- 1Research Group Mycology, Department of Biology, Ghent University, Ghent, Belgium
- 2Institute of Biological Sciences, Cardinal Stefan Wyszyński University, Warsaw, Poland
- 3Farlow Reference Library and Herbarium of Cryptogamic Botany, Harvard University, Cambridge, MA, United States
- 4Faculty of Science, University of South Bohemia, České Budějovice, Czechia
- 5Biology Centre of the Czech Academy of Sciences, Institute of Entomology, České Budějovice, Czechia
Laboulbeniales (Ascomycota, Laboulbeniomycetes) are biotrophic microfungi always attached to the exoskeleton of their arthropod hosts. They do not form hyphae or a mycelium; instead, they undergo determinate growth, developing from a two-celled ascospore to form a multicellular thallus. Hesperomyces virescens has been reported on over 30 species of ladybirds (Coleoptera, Coccinellidae); in reality, it represents a complex of species, presumably segregated by host genus association. In this study, we report on Hesperomyces thalli on Hyperaspis vinciguerrae from the Canary Islands and compare them with the Hesperomyces hyperaspidis described on Hyperaspis sp. from Trinidad. We generated the sequences of the internal transcribed spacer (ITS) region, the large subunit (LSU) nuclear ribosomal RNA gene, and the minichromosome maintenance complex component 7 (MCM7) protein-coding gene. Our phylogenetic reconstruction of Hesperomyces based on a concatenated ITS–LSU–MCM7 dataset revealed Hesperomyces sp. ex Hy. vinciguerrae as a member of the He. virescens species complex distinct from He. virescens sensu stricto (s.s.). It also revealed that the Hesperomyces sp. ex Chilocorus bipustulatus from Algeria is different from He. virescens s.s., which is associated with Chilocorus stigma from the USA. This suggests that the species of Hesperomyces are not solely segregated by host association, but that there is also a biogeographical component involved. Based on these data, we refrained from referring our material from Hy. vinciguerrae to He. hyperaspidis. Finally, we discuss the usefulness of MCM7 as a useful marker for species delimitation in Hesperomyces.
Introduction
There are various symbiotic interactions among insects and fungi, ranging from mutualistic, such as those of the fungus-farming leafcutter ants in the genera Acromyrmex and Atta (Hymenoptera, Formicidae), to pathogenic and parasitic, such as those of Beauveria spp. (Ascomycota, Sordariomycetes) and Laboulbeniales (Ascomycota, Laboulbeniomycetes) (Bustamante et al., 2019; Biedermann and Vega, 2020; Haelewaters et al., 2021b). The species of Laboulbeniales are biotrophic microfungi growing externally on the exoskeleton of their arthropod hosts. They are characterized by the formation of multicellular thalli instead of hyphae and a mycelium (Blackwell et al., 2020; Haelewaters et al., 2021a). The study of Laboulbeniales has been neglected by mycologists due to their minute size, insignificant morphological appearance, and inability to grow in axenic culture (Haelewaters et al., 2021b). Recent molecular work has indicated that morphology alone is not sufficient to delimitate species in several genera of Laboulbeniales: Arthrorhynchus Kolen. (Haelewaters et al., 2020), Chitonomyces Peyr. (Goldmann and Weir, 2012), Coreomyces Thaxt. (Sundberg et al., 2018), Gloeandromyces Thaxt. (Haelewaters and Pfister, 2019), Hesperomyces Thaxt. (Goldmann et al., 2013; Haelewaters et al., 2018), Laboulbenia Mont. and C.P. Robin (Haelewaters et al., 2019a), and Nycteromyces Thaxt. (Van Caenegem and Haelewaters, unpublished data). In some cases, too many morphological species have been previously recognized. For example, in the genus Chitonomyces, 13 morphological species actually represent six phylogenetic species (Goldmann and Weir, 2012). Alternatively, too few species have been recognized in other genera. This pertains to cryptic diversity, with one taxon consisting of several morphologically indistinguishable species. An example of this can be found in the genus Hesperomyces (Haelewaters et al., 2018).
The genus Hesperomyces was erected by Thaxter (1891) to accommodate Hesperomyces virescens Thaxt. on Chilocorus stigma (Say, 1835). Since its description, He. virescens, in a broad sense, has been reported on more than 30 species of ladybirds (Coleoptera, Coccinellidae) (Haelewaters and De Kesel, 2017). Haelewaters et al. (2018) revealed that He. virescens is a complex of species segregated according to host association based on landmark-based geometric, morphometric, ecological, and molecular phylogenetic data. Since then, three species within the complex have been described: Hesperomyces halyziae (Haelewaters and De Kesel, 2020), Hesperomyces harmoniae (Haelewaters et al., 2022b), and Hesperomyces parexochomi (Crous et al., 2021).
In this paper, we present the first molecular data for He. virescens sensu lato (s.l.) on Hyperaspis and expand our understanding of the diversity within this complex. We also evaluate the usefulness of the minichromosome maintenance complex component 7 (MCM7) gene as a secondary barcode for Laboulbeniales.
Materials and methods
Collection of insects and morphological study
Ladybirds were collected on the island of Fuerteventura, Canary Islands, Spain. Specimens were shaken down from host plants on a 1-m × 1-m white beating sheet and preserved in 90% ethanol. Identification of the ladybirds was based on morphological and anatomical features, including the type of reproductive organs (Romanowski et al., 2019).
Thalli were removed from the left elytron under a Novex RZB-PL 65.500 dissecting microscope (Novex, Arnheim, Netherlands) at ×10 to ×45 magnification using Minutien pins (#1208SA; BioQuip, Rancho Dominguez, CA, USA) inserted onto wooden rods. Permanent slides were made using the double-coverslip mounting technique as described by Liu et al. (2020), with one modification: the thalli were placed in a droplet of 1:1 Hoyer’s medium/glycerin mixture instead of pure Hoyer’s medium because our Hoyer’s medium dried quickly. Mounted thalli were viewed at ×200 to ×1,000 magnification under an Olympus BH-2 microscope (Olympus, Center Valley, PA, USA). Images were generated with a Nikon DS-Fi3 microscopy camera mounted on an Eclipse Ni-U compound microscope (Nikon, Nelville, NY, USA) equipped with differential interference contrast (DIC) optics and processed using NIS-Elements BR 5.0.03 imaging software (Nikon).
The thalli, cells, structures, and ascospores were measured using ImageJ 1.51h Image Processing and Analysis software (Abramoff et al., 2004). All measurements were taken as described and illustrated by Haelewaters et al. (2018). Measurements in the morphological description were noted as (a–)b–c–d(–e) [n], with a and e as extreme values, b and d denote the mean ± standard deviation, c represents the mean, and n is the number of structures measured. Ladybirds were preserved at the Purdue Entomology Research Collection, West Lafayette, Indiana, USA (PERC), and permanent slides of Laboulbeniales were deposited in the Herbarium Universitatis Gandavensis, Ghent, Belgium (GENT).
DNA extraction, PCR amplification, and sequencing
DNA extractions were performed using the REPLI-g Single Cell Kit (Qiagen, Stanford, CA, USA). A Minutien pin inserted onto a wooden rod for holdfast was submerged in glycerin to prevent the thalli from flying away during transfer. The thalli were detached from the host and placed in a droplet of glycerin on a microscope slide. To ensure successful lysis, we cut every perithecium transversally once using a no. 10 surgical blade on a disposable Bard-Parker handle (Aspen Surgical, Caledonia, MI, USA). The thalli were then placed in 0.2-ml PCR tubes with 4 µl of phosphate-buffered saline. After the addition of 3 ml of prepared D2 buffer, the tubes were incubated at 65°C for 30 min. Subsequent steps followed the manufacturer’s instructions (Qiagen).
The small subunit (SSU), the internal transcribed region (ITS), and the large subunit (LSU) of the nuclear ribosomal RNA gene were amplified using the following primer pairs: NSL1/NSL2 for SSU (Haelewaters et al., 2015), ITShespL/ITShespR for ITS (Haelewaters et al., 2019b), and LR0R/LR5 for LSU (Vilgalys and Hester, 1990; Hopple, 1994). Additionally, the MCM7 protein-coding gene was amplified using the primer pair MCM7-709for/MCM7-1384rev (Schmitt et al., 2009). The PCR reactions (25 µl in total) consisted of 13.3 µl of RedExtract Taq polymerase (Sigma-Aldrich, St. Louis, MO, USA), 2.5 µl of each 10 µM primer, 5.45 µl of ddH2O, and 1 µl of DNA extract. The PCR cycling conditions used were as follows: for SSU, an initial denaturation at 94°C for 5 min; 39 cycles of denaturation at 94°C for 30 s, annealing at 50°C for 45 s, and extension at 72°C for 90 s; and a final extension at 72°C for 10 min; for ITS, an initial denaturation at 94°C for 3 min; 34 cycles of denaturation at 94°C for 1 min, annealing at 50°C for 45 s, and extension at 72°C for 90 s; and a final extension at 72°C for 10 min; for LSU, an initial denaturation at 94°C for 5 min; 34 cycles of denaturation at 94°C for 30 s, annealing at 50°C for 45 s, and extension at 72°C for 1 min; and a final extension at 72°C for 7 min; for MCM7, an initial denaturation at 94°C for 5 min; 10 cycles of denaturation at 94°C for 45 s, annealing at 55°C (−1°C/cycle) for 50 s, and extension at 72°C for 1 min; 24 cycles of denaturation at 94°C for 45 s, annealing at 47°C for 50 s, and extension at 72°C for 1 min; and a final extension at 72°C for 5 min.
The PCR products were purified using 1.5 µl of Exo-FAP (0.5 µl exonuclease I and 1 µl FAST alkaline phosphatase) (Thermo Fisher Scientific, Waltham, MA, USA) per 10 µl of PCR product at 37°C for 15 min, followed by deactivation at 85°C for 15 min. The purified PCR products were sequenced using an automated ABI 3730 XL capillary sequencer (Life Technology at Macrogen, Amsterdam, Netherlands). The forward and reverse sequence reads were assembled and edited in Sequencher version 5.4.6 (Gene Codes Corporation, Ann Arbor, MI, USA). Newly generated sequences were submitted to NCBI GenBank (https://www.ncbi.nlm.nih.gov/genbank/; accession numbers are shown in Table 1).
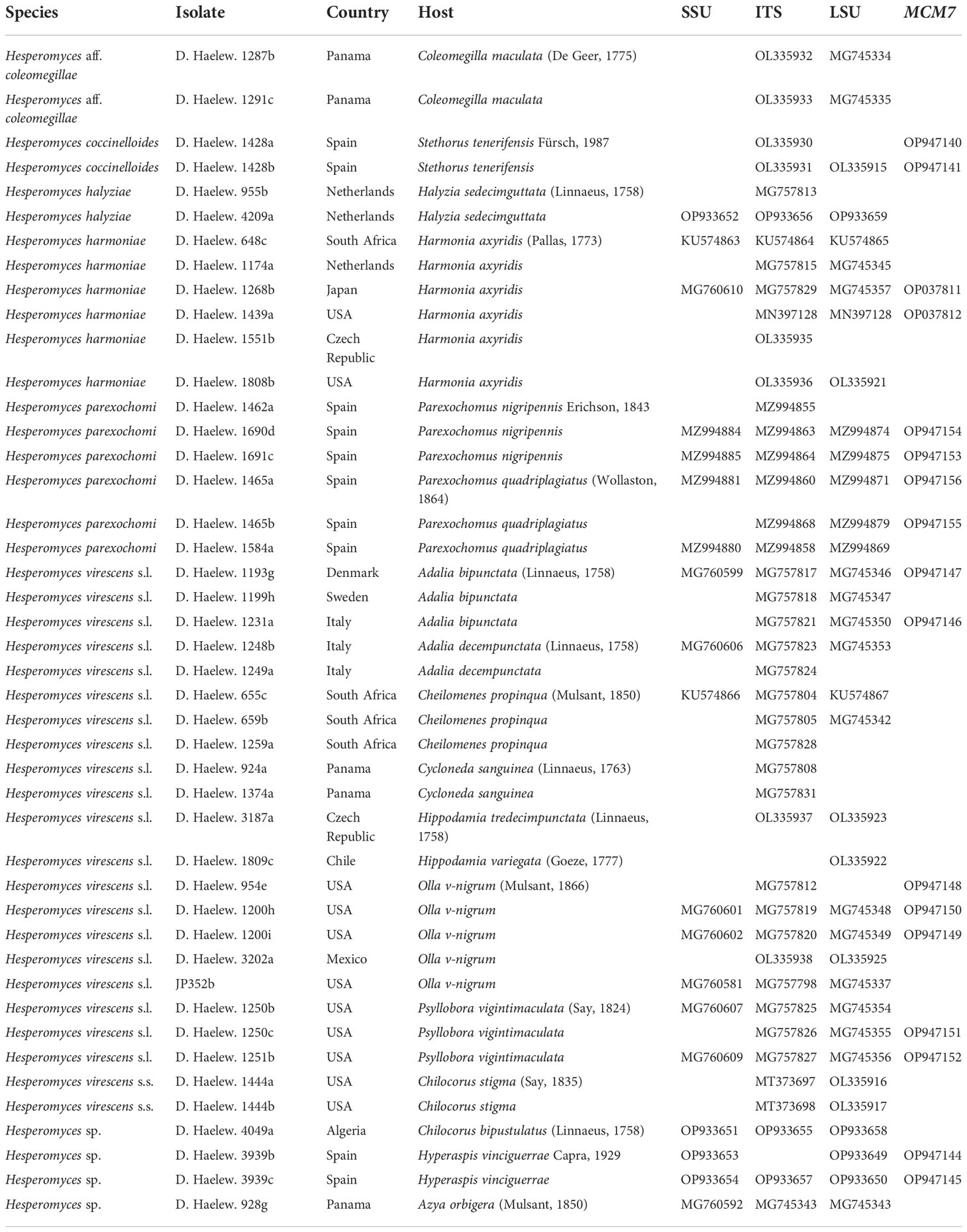
Table 1 Details of all the isolates used in the study, including species name, country of collection, host species, and the GenBank accession numbers of the small subunit (SSU), internal transcribed spacer (ITS), large subunit (LSU), and minichromosome maintenance complex component 7 (MCM7) sequences.
Phylogenetic analyses
Newly generated sequences were supplemented by the sequences downloaded from NCBI GenBank, resulting in 19 SSU, 42 ITS, 35 LSU, and 17 MCM7 sequences (Table 1). We used Hesperomyces ex Azya orbigera Mulsant, 1850, Hesperomyces coccinelloides (Thaxt.) Thaxt., and Hesperomyces coleomegillae W. Rossi and A. Weir as outgroups (Haelewaters et al., 2022b). We aligned the sequences by locus with the E-INS-i strategy using MAFFT (Multiple Alignment using Fast Fourier Transform) version 7 (Kuraku et al., 2013; Katoh et al., 2019). The sequences were manually trimmed using the BioEdit Sequence Alignment Editor version 7.2.6 (Hall, 1999) and concatenated in SequenceMatrix 1.9 (Vaidya et al., 2011).
We constructed five datasets: each marker individually (ITS, LSU, and MCM7) and two concatenated datasets (ITS–LSU and ITS–LSU–MCM7). The ITS dataset was partitioned into the ITS1 spacer, the conserved 5.8S gene, and the ITS2 spacer. The concatenated three-locus dataset included five partitions: the ITS1 and ITS2 spacer regions, the 5.8S gene, LSU, and MCM7. Likewise, the concatenated two-locus dataset included four partitions (ITS1, 5.8S, ITS2, and LSU). Following previous work in this species complex (Haelewaters et al., 2018; Haelewaters et al., 2022b), we only used a selection of publicly available sequences for the ITS, LSU, and the concatenated datasets. To examine the utility of MCM7 as a secondary barcode marker, we did use all available (17) Hesperomyces sequences in the single-partition dataset of this marker.
Models for nucleotide substitution were selected for each partition using ModelFinder (Kalyaanamoorthy et al., 2017) according to the corrected Akaike information criterion (AICc). Maximum likelihood (ML) analyses were inferred using IQ-TREE (Nguyen et al., 2015) under partitioned models (Chernomor et al., 2016). Ultrafast bootstrapping was performed with 1,000 replicates (Hoang et al., 2018). Phylogenetic trees were visualized in FigTree (http://tree.bio.ed.ac.uk/software/figtree/) and edited using Inkscape (http://www.inkscape.org).
Results
We generated 26 new sequences for this study (Table 1). The concatenated ITS–LSU–MCM7 dataset consisted of 2,362 characters for 44 isolates. The selected models for each partition in our concatenated dataset were: TPM2+F+G4 (ITS1, 414 bp; −lnL = 4,130.865), TNe (5.8S, 160 bp; −lnL = 579.759), TIM2+F+G4 (ITS2, 272 bp; −lnL = 3,194.845), TIM+F+G4 (LSU, 894 bp; −lnL = 4,549.320), and TIM3e+G4 (MCM7, 622 bp; −lnL = 2,790.124). The models for the unpartitioned alignments and the concatenated ITS–LSU dataset were identical. The reconstructed phylogenies of He. virescens s.l. are shown in Figure 1 (for the concatenated ITS–LSU–MCM7 dataset), Figure 2 (for the concatenated ITS–LSU dataset), and Figure 3 (for the ITS, LSU, and MCM7 datasets). The He. virescens s.l. complex consists of 12 lineages, each with maximum support. The SSU sequences are too conserved and cannot be used to delimit species or to identify isolates to the species level (Supplementary File 1).
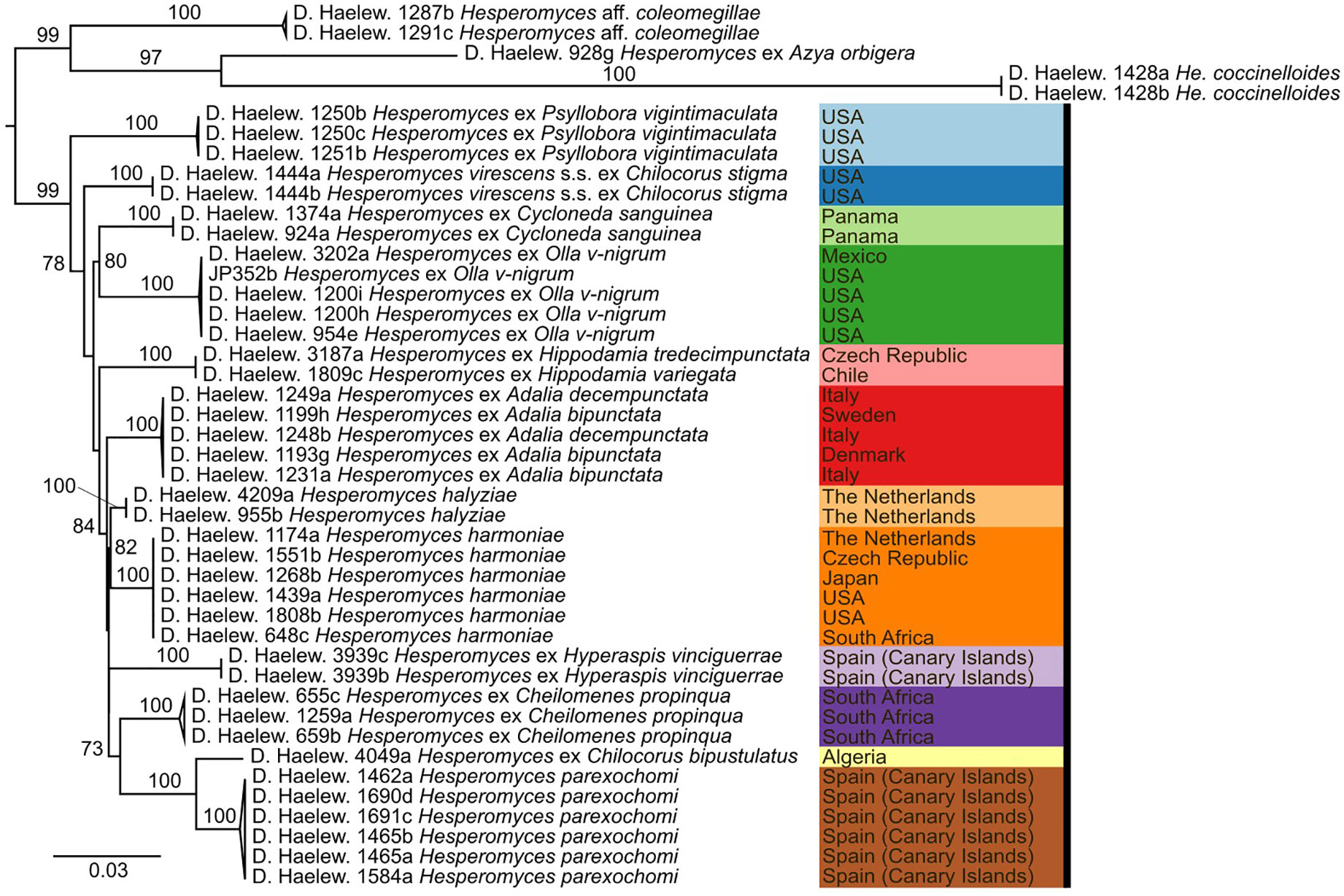
Figure 1 Phylogenetic tree obtained from the maximum likelihood (ML) analysis of a three-locus (ITS–LSU–MCM7) dataset. For every node, the ML bootstrap value (≥70) is given above or next to the branch leading to that node. Species within the Hesperomyces virescens complex are each indicated with their own color. Color scheme from https://colorbrewer2.org by C.A. Brewer, Geography, Pennsylvania State University.
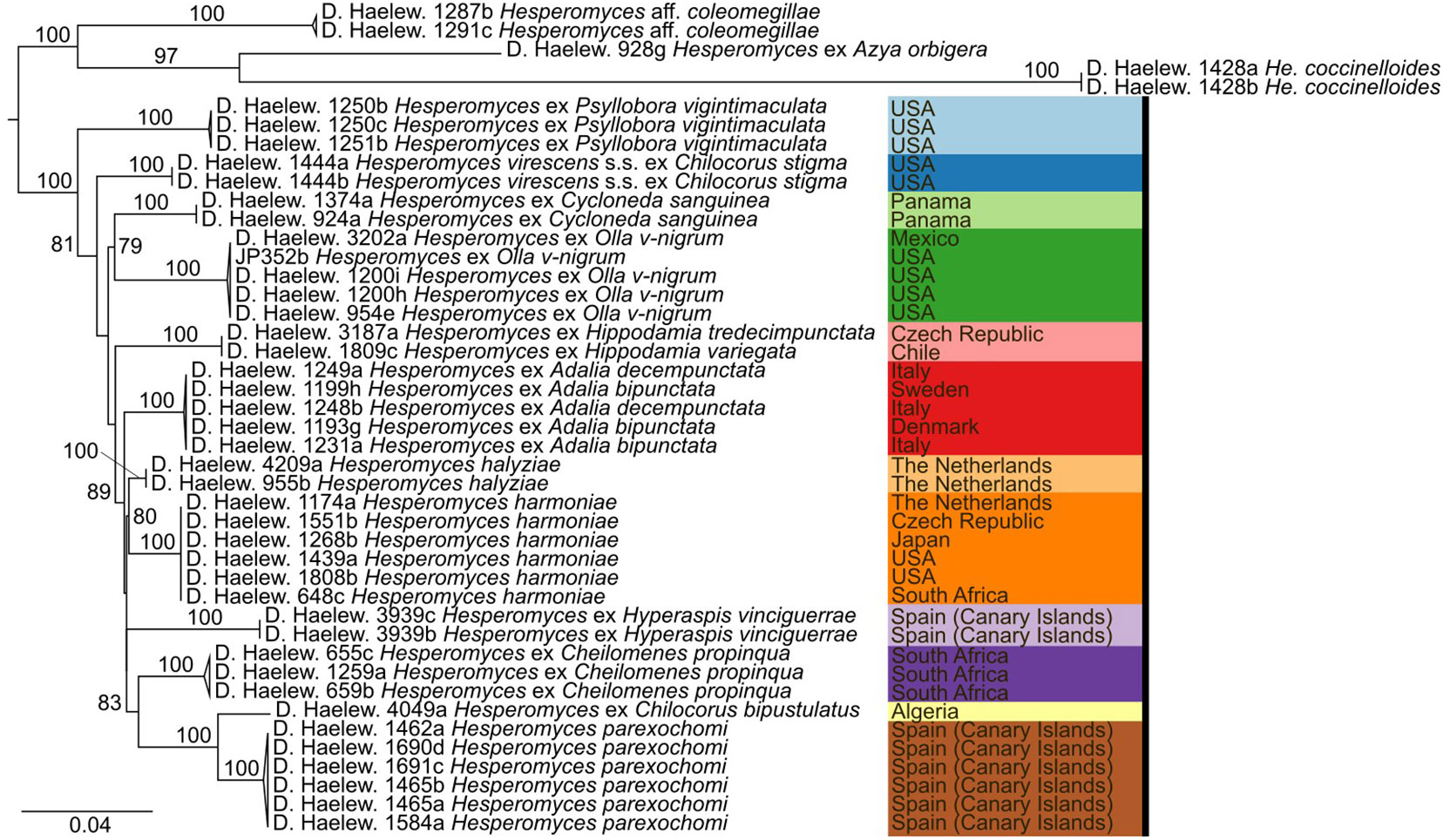
Figure 2 Phylogenetic tree obtained from the maximum likelihood (ML) analysis of a two-locus (ITS–LSU) dataset. For every node, the ML bootstrap value (≥70) is given above or next to the branch leading to that node. Species within the Hesperomyces virescens complex are each indicated with their own color, as in Figure 1.
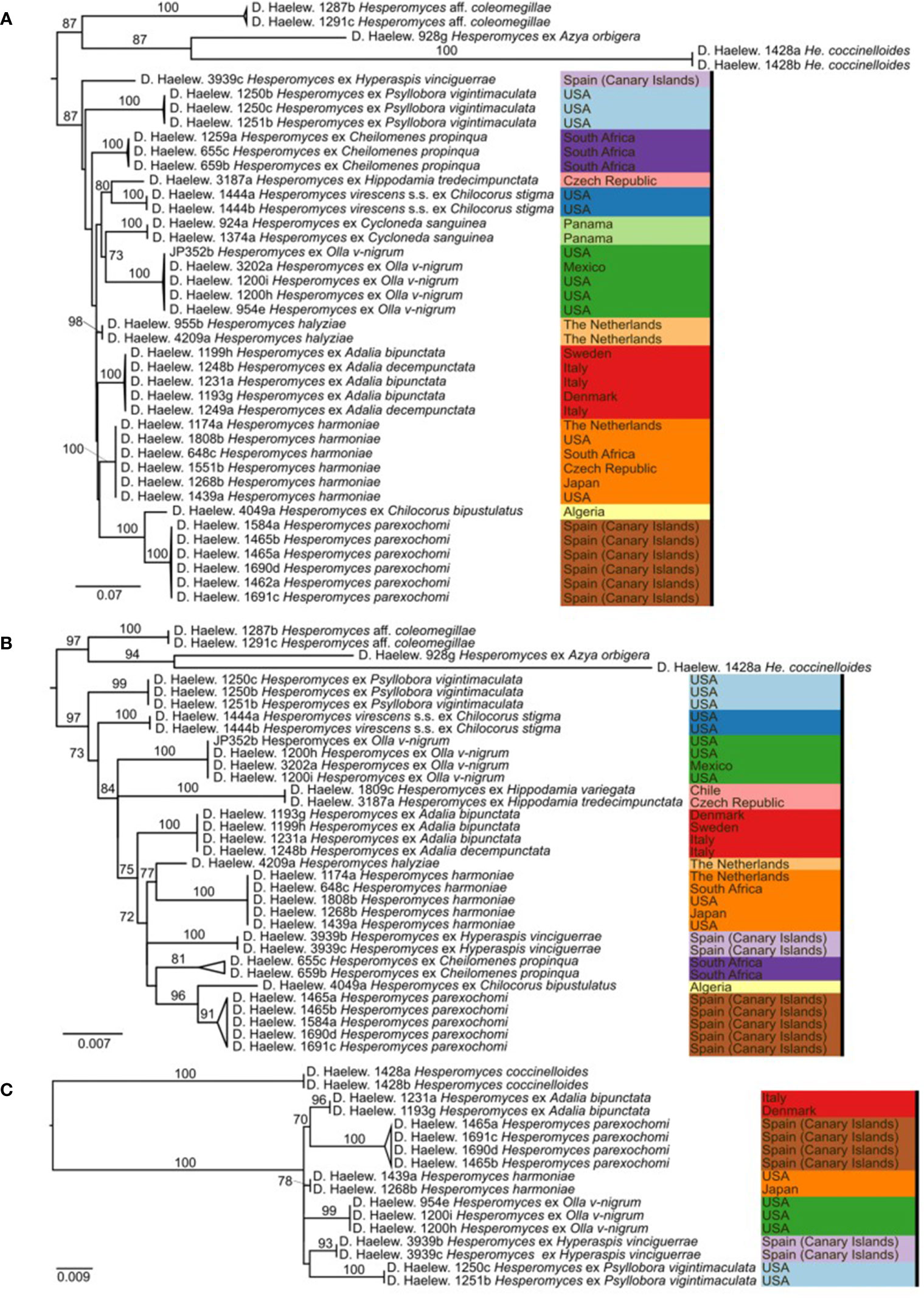
Figure 3 Phylogenies of the Hesperomyces virescens species complex, reconstructed from the internal transcribed spacer (ITS) (A), large subunit (LSU) (B), and minichromosome maintenance complex component 7 (MCM7) (C) datasets. For every node, the ML bootstrap value (≥70) is given above or next to the branch leading to that node. Species within the He. virescens complex are each indicated with their own color, as in Figure 1.
Taxonomy
Hesperomyces hyperaspidis Thaxt., Mem. Am. Acad. Arts Sci., ser. 2 16(1):111 (1931)
Material examined
Trinidad and Tobago. Trinidad Island, vicinity of the City of Port of Spain, no date, leg. R. Thaxter, on Hyperaspis sp. (Coleoptera, Coccinellidae) (no. 2896), slide FH 4989 (holotype at FH, one mature thallus and one broken thallus from elytra); Northern Range hills, slopes of El Tucuche, April 1929, leg. P.J. Darlington, on Hyperaspis sp. (Coleoptera, Coccinellidae) (no. 3637), slide FH 4990 (FH, one mature and two submature thalli).
Hosts and distribution
Described on Hyperaspis sp. from Trinidad and reported on Hyperapsis festiva Mulsant, 1850, from French Guiana (Bernardi et al., 2014), although only studied based on morphology.
Notes
The description of this species was based on only one mature thallus (Thaxter, 1931); thus, variations in morphology could not be evaluated. According to Thaxter (1931), He. hyperaspidis differs from other species of Hesperomyces by the length of the six lobes at the perithecial tip (see Figure 4). Bernardi et al. (2014) evaluated the usefulness of this morphological characteristic and concluded that the lengths of the lobes were overlapping among species. As a result, these authors synonymized He. hyperaspidis with He. virescens.
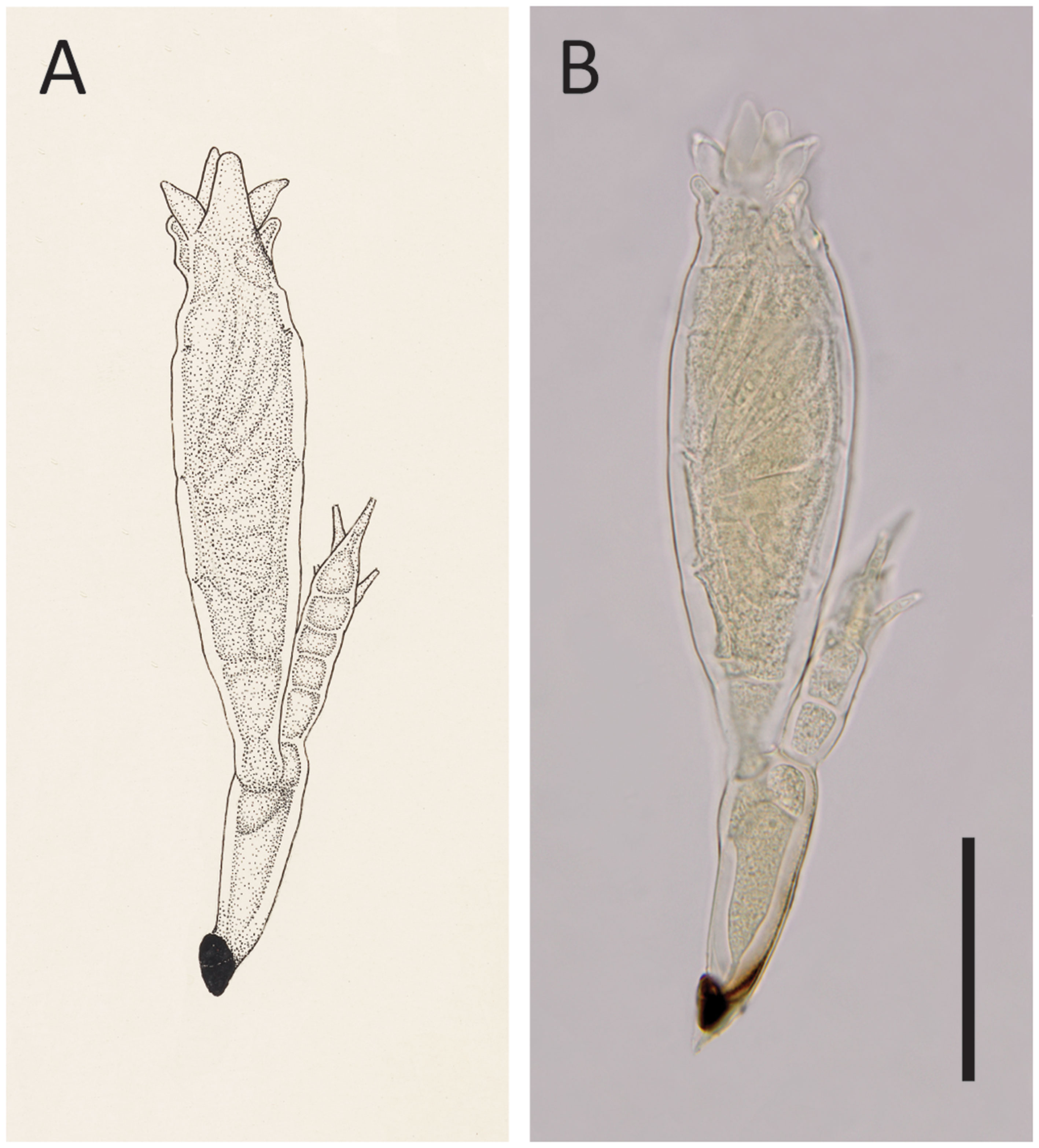
Figure 4 Hesperomyces hyperaspidis Thaxt., slide FH 4989 (holotype). (A) An enlargement of Thaxter’s (1931) original Plate XX: Figure 22. Courtesy of the Archives of the Farlow Herbarium of Cryptogamic Botany, Harvard University. (B) Photo of the same thallus that stood model for Thaxter’s drawing. Scale bar 50 μm.
We had access to the protologue material of He. hyperaspidis, which comprises the holotype slide (FH 4989) and one uncited slide (FH 4990) made by Thaxter. Our measurements of the single mature thallus in the holotype slide differed from those in the protologue (Thaxter, 1931). Thaxter’s measurements are sometimes at variance with modern work; Haelewaters and Rossi (2015) reported on measurements of thalli that differed from those reported by Thaxter. A comparison of these measurements is given in Table 2.

Table 2 Measurement of the different structures of the single mature thallus of Hesperomyces hyperaspidis in Thaxter’s holotype slide, as described in Thaxter (1931) and re-measured by us.
Hesperomyces sp. ex Hyperaspis vinciguerrae
Material examined
Spain. Canary Islands, Las Palmas, Fuerteventura Island, Jandia, Ventura Shopping Center, 28.053 N, 14.324 W, November 27, 2021, leg. Jerzy Romanowski, on Hy. vinciguerrae Capra, 1929 (PERC), slide D. Haelew. 3939a (GENT, nine mature thalli from the left elytron), and D. Haelew. 3939d (GENT, 20 mature thalli from the left elytron).
Description
The thallus is (174–)200–222–244(–254) μm long from the foot to the perithecial apex; hyaline to yellowish green [29]. The receptacle is (41–)45–48–51(–53) μm long [29]. Cell I is (28–)34–36–38(–39) × (13–)14–15–16(–17) μm, triangular to quadrangular, and is longer than broad [28]. Cell II is (15–)17–18–19(–20) × (9–)10–11–12(–13) μm, longer than broad, and subtrapezoidal [22]. Cell III is (11–)12–13–14(–16) × (8–)9–11–13(–16) μm, shorter than cell II, almost isodiametric, and is dorsally convex [26]. The primary appendage is (38–)43–45–47(–51) μm long, consisting of four superposed cells, with a distinct constricted septum between cell III and the basal cell. The basal cell is (12–)13–14–15(–17) μm long, longer than any of the other cells of the appendage, the remaining cells each bearing one antheridium directed outwardly and the uppermost cell bearing one or two antheridia and a terminal spinous process, which is the original ascospore apex [21]. The antheridia are (13–)16–17–18(–20) μm long, with outwardly straight to curved efferent necks, (6–)7–8–9(–10) μm [22]. Cell VI is (22–)25–29–33(–37) × (15–)16–18–20(–22) μm, rather stout, broadening distally [29]. The perithecium is (120–)134–152–169(–178) × (28–)31–37–43(–47) μm, on average four times longer than broad, asymmetric, fusiform, and broadest near the middle, then gradually tapering toward a short, broad, indistinct neck and an asymmetrical apex. The septa between the horizontal tiers of wall cells are marked by constrictions. The perithecial tip has two lower lobes, two upper lobes, and two prominent lips surrounding the ostiole; lower lobes are minute, while the upper lobes are (10–)11–12–13(–14) μm long, unicellular, thumb-like, usually curved outwards, and their tips not exceeding the perithecial apex. The ostiole has two lips, one lip triangular and the other slightly shorter, rounded [29]. The ascospores are two-celled, (46–)48–53–57(–57) × 4.1–4.6–5.1(–5.3) μm, with a gelatinous sheath covering the larger cell [7].
Material sequenced
Spain. Canary Islands, Las Palmas, Fuerteventura Island, Jandia, Ventura Shopping Center, 28.053 N, 14.324 W, November 27, 2021, leg. Jerzy Romanowski, on Hy. vinciguerrae (PERC), isolate D. Haelew. 3939b (six mature thalli, left elytron; SSU = OP933653, LSU = OP933649, MCM7 = OP947144); ibid., isolate D. Haelew. 3939c (five mature thalli, left elytron; SSU = OP933654, ITS = OP933657, LSU = OP933650, MCM7 = OP947145).
Hosts and distribution
Reported on Hy. vinciguerrae Capra, 1929, from Spain (this paper).
Notes
Hesperomyces sp. ex Hy. vinciguerrae is part of the near-cryptic He. virescens species complex (Haelewaters et al., 2018). Morphologically, it is very similar to the other species in this complex (Figure 5). Given that our material was associated with a Hyperaspis host, we compared the morphological characteristics with those of He. hyperaspidis. The thalli from our collection had short lobes at the tip of the perithecium, similar to those of He. hyperaspidis described by Thaxter (1931). Most of the thalli were bent in cell VI, mostly at a 90° angle toward anterior. This is different from the available material of He. hyperaspidis, but this difference could be attributed to morphological plasticity. The receptacle of the thallus in the holotype of He. hyperaspidis is 10 µm longer than the mean length of the receptacle we measured in our material. The total length of the thalli we studied is, on average, 30 µm longer than the holotype of He. hyperaspidis.
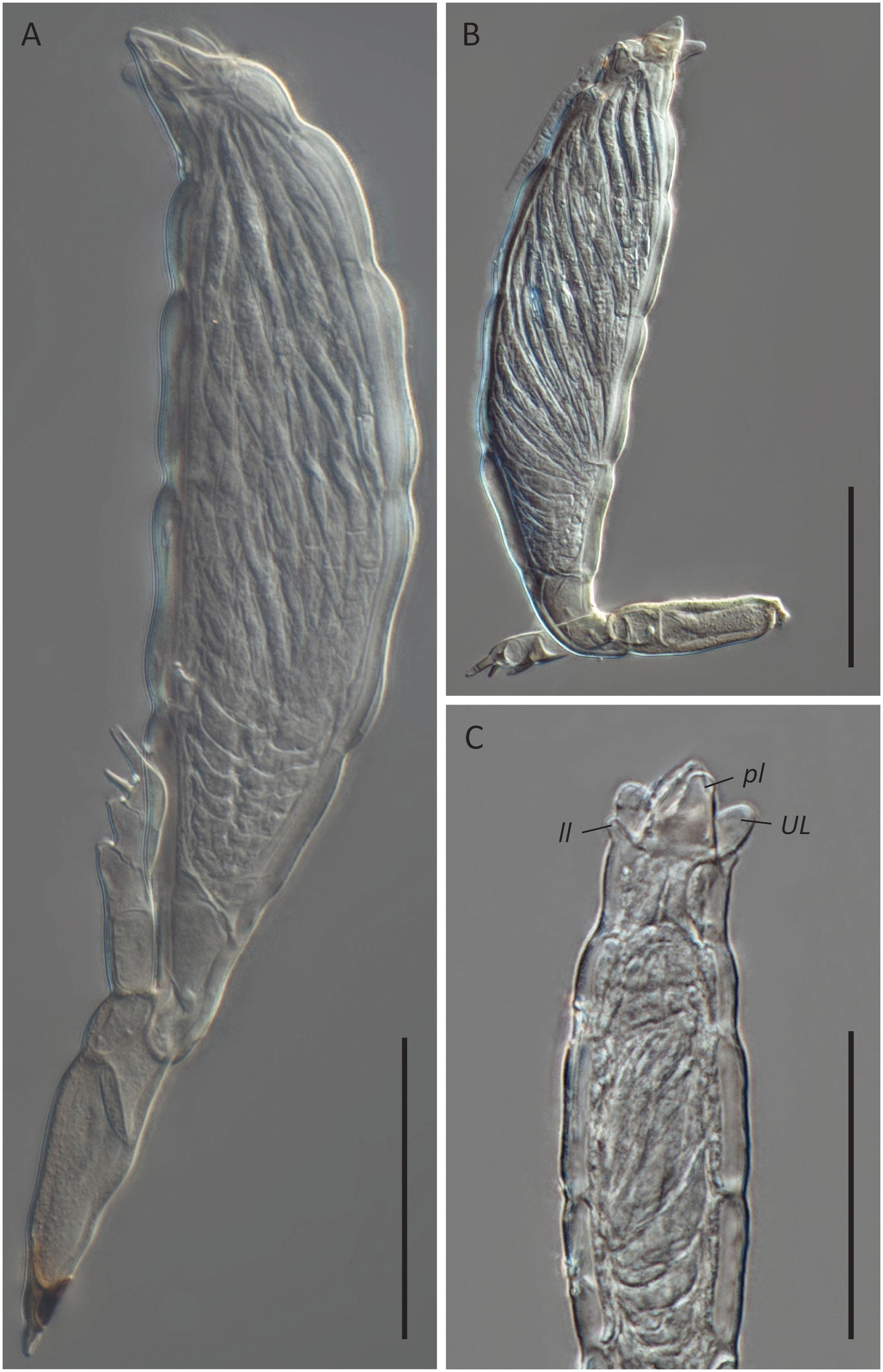
Figure 5 Hesperomyces sp. ex Hyperaspis vinciguerrae. (A) Mature thallus, slide D. Haelew. 3939a. (B) Mature thallus, slide D. Haelew. 3939a. Cell VI is highly bent. (C) Perithecial tip of a mature thallus, slide D. Haelew. 3939d. Indicated are the two lower lobes (ll), the two upper lobes (UL), and the two perithecial lips (pl), similar to Hesperomyces hyperaspidis. Scale bar 50 μm.
Hesperomyces sp. ex Hy. vinciguerrae formed a distinct species-level clade supported by ITS, LSU, and MCM7 data. Unique molecular autapomorphies and motifs in the ITS were found at positions 130–132 (5'-TTC-3') (insertion), 140 (C), 148 (A), 179 (G), 197 (C), 206 (G), 232 (G), 250 (G), 343 (G), 391 (C), 403 (T), 407 (T), 597 (T), 658 (T), 682 (C), 683 (A), 724 (G), 742 (A), 818 (G), and 827 (G); in the LSU at positions 116 (G), 173 (C) (insertion), 176 (A), 416 (T), 436 (C), 480 (C), and 490 (A); and in MCM7 at positions 126 (A), 288 (T), 504 (A), and 528 (A).
Using the NCBI BLAST tool, we searched for sequences with the highest similarity to our newly generated ITS and LSU sequences of Hesperomyces sp. ex Hy. vinciguerrae. For the ITS sequence, these include: He. halyziae [isolate D. Haelew. 955b, GenBank MG757813; identity = 717/765 (94%), 6 (0%) gaps]; He. harmoniae [isolate D. Haelew. 1439a, GenBank MN397128; identity = 720/772 (93%), 8 (1%) gaps]; He. harmoniae [isolate D. Haelew. 1268b, GenBank MG757829; identity = 720/772 (93%), 8 (1%) gaps]; He. harmoniae [isolate D. Haelew. 943b, GenBank MG757810; identity = 720/772 (93%), 8 (1%) gaps]; and He. harmoniae [isolate D. Haelew. 1808b, GenBank OL335936; identity = 720/772 (93%), 8 (1%) gaps]. For the LSU sequences, these are: He. virescens ex Adalia bipunctata [isolate D. Haelew. 1232a, GenBank MG745351; identity = 882/899 (98%), 1 (0%) gap]; He. virescens ex A. bipunctata [isolate D. Haelew. 1199h, GenBank MG745347; identity = 882/899 (98%), 1 (0%) gap]; He. virescens ex A. bipunctata [isolate D. Haelew. 1231a, GenBank MG745350; identity = 882/900 (98%), 2 (0%) gaps]; He. virescens ex A. bipunctata [isolate D. Haelew. 1231a, GenBank MG745350; identity = 882/900 (98%), 2 (0%) gaps]; He. parexochomi [isolate D. Haelew. 1584a, GenBank MZ994870; identity = 882/900 (98%), 2 (0%) gaps]; and He. parexochomi [isolate D. Haelew. 1693a, GenBank MZ994870; identity = 881/899 (98%), 1 (0%) gap].
Discussion
MCM7 as a secondary marker in Laboulbeniales
The ITS region has been proposed as a universal barcode for fungi (Schoch et al., 2012). General fungal ITS primers have been used in a few molecular studies to successfully amplify the ITS region to delimit the species of Laboulbeniales (e.g., Goldmann and Weir, 2012; Goldmann et al., 2013; Sundberg et al., 2018). However, the amplification success of the ITS region substantially differed among the taxa of Laboulbeniales when using general fungal primers such as ITS1f, ITS9mun, and ITS4 (Haelewaters et al., 2015; Haelewaters et al., 2018; Walker et al., 2018). A new primer pair, ITSHespL/ITSHespR, with improved specificity for Hesperomyces was described (Haelewaters et al., 2018). Since this design, we have successfully used these specific primers to amplify the ITS region of Hesperomyces, including in this study.
Recent studies have shown that LSU, in contrast to ITS, is easier to amplify using general fungal primer pairs such as LR0R/LR5, LIC24R/LR3, and NL1/NL4 (Haelewaters et al., 2015; Haelewaters et al., 2018; Walker et al., 2018). Haelewaters et al. (2018) reported that both the ITS and LSU datasets resulted in high support for the species-level clades within He. virescens s.l. and suggested further investigation of LSU as a secondary barcode in Laboulbeniales. Thus far, LSU has been used in several studies delimiting species within Arthrorhynchus, Coreomyces, Gloeandromyces, Hesperomyces, and Laboulbenia (Haelewaters et al., 2018; Sundberg et al., 2018; Haelewaters and Pfister, 2019; Haelewaters et al., 2020; Haelewaters and De Kesel, 2020; Liu et al., 2020).
Liu et al. (2020) generated the first sequences of the translation elongation factor 1α (TEF1) for Gloeandromyces and Nycteromyces species. The phylogeny based on these TEF1 sequences matched the one based on the LSU sequences, with high support for the species clades. To date, no other studies have used TEF1 for species delimitation in Laboulbeniales. We continued to test the amplification success of the TEF1 gene in different genera of Laboulbeniales and have generated sequences for isolates of Arthrorhynchus (seven sequences), Herpomyces Thaxt. (one sequence), Hesperomyces (10 sequences), and Laboulbenia (one sequence) (Haelewaters, unpublished data).
In this study, we expanded on the first efforts by Haelewaters et al. (2022b) to generate the MCM7 sequences of Hesperomyces species. We generated 15 new MCM7 sequences of the Hesperomyces species using the primer pair MCM7-709for/MCM7-1384rev and the two-step touchdown PCR protocol indicated above. The amplification of MCM7 proceeded without issue for all extracts of Hesperomyces in the trial. We also successfully amplified the MCM7 gene for the isolates of Appendiculina (one sequence), Corethromyces (one sequence), Herpomyces (three sequences), Laboulbenia (five sequences), and Nycteromyces (eight sequences) under the same cycling conditions (Van Caenegem and Haelewaters, unpublished data).
Here, we presented the first phylogeny of Hesperomyces based on MCM7. The dataset included Hesperomyces isolates removed from six host species. All isolates were resolved in six monophyletic clades, and each of the tip nodes (species) was highly to maximally supported in the MCM7-based phylogeny (Figure 3C), similar to what Haelewaters et al. (2018) reported for their phylogenetic reconstructions based on ITS and LSU. Based on available data, we can only compare ITS, LSU, and MCM7 for their efficacy to delimit species in Hesperomyces. Support for tip nodes was comparably high in all three markers. Conversely, neither marker has the discriminative power to resolve deeper nodes within the complex—but that was not the goal of this study. Given the difficulty to amplify the ITS region, we suggest exploring the utility of MCM7 as a secondary marker in other genera of the thallus-forming Laboulbeniomycetes. We conclude with the suggestion that MCM7 is a useful region for the delimitation and identification of Hesperomyces species.
Species of Hesperomyces are segregated by host association and geography
All species-level lineages within He. virescens s.l. received maximum support in our multilocus phylogeny (ITS–LSU–MCM7). The species within this complex are morphologically very similar. However, based on our observations, it seems that the thalli and ascospores of Hesperomyces sp. ex Hy. vinciguerrae (thallus, 174–254 µm; ascospores, 46–57 µm) are much smaller than those of He. halyziae (thallus, 335–453 µm; ascospores, 70–85 µm), He. harmoniae (thallus, 290–653 µm; ascospores, 66–106 µm), and He. parexochomi (thallus, 251–441 µm; ascospores, 59–74 µm) (Haelewaters and De Kesel, 2020; Crous et al., 2021; Haelewaters et al., 2022b).
The one-host-one-parasite (1H1P) model states that species of Laboulbeniales with a haustorium, like those in the genus Hesperomyces, have higher host specificity compared to species without a haustorium because the fungi are in closer contact with their host (Haelewaters et al., 2022a). The species within He. virescens s.l. are seemingly segregated by host genus. Support is provided by He. virescens s.l. isolates removed from Adalia, Hippodamia, and Parexochomus spp. An undescribed species of Hesperomyces has been found on Adalia bipunctata (Linnaeus, 1758) and A. decempunctata (Linnaeus, 1758) in Denmark, Italy, and Sweden (Haelewaters et al., 2018). Another undescribed species of Hesperomyces has been found on both Hippodamia variegata (Goeze, 1777) from Chile and Hippodamia tredecimpunctata (Linnaeus, 1758) from the Czech Republic. Finally, He. parexochomi from the Canary Islands is found on two host species: Parexochomus nigripennis Erichson, 1843, and Parexochomus quadriplagiatus (Wollaston, 1864) (Crous et al., 2021). In addition, He. harmoniae is found almost all over the world where its host, Harmonia axyridis (Pallas, 1773), has been introduced, and there have been some observations of Hesperomyces-infected Ha. quadripunctata (Pontoppidan, 1763), although no isolates are available to confirm their identity (Haelewaters et al., 2022b).
In an earlier version of this paper, we planned to reinstate He. hyperaspidis since we thought our thalli from Hy. vinciguerrae belonged to this species. However, during the review process, we generated the sequences of Hesperomyces sp. ex Chilocorus bipustulatus from Algeria. This is an interesting case, as species within the He. virescens complex are thought to be segregated by their host genus association and He. virescens sensu stricto (s.s.) is described from Ch. stigma from the USA (Thaxter, 1891; Haelewaters et al., 2018; Haelewaters et al., 2022b). To our surprise, Hesperomyces sp. ex Ch. bipustulatus does not form a monophyletic clade with He. virescens s.s. ex Ch. stigma and is retrieved sister to He. parexochomi in both the ITS and LSU phylogenies with maximum support. This result reshaped our thinking on the diversification within the He. virescens complex: it appears that species are not simply segregated by their host genus association and that there is a geographical component involved as well.
The host of the holotype material of He. hyperaspidis, an unidentified species of Hyperaspis, was collected in Trinidad. The infected Hy. vinciguerrae in this study was collected in the Canary Islands. To avoid future taxonomic issues, we refrained from 1) assigning our material of Hesperomyces sp. ex Hy. vinciguerrae to He. hyperaspidis; 2) reinstating said species; and 3) formally describing Hesperomyces sp. ex Hy. vinciguerrae as a new species. However, given all the available knowledge, it stands without a doubt that He. hyperaspidis should not be considered a synonym of He. virescens s.s., in contrast to the conclusion of Bernardi et al. (2014) based on morphology. Moving forward, Hyperaspis ladybirds should be collected in Trinidad and other locations around the world to screen for Hesperomyces thalli, extract DNA, and compare the sequences with the recent material from the Canary Islands. We also refrained from describing Hesperomyces sp. ex Ch. bipustulatus because we found only juvenile thalli.
Conclusion
Thalli of Hesperomyces from a Hy. vinciguerrae ladybird, recently collected in the Canary Islands, triggered an integrative taxonomy investigation into the identify of this fungus. Based on the phylogenies inferred from single-locus and multilocus ITS, LSU, and MCM7 data, we found that the thalli on Hy. vinciguerrae belong to another species than He. virescens s.s., as do the thalli on Ch. bipustulatus from Algeria. The hypothesis that species within the He. virescens complex are segregated by their host association appears to be complicated by geography. Thus, we have not assigned Hesperomyces sp. ex Hy. vinciguerrae to He. hyperaspidis, which is described from an unidentified Hyperaspis ladybird collected in Trinidad. However, there is good reason to consider He. hyperaspidis as a unique species separate from He. virescens s.s.
Data availability statement
All final alignments and unedited trees are available through GitHub: https://github.com/dannyhaelewaters/teamlaboul/tree/main/hesperomyces_hyperaspidis_paper. Newly generated sequences were submitted to the National Center for Biotechnology Information (NCBI) GenBank database (https://www.ncbi.nlm.nih.gov/genbank/), under the following accession numbers: OP933649–OP933659, OP947140–OP947141, and OP9471444–OP947156.
Author contributions
WVC and DH designed the study. WVC, PC, JR, and DHP collected the data. WVC performed data analysis. DH and JR acquired funding. WVC drafted the manuscript. WVC, DHP, and DH revised the manuscript. All authors contributed to the article and approved the submitted version.
Acknowledgments
This study was supported by the following funding agencies: Czech Science Foundation (GAČR; grant 20-10003S), En Arche Foundation (grant 2020/01/15 to JR), and Research Foundation—Flanders (Junior Postdoctoral Fellowship 1206620N to DH). We thank Aaron D. Smith (Purdue Entomological Research Collection) for curatorial support and the participants of the #TeamLaboul meetings for valuable comments on earlier drafts of the manuscript.
Conflict of interest
The authors declare that the research was conducted in the absence of any commercial or financial relationships that could be construed as a potential conflict of interest.
Publisher’s note
All claims expressed in this article are solely those of the authors and do not necessarily represent those of their affiliated organizations, or those of the publisher, the editors and the reviewers. Any product that may be evaluated in this article, or claim that may be made by its manufacturer, is not guaranteed or endorsed by the publisher.
Supplementary material
The Supplementary Material for this article can be found online at: https://www.frontiersin.org/articles/10.3389/ffunb.2022.1040102/full#supplementary-material
Supplementary File 1 | Phylogeny of the Hesperomyces virescens species complex, reconstructed from the SSU dataset. No ML bootstrap value was ≥70. Species within the Hesperomyces virescens complex are each indicated with their own color, as in Figure 1.
References
Abramoff M., Magalhães P., Ram S. J. (2004). Image processing with ImageJ. Biophotonics Int. 11, 36–42.
Bernardi M., Barragán A., Rossi W. (2014). New records of Laboulbeniales (Fungi: Ascomycota) from Ecuador and other countries. Webbia 69, 281–289. doi: 10.1080/00837792.2014.953369
Biedermann P., Vega F. (2020). Ecology and evolution of insect–fungus mutualisms. Annu. Rev. Entomol. 65, 431–455. doi: 10.1146/annurev-ento-011019-024910
Blackwell M., Haelewaters D., Pfister D. H. (2020). Laboulbeniomycetes: Evolution, natural history, and thaxter’s final word. Mycologia 112, 1048–1059. doi: 10.1080/00275514.2020.1718442
Bustamante D. E., Oliva M., Leiva S., Mendoza J. E., Bobadilla L., Angulo G., et al. (2019). Phylogeny and species delimitations in the entomopathogenic genus Beauveria (Hypocreales, Ascomycota), including the description of B. peruviensis sp. nov. MycoKeys 58, 47–68. doi: 10.3897/mycokeys.58.35764
Chernomor O., von Haeseler A., Minh B. Q. (2016). Terrace aware data structure for phylogenomic inference from supermatrices. Syst. Biol. 65, 997–1008. doi: 10.1093/sysbio/syw037
Crous P. W., Osieck E. R., Jurjevi Ž, Boers J., Van Iperen A. L., Starink-Willemse M., et al. (2021). Fungal planet description sheets: 1284–1382. Persoonia 47, 178–374. doi: 10.3767/persoonia.2021.47.06
Goldmann L., Weir A. (2012). Position specificity in Chitonomyces (Ascomycota, Laboulbeniomycetes) on Laccophilus (Coleoptera, Dytiscidae): a molecular approach resolves a century-old debate. Mycologia 104, 1143–1158. doi: 10.3852/11-358
Goldmann L., Weir A., Rossi W. (2013). Molecular analysis reveals two new dimorphic species of Hesperomyces (Ascomycota, Laboulbeniomycetes) parasitic on the ladybird Coleomegilla maculata (Coleoptera, Coccinellidae). Fungal Biol. 117, 807–813. doi: 10.1016/j.funbio.2013.10.004
Haelewaters D., Blackwell M., Pfister D. H. (2021a). Laboulbeniomycetes: Intimate fungal associates of arthropods. Annu. Rev. Entomol. 66, 257–276. doi: 10.1146/annurev-ento-013020-013553
Haelewaters D., De Kesel A. (2017). De schimmel Hesperomyces virescens, een natuurlijke vijand van lieveheersbeestjes. Entomol. Ber. 77, 106–118.
Haelewaters D., De Kesel A. (2020). Checklist of thallus-forming Laboulbeniomycetes from Belgium and the Netherlands, including Hesperomyces halyziae and Laboulbenia quarantenae spp. nov. MycoKeys 71, 23–86. doi: 10.3897/mycokeys.71.53421
Haelewaters D., De Kesel A., Gorczak M., Bao K., Gort G., Zhao S. Y., et al. (2019a). Laboulbeniales (Ascomycota) of the Boston Harbor Islands II (and other localities): Species parasitizing Carabidae, and the Laboulbenia flagellata species complex. Northeast. Nat. 25, 110–149. doi: 10.1656/045.025.s906
Haelewaters D., De Kesel A., Pfister D. H. (2018). Integrative taxonomy reveals hidden species within a common fungal parasite of ladybirds. Sci. Rep. 8, 15966. doi: 10.1038/s41598-018-34319-5
Haelewaters D., Dima B., Abdel-Hafiz A., Abdel-Wahab M. A., Abul-Ezz S. R., Acar I., et al. (2020). Fungal systematics and evolution: FUSE 6. Sydowia 72, 231–356. doi: 10.12905/0380.sydowia72-2020-0231
Haelewaters D., Gorczak M., Kaishian P., De Kesel A., Blackwell M. (2021b). “Laboulbeniomycetes, enigmatic fungi with a turbulent taxonomic history,” in Encyclopedia of mycology, vol. 1 . Eds. Zaragoza Ó., Casadevall A. (Oxford: Elsevier), 263–283.
Haelewaters D., Gorczak M., Pfliegler W. P., Tartally A., Tischer M., Wrzosek M., et al. (2015). Bringing Laboulbeniales to the 21st century: enhanced techniques for extraction and PCR amplification of DNA from minute ectoparasitic fungi. IMA Fungus 6, 363–372. doi: 10.5598/imafungus.2015.06.02.08
Haelewaters D., Lubbers M., De Kesel A. (2022a). The haustorium as a driving force for speciation in thallus-forming Laboulbeniomycetes. IMA Fungus 13, 1. doi: 10.1186/s43008-021-00087-7
Haelewaters D., Pfister D. H. (2019). Morphological species of Gloeandromyces (Ascomycota, Laboulbeniales) evaluated using single-locus species delimitation methods. Fungal Syst. Evol. 3, 19–34. doi: 10.3114/fuse.2019.03.03
Haelewaters D., Pfliegler W. P., Gorczak M., Pfister D. H. (2019b). Birth of an order: Comprehensive molecular phylogenetic study excludes Herpomyces (Fungi, Laboulbeniomycetes) from Laboulbeniales. Mol. Phylogenet. Evol. 133, 286–301. doi: 10.1016/j.ympev.2019.01.007
Haelewaters D., Rossi W. (2015). Three new species of Laboulbenia from Roland Thaxter’s backlog of slides and a brief review of Laboulbeniales associated with Chrysomelidae. Mycologia 107, 142–148. doi: 10.3852/14-022
Haelewaters D., Van Caenegem W., De Kesel A. (2022b). Hesperomyces harmoniae, a new name for a common ectoparasitic fungus on the invasive alien ladybird Harmonia axyridis. Sydowia 75, 53–74. doi: 10.12905/0380.sydowia75-2023-0053
Hall T. A. (1999). BioEdit: a user-friendly biological sequence alignment editor and analysis program for windows 95/98/NT. Nucleic Acids Symp. Ser. 41, 95–98.
Hoang D. T., Chernomor O., von Haeseler A., Minh B. Q., Vinh L. S. (2018). UFBoot2: Improving the ultrafast bootstrap approximation. Mol. Biol. Evol. 35, 518–522. doi: 10.1093/molbev/msx281
Hopple J. S. (1994). Phylogenetic investigations in the genus Coprinus based on morphological and molecular characters. Ph.D. dissertation (Durham, NC: Duke University).
Kalyaanamoorthy S., Minh B., Wong T., von Haeseler A., Jermiin L. (2017). ModelFinder: Fast model selection for accurate phylogenetic estimates. Nat. Methods 14, 587–589. doi: 10.1038/nmeth.4285
Katoh K., Rozewicki J., Yamada K. D. (2019). MAFFT online service: multiple sequence alignment, interactive sequence choice and visualization. Brief. Bioinform. 20, 1160–1166. doi: 10.1093/bib/bbx108
Kuraku S., Zmasek C. M., Nishimura O., Katoh K. (2013). aLeaves facilitates on-demand exploration of metazoan gene family trees on MAFFT sequence alignment server with enhanced interactivity. Nucleic Acids Res. 41, W22–W28. doi: 10.1093/nar/gkt389
Liu J., Haelewaters D., Pfliegler W. P., Page R. A., Dick C. W., Aime M. C. (2020). A new species of Gloeandromyces from Ecuador and Panama revealed by morphology and phylogenetic reconstruction, with a discussion of secondary barcodes in Laboulbeniomycetes taxonomy. Mycologia 112, 1192–1202. doi: 10.1080/00275514.2020.1781496
Nguyen L.-T., Schmidt H. A., von Haeseler A., Minh B. Q. (2015). IQ-TREE: A fast and effective stochastic algorithm for estimating maximum-likelihood phylogenies. Mol. Biol. Evol. 32, 268–274. doi: 10.1093/molbev/msu300
Romanowski J., Ceryngier P., Větrovec J., Szawaryn K. (2019). The Coccinellidae (Coleoptera) from Fuerteventura, Canary Islands. Zootaxa 4646, 101–123. doi: 10.11646/zootaxa.4646.1.6
Schmitt I., Crespo A., Divakar P. K., Fankhauser J. D., Herman-Sackett E., Kalb K., et al. (2009). New primers for promising single-copy genes in fungal phylogenetics and systematics. Persoonia 23, 35–40. doi: 10.3767/003158509X470602
Schoch C. L., Seifert K. A., Huhndorf S., Robert V., Spouge J. L., Levesque C. A., et al. (2012). Nuclear ribosomal internal transcribed spacer (ITS) region as a universal DNA barcode marker for Fungi. Proc. Natl. Acad. Sci. U.S.A. 109, 6241–6246. doi: 10.1073/pnas.1117018109
Sundberg H., Kruys Å., Bergsten J., Ekman S. (2018). Position specificity in the genus Coreomyces (Laboulbeniomycetes, Ascomycota). Fungal Syst. Evol. 1, 217–228. doi: 10.3114/fuse.2018.01.09
Thaxter R. (1891). Supplementary note on North American Laboulbeniaceae. Proc. Am. Acad. Arts Sci. 25, 261–270. doi: 10.2307/20020441
Thaxter R. (1931). Contribution towards a monograph of the Laboulbeniaceae. Part V. Mem. Am. Acad. Arts Sci. 16, 7–435. doi: 10.2307/25058136
Vaidya G., Lohman D. J., Meier R. (2011). SequenceMatrix: concatenation software for the fast assembly of multi-gene datasets with character set and codon information. Cladistics 27, 171–180. doi: 10.1111/j.1096-0031.2010.00329.x
Vilgalys R., Hester M. (1990). Rapid genetic identification and mapping of enzymatically amplified ribosomal DNA from several Cryptococcus species. J. Bacteriol. 172, 4238–4246. doi: 10.1128/jb.172.8.4238-4246.1990
Keywords: arthropod-associated fungi, Coccinellidae, DNA barcoding, integrative taxonomy, MCM7, phylogeny, nuclear ribosomal DNA
Citation: Van Caenegem W, Ceryngier P, Romanowski J, Pfister DH and Haelewaters D (2023) Hesperomyces (Fungi, Ascomycota) associated with Hyperaspis ladybirds (Coleoptera, Coccinellidae): Rethinking host specificity. Front. Fungal Biol. 3:1040102. doi: 10.3389/ffunb.2022.1040102
Received: 08 September 2022; Accepted: 22 November 2022;
Published: 09 January 2023.
Edited by:
Donald O. Natvig, University of New Mexico, United StatesReviewed by:
Vinson P. Doyle, Louisiana State University, United StatesMilan C. Samarakoon, Chiang Mai University, Thailand
Copyright © 2023 Van Caenegem, Ceryngier, Romanowski, Pfister and Haelewaters. This is an open-access article distributed under the terms of the Creative Commons Attribution License (CC BY). The use, distribution or reproduction in other forums is permitted, provided the original author(s) and the copyright owner(s) are credited and that the original publication in this journal is cited, in accordance with accepted academic practice. No use, distribution or reproduction is permitted which does not comply with these terms.
*Correspondence: Warre Van Caenegem, warre.vancaenegem@ugent.be; Danny Haelewaters, danny.haelewaters@gmail.com