, UPC2, and MRR1 were amplified by PCR and sequenced using conditions described previously (Arastehfar et al., 2020b). Contigs were assembled using SeqMan Pro (DNASTAR, Madison, WI, USA), and fully assembled and curated sequences were aligned to the reference sequences for TAC1 (HE605204), MRR1 (HE605205), and UPC2 (HE605206).
Antifungal susceptibility and checkerboard testing
Antifungal susceptibility testing used the broth microdilution of the CLSI M27-A3 protocol, which included fluconazole, micafungin, and MOX (all from Sigma-Aldrich, St. Louis, MO, USA). Plates were incubated at 37°C for 24 hours, and the MIC50 data (50% growth inhibition compared to control without drug for each given isolate) were determined by a Tecan® Infinite 200 Pro microplate reader (Männedorf, Switzerland). Fluconazole MICs were reported per established clinical breakpoints as reported (Pfaller and Diekema, 2012), where a given isolate with an MIC ≥8µg/L was considered as being fluconazole resistant.
The checkerboard assay included either MOX-fluconazole or MOX-micafungin, and the MIC50 was determined as described above. Fractional inhibitory concentrations (FICs) were determined using the following formula: ΣFIC = FICA + FICB = (CFLZ/MICFLZ) + (CMOX/MICMOX), where MICFLZ and MICMOX refer to the MIC of each drug alone, while CFLZ and CMOX refer to the combination MIC of fluconazole and MOX. FIC values ≤0.5, >0.5 to ≤1, and >1 to <4 were considered synergistic, additive, and indifferent, respectively (Silva et al., 2013).
Results and discussion
In this study, we used a comprehensive collection of C. parapsilosis isolates collected during outbreaks in Brazil and Turkey. ERG11 sequencing showed that all Turkish FLZR isolates carried Y132F (n=7), Y132F+G307A (n=3), and G458S (n= 4), while the Brazilian counterparts only harbored Y132F (n=7) (Table 1). To determine whether changes in ERG11, CDR1, and MDR1 expression were also involved in C. parapsilosis FLZR, the isolates were exposed to fluconazole for 90 minutes, and the expression profile of the aforementioned genes was normalized against the initial inoculum not exposed to fluconazole. Interestingly, ERG11 showed the highest degree of induction by fluconazole regardless of susceptibility profile, ERG11 mutation type, and country of origin (Figures 1A, B; Table 2). Moreover, the basal expression level of ERG11 was highest in Turkish FLZR isolates carrying G458S (P< 0.05) but unchanged in the rest of the strains (Supplementary Figure 1A), raising the question about the mechanism that may confer this difference. It is possible that this mutation impairs Erg11 enzyme catalytic activity and that higher basal expression could be a strategy to compensate for the lower level of ergosterol. Because overexpression of ERG11 is associated with a hyperactive Upc2, we sequenced this gene in all isolates, but none of the isolates harbored any mutations in UPC2. Therefore, ERG11 induction is likely a universal response employed by C. parapsilosis to effectively counteract azole activity.
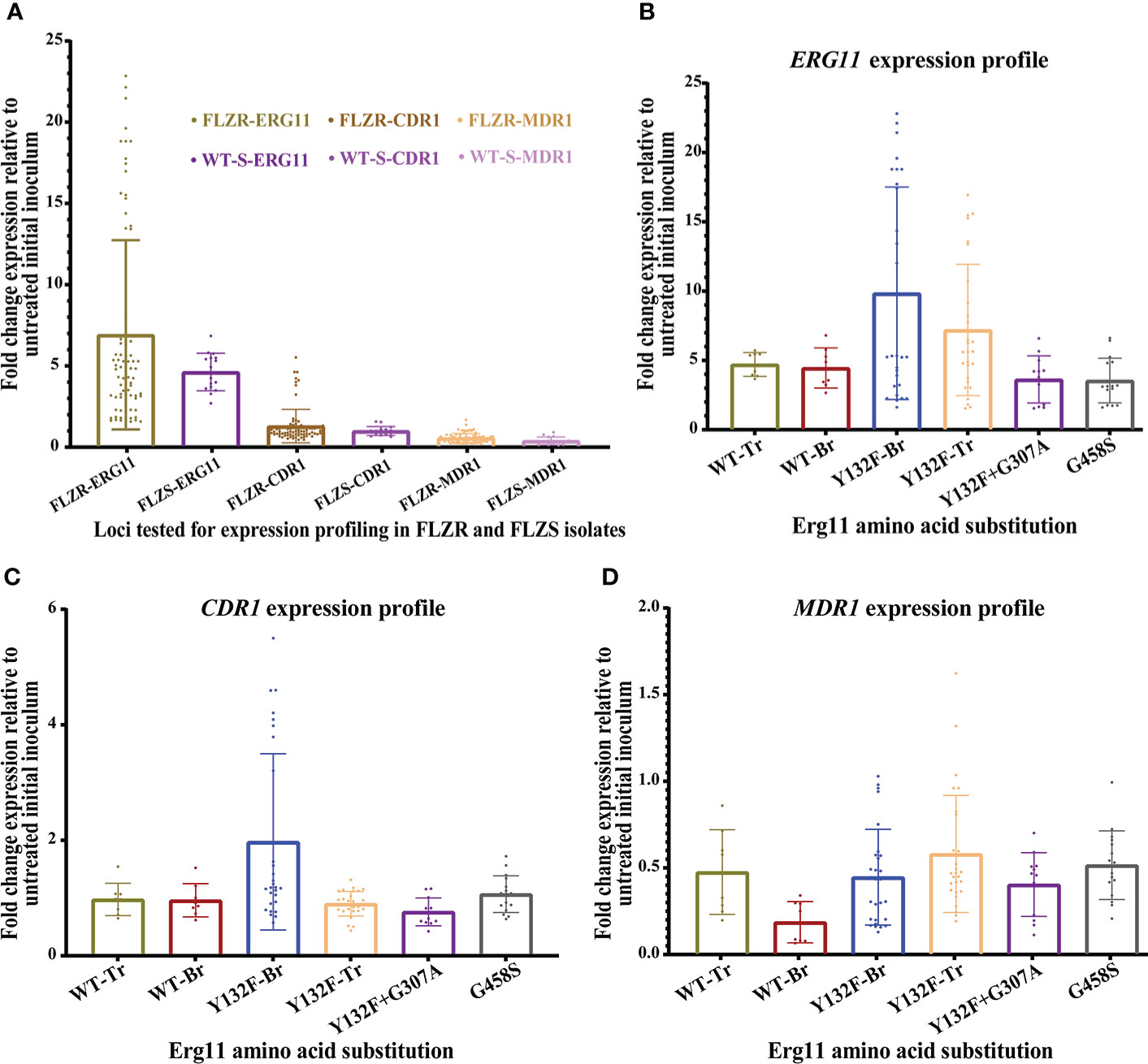
Figure 1 (A) Analysis of the expression profile of ERG11, CDR1, and MDR1 among 25 C parapsilosis isolates collected from Brazilian and Turkish outbreaks. Isolates grown in logarithmic phase were treated with one dilution below the minimum inhibitory concentration of fluconazole for 90 minutes and incubated at 37°C and 250 rpm, and treated cells were subjected to RNA extraction, followed by RNase treatment and further purification of treated RNA samples, followed by qPCR. The expression data of treated cells were normalized against untreated cells, and Actin1 was used as our reference gene. The expression fold change was calculated using 2-ΔΔCt. (B) ERG11 showed the highest level of induction irrespective of the azole susceptibility profile. (C) Overexpression of CDR1 after fluconazole exposure was noted only for two Brazilian isolates. (D) None of the isolates overexpressed MDR1.
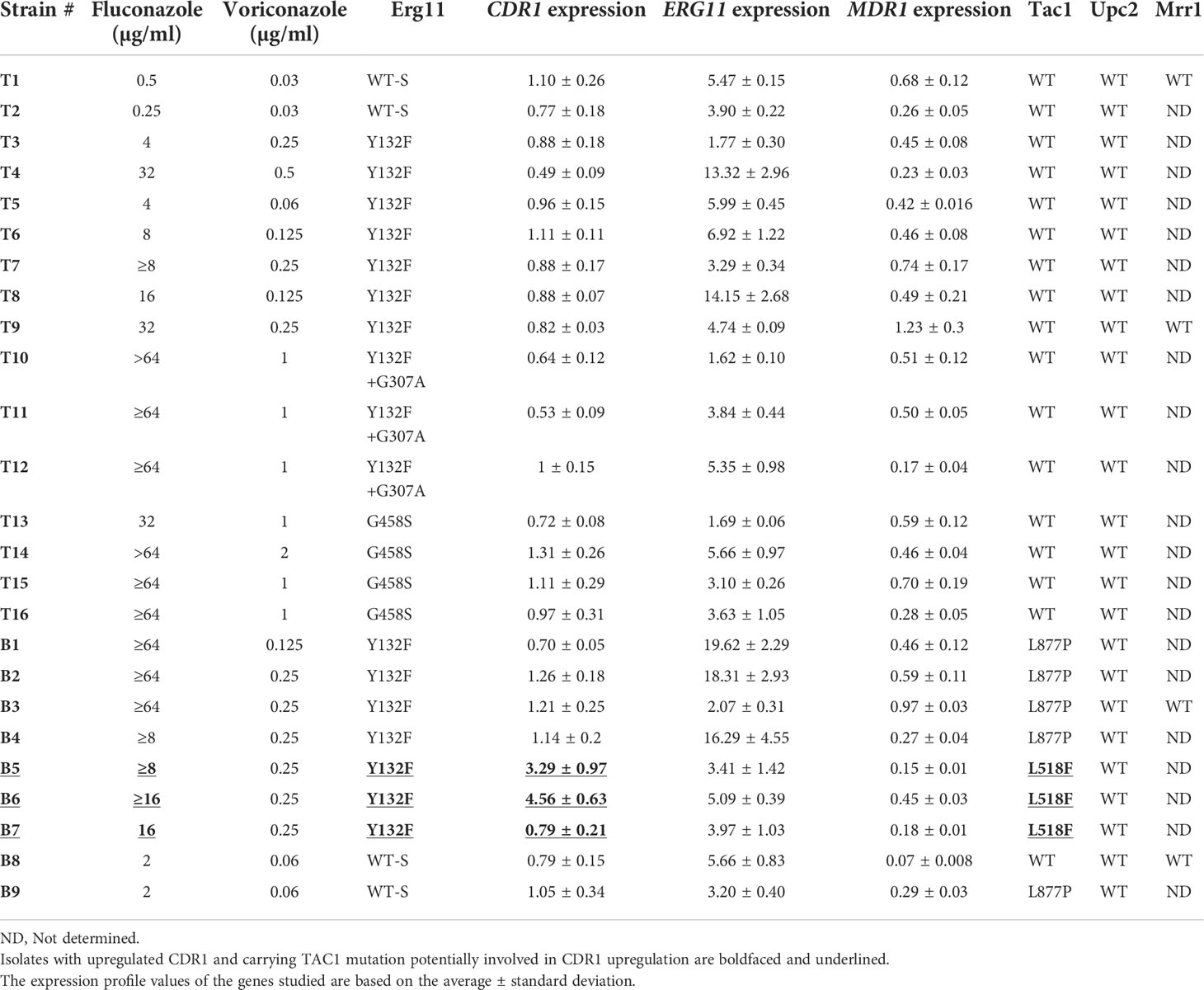
Table 2 The characteristics of Candida parapsilosis isolates collected from Turkish (shown by T) and Brazilian isolates.
Next, we assessed the expression of CDR1. Unlike ERG11, only two Brazilian isolates showed CDR1 induction upon fluconazole exposure (Figure 1C; Table 2). Since this overexpression only occurred in FLZR isolates, we suspected that TAC1 may harbor a specific GOF mutation driving the overexpression of CDR1. Indeed, while Turkish C. parapsilosis isolates were all WT for TAC1, we found two mutations among Brazilian counterparts, namely, L877P and L518F, the latter occurring exclusively in the three FLZR isolates. Of these three isolates, two overexpressed CDR1 after fluconazole induction, while the other isolate had significantly increased basal expression of CDR1 (Table 2, Supplementary Figure 1B). Therefore, strains belonging to specific lineages may have acquired additional changes to keep TAC1 in check, and overexpression of TAC1 followed by CDR1 may occur only in the presence of azoles. Such adaptation is in line with the observations that Candida lusitaniae isolates carrying hyperactive MRR1 frequently acquire secondary mutations to either completely abolish MRR1 activity or decrease the expression of MRR1 to adapt to the dynamic environment of the host (Demers et al., 2021).
Consistent with our findings, both azole-susceptible and azole-resistant C. parapsilosis isolates recovered from South Korean hospitals harbored L877P in TAC1 (Choi et al., 2018), suggesting that L877P may represent a polymorphism rather than a GOF mutation, while the exclusive occurrence of the novel GOF mutation, L518F, among FLZR isolates combined with the overexpression of CDR1 reinforces the possibility that this mutation could result in hyperactivity of TAC1 and consequent CDR1 overexpression. Interestingly, L518F mapped to the middle homology region (MHR), which is a presumed inhibitory domain of TAC1 that suppresses the activity of the C-terminal activating domain (Nishimoto et al., 2020). Notably, the basal expression level of CDR1 was not different among the isolates included in the current study (Supplementary Figure 1B). Therefore, our data support the conclusion that CDR1 overexpression (either basal or induced) is likely a specific mechanism employed by some FLZR C. parapsilosis strains and that L518F is a potential GOF mutation candidate associated with overexpression of CDR1.
Although infrequent, MDR1 has also been suggested to play a role in azole resistance among clinical isolates of C. albicans (Flowers et al., 2015; Nishimoto et al., 2020). Therefore, we examined the expression of MDR1, and to our surprise, not only did none of the isolates overexpress MDR1, but most of the isolates even downregulated MDR1 in response to fluconazole (Figure 1D and Table 2). Among the 25 isolates tested, only two isolates (one from Brazil and one from Turkey) did not downregulate MDR1, and hence, we assumed that they may have some exclusive mutations in MRR1 maintaining the expression of MDR1. However, MRR1 sequencing in these two FLZR isolates and two susceptible counterparts revealed a lack of MRR1 mutations. Moreover, unlike CDR1 and ERG11, basal overexpression of MDR1 was not observed (Supplementary Figure 1C). Therefore, we suspect that MDR1 may not represent a frequent player in azole resistance in C. parapsilosis collected from Turkey and Brazil. These findings contrast those obtained from C. parapsilosis isolates from the USA (Grossman et al., 2015), South Korea (Zhang et al., 2015), and those obtained from a Chinese patient (Choi et al., 2018), where the exclusive occurrence of “potential” MRR1 GOF mutations was reported for FLZR isolates. Of note, MDR1 overexpression in the absence of MRR1 mutation was also observed (Grossman et al., 2015), which further highlights the complexity of the mechanisms underlying azole resistance. Of the mutations exclusively found in MRR1 in the clinical FLZR isolates, only G583R has been proven to be associated with MDR1 overexpression and azole resistance (Branco et al., 2015), and the involvement of the rest in azole resistance has yet to be studied.
Although the C. parapsilosis isolates included in the current study were randomly selected, we still observed that fluconazole resistance mechanisms varied depending on the country of origin, and our gene expression data indicated that fluconazole resistance in Turkish C. parapsilosis isolates is mainly driven by the acquisition of ERG11 mutation, while those from Brazilian hospitals concomitantly overexpressed CDR1, possibly due to a GOF mutation in TAC1. This phenomenon might be explained by the clonal expansion of C. parapsilosis isolates in clinical settings, where the azole resistance mechanisms in a specific hospital could be determined by the most adaptable and abundant genotype/lineage. This hypothesis is in line with a previous study from Brazil, where all the FLZR C. parapsilosis isolates overexpressed CDR1 (Souza et al., 2015). This observation contrasts with FLZR C. albicans isolates, which are believed not to be horizontally transmitted and show significant genetic diversity. This phenomenon also has implications for mutants lacking either of those transcription factors, and the results from a given background may not be applicable to others, which again warrants further research.
The fact that Brazilian C. parapsilosis had an extra mutation in CDR1 may also point to the fact that such isolates may have a higher tendency to acquire mutations. For instance, specific genotypes of C. glabrata harboring specific mutations of the mismatch repair gene MSH2 were hypothesized to have a higher rate of mutation frequency (Healey et al., 2016). Whether such a phenomenon is applicable to C. parapsilosis is yet to be defined and is worth exploring. Given the region-specific diversity and to gain deeper insight into azole resistance in C. parapsilosis, our study warrants collecting isolates from multiple clinical centers most severely affected by clonal outbreaks, combined with gene expression analysis, sequencing, and application of precise genome editing tools to unravel mechanisms underpinning azole resistance.
Because azole resistance is a growing clinical problem, we sought to determine the efficacy of MOX in combination with fluconazole, and we included FLZR C. parapsilosis isolates harboring various ERG11 mutations from both countries (Table 3). Our data revealed that MOX per se does not have antifungal activity (Table 3), which is unlike the observations made for C. albicans and C. glabrata (Silva et al., 2013). Yet, in line with the observation that MOX may have activity beyond efflux pump inhibition (Silva et al., 2013), we observed that when combined with fluconazole, MOX significantly potentiated fluconazole activity by reducing fluconazole MIC 8– to 32–fold (Table 3) regardless of the underlying fluconazole resistance mechanisms (ERG11 mutation alone or in combination with CDR1 overexpression). Likewise, fluconazole dramatically decreased the MIC of MOX 64– to 128–fold, which mirrors the potent synergistic activities exerted by their combination.
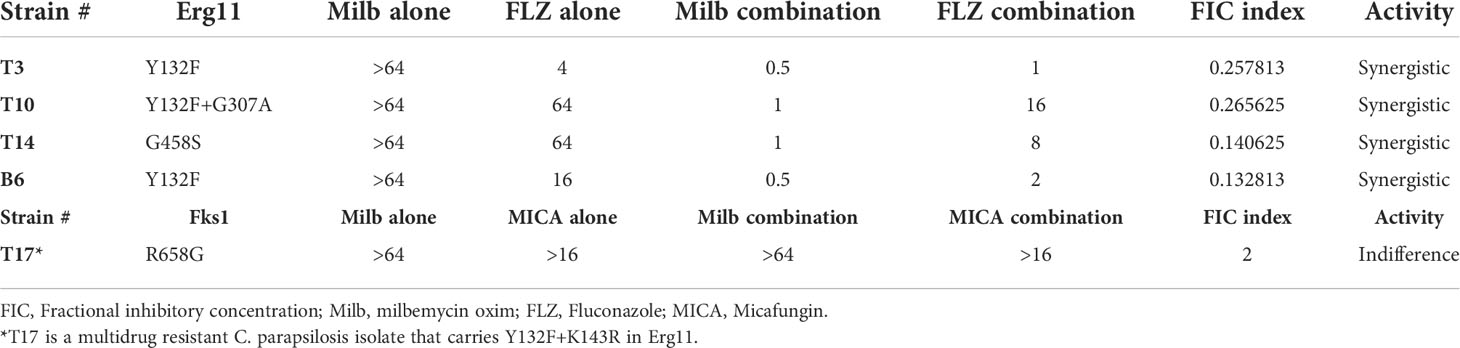
Table 3 The minimum inhibitory concentrations of fluconazole and/or micafungin in combination with milbemycin oxim.
As MDR is an emerging clinical problem in our center in Turkey, we were curious to explore the efficacy of the combination of micafungin and MOX against an MDR isolate. Of note, the MOX and micafungin combination did not yield synergistic or additive activities, and the MICs did not change compared to each drug alone. We note that in vivo studies are required to validate the synergistic and indifferent activities observed for fluconazole-MOX and micafungin-MOX, respectively. Of note, given that checkerboard assay is labor-intensive and time-consuming, here we just included a limited number of isolates and the lack of efficacy of MOX and micafungin warrants inclusion of further isolates.
Collectively, our study shows that fluconazole resistance mechanisms employed by C. parapsilosis isolates can vary from hospital to hospital and among geographical regions and that efflux pump inhibitors such as MOX in combination with fluconazole showed promising activities against FLZR isolates.
Data availability statement
The original contributions presented in the study are included in the article/supplementary material. Further inquiries can be directed to the corresponding authors.
Ethics statement
This study is part of a FAPESP GRANT (2017/02203-7) that was reviewed and approved by local Ethical Committee at UNIFESP.
Author contributions
Conceptualization, AA, FD, and DP. Methodology, FD and AA. Software, FD, AA, and ES. Validation, FD and AA. Formal analysis, FD and AA. Investigation, FD and AA. Resources, MI, SH-P, JA, AC, and LF. Data curation, FD and AA. Writing – original draft, FD, AA, and ES. Writing – review and editing, all co-authors. Visualization, FD, AA, and ES. Supervision, DP, FD, and AA. Project administration, FD and AA. Funding acquisition, DP and AC. All authors contributed to the article and approved the submitted version.
Funding
This work was supported by internal support by the Center for Discovery and Innovation to DP and Grant 2017/02203-7, Fundação de Amparo a Pesquisa de Sao Paulo (FAPESP) to AC.
Conflict of interest
AC received educational Grants from Angem, Eurofarma, Knight-United Medical, Gilead, Pfizer and travel grant support from Eurofarma and Knight-United Medical.
DP receives research support and/or serves on advisory boards for Amplyx, Cidara, Scynexis, N8 Medical, Merck, Regeneron, and Pfizer. He also has a patent covering the detection of fungal species and drug resistance, as well as a pending patent on COVID-19 detection licensed to T2 Biosystems.
The remaining authors declare that the research was conducted in the absence of any commercial or financial relationships that could be construed as a potential conflict of interest.
Publisher’s note
All claims expressed in this article are solely those of the authors and do not necessarily represent those of their affiliated organizations, or those of the publisher, the editors and the reviewers. Any product that may be evaluated in this article, or claim that may be made by its manufacturer, is not guaranteed or endorsed by the publisher.
Supplementary material
The Supplementary Material for this article can be found online at: https://www.frontiersin.org/articles/10.3389/ffunb.2022.906681/full#supplementary-material
Supplementary Figure 1 | (A) Basal expression of Turkish isolates carrying G458S was the highest, while the rest had the same level of expression. (B) Basal overexpression of CDR1 was noted only for one Brazilian isolate carrying L518F in TAC1. (C) All of the isolates tested showed the same basal level of MDR1 expression. The basal expression level was calculated using 2-ΔCt, where ΔCt refers to the Ct of the target gene- Ct of ACT1.
References
Ahangarkani F., Shokohi T., Rezai M. S., Ilkit M., Mahmoodi Nesheli H., Karami H., et al. (2020). Epidemiological features of nosocomial candidaemia in neonates, infants and children: a multicentre study in Iran. Mycoses 63, 382–394. doi: 10.111/myc.13053
Arastehfar A., Daneshnia F., Hilmioğlu-Polat S., Fang W., Yaşar M., Polat F., et al. (2020b). First report of candidemia clonal outbreak caused by emerging fluconazole-resistant Candida parapsilosis isolates harboring Y132F and/or Y132F+K143R in Turkey. Antimicrob. Agents Chemother. 64, e01001–e01020. doi: 10.1128/AAC.01001-20
Arastehfar A., Daneshnia F., Hilmioglu-Polat S., Ilkit M., Yasar M., Polat F., et al. (2020c). Genetically related micafungin-resistant Candida parapsilosis blood isolates harbouring novel mutation R658G in hotspot 1 of Fks1p: a new challenge? J. Antimicrob. Chemother. 76, 418–422. doi: 10.1093/jac/dkaa419
Arastehfar A., Gabaldón T., Garcia-Rubio R., Jenks J. D., Hoenigl M., Salzer H. J. F., et al. (2020a). Drug-resistant fungi: An emerging challenge threatening our limited antifungal armamentarium. Antibiotics 9, 877. doi: 10.3390/antibiotics9120877
Arastehfar A., Hilmioğlu-Polat S., Daneshnia F., Pan W., Ahmed Hafez A., Fang W., et al. (2021). Clonal candidemia outbreak by candida parapsilosis carrying Y132F in Turkey: evolution of a persisting challenge. Front. Cell. Infect. Microbiol. 11. doi: 10.3389/fcimb.2021.676177
Arastehfar A., Wickes B. L., Ilkit M., Pincus D. H., Daneshnia F., Pan W., et al. (2019). Identification of mycoses in developing countries. J. Fungi 5, 90. doi: 10.3390/jof5040090
Arikan-Akdagli S., Gülmez D., Doğan Ö., Çerikçioğlu N., Doluca Dereli M., Birinci A., et al. (2019). First multicenter report of in vitro resistance rates in candidaemia isolates in Turkey. J. Glob. Antimicrob. Resist. 18, 230–234. doi: 10.1016/j.jgar.2019.04.003
Branco J., Silva A. P., Silva R. M., Silva-Dias A., Pina-Vaz C., Butler G., et al. (2015). Fluconazole and voriconazole resistance in Candida parapsilosis is conferred by gain-of-function mutations in MRR1 transcription factor gene. Antimicrob. Agents Chemother. 59, 6629–6633. doi: 10.1128/AAC.00842-15
Brown G. D., Denning D. W., Gow N. A. R., Levitz S. M., Netea M. G., White T. C. (2012). Hidden killers: Human fungal infections. Sci. Transl. Med. 4, 165rv13. doi: 10.1126/scitranslmed.3004404
Choi Y. J., Kim Y.-J., Yong D., Byun J.-H., Kim T. S., Chang Y. S., et al. (2018). Fluconazole-resistant Candida parapsilosis bloodstream isolates with Y132F mutation in ERG11 gene, south Korea. Emerg. Infect. Dis. 24, 1768–1770. doi: 10.3201/eid2409.180625
Corzo-Leon D. E., Peacock M., Rodriguez-Zulueta P., Salazar-Tamayo G. J., MacCallum D. M. (2021). General hospital outbreak of invasive candidiasis due to azole-resistant Candida parapsilosis associated with an Erg11 Y132F mutation. Med. Mycol. 59, 664–671. doi: 10.1093/mmy/myaa098
Cotreau M. M., Warren S., Ryan J. L., Fleckenstein L., Vanapalli S. R., Brown K. R., et al. (2003). The antiparasitic moxidectin: safety, tolerability, and pharmacokinetics in humans. J. Clin. Pharmacol. 43, 1108–1115. doi: 10.1177/0091270003257456
Demers E. G., Stajich J. E., Ashare A., Occhipinti P., Hogan D. A. (2021). Balancing positive and negative selection: In vivo evolution of Candida lusitaniae MRR1. mBio 12, e03328–e03320. doi: 10.1128/mBio.03328-20
Fekkar A., Blaize M., Bouglé A., Normand A.-C., Raoelina A., Kornblum D., et al. (2021). Hospital outbreak of fluconazole-resistant Candida parapsilosis: arguments for clonal transmission and long-term persistence. Antimicrob. Agents Chemother. 65, e02036–e02020. doi: 10.1128/AAC.02036-20
Ferrari S., Ischer F., Calabrese D., Posteraro B., Sanguinetti M., Fadda G., et al. (2009). Gain-of-function mutations in CgPDR1 of Candida glabrata not only mediate antifungal resistance but also enhance virulence. PLoS Pathog. 5, e1000268. doi: 10.1371/journal.ppat.1000268
Flowers S. A., Colón B., Whaley S. G., Schuler M. A., Rogers P. D. (2015). Contribution of clinically derived mutations in ERG11 to azole resistance in Candida albicans. Antimicrob. Agents Chemother. 59, 450–460. doi: 10.1128/AAC.03470-14
Govender N. P., Patel J., Magobo R. E., Naicker S., Wadula J., Whitelaw A., et al. (2016). Emergence of azole-resistant Candida parapsilosis causing bloodstream infection: results from laboratory-based sentinel surveillance in south Africa. J. Antimicrob. Chemother. 71, 1994–2004. doi: 10.1093/jac/dkw091
Grossman N. T., Pham C. D., Cleveland A. A., Lockhart S. R. (2015). Molecular mechanisms of fluconazole resistance in Candida parapsilosis isolates from a U.S. surveillance system. Antimicrob. Agents Chemother. 59, 1030–1037. doi: 10.1128/AAC.04613-14
Guo J., Zhang M., Qiao D., Shen H., Wang L., Wang D., et al. (2021). Prevalence and antifungal susceptibility of Candida parapsilosis species complex in Eastern China: A 15-year retrospective study by ECIFIG. Front. Microbiol. 12. doi: 10.3389/fmicb.2021.644000
Healey K. R., Zhao Y., Perez W. B., Lockhart S. R., Sobel J. D., Farmakiotis D., et al. (2016). Prevalent mutator genotype identified in fungal pathogen Candida glabrata promotes multi-drug resistance. Nat. Commun. 7, 11128. doi: 10.1038/ncomms11128
Iyer K. R., Camara K., Daniel-Ivad M., Trilles R., Pimentel-Elardo S. M., Fossen J. L., et al. (2020). An oxindole efflux inhibitor potentiates azoles and impairs virulence in the fungal pathogen Candida auris. Nat. Commun. 11, 6429. doi: 10.1038/s41467-020-20183-3
Kakeya H., Shibata W., Yamada K., Kaneko Y. (2019). National trends in the Japanese distribution of major Candida species causing candidemia during 2003–2017: A report by the epidemiological investigation committee for human mycoses in Japan. Open Forum Infect. Dis. 6 (Suppl. 2), S142. doi: 10.1093/ofid/ofz360.329
Livak K. J., Schmittgen T. D. (2001). Analysis of relative gene expression data using real-time quantitative PCR and the 2(-delta delta C(T)) method. Methods 25, 402–408. doi: 10.1006/meth.2001.1262
Martini C., Torelli R., de Groot T., De Carolis E., Morandotti G. A., De Angelis G., et al. (2020). Prevalence and clonal distribution of azole-resistant Candida parapsilosis isolates causing bloodstream infections in a Large Italian hospital. Front. Cell. Infect. Microbiol. 10. doi: 10.3389/fcimb.2020.00232
Nishimoto A. T., Sharma C., Rogers P. D. (2020). Molecular and genetic basis of azole antifungal resistance in the opportunistic pathogenic fungus Candida albicans. J. Antimicrob. Chemother. 75, 257–270. doi: 10.1093/jac/dkz400
Nucci M., Queiroz-Telles F., Alvarado-Matute T., Tiraboschi I. N., Cortes J., Zurita J., et al. (2013). Epidemiology of candidemia in Latin America: A laboratory-based survey. PLoS One 8, e59373. doi: 10.1371/journal.pone.0059373
Pekmezovic M., Hovhannisyan H., Gresnigt M. S., Iracane E., Oliveira-Pacheco J., Siscar-Lewin S., et al. (2021). Candida pathogens induce protective mitochondria-associated type I interferon signalling and a damage-driven response in vaginal epithelial cells. Nat. Microbiol. 6, 643–657. doi: 10.1038/s41564-021-00875-2
Pfaller M. A., Diekema D. J. (2012). Progress in antifungal susceptibility testing of Candida spp. by use of clinical and laboratory standards institute broth microdilution methods 2010 to 2012. J. Clin. Microbiol. 50, 2846–2856. doi: 10.1128/JCM.00937-12
Silva L. V., Sanguinetti M., Vandeputte P., Torelli R., Rochat B., Sanglard D. (2013). Milbemycins: more than efflux inhibitors for fungal pathogens. Antimicrob. Agents Chemother. 57, 873–886. doi: 10.1128/AAC.02040-12
Singh A., Singh P. K., de Groot T., Kumar A., Mathur P., Tarai B., et al. (2019). Emergence of clonal fluconazole-resistant Candida parapsilosis clinical isolates in a multicentre laboratory-based surveillance study in India. J. Antimicrob. Chemother. 74, 1260–1268. doi: 10.1093/jac/dkz029
Siopi M., Tarpatzi A., Kalogeropoulou E., Damianidou S., Vasilakopoulou A., Vourli S., et al. (2020). Epidemiological trends of fungemia in Greece with a focus on candidemia during the recent financial crisis: A 10-year survey in a tertiary care academic hospital and review of literature. Antimicrob. Agents Chemother. 64, e01516-19. doi: 10.1128/AAC.01516-19
Souza A. C. R., Fuchs B. B., Pinhati H. M. S., Siqueira R. A., Hagen F., Meis J. F., et al. (2015). Candida parapsilosis resistance to fluconazole: Molecular mechanisms and in vivo impact in infected Galleria mellonella larvae. Antimicrob. Agents Chemother. 59, 6581–6587. doi: 10.1128/AAC.01177-15
Thomaz D. Y., de Almeida J. N. J., Lima G. M. E., Nunes M., de O., Camargo C. H., et al. (2018). An azole-resistant Candida parapsilosis outbreak: Clonal persistence in the intensive care unit of a Brazilian teaching hospital. Front. Microbiol. 9. doi: 10.3389/fmicb.2018.02997
Thomaz D. Y., de Almeida J. N. J., Sejas O. N. E., Del Negro G. M. B., Carvalho G. O. M. H., Gimenes V. M. F., et al. (2021). Environmental clonal spread of azole-resistant Candida parapsilosis with Erg11-Y132F mutation causing a large candidemia outbreak in a Brazilian cancer referral center. J. Fungi 7, 259. doi: 10.3390/jof7040259
Keywords: Candida parapsilosis, outbreak, candidemia, fluconazole resistance, fluconazole potentiation
Citation: Daneshnia F, Hilmioğlu Polat S, Ilkit M, Shor E, de Almeida Júnior JN, Favarello LM, Colombo AL, Arastehfar A and Perlin DS (2022) Determinants of fluconazole resistance and the efficacy of fluconazole and milbemycin oxim combination against Candida parapsilosis clinical isolates from Brazil and Turkey. Front. Fungal Biol. 3:906681. doi: 10.3389/ffunb.2022.906681
Received: 28 March 2022; Accepted: 11 July 2022;
Published: 28 July 2022.
Edited by:
Kaustuv Sanyal, Jawaharlal Nehru Centre for Advanced Scientific Research, IndiaReviewed by:
Aylin Döğen, Mersin University, TurkeyShahram Mahmoudi, Iran University of Medical Sciences, Iran
Emeka Nweze, University of Nigeria, Nsukka, Nigeria
Fatemeh Ahangarkani, Mazandaran University of Medical Sciences, Iran
Copyright © 2022 Daneshnia, Hilmioğlu Polat, Ilkit, Shor, de Almeida Júnior, Favarello, Colombo, Arastehfar and Perlin. This is an open-access article distributed under the terms of the Creative Commons Attribution License (CC BY). The use, distribution or reproduction in other forums is permitted, provided the original author(s) and the copyright owner(s) are credited and that the original publication in this journal is cited, in accordance with accepted academic practice. No use, distribution or reproduction is permitted which does not comply with these terms.
*Correspondence: Amir Arastehfar, a.arastehfar.nl@gmail.com; David S. Perlin, David.perlin@hmh-cdi.org
†ORCID: Farnaz Daneshnia, orcid.org/0000-0002-8782-2036
Süleyha Hilmioğlu Polat, orcid.org/0000-0001-8850-2715
Macit Ilkit, orcid.org/0000-0002-1174-4182
Erika Shor, orcid.org/0000-0003-3655-0963
João Nobrega de Almeida Júnior, orcid.org/0000-0002-3766-026X
Arnaldo Lopes Colombo, orcid.org/0000-0003-0793-8491
Amir Arastehfar, orcid.org/0000-0002-4361-4841
David S. Perlin, orcid.org/0000-0002-9503-3184