- 1Instituto de Ciencias Forestales (ICIFOR-INIA), CSIC, Madrid, Spain
- 2Departamento de Sistemas y Recursos Naturales, Escuela Técnica Superior de Ingeniería de Montes, Forestal y del Medio Natural, Universidad Politécnica de Madrid, Madrid, Spain
- 3Centre de Ciència i Tecnologia Forestal de Catalunya, Solsona, Spain
- 4Department of Forestry and Agricultural Science and Engineering, Universitat de Lleida, Lleida, Spain
- 5Austrian Research Centre for Forests (BFW), Vienna, Austria
- 6Eidgenösische Technische Hochschule Zürich (ETH), Zürich, Switzerland
Restoration involves the recovery and repair of environments because environmental damage is not always irreversible, and communities are not infinitely resilient to such harm. When restoration projects are applied to nature, either directly or indirectly these may take the form of ecological, forestry or hydrological restoration, for example. In the current scenario of global climate change and increasing intensity of disturbances the importance of restoration in all types of ecosystems in order to adapt to the new conditions (so called prestoration) is evident. Whatever the objective of the restoration initiative, there is a lack of consensus as regards common indicators to evaluate the success or failure of the different initiatives implemented. In this study, we have carried out an extensive meta-analysis review of scientific papers aiming to evaluate the outcomes of restoration projects. We have done a review and selected 95 studies implemented in Europe. We explored the main pre-restoration land cover in which restoration initiatives have been implemented, the main causes of degradation, the objective of the restoration action and the indicators selected to analyze the success or failure of the action. We identified a total of 84 indicators in the analyzed papers and compared with the ones proposed for forest in the recent Nature Restoration Law. The analysis revealed five indicators commonly used for the evaluation of restoration initiatives (abundance, coverage, density, Ellenberg indicator, and richness), even where the initial objective has not yet been achieved. Our findings underscore both the benefits and challenges associated with a specific set of harmonized indicators for evaluating the success or failure of restoration initiatives.
1 Introduction
Among the manifestations of global climate change are those of increased temperature due to human-induced atmospheric emissions and loss of biodiversity (Butchart et al., 2010; Halpern et al., 2008). The emerging risks associated with climate change include ecosystem degradation and biodiversity loss, which result from interactions between climatic factors, ecosystems (including biodiversity) and society (Field et al., 2018). The United Nations Intergovernmental Panel on Climate Change (IPCC) has warned that the global climate is changing and that it is likely to continue changing at an increased rate into the future as a consequence of increasing greenhouse gas emissions (IPCC, 2007a,b; Pörtner et al., 2023). These changes have led to a general biotic homogenization (Mori et al., 2018) and widespread loss of biodiversity (Urban, 2015). On the one hand, due to the increasing temperatures drought periods will be more frequent and longer, making conditions less suitable for plant growth (Stylianou et al., 2020). On the other hand, these longer drought periods will further increase the risk and magnitude of wild fire occurrence (Casas et al., 2016; Flannigan et al., 2009; Stephens et al., 2013), leading to increased soil erosion and land degradation (Shakesby, 2011; Shakesby and Doerr, 2006), especially in more susceptible areas such as the Mediterranean basin (Caon et al., 2014; Doerr and Cerdà, 2005; Koutsias et al., 2013; Mayor et al., 2007; Ramón Vallejo et al., 2012). In the case of forests, these effects will alter the environments in which they grow, modifying site conditions such as soil and air temperatures, atmospheric humidity, soil water content or snow cover depth, as well as influencing the duration of the growing-season (Ashraf et al., 2015). The future of forests is particularly important as they are major carbon sinks (Harris et al., 2021) and embrace much of the terrestrial biodiversity (FAO and UNEP, 2020). Moreover, deforestation has a highly negative impact on habitat provision, since the capacity to support a diversity of wildlife is closely linked to the structural diversity of vegetation and tree dominance or coverage (Berg, 1997; Breuste et al., 2013). Changes in society since the 1960s have exacerbated the natural climate-change driven consequences. These human-induced changes include increased water demand, alterations in stream-flow patterns, and the expansion of intensive agriculture at the expense of abandoned farmland in other areas (Moreira et al., 2011; Rey Benayas and Bullock, 2012). About 40% of terrestrial natural ecosystems have been converted to agricultural land (FAO, 2018) in order to meet the demands for commodities from an increased population, leading to a decline in provisioning, cultural and regulatory ecosystem service (Bullock et al., 2011; Landis, 2017). These shifts in land use have been significant drivers of habitat loss, fragmentation, and reduced spatial diversity (Gavier-Pizarro et al., 2012; Klar et al., 2012).
Given the state of deterioration of our ecosystems and the abovementioned effect of global climate change, restoration is of prime importance. This has been reflected in the declaration by the United Nations as the “Decade of Restoration” (Dollinger et al., 2023). Consequently, legislation and strategies have been developed to address restoration as a necessary aspect of management at international, national, and regional levels. In September 2011, The Bonn Challenge, launched by the Government of Germany and the International Union for Conservation of Nature (IUCN), focused on bringing 150 million hectares of degraded and deforested landscapes into restoration by 2020 and 350 million hectares by 2030. Ten years after in 2021, the European Commission adopted the New EU Forest Strategy for 2030, mainly focused on the greenhouse gas emission reduction target of at least 55% by 2030 and climate neutrality by 2050, to contribute to achieving the EU’s biodiversity objectives (European Commission, 2021). In June 2022, the European Commission proposed a binding “Nature Restoration Law” (NRL) (European Commission, 2022) aimed at restoring ecosystems, habitats, and species across both land and sea areas as a fundamental component of the EU Biodiversity Strategy (European Commission, 2020). In November 2023, representatives from the Council presidency and the European Parliament reached a provisional political agreement on the proposal. However, before the final text can become EU law, each institution must adopt the provisional agreement. This proposal mandates that member states undertake restoration measures to cover at least 20% of the EU’s land and sea areas by 2030. It is also noteworthy that the Kumming-Montreal Global Biodiversity Framework (United Nations, 2022) was adopted as a global policy framework in December 2022 at the 15th meeting of the Conference of the Parties to the Convention on Global Biodiversity. This framework aims to halt and reverse biodiversity loss by 2030, with particular emphasis on Target 2, which focuses on ecosystem restoration.
However, there is no consensus within the scientific community as to what is considered ecosystem restoration. In most cases, the main concept is the same but with slight nuances and differences in details. In Lenz and Haber (1992) stated that restoration requires a thorough investigation and understanding both of the causes of disturbances and the possibilities for restoration. The International Society for Ecological Restoration defined restoration as the process of assisting the recovery of an ecosystem that has been degraded, damaged or destroyed, in order to retrieve its environmental functions and ecosystem services (Carabassa et al., 2019). Furthermore, the Society of Ecological Restoration International Science and Policy Working Group (SER) (Martelletti et al., 2020) focuses the concept of restoration ecology on counteracting the human impact on the environment, through the creation of an ecosystem that is self-supporting and resilient to future disturbances. The SER has also defined a list of ecological attributes. However, they did not consider natural disasters and other non-human negative impacts of the environment as possible causes of environmental damage in their definition. Some authors also include in their definition the idea of recovery of degraded ecosystems and habitats through revegetation and multi-species planting to restore biodiversity, ecosystem functioning and the supply of ecosystem services (Ibáñez and Rodríguez, 2020; McDonald et al., 2016). By contrast, the main idea of the definitions proposed by White and Walker (1997), Blázquez-Cabrera et al. (2018) and Heitkamp et al. (2008) is the recovery of the previous conditions of the ecosystem to be restored, using nearby, undisturbed areas as a reference. However, the previous state is not always know. Seastedt et al. (2008) pointed out that, at times, after restoration measures are implemented, nature may follow its own trajectory, which may differ from its previous state. This does not necessarily mean that the restoration was unsuccessful. In addition, there are several other terms commonly used as synonyms for restoration, such as rehabilitation, reclamation or compensation. However, as stated by Lenz and Haber (1992), these terms only refer to certain aspects of the restoration. The first of these terms, rehabilitation, refers to restoration in terms of a “demanding reconstruction.” The second term, reclamation, refers to the re-creation of a socially acceptable and productive ecosystem. Finally, the term compensation embraces the balance of detrimental and beneficial influences. In the EU Nature Restoration Law, “restoration” means the “process of actively or passively assisting the recovery of an ecosystem toward or to good condition, of a habitat type to the highest level of condition attainable and to its favorable reference area, of a habitat of a species to a sufficient quality or quantity, or of species populations to satisfactory levels, as a means of conserving or enhancing biodiversity and ecosystem resilience.” Additionally, Seastedt et al. (2008) introduced the concept of “prestoration” in 2008, suggesting that global climate change should be addressed by acknowledging that aiming to restore ecosystems to their previous state within the historical range of biotic and abiotic characteristics and processes may not always be feasible. Therefore, management activities should focus on maintaining genetic and species diversity while promoting desirable species under the new environmental conditions.
In addition to the lack of a consensual definition for restoration, there is an even greater lack of consensus on what success means in restoration initiatives and still more on how this success should be measured. There is also an absence of common indicators for evaluating the success or failure of a restoration initiative (Di Filippo et al., 2017; Engst et al., 2016; Turnhout et al., 2007; Vermaat et al., 2016; Versluijs et al., 2019). SER and partners have undertaken large stocktaking exercises to develop lists of indicators. However, they present the information from a different perspective. Sometimes, even where information is available, outcome indicators are inconsistent from one project to another (Gatt et al., 2022). In most cases, restoration processes require very long periods of time before the success or failure of the measures implemented in the ecosystem can be evaluated. In many cases, this time requirement together with the absence of monetary policies for monitoring the ecosystem after restoration makes it even more difficult to evaluate the success or failure of the measures implemented. In the Nature Restoration Law, six indicators were proposed for forest monitoring regardless of the restoration objectives pursued: standing deadwood; lying deadwood; share of forests with uneven-aged structure; forest connectivity; common forest bird index; and stock of organic carbon. However, it has not been analyzed if these indicators are commonly used to monitor and evaluate forest restoration.
In this context, we performed an analysis of the existing scientific literature from a broad range of restoration initiatives aiming to compile the state of the art about the indicators used to evaluate restoration outcomes. To achieve this, the secondary objectives of this study were to: (i) evaluate the indicators used to analyze the results of the restoration initiatives; (ii) identify the main causes degradation in European ecosystems; (iii) examine the feasibility of harmonizing indicators for assessing restoration outcomes based on the specific objectives of each restoration action; and (iv) identify and outline the main knowledge gaps in current restoration projects.
2 Methodology
2.1 Data collection
We conducted an extensive meta-analysis of the existing scientific literature that was not limited to any particular country or journal. This was carried out in October 2023 through the Web of Science (Core collection of Web of Science) using different key words for the search. Specifically, the combination of keywords used in the search was: ((indicator* OR descriptor* OR parameter* OR measure*) AND (restoration* OR reveget* OR rehabilit* OR rewilding* OR renaturaliz*) AND (evaluat* OR success* OR monitor* OR assess) AND (Europe*) AND (forest* OR broadleaf* OR conifer* OR woodland* OR shrubland* OR grassland* OR riverside*)). Each paper was examined to determine whether the study was related to ecological restorations. The search returned an initial result of 1,030 articles, with 945 articles finally being selected after filtering according to type of article and removing those in the form of proceedings, book chapters or editorial materials. The studies not related to restoration initiatives, not undertaken in a European country, and not written in English were removed. Where there was uncertainty, we included the study and critically evaluated its suitability by reviewing the full text. Full-text screening was performed on the 715 studies. Within the scope of the SUPERB Project (H2020-LC-GD-2020, Grant Agreement number 101036849), the objectives have been outlined to categorize all restoration initiative undertaken in recent years at the European level (Table 1).
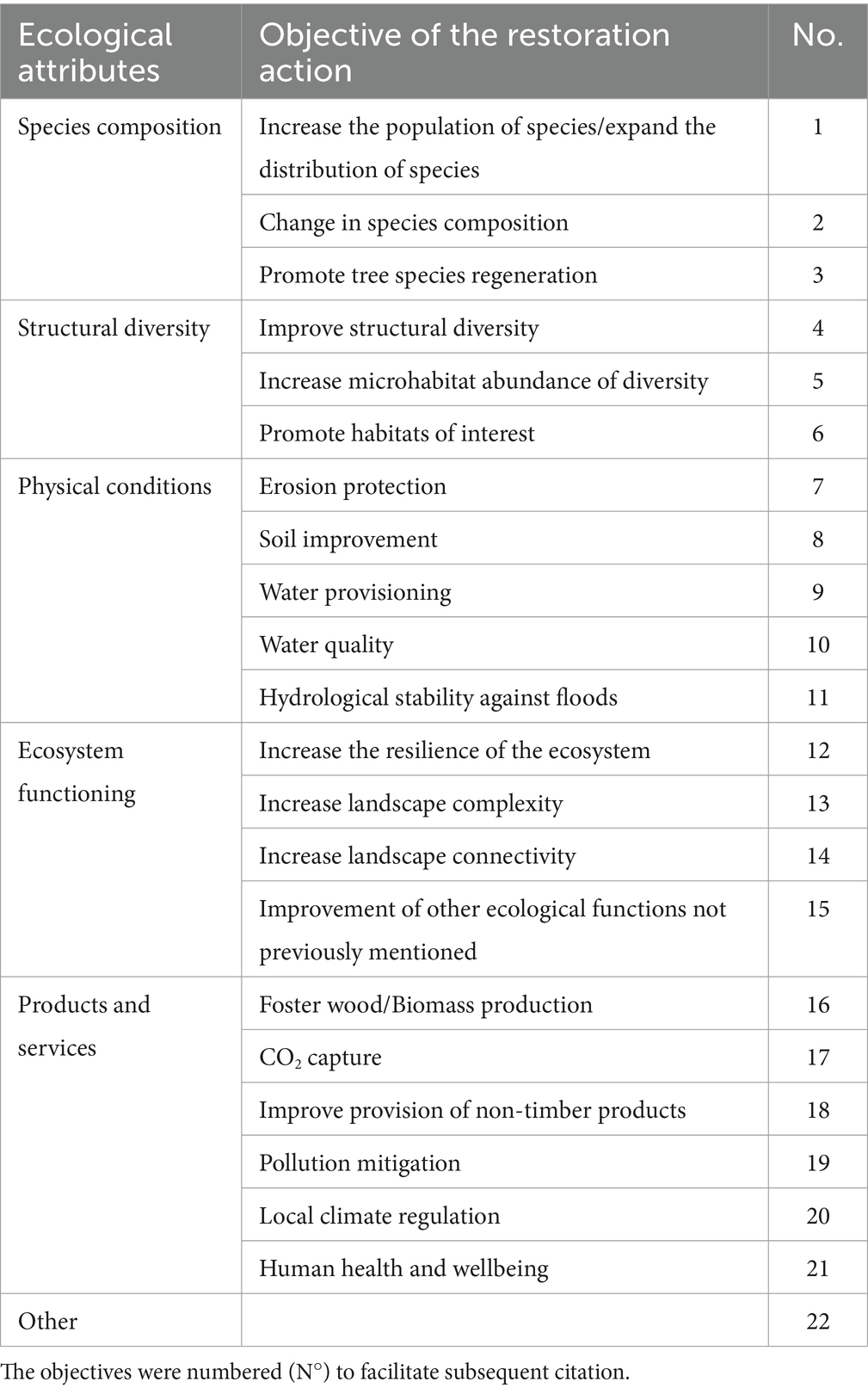
Table 1. Ecological attributes considered and the categorization of the objectives of the selected restoration papers to be analyzed.
Additionally, based on the study by Evju et al. (2020), we used the definition of ecological attributes in the SER standards (Gann et al., 2019) and key ecosystem attributes necessary to evolve toward the short and long-term objectives in ecological restoration. The first four categories coincide exactly with SER ecological attributes. Additionally to these ecological attributes, we included two extra categories, as none of the SER attributes cover these adequately. The fifth category was “Products and services” in order to embrace restorations focused on local climate regulation, pollution mitigation, CO2 capture, fostering of wood/biomass production, improvement of the provision of non-timber products, or human health and wellbeing. The last new category established was “Other,” to consider other restoration aspects not considered in any of the other categories. Table 1 presents the categories of ecological attributes considered for the corresponding objectives of the restoration action established within the framework of SUPERB Project, subcategorized within the ecological attributes.
A minimum threshold of more than 5% of the selected articles was set to ensure that all ecological attributes considered in this article, encompassing the restoration objectives proposed within the SUPERB Project, were representatively analyzed. The articles were then classified according to the impact factor of the journal and number of citations of each paper so as to identify those focusing on the methods and indicators most commonly used and of greatest impact. As a result, 95 articles related to ecological restorations were selected. A PRISMA (Page et al., 2021) diagram of the identification and screening process can be found in Supplementary material.
2.2 Classification of papers
The set of relevant papers selected were examined further to extract information about the restoration projects, detailed attributes such as country in which the projects were carried out, year of implementation, study period, ecological or socioeconomic aspects analyzed and indicators used to measure the outcome of the restoration. Figure 1 provides a comprehensive overview of the distribution of selected European restoration papers. This figure also includes two studies carried out in Russia in close proximity to the European border. Certain studies were not included in Figure 1 because they embrace more than one country. These studies were undertaken in: (i) The Mediterranean area, (ii) Worldwide, (iii) Portugal and France, (iv) USA and Spain, and (v) Netherlands, Germany, Norway, UK, Sweden and France.
We categorized the papers according to the different pre-restoration land cover in which the studies were undertaken. These categories were as follows: Forest ecosystems including all forest types, other woodlands or other lands, according to Food and Agriculture Organization (2020); Grasslands included semi-natural grasslands, inland grasslands on sandy soils; Bare areas; Agricultural lands; and Wetlands including riversides. We also included a category for “other” pre-restoration land covers, including for example spontaneous vegetation growth after restorations, heathlands or shrublands.
The types of restoration targets varied widely across the studies, encompassing diverse ecosystems and objectives such as reforestation, afforestation, and ecological restoration. Additionally, several studies addressed measures aimed at mitigating the negative impacts of factors like wildfires. Depending on the type of restoration implemented or evaluated in the study, we established five categories: post-disturbances such as fires, wind, storms, pests or mining; hydromorphology, species restoration (such as animals, shrubs or trees); landscape fragmentation; and degradation/abandonment recovery.
Once all the papers had been classified into different categories, a correspondence analysis of the keywords used in the papers was conducted to qualitatively analyze any common words typically selected as keywords in restoration studies using T-LAB 10 (T-LAB di Lancia Franco) software.
We also extracted data on the different evaluation indicators generated by the studies. In the analysis conducted in this study, any variable directly measured in the field, derived from others, parameter, etc., utilized in the various articles to assess the success or failure of the restoration efforts, has been regarded as an “indicator.” This compilation and posterior analysis allowed us to establish indicator categories depending on the type of restoration or ecosystem. All the aspects and parameters evaluated for classifying the selected papers are summarized in Table 2.
3 Results
3.1 General description of the dataset
In total, our dataset included 95 papers related to different types of restoration initiatives carried out in the Eurasia area. Almost half of these papers (54.55%) were published in the first quartile of journals in this field (Q1 journals), 32.95% in Q2, 11.36% in Q3, and only one paper (1.14%) was published in a Q4 Journal. The number of citations of the analyzed papers ranged from 0 citations in the case of the two articles published last year (2023) to a maximum of 189 citations of an article published in 2003. The mean number of citations for all these studies was 50.5, with almost half of the analyzed papers (49.44%) being cited less than 20 times. The selected papers were published between 1992 and 2023.
A first analysis of the keywords in the selected papers revealed “RESTORATION” as the main keyword considered by the authors among the 49 most common keywords used. This keyword frequently appears alongside others like “SOIL,” “MANAGEMENT,” “FOREST” and “CONSERVATION” (sand color, Figure 2), as indicated by their shared color in the co-occurrence analysis shown in Figure 2 (T-LAB 10 software, T-LAB di Lancia Franco). This analysis, based on Sammon method for Multidimensional Scaling (MDS), also highlighted the frequent co-occurrence of keywords like “INDICATOR” and “WILDFIRE” (dark blue, Figure 2), “DISTURBANCE,” “ECOLOGICAL” and “EROSION” (yellow, Figure 2), or “ABANDONMENT,” “LAND” and “CHANGE” (red color, Figure 2).
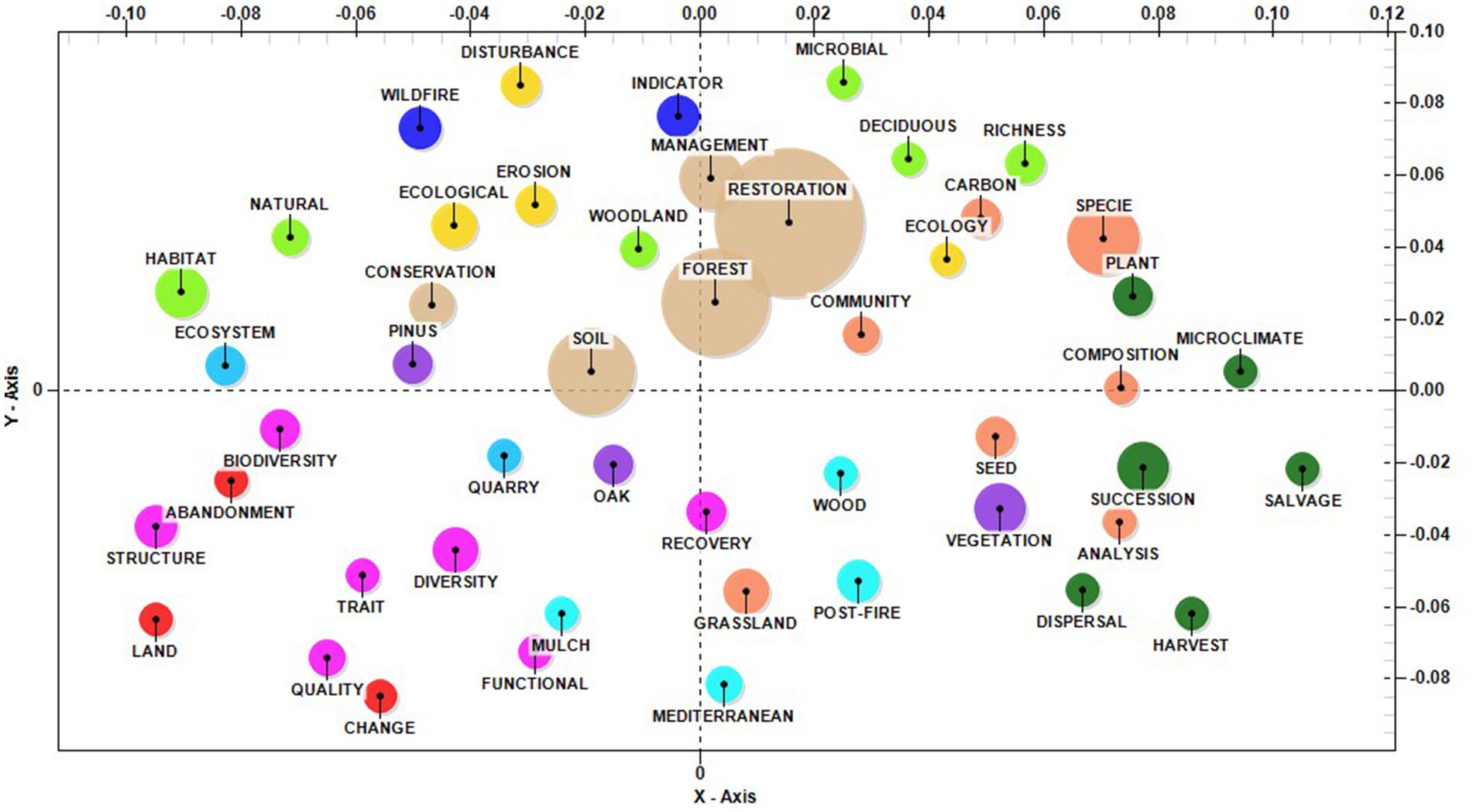
Figure 2. Co-occurrence analysis of the 49 most repeated keywords selected by the authors in the 95 analyzed papers. Each color represents the keywords that frequently co-occur in the analyzed studies. The size of the circles represents the number of times these words have been employed as a keyword in the study papers.
The restauration projects addressed in most of the analyzed papers were undertaken in Germany or Spain (18 and 22, respectively, Figure 1). These two countries accounted for almost the same number of papers as the remaining 15 countries together. The time since the restoration initiative was implemented varied greatly, from 1930 to 2014. More than half of the studies the timing of the restoration project (year in which the restoration action was implemented) was not stated, some were ongoing, and in other cases the restoration measures had been implemented repeatedly. Hence, the variable of time since restoration was not further employed. The times or periods in which measurements of the restoration sites were carried out also varied among the studies. In some cases there was no mention of the measurement period or date and in those studies where it was mentioned, measurements tended to be taken directly after the implementation of the restoration initiative, with repeated measurements after a given number of years.
The most frequently studied pre-restoration land cover was Forest (61), followed at a considerable distance by Agricultural lands (9) or Bare areas (8), whereas the other pre-restoration land covers were more sparsely represented (Figure 3). The analysis revealed that in some cases the studies were undertaken in more than one pre-restoration land cover. In this regard, four types of pre-restoration land cover combinations were observed: (i) Forest + Grasslands, (ii) Bare areas + Agricultural lands + Grasslands, (iii) Forest + Bare areas, and (iv) Forest + Bare areas + Agricultural lands.
Among the different reasons for restoration action to be undertaken, the analyzed articles reflected that “post-disturbance” and “degradation/abandonment” recovery were the most common. Restoration initiatives related to hydromorphology were the least common. As with the studies dealing with multi pre-restoration land cover, there were two case studies which described a combination of two types of restoration initiative (species restoration + degradation/abandonment recovery) (Figure 4). An in-depth analysis of post-disturbance studies (Figure 5), highlighted the importance of fire disturbance in the analyzed papers, this being the main type of disturbance requiring subsequent restoration (20 out of a total of 43 articles dealt with restoration initiatives following fire disturbance). After fire disturbance, the next most common were mining and other disturbances (intensive management).
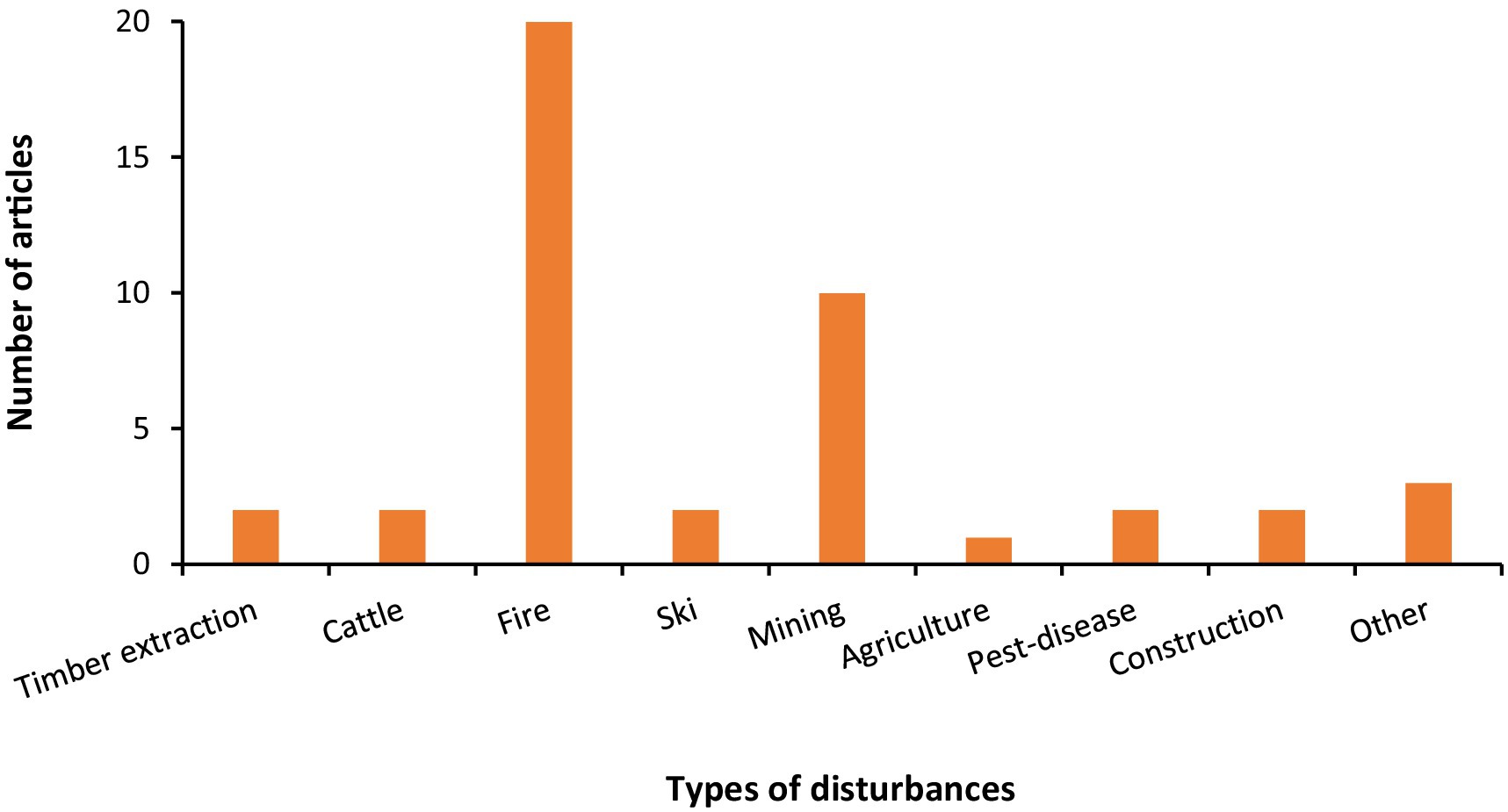
Figure 5. Detail of the types of disturbances that affect the ecosystems in which a post-disturbance restoration initiative was implemented.
Depending on the type of pre-restoration land cover, the type of restoration implemented varied slightly (Figure 6). As shown in Figure 4, post-disturbance was the most common type of restoration, this type being categorized in different pre-restoration land covers, specifically 31% in the case of Forests, 7% in Bare areas, 2% in Wetlands and 1% in Other pre-restoration land covers, respectively. The second most frequent type of restoration initiatives addressed were those for degradation/abandonment recovery, this type being present in all the pre-restoration land covers except for the Bare areas, for which the studies only addressed Post-disturbance and Species restoration initiatives. Studies dealing with landscape fragmentation and Degradation/Abandonment recovery are common to both Agricultural lands and Forest.
Overall, 67 studies (70.53%) dealt with more than one restoration objective. The remaining studies (29.47%) only dealt with one main objective of the restoration initiative analyzed. Within this data it was found that the 22 possible objectives of the restoration projects were reflected in 207 cases (Figure 7). Almost half of these cases (40%) were corresponded to objectives 1, 2 and 8 (see Table 1. Increase the population of species/expand the distribution of the species, Change in species composition, and Soil improvement, respectively). In terms of number of cases, the next most prevalent were objectives 3, 4, and 7 (To promote tree/plant regeneration, Improve structural diversity, and Erosion protection, respectively), which were dealt with in 7, 7 and 8% of the analyzed papers, respectively. Among the Other objectives of the restoration project, 5% of the analyzed papers addressed aspects such as conservation of species, economic rehabilitation, climate change adaptation, or hydrologic retention. The analysis revealed that there were no studies which focused on Hydrological stability against floods as the main objective of the restoration initiative.
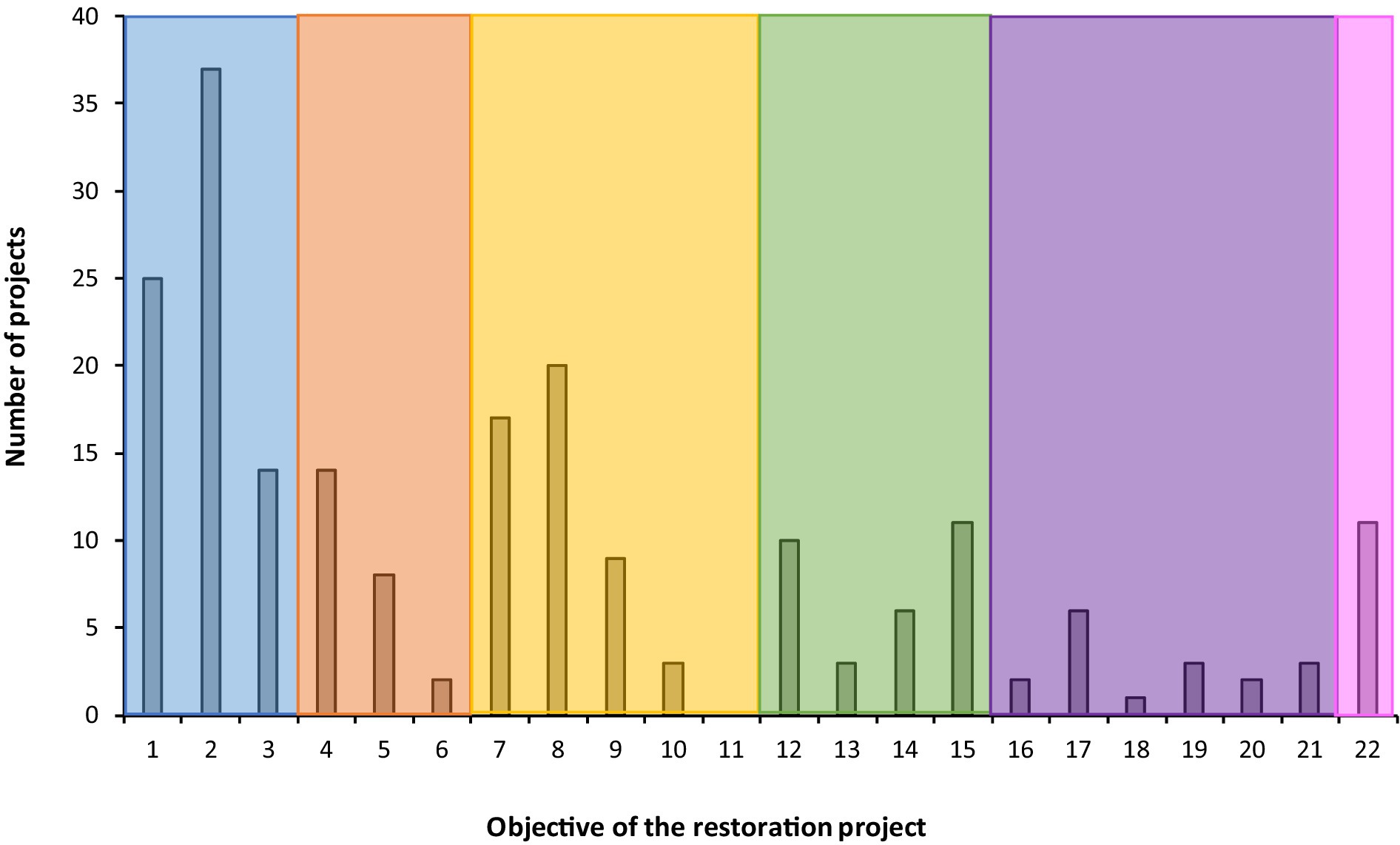
Figure 7. Distribution of the main objectives of the restoration initiatives in the 95 analyzed studies. Numbers on the X-axis correspond to the numbering of the objectives presented in Table 1. Colors correspond to the different ecological attributes that comprises the objectives (Table 1); blue corresponds to Species composition, orange to Structural diversity, yellow to Physical conditions, green to Ecosystem functioning, purple to Products and services, and pink to Other attributes.
3.2 Identification of indicators
We identified a total of 84 indicators in the analyzed papers. Supplementary Table S2 summarizes all the indicators according to the objective of the restoration initiative implemented. Table 3 provides a summary of the quantified indicators identified in alignment with the corresponding objectives of the restoration initiative. Indicators provide the most objective way to monitor and analyze the success or failure of a restoration initiative. Despite the importance of such indicators, this information is still lacking in some cases. In the analyzed studies, the authors sometimes proposed more than one objective of the restoration projects. Therefore, the detail among the different indicators for each objective varies substantially. The concept underlying the selected indicators in the studies was often the same, such as assessing abundance. In these cases, the various indicators have been grouped as a single indicator to streamline the analysis. However, in seven of the studies, the authors made no mention of any success/failure indicators related to the proposed objective in the restoration initiative analyzed. This aspect leaved objectives 11, 13, and 18 without any proposed indicator. There was no consensus around a common indicator for any of the proposed objectives of the restoration initiatives. However, the analysis of the selected papers revealed five indicators, mainly focused on biodiversity aspects, which are commonly used for the analysis of different restoration objectives. Table 4 presents these shared indicators and the corresponding restoration objectives.
4 Discussion
This review of scientific literature on restoration initiatives reveals a high diversity of ecological indicators used to evaluate the success or failure of restoration projects. These differences are even more pronounced when considering the methods for measuring each indicator in the field.
Ecological restoration initiatives have become more frequent globally (Evju et al., 2020) because of their importance in mitigating the impacts of climate change by restoring ecosystems and enhancing their resilience. Many scientific papers have emphasized the necessity for adequate evaluation documenting the impacts and progress of restoration initiatives in degraded areas (Kurth and Schirmer, 2014; Palmer et al., 2005; Wortley et al., 2013). In fact, the number of scientific studies based on empirical evaluations of the outcomes of restoration initiatives has increased in recent years (Ruiz-Jaen and Aide, 2005; Wortley et al., 2013), mainly due to awareness of the necessity for such studies in the context of global climate change. Monitoring and evaluation are often regarded as the most cost-effective phases in the implementation of a restoration project. Despite the fact that in many cases, budget constraints of other factors mean that monitoring and evaluation are not carried out, they are perhaps the best way to evaluate the success or failure of a restoration project (Nilsson et al., 2016), given that attribute and indicator measurements provide the necessary data to perform such evaluations. Hence, the EU Nature Restoration Law considers it necessary to compile an inventory of the various restoration initiatives implemented. Unfortunately, there is a lack of common information for assessing the outcomes of restoration projects, as observed in this review of the scientific literature. The diversity of indicators reported in this study clearly reflects the complexity of standardization in this regard. Evju et al. (2020) and Strange et al. (2024) also acknowledge this situation, primarily associated with the wide range of physical conditions, ecosystem types, life forms or variety of goals pursued in the restoration projects. However, without clear metrics and evaluation criteria, it is very difficult to determine the efficacy of restoration initiatives.
4.1 Indicators based on restoration objectives
Our analysis revealed five indicators as those most commonly used in restoration projects: abundance, canopy/plant/species cover, density, Ellenberg indicator, and species richness. These indicators were used to quantify the success or failure of the same or of different restoration objectives. Abundance was used as an indicator when analyzing species composition or structural diversity attributes, specifically when the objective of the restoration initiative was to increase the population of species, induce a change in species composition or increase microhabitat abundance or diversity. For instance, in studies such as Alday et al. (2017), Castro et al. (2010), Sampaio et al. (2021), Bell et al. (2015), Vymazalová et al. (2021) or Woodcock et al. (2012), abundance was used to quantify the success or failure of their respective initiatives in terms of increase in species population or expansion of their distribution (objective 1). On the other hand, while maintaining the concept of abundance, other authors have expressed it in terms of the number of species, eggs or fledglings, thus adapting it in more detail to their studies (Ganatsas et al., 2012; Spanos et al., 2010; Versluijs et al., 2019). When considering objectives such as change in species composition (objective 2) or increased microhabitat abundance or diversity (objective 5), all the studies reviewed explicitly mentioned abundance as an indicator of success (Albrecht et al., 2010; Palo et al., 2013; Penttilä et al., 2013). The analysis by Evju et al. (2020) of restoration projects also reflects the importance of abundance as an indicator, this being the most commonly used indicator (74% of the analyzed studies) for assessing species composition attributes. The concept of coverage in ecosystems was commonly applied as an indicator in studies focused on increasing the population of species (objective 1), changing species composition (objective 2), promoting tree/plant regeneration (objective 3), improving structural diversity (objective 4), increasing microhabitat abundance or diversity (objective 5) or soil improvement (objective 8). Depending on the type of study, this coverage concept took the form of vegetation cover (Baeten et al., 2010; Borchard et al., 2014; Borchard and Fartmann, 2014; Carabassa et al., 2019; Fernández and Vega, 2016; Fernández and Vega, 2014; Halassy et al., 2021; Hekkala et al., 2014; Jírová et al., 2012; Maccherini et al., 2019; Mench et al., 2003; Mudrák et al., 2010; Pasanen et al., 2016; Príncipe et al., 2014; Sampaio et al., 2021; Spanos et al., 2010; Strubelt et al., 2019), canopy cover (Brunet et al., 2012; Ganatsas et al., 2012; Turunen et al., 2017; Vega et al., 2015) or basal area (Mazziotta et al., 2016). Density (number of individuals per unit of surface area) was considered as an indicator in studies focused on increasing the population of species (objective 1) (Tomao et al., 2018), changing species composition (objective 2) (Borchard et al., 2014; Borchard et al., 2013; Borchard and Fartmann, 2014; Penttilä et al., 2013), promoting tree/plant regeneration (objective 3) (Trout et al., 2012) and increasing microhabitat abundance or diversity (objective 5) (Paquet et al., 2006). Ruiz-Jaen and Aide (2005) also observed these slight variations in the concept of coverage as an indicator for evaluating outcomes in restoration projects in which planting or seeding is used to restore a site. The Ellenberg indicator was selected as an indicator for restoration initiatives in degraded or abandoned areas aimed at increasing the population of species (objective 1) (Helbing et al., 2023), changing species composition (objective 2) (Douda et al., 2017), improving structural diversity (objective 4) (Palo et al., 2013), or soil improvement (objective 8) (Oelmann et al., 2017). All these objectives are covered, to a greater or lesser extent, by the definition of this indicator, which is based on an ordinal classification of plants according to their position or realized ecological niche along an environmental gradient. This provides an expert-based estimate of the niche position of each species on ordinal scales representing gradients of light, temperature, continental characteristics, moisture, soil reaction, nutrients and salinity. Finally, species richness was employed to express the success/failure of restoration initiatives in agricultural land, grasslands and forest areas. The main objectives included increasing the population of species (objective 1) (Albrecht et al., 2010; Bell et al., 2015; Carabassa et al., 2019; Carmona-Yáñez et al., 2023; Castro et al., 2010; Edwards et al., 2007; González et al., 2016), changing species composition (objective 2) (Achilles et al., 2021; Caprio et al., 2016; Douda et al., 2017; Ganatsas et al., 2012; Helbing et al., 2023; Palo et al., 2013; Penttilä et al., 2013; Pilotto et al., 2018; Vymazalová et al., 2021; Woodcock et al., 2012), promoting tree/plant regeneration (objective 3) (Castellano et al., 2022), increasing microhabitat abundance or diversity (objective 5) (Paquet et al., 2006; Turunen et al., 2017), improving other ecological functions not previously mentioned (objective 15) (Mazziotta et al., 2016) and local climate regulation (objective 21) (Stoltz et al., 2016). This diversity of main objectives reflects the fact that this indicator is widely used as a measure of biodiversity and habitat quality. The first indicators mentioned (abundance, canopy/plant/species cover, density, Ellenberg indicator, and species richness) are closely related, with the first four combining both concepts of species composition and frequency.
As regards the studies with erosion protection (objective 7), most addressed post-disturbance restoration initiatives in forest areas, and either disturbances caused by fires or mining activities. Despite the lack of consensus on a single indicator, all the studies converged on the importance of soil cover and the capacity of materials to remain on-site as key factors. On the one hand, some studies focused more on analyzing aspects related to climate and weather, such as rainfall intensity, wind and rain events, with indicators such as average runoff coefficient, rainfall erosivity, rate of associated runoff, maximum rainfall intensity in 10 min, total precipitation or average yearly runoff (Carabassa et al., 2019; Carmona-Yáñez et al., 2023; Cerdà et al., 2017; Fernández and Vega, 2014; Girona-García et al., 2021; Martelletti et al., 2020; Vega et al., 2015). On the other, some studies focused the analysis of erosion protection on soil-related factors, with indicators such as soil sorptivity, erosion rates, soil organic carbon content, soil loss, sediment yield or area affected by concentrated erosion (Castellano et al., 2022; Díaz-Raviña et al., 2012; Ferreira et al., 2015; Krautzer et al., 2011; Zethof et al., 2019).
Soil improvement was considered the primary objective in post-disturbance and degradation/abandonment recovery restoration initiatives implemented in agricultural lands, forest, bare areas and heathlands, according to the analyzed papers. Among these studies, as with soil erosion, there was no consensus around a single indicator for soil improvement. Despite this lack of consensus, all the studies converged on two key ideas: (i) restoration of degraded lands should at least be aimed at reversing the effects of degradation by improving soil conditions; and (ii) short-term monitoring following a disturbance is insufficient to clearly determine the effect of restoration initiatives, especially with regard to soils. The recovery of soil properties in both the topsoil and the subsoil is very poor. Díaz-Raviña et al. (2012) highlighted the fact that there was no notable attenuation in most of the negative physical, chemical and microbiological effects of the fire disturbance on soil properties after 4 months. Moreover, Kalinina et al. (2013) only observed the first changes in chemical properties 4 years into the restoration initiative, reporting leaching of bases from the topsoil and increasing soil acidification. These authors also noted further changes after 12 years, including a downsizing of soil structure elements accompanied by a decrease in bulk density, and after 17 years, they found a decrease in pH, loss of exchangeable cations and depletion of base saturation. Authors such as Oelmann et al. (2017) and Zethof et al. (2019) discussed longer intervals, ranging from 20 to 40 years, in order to observe changes in soil components following the implementation of restoration initiatives. All the studies addressing post-fire restoration initiatives emphasized the importance of mulching the burned area to reduce evapotranspiration, increase soil moisture, decrease soil temperature in the first 10 cm, or decelerate the reduction in bioavailable P fractions over time (Carmona-Yáñez et al., 2023; Jiménez et al., 2016; Oelmann et al., 2017). Primarily, the analysis of restoration success in the analyzed papers was based on three aspects: (i) changes related to the chemical composition of the soil, such as pH, soil organic carbon, exchangeable cations, nutrients or available N, P, K (Achilles et al., 2021; Baeten et al., 2010; Caon et al., 2014; Cihlářová et al., 2017; Jiménez et al., 2016; Kalinina et al., 2013; Oelmann et al., 2017); (ii) structural changes measured by soil depth, type and number of particles, bulk density, soil acidity or process of deposition (Carabassa et al., 2019; Heitkamp et al., 2008; Lenz and Haber, 1992); or (iii) changes related to the microbial activity of soil, assessed through indicators such as microbial composition, biomass determination or respiration (Castellano et al., 2022; Duddigan et al., 2020; Kurganova et al., 2019).
Studies focusing on both water provisioning (objective 9) and water quality (objective 10) were based on research conducted in riparian forest affected by degradation/abandonment or post-mining disturbance, as well as riverside forests also affected by disturbances. In both cases, conductivity was a common indicator of success following restoration initiatives. Other indicators considered included pH, sediment deposition or nutrient retention/concentration. Most of the studies with water-related objectives point to the importance of considering the effects of interaction among water, soil variables and vegetation to optimize the design of restoration initiatives (Castellano et al., 2022; Crowell and Lane, 2001; Stylianou et al., 2020; Vermaat et al., 2016).
The objective of increasing ecosystem resilience through restoration projects was only observed in post-disturbance studies, whether the disturbance was due to fire, mining, or intensive timber extraction. The authors of these studies focused their analyses of success on aspects related to the vegetation, using indicators such as vegetation/regeneration cover (Ascoli et al., 2013), structure (Ibáñez and Rodríguez, 2020; Scotton, 2018), diversity (Mazziotta et al., 2016) or even the area occupied by exotic/invasive species (Carabassa et al., 2019). Holl and Aide (2011) also highlighted the importance of habitat cover affecting ecosystem resilience when analyzing biodiversity conservation in a restored area. Recent studies have suggested that landscape resilience may be related to landscape structure, primarily landscape cover and connectivity (Pardini et al., 2010; Tscharntke et al., 2005). Additionally, Hobbs et al. (2016) suggested that this association of landscape cover and connectivity with biodiversity conservation and landscape resilience could potentially be of great help to those responsible for designing restoration initiatives in order to distinguish areas that would most benefit from restoration initiatives from those that can be recovered without intervention. This association was clearly observed in the study by Carabassa et al. (2019), who focused their research on the combination of objective 12 (increase the resilience of the ecosystem) and objectives 13 (increase landscape complexity) and 14 (increase landscape connectivity) when restoring a bare area following disturbance from mining activities. In the cases of these last two objectives (13 and 14), the number and length of the connections, in terms of corridors, paths, or networks, are key indicators of the analyzed restoration initiatives (Carabassa et al., 2019; Quine and Watts, 2009). However, as with other restoration objectives, there was no consensus around a definition of common indicators for these objectives. There were slight differences among studies with regard to the importance of patches when analyzing both the complexity and the connectivity of the landscape, with several different indicators being used, such as the index of connectivity, number of patches, mean patch size, number of times/observations or effective distance (Aavik et al., 2013; Blázquez-Cabrera et al., 2018; Carabassa et al., 2019; Klar et al., 2012; Quine and Watts, 2009).
Fostering wood/biomass production (objective 16) and CO2 capture (objective 17) frequently appeared together in the analyzed papers, in most cases focusing on forest ecosystems or wetlands, including heathlands and riverside forests. This alignment reflects the understanding that biomass is one of the most significant sources of carbon fixation in any ecosystem. On the one hand, the analysis of success in the case of the first of these objectives was determined by quantifying the total amount of wood harvested, as observed by Evju et al. (2020) in their review, or the total basal area (Carmona-Yáñez et al., 2023; Vermaat et al., 2016). On the other hand, success with regard to CO2 capture was commonly estimated in terms of soil organic carbon stocks, encompassing both soil, and above/belowground biomass (Castellano et al., 2022; Escobar et al., 2022; De Gato et al., 2009; Kalinina et al., 2013; Marañón-Jiménez et al., 2013).
Among the 95 analyzed papers, only four studies considered either pollution mitigation (objective 19) or local climate regulation (objective 20) as one of the restoration objectives, particularly when analyzing post-disturbances, species restoration or degradation/abandonment recovery. The small number of studies covering these restoration objectives is compounded by the lack of consensus on the indicator used, even in these few studies. While Castellano et al. (2022) emphasized aspects related to climate using mean daily air temperature or mean daily air humidity as indicators, Carmona-Yáñez et al. (2023) considered total organic carbon after fire disturbance as a better indicator, and El Kateb et al. (2004) focused more on aspects related to vegetation, using indicators such as production index, species cover or soil storage capacity for organic pollutants. However, Stylianou et al. (2020) did not consider any indicators in their study focused on these objectives.
The consideration of human health and wellbeing (objective 21) as an objective of restoration appeared in only three of the studies analyzed, each carried out in a different pre-restoration land cover (bare areas, forest and wetlands) and for different reasons (species restoration, degradation/abandonment recovery, and post-disturbances, respectively). Despite these differences, all three studies coincided on two aspects: (i) individual attributes as well as broader contextual characteristics might also be taken into account in addition to environmental variables; and (ii) a combination of quantitative and qualitative indicators is necessary to analyze the success or failure of this type of restoration initiative (Stoltz et al., 2016; Tomao et al., 2018; Vermaat et al., 2016). While the authors selected species richness, extension of the area, biodiversity, or number of fishing licenses among the quantitative indicators; some of the qualitative indicators were fascination, serenity, culture, or physiological benefits.
The analyzed papers encompassing other restoration objectives included studies focusing on species conservation, the indicators for which—species richness, abundance and number of species—(Penttilä et al., 2013; Rey-Benayas et al., 2010) coincided with those used in studies focused on objectives 1, 2, 4, or 5 (increase the population of species, change in species composition, improve structural diversity, and increase microhabitat abundance or diversity, respectively). A study by Turunen et al. (2017) considered hydrologic retention as one of the restoration objectives, using the percentage of sediment as an indicator, this being a common indicator used in studies focused on erosion protection, for example.
4.2 Assessment and challenges of indicators for forest restoration
The Nature Restoration Law (NRL), proposed by European Commission (2022), plays crucial roles in shaping policies and actions for the conservation and sustainable management of forest and biodiversity across the EU member states, and introduces six key indicators for assessing forest: (i) standing deadwood; (ii) lying deadwood; (iii) share of forests with uneven-aged structure; (iv) forest connectivity; (v) common forest bird index; and (vi) stock of organic carbon. The first two indicators (standing and lying deadwood) have been evaluated based on deadwood volume in studies such as Mazziotta et al. (2016) and Turunen et al. (2017), which focused on enhancing other ecological functions during restoration efforts. The stock of organic carbon, the last of the six indicators, has been examined in studies such as Di Filippo et al. (2017), Castellano et al. (2022), De Gato et al. (2009) or Kalinina et al. (2015) when analyzing the CO2 capture potential. However, comprehensive data on these NRL-proposed indicators is often lacking, and in some cases, such as the stock of organic carbon and the common bird index, data are particularly challenging and costly to obtain.
Furthermore, a holistic approach should be applied in restoration efforts, acknowledging not just the ecological implications of such initiatives but also the potential economic benefits for the region, employment opportunities, and the intricate interplay between climate, ecosystems and society as outlined by the IPCC. However, none of these dimensions were observed in the studies examined in this review, possibly reflecting the challenges associated with their comprehensive monitoring.
The review of restoration studies carried out revealed the heterogeneity in indicators for restoration initiatives implemented in the different pre-restoration land covers proposed. Krebs (2014) defined the concept of ecosystem as the biotic community and its abiotic environment. As a combination of different factors, both abiotic and biotic, ecosystems should be analyzed from different perspectives to cover all possible aspects acting on and evolving in them. This global vision was observed in many of the analyzed studies that combine more than one restoration objective dependent on the different actors involved in the given ecosystem. The lack of common indicators not only impedes our ability to determine whether restoration initiatives can be considered a success or a failure but also inhibits our capacity to learn from past endeavors and improve future restoration strategies. Therefore, establishing a harmonized set of indicators and evaluation frameworks is advisable to ensure that restoration initiatives effectively contribute to climate change adaptation and mitigation, ultimately safeguarding biodiversity and ecosystem services for future generations. Despite the problem of establishing common indicators for successful restoration projects, certain common points have been observed during this review, which could be considered a starting point for future restoration initiatives. Furthermore, we suggest that the common points identified in this study, along with the monitoring costs required for estimating indicators, be taken into consideration to enhance the set of NRL indicators for forests being relevant the consideration of not only ecological but also economic and social aspects.
It is striking that, despite recent studies highlighting the extensive damage caused by natural disturbances in Europe −17% of the 43.8 million m3 of wood damaged annually by bark beetles, 46% by storms and 24% by fires (Forzieri et al., 2023; Patacca et al., 2023), this significance has not been clearly reflected in the scientific review we conducted. Surprisingly, the number of studies focused on restoration efforts following wind disturbances or pest and diseases outbreaks has been remarkably low. Additionally, we would like to highlight that representative of results for Europe could be limited due to the consideration of English scientific papers and not subjected to specific regions (therefore the representation of countries is not balanced as shown in Figure 1) or objectives (Table 3).
To enhance the aforementioned efforts, it is imperative to gather additional data on past and ongoing restoration initiatives worldwide. Specifically, we suggest broadening research to encompass a diverse range of sources, including not only scientific research papers but also gray literature, internal documents, reports, and unpublished papers. It would also be highly beneficial to include documentation on local and regional activities along with their resulting reports.
5 Conclusion
We have provided a comprehensive overview of the types of restoration initiatives implemented in the European area in recent years and the main causes of degradation that necessitate this type of recovery action. To improve our knowledge of restoration, there is a need for integrated research that bridges scientific studies and on-the-ground initiatives. This review of the literature partially compiles the existing knowledge on restoration studies in Europe within the scope of scientific research. Based on the compiled information, it is evident that the variability of pre-restoration land covers in which the restoration initiatives are implemented is reflected in the wide array of different indicators applied to quantify their success or failure. However, five common indicators have been identified for various types of restoration action (abundance, coverage, density, Ellenberg indicator, and species richness). These indicators were used to quantify the success or failure of the same or different restoration objectives. In order to compare the success or failure of different restoration initiatives, it is necessary to harmonize the indicators used to evaluate them. Moreover, it is crucial that they are evaluated in a consistent manner. Establishing standardized evaluation frameworks is advisable to ensure that restoration efforts effectively contribute to climate change goals and safeguard biodiversity for future generations. Therefore, a continued search for common indicators is necessary to facilitate future restoration strategies, while also recognizing the importance of combining scientific knowledge with insights gained from on-the-ground and in-situ initiatives. Additionally, it is fundamental to consider a holistic understanding of the restoration ecosystem and thus, assessing not only ecological but also social, economic and governance aspects. Finally, we recommend that the common indicators identified in this study, along with the associated monitoring costs required for their estimation, be taken into account to enhance the NRL set of indicators for forests.
Author contributions
MM-M: Writing – review & editing, Writing – original draft, Methodology, Formal analysis, Data curation, Conceptualization. ÁR-C: Writing – review & editing, Formal analysis. IC: Writing – original draft, Methodology, Funding acquisition, Conceptualization. ME: Writing – review & editing. SM: Writing – review & editing. KL: Writing – review & editing. JH: Writing – review & editing. LW: Writing – review & editing. IA: Writing – original draft, Methodology, Conceptualization.
Funding
The author(s) declare that financial support was received for the research, authorship, and/or publication of this article. This research was funded by the H2020-LC-GD-2020 (SUPERB Project, Grant Agreement number 101036849), and Biodiversity Foundation of Spanish Ministry for the Ecological Transition and the Demographic Challenge (Spain) (REFOREADAPT Project, Grants call in competition regime for the execution of projects that contribute to implementing the National Plan for Adaptation to Climate Change 2021–2030).
Acknowledgments
We thank Adam Collins for revising and editing the English grammar. We also thank the reviewers and the academic editor who provided useful comments to improve the paper. We thank Johan Svensson too for his comments on the manuscript.
Conflict of interest
The authors declare that the research was conducted in the absence of any commercial or financial relationships that could be construed as a potential conflict of interest.
Publisher’s note
All claims expressed in this article are solely those of the authors and do not necessarily represent those of their affiliated organizations, or those of the publisher, the editors and the reviewers. Any product that may be evaluated in this article, or claim that may be made by its manufacturer, is not guaranteed or endorsed by the publisher.
Supplementary material
The Supplementary material for this article can be found online at: https://www.frontiersin.org/articles/10.3389/ffgc.2024.1420127/full#supplementary-material
References
Aavik, T., Holderegger, R., and Bolliger, J. (2014). The structural and functional connectivity of the grassland plant Lychnis flos-cuculi. Heredity 112, 471–478. doi: 10.1038/hdy.2013.120
Aavik, T., Holderegger, R., Edwards, P. J., and Billeter, R. (2013). Patterns of contemporary gene flow suggest low functional connectivity of grasslands in a fragmented agricultural landscape. J. Appl. Ecol. 50, 395–403. doi: 10.1111/1365-2664.12053
Achilles, F., Tischer, A., Bernhardt-Römermann, M., Heinze, M., Reinhardt, F., Makeschin, F., et al. (2021). European beech leads to more bioactive humus forms but stronger mineral soil acidification as Norway spruce and Scots pine – results of a repeated site assessment after 63 and 82 years of forest conversion in Central Germany. For. Ecol. Manag. 483:118769. doi: 10.1016/j.foreco.2020.118769
Albrecht, M., Schmid, B., Obrist, M. K., Schüpbach, B., Kleijn, D., and Duelli, P. (2010). Effects of ecological compensation meadows on arthropod diversity in adjacent intensively managed grassland. Biol. Conserv. 143, 642–649. doi: 10.1016/j.biocon.2009.11.029
Alday, J. G., Etxeberria, E., and Ametzaga, I. (2017). Conversion of Pinus radiata plantations to native forest after harvest operations: a North Iberian Peninsula case study. Eur. J. For. Res. 136, 801–810. doi: 10.1007/s10342-017-1071-2
Andrés, P., and Mateos, E. (2006). Soil mesofaunal responses to post-mining restoration treatments. Appl. Soil Ecol. 33, 67–78. doi: 10.1016/j.apsoil.2005.08.007
Anlanger, C., Attermeyer, K., Hille, S., Kamjunke, N., Koll, K., König, M., et al. (2022). Large wood in river restoration: a case study on the effects on hydromorphology, biodiversity, and ecosystem functioning. Int. Rev. Hydrobiol. 107, 34–45. doi: 10.1002/iroh.202102089
Arsénio, P., Rodríguez-González, P. M., Bernez, I., Dias, S., Bugalho, M. N., and Dufour, S. (2020). Riparian vegetation restoration: does social perception reflect ecological value? River Res. Appl. 36, 907–920. doi: 10.1002/rra.3514
Ascoli, D., Castagneri, D., Valsecchi, C., Conedera, M., and Bovio, G. (2013). Post-fire restoration of beech stands in the Southern Alps by natural regeneration. Ecol. Eng. 54, 210–217. doi: 10.1016/j.ecoleng.2013.01.032
Ashraf, M. I., Meng, F.-R., Bourque, C. P. A., and MacLean, D. A. (2015). A novel modelling approach for predicting forest growth and yield under climate change. PLoS One 10:e0132066. doi: 10.1371/journal.pone.0132066
Baeten, L., Velghe, D., Vanhellemont, M., De Frenne, P., Hermy, M., and Verheyen, K. (2010). Early trajectories of spontaneous vegetation recovery after intensive agricultural land use. Restor. Ecol. 18, 379–386. doi: 10.1111/j.1526-100X.2009.00627.x
Balogh, L. (2004). “Actual list of neophytes in Hungary and their classification according to their success” in Invasive plants. eds. B. Mihály and Z. Botta-Dukát (Budapest: TermészetBÚVÁR Alapítvány Kiadó), 61–92.
Bell, D., Hjältén, J., Nilsson, C., Jørgensen, D., and Johansson, T. (2015). Forest restoration to attract a putative umbrella species, the white-backed woodpecker, benefited saproxylic beetles. Ecosphere 6:art278. doi: 10.1890/ES14-00551.1
Berg, Å. (1997). Diversity and abundance of birds in relation to forest fragmentation, habitat quality and heterogeneity. Bird Study 44, 355–366. doi: 10.1080/00063659709461071
Blázquez-Cabrera, S., Ciudad, C., Gastón, A., Simón, M., and Saura, S. (2018). Identification of strategic corridors for restoring landscape connectivity: application to the Iberian lynx. Anim. Conserv. 22, 210–219. doi: 10.1111/acv.12464
Borchard, F., Buchholz, S., Helbing, F., and Fartmann, T. (2014). Carabid beetles and spiders as bioindicators for the evaluation of montane heathland restoration on former spruce forests. Biol. Conserv. 178, 185–192. doi: 10.1016/j.biocon.2014.08.006
Borchard, F., and Fartmann, T. (2014). Effects of montane heathland restoration on leafhopper assemblages (Insecta: Auchenorrhyncha). Restor. Ecol. 22, 749–757. doi: 10.1111/rec.12135
Borchard, F., Schulte, A. M., and Fartmann, T. (2013). Rapid response of orthoptera to restoration of montane heathland. Biodivers. Conserv. 22, 687–700. doi: 10.1007/s10531-013-0438-z
Breuste, J., Schnellinger, J., Qureshi, S., and Faggi, A. (2013). Investigations on habitat provision and recreation as ecosystem services in urban parks-two case studies in Linz und Buenos Aires. Bird Study 44, 355–366.
Brunet, J., De Frenne, P., Holmström, E., and Mayr, M. L. (2012). Life-history traits explain rapid colonization of young post-agricultural forests by understory herbs. For. Ecol. Manag. 278, 55–62. doi: 10.1016/j.foreco.2012.05.002
Bullock, J. M., Aronson, J., Newton, A. C., Pywell, R. F., and Rey-Benayas, J. M. (2011). Restoration of ecosystem services and biodiversity: conflicts and opportunities. Trends Ecol. Evol. 26, 541–549. doi: 10.1016/j.tree.2011.06.011
Butchart, S., Walpole, M., Collen, B., van Strien, A., Scharlemann, J., Almond, R., et al. (2010). Global biodiversity: indicators of recent declines. Science 1979:1164. doi: 10.1126/science.1186777
Caon, L., Vallejo, V. R., Coen, R. J., and Geissen, V. (2014). Effects of wildfire on soil nutrients in Mediterranean ecosystems. Earth Sci. Rev. 139, 47–58. doi: 10.1016/j.earscirev.2014.09.001
Caprio, E., Chamberlain, D., and Rolando, A. (2016). Ski-piste revegetation promotes partial bird community recovery in the European Alps. Bird Study 63, 470–478. doi: 10.1080/00063657.2016.1216520
Carabassa, V., Ortiz, O., and Alcañiz, J. M. (2019). RESTOQUARRY: indicators for self-evaluation of ecological restoration in open-pit mines. Ecol. Indic. 102, 437–445. doi: 10.1016/j.ecolind.2019.03.001
Carmona-Yáñez, M. D., Francos, M., Miralles, I., Soria, R., Ahangarkolaee, S. S., Vafaie, E., et al. (2023). Short-term impacts of wildfire and post-fire mulching on ecosystem multifunctionality in a semi-arid pine forest. For. Ecol. Manag. 541:121000. doi: 10.1016/j.foreco.2023.121000
Carnell, R., and Anderson, M. A. (1986). A technique for extensive field measurement of soil anaerobism by rusting of steel rods. Forestry 59, 129–140. doi: 10.1093/forestry/59.2.129
Casas, Á., García, M., Siegel, R. B., Koltunov, A., Ramírez, C., and Ustin, S. (2016). Burned forest characterization at single-tree level with airborne laser scanning for assessing wildlife habitat. Remote Sens. Environ. 175, 231–241. doi: 10.1016/j.rse.2015.12.044
Castellano, C., Bruno, D., Comín, F. A., Rey Benayas, J. M., Masip, A., and Jiménez, J. J. (2022). Environmental drivers for riparian restoration success and ecosystem services supply in Mediterranean agricultural landscapes. Agric. Ecosyst. Environ. 337:108048. doi: 10.1016/j.agee.2022.108048
Castro, J., Moreno-Rueda, G., and Hódar, J. A. (2010). Experimental test of postfire Managemetn in pine forests: impact of salvage Loggin versus partial cutting and nonintervention on bird-species assemblages. Conserv. Biol. 24, 810–819. doi: 10.1111/j.1523-1739.2009.01382.x
Cerdà, A., Lucas Borja, M. E., Úbeda, X., Martínez-Murillo, J. F., and Keesstra, S. (2017). Pinus halepensis M. versus Quercus ilex subsp. rotundifolia L. runoff and soil erosion at pedon scale under natural rainfall in eastern Spain three decades after a forest fire. For. Ecol. Manag. 400, 447–456. doi: 10.1016/j.foreco.2017.06.038
Chytrý, M., and Tichý, L. (2003). Diagnostic, constant and dominant species of vegetation classes and alliances of the Czech Republic: a statistical revision. Folia SCI. Nat. Univ. Masaryk. Brun. 231.
Cihlářová, H., Koutecký, T., Hladký, J., Rosická, Z., Botková, K., Brtnický, M., et al. (2017). Can soil properties determine vegetation of spontaneously recovered postmined areas? Case study of limestone quarry Mokrá. Environ. Eng. Sci. 34, 638–647. doi: 10.1089/ees.2016.0505
Creamer, R. E., Stone, D., Berry, P., and Kuiper, I. (2016). Measuring respiration profiles of soil microbial communities across Europe using MicroResp™ method. Appl. Soil Ecol. 97, 36–43. doi: 10.1016/j.apsoil.2015.08.004
Crowell, M. D., and Lane, S. N. (2001). Evaluating interactions between soil drainage and seedling performance in a restoration of Pinus sylvestris woodland, Scotland. Glob. Ecol. Biogeogr. 10, 147–160. doi: 10.1046/j.1466-822x.2001.00219.x
De Gato, G. D., Loperfido, L., de Angelis, P., and Valentini, R. (2009). Establishment of a planted field with Mediterranean shrubs in Sardinia and its evaluation for climate mitigation and to combat desertification in semi-arid regions. IForest 2, 77–84. doi: 10.3832/ifor0498-002
Di Filippo, A., Biondi, F., Piovesan, G., and Ziaco, E. (2017). Tree ring-based metrics for assessing old-growth forest naturalness. J. Appl. Ecol. 54, 737–749. doi: 10.1111/1365-2664.12793
Díaz-Raviña, M., Martín, A., Barreiro, A., Lombao, A., Iglesias, L., Díaz-Fierros, F., et al. (2012). Mulching and seeding treatments for post-fire soil stabilisation in NW Spain: short-term effects and effectiveness. Geoderma 191, 31–39. doi: 10.1016/j.geoderma.2012.01.003
Doerr, S. H., and Cerdà, A. (2005). Fire effects on soil system functioning: new insights and future challenges. Int. J. Wildland Fire 14, 339–342. doi: 10.1071/WF05094
Dollinger, C., Rammer, W., and Seidl, R. (2023). Climate change accelerates ecosystem restoration in the mountain forests of Central Europe. J. Appl. Ecol. 60, 2665–2675. doi: 10.1111/1365-2664.14520
Douda, J., Boublík, K., Doudová, J., and Kyncl, M. (2017). Traditional forest management practices stop forest succession and bring back rare plant species. J. Appl. Ecol. 54, 761–771. doi: 10.1111/1365-2664.12801
Duddigan, S., Gil-Martínez, M., Fraser, T., Green, I., Diaz, A., Sizmur, T., et al. (2020). Evaluating heathland restoration belowground using different quality indices of soil chemical and biological properties. Agronomy 10:1140. doi: 10.3390/agronomy10081140
Dufrêne, M., and Legendre, P. (1997). Species assemblages and indicator species: the need for a flexible asymmetrical approach. Ecol. Monogr. 67, 345–366. doi: 10.2307/2963459
Edwards, A. R., Mortimer, S. R., Lawson, C. S., Westbury, D. B., Harris, S. J., Woodcock, B. A., et al. (2007). Hay strewing, brush harvesting of seed and soil disturbance as tools for the enhancement of botanical diversity in grasslands. Biol. Conserv. 134, 372–382. doi: 10.1016/j.biocon.2006.08.025
El Kateb, H., Benabdellah, B., Ammer, C., and Mosandl, R. (2004). Reforestation with native tree species using site preparation techniques for the restoration of woodlands degraded by air pollution in the Erzgebirge, Germany. Eur. J. For. Res. 123, 117–126. doi: 10.1007/s10342-004-0027-5
Engst, K., Baasch, A., Erfmeier, A., Jandt, U., May, K., Schmiede, R., et al. (2016). Functional community ecology meets restoration ecology: assessing the restoration success of alluvial floodplain meadows with functional traits. J. Appl. Ecol. 53, 751–764. doi: 10.1111/1365-2664.12623
Escobar, D., Belyazid, S., and Manzoni, S. (2022). Back to the future: restoring northern drained forested peatlands for climate change mitigation. Front. Environ. Sci. 10, 1–18. doi: 10.3389/fenvs.2022.834371
European Commission (2020). Communication from the commission to the European Parliament, the council, the European economic and social committee and the committee of the regions. EU biodiversity strategy for 2030. Bringin nature back into our lives.
European Commission (2022). Proposal for a Regulation of the European Parliament and of the Council on nature restoration.
European Commission (2021). Communication from the commission to the European Parliament, the council, the European economic and social committee and the Committee of the Regions. New EU Forest Strategy for 2030.
Evju, M., Hagen, D., Kyrkjeeide, M. O., and Köhler, B. (2020). Learning from scientific literature: can indicators for measuring success be standardized in “on the ground” restoration? Restor. Ecol. 28, 519–531. doi: 10.1111/rec.13149
FAO and UNEP . (2020). The state of the World’s forests 2020. Forests, biodiversity and people, geographical review. Rome.
Fernández, C., and Vega, J. A. (2014). Efficacy of bark strands and straw mulching after wildfire in NW Spain: effects on erosion control and vegetation recovery. Ecol. Eng. 63, 50–57. doi: 10.1016/j.ecoleng.2013.12.005
Fernández, C., and Vega, J. A. (2016). Shrub recovery after fuel reduction treatments in a gorse shrubland in northern Spain. J. Environ. Manag. 166, 211–216. doi: 10.1016/j.jenvman.2015.10.028
Ferreira, A. J. D., Alegre, S. P., Coelho, C. O. A., Shakesby, R. A., Páscoa, F. M., Ferreira, C. S. S., et al. (2015). Strategies to prevent forest fires and techniques to reverse degradation processes in burned areas. Catena 128, 224–237. doi: 10.1016/j.catena.2014.09.002
Field, C. B., Barros, V., Stocker, T. F., Dabe, Q., Dokkern, D. J., Ebj, K. L., et al. (eds). (2018). “IPCC, 2012: summary for policymakers: managing the risks of extreme events and disasters to advance climate change adaptation” in A Special Report of Working Groups I and II of the Intergovernmental Panel on Climate Change, (Cambridge, UK, and New York, NY, USA: Cambridge University Press), pp. 1–19.
Flannigan, M. D., Krawchuk, M. A., De Groot, W. J., Wotton, B. M., and Gowman, L. M. (2009). Implications of changing climate for global wildland fire. Int. J. Wildland Fire 18, 483–507. doi: 10.1071/WF08187
Food and Agriculture Organization (2020). Terms and definitions FRA 2020. Global Forest Resources Assessment.
Forzieri, G., Dutrieux, L. P., Elia, A., Eckhardt, B., Caudullo, G., Taboada, F. Á., et al. (2023). The database of European Forest insect and disease disturbances: DEFID2. Glob. Chang. Biol. 29, 6040–6065. doi: 10.1111/gcb.16912
Ganatsas, P., Tsitsoni, T., Tsakaldimi, M., and Zagas, T. (2012). Reforestation of degraded kermes oak shrublands with planted pines: effects on vegetation cover, species diversity and community structure. New For 43, 1–11. doi: 10.1007/s11056-011-9262-z
Gann, G. D., Mcdonald, T., Walder, B., Aronson, J., Nelson, C. R., Hallett, J. G., et al. (2019). International standards and principles for the practice of ecological restoration. 2nd Edn. Restoration Ecology 27, S1–S46. United Nations.
Gatt, Y. M., Andradi-Brown, D. A., Ahmadia, G. N., Martin, P. A., Sutherland, W. J., Spalding, M. D., et al. (2022). Quantifying the reporting, coverage and consistency of key indicators in mangrove restoration projects. Front. For. Glob. Change 5, 1–15. doi: 10.3389/ffgc.2022.720394
Gavier-Pizarro, G. I., Calamari, N. C., Thompson, J. J., Canavelli, S. B., Solari, L. M., Decarre, J., et al. (2012). Expansion and intensification of row crop agriculture in the pampas and Espinal of Argentina can reduce ecosystem service provision by changing avian density. Agric. Ecosyst. Environ. 154, 44–55. doi: 10.1016/j.agee.2011.08.013
Girona-García, A., Badía-Villas, D., Martí-Dalmau, C., Ortiz-Perpiñá, O., Mora, J. L., and Armas-Herrera, C. M. (2018). Effects of prescribed fire for pasture management on soil organic matter and biological properties: a 1-year study case in the Central Pyrenees. Sci. Total Environ. 618, 1079–1087. doi: 10.1016/j.scitotenv.2017.09.127
Girona-García, A., Vieira, D. C. S., Silva, J., Fernández, C., Robichaud, P. R., and Keizer, J. J. (2021). Effectiveness of post-fire soil erosion mitigation treatments: a systematic review and meta-analysis. Earth Sci. Rev. 217:103611. doi: 10.1016/j.earscirev.2021.103611
González, E., Masip, A., and Tabacchi, E. (2016). Poplar plantations along regulated rivers may resemble riparian forests after abandonment: a comparison of passive restoration approaches. Restor. Ecol. 24, 538–547. doi: 10.1111/rec.12351
Grime, J. P. (1977). Evidence for the existence of three primary strategies in plants and its relevance to ecological and evolutionary theory. Am. Nat. 111, 1169–1194. doi: 10.1086/283244
Gruppe, A., Kilg, M., and Schopf, R. (2018). Restoration of a Danube floodplain forest: what happens to species richness of terrestrial beetles? Restor. Ecol. 26, 729–739. doi: 10.1111/rec.12627
Halassy, M., Kövendi-Jakó, A., Paolinelli Reis, B., Szitár, K., Seyidova, Z., and Török, K. (2021). N immobilization treatment revisited: a retarded and temporary effect unfolded in old-field restoration. Appl. Veg. Sci. 24, 1–12. doi: 10.1111/avsc.12555
Halpern, B., Walbridge, S., Selkoe, K., Kappel, C., Micheli, F., D’Agrosa, C., et al. (2008). A global map of human impact on marine ecosystems. Science 1979, 948–952. doi: 10.1126/science.1151084
Harris, N. L., Gibbs, D. A., Baccini, A., Birdsey, R. A., de Bruin, S., Farina, M., et al. (2021). Global maps of twenty-first century forest carbon fluxes. Nat. Clim. Chang. 11, 234–240. doi: 10.1038/s41558-020-00976-6
Heitkamp, F., Glatzel, S., Michalzik, B., Fischer, E., and Gerold, G. (2008). Soil microbiochemical properties as indicators for success of heathland restoration after military disturbances. Land Degrad. Dev. 19, 408–420. doi: 10.1002/ldr
Hekkala, A. M., Tarvainen, O., and Tolvanen, A. (2014). Dynamics of understory vegetation after restoration of natural characteristics in the boreal forests in Finland. For. Ecol. Manag. 330, 55–66. doi: 10.1016/j.foreco.2014.07.001
Helbing, F., Fartmann, T., Morkel, C., and Poniatowski, D. (2023). Rapid response of vascular plants and insects to restoration of montane grasslands. Front. Ecol. Evol. 11, 1–15. doi: 10.3389/fevo.2023.1148266
Hobbs, T. J., Neumann, C. R., Meyer, W. S., Moon, T., and Bryan, B. A. (2016). Models of reforestation productivity and carbon sequestration for land use and climate change adaptation planning in South Australia. J. Environ. Manag. 181, 279–288. doi: 10.1016/j.jenvman.2016.06.049
Holl, K. D., and Aide, T. M. (2011). When and where to actively restore ecosystems? For. Ecol. Manag. 261, 1558–1563. doi: 10.1016/j.foreco.2010.07.004
Ibáñez, I., and Rodríguez, A. (2020). Understanding neighborhood effects to increase restoration success of woody plant communities. Ecol. Appl. 30:e02098. doi: 10.1002/eap.2098
IPCC (2007a). “Climate change 2007: synthesis report. Contribution of working groups I” in II and III to the fourth assessment report of the intergovernmental panel on climate change, intergovernmental panel on climate change (Geneva: Intergovernmental Panel on Climate Change).
IPCC (2007b). “Climate change 2007: impacts, adaptation and vulnerability” in Contribution of working group II to the fourth assessment report of the intergovernmental panel on climate change (Cambridge: Camridge University Press).
Jiménez, M. N., Fernández-Ondoño, E., Ripoll, M. Á., Castro-Rodríguez, J., Huntsinger, L., and Navarro, F. B. (2016). Stones and organic mulches improve the Quercus Ilex L. afforestation success under Mediterranean climatic conditions. Land Degrad. Dev. 27, 357–365. doi: 10.1002/ldr.2250
Jiménez-González, M. A., De la Rosa, J. M., Jiménez-Morillo, N. T., Almendros, G., González-Pérez, J. A., and Knicker, H. (2016). Post-fire recovery of soil organic matter in a Cambisol from typical Mediterranean forest in southwestern Spain. Sci. Total Environ. 572, 1414–1421. doi: 10.1016/j.scitotenv.2016.02.134
Jírová, A., Klaudisová, A., and Prach, K. (2012). Spontaneous restoration of target vegetation in old-fields in a central European landscape: a repeated analysis after three decades. Appl. Veg. Sci. 15, 245–252. doi: 10.1111/j.1654-109X.2011.01165.x
João, T., João, G., Bruno, M., and João, H. (2018). Indicator-based assessment of post-fire recovery dynamics using satellite NDVI time-series. Ecol. Indic. 89, 199–212. doi: 10.1016/j.ecolind.2018.02.008
Kalinina, O., Barmin, A. N., Chertov, O., Dolgikh, A. V., Goryachkin, S. V., Lyuri, D. I., et al. (2015). Self-restoration of post-agrogenic soils of Calcisol-solonetz complex: soil development, carbon stock dynamics of carbon pools. Geoderma 237, 117–128. doi: 10.1016/j.geoderma.2014.08.013
Kalinina, O., Chertov, O., Dogikh, A. V., Goryachkin, S. V., Lyuri, D. I., Vormstein, S., et al. (2013). Self-restoration of post-agrogenic Albeluvisols: soil development, carbon stocks and dynamics of carbon pools. Geoderma 207–208, 221–233. doi: 10.1016/j.geoderma.2013.05.019
Kjær, E. D., McKinney, L. V., Nielsen, L. R., Hansen, L. N., and Hansen, J. K. (2012). Adaptive potential of ash (Fraxinus excelsior) populations against the novel emerging pathogen Hymenoscyphus pseudoalbidus. Evol. Appl. 5, 219–228. doi: 10.1111/j.1752-4571.2011.00222.x
Klar, N., Herrmann, M., Henning-Hahn, M., Pott-Dörfer, B., Hofer, H., and Kramer-Schadt Stephanie, S. (2012). Between ecological theory and planning practice: (re-) connecting forest patches for the wildcat in Lower Saxony, Germany. Landsc. Urban Plan. 105, 376–384. doi: 10.1016/j.landurbplan.2012.01.007
Klimkowska, A., Van Diggelen, R., Bakker, J. P., and Grootjans, A. P. (2007). Wet meadow restoration in Western Europe: a quantitative assessment of the effectiveness of several techniques. Biol. Conserv. 140, 318–328. doi: 10.1016/j.biocon.2007.08.024
Koutsias, N., Xanthopoulos, G., Founda, D., Xystrakis, F., Nioti, F., Pleniou, M., et al. (2013). On the relationships between forest fires and weather conditions in Greece from long-term national observations (1894-2010). Int. J. Wildland Fire 22, 493–507. doi: 10.1071/WF12003
Krautzer, B., Graiss, W., Peratoner, G., Partl, C., Venerus, S., and Klug, B. (2011). The influence of recultivation technique and seed mixture on erosion stability after restoration in mountain environment. Nat. Hazards 56, 547–557. doi: 10.1007/s11069-009-9491-z
Krebs, C. J. (2014). Ecology: the experimental analysis of distribution and abundance. 6th Edn. Harlow: Pearson Education Limited.
Kurganova, I., Merino, A., Lopes de Gerenyu, V., Barros, N., Kalinina, O., Giani, L., et al. (2019). Mechanisms of carbon sequestration and stabilization by restoration of arable soils after abandonment: a chronosequence study on phaeozems and chernozems. Geoderma 354:113882. doi: 10.1016/j.geoderma.2019.113882
Kurth, A. M., and Schirmer, M. (2014). Thirty years of river restoration in Switzerland: implemented measures and lessons learned. Environ. Earth Sci. 72, 2065–2079. doi: 10.1007/s12665-014-3115-y
Landis, D. A. (2017). Designing agricultural landscapes for biodiversity-based ecosystem services. Basic Appl. Ecol. 18, 1–12. doi: 10.1016/j.baae.2016.07.005
Lenz, R., and Haber, W. (1992). Approaches for the restoration of forest ecosystems in northeastern Bavaria. Ecol. Model. 63, 299–317. doi: 10.1016/0304-3800(92)90074-O
Levins, R. (1968). Evolution in changing environments: some theoretical explorations, Monographs in population biology. Princeton, NJ: Princeton University Press.
Maccherini, S., Bacaro, G., Giovannetti, G., Angiolini, C., and Chiarucci, A. (2019). Analysing methodological issues in short-term monitoring of rare European beech forests restoration. Plant Biosyst. 153, 60–67. doi: 10.1080/11263504.2018.1454529
Marañón-Jiménez, S., Castro, J., Kowalski, A. S., Serrano-Ortiz, P., Reverter, B. R., Sánchez-Cañete, E. P., et al. (2011). Post-fire soil respiration in relation to burnt wood management in a Mediterranean mountain ecosystem. For. Ecol. Manag. 261, 1436–1447. doi: 10.1016/j.foreco.2011.01.030
Marañón-Jiménez, S., Castro, J., Querejeta, J. I., Fernández-Ondoño, E., and Allen, C. D. (2013). Post-fire wood management alters water stress, growth, and performance of pine regeneration in a Mediterranean ecosystem. For. Ecol. Manag. 308, 231–239. doi: 10.1016/j.foreco.2013.07.009
Martelletti, S., Meloni, F., Freppaz, M., Paone, A., Lonati, M., Pittarello, M., et al. (2020). Soil and plant characteristics in a restored area under mid-term site management. Sustainability 12, 1–16. doi: 10.3390/su12114433
Mayor, A. G., Bautista, S., Llovet, J., and Bellot, J. (2007). Post-fire hydrological and erosional responses of a Mediterranean landscpe: seven years of catchment-scale dynamics. Catena 71, 68–75. doi: 10.1016/j.catena.2006.10.006
Mazziotta, A., Heilmann-Clausen, J., Bruun, H. H., Fritz, Ö., Aude, E., and Tøttrup, A. P. (2016). Restoring hydrology and old-growth structures in a former production forest: modelling the long-term effects on biodiversity. For. Ecol. Manag. 381, 125–133. doi: 10.1016/j.foreco.2016.09.028
McDonald, T., Gann, D., Jonson, J., and Dixon, K. W. (2016). International standards for the practice of ecological restoration-including principles and key concepts. SSRN Electron. J. doi: 10.2139/ssrn.3645563
Mench, M., Bussière, S., Boisson, J., Castaing, E., Vangronsveld, J., Ruttens, A., et al. (2003). Progress in remediation and revegetation of the barren Jales gold mine spoil after in situ treatments. Plant Soil 249, 187–202. doi: 10.1023/A:1022566431272
Moreira, F., Viedma, O., Arianoutsou, M., Curt, T., Koutsias, N., Rigolot, E., et al. (2011). Landscape - wildfire interactions in southern Europe: implications for landscape management. J. Environ. Manag. 92, 2389–2402. doi: 10.1016/j.jenvman.2011.06.028
Mori, A. S., Isbell, F., and Seidl, R. (2018). β-Diversity, community assembly, and ecosystem functioning. Trends Ecol. Evol. 33, 549–564. doi: 10.1016/j.tree.2018.04.012
Morrice, J. A., Valett, H. M., Dahm, C. N., and Campana, M. E. (1997). Alluvial characteristics, groundwater-surface water exchange and hydrological retention in headwater streams. Hydrol. Process. 11, 253–267. doi: 10.1002/(SICI)1099-1085(19970315)11:3<253::AID-HYP439>3.0.CO;2-J
Mudrák, O., Frouz, J., and Velichová, V. (2010). Understory vegetation in reclaimed and unreclaimed post-mining forest stands. Ecol. Eng. 36, 783–790. doi: 10.1016/j.ecoleng.2010.02.003
Nilsson, C., Aradottir, A. L., Hagen, D., Halldórsson, G., Høegh, K., Mitchell, R. J., et al. (2016). Evaluating the process of ecological restoration. Ecol. Soc. 21:18. doi: 10.5751/ES-08289-210141
Nováková, M. H., and Edwards-Jonášová, M. (2015). Restoration of central-European mountain Norway spruce forest 15 years after natural and anthropogenic disturbance. For. Ecol. Manag. 344, 120–130. doi: 10.1016/j.foreco.2015.02.010
Oelmann, Y., Brauckmann, H. J., Schreiber, K. F., and Broll, G. (2017). 40 years of succession or mulching of abandoned grassland affect phosphorus fractions in soil. Agric. Ecosyst. Environ. 237, 66–74. doi: 10.1016/j.agee.2016.12.014
Page, M. J., McKenzie, J. E., Bossuyt, P. M., Boutron, I., Hoffmann, T. C., Mulrow, C. D., et al. (2021). The PRISMA 2020 statement: an updated guideline for reporting systematic reviews. BMJ 372:n71. doi: 10.1136/bmj.n71
Palmer, M. A., Bernhardt, E. S., Allan, J. D., Lake, P. S., Alexander, G., Brooks, S., et al. (2005). Standards for ecologically successful river restoration. J. Appl. Ecol. 42, 208–217. doi: 10.1111/j.1365-2664.2005.01004.x
Palo, A., Ivask, M., and Liira, J. (2013). Biodiversity composition reflects the history of ancient semi-natural woodland and forest habitats - compilation of an indicator complex for restoration practice. Ecol. Indic. 34, 336–344. doi: 10.1016/j.ecolind.2013.05.020
Paquet, J. Y., Vandevyvre, X., Delahaye, L., and Rondeux, J. (2006). Bird assemblages in a mixed woodland-farmland landscape: the conservation value of silviculture-dependant open areas in plantation forest. For. Ecol. Manag. 227, 59–70. doi: 10.1016/j.foreco.2006.02.009
Pardini, R., de Bueno, A. A., Gardner, T. A., Prado, P. I., and Metzger, J. P. (2010). Beyond the fragmentation threshold hypothesis: regime shifts in biodiversity across fragmented landscapes. PLoS One 5:e13666. doi: 10.1371/journal.pone.0013666
Pasanen, H., Rouvinen, S., and Kouki, J. (2016). Artificial canopy gaps in the restoration of boreal conservation areas: long-term effects on tree seedling establishment in pine-dominated forests. Eur. J. For. Res. 135, 697–706. doi: 10.1007/s10342-016-0965-8
Patacca, M., Lindner, M., Lucas-Borja, M. E., Cordonnier, T., Fidej, G., Gardiner, B., et al. (2023). Significant increase in natural disturbance impacts on European forests since 1950. Glob. Chang. Biol. 29, 1359–1376. doi: 10.1111/gcb.16531
Penttilä, R., Junninen, K., Punttila, P., and Siitonen, J. (2013). Effects of forest restoration by fire on polypores depend strongly on time since disturbance - a case study from Finland based on a 23-year monitoring period. For. Ecol. Manag. 310, 508–516. doi: 10.1016/j.foreco.2013.08.061
Pilotto, F., Nilsson, C., Polvi, L. E., and McKie, B. G. (2018). First signs of macroinvertebrate recovery following enhanced restoration of boreal streams used for timber floating. Ecol. Appl. 28, 587–597. doi: 10.1002/eap.1672
Pons, P., Lambert, B., Rigolot, E., and Prodon, R. (2003). The effects of grassland management using fire on habitat occupancy and conservation of birds in a mosaic landscape. Biodivers. Conserv. 12, 1843–1860. doi: 10.1023/A:1024191814560
Pörtner, H.-O., Roberts, D. C., Adams, H., Adler, C., Aldunce, P., Ali, E., et al. (2023). “IPCC 2022: summary for policymakers” in Climate change 2022 – impacts, adaptation and vulnerability (UK and New York: Cambridge University Press), 3–34.
Príncipe, A., Nunes, A., Pinho, P., do Rosário, L., Correia, O., and Branquinho, C. (2014). Modeling the long-term natural regeneration potential of woodlands in semi-arid regions to guide restoration efforts. Eur. J. For. Res. 133, 757–767. doi: 10.1007/s10342-014-0787-5
Quine, C. P., and Watts, K. (2009). Successful de-fragmentation of woodland by planting in an agricultural landscape? An assessment based on landscape indicators. J. Environ. Manag. 90, 251–259. doi: 10.1016/j.jenvman.2007.09.002
Ramón Vallejo, V., Smanis, A., Chirino, E., Fuentes, D., Valdecantos, A., and Vilagrosa, A. (2012). Perspectives in dryland restoration: approaches for climate change adaptation. New For. 43, 561–579. doi: 10.1007/s11056-012-9325-9
Rey Benayas, J. M., and Bullock, J. M. (2012). Restoration of biodiversity and ecosystem services on agricultural land. Ecosystems 15, 883–899. doi: 10.1007/s10021-012-9552-0
Rey-Benayas, J. M., Galván, I., and Carrascal, L. M. (2010). Differential effects of vegetation restoration in Mediterranean abandoned cropland by secondary succession and pine plantations on bird assemblages. For. Ecol. Manag. 260, 87–95. doi: 10.1016/j.foreco.2010.04.004
Ruiz-Jaen, M. C., and Aide, T. M. (2005). Restoration success: how is it being measured? Restor. Ecol. 13, 569–577. doi: 10.1111/j.1526-100X.2005.00072.x
Sampaio, A. D., Pereira, P. F., Nunes, A., Clemente, A., Salgueiro, V., Silva, C., et al. (2021). Bottom-up cascading effects of quarry revegetation deplete bird-mediated seed dispersal services. J. Environ. Manag. 298:113472. doi: 10.1016/j.jenvman.2021.113472
Scotton, M. (2018). Calcareous grassland restoration at a coarse quarry waste dump in the Italian Alps. Ecol. Eng. 117, 174–181. doi: 10.1016/j.ecoleng.2018.04.012
Seastedt, T. R., Hobbs, R. J., and Suding, K. N. (2008). Management of novel ecosystems: are novel approaches required? Front. Ecol. Environ. doi: 10.1890/070046
Seliger, A., Ammer, C., Kreft, H., and Zerbe, S. (2023). Diversification of coniferous monocultures in the last 30 years and implications for forest restoration: a case study from temperate lower montane forests in Central Europe. Eur. J. For. Res. 142, 1353–1368. doi: 10.1007/s10342-023-01595-4
Semizer-Cuming, D., Chybicki, I. J., Finkeldey, R., and Kjær, E. D. (2021). Gene flow and reproductive success in ash (Fraxinus excelsior L.) in the face of ash dieback: restoration and conservation. Ann. For. Sci. 78:14. doi: 10.1007/s13595-020-01025-0
Shakesby, R. A. (2011). Post-wildfire soil erosion in the Mediterranean: review and future research directions. Earth Sci. Rev. 105, 71–100. doi: 10.1016/j.earscirev.2011.01.001
Shakesby, R. A., and Doerr, S. H. (2006). Wildfire as a hydrological and geomorphological agent. Earth Sci. Rev. 74, 269–307. doi: 10.1016/j.earscirev.2005.10.006
Spanos, I., Ganatsas, P., and Tsakaldimi, M. (2010). Evaluation of postfire restoration in suburban forest of Thessaloniki, northern Greece. Global NEST J. 12, 390–400. doi: 10.30955/gnj.000546
Stephens, S. L., Agee, J. K., Fulé, P. Z., North, M. P., Romme, W. H., Swetnam, T. W., et al. (2013). Managing forests and fire in changing climates. Science 1979, 41–42. doi: 10.1126/science.1240294
Stoltz, J., Lundell, Y., Skärbäck, E., van den Bosch, M. A., Grahn, P., Nordström, E. M., et al. (2016). Planning for restorative forests: describing stress-reducing qualities of forest stands using available forest stand data. Eur. J. For. Res. 135, 803–813. doi: 10.1007/s10342-016-0974-7
Strange, N., Ermgassen, S., Marshall, E., Bull, J. W., and Jacobsen, J. B. (2024). Why it matters how biodiversity is measured in environmental valuation studies compared to conservation science. Biol. Conserv. 292:110546. doi: 10.1016/j.biocon.2024.110546
Streitberger, M., Holtmann, L., Bültmann, H., Schmidt, C., and Fartmann, T. (2021). Effects of montane heathland rejuvenation on phytodiversity depend on management intensity and succession. Ecol. Eng. 168:106292. doi: 10.1016/j.ecoleng.2021.106292
Strubelt, I., Diekmann, M., Griese, D., and Zacharias, D. (2019). Inter-annual variation in species composition and richness after coppicing in a restored coppice-with-standards forest. For. Ecol. Manag. 432, 132–139. doi: 10.1016/j.foreco.2018.09.014
Stylianou, M., Gavriel, I., Vogiatzakis, I. N., Zorpas, A., and Agapiou, A. (2020). Native plants for the remediation of abandoned sulphide mines in Cyprus: a preliminary assessment. J. Environ. Manag. 274:110531. doi: 10.1016/j.jenvman.2020.110531
Tomao, A., Secondi, L., Carrus, G., Corona, P., Portoghesi, L., and Agrimi, M. (2018). Restorative urban forests: exploring the relationships between forest stand structure, perceived restorativeness and benefits gained by visitors to coastal Pinus pinea forests. Ecol. Indic. 90, 594–605. doi: 10.1016/j.ecolind.2018.03.051
Trout, R. C., Brooks, S. E., Rudlin, P., and Neil, J. (2012). The effects of restoring a conifer plantation on an ancient woodland site (PAWS) in the UK on the habitat and local population of the hazel dormouse (Muscardinus avellanarius). Eur. J. Wildl. Res. 58, 635–643. doi: 10.1007/s10344-012-0611-9
Tscharntke, T., Klein, A. M., Kruess, A., Steffan-Dewenter, I., and Thies, C. (2005). Landscape perspectives on agricultural intensification and biodiversity - ecosystem service management. Ecol. Lett. 8, 857–874. doi: 10.1111/j.1461-0248.2005.00782.x
Turnhout, E., Hisschemöller, M., and Eijsackers, H. (2007). Ecological indicators: between the two fires of science and policy. Ecol. Indic. 7, 215–228. doi: 10.1016/j.ecolind.2005.12.003
Turunen, J., Aroviita, J., Marttila, H., Louhi, P., Laamanen, T., Tolkkinen, M., et al. (2017). Differential responses by stream and riparian biodiversity to in-stream restoration of forestry-impacted streams. J. Appl. Ecol. 54, 1505–1514. doi: 10.1111/1365-2664.12897
Urban, M. C. (2015). Accelerating extinction risk from climate change. Science 1979, 571–573. doi: 10.1126/science.aaa4984
Vega, J. A., Fernández, C., and Fonturbel, T. (2015). Comparing the effectiveness of seeding and mulching+seeding in reducing soil erosion after a high severity fire in Galicia (NW Spain). Ecol. Eng. 74, 206–212. doi: 10.1016/j.ecoleng.2014.10.019
Vermaat, J. E., Wagtendonk, A. J., Brouwer, R., Sheremet, O., Ansink, E., Brockhoff, T., et al. (2016). Assessing the societal benefits of river restoration using the ecosystem services approach. Hydrobiologia 769, 121–135. doi: 10.1007/s10750-015-2482-z
Versluijs, M., Roberge, J. M., Eggers, S., Boer, J., and Hjältén, J. (2019). Ecological restoration for biodiversity conservation improves habitat quality for an insectivorous passerine in boreal forest. Biol. Conserv. 237, 90–96. doi: 10.1016/j.biocon.2019.06.025
Vymazalová, P., Košulič, O., Hamřík, T., Šipoš, J., and Hédl, R. (2021). Positive impact of traditional coppicing restoration on biodiversity of ground-dwelling spiders in a protected lowland forest. For. Ecol. Manag. 490:119084. doi: 10.1016/j.foreco.2021.119084
White, P. S., and Walker, J. L. (1997). Approximating nature’s variation: selecting and using reference information in restoration ecology. Restor. Ecol. 5, 338–349. doi: 10.1046/j.1526-100X.1997.00547.x
Woodcock, B. A., Bullock, J. M., Mortimer, S. R., Brereton, T., Redhead, J. W., Thomas, J. A., et al. (2012). Identifying time lags in the restoration of grassland butterfly communities: a multi-site assessment. Biol. Conserv. 155, 50–58. doi: 10.1016/j.biocon.2012.05.013
Wortley, L., Hero, J. M., and Howes, M. (2013). Evaluating ecological restoration success: a review of the literature. Restor. Ecol. 21, 537–543. doi: 10.1111/rec.12028
Zanini, E., Bonifacio, E., Albertson, J. D., and Nielsen, D. R. (1998). Topsoil aggregate breakdown under water-saturated conditions. Soil Sci. 163, 288–298. doi: 10.1097/00010694-199804000-00004
Zethof, J. H. T., Cammeraat, E. L. H., and Nadal-Romero, E. (2019). The enhancing effect of afforestation over secondary succession on soil quality under semiarid climate conditions. Sci. Total Environ. 652, 1090–1101. doi: 10.1016/j.scitotenv.2018.10.235
Keywords: forest monitoring, disturbances, abundance, richness, restoration reporting, practical knowledge
Citation: Menéndez-Miguélez M, Rubio-Cuadrado &, Cañellas I, Erdozain M, de Miguel S, Lapin K, Hoffmann J, Werden L and Alberdi I (2024) How to measure outcomes in forest restoration? A European review of success and failure indicators. Front. For. Glob. Change. 7:1420127. doi: 10.3389/ffgc.2024.1420127
Edited by:
Erkki Tomppo, University of Helsinki, FinlandReviewed by:
Markku Simula, University of Helsinki, FinlandDzenan Becirovic, University of Sarajevo, Bosnia and Herzegovina
Copyright © 2024 Menéndez-Miguélez, Rubio-Cuadrado, Cañellas, Erdozain, de Miguel, Lapin, Hoffmann, Werden and Alberdi. This is an open-access article distributed under the terms of the Creative Commons Attribution License (CC BY). The use, distribution or reproduction in other forums is permitted, provided the original author(s) and the copyright owner(s) are credited and that the original publication in this journal is cited, in accordance with accepted academic practice. No use, distribution or reproduction is permitted which does not comply with these terms.
*Correspondence: María Menéndez-Miguélez, bWVuZW5kZXoubWFyaWFAaW5pYS5jc2ljLmVz