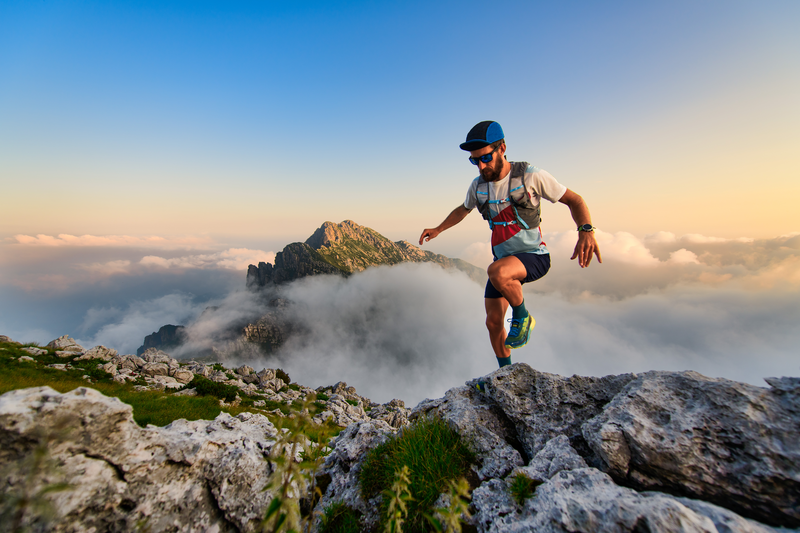
95% of researchers rate our articles as excellent or good
Learn more about the work of our research integrity team to safeguard the quality of each article we publish.
Find out more
ORIGINAL RESEARCH article
Front. For. Glob. Change , 11 December 2024
Sec. Forests and the Atmosphere
Volume 7 - 2024 | https://doi.org/10.3389/ffgc.2024.1472857
Picea neoveitchii Mast., an endemic and rare species in China, classified as Critically Endangered (CR) in the IUCN Red List of Threatened Species, possesses significant research value due to its unique biological characteristics that contribute to plant taxonomy and the conservation of the genus Picea. Despite its excellent timber quality and high ornamental value, it has not been widely used and well protected. This study employed the MaxEnt modeling approach, incorporating field survey data on species distribution and species distribution data collected online, along with climatic and environmental data sourced from IPCC reports and climate databases. It assessed the current and future distribution ranges, influential variables, and conservation status of P. neoveitchii. The results indicate that Minimum Temperature of the Coldest Month, Annual Precipitation, Temperature Seasonality, and Altitude are the key factors influencing the distribution of P. neoveitchii. Across all future climate scenarios, the suitable habitat for P. neoveitchii consistently exhibits a trend of shifting northwestwards. Under SSP2-4.5, SSP5-5.8 scenario, the suitable area decreases in all periods. Under SSP1-2.6 scenario, the suitable area decreases, except the period from 2080 to 2,100, which sightly increases. Notably, the proportion of habitat within natural reserves increased. To conserve P. neoveitchii, it is necessary to adopt in-situ and ex-situ conservation measures, conserve germplasm resources through seed banking and tissue culture, and implement strict enclosure policies to minimize human disturbance and promote natural regeneration. These findings highlight the need for targeted conservation measures to address the ongoing threat to this species.
According to the Intergovernmental Panel on Climate Change (IPCC) Sixth Assessment Report (Intergovernmental Panel on Climate, 2023), human activities have already caused global temperatures to rise by 1.1°C, with projections indicating an increase exceeding 1.5°C within the next 20 years. In China, the changes have been notably pronounced, with temperatures rising by 0.90–1.52°C in recent years, exceeding the global average level (Ding and Wang, 2016). Anticipated consequences of global warming encompass alterations in species distributions, fluctuations in population sizes, and modifications to genetic diversity (Bertrand et al., 2011), These phenomena precipitate species migrations, increase vulnerability to endangerment, and potentially culminate in extinction events (He et al., 2023; Li R. et al., 2024; Tiwary et al., 2024; Wang et al., 2020). The mid-Holocene epoch witnessed a pronounced warm period, which facilitated a modest northward shift of the forest belt in Eurasia’s mid-latitude regions, paralleling the relocation trends observed in China’s warm-temperate evergreen broadleaf and mixed forest ecosystems due to augmented temperatures and humidity levels (Ni et al., 2010; Yu, 2008). Clearly, climate profoundly influences species distribution ranges at a large scale, serving as a key factor (Tiwary et al., 2024; Wilson et al., 2021). Understanding these alterations is crucial for developing effective conservation strategies aimed at protecting endangered plant species and maintaining biodiversity.
Species distribution models (SDMs) have emerged as pivotal tools for predicting species’ spatial distributions, with a particular emphasis on their invaluable contribution to endangered species conservation (Bhardwaj, 2023; Noce et al., 2023). In the context of climate change, these models offer crucial insights into the potential impacts on species distributions and habitats, thereby guiding conservation efforts and strategies (Li Y. et al., 2024). Advances in mathematical modeling and Geographic Information Systems (GIS) have facilitated the widespread utilization of diverse SDM frameworks, notably the Maximum Entropy (MaxEnt) model (Phillips et al., 2006), the Bioclimatic Climate Model (BIOCLM) (Booth et al., 2014), the Genetic Algorithm for Rule Set Production (GARP) (Stockwell, 1999), and the Generalized Additive Model (GAM) (Yee and Mitchell, 1991), among others. Each of these models contributes uniquely to our understanding of species distributions and their vulnerabilities to climate change, ultimately informing more effective conservation interventions for endangered species. The MaxEnt model stands out due to its ability to generate highly accurate predictions even with limited sample sizes, and its capacity to produce spatially resolved habitat suitability maps that efficiently quantify the importance of individual environmental factors (Dong et al., 2024; Guisan et al., 2007; Mothes et al., 2019). Its extensive application in forecasting plant distributions, particularly those of endangered species, at both national and global extents (Lu et al., 2021; Srivastava et al., 2021; Tiwary et al., 2024; Yang et al., 2024), underscores its pivotal role in bolstering conservation and restoration endeavors focused on imperiled plant species.
Picea neoveitchii Mast., an evergreen conifer belonging to the Pinaceae family and specifically the Picea genus, classified as Critically Endangered (CR) in the IUCN Red List of Threatened Species (Qin et al., 2017; Zhang et al., 2013), has been designated as a second-tier protected wild plant within the jurisdiction of China, underscoring its significance and vulnerability in natural ecosystems (Qin et al., 2017). It is a rare and endangered species endemic to central and northwestern China. P. neoveitchii features straight trunks and high-quality wood, which is an ideal material for greening, construction, and furniture manufacturing. It is contributing significantly to the study of the formation history, developmental dynamics of plant floristics in the Qinling Mountains, and the evolutionary aspects of gymnosperms (Song et al., 2011). However, over the past few decades, excessive logging has led to a drastic decline in the population of P. neoveitchii (Chang et al., 2019). The high habitat requirements and the lack of efficient reproduction methods have made population recovery challenging for this species (Bond, 2008; Ma et al., 2016). Moreover, recent climatic alterations have intensified anxieties regarding the persistence of the species, compelling reassessments of its climatic adaptability and spatial distribution boundaries (Ballardin et al., 2024; Paudel et al., 2024). Currently, extensive pure stands of P. neoveitchii have nearly disappeared, and the remaining populations face severe threats to their survival (Liang et al., 2022).
Recently, considerable attention has been devoted to the effects of climate change on angiosperm distribution ranges, as evidenced by prior studies (Li Y. et al., 2024; Ouahzizi et al., 2024; Zhang et al., 2018; Zhao et al., 2021). However, research on how climate change affects the distribution ranges of gymnosperms remains relatively limited. Gymnosperms, distinguished by their distinct growth environments, reproductive methods, and adaptability to diverse conditions, exhibit a higher vulnerability to the impacts of climate change compared to angiosperms (Dad et al., 2023). This vulnerability is exacerbated by their tendency to be outcompeted by angiosperms due to the latter’s superior reproductive strategies, biochemical defenses, and growth form diversity (Ma et al., 2016; Shi et al., 2024). Consequently, gymnosperms often inhabit higher latitudes or elevations, making them a marginal and threatened group within spermatophytes (Rudley et al., 2023).
Addressing this research gap, particularly in relation to gymnosperm species, is crucial for understanding and mitigating the potential impacts of climate change on global biodiversity. Hence, amidst the intensification of global climate change impacts, a pressing necessity arises to enhance scrutiny of the viability of gymnosperms, notably P. neoveitchii, and expeditiously adopt conservation interventions. Ensuring the persistence of these endangered species’ existence conditions is paramount. Currently, while the endangered status of P. neoveitchii has garnered attention among some Chinese scholars, the majority of research has focused on in-situ conservation, endangered mechanisms, and community characteristics. However, these studies have not clearly elucidated the coupled effects between P. neoveitchii and environmental factors, nor have they predicted population trends and survival strategies (Chang et al., 2019; Chen et al., 2012; Song et al., 2011). Therefore, there is an urgent need for further in-depth studies to address these issues, facilitating more effective conservation of this endangered species.
To assess the habitat distribution characteristics of P. neoveitchii and the environmental factors influencing its habitat suitability, this study employed the Maxent model to predict its distribution in China. This prediction was based on a comprehensive dataset comprising 103 distribution records of P. neoveitchii retrieved from online databases containing plant distribution information, as well as from three field surveys conducted in the Qinling Mountains and Gansu Province. The primary objectives of the present investigation encompassed: (i) identifying the paramount environmental variables influencing the geographic distribution of P. neoveitchii; (ii) modeling contemporary and projected suitable habitats for P. neoveitchii across diverse climatic scenarios; (iii) quantifying the temporal and scenario-based shifts in the centroid of its suitable habitat; and (iv) evaluating the distribution of protected areas within the species’ range to inform the development of effective conservation strategies.
The overarching workflow employed for conducting the analyses is outlined in Figure 1.
To gather a comprehensive dataset of the plant’s distribution within its range, we conducted exhaustive literature reviews utilizing online databases, incorporating references sourced from the Global Biodiversity Information Facility (GBIF, https://www.gbif.org/) and the Chinese Virtual Herbarium (CVH, http://v5.cvh.org.cn/). In cases where distribution records lacked complete geographic coordinates, we employed Google Earth1 to ascertain precise latitudes and longitudes.
Fieldwork was conducted from July 2022 to September 2023. It covered potential distribution zones such as the Qinling-Bashan Mountains and Daba Mountains (Supplementary Figure S1). Survey methods included setting up systematic transects in different ecological habitats, making direct observations to record individual plants, using high-precision GPS for precise localization, and, in some cases, collecting specimens for later morphological identification. To ensure data quality, duplicate records were eliminated, and spatial filtering using ENM Tools was applied to guarantee one locality per 1 km2 grid cell. This resulted in a final dataset comprising 77 unique distribution points. ArcGIS 10.3 software was then utilized to create a distribution map based on these data, providing valuable insights for subsequent research endeavors and confirming the accuracy and reliability of the distribution dataset (Figure 2).
To fully consider the relationship between the distribution of suitable area for tree species and environmental factors, data on climate, soil, topography and vegetation classification were selected for model calculations, with a spatial resolution of 30s. Current and projected (2021–2,100) climate data were obtained from WorldClim.2 Future climate scenarios were chosen from the BCC-CSM2-MR climate model within CMIP6, suitable for China’s geographical environment, which includes the sustainable development pathway limiting temperature increase to 2°C (SSP1-2.6), the moderate development pathway limiting temperature increase to 3°C (SSP2-4.5), and the conventional development pathway limiting temperature increase to 5°C (SSP5-8.5). Topographical variables, including elevation, slope, and aspect, were acquired from the Geographical National Condition Monitoring Cloud Platform3 and further processed using ArcGIS software. Additionally, soil variables comprising 56 factors were sourced from the Harmonized World Soil Database. The 1:1,000,000 Vegetation Data Set, sourced from the National Cryosphere Desert Data Center,4 was utilized for acquiring vegetation classification data for China. The distribution data of nature reserves in China were sourced from the Resource and Environment Data Cloud Platform of the Chinese Academy of Sciences.5 The study employed a 1:4,000,000 scale map of China along with its corresponding administrative division map, both sourced from the National Fundamental Geographic Information System.6
To mitigate the risk of overfitting in model predictions due to multicollinearity among environmental variables, correlation analysis was conducted using ENMTools. A Pearson correlation coefficient of 0.8 was defined as the threshold. For any two environmental factors with an absolute correlation coefficient ≥ 0.8, the one with the higher contribution rate based on the initial MaxEnt model results was retained (Supplementary Figure S2). Ultimately, 32 variables were selected from the initial 68 for evaluation purposes, as detailed in Supplementary Table S1.
The species distribution spot data, along with the processed environmental variables, were incorporated into the MaxEnt model for comprehensive analysis (Phillips et al., 2006) (Maxent version 3.4.4; http://www.cs.princeton.edu/wschapire/Maxent/). During model operation, data were randomly sampled and divided into a training subset (75% of the total data) and a testing subset (25% of the total data). The bootstrap method was chosen for replicated runs, while the jackknife technique was employed to quantify the individual contribution of environmental factors to model development (Peterson and Cohoon, 1999). Response curves were generated to show the relationship between species suitable area and climate factors (Moreno et al., 2011). The model was run 10 times, and the average of these ten runs was used as the final output. The accuracy of the model was evaluated with the area under the Receiver Operating Characteristic curve (AUC) (Supplementary Figure S3).
The final output is the potential habitat suitability distribution data for the species in the form of a habitat suitability index (HSI) ranging from 0 to 1. Regions with HSI values greater than or equal to the maximum test sensitivity plus specificity logistic threshold are considered suitable area for the species. These regions were subsequently categorized into four potential habitat classes: “high suitable” (>0.6), “medium suitable” (0.4–0.6), “low suitable” (0.2–0.4), and “not suitable” (<0.2).
Utilizing current climate data, this study simulated the suitable area range of P. neoveitchii and then conducted predictive simulations for three prospective climate scenarios (SSP1-2.6, SSP2-4.5, SSP5-8.5) to anticipate future habitat suitability. The simulation outcomes categorized future habitat polygons into three distinct groups: Expansion, indicating areas where suitability is projected to increase; Shrinkage, denoting regions where suitability is anticipated to decrease; and Stability, reflecting zones where suitability is forecasted to remain unchanged. Following this classification, the spatial extents of these categories were quantified and comprehensively described.
Utilizing the SDM Toolbox, a Python-powered GIS application, regions exhibiting HSI values exceeding 0.2 were designated as potentially suitable habitats, whereas those with HSI values below 0.2 were classified as unsuitable for the species’ persistence. This categorical distinction facilitated the subsequent analysis of habitat distributional changes. Subsequently, the changes in potential suitable area for P. neoveitchii under different climate scenarios were analyzed and categorized into expansion areas, stable areas, and contraction areas. The areas of these regions were then calculated (Cong et al., 2020).
Furthermore, utilizing the SDM Toolbox, we conducted a comparative analysis of the central points (centroids) within the current and projected suitable habitat areas to discern potential shifts in spatial distribution (Brown, 2014). Specifically, the centroids of P. neoveitchii distribution points under different climate scenarios were calculated first. Then, centroid vector files containing distance and direction information were created. Finally, the movement trajectories of the centroids were analyzed to confirm the change trends of the distribution of P. neoveitchii (Kong et al., 2021).
In this study, the ArcGIS platform was employed to quantitatively assess and interpret the spatial intersection between the projected suitable habitats of P. neoveitchii and the boundaries of designated nature reserves across varying temporal horizons. By adopting this methodology, we gain insights into the conservation priorities for P. neoveitchii, encompassing both contemporary climatic regimes and projected future scenarios, thereby facilitating the assessment of its vulnerability and resilience. Additionally, based on the overlap analysis results, this research identifies key areas for future conservation efforts, providing a basis for developing scientific conservation strategies.
The species distribution model was evaluated on the designated training and testing datasets. It exhibited an AUC score of 0.985, and the standard deviation is 0.004. This score is comparable to or even surpasses the typical AUC values reported in similar studies. For example, in a vulnerability assessment of Taxus wallichiana in the Indian Himalayan Region by Tiwary et al. (2024), the average training AUC was 0.96. The high AUC score of our model indicates excellent performance and reliable predictive results (Supplementary Figure S3), supporting the robustness and accuracy of the findings. Based on the current assessment, the total area of “high suitable” and “medium suitable” habitats for P. neoveitchii is approximately 1,740,000 square kilometers, primarily distributed in southeastern Gansu, southern Shaanxi, western Henan, and western Hubei. Observing the topographical map of China, the distribution areas are mainly located in the sunny slopes, semi-shaded slopes, and cliff areas of the Qinling-Bashan Mountains, Wushan Mountains, Wuyi Mountains, Hengduan Mountains, and their surrounding areas (Figure 3).
The MaxEnt framework evaluated the significance of various factors. The minimum temperature during the coldest month (Bio 6) emerged as the most influential, contributing 29.1% to model variation. Annual precipitation (Bio 12) followed closely, with a contribution of 25.1%. Temperature seasonality (Bio 4) and altitude also played significant roles, accounting for 9 and 7.7%, respectively. Together, these four factors comprised 70.9% of the model’s predictive power (Supplementary Table S1).
Response curves were utilized to determine the optimal bioclimatic conditions for P. neoveitchii. An HSI threshold of 0.2 was established. Climates conducive to P. neoveitchii’s growth are characterized by relatively cold temperatures that are not excessively low, moderate and stable precipitation, and distinct seasonal temperature variations. Higher altitudes, approximately 2,100 meters, provide a more favorable growth environment for the species (Figure 4).
The MaxEnt model simulations projected an estimated potential suitable habitat of approximately 4,226,000 km2 for P. neoveitchii within China’s borders, constituting 4.48% of the country’s total territorial area (Supplementary Table S2). Under various projected future climatic scenarios, the extent of viable habitats exhibits a declining trend punctuated by periods of variability. Notably, the SSP5-8.5 scenario manifests the most pronounced decline in the extent of suitable habitats, particularly those of high suitability, while the SSP2-4.5 scenario exhibits a lesser yet notable decrease. In contrast, the SSP1-2.6 scenario demonstrates minimal alteration, preserving the largest extent of viable habitats. Remarkably, within this climate trajectory, the suitable habitat area experiences a marginal increase of 0.05% between 2080 and 2,100, as compared to the current projected distribution (Figure 5), underscoring its resilience under mitigated climate change. Regarding geographical shifts in habitat suitability, the SSP5-8.5 scenario elicits the most pronounced alterations in the extent of suitable habitat for P. neoveitchii, particularly evident in the northwestward and southeastward directions. The SSP2-4.5 scenario follows with a lesser degree of variation, while the SSP1-2.6 scenario exhibits minimal geographical redistribution (Figure 6).
Figure 5. The area of different grades of suitable habitats under various scenario periods and the trend of changes over time in the total suitable habitat area under each scenario (* denotes the maximum and minimum areas, respectively; dashed lines indicate trends in area changes).
Figure 6. Example diagram of Shrinkage and expansion of suitable area (including some example maps).
The observed changes are closely linked to the degree of temperature increase under the three different climate scenarios. From SSP1-2.6 to SSP5-8.5, global warming increases significantly. Under the SSP1-2.6 scenario, global temperature increase is strictly limited to 2°C, resulting in relatively minor impacts on P. neoveitchii. Therefore, although the area of suitable habitats may decrease in the mid-term (2040–2060), it can recover to slightly above the current level in the long term (2080–2,100). This finding suggests that P. neoveitchii, under the auspices of a sustainable development trajectory, possesses a degree of climatic adaptability, potentially maintaining or even slightly expanding its suitable habitat area. In contrast, under the SSP5-8.5 scenario, global warming reaches 5°C, a relatively high level of temperature increase. The area of suitable habitats for P. neoveitchii decreases significantly, suggesting that under the conventional development pathway, P. neoveitchii will face immense survival pressure, leading to a substantial reduction in its suitable habitat range. The spatial distribution changes are also the most significant under this scenario, probably due to its sensitivity to extreme climate conditions such as high temperatures and droughts. In comparison, under the SSP2-4.5 scenario, the global temperature increase was limited to 3°C, falling between the other two scenarios. Therefore, both the area and spatial distribution changes of suitable habitats are intermediate between those observed in the SSP1-2.6 and SSP5-8.5 scenarios.
Currently, the centroid of P. neoveitchii distribution is situated in Ziyang County, Ankang City, Shaanxi Province, China (108°18′E, 32°33′N). Predictions under three distinct climate scenarios indicate that by 2080–2,100, the centroid’s migration trend predominantly leans northwestward, albeit with scenario-specific variations in path and distance. Specifically, under SSP1-2.6, the centroid is projected to relocate to Huixian County, Longnan City, Gansu Province (106°24′E, 33°40′N), approximately 215 km from its current position. In SSP2-4.5, the migration path is basically the same as in SSP1-2.6, but extends further, concluding in Huixian County, Longnan City, Gansu Province (105°43′E, 34°9′N), about 300 km away. SSP5-8.5 forecasts a more significant northward shift, settling in Lingtai County, Pingliang City, Gansu Province (107°9′E, 34°59′N), approximately 290 km distant from the current position (Figure 7). Furthermore, the importance of geographical variability, particularly in relation to conservation efforts at higher altitudes, is underscored by the observed migration trends of P. neoveitchii. As temperatures rise, the species is projected to migrate further towards higher latitudes and altitudes, seeking cooler environments. This migration towards higher altitudes not only highlights the species’ adaptive capacity but also poses significant conservation challenges. Effective conservation strategies must consider the shifting habitat requirements of P. neoveitchii and prioritize the protection of habitats at higher altitudes, which are likely to become crucial refuge areas under future climate scenarios.
Figure 7. Predicted the centroid distributional shifts under different climate scenario/year for P. neoveitchii.
It is noteworthy that across the four time periods from present to 2,100 under the three scenarios, the centroid exhibits a significant fluctuation towards the northwest direction during the period of 2060–2080. However, the amplitude of these fluctuations varies among scenarios, with SSP5-8.5 showing the largest fluctuation. Under this scenario, the centroid even shifts to the northeast of Gansu Province during 2060–2080, before settling approximately 107 kilometers northeast of its position during 2040–2060 by 2080–2,100. SSP2-4.5 exhibits a lesser fluctuation, with the centroid ending up approximately 40 kilometers away from its position during 2040–2060. SSP1-2.6, on the other hand, displays the smallest fluctuation, with the centroid in 2080–2,100 closely approximating its position during 2040–2060, just 5 kilometers away. These fluctuations are speculated to correlate with future climate variability. P. neoveitchii shows stronger adaptability and resilience under scenarios with lower temperature increases. Conversely, under SSP5-8.5, characterized by a higher temperature increase within the conventional development pathway, the centroid’s fluctuation is the most significant. This reflects significant challenges P. neoveitchii faces in adapting to new environments under extreme climate conditions. While the centroid returns somewhat by 2080–2,100, its final position is considerably farther northeast, approximately 107 kilometers away from its original location. This suggests that high temperatures pose substantial pressure and uncertainty during the migration of P. neoveitchii, making it difficult to find stable habitats and moving away from its ecological resources.
The protection work of P. neoveitchii currently faces severe challenge, with protected areas covering only 6.48% of its distribution range. These protected areas are mainly concentrated in central Sichuan Province and the border areas of Chongqing, Hubei, and parts of Hubei. However, in critical regions of its native distribution, such as western Gansu, southern Shaanxi, western Hubei, and western Henan, the coverage of nature reserves is notably insufficient. Moreover, these protected areas are severely fragmented, posing serious challenges to the effectiveness in their conservation (Figure 8).
Figure 8. Distribution of protected areas within the suitable area for P. neoveitchii (including some example maps).
Facing the uncertainties brought by future climate change, the conservation status of P. neoveitchii appears to be undergoing a positive transformation. Across three distinct climate scenarios (SSP1-2.6, SSP2-4.5, SSP5-8.5), the projected gradual reduction in the suitable habitat area for P. neoveitchii is counterbalanced by an impending increase in the proportion of national nature reserves encompassed within these areas (Supplementary Figure S2). Of particular note is the projection that, within the timeframe spanning 2080 to 2,100, under the most severe climate scenario (SSP5-8.5), the proportion of national natural reserves residing within the suitable habitat area of P. neoveitchii is anticipated to reach 10.2%. For SSP2-4.5 and SSP1-2.6, these proportions are projected to be 8.66 and 7.52%, respectively. This positive trend may be associated with the potential northwestward migration of P. neoveitchii as predicted by the models. Natural reserves in northwest China are relatively more intact and more extensive. Thus, although the suitable habitat area for P. neoveitchii decreases, they may migrate to these more comprehensive natural reserves, which provides a relatively optimistic outlook for the conservation of P. neoveitchii.
P. neoveitchii holds the status of a second-tier key protected wild plant in China, distinguished as a rare and endangered species that is uniquely endemic to the country. Employing 77 distribution records and 32 environmental variables, this research endeavor leveraged the MaxEnt model to forecast the habitat suitability of P. neoveitchii across China. Notably, for the first time, exhaustive assessments were undertaken to scrutinize both the present and projected suitable habitats of P. neoveitchii amidst varying climate scenarios.
The distribution patterns of species are shaped by a complex interplay of both abiotic and biotic factors. Abiotic factors include climate, terrain, and soil properties, while biotic factors encompass competition dynamics, growth traits, dispersal and migration capabilities, natural regeneration processes, herbivory, and various human-induced impacts such as deforestation, overgrazing, tourism pressures, and industrial and mining activities (Ballardin et al., 2024; Campedelli et al., 2015; Wesemeyer et al., 2023). Exemplified by the Qilian Mountains, where from 1989 to 1998, a surge in human activities resulted in a marked decline of 33.5% in forest vegetation, thereby contributing to the degradation of forest ecosystems (Guo et al., 2019). Furthermore, limitations in species occurrence data can introduce deviations between simulated results and actual species locations. Additionally, the modeling accuracy for estimating species’ geographical distribution ranges may be impacted by variations in the resolution of selected environmental variables. This study aimed to mitigate such errors by utilizing field-based distribution data collected since 2022, eliminating redundant distribution data, removing some environmental data with high correlation coefficients, and performing multiple iterations. The evaluation metrics, notably the Area Under the Receiver Operating Characteristic Curve (AUC) and the training-based omission rate, underscore the reasonability of the delineated distribution range for P. neoveitchii in this investigation. These findings carry substantial implications for the strategic cultivation, effective conservation, comprehensive management, and sustainable utilization strategies of this species.
The spatial distribution of plant species at a given scale is primarily governed by climatic determinants, with hydrothermal parameters (pertaining to water and heat) occupying a paramount position in influencing this distribution (Tiwary et al., 2024). Model simulations have revealed that Min Temperature of Coldest Month, Annual Precipitation and Temperature Seasonality are the primary climatic factors influencing the distribution of P. neoveitchii. Specifically, lower temperatures, moderate and stable precipitation and distinct seasonal temperature variations are particularly favorable for the growth of P. neoveitchii. Our discovery converges significantly with the findings presented by Dad et al. (2023), who identified Annual Precipitation and annual mean temperature as the three pivotal environmental factors that exert the most profound influence on the distribution of wild gymnosperms in the northwestern Himalayan region. The slight differences between the two conclusions can be attributed to variations in plant species and study areas.
In the modeling calculations, apart from climate, soil conditions also have a significant, albeit relatively lesser, impact on the growth of P. neoveitchii. The species prefers gravelly alluvial soil, which offers excellent drainage and aeration properties, essential for healthy root growth. From a topographical perspective, the formation of alluvial soil is closely associated with hydrodynamic processes such as rivers and floods, indicating that P. neoveitchii may be more adapted to environments like wide river valleys, riverbanks, and floodplains adjacent to plains. Simultaneously, the survival probability of P. neoveitchii is closely associated with the vegetation type in which it resides. According to model analyses, the most suitable vegetation type for P. neoveitchii is subtropical coniferous and broad-leaved mixed forests. However, this vegetation type has a relatively small distribution area in China, primarily located in the south-central and western regions of Yunnan, the western part of Sichuan, the southeastern section of Tibet, and the central mountainous areas of Taiwan. These vegetation types are mainly distributed in the mountainous environments of the mid-subtropical and temperate zones, which aligns with their actual distribution. This discovery not only reveals the ecological habits and distribution patterns of P. neoveitchii, but also provides important insights for the development of relevant protection measures. In future conservation efforts, we should prioritize areas with suitable vegetation types and employ measures such as afforestation and ecological restoration to create a better habitat for rare plants like P. neoveitchii.
The future climate scenarios are projected to have significant impacts on both the size and distribution range of viable habitats for P. neoveitchii. Specifically, adhering to the Sustainable Development Scenario (SSP1-2.6), the predicted trend indicates that the suitable habitat area for P. neoveitchii will maintain stability or undergo a modest expansion. However, under the Intermediate Development Pathway (SSP2-4.5) and the Conventional Development Pathway (SSP5-8.5), its suitable habitat area will be reduced and disrupted to varying degrees. Our forecast concurs with the conclusions outlined in the Sixth Assessment Report of the Intergovernmental Panel on Climate Change (IPCC) published in 2023 (Intergovernmental Panel on Climate, 2023), reinforcing a shared understanding. Additionally, it harmonizes with the research findings reported by Gao et al. (2021), who explored the evolving patterns of suitable habitat for European spruce under future climatic scenarios. The modeling results indicate that under current climatic conditions, the distribution center of P. neoveitchii is located in Ziyang County, Ankang City, Shaanxi Province (108°18′E, 32°33′N). Under three different future climate scenarios, the migration direction of P. neoveitchii is generally northwest, though the specific paths and distances vary depending on the scenario. Our findings are congruent with the conclusions presented in the IPCC’s AR6 (Intergovernmental Panel on Climate, 2023), indicating that climatic alterations are anticipated to exert pronounced effects on ecological systems located within specific low-latitude areas of the Northern Hemisphere. Many plant species may adapt to climate change by migrating to higher latitudes or altitudes. Furthermore, our findings resonate with the projections made by He et al. (2023) and Xiao et al. (2024), as well as those reported in another study (Duan et al., 2022), which showed that the distribution center of P. densiflora shifts to a small northward migration from current to future climate scenarios. While all these studies anticipate a northwestward migration of alpine coniferous forests in response to ongoing climate warming trends, the specific migration distances and angles may vary slightly among different plant species.
Facing the uncertainties brought by future climate change, the conservation status of P. neoveitchii shows a positive shift. Despite the projected gradual reduction in the extent of suitable habitat for P. neoveitchii, the fraction of this habitat encompassed within national nature reserves demonstrates an upward trajectory, affording a moderately optimistic perspective on its conservation prospects. Nonetheless, it is crucial to acknowledge that the model’s projections solely rely on climatic simulations and may therefore possess inherent limitations. Given that P. neoveitchii is currently endangered and the populations are highly isolated from each other, it remains uncertain whether P. neoveitchii can migrate northwestward through its own dispersal mechanisms and the extent of such migration.
To address this scenario, strategic interventions encompassing in-situ and ex-situ conservation efforts, safeguarding germplasm repositories, implementing stringent enclosure measures, and enhancing reproductive programs on a larger scale ought to be prioritized. (1) Firstly, efforts should be intensified to protect the native distribution areas of P. neoveitchii, especially the regions where protected areas are severely fragmented. By restoring and connecting fragmented reserves, a more stable and continuous habitat for P. neoveitchii can be provided. (2) Secondly, a germplasm bank for P. neoveitchii should be established. The favorable conditions of mature individuals, such as good seed production and high germination rates, should be utilized optimally by timely and appropriate seed collection. Nurseries should be established locally to expand the artificial populations and study rapid propagation methods for P. neoveitchii. Additionally, transplantation and seedling cultivation among natural populations should be conducted to ensure genetic exchange and maximize the genetic diversity of P. neoveitchii. This would provide germplasm and materials for further breeding and in-depth conservation efforts. (3) Furthermore, the migration process of P. neoveitchii needs to be monitored and studied to understand its migration routes, speed, and quantity. This information would help in accurately predicting its future distribution and guide the planning and construction of protected areas to ensure comprehensive protection in new distribution areas. (4) Finally, promoting the implementation of sustainable development strategies to limit global warming levels is also crucial for preserving the habitat of P. neoveitchii and other organisms.
Currently, research on P. neoveitchii primarily focuses on population size, community characteristics, and endangerment mechanisms, with relatively little attention given to habitat simulation, cultivation methods, and seed germination. Research on P. neoveitchii should be deepened further into ecological physiology and molecular levels, thereby gaining a detailed understanding of their growth dynamics and other related issues. An in-depth study of P. neoveitchii would allow for a comprehensive analysis of the causes of its endangerment, intra-population relationships, seed germination patterns, and genetic differentiation. These insights could then help to develop more effective conservation measures, ultimately aiming to rescue this “living fossil” from endangerment.
This study thoroughly analyzed the suitable habitats of P. neoveitchii under current climate conditions and future climate scenarios, providing a scientific basis for formulating conservation strategies. Our findings underscore Minimum Temperature of the Coldest Month, Annual Precipitation, Temperature Seasonality, and Altitude as pivotal determinants of P. neoveitchii’s distribution. Across diverse climate projections, the species’ suitable habitat exhibits a northwestern shift. Notably, under SSP2-4.5 and SSP5-8.5 scenarios, a decline in suitable area is observed across all time frames. Conversely, under SSP1-2.6, while the suitable area diminishes in most periods, a marginal expansion is discernible from 2080 to 2,100, accompanied by an increase in the proportion of reserve-encompassed habitat.
To address these findings, we propose: (1) In-situ and ex-situ conservation to safeguard populations and genetic diversity; (2) Germplasm resource conservation through seed banking and tissue culture; and (3) Strict enclosure policies to minimize human disturbance and promote natural regeneration. These findings are critical for shaping future forest management and targeted conservation strategies, with profound implications for the preservation of P. neoveitchii, the Picea genus, and the broader forest ecosystem. They offer invaluable insights for guiding future forest conservation and management endeavors.
The original contributions presented in the study are included in the article/Supplementary material, further inquiries can be directed to the corresponding authors.
NX: Writing – original draft. KL: Writing – review & editing. KC: Writing – review & editing. PL: Writing – review & editing. XJ: Writing – review & editing. ZM: Supervision, Writing – review & editing, Investigation. WJ: Funding acquisition, Supervision, Writing – review & editing.
The author(s) declare financial support was received for the research, authorship, and/or publication of this article. This study was financially supported by Shaanxi Provincial Forestry Reform and Development Project 2023 (SXDY2023-01) and Shaanxi Province Key Research and Development Plan (2023-YBNY-002).
The authors declare that the research was conducted in the absence of any commercial or financial relationships that could be construed as a potential conflict of interest.
All claims expressed in this article are solely those of the authors and do not necessarily represent those of their affiliated organizations, or those of the publisher, the editors and the reviewers. Any product that may be evaluated in this article, or claim that may be made by its manufacturer, is not guaranteed or endorsed by the publisher.
The Supplementary material for this article can be found online at: https://www.frontiersin.org/articles/10.3389/ffgc.2024.1472857/full#supplementary-material
Ballardin, S., Belladona, R., De Vargas, T., Bosco, V. D., Bortolin, T. A., and Roehe Reginato, P. A. (2024). MaxEnt machine learning model predicts high groundwater potential areas in a fractured volcanic aquifer system. J. S. Am. Earth Sci. 135:104794. doi: 10.1016/j.jsames.2024.104794
Bertrand, R., Lenoir, J., Piedallu, C., Riofrío-Dillon, G., de Ruffray, P., Vidal, C., et al. (2011). Changes in plant community composition lag behind climate warming in lowland forests. Nature 479, 517–520. doi: 10.1038/nature10548
Bhardwaj, V. (2023). “Taxus wallichiana Zucc. (Himalayan yew): a medicinal plant exhibiting antibacterial properties” in Advances in microbiology, infectious diseases and public health. ed. G. Donelli, vol. 17 (Cham: Springer International Publishing), 145–153. doi: 10.1007/5584_2023_772
Bond, W. (2008). The tortoise and the hare: ecology of angiosperm dominance and gymnosperm persistence. Biol. J. Linn. Soc. 36, 227–249. doi: 10.1111/j.1095-8312.1989.tb00492.x
Booth, T. H., Nix, H. A., Busby, J. R., and Hutchinson, M. F. (2014). BIOCLIM: the first species distribution modelling package, its early applications and relevance to most current MAXENT studies. Diversity and Distributions, 20, 1–9. doi: 10.1111/ddi.12144
Brown, J. L. (2014). SDMtoolbox: a python-based GIS toolkit for landscape genetic, biogeographic and species distribution model analyses. Methods Ecol. Evol. 5, 694–700. doi: 10.1111/2041-210X.12200
Campedelli, T., Londi, G., Gioia, G. L., Frassanito, A. G., and Florenzano, G. T. (2015). Steppes vs. crops: is cohabitation for biodiversity possible? Lessons from a national park in southern Italy. Agric. Ecosyst. Environ. 213, 32–38. doi: 10.1016/j.agee.2015.07.012
Chang, P., Zhu, L., Zhao, M., Li, C., Zhang, Y., and Li, L. (2019). The first transcriptome sequencing and analysis of the endangered plant species Picea neoveitchii mast. And potential EST-SSR markers development. Biotechnol. Biotechnol. Equip. 33, 967–973. doi: 10.1080/13102818.2019.1632739
Chen, W.-Q., Song, Z.-J., and Xu, H.-H. (2012). A new antifungal and cytotoxic C-methylated flavone glycoside from Picea neoveitchii. Bioorg. Med. Chem. Lett. 22, 5819–5822. doi: 10.1016/j.bmcl.2012.07.089
Cong, M., Xu, Y., Tang, L., Yang, W., and Jian, M. (2020). Predicting the dynamic distribution of Sphagnum bogs in China under climate change since the last interglacial period. PLoS One 15:e0230969. doi: 10.1371/journal.pone.0230969
Dad, J. M., Rashid, I., and Chen, A. P. (2023). Is climate change pushing gymnosperms against the wall in the northwestern Himalayas? Reg. Environ. Chang. 23:51. doi: 10.1007/s10113-023-02050-1
Ding, Y., and Wang, H. (2016). Newly acquired knowledge on the scientific issues related to climate change over the recent 100 years in China. Chin. Sci. Bull. 61, 1029–1041. doi: 10.1360/N972015-00638
Dong, J., Bai, T., Gao, Z. S., Yang, S. W., Zhang, J. L., Wu, Y. W., et al. (2024). Prediction of potential habitat areas of Rhododendron delavayi in China based on maximum entropy model MaxEnt. Front. Forests Glob. Change 7:823. doi: 10.3389/ffgc.2024.1360823
Duan, X. G., Li, J. Q., and Wu, S. H. (2022). ). MaxEnt modeling to estimate the impact of climate factors on distribution of Pinus densiflora. Forests 13:402. doi: 10.3390/f13030402
Gao, Y., He, Z. B., Zhu, X., Chen, L. F., Du, J., Lin, P. F., et al. (2021). Potential distribution and habitat suitability of Picea crassifolia with climate change scenarios. Can. J. For. Res. 51, 1903–1915. doi: 10.1139/cjfr-2020-0507
Guisan, A., Zimmermann, N. E., Elith, J., Graham, C. H., Phillips, S., and Peterson, A. T. (2007). What matters for predicting the occurrences of trees: techniques, data, or species' characteristics? Ecol. Monogr. 77, 615–630. doi: 10.1890/06-1060.1
Guo, Y., Guo, J., Shen, X., Wang, G. B., and Wang, T. L. (2019). Predicting the bioclimatic habitat suitability of Ginkgo biloba L. in China with field-test validations. Forests 10:705. doi: 10.3390/f10080705
He, Y., Ma, J., and Chen, G. (2023). Potential geographical distribution and its multi-factor analysis of Pinus massoniana in China based on the maxent model. Ecol. Indic. 154:110790. doi: 10.1016/j.ecolind.2023.110790
Intergovernmental Panel on Climate (2023). Climate change 2021 – the physical science basis: working group I contribution to the sixth assessment report of the intergovernmental panel on climate change. Cambridge: Cambridge University Press.
Kong, F., Tang, L., He, H., Yang, F. X., Tao, J., and Wang, W. C. (2021). Assessing the impact of climate change on the distribution of Osmanthus fragrans using Maxent. Environ. Sci. Pollut. Res. 28, 34655–34663. doi: 10.1007/s11356-021-13121-3
Li, R., Hu, X., Li, Q., Liu, L., He, Y., and Chen, C. (2024). Gap analysis of Firmiana danxiaensis, a rare tree species endemic to southern China. Ecol. Indic. 158:111606. doi: 10.1016/j.ecolind.2024.111606
Li, Y., Wang, Y., Zhao, C., Du, X., He, P., and Meng, F. (2024). Predicting the spatial distribution of three Ephedra species under climate change using the MaxEnt model. Heliyon 10:e32696. doi: 10.1016/j.heliyon.2024.e32696
Liang, M. C., Gong, F. J., Jin, T., Sun, B., Yang, Y. J., Hu, D., et al. (2022). Characteristics of Picea neoveitchii tree growth in mountain areas of Central China: insights from isotopic compositions and satellite-derived indices. Isot. Environ. Health Stud. 58, 121–140. doi: 10.1080/10256016.2022.2047961
Lu, Y., Liu, H., Chen, W., Yao, J., Huang, Y., Zhang, Y., et al. (2021). Conservation planning of the genus Rhododendron in Northeast China based on current and future suitable habitat distributions. Biodivers. Conserv. 30, 673–697. doi: 10.1007/s10531-020-02110-6
Ma, Z., Sandel, B., and Svenning, J.-C. (2016). Phylogenetic assemblage structure of north American trees is more strongly shaped by glacial-interglacial climate variability in gymnosperms than in angiosperms. Ecol. Evol. 6, 3092–3106. doi: 10.1002/ece3.2100
Moreno, R., Zamora, R., Molina, J. R., Vasquez, A., and Herrera, M. A. (2011). Predictive modeling of microhabitats for endemic birds in south Chilean temperate forests using maximum entropy (Maxent). Eco. Inform. 6, 364–370. doi: 10.1016/j.ecoinf.2011.07.003
Mothes, C. C., Stroud, J. T., Clements, S. L., and Searcy, C. A. (2019). Evaluating ecological niche model accuracy in predicting biotic invasions using South Florida's exotic lizard community. J. Biogeogr. 46, 432–441. doi: 10.1111/jbi.13511
Ni, J., Yu, G., Harrison, S. P., and Prentice, I. C. (2010). Palaeovegetation in China during the late quaternary: biome reconstructions based on a global scheme of plant functional types. Palaeogeogr. Palaeoclimatol. Palaeoecol. 289, 44–61. doi: 10.1016/j.palaeo.2010.02.008
Noce, S., Cipriano, C., and Santini, M. (2023). Altitudinal shifting of major forest tree species in Italian mountains under climate change. Front. Forests Glob. Change 6:651. doi: 10.3389/ffgc.2023.1250651
Ouahzizi, B., Elbouny, H., Sellam, K., Bammou, M., Alem, C., and Bakali, A. H. (2024). Predicting potential distribution of Thymus atlanticus (ball) Roussine an endemic species in Morocco using MaxEnt modeling. Ecol. Front. 44, 966–971. doi: 10.1016/j.ecofro.2024.02.011
Paudel, G., Pandey, K., Lamsal, P., Bhattarai, A., Bhattarai, A., and Tripathi, S. (2024). Geospatial forest fire risk assessment and zoning by integrating MaxEnt in Gorkha District, Nepal. Heliyon 10:e31305. doi: 10.1016/j.heliyon.2024.e31305
Peterson, A. T., and Cohoon, K. P. (1999). Sensitivity of distributional prediction algorithms to geographic data completeness. Ecol. Model. 117, 159–164. doi: 10.1016/s0304-3800(99)00023-x
Phillips, S. J., Anderson, R. P., and Schapire, R. E. (2006). Maximum entropy modeling of species geographic distributions. Ecol. Model. 190, 231–259. doi: 10.1016/j.ecolmodel.2005.03.026
Qin, H., Yang, Y., Dong, S., He, Q., Jia, Y., Zhao, L., et al. (2017). Threatened species list of China’s higher plants. Biodivers. Sci. 25, 696–744. Available at: https://www.biodiversity-science.net
Rudley, D., DeSoto, L., Rodríguez-Echeverría, S., and Nabais, C. (2023). Climate effect on the growth and hydraulic traits of two shrubs from the top of a Mediterranean mountain. Sci. Total Environ. 902:165911. doi: 10.1016/j.scitotenv.2023.165911
Shi, X., Yu, J. X., Sun, Y. W., Xu, Z., and Li, H. (2024). A novel gymnosperm wood from the Lopingian (late Permian) in Zhangzi, Shanxi, North China and its Paleoecological and Paleogeographic implications. J. Earth Sci. 35, 167–176. doi: 10.1007/s12583-021-1510-3
Song, Z., Chen, W., Du, X., Zhang, H., Lin, L., and Xu, H. (2011). Chemical constituents of Picea neoveitchii. Phytochemistry 72, 490–494. doi: 10.1016/j.phytochem.2011.01.018
Srivastava, V., Roe, A. D., Keena, M. A., Hamelin, R. C., and Griess, V. C. (2021). Oh the places they’ll go: improving species distribution modelling for invasive forest pests in an uncertain world. Biol. Invasions 23, 297–349. doi: 10.1007/s10530-020-02372-9
Stockwell, D. (1999). The GARP modelling system: problems and solutions to automated spatial prediction. Int. J. Geogr. Inf. Sci. 13, 143–158. doi: 10.1080/136588199241391
Tiwary, R., Singh, P. P., Adhikari, D., Behera, M. D., and Barik, S. K. (2024). Vulnerability assessment of Taxus wallichiana in the Indian Himalayan region to future climate change using species niche models and global climate models under future climate scenarios. Biodivers. Conserv. 33, 3475–3494. doi: 10.1007/s10531-024-02859-0
Wang, G., Wang, C., Guo, Z., Dai, L., Wu, Y., Liu, H., et al. (2020). Integrating Maxent model and landscape ecology theory for studying spatiotemporal dynamics of habitat: suggestions for conservation of endangered red-crowned crane. Ecol. Indic. 116:106472. doi: 10.1016/j.ecolind.2020.106472
Wesemeyer, M., Kamp, J., Schmitz, T., Müller, D., and Lakes, T. (2023). Multi-objective spatial optimization to balance trade-offs between farmland bird diversity and potential agricultural net returns. Agric. Ecosyst. Environ. 345:108316. doi: 10.1016/j.agee.2022.108316
Wilson, B., Dolotbakov, A., Burgess, B. J., Clubbe, C., Lazkov, G., Shalpykov, K., et al. (2021). Central Asian wild tulip conservation requires a regional approach, especially in the face of climate change. Biodivers. Conserv. 30, 1705–1730. doi: 10.1007/s10531-021-02165-z
Xiao, S., Li, S., Huang, J., Wang, X., Wu, M., Karim, R., et al. (2024). Influence of climate factors on the global dynamic distribution of Tsuga (Pinaceae). Ecol. Indic. 158:111533. doi: 10.1016/j.ecolind.2023.111533
Yang, G., Liu, N., Zhang, X., Zhou, H., Hou, Y., Wu, P., et al. (2024). Prediction of the potential distribution of Chimonobambusa utilis (Poaceae, Bambusoideae) in China, based on the MaxEnt model. Biodiv. Data J. 12:e126620. doi: 10.3897/BDJ.12.e126620
Yee, T. W., and Mitchell, N. D. (1991). Generalized additive models in plant ecology. J. Veg. Sci. 2, 587–602. doi: 10.2307/3236170
Yu, C.-G. (2008). Quantitative PALAEOVEGETATION reconstruction at large scale based on pollen records. J. Plant Ecol. 135:104794.
Zhang, D., Katsuki, T., and Rushforth, K. (2013). Picea neoveitchii the IUCN red list of threatened species. Ecology and Evolution. 6, 3092–3106.
Zhang, K., Yao, L., Meng, J., and Tao, J. (2018). Maxent modeling for predicting the potential geographical distribution of two peony species under climate change. Sci. Total Environ. 634, 1326–1334. doi: 10.1016/j.scitotenv.2018.04.112
Keywords: climate change, MaxEnt, habitat shift, Picea neoveitchii Mast., potential geographic distribution, conservation strategies
Citation: Xue N, Li K, Chen K, Li P, Ji X, Ma Z and Ji W (2024) Predicting climate change impacts on distribution and conservation of critically endangered Picea neoveitchii using MaxEnt. Front. For. Glob. Change. 7:1472857. doi: 10.3389/ffgc.2024.1472857
Received: 30 July 2024; Accepted: 26 November 2024;
Published: 11 December 2024.
Edited by:
Daniel M. Ricciuto, Oak Ridge National Laboratory (DOE), United StatesReviewed by:
Jieshi Tang, Chinese Academy of Agricultural Sciences, ChinaCopyright © 2024 Xue, Li, Chen, Li, Ji, Ma and Ji. This is an open-access article distributed under the terms of the Creative Commons Attribution License (CC BY). The use, distribution or reproduction in other forums is permitted, provided the original author(s) and the copyright owner(s) are credited and that the original publication in this journal is cited, in accordance with accepted academic practice. No use, distribution or reproduction is permitted which does not comply with these terms.
*Correspondence: Zhilin Ma, NTQwNzM4ODgxQHFxLmNvbQ==; Wenli Ji, aml3ZW5saUBud2FmdS5lZHUuY24=
Disclaimer: All claims expressed in this article are solely those of the authors and do not necessarily represent those of their affiliated organizations, or those of the publisher, the editors and the reviewers. Any product that may be evaluated in this article or claim that may be made by its manufacturer is not guaranteed or endorsed by the publisher.
Research integrity at Frontiers
Learn more about the work of our research integrity team to safeguard the quality of each article we publish.