- W. Harry Feinstone Department of Molecular Microbiology and Immunology, Bloomberg School of Public Health, Johns Hopkins University, Baltimore, MD, United States
The increased application of chemical control programs has led to the emergence and spread of insecticide resistance in mosquitoes. Novel environmentally safe control strategies are currently needed for the control of disease vectors. The use of entomopathogenic fungi could be a suitable alternative to chemical insecticides. Currently, Beauveria spp. and Metarhizium spp. are the most widely used entomopathogenic fungi for mosquito control, but increasing the arsenal with additional fungi is necessary to mitigate the emergence of resistance. Entomopathogenic fungi are distributed in a wide range of habitats. We have performed a comprehensive screen for candidate mosquitocidal fungi from diverse outdoor environments in Maryland and Puerto Rico. An initial screening of 22 fungi involving exposure of adult Anopheles gambiae to 2-weeks-old fungal cultures identified five potent pathogenic fungi, one of which is unidentified and the remaining four belonging to the three genera Galactomyces sp., Isaria sp. and Mucor sp. These fungi were then screened against Aedes aegypti, revealing Isaria sp. as a potent mosquito killer. The entomopathogenic effects were confirmed through spore-dipping assays. We also probed further into the killing mechanisms of these fungi and investigated whether the mosquitocidal activities were the result of potential toxic fungus-produced metabolites. Preliminary assays involving the exposure of mosquitoes to sterile filtered fungal liquid cultures showed that Galactomyces sp., Isaria sp. and the unidentified isolate 1 were the strongest producers of factors showing lethality against An. gambiae. We have identified five fungi that was pathogenic for An. gambiae and one for Ae. aegypti, among these fungi, four of them (two strains of Galactomyces sp., Mucor sp., and the unidentified isolate 1) have never previously been described as lethal to insects. Further characterization of these entomopathogenic fungi and their metabolites needs to be done to confirm their potential use in biologic control against mosquitoes.
Introduction
Vector-borne disease accounts for more than 17% of all infectious disease, causing more than 700,000 deaths annually. Anopheles gambiae is the most important malaria vector, and Aedes aegypti is responsible for the transmission of dengue fever, Zika virus disease, chikungunya, Rift Valley fever, and yellow fever, among others [World Health Organization (WHO), 2020]. Current mosquito vector control strategies are primarily based on synthetic insecticides. However, the increased implementation of chemical control programs has led to the emergence and spread of insecticide resistance in Anopheles sp. (An.) and Aedes sp. (Ae.) populations and is also affecting non-target organisms, including humans (Moyes et al., 2017; Riveron et al., 2018).
Several studies have demonstrated the potential of using entomopathogenic fungi for controlling mosquito vectors (Charnley and Collins, 2007), as an effective and environmentally safe strategy. Since one of the mode of action of entomopathogenic fungi is mediated through surface contact with adult mosquitoes, these agents would be applicable to a variety of deployment strategies, some of which are already in use for chemical insecticides (Scholte et al., 2005; Farenhorst et al., 2011). More importantly, they are effective against mosquito strains that have developed resistance to the available chemical insecticides (Farenhorst et al., 2009, 2010; Blanford et al., 2011). Furthermore, in contrast to chemical insecticides that generally kill mosquitoes within 24 h, fungal biopesticides usually require more than a week to kill exposed mosquitoes and thereby the probability is decreased that resistance will emerge (Ffrench-Constant, 2005; Read et al., 2009).
Metarhizium spp. and Beauveria spp. are the best characterized and most widely used fungi in biological control programs (Scholte et al., 2005; Hancock, 2009; Knols et al., 2010; Blanford et al., 2011; Farenhorst et al., 2011). About 13 species and sub-species of both these fungi have been formulated and registered as mycoinsecticides or mycoacaricides (de Faria and Wraight, 2007). While their mode of action against adult mosquitoes is based on slow and gradual penetration and invasion through the invertebrate cuticle, the emergence of resistance is still a likely event, as has been demonstrated by Dubovskiy et al. (2013). It is therefore necessary to expand the repertoire of fungal biopesticides with additional fungi in order to mitigate the emergence of resistance; the availability of additional fungal biopesticides will potentially also enable a greater versatility of exposure routes that may be dependent of the biology of the fungus. Key stages of the infection process by entomopathogenic fungi are: (a) adhesion of infectious spores to the surface of the insect cuticle; (b) penetration of the cuticle via enzymes and mechanical force; (c) colonization of the hemocoel; and (d) emergence of the conidiophores for external sporulation on the insect cadaver (Butt et al., 2016). In addition, entomopathogenic fungi produce a variety of secondary metabolites, some of which are highly toxic to the larval, pupal, and adult mosquito stages, as well as to some pathogens such as the malaria parasite (Singh and Prakash, 2012; Niu et al., 2015; Vivekanandhan et al., 2018a, 2020a). A better understanding of the toxic metabolites produced by fungi can help unravel the relevant mosquitocidal mechanisms and enable the development of novel natural product-based mosquito control agents.
Entomopathogenic fungi are present in a wide range of habitats (Lacey and Fransen, 1996; Chandler et al., 1997; Sánchez-Peña et al., 2011). Here we aimed at discovering novel mosquitocidal fungi from diverse habitats with activity against An. gambiae and Ae. aegypti adults. We use three different ways to expose adult mosquitoes to the isolated environmental fungi, the first exposure assay was primarily used as a screening method to differentiate pathogenic from non-pathogenic environmental fungi. This method was a direct exposure of adult mosquitoes to fungal cultures on agar plates containing fungal mycelia, the growing hyphae, spores and possible toxic compounds all together. The second methodology involved the use of purified spores in solution (108 spores/ml) alone. The third way to expose mosquitoes, to determine whether the killing effect is mediated by toxic metabolites and/or requires actual exposure to live fungi, was sugar fed adult mosquitoes with a sugar solution containing only the fungal metabolites.
Results
Fungus Sample Collection
To maximize the probability of identifying novel fungi with entomopathogenic properties, samples of fungus-containing plants, decomposing material, soil, and stagnant water were collected from outdoor mosquito habitats in Maryland (MD) and Puerto Rico (Table 1).
In Baltimore, MD, samples were collected from six different sources, including stagnant water from containers, a fountain, and a pond, and from soil in an outdoor pot and two tree flowers. In the rural area of Maunabo, Puerto Rico, 30 fungal samples were collected from 15 different types of leaves, five different decomposing materials, nine types of flowers, and one sample was collected from moss.
Culturing and Identification of Fungi
The 36 environmental samples were manually homogenized and plated on rich fungal solid growth medium to allow the growth of as many fungi as possible. Each morphologically distinct fungal colony was re-streaked on a new agar plate until an axenic fungal culture was attained for each of the different fungal colonies. Once these were separated and streaked on individual plates, several isolates did not grow for more than one generation and were thus excluded from the study. Possible explanations for this could be that these isolates had an obligate symbiosis relationship with microorganisms in the collected sample, or that they could not produce a sufficient number of spores necessary to propagate under laboratory conditions. The 36 collected samples generated a total of 76 fungal isolates, 22 of which could be successfully cultured under the specified laboratory conditions (Figure 1). DNA was extracted from these 22 fungi and used for ribosomal 18S gene amplification and sequencing for identification (Table 1). After the DNA extraction, ribosomal 18S gene amplification was performed using internal transcribed spacer (ITS) degenerate primers, with either of two forward primers (ITS1, ITS3) in combination with one common reverse primer (ITS4) (Supplementary Table 1).
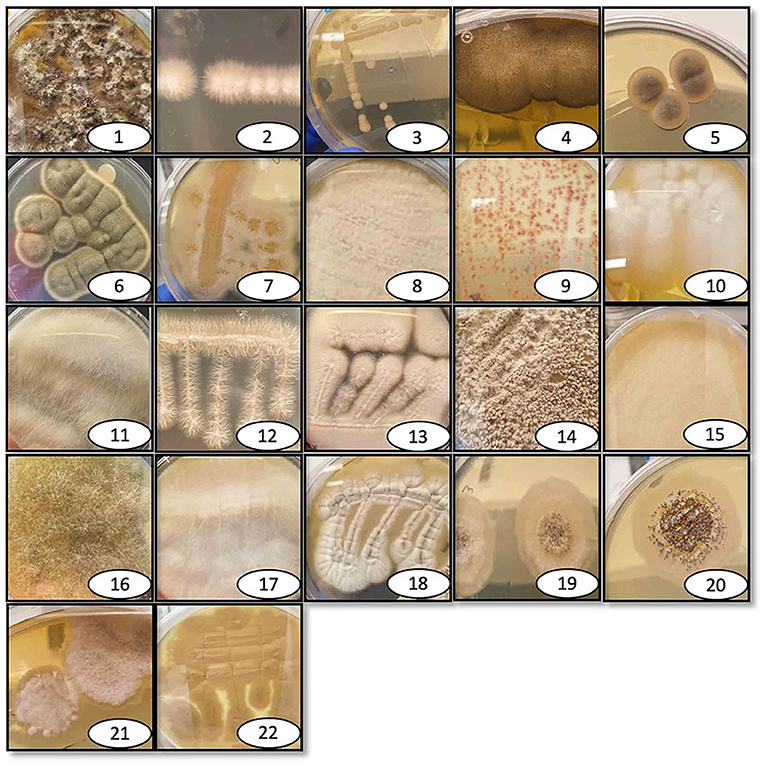
Figure 1. Pictures of isolated and identified fungi. Individual photographs of the isolated fungi that are identified here, labeled with the reference number, and listed in Table 1. The fungi were collected at various outdoor locations and maintained in the lab on either rich growth agar medium brain heart infusion or Sabouraud dextrose, depending on the fungal species.
The sequence identity was determined through BLAST nucleotide searches against the NCBI database (Table 1). The identity of the fungi was determined through analysing phylogenetic trees constructed using MEGAX software with the the Bootstrap and the Neighbor-joining statistic method (Supplementary Files 1–3).
As many as 20 of the 22 isolated fungi were identified down to genus level using a cut-off of 98% for query coverage and identity. One isolate (Ascomycota) was identified only at the phylum level, and the identity of another isolate was ambiguous and was therefore called fungal isolate 1. The top hit for fungal isolate 1 when BLASTed was Aaosphaeria sp., but as can be seen in the phylogenetic tree this result was not conclusive as several other genera appears equally identical. The 22 fungal isolates belonged to the following 12 different genera and one phylum: phylum Ascomycota, Aureobasidium sp., Cercospora sp., Cladosporium sp., Colletotrichum sp., Fusarium sp., Galactomyces sp., Isaria sp., Mucor sp., Penicillium sp., Phomopsis sp., Pleosporales sp. and Scopulariopsis sp. (ITS-based sequences are presented in Supplementary File 1). Some of the identified fungi have different nomenclature depending on the sexual stage; Galactomyces, Isaria, Fusarium, Mucor and Phomopsis are synonymous to Geotricum, Cordyceps/Paecylomyces, Fusicola, Rhizomucor and Diaporthe, respectively (Eliskases-Lechner et al., 2011; Gräfenhan et al., 2011; Udayanga et al., 2011; Sun et al., 2020).
The most common genus was Fusarium sp., being represented by four isolates that originated from both Puerto Rico and Maryland. Two fungal isolates each of Cladosporium sp. Galactomyces sp. and Phomopsis sp. were represented in our collection. Cladosporium sp. was identified in both Puerto Rico and Maryland, while Galactomyces sp. and Phomopsis sp. isolates were only identified in Maryland or Puerto Rico, respectively. Single isolates were identified for the remaining fungal genera.
Screening for Entomopathogenic Activity With a Fungus Direct-Exposure Assay Against An. gambiae Adults
As an initial screen to identify potential entomopathogenic isolates, our 22 isolated fungi were tested for mosquitocidal activity through a direct exposure assay against adult non-blood-fed females of the major malaria vector An. gambiae. This mosquito species is in general more delicate and therefore more susceptible to pathogens than is the more robust arboviral vector Ae. aegypti (Beckage et al., 2004; Alkhaibari et al., 2017). In short, adult females were placed directly on the fungus culture agar plate, which was then shaken for 30 s to assure extensive mosquito surface exposure. Sterile BHI agar plates were used as a negative control. The mosquitoes were then transferred to cups, and survival was monitored for 20 days. Fungus-mediated lethality was estimated by calculating the p-value with a long-rank Mantel-Cox analysis, and a value of <0.0001 was considered indicative of highly potent entomopathogenic activity. From this first screening, a total of five fungal isolates (isolate 1, two Galactomyces sp., Isaria sp., Mucor 3 sp.) showed potent killing activity against An. gambiae females, with these mosquitoes having a significantly (p < 0.0001) shorter lifespan than did the non-fungus-exposed mosquitoes (Table 2, Supplementary Figure 1).
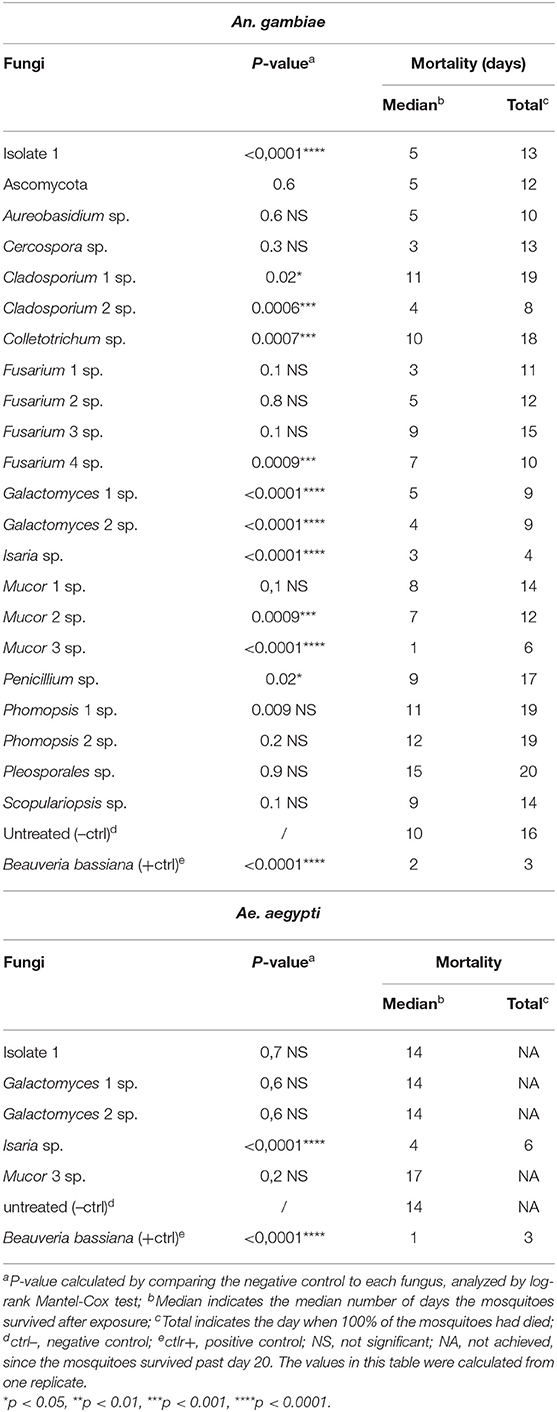
Table 2. Mortality of An. gambiae and Ae. aegypti mosquitoes exposed through fungal direct-exposure assay.
The median survival of the non-fungus-exposed mosquitoes was 10 days; Mucor 3 sp. displayed the most potent lifespan-shortening activity, resulting in a median mosquito survival period of 1 day, followed by Isaria sp. (3 days), Galactomyces 2 sp. (4 days), and fungal isolate 1 and Galactomyces 1 sp. (5 days). Exposure to the positive control B. bassiana resulted in a median mosquito survival of 2 days (Table 2). All non-fungus-exposed mosquitoes had died by 16 days, all isolate 1-exposed mosquitoes had died by 13 days, all mosquitoes exposed to either of the two Galactomyces sp. isolates had died by 9 days, those exposed to Isaria had died by 4 days, and the Mucor 3 sp.-exposed mosquitoes had died by 6 days. Mosquitoes exposed to the positive control B. bassiana were all dead at day 3 (Table 2). Three of the entomopathogenic fungi we discovered, isolate 1, Isaria sp., and Mucor 3 sp., displayed an intense fungal proliferation/growth on the dead mosquito carcasses. Mosquitoes that had succumbed after Galactomyces sp. exposure showed a darker coloration than that of the non-fungus-exposed mosquitoes.
The interesting findings from the screen made us re-evaluate the identification of four of the five potent fungal isolates. Based on the ITS, ribosomal small subunit (SSU) and ribosomal large subunit (LSU)-sequencing data, the phylogenetic trees (Supplementary Files 1–3) and spore photos of the four interesting fungi (Supplementary Figure 2) we were able to suggest a species for four of the five fungal isolates. These are Galactomyces candidum 1, Galactomyces candidum 2, Isaria fumosorosea, and Mucor hiemalis.
Testing for Entomopathogenic Activity Against Ae. aegypti Adults
Next, we decided to evaluate the five fungi (isolate 1, G. candidum 1, G. candidum 2, I. fumosorosea, and M. hiemalis) that had been shown to be entomopathogenic in the An. gambiae-based screen against Ae. aegypti using the same direct exposure assay. Of these five fungi, only I. fumosorosea showed a highly potent entomopathogenic activity against Ae. aegypti that was comparable to the activity of the positive control B. bassiana; they both had a p-value of <0.0001 (Table 2, Supplementary Figure 1). While the median lifespan of the non-exposed mosquitoes was 14 days, the lifespan for those exposed to Isaria sp. or the positive control B. bassiana were 4 days and 1 day, respectively. For the Isaria sp.-exposed mosquitoes, 100% mortality was reached on day 6, whereas those exposed to the positive control B. bassiana died within a 3-day period after exposure (Table 2).
Determining Entomopathogenic Activity With Spore-Dipping Fungus Exposure Assays Against An. gambiae and Ae. aegypti Adults
In order to confirm the outcome of the direct-exposure mosquitocidal assays and to further explore the entomopathogenic activities of the five selected fungal isolates, We performed spore-dipping assays against An. gambiae and Ae. aegypti. This type of assay allowed us to better control the concentration of spores to which mosquitoes were exposed and thereby obtain more reproducible and comparable data. In contrast to the direct exposure assay, in this method the mosquitoes are only exposed to spores and not fungal mycelia, growing hyphae, or possible toxic compounds. We used a spore concentration corresponding to that used in previous studies (Dong et al., 2012; Vivekanandhan et al., 2020b). Adult female mosquitoes were dipped for 1 min into a spore solution, to allow the spores to come in contact with the mosquito surface. As a negative control, mosquitoes were dipped in a non-spore-containing solution. The spore-dipping experiments were performed in triplicate, and the two mosquito species were tested with the respective fungi that had shown a significant (p < 0.0001) killing activity in the direct-exposure assay. Five-days-old An. gambiae females were tested against isolate 1, G. candidum 1 and 2, I. fumosorosea, and M. hiemalis. All five fungi displayed a strong and significant (p < 0.0001) mosquitocidal activity after dipping of the mosquitoes into the spore solution (Table 3, Figure 2A). The median lifespan of the negative control cohort was 8 days, and the mosquitoes exposed to isolate 1, G. candidum 2, or I. fumosorosea displayed a 2-day median survival time. Mosquitoes exposed to spores of G. candidum 1 or M. hiemalis showed a median survival time of 4 and 3 days, respectively. Mosquitoes exposed to the positive control B. bassiana had a median survival time of 3 days. The spores of these fungal isolates killed all the adults within 6–9 days, depending on the replicates, whereas the non-spore-treated mosquitoes died within 14–18 days in the three biological replicates. These findings were also confirmed with a hazard ratio analysis, which expresses the probability of the death event. For example, isolate 1-exposed mosquitoes that had all succumbed by 6 days after exposure had a hazard ratio of 4.4, indicating that death was 4.4 times more likely after exposure to this fungus than if not exposed. For G. candidum 1- and G. candidum 2-exposed mosquitoes, the hazard ratios were 3.9 and 4.5, respectively. For I. fumosorosea-exposed mosquitoes, which had all died by 6 days after exposure, the hazard value was 5.1, and for M. hiemalis-exposed mosquitoes it was 2.5. Mosquitoes exposed to the positive control B. bassiana showed a hazard ratio of 5.4 and 100% mortality was observed after 7 days (Table 3). We also screened adult female Ae. aegypti against Isaria sp. spores, and the results showed a potent reduction of the lifespan (p < 0.0001) (Figure 2B), with a median of survival time of 11 days, as compared to 16 days for the negative control and 9 days for the B. bassiana-exposed positive control (Table 3).
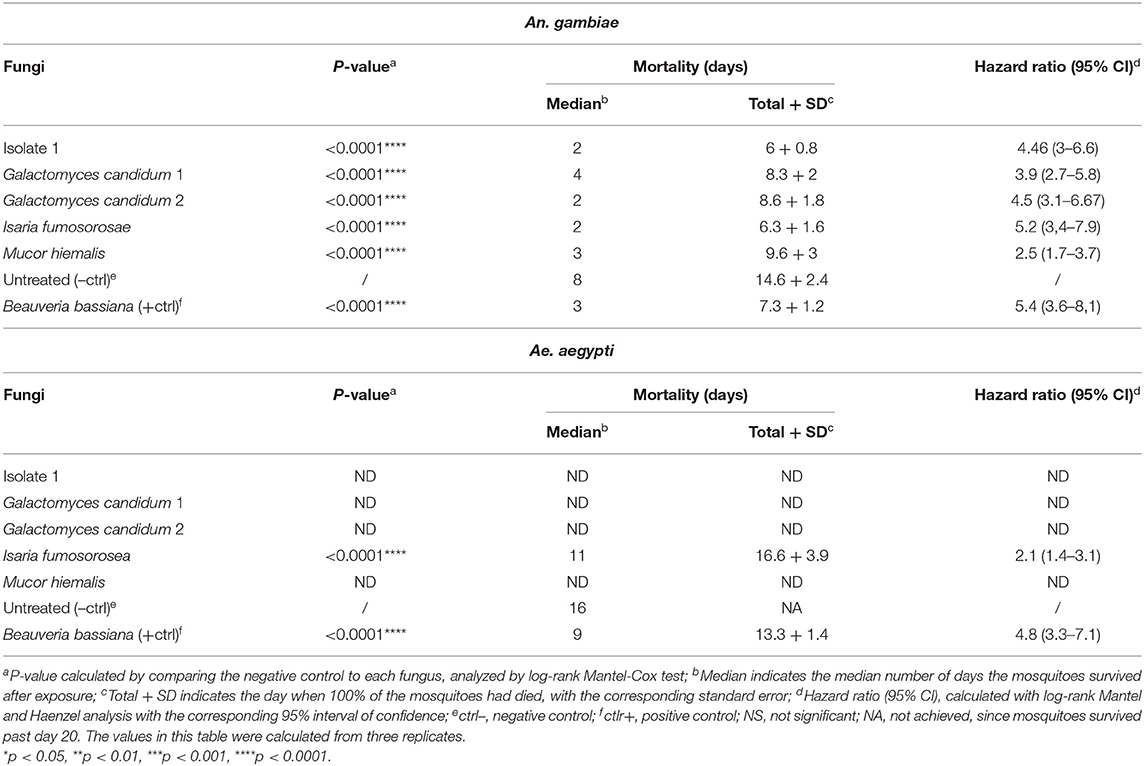
Table 3. Mortality of An. gambiae and Ae. aegypti mosquitoes exposed to spore solution in the dipping assay.
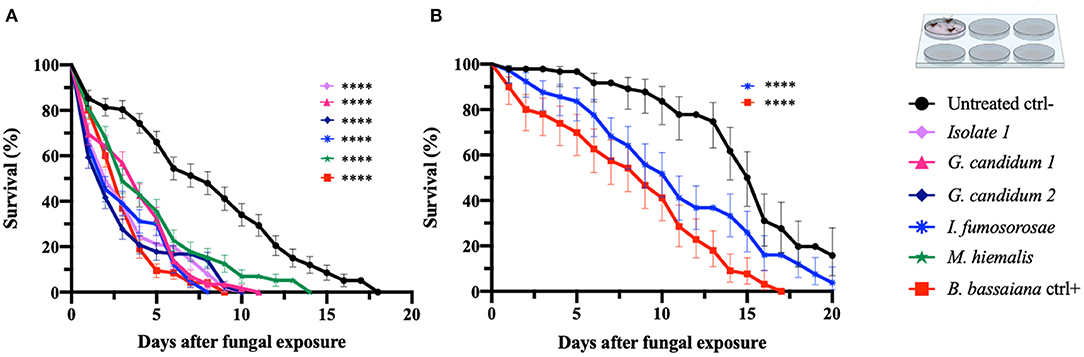
Figure 2. Spore-dipping assays of An. gambiae and Ae. aegypti mosquitoes. Mosquitoes were exposed via a dipping assay to a spore solution (108 spores/ml) for 1 min, and the mosquito survival was monitored for 20 days. Data are here presented for An. gambiae (A) and Ae. aegypti (B). The fungal species tested on each mosquito species were the ones identified as active in the respective direct-exposure experiment. The graphs represent the average and standard deviation of three biological replicates, N = 30 females per replicate. As a negative control, mosquitoes were exposed to a spore-free solution, and as a positive control they were exposed to a spore solution of B. bassiana. The statistical significance of the results for each fungus was compared to the negative control and analyzed by log rank-Mantel-Cox test; p-values are presented in Table 3. *p < 0.05, **p < 0.01, ***p < 0.001, ****p < 0.0001.
All Ae. aegypti exposed to I. fumosorosea had died by 16 days after exposure, yielding a hazard ratio of 2.1, whereas 57% of the non-spore-exposed control cohort had succumbed by day 20. All mosquitoes had succumbed after exposure to B. bassiana spores at 13 days post-treatment, yielding a hazard ratio of 4.8 (Table 3).
Mosquitocidal Activity of Fungus Culture Filtrates Against An. gambiae and Ae. aegypti Adults
To initiate an investigation into the nature of the entomopathogenic activity, we designed assays that would discriminate between killing mechanisms that are mediated by secreted fungus-produced metabolites and those requiring exposure to living fungi. Adult female An. gambiae and Ae. aegypti were fed on a sucrose solution containing a fungal liquid potato dextrose (PD) culture filtrate that presumably would contain the secreted factors of interest. We examined the five selected mosquitocidal fungi identified in the initial screening against adult An. gambiae females. As expected, both mosquito species showed an overall greater survival after ingestion of the fungal culture filtrates than after direct exposure to live fungi or spore solution. In fact, the hazard ratio of An. gambiae was between 0.8 and 2.4 and of Ae. aegypti mosquitoes <1, for the tested fungal isolates (Table 4, Figure 3).
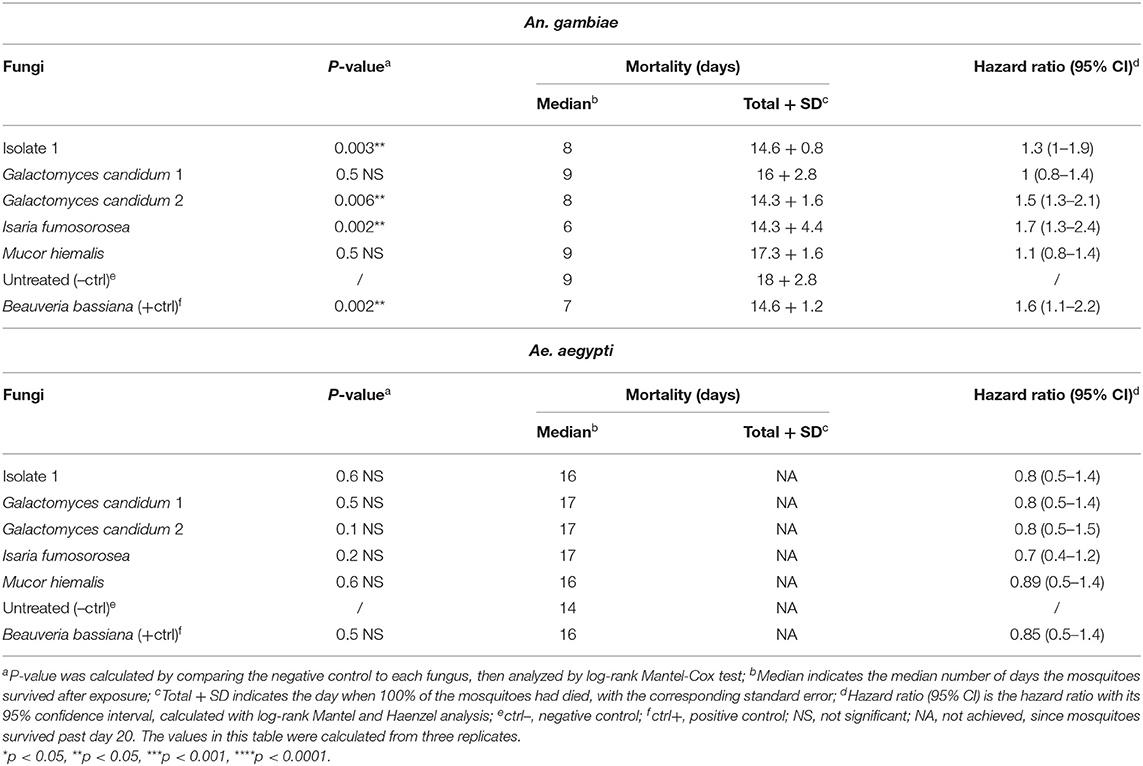
Table 4. Mortality of An. gambiae and Ae. aegypti mosquitoes exposed to fungal metabolites by feeding assay.
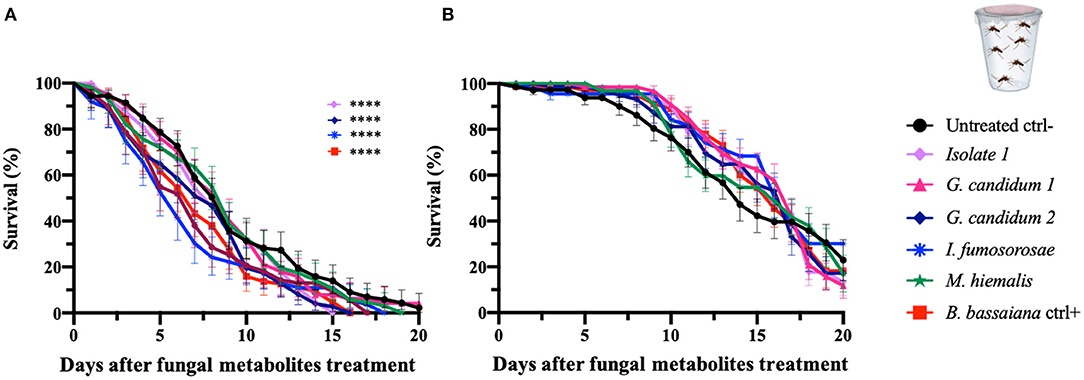
Figure 3. Fungal metabolite assays of An. gambiae and Ae. aegypti mosquitoes. Mosquitoes were allowed to feed on a sucrose solution containing fungal metabolites (free from spores and mycelium) for 48 h, and the mosquito survival was monitored for 20 days. Data here are presented for An. gambiae (A) and Ae. aegypti (B). The metabolites used in the experiments are derived from the fungi identified as active against An. gambiae in the initial screen (direct exposure experiment) and were collected from fungal liquid culture. The graphs represent the average and standard deviation of three biological replicates, N = 30 females per replicate. As a negative control, mosquitoes were fed on a metabolite-free solution, and as a positive control on metabolite solutions from B. bassiana. The statistical significance of the results for each fungus was compared to the negative control and analyzed by log-rank Mantel-Cox test; p-values are presented in Table 4.
An. gambiae mosquitoes that had ingested isolate 1 and G. candidum 2 culture filtrates showed a median of survival of 8 days and a significant reduction in the lifespan when compared to untreated mosquitoes (p = 0.003 and p = 0.006, respectively). An. gambiae mosquitoes that fed on the I. fumosorosea culture filtrate showed a median survival time of 6 days (p = 0.002), and those ingesting the B. bassiana culture filtrate showed a median survival of 7 days and a p-value of 0.002. Ingestion of G. candidum 1 or M. hiemalis culture filtrate did not have any effect on An. gambiae longevity when compared to the non-culture filtrate-fed control mosquitoes (Table 4, Figure 3A). All An. gambiae mosquitoes that were fed on isolate 1, I. fumosorosea or G. candidum 2 culture filtrates died within 14 days after ingestion, whereas those fed on G. candidum 1 or M. hiemalis culture filtrates, as well as the untreated mosquitoes, survived up to 16–18 days. The positive control mosquitoes that fed on a B. bassiana culture filtrate had all died at 7 days post-feeding (Table 4). As compared to the untreated control mosquitoes, none of the culture filtrates of the five selected fungi affected the survival of Ae. aegypti after ingestion, not even the I. fumosorosea isolate, which exerted potent killing activity against this mosquito species in the direct-exposure assay (Table 4, Figure 3B). Our results show that some of the fungi do produce and secrete factors that are toxic to the mosquitoes, but the most efficient killing requires exposure to live fungi and presumably involves an active infection process.
Discussion
The failure of disease control programs resulting from insecticide resistance among mosquito populations highlights the urgent need for new tools for mosquito control, including new insecticides. In the present study, we sampled diverse habitats to find entomopathogenic fungi that could satisfy the requirements for further development into mosquitocidals for use in vector control programs. We collected a total of 36 samples from various habitats and sources in Maryland and Puerto Rico that yielded 76 fungal isolates, 22 of which were successfully cultured under laboratory conditions. We were unsuccessful to maintain all the isolated fungi in the lab for several reasons; some fungi were simply not able to survive in the lab-condition of temperature and humidity, considering that they were isolated in Puerto Rico, some of them could have been in strict symbiosis with other fungi or source like flowers or other plants, some of them were perhaps not able to produce enough spores to propagate.
Analyses of their ribosomal 18S gene, through sequences blasting and through phylogenetic trees generation, revealed that the 22 isolates belonged to 12 different genera: Ascomycota phylum, Aureobasidium sp., Cercospora sp., Cladosporium sp., Colletotrichum sp., Fusarium sp., Galactomyces sp., Isaria sp., Mucor sp., Penicillium sp., Phomopsis sp., Pleosporales sp. and Scopulariopsis sp. Members belonging to the same genus as at least eight of our isolates have previously been shown to possess entomopathogenic properties against various types of insects, including various mosquito species (Ascomycota, Cladosporium sp., Diaporthe sp., Fusarium sp., Isaria sp., Mucor sp., Penicillium sp., and Scopulariopsis). These results further confirm the presence of entomopathogenic fungi in a wide variety of outdoor environments (Lacey and Fransen, 1996; Chandler et al., 1997; Sánchez-Peña et al., 2011). Within the Ascomycota phylum we find the most important entomopathogenic fungi (Beauveria spp. and Metarhizium spp.) that are used a biopesticides to control mosquitoes (Blanford et al., 2005; Scholte et al., 2005; Hancock, 2009; Hancock et al., 2009; Knols et al., 2010; Farenhorst et al., 2011). Several species of Cladosporium sp. (also Ascomycota phylum) have been found to exert pathogenic activity against insects; for example, lab trials showed that a C. velox fungus is able to kill Spodoptera litura larvae, one of the most important insect pests of agricultural crops in tropical regions of Asia (Singh et al., 2015), and extracts of C. cladosporioides have been shown to kill Aphis gossypii, which is a major pest of the cotton industry (Elbanhawy et al., 2019). Diaporthe sp. filtrates have been shown to have both larvicidal and adulticidal activity against Ae. aegypti mosquitoes (Meepagala et al., 2018), and exposure to its conidia has been shown to have pathogenic activity against the strawberry pest Duponchelia fovealist (Amatuzzi et al., 2018). The Fusarium sp. genus belongs to the Hypocreales order, as do Beaveria spp. and Metarhizium spp.; species within this genus have been shown to possess entomopathogenic activity against Tribolium sp., is an insect pest of stored grains (Chehri, 2017); a F. verticillioides isolate that is a grasshopper pathogen (Pelizza et al., 2011); and F. oxysporum extracts, which are lethal against larvae and pupae of multiple mosquito vectors such as An. stephensi, Ae. aegypti, and Culex (Cx) quinquefasciatus (Vivekanandhan et al., 2018a). Isaria sp. is a well-known entomopathogenic fungus, already commercialized for the control of different insect species in several countries. The only insecticidal activity of Mucor hiemalis has been shown to be correlated with its ability to secrete insecticidal metabolites against the adult stages of the agricultural pests Bactrocera oleae and Ceratitis capitata (Konstantopoulou et al., 2006). Penicillium citrum exerts lethal activity against larvae and adults of Cx. quinquefasciatus (Maketon et al., 2014). Finally, Scopulariopsis sp. has been found to be lethal to the agricultural pest Bemisia tabaci (Niu et al., 2019) (Table 1).
But among these eight already known as EPFs, we have here discovered three novel pathogenic fungi: isolate 1 that was previously unknown and un-characterized, two strains of Galactomyces candidum and Mucor hiemalis.
Our first screening for mosquitocidal activity was performed through surface exposure of female An. gambiae to plated 2-week-old fungal cultures that usually contained between 4 × 108 and 1 × 1010 spores/ml, depending on the fungal colony. This assay, that involves the shaking of mosquitoes on the fungal plate ensured an extensive surface exposure to not only spores, but also the fungal mycelia and hyphae and eventual toxic compounds produced by the fungi; however, the somewhat short lifespan of the control mosquitoes suggests that this procedure adversely affected An. gambiae viability, maybe due to the mechanical stress during the shaking on the agar plate. Nevertheless, we saw significant differences in survival between the non-exposed mosquitoes and several of the fungus-exposed cohorts, with a stringent statistical evaluation yielding a p-value of <0.0001. We identified five fungal isolates that significantly reduced the An. gambiae mosquitoes' lifespan and therefore were chosen for further investigation. These five were: isolate 1, Galactomyces candidum 1, Galactomyces candidum 2, Isaria fumosorosea, and Mucor hiemalis. I. fumosorosea also displayed a significant (p < 0.0001) killing activity against Ae. aegypti with the same direct exposure assay.
The isolate 1 fungus that displayed adulticidal activity against An. gambiae appears to be a novel mosquitocidal fungus that has not been previously investigated for pathogenic effects on insects, plants, or humans. It did display relatedness to Aaosphaeria sp. that has not been addressed with regards to these properties. G. candidum is a Saccharomycotina, a yeast-like fungus whose potential pathogenic activities have been investigated in previous studies, and recent laboratory-based experiments have shown killing activity against Botrytis cinera, a gray mold causing plant disease (Chen et al., 2018). Studies performed by Chen et al. have suggested that Galactomyces sp. is able to produce volatile compounds and chitinase enzymes that inhibit fungal growth. Galactomyces sp. is also used in dairy industry (Perkins et al., 2002). I. fumosorosea belongs to the Hypocreales order, which includes the well-studied entomopathogenic fungi Beauveria spp. and Metarhizium spp. Isaria sp. has been used as an environmentally friendly pest biocontrol agent in many countries, being registered as a biopesticide in the USA (United States Environmental Protection Agency (EPA)., 2020), in the European Union (European Commission (EU), 2020), and in Brazil (Ministerio de Agricultura, 2020). In China, although this fungus has not been registered as a myco-pesticide, it is widely used to control whiteflies and aphids (Zhang et al., 2016; Xu et al., 2017). Several studies have identified the genus Isaria sp. as a source of promising entomopathogenic candidates against adult Ae. aegypti and An. stephensi as well as Ae. aegypti eggs (Luz et al., 2007; Leles et al., 2010; Blanford et al., 2012; Ramirez et al., 2018). Furthermore, Isaria sp. mycelia extracts have been used for the development of silver nanoparticles that are able to kill Ae. aegypti and Cx. quinquefasciatus larvae (Banu and Balasubramanian, 2014). M. hiemalis is a saprophytic species belonging to the Zygomycetes, Mucorales. It is frequently found to infect injured insects (Heitor, 1962), but as far as we know it has not been previously studied with regard to mosquitocidal activity.
We also used a spore-based dipping assay that relied on exposure of mosquitoes to a liquid spore-containing solution to further study the fungi that had shown confirmed entomopathogenic activity in the direct-exposure assay. We reasoned that this method would enable us to expose the mosquitoes to the same concentration of spores in each replicate and that with 1 min of spore exposure we could assess the fungal infection in the mosquitoes, if the fungus was pathogenic. The actual procedure of dipping An. gambiae mosquitoes into the spore-free PBS solution seemed to affect mosquito viability, since the control cohort displayed a lifespan of only 14 days. A possible contributing factor to this decreased viability could have been the presence of detergent (Tween-80) that to some degree could permeabilize the external wax layer of the cuticle (Vincent, 2002). However, the generally more robust Ae. aegypti mosquitoes were not affected by this treatment. We found that An. gambiae exposed to I. fumosorosea as well as M. hiemalis and Ae. aegypti exposed to I. fumosorosea through direct exposure, when we assume a more extensive contact with spores occurred, displayed a shorter lifespan than did the mosquitoes exposed to the same fungi in the 108 spores/ml dipping assay. This likely spore dosage-dependent effect was also observed with B. bassiana fungus, for which an increased dose of spores was associated with a greater mortality in Ae. aegypti mosquitoes (Dong et al., 2012). These results confirm a previous study showing a dosage-dependent entomopathogenic activity of I. fumosorosea against B. tabaci nymphs (Gao et al., 2017). We also demonstrate here a spore dosage-dependence with regard to M. hiemalis. The killing activity caused by the fungal spores did not seem to be exposure method-dependent for all our fungal isolates. For example, exposure to isolate 1 resulted in a 100% mortality of An. gambiae by 6 days after dipping in the spore solution, whereas all the mosquitoes of this species treated with the direct-exposure method survived longer and died within 13 days after exposure. This observation highlights the importance of adapting the delivery, or exposure, method to the physiological and morphological characteristics of a particular fungus. However, An. gambiae subjected to G. candidum 1 in the two different assays displayed an identical median and total mortality time-course, demonstrating a fungal killing mechanism that is not dependent on the mode of exposure. G. candidum 2, in contrast, showed higher mortality in the spore-dipping assay than in direct-exposure assay, and this result could potentially be explained by the presence of toxic compounds that, in the spore solution, were able to more efficiently come in contact with the mosquitoes. Mosquitoes, this result was also corroborate with the fungus-culture-filtrate experiment result. The ability of G. candidum to produce pathogenic compounds had already been shown in the work of Chen and co-workers, in which they demonstrated the production of volatile toxic compounds and a chitinase by Galactomyces that can affect the longevity of adult mosquitoes (Chen et al., 2018).
To investigate whether the mechanism of fungus-mediated killing required interaction with live fungi or was caused by a secreted fungus factor, we exposed An. gambiae and Ae. aegypti mosquitoes to a fungus-culture filtrate through a sugar meal. Our results indicated that isolate 1, G. candidum 2, and I. fumosorosea produce mosquitocidal compound(s) against An, gambiae. While isolate 1 has not been previously studied with regard to mosquitocidal activity, G. candidum and I. fumosorosea have previously been shown to produce entomopathogenic factors (Chen et al., 2018). Interestingly, only one of our two Galactomyces sp. isolates had this property, thereby demonstrating that different isolates of the same fungal species can produce different metabolites and thus display different entomopathogenic activities. I. fumosorosea has previously been shown to produce metabolites with antibacterial, insecticidal, antiviral, and cytotoxic activity (Weng et al., 2019). Ingestion of the fungal-culture filtrates, including that of B. bassiana, which is known to produce entomopathogenic metabolites (Bukhari et al., 2011; Mnyone et al., 2011; Vivekanandhan et al., 2018b), had no effect on Ae. aegypti. This result likely reflects the greater robustness of this mosquito species and also raises the possibility that some of the fungi-produced toxic factors were in too low concentration to exert an effect. It is also possible that a different route of exposure (i.e., surface exposure) could have yielded a different result with some of the tested fungal culture filtrates.
In summary, in this study we have isolated and characterized five potentially entomopathogenic fungi with activity against two major mosquito disease vectors. Among these fungi, four have never been tested on insect pests before: isolate 1, two strains of Galactomyces candidum and Mucor hiemalis. We further show and corroborate the particular potential of Isaria fumosorosea as a potential biopesticide candidate against various mosquito species.
These fungi merit further investigation as the source of novel biological agents for mosquito control.
Materials and Methods
Ethics Statement
This study was carried out in strict accordance with the recommendations in the Guide for the Care and Use of Laboratory Animals of the National Institutes of Health. The protocol was approved by the Animal Care and Use Committee of the Johns Hopkins University.
Sample Collection
Organic material with potential fungus growth was collected from different outdoor environments in Maryland and in Puerto Rico. Each sample was collected with sterile forceps, placed in a 1.5-ml tube with 200 μl of sterile 1X PBS and kept at 4°C until further use.
Culture and Isolation of Fungi
Each sample was manually homogenized with a pestle and plated on brain heart infusion (BHI) agar containing 50 μg/ml of chloramphenicol and left at room temperature until fungal growth was observed. From each plate, every different fungus that grew was single-subcultured until the fungal culture was axenic. All the fungi were maintained on BHI except for Aaosphaeria arxii, which was maintained on Sabouraud dextrose agar medium. Beauveria bassiana strain 80.2, kindly provided by Dr. Silverman, was used as a positive control and was maintained under the same conditions as the other fungi.
Identification of Isolated Fungi
DNA from 0.5 cm of fungal culture was extracted with a DNeasy Blood and Tissue Kit (Qiagen) according to the manufacturer's instructions. The fungal biomass was homogenized for 2 min in 200 μl of lysis buffer using 0.5-mm sterile glass beads. Two pairs of degenerate fungal primers were used to amplify the ITS ribosomal 18S region: ITS1 forward and ITS3 forward (ITS1 5′-CTHGGTCATTTAGAGGAASTAA, ITS3 5′-FAHCGATGAAGAACRYAG), and ITS4 reverse (ITS4 5′-RTCCTCCGCTTWTTGWTWTGC) (Toju et al., 2012). One primer pair was used to amplify the 18S small subunit-SSU (NS1-F 5′-GTAGTC ATATGCTTGTCTC, NS4-R 5′- CTT CCGTCAATTCCTTTAAG) and one pair for the 28S large sub unit LSU (LROR-F 5′-ACCCGCTGAACTTAAGC, LR6-R 5′-CGCCAGTTCTGCTTACC) (Raja et al., 2017).
The target amplicon was column-purified using a ZYMO kit and sent to Quintara Biosciences for Sanger sequencing, and the returned sequence was blasted in the NCBI database against fungus taxa for species identification. The ITS-18S sequences were also used to generate phylogenetic trees using the Bootstrap and Neighbor-joining statistical method with the p-distance model through the MEGAX software.
A morphological analysis of spores from a selection of fungal isolates was performed. Spores were obtained from 2 week old fungal cultures. PBS+ 0,02% tween 80 was added to the agar plate and the fungi was scraped loose, filtered through glass wool and transferred to Eppendorf tubes. Samples were centrifuged at 5,000 rpm for 5 min to remove any remaining debris. The supernatant was analyzed under bright field microscope and photos of spores were acquired at 40x magnification using a Leica DM2500 microscope and a DFC310 FX Digital Color Camera (Leica Microsystems).
Mosquito Rearing
Rockefeller strain Ae. aegypti and Keele strain An. gambiae were maintained in an insectary chamber at 27°C and 80% humidity on a 12-h light/dark cycle according to standard rearing procedures. The larvae were fed on sterile pulverized fish food, and adult mosquitoes were provided with a 10% sucrose solution. The mosquitoes for the experiments were kept in cups inside a large incubator provided with the same environmental conditions as in the insectary chamber described above.
Fungal Direct Exposure Assays
To assess the first screening experiments, 30 not blood-fed females, each 3–5 days old, were tested against fungi. Mosquitoes were cold-anesthetized, placed directly into an agar plate containing a 2-week-old fungal culture, and shaken for 30 s. Here, the mosquitoes are exposed to a complex content of fungal spores, fungal mycelia, the growing hyphae, and possible toxic compounds. Thereafter, the mosquitoes were placed in cups with 10% sucrose solution; to avoid bacterial and fungal growth, the sucrose paper was changed three times per week. A plate with B. bassiana was used as a positive control, and sterile BHI agar medium was used as a negative control.
All the direct exposure experiments were done once, and mosquito survival was checked daily for 20 days. For logistic reasons, the 22 fungal isolates against An. gambiae were tested at three different times, always including the positive and negative controls, and p-values were calculated against the respective negative controls.
Fungal Dipping-Based Exposure Assays
To confirm the mosquitocidal potential of the five pathogenic fungi, a fungal spore exposure assay was performed on 30 female An. gambiae and 30 female Ae. aegypti mosquitoes, only against those fungi for which a p-value of <0.0001 was obtained from direct exposure. The fungal spore exposure was done using purified spores and the dipping procedure (Dong et al., 2012), with the mosquitoes exposed to a solution of 108 spores/ml. The spores were collected from 2-week-old fungal plate cultures, counted with a Neubauer hemocytometer, and adjusted to 108 spores/ml. In brief, mosquitoes were placed, back first, into 2 ml of the spore solution for 1 min. Only the back of the mosquito came in contact with the spore solution. B. bassiana spore solution was used as a positive control, and 1X PBS with 0.02% tween-80, used to collect spores, was used as a negative control. Mosquito sugar papers were changed three times per week. The dipping experiments were done in triplicate, and mosquito survival was checked daily for 20 days.
Fungus Culture Filtrate-Feeding Assays
To discriminate the fungal killing activity as a result of fungal proliferation on mosquito bodies from the potential presence of toxic compounds, 30 females of An. gambiae and Ae. aegypti mosquitoes were exposed to fungal metabolites through a feeding assay. Fungal metabolites were collected from a 2-week-old fungal liquid culture (potato dextrose medium) (Vandermolen et al., 2013) by filtration through a 0.2-μm filter, then added to a 10% sucrose solution to give a final concentration of 5% sucrose. Metabolites produced by B. bassiana were used as a positive control, and filtered potato dextrose broth with sucrose was used as a negative control. Mosquitoes were fed on the metabolite/sucrose solution for 48 h, and then the solution was replaced with 10% sucrose. The sucrose papers were changed three times per week as for all other experiments. To confirm that the mosquitoes had died as a consequence of the activity of the metabolites and not because of fungal growth, dead mosquitoes were checked each week under an optical microscope to confirm the absence of fungal proliferation on the mosquito bodies. These assays were run in triplicate, and the mosquito survival rate was checked daily for 20 days.
Statistical Analysis
Mortality was expressed as a median, and the final day of mosquito survival and the hazard ratio were calculated by Manten and Haenzel analysis. The statistical significance of survival curves was set to the conventional α <0.05 level, calculated with a long-rank Mantel-Cox analysis and using Graphpad Prism software, version 8.
Data Availability Statement
The original contributions presented in the study are included in the article/Supplementary Material, further inquiries can be directed to the corresponding author.
Ethics Statement
The animal study was reviewed and approved by Animal Care and Use Committee of the Johns Hopkins University.
Author Contributions
AA and CE performed the experiments. All authors conceived experiments, analyzed the data obtained, and wrote the manuscript.
Funding
This work has been supported by National Institutes of Health, National Institute of Allergy and Infectious Diseases grant R21AI136456 as well as the Swedish Research Council grant nr 2018-00334. We also thank the Bloomberg Philanthropies.
Conflict of Interest
The authors declare that the research was conducted in the absence of any commercial or financial relationships that could be construed as a potential conflict of interest.
Publisher's Note
All claims expressed in this article are solely those of the authors and do not necessarily represent those of their affiliated organizations, or those of the publisher, the editors and the reviewers. Any product that may be evaluated in this article, or claim that may be made by its manufacturer, is not guaranteed or endorsed by the publisher.
Acknowledgments
We would like to thank the Johns Hopkins Malaria Research Institute Insectary as well as Dr. Deborah McClellan for editing the manuscript.
Supplementary Material
The Supplementary Material for this article can be found online at: https://www.frontiersin.org/articles/10.3389/ffunb.2021.637234/full#supplementary-material
References
Alkhaibari, A. M., Carolino, A. T., Bull, J. C., Samuels, R. I., and Butt, T. M. (2017). Differential pathogenicity of metarhizium blastospores and conidia against larvae of three mosquito species. J. Med. Entomol. 54, 696–704. doi: 10.1093/jme/tjw223
Amatuzzi, R. F., Cardoso, N., Poltronieri, A. S., Poitevin, C. G., Dalzoto, P., Zawadeneak, M. A., et al. (2018). Potential of endophytic fungi as biocontrol agents of Duponchelia fovealis (Zeller) (Lepidoptera:Crambidae). Braz. J. Biol. 78, 429–435. doi: 10.1590/1519-6984.166681
Banu, A. N., and Balasubramanian, C. (2014). Optimization and synthesis of Isaria delivered by silver nanoparticles resulted lethal for Ae aegypti and Cx quinquefasciatus larvae. Parasitol. Res. 113, 3843–3851. doi: 10.1007/s00436-014-4052-0
Beckage, N. E., Marin, K. M., Walton, W. E., Wirth, M. C., and Tan, F. F. (2004). Comparative larvicidal toxicities of three ecdysone agonists on the mosquitoes Aedes aegypti, Culex quinquefasciatus, and Anopheles gambiae. Arch. Insect Biochem. Physiol. 57, 111–122. doi: 10.1002/arch.20021
Blanford, S., Chan, B. H., Jenkins, N., Sim, D., Turner, R. J., Read, A. F., et al. (2005). Fungal pathogen reduces potential for malaria transmission. Science 308, 1638–1641. doi: 10.1126/science.1108423
Blanford, S., Jenkins, N. E., and Read, A. F. (2012). Evaluating the lethal and pre-lethal effects of a range of fungi against adult Anopheles stephensi mosquitoes. Malar. J. 11:365. doi: 10.1186/1475-2875-11-365
Blanford, S., Shi, W., Christian, R., Marden, J. H., Koekemoer, L. L., Brooke, B. D., et al. (2011). Lethal and pre-lethal effects of a fungal biopesticide contribute to substantial and rapid control of malaria vectors. PLoS ONE 6:e23591. doi: 10.1371/journal.pone.0023591
Bukhari, T., Takken, W., and Koenraadt, C. J. M. (2011). Development of Metarhizium anisopliae and Beauveria bassiana formulations for control of malaria mosquito larvae. Parasit. Vectors 4:23. doi: 10.1186/1756-3305-4-23
Butt, T. M., Coates, C. J., Dubovskiyx, I. M., and Ratcliffe, N. A. (2016). Entomopathogenic fungi: new insights into host pathogen interactions. Adv. Genet. 94, 307–364. doi: 10.1016/bs.adgen.2016.01.006
Chandler, D., Hay, D., and Reid, A. P. (1997). Sampling and occurrence of entomopathogenic fungi and nematodes in UK soils. Appl. Soil Ecol. 5, 133–141. doi: 10.1016/S0929-1393(96)00144-8
Charnley, A. K., and Collins, S. A. (2007). “Entomopathogenic fungi and their role in pest control,” in The Mycota IV: Environmental and Microbial Relationships, 2nd Edn, eds C. P. Kubicek and I. S. Druzhinina (Cham: Springer), 159–187.
Chehri, K. (2017). Molecular identification of entomopathogenic Fusarium species associated with Tribolium species in stored grains. J. Invertebr. Pathol. 144, 1–6. doi: 10.1016/j.jip.2017.01.003
Chen, P. H., Chen, R. Y., and Chou, J. Y. (2018). Screening and evaluation of yeast antagonists for biological control of Botrytis cinerea on strawberry fruits. Mycobiology 46, 33–46. doi: 10.1080/12298093.2018.1454013
de Faria, M. R., and Wraight, S. P. (2007). Mycoinsecticides and Mycoacaricides: a comprehensive list with worldwide coverage and international classification of formulation types. Biol. Control 43, 237–256. doi: 10.1016/j.biocontrol.2007.08.001
Dong, Y., Morton, J. C., Ramirez, J. L., and Souza-Neto, J. A. (2012). The entomopathogenic fungus Beauveria bassiana activate toll and JAK-STAT pathway-controlled effector genes and anti-dengue activity in Aedes aegypti. Insect Biochem. Mol. Biol. 42, 126–132. doi: 10.1016/j.ibmb.2011.11.005
Dubovskiy, M., Whitten, M. M. A., Yaroslavtseva, O. N., Greig, C., Kryukov, V. Y., Grizanova, E. V., et al. (2013). Can insects develop resistance to insect pathogenic fungi? PLoS ONE 8:e60248. doi: 10.1371/journal.pone.0060248
Elbanhawy, A. A., Elsherbiny, E. A., El-Mageed, A. E. A., and Abdel-Fattah, G. M. (2019). Potential of fungal metabolites as a biocontrol agent against cotton aphid, Aphis gossypii Glover and the possible mechanisms of action of fungal metabolites as a biocontrol agent against cotton aphid. Pestic. Biochem. Physiol. 159, 34–40. doi: 10.1016/j.pestbp.2019.05.013
Eliskases-Lechner, F., Guéguen, M., and Panoff, J. M. (2011). “Yeasts and molds, Geotrichum candidum,” in Encyclopedia of Dairy Sciences, 2nd Edn, ed J. W. Fuquay (Amsterdam: Academic Press), 765–771.
European Commission (EU) (2020). Pesticides. Available online at: http://ec.europa.eu/food/plant/pesticides/eu-pesticides-database/ (accessed October 13, 2020).
Farenhorst, M., Hilhorst, A., Thomas, M. B., and Knols, B. G. J. (2011). Development of fungal applications on netting substrates for malaria vector control. J. Med. Entomol. 48, 305–313. doi: 10.1603/ME10134
Farenhorst, M., Knols, B. G. J., Thomas, M. B., Howard, A. F. V., Takken, W., Rowland, M., et al. (2010). Synergy in efficacy of fungal entomopathogens and permethrin against West African insecticide-resistant Anopheles gambiae mosquitoes. PLoS ONE 5:e12081. doi: 10.1371/journal.pone.0012081
Farenhorst, M., Mouatcho, J. C., Kikankie, C. K., Brooke, B. D., Hunt, R. H., Thomas, M. B., et al. (2009). Fungal infection counters insecticide resistance in African malaria mosquitoes. Proc. Natl. Acad. Sci. U. S. A. 106, 17443–17447. doi: 10.1073/pnas.0908530106
Ffrench-Constant, R. H. (2005). Something old, something transgenic, or something fungal for mosquito control? Trends Ecol. Evol. 20, 577–579. doi: 10.1016/j.tree.2005.08.007
Gao, T., Wang, Z., Huang, Y., Keyhani, N. O., and Huang, Z. (2017). Lack of resistance development in Bemisia tabaci to Isaria fumosorosea after multiple generations of selection. Sci. Rep. 7:42727. doi: 10.1038/srep42727
Gräfenhan, T., Schroers, H. J., Nirenberg, H. I., and Seifert, K. A. (2011). An overview of the taxonomy, phylogeny, and typification of nectriaceous fungi in Cosmospora, Acremonium, Fusarium, Stilbella, and Volutella. Stud. Mycol. 68, 79–113. doi: 10.3114/sim.2011.68.04
Hancock, P. A. (2009). Combining fungal biopesticides and insecticide-treated bednets to enhance malaria control. PLoS Comput. Biol. 5:e1000525. doi: 10.1371/journal.pcbi.1000525
Hancock, P. A., Thomas, M. B., and Godfray, H. C. J. (2009). An age-structured model to evaluate the potential of novel malaria-control interventions: a case study of fungal biopesticide sprays. Proc. Biol. Sci. 276, 71–80. doi: 10.1098/rspb.2008.0689
Heitor, F. (1962). Parasitisme de blessure par le champignon Mucor hiemalis Wehmer chez les insectes. Ann. Epiphyt. 13, 179–203.
Knols, B. G., Bukhari, T., and Farenhorst, M. (2010). Entomopathogenic fungi as the next-generation control agents against malaria mosquitoes. Future Microbiol. 5, 339–341. doi: 10.2217/fmb.10.11
Konstantopoulou, M. A., Milonas, P., and Mazomenos, B. E. (2006). Partial purification and insecticidal activity of toxic metabolites secreted by a Mucor hiemalis Strain (SMU-21) against adults of Bactrocera oleae and Ceratitis capitata (Diptera: Tephritidae). J. Econ. Entomol. 99,1657–1664. doi: 10.1093/jee/99.5.1657
Lacey, L. A., Fransen, J. J, and Carruthers, R. (1996). “Global distribution of naturally occurring fungi of Bemisia, their biologies and use as biological control agents,” in Bemisia, Taxonomy, Biology, Damage, Control and Management, eds D. Gerling and R. T. Mayer (Andover: Intercept), 401–433.
Leles, R. N., Sousa, N. A., Rocha, L. F. N., Santos, A. H., Silva, H. H. G., and Luz, C. (2010). Pathogenicity of some hypocrealean fungi to adult Aedes aegypti (Diptera: Culicidae). Parasitol. Res. 107, 1271–1274. doi: 10.1007/s00436-010-1991-y
Luz, C., Tai, M. H. H., Santos, A. H., Rocha, L. F. N., Albernaz, D. A. S., and Silva, H. H. G. (2007). Ovicidal activity of entomopathogenic hyphomycetes on Aedes aegypti (Diptera: Culicidae) under laboratory conditions. J. Med. Entomol. 44, 799–804. doi: 10.1093/jmedent/44.5.799
Maketon, M., Amnuaykanjanasin, A., and Kaysorngup, A. (2014). A rapid knockdown effect of Penicillium citrinum for control of the mosquito Culex quinquefasciatus in Thailand. World J. Microbiol. Biotechnol. 30, 727–736. doi: 10.1007/s11274-013-1500-4
Meepagala, K. M., Estep, A. S., Clausen, B. M., and Becnel, J. J. (2018). Mosquitocidal activity of a naturally occurring isochroman and synthetic analogs from the plant pathogenic fungus, Diaporthe eres against Aedes aegypti (Diptera: Culicidae). J. Med. Entomol. 55, 969–974. doi: 10.1093/jme/tjy016
Ministerio de Agricultura, Agrofit Consulta Aberta. (2020). Ingredientes ativos. Available online at: http://agrofit.agricultura.gov.br/agrofit_cons/ (accessed October 13, 2020).
Mnyone, L. L., Kirby, M. J., Mpingwa, M. W., Lwetoilera, D. W., Knols, B. G. J., Takken, W., et al. (2011). Infection of Anopheles gambiae mosquitoes with entomopathogenic fungi: effect of host age and blood feeding stage. Parasitol. Res. 108, 317–322. doi: 10.1007/s00436-010-2064-y
Moyes, C. L., Vontas, J., Martins, A. J., Ching, N.g L., Koou, S. Y., Dusfour, I., et al. (2017). Contemporary status of insecticide resistance in the major Aedes vectors of arboviruses infecting humans. PLoS Negl. Trop. Dis. 11:e0005625. doi: 10.1371/journal.pntd.0005625
Niu, G., Wang, B., Zhang, G., King, J. B., Cichewicz, T. R. H., and Li, J. (2015). Targeting mosquito FREP1 with a fungal metabolite blocks malaria transmission. Sci. Rep. 5:14694. doi: 10.1038/srep14694
Niu, X., Xie, W., Zhang, J., and Hu, Q. (2019). Biodiversity of entomopathogenic fungi in the soils of South China. Microorganisms 7:311. doi: 10.3390/microorganisms7090311
Pelizza, S. A., Stenglein, S. A., Cabello, M. N., Dinolfo, M. I., and Lange, C. E. (2011). First record of Fusarium verticillioides as an entomopathogenic fungus of grasshoppers. J. Insect Sci. 11:70. doi: 10.1673/031.011.7001
Perkins, V., Vignola, S., Lessard, M. H., Plante, P. L., Corbeil, J., Dugat-Bony, E., et al. (2002). Phenotypic and genetic characterization of the cheese ripening yeast Geotrichum candidum. Front. Microbiol. 11:737. doi: 10.3389/fmicb.2020.00737
Raja, H. A., Miller, A. N., Pearce, C. J., and Oberlies, N. H. (2017). Fungal identification using molecular tools: a primer for the natural products research community. J. Nat. Prod. 80, 756–770. doi: 10.1021/acs.jnatprod.6b01085
Ramirez, J. L., Muturi, E. J., Dunlap, C., and Rooney, A. P. (2018). Strain-specific pathogenicity and subversion of phenoloxidase activity in the mosquito Aedes aegypti by members of the fungal entomopathogenic genus Isaria. Sci. Rep. 8:9896. doi: 10.1038/s41598-018-28210-6
Read, A. F., Lynch, P. A., and Thomas, M. B. (2009). How to make evolution- proof insecticides for malaria malaria control. PLoS Biol. 7:e1000058. doi: 10.1371/journal.pbio.1000058
Riveron, J. M., Tchouaku, M., Mugenzi, L., Menze, B. D., Chiang, M. C., and Wondji, C. S. (2018). “Insecticide resistance in malaria vectors: an update at a global scale,” in Towards Malaria Elimination: A Leap Forward, eds S. Manguin and V. Dev (London: IntechOpen Limited), 150–175. doi: 10.5772/intechopen.78375
Sánchez-Peña, S. R., Lara, J. S. J., and Medina, R. F. (2011). Occurrence of entomopathogenic fungi from agricultural and natural ecosystems in Saltillo, Mexico, and their virulence towards thrips and whiteflies. J. Insect Sci. 11:1. doi: 10.1673/031.011.0101
Scholte, E., Ng'habi, K., Kihonda, J., Takken, W., Paaijmans, K., Abdulla, S., et al. (2005). An Entomopathogenic fungus for control of adult african malaria mosquitoes. Science 308, 1641–1642. doi: 10.1126/science.1108639
Singh, B., Kaur, T., Kaur, S., Manhas, R. K., and Kaur, A. (2015). Insecticidal potential of an endophytic Cladosporium velox against Spodoptera litura mediated through inhibition of alpha glycosidases. Pestic. Biochem. Physiol. 131, 46–52. doi: 10.1016/j.pestbp.2016.01.004
Singh, G., and Prakash, S. (2012). Lethal effects of Aspergillus niger against mosquitoes vector of filaria, malaria, and dengue: a liquid mycoadulticide. Sci. World J. 2012:603984. doi: 10.1100/2012/603984
Sun, T., Shen, Z., Shaukat, M., Du, C., and Ali, S. (2020). Endophytic isolates of Cordyceps fumosorosea to enhance the growth of solanum melongena and reduce the survival of whitefly (Bemisia tabaci). Insects 11:78. doi: 10.3390/insects11020078
Toju, H., Tanabe, A. S., Yamamoto, S., and Sato, H. (2012). High-coverage ITS primers for the DNA-based identification of ascomycetes and basidiomycetes in environmental samples. PLoS ONE 7:e40863. doi: 10.1371/journal.pone.0040863
Udayanga, D., Liu, X., McKenzie, E. H. C., Chukeatirote, E., Bahkali, A. H. A., and Hyde, K. D. (2011). The genus Phomopsis: biology, applications, species concepts and names of common phytopathogens. Fungal Divers. 50:189. doi: 10.1007/s13225-011-0126-9
United States Environmental Protection Agency (EPA). (2020). Eu Pesticides Database. Available online at: https://www.epa.gov/pesticides/ (accessed October 13, 2020).
Vandermolen, K. M., Raja, H. A., El-Elimat, T., and Oberlies, N. H. (2013). Evaluation of culture media for the production of secondary metabolites in a natural products screening program. AMB Express. 3:71. doi: 10.1186/2191-0855-3-71
Vincent, J. F. V. (2002). Arthropod cuticle: a natural composite shell system. Appl. Sci. Manuf. 33, 1311–1315. doi: 10.1016/S1359-835X(02)00167-7
Vivekanandhan, P., Bedinib, S., and Shivakumar, M. S. (2020b). Isolation and identification of entomopathogenic fungus from Eastern Ghats T of South Indian forest soil and their efficacy as biopesticide for mosquito control. Parasitol. Int. 76:102099. doi: 10.1016/j.parint.2020.102099
Vivekanandhan, P., Karthi, S., Shivakumar, M. S., and Benelli, G. (2018a). Synergistic effect of entomopathogenic fungus Fusarium oxysporum extract in combination with temephos against three major mosquito vectors. Pathog. Glob. Health 112, 37–46. doi: 10.1080/20477724.2018.1438228
Vivekanandhan, P., Kavitha, T., Karthi, S., Senthil-Nathan, S., and Shivakumar, M. (2018b). Toxicity of Beauveria bassiana-28 mycelial extracts on larvae of Culex quinquefasciatus mosquito (Diptera: Culicidae). Int. J. Environ. Res. Public Health 15:440. doi: 10.3390/ijerph15030440
Vivekanandhan, P., Swathy, K., Kalaimurugan, D., Ramachandran, M., and Yuvaraj, A. (2020a). Larvicidal toxicity of Metarhizium anisopliae metabolites against three mosquito species and non-targeting organisms. PLoS ONE 15:e0232172. doi: 10.1371/journal.pone.0232172
Weng, Q., Zhang, X., Chen, W., and Hu, Q. (2019). Secondary metabolites and the risks of Isaria fumosorosea and Isaria farinose. Molecules 24:664. doi: 10.3390/molecules24040664
World Health Organization (WHO). (2020). Vector-borne diseases. Available online at: https://www.who.int/news-room/fact-sheets/detail/vector-borne-diseases (accessed October 1, 2020).
Xu, J., Xu, X., Shakeel, M., Li, S., Wang, S., Zhou, X., et al. (2017). The entomopathogenic fungi Isaria fumosorosea plays a vital role in suppressing the immune system of Plutella xylostella: Rna-seq and dge analysis of immunity-related genes. Front. Microbiol. 8:1421. doi: 10.3389/fmicb.2017.01421
Keywords: Anopheles gambiae, Aedes aegypti, entomopathogenic fungi, biopesticides, mosquito control, vector-borne diseases
Citation: Accoti A, Engdahl CS and Dimopoulos G (2021) Discovery of Novel Entomopathogenic Fungi for Mosquito-Borne Disease Control. Front. Fungal Biol. 2:637234. doi: 10.3389/ffunb.2021.637234
Received: 03 December 2020; Accepted: 28 June 2021;
Published: 27 July 2021.
Edited by:
Almudena Ortiz-Urquiza, Swansea University, United KingdomReviewed by:
Ivan Dubovskiy, Novosibirsk State Agrarian University, RussiaSibao Wang, Shanghai Institutes for Biological Sciences (CAS), China
Copyright © 2021 Accoti, Engdahl and Dimopoulos. This is an open-access article distributed under the terms of the Creative Commons Attribution License (CC BY). The use, distribution or reproduction in other forums is permitted, provided the original author(s) and the copyright owner(s) are credited and that the original publication in this journal is cited, in accordance with accepted academic practice. No use, distribution or reproduction is permitted which does not comply with these terms.
*Correspondence: George Dimopoulos, gdimopo1@jhu.edu