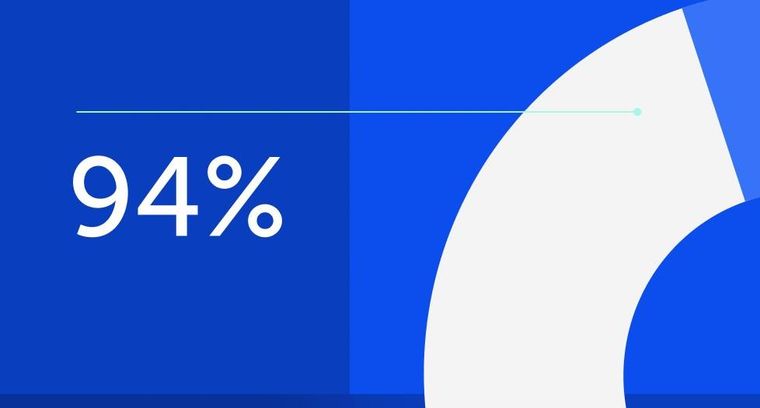
94% of researchers rate our articles as excellent or good
Learn more about the work of our research integrity team to safeguard the quality of each article we publish.
Find out more
ORIGINAL RESEARCH article
Front. For. Glob. Change, 15 April 2025
Sec. People and Forests
Volume 8 - 2025 | https://doi.org/10.3389/ffgc.2025.1513140
Natural Climate Solutions (NCS) aim to enhance carbon sequestration by restoring and managing various ecosystems while ensuring environmental and socio-economic stability. They also focus on identifying and implementing management actions with the highest mitigation potential. In the Ecuadorian Amazon, Indigenous communities have traditionally practiced a unique form of agriculture that integrates remnant trees within cultivated areas, known as Chakras or Ajas. These areas provide essential resources such as food, medicine, and other products. Despite the persistence of these practices, there has been a shift toward more market-oriented systems. The mitigation capacity of Chakras and Ajas is under-researched, leaving a gap in understanding their characteristics and potential to mitigate climate change. This study utilized inventory data to estimate the variability and range of carbon stocks in Chakras and Ajas at different stages and other typical land uses in the area. Data were collected through an extensive field survey comprising 171 measurement plots, 4.592 trees, 731 palms, and various crops. To represent the landscape heterogeneity of above-ground biomass (AGB) carbon stocks, plots were randomly located, representing a stratified sample of eight different land uses. This sampling was implemented with a 95% confidence interval and a 10% error margin. Additionally, two other land uses (primary forest and an expert-identified best agroforestry - Model Chakra) were included, although they were not statistically defined. The results indicate that on average, Chakras/Ajas have more AGB carbon than pastures and monocultures (25.5 vs. 4.38 MgCha−1). The best Chakras and Ajas can achieve a carbon mitigation potential comparable to secondary forests, with a maximum of 105.6 MgCha−1, further emphasizing the need to support these traditional practices. Additionally, these systems provide co-benefits such as enhanced biodiversity (Shannon index of 1.98 in Model Chakras), improved ecosystem structure, and valuable provisioning services. This study also highlights successful cases that can serve as models for implementing NCS strategies. Based on these findings, it is evident that Chakras and Ajas in the Ecuadorian Amazon possess significant carbon mitigation potential and provide essential resources, highlighting the importance of supporting these traditional agricultural practices as NCS over monocultures.
There is a need to find and integrate cost-effective solutions that can be replicable to avoid an increase in green house gases (GHG) and reduce the effects of climate change (Griscom et al., 2017; Calvin et al., 2023). Natural Climate Solutions (NCS) encompass strategies for climate change mitigation through protecting, restoring, and improving the management of terrestrial and marine ecosystems (Ellis et al., 2024). These solutions improve carbon sequestration, protect local biodiversity, ensure food security, and preserve social and cultural values (Leavitt et al., 2021).
Agroforestry systems (AFS) are part of NCS's improving management strategy. AFS integrates agriculture and forest conservation while effectively providing environmental and socioeconomic benefits (Hart et al., 2023).
In the Ecuadorian Amazon, Indigenous agroforestry systems, locally known as “Amazonian Chakra” and “Amazonian Aja” for the Kichwas and Shuar nationalities, respectively, are ancient practices that combine agriculture and forest for subsistence. They are characterized by being diverse, providing food and medicine (Coq-Huelva et al., 2017, 2018; Torres et al., 2015), and maintaining cultural and spiritual customs (Kohn, 2013). This traditional agroforestry system does not involve fertilizers, pesticides, or heavy machinery, with the advantage of preserving mature native trees for natural regeneration (Torres et al., 2022). Establishing and managing these systems follow a pattern that starts with the selective logging of easily accessible primary forests (near rivers and roads) (Luna and Barcellos-Paula, 2024). The subsequent clearing is then carried out to establish mixed-crop cultivation systems (Chakras/Ajas), followed by a fallow stage for land recovery (Gray et al., 2008).
Due to its importance, the Amazonian Chakra is part of the Globally Important Agricultural Heritage System (GIAHS) (FAO, 2023; Torres et al., 2022). However, these ancestral systems are threatened, as Indigenous peoples increasingly engaged in the market economy and adopt unsustainable agricultural practices (Tinoco-Jaramillo et al., 2024), as illegal timber extraction (Vasco et al., 2017), extensive cattle ranching (Gray et al., 2008; Torres et al., 2023b), and monoculture (Vasco et al., 2021). For instance, Borja et al. (2023) showed that Ecuador's largest expansion of agriculture occurred in the Amazon, with a loss of 500.000 ha of forest from 1985 to 2023.
This land-use dynamic has created a heterogeneous and fragmented landscape in the Ecuadorian Amazon (Figure 1b), resulting in a complex mosaic of various land uses ranging from remnant forests and Chakras and Ajas in different stages to monocultures. The social and environmental benefits of the Chakra/Aja are documented in different studies (Huera-Lucero et al., 2024; Tinoco-Jaramillo et al., 2024; Torres et al., 2022; Doyle et al., 2017; Bremner and Lu, 2006) but their mitigation capacity is little studied and lacks comprehensive and comparative analysis between the different land uses in the Ecuadorian Amazon. Thus to recognize and strengthen ancestral agroforestry systems as an NCS, it is necessary to assess possible losses, identify their mitigation potential (Keith et al., 2024), their characteristics that drive carbon storage, and determine the benefits associated with AFS (Bremner and Lu, 2006; Keith et al., 2024). This information is also essential to support policymakers and practitioners (Ellis et al., 2024) in identifying management priorities to avoid further carbon losses and restore degraded land (Berenguer et al., 2014).
Figure 1. Study area location and characteristics. (a) Location of the study area in Ecuador. (b) Landscape view of the study area. Source: Subset of Planet SuperDove image 2023.
To address these gaps, an integrated landscape approach provides a valuable framework for understanding the role of agroforestry systems like Chakra and Aja within the broader productive mosaic. This perspective is essential for assessing trade-offs and synergies, allowing for management practices that enhance carbon sequestration, biodiversity conservation, and local livelihoods, thereby strengthening their potential as NCS (Sayer et al., 2013; Reed et al., 2020). Therefore, this study applies a robust and statistically rigorous methodological framework, enabling a precise assessment of carbon stock across a diverse range of land uses through field data collection and data analysis to ask the following research questions: How do carbon stocks in aboveground biomass (AGB) in Amazonian Chakra and Aja systems compare to other land uses in the surrounding landscapes?, what are the characteristics of the most successful agroforestry practices in the Amazonian Chakra and Aja systems?, how do the Amazonian Chakra and Aja systems comply with the criteria for being recognized as a Natural Climate Solution (NCS) strategy?
The study was conducted in the Central Ecuadorian Amazon, within the globally significant Andean-Amazonian biodiversity hotspot (Figure 1a) (Myers et al., 2000; Mittermeier et al., 1998), encompassing the provinces of Napo and Pastaza (Figure 2). This region, ancestrally inhabited by Amazonian Kichwa and Shuar indigenous communities and influenced by migrant settlers for over 400 years, exemplifies a unique convergence of cultural heritage and ecological richness. Its critical role in global biodiversity and endemism makes it a priority area for conservation and sustainable development initiatives. The area is also characterized by dense water drainage (de Castro et al., 2018), with humidity exceeding 80%, temperatures ranging from 24-27°C, and year-round rainfall (4,000 mm/year) (Zambrano et al., 2019), making it one of the wettest region in the world (Cerón et al., 2024).
Figure 2. Plot design. The image shows a measured Chakra. The red dots are the coordinates of the corner of the plot. The graphics shows the plot dimension and the AGB pools.
The two provinces are mostly covered by native vegetation but, there is an intervention front dispersed around the main population centers, roads, and rivers. The study was carried out in households with different land uses, predominating the Amazonian Chakra and Aja systems. The study area focuses on the transformed land from indigenous territories. To determine these areas, spatial information was edited from the map of indigenous territories of the Amazon Network of Georeferenced Socio-Environmental Information (RAISG, 2022) and the Land Use Map of the Ecuador Ministry of Environment of 2018 (MAATE, 2019). As shown in Figure 1b, the area is highly fragmented, with remaining forest patches within agricultural land. In some cases, the properties are fully deforested while others keep some trees remaining, the last being an ancient practice. The selected sample in the two provinces covers 117,147.58 ha.
The design of the plots was based on the methodology of Torres et al. (2022), who defined an optimal plot size of 40 × 40 m (1,600 m2) (Figure 2) to capture biodiversity in indigenous agroforestry systems - Amazon Chakras - considering that the size range of each farm is between 1.2 and 4 ha. Data was collected from 171 plots distributed in the study area (see Supplementary Figure S1).
The collected data included plot code, plot geographic coordinates, common and scientific names of each species, diameter at breast height (DBH), commercial height, total height, social position, stem quality, and crown width. Precision instruments were used in the field phase, vertex to measure tree height shrubs, palms, bamboo, and the different crops were measured. All trees, shrubs, palms bamboo, and crops were measured.
To capture the variability of carbon in the landscape, we selected eight predominant land uses in the area, focusing on including Chakra/Ajas in their different stages. This selection was supported through workshops with experts who reviewed and validated the approach. The characteristics of these strata or land uses are described in the Table 1 (see Supplementary Figures S2–S4, for reference photos of each strata).
A two-phase stratified random sampling method was then applied. The first phase involved pilot sampling to validate the number of strata based on the variance of AGB and the landscape proportions of different land uses. The second phase further refined the sample. This methodological framework establishes that the principal unit of analysis is individual plots, distributed across a broad set of land use practices, enabling the comparison of AGB of these different practices
We also added two strata. The first was the primary forest (J) and Model Chakras/Ajas (G), which are not statistically representative. Their inclusion is explained in the following paragraphs.
In the pilot phase, data came from 44 randomly located plots to establish the variance of the AGB and the landscape proportion of the different land uses under study and thus refine the final sample (second phase) with a confidence interval of 95% and an error of 10%. Out of those 44 plots, 8 landed in forests since the original map extended a buffer around the agricultural areas to allow for possible mapping errors. Since this study focuses on evaluating carbon stocks in indigenous traditional agroforestry systems, we used the 36 plots located outside forests to define our final eight land use categories (A-F and H-I). We then calculated their respective statistics and determined the sample size for the second phase (Table 2). Hence, with the information from the pilot phase, we used the formula below to calculate stratified sampling following the recommended methodology of UNFCCC (2010) to assess biomass content:
where:
n = Number of sample plots required for estimation of biomass stocks within the project boundary; dimensionless.
N = Total number of possible sample plots within the project boundary; dimensionless.
tVAL = Two-sided Student's t-value, at infinite degrees of freedom, for the required confidence level; dimensionless.
wi = Relative weight of the area of stratum i.
si = Estimated standard deviation of biomass stock in stratum i.
E2 = Acceptable margin of error in estimation of biomass stock within the project boundary.
i = 1, 2, 3, … biomass stock estimation strata within the project boundary.
The total stratified sample required was 153 plots (Table 2). During the second phase of fieldwork, data was collected from an additional 117 randomly selected plots. The combined results from both phases were used to update the standard deviation and landscape proportions of the eight land uses, leading to a revised stratified sample (Table 3). We confirmed that the initial estimated sample size was accurate, and the actual total number of plots needed was smaller (n = 94). Additionally, all strata groups had sufficient observations to achieve a 95% confidence interval with a 10% margin of error (Table 3).
However, there were six missing values, due to the absence of specific allometric equations or because crops were in a very early-stage. This included five missing values for group D and one for group A. To maximize the utility of the collected data, 147 plots were utilized to estimate AGB carbon in the different land uses that are statistically representative. Furthermore, information from 8 plots from the primary forest (J) and 10 from the model chakra (G) are included for comparison purposes.
In addition to assessing carbon stocks in traditional agroforestry systems, this study aims to identify best practices, referred to as “Model Chakra” (G). The Model Chakra/Aja systems were required to meet specific criteria determined by local experts: high tree diversity, the application of local knowledge, and the provision of income and economic resources for their owners. To achieve this, the project presented its objectives and identified these best practices during two workshops with local stakeholders in the Ecuadorian Amazon, held in Puyo and Tena. As a result, 10 plots were selected, and their outcomes will be presented in the following sections. These results are compared to average systems and practices; and the 8 plots from the primary forest (J) to understand the potential for carbon storage better.
Finally, to evaluate the most successful cases related to carbon content, the plots with the highest carbon values - above the third quartile Q3 - from the different Chakra/Aja types are selected to analyze their characteristics concerning carbon in AGB, tree number, and DBH.
To estimate AGB, allometric equations were applied, using data collected in the floristic inventory of each plot (trees with DBH 1.30 ≥ 10 cm), in combination with species-specific values of wood density p = gcm−3 (Chave et al., 2006; Baker et al., 2004; Zanne et al., 2009). In the case of palms, DBH was replaced by height. The equations are specified in Table 4.
The AGB is measured in kilograms of dry mass, where ρ is the specific gravity of the wood (in gcm−3), and DBH represents the diameter at the height of the breast (cm), considering all trees with DBH1.30 ≥ 10 cm. The wood-specific gravity values for each species were primarily derived from Baker et al. (2004). When specific gravity data were unavailable, a mean value of 0.47 gcm−3 was employed, as recommended for tropical secondary forests by van Breugel et al. (2011) and McGroddy et al. (2015). The total carbon content in the AGB (expressed as MgCha−1) was estimated by multiplying each plot's average AGB (IPCC, 2018) by a wood-carbon ratio of 47% (Manickam et al., 2014).
Table 5, Figure 3 show the carbon statistics in AGB for each chakra type and forest. The primary forest (J) has the highest mean 140.0 and max 200.0 MgCha−1 values followed by the secondary forest > 15y (I) with a mean of 79.4 MgCha−1. These values agree with Berenguer et al. (2014), Mackey et al. (2020), and Cardozo et al. (2022) respectively. Primary forest and regeneration forests will be only used as a reference to compare the mitigation capacity with the Chakras/Ajas types, as they don't include intensive management.
Figure 3. Above ground biomass carbon statistics per strata (MgCha−1). Black dots indicate the amount of carbon in each plot.
Chakras/Ajas types C, B, F, and G have mean values ranging from 23.1 to 49.1. and maximum values from 42.8 to 105.6 MgCha−1. Their variability is SD = 20.9-31.1. The lowest AGB carbon stocks are for stratas A, D, and E, with mean values ranging from 5.61 to 21.0 MgCha−1, and maximum values of 19.7 to 30.2 MgCha−1. It is important to notice that the mean value for D is affected by cero values as the allometric equations e.g. Ipomoea batatas, Solanum quitoense and Selenicereus undatus are not available. They also show a low variability (SD = 4.82-30.2).
Also, the Model Chakra (G) outperforms, on average, other Chakras/Ajas types (A,B,C). Although G plots were deliberately selected to meet specific criteria, the results show that they vary within the AGB carbon stocks (SD = 13.91). This variation is also observed in the Mature market Chakra (C) with a variation of SD = 20.89. Interestingly, within this strata, high values are also found. There are 9 plots with values higher than the average of Model Chakra, and the highest value 105.5 MgCha−1, is also found in this strata.
The plots for stratas G and C with values greater 50 MgCha−1 shows the mitigation capacity of these ancestral systems, which resembles the capacity of a secondary forest > 15 years (I) (Paniagua-Ramirez et al., 2021; Sugiyama et al., 2024; Rodrguez-Len et al., 2025).
Figure 4 shows the percentage of contribution of each measured carbon pool for each strata. Living trees are the major carbon AGB pool in the study area, except for (D) where trees are the scarcest as shown in Figure 5. It also highlights the presence of palms within the landscape. Palms are a valuable ecological and social feature. They are important contributors to biodiversity, and animal food and are a source of nourishment, fibers, and construction materials, especially within Indigenous communities (Byg and Balslev, 2006). The figure also shows that trees are still part of the production system and are found within the categories focused on agriculture.
Figure 4. Percentage contribution of the main aboveground biomass carbon pools by strata. Strata: A, Initial Chakra; B, Mature subsistence Chakra; C, Mature market Chakra; D, Monoculture; E, Forest plantations; F, Silvopasture; G, Model Chakra; H, Secondary forest < 15y; I, Secondary forest > 15y; J, Primary forest.
The aboveground carbon in the Chakras/Ajas with the largest stocks (3-4 quartiles) is explained mostly by a quadratic regression based on the average diameter at the breast height (DBH) and the number of trees (#trees). All the terms in the quadratic polynomial are statistically significant at least at a 95% confidence interval and explain 93% of the variation in the data (r2 = 0.9393).
There is a reduction of tree density in contrast with higher DBH classes, as shown in Figure 5. Forest types (H, I, J) have a higher density for all the DBH classes except for the ≤ 29.99 cm class in which Forest plantations (E) has the higher number. The model chakra (G) has a similar pattern to the Forest types followed by the Mature market chakra (C). This variability in DBH is important to maintain the forest structure related to ecosystem function and diversity (Spies, 1998).
Table 6 shows the floristic diversity for the different land uses. Primary Forests have the highest tree species diversity (average 45.75 species). Secondary forests, both ≤ 15 years (23 sp.) and ≥ 15 years (27.4 sp.) show a progressive increase in biodiversity with age, demonstrating a natural successional process enhancing floristic diversity (Garate-Quispe et al., 2024; de Jesus Silva et al., 2016).
A key finding of this study is the significant role of trees in managed land use systems and its effects on biodiversity. Model Chakra (G) stands out with the highest Richness (14.10) and Shannon index (1.98) among strata A to G. However, other Chakra types (Strata B and C) and pastures with dispersed trees (Strata F) also maintain a noticeable level of diversity, with Shannon indices of 1.72, 1.62, and 1.75, respectively. This emphasizes the importance of Indigenous practices to maintain diversity and balanced ecosystems in managed land use systems (Levis et al., 2024).
This study integrated the Model Chakras (G) to identify the best examples of landscape management. However, our analysis revealed that other strata also contain significant examples of mitigation capacity. This section highlights those cases.
The types of Chakras/Ajas with the highest carbon values are Mature market Chakra (C), Model Chakra (G) and Silvopasture (F) carbon (see Table 5, Figure 3). To identify the most successful cases related to AGB, values greater than the third quartile (Q3) were selected, resulting in 22 plots. Q3 values for each Chakra are: C ≥ 38.1, F ≥ 49.3, and G ≥ 64.9.
Figure 6 shows the 22 plots, highlighting trees as the main contributor to the AGB. The figure also illustrates the trend between tree number, DBH, and carbon content.
Figure 6. Sucessfull chakra plots–for Stratas: C, Mature market Chakra; F, Silvopasture; G, Model Chakra—in relation to diameter classes, number of trees per diameter class, and above ground biomass carbon per hectare (MgCha−1).
It is important to note that the 15069 and 15090 plots have lower values, 9.49 and 11.18 MgCha−1 respectively, than their Q3. This is because, in the case of 15069, an edible palm Bactris gasipaes is the main contributor with 34.65 MgCha−1, while in plot 15090 cacao crops add 27.90 MgCha−1.
Another special case is plot 15003. Although trees are the largest carbon pool 67.14 MgCha−1, there is also a significant gain from cocoa cultivation 38.52 MgCha−1, bringing the total to 105.6 MgCha−1.
Table 7 presents the most successful values, considering only trees as contributors to AGB carbon, for each Chakra Type (C, G, and F). Plot IDs 15003, 15101, and 16044 demonstrate that large trees (DBH > 50 cm) significantly contribute to ABG carbon stocks, even if they are few in number. Additionally, these plots are linked to agricultural products, providing environmental benefits and boosting the local economy in economically depressed areas.
Within the study area, 299 tree species were identified. In the three most successful plots, 22 species of trees and 3 species of palms are found. Table 8 shows the main uses of these tree species according to Rios et al. (2007). One of the key characteristics of the Amazonic Chakra/Aja is its diversity, which ensures access to natural resources for indigenous communities. Many of these species provide nourishment, thereby ensuring food availability. Some of these trees, such as Cedrelinga cateniformis and Swietenia macrophylla, are valuable fine wood species. This diversity not only guarantees access to food or valuable wood but also medicine and sources for rituals.
NCS offers a range of land stewardship to achieve the Paris Agreement and maintain temperatures below 2°C (Griscom et al., 2017). AFS is one land use with great potential to fulfill commitments to reduce emissions from improved management agriculture (Duguma et al., 2023). To successfully implement AFS, local conditions need to be understood. This study provides a reference level for understanding the carbon dynamics within Indigenous agroforestry systems in the Ecuadorian Amazon. It shows its potential as an NCS by understanding the different land uses within the study area and their effects on AGB carbon stocks.
The results show that the Ecuadorian Amazon's Indigenous agroforestry systems (strata B, C, F, and G) average more AGB carbon stock than crops and pastures (stratum D) (p = 0.000 and p = 0.001, see Supplementary material). This emphasizes the importance of recognizing and learning from local Indigenous knowledge and practices that help mitigate climate change. But also highlights its potential for carbon addition if Chakras/Ajas is strengthened and expanded instead of monocultures and pastures. This outcome is expected, as agroforestry produces more AGB than monocultures in the same conditions (Nair, 2012), highlighting the importance of integrating trees into production systems. Notably, model chakras (G), which represent some of the best Indigenous agroforestry practices (group G) have, on average, more carbon than forest plantations (strata E) (p = 0.030; see Supplementary material) that still had a low DBH in this study. While forest plantations are recognized for their carbon stock potential, they are generally low in biodiversity (Wungshap et al., 2023). In this study strata E didn't show high AGB carbon stocks because they were young plantation (DBH < 29.99 cm) of Ochroma Pyramidale which is characterized for its low wood density.
The production area of the indigenous communities includes intermingled areas of forest recovery and patches of primary forest (Gray et al., 2008). This allowed the primary forest to be included as a reference level, showing that the mean carbon stock difference between the primary forest (J) and monoculture (D) is 135.62 MgCha−1. This value illustrates the significant loss in carbon storage capacity when forests are converted to crops. Even if all the equations for monocultures are unavailable, the values won't change significantly, as non-woody plants have low biomass. For instance, Bonini et al. (2018) quantified a similar loss of 130.5 MgCha−1 when Amazonian forests are converted to soybean plantations. Although the loss of carbon stocks from forest to Chakra/Ajas is lower than monocultures, the NCS strategy focuses on additionality. Therefore, the most effective approach is targeting and enhancing areas with lower carbon stocks.
The landscape can recover approximately 21.12 MgCha−1 (p = 0.000) of its mitigation capacity when monoculture and pastures (D) are converted to ancestral systems (AFS), considering the average values for mature Chakra (B), mature market Chakra (C), and silvopasture (F). If model Chakra is regarded as the sequestration potential, it will be ≈49 MgCha−1 (p = 0.001). This highlights the potential for carbon addition if Chakras/Ajas are strengthened and expanded instead of monocultures.
This study also highlights successful cases where Chakra/Ajas systems can achieve carbon stock values comparable to those of a secondary forest in its later stages. Specifically, these systems can reach 90.81 MgCha−1 when only trees are assessed, and a total of 105.6 MgCha−1 when crops or palms are included. These results align with Huera-Lucero et al. (2024), who measured 90.4 MgCha−1 in Chakra systems mixed with cacao and 97.8 MgCha−1 when mixed with fruits. These values are higher than those reported by Jadán et al. (2012), who found a mean value of 52.8 MgCha−1 in Chakra systems mixed with cacao. Additionally, we found that sylvopastures can add 56.08 MgCha−1 to carbon stocks, while Torres et al. (2023a) reported a potential range of 45.31 to 87.57 MgCha−1 in a nearby region. The best cases demonstrate the significant potential for climate mitigation if successful models are established, highlighting the power of Chakras and Ajas as NCS.
To successfully implement AFS, local conditions need to be understood broadly. Analyzing the landscape surrounding Amazonian Chakra/Aja allows understanding the components that affect the quantity of carbon in AGB.
This study shows that trees are the main contributors to AGB pool carbon in the landscape. The factors that affect the amount of carbon in above-ground biomass depend on tree density, DBH, and species richness, as shown in this and other studies (Cardozo et al., 2022; Ameray et al., 2021). Younger trees (low DBH–high density) have a higher rate of carbon uptake as they grow, while larger trees store more carbon, impact soil carbon, and act as seed sources (Sist et al., 2014; Berenguer et al., 2014; Mackey et al., 2020; Ameray et al., 2021). Chakras/Ajas have different DBH classes and tree density which is crucial for creating structure and maintaining ecosystem function (Spies, 1998).
Since Chakras/Ajas are harvesting systems, it is essential to establish management plans that allow the extraction of some trees while permanently protecting others by setting mitigation targets. For instance, maintaining large trees is a key strategy to secure carbon stocks (Sist et al., 2014) and Figure 6. Our research also demonstrated that palms are crucial for climate mitigation and as a source of livelihood (zur Lage et al., 2023) through the different land uses. This feature is also important to increase structure and biodiversity, which is higher in AFS than other land uses.
Traditional knowledge influences forest management practices (Doyle et al., 2017) and can be leveraged as an advantage. As shown in Table 8, trees in ancestral agroforestry systems provide various services to their owners. Considering the benefit that the remaining trees can generate for their owner is key to ensuring permanence and securing carbon stocks in the area (Tavares et al., 2024; Blicharska and Mikusinski, 2014).
Following the aforementioned characteristics, this study suggests a positive change in land use management by reversing the degradation and transition from primary forests to monocultures (Figure 7). This approach avoids the loss of carbon stocks, biodiversity, and livelihoods (Tinoco-Jaramillo et al., 2024). The main focus is on transitioning from crops or pastures to more complex systems that can be tailored to landowners' specific needs through diversified management options. For example, adding trees in recovery areas after leaving the Chakas/Ajas, where secondary forests are established, can accelerate the mitigation process, contributing approximately 16.2 MgCha−1 (Sugiyama et al., 2024). Integrating trees within active production systems, such as pastures, or systems like Chakas/Ajas, which can function similarly to secondary forests regarding their mitigation capacity, opens up opportunities for these systems to be adopted and strengthened, benefiting also biodiversity and livelihoods that are based principles of an NCS strategy.
Figure 7. Agroforestry system management alternatives to monoculture in relation to above ground biomass carbon per hectare (MgCha−1).
Although this study highlights cases of good management throughout the study area, resulting in effective mitigation capacity, biodiversity, and improved resource access, further analysis is needed to understand why these practices have not been widely adopted. Thus, a cost-benefit analysis is essential to comprehend this effect.
In addition to their significant mitigation potential, agroforestry systems offer multiple benefits, such as regulating temperature, maintaining soil moisture, and diversifying food availability, among others (Rosenstock et al., 2019; Montagnini, 2024; Rijal, 2019). In the study area, these systems are critical because they can also enhance residents' economic well-being, as many live in extreme poverty (Luna-Ojeda et al., 2020). For instance, AFS integrated with cash crops such as cacao, coffee, and timber offer a viable economic solution while enhancing productive sustainability for associated producers (Torres et al., 2024; Garrett et al., 2024). Ilex guayusa a medicinal plant grown in Chakras/Ajas and widely used within communities (Saltos et al., 2016), is now being successfully commercialized through the creation of sustainable and equitable supply chains based on local products (Jarrett et al., 2024). Additionally, AFS enhances yields, thereby boosting income for farmers (Tinoco-Jaramillo et al., 2024). Alleviating poverty can reduce forest clearing without diminishing agricultural rent (Schroth et al., 2002; Miyamoto, 2020). However, it is necessary to develop a strategy or framework to prevent leakage in primary forests (Aukland et al., 2003), such as providing monetary recognition to are already engaged in protection and mitigating efforts (Eguiguren et al., 2019).
Land management strategies, including mitigation, are crucial for addressing biodiversity loss (Eguiguren et al., 2019). The Amazon rainforest is renowned for its rich biodiversity. Chakras and Ajas, which are agroecological zones characterized play a significant role in maintaining characteristics of a healthy environment (zur Lage et al., 2023). Ancestral agroforestry systems, unlike monocultures, have shown a remarkable ability to conserve plant biodiversity, as evidenced in Table 6 and supported by various studies (Vera et al., 2017; Huera-Lucero et al., 2024). Chakras and Ajas also function as buffer zones and natural corridors, reducing pressure on natural reserves and providing valuable resources for both local indigenous communities and wildlife (Torres et al., 2015; Vera et al., 2017). Reversing biodiversity loss in synergy with climate change mitigation efforts can contribute to biodiversity conservation rather than exacerbating its decline (Leclre et al., 2020).
Finally, agroforestry systems also help adapt to climate change's effects. The addition of forest cover helps regulate temperatures, control torrential rains, and improve the soil, which in turn serves as a water reservoir. These benefits make Chakras/Ajas invaluable for building the resilience of local ecosystems and communities adaptation to the adverse impacts of climate change (Torres et al., 2015; Vizuete-Montero et al., 2024). Strengthening these systems can provide long-term environmental and socio-economic stability in vulnerable regions.
This study underscores the importance of landscape-level analyses in evaluating traditional agroforestry systems (AFS) like Chakra and Aja within the Amazon, a critical global carbon reservoir. By demonstrating that these systems can achieve carbon storage levels comparable to secondary forests, the findings highlight their effectiveness as NCS. By integrating these ancestral practices into landscapes dominated by monoculture or pastureland, there is a significant potential for enhancing regional carbon sequestration. These approaches emphasizes the value of combining ecological and cultural knowledge to strengthen climate mitigation efforts and promote sustainable land management. Carbon storage in these areas depends on the diameter at breast height (DBH) and the number of trees in the ancestral agroforestry systems, with large trees being serving as important contributors to carbon storage. By showcasing the capacity of ancestral agroforestry systems in the Ecuadorian Amazon, this study highlights their potential for enhancing climate mitigation and maintaining biodiversity and ecosystem complexity, key features of a healthy environment. In addition to their environmental benefits, Chakra and Aja systems likely offer significant social and economic advantages, although this study did not address these. Future research should investigate the role of these sysmtes in supporting local livelihoods and preserving cultural heritage, providing insights into how they contribute to community resilience and sustainable management. For instance, trees found in these systems are a source of livelihood for Indigenous communities, which is crucial for ensuring access to resources. Moreover, when combined with cash crops, they can help alleviate poverty. The documented benefits of agroforestry, combined with the identified best practices, provide a robust foundation for developing extension and public policy programs to implement NCS strategies. These programs can help scale up agroforestry practices, offering viable economic solutions for Indigenous communities while promoting sustainable land use and conservation efforts. Future research should explore carbon dynamics and their long-term potential for biodiversity conservation. Additionally, investigating the socio-economic impacts of scaling up these systems could further inform sustainable development and climate adaptation strategies.
The raw data supporting the conclusions of this article will be made available by the authors, without undue reservation.
The Nature Conservancy (TNC) respects and promotes the right of Indigenous Peoples to self-determination. TNC is committed to seeking and honoring Free, Prior, and Informed Consent when engaging with Indigenous Peoples and local communities. Thus, all data for this study was collected after explaining the study's scope and obtaining permission from each Chakra or Aja owner.
PÁ-N: Methodology, Data curation, Formal analysis, Investigation, Visualization, Writing – original draft. BT: Conceptualization, Methodology, Investigation, Data curation, Formal analysis, Visualization, Writing – original draft. MC: Methodology, Investigation, Formal analysis, Writing – review & editing. MR: Conceptualization, Methodology, Funding acquisition, Writing – review & editing.
The author(s) declare that financial support was received for the research and/or publication of this article. Funding support for this research has been provided by the Bezos Earth Fund (BEF). Ideas expressed do not necessarily reflect the views of BEF.
The authors thank the Bezos Earth Fund (BEF) for funding. Also, want to thank the field team: Lady Shiguango, Gabriel Grefa, Joseph Moreira, Luis Vallejo, Thony Huera, Gerardo Machoa, Christian Tipán, Luz Huera, Víctor Torres, Edwin Collahuaso, and the Kichwa and Shuar people who kindly let us do this research in their properties. The authors also want to thank the reviewers for their valuable comments.
The authors declare that the research was conducted in the absence of any commercial or financial relationships that could be construed as a potential conflict of interest.
The author(s) declare that no Gen AI was used in the creation of this manuscript.
All claims expressed in this article are solely those of the authors and do not necessarily represent those of their affiliated organizations, or those of the publisher, the editors and the reviewers. Any product that may be evaluated in this article, or claim that may be made by its manufacturer, is not guaranteed or endorsed by the publisher.
The Supplementary Material for this article can be found online at: https://www.frontiersin.org/articles/10.3389/ffgc.2025.1513140/full#supplementary-material
Ameray, A., Bergeron, Y., Valeria, O., Girona, M. M., and Cavard, X. (2021). Forest carbon management: a review of silvicultural practices and management strategies across boreal, temperate and tropical forests. Curr.Forest. Rep. 7, 245–266. doi: 10.1007/s40725-021-00151-w
Aukland, L., Costa, P. M., and Brown, S. (2003). A conceptual framework and its application for addressing leakage: the case of avoided deforestation. Climate Policy 3, 123–136. doi: 10.3763/cpol.2003.0316
Baker, T. R., Phillips, O. L., Malhi, Y., Almeida, S., Arroyo, L., Fiore, A. D., et al. (2004). Variation in wood density determines spatial patterns inamazonian forest biomass. Glob. Chang. Biol. 10, 545–562. doi: 10.1111/j.1365-2486.2004.00751.x
Berenguer, E., Ferreira, J., Gardner, T. A., Arago, L. E. O. C., Camargo, P. B. D., Cerri, C. E., et al. (2014). A large-scale field assessment of carbon stocks in human-modified tropical forests. Glob. Chang. Biol. 20, 3713–3726. doi: 10.1111/gcb.12627
Blicharska, M., and Mikusinski, G. (2014). Incorporating social and cultural significance of large old trees in conservation policy. Conservat. Biol. 28, 1558–1567. doi: 10.1111/cobi.12341
Bonini, I., Marimon-Junior, B. H., Matricardi, E., Phillips, O., Petter, F., Oliveira, B., et al. (2018). Collapse of ecosystem carbon stocks due to forest conversion to soybean plantations at the amazon-cerrado transition. For. Ecol. Manage. 414, 64–73. doi: 10.1016/j.foreco.2018.01.038
Borja, M. O., Holgun, W., Aguilar, C., Tern, K., Rodriguez, A., and Josse, C. (2023). Documento de bases tericas de algoritmo (ATBD) raisg - mapbiomas ecuador coleccin 1.0 de mapas anuales de cobertura y uso del suelo de ecuador. Quito. Available online at: https://ecuador.mapbiomas.org/wp-content/uploads/sites/7/2023/12/ATBD-Ecuador-Coleccion-1.0-MapBiomas-Ecuador.pdf (accessed September 16, 2024).
Bremner, J., and Lu, F. (2006). Common property among indigenous peoples of the ecuadorian amazon. Conserv. Soc. 4, 499–521. Available online at: https://www.ciesin.columbia.edu/repository/pern/papers/Bremmer_Lu.pdf
Byg, A., and Balslev, H. (2006). Palms in indigenous and settler communities in southeastern ecuador: Farmers perceptions and cultivation practices. Agroforest. Syst. 67, 147–158. doi: 10.1007/s10457-005-1704-1
Calvin, K., Dasgupta, D., Krinner, G., Mukherji, A., Thorne, P. W., Trisos, C., et al. (2023). “IPCC, 2023: Climate change 2023: Synthesis report. contribution of working Groups I, II, and III to the sixth assessment report of the intergovernmental panel on climate change,” in Technical Report, Intergovernmental Panel on Climate Change (Geneva: IPCC).
Cardozo, E. G., Celentano, D., Rousseau, G. X., Silva, H. R., Muchavisoy, H. M., and Gehring, C. (2022). Agroforestry systems recover tree carbon stock faster than natural succession in eastern amazon, brazil. Agroforest. Syst. 96, 941–956. doi: 10.1007/s10457-022-00754-7
CATIE (2009). “Propuesta metodolgica para la evaluacin de servicios ambientales. adaptado de metodolog para la evaluacin de servicios ambientales,” in Technical report, CATIE-Centro Agronmico Tropical de Investigacin y Enseanza (Turrialba). Available online at: https://repositorio.catie.ac.cr/handle/11554/9810 (accessed May 10, 2023).
Cerón, W. L., Kayano, M. T., Andreoli, R. V., Canchala, T., Avila-Diaz, A., Ribeiro, I. O., et al. (2024). New insights into trends of rainfall extremes in the amazon basin through trend-empirical orthogonal function (1981–2021). Int. J. Climatol. 44, 3955–3975. doi: 10.1002/joc.8561
Chave, J., Andalo, C., Brown, S., Cairns, M. A., Chambers, J. Q., Eamus, D., et al. (2005). Tree allometry and improved estimation of carbon stocks and balance in tropical forests. Oecologia 145, 87–99. doi: 10.1007/s00442-005-0100-x
Chave, J., Helene, M.-L., Baker, T., Toms, E., terr Steege, H., and Webb, C. (2006). Regional and phylogenetic variation of wood density across 2456 neotropical tree species. Ecol. Applicat. 16, 2356–2367. doi: 10.1890/1051-0761(2006)016[2356:RAPVOW]2.0.CO;2
Coq-Huelva, D., Higuchi, A., Alfalla-Luque, R., Burgos-Morn, R., and Arias-Gutirrez, R. (2017). Co-evolution and bio-social construction: the kichwa agroforestry systems (chakras) in the ecuadorian amazonia. Sustainability 9:1920. doi: 10.3390/su9101920
Coq-Huelva, D., Torres-Navarrete, B., and Bueno-Surez, C. (2018). Indigenous worldviews and western conventions: Sumak kawsay and cocoa production in ecuadorian amazonia. Agric. Human Values 35, 163–179. doi: 10.1007/s10460-017-9812-x
de Castro, A. A., Cuartas, L. A., Coe, M. T., Randow, C. V., Castanho, A., Ovando, A., et al. (2018). Coupling the terrestrial hydrology model with biogeochemistry to the integrated land surface model: Amazon basin applications. Hydrol. Sci. J. 63, 1954–1966. doi: 10.1080/02626667.2018.1538592
de Jesus Silva, C. V., dos Santos, J. R., Galvo, L. S., da Silva, R. D., and Moura, Y. M. (2016). Flor-stica e estrutura de uma floresta primria e uma cronossequncia de sucesso secundria na amaznia. Acta Amazonica 46, 133–150. doi: 10.1590/1809-4392201504341
Douterlungne, D., Herrera-Gorocica, A.-M., G, F. B., Siddique, I., and Soto-Pinto, L. (2013). Allometric equations used to estimate biomass and carbon in four neotropical tree species with restoration potential. Agrociencia 2013, 385–397.
Doyle, B. J., Asiala, C. M., and Fernndez, D. M. (2017). Relative importance and knowledge distribution of medicinal plants in a kichwa community in the ecuadorian amazon. Ethnobiol. Letters 8:777. doi: 10.14237/ebl.8.1.2017.777
Duguma, L. A., Minang, P. A., Watson, C., Nath, A. J., Muthee, K. W., van Noordwijk, M., et al. (2023). Agroforestry as a Key Intervention to Achieve Nationally Determined Contribution (NDC) Targets. Cham: Springer, 641–664.
Eguiguren, P., Fischer, R., and Gnter, S. (2019). Degradation of ecosystem services and deforestation in landscapes with and without incentive-based forest conservation in the ecuadorian amazon. Forests 10:442. doi: 10.3390/f10050442
Ellis, P. W., Page, A. M., Wood, S., Fargione, J., Masuda, Y. J., Denney, V. C., et al. (2024). The principles of natural climate solutions. Nat. Commun. 15:547. doi: 10.1038/s41467-023-44425-2
FAO (2023). The Amazonian Chakra, a Traditional Agroforestry System Managed by Indigenous Communities in Napo Province, Ecuador. Available online at: https://www.fao.org/giahs/giahsaroundtheworld/ecuador-amazonian-chakra/en (accessed October 17, 2024).
Frangi, J. L., and Lugo, A. E. (1985). Ecosystem dynamics of a subtropical floodplain forest. Ecol. Monogr. 55, 351–369. doi: 10.2307/1942582
Garate-Quispe, J., Canahuire-Robles, R., Alarcn-Aguirre, G., Dueas-Linares, H., and Roman-Daobeytia, F. (2024). Changes in floristic and vegetation structure in a chronosequence of abandoned gold-mining lands in a tropical amazon forest. Heliyon 10:e29908. doi: 10.1016/j.heliyon.2024.e29908
Garrett, R., Ferreira, J., Abramovay, R., Brando, J., Brondizio, E., Euler, A., et al. (2024). Transformative changes are needed to support socio-bioeconomies for people and ecosystems in the Amazon. Nat. Ecol. Evolut. 8, 1815–25. doi: 10.1038/s41559-024-02467-9
GIZ (2011). Secuestro de carbono en sistemas agroforestales de cacao y caf ubicados en la reserva de biosfera sumaco. Quito. Available online at: https://cadenacacaoca.info/CDOC-Deployment/documentos/proyectocarbono.pdf (accessed March 18, 2023).
Gray, C. L., Bilsborrow, R. E., Bremner, J. L., and Lu, F. (2008). Indigenous land use in the ecuadorian amazon: a cross-cultural and multilevel analysis. Hum. Ecol. 36, s97–109. doi: 10.1007/s10745-007-9141-6
Griscom, B. W., Adams, J., Ellis, P. W., Houghton, R. A., Lomax, G., Miteva, D. A., et al. (2017). Natural climate solutions. Proc. Natl. Acad. Sci. USA. 114, 11645–11650. doi: 10.1073/pnas.1710465114
Hart, D. E. T., Yeo, S., Almaraz, M., Beillouin, D., Cardinael, R., Garcia, E., et al. (2023). Priority science can accelerate agroforestry as a natural climate solution. Nat. Clim. Chang. 13, 1179–1190. doi: 10.1038/s41558-023-01810-5
Huera-Lucero, T., Lopez-Pieiro, A., Torres, B., and Bravo-Medina, C. (2024). Biodiversity and carbon sequestration in chakra-type agroforestry systems and humid tropical forests of the ecuadorian amazon. Forests 15:557. doi: 10.3390/f15030557
IPCC (2018). “Summary for policymakers,” in Global Warming of 1.5°C. An IPCC Special Report on the Impacts of Global Warming of 1.5°C above Pre-Industrial Levels and Related Global Greenhouse Gas Emission Pathways, in the Context of Strengthening the Global Response To (Geneva: IPCC).
Jadán, O., Navarrete, S. B. T., and Gnter, S. (2012). Influencia del uso de la tierra sobre almacenamiento de carbono en sistemas productivos y bosque primario en Napo, Reserva de Biosfera Sumaco, Ecuador. Revista Amazónica: Ciencia y Tecnología 1, 173–186. doi: 10.59410/RACYT-v01n03ep02-0017
Jarrett, C., Cummins, I., and Logan-Hines, E. (2024). Lessons Learned from Developing Supply Chains Based on Indigenous Agroforestry and Community Forestry in Napo, Ecuador. 359–388. doi: 10.1007/978-3-031-54270-1_11
Keith, H., Kun, Z., Hugh, S., Svoboda, M., Mikol, M., Adam, D., et al. (2024). Carbon carrying capacity in primary forests shows potential for mitigation achieving the european green deal 2030 target. Commun. Earth Environm. 5:5. doi: 10.1038/s43247-024-01416-5
Kohn, E. (2013). How Forests Think: Toward an Anthropology Beyond the Human. Berkeley: Unov. Calif. Press.
Leavitt, S., Cook-Patton, S., Marx, L., Drever, R., Carrasco-Denney, V., Kroeger, T., et al. (2021). “Natural climate solutions handbook a technical guide for assessing nature-based mitigation opportunities in countries,” in Technical Report, The Nature Conservancy (Arlington, VA). Available online at: https://www.nature.org/content/dam/tnc/nature/en/documents/TNC_Natural_Climate_Solutions_Handbook.pdf (accessed June 08, 2024).
Leclre, D., Obersteiner, M., Barrett, M., Butchart, S. H., Chaudhary, A., Palma, A. D., et al. (2020). Bending the curve of terrestrial biodiversity needs an integrated strategy. Nature 585, 551–556. doi: 10.1038/s41586-020-2705-y
Levis, C., Flores, B. M., Campos-Silva, J. V., Peroni, N., Staal, A., Padgurschi, M. C., et al. (2024). Contributions of human cultures to biodiversity and ecosystem conservation. Nat. Ecol. Evol. 8, 866–879. doi: 10.1038/s41559-024-02356-1
Luna, M., and Barcellos-Paula, L. (2024). Structured equations to assess the socioeconomic and business factors influencing the financial sustainability of traditional amazonian chakra in the ecuadorian amazon. Sustainability 16:2480. doi: 10.3390/su16062480
Luna-Ojeda, T., Eguiguren, P., Gter, S., Torres, B., and Dieter, M. (2020). What drives household deforestation decisions? Insights from the ecuadorian lowland rainforests. Forests 11, 1–20. doi: 10.3390/f11111131
MAATE (2019). Cobertura y uso de la tierra (cut 2018). Quito. Available online at: http://ide.ambiente.gob.ec/mapainteractivo/ (accessed October 17, 2022).
Mackey, B., Kormos, C. F., Keith, H., Moomaw, W. R., Houghton, R. A., Mittermeier, R. A., et al. (2020). Understanding the importance of primary tropical forest protection as a mitigation strategy. Mitigat. Adapt. Strat. Global Change 25, 763–787. doi: 10.1007/s11027-019-09891-4
Manickam, V., Krishna, I. V. M., Shanti, S. K., and Radhika, R. (2014). Biomass calculations for carbon sequestration in forest ecosystem. J. Energy Chem. Eng. 2, 30–38.
McGroddy, M. E., Lerner, A. M., Burbano, D. V., Schneider, L. C., and Rudel, T. K. (2015). Carbon stocks in silvopastoral systems: a study from four communities in southeastern Ecuador. Biotropica 47, 407–415. doi: 10.1111/btp.12225
Mittermeier, R. A., Myers, N., Thomsen, J. B., Fonseca, G. A. B. D., and Olivieri, S. (1998). Biodiversity hotspots and major tropical wilderness areas: Approaches to setting conservation priorities. Conservatt. Biol. 12, 516–520. doi: 10.1046/j.1523-1739.1998.012003516.x
Miyamoto, M. (2020). Poverty reduction saves forests sustainably: Lessons for deforestation policies. World Dev. 127:104746. doi: 10.1016/j.worlddev.2019.104746
Montagnini, F. (2024). Integrating Landscapes: Agroforestry for Biodiversity Conservation and Food Sovereignty. Cham: Springer International Publishing.
Myers, N., Mittermeier, R. A., Mittermeier, C. G., da Fonseca, G. A. B., and Kent, J. (2000). Biodiversity hotspots for conservation priorities. Nature 403, 853–858. doi: 10.1038/35002501
Paniagua-Ramirez, A., Krupinska, O., Jagdeo, V., and Cooper, W. J. (2021). Carbon storage estimation in a secondary tropical forest at ciee sustainability center, monteverde, costa rica. Sci. Rep. 11:23464. doi: 10.1038/s41598-021-03004-5
RAISG (2022). Cartographic Data Visualization of Geospatial Information About the Amazon. Available online at: https://www.raisg.org/es/mapas/ (accessed October 1, 2022).
Reed, J., Oldekop, J., Barlow, J., Carmenta, R., Geldmann, J., Ickowitz, A., et al. (2020). The extent and distribution of joint conservation-development funding in the tropics. One Earth 3:753–762. doi: 10.1016/j.oneear.2020.11.008
Rijal, S. (2019). Agroforestry system: approaches for climate mitigation and adaptation. Big Data Agricult. 1, 23–25. doi: 10.26480/bda.02.2019.23.25
Rios, M., Koziol, M. J., Pedersen, H. B., and Granda, G. (2007). Plantas tiles del Ecuador: aplicaciones, retos y perspectivas/Useful Plants of Ecuador: Applications, Challenges, and Perspectives. Quito: Abya-Yala, 652.
Rodrguez-Len, C. H., Sterling, A., Trujillo-Briez, A., Surez-Crdoba, Y. D., and Roa-Fuentes, L. L. (2025). Forest attribute dynamics in secondary forests: Insights for advancing ecological restoration and transformative territorial management in the amazon. Diversity 17:39. doi: 10.3390/d17010039
Rojas Quiroga, R. A., Li, T., Lora, G., and Andersen, L. E. (2013). “A measurement of the carbon sequestration potential of Guadua angustifolia in the Carrasco National Park, Bolivia,” in Development Research Working Paper Series, No. 04/2013. La Paz: Institute for Advanced Development Studies (INESAD).
Rosenstock, T. S., Dawson, I. K., Aynekulu, E., Chomba, S., Degrande, A., Fornace, K., et al. (2019). A planetary health perspective on agroforestry in sub-saharan africa. One Earth 1, 330–344. doi: 10.1016/j.oneear.2019.10.017
Saltos, R. V. A., Vsquez, T. E. R., Lazo, J. A., Banguera, D. V., Guayasamn, P. D. R., Vargas, J. K. A., et al. (2016). Uso de plantas medicinais por populaes rurais da prov-ncia de pastaza, na amaznia equatoriana. Acta Amazonica 46, 355–366. doi: 10.1590/1809-4392201600305
Sayer, J., Sunderland, T., Ghazoul, J., Pfund, J.-L., Sheil, D., Meijaard, E., et al. (2013). Ten principles for a landscape approach to reconciling agriculture, conservation, and other competing land uses. Proc. Nat. Acad. Sci. 110, 8349–8356. doi: 10.1073/pnas.1210595110
Schroth, G., D'Angelo, S. A., Teixeira, W. G., Haag, D., and Lieberei, R. (2002). Conversion of secondary forest into agroforestry and monoculture plantations in amazonia: consequences for biomass, litter and soil carbon stocks after 7 years. For. Ecol. Manage. 163, 131–150. doi: 10.1016/S0378-1127(01)00537-0
Sist, P., Mazzei, L., Blanc, L., and Rutishauser, E. (2014). Large trees as key elements of carbon storage and dynamics after selective logging in the eastern amazon. For. Ecol. Manage. 318:103–109. doi: 10.1016/j.foreco.2014.01.005
Spies, T. A. (1998). “Forest structure: a key to the ecosystem,” in Proceedings of a workshop on Structure, Process, and Diversity in Successional Forests of Coastal British Columbia 72, 34–39.
Sugiyama, A., Game, E. T., and Wright, S. J. (2024). Planting exceptional tropical tree species to increase long-term carbon storage in assisted secondary succession. J. Appl. Ecol. 61, 7–12. doi: 10.1111/1365-2664.14533
Szott, L., Arevalo-Lopez, L., and Perez, J. (1993). “Allometric relationships in pijuayo (Bactris gasipaes h.b.k),” in Internacional sobre Biolog-a, Agronom-a e Industrializacin del Pijuayo, eds. J. Mora-Urpi, L. Szott, M. Murillo, and V. Patino Congreso (Costa Rica: Editorial de la Universidad de Costa Rica), 91–114.
Tavares, M. F., Gallo, P., Nascimento, N., Bauhus, J., Brancalion, P. H., and Feurer, M. (2024). Smallholders' perspectives, motivations, and incentives for restoring the Brazilian Atlantic forest. Restorat. Ecol. 32:e14270. doi: 10.1111/rec.14270
Tinoco-Jaramillo, L., Vargas-Tierras, Y., Habibi, N., Caicedo, C., Chanaluisa, A., Paredes-Arcos, F., et al. (2024). Agroforestry systems of cocoa (Theobroma cacao l.) in the ecuadorian amazon. Forests 15:f15010195. doi: 10.3390/f15010195
Torres, B., Andrade, A. K., Enriquez, F., Luna, M., Heredia, -R M., et al. (2022). Estudios sobre medios de vida, sostenibilidad y captura de carbono en el sistema agroforestal Chakra con cacao en comunidades de pueblos originarios de la provincia de Napo: casos de las asociaciones Kallari, Wiak y Tsatsayaku, Amazon-a Ecuatoriana. Rome: FAO.
Torres, B., Bravo, C., Torres, A., Tipn-Torres, C., Vargas, J. C., Herrera-Feijoo, R. J., et al. (2023a). Carbon stock assessment in silvopastoral systems along an elevational gradient: a study from cattle producers in the sumaco biosphere reserve, ecuadorian amazon. Sustainability 15:449. doi: 10.3390/su15010449
Torres, B., Luna, M., Tipn-Torres, C., Ramrez, P., Muoz, J. C., and Garca, A. (2024). A simplified integrative approach to assessing productive sustainability and livelihoods in the “amazonian chakra” in ecuador. Land 13:2247. doi: 10.3390/land13122247
Torres, B., Maza, O. J., Aguirre, P., Hinojosa, L., and Gnter, S. (2015). The Contribution of Traditional Agroforestry to Climate Change Adaptation in the Ecuadorian Amazon: The Chakra System. Berlin: Springer Berlin Heidelberg, 1973–1994.
Torres, B., talo Espinoza, T. A., Herrera-Feijoo, R., Luna, M., and Garca, A. (2023b). Livelihood capitals and opportunity cost for grazing areas restoration: a sustainable intensification strategy in the ecuadorian amazon. Animals 13:714. doi: 10.3390/ani13040714
UNFCCC (2010). Calculation of the Number of Sample Plots for Measurements Within A/R CDM Project Activities. Available online at: https://cdm.unfccc.int/methodologies/ARmethodologies/tools/ar-am-tool-03-v2.1.0.pdf (accesed March 25, 2023).
van Breugel, M., Ransijn, J., Craven, D., Bongers, F., and Hall, J. S. (2011). Estimating carbon stock in secondary forests: decisions and uncertainties associated with allometric biomass models. For. Ecol. Manage. 262, 1648–1657. doi: 10.1016/j.foreco.2011.07.018
Vasco, C., Torres, B., Jcome, E., Torres, A., Eche, D., and Velasco, C. (2021). Use of chemical fertilizers and pesticides in frontier areas: a case study in the northern Ecuadorian Amazon. Land Use Policy 107:105490. doi: 10.1016/j.landusepol.2021.105490
Vasco, C., Torres, B., Pacheco, P., and Griess, V. (2017). The socioeconomic determinants of legal and illegal smallholder logging: Evidence from the ecuadorian amazon. Forest Policy Econ. 78:133–140. doi: 10.1016/j.forpol.2017.01.015
Vera, R. R., Cota-Snchez, J. H., and Olmedo, J. E. G. (2017). Biodiversity, dynamics, and impact of chakras on the ecuadorian amazon. Journal of Plant Ecology 12:34–44. doi: 10.1093/jpe/rtx060
Vizuete-Montero, M., Carrera-Oscullo, P., Barreno-Silva, N. D. L. M., Snchez, M., Figueroa-Saavedra, H., and Moya, W. (2024). Agroecological alternatives for small and medium tropical crop farmers in the ecuadorian amazon for adaptation to climate change. Agric. Syst. 218:103998. doi: 10.1016/j.agsy.2024.103998
Wungshap, H., Paul, A., Bordoloi, R., Das, B., Sahoo, U. K., Tripathi, S. K., et al. (2023). Carbon stock assessment in natural forests and plantations using geo-informatics in manipur, northeast india. Agronomy 13, 1–17. doi: 10.3390/agronomy13082023
Zambrano, M., Robles, M., Izuriea, S., Torres, B., Bravo, C., and Martinez, C. (2019). “Atlas geogrfico de la provincia de pastaza,” in Technical Report, Gobierno Provincial de Pastaza, The Nature Conservancy. Santa Clara: Universidad Estatal Amaznica y Conservacin Internacional.
Zanne, A. E., Lopez-Gonzalez, G., Coomes, D. A., Ilic, J., Jansen, S., Lewis, S. L., et al. (2009). Data from: Towards a worldwide wood economics spectrum [Dataset]. Dryad. doi: 10.5061/dryad.234
Keywords: Natural Climate Solutions, agroforestry, above ground carbon biomass, indigenous territories, climate change mitigation
Citation: Álava-Núñez P, Torres B, Castro M and Robles M (2025) AGB carbon stock analysis in the Indigenous agroforestry of the Ecuadorian Amazon: Chakra and Aja as Natural Climate Solutions. Front. For. Glob. Change 8:1513140. doi: 10.3389/ffgc.2025.1513140
Received: 22 October 2024; Accepted: 11 March 2025;
Published: 15 April 2025.
Edited by:
Sumit Chakravarty, Uttar Banga Krishi Viswavidyalaya, IndiaReviewed by:
David Ellison, University of Bern, SwitzerlandCopyright © 2025 Álava-Núñez, Torres, Castro and Robles. This is an open-access article distributed under the terms of the Creative Commons Attribution License (CC BY). The use, distribution or reproduction in other forums is permitted, provided the original author(s) and the copyright owner(s) are credited and that the original publication in this journal is cited, in accordance with accepted academic practice. No use, distribution or reproduction is permitted which does not comply with these terms.
*Correspondence: Paulina Álava-Núñez, cGF1bGluYS5hbGF2YW51bmV6QGdtYWlsLmNvbQ==
†ORCID: Marco Robles orcid.org/0000-0002-0940-3039
Disclaimer: All claims expressed in this article are solely those of the authors and do not necessarily represent those of their affiliated organizations, or those of the publisher, the editors and the reviewers. Any product that may be evaluated in this article or claim that may be made by its manufacturer is not guaranteed or endorsed by the publisher.
Research integrity at Frontiers
Learn more about the work of our research integrity team to safeguard the quality of each article we publish.