- 1Klamath Center for Conservation Research, Orleans, CA, United States
- 2Department of Fish, Wildlife and Conservation Biology, Colorado State University, Fort Collins, CO, United States
- 3Trinity College, Hartford, CT, United States
- 4Center for Landscape Conservation Planning, University of Florida, Gainesville, FL, United States
Conservation of old-growth forests and their biodiversity and climate benefits requires coordinated actions across spatiotemporal scales, including restrictions on harvest of old and mature trees and longer-term landscape planning for old-growth recovery. In 2024, the US government drafted a National Old-Growth Amendment (NOGA) to enhance consideration of old growth in planning. The amendment was never finalized due to a change in administrations. However, the debate associated with the proposed NOGA remains relevant for several reasons. The key points of debate also underpin discussion regarding ongoing US regional policy initiatives such as amendments to the Northwest Forest Plan (NWFP). The NOGA policy debate illustrates questions that should inform US national old-growth initiatives under future administrations. Lastly, the debate highlights challenges common to such initiatives globally as nations implement the Kunming-Montreal Global Biodiversity Framework. Although the proposed NOGA provides a useful foundation for future initiatives, more attention must be paid to the policy implications of variation in the context in which old growth occurs across ecosystems. New initiatives must learn from regional efforts, including the NWFP’s insights that landscape design including reserves is essential for conservation of species, services, and processes associated with old-growth ecosystems. Reserves, conceived as places where extractive uses are restricted but beneficial human activities are supported, are compatible with strategies for ecosystem restoration and Indigenous-led conservation. An approach that builds on the NOGA’s adaptive strategies can form a foundation for long-term conservation of forest ecosystems by protecting climate refugia, addressing barriers to connectivity, and enhancing monitoring capacity. Ecosystem-based standards are needed to ensure protection of mature forest so recruitment into the old-growth stage shifts ecosystems towards historic proportions of old growth. In addition to clarifying goals regarding ecological integrity, comprehensive policy must incorporate goals for recovering at-risk species based on relationships across scales of biodiversity between forest habitat and species viability. Land management agencies need to articulate a long-term vision for recovery of depleted ecosystem elements (including both old growth and naturally disturbed younger stands) via designation of large areas anchored by remaining old-growth stands, surrounded by areas managed for recovery of ecological integrity, native biodiversity, and ecosystem services.
1 Introduction
Halting and reversing global loss of biodiversity requires conservation strategies and policies coordinated across multiple spatial scales and levels of biological organization (IPBES, 2019). Ecological theory provides insights that can help society address such complex conservation challenges by understanding key mechanisms underlying the patterns and processes observed in ecological systems (Twombly and Hastings, 2022). Old trees and old-growth forests are key features supporting biodiversity, ecological integrity, and ecosystem services whose conservation exemplifies the challenges of coordinating actions across scales (Lindenmayer et al., 2012; Mackey et al., 2014; Lindenmayer and Laurance, 2017).
Various definitions for old-growth forest have been proposed based on tree age or size but also the unique characteristics associated with older stands (a spatial scale intermediate between tree and landscape, representing areas of relatively uniform site conditions; McElhinny et al., 2005) that maintain an array of native species, processes, and functions (DellaSala et al., 2022b; Barnett et al., 2023; USDA, 2024b). By any definition, old-growth forests are rare in the US (<7% of the forested landscape in the conterminous US; Barnett et al., 2023). Ecologically, large old trees are “keystone structures,” defined as distinct spatial structures having a disproportionately large effect on the presence and abundance of other species (Lindenmayer and Laurance, 2017). The structural complexity of old trees adds both vertical and horizontal heterogeneity to a forest stand and creates diverse niches that enhance and maintain species diversity (Tews et al., 2004; Lindenmayer and Laurance, 2017). Old-growth forests typically support a wide distribution of tree sizes and a species-rich understory of younger-aged trees and canopy gaps reflecting recovery from natural disturbances such as wildfires, windstorms, and insect outbreaks (Swanson et al., 2011; Johnson et al., 2023).
In 2024, in response to concerns about old-growth forest loss and forest degradation (i.e., forests whose ecological processes are diminished or severely constrained; Ghazoul et al., 2015) on federal lands, the US government drafted a national strategy [the National Old-Growth Amendment (NOGA)] to enhance consideration of old-growth conservation in planning by the USDA Forest Service, the primary US federal agency managing forested lands (USDA, 2024a). The amendment was never finalized due to a change in administrations and consequent abrupt policy shift. However, the science and policy debate associated with the proposed NOGA remains highly relevant for several reasons. First, the key points of debate also underpin discussion regarding ongoing US regional forest policy initiatives such as amendments to the 1994 Northwest Forest Plan (NWFP). Second, the NOGA policy debate illustrates questions that should inform US national old-growth initiatives under future administrations. Lastly, the debate highlights challenges common to such initiatives globally as nations work to implement the recently developed Kunming-Montreal Global Biodiversity Framework (see Table 1 for key questions addressed in the essay).
We address three limitations that are common to biodiversity-related policy initiatives in the US and elsewhere. First, insufficient attention is often paid to ecological context, in terms of the interaction of ecological processes across multiple scales but also the policy implications of variation among ecosystems. The structural and ecological characteristics of old-growth forests differ substantially among forest types and associated tree species, spanning a range from closed-canopy forests to open-canopied woodlands and savannas (Noss, 2012), which makes formulation of universal management strategies and conservation policies challenging. A key priority for any national policy will be balancing effective national guidance while allowing for flexibility to address the context in which old growth occurs across a diverse range of ecosystems. We explore an example of how the relative emphasis on “active” (e.g., mechanized vegetation management or thinning) versus “passive” (non-mechanized, including prescribed fire) management of old growth may vary among ecosystems.
Second, policy initiatives often lack institutional memory and fail to learn from previous efforts. For example, the current US administration immediately removed both science and policy documents associated with the NOGA from government websites. Peer-reviewed journal articles such as this essay play an important role in counteracting this attempted erasure of previous efforts. To ground the NOGA in historical context, we describe how the evolution of forest policy in the US Pacific Northwest (PNW) illustrates the challenges faced when developing old-growth conservation strategies. Many of the ecological concepts underlying the 1994 Northwest Forest Plan (NWFP) are relevant to old-growth conservation in other temperate forest types in the US and elsewhere. Lastly, US biodiversity policy would benefit from greater awareness of the global and international context. We compare the challenges to devising effective US old-growth conservation policy across scales (Table 2) with analogous issues in Canada and globally as nations implement the recently adopted Kunming-Montreal Global Biodiversity Framework (KMGBF) (CBD, 2023). Although political winds may shift, the themes we explore will remain fundamental to any future effort to develop precautionary and aspirational policies that ensure long-term sustainability of our lands and waters (Bell-James and Watson, 2025).
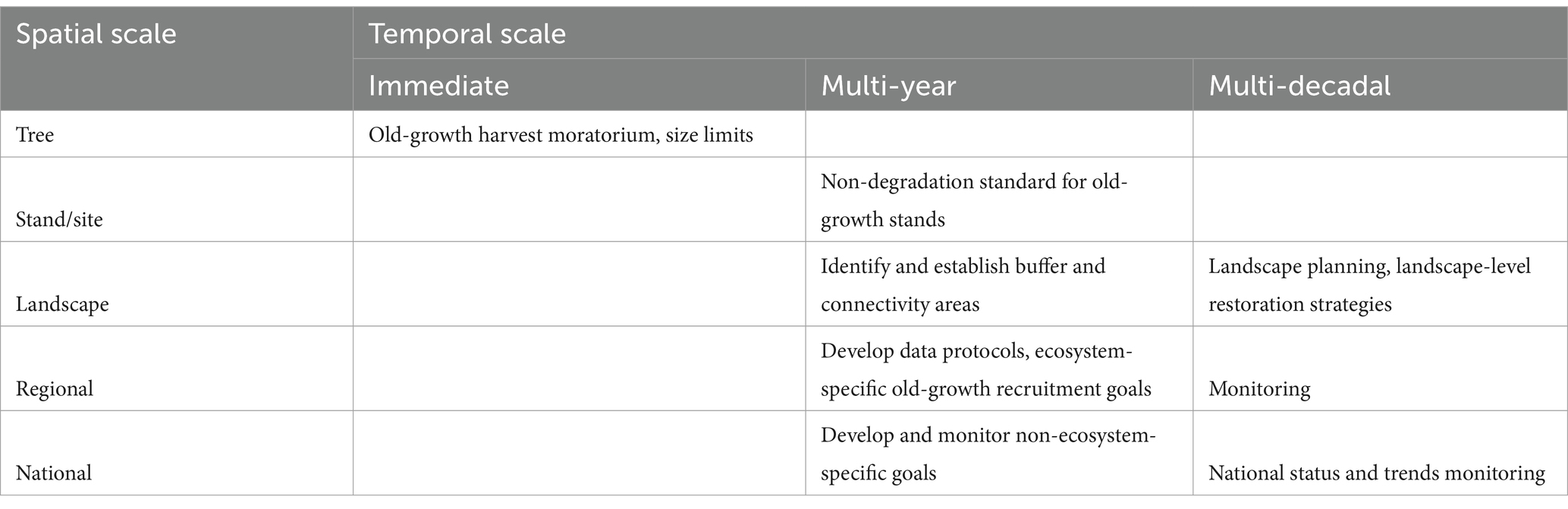
Table 2. Examples of correspondence between spatial and temporal scales of policy action for old-growth conservation.
2 Parallels between US and global old-growth conservation policy questions
Despite global focus on the general goal of protecting old trees and old-growth forests (along with primary forests and intact forest landscapes; Watson et al., 2018), many specific old-growth policy issues remain unresolved in the US and other nations (Lindenmayer et al., 2014). The issues that emerged in the context of efforts to develop a US national old-growth policy are also evident in similar policy efforts in other nations. A key issue is the tension between the need for immediate decision-making in the face of acute threats to and loss of old growth and the necessity for long-term planning for ecosystem integrity, recovery and restoration.
For example, in the province of British Columbia, Canada, which encompasses extensive areas of coastal and interior old-growth forest (DellaSala et al., 2021), temporary deferral of logging has been implemented in a subset of priority remnant old-growth stands. The provincial government implemented these “emergency” deferrals in response to advocacy by communities, Indigenous peoples (termed First Nations in Canada), and non-governmental organizations, and in response to the threat of federal intervention to protect at-risk species [Northern Spotted Owl (Strix occidentalis caurina) and mountain caribou (Rangifer tarandus caribou)] under the Canada’s Species At Risk Act (SARA). Concurrently, longer-term regional planning processes have been initiated in coordination with First Nations but have progressed at a slow pace in comparison to ongoing loss of old-growth forest (Carroll and Ray, 2021). Coordinating immediate stand-level and long-term landscape-level strategies is challenging in Canada as in the US.
Unlike the US, Canada is party to the Convention on Biological Diversity (CBD, 2023). In 2022, parties to the CBD adopted the Kunming-Montreal Global Biodiversity Framework (KMGBF), consisting of goals, targets, and indicators designed to reverse the decline of biodiversity and ecosystem services via transformative societal changes that also addresses equity issues (CBD, 2023). The US, the only nation not a formal party to the CBD, nevertheless has endorsed many elements of the KMGBF, as well as related international pledges and agreements such as the Glasgow Leaders Forest Declaration and Paris Climate Agreement (White House, 2021, 2022).
Although provinces control most land-use decisions under Canada’s federalized governance structure, British Columbia’s old-growth deferrals and regional planning have received support from the federal government, in part to fulfill national-level commitments to KMGBF targets. Understanding the goals, targets, and indicators that make up the KMGBF is key in evaluating efforts by Canada and other CBD parties to implement meaningful old-growth policy. Although the KMGBF commits nations to halting the extinction of species (analogous to the mandates of the US Endangered Species Act and Canada’s SARA), many KMGBF targets focus on concepts such as ecological integrity that remain poorly defined (analogous to the NOGA; Carroll et al., 2022).
KMGBF target 1 calls for expansion of biodiversity-aware spatial planning to halt the loss of “areas of high biodiversity importance, including ecosystems of high ecological integrity” (CBD, 2023). KMGBF targets 2 and 3, respectively, establish goals for restoring the ecological integrity of at least 30% of degraded areas and establishing protected and conserved areas (the “30×30” commitment to protect 30% of the globe by 2030) in landscapes of particular importance for biodiversity and ecosystem services. These three targets in turn support KMGBF target 4’s biodiversity-element-based objectives to halt anthropogenically caused species extinctions and significantly reduce extinction risks. KMGBF target 8 (promoting climate adaptation and mitigation), and target 10 (ensuring sustainable forestry practices) are also highly relevant. Lastly, several targets focus on policy and planning processes: target 14 calls for better integration of biodiversity conservation into policy, target 21 for strengthening monitoring and data from both Western and Indigenous knowledge systems, and target 22 for ensuring participation of Indigenous peoples and historically unrepresented groups in decision-making.
The history of the CBD suggests that nations have struggled to implement individual targets in a holistic manner (Hughes and Grumbine, 2023). For example, although target 3 and its 30% goal for protected areas (30 × 30) has received most attention, protected areas are most effective when situated within sustainably managed landscapes (Carroll and Noss, 2022; Carroll et al., 2024). This suggests that global and national strategies focused on conservation of keystone ecosystem elements, such as old trees, should be embedded in broader landscape-scale strategies supporting viability of threatened species and ecosystem restoration efforts (Lindenmayer and Franklin, 2002; Carroll et al., 2022). Debate over expansion of protected areas in relation to Indigenous land rights has also been a key issue in implementation of the KMGBF and previous CBD targets (ICE, 2018). An inclusive paradigm of “land relationship” planning, guided by integration of Western and traditional knowledge, as set forth in KMGBF targets 21 and 22, will be a key element of such strategies (ICE, 2018).
3 Lessons from the evolution of old-growth conservation policy on US federal lands
In the US, old-growth forest conservation, especially on federal land, has historically been the subject of extensive research and policy debate (Spies et al., 2019; DellaSala et al., 2022b; Johnson et al., 2023). We can categorize historic and current policy into three types of strategies (Table 3). First, procedural requirements can be adopted to promote consideration of old-growth conservation during planning processes but not be referenced to specific areas of the landscape. Secondly, stand-level old-growth characteristics may be conserved by restricting logging of trees above a certain size or by protecting old growth at the stand scale. Thirdly, landscape-level conservation strategies can be implemented to constrain or prohibit certain management actions (e.g., timber harvest) within designated areas (e.g., reserves). In theory, these three approaches are complementary but have more commonly been implemented separately.
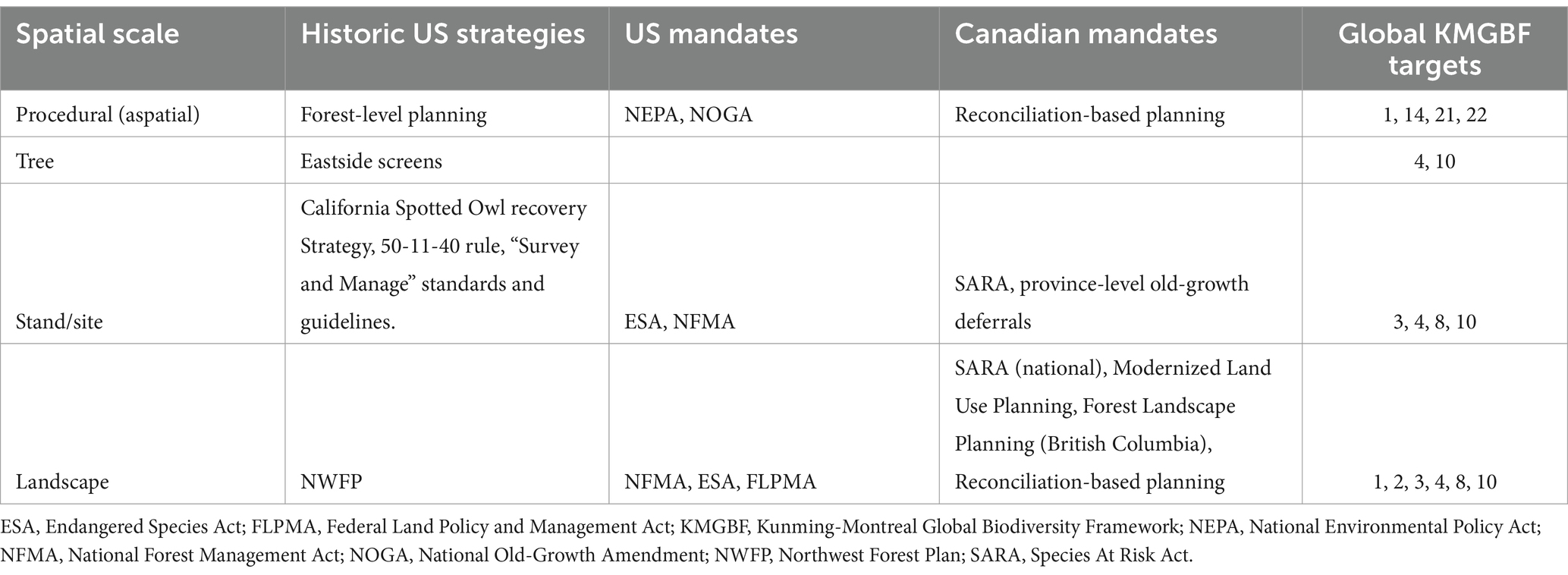
Table 3. Spatial scales of implementation of old-growth conservation, including past and current old-growth management policies and corresponding laws and mandates.
For US federal land management agencies, the procedural approach has the longest history. The USDA Forest Service (henceforth Forest Service), the primary federal agency charged with management of forest ecosystems, has historically operated under a mandate of “multiple use” management in which extractive and non-extractive land uses would in theory be balanced in local project-level decisions by managers to meet Forest-level objectives. However, in the period from the 1950s to 1990s, management objectives emphasized timber harvest largely to the exclusion of other ecological requirements (Johnson et al., 2023). By the 1990s this approach became socially and legally untenable, particularly in the US Pacific Northwest (PNW), due to incompatibility with legal mandates to maintain viable populations of old-growth dependent species. Public acceptance of the multiple-use approach also diminished due to increased awareness of the ecological, recreational, and aesthetic values provided by old-growth forests (Johnson et al., 2023).
In response to the inadequacy of existing procedural safeguards to ensure viability of at-risk species, the Forest Service implemented new restrictions on the harvest of large, old trees. For example, the 1994 “Eastside Screens” sought to limit the harvest of old-growth trees by restricting logging on federal lands of trees greater than 53 cm dbh (diameter at breast height) east of the Cascade crest in Oregon and Washington. Initial conservation strategies for the Northern Spotted Owl also sought to maintain habitat conditions suitable for dispersal between stands of old forest by imposing tree- and stand-level constraints on timber harvest (e.g., the “50-11-40 rule” to retain 50% of every quarter-township (2,300 ha area) in stands with a minimum average size and canopy closure) (Thomas et al., 2006; Noon and McKelvey, 1996).
Ultimately, size-based and stand-level guidelines were inadequate to address the cumulative effects of past logging practices and the subsequent regional decline in old-growth ecosystems and associated species of concern (Johnson et al., 2023). In 1994, multiple federal land management agencies developed the Northwest Forest Plan (NWFP) to guide management of ~100,000 km2 of federal lands in the PNW (Spies et al., 2019; Johnson et al., 2023). Two aspects of the NWFP were comparatively novel for US land management planning. First, the NWFP coordinated actions of 10 federal agencies across 17 Forests, plus dozens of areas managed by other federal agencies. Second, broad-scale management direction sought to maintain viability of species and sustain ecosystem services by partitioning the landscape into distinct land-use designations wherein different management objectives would be emphasized (e.g., Late Successional Reserves (LSR) and Riparian Reserves received greater protection, whereas Matrix areas between Reserves provided for the majority of timber harvest) (Murphy and Noon, 1992; Noon and Blakesley, 2006; Johnson et al., 2023). Conserving entire landscape mosaics made up of old-growth reserves surrounded by a multi-aged forest matrix provided for dispersal among reserves and increased the likelihood of persistence of old-growth-associated species (Harris, 1984; Molina et al., 2006). Landscape planning that resulted in “lines on a map” also created greater transparency in what management the public could expect to see in different areas, thus potentially increasing societal buy-in and reducing litigation around individual projects.
US laws, including the National Forest Management Act (NFMA), historically required the Forest Service and other federal agencies that manage older forests to maintain viable populations of old-growth-associated species (Johnson et al., 2023). Successful legal challenges to forest management in the PNW focused on flagship species such as the Northern Spotted Owl, Marbled Murrelet (Brachyramphus marmoratus), and at-risk salmonids (Oncorhynchus spp.). The NWFP sought to resolve litigation and recover these species by means of a landscape-level reserve design (Thomas et al., 2006). The NWFP’s focus was not limited to old growth but sought to conserve late-successional stands more broadly, in part because Northern Spotted Owls select mature forests with residual large old trees (Noon and McKelvey, 1996; Johnson et al., 2023).
The NWFP was both a species- and ecosystem-focused strategy, combining viability modeling of individual species with landscape planning to conserve ecological integrity and ecosystem services (Noon and McKelvey, 1996). At the time, public attention was particularly focused on cumulative effects of timber harvest and associated road construction on water quality for downstream communities and at-risk salmonid populations. At the watershed scale, old-growth forests maintain hydrological cycles critical to sustaining aquatic and other biodiversity elements (Johnson et al., 2023). The NWFP’s Aquatic Conservation Strategy was designed to ensure protection and restoration of water quality and associated aquatic biodiversity at an appropriate scale. The Strategy prioritized protection of Key Watersheds that retained high-quality habitat and healthy fish populations, with the long-term goal of restoring a NWFP-wide network of watersheds that supported viable populations of aquatic species (Reeves et al., 2006). The NWFP’s regionally coordinated landscape planning model informed subsequent ecosystem-focused plans including the Desert Renewable Energy Conservation Plan (DRECP) that coordinated biodiversity conservation in southeastern California to minimize conflicts with expansion of energy infrastructure (Kreitler et al., 2015).
4 What can we learn from the strengths and shortfalls of the Northwest Forest Plan?
In the three decades since the NWFP was developed, scientists and policymakers have sought to assess the degree to which it succeeded or fell short of its objectives (Spies et al., 2019). This assessment is complicated by the fact that on-the-ground implementation of the NWFP inevitably varied across the 17 Forests over time (Johnson et al., 2023). The NWFP’s ecological objectives included protecting and restoring late-successional forest and associated species and ecosystem services. A strategic plan focused on long-term ecological recovery and integrity is a cost-effective approach to sustaining these species and others on the landscape. The NWFP succeeded in significantly slowing the loss of late-successional forest to timber harvest on federal lands. From the mid-1950s to 1989, annual timber sales on national forests exceeded 10 billion board feet. From 1989 onward federal timber sales have been <3 billion board feet (Johnson et al., 2023: Fig. 11.1). However, Northern Spotted Owls, and other late successional species, have not shown strong increases in either abundance or geographic distribution (Spies et al., 2019). In the case of the Northern spotted owl, this is largely attributable to range expansion by the barred owl (Strix varia) (Franklin et al., 2021). For other species, threats beyond the control of federal land managers (e.g., harvest on private timber lands, climate change) and the slow pace of successional change suggest that any responses will be long-term (Spies et al., 2019).
Most criticism of the NWFP as implemented focused on the practical limitations on the ability of the Forest Service to facilitate timber harvest due to the increased procedural hurdles created by the NWFP. To some extent, these are inherent in the agency’s new requirement to consider factors such as species viability and ecosystem services that were previously ignored as externalities. The extent of these new procedural hurdles (e.g., surveys for rare species; Molina et al., 2006) and other factors contributed to several outcomes. Volume of timber harvest and consequent economic benefits did not meet the NWFP’s projections (USDA, 2024c). Some of the NWFP land management zones, particularly the Adaptive Management Areas (AMA), did not meet their intended purpose of facilitating assessment of novel management approaches (Spies et al., 2019). Lastly, some authors concluded that the existing reserve-based strategy (e.g., the NWFP LSR) created hurdles to fuels reduction via mechanical thinning and commercial logging, and that these management activities are needed to avoid loss of biodiversity and ecosystem services due to high-severity fires (Spies et al., 2018, 2019). Other studies have reached different conclusions, and several of these issues remain foci of continued scientific and societal debate (Dellasala et al., 2022a).
At the same time as the NOGA was in development, the Forest Service also began the process of amending the NWFP (USDA, 2024c). The stated goals of the NWFP amendment include facilitating active management for addressing fire disturbance and climate change, incorporating Indigenous knowledge, and ensuring a more predictable supply of timber from federal lands (USDA, 2023; USDA, 2024c). The management alternatives developed in the NWFP Amendment process differ in the degree of emphasis placed on “active” (e.g., mechanized vegetation management or thinning) versus “passive” (non-mechanized, including prescribed fire) approaches, and in the priority given to increasing timber harvest volume. This contrast resonates with the broader debate over national old-growth policy that was evident during development of the NOGA.
5 How can a national old-growth policy apply consistent scientific principles across a range of ecosystem contexts?
In the context of this debate, we address whether a landscape-level conservation strategy grounded in a system of reserves remains appropriate, both in the PNW and more generally to the diversity of old-growth forest types occurring across all Forest Service lands We discussed the NWFP at length because we believe that such regional landscape-scale strategies that are anchored by reserves and combine species- and ecosystem-focused elements should be the building blocks of any future US national or regional old-growth conservation strategy. The example of the hurdles to conserving the owl is relevant beyond the specific species in that it exemplifies the challenges of linking species viability and ecosystem integrity. Although the NOGA process itself was ultimately inconclusive, we believe that the debate it engendered remains relevant, and that key elements of the NOGA and the NWFP can be built upon under future administrations to create more effective and aspirational policy.
The NOGA effort built on previous US Executive Orders focused on old-growth conservation and climate change mitigation and adaptation (White House, 2021, 2022). The NOGA included the goal of ensuring long-term resilience and ecological integrity of old-growth forests under “rapidly changing climate conditions” and also sought to establish a clearer role for Indigenous knowledge and tribal leadership in decision-making (USDA, 2024a). The NOGA was primarily focused on amending the procedures by which management planning occurs at the level of the Forest Service’s subunits (the National Forests, averaging ~5,000 km2 in size), to ensure “a consistent management framework for conserving, stewarding, recruiting and monitoring old-growth forests” (USDA, 2024a). The amendment was issued in draft form in June 2024 (USDA, 2024a), but was never finalized as a formal regulation due to the change in US administrations in January 2025.
The NOGA favored procedural changes over prescriptive requirements and limitations on agency discretion, including decisions regarding timber harvest. In place of a nationally coordinated and mapped landscape strategy, the NOGA called for each of the 122 Forests (or groups of adjacent Forests) to develop an “adaptive strategy for old-growth forest conservation.” These adaptive strategies would set quantitative goals for old-growth forest conservation and determine if a need exists for changes in management practices to reach these goals. Although the NOGA provided little detail on what the adaptive strategies would entail, the “adaptive strategy” approach has potential and could be built upon in future policy initiatives that are grounded more clearly in ecological principles.
There are previous examples of where the Forest Service implemented a national strategy based on dividing the landscape into zones with different management restrictions. The 2001 National Roadless Area Conservation Rule added prohibitions on road construction and most timber harvesting on 237,000 km2 of inventoried roadless areas nationwide that were > 2023 ha (5,000 acres), to maintain these areas’ unique ecological and recreational values (Talty et al., 2020). A more locally driven approach, in which individual Forests would adopt procedures to conserve their roadless areas, was initially considered for the 2001 Rule but rejected because potentially high variance in conservation strategies among Forests would undermine consistent application of the rule. A “top-down” approach similar to the 2001 Roadless Area Conservation Rule that delineated management zones at the national scale was rejected by the NOGA’s authors because they concluded that “old-growth forests are dynamic systems and the intent is not to manage all of these areas in the same manner”; and that “strictly reserving mature and old-growth forest may not always ensure that it is protected from future losses” (USDA, 2024a).
We agree that delineating priority areas for old-growth conservation nationally is more challenging than delineating roadless areas. There is wide variation among regions in the structural role of old trees in ecosystems. Although the closed-canopy forest of the coastal PNW is often used to illustrate “classic” old-growth forest, many old-growth “forests” are more accurately categorized as woodlands or savannas (Veldman et al., 2015). For example, in eastside Cascades dry pine (Pinus ponderosa) and mixed conifer forests, large remnant trees are often scattered in small clusters across different age classes reflecting the consequences of recurring fires of mixed severity (Hessburg et al., 2015).
Although old-growth forests in many regions represent the terminal age class of forest succession (Powell, 2012), the succession-to-climax model does not apply to all ecosystems. For example, longleaf pine (Pinus palustris) and similar savanna ecosystems in the southeastern US, which were once thought of as a “fire climax,” are actually non-successional ecosystems, with different age classes of one dominant tree species but no discernable successional stages leading up to a climax state (Noss, 2018). Regeneration is limited to canopy gaps among patches of older trees due to higher temperatures of surface fires in areas with abundant fallen needles and potentially other factors such as root competition for water or nutrients (Ellair and Platt, 2013). Old trees in these savannas are keystone structures that facilitate development of herbaceous biodiversity by converting lightning strikes to surface fires (Platt et al., 1988).
The key challenge in designing a successful national old-growth conservation strategy lies in balancing the flexibility to sustain and restore old-growth forest ecosystems across the varying ecological context of diverse forest types with a strong national policy that ensures consistency and effectiveness. National guidance must frame a vision for ecological restoration and recovery that shifts management away from past practices that ignored cumulative effects and responded primarily to socioeconomic pressures that promote unsustainable logging practices including the harvesting of old-growth stands.
Federal land management agencies do not have a strong record of assessing cumulative effects absent coordinated policy direction such as seen in the NWFP. In the past, most National Forests in the PNW failed to comply with similar legal requirements that ultimately led to the lengthy litigation resulting in the NWFP. This failure is the result of multiple factors including: (1) an interpretation of the agency’s multiple-use mandate as favoring extractive uses, (2) a “philosophical” bias and educational focus in forestry to favor active management; (3) a desire to maximize agency discretion; (3) bureaucratic and internal economic incentives that tie budgets to timber volume (e.g., Knutson-Vandenberg Act funds); and (5) pressure from external industry groups that receive economic benefits from logging on federal lands (DellaSala et al., 2022a).
Although our primary focus in this essay is on landscape-level strategies, these must be combined with strong protections for old-growth at the stand and site level (Table 2). The NOGA fell short in this area: instead stating that “[t]here is no requirement that [old-growth forests] continue to meet the definition of old-growth when managed for the purpose of proactive stewardship” (USDA, 2024a). The current scarcity and long regeneration timelines of old trees make old-growth forests inherently vulnerable to cumulative impacts from active management (DellaSala et al., 2022a, 2022b; Kellett et al., 2023). Comprehensive old-growth conservation policy can address this vulnerability in the short term via policies acting at the relevant scale of trees and stands: (1) an immediate moratorium on commercial harvest of existing old-growth trees, and (2) a standard (i.e., a mandatory constraint on project and activity decision-making) to ensure activities within old-growth stands to not degrade or impair old-growth condition, similar to standards included in the NWFP in relation to LSRs and Key Watersheds.
The NOGA also fell short in meeting the challenge of crafting consistent national policy for a diversity of ecosystem types. The NOGA included broad exemptions to its procedural requirements where managers judged that these were “not relevant or beneficial to a particular forest ecosystem type” (USDA, 2024a). We agree that there are important contrasts between the ecological context of old-growth conservation in different regions of the US. Many of these arise from contrasts in disturbance regimes, recovery trajectories, and the role of large trees and other vegetation layers in supporting biodiversity. However, the ecological value of old growth is evident even in those ecosystems that do not conform to classic successional models. In every ecoregion it is critical to allow connected areas of intact forests to evolve and adapt over space and time. Effective national old-growth policy must consequently avoid overly broad discretion at the level of individual Forests that may undermine the consistency of the strategy and contradict national and international goals to retain ecological integrity and climate-regulating ecosystems.
6 Are reserve-based landscape strategies relevant across ecosystems?
We propose that a landscape-level strategy analogous to the NWFP that identifies, protects, and connects old-growth trees and forests is broadly feasible and applicable across the diversity of forest types that support old growth in a range of ecological contexts and across multiple forest, woodland, and savanna communities in the United States and elsewhere.
Among the goals that the NOGA described for its proposed adaptive strategies were ensuring adequate amounts, representativeness, redundancy, and connectivity of old-growth forest areas, as well as areas that function as fire refugia and climate refugia (areas relatively buffered from the effects of a changing climate; Keppel et al., 2024). Because these criteria inherently involve mapped landscape elements, this suggests the need for landscape planning such as underpinned the NWFP. Commonalities evident across US federal forestlands include the need for coordination among multiple land management units (e.g., Forests) within a region, a key element of the NWFP.
Coordinated landscape strategies also enhance the conservation of ecosystem services. The NWFP’s Aquatic Conservation Strategy approach is also broadly relevant across ecosystem types. The Aquatic Conservation Strategy is a zoning-based strategy for conserving water quality and associated aquatic species, based on prioritizing coordinated conservation actions in Key Watersheds identified based on their ability to contribute to viability of populations of salmonids and other at-risk aquatic species (Reeves et al., 2006).
Although landscape plans do not necessarily involve reserves (i.e., areas where most forms of extractive use are restricted), “lines on a map” do by their nature limit the discretion of local managers. The NOGA’s emphasis on the widespread need for “proactive stewardship” suggests an aversion to establishment of designated landscape units with constraints on allowable actions (e.g., similar to the NWFP’s LSR), in favor of an approach that permits intensive management and resource extraction, including commercial thinning and other timber harvest, wherever local managers deem it appropriate (Spies et al., 2018, 2019). This tension between reserves and management constraints is also key to the debate surrounding proposed amendments to the NWFP (USDA, 2024c).
In the three decades since development of the NWFP, there has been increasing recognition of the dynamic nature of forest ecosystems resulting from both historical disturbance processes and novel stressors (e.g., anthropogenic climate change, invasive species) (Newman, 2019). This awareness has led some to question whether an approach that uses “lines on a map” to encourage or prohibit certain management practices is an appropriate and adequate management response to highly dynamic ecosystems (Spies et al., 2019). The effects of climate change, especially on increasing severity and frequency of fire disturbance, had led some authors to suggest moving away from the 1994 NWFP’s reserve-based strategy (Spies et al., 2018, 2019).
In many respects, however, climate change and associated extreme events makes landscape zoning and reserves even more relevant (DellaSala et al., 2015). Conservation of old-growth landscapes is increasingly seen as an essential contributor to climate mitigation and stabilization, enhancing the resilience of forest ecosystems and the adaptation potential of their components (Faison et al., 2023a). Maintaining and recovering climate-stabilizing forest ecosystems is critical to long-term human well-being (Dasgupta, 2021; Makarieva et al., 2023). Comprehensive carbon accounting on federal lands, including a focus on the value of old forest and its soils as long-term carbon stores, also benefits from a zoning-based strategy for linking landscape pattern to ecosystem services (Law et al., 2021).
Protection and restoration of old-growth landscapes is an effective climate change mitigation strategy via carbon sequestration and long-term storage due to sustained carbon accumulation rates as trees mature (Krankina et al., 2012). The largest trees in old-growth forests in the eastern portion of the PNW represented just 3% of all stems yet stored 42% of above-ground carbon (Mildrexler et al., 2020). The generality of this pattern of increased carbon stocks with tree size and age has been documented globally (Stephenson et al., 2014). At the stand level, old-growth forests store 35 to 70% more carbon, including in the soils, compared to logged stands, highlighting their potential role in supporting natural climate solutions (Mackey et al., 2014; Law et al., 2021). Using matched plots, unmanaged forests in the Northeastern US were found to have higher carbon accumulation and increased structural complexity and tree diversity compared to managed forests (Faison et al., 2023a). Similarly, a recent broad-scale survey of New Brunswick (Canada), a province bordering the northeastern US, found strong positive correlations between locations with declines in above-ground carbon and habitats for old-forest bird species (which have lost >20% habitat over 35 years), and thus high congruence between biodiversity and forest carbon conservation goals (Betts et al., 2024). Landscape planning for carbon accumulation should be considered holistically with effects on other ecosystem- and climate-regulating cycles including methane (Wigley et al., 2024) and water (Farinacci et al., 2024).
The capacity of different portions of the landscape to act as climate refugia also supports a landscape planning strategy that identifies and prioritizes refugia (an element of the NOGA’s adaptive strategies) and connectivity among refugia (Carroll and Ray, 2021). Old-growth and mature stands, by creating microclimatic refugia, are ecosystem elements that inherently enhance the climate resilience of landscapes (Lesmeister et al., 2019). Fire disturbance frequency and severity differ widely across US forest ecosystems, but are typically influenced by landscape position, causing some stands to serve as transient or persistent topoclimatic fire refugia. Conservation of such refugia via a zoning-based strategy can enhance pyrodiversity (landscape-scale variation in fire severity and frequency) and promote landscape resilience (e.g., by furnishing seed sources during post-fire recovery) (Krawchuk et al., 2016, 2020). Compared to younger forests, old-growth forests are expected to show considerable inertia in the face of climate change (Noss, 2001). In some cases, forests with very long-lived trees may be able to survive centuries of unsuitable climate and the return of a more favorable regeneration environment.
Independent of fire disturbance patterns, protection of old-growth stands situated in microclimatic refugia resulting from landscape position (e.g., via cold air pooling) can also promote ecological resilience and adaptive potential (Morelli et al., 2020). Agency activities including timber harvest, wildfire suppression, and prescribed fire should incorporate refugia protection goals. However, implementing such refugia protection goals will require substantial effort by agencies to develop and validate maps of refugia potential and connectivity (Krawchuk et al., 2016; Stralberg et al., 2020; Keppel et al., 2024).
Finally, a strong case can be made that old-growth reserves are essential to recover and sustain populations of species dependent on late-seral forests. Principles of reserve design for at-risk species contributed significantly to the NWFP (Noon and McKelvey, 1996) and the Forest Service has strict legal requirements to sustain these species (Schultz et al., 2013). A reserve design is described according to the number, size and spacing of the reserves and requires lines on a map (Kukkala and Moilanen, 2013), In this context, reserves have two main roles (Margules and Pressey, 2000). First, forests within the reserve boundaries must have the compositional and structural attributes of high quality habitat, now or in the future. Second, reserves should exclude human actions that threaten the persistence of this biodiversity. Effective reserve-based planning must also acknowledge the need for management across administrative boundaries by ensuring that the matrix within which reserves are embedded is managed to sustain connectivity and ecological integrity (Carroll and Noss, 2022).
7 Can reserve-based landscape strategies be too restrictive? The nuanced role of mechanical vegetation management
The appropriate role of mechanical vegetation treatments in old-growth conservation is a key policy question that exemplifies how conservation strategies vary with ecological context across the diversity of forest ecosystems. The NOGA identified “proactive stewardship” as a key element of old-growth conservation, defining the term as vegetation management (including commercial timber harvest) that “promotes the quality, composition, structure, pattern, or ecological processes necessary for old-growth forests to be resilient and adaptable to stressors and likely future environments” (USDA, 2024a). A key motivation for proposed amendments to the NWFP has been the perception that the NWFP’s reserve strategy unduly restricts mechanical fuel treatments called for under the Forest Service’s recently developed Wildfire Crisis Strategy (USDA, 2022).
These assertions may overgeneralize the role of mechanical vegetation treatments across diverse ecological contexts. The appropriateness of vegetation management via commercial and non-commercial thinning depends on several factors, including the type of ecosystem, its fire and other disturbance ecology, the site-specific disturbance history, and current stand structure (Noss, 2018). In many cases there is a focus on the expected benefits of treatments without considering negative impacts, especially to old-growth and future old-growth forests (Kellett et al., 2023). Thinning of small-diameter understory trees (coupled with retention of old or large diameter trees) can be beneficial for biodiversity and ecosystem services, especially at the stand scale, in some forest types. For example, many pine (Pinus spp.) woodlands and savannas of the southeastern US have experienced declines in biodiversity due to fire exclusion and subsequent invasion by mesic hardwood tree species, which shade out the species-rich herbaceous layer (Noss, 2018). Restoration of these pine ecosystems may sometimes benefit from understory thinning, followed by prescribed or cultural burning practices. Among the potential benefits are reduced risk of severe fire and accompanying carbon loss and emissions (Hurteau et al., 2008), although the extent of such reductions is often overestimated (Stenzel et al., 2019). Cutting and removing hardwood species, especially those with relatively non-flammable litter, is often necessary before fire can be reintroduced, but successful fire restoration is then essential for recovery of biodiversity (Noss, 2018).
Effects of historic and ongoing fire exclusion, along with increased fire frequency and severity due to climate change, have also altered dry forest ecosystems of the western US (USDA, 2024c). The California Spotted Owl (Strix occidentalis) Conservation Strategy for the southern Cascades and Sierra Nevada sought to preserve large trees without dividing the landscape into zones of differing management emphasis, due to the perceived need for continued fuels reduction including commercial logging throughout the landscape (Verner, 1992). However, several factors qualify or limit the role of thinning as an ecological restoration tool in such contexts.
In ecosystems where fire is the dominant disturbance process, the appropriate role of thinning is as a pretreatment for prescribed burning to restore natural stand structure and groundcover biodiversity. In such cases, the objective is to segue to fire treatments alone as quickly as possible (Rickey et al., 2013). Thinning is not an optimal strategy for restoring historic fire regimes where fire can be introduced without thinning (DellaSala et al., 2022a). Thinning, although often an effective element of stand-level restoration in contexts such as southeastern pine savannas, is often less effective at addressing fire behavior or insect outbreaks at broad landscape scales (DellaSala et al., 2004; DellaSala et al., 2022a).
Given existing budgets and cultures of US land management agencies and ongoing scientific uncertainties, there are significant challenges to implementing landscape-scale thinning strategies in a “nature-positive” manner. Especially in mountainous regions, federal lands that retain old-growth stands are primarily areas costly to access and log, compared to previously harvested federal forested lands, flatter landscapes, and private timberlands with extensive road networks (DellaSala et al., 2022b). Subsequent to the initial harvest of primary forest, thinning of small trees is often not economically feasible unless remnant large old trees are harvested or funding is dedicated to restoration (e.g., “stewardship contracts” without a commercial logging component or additional road construction). Road construction to enable commercial thinning and fuels reduction may have significant long-term negative effects on soil and water cycles and aquatic species that are still poorly understood (Forman et al., 2003).
A broader spatial and temporal context and a more precautionary approach is needed when considering disturbance processes and discussing threats to old-growth ecosystems. The recent federal old-growth threat analysis focuses on fire and insect disturbance but mostly omits consideration of ongoing effects of historic deforestation and degradation, especially on non-federal ownerships within which federal forestlands are situated (USDA and USDI, 2024). Increasing disturbance frequency and severity (e.g., due to climate change) is a potential threat to some forest ecosystems, yet disturbance itself is an essential ecosystem process (Newman, 2019). Maintaining and restoring ecological integrity (a stated goal of the NOGA and Forest Service policy generally) require a dynamic and data-driven view of ecosystems that involves conserving key ecosystem processes such as fire and insect disturbances, which typically have effects distinctly different from anthropogenic disturbances such as logging (Swanson et al., 2011).
In ecosystems with a historic fire deficit (Ryan et al., 2013), old-growth conservation policies should incentivize working with naturally occurring wildland fire for ecosystem benefits under appropriate conditions by preserving and restoring landscape-scale pyrodiversity within a historic range of variability (Supplementary Table S1). This goal can be promoted by expanding opportunities for managed wildland fire and Indigenous cultural fire management, and by eliminating current restrictions on use of fire suppression funds for managed wildland fire (Stephens et al., 2016). Landscape planning can facilitate increased use of naturally occurring or prescribed fire (e.g., by designation of Strategic Fire Zones; North et al., 2024).
A comprehensive review of optimal management practices to restore beneficial fire regimes in different forest types goes beyond the scope of our paper. However, we note that there is greater societal and scientific consensus concerning positive effects of (1) fuels reduction around human communities, (2) changes in fire suppression strategies to facilitate managed wildland fire, (3) increased agency support for prescribed fire, and (4) restoration of Indigenous cultural fire practices that were historically suppressed by US land management agencies (Stephens et al., 2016; Spies et al., 2018; North et al., 2024). All four approaches are compatible with an “adaptive strategy for old-growth forest conservation” that includes reserves as a major component. Even in contexts such as the southeastern US where thinning may be initially necessary to open the canopy or midstory, successful fire restoration can avoid the need for repeated mechanized entry and allow implementation of a reserve-based strategy.
Support for Indigenous cultural fire practices forms part of our evolving concept of “reserves” as places where beneficial human activities are supported (Eisenberg et al., 2024). Indigenous communities hold diverse perspectives on appropriate “land relationship” frameworks that would require lengthy text to adequately review, and the economies of many Indigenous communities are supported by extractive industries including logging. However, Indigenous-led regional planning processes in Canada and elsewhere have designated extensive reserves where industrial activities are restricted to limit negative impacts on ecological and cultural resources (ICE, 2018). These examples demonstrate that reserve-based landscape conservation is compatible with Indigenous co-management and knowledge including cultural fire. Recent progress has been made at the global level in coordinating conservation goals with empowerment of Indigenous peoples via new paradigms for Indigenous Protected and Conserved Areas (IPCA) and “other effective area-based conservation measures” (OECM) (IUCN, 2019).
As in medicine, restoration treatments should be guided by the rule of “first do no harm” (Marler and Lindström, 2017). It is important to recognize the implicit human bias to “do more” and to systematically overlook opportunities to do less (Adams et al., 2021). Any emphasis on “proactive” vegetation management in policies such as the NOGA must be tempered by an acknowledgement that “passive” (i.e., non-mechanized) management or even “benign neglect” (Müller et al., 2010) may often be the most appropriate strategy. For example, the NWFP reserve strategy was developed for the coastal Pacific Northwest where many forest ecosystems historically experienced infrequent, broad-scale fires. As a result, restoration of these ecosystems is unlikely to require or benefit from mechanical vegetation treatment in the same way as xeric western forests (Davis et al., 2024).
Forest ecosystems of the northeastern US were historically structured by disturbance associated with wind and insect outbreaks (Sprugel, 1976). Although some authors have proposed the need for mechanized timber harvest to increase early-seral habitat in these ecosystems (e.g., Littlefield and D'Amato, 2022), there are persistent questions in estimating historical and current prevalence of these habitats (Kellett et al., 2023). There are also several factors that limit the utility of logging and clearing as a tool for landscape-scale ecological restoration. First, retention of a significant fraction of current mature stands is necessary to provide recruitment into the highly depleted old-growth stage (Moomaw et al., 2019; Foster, 2023). Second, mechanically harvested stands do not replicate early seral stands resulting from natural disturbance processes (Swanson et al., 2011). Mechanical harvest results in soil disruption and favorable conditions for invasive plants and species with negative impacts on forest regeneration or human health. Third, in many parts of the region, federal and state forest lands are embedded in a landscape of private commercial timberlands managed under a silvicultural strategy that harvests stands at early to mid-successional stages when their growth rate reaches a maximum and begins to decline (Trombulak, 2007).
8 What ecological concepts can inform a nationally coordinated and regionally contextualized old-growth policy?
The NWFP’s landscape planning approach was informed by foundational principles and concepts of conservation biology, including metapopulation dynamics, species-area relationships, and allometric scaling laws (Supplementary Table S1) (Noon et al., 2009). These principles describe how ecosystems are governed by universal patterns and processes that operate at the level of the individual organism and transcend species identities in shaping patterns of biodiversity. If we accept that the NWFP’s landscape-level planning approach is relevant to other regions, we need to evaluate how the ecological concepts and principles underpinning the NWFP can be applied elsewhere. These concepts and principles can be broadly divided into those that focus on species- or population-level and on ecosystem or landscape-level objectives, although some concepts fall into both categories (Table 4).
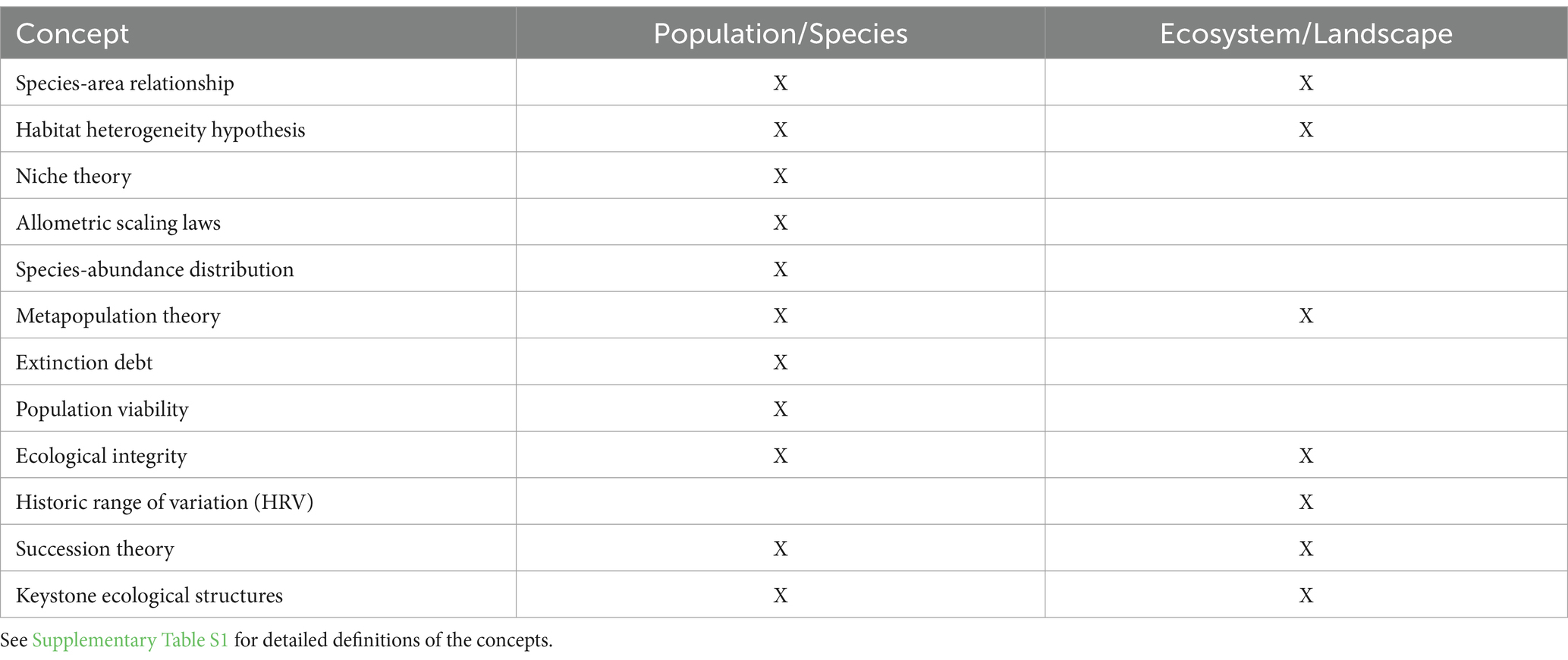
Table 4. Categorization of ecological concepts relevant to old-growth conservation discussed in section 8 of this essay.
The species-area relationship is a basic theory of the relationship between species richness (S) and habitat area (A) (MacArthur and Wilson, 1967; Harte et al., 2009). One explanation for the positive species-area relationship is based on the habitat heterogeneity hypothesis—that is, larger areas provide more habitat types. Due to their size and horizontal and vertical complexity, old-growth trees and stands have a substantial positive effect on landscape-scale species richness via their contribution to high habitat heterogeneity (Franklin et al., 1981; Clebsch and Busing, 1989).
The relevance of this theory to restoration objectives for old-growth habitat is illustrated by an example concerning the effects of loss of old-growth forests for late-successional obligate forest birds breeding in the Douglas-fir (Pseudotsuga menziesii) forest type. Pre-1900 estimates of the percent of the forested landscape in old-growth Douglas-fir were approximately 40% (Spies, 2009). The current estimate for federal lands in the region is 17% (USDA, 2024a). The species-area relationship predicts that this decline in the extent of old-growth in the Douglas-fir type would result in a ~ 36% decline in number of breeding bird species dependent on late successional forest, given estimates for c and z from Boecklen (1986). Although the species-area relationship is a highly simplified model of the relationship between habitat and viability, it can provide context to conclusions from more complex models such as described below.
Old-growth-centered landscapes have emergent properties for biodiversity beyond those provided by individual stands. Many animal species that select old-growth forest ecosystems have slow life histories (i.e., delayed age at first reproduction, low reproductive rates, and high survival rates) (Stearns, 1998). Given the scarcity and fragmentation of old-growth forests, populations of dependent species primarily persist as isolated small populations in remnant patches. Given small patch sizes, species richness is low and extinction rates are high especially for species with large area requirements (e.g., apex predators, wide-ranging species) and those with limited mobility including range-restricted endemics. For old-growth dependent species experiencing habitat loss, theory predicts that many will eventually become extirpated as the forest reaches a new equilibrium (Kuussaari et al., 2009). Due to low reproductive rates, delayed maturity, and long life spans, species with slow life histories often show a delayed response to declines in the quality and extent of their focal habitats (Bennett and Owens, 1997; Sodhi et al., 2008; Cardillo et al., 2005). The number of extant specialist species of the focal habitat expected to eventually become extinct as the community reaches a new equilibrium is called the “extinction debt” (Hanski and Ovaskainen, 2002; Kuussaari et al., 2009).
For a given species, individual old-growth patches may provide colonists to other old-growth patches (source patches) or act as receivers (sink patches) of colonists from source patches (Pulliam, 1988). This group of spatially separated populations of the same species, interacting at some level via local colonization and extinctions, is called a metapopulation (Levins, 1969). In general, for a metapopulation to be stable requires that dispersal be sufficient to recolonize vacant patches. Subpopulations can persist over time in a dynamic balance of extinction and recolonization events provided patches are sufficiently connected (Lande, 1988). The NWFP designed its network of Late-Successional Reserves in part to ensure a stable metapopulation, a result of including stands in sufficiently close proximity to allow for connectivity via dispersal and sufficiently dispersed so as to achieve spatial independence from disturbance events and other stochastic processes. In place of the previous focus on individual old-growth stands, this strategy focused on multiple stands dispersed broadly across the landscape (Noon and McKelvey, 1996).
These ecological concepts are highly relevant to old-growth-associated species in other regions. Populations of old forest-associated species outside the PNW may, like the Northern Spotted Owl, still be responding to historic loss of old-growth forest, leaving them vulnerable to additional cumulative losses absent rapid conservation actions to prevent further habitat loss. In longleaf pine savanna ecosystems of the southeastern US, the spatial patchiness of old-growth trees leads to relatively low densities of old pines required by the federally listed Red-cockaded Woodpecker (Dryobates borealis) and other old-growth dependent species. Large and well-connected networks of pine savanna require protection to maintain viable populations of such species.
Complementing these species-focused concepts are principles relevant to ecosystem-level attributes. The US Forest Service uses ecological integrity as the fundamental concept to guide its assessment, land-use planning, and monitoring of forest ecosystems (Wurtzebach and Schultz, 2016). Ecological integrity has been defined as a measure of the composition, structure, and function of an ecosystem in relation to the system’s natural range of variation (Mackey et al., 2024). For forest ecosystems, Rogers et al. (2022) propose several key foundational elements including: (1) physiological structures that efficiently use and dissipate energy (e.g., forest structural complexity and carbon sequestration in the case of late successional forests); (2) productivity (e.g., nutrient cycling) and regenerative capacity following disturbance; and (3) ecosystem resistance/constancy, resilience, and persistence in the context of environmental variation.
The historic range of variation (HRV) concept is widely cited by the Forest Service as a useful metric to guide the management of forest ecosystems (Keane and Loehman, 2019). The concept is based on the premise that keeping ecosystems within their historic bounds of variation will ensure their ecological integrity. Many definitions of HRV exist, but one closely tied to sustaining ecosystem integrity states that HRV is “…the spatial and temporal variation in composition, structure, and function experienced in an ecosystem from about 1600 to 1850” (Dillon et al., 2005). Based on this definition, estimates of composition and structure could provide a rough approximation of the proportion of the landscape that was old-growth within the time period preceding Euro-American settlement. For biodiversity objectives, management might focus on the distribution of stand structural classes most likely to support native species and reflect environmental conditions prior to extensive harvest of mature and old-growth forests.
It would be possible to estimate the proportion of its landscape that would have historically been old growth for each Forest or ecoregion. Such an analysis was recently completed in New England (Foster, 2023). This historic estimate can be used to set baseline landscape-scale targets for forest composition, structure, and subsequent management actions, based on a commitment to protect and restore old growth representing all relevant ecosystem types, including by retention of mature forest that is aging into the old-growth category (Moomaw et al., 2019). Guidance provided by HRV estimates will need to reflect constraints imposed by data limitations, human land-use impacts, and accelerating climate change (Romme et al., 2012). Because biotic interactions and hydrologic processes span ownership boundaries, HRV should typically be considered on an ecoregional scale. If management on non-federal lands favors shorter rotations, this implies that federal lands will have to be managed primarily for recovery of now-rare seral states (including old growth and naturally disturbed, unsalvaged, and regenerating younger forests; Swanson et al., 2011).
The application of concepts such as HRV emphasizes the broader spatiotemporal scale needed for ecologically based forest planning. A hallmark of the NWFP, when compared to previous project-level forest management plans, is that it considered conservation goals over longer time horizons and greater spatial extents (DellaSala et al., 2015; Johnson et al., 2023). Under the NWFP, some proportion of areas between old-growth stands were to be restored to a mosaic of patches of different ages including mature, naturally disturbed, and naturally regenerated early seral stands that retain standing old living and dead trees as biological legacies (Swanson et al., 2011). Because individual stands of old growth will eventually experience loss to disturbance or senescence, a conservation strategy focused on sustaining and increasing the amount of old growth must also protect a significant amount of mature forest, especially in areas adjacent to existing old-growth stands (Moomaw et al., 2019). Assuring that recruitment of mature forest, at the landscape scale, exceeds the rate of old-growth mortality will move Forest Service lands closer to their historic range of variability. Large, old trees occurring individually or in remnant patches outside designated old-growth reserves also merit protection due to their ecological role as keystone structures that buffer and connect old-growth stands (Manning et al., 2006).
The use of the HRV concept to guide forest management into the future has been challenged based on the argument that in the context of rapid climate change and extensive human transformation of the landscape, current conditions of the earth have no precedent in the past (Millar and Woolfenden, 1999; Millar et al., 2007). In many respects, forest management is an ongoing experiment in a dynamic and evolving system with many unknowns. Unfortunately, there are few available metrics beyond HRV that characterize the environmental conditions that supported the current cohort of species in the recent past. How these species responded to past environmental variation may prove some guidance to their responses to future environmental variation (Keane et al., 2018). Keane et al. (2018) and Keane and Loehman (2019) addressed some deficiencies of current estimates of HRV by comparing them to projected future HRVs using multivariate simulation models that incorporate multiple environmental drivers. These models allow estimates of the degree of overlap among HRV metrics between past and projected future HRV distributions. Falk et al. (2022) offered support for HRV by arguing that forests may show extensive resilience to changing environmental conditions with novel states being transitory, representing successional stages that may ultimately revert to the pre-disturbance condition.
Statutes and regulations governing management of federal forestlands include both species- and ecosystem-focused goals, but do not always establish coherent linkages between conservation objectives for the two levels of biodiversity (Carroll et al., 2022). In 2012, revisions to the regulations implementing NFMA de-emphasized the previous mandate for viability of individual species in favor of more general goals based on ecosystem integrity and a more limited group of species of conservation concern (USDA, 2012; Schultz et al., 2013). Though not defined in measurable terms, ecological integrity is a central component of the Forest Service 2012 Planning Rule (USDA, 2012). The foundational elements of ecosystem integrity – ecosystem processes, stability, and adaptive capacity (Rogers et al., 2022) – are all derivatives of the underlying above- and below-ground biodiversity of a forest ecosystem (Mackey et al., 2024; Faison et al., 2023a). However, the more generalized goals of the 2012 Planning Rule allow agencies more discretion and insulation from litigation than did the previous requirement to sustain the viability of all native species (Schultz et al., 2013). This is especially of concern given how much is not yet known regarding the community of old-growth-associated species and their interactions. It is important that old-growth conservation strategies integrate species- and ecosystem-level metrics rather than assuming that ecosystem-level metrics in isolation will be sufficient to conserve biodiversity.
9 Key principles of a nationally coordinated and regionally contextualized strategy for conservation and restoration of old-growth landscapes
Future old-growth conservation policy should incorporate landscape-level planning similar to the NOGA’s “adaptive strategies,” but must add substantive detail, including guidance regarding ensuring viability of old-growth-associated species. To assist in this effort, we propose essential elements that should be included in future national or subnational old-growth policy, including amendments to existing regional plans such as the NWFP. We structure our recommendations as a series of key principles.
9.1 Create a comprehensive map and estimate the amount and spatial distribution of old-growth by major forest type
Recent progress has been made on mapping the distribution and extent of mature and old-growth forest at multiple spatial scales within the continental US (DellaSala et al., 2022b; Barnett et al., 2023; USDA, 2024b). Similar mapping efforts will be essential for any nation committed to the protection of old-growth forest types and recruitment of younger forests into this state. Such maps should discriminate private from public ownership of forested areas and be based on the best available geospatial data so as to achieve sufficient spatial resolution to inform management decisions (Gray et al., 2023). This type of mapping exercise is essential for an initial delineation of candidate reserve areas and to ensure connectivity among candidate reserves.
9.2 Combine immediate measures to protect old-growth trees and stands with long-term landscape planning for old-growth ecosystem protection and restoration
Given the rarity of old-growth trees and stands in most regions and forest types, their immediate protection from harvest or other destruction is paramount. Nevertheless, a strategy of protection focused on trees and stands by itself will not assure maintenance of sufficient old growth and naturally disturbed younger forests across the regional landscape over time. A landscape-level strategy involving large and well-connected reserves and buffer zones is needed to ensure that natural disturbance-recovery cycles are able to continue operating and recruiting new old-growth trees and stands into the future.
9.3 Design a protection and restoration strategy to ensure
“Adequate amounts, representativeness, redundancy, and connectivity of old-growth forest areas” (text from NOGA; USDA, 2024a) via an intelligently designed network of old-growth reserves across landscapes. Evaluate current status and set specific targets in terms of total area, size, and distribution of old-growth forest stands at the Forest, ecoregional, and national levels. Without aspirational quantitative targets, management by agency discretion is highly likely to reduce the area of old growth and limit its recruitment over time.
9.4 Base protection and restoration goals on historic range of variation
Although we are in an era of rapid climate change unprecedented for millennia, the evolution of tree species and development of forest communities extends back much further in time. These communities have changed over time with changing climate, but this change has occurred within a range of variation that can be estimated through historic reconstructions. The time scale over which to consider this range of variability will vary depending on the historical biogeography and climate of each region (e.g., glaciated vs. unglaciated, refugial or non-refugial), but can be informed by paleontology, dated phylogenies, climatic reconstructions, and other scientific evidence (Noss, 2001).
9.5 Base conservation strategy on landscape-level design including reserves
We consider reserves to be areas where most forms of extractive use and associated impacts (e.g., road construction) are restricted and where the conservation and restoration of biodiversity and ecological integrity is a major, if not the primary, concern. Reserves therefore encompass IUCN protected area categories I through IV and US Gap Analysis categories 1 and 2, including areas protected under “Other Effective Conservation Measures” (OECM) (IUCN, 2019). In line with well-accepted landscape management principles (e.g., Noss and Cooperrider 1994), reserves should be multiple, well-connected, and buffered from intensive land uses. Such reserves can help meet national “30×30” commitments to protect 30% or more of lands and waters (Carroll and Noss, 2022).
9.6 Consider habitat area and configuration requirements for species viability and ecosystem services
Species have widely varied spatial requirements determined by their body size (for animals), trophic position, and behavior. Appropriate focal species for conservation include area-limited, dispersal-limited, resource-limited, and process-limited species (Lambeck, 1997). For conservation planning on a regional landscape scale, area-limited and dispersal-limited animal species typically receive the most attention because of their sensitivity to landscape configuration, especially the size, connectivity, and juxtaposition of habitat blocks. Identifying the species that fall into these categories for any region or forest type is a relatively straightforward exercise, though determining the specific requirements for species viability still requires further research for many regions.
9.7 Identify and protect climate and fire refugia
Climate refugia exist at scales ranging from regional (which encompass most global hotspots of species endemism; Harrison and Noss, 2017) to local, such as sinkholes, springs, and other karst features and relatively cool north-facing slopes (e.g., Bátori et al., 2016). Many of these same areas, as well as relatively isolated areas such as islands, peninsulas, and north-facing slopes will also serve as fire refugia (Noss, 2018). Identifying, protecting and connecting climate and fire refugia at all relevant spatial scales is one of the most promising strategies for reducing the impacts of climate change on biodiversity (Carroll and Ray, 2021; Keppel et al., 2024).
9.8 Address barriers to connectivity
Connectivity, in addition to its benefits for maintaining viable metapopulations, as discussed above, also joins protection of climate refugia as a key strategy for adaptation to climate change. Connectivity at finer scales is also critical to allow barrier-sensitive small animals to meet their daily and seasonal life history needs. In addition to habitat fragmentation generally, roads are among the most important barriers to the movements of non-volant animals, in addition to many other negative effects such as erosion and promotion of non-native species invasions (Forman et al., 2003). Decommissioning, recontouring, and revegetation of unnecessary roads in forest landscapes, and establishing wildlife crossings where needed, is an important strategy for restoring forest ecosystem integrity.
9.9 Enhance monitoring capacity and data protocols
One of the NWFP’s key innovations was a commitment to comprehensive monitoring focused on multiple facets of planning (implementation, effectiveness, etc.) (Johnson et al., 2023). Adaptive management implies long-term data collection across a system of controls and treatments, i.e., lines on a map with differing management practices in different areas (Faison et al., 2023b). The success of any “adaptive strategies” similar to those proposed in the NOGA is contingent on developing improved methods for monitoring the status and trends of biodiversity across scales of space and time (Noss, 1990; Lindenmayer et al., 2012; Kühl et al., 2020). Although the NOGA included aspirational goals regarding ecological integrity and connectivity, it lacked guidance on how to establish control groups, baselines, measurable indicators, metrics, and targets to monitor achievement towards goals (Schultz et al., 2013; Brown and Williams, 2016; Mackey et al., 2024).
Achieving the goals of an old-growth conservation strategy such as the NOGA also requires development an old-growth monitoring network (USDA, 2024a). To be useful in estimating temporal trends and management effectiveness, long-term, comprehensive site-level monitoring information will need to be integrated with remotely sensed data sources. In turn, data on old-growth status and trends can be integrated into more broadly focused analyses such as federal lands carbon budgeting and national nature assessments (Noon et al., 2003; Carroll et al., 2023).
10 Conclusion
Effective conservation of old-growth forests, along with their unique biodiversity and climate benefits, requires coordinated actions from the scale of individual trees to landscapes. Because large, old trees and old-growth forests can be removed quickly, but require hundreds of years to be renewed, old-growth trees and stands are classic examples of remnant biodiversity or ecosystem elements that require immediate conservation action (Lindenmayer and Laurance, 2017). While immediate site-level conservation actions are a priority, such efforts will be insufficient to restore ecological integrity unless coupled with recovery and recruitment of old growth at the landscape scale. This will require broad-scale spatial planning that addresses current threats and the ecological legacies of past deforestation and forest degradation (Lindenmayer et al., 2014). The recent effort to develop a US National Old-Growth Amendment (NOGA) offers lessons that can be built upon to inform long-term development of effective old-growth policy in the US and elsewhere.
An ecologically informed old-growth conservation strategy must include elements at three spatiotemporal scales: immediate restrictions on harvest of old and mature trees, measures to ensure management activities do not degrade old growth at the stand scale, and longer-term landscape planning for old-growth restoration and recovery. A landscape-scale planning approach analogous to the NOGA’s proposed adaptive strategies can form a key foundation of long-term conservation of forest ecosystems, especially if informed by viability requirements for old-growth-associated species. Reversing extinction debt and ensuring long-term adaptation potential requires designation of large areas anchored by remaining old-growth stands, surrounded by areas managed for restoration of ecological integrity, native biodiversity, and ecosystem services where management objectives explicitly include recruitment of additional old growth by allowing mature forests to develop over time (Moomaw et al., 2019). Ecosystem-based standards should be developed to ensure sufficient extent of mature forest is protected so that recruitment of these stands into the old-growth stage, minus cumulative loss and transition of old growth due to mortality and other factors, shifts ecosystems towards to the approximate proportion within each forest ecosystem type that would have historically been old growth.
A focus on old-growth-associated species and their viability is a necessary complement to ecosystem metrics. Bedrock US environmental laws such as the ESA (Endangered Species Act) and NFMA contain a fundamental responsibility to prevent species’ extinctions, with habitat protection as the principal foundation of this effort. Compared to the 1994 NWFP, the NOGA de-emphasized the linkages between forest landscape composition and species viability, instead referencing goals such as ecological integrity and resilience. The lack of specific goals for recovering at-risk species dependent on old-growth forests is a weakness in current forest policy that must be addressed so that biodiversity is conserved across all scales from populations and species to ecosystems (Carroll et al., 2022).
Conserving and restoring ecological integrity is a foundational goal and legal requirement for the Forest Service (USDA, 2012; section 219.9a), and at the global scale is an element of multiple KMGBF targets (CBD, 2023). Because of their physical dominance and structural complexity, old-growth trees are a key biological entity in conserving ecological integrity (Brown and Williams, 2016). Conserving old-growth stands and native species is central to conserving the ecological integrity of forest ecosystems (Lindenmayer et al., 2014; Rogers et al., 2022). However, many efforts to operationalize ecological integrity goals, including those in the NOGA, suffer from a lack of specific metrics. Absent measurable targets, standards, and indicators, the commitment to ecological integrity will remain a purely aspirational goal (Brown and Williams, 2016; Mackey et al., 2024).
US policy concerning old-growth conservation and other biodiversity-related issues has been weakened by its tendency towards ahistorical and parochial debate. Here, we have sought to strengthen our discussion of the NOGA and related efforts by providing ecological context, and greater global and international context. The 1994 NWFP’s insights that spatial landscape design is essential for effective conservation of species, services, and processes associated within old-growth forest ecosystems remains broadly relevant. Systematic conservation planning and landscape design are widely accepted fundamentals of conservation science and practice (Margules and Pressey, 2000; Kukkala and Moilanen, 2013). In place of the NOGA’s broad generalizations regarding the need for “proactive stewardship,” substantive discussion is needed of the varied ecological contexts presented by diverse old-growth types (including non-successional examples such as longleaf pine and other savanna ecosystems) and their implications for spatial landscape design.
In grappling with these conceptually and practically challenging questions, US conservation policy can be strengthened by consideration of the parallels between US and global policy development as the world’s nations work to implement the KMGBF by coordinating targets regarding inclusive spatial planning, protected areas, species viability, and ecosystem integrity (Bell-James and Watson, 2025). In most contexts, reserves (areas where forms of extractive use are restricted) in the broad sense (IUCN, 2019) are necessary and compatible with practical strategies for ecosystem recovery and restoration and Indigenous co-management practices (e.g., cultural fire). Such areas can also help nations fulfill “30×30” commitments to conserve at least 30% of lands and waters in a natural or near-natural condition by 2030 (Carroll and Noss, 2022; CBD, 2023). Practitioners and policymakers in other nations can also learn from the NOGA debate and the multi-decadal evolution of US old-growth policy and research. Our recommendations are relevant to the global conservation of old-growth forests, primary forests (i.e., unlogged forests of all age classes), and intact forest areas (Watson et al., 2018).
We recognize that debate over management of forest ecosystems (including the relative emphasis on “active” versus “passive” management) is in part normative or values-based, and influenced by social, financial, and cognitive biases. For example, the relative priority placed on short-term economic benefits versus long-term sustainability of ecosystems and their services varies between successive US administrations. The science-informed insights that we develop further the stated conservation goals of the NOGA and related global accords (CBD, 2023) in promoting long-term sustainability and recovery of forest ecosystems and biodiversity (White House, 2021, 2022).
Although our focus here is on how ecological and interdisciplinary science can best inform policy development, we recognize the complexity of sociopolitical and organizational constraints on application of ecological science. US agencies such as the Forest Service face substantial informational and organizational challenges and pressures in designing and implementing landscape planning, as seen in the case of NWFP implementation (Spies et al., 2019). Investments in building this capacity are warranted, however, due to the essential value to society of forest ecosystem integrity.
In addition to any technical and information needs, land management agencies need to articulate and adopt a long-term vision and strategy for ecosystem integrity and recovery that focuses not only on old growth but also other depleted forest types such as naturally disturbed stands across all age classes, and that values disturbance as an ecological process rather than as solely a threat to human control of forest succession. The ultimate impetus for old-growth conservation efforts arises not only from our increased scientific understanding of these forests’ contributions to biodiversity, climate regulation, and ecosystem services; the impetus also lies in the evolution of society’s vision for the role of forest lands, especially those held in the public trust by the federal government. Ongoing conflict between ambitious aspirational goals for recovery and restoration of nature and the continued support for extractive industries by most national governments has also undermined efforts at the global level including implementation of the KMGBF. Conservation science cannot in itself resolve this values-based debate but can help inform society as to the rising costs of the status quo, and of the ecological and social benefits of maintaining, recovering, and restoring functioning old-growth ecosystems.
Author contributions
CC: Writing – original draft, Writing – review & editing. BN: Writing – original draft, Writing – review & editing. SM: Writing – review & editing. RN: Writing – original draft, Writing – review & editing.
Funding
The author(s) declare that financial support was received for the research and/or publication of this article. This work was supported by the Wilburforce Foundation, which provided support for CC.
Acknowledgments
The manuscript benefited from comments by L. Rosa, D. Rohlf, and D. Werntz. CC acknowledges support from the Wilburforce Foundation.
Conflict of interest
The authors declare that the research was conducted in the absence of any commercial or financial relationships that could be construed as a potential conflict of interest.
Publisher’s note
All claims expressed in this article are solely those of the authors and do not necessarily represent those of their affiliated organizations, or those of the publisher, the editors and the reviewers. Any product that may be evaluated in this article, or claim that may be made by its manufacturer, is not guaranteed or endorsed by the publisher.
Supplementary material
The Supplementary material for this article can be found online at: https://www.frontiersin.org/articles/10.3389/ffgc.2025.1493879/full#supplementary-material
References
Adams, G. S., Converse, B. A., Hales, A. H., and Klotz, L. E. (2021). People systematically overlook subtractive changes. Nature 592, 258–261. doi: 10.1038/s41586-021-03380-y
Barnett, K., Aplet, G. H., and Belote, R. T. (2023). Classifying, inventorying, and mapping mature and old-growth forests in the United States. Front. For. Global Change 5:1070372. doi: 10.3389/ffgc.2022.1070372
Bátori, Z., Vojtkó, A., Farkas, T., Szabó, A., Havadtői, K., Vojtkó, A. E., et al. (2016). Large- and small-scale environmental factors drive distributions of cool-adapted plants in karstic microrefugia. Ann. Bot. 119, 301–309. doi: 10.1093/aob/mcw233
Bell-James, J., and Watson, J. E. (2025). Ambitions in national plans do not yet match bold international protection and restoration commitments. Nature Ecol. Evol. 9, 417–424. doi: 10.1038/s41559-025-02636-4
Bennett, P. M., and Owens, I. P. F. (1997). Variation in extinction risk among birds: chance or evolutionary predisposition? Proceedings of the Royal Society of London. Series B Biol. Sci. 264, 401–408. doi: 10.1098/rspb.1997.0057
Betts, M. G., Yang, Z., Gunn, J. S., and Healey, S. P. (2024). Congruent long-term declines in carbon and biodiversity are a signature of forest degradation. Glob. Chang. Biol. 30:e17541. doi: 10.1111/gcb.17541
Boecklen, W. J. (1986). Effects of habitat heterogeneity on the species-area relationships of forest birds. J. Biogeogr. 13, 59–68. doi: 10.2307/2844849
Brown, E. D., and Williams, B. K. (2016). Ecological integrity assessment as a metric of biodiversity: are we measuring what we say we are? Biodivers. Conserv. 25, 1011–1035. doi: 10.1007/s10531-016-1111-0
Cardillo, M., Mace, G. M., Jones, K. E., Bielby, J., Bininda-Emonds, O. R. P., Sechrest, W., et al. (2005). Multiple causes of high extinction risk in large mammal species. Science 309, 1239–1241. doi: 10.1126/science.1116030
Carroll, C., Hoban, S., and Ray, J. C. (2024). Lessons from COP15 on effective scientific engagement in biodiversity policy processes. Conserv. Biol. 38:e14192. doi: 10.1111/cobi.14192
Carroll, C., and Noss, R. F. (2022). How percentage-protected targets can support positive biodiversity outcomes. Conserv. Biol. 36:e13869. doi: 10.1111/cobi.13869
Carroll, C., Noss, R. F., Dreiss, L. M., Hamilton, H., and Stein, B. A. (2023). Four challenges to an effective national nature assessment. Conserv. Biol. 37:e14075. doi: 10.1111/cobi.14075
Carroll, C., and Ray, J. C. (2021). Maximizing the effectiveness of national commitments to protected area expansion for conserving biodiversity and ecosystem carbon under climate change. Glob. Chang. Biol. 27, 3395–3414. doi: 10.1111/gcb.15645
Carroll, C., Rohlf, D. J., and Epstein, Y. (2022). Mainstreaming the ambition, coherence, and comprehensiveness of the Post-2020 global biodiversity framework into conservation policy. Front. Conserv. Sci. 3:906699. doi: 10.3389/fcosc.2022.906699
CBD (2023). Kunming-Montreal global biodiversity framework. Montreal: Secretariat of the Convention on Biological Diversity.
Clebsch, E. E. C., and Busing, R. T. (1989). Secondary succession, gap dynamics, and community structure in a southern Appalachian cove forest. Ecology 70, 728–735. doi: 10.2307/1940223
Davis, K. T., Peeler, J., Fargione, J., Haugo, R. D., Metlen, K. L., Robles, M. D., et al. (2024). Tamm review: a meta-analysis of thinning, prescribed fire, and wildlife fire effects on subsequent wildfire severity in conifer dominated forest of the western US. For. Ecol. Manag. 561, 1–13. doi: 10.1016/j.foreco.2024.121885
DellaSala, D. A., Baker, B. C., Hanson, C. T., Ruediger, L., and Baker, W. (2022a). Have western USA fire suppression and megafire active management approaches become a contemporary Sisyphus? Biol. Conserv. 268:109499. doi: 10.1016/j.biocon.2022.109499
DellaSala, D. A., Baker, R., Heiken, D., Frissell, C. A., Karr, J. R., Nelson, S. K., et al. (2015). Building on two decades of ecosystem management and biodiversity conservation under the northwest Forest plan, USA. Forests 6, 3326–3352. doi: 10.3390/f6093326
DellaSala, D. A., Mackey, B., Norman, P., Campbell, C., Comer, P. J., Kormos, C. F., et al. (2022b). Mature and old-growth forests contribute to large-scale conservation targets in the conterminous United States. Front. For. Global Change 5:979528. doi: 10.3389/ffgc.2022.979528
DellaSala, D. A., Strittholt, J. R., Degagne, R., Mackey, B., Werner, J. R., Connolly, M., et al. (2021). Red-listed ecosystem status of interior wetbelt and inland temperate rainforest of British Columbia, Canada. Land 10:775. doi: 10.3390/land10080775
DellaSala, D. A., Williams, J. E., and Williams, C. D., And Franklin, J. F. (2004). Beyond smoke and mirrors: a synthesis of fire policy and science. Conserv. Biol. 18, 976–986. doi: 10.1111/j.1523-1739.2004.00529.x
Dillon, G., Knight, D., and Meyer, C. (2005). Historic range of variability for upland vegetation in the medicine bow National Forest, Wyoming, USDA Forest Service general technical report RMRS-GTR-139. Fort Collins, CO: USDA Forest Service.
Eisenberg, C., Prichard, S., Nelson, M. P., and Hessburg, P. (2024). Braiding Indigenous and Western knowledge for climate-adapted forests: An ecocultural state of science report. Available online at: adaptiveforeststewardship.org (Accessed January 1, 2025).
Ellair, D. P., and Platt, W. J. (2013). Fuel composition influences fire characteristics and understorey hardwoods in pine savanna. J. Ecol. 101, 192–201. doi: 10.1111/1365-2745.12008
Faison, E. K., Laflower, D., Morreale, L. L., Foster, D. R., Hall, B., Johnson, E., et al. (2023a). Adaptation and mitigation capacity of wildland forests in the northeastern United States. For. Ecol. Manag. 544:121145. doi: 10.1016/j.foreco.2023.121145
Faison, E. K., Masino, S. A., and Moomaw, W. R. (2023b). The importance of natural forest stewardship in adaptation planning in the United States. Conserv. Sci. Pract. 5:e12935. doi: 10.1111/csp2.12935
Falk, D. A., van Mantgem, P. J., Keeley, J. E., Gregg, R. M., Guiterman, C. H., Tepley, A. J., et al. (2022). Mechanisms of forest resilience. For. Ecol. Manag. 512:120129. doi: 10.1016/j.foreco.2022.120129
Farinacci, M. D., Jones, J., and Silva, L. C. R. (2024). Carbon-water tradeoffs in old-growth and young forests of the Pacific northwest. AGU Adv. 5:e2024AV001188. doi: 10.1029/2024AV001188
Forman, R. T., Sperling, D., Bissonette, J. A., Clevenger, A. P., Cutshall, C. D., Dale, V. H., et al. (2003). Road ecology. Washington, DC: Island Press.
Foster, D. R. (2023). Wildlands in New England: Past, present, and future. Harvard: Harvard University Press.
Franklin, J. F., Denison, W., McKee, A., Maser, C., Sedell, J., Swanson, F., et al. (1981). Ecological characteristics of old-growth Douglas-fir forests. Gen. Tech. Rep. 48:118.
Franklin, A. B., Dugger, K. M., Lesmeister, D. B., Davis, R. J., Wiens, J. D., White, G. C., et al. (2021). Range-wide declines of northern spotted owl populations in the Pacific northwest: a meta-analysis. Biol. Conserv. 259:109168. doi: 10.1016/j.biocon.2021.109168
Ghazoul, J., Burivalova, Z., Garcia-Ulloa, J., and King, L. A. (2015). Conceptualizing forest degradation. Trends Ecol. Evol. 30, 622–632. doi: 10.1016/j.tree.2015.08.001
Gray, A. N., Pelz, K., Hayward, G. D., Schuler, T., Salverson, W., Palmer, M., et al. (2023). Perspectives: the wicked problem of defining and inventorying mature and old growth forests. For. Ecol. Manag. 546:121350. doi: 10.1016/j.foreco.2023.121350
Hanski, I., and Ovaskainen, O. (2002). Extinction debt at extinction threshold. Conserv. Biol. 16, 666–673. doi: 10.1046/j.1523-1739.2002.00342.x
Harris, L. D. (1984). The fragmented forest: Island biogeography theory and the preservation of biotic diversity. Chicago, IL: University of Chicago Press.
Harrison, S., and Noss, R. (2017). Endemism hotspots are linked to stable climatic refugia. Ann. Bot. 119, 207–214. doi: 10.1093/aob/mcw248
Harte, J., Smith, A. B., and Storch, D. (2009). Biodiversity scales from plots to biomes with a universal species–area curve. Ecol. Lett. 12, 789–797. doi: 10.1111/j.1461-0248.2009.01328.x
Hessburg, P. F., Churchill, D. J., Larson, A. J., Haugo, R. D., Miller, C., Spies, T. A., et al. (2015). Restoring fire-prone inland Pacific landscapes: seven core principles. Landsc. Ecol. 30, 1805–1835. doi: 10.1007/s10980-015-0218-0
Hughes, A. C., and Grumbine, R. E. (2023). The Kunming-Montreal global biodiversity framework: what it does and does not do, and how to improve it. Front. Environ. Sci. 11. doi: 10.3389/fenvs.2023.1281536
Hurteau, M. D., Koch, G. W., and Hungate, B. A. (2008). Carbon protection and fire risk reduction: toward a full accounting of forest carbon offsets. Front. Ecol. Environ. 6, 493–498. doi: 10.1890/070187
ICE (2018). We rise together: Achieving pathway to target 1 through the creation of indigenous protected and conserved areas in the spirit and practice of reconciliation: the Indigenous Circle of Experts’ report and recommendations. Gatineau, QC: Parks Canada.
IPBES (2019). Summary for policymakers of the global assessment report on biodiversity and ecosystem services of the intergovernmental science-policy platform on biodiversity and ecosystem services. Bonn, Germany: IPBES secretariat.
IUCN (2019). Recognising and reporting other effective area-based conservation measures. Gland, Switzerland: International Union for the Conservation of Nature.
Johnson, K. N., Franklin, J. F., and Reeves, G. H. (2023). The making of the Northwest Forest Plan: The wild science of saving old growth ecosystems. Corvallis, OR: Oregon State University Press.
Keane, R. E., and Loehman, R. (2019) in Historic range and variation (HRV). Encyclopedia of wildlife fires and wildland-urban interface (WUI) fires. ed. S. L. Manzello (Cham, Switzerland: Springer Nature Switzerland). doi: 10.1007/978-3-319-51727-8_255-1
Keane, R. E., Loehman, R. A., Holsinger, L. M., Falk, D. A., Higuera, P., Hood, S. M., et al. (2018). Use of landscape simulation modeling to quantify resilience for ecological applications. Ecosphere 9:e02414. doi: 10.1002/ecs2.2414
Kellett, M. J., Maloof, J. E., Masino, S. A., Frelich, L. E., Faison, E. K., Brosi, S. L., et al. (2023). Forest-clearing to create early-successional habitats: questionable benefits, significant costs. Front. For. Global Change 5. doi: 10.3389/ffgc.2022.1073677
Keppel, G., Stralberg, D., Morelli, T. L., and Bátori, Z. (2024). Managing climate-change refugia to prevent extinctions. Trends Ecol. Evol. 39, 800–808. doi: 10.1016/j.tree.2024.05.002
Krankina, O. N., Harmon, M. E., Schnekenburger, F., and Sierra, C. A. (2012). Carbon balance on federal forest lands of Western Oregon and Washington: the impact of the Northwest Forest Plan. For. Ecol. Manag. 286, 171–182. doi: 10.1016/j.foreco.2012.08.028
Krawchuk, M. A., Haire, S. L., Coop, J., Parisien, M.-A., Whitman, E., Chong, G., et al. (2016). Topographic and fire weather controls of fire refugia in forested ecosystems of northwestern North America. Ecosphere 7:e01632. doi: 10.1002/ecs2.1632
Krawchuk, M. A., Meigs, G. W., Cartwright, J. M., Coop, J. D., Davis, R., Holz, A., et al. (2020). Disturbance refugia within mosaics of forest fire, drought, and insect outbreaks. Front. Ecol. Environ. 18, 235–244. doi: 10.1002/fee.2190
Kreitler, J., Schloss, C. A., Soong, O., Hannah, L., and Davis, F. W. (2015). Conservation planning for offsetting the impacts of development: a case study of biodiversity and renewable energy in the Mojave Desert. PLoS One 10:e0140226. doi: 10.1371/journal.pone.0140226
Kühl, H. S., Bowler, D. E., Bösch, L., Bruelheide, H., Dauber, J., Eichenberg, D., et al. (2020). Effective biodiversity monitoring needs a culture of integration. One Earth 3, 462–474. doi: 10.1016/j.oneear.2020.09.010
Kukkala, A. S., and Moilanen, A. (2013). Core concepts of spatial prioritisation in systematic conservation planning. Biol. Rev. 88, 443–464. doi: 10.1111/brv.12008
Kuussaari, M., Bommarco, R., Heikkinen, R. K., Helm, A., Krauss, J., Lindborg, R., et al. (2009). Extinction debt: a challenge for biodiversity conservation. Trends Ecol. Evol. 24, 564–571. doi: 10.1016/j.tree.2009.04.011
Lambeck, R. J. (1997). Focal species: a multi-species umbrella for nature conservation. Conserv. Biol. 11, 849–856. doi: 10.1046/j.1523-1739.1997.96319.x
Lande, R. (1988). Demographic models of the northern spotted owl (Strix occidentalis caurina). Oecologia 75, 601–607. doi: 10.1007/BF00776426
Law, B. E., Berner, L. T., Buotte, P. C., Mildrexler, D. J., and Ripple, W. J. (2021). Strategic Forest Reserves can protect biodiversity in the western United States and mitigate climate change. Commun. Earth Environ. 2:254. doi: 10.1038/s43247-021-00326-0
Lesmeister, D. B., Sovern, S. G., Davis, R. J., Bell, D. M., Gregory, M. J., and Vogeler, J. C. (2019). Mixed-severity wildfire and habitat of an old-forest obligate. Ecosphere 10:e02696. doi: 10.1002/ecs2.2696
Levins, R. (1969). Some demographic and genetic consequences of environmental heterogeneity for biological control. Bull. Entomol. Soc. Am. 15, 237–240. doi: 10.1093/besa/15.3.237
Lindenmayer, D. B., and Franklin, J. F. (2002). Conserving forest biodiversity: A comprehensive multiscaled approach. Washington, DC: Island Press.
Lindenmayer, D. B., and Laurance, W. F. (2017). The ecology, distribution, conservation and management of large old trees. Biol. Rev. 92, 1434–1458. doi: 10.1111/brv.12290
Lindenmayer, D. B., Laurance, W. F., and Franklin, J. F. (2012). Global decline in large old trees. Science 338, 1305–1306. doi: 10.1126/science.1231070
Lindenmayer, D. B., Laurance, W. F., Franklin, J. F., Likens, G. E., Banks, S. C., Blanchard, W., et al. (2014). New policies for old trees: averting a global crisis in a keystone ecological structure. Conserv. Lett. 7, 61–69. doi: 10.1111/conl.12013
Littlefield, C. E., and D'Amato, A. W. (2022). Identifying trade-offs and opportunities for forest carbon and wildlife using a climate change adaptation lens. Conserv. Sci. Pract. 4:e12631. doi: 10.1111/csp2.12631
MacArthur, R. H., and Wilson, E. O. (1967). The theory of island biogeography. Princeton, NJ: Princeton University Press.
Mackey, B., DellaSala, D. A., Kormos, C., Lindenmayer, D., Kumpel, N., Zimmerman, B., et al. (2014). Policy options for the world's primary forests in multilateral environmental agreements. Conserv. Lett. 8, 139–147. doi: 10.1111/conl.12120
Mackey, B., Morgan, E., and Keith, H. (2024). Evaluating forest landscape management for ecosystem integrity. Landsc. Res. 49, 246–267. doi: 10.1080/01426397.2023.2284938
Makarieva, A. M., Nefiodov, A. V., Rammig, A., and Nobre, A. D. (2023). Re-appraisal of the global climatic role of natural forests for improved climate projections and policies. Front. For. Global Change 6:1150191. doi: 10.3389/ffgc.2023.1150191
Manning, A. D., Fischer, J., and Lindenmayer, D. B. (2006). Scattered trees are keystone structures – implications for conservation. Biol. Conserv. 132, 311–321. doi: 10.1016/j.biocon.2006.04.023
Margules, C. R., and Pressey, R. L. (2000). Systematic conservation planning. Nature 405, 243–253. doi: 10.1038/35012251
Marler, T. E., and Lindström, A. J. (2017). First, do no harm. Commun. Integr. Biol. 10:e1393593. doi: 10.1080/19420889.2017.1393593
McElhinny, C., Gibbons, P., Brack, C., and Bauhus, J. (2005). Forest and woodland stand structural complexity: its definition and measurement. For. Ecol. Manag. 218, 1–24. doi: 10.1016/j.foreco.2005.08.034
Mildrexler, D. J., Berner, L. T., Law, B. E., Birdsey, R. A., and Moomaw, W. R. (2020). Large trees dominate carbon storage in forests east of the Cascade crest in the United States Pacific Northwest. Front. For. Global Change 3:594274. doi: 10.3389/ffgc.2020.594274
Millar, C. I., Stephenson, N. L., and Stephens, S. L. (2007). Climate change and forests of the future: managing in the face of uncertainty. Ecol. Appl. 17, 2145–2151. doi: 10.1890/06-1715.1
Millar, C. I., and Woolfenden, W. B. (1999). The role of climate change in interpreting historical variability. Ecol. Appl. 9, 1207–1216. doi: 10.1890/1051-0761(1999)009[1207:TROCCI]2.0.CO;2
Molina, R., Marcot, B. G., and Lesher, R. (2006). Protecting rare, old-growth, forest-associated species under the survey and manage program guidelines of the Northwest Forest Plan. Conserv. Biol. 20, 306–318. doi: 10.1111/j.1523-1739.2006.00386.x
Moomaw, W. R., Masino, S. A., and Faison, E. K. (2019). Intact forests in the United States: Proforestation mitigates climate change and serves the greatest good. Front. For. Global Change 2:27. doi: 10.3389/ffgc.2019.00027
Morelli, T. L., Barrows, C. W., Ramirez, A. R., Cartwright, J. M., Ackerly, D. D., Eaves, T. D., et al. (2020). Climate-change refugia: biodiversity in the slow lane. Front. Ecol. Environ. 18, 228–234. doi: 10.1002/fee.2189
Müller, J., Noss, R. F., Bussler, H., and Brandl, R. (2010). Learning from a “benign neglect strategy” in a national park: response of saproxylic beetles to dead wood accumulation. Biol. Conserv. 143, 2559–2569. doi: 10.1016/j.biocon.2010.06.024
Murphy, D. D., and Noon, B. R. (1992). Integrating scientific methods with habitat conservation planning: reserve design for northern spotted owls. Ecol. Appl. 2, 3–17. doi: 10.2307/1941885
Newman, E. A. (2019). Disturbance ecology in the Anthropocene. Front. Ecol. Evol. 7:147. doi: 10.3389/fevo.2019.00147
Noon, B. R., and Blakesley, J. A. (2006). Conservation of the northern spotted owl under the Northwest Forest Plan. Conserv. Biol. 20, 288–296. doi: 10.1111/j.1523-1739.2006.00387.x
Noon, B. R., and McKelvey, K. S. (1996). Management of the Spotted owl: a case history in conservation biology. Annu. Rev. Ecol. Evol. Syst. 27, 135–162. doi: 10.1146/annurev.ecolsys.27.1.135
Noon, B. R., McKelvey, K. S., and Dickson, B. G. (2009). “Multispecies conservation planning on U.S. federal lands” in Models for planning wildlife conservation in large landscapes. eds. J. J. Millspaugh and F. R. ThompsonIII (New York, NY: Academic Press), 51–84.
Noon, B. R., Murphy, D. D., Beissinger, S. R., Shaffer, M. L., and DellaSala, D. (2003). Conservation planning for US National Forests: conducting comprehensive biodiversity assessments. Bioscience 53, 1217–1220. doi: 10.1641/0006-3568(2003)053[1217:Cpfunf]2.0.Co;2
North, M. P., Bisbing, S. M., Hankins, D. L., Hessburg, P. F., Hurteau, M. D., Kobziar, L. N., et al. (2024). Strategic fire zones are essential to wildfire risk reduction in the Western United States. Fire Ecol. 20:50. doi: 10.1186/s42408-024-00282-y
Noss, R. F. (1990). Indicators for monitoring biodiversity: a hierarchical approach. Conserv. Biol. 4, 355–364. doi: 10.1111/j.1523-1739.1990.tb00309.x
Noss, R. F. (2001). Beyond Kyoto: forest management in a time of rapid climate change. Conserv. Biol. 15, 578–590. doi: 10.1046/j.1523-1739.2001.015003578.x
Noss, R. F. (2012). Forgotten grasslands of the south: Natural history and conservation. Washington, DC: Island Press.
Noss, R. F. (2018). Fire ecology of Florida and the southeastern coastal plain. Florida: University Press of Florida.
Noss, R. F., and Cooperrider, A. Y. (1994). Saving nature’s legacy: protecting and restoring biodiversity. Washington, DC: Island Press.
Platt, W. J., Evans, G. W., and Rathbun, S. L. (1988). The population dynamics of a long-lived conifer (Pinus palustris). Am. Nat. 131, 491–525. doi: 10.1086/284803
Powell, D. C. (2012). A stage is a stage is a stage, or is it? Successional stages, structural stages, seral stages. Silvicultural papers no. 12. USDA Forest Service. White Paper F14-SO-WP-SILV-10.
Reeves, G. H., Williams, J. E., Burnett, K. M., and Gallo, K. (2006). The aquatic conservation strategy of the Northwest Forest Plan. Conserv. Biol. 20, 319–329. doi: 10.1111/j.1523-1739.2006.00380.x
Rickey, M. A., Weekley, C. W., and Menges, E. S. (2013). Felling as a pre-treatment for prescribed fire promotes restoration of fire-suppressed Florida Sandhill. Nat. Areas J. 33, 199–213. doi: 10.3375/043.033.0210
Rogers, B. M., Mackey, B., Shestakova, T. A., Keith, H., Young, V., Kormos, C. F., et al. (2022). Using ecosystem integrity to maximize climate mitigation and minimize risk in international forest policy. Front. For. Global Change 5:929281. doi: 10.3389/ffgc.2022.929281
Romme, W. H., Wiens, J. A., and Safford, H. D. (2012). “Setting the stage: theoretical and conceptual background of historical range of variation” in Historical environmental variation in conservation and natural resource management. eds. C. Giffen, G. D. Hayward, and H. D. Safford, (Hoboken, NJ: John Wiley & Sons) 3–18.
Ryan, K. C., Knapp, E. E., and Varner, J. M. (2013). Prescribed fire in North American forests and woodlands: history, current practice, and challenges. Front. Ecol. Environ. 11, e15–e24. doi: 10.1890/120329
Schultz, C. A., Sisk, T. D., Noon, B. R., and Nie, M. A. (2013). Wildlife conservation planning under the United States Forest Service's 2012 planning rule. J. Wildl. Manag. 77, 428–444. doi: 10.1002/jwmg.513
Sodhi, N. S., Bickford, D., Diesmos, A. C., Lee, T. M., Koh, L. P., Brook, B. W., et al. (2008). Measuring the meltdown: drivers of global amphibian extinction and decline. PLoS One 3:e1636. doi: 10.1371/journal.pone.0001636
Spies, T. A. (2009). “Science of old growth, or a journey into wonderland” in Old growth in a New World: A Pacific Northwest icon reexamined. eds. T. A. Spies and S. L. Duncan (Covelo, CA: Island Press).
Spies, T. A., Long, J. W., Charnley, S., Hessburg, P. F., Marcot, B. G., Reeves, G. H., et al. (2019). Twenty-five years of the Northwest Forest Plan: what have we learned? Front. Ecol. Environ. 17, 511–520. doi: 10.1002/fee.2101
Spies, T. A., Stine, P. A., and Gravenmier, R. (2018). Synthesis of science to inform land management within the Northwest Forest Plan area. Portland, OR: US Department of Agriculture Forest Service, Pacific Northwest Research Station.
Sprugel, D. G. (1976). Dynamic structure of wave-regenerated Abies balsamea forests in the north- eastern United States. J. Ecol. 64, 889–911. doi: 10.2307/2258815
Stenzel, J. E., Bartowitz, K. J., Hartman, M. D., Lutz, J. A., Kolden, C. A., Smith, A. M., et al. (2019). Fixing a snag in carbon emissions estimates from wildfires. Glob. Chang. Biol. 25, 3985–3994. doi: 10.1111/gcb.14716
Stephens, S. L., Collins, B. M., Biber, E., and Fulé, P. Z. (2016). US federal fire and forest policy: emphasizing resilience in dry forests. Ecosphere 7:e01584. doi: 10.1002/ecs2.1584
Stephenson, N. L., Das, A. J., Condit, R., Russo, S. E., Baker, P. J., Beckman, N. G., et al. (2014). Rate of tree carbon accumulation increases continuously with tree size. Nature 507, 90–93. doi: 10.1038/nature12914
Stralberg, D., Carroll, C., and Nielsen, S. E. (2020). Toward a climate-informed North American protected areas network: incorporating climate-change refugia and corridors in conservation planning. Conserv. Lett. 13:e12712. doi: 10.1111/conl.12712
Swanson, M. E., Franklin, J. F., Beschta, R. L., Crisafulli, C. M., DellaSala, D. A., Hutto, R. L., et al. (2011). The forgotten stage of forest succession: early-successional ecosystems on forest sites. Front. Ecol. Environ. 9, 117–125. doi: 10.1890/090157
Talty, M. J., Mott Lacroix, K., Aplet, G. H., and Belote, R. T. (2020). Conservation value of national forest roadless areas. Conserv. Sci. Pract. 2:e288. doi: 10.1111/csp2.288
Tews, J., Brose, U., Grimm, V., Tielbörger, K., Wichmann, M. C., Schwager, M., et al. (2004). Animal species diversity driven by habitat heterogeneity/diversity: the importance of keystone structures. J. Biogeogr. 31, 79–92. doi: 10.1046/j.0305-0270.2003.00994.x
Thomas, J. W., Franklin, J. F., Gordon, J., and Johnson, K. N. (2006). The Northwest Forest Plan: origins, components, implementation experience, and suggestions for change. Conserv. Biol. 20, 277–287. doi: 10.1111/j.1523-1739.2006.00385.x
Trombulak, S. C. (2007). “Wilderness and wild lands in the northern Appalachian region of North America: an ecological perspective” in Science and stewardship to protect and sustain wilderness values: Eighth world wilderness congress symposium; September 30–October 6, 2005; Anchorage, AK. Proceedings RMRS-P-49. eds. A. Watson, J. Sproull, and L. Dean (Fort Collins, CO: US Department of Agriculture, Forest Service, Rocky Mountain Research Station), 510–514.
Twombly, S., and Hastings, A. (2022). New theory for increasingly tangled banks. Issues Sci. Technol. 38, 39–44.
USDA (2012). National Forest System Land Management Planning, final rule and record of decision. Fed. Regist. 77:6821162.
USDA (2022). Confronting the wildfire crisis: A 10-year implementation plan. Washington, DC: U.S. Department of Agriculture Forest Service.
USDA (2023). Forest plan amendment for planning and management of northwest forests within the range of the northern spotted owl. Fed. Regist. 88:87393.
USDA. (2024a). Amendments to land management plans to address old-growth forests across the National Forest System, Draft Environmental Impact Statement. Available online at: https://www.fs.usda.gov/project/?project=65356 (Accessed January 1, 2025).
USDA. (2024b). Mature and old-growth forests: Definition, identification, and initial inventory on lands managed by the Forest Service and Bureau of Land Management. Publication FS-1215a, Washington, DC: USDA Forest Service.
USDA. (2024c). Northwest Forest plan amendment, Draft Environmental Impact Statement. Portland, OR: USDA Forest Service Pacific Southwest and Pacific Northwest Regions.
USDA and USDI. (2024). Mature and old-growth forests: Analysis of threats on lands managed by the Forest Service and Bureau of Land Management.Publication FS-1215c. Washington, DC: USDA Forest Service.
Veldman, J. W., Buisson, E., Durigan, G., Fernandes, G. W., Le Stradic, S., Mahy, G., et al. (2015). Toward an old-growth concept for grasslands, savannas, and woodlands. Front. Ecol. Environ. 13, 154–162. doi: 10.1890/140270
Verner, J. (1992). The California spotted owl: A technical assessment of its current status : Pacific Southwest Research Station.Albany, CA: USDA Forest Service.
Watson, J. E. M., Evans, T., Venter, O., Williams, B., Tulloch, A., Stewart, C., et al. (2018). The exceptional value of intact forest ecosystems. Nat. Ecol. Evol. 2, 599–610. doi: 10.1038/s41559-018-0490-x
White House. (2021). Executive order on tackling the climate crisis at home and abroad. Available online at: https://www.federalregister.gov/documents/2021/02/01/2021-02177/tackling-the-climate-crisis-at-home-and-abroad (Accessed January 1, 2025).
White House. (2022). Executive order on strengthening the nation’s forests, communities, and economies. Available online at: https://www.federalregister.gov/documents/2022/04/27/2022-09138/strengthening-the-nations-forests-communities-and-local-economies (Accessed January 1, 2025).
Wigley, K., Armstrong, C., Smaill, S. J., Reid, N. M., Kiely, L., and Wakelin, S. A. (2024). Methane cycling in temperate forests. Carbon Balance Manag. 19:37. doi: 10.1186/s13021-024-00283-z
Keywords: biodiversity monitoring, conservation planning, ecological scale, global biodiversity framework, old-growth forest, northwest forest plan
Citation: Carroll C, Noon BR, Masino SA and Noss RF (2025) Coordinating old-growth conservation across scales of space, time, and biodiversity: lessons from the US policy debate. Front. For. Glob. Change. 8:1493879. doi: 10.3389/ffgc.2025.1493879
Edited by:
Maxence Martin, Université du Québec en Abitibi Témiscamingue, CanadaReviewed by:
Johan Svensson, Swedish University of Agricultural Sciences, SwedenJames H. Thorne, University of California, Davis, United States
Copyright © 2025 Carroll, Noon, Masino and Noss. This is an open-access article distributed under the terms of the Creative Commons Attribution License (CC BY). The use, distribution or reproduction in other forums is permitted, provided the original author(s) and the copyright owner(s) are credited and that the original publication in this journal is cited, in accordance with accepted academic practice. No use, distribution or reproduction is permitted which does not comply with these terms.
*Correspondence: Carlos Carroll, Y2FybG9zQGtsYW1hdGhjb25zZXJ2YXRpb24ub3Jn