- Department of Forestry, Mississippi State University, Starkville, MS, United States
Introduction: Populus and its hybrids are attractive bioenergy crops and the southeastern United States has broad ability to supply bioenergy markets with woody biomass. Breeding and hybridization have led to superior eastern cottonwood (Populus deltoides W. Bartram ex Marshall) and hybrid poplars adapted to a wide variety of site types not suited for agricultural production. In order to maximize productivity and minimize inputs, genotypes need to efficiently use available site resources and tolerate environmental stresses. In addition, we need to determine plasticity of traits and their coordination across sites to select traits that will broadly characterize genotypes. Therefore, our study objectives were to determine (1) which leaf traits were correlated with growth, (2) if traits and genotypes exhibited significant plasticity across sites, and (3) how traits were coordinated within and across sites and Populus taxa.
Methods: We measured trees at two sites in northeastern Mississippi, United States: one upland and one alluvial terrace site. Genotypes included eastern cottonwoods as well as F1 crosses of eastern cottonwood and P. maximowiczii (Henry), P. nigra (L.) and P. trichocarpa (Torr. & Gray).
Results: We found that sites differed in which leaf traits were correlated with productivity; with water use efficiency specifically being positively correlated with growth at an alluvial terrace site, but negatively correlated with growth at an upland site. Tree height growth, leaf isotope composition (δ13C and δ15N), as well as leaf mass per area (LMA) exhibited the least plasticity across sites, while physiological gas exchange parameters and leaf nitrogen concentration exhibited the highest plasticity. Broadly across taxa, leaf carbon isotope ratios were correlated with intrinsic water use efficiency, and stomatal conductance was positively correlated with photosynthetic nitrogen use efficiency across sites, while leaf nitrogen isotope ratios exhibited contrasting relationships with leaf nitrogen concentration.
Discussion: Overall, these results allow us to refine selections of productive genotypes based on site conditions and site-specific relationships with physiological parameters to better match Populus taxa with sites and landowner objectives.
1 Introduction
Sustainable biomass production for bioenergy relies on low resource input crop production, and short-rotation poplar (Populus spp.) are a promising crop for bioenergy systems due to their fast growth, wide adaptability, short rotation lengths, and limited management requirements (Azar et al., 2013; An et al., 2021; Xu et al., 2021). According to the Department of Energy’s Billion Ton Update (U.S. Department of Energy, 2016), the southeastern United States (US) is forecasted to provide 68% of woody biomass for energy usage in the US by 2040 and Populus is a prime candidate to supply this feedstock because it has historically been grown for pulpwood production or low-cost timber (Ridge et al., 1986; Stanton et al., 2002; Spinelli and Hartsough, 2006). Therefore, much of the knowledge base and genetic improvement needed for fast growth, and harvesting technology is already available. Moreover, genetic breeding has enabled poplar to be more adaptive and resistant to biotic and abiotic factors (Han et al., 2013; Polle et al., 2013; Thakur et al., 2021) and grow on land not considered suitable for food crops (Robinson et al., 2000; Vose et al., 2000; Guerra et al., 2011). Nevertheless, poplar for traditional timber products have been grown on fertile, alluvial sites, therefore commercial breeding efforts seldomly focused on selecting for conservative water use strategies (Monclus et al., 2006). The next generation of poplar genotypes for bioenergy production will need to display fast growth in addition to efficient use of resources (water and nutrients) and the ability to tolerate marginal sites in order to provide bioenergy feedstocks with minimal ecosystem impacts.
Tree breeding and improvement in Populus have led to clonal material that exhibit no genetic variability within individuals of the same genotype, but potentially substantial genetic variability across genotypes. Physiologically, this can aid in the identification of suites of traits that vary together or broadscale tradeoffs among traits. Likewise, leaf traits can be categorized as “functional” if they predict plant performance metrics like growth and productivity (Violle et al., 2007). In the fast-slow plant economics spectrum, suites of traits are coordinated such that high rates of resource acquisition and processing are associated with high growth rates in resource-rich environments and traits need to be coordinated throughout the organism so that there are no bottlenecks or wasteful allocation of carbon and resources (Reich, 2014). Broadly, studies have found that total leaf area and water use were positively correlated with growth in Populus spp. (Pellis et al., 2004; Marron et al., 2005; Monclus et al., 2005; Monclus et al., 2009).
Additionally, planting of specific genotypes at differing sites allows for the identification of genotype by environment (G × E) interactions to determine which physiological characteristics and suites of traits are site dependent and which exhibit consistent patterns across sites. The presence of G × E interactions, particularly in productivity or resource-use parameters, can limit the selection of superior genotypes to site-specific locations (Marron et al., 2007; Miller, 2018). Alternatively, suites of plant traits can differ across sites, but potentially yield similar growth rankings among genotypes depending on the trait plasticity within individual genotypes. In total, plant functional traits can be used to select genotypes with high performance and resource use efficiency and identify trait plasticity across sites to identify the most robust plant traits to use for broadscale genotype selection.
In this study, we compared leaf physiological, anatomical, and biochemical traits as well as productivity from 168 genotypes of eastern cottonwood (Populus deltoides W. Bartram ex Marshall) and hybrid poplars planted at two sites (40 genotypes were planted at both sites and 128 were unique to only one site) differing in belowground water availability and located in the southeastern US. Genotypes included selected and improved eastern cottonwoods as well as hybrid poplars resulting from F1 crosses of Populus deltoides, Populus maximowiczii (Henry), Populus nigra (L.) and Populus trichocarpa (Torr. & Gray). Populus species crosses generally exhibit hybrid vigor particularly in first generation (F1) hybrids (Cronk, 2005). The objectives of the study were (1) to compare leaf traits and determine which traits were significantly correlated with growth at each site and potentially across sites, (2) identify suites of traits that were coordinated or exhibited tradeoffs and determine if these relationships were site and/or taxon specific and (3) determine which traits and genotypes had significant G × E interactions and exhibited the greatest plasticity. Overall, these data will increase understanding of leaf physiological traits and the tradeoffs among them in Populus genotypes that differ greatly in growth rate and site conditions. Assessing physiological performance and leaf traits in relation to productivity of numerous unique eastern cottonwood and hybrid poplars, many of which have not been studied before, will also improve our collective knowledge on the functioning and culture of these potential bioenergy feedstocks to aid in future breeding and selection of optimal genotypes for the southeastern US.
2 Materials and methods
2.1 Site descriptions and planting
The study was conducted at two field trials in Monroe (33°51’ N, 88°17’ W) and Pontotoc (34°08’ N, 88°59’ W) counties, in northeastern Mississippi, US. These sites are located about 70 km apart and are located on the Gulf Coastal Plain of the US. The climate of the region is humid subtropical characterized by mild winters and long, hot summers. These sites have a 30 year (1991–2020) mean annual rainfall of about 1,490 mm, mean maximum and minimum temperatures of 22.5°C and 10.3°C, respectively and a mean annual temperature of 16.4°C (NOAA, 2023). Table 1 contains specifics on site elevation, depth to the water table and soil composition. Specifically, the Monroe site is categorized as an “alluvial terrace” located approximately 500 m from the Buttahatchee River with fine sandy loam soils and a water table within 2 m of the surface (Natural Resources Conservation Service, 2023). The Pontotoc site is characterized as an “upland” site with an elevation about 65 m higher than the Monroe site and a water table over 2 m below the surface. Soils are categorized as silt loams (Table 1; Natural Resources Conservation Service, 2023). Volumetric soil moisture contents were measured at each field site with 30 cm long time domain reflectometry probes (Campbell Scientific Inc. Logan UT, USA) attached to a CR1000 datalogger (Campbell Scientific Inc.) that recorded data half-hourly. Relative soil moisture content in the top 30 cm was calculated as soil moisture divided by the highest soil moisture in the sample period (representing field capacity) and converted to a percentage. Figure 1 presents relative soil moisture contents for both the Monroe (alluvial terrace) and Pontotoc (upland) sites and shows that they did not differ significantly from one another during the growing season with the alluvial terrace site being near field capacity during the dormant season (Figure 1).
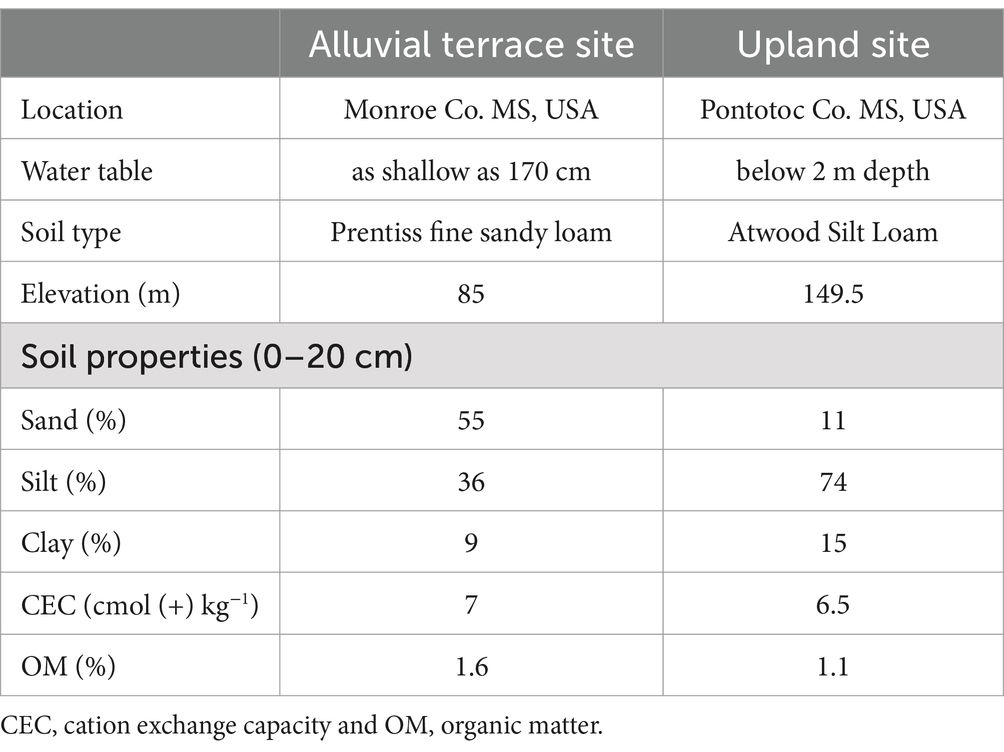
Table 1. Location, elevation and soil data for the study sites (Natural Resources Conservation Service, 2023).
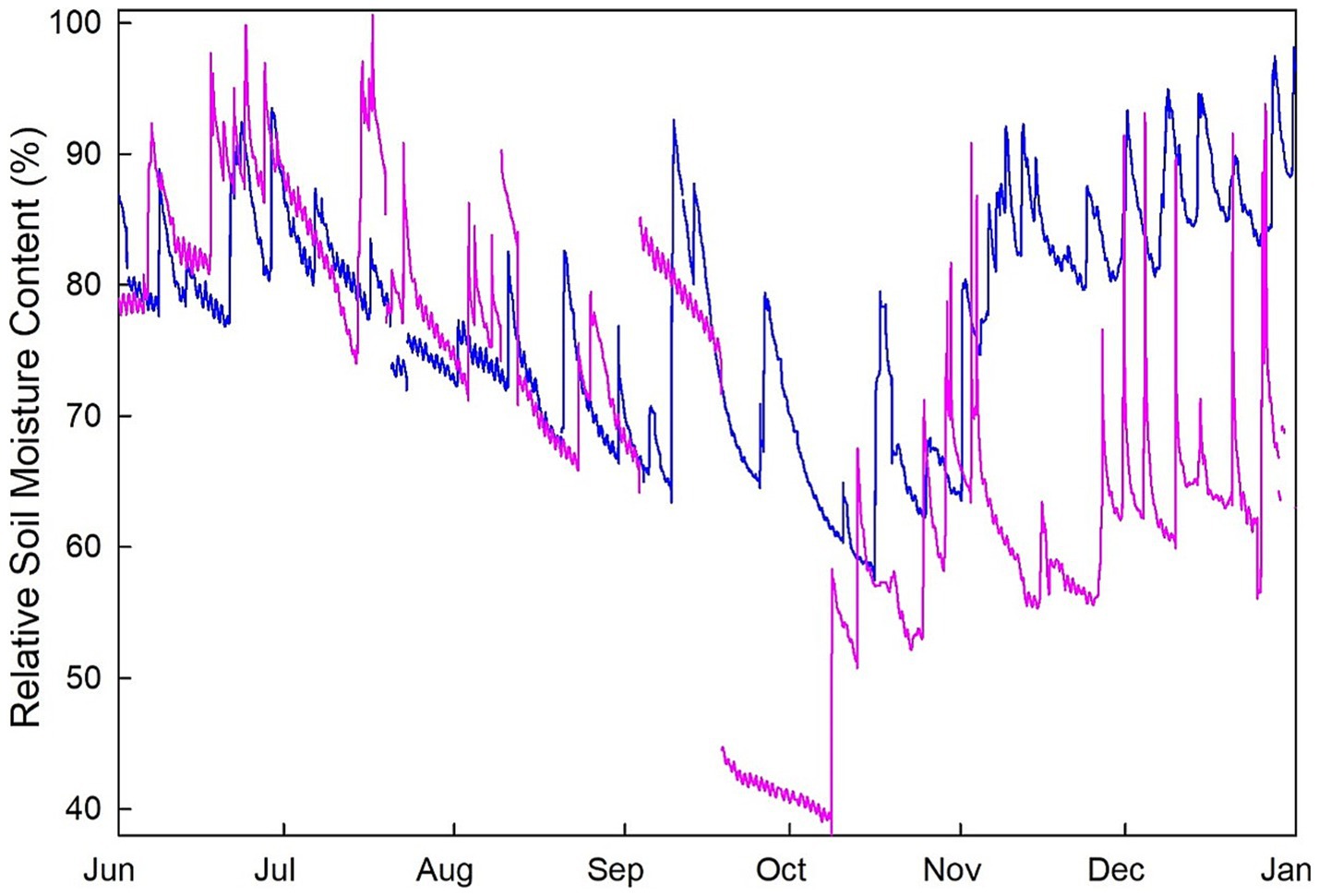
Figure 1. Relative soil moisture content for sensors located in the top 30 cm of soil for the alluvial terrace (blue) and upland (pink) field sites.
The alluvial terrace was established and planted in April 2018, and the previous use of this site was peanut production. The upland site was established and planted in April 2019 and was previously used for annual row crop production. Both sites were tilled and subsoiled along planting rows that were 2.7 m apart to a depth of 45.7 cm and treated with pre-emergent herbicide before planting. One hundred and 108 unique genotypes were planted at the alluvial terrace and upland sites, respectively, (Table 2). Six taxa including improved eastern cottonwoods (D × D) as well as hybrid poplars were used including P. deltoides × P. maximowiczii (D × M), P. deltoides × P. nigra (D × N), P. deltoides × P. trichocarpa (D × T), P. trichocarpa × P. deltoides (T × D), P. trichocarpa × P. maximowiczii (T × M) and P. deltoides × P. nigra × P. maximowiczii, (D × N × M) which was grouped with D × N genotypes since only one genotype was represented (Supplementary Table S1). Dormant and unrooted hybrid poplar cuttings (22.9 cm long) were obtained from GreenWood Resources (Portland, OR, USA) and kept in a walk-in freezer until planting. Dormant, unrooted, eastern cottonwood cuttings (40.6 cm long) were obtained from the Department of Forestry Cottonwood Cutting Orchard at Mississippi State University. Before planting, hybrid poplar cuttings were soaked in insecticide (Admire® Pro, Bayer Corp., Whippany, NJ, USA) for 3–4 days, and eastern cottonwood cuttings were also soaked in water and insecticide for 18 h, wrapped in plastic film, and placed in the freezer prior to planting. Cuttings were planted in subsoiled rows at a 1.8 m spacing and two ramets of the same genotype were planted adjacent to one another in each block. Taxa were contained together in subplots within blocks, when possible, given the number of genotypes per taxa at each site, to provide consistent competition around trees.
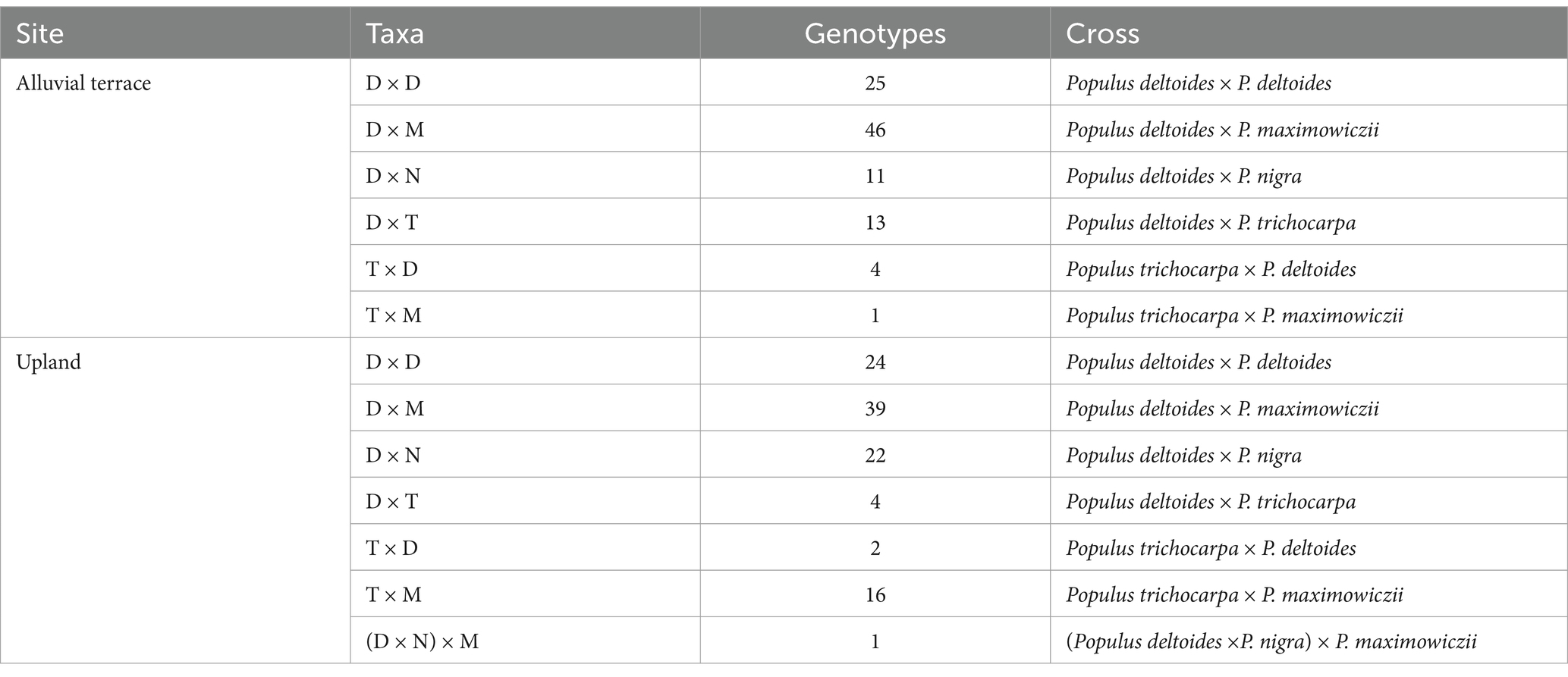
Table 2. Selected Populus taxa and number of genotypes planted at the Alluvial terrace (Monroe) and Upland (Pontotoc) field sites located in northeastern Mississippi, US.
For the first growing season, sites were maintained with minimal competition from herbaceous vegetation through hand weeding combined with tilling and chemical herbicide (Select, Valent USA LLC., Walnut Creek CA, USA) application. Additional fertilization and irrigation were not added to the site. If trees were being impacted by cottonwood leaf beetle (Chrysomela scripta Fabricius) larval feeding, sites were aerially sprayed with insecticide (Admire® Pro, Bayer Corp., Whippany, NJ, USA). Tree heights were measured at the end of the first growing season using a height pole.
2.2 Leaf physiology, morphology, and chemical composition
During approximately July and early August of the first growing season at each site, leaf gas exchange measurements were made on an attached, fully expanded and developed leaf near the top of the tree using a LiCOR 6400 portable photosynthesis system (calibrated in 2015; LI-COR Biosciences Inc., Lincoln, NE, USA). One individual per genotype (generally the larger of the two) in three blocks at each site was measured for leaf gas exchange during midmorning on sunny days after dew had evaporated, but before significant midday leaf stress. Generally, one replicate block was measured per day and blocks were chosen that represented a range of study site conditions within the larger study sites. Light levels in the leaf chamber were 1,500 μmol m−2 s−1, CO2 concentrations were 400 ppm and relative humidity was controlled to be between 40 and 60%. Measurements were made between about 8:30 and 12:30 local time, leaf temperatures ranged from about 27 to 37°C and leaf VPD ranged from about 0.8 to 3.8 kPa. Net assimilation rates (Anet; μmol m−2 s−1) and stomatal conductance (gs; mol m−2 s−1) were monitored and allowed to equilibrate for approximately 2 minutes before measurements were taken with the ratio of the two representing intrinsic water use efficiency (iWUE).
After gas exchange measurements, leaves were placed in labeled, zip top bags and into a cooler to be transported back to the laboratory. Leaves were kept in a refrigerator until their fresh areas could be measured using a LI-COR 3100 leaf area scanner. Leaves were then placed in envelopes and oven dried for approximately 1 week at 65°C, then their dry weights were obtained. The ratio of leaf fresh area and dry mass was used to calculate leaf dry mass per unit area (LMA; g m−2) which was used to calculate net assimilation on a per unit leaf mass basis (Amass). Dry leaves were then separated from the petiole and midrib and ground to pass through a 2 mm sieve. Ground leaf material was encapsulated in tin capsules, and sent to the University of California, Davis Stable Isotope Facility (Davis, CA, USA) for total sample carbon (C), and total sample nitrogen (N), and stable isotopes of carbon and nitrogen (δ13C, δ15N) using elemental combustion analysis (PDZ Europa ANCA-GSL elemental analyzer; Sercon Ltd., Cheshire, UK) and inductively coupled plasma optical emission spectrometry (PDZ Europa 20–20 isotope mass spectrometer; Sercon Ltd., Cheshire, UK) respectively. Leaf nitrogen content was calculated on a per unit area basis (Narea; g N m−2) by multiplying leaf N concentration by LMA. Photosynthetic nitrogen use efficiency (PNUE; μmol g−1 N s−1) was calculated by dividing leaf photosynthetic rates by nitrogen content on a per unit area basis.
Carbon isotope analysis provides an indirect measure of stomatal diffusion, but more importantly, of water use efficiency due to the inherent discrimination of the RuBisCO enzyme against 13C (Farquhar et al., 1989) and potentially provides a temporally and spatially integrated measure of intrinsic water use efficiency. Nitrogen isotope analysis can be more difficult to interpret compared with δ13C due to the multitude of soil nitrogen transformations and soil microbial species performing these chemical reactions and each exhibiting differing discrimination against 15N (Craine et al., 2015). However, in general, δ15N can distinguish between sources of N (soil or atmospheric; Craine et al. (2015)) as well as potentially indicating N availability and if a site is nearing N saturation (Craine et al., 2009).
2.3 Data and statistical analysis
Because gas exchange data were measured throughout the day and on multiple days, net assimilation and stomatal conductance data were scaled to a common temperature before further analysis (Willis et al., 2023). Using these corrected data, intrinsic water use efficiency was calculated as the ratio of net assimilation and stomatal conductance. Significant differences in physiological parameters across sites were accessed using ANOVA (aov) in R version 4.2.2 (R Core Team, 2022). Graphs were produced and p-values, correlation coefficients, and data quartiles were calculated using Sigmaplot version 13 (Systat Software Inc. San Jose, California, USA). Spearman rank correlations (ρ) and p-values were calculated in R using the “corr.test” function on genotypes that were present at both sites to evaluate ranking stability between sites (40 genotypes; Supplementary Table S1). Weak correlation between parameters (high p-value, low ρ) indicate a large difference in genotype ranks between sites and suggests the presence of a G × E interaction and traits with high plasticity. To determine total rank differences, genotypes were ranked from high to low values for each physiological parameter and absolute differences between sites for each genotype were calculated. These absolute differences were summed for each genotype to determine their total rank difference. Principal component analysis was performed for each site in R using the “prcomp” function with the datasets scaled and zero centered.
3 Results
3.1 Site differences
Although growth was higher across taxa at the alluvial terrace site, many leaf physiological and biochemical metrics were higher at the upland site including Anet, gs, LMA, leaf N concentrations and δ13C (signifying more moisture stress and/or more water use efficiency; Table 3). The alluvial terrace site also had higher δ15N across taxa and higher leaf-level iWUE in D × D and D × M individuals (Table 3).
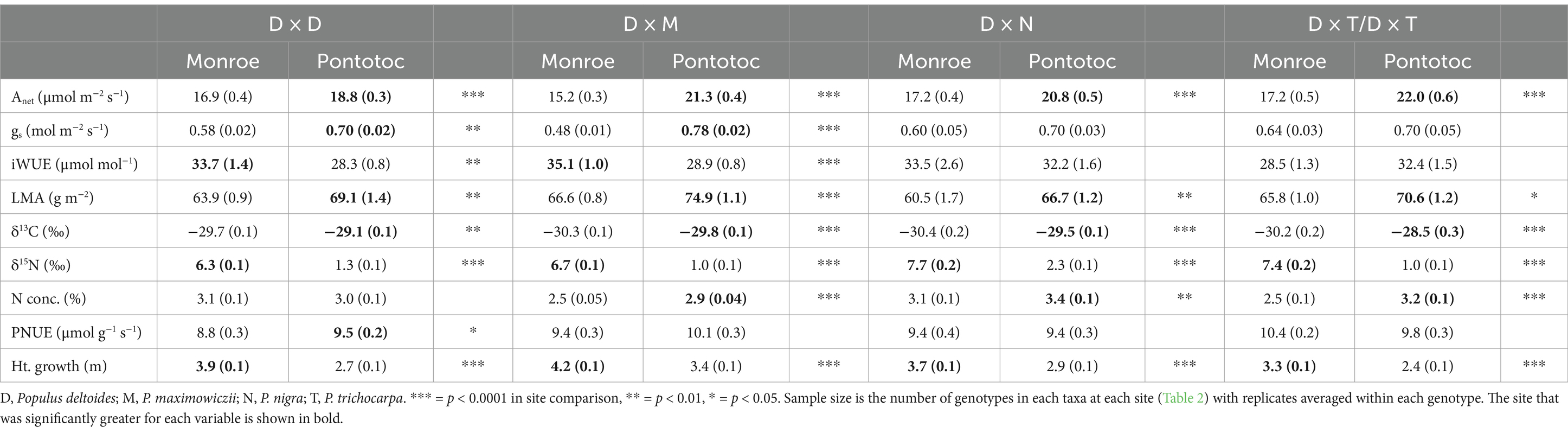
Table 3. Mean (standard error in parentheses) for leaf physiological, biochemical and anatomical parameters and tree height growth for Populus taxa planted at the Monroe (alluvial terrace) and Pontotoc (upland) sites.
3.2 Productivity predictors
Physiological and biochemical parameters to predict productivity in terms of yearly height growth were site specific with no common parameters across sites (Figure 2). At the alluvial terrace site, height growth was positively correlated with leaf-level iWUE, and negatively correlated with Anet, gs and PNUE (less so for D × M than other taxa; Figure 3). At the upland site, height growth was negatively correlated with leaf level iWUE and δ13C and positively correlated with gs (Figures 2, 3). When separating out D × D and hybrid taxa that were planted at both field sites, no parameters were significantly correlated with height growth for D × D genotypes at the upland site while height growth was negatively correlated with Anet and positively correlated with δ15N at the alluvial terrace site (Figure 4). For hybrid poplar taxa, height growth at the alluvial terrace site was negatively correlated with water use efficiency in terms of δ13C and negatively correlated with N concentrations, while being positively correlated with δ15N and negatively correlated with Anet at the upland site (Figure 4).
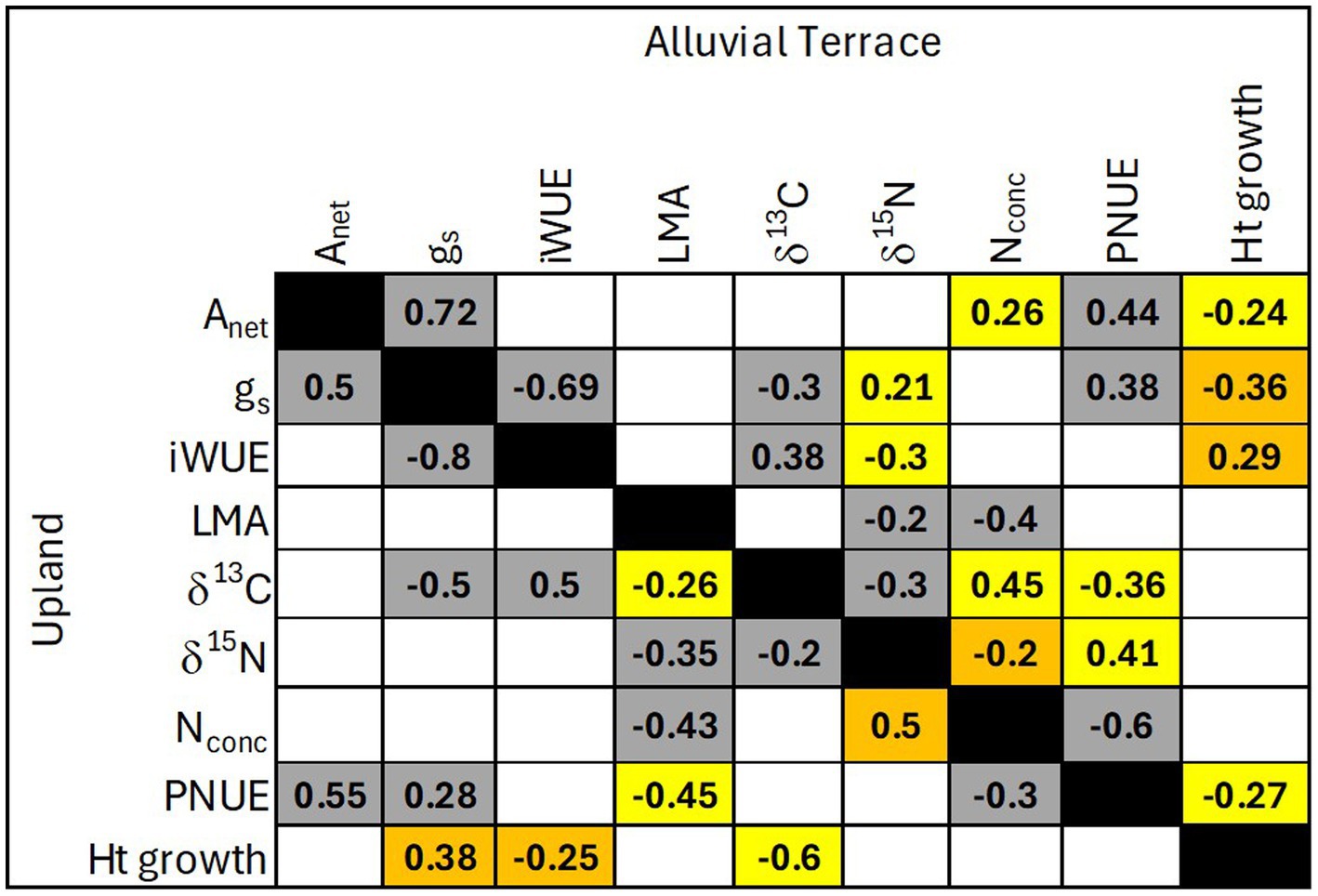
Figure 2. Correlations among leaf parameters and height growth for the alluvial terrace (top diagonal) and upland (bottom diagonal) field sites. Empty squares exhibited a non-significant correlation (p > 0.05). Gray squares represent statistically significant (p < 0.05) correlations that were similar in direction between sites, yellow squares represent correlations that were only significant at one site and orange squares represent statistically significant but opposing correlations between sites.
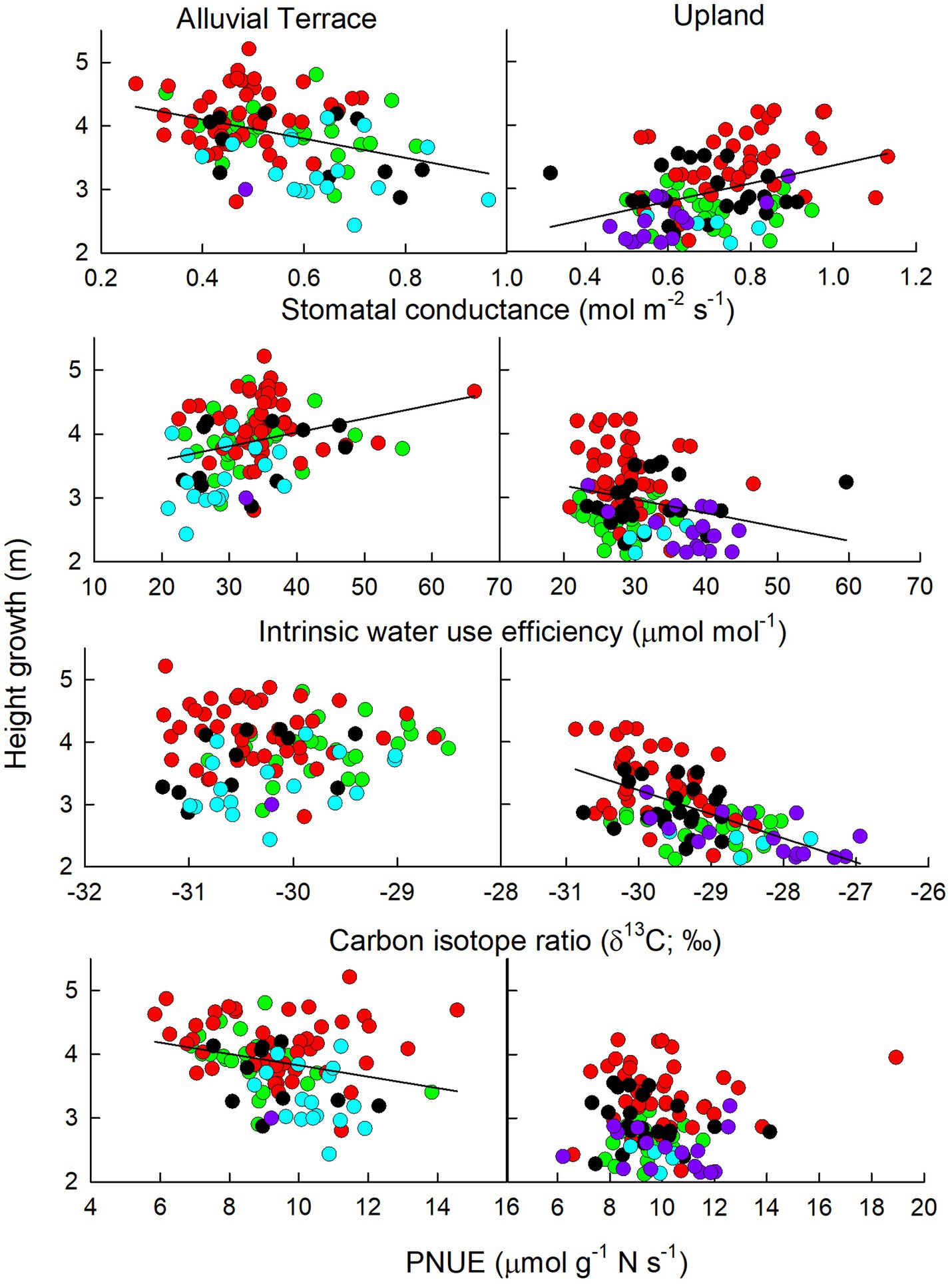
Figure 3. Physiological parameters correlated tree height growth (m) at the alluvial terrace (left) and upland (right) sites for P. deltoides × P. deltoides (D × D; green), P. deltoides × P. maximowiczii (D × M; red), P. deltoides × P. nigra (D × N; black), P. deltoides × P. trichocarpa (D × T) and P. trichocarpa × P. deltoides (T × D; light blue), and P. trichocarpa × P. maximowiczii (T × M; purple).
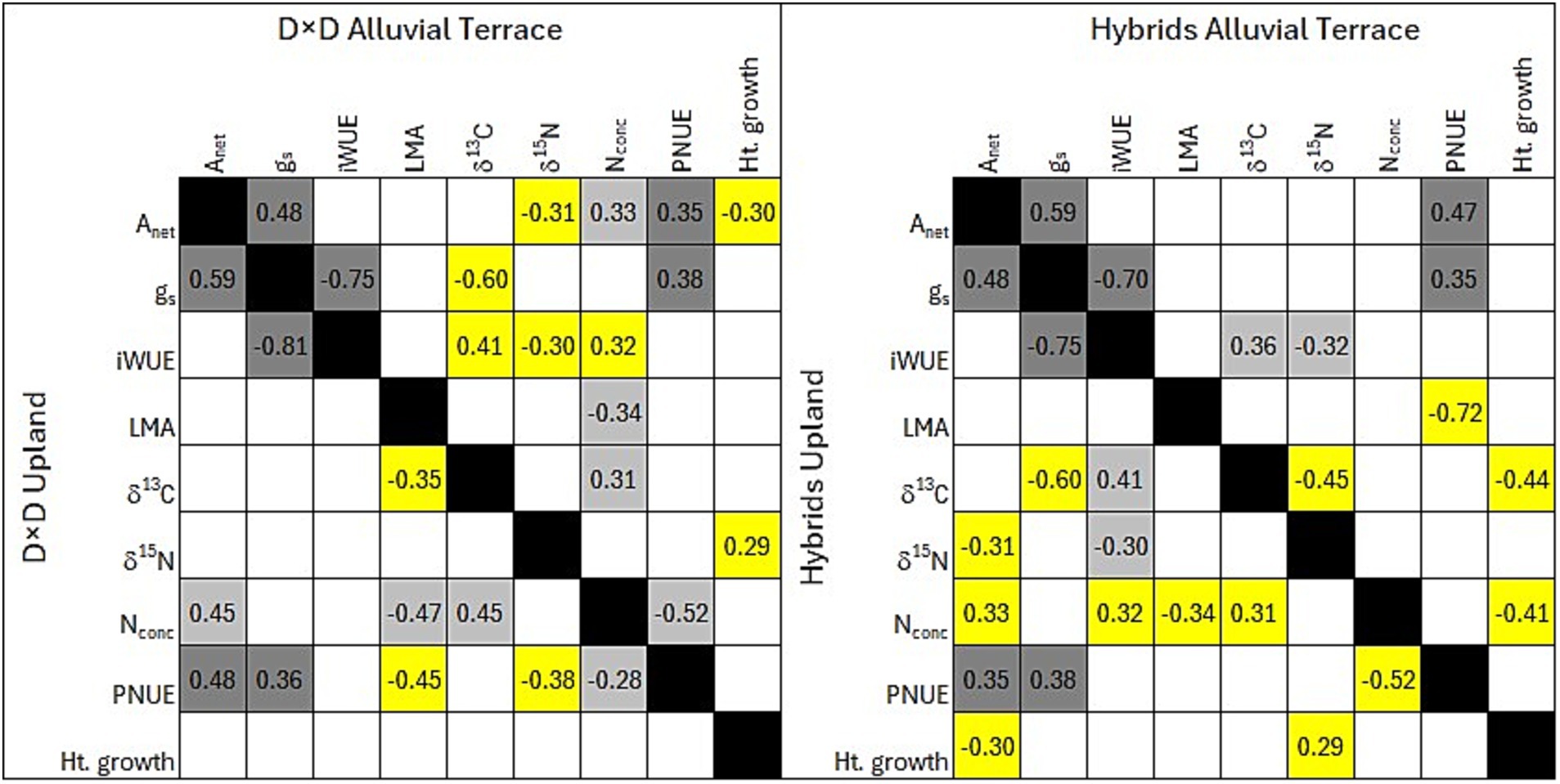
Figure 4. Correlations among leaf parameters and height growth for Populus deltoides × P. deltoides (D × D; left) and hybrid poplars (D × M, D × N, D × T, T × D) at the alluvial terrace (top diagonals) and upland (bottom diagonals) field sites. Empty squares exhibited a non-significant correlation (p > 0.05). Dark gray squares represent statistically significant correlations (p < 0.05) that were similar in direction across taxa groupings and sites. Light gray squares represent statistically significant correlations that were similar within taxa grouping between sites, yellow squares represent correlations that were only significant at one site within taxa groupings.
3.3 Coordination and tradeoffs among physiological parameters
Several physiological parameters exhibited consistent relationships across all planted Populus taxa at both the alluvial terrace and upland sites including positive relationships between Anet and gs as well as PNUE, negative relationships between gs and iWUE and δ13C (Figure 2), and negative correlations between leaf N concentration and LMA (Figure 5) and well as PNUE (Figure 2). Particularly, δ13C and iWUE were positively correlated at both sites (Figure 5). Stomatal conductance was also positively correlated with PNUE across taxa and sites suggesting a consistent relationship between water use and nitrogen use efficiency (Figure 5). LMA was negatively correlated with δ15N at both sites across all taxa (Figure 5) and δ15N and δ13C were also negatively correlated with one another (Figure 2). However, δ15N exhibited opposing relationships with leaf N concentration being positively correlated at the upland site, but negatively correlated at the alluvial site (Figure 5). For the 40 common genotypes (D × D and hybrids) planted at both sites, physiological relationships including positive correlations between Anet and gs as well as PNUE and negative relationships between gs and iWUE were evident (Figure 4). PNUE and gs were also positively correlated across sites and Populus types suggesting a broad relationship between water use and PNUE. For D × D genotypes, leaf nitrogen parameters exhibited more similar relationships across sites with leaf N concentration being positively correlated with Anet, and δ13C and negatively correlated with LMA and PNUE (Figure 4). For Populus hybrids, fewer consistent relationships were evident across sites except for iWUE which was positively correlated with δ13C and negatively correlated with δ15N at both sites (Figure 4). Nevertheless, P. deltoides tended to have more significant correlations among leaf traits particularly with regard to iWUE at the alluvial terrace site, while hybrid poplars exhibited more trait correlation particularly for leaf nitrogen traits at the upland site (Figure 4).
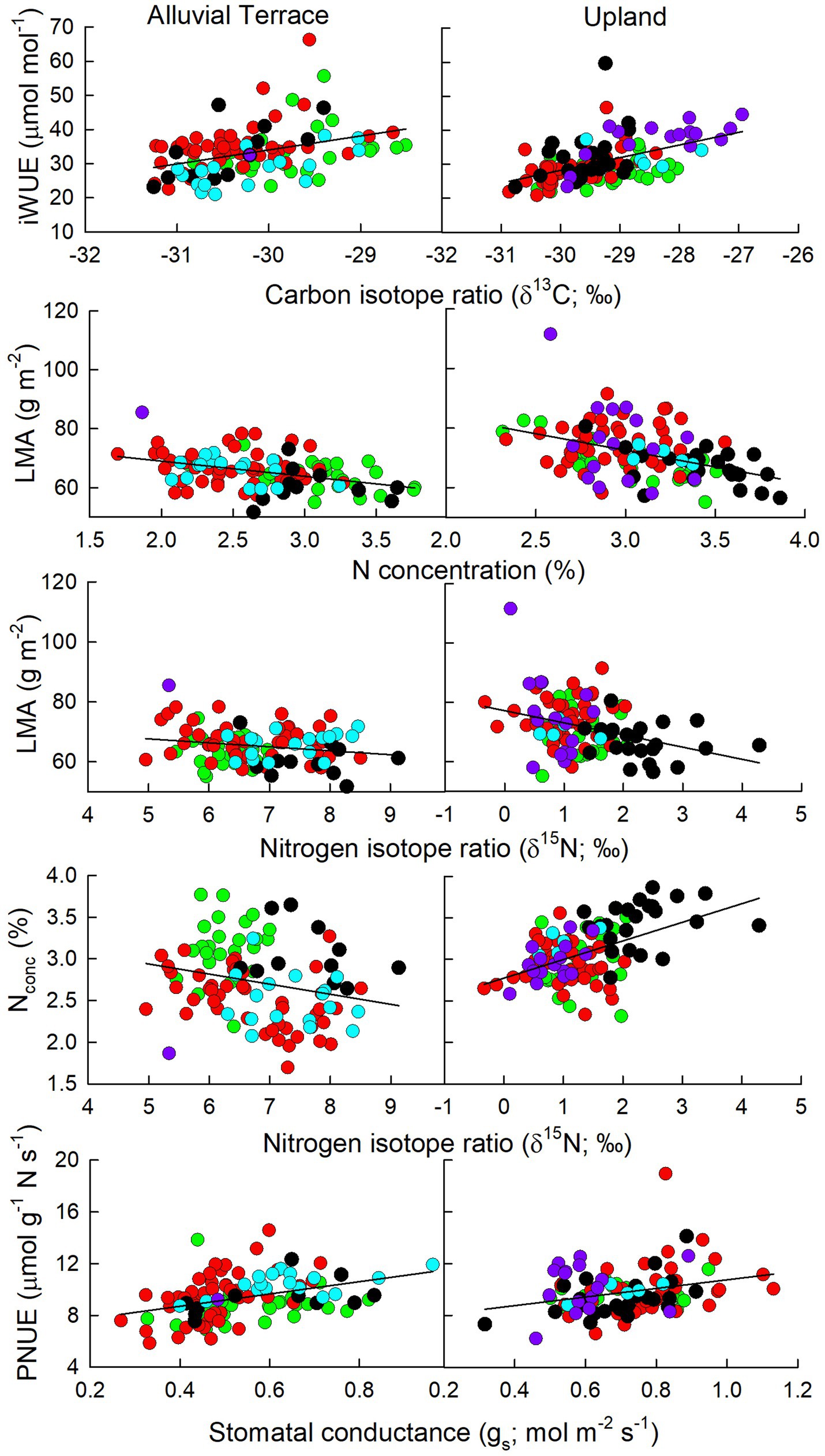
Figure 5. Relationships among leaf physiological parameters at the alluvial terrace (left) and upland (right) sites for genotypes in the P. deltoides × P. deltoides (D × D; green), P. deltoides × P. maximowiczii (D × M; red), P. deltoides × P. nigra (D × N; black), P. deltoides × P. trichocarpa (D × T) and P. trichocarpa × P. deltoides (T × D; light blue), and P. trichocarpa × P. maximowiczii (T × M; purple) taxa.
For principal component analysis, the first two PCs explained about 59 and 53% of the variation in data for the alluvial terrace and upland field sites, respectively, (Figure 6). At both sites, iWUE and δ13C were positively correlated and δ15N and PNUE were positively correlated with one another. At the alluvial terrace site, assimilation rates were positively correlated with gs and negatively correlated with LMA, while at the upland site, assimilation rates were positively correlated with leaf Nconc and PNUE and negatively correlated with LMA and leaf CN ratios (Figure 6). At the alluvial terrace site, yearly height growth was positively correlated with iWUE and negatively correlated with PNUE and δ15N. At the upland site, height growth was positively correlated with gs and negatively correlated with δ13C and iWUE (Figure 6).
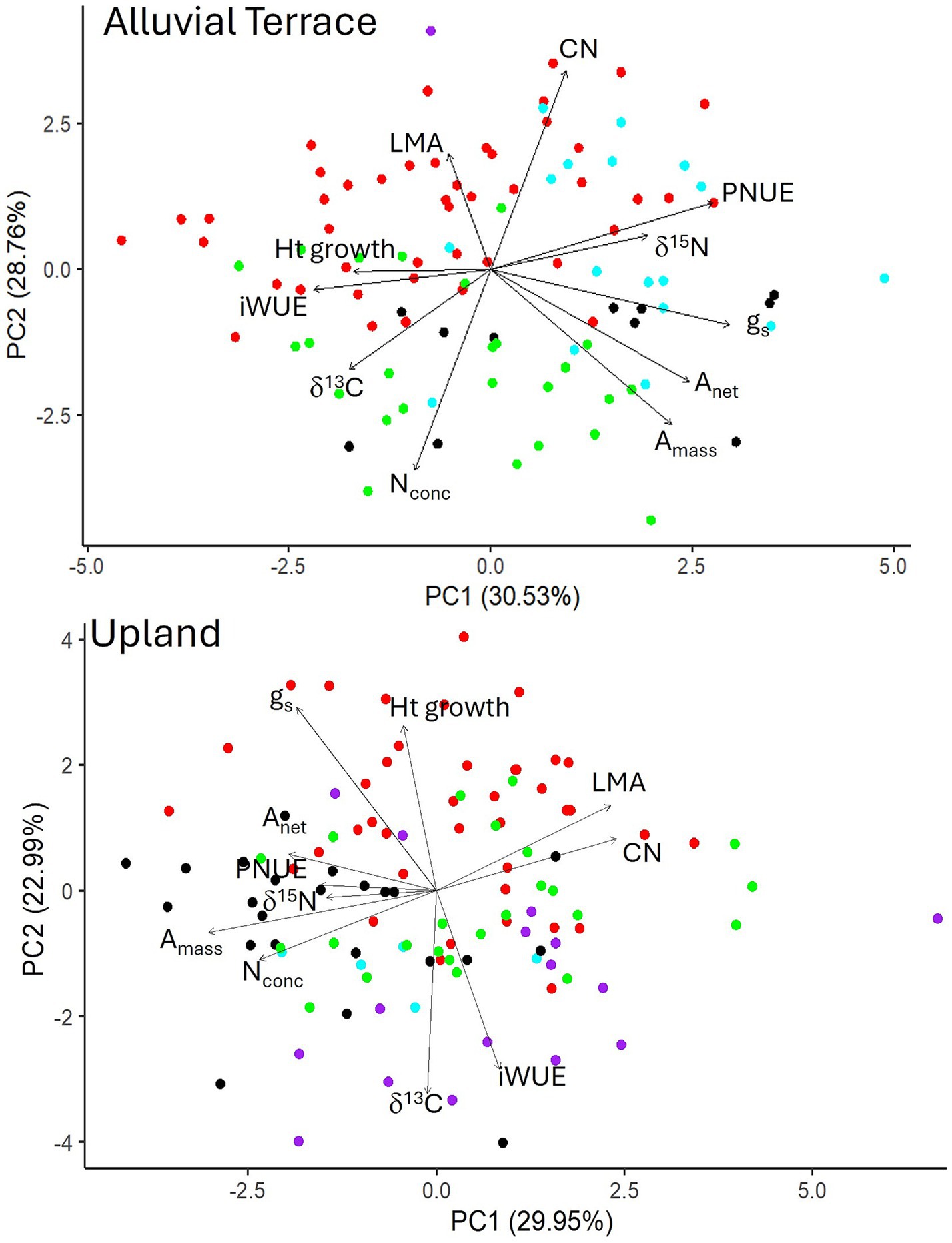
Figure 6. Principal component analysis (PCA) for the alluvial terrace (top) and upland (bottom) sites showing the loading of physiological parameters (arrows) in relation to the PC scores for all genotypes in the P. deltoides × P. deltoides (D × D; green), P. deltoides × P. maximowiczii (D × M; red), P. deltoides × P. nigra (D × N; black), P. deltoides × P. trichocarpa and P. trichocarpa × P. deltoides (D × T and T × D; blue), and P. trichocarpa × P. maximowiczii (T × M; purple) taxa.
3.4 G × E interactions and trait plasticity
For the 40 genotypes planted at both field sites, rankings among genotypes changed the most, and G × E interactions were the most evident, in terms of physiological parameters, in particular Anet, nitrogen parameters, and to a lesser extent, water use parameters (Table 4). For Anet, D × M genotypes were the most likely to have high rates at the upland site but low rates at the alluvial terrace site, while the opposite was true for D × D which tended to have high rates at the alluvial terrace site as opposed to the upland site (Figure 7). For leaf N concentrations, D × D genotypes were more likely to be in the extremes with high leaf N concentration at one site and low leaf N for the same genotype at the other field site (Figure 7). Most other physiological traits exhibited a mix of taxa with opposing ranking at one site vs. another (Figure 7). Leaf isotope ratios (δ13C and δ15N) and leaf anatomical parameters (LMA) tended to have the lowest plasticity and low G × E interaction as they were correlated across sites (Table 4 and Figure 7). Finally, height growth was consistent across sites suggesting minimal site effects on height rankings among genotypes (Figure 7).
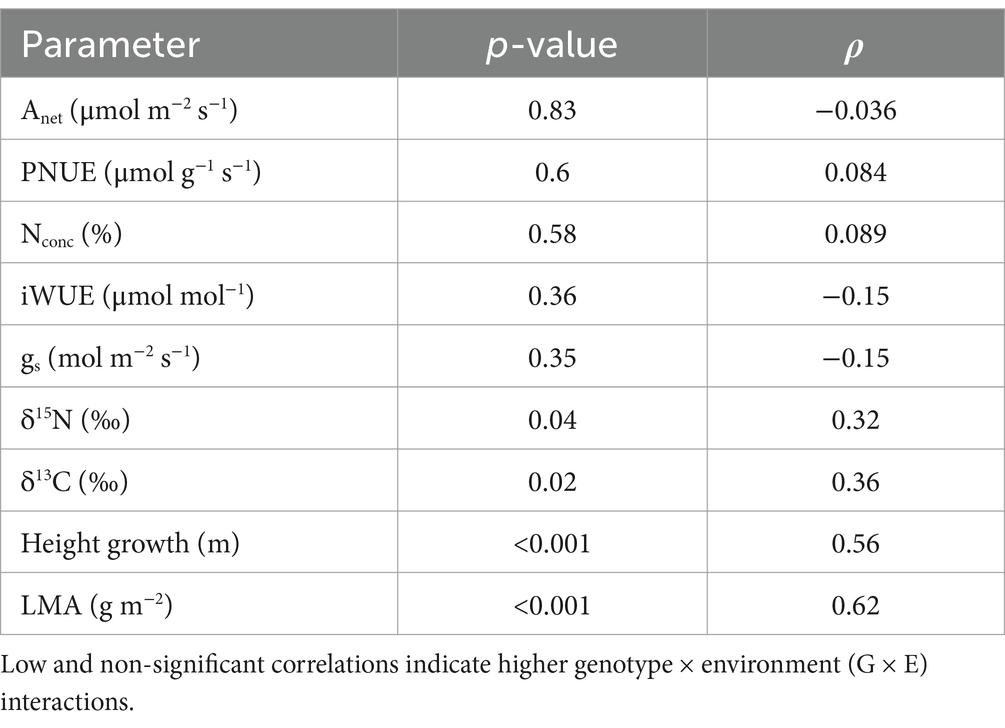
Table 4. Spearman rank correlation coefficient (ρ) and p-values comparing correlations among 40 genotypes planted at the Monroe (alluvial terrace) and Pontotoc (upland) sites for leaf physiological, biochemical, and anatomical parameters and well as tree height growth.
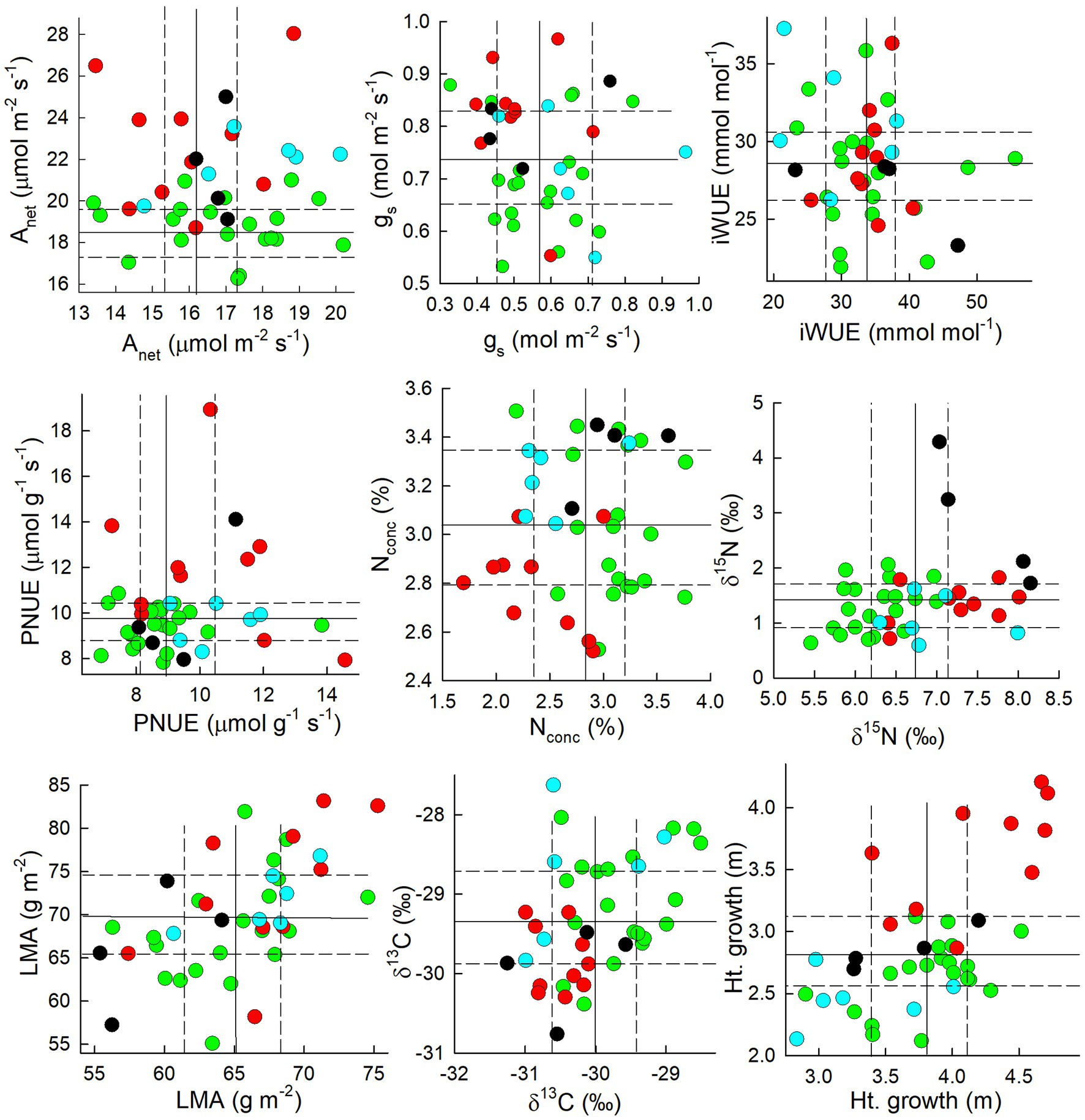
Figure 7. Physiological parameters measured in the same genotypes from P. deltoides × P. deltoides (D × D; green), P. deltoides × P. maximowiczii (D × M; red), P. deltoides × P. nigra (D × N; black), P. deltoides × P. trichocarpa (D × T) and P. trichocarpa × P. deltoides (T × D; light blue) planted at both the alluvial terrace (x axes) and upland (y axes) field sites (Table 4). Solid lines represent the median and dashed lines represent the upper and lower quartiles for each parameter.
Among the genotypes that were common at both field sites, certain genotypes were more likely to exhibit trait plasticity across sites when all measured parameters were taken into account while others exhibited fairly low plasticity (Figure 8). Taxa did not factor into trait plasticity with each taxa containing genotypes that exhibited high and low plasticity across sites. For D × D genotypes, ‘83–2’, ‘S7C2’, ‘S7C4’ and ‘27–5’ exhibited high trait plasticity while genotypes ‘122’, ‘95A-6’, ‘6–1’ and ‘6–5’ exhibited relatively low plasticity (Figure 8). For D × T and T × D genotypes ‘5077’ and ‘9552’ (both T × D) exhibited high plasticity while ‘10029’ and ‘7903’ (both D × T) exhibited relatively low plasticity (Figure 8). Finally, for D × M genotypes ‘9252’, ‘13724’, ‘8019’ and ‘13738’ exhibited relatively high plasticity while ‘9225’ exhibited low plasticity between sites (Figure 8). Overall, trait plasticity was not correlated with height growth (p = 0.52; data not shown) with both low and high plasticity genotypes of each taxa capable of high growth.
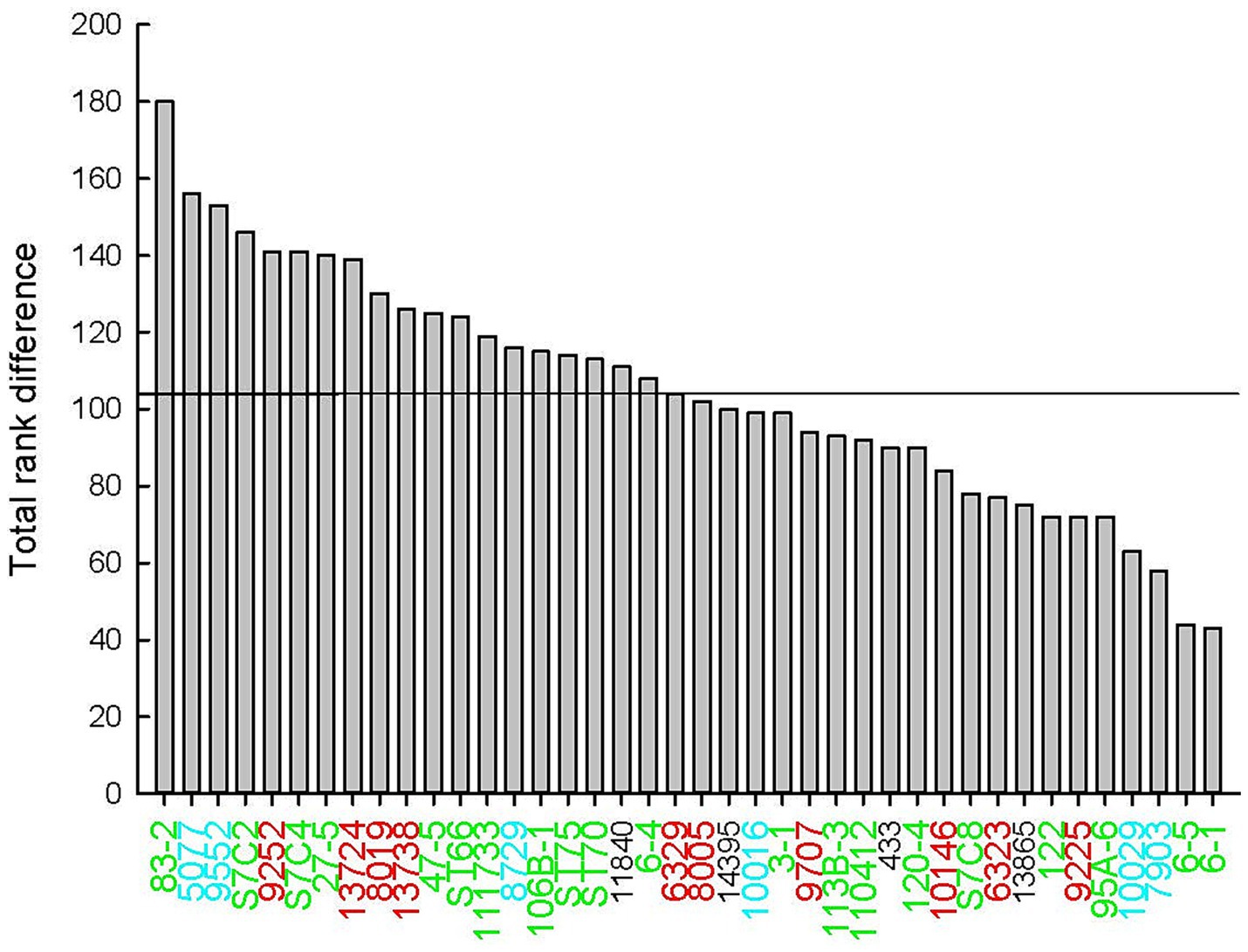
Figure 8. Total of rank differences between the alluvial terrace and upland field sites summed across each measured physiological parameter for genotypes from taxa including P. deltoides × P. deltoides (D × D; green), P. deltoides × P. maximowiczii (D × M; red), P. deltoides × P. nigra (D × N; black), P. deltoides × P. trichocarpa (D × T) and P. trichocarpa × P. deltoides (T × D; light blue) planted at both sites. The horizontal line represents the average rank difference summed across all parameters.
4 Discussion
4.1 Productivity predictors
Year one height growth was significantly greater at the alluvial terrace site for all taxa groups compared with the upland site and site and taxonomic differences meant that no single measured physiological parameter predicted growth across sites and taxa. Across all taxa at the alluvial terrace site, a tradeoff was evident in terms of either using water or nitrogen efficiently with a positive correlation between height growth and iWUE but a negative correlation between height growth and stomatal conductance as well as PNUE. Several other studies found a tradeoff between water and nitrogen use efficiency (Sage and Pearcy, 1987; DeLucia and Schlesinger, 1991; Hikosaka et al., 1998; Chen et al., 2005; Broeckx et al., 2014), since restricting CO2 intake and exhibiting a high water use efficiency should make photosynthetic enzymes, and the nitrogen used to make them, less efficiently utilized. Other studies have also reported a positive relationship between growth and water use efficiency in Populus (Zhang et al., 2004; Monclus et al., 2009; King et al., 2013; Renninger et al., 2021b; Renninger et al., 2023). At the upland site, the opposite was true with height growth being negatively correlated with both iWUE and δ13C and positively correlated with stomatal conductance which was seen in other studies as well (Monclus et al., 2009; Renninger et al., 2021a) and fits with the fast/slow hypothesis (Reich, 2014) of high resource acquisition leading to higher growth rates. These data as well as the results from other research suggest that whether growth is positively or negatively related with water use efficiency is related to site characteristics and potentially water availability. However, it is surprising that at the alluvial terrace site with a seemingly more abundant water supply, height growth would be positively associated with water use efficiency, while height growth was negatively correlated with water use efficiency parameters at the drier upland site. This could suggest that access and acquisition to water leads to greater height growth at the upland site, while at the alluvial terrace site, efficient use of water at the leaf level may allow for more overall leaf area and therefore greater growth. This is corroborated by the results of the PCA analysis wherein height growth was positively correlated with iWUE at the alluvial terrace site but was inversely correlated with iWUE at the upland site. Other studies found that leaf area was correlated with productivity in Populus (Pellis et al., 2004; Marron et al., 2005; Monclus et al., 2005; Renninger et al., 2021b). Likewise, the large growth rates seen at the alluvial terrace site may have led to water resource limitations, or at least limitations in resource acquisition compared with the rate of growth occurring.
4.2 Coordination and tradeoffs among physiological parameters
Across sites and taxa, broadscale relationships and coordination among physiological parameters were evident including well established relationships between Anet and gs as well as PNUE, negative relationships between gs and iWUE and δ13C and negative correlations between leaf N concentration and LMA and well as PNUE. The negative correlation between LMA and leaf N concentration is in line with the worldwide leaf economic spectrum in which “quick-return” leaves exhibit high leaf N and low LMA with the opposite being true of “slow-return” leaves (Wright et al., 2004). Other broadly significant relationships were the positive correlation between leaf gas exchange-estimated iWUE and δ13C, an estimate of water use efficiency based on carbon isotope ratios which has been seen in other studies as well (Soolanayakanahally et al., 2009; Bogeat-Triboulot et al., 2019). This suggests that the instantaneous measures from single leaves on the canopy provided a broad representation of the water use efficiency of the individual as is captured by leaf isotope data.
Another broad-scale relationship is the positive correlation between stomatal conductance and PNUE likely explained by the aforementioned mechanism in which higher stomatal conductance allows leaf nitrogen in photosynthetic enzymes to be used more efficiently (Field et al., 1983). Additionally, a positive correlation between leaf N concentration and δ13C seen across taxa at the alluvial terrace site, in D × D at both sites and in hybrids at the upland site suggests the same scenario in which greater leaf nitrogen and photosynthetic enzymes allow water to be used more efficiently (Brueck, 2008). Finally, a negative correlation between LMA and δ15N was observed at both sites. This relationship is more difficult to interpret as a wide variety of soil characteristics and processes affect δ15N of nutrients taken up by plants (Craine et al., 2015). However, N saturated sites have been shown to have higher δ15N values (Craine et al., 2009) and more microsite N availability within sites may lead to “quick return” leaves with lower LMA, particularly given the negative relationship between leaf N and LMA. This explanation is corroborated by the positive relationship between leaf N concentration and δ15N at the upland site, however the opposite relationship is seen at the alluvial terrace site. Nitrogen isotope ratios at the alluvial terrace site may be further complicated by the loss of the lighter isotopes of N due to denitrification (Blackmer and Bremner, 1977) which potentially explains why that site has more positive δ15N values than the upland site. Therefore, issues of greater denitrification and potential nitrate leaching (Ostrom et al., 1998) due to the greater soil saturation at the alluvial terrace site may be affecting the isotopic ratio of nitrate and ammonium and affecting its relationship with leaf N concentration. Overall, these differing relationships help determine what may be site-specific trait interactions and which are more broadly held across environmental conditions.
4.3 G × E interactions and trait plasticity
For genotype × environment interactions, leaf gas exchange, particularly Anet and PNUE, as well as leaf N parameters exhibited the most plasticity between sites, while tree height growth and leaf anatomical and isotopic parameters were most similar in terms of displaying similar rankings among genotypes between sites. This means that site effects should not impact studies that aim to identify the most productive genotypes in field trials at least for similar geographic conditions. Similar findings were reported by Marron and Ceulemans (2006) and Nelson et al. (2018), but differ from findings from Renninger et al. (2021a) who found significant G × E interactions in productivity parameters across study sites, although sites were in different physiographic regions and exhibited different disease pressures from Septoria stem canker (Sphaerulina musiva). Other studies also report G × E interactions in terms of productivity (Marron et al., 2006; Marron et al., 2007; Pliura et al., 2007; Rönnberg-Wästljung et al., 2022) with some genotypes exhibiting more stability in productivity across sites than others (Alvarez et al., 2020). Plasticity in physiological functioning has been reported by other researchers who found changes in gene expression as a result of water stress conditions (Vigar et al., 2016) and plasticity in sylleptic branching (Wu and Stettler, 1998; Cooke et al., 2005; Marron et al., 2006), as well as in xylem hydraulic traits (Plavcová and Hacke, 2012; Garavillon-Tournayre et al., 2018; Lemaire et al., 2021) and leaf physiological traits (Garavillon-Tournayre et al., 2018). For leaf metrics, isotope (δ13C and δ15N) and anatomical (LMA) parameters had the lowest plasticity with genotype rankings remaining similar across sites. Renninger et al. (2021a) also found that LMA, δ13C and δ15N exhibited similar rankings among genotypes across different field sites and Himes et al. (2021) and Voltas et al. (2006) found that δ13C exhibited low plasticity across irrigation treatments. However, Guet et al. (2015) found that LMA and δ13C exhibited significant phenotypic plasticity across sites in P. nigra genotypes. Nevertheless, these leaf traits may represent robust parameters for broad phenotypic comparison since genotypes maintained their ranking for these parameters regardless of site.
5 Conclusion
In total, these data can aid in future breeding of superior Populus genotypes for growth in the southeastern US as well as selection of optimal genotypes based on site conditions. In terms of physiological parameters that predict growth, parameters were site specific due to the significant impacts of resource availability and the need for physiological adjustments to perform optimally at each site. Likewise, physiological and leaf N parameters exhibited the highest trait plasticity with genotypes changing rank in these traits while height growth remained consistent in genotype rankings. Therefore, the best Populus genotypes in terms of growth were able to adjust their physiology and N uptake to match site conditions and optimally grow under divergent site conditions. LMA and isotope ratios (δ13C and δ15N) exhibited the least plasticity across sites suggesting that, like overall height growth, they represent cumulative functioning with genotype rankings remaining consistent even as small-scale alterations in leaf functioning are made. Due to their low site plasticity, LMA and leaf isotopes may be broadly useful in categorizing genotypes in terms of their intrinsic physiology in terms of water use efficiency and nutrient uptake in comparison with one another. We also found that coordination of some leaf traits was maintained across sites and taxa, specifically relationships between leaf N (and δ15N) and LMA, gas exchange and carbon isotope metrics for water use efficiency, stomatal conductance and PNUE. Other relationships among leaf traits differed by site suggesting environmental controls on these relationships. Overall, these data can broaden our understanding of coordination among leaf traits and their relationship with tree growth as well as select optimal genotypes of Populus for bioenergy feedstock production across environmental site conditions.
Data availability statement
The datasets presented in this study can be found in online repositories. The names of the repository/repositories and accession number(s) can be found at: https://scholarsjunction.msstate.edu/fwrc-publications/13/.
Author contributions
HR: Conceptualization, Formal analysis, Funding acquisition, Investigation, Methodology, Supervision, Writing – original draft. JP: Investigation, Methodology, Writing – review & editing. JW: Investigation, Methodology, Writing – review & editing.
Funding
The author(s) declare that financial support was received for the research, authorship, and/or publication of this article. This research was supported by grants from the National Institute of Food and Agriculture, U.S. Department of Agriculture (2018–68005-27636 and McIntire-Stennis project MISZ-067050), and the United States Department of Energy (DE-EE0009280).
Acknowledgments
The authors thank R. Rousseau, C. James, M. Murphy, T. Bowling, W. Booth, and T. Y. Kyaw for site logistics and fieldwork assistance. This publication is a contribution of the Forest and Wildlife Research Center, Mississippi State University.
Conflict of interest
The authors declare that the research was conducted in the absence of any commercial or financial relationships that could be construed as a potential conflict of interest.
The author(s) declared that they were an editorial board member of Frontiers, at the time of submission. This had no impact on the peer review process and the final decision.
Publisher’s note
All claims expressed in this article are solely those of the authors and do not necessarily represent those of their affiliated organizations, or those of the publisher, the editors and the reviewers. Any product that may be evaluated in this article, or claim that may be made by its manufacturer, is not guaranteed or endorsed by the publisher.
Supplementary material
The Supplementary material for this article can be found online at: https://www.frontiersin.org/articles/10.3389/ffgc.2024.1467381/full#supplementary-material
References
Alvarez, J., Cortizo, S., and Gyenge, J. (2020). Yield stability and phenotypic plasticity of Populus spp. clones growing in environmental gradients: I-yield stability under field conditions. For. Ecol. Manag. 463:117995. doi: 10.1016/j.foreco.2020.117995
An, Y., Liu, Y., Liu, Y., Lu, M., Kang, X., Mansfield, S. D., et al. (2021). Opportunities and barriers for biofuel and bioenergy production from poplar. GCB Bioenergy 13, 905–913. doi: 10.1111/gcbb.12829
Azar, C., Johansson, D. J., and Mattsson, N. (2013). Meeting global temperature targets—the role of bioenergy with carbon capture and storage. Environ. Res. Lett. 8:034004. doi: 10.1088/1748-9326/8/3/034004
Blackmer, A. M., and Bremner, J. M. (1977). Nitrogen isotope discrimination in denitrification of nitrate in soils. Soil Biol. Biochem. 9, 73–77. doi: 10.1016/0038-0717(77)90040-2
Bogeat-Triboulot, M., Buré, C., Gerardin, T., Chuste, P., Le Thiec, D., Hummel, I., et al. (2019). Additive effects of high growth rate and low transpiration rate drive differences in whole plant transpiration efficiency among black poplar genotypes. Environ. Exp. Bot. 166:21:103784. doi: 10.1016/j.envexpbot.2019.05.021
Broeckx, L., Fichot, R., Verlinden, M., and Ceulemans, R. (2014). Seasonal variations in photosynthesis, intrinsic water-use efficiency and stable isotope composition of poplar leaves in a short-rotation plantation. Tree Physiol. 34, 701–715. doi: 10.1093/treephys/tpu057
Brueck, H. (2008). Effects of nitrogen supply on water-use efficiency of higher plants. J. Plant Nutr. 171, 210–219. doi: 10.1002/jpln.200700080
Chen, S., Bai, Y., Zhang, L., and Han, X. (2005). Comparing physiological responses of two dominant grass species to nitrogen addition in Xilin River basin of China. Environ. Exp. Bot. 53, 65–75. doi: 10.1016/j.envexpbot.2004.03.002
Cooke, J., Martin, T., and Davis, J. (2005). Short-term physiological and developmental responses to nitrogen availability in hybrid poplar. New Phytol. 167, 41–52. doi: 10.1111/j.1469-8137.2005.01435.x
Craine, J. M., Brookshire, E. N. J., Cramer, M. D., Hasselquist, N. J., Koba, K., Marin-Spiotta, E., et al. (2015). Ecological interpretations of nitrogen isotope ratios of terrestrial plants and soils. Plant Soil 396, 1–26. doi: 10.1007/s11104-015-2542-1
Craine, J. M., Elmore, A. J., Aidar, M. P. M., Bustamante, M., Dawson, T. E., Hobbie, E. A., et al. (2009). Global patterns of foliar nitrogen isotopes and their relationships with climate, mycorrhizal fungi, foliar nutrient concentrations, and nitrogen availability. New Phytol. 183, 980–992. doi: 10.1111/j.1469-8137.2009.02917.x
Cronk, Q. (2005). Plant eco-devo: the potential of poplar as a model organism. New Phytol. 166, 39–48. doi: 10.1111/j.1469-8137.2005.01369.x
Delucia, E. H., and Schlesinger, W. H. (1991). Resource-use efficiency and drought tolerance in adjacent Great Basin and Sierran plants. Ecology 72, 51–58. doi: 10.2307/1938901
Farquhar, G. D., Ehleringer, J. R., and Hubick, K. T. (1989). Carbon isotope discrimination and photosynthesis. Annu. Rev. Plant Physiol. Plant Mol. Biol. 40, 503–537. doi: 10.1146/annurev.pp.40.060189.002443
Field, C., Merino, J., and Mooney, H. A. (1983). Compromises between water-use efficiency and nitrogen-use efficiency in five species of California evergreens. Oecologia 60, 384–389. doi: 10.1007/BF00376856
Garavillon-Tournayre, M., Gousset-Dupont, A., Gautier, F., Benoit, P., Conchon, P., Souchal, R., et al. (2018). Integrated drought responses of black poplar: how important is phenotypic plasticity? Physiol. Plant 163, 30–44. doi: 10.1111/ppl.12646
Guerra, F., Gainza-Cortés, F., Pérez-Castro, R., and Zamudio, F. (2011). “Phytoremediation of heavy metals using poplars (Populus spp.): a glimpse of the plant responses to copper, cadmium and zinc stress” in Handbook of Phytoremediation. ed. I. A. Golubev (New York, NY: Nova Science), 387–413.
Guet, J., Fabbrini, F., Fichot, R., Sabatti, M., Bastien, C., and Brignolas, F. (2015). Genetic variation for leaf morphology, leaf structure and leaf carbon isotope discrimination in European populations of black poplar (Populus nigra L.). Tree Physiol. 35, 850–863. doi: 10.1093/treephys/tpv056
Han, M., Noh, E., and Han, S. (2013). Enhanced drought and salt tolerance by expression of AtGSK1 gene in poplar. Plant Biotechnol. Rep. 7, 39–47. doi: 10.1007/s11816-012-0258-8
Hikosaka, K., Hanba, Y., Hirose, T., and Terashima, I. (1998). Photosynthetic nitrogen-use efficiency in leaves of woody and herbaceous species. Funct. Ecol. 12, 896–905. doi: 10.1046/j.1365-2435.1998.00272.x
Himes, A., Emerson, P., McClung, R., Renninger, H., Rosenstiel, T., and Stanton, B. (2021). Leaf traits indicative of drought resistance in hybrid poplar. Agric. Water Manag. 246:106676. doi: 10.1016/j.agwat.2020.106676
King, J., Ceulemans, R., Albaugh, J., Dillen, S. Y., Domec, J. C., Fichot, R., et al. (2013). The challenge of lignocellulosic bioenergy in a water-limited world. Bioscience 63, 102–117. doi: 10.1525/bio.2013.63.2.6
Lemaire, C., Blackman, C. J., Cochard, H., Menezes-Silva, P., Torres-Ruiz, J. M., and Herbette, S. (2021). Acclimation of hydraulic and morphological traits to water deficit delays hydraulic failure during simulated drought in poplar. Tree Physiol. 41, 2008–2021. doi: 10.1093/treephys/tpab086
Marron, N., Bastien, C., Sabatti, M., Taylor, G., and Ceulemans, R. (2006). Plasticity of growth and sylleptic branchiness in two poplar families grown at three sites across Europe. Tree Physiol. 26, 935–946. doi: 10.1093/treephys/26.7.935
Marron, N., and Ceulemans, R. (2006). Genetic variation of leaf traits related to productivity in a Populus deltoides × Populus nigra family can. J. For. Res. 36, 390–400. doi: 10.1139/x05-245
Marron, N., Dillen, S. Y., and Ceulemans, R. (2007). Evaluation of leaf traits for indirect selection of high yielding poplar hybrids. Environ. Exp. Bot. 61, 103–116. doi: 10.1016/j.envexpbot.2007.04.002
Marron, N., Villar, M., Dreyer, E., Delay, D., Boudouresque, E., Petit, J. M., et al. (2005). Diversity of leaf traits related to productivity in 31 Populus deltoides × Populus nigra clones. Tree Physiol. 25, 425–435. doi: 10.1093/treephys/25.4.425
Miller, R. (2018). Growth variation among hybrid poplar varieties in Michigan, USA and the implications for commercial biomass production. Bioenergy Res. 11, 816–825. doi: 10.1007/s12155-018-9937-9
Monclus, R., Dreyer, E., Delmotte, F., Villar, M., Delay, D., Boudouresque, E., et al. (2005). Productivity, leaf traits and carbon isotope discrimination in 29 Populus deltoides × P. nigra clones. New Phytol. 167, 53–62. doi: 10.1111/j.1469-8137.2005.01407.x
Monclus, R., Dreyer, E., Villar, M., Delmotte, F., Delay, D., Petit, J., et al. (2006). Impact of drought on productivity and water use efficiency in 29 genotypes of Populus deltoides x Populus nigra. New Phytol. 169, 765–777. doi: 10.1111/j.1469-8137.2005.01630.x
Monclus, R., Villar, M., Barbaroux, C., Bastien, C., Fichot, R., Delmotte, F., et al. (2009). Productivity, water-use efficiency and tolerance to moderate water deficit correlate in 33 poplar genotypes from a Populus deltoides x Populus trichocarpa F1 progeny. Tree Physiol. 29, 1329–1339. doi: 10.1093/treephys/tpp075
Natural Resources Conservation Service (2023). United states department of agriculture. Web Soil Survey. Available at: (https://websoilsurvey.sc.egov.usda.gov/).
Nelson, N., Berguson, W., Mcmahon, B., Cai, M., and Buchman, D. (2018). Growth performance and stability of hybrid poplar clones in simultaneous tests on six sites. Biomass Bioenergy 118, 115–125. doi: 10.1016/j.biombioe.2018.08.007
NOAA (2023). U.S. climate normals: national centers for environmental information. Available: (https://www.ncei.noaa.gov/products/land-based-station/us-climate-normals).
Ostrom, N. E., Knoke, K. E., Hedin, L. O., Robertson, G. P., and Smucker, A. J. M. (1998). Temporal trends in nitrogen isotope values of nitrate leaching from an agricultural soil. Chem. Geol. 146, 219–227. doi: 10.1016/S0009-2541(98)00012-6
Pellis, A., Laureysens, I., and Ceulemans, R. (2004). Growth and production of a short rotation coppice culture of poplar I. Clonal differences in leaf characteristics in relation to biomass production. Biomass Bioenergy 27, 9–19. doi: 10.1016/j.biombioe.2003.11.001
Plavcová, L., and Hacke, U. (2012). Phenotypic and developmental plasticity of xylem in hybrid poplar saplings subjected to experimental drought, nitrogen fertilization, and shading. J. Exp. Bot. 63, 6481–6491. doi: 10.1093/jxb/ers303
Pliura, A., Zhang, S., Mackay, J., and Bousquet, J. (2007). Genotypic variation in wood density and growth traits of poplar hybrids at four clonal trials. For. Ecol. Manag. 238, 92–106. doi: 10.1016/j.foreco.2006.09.082
Polle, A., Janz, D., Teichmann, T., and Lipka, V. (2013). Poplar genetic engineering: promoting desirable wood characteristics and pest resistance. Appl. Microbiol. Biotechnol. 97, 5669–5679. doi: 10.1007/s00253-013-4940-8
R Core Team (2022). R: a language and environment for statistical computing. Vienna, Austria: R Foundation for Statistical Computing.
Reich, P. B. (2014). The world-wide ‘fast–slow’ plant economics spectrum: a traits manifesto. J. Ecol. 102, 275–301. doi: 10.1111/1365-2745.12211
Renninger, H. J., Pitts, J. J., and Rousseau, R. (2023). Comparisons of biomass, water use efficiency and water use strategies across five genomic groups of Populus and its hybrids. Glob. Change Biol. Bioenergy. 15, 99–112. doi: 10.1111/gcbb.13014
Renninger, H. J., Stewart, L. F., Freeman, J. L., and Rousseau, R. J. (2021a). Physiological functioning and productivity in eastern cottonwood and hybrid poplars on contrasting sites in the southeastern US. Bioenergy Res. 15, 1057–1070. doi: 10.1007/s12155-021-10377-y
Renninger, H. J., Stewart, L. F., and Rousseau, R. J. (2021b). Water use, efficiency, and stomatal sensitivity in eastern cottonwood and hybrid poplar varietals on contrasting sites in the southeastern U.S. Frontiers in forests and global. Change 4:704799. doi: 10.3389/ffgc.2021.704799
Ridge, C. R., Hinckley, T. M., Stettler, R. F., and Van Volkenburgh, E. (1986). Leaf growth characteristics of fast-growing poplar hybrids Populus trichocarpa × P. deltoides. Tree Physiol. 1, 209–216. doi: 10.1093/treephys/1.2.209
Robinson, B., Mills, T., Petit, D., Fung, D., Green, S., and Clothier, B. (2000). Natural and induced cadmium-accumulation in poplar and willow: implications for phytoremediation. Plant Soil 227, 301–306. doi: 10.1023/A:1026515007319
Rönnberg-Wästljung, A., Adler, A., Karacic, A., Liepins, K., Richards, T., Ingvarsson, K., et al. (2022). Phenotypic plasticity in Populus trichocarpa clones across environments in the Nordic–Baltic region. Scand. J. For. Res. 37, 1–5. doi: 10.1080/02827581.2022.2039279
Sage, R. F., and Pearcy, R. W. (1987). The nitrogen use efficiency of C3 and C4 plants: II. Leaf nitrogen effects on the gas exchange characteristics of Chenopodium album (L.) and Amaranthus retroflexus (L.). Plant Physiol. 84, 959–963. doi: 10.1104/pp.84.3.959
Soolanayakanahally, R. Y., Guy, R. D., Silim, S. N., Drewes, E. C., and Schroeder, W. R. (2009). Enhanced assimilation rate and water use efficiency with latitude through increased photosynthetic capacity and internal conductance in balsam poplar (Populus balsamifera L.). Plant Cell Environ. 32, 1821–1832. doi: 10.1111/j.1365-3040.2009.02042.x
Spinelli, R., and Hartsough, B. R. (2006). Harvesting SRF poplar for pulpwood: experience in the Pacific northwest. Biomass Bioenergy 30, 439–445. doi: 10.1016/j.biombioe.2005.11.021
Stanton, B., Eaton, J., Johnson, J., Rice, D., Schuette, B., and Moser, B. (2002). Hybrid poplar in the Pacific northwest: the effects of market-driven management. J. For. 100, 28–33. doi: 10.1093/jof/100.4.28
Thakur, A. K., Kumar, P., Parmar, N., Shandil, R. K., Aggarwal, G., Gaur, A., et al. (2021). Achievements and prospects of genetic engineering in poplar: a review. New For. 52, 889–920. doi: 10.1007/s11056-021-09836-3
U.S. Department of Energy (2016). 2016 billion-ton report: Advancing domestic resources for a thriving bioeconomy. Volume 1: Economic availability of feedstocks. Oak Ridge, TN, USA: Oak Ridge National Laboratory.
Vigar, M., Smith, H., Cohen, D., Dewoody, J., Trewin, H., Steenackers, M., et al. (2016). Adaptive mechanisms and genomic plasticity for drought tolerance identified in European black poplar (Populus nigra L.). Tree Physiol. 36, 909–928. doi: 10.1093/treephys/tpw017
Violle, C., Navas, M., Vile, D., Kazakou, E., Fortunel, C., Hummel, I., et al. (2007). Let the concept of trait be functional! Oikos 116, 882–892. doi: 10.1111/j.0030-1299.2007.15559.x
Voltas, J., Serrano, L., Hernández, M., and Pemán, J. (2006). Carbon isotope discrimination, gas exchange and stem growth of four Euramerican hybrid poplars under different watering regimes. New For. 31, 435–451. doi: 10.1007/s11056-005-0879-7
Vose, J., Swank, W., Harvey, G., Clinton, B., and Sobek, C. (2000). Leaf water relations and sapflow in eastern cottonwood (Populus deltoides Bartr.) trees planted for phytoremediation of a groundwater pollutant. Int. J. Phytoremediation 2, 53–73. doi: 10.1080/15226510008500030
Willis, J., Renninger, H., Schnake, D., and Alexander, H. (2023). Xerophytic hardwood retention promotes competition over facilitation in longleaf pine woodlands in the absence of fire. For. Ecol. Manag. 531:120792. doi: 10.1016/j.foreco.2023.120792
Wright, I. J., Reich, P. B., Westoby, M., Ackerly, D. D., Baruch, Z., Bongers, F., et al. (2004). The worldwide leaf economics spectrum. Nature 428, 821–827. doi: 10.1038/nature02403
Wu, R., and Stettler, R. (1998). Quantitative genetics of growth and development in Populus. III. Phenotypic plasticity of crown structure and function. Heredity 81, 299–310. doi: 10.1046/j.1365-2540.1998.00397.x
Xu, B., Long, Y., Feng, X., Zhu, X., Sai, N., Chirkova, L., et al. (2021). GABA signalling modulates stomatal opening to enhance plant water use efficiency and drought resilience. Nat. Commun. 12:1952. doi: 10.1038/s41467-021-21694-3
Keywords: short rotation woody crops, cottonwood, bioenergy feedstocks, genotype×environment interactions, leaf mass per area, leaf gas exchange, stable isotopes
Citation: Renninger HJ, Pitts JJ and Wang J (2024) Leaf-level physiological strategies related to productivity and plasticity of Populus in the Southeastern United States. Front. For. Glob. Change. 7:1467381. doi: 10.3389/ffgc.2024.1467381
Edited by:
Ned Fetcher, Wilkes University, United StatesReviewed by:
Alexander Wait, Missouri State University, United StatesMichael Tyree, Indiana University of Pennsylvania, United States
Copyright © 2024 Renninger, Pitts and Wang. This is an open-access article distributed under the terms of the Creative Commons Attribution License (CC BY). The use, distribution or reproduction in other forums is permitted, provided the original author(s) and the copyright owner(s) are credited and that the original publication in this journal is cited, in accordance with accepted academic practice. No use, distribution or reproduction is permitted which does not comply with these terms.
*Correspondence: Heidi J. Renninger, aHI0MjdAbXNzdGF0ZS5lZHU=
†Present addresses: Justin J. Pitts, Department of Agronomy, University of Florida, Gainesville, FL, United StatesJiaxin Wang, Department of Forest Resources and Environmental Conservation, Virginia Polytechnic and State University, Blacksburg, VA, United States