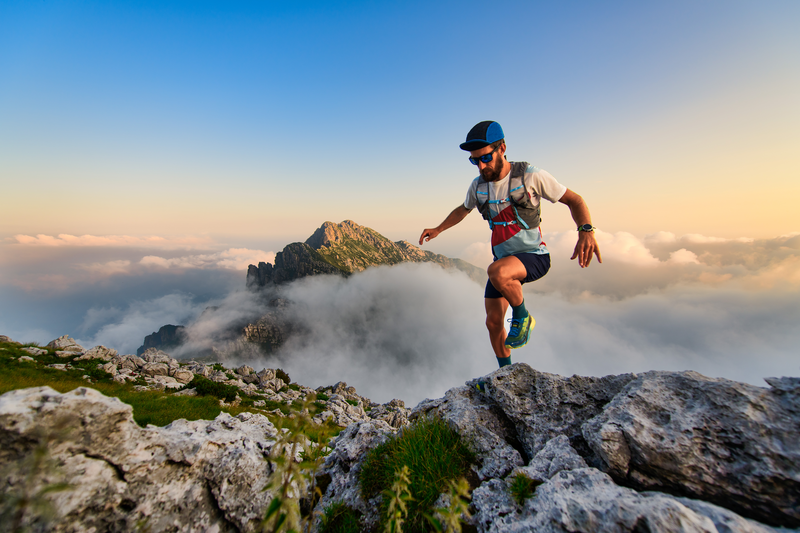
94% of researchers rate our articles as excellent or good
Learn more about the work of our research integrity team to safeguard the quality of each article we publish.
Find out more
ORIGINAL RESEARCH article
Front. For. Glob. Change , 07 January 2025
Sec. People and Forests
Volume 7 - 2024 | https://doi.org/10.3389/ffgc.2024.1461932
Climate change-associated disturbances such as storms, wildfires, and pest outbreaks increasingly destabilize forest systems, threatening their ecological, economic, and social functions. These disruptions impact the forest value chain (FVC) by causing fluctuations in timber supply, from a quantity and quality perspective. This study employed the operational resilience framework (ORF) to assess FVC resilience in five European case studies (CZ, HR, DE, FIN, and ESP), focusing on timber supply as a key system variable. A resilience assessment was conducted using resilience thresholds, considering sustainability from both ecological and economic perspectives. Principal component analysis (PCA) identified three predictor groups that influenced FVC resilience: wood production (WP), harvesting systems (HS), and management and silviculture (MS). Findings revealed that regions with proactive management and sufficient processing capacities (CZ, HR, and ESP) maintained relative stability despite natural disturbances, while others (DE and FIN) experienced prolonged instability due to market-driven logging practices and limited adaptive measures. The study highlighted the frequent breaching of resilience thresholds, particularly during high-volume salvage logging following disturbances such as bark beetle outbreaks, windstorms, and wildfires. The results emphasized the importance of integrating adaptive and proactive strategies to mitigate these impacts. The ORF demonstrated potential for operationalizing FVC resilience and provided guidance for improving preparedness against future disturbances.
Climate change increasingly threatens the stability of forest social-ecological systems, as the frequency and severity of natural disturbances such as storms, wildfires, and pest outbreaks intensify across broader regions (Seidl and Senf, 2024). The changes alter forest dynamics, decreasing forest health, and weakening ecosystem resilience, with cascading effects on the forest value chain (FVC; Patacca et al., 2023; Nikinmaa et al., 2024). Issues such as inconsistent timber supply, fluctuating wood quality, and market instability challenge stakeholders in maintaining steady operations. While substantial research exists on resilience frameworks at national and international levels (Seidl et al., 2017; Messier et al., 2019; Asada et al., 2023), local-scale operations need context-specific strategies to optimally adapt to disruptions and ensure long-term sustainability of their FVCs, which is an under-researched outlook on value chain resilience.
According to Porter (1985) a value chain “is a series of activities that create value at each step, with the total value being the sum of all contributions throughout the company.” In the context of forest social-ecological systems (forest systems), the FVC refers to a sequence of activities that use forest-based resources as inputs for processing and production of goods and services that are delivered to final consumers and ultimately disposed. Primary activities include harvesting, logistics, and packaging while supporting activities include marketing, planning, and managing (Kaplinsky and Morris, 2000; D’Amours et al., 2017). The FVC is vital for sustainable resource management, economic growth, and ecosystem service provisioning. Besides the usefulness of the value chain approach in optimizing the systems for their performance or efficiency of resource use, FVCs can be used to provide useful insights into the resilience of the forest-based industries and their constituent parts. Resilience of European FVCs needs to be considered for a diverse set of stressors, e.g., the disturbances in Central Europe, such as prolonged droughts, are causing widespread tree mortality, partly due to bark beetle outbreaks (Schuldt et al., 2020). These outbreaks have led to extensive salvage logging of spruce forests in the Czechia and Germany, saturating wood markets and collapsing sawlog prices (Hlásny et al., 2021; Sommerfeld et al., 2021). Coniferous species, which dominate the region, are more vulnerable to climate-induced disturbances than broadleaf and mixed forests (Seidl et al., 2017; Steckel et al., 2020). Moreover, in the Mediterranean region, wildfires are becoming larger and more severe, causing direct damage to the existing forest stock (De Rigo et al., 2017; López-Rodríguez et al., 2021). These challenges underscore the importance of improving resilience, not only in forest ecosystems but also across the FVC, to sustain operations and minimize cascading impacts.
Ensuring adaptability in forest systems depends on proactive measures that synergistically enhance their resilience, enabling them to withstand and recover from disturbances (Pingoud and Wagner, 2006). The widespread use of resilience has led to various definitions and approaches in the context of the forest system, and three key levels can be distinguished: engineering, ecological, and social-ecological resilience (SER) (Nikinmaa et al., 2020, Lindner et al., 2020). Engineering resilience refers to a system’s ability to return to its equilibrium state after a disturbance (Holling, 1996; Pimm, 1984). Ecological resilience, on the other hand, is defined as a system’s ability to withstand disturbances from its equilibrium state (Holling, 1996; Scheffer et al., 2015). Finally, SER represents the capacity of the system to continue providing ecosystem services while undergoing disturbances (Biggs et al., 2015).
Based on the concept of SER, Lloret et al. (2024) proposed a novel approach for operationalizing the resilience of forest systems, termed the operational resilience framework (ORF). This approach incorporates various system boundaries, disturbances or stressors, resilience descriptors (so-called system variables) and resilience indicators in the form of predictors or co-drivers to quantitatively assess the resilience levels of forest systems at different scales. Specifically, in this context, operationalization involves analyzing the performance of system variables (i.e., characteristics of ecosystem services or the FVC) and describing the state of a system in response to disturbances, relative to some reference state (Lloret et al., 2024). The performance of the system variable considered, along with the relevant disturbances, is observed over a specific period relative to a reference state (i.e., which may correspond to the hypothetical absence of stressors or to a desired state of the system variable) and pertinent environmental, climatic or management changes, thus enabling the comparison of resilience across various contexts such as regions, management practices, and policies (Cantarello et al., 2024). Following this, the relationships between system variables, resilience predictors (i.e., variables that can be manipulated), and co-drivers (i.e., variables that cannot be manipulated meaningfully, but provide valuable information about the context) are observed, thus enabling an operational assessment of the system’s resilience (Lloret et al., 2024).
The system variable that reflects resilience in both forest as ecosystem and their related FVCs is timber supply—the volume of harvested timber available for supply to the market (Prestemon and Wear, 1999). Timber supply depends on market and nonmarket factors, including the merchantable stock of standing timber and stakeholder-specific circumstances (Tian et al., 2017; Hurmekoski et al., 2018). In literature, various resilience predictors and co-drivers can be found that impact FVCs, some of which apply to international or national levels, while others are region-specific (e.g., harvesting technologies, processing capacities, tree species composition, etc.). Moreover, strengthening local forestry networks can enhance resilience by reducing costs, improving resource access, and adapting to market trends (Macqueen, 2010), so variables connected to network structure, performance or efficiency can work as effective resilience predictors or co-drivers.
Value chain actors need concrete, operational decision-making support to enhance FVC resilience. However, there is a lack of integrated operational assessment strategies tailored to regional-level actors. This study aims to fill this gap with two main objectives, (1) integrate an operational resilience assessment to natural disturbances based on quantitative analysis which could help regional-level actors in mitigating and adapting their operations to the changing disturbance regimes, and (2) provide a (non-exhaustive) list of resilience predictors and co-drivers based on their relationship with the supply of timber to the market, considered as system variable.
The research focused on the assessment of FVC resilience based on historical, regional data. Because we researched resilience from a regional perspective, the study was conducted using a case study approach (which encompasses the entire region, including other actors within it), covering five introducing European countries: Kostelec, Czechia (CZ), Upper Rhine Valley, Germany (DE), Istria, Croatia (HR), Kainuu, Finland (FIN), and Galicia, Spain (ESP; Figure 1). These case studies were selected to cover major bio-geographic regions and exemplars (the specific company which we are studying in each region) were chosen to represent diverse forest management systems and FVC setups across Europe. For instance, CZ, DE, and HR had mid-scale wood processing industries, in which the use of timber and forest products was mostly confined to the regional level due to the limited export capabilities of the forest-based industries. In contrast, FIN and ESP had more diverse processing industries with broad access to international markets, though in the case of FIN, the industry was driven by large companies while in ESP, the industry consisted of a large number of small-scale community driven companies. Given these regional differences, the species composition varied across the studied regions. In CZ, the forests were predominantly composed of Norway spruce, with overall low species diversity. In HR, the forests exhibited high diversity, with broadleaved species dominating, followed by beech, oaks, and conifers. Then in DE, forests managed by the case study featured primarily beech, along with fir and Norway spruce; however, conifer dominance has declined due to repeated disturbances. In FIN, forests were predominantly coniferous, mainly pines, with a preference for harvesting softwood species. Finally, in ESP, broadleaved species dominated, particularly eucalyptus, followed by pine and other softwoods. Also, the main disturbances during the study period varied across countries, with bark beetle outbreaks playing a prominent role in CZ, windstorms in DE and FIN, ice storms and windstorms in HR, and wildfires in ESP.
Figure 1. Five European case studies: Kostelec, Czechia (CZ), Upper Rhine Valley, Germany (DE), Istria, Croatia (HR), Kainuu, Finland (FIN), and Galicia, Spain (ESP). Illustration by Gabriela Rueda, EFI (adapted by Rueda, 2022).
Within each country, a forest enterprise was selected as an exemplar (Figure 2). To ensure comparability between the case studies, exemplars were selected based on the spatial range of forests they managed—between 500 and 5,000 ha. The spatial range allowed the integration of smaller case studies characterized by lower processing capacities while incorporating larger enterprises with greater economic capacities, all representing key aspects of the forest-based industries. If an exemplar that fit the spatial range could not be found within a case study, a suitable and cooperative exemplar enterprise was selected and the spatial range of its operation was scaled up or down by multiplying the original size of forests managed by an exemplar by the ratio of the lower or upper bound of the spatial range to the original size of the area of the forests managed by said exemplar. The case studies which did not need readjustments were CZ and DE while HR, FIN, and ESP needed a downscaling in their data.
Two formats for primary data collection were used: a standardized dataset template and a survey. To streamline the data collection process, we began by establishing a common dataset structure. Working closely with case study representatives, we developed a standardized dataset template, containing a unified list of variables to be gathered, thus ensuring consistency and reliability of collected data (Supplementary Annex I). Additionally, each topic was further elaborated into specific questions, case study reports, related to resilience predictors and co-drivers to accompany the gathered data and provide context. These case study reports were specifically tailored to the unique conditions and characteristics of each case study, ensuring filling information gaps and ensured that the collected data remained relevant and informative. Primary data collection included gathering operational data from the exemplar and operational and industrial data from stakeholders through case study reports. If primary data was not sufficient to satisfy data needs, secondary data was obtained through reviews of literature, databases, and gray literature.
Annual data were collected for the period from 2001 to 2021, the following topics we covered: regulatory frameworks, forest management practices, business models, resource availabilities, technologies, harvesting and storage capacities, network properties, particularly wood processing capabilities (such as sawmilling, wood-based panels, pulp and paper, downstream industries, and bioenergy production) of the exemplars or their networks. Additionally, we gathered data on disturbances, expressed as annual volumes of salvage logging (m3 year−1) and disturbance agents, such as wildfires, windstorms, and insect outbreaks, which impacted the volume and quality of salvaged timber.
We chose the annual supply of timber to the market (henceforth timber supply) as the system variable. The system variable was formulated as the sum of the annual provisioning of timber (i.e., voluntary and salvage logging) by the forest enterprise to other value chain actors involved in the timber processing, i.e., lumber and other sawmill producers, pulp and paper producers, and heat and power producers (Supplementary Annex II, correspondence of the components of the assessment of the FVC with the ORF).
In our study, we defined a forest enterprise as sustainable and resilient when it was able to maintain operations without depleting the forest it managed—establishing an upper resilience threshold (UTH)—while also generating stable revenues that exceed costs, thereby setting a lower resilience threshold (LTH). Resilience in this context aligned with sustainability but emphasized the impact of disturbances, such as bark beetle outbreaks in Central Europe (quantified in our case through salvage logging, Figure 3), as potential disruptors of system functioning. To support regional value chain actors, we developed a resilience assessment.
Figure 3. Representation of resilience metrics and measurement for resilience assessment. The blue line represents the System variable (a), which in this case is timber supply, the red line represents the Disturbance (b), summarized as salvage logging in this study, and the gray straight lines are upper (UTH) and lower thresholds (LTH). The time series from 1 to n, corresponds to a selected number of time intervals (e.g., years). In this example, the dotted gray rectangles highlight periods where the system exhibits a lack of resilience, i.e., when timber supply exceeds both UTH and LTH. A vertical double arrow (black) is used to depict the resilience metric, and horizontal double arrows (orange) represent the resilience measure, illustrating the system’s ability to stay within thresholds despite disturbances.
Resilience was assessed by comparing the observed development of the system variable, timber supply, over time against a reference state framed by upper (Eq. 1), and lower (Eq. 2) thresholds. That meant that the enterprise–based system was considered to be fully resilient if its related timber supply remained within these thresholds defined by sustainable timber extraction from the forest (UTH) and sustainable enterprise operation (LTH).
Where, UTH is the upper resilience threshold, defined as the mean annual logging prescriptions according to forest management plan from year 1 (2001) to year n (2021) of the observed period; and n is the number of years within the observed period.
Where, LTH is the lower resilience threshold, defined as the minimal annual timber supply by the exemplar, expressed as the profitability of forest enterprises (x%) in particular case study country based on literature, since empirical data on the profitability of the exemplars were not available.
The LTH was defined in terms of the exemplar’s capacity to generate profit (Table 1). So, the LTH then considers the exemplar’s financial performance in relation to its lowest level of timber supply.
The calculation of the system variable referred to the annual values of the timber supply during the observed period. We devised a resilience metric, given by the vertical distance between timber supply and the UTH or LTH in a given year. The measure of the resilience of the exemplar was then assessed based on both the vertical distance between timber supply and UTH/LTH as well as the time lapse between the year when the system variable breached the reference state (i.e., UTH or LTH) and the year when it returned to the reference state. If a exemplar was able to promptly return the system variable to its reference state, it likely demonstrated greater resilience compared to a situation when the exemplar continuously operated so that its system variable was outside of its reference state, as exemplified in Figure 3.
An exploratory overview of all variables collected (Supplementary Annex I) in the standardized data set took place to select meaningful variables for quantitative analysis and to ensure applicability across all countries (Table 2). We identified 11 variables explaining the resilience dynamics of timber supply in each case study (resilience predictors, which correspond to actions suitable to be implemented, and co-drivers, which correspond to contextual variables, sensu Lloret et al., 2024). It is important to note that due to the challenges they posed from an analytical point of view, binary variables were omitted from analyses (e.g., species presence). Variables with minimal variation or limited data availability throughout the observed period were also omitted. Nonetheless, excluded variables were leveraged to contextualize the selected variables, contributing to the holistic narrative for each case study.
First, descriptive statistics were computed to provide a summary of the main aspects of the dataset. Spearman correlation analyses were conducted to assess the strength of the associations between the timber supply and the resilience predictors and co-drivers in the different case studies. These correlations were used to interpret timber supply resilience, according to its dynamics referred to UTH and LTH boundaries.
Following the correlation analysis, we employed a biplot principal component analysis (PCA), as a dimensionality reduction technique (Gower et al., 2011) adopted in several analytical settings in forestry (Liubachyna et al., 2017; Ficko et al., 2019; Riccioli et al., 2020). The PCA enabled the identification of factors driving variation in each case study, revealing patterns and correlations within the dataset by showing the tendencies of variables to move in similar directions. Importantly, PCA facilitated the visualization of temporal impacts by observing data points representing different periods, tracking shifts over time, and identifying trends. An integrated vision of the various predictors created a multivariate space, allowing us to visualize their characteristics and patterns of change over time, thus helping to understand the temporal sequence and interactions among the predictors. The PCAs were conducted using R Studio, with R version 4.3.1 utilized for the analyses (RStudio Team, 2020).
The findings showed notable trends concerning harvesting systems across different case studies. Cases such as CZ and DE showed higher volumes in both HS-PL (54,195 and 7,310 m3 year−1, respectively) and HS-SL (24,483 and 4,580 m3 year−1, respectively). The CZ demonstrated the highest mean volume of harvested timber using the cut-to-length (CTL) logging method (16,789 m3 year−1), while HR was the only country where animals were used in forest harvesting (1,283 m3 year−1). On the other hand, FIN and ESP, with their complex forest industries, showed high production volumes, especially evident from the mean volumes of produced sawlogs (2,942 and 11,928 m3 year−1), pulpwood (44,773 and 3,561 m3 year−1, respectively) and energy wood (2,794 m3 year−1 in ESP). These significant production volumes likely contributed substantially to the output of the forest-based sectors of these countries. Further details of the descriptive statistics of each case study can be found in Supplementary Annex III.
To uncover the relationships between timber supply and the 11 predictors and co-drivers, a Spearman correlation analysis was performed for each case study (Table 3). Timber supply was significantly positively correlated with salvage logging (HS-SL) in all case studies except for the CZ. The use of cut-to-length harvesting systems (HS.CTL) showed a strong positive correlation with timber supply in CZ, DE, and ESP. In contrast, only HR exhibited a significant positive correlation between timber supply and the management and silviculture co-drivers (MS.HTS and MS.TRA). Timber production (including sawlogs, pulpwood, and energy wood) showed moderate to strong significant correlations with timber supply across all case studies.
Table 3. Correlation analysis for each case study between system variable, timber supply, and resilience predictors and co-drivers.
Figure 4 illustrates the outcomes of resilience assessments for case studies. Beginning with the first block of countries, CZ, HR, and DE were categorized within the mid-scale wood processing industry. In the CZ (Figure 4, CZ), timber supply remained mostly within resilience thresholds during the first decade of the study. In 2015, salvage logging increased to about 50% of total felling (50,051 mł year−1), rising to 99% between 2018 and 2021 due to a large-scale bark beetle outbreak. This peak reached 72,000 mł year−1, exceeding resilience thresholds and signaling unsustainable operational mode if continued. Under the Forestry Act of 1995, salvage logging is mandatory and reforestation must occur within 2 years. Thus, regulation, coupled with market pressure often required rapid timber sales to avoid fines and degradation of timber.
Figure 4. Representation of resilience metrics for assessment CZ, HR, DE, FIN, and ESP from 2001 to 2021. The blue line shows the timber supply; the red line represents the disturbance (salvage logging volume), and the gray lines indicate the upper (UTH) and lower thresholds (LTH) specific for each case study. It highlights each case study’s ability to maintain timber supply within these thresholds despite natural disturbances.
Similar to CZ, HR stayed within its thresholds in years with low disturbance occurrence, due to its adherence to forest management plans (Figure 4, HR). However, disturbances in 2016 and 2020 caused timber supply to exceed thresholds, with salvage logging reaching 1,590 and 2,021 mł year−1, respectively. Sustained high salvage logging volumes, similar to those in CZ, could pose risks to long-term sustainability.
The German case study (DE) frequently exceeded its UTH throughout the observed period, reflecting high logging activity driven by frequent disturbances (Figure 2, DE). Notably, salvage logging accounted for 99% of timber supply in 2001 after the Lothar windstorm. From 2009 to 2021, timber supply consistently exceeded thresholds, with reductions only to compensate for earlier overharvesting. This underscores a reactive rather than preventive approach to forest management.
Then FIN and ESP were characterized by a diverse industry. Finland (FIN) exhibited a distinct trend, with a dramatic spike in timber supply in 2018 driven by a large-scale disturbance. During said year, salvage logging exceeded 100% of timber supply (Figure 4, FIN), which means that the exemplar was able to store the surplus salvaged timber and supply it to the market in later years. While historically affected by windstorms and snow damage, the impacts of these disturbances were relatively limited. Nevertheless, timber supply consistently exceeded UTH since 2006, likely due to increased timber supply driven by market demand. Notably, FIN provided indirect data, but national disturbance trends reflected the case study’s performance.
In ESP, timber supply showed a sharp increase from 2018 onward, with a peak at 22,134 mł year−1, corresponding to wildfire-driven logging in Galicia (Figure 4, ESP). With the exception of 2007, the earlier increases in timber supply (2000–2010) were likely driven by market demand rather than disturbances, though the 2006 fire season was particularly severe. In the Spanish region of Galicia (ESP), the Forestry Act of 2012 introduced Forest Management Plans to enhance sustainable forestry practices. However, due to the predominance of communal-private ownership and fragmented family forests, by 2022, only 20.6% of the forest area was covered by such plans. Moreover, most of these plans were advisory in nature, significantly limiting their impact on forest management practices.
Overall, CZ and HR demonstrated effective disturbance management, enabling them to maintain a resilient timber supply within their resources. In contrast, DE and FIN faced challenges due to disturbance-driven logging, which undermined long-term stability. Finally, ESP appeared resilient, but additional context is needed to confirm whether its situation was primarily driven by market demand rather than disturbances. Thus, these insights provide a broader understanding of resilience of forest systems over time.
The PCA for the case studies highlighted similarities and differences in forestry practices, temporal trends, and responses to disturbances across the regions (CZ, HR, DE, FIN, and ESP). Dim1 represented the most significant patterns in the data. The distribution of dots (observed years) across the quadrants (Figure 5) reflected temporal trends, highlighting shifts in forestry practices and resource usage over time.
Figure 5. In principal component analysis (PCA) biplot, variables per case study – CZ, HR, DE, FIN, and ESP – are represented by arrows within dimension 1 (Dim1) and dimension 2 (Dim2). These arrows illustrate the direction and the magnitude of each variable’s influence. The respective contributions of these variables to the timber supply (TS) are indicated by a color gradient. The observations, depicted as dots, correspond to different time periods, where “1” signifies the year 2001 and “21” represents 2021. The complete names of the variable abbreviations are provided in Table 2 of the section “2 Materials and methods.”
First, temporal trends aligned with the resilience assessment graphs and revealed two distinct periods, each lasting around 10 years, consistent with the typical duration of forest management plans. These changes reflected modifications in voluntary logging, harvesting systems, and timber production practices. However, deviations in specific years are evident, likely caused by external market disturbances or other contextual factors.
Second, the explained variance reflected a substantial portion of the variability in the data, ranging from 72.7% in FIN (Figure 5, FIN) to 87.5% in DE and ESP (Figure 5, DE and ESP). Dim1 consistently captured the dominant trends in forestry practices and resource usage, while Dim2 explained additional variability. In CZ, HR, DE, and ESP (Figure 5), the PCA explained over 84% of the variance, reflecting well-defined patterns in forest operations and responses to disturbances. While for FIN, with 72.7%, Dim1 and 2 explained slightly less variance, suggesting that additional factors (not captured in the PCA) may have played a more significant role in this case study.
About key predictors (Dim1 Drivers), a consistent pattern was observed across all case studies: TP.SP (Volume of Sawlogs Produced) was a common contributor to Dim1. However, additional predictors varied among region, reflecting differences in management priorities and regional contexts. For instance, CZ, DE, and ESP emphasized mechanized operations and market demand, with HS.CTL (cut-to-length harvesting) playing a key role. HS.SL (salvage logging) contributed either positively or negatively to Dim1 and 2 across all case studies, but FIN stood out with a negative loading, suggesting challenges related to overexploitation during periods of disturbance. In ESP, HS.PL likely reflected an increase in logging operations over time due to market demand. Lastly, in HR, MS.TRA (total resource availability) contributed positively to Dim1, indicating that sustainable resource management was a central strategy.
The PCAs revealed consistent patterns of adaptation and change in forestry practices over the studied period. Later years were characterized by increased mechanization, salvage logging, and market-driven shifts in timber production, while earlier years reflected a combination of less-intensive practices, species composition, and external factors not fully captured by the PCA.
Based on our observation, the frequency and severity of natural disturbances increased in all case studies over the observed period, which is in line with reporting in the literature (Bauhus et al., 2017; Johnstone et al., 2016; Patacca et al., 2023). Figure 4 showed that case study representatives reacted to the occurrence of natural disturbances by increasing the amount of salvage logging and consequently the amount of timber supply to the market (Table 2), though the changes in total timber supply were relatively small in most cases. On the other hand, we observed several instances of substantial natural disturbances across all case studies, which caused swings in timber supply beyond the resilience thresholds. For example, DE and CZ experienced significant disruptions to normal operation from windstorms like Lothar and Kyrill, both followed by secondary biotic disturbances, especially in the case of CZ. Also, FIN dealt with windstorms coupled with snow damage, HR faced challenges from ice storms and drought, while in Spain, wildfire activity was rising over the observed period.
Besides their quantitative effects, disturbances also influence the quality grades of timber harvested and supplied to the market, with timber downgrading varying based on the type and severity of the disturbances (Gunnarsson et al., 2004; Hlásny et al., 2019). Moreover, excess salvage logging involves hiring more people, increasing harvests, and reestablishing forest stands thus incurring excessive costs on the exemplars. The joint effects of oversupply and degraded quality of salvaged timber likely caused the enterprises to operate with lower profit margins per unit of timber sold (Fuchs et al., 2022; Greenwood et al., 2023). The combined effects of natural disturbances, therefore, posed a substantial challenge to the resilience of the operations of the case studies.
The resilience assessment (Figures 3, 4) showed that CZ and HR largely operated within resilience boundaries, only breaching the UTH under severe disturbances occurring toward the end of the observed period. In ESP, the timber supply had a relatively stable increasing trend throughout the observed period as can be seen in Figure 4. In ESP, however, the lack of peaks in timber supply when disturbances occurred was likely due to the nature of the disturbance agent (wildfire), which limits the amount of timber available for salvage logging (De Rigo et al., 2017). In the DE and FIN cases the UTHs were consistently exceeded by the companies, the breach was spanning 12 and 13 years, respectively. The duration of the breach hints at the lower ability of these exemplars to mitigate the effects of windthrow by decreasing voluntary logging (Kärhä et al., 2018; Sanginés de Cárcer et al., 2021).
In addition to the disturbance agent, factors such as exemplar size, its processing capacities and network size can substantially influence the ability to absorb disturbances through decreasing the amount of voluntary logging, as demonstrated by CZ, HR and to some extent, ESP. For instance, CZ leveraged its sawmill to efficiently process timber in-house and sell finished lumber products instead of supplying timber to the market. Similarly, the acquisition of a sawmill in 2020 in HR marks a step toward enhancing local processing capacity. In the case of ESP, even if the community-oriented forest sector relied on small-scale processing within the region, having a complex network of small players promoted the regional capacity to process excess timber. Conversely, DE’s limited processing capabilities hindered its ability to stabilize timber supply during disturbances. Indeed, the data showed that being able to process timber and having a well-developed network played a role in mitigating disturbance impacts and maintaining supply stability.
To further investigate relationships between timber supply, disturbances and the mode of operation of particular exemplars, we used a PCA-based procedure, which led to the identification of three clusters of variables that could serve as predictors or co-drivers of FVC resilience: timber production (TP), harvesting systems (HS), and management and silviculture (MS). The TP cluster, encompassing sawlogs, pulpwood, and energy wood, underscores the dual importance of monitoring routine timber production and the system’s responses to disturbances (Pettenella and Maso, 2011; Badini et al., 2018). In line with these findings, our study showed (Figure 5) that increased salvage logging goes hand in hand with a change of assortment structure (the share of pulpwood and energy wood produced). Therefore, the companies can increase their preparedness for disturbances by adapting their network structures and sawmill capacities.
In synergy with the adaptation of the customer base, companies can also increase their preparedness by introducing high productivity harvesting systems either by expanding their network of harvesting contractors or by increasing their own capacity to harvest more efficiently. For example, using a CTL system in favor of conventional logging systems can enhance extraction efficiency in regions with favorable terrain and species composition (Mederski et al., 2022; Udali et al., 2022). Still, fully mechanized harvesting systems are less common in more complex landscapes, such as Croatia (Vuletić et al., 2014) or countries where forestry is undercapitalized such as the Central and Eastern Europe region (Dvoøák et al., 2020). Rapid salvage logging operations can substantially limit the deterioration of the quality of timber supplied to the market in the case of disturbance and coupled with other recovery techniques—such as debarking, treatment and adequate storage—are essential for maintaining FVC resilience (Pischedda, 2004; Hlásny et al., 2019; Greenwood et al., 2023).
Finally, variables within the MS cluster, i.e., Harvestable timber stock (MS.HTS) and Total resource availability (MS.TRA) connected to forest growth and as such their change was gradual. Their sudden change would point toward a devastating extent of a disturbance event (or substantial malpractice); therefore, they were considered co-drivers. We observed no such instances in the data, and the behavior of MS.HTS and MS.TRA showed that they had a small immediate effect on timber supply. Even so, Braatz (2012) and Hörl et al. (2020) state that proactive and adaptive management strategies enhance long-term sustainability. Another important co-driver connected to the resource base (the resilience of forest ecosystems) is the tree species composition or biodiversity in general. Mixed-species stands have demonstrated better resilience to natural disturbances (Muñoz-Gálvez et al., 2021; Jactel et al., 2017; Pukkala, 2018) through the reduced susceptibility to windthrow and pest infestations (Thom et al., 2017; Romeiro et al., 2022). The challenges in improving FVC resilience lie also in the coordination of tactical to operational level measures with strategic level measures related to forest ecosystems and their resilience.
Indeed, diversifying forest structure and species composition is widely recognized as a key strategy for enhancing the ecological resilience of forests to climate change and related stressors (Pretzsch et al., 2021; Messier et al., 2022). Forest owners are often hesitant to implement measures such as replacing vulnerable species with more resilient variants or introducing alternative management systems due to perceived economic trade-offs of these measures (Knoke et al., 2017; Zamora-Pereira et al., 2021). While these approaches can strengthen forest resilience, many forest owners perceive that downstream industries are not yet adequately prepared to take the timber in quantities sufficient for the sustainable operation of timber producers (Lawrence, 2020; Mazziotta et al., 2023). Adopting a participatory networking structure integrating diverse expertise and stakeholder input can foster better information-sharing (Nikinmaa et al., 2023) and goal alignment along the FVC. Additionally, promoting innovation can support diversification, enhance efficiency, and improve flexibility in forest management and the processing industry while strengthening communication between these stakeholders (Hoeben et al., 2023). Furthermore, providing economic incentives (Juutinen et al., 2022; Haeler et al., 2023) can address forest owners’ short-term financial concerns, enabling them to sustain operations while markets for alternative timber species continue to develop, thus promoting long-term ecological and value chain resilience. The predictors and co-drivers of resilience identified within this study are tied within the broader scope of the ORF (Lloret et al., 2024) which provides a comprehensive multiscale resilience assessment of forest social-ecological systems. Literature provides insights into FVC resilience at national and international levels (Fuchs et al., 2022; Loeffler and Anderson, 2018; Seidl et al., 2017), methods focusing on resilience assessment on a local level are scarce and do not cover the variability of how companies operate in different world regions. For example, European FVCs are much more industry-driven and frequently companies are connected to well-integrated markets (Toivonen et al., 2002; Toppinen and Kuuluvainen, 2010; Dzian et al., 2019). In contrast, the more community-oriented approaches observed in Asia and Latin America create an environment where small- and medium-sized enterprises can grow and compete with larger enterprises through policy setting. These approaches often prioritize long-term resource management, balancing the goals of generating employment with conserving the diverse services provided by forests that sustain local communities and ecosystems (Molnar et al., 2007; Hajjar et al., 2013; Baynes et al., 2015). Understanding these structural differences highlights opportunities for developing context-specific strategies that align with economic, social, and operational needs.
This study focused on the local-level resilience assessment of five European case studies. Aside from its geographical focus, it also focused on the interface between forest management and first-stage timber processing of the FVC. Furthermore, the observed period was limited from the point of view of our ability to observe long-term trends connected mainly to forest management and silvicultural data. However, this was a trade-off necessitated by the use of exemplar operational records. Despite these limitations, our findings emphasize that by integrating adaptive management practices and strategies, the FVC can become more sustainable and better equipped to address evolving environmental challenges.
This study provides a methodical framework for the operationalization of FVC resilience. We proposed using timber supply as a system variable because, on the one hand, it relates to an essential provisioning ecosystem service, and on the other hand, it provides information about the amount of timber available as input material for value chain actors. We also proposed thresholds of resilience based on the sustainability of exemplar operations, from both ecological and economic perspectives. The resilience metric was defined by the system’s ability to remain within thresholds, while its measurement focused on the magnitude of breaches and the time required for recovery to the reference state set by the thresholds. Since this study represents an initial step in developing this type of resilience assessment, we chose not to establish specific criteria for evaluating resilience levels and instead provided a qualitative, verbal assessment. Aside from the resilience assessment itself, we identified key predictors and co-drivers based on our analysis. These findings support companies in enhancing their preparedness for future disturbances by connecting key operations to adaptive and proactive management strategies. Literature suggests additional potential predictors and co-drivers, however, testing their viability requires adapting data-gathering methods and extending observation periods, which poses a challenge due to the limited availability of past operational records of companies.
Future studies of local-level FVC resilience should incorporate additional predictors, such as tree species composition and storage capacity, and examine later stages of the value chain. Moreover, the ORF approach developed within the RESONATE project and first used here in this setting, has the potential for broader global application and can be adapted to assess resilience across different regions and systems. In this sense, future research should test its applicability across scales and explore its integration into other social-ecological systems.
The original contributions presented in this study are included in this article/Supplementary material, further inquiries can be directed to the corresponding author.
SG-J: Data curation, Formal analysis, Investigation, Methodology, Software, Visualization, Writing – original draft, Writing – review & editing. MJ: Conceptualization, Data curation, Formal analysis, Methodology, Supervision, Visualization, Writing – original draft, Writing – review & editing. AH: Methodology, Investigation, Visualization, Writing – original draft, Writing – review & editing. ML: Project administration, Supervision, Validation, Writing – review & editing. SU: Methodology, Visualization, Writing – review & editing. TS: Conceptualization, Writing – original draft. ON: Data curation, Methodology, Writing – original draft. DV: Data curation, Writing – review & editing. HM: Data curation, Writing – review & editing. JP: Data curation, Writing – review & editing. MP: Data curation, Writing – review & editing. LB: Data curation, Writing – review & editing. FL: Conceptualization, Formal analysis, Methodology, Supervision, Visualization, Writing – review & editing, Writing – original draft.
The research was supported by the RESONATE Project funded by the European Union’s Horizon 2020 Research and Innovation Program under grant agreement number 101000574. This work was supported by the Internal Grant Agency (IGA) of the Faculty of Forestry and Wood Sciences of the Czech University of Life Sciences in Prague No. A_07_23.
The authors declare that the research was conducted in the absence of any commercial or financial relationships that could be construed as a potential conflict of interest.
All claims expressed in this article are solely those of the authors and do not necessarily represent those of their affiliated organizations, or those of the publisher, the editors and the reviewers. Any product that may be evaluated in this article, or claim that may be made by its manufacturer, is not guaranteed or endorsed by the publisher.
The Supplementary Material for this article can be found online at: https://www.frontiersin.org/articles/10.3389/ffgc.2024.1461932/full#supplementary-material
Asada, R., Hurmekoski, E., Hoeben, A. D., Patacca, M., Stern, T., and Toppinen, A. (2023). Resilient forest-based value chains? Econometric analysis of roundwood prices in five European countries in the era of natural disturbances. For. Policy Econ. 153:102975. doi: 10.1016/j.forpol.2023.102975
Badini, O. S., Hajjar, R., and Kozak, R. (2018). Critical success factors for small and medium forest enterprises: a review. For. Policy Econ. 94, 35–45. doi: 10.1016/j.forpol.2018.06.005
Bauhus, J., Forrester, D. I., Gardiner, B., Jactel, H., Vallejo, R., and Pretzsch, H. (2017). “Ecological stability of mixed-species forests,” in Mixed-Species Forests: Ecology and Management, eds H. Pretzsch, D. Forrester, and J. Bauhus (Berlin: Springer), 337–382. doi: 10.1007/978-3-662-54553-9_7
Baynes, J., Herbohn, J., Smith, C., Fisher, R., and Bray, D. (2015). Key factors which influence the success of community forestry in developing countries. Glob. Environ. Change 35, 226–238. doi: 10.1016/j.gloenvcha.2015.09.011
Biggs, R., Schlüter, M., and Schoon, M. L. (eds) (2015). “An introduction to the resilience approach and principles to sustain ecosystem services in social–ecological systems,” in Principles for Building Resilience: Sustaining Ecosystem Services in Social-Ecological Systems, (Cambridge: Cambridge University Press), 1–31.
Braatz, S. (2012). Building resilience for adaptation to climate change through sustainable forest management. Build. Resilience Adapt. Climate Change Agric. Sector 23:117.
Cantarello Elena Jacobsen Jette Lloret Francisco, et al. (2024). Shaping and enhancing resilient forests for a resilient society. Ambio 53, 1095−1108. doi: 10.1007/s13280-024-02006-7
D’Amours, S., Ouhimmou, M., Audy, J., and Feng, Y. (2017). Forest Value Chain Optimization and Sustainability. Boca Raton, FL: CRC Press.
De Rigo, D., Libertà, G., Durrant, T. H., Vivancos, T. A., and San-Miguel-Ayanz, J. (2017). Forest Fire Danger Extremes in Europe Under Climate Change: Variability and Uncertainty (Doctoral Dissertation). Luxembourg: Publications Office of the European Union.
Dvoøák, J., Löwe, R., Natov, P., Jankovskı, M., and Sedmíková, M. (2020). Unrecorded volume of Norway spruce timber in cut-to-length harvesting. Scand. J. For. Res. 35, 383–393. doi: 10.1080/02827581.2020.1815829
Dzian, M., Paluš, H., and Parobek, J. (2019). “Global trends affecting the EU timber trade,” in Proceedings of the 12th WoodEMA Annual International Scientific Conference on Digitalisation and Circular Economy: Forestry and Forestry Based Industry Implications. Varna, 297.
Ficko, A., Lidestav, G., Dhubháin, ÁN., Karppinen, H., Zivojinovic, I., and Westin, K. (2019). European private forest owner typologies: a review of methods and use. For. Policy Econ. 99, 21–31. doi: 10.1016/j.forpol.2017.09.010
Fuchs, J., Bodelschwingh, H., Lange, A., Paul, C., and Husmann, K. (2022). Quantifying the consequences of disturbances on wood revenues with impulse response functions. For. Policy Econ. 140:102738. doi: 10.1016/j.forpol.2022.102738
Gower, J. C., Lubbe, S. G., and Le Roux, N. J. (2011). Understanding Biplots. Hoboken, NJ: John Wiley and Sons.
Greenwood, L. F., Coyle, D. R., Guerrero, M. E., Hernández, G., MacQuarrie, C. J., Trejo, O., et al. (2023). Exploring pest mitigation research and management associated with the global wood packaging supply chain: what and where are the weak links? Biol. Invas. 25, 2395–2421. doi: 10.1007/s10530-023-03058-8
Gunnarsson, H., Rönnqvist, M., and Lundgren, J. T. (2004). Supply chain modelling of forest fuel. Eur. J. Operat. Res. 158, 103−123. doi: 10.1016/S0377-2217(03)00354-0
Haeler, E., Bolte, A., Buchacher, R., Hänninen, H., Jandl, R., Juutinen, A., et al. (2023). Forest subsidy distribution in five European countries. For. Policy Econ. 146:102882. doi: 10.1016/j.forpol.2022.102882
Hajjar, R., Kozak, R. A., El-Lakany, H., and Innes, J. L. (2013). Community forests for forest communities: integrating community-defined goals and practices in the design of forestry initiatives. Land Use Policy 34, 158−167. doi: 10.1016/j.landusepol.2013.03.002
Hlásny, T., Krokene, P., Liebhold, A., Montagné-Huck, C., Müller, J., Qin, H., et al. (2019). Living with Bark Beetles: Impacts, Outlook and Management Options (No. 8). Joensuu: European Forest Institute.
Hlásny, T., Zimová, S., Merganičová, K., Štìpánek, P., Modlinger, R., and Turčáni, M. (2021). Devastating outbreak of bark beetles in the Czech Republic: drivers, impacts, and management implications. For. Ecol. Manag. 490:119075. doi: 10.1016/j.foreco.2021.119075
Hoeben, A. D., Stern, T., and Lloret, F. (2023). A review of potential innovation pathways to enhance resilience in wood-based value chains. Curr. For. Rep. 9, 301–318. doi: 10.1007/s40725-023-00191-4
Holling, C. S. (1996). “Engineering resilience versus ecological resilience,” in Engineering within Ecological Constraints, ed. P. E. Schulze , (Washington, DC: National Academy Press), 31–43.
Hörl, J., Keller, K., and Yousefpour, R. (2020). Reviewing the performance of adaptive forest management strategies with robustness analysis. For. Policy Econ. 119:102289. doi: 10.1016/j.forpol.2020.102289
Hurmekoski, E., Ragnar, J., Korhonen, J., Jänis, J., Mäkinen, M., and Hetemäki, L. (2018). Diversification of the forest industries: role of new wood-based products. Can. J. For. Res. 48, 1417−1432. doi: 10.1139/cjfr-2018-0116
Instituto Galego de Estatística (2021). Análise da Cadea Forestal-Madeira. Xunta de Galicia. Santiago de Compostela: Instituto Galego de Estatística.
Jactel, H., Bauhus, J., Boberg, J., Bonal, D., Castagneyrol, B., Gardiner, B., et al. (2017). Tree diversity drives forest stand resistance to natural disturbances. Curr. For. Rep. 3, 223–243. doi: 10.1007/s40725-017-0064-1
Johnstone, J. F., Allen, C. D., Franklin, J. F., Frelich, L. E., Harvey, B. J., Higuera, P. E., et al. (2016). Changing disturbance regimes, ecological memory, and forest resilience. Front. Ecol. Environ. 14:369–378. doi: 10.1002/fee.1311
Juutinen, A., Haeler, E., Jandl, R., Kuhlmey, K., Kurttila, M., Mäkipää, R., et al. (2022). Common preferences of European small-scale forest owners towards contract-based management. For. Policy Econ. 144:102839. doi: 10.1016/j.forpol.2022.102839
Kaplinsky, R., and Morris, M. (2000). A Handbook for Value Chain Research, Vol. 113. Brighton: University of Sussex, Institute of Development Studies.
Kärhä, K., Anttonen, T., Poikela, A., Palander, T., Laurén, A., Peltola, H., et al. (2018). Evaluation of salvage logging productivity and costs in windthrown Norway spruce-dominated forests. Forests 9:280. doi: 10.3390/f9050280
Knoke, T., Messerer, K., and Paul, C. (2017). The role of economic diversification in forest ecosystem management. Curr. Forestry Rep. 3, 93−106. doi: 10.1007/s40725-017-0054-3
Kovalčík, M. (2011). Profitability and competitiveness of forestry in European countries. J. For. Sci. 57, 369–376. doi: 10.17221/138/2010-JFS
Lawrence, A. (2020). Diversifying Conifers in Productive Forests: Stakeholders’ Perspectives. Edinburgh: Edinburgh Climate Change Institute.
Lindner, M., Nikinmaa, L., Brang, P., Cantarello, E., and Seidl, R. (2020). Enhancing Resilience to Address Challenges in Forest Management, eds F. Krumm, A. Schuck, and A. Rigling (Birmensdorf: European Forest Institute (EFI); Swiss Federal Institute for Forest, Snow and Landscape Research (WSL)), 147−155.
Liubachyna, A., Bubbico, A., Secco, L., and Pettenella, D. (2017). Management goals and performance: clustering state forest management organizations in Europe with multivariate statistics. Forests 8:504. doi: 10.3390/f8120504
Lloret, F., Hurtado, P., Espelta, J. M., Jaime, L., Nikinmaa, L., Lindner, M., et al. (2024). ORF, an operational framework to measure resilience in social–ecological systems: the forest case study. Sustain. Sci. 19, 1579−1593. doi: 10.1007/s11625-024-01518-1
Loeffler, D., and Anderson, N. (2018). Impacts of the mountain pine beetle on sawmill operations, costs, and product values in Montana. For. Prod. J. 68, 15–24.
López-Rodríguez, G., Rodríguez-Vicente, V., and Marey-Pérez, M. F. (2021). Study of forest productivity in the occurrence of forest fires in Galicia (Spain). Sustainability 13:8472. doi: 10.3390/su13158472
Luke (2021). Statistics database: Finish Natural Resources Institute (Luke). Available online at: https://statdb.luke.fi/PXWeb/pxweb/en/LUKE/LUKE__04%20Metsa__08%20Muut__Metsateollisuus/?tablelist=true&rxid=001bc7da-70f4-47c4-a6c2-c9100d8b50db (accessed August 31, 2023).
Macqueen, D. (2010). Building Profitable and Sustainable Community Forest Enterprises: Enabling Conditions. Edinburgh: International Institute for Environment and Development.
Mazziotta, A., Borges, P., Kangas, A., Halme, P., and Eyvindson, K. (2023). Spatial trade-offs between ecological and economical sustainability in the boreal production forest. J. Environ. Manag. 330:117144. doi: 10.1016/j.jenvman.2022.117144
Mederski, P. S., Schweier, J., Ðuka, A., Tsioras, P., Bont, L. G., and Bembenek, M. (2022). Mechanised harvesting of broadleaved tree species in Europe. Curr. For. Rep. 8, 1−19. doi: 10.1007/s40725-021-00154-7
Messier, C., Bauhus, J., Doyon, F., Maure, F., Sousa-Silva, R., Nolet, P., et al. (2019). The functional complex network approach to foster forest resilience to global changes. For. Ecosyst. 6:21. doi: 10.1186/s40663-019-0166-2
Messier, C., Bauhus, J., Sousa-Silva, R., Auge, H., Baeten, L., Barsoum, N., et al. (2022). For the sake of resilience and multifunctionality, let’s diversify planted forests! Conserv. Lett. 15:e12829. doi: 10.1111/conl.12829
Molnar, A., Liddle, M., Bracer, C., Khare, A., White, A., and Bull, J. (2007). Community-Based Forest Enterprises in Tropical Forest Countries: Status and Potential. Washington, DC: RRI.
Motik, D., Posavec, S., and Vuletić, D. (2005). Croatia. Acta Silvatica Lignaria Hungarica 1(Spec.). 103−143.
Muñoz-Gálvez, F. J., Herrero, A., Esther Pérez-Corona, M., and Andivia, E. (2021). Are pine-oak mixed stands in Mediterranean mountains more resilient to drought than their monospecific counterparts? For. Ecol. Manag. 484:118955. doi: 10.1016/J.FORECO.2021.118955
MZe (2021). Zpráva o Stavu lesa a Lesního Hospodáøství české Republiky v Roce 2020. Praha: Ministerstvo zemìdìlství.
Nikinmaa, L., de Koning, J. H. C., Derks, J., Grabska-Szwagrzyk, E., Konczal, A. A., Lindner, M., et al. (2024). The priorities in managing forest disturbances to enhance forest resilience: a comparison of a literature analysis and perceptions of forest professionals. For. Policy Econ. 158:103119. doi: 10.1016/j.forpol.2023.103119
Nikinmaa, L., Lindner, M., Cantarello, E., Gardiner, B., Jacobsen, J. B., Jump, A. S., et al. (2023). A balancing act: principles, criteria and indicator framework to operationalize social-ecological resilience of forests. J. Environ. Manag. 331:117039. doi: 10.1016/j.jenvman.2022.117039
Nikinmaa, L., Lindner, M., Cantarello, E., Jump, A. S., Seidl, R., Winkel, G., et al. (2020). Reviewing the use of resilience concepts in forest sciences. Curr. For. Rep. 6, 61–80. doi: 10.1007/s40725-020-00110-x
Patacca, M., Lindner, M., Lucas-Borja, M. E., Cordonnier, T., Fidej, G., Gardiner, B., et al. (2023). Significant increase in natural disturbance impacts on European forests since 1950. Glob. Change Biol. 29, 1359−1376. doi: 10.1111/gcb.16531
Pettenella, D., and Maso, D. (2011). Networks of Small-Medium Enterprises Operating in Forestry: Some Theoretical Concepts and Empirical Evidence. Innovation in Forestry: Territorial and Value Chain Relationship. Wallingford: CABI, 35–47.
Picos, J. (2023). A Cadea Forestal -Madeira de Galicia*. Axencia Galega da Industria Forestal. Consellerí a do Medio Rural. Vigo: Universidade de Vigo. 68. doi: 10.13140/RG.2.2.31367.16801
Pingoud, K., and Wagner, F. (2006). Methane emissions from landfills and carbon dynamics of harvested wood products: the first-order decay revisited. Mitig. Adapt. Strat. Glob. Change 11, 961–978. doi: 10.1007/s11027-006-9029-6
Pischedda, D. (2004). Technical Guide on Harvesting and Conservation of Storm Damaged Timber. Paris: CTBA.
Porter, M. (1985). Competitive Advantage: Creating and Sustaining Superior Performance: With a New Introduction. New York, NY: Free Press.
Posavec, S., Keča, L., Delić, S., Stojanovska, M., and Malovrh, ŠP. (2021). Comparative analysis of selected business indicators of state forest companies. J. For. Soc. Croatia 145:12.
Prestemon, J. P., and Wear, D. N. (1999). “Inventory effects on aggregate timber supply,” in SOFEW ’98, Proceedings of the 1998 Southern Forest Economics Workshop, March 25–27, 1999, Williamsburg, Virginia, eds K. L. Abt and R. C. Abt (Williamsburg), 26–32.
Pretzsch, H., Poschenrieder, W., Uhl, E., Brazaitis, G., Makrickiene, E., and Calama, R. (2021). Silvicultural prescriptions for mixed-species forest stands. A European review and perspective. Eur. J. For. Res. 140, 1267−1294. doi: 10.1007/s10342-021-01388-7
Pukkala, T. (2018). Effect of species composition on ecosystem services in European boreal forest. J. For. Res. 29, 261–272. doi: 10.1007/s11676-017-0576-3
Riccioli, F., Fratini, R., Marone, E., Fagarazzi, C., Calderisi, M., and Brunialti, G. (2020). Indicators of sustainable forest management to evaluate the socio-economic functions of coppice in Tuscany, Italy. Soc. Econ. Plann. Sci. 70:100732. doi: 10.1016/j.seps.2019.100732
Romeiro, J. M. N., Eid, T., Antón-Fernández, C., Kangas, A., and Trømborg, E. (2022). Natural disturbances risks in European Boreal and Temperate forests and their links to climate change–A review of modelling approaches. For. Ecol. Manag. 509:120071. doi: 10.1016/j.foreco.2022.120071
Rueda, G. (2022). Regional case studies & European scale analysis. Modified to apply to the 5 Case studies in this research. Available at: https://resonateforest.org
Sanginés de Cárcer, P., Mederski, P. S., Magagnotti, N., Spinelli, R., Engler, B., Seidl, R., et al. (2021). The management response to wind disturbances in European forests. Curr. For. Rep. 7, 167−180.
Saramä,ki, T. (2012). The Profitability of Forestry in Finland and Russia. LUKE. Available online at: http://urn.fi/URN:ISBN:978-951-40-2401-6 (accessed September 6, 2023).
Scheffer, M., Carpenter, S. R., Dakos, V., and van Nes, E. H. (2015). Generic indicators of ecological resilience: inferring the chance of a critical transition. Annu. Rev. Ecol. Evol. Syst. 46, 145−167. doi: 10.1146/annurev-ecolsys-112414-054242
Schuldt, B., Buras, A., Arend, M., Vitasse, Y., Beierkuhnlein, C., Damm, A., et al. (2020). A first assessment of the impact of the extreme 2018 summer drought on Central European forests. Basic Appl. Ecol. 45, 86−103. doi: 10.1016/j.baae.2020.04.003
Seidl, R., and Senf, C. (2024). Changes in planned and unplanned canopy openings are linked in Europe’s forests. Nat. Commun. 15:4741. doi: 10.1038/s41467-024-49116-0
Seidl, R., Thom, D., Kautz, M., Martin-Benito, D., Peltoniemi, M., Vacchiano, G., et al. (2017). Forest disturbances under climate change. Nat. Clim. Change 7, 395–402. doi: 10.1038/nclimate3303
Sommerfeld, A., Rammer, W., Heurich, M., Hilmers, T., Müller, J., and Seidl, R. (2021). Do bark beetle outbreaks amplify or dampen future bark beetle disturbances in Central Europe? J. Ecol. 109, 737–749. doi: 10.1111/1365-2745.13502
Steckel, M., del Río, M., Heym, M., Aldea, J., Bielak, K., Brazaitis, G., et al. (2020). Species mixing reduces drought susceptibility of Scots pine (Pinus sylvestris L.) and oak (Quercus robur L., Quercus petraea (Matt.) Liebl.)–Site water supply and fertility modify the mixing effect. For. Ecol. Manag. 461:117908. doi: 10.1016/j.foreco.2020.117908
Thom, D., Rammer, W., and Seidl, R. (2017). Disturbances catalyze the adaptation of forest ecosystems to changing climate conditions. Glob. Change Biol. 23, 269–282. doi: 10.1111/gcb.13506
Tian, N., Poudyal, N., Auge, R., Hodges, D., and Young, T. (2017). Meta-analysis of price responsiveness of timber supply. For. Prod. Soc. 67, 152−163. doi: 10.13073/FPJ-D-16-00017
Toivonen, R., Toppinen, A., and Tilli, T. (2002). Integration of roundwood markets in Austria, Finland and Sweden. For. Policy Econ. 4, 33–42. doi: 10.1016/S1389-9341(01)00071-5
Toppinen, A., and Kuuluvainen, J. (2010). Forest sector modelling in Europe—the state of the art and future research directions. For. Policy Econ. 12, 2–8.
Udali, A., Garollo, L., Cavalli, R., and Grigolato, S. (2022). “Quantity and type of logging residues following cut-to-length and full-tree salvage logging systems in damaged mountain forests,” in Proceedings of the Conference of the Italian Society of Agricultural Engineering, (Cham: Springer International Publishing), 967–977. doi: 10.1007/978-3-031-30329-6_100
Vuletić, D., Kauzlarić, Ž, Balenović, I., and Krajter Ostoić, S. (2014). Assessment of forest damage in Croatia caused by natural hazards in 2014. South East Eur. For. 5, 65–79. doi: 10.15177/seefor.14-07
Keywords: disturbances, timber supply, social-ecological systems, forest management, thresholds, wood production
Citation: García-Jácome SP, Jankovský M, Hoeben AD, Lindner M, Uzquiano S, Stern T, Nuhlíček O, Vuletić D, Marjanović H, Picos J, Peltoniemi M, Baumbach L and Lloret F (2025) Forest value chain resilience from a local perspective in five European countries: analysis of predictors and co-drivers. Front. For. Glob. Change 7:1461932. doi: 10.3389/ffgc.2024.1461932
Received: 09 July 2024; Accepted: 19 December 2024;
Published: 07 January 2025.
Edited by:
Ari Mikko Hietala, Norwegian Institute of Bioeconomy Research (NIBIO), NorwayReviewed by:
Pablo Torres-Lima, Metropolitan Autonomous University, MexicoCopyright © 2025 García-Jácome, Jankovský, Hoeben, Lindner, Uzquiano, Stern, Nuhlíček, Vuletić, Marjanović, Picos, Peltoniemi, Baumbach and Lloret. This is an open-access article distributed under the terms of the Creative Commons Attribution License (CC BY). The use, distribution or reproduction in other forums is permitted, provided the original author(s) and the copyright owner(s) are credited and that the original publication in this journal is cited, in accordance with accepted academic practice. No use, distribution or reproduction is permitted which does not comply with these terms.
*Correspondence: Sandra P. García-Jácome, Z2FyY2lhX2phY29tZUBmbGQuY3p1LmN6
Disclaimer: All claims expressed in this article are solely those of the authors and do not necessarily represent those of their affiliated organizations, or those of the publisher, the editors and the reviewers. Any product that may be evaluated in this article or claim that may be made by its manufacturer is not guaranteed or endorsed by the publisher.
Research integrity at Frontiers
Learn more about the work of our research integrity team to safeguard the quality of each article we publish.