- 1Collaborative Innovation Center of Sustainable Forestry in Southern China, College of Forestry, Nanjing Forestry University, Nanjing, China
- 2Department of Applied Foreign Languages, College of Continuing Education, Nanjing University of Aeronautics and Astronautics, Nanjing, China
Fungi perform crucial roles in nutrient cycles, but there is limited information on how soil fungal communities vary with stand age and tree species. Eucalyptus has been extensively planted in China, which has caused severe soil erosion and water deficiency due to short rotation management. In this study, the fungal community structure and potential function in Eucalyptus plantations with different ages (1–5+ years) and species (Eucalyptus urophylla × Eucalyptus grandis, Eucalyptus camaldulens, and Eucalyptus pellita) under a tropical monsoon climate in China were characterized by Illumina Miseq coupled with FUNGuild analysis. The results showed that the fungal alpha diversity decreased with an increase in the age of the plantation. Plantations of different ages and species formed distinct fungal communities and potential functional structures, respectively (p < 0.05), in which the age of the plantation contributed more to the variations. At high taxonomic levels, the soil fungal community changed from the dominance of orders belonging to Ascomycota (Pleosporales, Chaetothyriales, and Eurotiales) to orders belonging to Basidiomycota (Agaricales, Sebacinales, Cantharellales, and Russulales) with increasing plantation age. The community potential function shifted from the dominance of plant pathogens to a higher abundance of saprotrophs and symbiotrophs. The organic carbon of the soil was the key environmental driver to both the fungal community and potential functional structure. The results provide useful information on the importance of fungi for the management of Eucalyptus plantations.
Introduction
Eucalyptus, belonging to the genera of Myrtaceae, is originally from Australia and nearby islands (Grattapaglia et al., 2012). It has been extensively planted on over ~20 million hectares in subtropical and tropical regions because of its fast growth, strong adaptability, and short rotation cycle (Cook et al., 2016). In subtropical and tropical regions of China, farmers have frequently changed their fields to Eucalyptus plantations over the past two decades (Yang et al., 2017). Long-term Eucalyptus plantations with short rotations and human disturbance have led to a decrease in the quality of soil due to the demand for nutrients, resulting in soil ecological issues, e.g., soil erosion and water deficiency (Laclau et al., 2010; Yang et al., 2017). Furthermore, allelochemicals, such as phenolic acids and terpenoids, are normally released from Eucalyptus tissues and accumulate under natural conditions to produce allelopathic effects that can reduce the native biological diversity in Eucalyptus plantations (Qin et al., 2018). For instance, the essential oil from Eucalyptus can inhibit the proliferation of pathogenic fungi and pupation and feeding of pest insects (Nakahara et al., 2003; Liu et al., 2008; Kaur et al., 2012).
Fungi as decomposers, mutualists, or pathogens (i.e., parasites) in terrestrial ecosystems perform crucial roles in the carbon and nitrogen cycles, which can affect soil quality and plant growth (Peay et al., 2008; Mcguire et al., 2010; Burke et al., 2011). Generally, fungi have stronger effects on the rate of soil organic matter turnover than bacteria because of their higher decomposition ability; as a matter of fact, it is possible to find up to 1010 copies of fungal 18S rRNA genes per gram of soil (Ekblad et al., 2013; Lazzaro et al., 2015; Crowther et al., 2019). The relative abundance and occurrence of rare species can shift because of changes in vegetation, soil properties, and forest management (Shade et al., 2014; Uroz et al., 2016). However, because of the complexity of soil and the limitations of identification methods, variations in soil fungal communities and their driving forces remain less understood (Schmidt et al., 2014; Tedersoo et al., 2014; Liu et al., 2015). In recent years, high-throughput DNA sequencing platforms have been proven to be an effective tool for studying environmental microbial communities and how they relate to environmental factors in forests (Schmidt et al., 2014; Uroz et al., 2016).
Vegetation factors, such as stand age and site species composition, can affect fungal communities directly through host–fungi specificity or indirectly through resource input such as root exudates and litterfall (Alguacil et al., 2015; Alfaro et al., 2017). Previous studies have shown that tree species identity is one of the key factors governing soil microbial communities, especially mycorrhizal fungi (Debellis et al., 2006; Horn et al., 2017). For instance, the structure and diversity of fungal communities in temperate and boreal forest ecosystems are strongly dependent on one or few dominant tree species (Sun et al., 2016; van der Linde et al., 2018). Meanwhile, forest stand age can affect fungal community structure and function through changes in the quantity and quality of litter (Schilling et al., 2016). For example, a recent study showed that increasing stand age favored the dominance of soil saprotrophic fungi (e.g., Mortierellales, Trichoderma, and Scheffersomyces); however, the results of other research studies showed the opposite trend (Li et al., 2017; Hagenbo et al., 2018). These studies provide useful information for understanding the response of fungal communities to vegetation changes. However, such studies mentioned above are rare in tropical monsoon forests.
Eucalyptus camaldulens, Eucalyptus pellita, and Eucalyptus urophylla × Eucalyptus grandis (female parent E. urophylla and male parent Eucalyptus grandis), which were selected in this study, and their hybrids are among the nine most planted Eucalyptus species, together accounting for more than 90% of Eucalyptus plantations (Stanturf et al., 2013). Because of the fact that its adaptability is strong, E. camaldulens is the most widely distributed Eucalyptus species in the entire Australian continent (Mcdonald et al., 2009). E. pellita is naturally distributed in the tropical regions of northeastern Australia, while E. grandis usually grows on the edge of rainforests with deep and easy-draining soils on the eastern middle coast (Chippendale, 1981). Unlike most Eucalyptus, E. urophylla is one of the few Eucalyptus species distributed in the Lesser Sunda Islands of Indonesia outside of Australia, where ecological conditions are similar to those of the east coast of Australia (Pryor et al., 1995). Previous studies on Eucalyptus plantations have mainly focused on the effects on soil parameters and mycorrhizal fungal communities, e.g., ectomycorrhiza (ECM) and arbuscular mycorrhiza (Malajczuk et al., 2010; Grbić et al., 2015; Sulzbacher et al., 2018). Recent studies showed that the age and species of Eucalyptus plantations significantly affected soil bacterial community, microbial biomass, and enzymatic activities (Qu et al., 2020; Xu et al., 2020). Studies on soil fungal community as a whole in Eucalyptus plantations, however, are less common (Castaño et al., 2019). To better understand the impact of Eucalyptus planting on soil ecology, it is necessary to assess the dynamic response of soil fungi to Eucalyptus plantations. The hypothesis of the authors is that Eucalyptus plantations with different ages and species form distinct soil fungal communities. Therefore, we profiled the soil fungal community composition and structure in Eucalyptus plantations with different ages and species using high-throughput Illumina Miseq together with FUNGuild. The aims of this study were to (1) investigate how the age of Eucalyptus plantations and species impact soil fungal community and functional structures and (2) determine the environmental factors contributing to the variations in fungal communities and functional structures.
Materials and Methods
Study Sites
The study sites were located in the nursery base of the China Eucalypt Research Center (CERC, a member of the Chinese Academy of Forestry), northern Leizhou Peninsula, Zhanjiang, southern China (21°27′N, 110°11′E). This region belongs to the northern edge of the Leiqiong district in the humid tropical zone of southern China, and it is situated in the transitional zone between the subtropical humid and marginal tropics zone. The annual average precipitation in the area is ~1,500 mm with an 85.5% rainfall in the rainy season from May to September (Li et al., 2013). The annual average temperature is ~23.1°C, ranging from 17.2°C in January to 38.1°C in July. The soil is orthic ferralsols or brick red based on international or Chinese soil classification, respectively (Liu et al., 2009).
Sample Collection
In total, six plantations, ~2.2 hectares per plantation, were selected, representing three Eucalyptus species [Eucalyptus urophylla × Eucalyptus grandis (EUG), Eucalyptus camaldulensis (EC), and Eucalyptus pellita (EP)] with different ages (1, 5, and 11 years old). For EUG and EC, we sampled 1- and 5-year-old plantations; while for EP, we sampled 1- and 11-year-old plantations. The term 5+-year-old plantation hereafter refers to the 5-year-old (EUG5y and EC5y) and 11-year-old (EP11y) plantations. The 1-year-old plantations were established from a clear-cut Eucalyptus robusta Smith site in early March 2016 and were under similar management with weed control in August 2016 and March 2017 and fertilization with ammonium sulfate in March 2017. The 5+-year-old plantations received weed control once a year in August, and the common underground herbs were Mimosa pudica, Arachis duranensis, and Desmodium gangeticum. The distance between the trees in each plantation was 2 m × 3 m. The depth of soil was ~1 m, and the pH of the soil ranged from 4.3 to 5.4 (Liu et al., 2009). The distance between the 1- and 5+-year-old plantations was ~100 m. In each plantation, three subplots (15 m × 15 m) were selected randomly according to the S-shaped route in which three trees were chosen randomly per subplot for sampling. After the removal of litter, three soil cores (100 cm3) around each tree with a 1-m distance from the trunk were collected at a depth of 0–10 cm from three directions with 120° and then mixed as one sample, resulting in 9 samples per plantation and 54 samples in total. The samples were put into a carry-on icebox immediately and delivered to the laboratory. All the soil samples were taken on the same day in the rainy season on May 18, 2017. In the laboratory, each soil sample was subdivided into two subsamples after being sieved with a mesh (2 mm) to remove stones, litter, roots, and large particles. One subsample was used to measure the physicochemical properties of the soil, and one was stored at −20°C for DNA isolation until further processing.
Determination of Soil Physicochemical Properties
Soil water content (SWC) was measured by gravimetry after the soil samples were dried at 105°C for 24 h. The pH of the soil was measured with a digital pH meter in a 1:2.5 soil–water suspension (Kooch et al., 2017). Soil organic carbon (SOC) was measured by the wet oxidation method with potassium dichromate and titration with ferri sulphas (Klute, 1988). The total nitrogen (TN) content of the soil was determined by the semi-micro Kjeldahl method. In addition, the data for the soil microbial biomass, soil fungal biomass (FB), and soil bacterial biomass (BB) in the same study sites were retrieved from Xu et al. (2020), which were used in the analysis of the correlation between community structure and environmental variables. The ratios of soil organic carbon to total nitrogen (C:N ratio) and fungal to bacterial biomass (FB:BB ratio) were calculated.
DNA Extraction, Amplification of ITS rRNA Region, and Illumina Mise Sequencing
Soil genomic DNA was extracted from 0.3 g fresh soil using the PowerSoil DNA isolation kit (Omega Bio-Tek, Norcross, GA, United States) following the instructions of the manufacturer. The extracted DNA was measured using a Nanodrop-1000 spectrometer (NanoDrop Technologies, Wilmington, DE, United States) and checked by gel electrophoresis. The DNA was subjected to PCR amplification of fungal internal transcribed spacer 1 (ITS1) region using the primer set ITS1F (5′-CTTGGTCATTTAGAGGAAGTAA-3′) and ITS2R (5′-GCTGCGTTCTTCATCGATGC-3′) in a thermocycler 291 PCR system (GeneAmp 9700; Applied Biosystem (ABI), Massachusetts, United States) (Li et al., 2018). PCR reactions were performed in triplicate in a 20-μl mixture containing 4 μl of 5 × FastPfu Buffer (TransGen Biotech, Beijing, China), 2 μl of 2.5 mM deoxyribonucleotide triphosphate (dNTP), 0.8 μl of each primer (5 μM), 0.4 μl of FastPfu Polymerase (ransGen Biotech, Beijing, China), 0.2 μl of bovine serum albumin (BSA), and 10 ng of template DNA. The PCR reactions were conducted using the following program: 3 min of denaturation at 95°C, 36 cycles of 30 s at 95°C, 30 s for annealing at 55°C, and 45 s for elongation at 72°C, and final extension at 72°C for 10 min. The PCR products were checked from a 2% agarose gel and then further purified using the AxyPrep DNA Gel Extraction Kit (Axygen Biosciences, Union City, CA, United States) and quantified using QuantiFluor™-ST (Promega, Madison, WI, United States) according to the protocol of the manufacturer. The purified amplicons were subjected to sequencing with paired-end (PE = 300) on the Illumina MiSeq platform (Illumina, San Diego, CA, United States) according to the standard protocols at Majorbio Bio-Pharm Technology Co. Ltd. (Shanghai, China). The raw reads were deposited into the National Center for Biotechnology Information (NCBI) Sequence Read Archive (SRA) database (accession number: PRJNA580298).
Sequence Data Processing and Statistical Analysis
The paired-end sequences were processed using the Mothur software (version 1.39.1) following the standard operating procedure (SOP), with the following modifications (Schloss et al., 2009): sequences containing ambiguous (N) bases, homopolymers longer than 8 bp, average quality score lower than 25, putative chimeras (using UCHIME in Mothur), and fewer than 200 bp were omitted (Edgar et al., 2011). Amplification errors were checked using the PCR.seq command. The sequences were pairwise aligned using the align.seq command and pre-clustered with two base-pair differences to remove sequences that were likely due to sequencing errors. The high-quality and unique sequences were classified against the UNITE database (version 8) with a bootstrap cutoff of 80 (Abarenkov et al., 2010). Non-fungal sequences were removed using the remove.lineage command. All the fungal sequences were clustered into operational taxonomic units (OTUs) with 97% similarity using the average neighbor-joining algorithm. All global singletons (OTUs containing only one sequence across all samples) were omitted because of their uncertain origin (Tedersoo et al., 2010). Fungal alpha diversity indexes, such as species richness (Chao 1), diversity (Inverse Simpson's index 1/D), and species evenness (Simpsoneven) were calculated by subsample using the samples with the minimum number of sequences (23,500).
The operational taxonomic units were assigned into functional groups using the FUNGuild database in which the fungi were divided into three basic trophic modes: pathotrophs, symbiotrophs, and saprotrophs (Tedersoo et al., 2014; Nguyen et al., 2016). In addition, the trophic mode can overlap a single fungal taxon. Within these trophic modes, fungi were further divided into a total of 21 functional categories broadly referred to as guilds. For example, the pathotrophs included plant pathogens, animal pathogens, and fungal parasite guilds. The symbiotrophs included arbuscular mycorrhizal fungi, ECM, ericoid mycorrhizal fungi (ErM), and endophyte guilds. The saprotrophs included plant saprotroph, dung saprotroph, litter saprotroph, undefined saprotroph, and wood saprotroph guilds (Nguyen et al., 2016).
All the statistical analyses, except linear discriminant analysis (LDA) coupled with effect size (LEfSe), were performed in the R environment (v3.6.2 or v3.6.3; http://www.r-project.org/). A two-way ANOVA was performed to determine significant differences (p < 0.05) in community composition and diversity with plantation age, species, and their interaction as explanatory variables (car, package). One-way ANOVA and Tukey HSD post-hoc pairwise comparison tests at p < 0.05 were performed (multcomp package) for community diversity. If the data did not conform to a normal distribution, two-tailed Mann–Whitney U-tests were performed (spdep and reshape2 packages). Spearman's correlation coefficient and significance were calculated using the package “psych” in R, and the data were scaled by row using the “pheatmap” package. Principle coordination analysis (PCoA) was performed to visualize and detect the difference in fungal community structure or in functional community structure using the “vegan” package with the Bray–Curtis metric (Anderson, 2001). Permutational multivariate analysis of variance (PERMANOVA, adonis in the “vegan” package) was implemented to discern the amount of variation attributed to plantation age, species, and their interaction based on Bray–Curtis distance and 999 permutations. PERMANOVA was also performed to determine the dissimilarity between the community and functional structures of each site. Based on the long length of the first axis of the detrended correspondence analyses (DCA) (i.e., 4.33 for fungi and 4.21 for function), the unimodal ordination method and canonical correspondence analyses (CCAs) were chosen to disentangle relationships between the fungal community or functional structure and environmental variables. The species specialization index (SSI), which is the coefficient of variation in the distribution of fungal performances on host tree species, was computed to quantify the degree of specificity of each fungal species using the ESM package (Poisot et al., 2012). Linear discriminant analysis (LDA) coupled with effect size (LEfSe; http://huttenhower.sph.harvard.edu/galaxy) was performed to identify the fungal or FUNGuild taxa differentially represented among the plantations (Segata et al., 2011). LEfSe was performed with an average relative abundance of taxa of >0.001 in order to hold as many taxa as possible for meaningful comparisons and to remove all rare taxa in the analysis. The criteria for a significance level of fungal taxonomic and functional level were set up with a threshold of an LDA score of >3 and p < 0.05.
Results
General Analyses of the MiSeq Sequence Data
In total, 1,791,002 reads were obtained after quality control, which were clustered into 3,129 OTUs based on a 97% similarity. Most of the reads (98.69%) could be classified into the phyla of fungi (Supplementary Figure 1). Ascomycota was the most abundant phylum with 49.14% of reads, followed by Basidiomycota (49.09%), Mortierellomycota (0.24%), and Chytridiomycota (0.21%). The order Boletales accounted for 16.13% of the reads, followed by Eurotiales (13.55%), Thelephorales (11.28%), Sebacinales (9.36%), Agaricales (8.19%), and Hypocreales (7.68%). The most abundant genus was Scleroderma, a genus of Boletales, which accounted for 11.23% of the reads. Other genera with more than 5% of the reads were Talaromyces (7.88%), Sebacina (7.22%), and Thelephora (5.81%). In addition, 39 OTUs were identified as core OTUs, which existed in more than four soils from each Eucalyptus plantation and had an average relative abundance of >0.1% in at least one plantation (Supplementary Figure 2). Of these OTUs, Ascomycota (37 core OTUs, 95.37% of core reads) was more widely distributed than Basidiomycota (2 core OTUs, 4.63% of core reads).
The Alpha- and Beta-Diversity of Fungal Community
All fungal alpha diversity indices (richness, diversity, and evenness) of the 1-year-old plantations differed from that of the 5+-year-old plantations (Figure 1). The 5+-year-old plantations had significantly higher richness than the 1-year-old plantations (p = 0.018). In contrast, the 1-year-old plantations had a higher diversity and evenness than the 5+-year-old plantations (p = 0.024 and p = 0.002, respectively). In addition, the fungal richness in the EP 1-year-old plantation differed from that in the EC 1-year-old plantation, but no significant difference was observed for fungal richness, diversity, and evenness among the Eucalyptus species. However, the interaction between plantation age and species showed a significant effect on fungal richness (p = 0.021). The fungal diversity showed a positive correlation with evenness (Supplementary Table 1; R = 0.9184, p < 0.001).
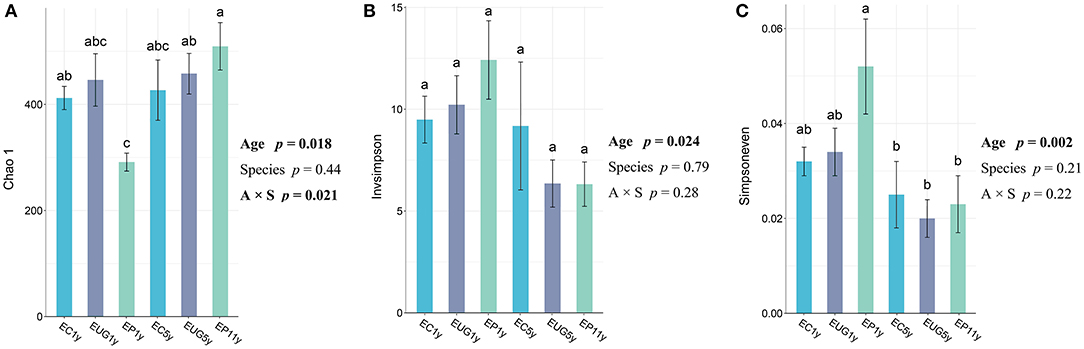
Figure 1. Fungal alpha diversity indexes of (A) richness, (B) diversity, and (C) evenness in Eucalyptus plantations with different ages and species. Two-way ANOVA model summary for responses of alpha diversity indexes and explanatory variables of Eucalyptus plantation age, species, and their interaction. Explanatory variables deemed statistically significant are bolded. One-way ANOVA and Tukey [honestly significant difference (HSD) post-hoc pairwise comparison tests (p < 0.05) were performed after checking for assumptions of normality and homoscedasticity. Accessions with the same letter were not significantly different. EC, Eucalyptus camaldulensis; EP, Eucalyptus pellita; EUG, Eucalyptus urophylla × Eucalyptus grandis; 1y, 1 year; 5y, 5 years; 11y, 11 years.
The principle coordination analysis showed that the six plantations formed five distinct fungal communities (Figure 2A; PERMANOVA, pseudo-F > 4.89, R2 >0.23, p < 0.01). The EP1y- and EC1y-plantations did not separate by fungal community (PERMANOVA, pseudo-F = 1.35, R2 = 0.08, p >0.05). Correspondingly, the 1- and 5+-year-old plantations harbored distinct fungal compositions and confirmed a clear separation along PCo 1 (explaining 16.2% of the overall variation), whereas PCo 2 explained 12.1% of the overall variation and separated mainly the Eucalyptus species. Moreover, plantation age accounted for a larger proportion of variation (PERMANOVA, pseudo-F = 7.09, R2 = 0.22, p < 0.001) in fungal community structure compared with the species (PERMANOVA, pseudo-F = 4.74, R2 = 0.16, p < 0.001).
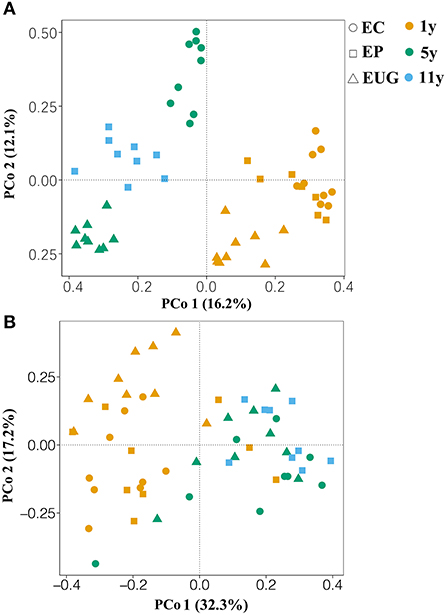
Figure 2. Principle coordination analysis (PCoA) based on Bray–Curtis similarity matrices showing the (A) fungal and (B) functional community structures in different soils. EC, E. camaldulensis; EP, E. pellita; EUG, E. urophylla × E. grandis; 1y, 1 year; 5y, 5 years; 11y, 11 years.
Fungal Taxa Differences in Eucalyptus Plantations
At higher taxonomic levels, Ascomycota (the phylum and its orders Pleosporales, Chaetothyriales, and Eurotiales) and Chytridiomycota had a higher abundance in the 1-year-old plantations, whereas there was a distinct increase in the relative abundance of Basidiomycota (the phylum and its orders Agaricales, Sebacinales, Cantharellales, and Russulales), Mortierellomycota (the phylum and its order Mortierellales), and ascomycetes Helotiales in the 5+-year-old plantations (Figure 3A, p < 0.01). At lower taxonomic levels, the genera Curvularia, Coniosporium, Aspergillus, Penicillium, Talaromyces, and Clonostachys were more abundant in the 1-year-old plantations, whereas Arthropsis, Purpureocillium, Sebacina, and Mortierella were more abundant in the 5+-year-old plantations (p < 0.05). The other taxa (unique to a specific site) with significant differences in abundance between plantations are listed in Figure 3A.
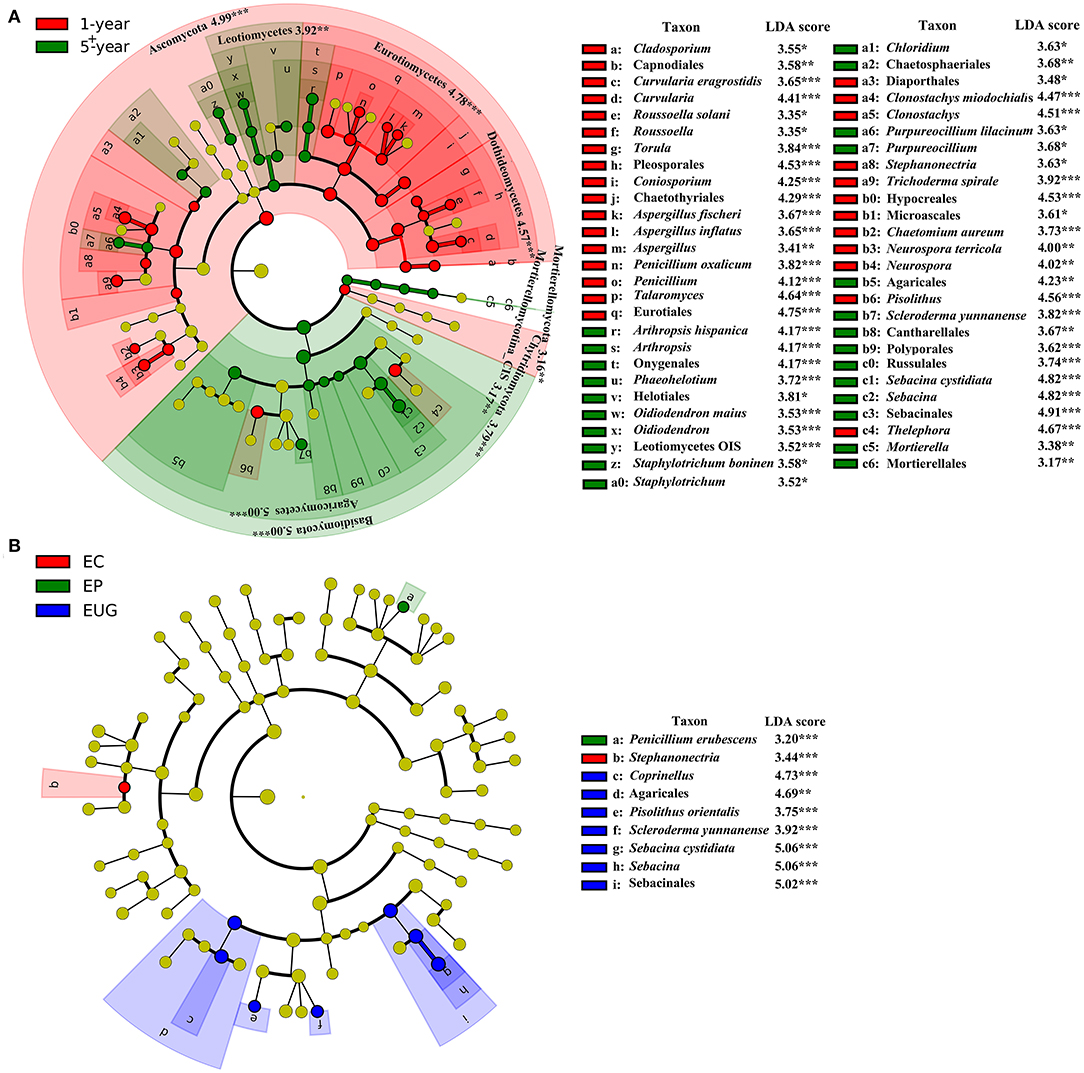
Figure 3. Fungal taxa (without family) significantly differentiated in Eucalyptus plantations with (A) different ages and (B) different species, identified by linear discriminant analysis coupled with effect size (LEfSe) using parameters of a threshold of linear discriminant analysis (LDA) score > 3 and p < 0.05. From the inner circle to the outer circle of tree diagrams are fungal taxa of phylum, class, order, genus, and species. The red and green nodes and shades shown in (A) represent fungal taxa that play an important role in the 1- and 5+-year-old plantations, respectively; the red, green, and blue nodes and shades shown in (B) represent fungal taxa that play an important role in EC, EP, and EUG plantations, respectively; the yellow nodes represent fungal taxa that do not play an important role in each treatment. EC, E. camaldulensis; EP, E. pellita; EUG, E. urophylla × E. grandis; CIS, Class Incertae sedis; OIS, Order Incertae sedis. *signifiant level of 5%; **significant level of 1%, and ***significant level of 0.1%.
Only a small number of taxa differed significantly among the Eucalyptus species. The order Agaricales and its genus Coprinellus, Sebacinales, and its genus, Sebacina and Pisolithusorientalis, were more abundant in the EUG plantations, whereas the genus Stephanonectria was more abundant in the EC plantations (Figure 3B; p < 0.01). Sebacinales was the most specialized order with the highest mean SSI (Supplementary Figure 3A). In addition, Agaricales and its genus Coprinellus had a higher mean SSI (Supplementary Figure 3).
Fungal Community Structure of Functional Guilds
For the functional groups, a total of 1,060,621 reads (59.22% of the total reads) were assigned to the three functional guilds (Supplementary Figure 4). The obligate saprotrophs were the most dominant functional guilds, covering 39.34% of the assigned reads, followed by obligate symbiotrophs (27.1% of the assigned reads), obligate pathotrophs (14.74%), pathotrophs–saprotrophs–symbiotrophs (12.13%), pathotrophs–saprotrophs (4.5%), pathotrophs–symbiotrophs (1.32%), and saprotrophs–symbiotrophs (0.87%) (Supplementary Figure 4). Similar to the fungal community structure, the PCoA showed that the six plantations formed five distinct functional structures (Figure 2B; PERMANOVA, pseudo-F = 2.06, R2 >0.12, p < 0.05). The EP1y- and EC1y-plantations did not differ in functional structure (PERMANOVA, pseudo-F = 1.54, R2 = 0.09, p >0.05).
For different plantation ages, the 1-year-old plantations had a higher abundance of plant pathogens, whereas the 5+-year-old plantations had a higher abundance of wood saprotrophs, endophytes–undefined saprotrophs, animal pathogens, fungal parasites, ericoid mycorrhizal fungi, and animal pathogens–dung, saprotrophs–endophytes–epiphytes–plant, and saprotrophs–wood saprotrophs (Figure 4A; p < 0.05). On the contrary, undefined saprotrophs and epiphytes–plant pathogens were more abundant in the EUG plantations (Figure 4B; p < 0.001). Out of the four dominant functional groups, plant pathogens were the most specialized group, followed by undefined saprotrophs, wood saprotrophs, and ECM (Supplementary Figure 5).
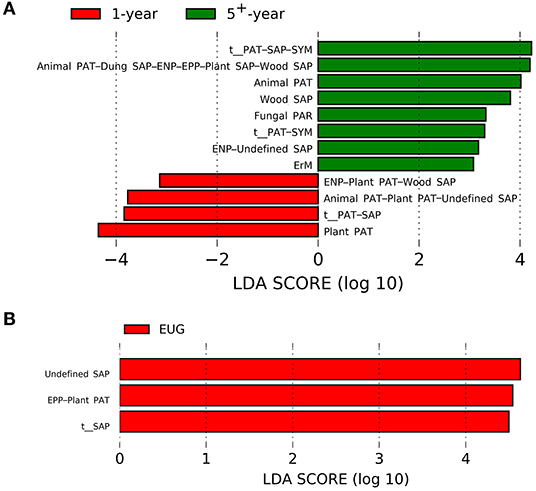
Figure 4. Fungal functional guilds significantly differentiated in Eucalyptus plantations with different (A) ages and (B) species identified by linear discriminant analysis coupled with effect size (LEfSe) using parameters of a threshold of LDA score > 3 and p < 0.05. EUG, E. urophylla × E. grandis; PAT, pathotroph; PAR, parasite; SAP, saprotroph; SYM, symbiotroph; ENP, endophyte; EPP, epiphyte; ErM, ericoid mycorrhizal.
Soil Physicochemical Properties in Eucalyptus Plantations
The physicochemical properties measured in the Eucalyptus plantations are summarized in Table 1. Among the sites, the mean SOC ranged from 2.96 to 4.06 g/kg, the TN content ranged from 0.089 to 0.266 g/kg, and the SWC ranged from 18.84 to 26.41%. The pH value in the EC5y-plantations was higher than those in the other sites (Table 1; p < 0.05). The C:N ratio, pH value, and the SOC content in the 5+-year-old plantations were higher than those in the 1-year-old plantations (Table 2; p < 0.05). On average, the pH value and the SWC were strongly affected by species (Table 2; p < 0.001). The interaction between plantation age and species had significant effects on the pH value, SWC, and TN (Table 2; p < 0.05).
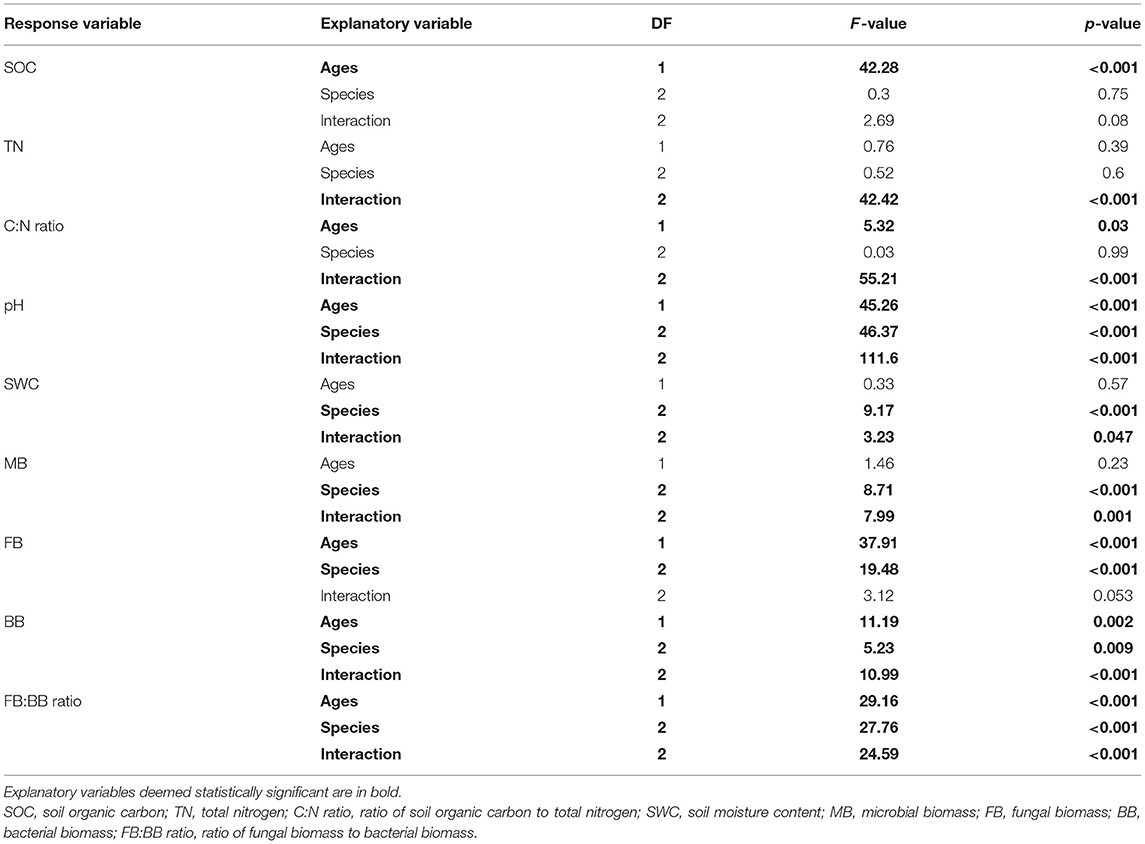
Table 2. Summary of two-way ANOVA model for responses of physicochemical and microbial characteristics to explanatory variables of Eucalyptus plantation age, species, and their interaction.
The results of the Spearman correlation showed that the total nitrogen content of the soil was significantly negatively correlated with pH value (Supplementary Figure 6; pH vs. TN: R = −0.5758, p < 0.001), whereas the C:N ratio and FB:BB ratio showed an opposite correlation with pH (C:N vs. pH: R = 0.6527, p < 0.001; FB:BB vs. pH: R = 0.4504, p = 0.039). Linear regression analysis showed that the C:N ratio, pH value, SOC, and soil TN content were significantly correlated with Eucalyptus plantation age (Supplementary Figure 7; Adj. R2 >0.25, p < 0.01). Furthermore, fungal richness and some dominant fungal taxa and functional groups also showed significant correlations with various physicochemical and microbial factors. For instance, Eurotiales, Curvularia, Coniosporium, Talaromyces, and Pisolithus exhibited a significantly negative correlation with SOC (Supplementary Figure 8; R < −0.51, p < 0.05). In addition, plant pathogens and undefined saprotrophs exhibited a negative correlation with SOC (Supplementary Figure 9; R < -0.51, p < 0.05).
Environmental Factors Contributing to Fungal Community and Potential Functional Structures
The results of the canonical correspondence analyses showed that the physicochemical and microbial factors together explained 51.49% (CCA1: 26.83% and CCA2:24.66%) of the total variations in fungal community structure (Figure 5A). According to the correlation coefficients of the CCA, the C:N ratio, SOC, and TN content were the top three explanatory factors contributing to the variations in fungal community structures (Figure 5A; R2 >0.5, p < 0.001). In addition, the C:N ratio and MB were significantly correlated with fungal community structures in the 1-year-old plantations. The C:N ratio, BB, and TN content were the top three factors explaining the total variations in functional structures (83.22%) (Figure 5B; R2 >0.3, p < 0.001). Similar to the correlation coefficient of the fungal community structure, MB was significantly correlated with the functional community structures in the 1-year-old plantations.
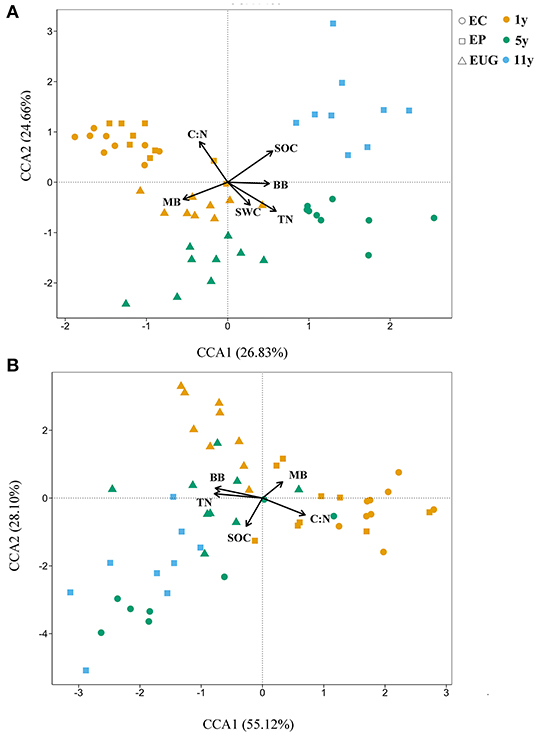
Figure 5. Canonical correspondence analyses (CCAs) showing (A) fungal and (B) functional community structures in Eucalyptus plantations with different ages and species with environmental variables as explanatory factors. EC, E. camaldulensis; EP, E. pellita; EUG, E. urophylla × E. grandis; 1y, 1 year; 5y, 5 years; 11y, 11 years; SOC, soil organic carbon; TN, total nitrogen; C:N ratio, ratio of soil organic carbon to total nitrogen; SWC, soil moisture content; MB, microbial biomass; BB, bacterial biomass.
Discussion
In this study, we investigated the responses of fungal community and potential function to Eucalyptus plantation age and species. Plantation age seemed to have the largest contribution to the variations in fungal community structure compared with species, particularly for certain taxa of Basidiomycota. Plantation age, species, and their interaction also impacted the major soil physicochemical and microbial characteristics in the Eucalyptus plantations which, in turn, affected the fungal community. Previous studies have shown that the indirect influence between fungal community structure and soil pH is mediated by fungal and bacterial communities (Rousk et al., 2010), which is in line with the findings that the FB:BB ratio was positively correlated with fungal biomass (FB) and showed a negative correlation with pH (Supplementary Figure 6). Meanwhile, the abundance of several taxa of Ascomycota varied along with plantation age, and the abundance of Eurotiales and soil organic carbon (SOC) showed a negative correlation. Moreover, only Pisolithus and Sebacina, belonging to Basidiomycota, differentially changed in response to physicochemical and microbial characteristics. These data may indicate that, similar to poplar plantations (Zheng et al., 2017), Ascomycota is relatively more sensitive to changes in soil physicochemical properties in the Eucalyptus plantations.
Three of the most popular planted Eucalyptus species and two plantation ages were studied in this experiment. Especially for the species of Eucalyptus pellita, there was no 5-year-old plantation to compare with the other two species, which might limit the observations. In forest practice, it is easy to have mixed plantations of different Eucalyptus species. Mixed plantations of equal tree age are uncommon. The EP plantations of 1 and 11 years of age, however, showed more or less a similar trend with the other two species with 1- and 5-year-old plantations. We suggest that the results may still reflect the general effect of the age of the plantations. However, further investigations with more species and different plantation ages are needed to draw solid conclusions. In addition, a designated negative control, which could be unplanted soil next to the existing plantations, was lacking in this study and is necessary to validate the observations.
The age of the Eucalyptus plantations was an important driver of fungal alpha diversity and structure in this study. This is consistent with previous studies showing that changes in soil biodiversity in Eucalyptus plantations depend on the age of the plantation and are in parallel with the succession process of Eucalyptus trees (Chen et al., 2011; Zhang et al., 2013). Similar observation results were obtained in boreal Pinus sylvestris (Lim and Berbee, 2013) and Tsuga mertensiana (Kyaschenko et al., 2017) forests, northern temperate Quercus mongolica (He et al., 2016) and Pinus koraiensis (Zhiguang et al., 2016) forests, and Chinese subtropical Phyllostachys praecox (Li et al., 2017) forest, in which the changes in the fungal community were likely parallel with shifts in soil properties and vegetation composition caused by potential feedback on the tree stand age.
The age of Eucalyptus plantations affects fungal community richness, alpha diversity, and evenness in a different way. With the increase in the age of Eucalyptus plantations, the richness of the fungal communities increased, whereas the diversity and evenness decreased. Fungi are capable of degrading plant residues, although some of the litter inputs (e.g., lignin) were recalcitrant (Zheng et al., 2017). The higher fungal richness in older Eucalyptus plantations agreed with previous observations that the 5+-year-old plantations received more litter inputs with lower disturbance (e.g., fertilization and weeding) than the 1-year-old plantations (Chen et al., 2011). With increased plantation age, Eucalyptus root exudates contained more allelochemicals, which have inhibiting effects on the production and germination of fungal spores (Batish et al., 2008; Chen et al., 2011). This may cause changes in the abundance of certain fungal groups, resulting in a decrease in evenness and alpha diversity of fungal communities. This conclusion is also in line with the result that fungal diversity and evenness had a positive correlation.
With the increased age of Eucalyptus plantations, changes in fungal community structure were apparent at both low and high taxonomic levels. Several studies have shown that the abundance of Ascomycota decreased, while that of Basidiomycota increased in forest soil across plantation age (Zhiguang et al., 2016; Zheng et al., 2017). In this study, the relative abundance of phylum Ascomycota and its orders Pleosporales, Chaetothyriales, and Eurotiales decreased, whereas that of phylum Basidiomycota and its orders Agaricales, Sebacinales, Cantharellales, and Russulales increased with the increase in the age of the Eucalyptus plantations. The increased plant residues and components (e.g., lignin), with the increase in the age of the Eucalyptus plantations, might contribute to the observations. In the 1-year-old plantations, Eucalyptus trees were in a fast-growing stage with less plant residues containing little lignin after a clear-cutting of the vegetation cover (Cortez et al., 2014). Many fungal groups with cellulolytic ability within Ascomycota, including Pleosporales, Chaetothyriales, Eurotiales, Talaromyces, Penicillium, and Aspergillus, had a high abundance in 1-year-old plantations. These fungi are able to degrade cellulose in the early stages of decomposition (Ma et al., 2013; Galitskaya et al., 2017; Zheng et al., 2017; Zhao et al., 2018). Recalcitrant plant components became more common in the 5+-year-old plantations, where the orders Agaricales, Cantharellales, and Russulales, belonging to Basidiomycota, with the ability to degrade complex polymers (e.g., lignin) were expected to be more abundant (Žifcakova et al., 2011; Nagy et al., 2015). Unlike most of the taxa in Ascomycota, the order Helotiales, which was described as saprotrophs in this study, was enriched in the 5+-year-old plantations similar to Basidiomycota. Previous studies have shown that Helotiales fungi can effectively degrade the allelochemicals produced by Eucalyptus, whereas the activities of members of Aspergillus, Penicillium, and Trichoderma, which were also described as saprotrophs, were strongly inhibited by Eucalyptus essential oil (Batish et al., 2008; Liu et al., 2018).
Previous studies have shown that plantation age affects vegetation biomass and plant residue composition and indirectly changes the physical and chemical properties of soil (Zhiguang et al., 2016; Zheng et al., 2017). The SOC was the most important factor influencing the fungal community structure across stand age in a Chinese subtropical forest (Wu et al., 2013). In this study, most of the core OTUs were described as saprotrophs, and the SOCs were identified as one of the key drivers in the fungal community and functional structures. Members of Pleosporales, Chaetothyriales, and Eurotiales (Ascomycota) have been described previously as saprotrophs and play a crucial role in soil organic matter degradation, and their relative abundance had a significantly positive correlation with SOC mineralization rate (Zheng et al., 2017). In this study, the orders Pleosporales, Chaetothyriales, and Eurotiales (Ascomycota) decreased in abundance along with plantation age, and Eurotiales and its genus Talaromyces, Curvularia (Pleosporales), and Coniosporium (Chaetothyriales) exhibited a significantly negative correlation with the SOC level. These results support previous observations regarding the role of SOC on fungal community structure. In addition, the organic matter derived from fungi is biochemically more resistant and can enhance C storage and slow the turnover of soil organic matter depending on the fungal community (Bailey et al., 2002; Zheng et al., 2017). This could be another association between SOC and fungal community structure.
By FUNGuild analysis of potential function, both saprotrophs and pathotrophs shifted in abundance between plantation age, in which wood saprotrophs, dung saprotrophs, fungal parasites, and animal pathogens increased in abundance, whereas plant pathogens decreased in abundance along with an increase in plantation age. This shift was likely related to the species richness and diversity of epiphytes, bryophytes, and soil animals, which were positively correlated with plantation age because of the presence of numerous microhabitats and habitat continuity in the older forests (Kantvilas and Jarman, 2004; Paillet et al., 2010; Chen et al., 2011; Davey et al., 2014). In addition, the taxa of plant pathogens tended to be relatively more abundant in 1-year-old Eucalyptus plantations. This result is inconsistent with the observation results obtained from Xanthoceras sorbifolia and Populus spp. plantations, where the abundance of certain plant pathogens increased across stand age (Jing et al., 2011; Zhu et al., 2015). This might be due to Eucalyptus essential oils and their major constituent that possess antifungal activity against a wide range of pathogenic fungi, including several species of Aspergillus and Penicillium (Nakahara et al., 2003; Kaur et al., 2012), which had a lower abundance in 5+-year-old Eucalyptus plantations. Some species of Mortierella, which were mainly composed of Mortierellomycota, can produce potential antagonistic compounds against various plant pathogens (Tagawa et al., 2010; Qiao et al., 2017). Therefore, soils with a higher abundance of Mortierella might receive less soil-borne diseases (Qiao et al., 2017). Notably, it is important to bear in mind that the FUNGuild analysis performed in this study was based on species classification, which can only predict potential rather than true fungal functions. Due to such limitation, a more comprehensive functional analysis using methods, such as metagenomics or real-time quantitative PCR, is necessary to further elucidate and test the community functional structure.
In this study, the Eucalyptus species had no effects on fungal alpha diversity. This result partially supports the findings that tree species do not result in changes in fungal alpha diversity (Davey et al., 2014). The fungal communities included saprotrophs, symbiotrophs, and pathotrophs. Changes in tree species may cause a shift in the abundance of certain fungal groups. It is likely that fungal species with similar functions could replace others, resulting in no net change in alpha diversity and function. Although Eucalyptus species partially explained the variation in fungal community structure, only a small number of biomarkers and functional groups differed significantly among the different Eucalyptus species. For instance, both of the order Agaricales and its genus Coprinellus, and undefined saprotrophs, which had higher host specificity, were more abundant in the EUG plantation. This proves the significant correlation between fungal community structure and predicted functional community structure, because most of the members of Agaricales and Coprinellus were described as undefined saprotrophs in this study. Meanwhile, a recent study showed similar results that plant pathogens and saprotrophs can better highlight host preference and/or specificity than ECM fungi (Chen et al., 2019). Different tree species can influence soil microbial biomass, fungal community, and functional structure through the quantity and quality of plant residues (Zeng et al., 2015). The soil microbial biomass (MB), fungal biomass (FB), and bacterial biomass (BB) were strongly affected by Eucalyptus species (Xu et al., 2020). Interestingly, the SOC in this study was also affected by the Eucalyptus species, which showed significant correlations with the fungal community in the EC5- and EP11y-plantations, but a negative correlation in the EUG5y-plantation. The leaf traits were similar between closely related Eucalyptus species and differed among distantly related species (Jie et al., 2018). Molecular evidence showed that E. urophylla × E. grandis (EUG) and E. pellita (EP) had higher genetic similarities than E. camaldulensis (EC) (Zeng et al., 2010). The fungal community and functional structures in the plantations of E. pellita and E. camaldulensis were more similar than those of E. urophylla × E. grandis; especially, the EP1y- and EC1y-plantations were not significantly different by the fungal community. One explanation for this observed discrepancy might be that Eucalyptus root exudates significantly affect the soil fungal community structure besides the effects of SOC (Wu and Yu, 2019). A previous study has demonstrated that the root exudates of E. grandis (male parent of E. urophylla × E. grandis) can significantly stimulate the growth of members of Pisolithus (Lagrange et al., 2001). In this study, P. orientalis was more abundant in the EUG plantations. Therefore, how Eucalyptus root exudates affect the soil fungal community and the influence of Eucalyptus species on the compositions of root exudates need to be further investigated.
Conclusions
In brief, both the age of the Eucalyptus plantations and species contributed to the shifts in fungal community and functional structures, in which the age of the plantations had stronger effects than the Eucalyptus species. The age of the Eucalyptus plantations, rather than species, affected the fungal alpha community diversity. With the increase in the age of the plantations, the abundance of Ascomycota decreased, while that of Basidiomycota increased. The predicted fungal community function shifted from the dominance of plant pathogens to the abundance of saprotrophs and symbiotrophs. The SOC was the key driver contributing to the shift in the fungal community structure and potential function. The results highlight the importance of the age of Eucalyptus plantations and species on the fungal community structure under atropical monsoon climate.
Data Availability Statement
The datasets presented in this study can be found in online repositories. The names of the repository/repositories and accession number(s) can be found at: https://www.ncbi.nlm.nih.gov/, PRJNA580298.
Author Contributions
HS: conceptualization, investigation, project administration, and funding acquisition. HS and BL: methodology, software, and formal analysis. ZQ, YM, and JX: validation, resources, and supervision. BL: data curation, writing—original draft preparation, and visualization. HS and PC: writing—review and editing. All the authors have read and agreed to the published version of the manuscript.
Funding
This research was funded by the National Natural Science Foundation of China (31870474), research funding for the Jiangsu Specially Appointed Professor (project 165010015), research funding for the Postgraduate Research & Practice Innovation Program of Jiangsu Province (KYCX19_1076), and research funding for the Priority Academic Program Development (PAPD) of Jiangsu Higher Education Institutions.
Conflict of Interest
The authors declare that the research was conducted in the absence of any commercial or financial relationships that could be construed as a potential conflict of interest.
Publisher's Note
All claims expressed in this article are solely those of the authors and do not necessarily represent those of their affiliated organizations, or those of the publisher, the editors and the reviewers. Any product that may be evaluated in this article, or claim that may be made by its manufacturer, is not guaranteed or endorsed by the publisher.
Acknowledgments
We thank Shuai-fei Chen and Guo-qing Li at the China Eucalyptus Research Centre, Zhanjiang, for the assistance with the fieldwork.
Supplementary Material
The Supplementary Material for this article can be found online at: https://www.frontiersin.org/articles/10.3389/ffunb.2021.703467/full#supplementary-material
References
Abarenkov, K., Nilsson, R. H., Larsson, K., Alexander, I. J., Eberhardt, U., Erland, S., et al. (2010). The unite database for molecular identification of fungi-recent updates and future perspectives. New Phytol. 186, 281–285. doi: 10.1111/j.1469-8137.2009.03160.x
Alfaro, F. D., Manzano, M., Marquet, P. A., and Gaxiola, A. (2017). Microbial communities in soil chronosequences with distinct parent material: the effect of soil pH and litter quality. J. Ecol. 105, 1709–1722. doi: 10.1111/1365-2745.12766
Alguacil, M. M., Torrecillas, E., Lozano, Z., and Roldán, A. (2015). Arbuscular mycorrhizal fungi communities in a coral cay system Morrocoy, Venezuela. and their relationships with environmental variables. Sci. Total Environ. 505, 805–813. doi: 10.1016/j.scitotenv.2014.10.030
Anderson, M. J. (2001). A new method for non-parametric multivariate analysis of variance. Austral. Ecol. 26, 32–46. doi: 10.1046/j.1442-9993.2001.01070.x
Bailey, V. L., Smith, J. L., and Bolton, H. (2002). Fungal-to-bacterial ratios in soils investigated for enhanced C sequestration. Soil Biol. Biochem. 34, 997–1007. doi: 10.1016/S0038-0717(02)00033-0
Batish, D. R., Singh, H. P., Kohli, R. K., and Kaur, S. (2008). Eucalyptus essential oil as a natural pesticide. Forest Ecol. Manag. 256, 2166–2174. doi: 10.1016/j.foreco.2008.08.008
Burke, D. J., Weintraub, M. N., Hewins, C. R., and Kalisz, S. (2011). Relationship between soil enzyme activities, nutrient cycling and soil fungal communities in a northern hardwood forest. Soil Biol. Biochem. 43, 795–803. doi: 10.1016/j.soilbio.2010.12.014
Castaño, C., Dejene, T., Mediavilla, O., Geml, J., Juan Andrés Oria-de-Rueda, J. A., and Pablo Martín-Pinto, P. (2019). Changes in fungal diversity and composition along a chronosequence of Eucalyptus grandis plantations in Ethiopia. Fungal Ecol. 39, 328–335. doi: 10.1016/j.funeco.2019.02.003
Chen, L., Swenson, N. G., Ji, N., Mi, X., Ren, H., Guo, L., et al. (2019). Differential soil fungus accumulation and density dependence of trees in a subtropical forest. Science 366, 124–128. doi: 10.1126/science.aau1361
Chen, L., Zhang, D., and Zhang, J. (2011). Soil microbial biodiversity across a range of Eucalyptus grandis plantation ages. J. Sichuan Agric. Univ. 29, 472–476. doi: 10.3969/j.issn.1000-2650.2011.04.005
Chippendale, G. M. (1981). The Natural Distribution of Eucalyptus in Australia. Canberra: Special Publication - Australian National Parks and Wildlife Service. No. 6.
Cook, R. L., Binkley, D., and Stape, J. L. (2016). Eucalyptus plantation effects on soil carbon after 20 years and three rotations in brazil. Forest Ecol. Manag. 359, 92–98. doi: 10.1016/j.foreco.2015.09.035
Cortez, C. T., Nunes, L., Rodrigues, L. B., Eisenhauer, N., and Araujo, A. F. (2014). Soil microbial properties in Eucalyptus grandis plantations of different ages. Afr. Res. Bull. Polit. Soc. Cult. 48, 734–742. doi: 10.4067/S0718-95162014005000059
Crowther, T. W., Hoogen, J. V. D., Wan, J., Mayes, M. A., Keiser, A. D., Mo, L., et al. (2019). The global soil community and its influence on biogeochemistry. Science 365:6455. doi: 10.1126/science.aav0550
Davey, M. L., Kauserud, H., and Ohlson, M. (2014). Forestry impacts on the hidden fungal biodiversity associated with bryophytes. FEMS Microbiol. Ecol. 90, 313–325. doi: 10.1111/1574-6941.12386
Debellis, T., Kernaghan, G., Bradley, R., and Widden, P. (2006). Relationships between stand composition and ectomycorrhizal community structure in boreal mixed-wood forests. Microb. Ecol. 52, 114–126. doi: 10.1007/s00248-006-9038-8
Edgar, R. C., Haas, B. J., Clemente, J. C., Christopher, Q., and Rob, K. (2011). Uchime improves sensitivity and speed of chimera detection. Bioinformatics 27, 2194–2200. doi: 10.1093/bioinformatics/btr381
Ekblad, A., Wallander, H., Godbold, D. L., Cruz, C., Johnson, D., Baldrian, P., et al. (2013). The production and turnover of extramatrical mycelium of ectomycorrhizal fungi in forest soils: role in carbon cycling. Plant Soil 366, 1–27. doi: 10.1007/s11104-013-1630-3
Galitskaya, P., Biktasheva, L., Saveliev, A., Grigoryeva, T., Boulygina, E., and Selivanovskaya, S. (2017). Fungal and bacterial successions in the process of co-composting of organic wastes as revealed by 454 pyrosequencing. PLoS ONE 12:e0186051. doi: 10.1371/journal.pone.0186051
Grattapaglia, D., Vaillancourt, R. E., Shepherd, M., Thumma, B. R., Foley, W. J., Kulheim, C., et al. (2012). Progress in Myrtaceae genetics and genomics: Eucalyptus as the pivotal genus. Tree Genet. Genomes 8, 463–508. doi: 10.1007/s11295-012-0491-x
Grbić, M. V. L., Savković, D., and Stupar, M. C. (2015). Phyllospheremycobiota of Eucalyptus camaldulensis DehnhandE. globulusLabill. ZbornikMatice Srpske Za PrirodneNauke 129, 55–64. doi: 10.2298/ZMSPN1529055L
Hagenbo, A., Kyaschenko, J., Clemmensen, K. E., Lindahl, B., Fransson, P., and Wurzburger, N. (2018). Fungal community shifts underpin declining mycelial production and turnover across a pinus sylvestris chronosequence. J. Ecol. 106, 490–501. doi: 10.1111/1365-2745.12917
He, F., Yang, B. S., Wang, H., Yan, Q. L., Cao, Y. N., and He, X. H. (2016). Changes in composition and diversity of fungal communities along Quercus mongolica forests developments in northeast China. Appl. Soil Ecol. 100, 162–171. doi: 10.1016/j.apsoil.2015.12.014
Horn, S., Hempel, S., Verbruggen, E., Rillig, M. C., and Caruso, T. (2017). Linking the community structure of arbuscular mycorrhizal fungi and plants: a story of interdependence? ISME J. 11, 1400–1411. doi: 10.1038/ismej.2017.5
Jie, Q., Wanhong, L., Peng, L., Chubiao, W., Yan, L., and Jianzhong, L. (2018). Genetic variation patterns in leaf morphology on eucalypts and their hybrids. J. Trop. Subtrop. Botany 26, 589–596. doi: 10.11926/jtsb.3882
Jing, W., Lingjun, C., Jun, L., Xingyao, Z., Zhaoxin, Z., Huiqin, W., et al. (2011). Impact of stand features of short-rotation poplar plantations on canker disease incidence at a mesoscale landscape: a case study in qingfeng county, henan province, China. Acta Ecol. Sin. 31, 2757–2767. doi: 10.3969/j.issn.1000-7369.2001.12.039
Kantvilas, G., and Jarman, S. J. (2004). Lichens and bryophytes on Eucalyptus obliqua in Tasmania: management implications in production forests. Biol. Conserv. 117, 359–373. doi: 10.1016/j.biocon.2003.08.001
Kaur, S., Singh, H. P., Batish, D. R., and Kohli, R. K. (2012). Role of monoterpenes in Eucalyptus communities. Curr. Bioactive Compounds 8, 101–107. doi: 10.2174/157340712799828232
Klute, A. (1988). Methods of soil analysis. Part 1. Physical and mineralogical methods. Soil Sci. 146:138. doi: 10.1097/00010694-198808000-00014
Kooch, Y., Samadzadeh, B., and Hosseini, S. M. (2017). The effects of broad-leaved tree species on litter quality and soil properties in a plain forest stand. Catena 150, 223–229. doi: 10.1016/j.catena.2016.11.023
Kyaschenko, J., Clemmensen, K. E., Hagenbo, A., Karltun, E., and Lindahl, B. D. (2017). Shift in fungal communities and associated enzyme activities along an age gradient of managed Pinus sylvestris stands. ISME J. 11, 863–874. doi: 10.1038/ismej.2016.184
Laclau, J. P., Ranger, J., Goncalves, M. J. L., Maquère, V., Krusche, A. V., M'Bou, A. T., et al. (2010). Biogeochemical cycles of nutrients in tropical Eucalyptus plantations: main features shown by intensive monitoring in Congo and Brazil. Forest Ecol. Manag. 259, 1771–1785. doi: 10.1016/j.foreco.2009.06.010
Lagrange, H., Jayallgmand, C., and Lapeyrie, F. (2001). Rutin, the phenolglycoside from Eucalyptus root exudates, stimulates Pisolithushyphal growth at picomolar concentrations. New Phytol. 149, 349–355. doi: 10.1046/j.1469-8137.2001.00027.x
Lazzaro, A., Hilfiker, D., and Zeyer, J. (2015). Structures of microbial communities in alpine soils: seasonal and elevational effects. Front. Microbiol. 6:1330. doi: 10.3389/fmicb.2015.01330
Li, M. S., Mao, L. J., Shen, W. J., Liu, S. Q., and Wei, A. S. (2013). Change and fragmentation trends of Zhanjiang mangrove forests in southern China using multi-temporal landsat imagery (1977–2010). Estuar. Coast. Shelf Sci. 130, 111–120. doi: 10.1016/j.ecss.2013.03.023
Li, Z., Fu, J., Zhou, R., and Wang, D. (2018). Effects of phenolic acids from ginseng rhizosphere on soil fungi structure, richness and diversity in consecutive monoculturing of ginseng. Saudi J. Biol. Sci. 25, 1788–1794. doi: 10.1016/j.sjbs.2018.07.007
Li, Y., Li, Y., Chang, S. X., Liang, X., Qin, H., Chen, J., et al. (2017). Linking soil fungal community structure and function to soil organic carbon chemical composition in intensively managed subtropical bamboo forests. Soil Biol. Biochem. 107, 19–31. doi: 10.1016/j.soilbio.2016.12.024
Lim, S., and Berbee, M. L. (2013). Phylogenetic structure of ectomycorrhizal fungal communities of western hemlock changes with forest age and stand type. Mycorrhiza 23, 473–486. doi: 10.1007/s00572-013-0488-9
Liu, J., Sui, Y., Yu, Z., Shi, Y., Chu, H., Jin, J., et al. (2015). Soil carbon content drives the biogeographical distribution of fungal communities in the black soil zone of northeast China. Soil Biol. Biochem. 83, 29–39. doi: 10.1016/j.soilbio.2015.01.009
Liu, S., Qin, F., and Yu, S. (2018). Eucalyptusurophylla root-associated fungi can counteract the negative influence of phenolic acid allelochemicals. Appl. Soil Ecol. 127, 1–7. doi: 10.1016/j.apsoil.2018.02.028
Liu, X., Chen, Q., Wang, Z., Xie, L., and Xu, Z. (2008). Allelopathic effects of essential oil from Eucalyptus grandis × E. urophylla on pathogenic fungi and pest insects. Front. For. China 3, 232–236. doi: 10.1007/s11461-008-0036-5
Liu, X. J., Xu, D. P., Xie, Z. S., and Zhang, N. N. (2009). Effects of different culture media on the growth of Indian sandalwood (Santalum album L.) seedlings in Zhanjiang, Guangdong, southern China. Forestry Stud. China 11, 132–138. doi: 10.1007/s11632-009-0023-4
Ma, A., Zhuang, X., Wu, J., Cui, M., Lv, D., Liu, C., et al. (2013). Ascomycota members dominate fungal communities during straw residue decomposition in arable soil. PLoS ONE 8:e66146. doi: 10.1371/journal.pone.0066146
Malajczuk, N., Molina, R., and Trappe, J. M. (2010). Ectomycorrhiza formation in Eucalyptus pure culture synthesis, host specificity and mycorrhizal compatibility with Pinus radiata. New Phytol. 91, 467–482. doi: 10.1111/j.1469-8137.1982.tb03325.x
Mcdonald, M. W., Brooker, M. I., and Butcher, P. A. (2009). A taxonomic revision of Eucalyptus camaldulensis (Myrtaceae). Aust. Syst. Bot. 22, 257–285. doi: 10.1071/SB09005
Mcguire, K. L., Bent, E., Borneman, J., Majumder, A., Allison, S. D., and Treseder, K. K. (2010). Functional diversity in resource use by fungi. Ecology 91, 2324–2332. doi: 10.1890/09-0654.1
Nagy, L., Robert, R., Andrew, T., Adam, C., Daum, C., Floudas, D., et al. (2015). Comparative genomics of early-diverging mushroom-forming fungi provides insights into the origins of lignocellulose decay capabilities. Mol. Biol. Evol. 4:4. doi: 10.1093/molbev/msv337
Nakahara, K., Alzoreky, N. S., Yoshihashi, T., Nguyen, H. T. T., and Trakoontivakorn, G. (2003). Chemical composition and antifungal activity of essential oil from Cymbopogon nardus (citronella grass). Jpn. Agr. Res. Q. 4, 249–252. doi: 10.6090/jarq.37.249
Nguyen, N. H., Song, Z., Bates, S. T., Branco, S., Tedersoo, L., Menke, J., et al. (2016). FUNGuild: an open annotation tool for parsing fungal community datasets by ecological guild. Fungal Ecol. 20, 241–248. doi: 10.1016/j.funeco.2015.06.006
Paillet, Y., Berges, L., Hjalten, J., Odor, P., and Avon, C. (2010). Biodiversity differences between managed and unmanged forests: meta-analysis of species richness in Europe. Conserv. Biol. 24, 101–112. doi: 10.1111/j.1523-1739.2009.01399.x
Peay, K. G., Kennedy, P. G., and Bruns, T. D. (2008). Fungal community ecology: a hybrid beast with a molecular master. Bioscience 58, 799–810. doi: 10.1641/B580907
Poisot, T., Canard, E., Mouquet, N., and Hochberg, M. E. (2012). A comparative study of ecological specialization estimators. Methods Ecol. Evol. 3, 537–544. doi: 10.1111/j.2041-210X.2011.00174.x
Pryor, L. D., Williams, E. R., and Gunn, B. V. (1995). A morphometric analysis of Eucalyptus urophylla and related taxa with descriptions of two new species. Aust. Syst. Bot. 8, 57–70. doi: 10.1071/SB9950057
Qiao, S. S., Zhou, Y. N., Chai, B. F., Jia, T., and Li, C. (2017). Characteristics of fungi community structure and genetic diversity of forests in Guandi Mountains. Huanjingkexue 38, 2502–2512. doi: 10.13227/j.hjkx.201611101
Qin, F., Liu, S., and Yu, S. (2018). Effects of allelopathy and competition for water and nutrients on survival and growth of tree species in Eucalyptus urophylla plantations. For. Ecol. Manage. 424, 387–395. doi: 10.1016/j.foreco.2018.05.017
Qu, Z., Liu, B., Ma, Y., and Sun, H. (2020). Differences in bacterial community structure and potential functions among Eucalyptus plantations with different ages and species of trees. Appl. Soil Ecol. 149:103515. doi: 10.1016/j.apsoil.2020.103515
Rousk, J., Baath, E., Brookes, P. C., Lauber, C. L., Lozupone, C. A., Caporaso, J. G., et al. (2010). Soil bacterial and fungal communities across a pH gradient in an arable soil. ISME J. 4, 1340–1351. doi: 10.1038/ismej.2010.58
Schilling, E. M., Waring, B. G., Schilling, J. S., and Powers, J. S. (2016). Forest composition modifies litter dynamics and decomposition in regenerating tropical dry forest. Oecologia 182, 287–297. doi: 10.1007/s00442-016-3662-x
Schloss, P. D., Westcott, S. L., Ryabin, T., Hall, J. R., Hartmann, M., Hollister, E. B., et al. (2009). Introducing Mothur: open-source, platform-independent, community-supported software for describing and comparing microbial communities. Appl. Environ. Microbiol. 75, 7537–7541. doi: 10.1128/AEM.01541-09
Schmidt, S. K., Nemergut, D. R., Darcy, J. L., and Lynch, R. (2014). Do bacterial and fungal communities assemble differently during primary succession? Mol. Ecol. 23, 254–258. doi: 10.1111/mec.12589
Segata, N., Izard, J., Waldron, L., Gevers, D., Miropolsky, L., Garrett, W. S., et al. (2011). Metagenomic biomarker discovery and explanation. Genome biol. 12:R60. doi: 10.1186/gb-2011-12-6-r60
Shade, A., Jones, S. E., Caporaso, J. G., Handelsman, J., Knight, R., Fierer, N., et al. (2014). Conditionally rare taxa disproportionately contribute to temporal changes in microbial diversity. mBio 5:e01371–14. doi: 10.1128/mBio.01371-14
Stanturf, J. A., Vance, E. D., Fox, T. R., and Kirst, M. (2013). Eucalyptus beyond its native range: environmental issues in exotic bioenergy plantations. Int. J. For. Res. 2013, 1–5. doi: 10.1155/2013/463030
Sulzbacher, M. A., Grebenc, T., Bevilacqua, C. B., Steffen, R. B., Coelho, G., Silveira, A. O., et al. (2018). Co-invasion of ectomycorrhizal fungi in the Brazilian Pampa biome. Appl. Soil Ecol. 130, 194–201. doi: 10.1016/j.apsoil.2018.06.007
Sun, H., Terhonen, E., Kovalchuk, A., Tuovila, H., Chen, H., Oghenekaro, A. O., et al. (2016). Dominant tree species and soil type affect the fungal community structure in a boreal peatland forest. Appl. Environ. Microbiol. 82, 2632–2643. doi: 10.1128/AEM.03858-15
Tagawa, M., Tamaki, H., Manome, A., Koyama, O., and Kamagata, Y. (2010). Isolation and characterization of antagonistic fungi against potato scab pathogens from potato field soils. FEMS Microbiol. Lett. 305, 136–142. doi: 10.1111/j.1574-6968.2010.01928.x
Tedersoo, L., Bahram, M., and Põlme, S. (2014). Global diversity and geography of soil fungi. Science 346:1256688. doi: 10.1126/science.1256688
Tedersoo, L., Nilsson, R. H., Abarenkov, K., Jairus, T., Sadam, A., Saar, I., et al. (2010). 454 pyrosequencing and sanger sequencing of tropical mycorrhizal fungi provide similar results but reveal substantial methodological biases. New Phytol. 188, 291–301. doi: 10.1111/j.1469-8137.2010.03373.x
Uroz, S., Buèe, M., Deveau, A., Mieszkin, S., and Martin, F. (2016). Ecology of the forest microbiome: highlights of temperate and boreal ecosystems. Soil Biol. Biochem. 103, 471–488. doi: 10.1016/j.soilbio.2016.09.006
van der Linde, S., Suz, L. M., Orme, C. D. L., Cox, F., Andreae, H., Asi, E., et al. (2018). Environment and host as large-scale controls of ectomycorrhizal fungi. Nature 558, 243–248. doi: 10.1038/s41586-018-0189-9
Wu, J., and Yu, S. (2019). Effect of root exudates of Eucalyptus urophylla and Acacia mearnsii on soil microbes under simulated warming climate conditions. BMC Microbiol. 19, 1–12. doi: 10.1186/s12866-019-1604-6
Wu, Y. T., Wubet, T., Trogisch, S., Both, S., Scholten, T., and Bruelheide, H. (2013). Forest age and plant species composition determine the soil fungal community composition in a Chinese subtropical forest. PLoS ONE 8:e66829. doi: 10.1371/journal.pone.0066829
Xu, J., Liu, B., Qu, Z. L., Ma, Y., and Sun, H. (2020). Age and species of Eucalyptus plantations affect soil microbial biomass and enzymatic activities. Microorganisms 8:811. doi: 10.3390/microorganisms8060811
Yang, X., Li, D., McGrouther, K., Long, W., Li, Y., Chen, Y., et al. (2017). Effect of Eucalyptus forests on understory vegetation and soil quality. J. Soils Sediments 17, 2383–2389. doi: 10.1007/s11368-016-1431-4
Zeng, Z., Wang, S., Zhang, C., Tang, H., Li, X.i., Wu, Z., et al. (2015). Soil microbial activity and nutrients of evergreen broad-leaf forests in mid-subtropical region of China. J. For. Res. 26, 673–678. doi: 10.1007/s11676-015-0060-x
Zeng, Y. L., Tan, X. F., Zhang, D. Q., Xie, P., and Zeng, X. F. (2010). Analysis of genetic relationship of Eucalyptus by ISSR markers. Seed 29, 24–27. doi: 10.16590/j.cnki.1001-4705.2010.01.085
Zhang, D. J., Zhang, J., Yang, W. Q., Wu, F. Z., Huang, Y. M., and Zhang, Z. W. (2013). Plant′s and soil organism′s diversity across a range of Eucalyptus grandis plantation ages. Acta Ecol. Sin. 33, 3947–3962. doi: 10.5846/stxb201208021098
Zhao, C., Deng, L., and Fang, H. (2018). Mixed culture of recombinant Trichoderma reeseiand Aspergillus niger for cellulase production to increase the cellulose degrading capability. Biomass Bioenerg. 112, 93–98. doi: 10.1016/j.biombioe.2018.03.001
Zheng, J., Chen, J., Pan, G., Wang, G., Liu, X., Zhang, X., et al. (2017). A long-term hybrid poplar plantation on cropland reduces soil organic carbon mineralization and shifts microbial community abundance and composition. Appl. Soil Ecol.111, 94–104. doi: 10.1016/j.apsoil.2016.11.017
Zhiguang, H., Xin, S., and Mengsha, L. (2016). Effects of forest age on soil fungal community in a northern temperate ecosystem. Indian J. Microbiol. 56, 328–334. doi: 10.1007/s12088-016-0577-5
Zhu, L., Huang, J., Chen, T. Y., and Zhou, Y. B. (2015). Root-associated and endophytic fungal community diversity in Xanthocerassorbifolia Bunge plantation. J. Northeast Forestry University 43, 105–111. doi: 10.13759/j.cnki.dlxb.20150522.007
Keywords: fungal community structure, community function, plantation age, Eucalyptus species, tropical monsoon climate
Citation: Liu B, Qu Z, Ma Y, Xu J, Chen P and Sun H (2021) Eucalyptus Plantation Age and Species Govern Soil Fungal Community Structure and Function Under a Tropical Monsoon Climate in China. Front. Fungal Biol. 2:703467. doi: 10.3389/ffunb.2021.703467
Received: 05 May 2021; Accepted: 17 August 2021;
Published: 10 September 2021.
Edited by:
Anna Maria Pirttilä, University of Oulu, FinlandReviewed by:
Tine Grebenc, Slovenian Forestry Institute, SloveniaSamuele Voyron, University of Turin, Italy
Copyright © 2021 Liu, Qu, Ma, Xu, Chen and Sun. This is an open-access article distributed under the terms of the Creative Commons Attribution License (CC BY). The use, distribution or reproduction in other forums is permitted, provided the original author(s) and the copyright owner(s) are credited and that the original publication in this journal is cited, in accordance with accepted academic practice. No use, distribution or reproduction is permitted which does not comply with these terms.
*Correspondence: Hui Sun, hui.sun@njfu.edu.cn