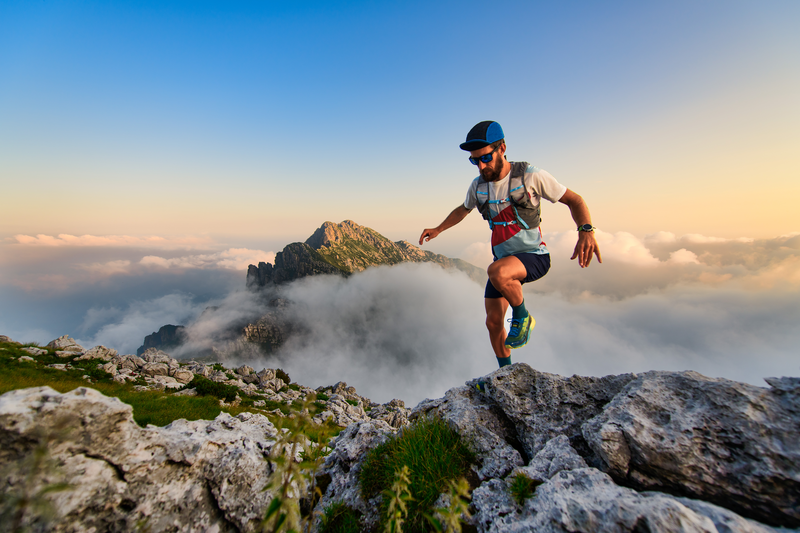
95% of researchers rate our articles as excellent or good
Learn more about the work of our research integrity team to safeguard the quality of each article we publish.
Find out more
REVIEW article
Front. For. Glob. Change , 23 August 2024
Sec. Forest Soils
Volume 7 - 2024 | https://doi.org/10.3389/ffgc.2024.1453615
This article is part of the Research Topic Understanding the Relationship between Extreme Climate Events and Forest Soil Hydrology: Implications for Ecosystem Functions View all 6 articles
The stability of soil organic carbon (SOC) is crucial for the global carbon cycle and serves as a key indicator of soil quality and eco-system function. In Karst areas, SOC stability is influenced by unique geological conditions. Studying SOC stability in these regions is significant for understanding regional ecosystem functions and the role of SOC in mitigating global climate change. This review examines the fractions and stability of SOC in Karst areas, analyzing the impacts of key factors such as rocky desertification, land use changes, and vegetation restoration on SOC stability, along with their underlying mechanisms. It also discusses the current challenges and opportunities in SOC stability. This review aims to guide the development of effective ecological rehabilitation and management strategies for degraded ecosystems in Karst areas.
The ability of soil organic carbon (SOC) to resist disturbance by external factors and to recover to its original level under current conditions is referred to as SOC stability (Zhang et al., 2019). The stability of SOC depends on organic carbon fractions as well as internal and external factors. Given that SOC stability significantly affects SOC storage, it also influences the entire SOC cycle (Yang et al., 2020). Internal factors influencing SOC stability include the molecular structure, types, and relative proportions of organic matter, while external factors encompass soil aggregate formation and fragmentation, organic matter adsorption, soil biological activity and biodegradation, and hydrothermal conditions (Xu et al., 2018). Different fractions of organic carbon arise from various sources and respond to influencing factors through distinct underlying mechanisms. Therefore, the processes driving the decomposition and transformation of these carbon fractions collectively impact SOC stability.
The study of SOC stability is important for understanding the role of the SOC pool in ameliorating global climate change. Consequently, the research focus on SOC stability has increased with increasing attention on global climate change and the associated environmental issues (Li et al., 2024; Xu et al., 2024). The unique geology of Karst areas, characterized by shallow and discontinuous soil layers and significant heterogeneity, has contributed to the diversity of Karst ecosystems and habitat types (Peng and Dai, 2022). The intricate cave systems, fractured rock formations, and thin soil layers in Karst areas can serve as both sinks and sources of organic carbon. Understanding the characteristics of SOC stability within these geological contexts is essential for predicting the response of Karst ecosystems to climate change and for managing carbon sequestration in these sensitive environments. Enhancing the stability of SOC in Karst areas through scientific management and regulatory measures has become a significant research focus and challenge. Therefore, thisreview explores recent advances in SOC stability studies in Karst areas, emphasizing the factors regulating SOC stability and the underlying mechanisms. This review aims to guide the rational utilization of land resources and promote ecosystem restoration and conservation in Karst areas.
Since the mechanisms regulating SOC stability play a central role in the soil carbon cycle, they are also crucial for maintaining soil ecological balance and regulating the Earth’s climate. Various physical, chemical, and biological processes influence SOC stability.
Organic matter in soil is essential for soil aggregate formation, and the stability of these aggregates plays a key role in the physical regulation of SOC stability. The formation of aggregates creates spatial separation between soil microorganisms, enzymes, and organic carbon, thereby slowing down SOC decomposition (Jastrow, 1996; Six et al., 2004). Soil aggregates of different sizes have different effects on SOC stability (Kunmala et al., 2023). Large soil aggregates, specifically, facilitate the infiltration of new organic matter into aggregates (Bhattacharyya et al., 2021), However, this organic carbon can decompose readily because large soil aggregates provide favorable conditions for microbial survival and activity. Consequently, the rate of organic carbon decomposition in large aggregates significantly exceeds that in micro-aggregates (Bimüller et al., 2016). Limited air in soil micro-aggregates restricts soil microbial activity, leading to a higher prevalence of long-term deposition of SOC in these micro-aggregates (Yu et al., 2024).
The stability of SOC is enhanced through the interaction between soil mineral particles and organic matter, which form organo-mineral complexes (Mastro et al., 2020). This process represents one of the main mechanisms regulating soil carbon sequestration. The cementation of organic carbon with clay silt decreases its decomposition rate, leading to SOC accumulation. Strong bonds occur between soil clay silt and specific organic acids, with the strength of these bonds regulated by mineral type, total electric charges, soil pH, and covalent bonding. The mechanisms by which SOC bonds to clay-silt minerals can be broadly categorized into two types: (1) cation adsorption to the clay layer and interlayer mineral sources; and (2) ion adsorption to the outer surface (Tournassat et al., 2004). Tisdall and Oades (1982) demonstrated that carbon from complexes formed through microbial degradation products, aromatic humus, amorphous iron-aluminum compounds, and aluminosilicates in clay accounts for 52–98% of soil carbon. The interaction between minerals, such as kaolinite and metal oxides, and organic carbon is particularly significant in soils with low organic matter (Li et al., 2023c). Han et al. (2016) proposed that the stability of organic carbon is enhanced through chemical bonding to amorphous oxides, due to the large specific surface area and high number of hydroxyl sites present on these oxides. Consequently, the influence of chemical processes on SOC stability is crucial for the sequestration of organic carbon in soil.
Biochemical factors contributing to SOC stability include the non-degradability of certain organic matter and the contribution of soil organisms to SOC stability. Complex, stabilized polymers formed during litter decomposition are resistant to microbial decomposition, and non-degradable fractions of litter, such as lignin, tannins, polyphenols, and waxes, can persist in the soil for long periods (Thevenot et al., 2010). Moreover, the storage of organic carbon by soil micro-and macro-organisms and their metabolites constitutes a portion of the SOC pool. These organic compounds, derived from soil organisms and difficult to degrade, gradually accumulate in the soil, forming an important part of the stability organic carbon (Lu et al., 2021). During the initial stages of litter decomposition, 68% of non-structural soluble organic matter interacts with microorganisms to form complex and stabilized SOC (Cotrufo et al., 2015). Nevertheless, previous studies have indicated that the biochemical stability of SOC is primarily observed in surface soil during the initial stages of organic carbon decomposition. In contrast, the chemical and physical processes contributing to SOC stability, as previously mentioned, play a dominant role in the later stages of organic carbon decomposition and deeper soil layers (Lützow et al., 2006; Rovira and Vallejo, 2007).
The pool of soil carbon reflects the balance between organic matter input and carbon loss (Mayer et al., 2020). The stability of SOC directly impacts soil carbon sequestration, and SOC fractions are typically used to quantitatively characterize the stability of SOC (Zhang et al., 2022). Carbon fractions are typically classified based on the three mechanisms regulating the persistence of organic carbon in soil, namely physical, chemical, and biological processes (Audette et al., 2021).
Physical processes include categorization by particle size, density, and aggregates, which act to preserve the chemical structure of SOC (Post and Kwon, 2000). Therefore, separated organic carbon fractions can reflect the structure, properties, and function of the original organic carbon, as well as its turnover characteristics. Particle size categorization is based on differences in surface areas and mineral compositions among soil particles of varying sizes, which in turn lead to differences in organic carbon content, composition, and chemical properties (Ma et al., 2024a). The quantity of organic carbon in silt and clay is positively related to SOC stability. During density categorization, organic carbon is separated into light-fraction organic carbon (LFOC) and heavy-fraction organic carbon (HFOC). LFOC is categorized as free organic matter with higher activity, resulting in a higher decomposition rate and shorter turnover time (Jia et al., 2020). HFOC comprises organic carbon that is combined with soil minerals, primarily humus. Since HFOC decomposes slowly due to the protection provided by soil minerals, it reflects the soil’s ability to retain organic carbon and represents the main organic carbon pool in soil (Guillaume et al., 2022). Aggregate categorization involves separating soil aggregates into different particle sizes using wet sieving. These aggregates play a crucial role in SOC conservation, where large aggregates primarily offer physical protection to organic carbon, while micro-aggregates provide chemical protection by forming organic-mineral complexes (Abrar et al., 2020; Qiao et al., 2023). Six et al. (2002) improved the original organic carbon aggregate separation method by combining density categorization with aggregate categorization. This approach led to the development of a comprehensive system for studying the variation and stability of organic carbon aggregates across different particle sizes.
Chemical categorization separates organic carbon fractions based on chemical stability. This is achieved using water, salt, and acid, relying on differences in hydrolysis, oxidation, and solubility among various chemical fractions. The most studied chemical fractions currently include dissolved organic carbon (DOC), readily oxidizable carbon (ROC), and hydrolyzable organic carbon (HOC) (Liu, 2019). Due to the rapid turnover and high biological activity of DOC (Guo et al., 2023), the content of this organic carbon fraction varies significantly within soil organic carbon. Therefore, studying DOC is crucial for understanding the accumulation and stability of SOC. The characteristics of ROC include high activity, instability, and a short turnover time in soil (Hurisso et al., 2016; He et al., 2024). The typical categorization of HOC into active and inert carbon pools facilitates the prediction of organic carbon mineralization (Zhao et al., 2021).
During biological categorization, SOC is separated into microbial biomass carbon (MBC) and potential mineralizable carbon (PMC). Although MBC constitutes a small proportion of total SOC, microorganisms serve as the driving force for material transformation in soil. Therefore, the metabolism of the microbial community plays an important role in promoting SOC decomposition and nutrient transformation (Das et al., 2023). MBC has a short turnover period and is considered the most active fraction in the SOC pool (Oren et al., 2018). The ratio of MBC to total organic carbon can act as an indicator of dynamic changes in the soil carbon pool (Insam and Domsch, 1988). Mineralization of carbon is associated with the microbial decomposition of organic matter, with the content of mineralizable carbon determined by measuring the released CO2 (Yang et al., 2019c). Since both PMC and MBC are part of the active SOC pool, they serve as indicators of soil microbial activity and soil fertility. Consequently, PMC and MBC are key components of the organic soil carbon cycle (Sainju et al., 2009).
The capacity of soil to store carbon is typically evaluated based on soil mineralogical properties, particularly the availability of mineral surfaces (Kang et al., 2024). Calcium-bound organic carbon and iron-aluminum-bound organic carbon in the soil are considered key pathways for the stabilization of SOC (Xu et al., 1999). An investigation by Yang et al. (2019a) into labile organic carbon, recalcitrant organic carbon, calcium-bound organic carbon, and iron-aluminum-bound organic carbon in soils from different bedrock lithologies revealed that higher contents of clay and calcium oxide in carbonate rocks contribute to stable organic carbon fractions. This stability exceeds that found in soils derived from siliceous rocks. The loss of SOC, mineral-protected organic carbon, and recalcitrant organic carbon fractions occurs concurrently with the evolution of calcareous soils. This can be attributed to the decreased capacity of stabilizing organic carbon due to adsorption of minerals through bridging of multivalent cations (Rasmussen et al., 2018; Yang et al., 2021).
Similarly, clay minerals and oxides, such as Fe2O3, play a key role in the stability of SOC (Eze et al., 2018). One of the main factors contributing to the high capacity of limestone-covered soils to store organic carbon is their elevated content of clay and fine silt (Valjavec et al., 2022). From the perspective of different soil structures and aggregate sizes, the contents of bulk soil organic carbon and aggregate organic carbon in Karst forests significantly exceed those found in other regions. In addition, microbial biomass and activity in micro-aggregates and mineral fractions far exceed those in large aggregates, reflecting higher biochemical stability (Wang et al., 2021). Particulate organic carbon (POC) has a high sensitivity index, effectively reflecting the dynamics of soil organic carbon. It influences the biochemical protection of SOC by impacting changes in the composition of bacteria and fungi in Karst areas (Lan, 2020).
Zhang et al. (2023a) showed that LFOC in calcareous soil is sensitive to vegetation restoration, whereas HFOC represents the most important and stable form of SOC storage. This stability increases with soil depth, consistent with the trend of SOC stability observed in non-Karst areas (Liu et al., 2023; Tang et al., 2023a). Huang et al. (2021b) identified differences in SOC variation patterns among four distinct topographic features of Karst, peak-cluster depression, plateau, canyon, and trough valley. They also found that SOC in Karst areas exceeds that in non-Karst areas. Soil of rocky desertification areas is typically stored in rocky soil surface, stone crevice, stone gully, stone cave, stone pit and soil surface. The highest carbon sequestration was found in soil of stone caves, but its proportion of mineral-associated organic carbon fraction in total organic carbon is lower than that in other Karst microhabitats; In contrast, soil surface showed a lower carbon sequestration capacity and highest proportion of mineral-associated organic carbon fractions (He et al., 2023). Compared to non-Karst areas (Zhao et al., 2023), Karst areas exhibit greater variations in SOC content, and relatively lower stability. This is primarily due to shallow soil layers, erosion, high habitat heterogeneity, and more complex factors influencing SOC stability (He et al., 2021; Lan et al., 2021).
The mechanisms involved in fixing organic carbon, ensuring its stability, and responding to influencing factors differ significantly among the various carbon fractions (Iqbal et al., 2009). Karst areas typically exhibit severe land degradation, with large expanses of exposed bedrock, leading to characteristic rocky desertification landscapes. The degree of rocky desertification can be categorized according to the rate of bedrock exposure and vegetation coverage, namely potential rocky desertification, slight rocky desertification, moderate rocky desertification, and severe rocky desertification (Tang et al., 2023b).
SOC is significantly affected by the degree of rocky desertification, with the greatest limitations on SOC observed under severe rocky desertification (Ma et al., 2020). Nevertheless, a study by Zheng et al. (2022) on the effects of bedrock exposure rate on SOC and its fractions showed that total organic carbon, MBC, and DOC in soil are maximized under severe rocky desertification, whereas LFOC decreased with the increase of bedrock exposure rate. These findings further confirm the diversity of habitats and the complexity of factors influencing SOC stability in Karst areas. Another study showed that a decrease in the degree of rocky desertification results in an increase in the number of aggregates with a diameter of 5–10 mm (Lu et al., 2023). The associated increase of organic carbon of these aggregates the main driver of the increase in total organic carbon.
The soil C:N ratio is less sensitive to the degree of rocky desertification, whereas the ratios of C:P and N:P increase with the degree of rocky desertification (Yang et al., 2019b). Further, both C:P and N:P ratios showed a strong correlation with soil free light organic carbon, occluded-particulate organic carbon, mineral-associated organic carbon and its proportion in total organic carbon. Soil microorganisms in ecosystems with rocky desertification exhibit different sensitivities to and mechanisms of coping with environmental degradation (Cheng et al., 2020). The abundance and diversity of soil bacteria and fungi generally decrease with the increasing degree of rocky desertification degree (Li et al., 2023a). While microorganisms can decompose and utilize some organic carbon, they also facilitate the formation of stable organic carbon (Gao et al., 2023). Therefore, the degree of rocky desertification further affects SOC stability by influencing soil stoichiometric ratios and microbial activity. In addition to altering the structure of soil microbiota, rocky desertification in Karst areas is often accompanied by changes in soil properties. Specifically, variations in Ca2+ and available P in the soil also impact carbon metabolism (Tang et al., 2019). The stability of SOC affected by soil erosion is primarily due to the displacement of soil organic matter (Nie et al., 2019). Overall, rocky desertification influences the fixation mechanisms and stability of different carbon fractions by altering soil microorganisms and physicochemical properties.
Since unsustainable land use is an important driver of rocky desertification, land use type has a significant effect on SOC stability. Factors influencing SOC are dependent on the diversity of geological conditions in Karst areas. For example, SOC in a Karst trough valley area is mainly affected by bedrock exposure, whereas land use is the key factor affecting SOC in a Karst plateau area (Huang et al., 2021b). Physicochemical properties of slope soil in Karst areas exhibit significant spatial differences among various land use types (Chen et al., 2024b), with both physical and chemical properties of soil significantly impacting SOC stability.
The rapid responses of labile organic carbon to changes in the soil environment have contributed to the significant impacts of land use changes on labile organic carbon in Karst areas (Yang et al., 2022). Since the proportion of different resistant organic carbon fractions in total organic carbon is directly or inversely proportional to SOC stability, it serves as a measure of SOC stability (He et al., 2023). A study by Chen et al. (2024a) on SOC stability based on the ratio of recalcitrant organic carbon and total organic carbon, found the lowest SOC stability in cultivated land. The soil pH was identified as a key factor influencing soil stability in Karst areas, as it affects the transformation of organic carbon by altering soil enzyme activity, and the abundance and diversity of microbial communities (Ma et al., 2024b). Paddy fields are regarded as a relatively crucial type of cultivated land for long-term soil carbon sequestration in the southwestern Karst areas. This is due to their higher contents of organic carbon in soil and aggregates compared to dry land and abandoned land, as well as a lower proportion of labile organic carbon to total organic carbon in paddy fields (Liao et al., 2015).
The 13C nuclear magnetic resonance spectroscopy is widely used to assess the composition of soil organic matter. Cui et al. (2023) conducted research on the soil of sugarcane field, corn field, pitaya field, etc., and found that the humic substances in Karst corn field has high stability, mainly due to the higher Alkyl C/O-alkyl C and Hydrophobic C/hydrophilic C ratios. Compared with other land use types such as grazing secondary forest, farmland often exhibits lower comprehensive quality of soil organic matter, but abandoned farmland has the highest ratio of Alkyl C/O-alkyl C (Chang et al., 2018).
A study by Yu et al. (2023) demonstrated that land use can significantly change the distribution of aggregates of different sizes, thus affecting the stability and contents of organic carbon aggregates. The conversion of farmland to bamboo forest can improve the capacity of soil to sequester carbon and resist soil erosion. While an increase in soil aggregate stability can effectively increase the stability and carbon sequestration capacity of SOC (Chen et al., 2022), organic carbon fractions may directly or indirectly affect aggregate stability. Wei et al. (2022) showed that woodland type has a considerable effect on the stability of soil aggregates, with POC being the main organic carbon fraction regulating the porosity and stability of aggregates. Similarly, Wen et al. (2020) confirmed that changes in organic carbon in soil aggregates affect their formation and stability during organic fertilizer application. A study of forest management interventions in Guangxi has shown that, despite having no significant impact on soil mineral associated organic carbon, forest management significantly reduces the content of particulate organic carbon (Li et al., 2023b), which is detrimental to the sequestration of soil carbon in forest ecosystems. The formation of persistent particulate organic matter and mineral-associated organic matter in the SOC pool is directly driven by the decomposition of particulate organic matter, with microbial activity serving as the governing factor (Witzgall et al., 2021). Regardless, the establishment of sustainable land use planning and the strengthening of scientific management are particularly crucial for Karst areas.
Ecological rehabilitation is regarded as a priority in the management of Karst areas, with vegetation restoration being particularly important due to its considerable influence on SOC stability. Indeed, the restoration of vegetation can lead to significant increases in SOC accumulation and the contents of MBC, ROC, and DOC, as well as their proportions in total organic carbon. Restored grassland has lower SOC compared to restored forests, shrubland, and vines, and the proportion of unstable organic carbon in restored grassland is higher (Wang et al., 2023), indicating the limitations of grassland rehabilitation for stabilizing SOC.
Vegetation type has a greater influence on labile organic carbon compared to total organic carbon and recalcitrant organic carbon (Song et al., 2022). An investigation by Bai et al. (2021) on different vegetation restoration measures for ecosystems with rocky desertification showed that total organic carbon, DOC, ROC, POC, and LFOC were maximized in Sabina chinensis plantations and under natural restoration, with POC being the dominant organic carbon fraction in soil. Lan et al. (2021) also found that POC has the highest sensitivity to vegetation restoration measures during the rehabilitation of Karst areas with rocky desertification, with its content dynamically reflecting trends in total SOC. Furthermore, cypress forests can significantly increase the proportion of recalcitrant organic carbon in the SOC pool over the long term (Zhang et al., 2023b).
Forests significantly increase aggregate stability and organic carbon fractions in soil (Lan et al., 2021). However, regeneration of natural vegetation is more effective in promoting the deposition of SOC in degraded ecosystems in Karst areas. Specifically, natural vegetation regeneration helps in sequestering unstable organic carbon in soil and maintaining microbiota stability (Cheng et al., 2023). Approximately 40% ~ 70% of the total organic carbon exists in the colloidal size fraction (Andersson et al., 2023). Vegetation restoration in Karst trough valley areas can increase the soil colloid content of particles larger than 2000 nm, where organic carbon is primarily distributed. In comparison to plantations, natural forests mainly enhance the content of organic carbon in soil colloids with particle sizes larger than 2000 nm (Zhang, 2023). Dissolved organic matter (DOM) serves as the material source for the physical protection mechanism of soil organic carbon. The activity of certain soil microorganisms, such as saprotrophic fungi, can significantly influence the colloidal properties of DOM (Gentile et al., 2024).
Vegetation succession acts as a key temporal factor impacting changes in SOC (Zhang et al., 2014). Zhang et al. (2023a) found that during succession in restored vegetation, both organic carbon and its fractions (LFOC and HFOC) increased. However, the proportion of LFOC in total organic carbon increased with succession, while the proportion of HFOC decreased. The formation of stable organic carbon, a gradual process, is the primary mechanism for long-term SOC storage.
The stability of SOC is mainly demonstrated by its resistance to decomposition, which is influenced by the proportions of different functional groups within the organic carbon (Huang et al., 2021a). The relative abundance of alkyl C increases (Hu et al., 2022) during vegetation restoration, with SOC stability proportional to the proportion of alkyl C. Aromatic C shows weak decomposability (Hall et al., 2020), whereas DOC in the soil is more easily lost in rocky desertification areas characterized by weak aromaticity and low humification (Wang et al., 2022). In addition, Hu et al. (2022) showed that the dominant functional groups during vegetation restoration are involved in the soil C cycle and play a key role in increasing the storage of stable SOC. Therefore, one of the mechanisms to enhance the stability of organic carbon is to alter the distribution of carbon functional groups in soil organic matter (Audette et al., 2021). Developing reasonable vegetation restoration plans and implementing scientific vegetation restoration techniques are key measures to enhance the stability of soil organic carbon in Karst areas (Figure 1).
This review highlights the extensive research on SOC stability in Karst areas and influencing factors. To effectively enhance SOC stability and promote the restoration and sustainable development of ecosystems, it is essential to improve vegetation restoration projects, optimize land use patterns, and intensify scientific research and policy support. However, several aspects of SOC stability in Karst areas necessitate further investigation due to the complexity of SOC stability and the unique geological conditions. These include:
(1) Previous studies on physical, chemical, and biological methods to classify different fractions of organic carbon, indicate that no single method can comprehensively characterize trends in SOC stability or the underlying mechanisms. This is because of the high complexity of decomposition and conversion of organic carbon and the diversity of influencing factors. Therefore, integrating physical, chemical, and biological categorization is the most effective way to reveal the dynamics of the soil carbon pool. This approach requires continuous exploration of and improvements to novel organic carbon fraction classification methods.
(2) There are ongoing controversies regarding the protective mechanisms of soil organic carbon, necessitating further exploration and verification. Specifically, the interactions among these mechanisms and their contributions to the long-term storage of soil organic carbon in Karst areas are not yet well understood. Future studies should focus on the relationships between these stabilizing mechanisms and their relative importance across different soil types and environmental conditions. This focus will help develop more targeted strategies to enhance SOC stability.
(3) While soil erosion in areas with rocky desertification remains of concern, the high SOC contents in these areas suggest that erosion may not solely reduce the SOC pool. Future studies should investigate the evolution of SOC stability in both erosion and deposition zones at the watershed scale. Moreover, efforts should be made to understand the mechanisms responsible for organic carbon stabilization during soil erosion.
(4) The stability of SOC is crucial for soil quality, fertility, and pollutant degradation. Research on the interaction between SOC stability and pollutants, using methods like stable isotope techniques, pollutant adsorption–desorption experiments, and molecular biological methods, can offer new perspectives and strategies for soil pollution remediation and management. These approaches can enhance our understanding of how SOC stability influences pollutant behavior and persistence in soils, thereby contributing to more effective environmental management practices.
(5) Unsustainable farming practices significantly contribute to rocky desertification, highlighting the need to investigate the effects of control measures, management strategies, and the duration of management on SOC stability. The findings of these studies should translate into concrete policies and practical actions for soil management, land use planning, and ecological rehabilitation to ensure the stability and sustainable development of Karst ecosystems.
(6) Past studies have primarily focused on small temporal and spatial scales, which limits a comprehensive understanding of SOC stability in Karst areas. Future studies on SOC stability in Karst areas should be conducted at larger spatial and temporal scales by establishing time-series databases and utilizing remote sensing technologies. This approach will help reveal the long-term changes and spatial distribution patterns of SOC stability in Karst areas.
JH: Writing – original draft, Conceptualization. XL: Writing – review & editing, Conceptualization. WM: Writing – review & editing, Investigation. XC: Writing – review & editing, Investigation.
The author(s) declare that financial support was received for the research, authorship, and/or publication of this article. This research was funded by Research on the Construction of Guizhou Karst Carbon Sink Resource Pool (2024RW318), the Science and Technology Fund of Guizhou [ZK(2023)-236] and Doctoral Fund of Guizhou Academy of Sciences [(2023)01].
The authors would like to thank all the reviewers who participated in the review, as well as MJEditor (www.mjeditor.com) for providing English editing services during the preparation of this manuscript.
The authors declare that the research was conducted in the absence of any commercial or financial relationships that could be construed as a potential conflict of interest.
All claims expressed in this article are solely those of the authors and do not necessarily represent those of their affiliated organizations, or those of the publisher, the editors and the reviewers. Any product that may be evaluated in this article, or claim that may be made by its manufacturer, is not guaranteed or endorsed by the publisher.
Abrar, M. M., Xu, M. G., Shah, S. A. A., Aslam, M. W., Aziz, T., Mustafa, A., et al. (2020). Variations in the profile distribution and protection mechanisms of organic carbon under long-term fertilization in a Chinese Mollisol. Sci. Total Environ. 723:138181. doi: 10.1016/j.scitotenv.2020.138181
Andersson, E., Meklesh, V., Gentile, L., Bhattacharya, A., Stålbrand, H., Tunlid, A., et al. (2023). Generation and properties of organic colloids extracted by water from the organic horizon of a boreal forest soil. Geoderma 432:116386. doi: 10.1016/j.geoderma.2023.116386
Audette, Y., Congreves, K. A., Schneider, K., Zaro, G. C., Nunes, A. L. P., Zhang, H., et al. (2021). The effect of agroecosystem management on the distribution of C functional groups in soil organic matter: a review. Biol. Fertil. Soils 57, 881–894. doi: 10.1007/s00374-021-01580-2
Bai, Y. X., Sheng, M. Y., Hu, Q. J., Zhao, C., and Xiao, H. L. (2021). Response of soil organic carbon and its active fractions to restoration measures in the Karst rocky desertification ecosystem, SW China. Pol. J. Environ. Stud. 30, 1991–2011. doi: 10.15244/pjoes/127916
Bhattacharyya, R., Rabbi, S. M. F., Zhang, Y. Q., Young, I. M., Jones, A. R., Dennis, P. G., et al. (2021). Soil organic carbon is significantly associated with the pore geometry, microbial diversity and enzyme activity of the macro-aggregates under different land uses. Sci. Total Environ. 778:146286. doi: 10.1016/j.scitotenv.2021.146286
Bimüller, C., Kreyling, O., Kölbl, A., von Lützow, M., and Kögel-Knabner, I. (2016). Carbon and nitrogen mineralization in hierarchically structured aggregates of different size. Soil Tillage Res. 160, 23–33. doi: 10.1016/j.still.2015.12.011
Chang, J. J., Zhu, J. X., Xu, L., Su, H. X., Gao, Y., Cai, X. L., et al. (2018). Rational land-use types in the Karst regions of China: insights from soil organic matter composition and stability. Catena 160, 345–353. doi: 10.1016/j.catena.2017.09.029
Chen, J. Q., Jia, Y. N., He, Q. F., Jiang, K., Chen, C., and Ye, K. (2024a). Effect of land use on the stability of soil organic carbon in a Karst region. Environ. Sci. 45, 335–342. doi: 10.13227/j.hjkx.202302142
Chen, L., Zhang, K. L., and Li, Y. T. (2024b). Spatial variability of soil physicochemical properties under different land uses impacted by erosion on Karst hillslopes in Southwest China. Earth Surf. Process. Landf. 49, 2245–2259. doi: 10.1002/esp.5827
Chen, M. M., Zhang, S. R., Liu, L., Liu, J. G., and Ding, X. D. (2022). Organic fertilization increased soil organic carbon stability and sequestration by improving aggregate stability and iron oxide transformation in saline-alkaline soil. Plant Soil 474, 233–249. doi: 10.1007/s11104-022-05326-3
Cheng, C., Li, Y. J., Long, M. Z., Gao, M., Zhang, Y. D., Lin, J. Y., et al. (2020). Moss biocrusts buffer the negative effects of Karst rocky desertification on soil properties and soil microbial richness. Plant Soil 475, 153–168. doi: 10.1007/s11104-020-04602-4
Cheng, H. T., Zhou, X. H., Dong, R. S., Wang, X. M., Liu, G. D., and Li, Q. F. (2023). Natural vegetation regeneration facilitated soil organic carbon sequestration and microbial community stability in the degraded Karst ecosystem. Catena 222:106856. doi: 10.1016/J.CATENA.2022.106856
Cotrufo, M. F., Soong, J. L., Horton, A. J., Campbell, E. E., Haddix, M. L., Wall, D. H., et al. (2015). Formation of soil organic matter via biochemical and physical pathways of litter mass loss. Nat. Geosci. 8, 776–779. doi: 10.1038/ngeo2520
Cui, T. T., Zhang, J. B., and Luo, W. Q. (2023). The quantity and quality of humic substances following different land uses in Karst peak-cluster depression in Guangxi, China. Agriculture 13:2246. doi: 10.3390/agriculture13122246
Das, S., Deb, S., Sahoo, S. S., and Sahoo, U. K. (2023). Soil microbial biomass carbon stock and its relation with climatic and other environmental factors in forest ecosystems: a review. Acta Ecol. Sin. 43, 933–945. doi: 10.1016/j.chnaes.2022.12.007
Eze, S., Palmer, S. M., and Chapman, P. J. (2018). Soil organic carbon stock and fractional distribution in upland grasslands. Geoderma 314, 175–183. doi: 10.1016/j.geoderma.2017.11.017
Gao, X., Berhe, A. A., Hu, Y. X., Du, L. L., Hou, F. B., Guo, S. L., et al. (2023). Role of soil organic matter composition and microbial communities on SOC stability: insights from particle-size aggregates. J. Soils Sediments 23, 2878–2891. doi: 10.1007/S11368-023-03528-5
Gentile, L., Floudas, D., Olsson, U., Persson, P., and Tunlid, A. (2024). Fungal decomposition and transformation of molecular and colloidal fractions of dissolved organic matter extracted from boreal forest soil. Soil Biol. Biochem. 195:109473. doi: 10.1016/j.soilbio.2024.109473
Guillaume, T., Makowski, D., Libohova, Z., Bragazza, L., Sallaku, F., and Sinaj, S. (2022). Soil organic carbon saturation in cropland-grassland systems: storage potential and soil quality. Geoderma 406:115529. doi: 10.1016/J.GEODERMA.2021.115529
Guo, Z. Y., Wang, Y. H., Liu, J. Z., He, L. Y., Zhu, X. H., Zuo, Y. J., et al. (2023). Mapping turnover of dissolved organic carbon in global topsoil. Sci. Total Environ. 906:167621. doi: 10.1016/j.scitotenv.2023.167621
Hall, S. J., Ye, C. L., Weintraub, S. R., and Hockaday, W. C. (2020). Molecular trade-offs in soil organic carbon composition at continental scale. Nat. Geosci. 13, 687–692. doi: 10.1038/s41561-020-0634-x
Han, L. F., Sun, K., Jin, J., and Xing, B. S. (2016). Some concepts of soil organic carbon characteristics and mineral interaction from a review of literature. Soil Biol. Biochem. 94, 107–121. doi: 10.1016/j.soilbio.2015.11.023
He, J., Dai, Q. H., Yi, X. S., Wang, Y., Peng, X. D., and Yan, Y. J. (2023). Effects of soil and rock microhabitats on soil organic carbon stability in a Karst peak-cluster depression region of southwestern China. Geoderma Reg. 32:e00623. doi: 10.1016/j.geodrs.2023.e00623
He, P. L., Xu, Q. X., Wang, Z. L., Zhang, Z. Y., Liu, Y., Gu, X. J., et al. (2024). Effects of stand age and soil layer on soil nutrients and readily Oxidizable organic carbon concentrations of Eucalyptus citriodora plantations. For. Res. 37, 115–123. doi: 10.12403/j.1001-1498.20230336
He, J., Yan, Y. J., Yi, X. S., Wang, Y., and Dai, Q. H. (2021). Soil heterogeneity and its interaction with plants in Karst areas. Chin. J. Appl. Ecol. 32, 2249–2258. doi: 10.13287/j.1001-9332.202106.006
Hu, L. N., Li, Q., Yan, J. H., Liu, C., and Zhong, J. X. (2022). Vegetation restoration facilitates belowground microbial network complexity and recalcitrant soil organic carbon storage in Southwest China Karst region. Sci. Total Environ. 820:153137. doi: 10.1016/j.scitotenv.2022.153137
Huang, R. L., Crowther, T. W., Sui, Y. Y., Sun, B., and Liang, Y. T. (2021a). High stability and metabolic capacity of bacterial community promote the rapid reduction of easily decomposing carbon in soil. Commun. Biol. 4:1376. doi: 10.1038/s42003-021-02907-3
Huang, X. F., Zhang, Z. M., Zhou, Y. C., Wang, X. F., Zhang, J. C., and Zhou, X. W. (2021b). Characteristics of soil organic carbon under different Karst landforms. Carbonates Evaporites. 36:40. doi: 10.1007/S13146-021-00711-Y
Hurisso, T. T., Culman, S. W., Horwath, W. R., Wade, J., Cass, D., Beniston, J. W., et al. (2016). Comparison of permanganate-Oxidizable carbon and Mineralizable carbon for assessment of organic matter stabilization and mineralization. Soil Sci. Soc. Amer. J. 80, 1352–1364. doi: 10.2136/sssaj2016.04.0106
Insam, H., and Domsch, K. H. (1988). Relationship between soil organic carbon and microbial biomass on chronosequences of reclamation sites. Microb. Ecol. 15, 177–188. doi: 10.1007/BF02011711
Iqbal, J., Hu, R., Lin, S., Ahamadou, B., and Feng, M. (2009). Carbon dioxide emissions from Ultisol under different land uses in mid–subtropical China. Geoderma 152, 63–73. doi: 10.1016/j.geoderma.2009.05.011
Jastrow, J. D. (1996). Soil aggregate formation and the accrual of particulate and mineral-associated organic matter. Soil Biol. Biochem. 28, 665–676. doi: 10.1016/0038-0717(95)00159-X
Jia, Y. F., Kuzyakov, Y., Wang, G. A., Tan, W. B., Zhu, B., and Feng, X. J. (2020). Temperature sensitivity of decomposition of soil organic matter fractions increases with their turnover time. Land Degrad. Dev. 31, 632–645. doi: 10.1002/ldr.3477
Kang, J., Qu, C. C., Chen, W. L., Cai, P., Chen, C. R., and Huang, Q. Y. (2024). Organo–organic interactions dominantly drive soil organic carbon accrual. Glob. Chang. Biol. 30, –e17147. doi: 10.1111/GCB.17147
Kunmala, P., Jindaluang, W., and Darunsontaya, T. (2023). Distribution of organic carbon fractions in soil aggregates and their contribution to soil aggregate formation of Paddy soils. Commun. Soil Sci. Plant Anal. 54, 1350–1367. doi: 10.1080/00103624.2022.2144875
Lan, J. C. (2020). Responses of soil organic carbon components and their sensitivity to Karst rocky desertification control measures in Southwest China. J. Soils Sediments 21, 978–989. doi: 10.1007/s11368-020-02840-8
Lan, G. Y., Liu, C., Wang, H., Cao, J. H., Tang, W., Li, Q., et al. (2021). Linking soil redistribution to soil organic carbon using 210Pbex along different complex toposequences in a Karst region, Southwest China. Catena 202:105239. doi: 10.1016/j.catena.2021.105239
Li, W. J., He, B., Feng, T., Bai, X. L., Zou, S., Chen, Y., et al. (2023a). Soil microbial communities in Pseudotsuga sinensis forests with different degrees of rocky desertification in the Karst region, Southwest China. Forests 15:47. doi: 10.3390/f15010047
Li, Q., Li, L. F., Du, H. H., Lin, X. Y., Hu, W. F., and Li, Y. C. (2024). Soil conditioners promote the formation of Fe-bound organic carbon and its stability. J. Environ. Manag. 349:119480. doi: 10.1016/J.JENVMAN.2023.119480
Li, Z., Meunier, J. D., and Delvaux, B. (2023c). Goethite affects phytolith dissolution through clay particle aggregation and pH regulation. Geochim. Cosmochim. Acta 349, 11–22. doi: 10.1016/J.GCA.2023.03.021
Li, X. J., Zhang, Q. F., Feng, J. G., Jiang, D. M., and Zhu, B. (2023b). Forest management causes soil carbon loss by reducing particulate organic carbon in Guangxi, southern China. For. Ecosyst. 10:100092. doi: 10.1016/j.fecs.2023.100092
Liao, H. K., Long, J., and Li, J. (2015). Soil organic carbon associated in size-fractions as affected by different land uses in Karst region of Guizhou, Southwest China. Environ. Earth Sci. 74, 6877–6886. doi: 10.1007/s12665-015-4690-2
Liu, J. D. (2019). Stability of soil organic carbon during different vegetation restoration stages in degraded red soil. Fuzhou: Fujian Agriculture and Forestry University.
Liu, D. W., Li, S. L., Zhu, W. X., Wang, Y. Y., Zhang, S. S., and Fang, Y. T. (2023). Storage and stability of soil organic carbon in two temperate forests in northeastern China. Land 12:1019. doi: 10.3390/land12051019
Lu, Y. H., Dai, Q. H., Yi, X. S., Li, H. M., Peng, H. D., and Tan, J. (2023). Impacts of abandoned sloping farmland on soil aggregates and aggregate-associated organic carbon in Karst rocky desertification areas. Environ. Monit. Assess. 195:1404. doi: 10.1007/s10661-023-12024-9
Lu, J. J., Li, S. P., Liang, G. P., Wu, X. P., and Degré, A. (2021). The contribution of microorganisms to soil organic carbon stock under fertilization varies among aggregate size classes. Agronomy 11:2126. doi: 10.3390/agronomy11112126
Lützow, M. V., Kögel-Knabner, I., Ekschmitt, K., Matzner, E., and Flessa, H. (2006). Stabilization of organic matter in temperate soils: mechanisms and their relevance under different soil conditions – a review. Eur. J. Soil Sci. 57, 426–445. doi: 10.1111/j.1365-2389.2006.00809.x
Ma, H. L., Chen, C. C., Yin, Y. F., and Gao, R. (2024a). Experimental study on carbon mineralization of different sizes particle in Forest soils. Acta Pedol. Sin. 61, 1–14.
Ma, T. S., Deng, X. W., Chen, L., and Xiang, W. H. (2020). The soil properties and their effects on plant diversity in different degrees of rocky desertification. Sci. Total Environ. 736:139667. doi: 10.1016/j.scitotenv.2020.139667
Ma, Y. Q., Yu, Y., Nan, S. Z., Chai, Y., Xu, W. Y., Qin, Y., et al. (2024b). Conversion of SIC to SOC enhances soil carbon sequestration and soil structural stability in alpine ecosystems of the Qinghai-Tibet plateau. Soil Biol. Biochem. 195:109452. doi: 10.1016/j.soilbio.2024.109452
Mastro, F. D., Traversa, A., Cocozza, C., Pallara, M., and Brunetti, G. (2020). Soil organic carbon stabilization: influence of tillage on mineralogical and chemical parameters. Soil Syst. 4:58. doi: 10.3390/soilsystems4030058
Mayer, M., Prescott, C. E., Abaker, W. E., Augusto, L., Cécillon, L., Ferreira, G. W., et al. (2020). Tamm review: influence of forest management activities on soil organic carbon stocks: a knowledge synthesis. For. Ecol. Manag. 466:118127. doi: 10.1016/j.foreco.2020.118127
Nie, X. D., Yuan, Z. J., Huang, B., Liao, Y. S., Zhang, X. Q., Li, Z. W., et al. (2019). Effects of water erosion on soil organic carbon stability in the subtropical China. J. Soils Sediments 19, 3564–3575. doi: 10.1007/s11368-019-02305-7
Oren, A., Rotbart, N., Borisover, M., and Bar-Tal, A. (2018). Chloroform fumigation extraction for measuring soil microbial biomass: the validity of using samples approaching water saturation. Geoderma 319, 204–207. doi: 10.1016/j.geoderma.2018.01.007
Peng, X. D., and Dai, Q. H. (2022). Drivers of soil erosion and subsurface loss by soil leakage during Karst rocky desertification in SW China. Int. Soil Water Conservat. Res. 10, 217–227. doi: 10.1016/j.iswcr.2021.10.001
Post, W. M., and Kwon, K. C. (2000). Soil carbon sequestration and land-use change: processes and potential. Glob. Chang. Biol. 6, 317–327. doi: 10.1046/j.1365-2486.2000.00308.x
Qiao, L. L., Zhou, H. K., Wang, Z. H., Li, Y. Z., Chen, W. J., Wu, Y., et al. (2023). Variations in soil aggregate stability and organic carbon stability of alpine meadow and shrubland under long-term warming. Catena 222:106848. doi: 10.1016/j.catena.2022.106848
Rasmussen, C., Heckman, K., Wieder, W. R., Keiluweit, M., Lawrence, C. R., Berhe, A. A., et al. (2018). Beyond clay: towards an improved set of variables for predicting soil organic matter content. Biogeochemistry 137, 297–306. doi: 10.1007/s10533-018-0424-3
Rovira, P., and Vallejo, V. R. (2007). Labile, recalcitrant, and inert organic matter in Mediterranean forest soils. Soil Biol. Biochem. 39, 202–215. doi: 10.1016/j.soilbio.2006.07.021
Sainju, U. M., Caesar-Tonthat, T., and Jabro, J. D. (2009). Carbon and nitrogen fractions in dryland soil aggregates affected by Long-term tillage and cropping sequence. Soil Sci. Soc. Amer. J. 73, 1488–1495. doi: 10.2136/sssaj2008.0405
Six, J., Bossuyt, H., Degryze, S., and Denef, K. (2004). A history of research on the link between (micro) aggregates, soil biota, and soil organic matter dynamics. Soil Tillage Res. 79, 7–31. doi: 10.1016/j.still.2004.03.008
Six, J., Callewaert, P., Lenders, S., De Gryze, S., Morris, S. J., Gregorich, E. G., et al. (2002). Measuring and understanding carbon storage in afforested soils by physical fractionation. Soil Sci. Soc. Am. J. 66, 1981–1987. doi: 10.2136/sssaj2002.1981
Song, R. P., Han, X. M., Yang, Q. F., Zheng, Z. H., and Xi, D. (2022). Effects of understory vegetation heterogeneity on soil organic carbon components in Cunninghamia lanceolata plantation. Land 11:2300. doi: 10.3390/LAND11122300
Tang, R. B., Huang, G. Y., Qiu, L. W., Hu, M. X., Fu, M. C., Wang, L., et al. (2023b). A new classification method of rocky desertification degree in different karst habitats of Southwest China. Earth Environ. 51, 441–454. doi: 10.14050/j.cnki.1672-9250.2023.51.101
Tang, J., Tang, X. X., Qin, Y. M., He, Q. S., Yi, Y., and Ji, Z. L. (2019). Karst rocky desertification progress: soil calcium as a possible driving force. Sci. Total Environ. 649, 1250–1259. doi: 10.1016/j.scitotenv.2018.08.242
Tang, K. Z., Wu, C., Wang, S., Liao, W. J., Yin, L. C., Zhou, W. J., et al. (2023a). Distribution characteristics of soil organic carbon fractions in paddy profiles with 40 years of fertilization under two groundwater levels. J. Soils Sediments 24, 681–691. doi: 10.1007/S11368-023-03664-Y
Thevenot, M., Dignac, M. F., and Rumpel, C. (2010). Fate of lignins in soils: a review. Soil Biol. Biochem. 42, 1200–1211. doi: 10.1016/j.soilbio.2010.03.017
Tisdall, J. M., and Oades, J. M. (1982). Organic matter and water-stable aggregates in soils. J. Soil Sci. 33, 141–163. doi: 10.1111/j.1365-2389.1982.tb01755.x
Tournassat, C., Greneche, J. M., Tisserand, D., and Charlet, L. (2004). The titration of clay minerals. J. Colloid Interface Sci. 273, 224–233. doi: 10.1016/j.jcis.2003.11.021
Valjavec, M. B., Čarni, A., Žlindra, D., Zorn, M., and Marinšek, A. (2022). Soil organic carbon stock capacity in Karst dolines under different land uses. Catena 218:106548. doi: 10.1016/j.catena.2022.106548
Wang, X. W., Liu, Z. Q., Xiong, K. N., He, Q. F., Li, Y., and Li, K. P. (2022). Characteristics and controlling factors of soil dissolved organic matter in the rainy season after vegetation restoration in a karst drainage area, South China. Catena 217:106483. doi: 10.1016/j.catena.2022.106483
Wang, L. J., Luo, N. N., Shi, Q. L., and Sheng, M. Y. (2023). Responses of soil labile organic carbon fractions and enzyme activities to long-term vegetation restorations in the karst ecosystems, Southwest China. Ecol. Eng. 194:107034. doi: 10.1016/j.ecoleng.2023.107034
Wang, Y. Q., Shahbaz, M., Zhran, M., Chen, A. L., Zhu, Z. K., Galal, Y. G. M., et al. (2021). Microbial resource limitation in aggregates in karst and non-karst soils. Agronomy 11:1591. doi: 10.3390/agronomy11081591
Wei, H., Deng, Y. S., Huang, J., Ling, H., Tang, Q. Y., and Xiao, Y. (2022). A quantitative study of the influence of soil organic carbon and pore characteristics on the stability of aggregates of the karst peak-cluster depression area in Southwest China. J. Soils Sediments 23, 312–330. doi: 10.1007/s11368-022-03318-5
Wen, Y. J., Tang, Y. F., Wen, J., Wang, Q., Bai, L. Y., Wang, Y. N., et al. (2020). Variation of intra-aggregate organic carbon affects aggregate formation and stability during organic manure fertilization in a fluvo-aquic soil. Soil Use Manag. 37, 151–163. doi: 10.1111/sum.12676
Witzgall, K., Vidal, A., Schubert, D. I., Höschen, C., Schweizer, S. A., Buegger, F., et al. (2021). Particulate organic matter as a functional soil component for persistent soil organic carbon. Nat. Commun. 12:4115. doi: 10.1038/S41467-021-24192-8
Xu, J. M., Cheema, S. U., and Yuan, K. N. (1999). Studies on organo-mineral complexes in soil ix. Characteristics of humus in calcium-bound and iron/aluminum-bound organo-mineral complexes in soil. Acta Pedol. Sin. 36, 168–178. doi: 10.11766/trxb199708050204
Xu, J. H., Sun, Y., Gao, L., and Cui, X. Y. (2018). A review of the factors influencing soil organic carbon stability. Chin. J. Eco-Agric. 26, 222–230. doi: 10.13930/j.cnki.cjea.170627
Xu, Y. L., Zhao, Y. Q., Cha, X. Y., Yang, W. L., Zheng, M. T., Liu, S., et al. (2024). Nitrogen addition-driven soil organic carbon stability depends on the fractions of particulate and mineral-associated organic carbon. Nutr. Cycl. Agroecosyst. 128, 269–281. doi: 10.1007/S10705-024-10343-Y
Yang, H., Peng, X. Y., Yang, S. Q., Zhang, Y. B., Zhao, C., and Huang, Y. (2022). Effects of land use types on soil organic carbon and soil labile organic carbon in karst faulted basin of southern Yunnan. Acta Ecol. Sin. 42, 7105–7117. doi: 10.5846/stxb202108112216
Yang, H., Prelovsek, M., Huang, F., Zhang, C. L., Cao, J. H., and Ravbar, N. (2019a). Quantification and evaluation of soil organic carbon and its fractions: case study from the classical karst, SW Slovenia. Acta Carsol. 48, 295–311. doi: 10.3986/ac.v48i3.7305
Yang, J. J., Wang, J., Li, A. Y., Li, G. H., and Zhang, F. (2020). Disturbance, carbon physicochemical structure, and soil microenvironment codetermine soil organic carbon stability in oilfields. Environ. Int. 135:105390. doi: 10.1016/j.envint.2019.105390
Yang, H., Xie, Y., Zhu, T., and Zhou, M. (2021). Reduced organic carbon content during the evolvement of calcareous soils in karst region. Forests 12:221. doi: 10.3390/f12020221
Yang, N., Yang, M. Y., Jiang, L., Wan, L., Wu, L., and Zou, D. S. (2019c). Properties of Mineralizable carbon (MC) Pool during re-vegetation on sloping-land with purple soils in Hengyang, Hunan Province. Acta Agrestia Sinica. 27, 320–325. doi: 10.11733/j.issn.1007-0435.2019.02.007
Yang, H., Zhang, P., Zhu, T. B., Li, Q., and Cao, J. H. (2019b). The characteristics of soil C, N, and P stoichiometric ratios as affected by geological background in a karst graben area, Southwest China. Forests 10:601. doi: 10.3390/f10070601
Yu, P. J., Liu, J. L., Tang, H. Y., Ci, E., Tang, X. G., Liu, S. W., et al. (2023). The increased soil aggregate stability and aggregate-associated carbon by farmland use change in a karst region of Southwest China. Catena 231:107284. doi: 10.1016/j.catena.2023.107284
Yu, Y. L., Xu, Y. N., Zhang, X. T., Li, Y. L., Virk, A. L., Li, F. M., et al. (2024). No-till reduced subsoil organic carbon due to decreased microbial necromass in micro-aggregates. Land Degrad. Dev. 35, 1792–1803. doi: 10.1002/ldr.5021
Zhang, Y. J. (2023). Soil colloid characteristics of typical forestcommunities under different vegetationrestoration in karst valley of Southwest China. Chong qing: Southwest University.
Zhang, Y., Gao, Y., Zhang, Y., Gregorich, E., Li, X. J., Chen, X. W., et al. (2022). Rock-Eval thermal analysis method for soil organic carbon measurement-a review. Soils Crops. 11, 282–289. doi: 10.11689/j.issn.2095-2961.2022.03.005
Zhang, X. Q., Li, Z. W., Nie, X. D., Huang, M., Wang, D. Y., Xiao, H. B., et al. (2019). The role of dissolved organic matter in soil organic carbon stability under water erosion. Ecol. Indic. 102, 724–733. doi: 10.1016/j.ecolind.2019.03.038
Zhang, S. L., Sheng, M. Y., Wang, L. J., He, X. X., and Luo, N. N. (2023b). Effects of long term vegetation restorations on soil organic carbon fractions in the karst rocky desertification ecosystem, Southwest China. Acta Ecologica Sinica 43, 8476–8492. doi: 10.20103/j.stxb.202208152346
Zhang, H., Song, T. Q., Wang, K. L., Du, H., Yue, Y. M., Wang, G. X., et al. (2014). Biomass and carbon storage in an age-sequence of Cyclobalanopsis glauca plantations in Southwest China. Ecol. Eng. 73, 184–191. doi: 10.1016/j.ecoleng.2014.09.008
Zhang, B., Xu, C. H., Zhang, Z. H., Hu, C., He, Y. Y., Huang, K. K., et al. (2023a). Response of soil organic carbon and its fractions to natural vegetation restoration in a tropical karst area, Southwest China. Front. Forests Glob. Change 6:1172062. doi: 10.3389/ffgc.2023.1172062
Zhao, L. L., Wu, Z. X., Sun, R., Yang, C., Fu, Q. M., and Tan, Z. H. (2021). An overview of research on classification and determination of soil organic carbon. Trop. Agricul. Eng. 45, 154–161.
Zhao, W. W., Zhang, Z. S., Zhang, X. H., Xu, L., Guan, Q., Lu, K. L., et al. (2023). Combination of mineral protection and molecular characteristics rather than alone to govern soil organic carbon stability in Qinghai-Tibetan plateau wetlands. J. Environ. Manag. 344:118757. doi: 10.1016/j.jenvman.2023.118757
Keywords: Karst areas, soil organic carbon stability, rocky desertification degree, land use, vegetation restoration
Citation: He J, Liu X, Meng W and Chen X (2024) Recent advances in studies of soil organic carbon stability in Karst areas. Front. For. Glob. Change. 7:1453615. doi: 10.3389/ffgc.2024.1453615
Received: 23 June 2024; Accepted: 13 August 2024;
Published: 23 August 2024.
Edited by:
Xi Zhang, Louisiana State University Agricultural Center, United StatesReviewed by:
Xiaolin Gou, Sichuan Academy of Natural Resource Sciences, ChinaCopyright © 2024 He, Liu, Meng and Chen. This is an open-access article distributed under the terms of the Creative Commons Attribution License (CC BY). The use, distribution or reproduction in other forums is permitted, provided the original author(s) and the copyright owner(s) are credited and that the original publication in this journal is cited, in accordance with accepted academic practice. No use, distribution or reproduction is permitted which does not comply with these terms.
*Correspondence: Xi Liu, bHhnejM4OUAxNjMuY29t
Disclaimer: All claims expressed in this article are solely those of the authors and do not necessarily represent those of their affiliated organizations, or those of the publisher, the editors and the reviewers. Any product that may be evaluated in this article or claim that may be made by its manufacturer is not guaranteed or endorsed by the publisher.
Research integrity at Frontiers
Learn more about the work of our research integrity team to safeguard the quality of each article we publish.