- 1Department of Forest Management, University of Montana, Missoula, MT, United States
- 2Mast Reforestation, Seattle, WA, United States
- 3Doerr School of Sustainability, Stanford University, Stanford, CA, United States
- 4Blue Forest, CA, United States
- 5The Nature Conservancy, Arlington, VA, United States
- 6Retired, Portland, OR, United States
- 7Vibrant Planet, PBC, Truckee, CA, United States
- 8PSW Research Station, USDA Forest Service, Davis, CA, United States
- 9School of Environmental and Forest Sciences, University of Washington, Seattle, WA, United States
- 10Colorado Forest Restoration Institute, Colorado State University, Fort Collins, CO, United States
- 11Department of Biology, University of New Mexico, Albuquerque, NM, United States
- 12Department of Environmental Science and Policy, University of California, Davis, Davis, CA, United States
- 13Department of Forestry, New Mexico Highlands University, Las Vegas, NM, United States
Tree establishment following severe or stand-replacing disturbance is critical for achieving U.S. climate change mitigation goals and for maintaining the co-benefits of intact forest ecosystems. In many contexts, natural post-fire tree regeneration is sufficient to maintain forest cover and associated ecosystem services, but increasingly the pattern and scale of disturbance exceeds ecological thresholds and active reforestation may be warranted. Our capacity to plant trees, however, is not keeping pace with reforestation needs. This shortfall is uniquely apparent in the western U.S., where wildfire size and severity have increased in recent decades and long-term divestment in the reforestation supply chain has limited our ability to respond to existing needs. Here we present an analysis of key facets of both the supply and demand side of reforestation in the western U.S. and address six questions: (1) What is the current backlog of potential reforestation needs driven by high-severity wildfire?; (2) How will increasing wildfire activity through the end of the century affect potential reforestation needs?; (3) What is our capacity to meet current and future reforestation needs?; (4) How can we scale the reforestation supply chain to meet current and future demands?; (5) What approaches to reforestation can promote forest resilience to climate change and wildfire?; and (6) Where are opportunities emerging from recent policy initiatives, innovative public-private partnerships, and natural capital markets for scaling reforestation? Between 1984 and 2000, annual tree planting capacity met post-fire needs but cumulatively over the last two decades (2000 to 2021) it has fallen short of fire-driven needs by an estimated 1.5 million ha (ca. 3.8 million ac). We anticipate this gap will increase 2 to 3 fold by 2050. Scaling up reforestation efforts to close this gap will require increased investment across all facets of the reforestation supply chain, public-private partnerships, and novel approaches to reforestation that increase the resilience of western forests to drought and wildfire. We highlight emerging opportunities from recent policy initiatives and conservation finance for expanding reforestation efforts.
Introduction
“Acts of creation are ordinarily reserved for gods and poets, but humbler folk may circumvent this restriction if they know how. To plant a pine, for example, one need be neither god nor poet; one need only own a shovel.”
— Aldo Leopold.
To address the intertwined threats of the climate and biodiversity crises requires that we promptly reduce fossil fuel emissions, sequester excess CO2, and conserve intact ecosystems (Pan et al., 2011; Dinerstein et al., 2020; Dobrowski et al., 2021; IPCC, 2023). Nature-based climate solutions play an important role in sequestering excess CO2 and could provide the equivalent of one-third of the net emissions reductions necessary to achieve the Paris Climate Agreement goal of keeping global warming below 2°C (Griscom et al., 2017). Forests account for roughly two-thirds of this potential carbon sink globally (Pan et al., 2013; Griscom et al., 2017).
Forests are also vital to meet the national climate mitigation goals of the United States (U.S.) (Domke et al., 2020) and sequester an estimated 11% of U.S. annual CO2 emissions as of 2019 (Giebink et al., 2022). However, climate-related risks to forests are accelerating and could undermine their capacity to sequester carbon (Hurteau et al., 2019; Anderegg et al., 2020, 2022). Specifically, the strength of the U.S. forest carbon sink, which is largely driven by disturbance dynamics and forest regrowth, is declining (Williams et al., 2016; Nave et al., 2019; Domke et al., 2020). This decline is especially apparent in the western US states (i.e., AZ, CA, CO, ID, MT, NM, NV, OR, UT, WA, WY), where accelerated rates of tree mortality due to wildfire, disease, pests, and drought have occurred during the last two decades (Allen et al., 2010; Williams and Abatzoglou, 2016; Anderegg et al., 2022). Additionally, relative to historical conditions, many western US forests are currently characterized by altered stand structure and composition due to a century of wildfire suppression, land management legacies, climate change, and their interactions resulting in compounding effects (Hagmann et al., 2021; Hessburg et al., 2021; Prichard et al., 2021).
Naturally occurring post-fire tree regeneration is a key mechanism of resilience to severe wildfire (Coop et al., 2020). Many fire-prone forests exhibit an innate capacity for post-fire tree seedling establishment and recovery following stand-replacing wildfire (e.g., Donato et al., 2009; Laughlin et al., 2023). In some cases (e.g., dry forests with historically frequent-fire regimes), lower post-fire tree densities and slower post-fire tree establishment may be effective in restoring resilience via stand structures that are more resistant to subsequent wildfire (North et al., 2019). In other cases (e.g., cold or wet forests with historically infrequent-fire regimes), slower post-fire tree establishment can allow for biologically diverse early-seral conditions (Swanson et al., 2011) that are currently in deficit in some forest types (e.g., Donato et al., 2009). However, the combined effects of uncharacteristically large and severe wildfires, short-interval high-severity reburns, and stressful post-fire climatic conditions is limiting post-fire tree regeneration in many areas of the western U.S. (e.g., Davis et al., 2019; Stevens-Rumann and Morgan, 2019; Turner et al., 2019).
There are two critical factors behind observed declines in post-fire natural tree regeneration in western US forests; a lack of available post-fire seed sources and declining climatic suitability for natural regeneration (Davis et al., 2018; Rodman et al., 2020; Stewart et al., 2021; Davis et al., 2023; Crockett and Hurteau, 2024). Larger wildfires create more severely burned areas that fall outside the typical seed dispersal radius of surviving parent trees (Buonanduci et al., 2023), and when seed is available, the climate may be too warm and dry for seedlings of the pre-fire tree species to persist (Davis et al., 2019). In combination, these factors are increasing the amount of area that may require active reforestation in order to recover pre-fire carbon and co-benefits of intact forests. For example, in the western US, wildfire causes an estimated 80% of replanting needs on National Forest System (NFS) lands (Dumroese et al., 2019), and expected increases in wildfire activity will likely continue these trends in the future.
Reforestation by artificial regeneration (e.g., tree planting) can enhance carbon sequestration rates compared with natural regeneration (Nave et al., 2019; 2019; Hemes et al., 2023). Consequently, recent studies have examined reforestation, afforestation, and increased stocking scenarios for the U.S. aimed at meeting climate mitigation goals (Cook-Patton et al., 2020; Domke et al., 2020; Fargione et al., 2021). Beyond climate change mitigation, there is also considerable interest in reforestation for a broader set of objectives (Clark et al., 2023), including diversifying and restoring ecosystem function in degraded forests (Stanturf et al., 2014), engineering structural and landscape heterogeneity (North et al., 2009; Safford and Vallejo, 2019), and developing climate-adaptive strategies designed to anticipate shifting species and climatic stressors (Palik et al., 2022). Forests are also critical for myriad co-benefits that stem from intact ecosystems. These benefits include biodiversity (Dinerstein et al., 2020; Dobrowski et al., 2021; Law et al., 2021), soil stabilization and fresh water supply (Neary et al., 2009; Liu et al., 2021), human health (Karjalainen et al., 2010; Burke et al., 2021), timber and non-timber forest products (Campbell et al., 2018; Weiss et al., 2020; Adlam et al., 2022), air quality (Nowak et al., 2014), and the maintenance of rural economies in forested regions (Keegan et al., 2011; Vaughan et al., 2022).
At the scale of the western US, our collective capacity to plant trees is not keeping pace with reforestation needs generated by wildfire. Reforestation efforts on NFS lands have fallen short of legally mandated targets for at least two decades (Dumroese et al., 2019; Nave et al., 2019). For example, only 6% of identified wildfire-driven replanting needs on USDA Forest Service (USFS) NFS lands are being met annually (USDA Forest Service, 2022). Although a reforestation backlog in the western U.S. is widely acknowledged, the scale of the shortfall remains unclear. The USFS has produced national estimates for NFS lands (Dumroese et al., 2019; USDA, 2023) but does not specify their estimation methods. Additionally, we know of no western US-wide estimates for reforestation shortfalls on other public lands or on tribal or private lands, the latter of which constitutes 30% of forestland in the western U.S. (Hoover and Riddle, 2021). Regional analyses that account for varying land-use pressures, climatic stressors, and disturbance dynamics are needed (Fargione et al., 2021). For example, since 2000, the median annual area burned in the western U.S. has quadrupled relative to the 1984–1999 period, and the largest wildfires have tripled in size (Iglesias et al., 2022).
The ability to keep pace with escalating reforestation needs depends on the integrity, functionality, and efficiency of the ‘reforestation pipeline’ (Fargione et al., 2021), which includes the people, supplies, services, and activities necessary for tree planting. In the western U.S., the pipeline and its associated supply chain evolved within a land management and market-based context that has changed significantly in the last four decades and will need to continue evolving to adapt to the needs of current and future climate and disturbance regimes.
The challenges for scaling the reforestation pipeline are many (Fargione et al., 2021; Clark et al., 2023; Kildisheva et al., 2023), but emerging opportunities for collective action exist. The reforestation pipeline in the western U.S. is principally supported by “compliance” forestry needs – restocking harvested areas in accordance with state-specific forest practice regulations. Beyond compliance, reforestation activities require diverse incentives to accommodate landowner types and motivations. Recent policy initiatives such as the REPLANT act and the Inflation Reduction Act (U.S. Congress, 2021a,b, 2022) have directed funding toward reforestation on public and private lands. Additionally, novel approaches to conservation finance, such as reforestation carbon removal projects and green/forest resilience bonds, offer a means for private, public, and tribal land managers to look beyond managing forests for timber as the sole source for funding reforestation.
Here, we present an analysis of key facets of both the supply and demand side of reforestation efforts in the western U.S. We explore the following questions: (1) What is the current backlog of potential reforestation needs driven by high-severity wildfire? (2) How will increasing wildfire activity through the end-of-century affect potential reforestation needs?; (3) What is our capacity to meet current and future reforestation needs?; (4) How can we scale the reforestation supply chain to meet current and future demands?; (5) What approaches to reforestation can promote forest resilience to climate change and wildfire?; and (6) Where are opportunities emerging from recent policy initiatives, innovative public-private partnerships, and natural capital markets?
What is the current backlog of potential reforestation needs driven by high-severity wildfires?
We define potential post-fire reforestation needs within the 11 western states as previously forested areas outside of designated wilderness areas and national parks that burned at high severity (Supplementary materials) and are found at distances that exceed a threshold dispersal distance from extant (unburned) seed sources. We consider these ‘potential’ needs because we cannot anticipate recovery trajectories in all cases, and post-fire management objectives include a wide array of goals, many of which do not require immediate post-fire tree establishment. Post-fire recovery trajectories vary by disturbance severity, biophysical setting, forest type, time since fire, and other factors (Stevens-Rumann and Morgan, 2019; Davis et al., 2023). The areas we identify that can benefit from replanting may see natural establishment and recruitment delayed for years, decades, or even centuries after wildfire, but may eventually recover. Other areas we identify may not recover without active planting efforts or will transition to alternate ecosystems (Coop et al., 2020).
Our approach assumes that seed availability is a first-order constraint on natural regeneration and does not consider climatic limitations of regeneration (Dobrowski et al., 2015; Kemp et al., 2016; Stevens-Rumann and Morgan, 2019; Davis et al., 2023). Published threshold distances for seed dispersal vary; those in the northwestern U.S. suggest thresholds of >200 m (656 ft) (Donato et al., 2009). Studies from the southwestern U.S. identify shorter threshold distances of ca. 50 m (164 ft) (Chambers et al., 2016), and studies from the northern Rockies define intermediate (ca. 100 m [328 ft]) distances (Kemp et al., 2016). This regional variability is expected because potential seed dispersal distances for wind-dispersed conifer seeds are largely a function of tree height and seed size and, thus, are specific to the species and the productivity of the site. In this study, we used an intermediate distance of 100 m (328 ft), while recognizing that there is variability in individual species dispersal distances across our study area.
We estimated the area within high-severity burns that exceed a threshold seed dispersal distance using the model described by Collins et al. (2017) fit with data from 1,615 wildfires from Buonanduci et al. (2023). The area in need of reforestation scales non-linearly with fire size given that it reflects an area:perimeter relationship (Supplementary materials). We use this relationship to estimate reforestation needs for 4,589 wildfires in the Monitoring Trends in Burn Severity (MTBS) database (MTBS, 2023). The MTBS database characterizes wildfires >4 km2 (1.5 mi2) in size from 1984 to 2021. Further details on our approach to estimating wildfire-driven reforestation needs are presented in the Supplementary Methods.
Between 1984 and 2021, 5.2 million ha (13 million ac) of forest area outside of wilderness and national parks burned in the western U.S. at high severity (Figure 1). Using the observations and model assumptions described above, we estimate that high-severity wildfire created approximately 2.4 million ha (5.9 million ac) of potential reforestation needs between 1984 and 2021 (Figure 1).
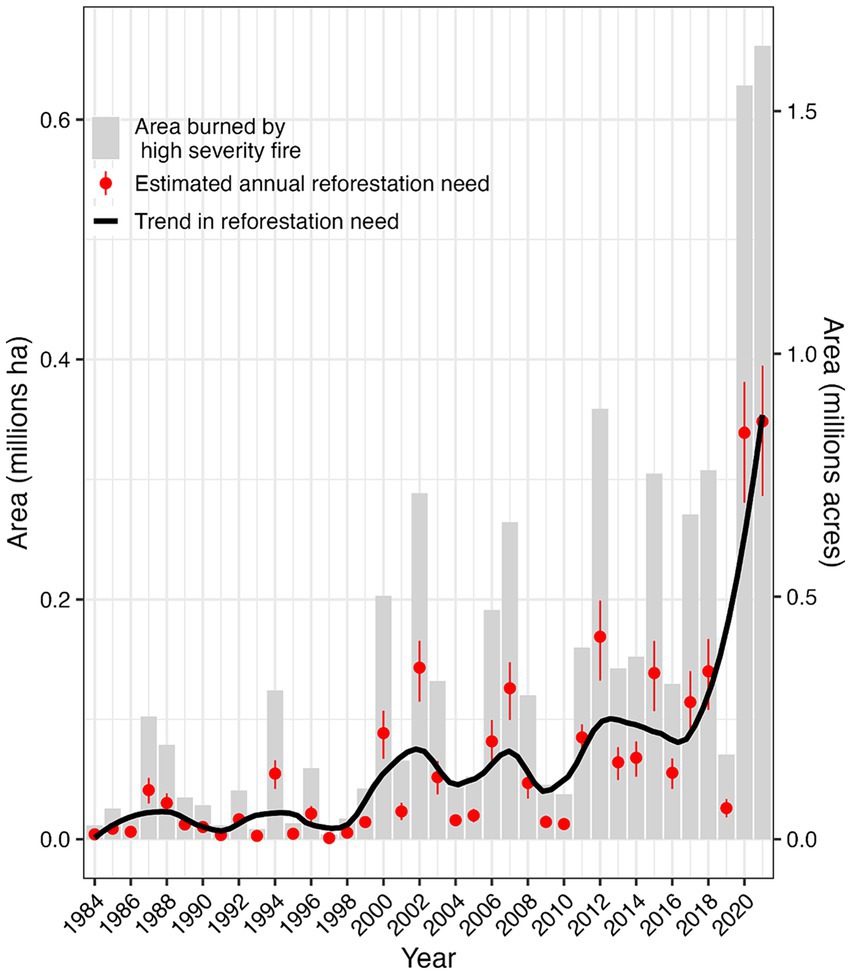
Figure 1. Estimated potential post-fire reforestation needs (derived from areas within high-severity wildfire patches that exceed 100 m [328 ft] from a viable seed source) have been increasing between 1984–2021. Error bars represent the interquartile range of estimates.
We found that the top 20 largest wildfires (out of 4,589) comprised 26% of the total reforestation needs between 1984 and 2021. All of these large wildfires occurred since 2000, and most occurred in 2020 and 2021 (Supplementary Figure S3). Thus, the proportion of area burned each year that requires reforestation, which depends on the size class distribution of wildfires within a given year, has also increased. The mean proportion of the total forest area burned between 1984 and 2021 that met our criteria for reforestation was 0.16 and varied from a minimum of 0.09 to a maximum of 0.24. Prior to 2000, the proportion of burned area in need of reforestation remained relatively flat but has trended upward as wildfires have become larger (Supplementary Figure S4).
Multiple assumptions underlie our models, which if varied, can increase or decrease estimates of reforestation needs. For example, we treat all forest types similarly in these calculations. This may overestimate reforestation needs for fire-obligate seeding forest types (although see Turner et al., 2019). Further, threshold seed dispersal distances are likely longer in moist forest types (e.g., Laughlin et al., 2023) and shorter in dry forest types, given average tree heights and their effects on dispersal distance (Thomson et al., 2011). Additionally, our approach does not account for individual live trees, patch shape, or small isolated patches of live trees that would act to reduce reforestation needs as we define them here. An explicit spatial analysis within burn perimeters of the MTBS dataset would improve upon our estimates but is beyond the scope of this study. Additionally, our estimates do not consider small fires (< 4km2), areas that have reburned that can further increase distances to live seed sources (Gill et al., 2021; Harvey et al., 2023), or other disturbances due to a lack of comprehensive data across land-ownerships in the western U.S. Nonetheless, we consider our approach to be a first-order estimate of fire-driven reforestation needs that can be refined in the future.
How will increasing wildfire activity through the end of the century affect potential reforestation needs?
In addition to using historical wildfire observations to estimate potential reforestation needs, we simulated post-wildfire reforestation needs through the end of the 21st century. We replicated the static log-linear model and predictions of Abatzoglou et al. (2021), which relates the annual area burned in the western U.S. to climatic water deficit (Supplementary Figure S5). Using this model, we predicted the annual area burned between 1985 and 2,100 for each of the 30 climate models in the Sixth Coupled Model Intercomparison Project (CMIP6) from the historical climate experiment for 1950–2014 and from the Shared Socioeconomic Pathway climate change scenario SSP2-45 experiment for 2015–2050 (Abatzoglou et al., 2021). Using the relationship between annual area burned in forests and reforestation needs, which we found to be strongly linear for the period 1984–2021 (Supplementary Figure S6), we forecasted annual post-wildfire reforestation needs given the annual area burned estimated from CMIP simulations for the period 1985–2,100 (Supplementary Methods).
The estimated median cumulative sum of post-fire reforestation needs between 1984 and 2021 (Figure 2) from the CMIP simulations was 1.9 million ha (4.7 million acres), which is lower than our estimates based on observed fire perimeter data for the same period (2.4 million ha [5.9 million acres, or about 160,000 acres per year]). By 2050, we estimate that annual reforestation needs will approach 200,000 ha (ca. 500,000 acres, more than triple the historical rate). For the period between 1984 and 2050, we estimate cumulative needs will be 5.5 million ha (13.7 million acres). We also note that the ensemble median CMIP6 simulations show far less year-to-year variation in reforestation needs compared to observations, suggesting that our estimates may be conservative (Figure 2).
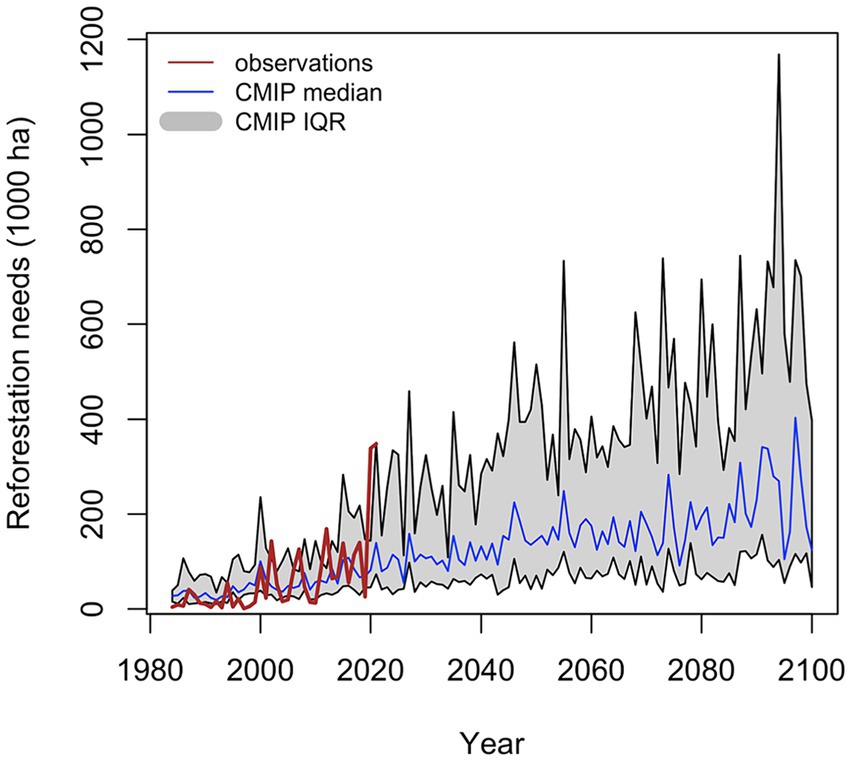
Figure 2. Projected post-fire reforestation needs through the end of the century from estimates of annual area burned derived from an ensemble of 30 CMIP6 climate models. The gray shaded area represents the interquartile range (IQR) of estimates. The blue line represents the median of these 30 projections. Estimates of reforestation needs derived from observed fires are shown in red and correspond to Figure 1.
What is our capacity to meet current and future reforestation needs?
To quantify potential reforestation capacity, we use seedling production as an imperfect proxy for the entirety of the reforestation pipeline. We distinguish between seedling production for post-fire planting needs and seedling production for non-fire ‘operational’ planting needs (e.g., compliance reforestation). We assume that operational planting capacity (and needs) will remain constant over the simulation period, whereas post-fire planting needs will increase based on the estimates described above. This assumption allows us to focus on fire-driven reforestation needs.
We start with 10-year (2012–2021) seedling production averages for western states from Haase et al. (2022) and assume that seedlings produced equate to seedlings planted. Further, we assume that 83% of total production comes from the private sector as per results for the western region described in Supplementary Table 2 of Fargione et al. (2021), with the remaining coming from the public sector (USFS, state, etc.). We translate seedling production numbers to total potential planting capacity in hectares based on estimated planting densities by state, which vary between 617 to 988 stems per ha (250 to 400 stems per ac) (Opalach and Arney, 2019). These values represent planting density estimates derived from surveys within the public and private sectors. These do not represent target stocking rates which may be lower than these values given expected seedling/sapling mortality or post-planting activities such as pre-commercial thinning (PCT).
We then partitioned planting capacity between post-fire and operational capacity for both the private and public sectors. To do this, we conducted a survey of organizations involved in reforestation within the region. In the private sector, we surveyed 37 participants, including TIMOs (Timber Investment Management Organizations), REITs (Real Estate Investment Trusts), and private landowners. These participants reported an average of 14% (SD = 24%) of their reforestation activities applied to post-fire planting needs. In the public sector (USFS, state agencies, etc.), 12 respondents reported an average of 55% (SD = 42%) of planting activities were for post-fire needs (Supplemental materials).
The 10-year (2012–2021) annual average seedling production for the western states was 142 million seedlings (Haase et al., 2022). Given target planting densities by state, this seedling production is enough to plant approximately 196,000 ha (401,000 ac) annually. Of this, we estimate that 34,048 ha (84,133 ac) is available annually on both public and private lands for post-fire replanting efforts after accounting for operational needs (e.g., post-harvest planting). Our estimates represent ‘potential’ planting capacity given that there are insufficient data on other facets of the pipeline that constrain planting efforts, including seed collection, outplanting capacity (labor availability), site preparation, prescription planning, or land management capacity (e.g., available foresters and restoration practitioners) across public and private ownerships. Consequently, our estimates represent an upper bound in terms of area planted.
Between 1984 and 2021, we estimate that post-fire planting capacity fell short of fire-driven needs by 1.5 million ha (ca. 3.8 million ac) (Figure 3). For comparison, the USFS reported that nationally 1.5 to 1.6 million ha (3.6–4.0 million ac) of NFS lands were in need of reforestation as of 2022 (USDA Forest Service, 2022; USDA, 2023). However, we caution against a direct comparison of these estimates given the varying geographic scope, land ownership types, and time periods examined. If planting capacity is maintained at current levels, we estimate that the cumulative gap will increase to ~3.3 million ha (8.3 million ac) by 2050, more than doubling the current gap of 1.5 million ha.
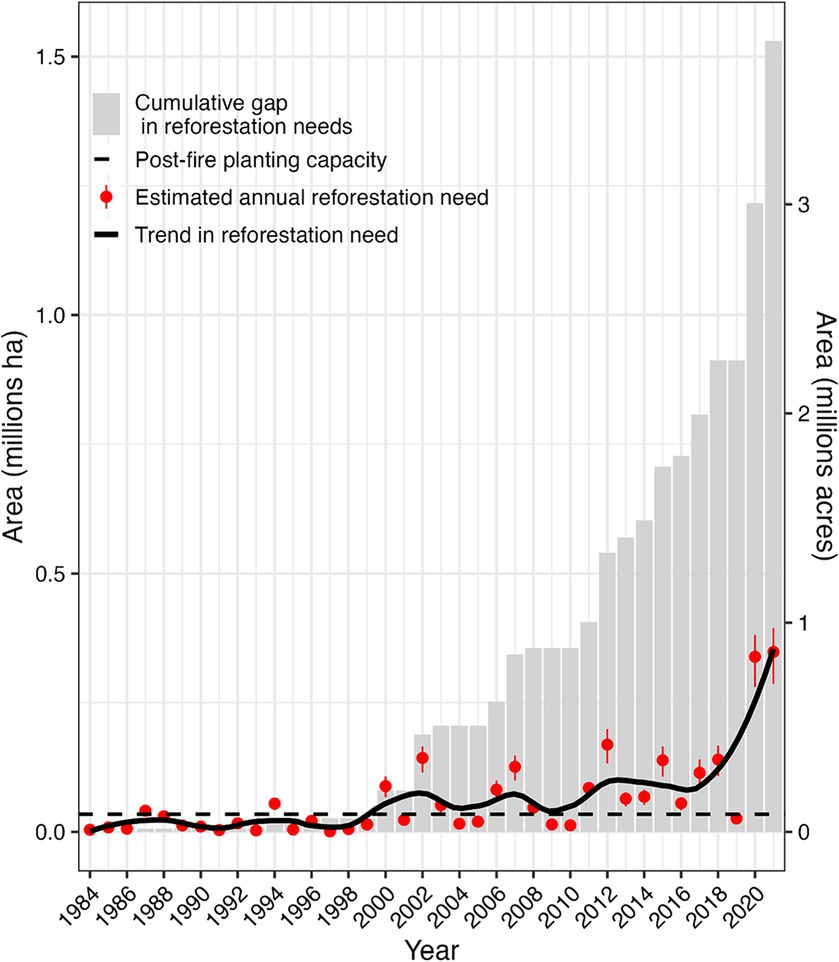
Figure 3. Cumulative difference between potential annual post-fire reforestation needs and potential post-fire planting capacity after accounting for operational (non-fire related) planting capacity for the period 1984–2021.
A geographic disparity exists between where seedlings are produced and where they are needed. Nearly all (95%) of seedling production in the western U.S. occurs in California, Oregon, and Washington, whereas wildfires in these states have resulted in 50% of the average annual post-fire planting needs since 1984. Post-fire planting needs outstrip post-fire planting capacity in all but two states – Washington and Oregon. Although some proportion of seedlings grown in Washington, Oregon, and California are shipped to other states, there remains a notable geographic disparity between total seedling production capacity and post-fire planting needs in the interior western states (Figure 4).
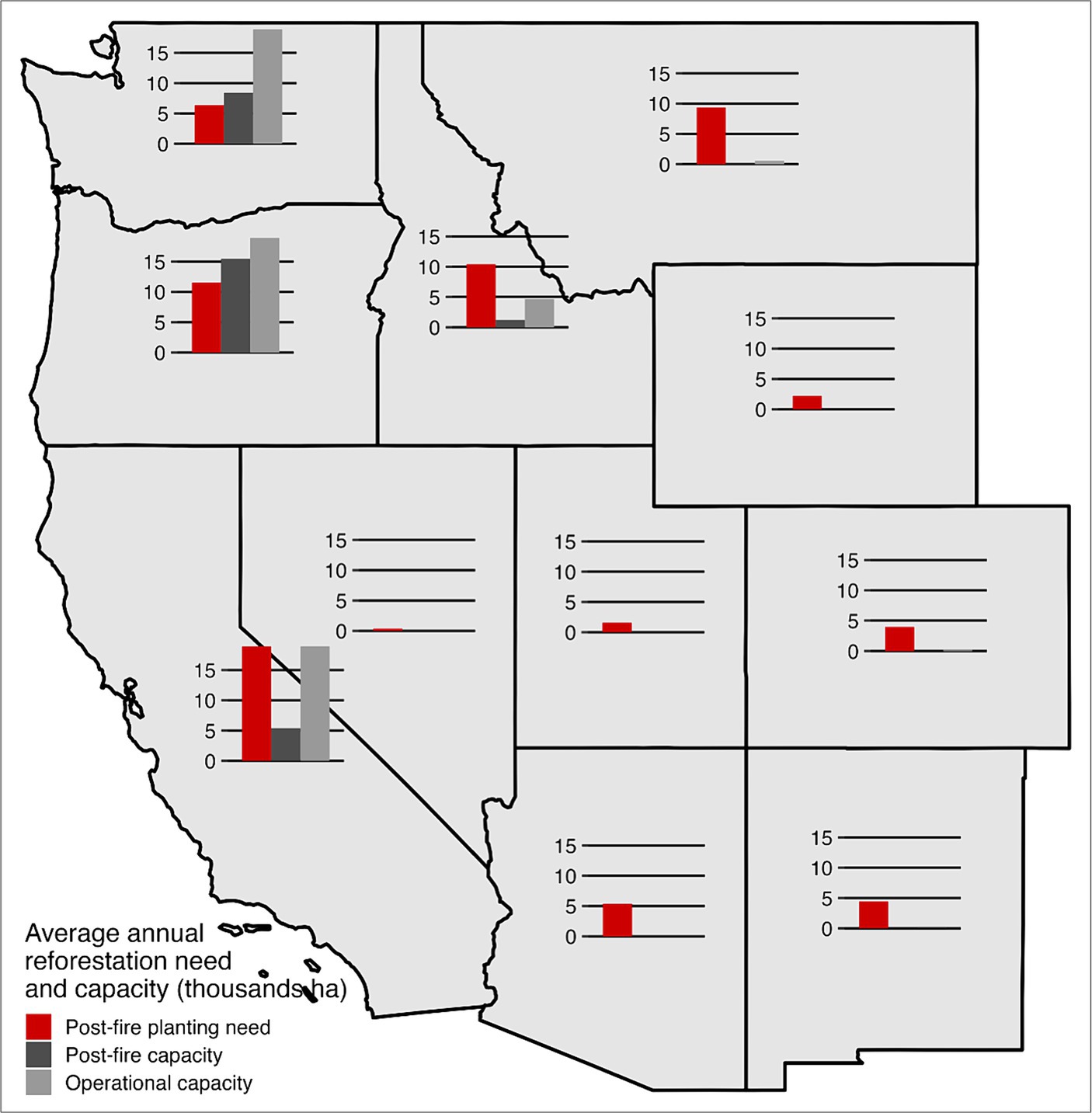
Figure 4. Average annual post-fire reforestation needs, post-fire planting capacity, and operational capacity (e.g., planting after harvests) by state show a disparity between where seedlings are produced and where post-fire planting needs have accrued. Planting capacity was estimated for the period 2012–2021 and derived from seedling production estimates by state. Post-fire potential planting needs were estimated using MTBS wildfire perimeter data.
How can we scale the reforestation supply chain to meet current and future demands?
The term “Reforestation Pipeline” includes the plant materials, logistical components, services, planning activities, and stakeholders that comprise the artificial forest regeneration supply chain (Fargione et al., 2021). The “pipeline” has historically been viewed as a component of silvicultural programs for industrial land managers but its use has become more inclusive of non-compliance needs as contemporary challenges like wildfire have grown.
The reforestation pipeline is principally supported by private enterprises. Private nurseries produce an estimated 83% of seedlings in the western U.S. (Fargione et al., 2021; Supplementary Table S2), and they provide a significant proportion of seedlings planted on federal lands through bidding on annual solicitations. Although we do not have data that resolve the proportion of privately grown seedlings planted on federal lands, there are currently only five USFS nurseries in the western states that produce an estimated 16 million seedlings a year (8% of western U.S. production). Similarly, state nurseries are limited in their production capacity. Of the 11 states examined here, seven have state-run tree nurseries (CA, CO, ID, MT, NM, NV, WA) that produce an estimated 17 million seedlings per year (9% of western U.S. production) out of the reported 142 million (10 year average) produced regionally for all ownership types (Haase et al., 2022).
Expanding the scope and inclusivity of the reforestation pipeline will require cooperation across landowners, land managers, and service providers to coordinate a wide array of operational activities (e.g., civil engineering, logging and biomass removal, site preparation, planting, monitoring, and maintenance), collection of appropriate plant materials (i.e., seed availability through orchards or wild collection and banking), seedling production (i.e., forest nurseries), and favorable site conditions. We recognize 10 steps that comprise the pipeline and 7 stakeholder categories associated with each component along various planning and execution timelines (Figure 5). The steps and the stakeholders are interdependent in a manner that directly impacts the successful implementation of a reforestation project.
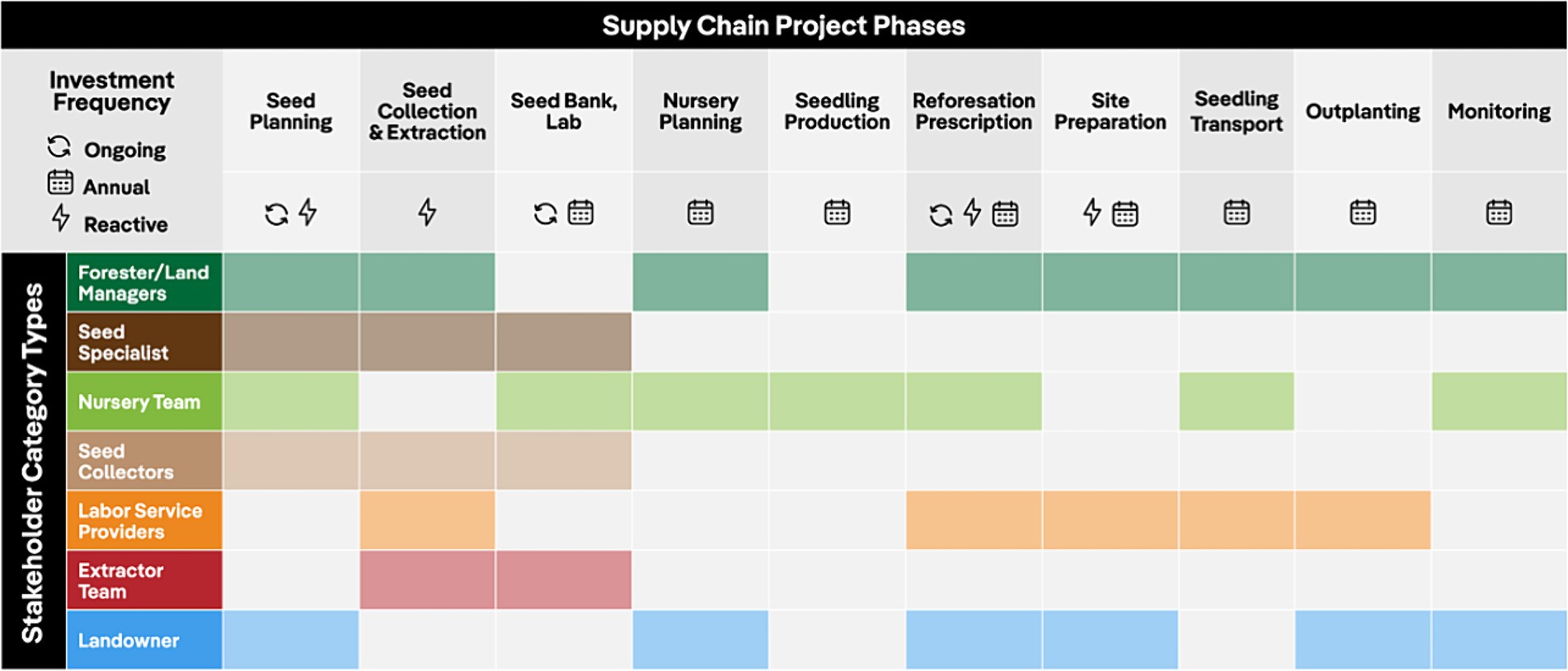
Figure 5. Requirements for artificial regeneration in the western U.S. are based on 10 steps and 7 stakeholder categories/types that provide inputs or are required for planning and implementation. Investments and activities at each step are designated as ongoing, annual, or reactive for managing a robust supply chain. Colored shading denotes stakeholder communication/engagement required for a supply chain process.
We categorize investments in the pipeline relative to the time frames in which they occur, from short-term or reactive (seasonal/annual) investments to cyclical or ongoing (e.g., decadal) investments (Figure 5). Multi-decadal investments will be needed for implementing emerging programs and policy initiatives to increase the scale of reforestation (see section below). In contrast, forest nursery crop planning, seedling production, seedling cold storage, transport, outplanting, and monitoring are distinct operations and are planned on an annual or biennium basis. Although elements of seed collection planning are reactive within the year (i.e., crop/mast event dependent), planning for seed needs for a region, seed collection, extraction, testing, and banking, requires ongoing management and staffing, base funding, equipment and facilities – all of which require a multi-year to decadal outlook (Kildisheva et al., 2023). Reforestation prescription planning is limited by available seed from appropriate provenances, and therefore timelines will vary from reactive to long-term outlooks. In general, the need for risk mitigation should catalyze the development of insurance pools, reinsurance, or other financial means to reduce the cost of mitigating losses after unplanned disturbances like wildfire.
Existing analyses of the reforestation pipeline (including ours) focus on seedling production as a proxy for reforestation capacity simply because we lack other public and private data sets on the up- and down-stream elements of the pipeline. However, seedling production is just one of many bottlenecks for scaling the pipeline. For instance, increasing seedling production without investing in workforce development and labor pools for time-sensitive site preparation and outplanting activities, will result in a glut of seedlings on the market without the capacity to plant them. For these reasons, few forest nurseries grow on speculation, even in years after major wildfire events. Similarly, the inability to conduct appropriate site preparation or plant in important climatic windows where soil moisture is conducive to root growth (Grossnickle, 2012) can result in wasted investments in reforestation planning and execution.
Compliance reforestation following harvest on industrial/managed forestlands has resulted in the development and persistence of the reforestation pipeline in regions where forests have sustained timber and fiber economies (Figure 4; Greeley, 1925; Rasker, 2021). Large TIMOs, REITs, and other timber- or fiber-generating organizations have contributed to keeping costs low by vertically integrating services and operations (e.g., seed and seedling production) or relying on large independent or regional corporate seedling producers, labor contractors, and forestry service providers. This model has sustained the supply chain while providing some additional capacity for seedling products and planting/site-preparation services for non-industrial ownerships. The downside of this model is that prices of goods and services are suppressed to meet the needs of the largest customers, often to the detriment of funding innovation, improved wage standards, and the development of small businesses in the sector. The “low cost” of reforestation is suitable for large ownerships that can leverage economies of scale but does not incentivize the development of locally derived reforestation capacity.
Current efforts to ramp up the pace and scale of reforestation would benefit from considering factors that have led to contractions of capacity, infrastructure, and technical expertise in the past. The annual harvesting cycles of industrial forestland ownerships will continue to provide materials to downstream consumers. Non-industrial ownerships competing for existing reforestation capacity will result in market turbulence if demand rises quickly, for instance, in response to large wildfires, resulting in price fluctuations which can, in turn, exacerbate bottlenecks in the supply chain like seed availability (Kildisheva et al., 2023) or labor availability for outplanting (Altieri et al., 2023). The development of net new capacity should be viewed as complementary to existing elements of the reforestation pipeline, with the ability to operate independently of the presence of industrial ownerships as primary consumers.
Further, developing new capacity will require a flexible and rapidly scalable supply chain that can accommodate the surges and contractions of demand driven by wildfire. Year-to-year variability in post-fire reforestation needs can vary significantly (Figure 1). Replanting tends to have the lowest costs and best outcomes within the first few years after disturbance (Haase et al., 2012; Webster et al., in press), but requires ongoing capacity and coordination across stakeholders (Figure 5). Consequently, a contemporary reforestation pipeline will require novel approaches to designing and implementing not only infrastructure (e.g., greenhouses), but also support seasonal or temporary labor for seedling production, site preparation, and outplanting. One possible solution may be to invest in regional hubs and more robust logistics to ensure seed collection and seedling production serve broader geographies from centralized locations. These hubs would act to maintain infrastructure, expertise, capital, and labor pools that can support reforestation ‘strike teams’ that travel regionally to where planting efforts are needed.
Third, the ongoing ability to support seed collection and seedling production from banked seed has largely been scalable due to the orthodox storage behavior of conifer species. With drying and proper cold storage conditions, conifer seed can be stored for decades with minimal impact on viability. However, a growing body of evidence suggests that wildfire and ongoing climate change in the western U.S. are promoting the expansion of hardwood species at the expense of conifers (Lenihan et al., 2003, 2008; Sheehan et al., 2015; Turner et al., 2015; Yospin et al., 2015; Case et al., 2021). As we consider the broader diversity of woody angiosperms that are important structural components of western forests, other factors may need to be considered. For example, because many important angiosperm species native to the western U.S. (e.g., many Quercus and Acer spp.) produce recalcitrant seeds, which do not maintain viability during storage and must be planted shortly after maturation, it is logistically challenging to integrate them into the standard supply chain. This will require more dynamic planning to take advantage of good seed years to ensure collection and seedling production align with site preparation and outplanting. Considerations of seed and seedling biology (and their phenologies) will be integral to developing a modern supply chain that is responsive to more diverse forest restoration objectives (Kildisheva et al., 2023) and for establishing more resilient forests.
Finally, expanding capacity across the interior western U.S. will create a more resilient reforestation pipeline for the western U.S. as a whole. The reforestation supply chain is underdeveloped in many non-coastal states with a growing backlog of planting needs (Figure 4). Although seedlings are shipped across state (and national) boundaries, most consumers require regionally sourced seed to ensure seedlings are seed zone appropriate (i.e., not maladapted to site conditions in the long term; O’Neill et al., 2017). Consumers often prefer seedling production to occur regionally for inspection, for communication between foresters (or other land managers) and nursery teams, to reduce costs of production, transport, and storage, and to improve quality assurance along the chain of custody by reducing the need for long-distance transport and cold storage. Building a resilient pipeline will be challenging in the interior western U.S. where forest productivity is lower than coastal regions and timber economies remain limited or have expired. Catalyzing an ecosystem of service providers in these regions will require novel (non-industrial forest management oriented) economies, and those supportive of ecologically based management objectives. Additionally, there are many different forest types, ownership types, and therefore contrasting management objectives across the western U.S. Progress will require that the costs of goods, services, and wages along the reforestation pipeline reflect the downstream value derived from maintaining intact forest ecosystems on our landscape – a value that extends benefits beyond the timber and fiber economy.
What approaches to reforestation can foster resilience to climate change and wildfire?
Successful artificial forest regeneration depends on the selection and availability of high-quality appropriate plant materials that meet management and ecological objectives (Dumroese et al., 2016). Seed provenance plays a critical role in the success of reforestation projects. Field trials indicate that seedlings planted outside of appropriate seed zones are maladapted and perform poorly in terms of growth and survival because of climatic differences between seed sources and planting sites (Leimu and Fischer, 2008; Alberto et al., 2013). Additionally, the broadening objectives of reforestation from timber production toward ecological services and function, climate mitigation, and resilience requires a wider array of species and seed sources to be considered for reforestation (Crowe and Parker, 2008; Clark et al., 2023; Warner et al., 2023). Many non-timber species (specifically native angiosperms, e.g., Quercus sp., Acer sp., Populus sp., etc.) do not have empirical seed zones or transfer guidelines (Pike et al., 2020; Crockett and Hurteau, 2024) which presents significant challenges to scaling reforestation, particularly in regions where there has been divestment from timber and fiber economies that previously sustained seed collection activities.
As climate conditions change, previously appropriate seed sources may be maladapted to the future climate of planting sites (St. Clair et al., 2022). Therefore, seed sources chosen for a particular outplanting site should be adapted to near-term as well as projected future climates at the planting site (O’Neill et al., 2017). Existing web-based tools like the Seedlot Selection Tool,1 Climate Adapted Seed Tool,2 and the Eastern Seed Zone Forum3 can facilitate matching seed lots with appropriate planting sites based on current and predicted climate change projections. Climate-smart planting strategies can take the form of assisted population migration or assisted species migration – the former consists of the movement of populations within the extant range of a species, whereas the latter consists of movement outside of and disjunct to the historic range of a given species (Williams and Dumroese, 2013). Current reforestation efforts principally focus on assisted population migration, but there are no clear policy frameworks that require climate-adapted planting strategies on public lands in the U.S. This lack of policy stands in contrast to Canadian forestry practices, where the British Columbia Ministry of Forests transitioned to a climate-based seed transfer system that mandates the use of assisted population migration to mitigate climate change impacts [O’Neill et al., 2008; BC Ministry of Forests, Lands, and Natural Resource Operations (MFLNRO), 2012; Pelai et al., 2021].
Enabling climate-resilient policies will require support for research on seedling performance as it relates to current and future climates. The USFS has recently initiated a network of operational seed-source trials across California, Oregon, and Washington – the Experimental Network for Assisted Migration and Establishment Silviculture.4 These trials should be expanded to a broader list of tree species and across a wider western geography to broaden insights and improve our understanding of seedling performance (O’Neill and Gómez-Pineda, 2021; Sáenz-Romero et al., 2021; Kildisheva et al., 2023). Additionally, inference from outplanting trials is limited by the lack of standardized and publicly available datasets on seed provenance and planting outcomes. To address this, a west-wide integrated network of seed source trials can be achieved by strategically selecting sites and seed sources that match desired climate ranges and establishing sites in recently burned and harvested locations. Cost-sharing, through collaborative projects that span jurisdictions can offset costs. Expanding collaborative opportunities for assisted population migration and range-expansion trials, especially on post-wildfire sites, is critical for building a knowledge base for informing regional planting guidelines.
Forest resilience to wildfire and drought can also be incorporated into reforestation practices by promoting greater heterogeneity (size class and vertical and horizontal discontinuity), in vegetation, and tree planting patterns. Historically, frequent fires in many western U.S. forests have maintained forest heterogeneity at fine scales (North et al., 2009; Moritz et al., 2011). In contrast, some contemporary wildfires produce extensive high-severity burned area with post-burn conditions that are more homogenous than historical patterns (Collins et al., 2017; Cova et al., 2023). These conditions challenge reforestation professionals to not only focus on the immediate goal of re-establishing forest cover but also to promote heterogeneity to restore mechanisms of resilience to future disturbance.
Managing post-fire biomass can present multiple competing objectives to balance landowner interests (e.g., timber vs. habitat), risks of future disturbance (i.e., persistent fuel loads), and reforestation potential (i.e., hazards, access, microclimatic conditions). High-severity wildfire combusts most of the finer fuels but can leave a concentration of large logs and snags, making tree planting difficult, hazardous, and, if left unaddressed, likely to produce lethal temperatures to seedlings in the case of a subsequent ignition (Peterson et al., 2015; Coppoletta et al., 2016). Industrial landowners often salvage log (i.e., remove standing dead trees and some down logs) immediately after a wildfire to minimize economic losses and to quickly re-establish trees ahead of germinating and resprouting shrubs. On public lands, salvage logging is subject to NEPA review (generally a 3–5 year process), in part because standing biomass and downed woody material are critical to ecosystem processes linked to biodiversity and resilience (Swanson and Franklin, 1992; Lindenmayer and Noss, 2006; Lindenmayer et al., 2012). On private, non-industrial lands, salvage logging and reforestation efforts often depend on grant funding, which may never materialize. Reforestation efforts on public and nonindustrial private lands are often limited to easily accessible areas with larger, more profitable snags (Beschta et al., 2004).
High density post-fire plantings with homogenous spatial patterns can also reduce a developing forest’s resilience to wildfire and drought (Figure 6). Tree seedlings are often planted at high densities in a regular, gridded pattern designed to optimize growth and shade out competing vegetation (Rubilar et al., 2018). Both the planting pattern and density can be detrimental to fostering resilience to future disturbance. Planting tree seedlings on a regular grid comes from an agronomic approach for maximizing growth, which has no analog in natural ecosystems (North et al., 2019). For example, in western U.S. conifer forests, a common pattern is to regularly plant seedlings every twelve feet, producing a density of 743 stems per ha (300 stems per ac) (White and Long, 2019). This regular pattern does not take advantage of wet, more shaded microsites that support higher survivorship and growth rates (Gray et al., 2005; Zald et al., 2008; Marshall et al., 2023), and lacks the spatial heterogeneity that can foster resilience to subsequent disturbance. Stands that retain high initial stem densities, are vulnerable to high burn severities (Thompson et al., 2011; Zald and Dunn, 2018) and are also at-risk during droughts. A recent analysis suggests historical forest resilience may have resulted, in part, from low tree densities that eliminated most competition and supported vigorous growth and tree defenses (North et al., 2022). The increasing size and intensity of wildfires and drought make ‘plantation-like’ reforestation practices ill-suited to current and projected climate conditions and disturbance regimes (North et al., 2019).
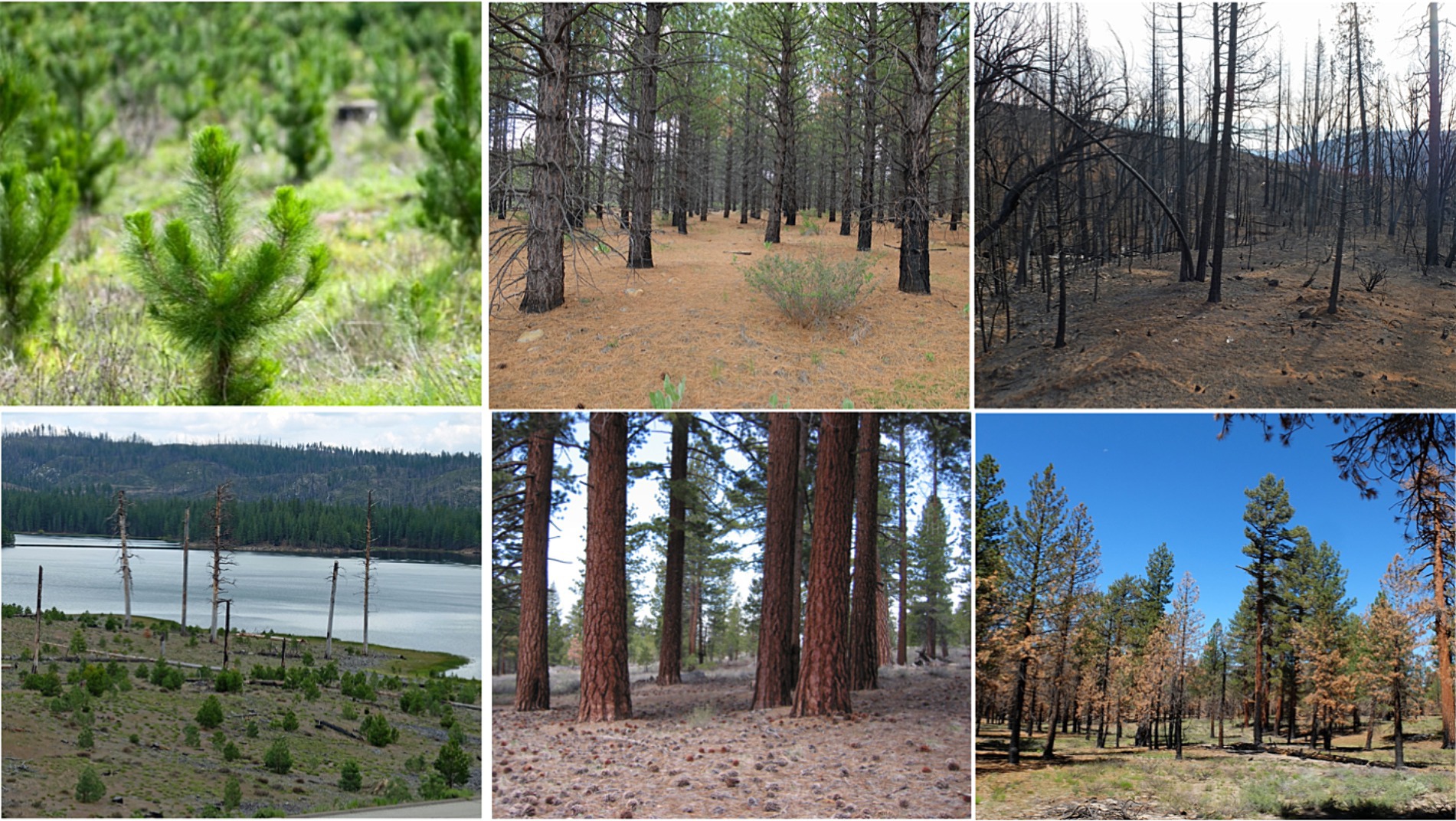
Figure 6. Which way forward? Top row from left to right: a 2-year-old gridded planting, a 60-year-old plantation, and a plantation burned in the 2021 Dixie Fire in California. Bottom row from left to right: an ‘ICO’ planting after the 2007 Moonlight fire in California, a mature tree clump in ponderosa pine with a restored fire regime, and an ICO pattern in Yosemite National Park after the 2013 Rim Fire.
Recent studies have found that historically, mature frequent-fire forests have a variable spatial structure, characterized as a combination of “Individual trees, Clumps of trees, and Openings” (ICO) (Churchill et al., 2013; Reynolds et al., 2013). Heterogeneous forest structure patterns have been shown to be more resistant to high-severity fire (Koontz et al., 2020) due to reduced surface fuels in openings and dampened wind patterns reducing convective and radiant heat transfer that can lead to crown fire (Ziegler et al., 2017, 2021). We’re not aware of studies that have directly compared fire effects in regularly spaced and ICO-patterned young forests, but the ICO pattern is commonly found in seedlings and saplings in old-growth forests with active fire regimes (Fry et al., 2014; Fertel et al., 2022; Stephens et al., 2023). Silviculturists have generally avoided planting tree seedlings in clumps assuming that inter-tree competition will reduce growth and survival rates. However, recent research focused on young (1–30 years of age) seedlings and saplings growing in clumps in fire-restored forests found higher diameter and height growth rates than individuals growing in the absence of neighboring seedlings and saplings (Fertel et al., 2022).
One concern with planting at lower densities is the resulting high light environment may favor shrubs, grasses, and other vegetation that can out compete tree seedlings for limited soil moisture. Current reforestation strategies can include chemical and mechanical treatments to eliminate or minimize shrub cover until saplings are tall enough to shade out competitors. Yet, in the warmer, drier conditions of the western U.S., increasing evidence suggests some shrubs can act to facilitate seedling survival by reducing incoming solar radiation (Crockett and Hurteau, 2022; Marsh et al., 2023) and can increase growth (Fertel et al., 2022; Goodwin and Hurteau, 2024). In place of herbicide and mechanical control approaches, planting some seedlings in clumps has the advantage of the clump holding the site and keeping shrub encroachment at bay. With this type of ‘nucleation’ planting, shrubs need not be entirely eliminated from reforestation areas. Shrubs also do better than tree seedlings in dry, shallow soil locations, and can diversify wildlife habitat and microclimate conditions (Marsh et al., 2023). Additionally, novel drought-conditioned seedling stock types are under development with the potential to improve post-transplant survival and performance, thereby reducing the planting density needed to achieve stocking targets on harsh sites faced with changing climates (Sloan et al., 2020; Pinto et al., 2023).
More broadly, reforestation projects would benefit from the consistent application of the ‘target plant concept’ (Dumroese et al., 2016; Davis and Pinto, 2021) – a framework for planting prescription development that focuses on limiting factors at outplanting sites and a feedback loop between field site and nurseries to improve planting outcomes. It accounts for planting objectives (e.g., target stocking rates), limiting factors at the site (i.e., expected mortality rates), plant materials (e.g., seed provenance and stocktype), outplanting practices, and expectations for post-planting management (e.g., pre-commercial thinning or prescribed fire) which inform initial planting density and spacing decisions. This framework is not commonly taught in silviculture or land management curricula and therefore not consistently practiced in reforestation work, to the detriment of improved outcomes.
Another means of increasing the resilience of reforested areas is with prescribed burning (North et al., 2019, 2021). Reduced planting densities and ICO planting patterns can facilitate the application of prescribed fire in young stands, which can maintain lower post-fire fuel loads in dry forests. While there are currently many limitations on the use of prescribed fire (Miller et al., 2020), some policy and administrative barriers are being addressed (Williams et al., 2024). Burning reduces surface fuels, a principal driver of fire behavior, and is effective with or without accompanying mechanical thinning at reducing crown fire potential (Agee and Skinner, 2005; Taylor et al., 2022). Compared with mechanical thinning, prescribed fire is more cost-effective (Hessein, 2000; North et al., 2012), maintains the evolutionary selection for fire-resistant trees [both species and individuals (Beaty and Taylor, 2007; Stevens et al., 2020)], and produces greater structural heterogeneity (Knapp et al., 2017). Prescribed burns can be timed to occur after recent rain events when rapid soil water uptake by shrubs (Royce and Barbour, 2001) can reduce fire intensity and increase post-burn heterogeneity. Factors affecting fire behavior in young conifer stands are markedly different than in mature stands (Weatherspoon and Skinner, 1995; Lyons-Tinsley and Peterson, 2012), and applying standard burn prescriptions developed for mature stands may not be successful. Nonetheless, studies have shown that fire can be applied in stands as young as 15 years old (Peterson et al., 2007; Kobziar et al., 2009; Knapp et al., 2011; Reiner et al., 2012; Bellows et al., 2016; York et al., 2021).
Lastly, whether sites have become marginal or unable to support forests under current or near future environmental conditions is an important consideration given changing climate and disturbance regimes. This is likely to change over time as a function of changing climate conditions and the physiological tolerances of the tree species targeted for reforestation (Lalor et al., 2023; Crockett and Hurteau, 2024). Mature trees can withstand a wider range of environmental conditions than seedlings; the presence of adult trees does not ensure that seedlings of the same species will survive and thrive at that site (Dobrowski et al., 2015). With fire suppression, forests have expanded into areas where they may not be viable under new climate conditions and fire regimes. Lower elevation forest cover is likely to decrease as the climate warms (Davis et al., 2019; Parks et al., 2019), and trying to reforest in these areas may be a waste of scarce resources.
Where are opportunities emerging from recent policy initiatives, innovative public-private partnerships, and natural capital markets?
As wildfire activity has increased, public and private land managers increasingly rely on active reforestation to ensure the recovery of forests after wildfire. Federal support for these efforts was recently included in the Infrastructure Investment and Jobs Act (IIJA), signed into law in 2021, and the Inflation Reduction Act (IRA), signed into law in 2022 (U.S. Congress, 2021a, 2022). Within the IIJA, the Repairing Existing Public Land by Adding Necessary Trees (REPLANT) Act eliminated the annual $30 million cap on the Reforestation Trust Fund, which effectively increased funds for reforestation on federal lands to $140 million or more per year (U.S. Congress, 2021b). In addition, it requires the USFS to eliminate the reforestation backlog on NFS lands within ten years (U.S. Congress, 2021a,b, 2022). The IIJA also provides USDA and the Department of Interior (DOI) with a total of $2.13 billion for ecosystem restoration programs, which includes $200 million to establish and implement a national revegetation effort, a national seed strategy, and $450 million for post-fire restoration. Reforestation investments in the IRA include $1.5 billion for urban and community forestry grants and $450 million for grants to support small and underserved landowners in participating in emerging markets for climate mitigation or forest resilience. In addition, the IRA provides agricultural conservation funding for private landowners that can be used for reforestation.
In 2022, Executive Order 14072 on Strengthening the Nation’s Forests, Communities, and Local Economies established a new federal policy for scaling up and optimizing climate-smart reforestation. It requires the Secretaries of the Interior and Agriculture to develop (i) a Federal goal to meet agency-specific reforestation targets by 2030, including an assessment of reforestation opportunities on Federal lands and through existing Federal programs and partnerships, and (ii) in collaboration with Federal, State, Tribal, and private-sector partners, a climate-informed plan to increase Federal seed collection and to ensure seed and seedling capacity is sufficient to meet anticipated reforestation demand (DOI and USDA, 2023).
While these laws make significant investments in reforestation on public and private lands through 2026, only the REPLANT Act permanently increases funding for reforestation on federal lands. Yet this level of funding is likely not sufficient to address the full scope of the reforestation backlog that has developed in the past 20 years or potential future needs.
Reforestation costs vary widely, and a lack of data on costs across planting objectives and ownership types presents barriers to delivering on proposed reforestation targets. Generally, reforestation costs are a function of site-preparation requirements (i.e., terrain, accessibility, post-fire biomass volume, and existing vegetation), species, seed availability, stocktype, planting density, planting methodology, and post-planting treatments. In particular, pre- and post-planting activities such as post-wildfire salvage logging, site preparation (mechanical and/or chemical), post-planting shrub control, and pre-commercial thinning (PCT) are often critical to long-term project success (Mousavijad et al., 2022), but are not consistently implemented on some federal plantings (North et al., 2019).
We estimate planting costs based on data from a 2019 survey from the Forest Biometrics Research Institute (FBRI), which summarizes silvicultural costs for both public and private land managers in the western U.S. (Opalach and Arney, 2019). The FBRI reforestation cost estimates range between $1,272 to $2,586 per ha ($515 to $1,047 per ac), depending on the nature and extent of pre- and post-planting activities. Using a midpoint, we assume a scenario of $1,929 per ha ($781 per ac) which includes site-prep, primary shrub control, and PCT. After adjusting for inflation, we estimate average costs to be $2,351 per ha ($951 per ac) in 2023. These estimates do not account for additional site preparation costs to reduce standing dead and downed materials in post-fire environments due to a lack of publicly available data on post-wildfire restoration costs. This can be an untenable expense for landowners if there is an absence of receptive mills or locations that will accept biomass to offset project costs.
Additionally, the cost of seed collection varies significantly by species, seed zone, and collection technique and is poorly captured by a bundled per-acre cost in survey data (like the FBRI data). The efficiency of seed collection from seed orchards allows for stockpiling seed for commercially important species within a limited range of provenances. Broader reforestation objectives including more diverse and climate-adapted plantings often require wild seed collection, with inherent risks of mixed seed quality and availability, and more complex supply chain logistics that increase the cost of banked seed. We also anticipate seed costs will rise as demand stretches the limited labor pool of skilled seed collectors.
Our analysis suggests that between 1984 and 2021, wildfire created approximately 1.5 million ha (3.8 million ac) of unmet post-fire reforestation needs in the western U.S., and this trend will likely accelerate in the coming decades. Given these numbers, $3.6 billion is an initial conservative estimate to cover the existing backlog of reforestation needs based on the per-acre costs described above. However, this estimate does not address additional investments in infrastructure or workforce capacity necessary to expand supply chain capacity to meet these objectives. Large wildfire seasons will increasingly stress the existing reforestation pipeline, increasing the need for rapid capital expenditures that are not within the scope of public funding initiatives or budget planning cycles of conventional forest managers.
Federal land managers have limited funding options as they seek to close the reforestation gap. Existing funding streams include funds received from the Reforestation Trust Fund and the Knutson-Vanderberg (K-V) Fund, from cooperative work agreements with partners, and through the annual budget and appropriations cycle (USDA Forest Service, 2023; USDA, 2024). Reducing the need for reforestation is also an integral part of a cohesive reforestation strategy. The IIJA invests $3.4 billion for wildfire risk reduction on 10 million acres of high-risk federal lands. As the impacts of a changing climate accelerate, sustained capacity and funding are needed to ensure that federal agencies can respond to time critical reforestation needs. As wildfires become more prevalent, federal agencies will be faced with a choice to embrace new ways of financing resource management on public lands (Quesnel Seipp et al., 2023) or persuade Congress to increase annual appropriations to match the scale of the problem.
Reforestation requires substantial up-front capital, while carbon, water, and biodiversity benefits accrue over time. Monetization of carbon as an ecosystem service began in 1997, with the signing of the Kyoto Protocol, an international effort to reduce carbon emission activities below a business-as-usual baseline. Forest carbon projects generate numerous co-benefits and natural capital markets have emerged in an effort to assign monetary value to a broad range of ecosystem services. The intention is for market participants to pay for these services (Kelemen et al., 2022; Sandoval-Solis et al., 2022) with the resulting finance used to support restoration activities. While these markets are nascent, they offer potential for landowners to generate future revenue from reforestation, distinct from traditional funding streams such as timber or fiber. These transactions can occur in emerging markets (Reig et al., 2019), through voluntary platforms (Kreibich and Hermwille, 2021; American Carbon Registry, 2024; Climate Action Reserve, 2024; Verra, 2024), or to meet regulatory requirements (Bang et al., 2017).
Within natural capital markets, carbon markets have the greatest potential to finance reforestation due to global interest in climate change mitigation. There are broadly two carbon credit classifications: ex-post credits and ex-ante credits. Ex-post credits are issued after the removal of carbon has occurred and is measured. U.S.-based ex-post reforestation carbon projects have not been broadly adopted, in part due to the slow growth rates of some North American forests, which result in comparatively long-time horizons for reforestation credit generation compared to other forest carbon project types (i.e., improved forest management and avoided deforestation) (Matzek et al., 2015). To address this barrier to adoption, the ex-ante credit was developed (Climate Action Reserve, Verra). Ex-ante credits are issued based on projections of future carbon removal (Climate Forward, 2023) and allow for up-front financing of reforestation projects. Ex-ante credits have also not been widely adopted. For example, the Integrity Council for the Voluntary Carbon Market (ICVCM) has indicated that ex-ante credits do not meet their Core Carbon Principle eligibility (The Integrity Council for the Voluntary Carbon Market, 2024). To increase rigor and buyer confidence, ex-ante credits are generally paired with a process to convert them to ex-post credits as a replanted forest grows and actual carbon removals are measured and verified. To date, capital is generally available to fund projects on productive sites with growth rates that support returns on investment over time frames that align with traditional investor expectations. Fire-impacted lands in the interior western U.S. may not meet these expectations, however, as they have high post-fire site preparation costs and longer time horizons for ex-post credit generation due to lower site productivity.
Landowners and land managers also face an array of barriers to participating in carbon markets (Stubbs et al., 2021; USDA, 2023). There are significant issues related to developing carbon projects on federal lands, including legal barriers to generating offsets, issues with achieving additionality given existing statutory management requirements, and administrative barriers within existing agency policies, regulations, and land management plans (Smith, 2012). Consequently, USDA is not directly engaged in carbon markets (Stubbs et al., 2021). Beyond federal lands, the Growing Climate Solutions Act, signed into law in December 2022 as a part of the Consolidated Appropriations Act of 2023, authorizes USDA to develop and implement a Greenhouse Gas Technical Assistance Provider and Third-Party Verifier Program to assist landowners interested in participating in carbon markets. Policy proposals to address financial barriers to participating in carbon markets include federal tax incentives (Mulligan et al., 2020; Stubbs et al., 2021; Food and Agriculture Climate Alliance (FACA), 2023) and federal loan guarantees to reduce the cost of capital for developers of grouped projects (U.S. Congress, 2023).
Natural capital markets also have shortcomings with respect to scaling reforestation in a manner that is effective for climate mitigation while avoiding potential negative impacts. For example, current and emerging protocols reward developers for planting fast-growing, dense plantations that maximize standing biomass (D’Amato et al., 2022). However, such plantations also increase the risk of wildfire losses and thus reduce the durability of carbon stocks (Hurteau et al., 2019; North et al., 2021). Further, market-based mechanisms have not historically rewarded nor incentivized ecologically informed reforestation practices due to the costs of implementation and the complexity of monitoring, reporting, and verification (MRV). Forest carbon projects are based on carbon standards that currently rely on growth and yield models developed for silvicultural regimes that align with commercial timber or fiber production. These models were not designed to simulate irregular, clumped, or spatially complex planting regimes (e.g., ICO methodology). As a result, this may incentivize project developers to prescribe reforestation plans that have fewer species and less structural and functional diversity in favor of meeting verification standards and maximizing carbon yields (D’Amato et al., 2022). Despite these shortcomings, there are clear pathways and principles for mitigating climate impacts by restoring forests while avoiding negative impacts (Griscom et al., 2017; Fargione et al., 2018; Ellis et al., 2024) including significant opportunities for reforestation in the western U.S. (Cook-Patton et al., 2020).
Debt markets have also become a national-level tool to direct finance toward reforestation. Countries, banks, and private institutions are exploring the use of ‘green bonds’, or bond issuances, that are tied to climate-positive or fire-resilient outcomes (Maltais and Nykvist, 2020). Green bonds and other innovative financing mechanisms like the Forest Resilience Bond (Blue Forest Conservation, 2017) can provide capital to implement work quickly and to generate new sources of funding from entities who would benefit from positive environmental and economic outcomes from reforestation (Madeira and Gartner, 2018; Mandle et al., 2019).
The outcomes attributed to reforestation include: recovered carbon storage (Looney et al., 2023), stabilized slopes to reduce erosion (González-Romero et al., 2022), sustained water supply, improved surface water quality (Keller and Fox, 2019), reduced flood risk (Papaioannou et al., 2023), improved snowpack storage and retention (Dickerson-Lange et al., 2021), maintained habitat for species of concern (Kemppinen et al., 2020), and others. Many entities stand to benefit from these outcomes, including electric and water utilities that operate on or downstream of projects, private companies with climate resilience, carbon removal, or water replenishment goals, and entities interested in providing recreation and tourism opportunities (Quesnel Seipp et al., 2023). These novel sources of funding can be paired with traditional state and federal funding programs to achieve the complete financial requirements for projects to be fully implemented.
Payments from stakeholders that benefit from reforestation, called beneficiaries, can be financed upfront to implement environmental restoration projects (Brand et al., 2021). This upfront deployment of capital ensures that projects can be implemented quickly, which is often critical for post-fire planting due to the negative influences of competing vegetation on seedling survival and growth (Stevens et al., 2021). Additionally, the effects of reforestation and associated activities last for decades, thus enabling a structure of outcome-based payments, in which beneficiaries can pay for projects over time as they see positive outcomes.
To leverage these new sources of funding, quantification of outcomes is often required. Outcomes need to be both projected over time and verified following implementation. A project developer and beneficiary paying for outcomes will need to agree upon the methods used to predict and verify these benefits, and, in the case of a pay-for-performance structure, they will need to identify which outcome benchmarks will be sought by the beneficiary prior to payment (Brand et al., 2021). Quantification and modeling of outcomes can be one of the most challenging steps of developing a conservation finance project, as tools available to evaluate outcomes may be resource intensive, have unsuitable levels of uncertainty, or may not be available for the exact application desired. However, western U.S. forests have relevant advantages in that they exist within structured markets and regulatory environments and are easily accessed for MRV activities. Additionally, industry and agency investments in allometric data sets for growth and yield models, along with the USFS Forest Inventory and Analysis (FIA) program (Heath et al., 2011; Westfall et al., 2022), support the ongoing improvement and validation of growth and yield models and their inclusion in multiple carbon registry standards. There is, of course, room for improvement and it will be important to develop better models, evaluation tools, and standardized practices. In the meantime, the inherent uncertainty involved in modeling outcomes should be acknowledged throughout the process of financing reforestation projects.
Conservation finance provides additional opportunities and benefits through public-private partnerships. To be able to finance larger reforestation projects, and cover financing costs, new funding from entities like utilities and private companies can partner with traditional sources of funding from state and federal agencies to access private capital markets and deploy significant upfront capital. Further, diversified financing can provide all elements of the supply chain an opportunity to increase production capacity. The medium- to long-term planning horizons required for building capacity for seed collection, nursery facilities and operations, and transportation/logistics often present challenges. With increased certainty provided by consistently financed and implemented projects, investments in local and regional nurseries can build up workforce, facilities, and operations to meet current and growing reforestation needs.
Summary and conclusions
We examined potential post-fire reforestation needs given historic and anticipated increases in the extent and severity of wildfires, highlighting the specific stressors, historical context, and opportunities that shape both the supply and demand sides of the reforestation pipeline in the western U.S. We estimate that between 1984 and 2021, high-severity wildfire created 2.4 million ha (5.9 million ac) of potential reforestation needs outside of wilderness areas and national parks; 26% of these needs were created by the 20 largest wildfires, all of which have occurred since 2000. The trend toward larger and more severe wildfire events brings the problem into focus; even two large wildfires, such as the 2021 Dixie fire (CA) or 2020 August Complex fire (CA), can create more reforestation needs in a single year than the entire western U.S. reforestation pipeline can respond to for that year.
The capacity to conduct reforestation at scale has principally been supported by private organizations working in the compliance forestry space. As investments in the timber and fiber economy have declined during the past 50 years, so has the capacity to plant trees for non-compliance needs – including in reaction to high-severity wildfire in areas where natural regeneration is unlikely. This divestment has manifested as a 1.5 million ha (ca. 3.8 million ac) gap between post-fire needs and post-fire planting capacity- a gap that has increased exponentially with the growth of severe wildfires since 2000. Additionally, a geographic gap has grown between where seed collection and seedling production is supported by an active forestry sector and where wood and fiber production have declined but wildfire has increased the need for reforestation materials and activities. These often semi-arid and low-productivity forests are also areas of greatest concern for ecological state-shifts after wildfires (Davis et al., 2019; Parks et al., 2019; Coop et al., 2020) and represent the greatest risk of divestment in local economies and communities.
The national discourse on how best to overcome barriers to increasing reforestation capacity has largely focused on seedling production capacity through nursery development and increasing seed availability through scaling collections (Fargione et al., 2021; Klein, 2021; American Forests, 2023; Clark et al., 2023; Kildisheva et al., 2023). Further innovation will require attention to a wider range of activities in the reforestation pipeline that are often overlooked (Figure 5). A key challenge will be to develop financing structures and workforces that can respond readily to the dramatic inter-annual variability of wildfire-driven reforestation needs while simultaneously supporting long-term infrastructure. Recent federal policy initiatives may partially address these challenges, but ephemeral funding cycles from policy initiatives have not historically sustained the capitalization required to manage healthy forests for non-timber, ecosystem-based objectives. Along with expanded appropriations on public lands, natural capital markets and green bonds can be important potential sources of finance for incentivizing and sustaining the recovery of forested ecosystems.
Much like wood and fiber economies incentivized the development and maturation of a reforestation supply chain, new economic drivers can modernize the reforestation pipeline and align tree planting efforts with broader ecosystem resilience and climate mitigation goals. Incentivizing tree planting using conservation finance can expand the reforestation pipeline. However, leveraging conservation finance to fund reforestation requires actions that promote wildfire-resilient landscapes so that western U.S. forests will continue to provide the ecosystem services those funds are predicated on. These investments, coupled with innovative reforestation approaches that include the use of climate-adapted seed zones, increased species diversity, and planting densities and patterns that more closely emulate natural fire-resistant stand structures, will increase the resilience of our forests into the future. Innovation and investments will require data-sharing structures (e.g., regional cooperative groups, online reporting platforms, centralized repositories) that will enable improved outcomes. These investments are critical to ensuring a functional reforestation supply chain in the short term and enabling the maintenance of our forests and local communities in the long term.
The changing patterns and scale of wildfire in the western U.S. is increasing the imperative to plant more trees, yet the challenge of scaling and sustaining this effort looms large. Time is not on our side. The area burned in the western U.S. is increasing with drought, warming, and human activities. Making progress will require public-private partnerships and leadership that extends beyond the conventional stakeholders of the reforestation pipeline – those invested in the production of wood and fiber. A robust call to action warrants the engagement of policymakers, corporations, financial institutions, investors, NGOs, and all those who stand to benefit from the value that trees and forests bring to the western U.S. and beyond.
Data availability statement
The original contributions presented in the study are included in the article/Supplementary material, further inquiries can be directed to the corresponding author.
Ethics statement
Ethical approval was not required for the studies involving humans because Survey was conducted by Mast Reforestation, a private company as part of their market research. No identifying information was collected. The participants provided their informed consent to participate in this study.
Author contributions
SD: Conceptualization, Data curation, Formal analysis, Methodology, Project administration, Resources, Supervision, Writing – original draft, Writing – review & editing. MA: Conceptualization, Data curation, Investigation, Methodology, Project administration, Resources, Supervision, Writing – original draft, Writing – review & editing. AC: Writing – review & editing. RD: Writing – review & editing. JF: Writing – review & editing. DH: Writing – review & editing. TH: Formal analysis, Writing – review & editing. OK: Writing – review & editing. AM: Writing – review & editing. SN: Writing – review & editing. MN: Writing – review & editing. PS: Writing – review & editing. MS: Writing – review & editing. TB: Writing – review & editing. MB: Writing – review & editing. MC: Writing – review & editing. LG-K: Writing – review & editing. BH: Writing – review & editing. MH: Writing – review & editing. JL: Writing – review & editing. HS: Writing – review & editing. JS: Writing – review & editing.
Funding
The author(s) declare financial support was received for the research, authorship, and/or publication of this article. Support for this project was provided by the R&D program at Mast Reforestation. SD was supported by USDA NIFA grant # 2022-67019-36438, the McIntire-Stennis program at the University of Montana, and Mast Reforestation.
Acknowledgments
We thank Dan Opalach of the Forest Biometrics Research Institute for providing insight into data on the costs of reforestation. We thank Shanna Hobbs of Mast Reforestation for supporting the compilation of survey results. Lastly, we thank Corrine Mock for generating Figure 5.
Conflict of interest
The authors declare that the research was conducted in the absence of any commercial or financial relationships that could be construed as a potential conflict of interest.
Publisher’s note
All claims expressed in this article are solely those of the authors and do not necessarily represent those of their affiliated organizations, or those of the publisher, the editors and the reviewers. Any product that may be evaluated in this article, or claim that may be made by its manufacturer, is not guaranteed or endorsed by the publisher.
Supplementary material
The Supplementary material for this article can be found online at: https://www.frontiersin.org/articles/10.3389/ffgc.2024.1402124/full#supplementary-material
Footnotes
1. ^https://seedlotselectiontool.org/sst/
2. ^https://reforestationtools.org/climate-adapted-seed-tool/
3. ^https://easternseedzones.com
4. ^https://www.fs.usda.gov/research/pnw/projects/enames#overview
References
Abatzoglou, J. T., Battisti, D. S., Williams, A. P., Hansen, W. D., Harvey, B. J., Kolden, C. A., et al. (2021). Projected increases in Western US Forest fire despite growing fuel constraints. Commun. Earth Environ. 2:227. doi: 10.1038/s43247-021-00299-0
Adlam, C., Almendariz, D., Goode, R. W., Martinez, D. J., and Middleton, B. R. (2022). Keepers of the flame: supporting the revitalization of indigenous cultural burning. Soc. Nat. Resour. 35, 575–590. doi: 10.1080/08941920.2021.2006385
Agee, J. K., and Skinner, C. N. (2005). Basic principles of Forest fuel reduction treatments. For. Ecol. Manag. 211, 83–96. doi: 10.1016/j.foreco.2005.01.034
Alberto, F. J., Aitken, S. N., Alía, R., González-Martínez, S. C., Hänninen, H., Kremer, A., et al. (2013). Potential for evolutionary responses to climate change – evidence from tree populations. Glob. Chang. Biol. 19, 1645–1661. doi: 10.1111/gcb.12181
Allen, C. D., Macalady, A. K., Chenchouni, H., Bachelet, D., McDowell, N., Vennetier, M., et al. (2010). A global overview of drought and heat-induced tree mortality reveals emerging climate change risks for forests. For. Ecol. Manag. 259, 660–684. doi: 10.1016/j.foreco.2009.09.001
Altieri, G., Downer, R., and Aghai, M. (2023). Outplanting seedlings in the Pacific northwest: historical efforts and contemporary constraints to success. Tree Planters’ Notes 66, 28–43. Available at: https://rngr.net/publications/tpn/66-1/outplanting-seedlings-in-the-pacific-northwest-historical-efforts-and-contemporary-constraints-to-success
American Carbon Registry. (2024). Available at: https://acrcarbon.org/ (Accessed February 20, 2024)
American Forests. (2023). American forests partners with USDA Forest Service to expand reforestation across national forests. https://www.americanforests.org/article/american-forests-partners-with-usda-forest-service-to-expand-reforestation-across-national-forests/ (Accessed January 31, 2024)
Anderegg, W. R. L., Chegwidden, O. S., Badgley, G., Trugman, A. T., Cullenward, D., Abatzoglou, J. T., et al. (2022). Future climate risks from stress, insects and fire across US forests. Ecol. Lett. 25, 1510–1520. doi: 10.1111/ele.14018
Anderegg, W. R. L., Trugman, A. T., Badgley, G., Anderson, C. M., Bartuska, A., Ciais, P., et al. (2020). Climate-driven risks to the climate mitigation potential of forests. Science 368:eaaz7005. doi: 10.1126/science.aaz7005
Balloffet, N., and Dumroese, R. K. (2022). “The National Reforestation Strategy and the REPLANT act: growing and nurturing resilient forests” in Foundational concepts in silviculture with emphasis on reforestation and early stand improvement – 2022 National Silviculture Workshop Proc. RMRS-P-80. eds. T. B. Jain and T. M. Schuler (Fort Collins, CO: Department of Agriculture, Forest Service, Rocky Mountain Research Station).
Bang, G., Victor, D. G., and Anderson, S. (2017). California’s cap-and-trade system: diffusion and lessons. Global Environ. Politics 17, 12–30. doi: 10.1162/GLEP_a_00413
Bare, B. B., and Waggener, T. R. (1980). Forest land values and return on investment. For. Sci. 26, 91–96. doi: 10.1093/forestscience/26.1.91
BC Ministry of Forests, Lands, and Natural Resource Operations (MFLNRO). (2012). Climate Change Adaptation: transitioning to a climate-based (Forest tree) genetic resource conservation and management system in British Columbia. Available at: https://www2.gov.bc.ca/assets/gov/farming-natural-resources-and-industry/forestry/tree-seed/climate-based-seed-transfer/cbst_projectcharter_july2012.pdf (Accessed February 20, 2024)
Beaty, R. M., and Taylor, A. H. (2007). Fire disturbance and Forest structure in old-growth mixed conifer forests in the northern Sierra Nevada, California. J. Veg. Sci. 18, 879–890. doi: 10.1111/j.1654-1103.2007.tb02604.x
Bellows, R. S., Thomson, A. C., Helmstedt, K. J., York, R. A., and Potts, M. D. (2016). Damage and mortality patterns in Young mixed conifer plantations following prescribed fires in the Sierra Nevada, California. For. Ecol. Manag. 376, 193–204. doi: 10.1016/j.foreco.2016.05.049
Beschta, R. L., Rhodes, J. J., Kauffman, J. B., Gresswell, R. E., Minshall, G. W., Karr, J. R., et al. (2004). Postfire management on forested public lands of the Western United States. Conserv. Biol. 18, 957–967. doi: 10.1111/j.1523-1739.2004.00495.x
Blue Forest Conservation (2017). Fighting fire with finance: A roadmap for collective action. Sacramento, CA: Blue Forest Conservation, 102.
Brand, M. W., Quesnel Seipp, K., Saksa, P., Ulibarri, N., Bomblies, A., Mandle, L., et al. (2021). Environmental impact bonds: a common framework and looking ahead. Environ. Res. 1:23001. doi: 10.1088/2634-4505/ac0b2c
Buonanduci, M. S., Donato, D. C., Halofsky, J. S., Kennedy, M. C., and Harvey, B. J. (2023). Consistent spatial scaling of high-severity wildfire can inform expected future patterns of burn severity. Ecol. Lett. 26, 1687–1699. doi: 10.1111/ele.14282
Burke, M., Driscoll, A., Heft-Neal, S., Xue, J., Burney, J., and Wara, M. (2021). The changing risk and burden of wildfire in the United States. Proc. Natl. Acad. Sci. 118:e2011048118. doi: 10.1073/pnas.2011048118
Campbell, R. M., Anderson, N. M., Daugaard, D. E., and Naughton, H. T. (2018). Financial viability of biofuel and biochar Production from Forest biomass in the face of market Price volatility and uncertainty. Appl. Energy 230, 330–343. doi: 10.1016/j.apenergy.2018.08.085
Case, M. J., Johnson, B. G., Bartowitz, K. J., and Hudiburg, T. W. (2021). Forests of the future: climate change impacts and implications for carbon storage in the Pacific northwest, USA. For. Ecol. Manag. 482:118886. doi: 10.1016/j.foreco.2020.118886
Chambers, M. E., Fornwalt, P. J., Malone, S. L., and Battaglia, M. A. (2016). Patterns of conifer regeneration following high severity wildfire in ponderosa pine – dominated forests of the Colorado front range. For. Ecol. Manag. 378, 57–67. doi: 10.1016/j.foreco.2016.07.001
Churchill, D. J., Larson, A. J., Dahlgreen, M. C., Franklin, J. F., Hessburg, P. F., and Lutz, J. A. (2013). Restoring Forest resilience: from reference spatial patterns to Silvicultural prescriptions and monitoring. For. Ecol. Manag. 291, 442–457. doi: 10.1016/j.foreco.2012.11.007
Clark, P. W., D’Amato, A. W., Palik, B. J., Woodall, C. W., Dubuque, P. A., Edge, G. J., et al. (2023). A lack of ecological diversity in Forest nurseries limits the achievement of tree-planting objectives in response to global change. Bioscience 73, 575–586. doi: 10.1093/biosci/biad049
Climate Action Reserve. (2024). Available at: https://www.climateactionreserve.org/ (Accessed February 20, 2024)
Climate Forward (2023). Reforestation forecast methodology version 2.0: Errata and clarifications. Los Angeles, CA: Climate Forward, a program of Climate Action Reserve, 79.
Collins, B. M., Stevens, J. T., Miller, J. D., Stephens, S. L., Brown, P. M., and North, M. P. (2017). Alternative characterization of Forest fire regimes: incorporating spatial patterns. Landsc. Ecol. 32, 1543–1552. doi: 10.1007/s10980-017-0528-5
Cook-Patton, S. C., Leavitt, S. M., Gibbs, D., Harris, N. L., Lister, K., Anderson-Teixeira, K. J., et al. (2020). Mapping carbon accumulation potential from global natural Forest regrowth. Nature 585, 545–550. doi: 10.1038/s41586-020-2686-x
Coop, J. D., Parks, S. A., Stevens-Rumann, C. S., Crausbay, S. D., Higuera, P. E., Hurteau, M. D., et al. (2020). Wildfire-driven Forest conversion in Western North American landscapes. Bioscience 70, 659–673. doi: 10.1093/biosci/biaa061
Coppoletta, M., Merriam, K. E., and Collins, B. M. (2016). Post-fire vegetation and fuel development influences fire severity patterns in Reburns. Ecol. Appl. 26, 686–699. doi: 10.1890/15-0225
Cova, G., Kane, V. R., Prichard, S., North, M., and Cansler, C. A. (2023). The outsized role of California’s largest wildfires in changing Forest burn patterns and coarsening ecosystem scale. For. Ecol. Manag. 528:120620. doi: 10.1016/j.foreco.2022.120620
Crockett, J. L., and Hurteau, M. D. (2022). Post-fire early successional vegetation buffers surface microclimate and increases survival of planted conifer seedlings in the southwestern United States. Can. J. For. Res. 52, 416–425. doi: 10.1139/cjfr-2021-0221
Crockett, J. L., and Hurteau, M. D. (2024). Ability of seedlings to survive heat and drought portends future demographic challenges for five southwestern US conifers. Tree Physiol. 44:136. doi: 10.1093/treephys/tpad136
Crowe, K. A., and Parker, W. H. (2008). Using portfolio theory to guide reforestation and restoration under climate change scenarios. Clim. Chang. 89, 355–370. doi: 10.1007/s10584-007-9373-x
D’Amato, A. W., Woodall, C. W., Weiskittel, A. R., Littlefield, C. E., and Murray, L. T. (2022). Carbon conundrums: do United States’ current carbon market baselines represent an undesirable ecological threshold? Glob. Chang. Biol. 28, 3991–3994. doi: 10.1111/gcb.16215
Davis, K. T., Dobrowski, S. Z., Higuera, P. E., Holden, Z. A., Veblen, T. T., Rother, M. T., et al. (2019). Wildfires and climate change push low-elevation forests across a critical climate threshold for tree regeneration. Proc. Natl. Acad. Sci. 116, 6193–6198. doi: 10.1073/pnas.1815107116
Davis, K. T., Higuera, P. E., and Sala, A. (2018). Anticipating fire-mediated impacts of climate change using a demographic framework. Funct. Ecol. 32, 1729–1745. doi: 10.1111/1365-2435.13132
Davis, A. S., and Pinto, J. R. (2021). The scientific basis of the target plant concept: an overview. Forests 12:1293. doi: 10.3390/f12091293
Davis, K. T., Robles, M. D., Kemp, K. B., Higuera, P. E., Chapman, T., Metlen, K. L., et al. (2023). Reduced fire severity offers near-term buffer to climate-driven declines in conifer resilience across the Western United States. Proc. Natl. Acad. Sci. 120:e2208120120. doi: 10.1073/pnas.2208120120
Dickerson-Lange, S. E., Vano, J. A., Gersonde, R., and Lundquist, J. D. (2021). Ranking Forest effects on snow storage: a decision tool for Forest management. Water Resour. Res. 57:e2020WR027926. doi: 10.1029/2020WR027926
Dinerstein, E., Joshi, A. R., Vynne, C., Lee, A. T. L., Pharand-Deschênes, F., França, M., et al. (2020). A ‘global safety net’ to reverse biodiversity loss and stabilize Earth’s climate. Sci. Adv. 6:eabb2824. doi: 10.1126/sciadv.abb2824
Dobrowski, S. Z., Littlefield, C. E., Lyons, D. S., Hollenberg, C., Carroll, C., Parks, S. A., et al. (2021). Protected-area targets could be undermined by climate change-driven shifts in ecoregions and biomes. Commun. Earth Environ. 2, 1–11. doi: 10.1038/s43247-021-00270-z
Dobrowski, S. Z., Swanson, A. K., Abatzoglou, J. T., Holden, Z. A., Safford, H. D., Schwartz, M. K., et al. (2015). Forest structure and species traits mediate projected recruitment declines in Western US tree species. Glob. Ecol. Biogeogr. 24, 917–927. doi: 10.1111/geb.12302
DOI and USDA. (2023). U.S. Department of the Interior and U.S. Department of Agriculture: reforestation goals and assessments, and a climate-informed plan to increase Federal Seed and nursery capacity. As directed by executive Order 14072. Available at: https://www.usda.gov/sites/default/files/documents/joint-reforestation-report.pdf (Accessed February 20, 2024)
Domke, G. M., Oswalt, S. N., Walters, B. F., and Morin, R. S. (2020). Tree planting has the potential to increase carbon sequestration capacity of forests in the United States. Proc. Natl. Acad. Sci. 117, 24649–24651. doi: 10.1073/pnas.2010840117
Donato, D. C., Fontaine, J. B., Campbell, J. L., Robinson, W. D., Kauffman, J. B., and Law, B. E. (2009). Conifer regeneration in stand-replacement portions of a large mixed-severity wildfire in the Klamath–Siskiyou Mountains. Can. J. For. Res. 39, 823–838. doi: 10.1139/X09-016
Donofrio, S., and Calderon, C. (2023). Paying for quality: State of the voluntary carbon markets. Washington, DC: Ecosystem Marketplace.
Dumroese, K., Balloffet, N., Crockett, J. W., Stanturf, J. A., and Nave, L. E. (2019). A National Approach to leverage the benefits of tree planting on public lands. New For. 50, 1–9. doi: 10.1007/s11056-019-09703-2
Dumroese, K. R., Landis, T. D., Pinto, J. R., Haase, D. L., Wilkinson, K. W., and Davis, A. S. (2016). Meeting Forest restoration challenges: using the target plant concept. Reforesta 1, 37–52. doi: 10.21750/REFOR.1.03.3
Ellis, P. W., Page, A. M., Wood, S., Fargione, J., Masuda, Y. J., Denney, V. C., et al. (2024). The principles of natural climate solutions. Nat. Commun. 15:547. doi: 10.1038/s41467-023-44425-2
Fargione, J. E., Bassett, S., Boucher, T., Bridgham, S. D., Conant, R. T., Cook-Patton, S. C., et al. (2018). Natural climate solutions for the United States. Sci. Adv. 4:eaat1869. doi: 10.1126/sciadv.aat1869
Fargione, J., Haase, D. L., Burney, O. T., Kildisheva, O. A., Edge, G., Cook-Patton, S. C., et al. (2021). Challenges to the reforestation pipeline in the United States. Front. Forests Global Change 4:629198. doi: 10.3389/ffgc.2021.629198
Fertel, H. M., North, M. P., Latimer, A. M., and Ng, J. (2022). Growth and spatial patterns of natural regeneration in Sierra Nevada mixed-conifer forests with a restored fire regime. For. Ecol. Manag. 519:120270. doi: 10.1016/j.foreco.2022.120270
Food and Agriculture Climate Alliance (FACA) (2023). Farm bill policy priorities recommendations to the 118th Congress. Food Agric. Clim. Alliance. 30, 1–30.
Fry, D. L., Stephens, S. L., Collins, B. M., North, M. P., Franco-Vizcaíno, E., and Gill, S. J. (2014). Contrasting spatial patterns in active-fire and fire-suppressed Mediterranean climate old-growth mixed conifer forests. PLoS One 9:e88985. doi: 10.1371/journal.pone.0088985
Gan, J., Kolison, S. H., and Colletti, J. P. (2001). Optimal Forest stock and harvest with valuing non-timber benefits: a Case of US coniferous forests. Forest Policy Econ. 2, 167–178. doi: 10.1016/S1389-9341(01)00051-X
Giebink, C. L., Domke, G. M., Fisher, R. A., Heilman, K. A., Moore, D. J. P., DeRose, R. J., et al. (2022). The policy and ecology of Forest-based climate mitigation: challenges, needs, and opportunities. Plant Soil 479, 25–52. doi: 10.1007/s11104-022-05315-6
Gill, N. S., Hoecker, T. J., and Turner, M. G. (2021). The propagule Doesn’t fall far from the tree, especially after short-interval, High-Severity Fire. Ecology 102:e03194. doi: 10.1002/ecy.3194
González-Romero, J., López-Vicente, M., Gómez-Sánchez, E., Peña-Molina, E., Galletero, P., Plaza-Álvarez, P., et al. (2022). Post-fire management effects on hillslope-stream sediment connectivity in a Mediterranean Forest ecosystem. J. Environ. Manag. 316:115212. doi: 10.1016/j.jenvman.2022.115212
Goodwin, M. J., and Hurteau, M. D. (2024). The effect of shrub cover on conifer water-use patterns, growth, and response to precipitation variability in the southern Sierra Nevada. Tree Physiol. 44:tpae004. doi: 10.1093/treephys/tpae004
Gray, A. N., Zald, H. S. J., Kern, R. A., and North, M. (2005). Stand conditions associated with tree regeneration in Sierran mixed-conifer forests. For. Sci. 51, 198–210. doi: 10.1093/forestscience/51.3.198
Greeley, W. B. (1925). The relation of geography to timber supply. Econ. Geogr. 1, 1–14. doi: 10.2307/140095
Griscom, B. W., Adams, J., Ellis, P. W., Houghton, R. A., Lomax, G., Miteva, D. A., et al. (2017). Natural climate solutions. Proc. Natl. Acad. Sci. 114, 11645–11650. doi: 10.1073/pnas.1710465114
Grossnickle, S. C. (2012). Why seedlings survive: influence of plant attributes. New For. 43, 711–738. doi: 10.1007/s11056-012-9336-6
Haase, D. L., Pike, C. C., Enebak, C., Mackey, L., Ma, Z., Silva, C., et al. (2022). Forest nursery seedling production in the United States—fiscal year 2021. Tree Planters Notes. 65, 79–86. Available at: https://rngr.net/publications/tpn/55-1/development-and-distribution-of-planted-seedlings-naturally-regenerated-seedlings-and-competing-vegetation-6-years-after-wildfire
Haase, D. L., Rose, R., and Henneman, D. (2012). Development and distribution of planted seedlings, naturally regenerated seedlings, and competing vegetation 6 years after wildfire. Tree Planters’ Notes 55, 37–44
Hagmann, R. K., Hessburg, P. F., Prichard, S. J., Povak, N. A., Brown, P. M., Fulé, P. Z., et al. (2021). Evidence for widespread changes in the structure, composition, and fire regimes of Western North American forests. Ecol. Appl. 31:e02431. doi: 10.1002/eap.2431
Harvey, B. J., Buonanduci, M. S., and Turner, M. G. (2023). Spatial interactions among short-interval fires reshape Forest landscapes. Glob. Ecol. Biogeogr. 32, 586–602. doi: 10.1111/geb.13634
Heath, L. S., Smith, J. E., Woodall, C. W., Azuma, D. L., and Waddell, K. L. (2011). Carbon stocks on forestland of the United States, with emphasis on USDA Forest Service ownership. Ecosphere 2, art6–art21. doi: 10.1890/ES10-00126.1
Hemes, K. S., Norlen, C. A., Wang, J. A., Goulden, M. L., and Field, C. B. (2023). The magnitude and pace of photosynthetic recovery after wildfire in California ecosystems. Proc. Natl. Acad. Sci. 120:e2201954120. doi: 10.1073/pnas.2201954120
Hessburg, P. F., Prichard, S. J., Hagmann, R. K., Povak, N. A., and Lake, F. K. (2021). Wildfire and climate change adaptation of Western North American forests: a Case for intentional management. Ecol. Appl. 31:e02432. doi: 10.1002/eap.2432
Hessein, H. (2000). The economics of prescribed burning: a research review. For. Sci. 46, 322–334. doi: 10.1093/forestscience/46.3.322
Hoover, K., and Riddle, A. A. (2021). U.S. Forest ownership and management: Background and issues for Congress. Washington, DC: Congressional Research Service, 44.
Hurteau, M. D., North, M. P., Koch, G. W., and Hungate, B. A. (2019). Managing for disturbance stabilizes Forest carbon. Proc. Natl. Acad. Sci. 116, 10193–10195. doi: 10.1073/pnas.1905146116
Iglesias, V., Balch, J. K., and Travis, W. R. (2022). U.S. fires became larger, more frequent, and more widespread in the 2000s. Sci. Adv. 8:eabc0020. doi: 10.1126/sciadv.abc0020
IPCC (2023) in Climate change 2023: Synthesis report. Contribution of working groups I, II and III to the sixth assessment report of the intergovernmental panel on climate change. eds. H. Lee and J. Romero (Geneva: IPCC).
Karjalainen, E., Sarjala, T., and Raitio, H. (2010). Promoting human health through forests: overview and major challenges. Environ. Health Prev. Med. 15, 1–8. doi: 10.1007/s12199-008-0069-2
Keegan, C. E., Sorenson, C. B., Morgan, T. A., Hayes, S. W., and Daniels, J. M. (2011). Impact of the great recession and housing collapse on the Forest products industry in the Western United States. For. Prod. J. 61, 625–634. doi: 10.13073/0015-7473-61.8.625
Kelemen, E., Subramanian, S., Nakangu, B., Islar, M., Kosmus, M., Nuesiri, E., et al. (2022). “Chapter 6: policy options and capacity development to operationalize the inclusion of diverse values of nature in decision-making” in Methodological assessment report on the diverse values and valuation of nature of the intergovernmental science-policy platform on biodiversity and ecosystem services (Bonn, Germany: IPBES Secretariat).
Keller, A. A., and Fox, J. (2019). Giving credit to reforestation for water quality benefits. PLoS One 14:e0217756. doi: 10.1371/journal.pone.0217756
Kemp, K. B., Higuera, P. E., and Morgan, P. (2016). Fire legacies impact conifer regeneration across environmental gradients in the U.S. Northern Rockies. Landscape Ecol. 31, 619–636. doi: 10.1007/s10980-015-0268-3
Kemppinen, K. M. S., Collins, P. M., Hole, D. G., Wolf, C., Ripple, W. J., and Gerber, L. R. (2020). Global reforestation and biodiversity conservation. Conserv. Biol. 34, 1221–1228. doi: 10.1111/cobi.13478
Kildisheva, O., Hobbs, S., Dowbrowski, S., Sloan, J., Shaw, N., and Aghai, M. (2023). Got seeds? Strengthening the reforestation pipeline in the Western United States. Tree Planters’ Notes. 66, 4–17. Available at: https://rngr.net/publications/tpn/66-1/got-seeds-strengthening-the-reforestation-pipeline-in-the-western-united-states/?searchterm=kildisheva%202023%20got%20seeds
Klein, J. (2021). Reforestation is great! But We’re Running out of seeds. Wired, April 6th, 2021. Available at: https://www.wired.com/story/reforestation-is-great-but-were-running-out-of-seeds/
Knapp, E. E., Lydersen, J. M., North, M. P., and Collins, B. M. (2017). Efficacy of variable density thinning and prescribed fire for restoring Forest heterogeneity to mixed-conifer Forest in the Central Sierra Nevada, CA. For. Ecol. Manag. 406, 228–241. doi: 10.1016/j.foreco.2017.08.028
Knapp, E. E., Varner, J. M., Busse, M. D., Skinner, C. N., and Shestak, C. J. (2011). Behaviour and effects of prescribed fire in masticated Fuelbeds. Int. J. Wildland Fire 20, 932–945. doi: 10.1071/WF10110
Kobziar, L. N., McBride, J. R., and Stephens, S. L. (2009). The efficacy of fire and fuels reduction treatments in a Sierra Nevada pine plantation. Int. J. Wildland Fire 18, 791–801. doi: 10.1071/WF06097
Koontz, M. J., North, M. P., Werner, C. M., Fick, S. E., and Latimer, A. M. (2020). Local Forest structure variability increases resilience to wildfire in dry Western U.S. Coniferous Forests. Ecol. Lett. 23, 483–494. doi: 10.1111/ele.13447
Kreibich, N., and Hermwille, L. (2021). Caught in between: credibility and feasibility of the voluntary carbon market post-2020. Clim. Pol. 21, 939–957. doi: 10.1080/14693062.2021.1948384
Lalor, A. R., Law, D. J., Breshears, D. D., Falk, D. A., Field, J. P., Loehman, R. A., et al. (2023). Mortality thresholds of juvenile trees to drought and heatwaves: implications for Forest regeneration across a landscape gradient. Front. Forests Global Change 6:1198156. doi: 10.3389/ffgc.2023.1198156
Laughlin, M. M., Rangel-Parra, L. K., Morris, J. E., Donato, D. C., Halofsky, J. S., and Harvey, B. J. (2023). Patterns and drivers of early conifer regeneration following stand-replacing wildfire in Pacific northwest (USA) temperate maritime forests. For. Ecol. Manag. 549:121491. doi: 10.1016/j.foreco.2023.121491
Law, B. E., Berner, L. T., Buotte, P. C., Mildrexler, D. J., and Ripple, W. J. (2021). Strategic Forest reserves can protect biodiversity in the Western United States and mitigate climate change. Commun. Earth Environ. 2:254. doi: 10.1038/s43247-021-00326-0
Leimu, R., and Fischer, M. (2008). A Meta-analysis of local adaptation in plants. PLoS One 3:e4010. doi: 10.1371/journal.pone.0004010
Lenihan, J. M., Bachelet, D., Neilson, R. P., and Drapek, R. (2008). Response of vegetation distribution, ecosystem productivity, and fire to climate change scenarios for California. Clim. Chang. 87, 215–230. doi: 10.1007/s10584-007-9362-0
Lenihan, J. M., Drapek, R., Bachelet, D., and Neilson, R. P. (2003). Climate change effects on vegetation distribution, carbon, and fire in California. Ecol. Appl. 13, 1667–1681. doi: 10.1890/025295
Lindenmayer, D. B., Burton, P. J., and Franklin, J. F. (2012). Salvage logging and its ecological consequences. Washington, DC: Island Press.
Lindenmayer, D. B., and Noss, R. F. (2006). Salvage logging, ecosystem processes, and biodiversity conservation. Conserv. Biol. 20, 949–958. doi: 10.1111/j.1523-1739.2006.00497.x
Liu, N., Caldwell, P. V., Dobbs, G. R., Miniat, C. F., Bolstad, P. V., Nelson, S. A. C., et al. (2021). Forested lands dominate drinking water supply in the conterminous United States. Environ. Res. Lett. 16:084008. doi: 10.1088/1748-9326/ac09b0
Looney, C. E., Stewart, J. A. E., and Wood, K. E. A. (2023). Mixed-provenance plantings and climatic transfer-distance affect the early growth of Knobcone-Monterey hybrid pine, a fire-resilient alternative for reforestation. New For. 55, 543–565. doi: 10.1007/s11056-023-09991-9
Lyons-Tinsley, C., and Peterson, D. L. (2012). Surface fuel treatments in Young, regenerating stands affect wildfire severity in a mixed conifer Forest, eastside Cascade Range, Washington, USA. For. Ecol. Manag. 270, 117–125. doi: 10.1016/j.foreco.2011.04.016
Madeira, L., and Gartner, T. (2018). Forest resilience bond Sparks innovative collaborations between water utilities and wide-ranging stakeholders. Am. Water Works Assoc. 110, 42–49. doi: 10.1002/awwa.1097
Maltais, A., and Nykvist, B. (2020). Understanding the role of green bonds in advancing sustainability. J. Sustain. Finance Investment, 1–20. doi: 10.1080/20430795.2020.1724864
Mandle, L., Ouyang, Z., Salzman, J. E., and Daily, G. (Eds.) (2019). Green growth that works: Natural capital policy and finance mechanisms from around the world. Washington, DC: Island Press/Center for Resource Economics.
Marsh, C., Blankinship, J. C., and Hurteau, M. D. (2023). Effects of nurse shrubs and biochar on planted conifer seedling survival and growth in a high-severity burn patch in New Mexico, USA. For. Ecol. Manag. 537:120971. doi: 10.1016/j.foreco.2023.120971
Marshall, L. A., Fornwalt, P. J., Stevens-Rumann, C. S., Rodman, K. C., Rhoades, C. C., Zimlinghaus, K., et al. (2023). North-facing aspects, shade objects, and microtopographic depressions promote the survival and growth of tree seedlings planted after wildfire. Fire Ecol. 19:26. doi: 10.1186/s42408-023-00181-8
Matzek, V., Puleston, C., and Gunn, J. (2015). Can carbon credits fund riparian forest restoration? Restor. Ecol. 23, 7–14. doi: 10.1111/rec.12153
Miller, R. K., Field, C. B., and Mach, K. J. (2020). Barriers and enablers for prescribed burns for wildfire Management in California. Nat. Sustain. 3, 101–109. doi: 10.1038/s41893-019-0451-7
Moritz, M. A., Hessburg, P. F., and Povak, N. A. (2011). “Native fire regimes and landscape resilience” in The landscape ecology of fire. eds. D. McKenzie, C. Miller, and D. A. Falk (Dordrecht, Netherlands: Springer), 51–86.
Mousavijad, M., LeBe, L., Lehoux, N., and Cloutier, C. (2022). Review of reforestation value chain planning and management: a conceptual framework. Trees Forests People 8:100258. doi: 10.1016/j.tfp.2022.100258
MTBS. (2023). MTBS. Available at: https://www.mtbs.gov/ (Accessed January 30, 2024)
Mulligan, J., Rudee, A., Lebling, K., Levin, K., Anderson, J., and Christensen, B. (2020). CarbonShot: Federal Policy Options for carbon removal in the United States. Washington, DC: World Resources Institute.
Nave, L. E., Walters, B. F., Hofmeister, K. L., Perry, C. H., Mishra, U., Domke, G. M., et al. (2019). The role of reforestation in carbon sequestration. New For. 50, 115–137. doi: 10.1007/s11056-018-9655-3
Neary, D. G., Ice, G. G., and Jackson, C. R. (2009). Linkages between Forest soils and water quality and quantity. For. Ecol. Manag. 258, 2269–2281. doi: 10.1016/j.foreco.2009.05.027
Nelson, S., Bigger, P., Elias, M., and Schuldt, A. (2022). High roads to resilience: building equitable forest restoration economies in California and beyond. Vancouver, BC: University of British Columbia Centre for Climate Justice, 63.
North, M., Brough, A., Long, J., Collins, B., Bowden, P., Yasuda, D., et al. (2015). Constraints on mechanized treatment significantly limit mechanical fuels reduction extent in the Sierra Nevada. J. For. 113, 40–48. doi: 10.5849/jof.14-058
North, M., Collins, B. M., and Stephens, S. (2012). Using fire to increase the scale, benefits, and future maintenance of fuels treatments. J. For. 110, 392–401. doi: 10.5849/jof.12-021
North, M. P., Stevens, J. T., Greene, D. F., Coppoletta, M., Knapp, E. E., Latimer, A. M., et al. (2019). Tamm review: reforestation for resilience in dry Western U.S. Forests. Forest Ecol. Manag. 432, 209–224. doi: 10.1016/j.foreco.2018.09.007
North, M., Stine, P., O’Hara, K., Zielinski, W., and Stephens, S. (2009). An ecosystem management strategy for Sierran mixed-conifer forests. Albany, CA: U.S. Department of Agriculture, Forest Service, Pacific southwest Research Station.
North, M. P., Tompkins, R. E., Bernal, A. A., Collins, B. M., Stephens, S. L., and York, R. A. (2022). Operational resilience in Western US frequent-fire forests. For. Ecol. Manag. 507:120004. doi: 10.1016/j.foreco.2021.120004
North, M. P., York, R. A., Collins, B. M., Hurteau, M. D., Jones, G. M., Knapp, E. E., et al. (2021). Pyrosilviculture needed for landscape resilience of dry Western United States forests. J. For. 119, 520–544. doi: 10.1093/jofore/fvab026
Nowak, D. J., Hirabayashi, S., Bodine, A., and Greenfield, E. (2014). Tree and Forest effects on air quality and human health in the United States. Environ. Pollut. 193, 119–129. doi: 10.1016/j.envpol.2014.05.028
O’Neill, G. A., and Gómez-Pineda, E. (2021). Local was best: sourcing tree seed for future climates. Can. J. For. Res. 51, 1432–1439. doi: 10.1139/cjfr-2020-0408
O’Neill, G. A., Ukrainetz, N. K., Carlson, M. R., Cartwright, C. V., Jaquish, B. C., King, J. N., et al. (2008). Assisted migration to address climate change in British Columbia: Recommendations for interim seed transfer standards. Victoria, B.C.: B.C. Ministry of Forests and Range Research Branch. Tech. Rep, 48.
O’Neill, G., Wang, T., Ukrainetz, N., Charleson, L., McAuley, L., Yanchuk, A., et al. (2017). A proposed climate-based seed transfer system for British Columbia. Victoria, BC: Province of British Columbia.
Opalach, D., and Arney, J. (2019). The results of a 2019 Silviculture and harvesting cost survey. Portland, OR: Forest Biometrics Research Institute, 46.
Palik, B. J., Clark, P. W., D’Amato, A. W., Swanston, C., and Nagel, L. (2022). Operationalizing forest-assisted migration in the context of climate change adaptation: examples from the eastern USA. Ecosphere 13:e4260. doi: 10.1002/ecs2.4260
Pan, Y., Birdsey, R. A., Fang, J., Houghton, R., Kauppi, P. E., Kurz, W. A., et al. (2011). A large and persistent carbon sink in the world’s forests. Science 333, 988–993. doi: 10.1126/science.1201609
Pan, Y., Birdsey, R. A., Phillips, O. L., and Jackson, R. B. (2013). The structure, distribution, and biomass of the world’s forests. Annu. Rev. Ecol. Evol. Syst. 44, 593–622. doi: 10.1146/annurev-ecolsys-110512-135914
Papaioannou, G., Alamanos, A., and Maris, F. (2023). Evaluating post-fire Erosion and flood protection techniques: a narrative review of applications. GeoHazards 4, 380–405. doi: 10.3390/geohazards4040022
Parks, S. A., and Abatzoglou, J. T. (2020). Warmer and drier fire seasons contribute to increases in area burned at high severity in Western US forests from 1985 to 2017. Geophys. Res. Lett. 47:858. doi: 10.1029/2020GL089858
Parks, S. A., Dobrowski, S. Z., Shaw, J. D., and Miller, C. (2019). Living on the Edge: trailing Edge forests at risk of fire-facilitated conversion to non-Forest. Ecosphere 10:e02651. doi: 10.1002/ecs2.2651
Pelai, R., Hagerman, S. M., and Kozak, R. (2021). Seeds of change? Seed transfer governance in British Columbia: insights from history. Can. J. For. Res. 51, 326–338. doi: 10.1139/cjfr-2020-0235
Peterson, D. W., Dodson, E. K., and Harrod, R. J. (2015). Post-fire logging reduces surface Woody fuels up to four decades following wildfire. For. Ecol. Manag. 338, 84–91. doi: 10.1016/j.foreco.2014.11.016
Peterson, D. W., Hessburg, P. F., Salter, B., Dahlgreen, M. C., and Barnes, J. A. (2007). “Reintroducing fire in regenerated dry forests following stand-replacing wildfire” in Restoring fire-adapted ecosystems: Proceedings of the 2005 national silviculture workshop. ed. R. F. Powers (Albany, CA: Pacific Southwest Research Station, Forest Service, U.S. Department of Agriculture), 79–86.
Pike, C., Potter, K. M., Berrang, P., Crane, B., Baggs, J., Leites, L., et al. (2020). New seed-collection zones for the eastern United States: the eastern seed zone forum. J. For. 118, 444–451. doi: 10.1093/jofore/fvaa013
Pinto, J. R., Sloan, J. L., Ervan, G., and Burney, O. T. (2023). Physiological and morphological responses of Pinus ponderosa seedlings to moisture limitations in the nursery and their implications for restoration. Front. Plant Sci. 14:1127656. doi: 10.3389/fpls.2023.1127656
Prichard, S. J., Hessburg, P. F., Hagmann, R. K., Povak, N. A., Dobrowski, S. Z., Hurteau, M. D., et al. (2021). Adapting Western North American forests to climate change and wildfires: 10 common questions. Ecol. Appl. 31:e02433. doi: 10.1002/eap.2433
Quesnel Seipp, K., Maurer, T., Elias, M., Saksa, P., Keske, C., Oleson, K., et al. (2023). A multi-benefit framework for funding Forest Management in Fire-Driven Ecosystems across the Western U.S. J. Environ. Manag. 344:118270. doi: 10.1016/j.jenvman.2023.118270
Rasker, R. (2021). The transition from Western timber dependence: Lessons for counties. Bozeman, MT: Headwaters Economics, 36.
Reig, P., Larson, W., Vionnet, S., and Bayart, J. B. (2019). Volumetric water benefit accounting (VWBA): A method for implementing and valuing water stewardship activities. Washington, DC: World Resources Institute.
Reiner, A. L., Vaillant, N. M., and Dailey, S. N. (2012). Mastication and prescribed fire influences on tree mortality and predicted fire behavior in ponderosa pine. West. J. Appl. For. 27, 36–41. doi: 10.1093/wjaf/27.1.36
Reynolds, R. T., Sánchez Meador, A. J., Youtz, J. A., Nicolet, T., Matonis, M. S., and Jackson, P. L. (2013). Restoring composition and structure in southwestern frequent-fire forests: A science-based framework for improving ecosystem resiliency. Fort Collins, CO: U.S. Department of Agriculture, Forest Service, Rocky Mountain Research Station.
Rodman, K. C., Veblen, T. T., Battaglia, M. A., Chambers, M. E., Fornwalt, P. J., Holden, Z. A., et al. (2020). A changing climate is snuffing out post-fire recovery in montane forests. Glob. Ecol. Biogeogr. 29, 2039–2051. doi: 10.1111/geb.13174
Royce, E. B., and Barbour, M. G. (2001). Mediterranean climate effects. I. conifer Water use across a Sierra Nevada ecotone. Am. J. Bot. 88, 911–918. doi: 10.2307/2657044
Rubilar, R. A., Allen, H. L., Fox, T. R., Cook, R. L., Albaugh, T. J., and Campoe, O. C. (2018). Advances in Silviculture of intensively managed plantations. Curr. For. Rep. 4, 23–34. doi: 10.1007/s40725-018-0072-9
Sáenz-Romero, C., O’Neill, G., Aitken, S. N., and Lindig-Cisneros, R. (2021). Assisted migration field tests in Canada and Mexico: lessons, limitations, and challenges. Forests 12:19. doi: 10.3390/f12010009
Safford, H. D., and Vallejo, V. R. (2019). “Chapter 12 – ecosystem management and ecological restoration in the Anthropocene: integrating global change, soils, and disturbance in boreal and Mediterranean forests” in Developments in soil science. eds. M. Busse, C. P. Giardina, D. M. Morris, and D. S. Page-Dumroese, vol. 36 (Amsterdam, Netherlands: Elsevier), 259–308.
Sandoval-Solis, S., Paladino, S., Garza-Diaz, L. E., Nava, L. F., Friedman, J. R., Ortiz-Partida, J. P., et al. (2022). Environmental flows in the Rio Grande – Rio Bravo Basin. Ecol. Soc. 27:20. doi: 10.5751/ES-12944-270120
Sheehan, T., Bachelet, D., and Ferschweiler, K. (2015). Projected major fire and vegetation changes in the Pacific northwest of the conterminous United States under selected CMIP5 climate futures. Ecol. Model. 317, 16–29. doi: 10.1016/j.ecolmodel.2015.08.023
Sloan, J. L., Burney, O. T., and Pinto, J. R. (2020). Drought-conditioning of quaking Aspen (Populus tremuloides Michx.) seedlings during nursery production modified seedling anatomy and physiology. Front. Plant Sci. 11:557894. doi: 10.3389/fpls.2020.557894
Smith, G. (2012). Forest offset projects on Federal Lands. Los Angeles, CA: Climate Action Reserve White Paper.
St. Clair, J. B., Richardson, B. A., Stevenson-Molnar, N., Howe, G. T., Bower, A. D., Erickson, V. J., et al. (2022). Seedlot selection tool and climate-smart restoration tool: web-based tools for sourcing seed adapted to future climates. Ecosphere 13:e4089. doi: 10.1002/ecs2.4089
Stanturf, J. A., Palik, B. J., and Dumroese, R. K. (2014). Contemporary Forest restoration: a review emphasizing function. For. Ecol. Manag. 331, 292–323. doi: 10.1016/j.foreco.2014.07.029
Steel, Z. L., Koontz, M. J., and Safford, H. D. (2018). The changing landscape of wildfire: burn pattern trends and implications for California’s yellow pine and mixed conifer forests. Landsc. Ecol. 33, 1159–1176. doi: 10.1007/s10980-018-0665-5
Stephens, S. L., Steel, Z. L., Collins, B. M., Fry, D. L., Gill, S. J., Rivera-Huerta, H., et al. (2023). Climate and fire impacts on tree recruitment in mixed conifer forests in northwestern Mexico and California. Ecol. Appl. 33:e2844. doi: 10.1002/eap.2844
Stevens, J. T., Haffey, C. M., Coop, J. D., Fornwalt, P. J., Yocom, L., Allen, C. D., et al. (2021). Tamm review: Postfire landscape Management in Frequent-Fire Conifer Forests of the southwestern United States. For. Ecol. Manag. 502:119678. doi: 10.1016/j.foreco.2021.119678
Stevens, J. T., Kling, M. M., Schwilk, D. W., Varner, J. M., and Kane, J. M. (2020). Biogeography of fire regimes in Western U.S. Conifer forests: a trait-based approach. Glob. Ecol. Biogeogr. 29, 944–955. doi: 10.1111/geb.13079
Stevens-Rumann, C. S., and Morgan, P. (2019). Tree regeneration following wildfires in the Western US: a review. Fire Ecol. 15:15. doi: 10.1186/s42408-019-0032-1
Stewart, J. A. E., van Mantgem, P. J., Young, D. J. N., Shive, K. L., Preisler, H. K., Das, A. J., et al. (2021). Effects of Postfire climate and seed availability on Postfire conifer regeneration. Ecol. Appl. 31:e02280. doi: 10.1002/eap.2280
Stubbs, M., Hoover, K., and Ramseur, J. L. (2021). Agriculture and forestry offsets in carbon markets: Background and selected issues. Washington, DC: Congressional Research Service, R46956.
Swanson, F. J., and Franklin, J. F. (1992). New forestry principles from ecosystem analysis of Pacific northwest forests. Ecol. Appl. 2, 262–274. doi: 10.2307/1941860
Swanson, M. E., Franklin, J. F., Beschta, R. L., Crisafulli, C. M., DellaSala, D. A., Hutto, R. L., et al. (2011). The forgotten stage of forest succession: early-successional ecosystems on forest sites. Front. Ecol. Environ. 33, 117–125. doi: 10.1890/090157
Taylor, A. H., Harris, L. B., and Skinner, C. N. (2022). Severity patterns of the 2021 Dixie fire exemplify the need to increase low-severity fire treatments in California’s forests. Environ. Res. Lett. 17:071002. doi: 10.1088/1748-9326/ac7735
The Integrity Council for the Voluntary Carbon Market (2024). “Section 4: assessment framework” in Core carbon principles, assessment framework and assessment procedure (The Integrity Council for the Voluntary Carbon Market), 50–102.
Thompson, J. R., Spies, T. A., and Olsen, K. A. (2011). Canopy damage to conifer plantations within a large mixed-severity wildfire varies with stand age. For. Ecol. Manag. 262, 355–360. doi: 10.1016/j.foreco.2011.04.001
Thomson, F. J., Moles, A. T., Auld, T. D., and Kingsford, R. T. (2011). Seed dispersal distance is more strongly correlated with plant height than with seed mass. J. Ecol. 99, 1299–1307. doi: 10.1111/j.1365-2745.2011.01867.x
Turner, M. G., Braziunas, K. H., Hansen, W. D., and Harvey, B. J. (2019). Short-interval severe fire erodes the resilience of subalpine Lodgepole pine forests. Proc. Natl. Acad. Sci. 116, 11319–11328. doi: 10.1073/pnas.1902841116
Turner, D. P., Conklin, D. R., and Bolte, J. P. (2015). Projected climate change impacts on forest land cover and land use over the Willamette River basin, Oregon, USA. Clim. Chang. 133, 335–348. doi: 10.1007/s10584-015-1465-4
U.S. Congress (2021a). Public law no: 117–58. Infrastructure investment and jobs act of 2021. Washington, DC: U.S. Congress.
U.S. Congress (2021b). Repairing existing public land by adding necessary trees act, S.866, 117th Cong. Washington, D.C: U.S. Congress.
U.S. Congress (2022). Public law no: 117–169. Inflation reduction act of 2022. Washington, DC: U.S. Congress.
U.S. Congress (2023). Rural Forest markets act of 2023, H.R.5315, 118th Cong. (2023). Washington, DC: U.S. Congress.
USDA (2023). Report to the secretary USDA strike team for accelerating tree establishment. Washington, DC: USDA, 51.
USDA Forest Service (2022). National Forest System Reforestation Strategy Growing and nurturing resilient forests. Washington, DC: USDA Forest Service National Forest System FS-1198, 32.
Vaughan, D., Edgeley, C., and Han, H. S. (2022). Forest contracting businesses in the US southwest: current profile and workforce training needs. J. For. 120, 186–197. doi: 10.1093/jofore/fvab060
Verra. (2024). Available at: https://verra.org/ (Accessed February 20, 2024)
Warner, E., Cook-Patton, S. C., Lewis, O. T., Brown, N., Koricheva, J., Eisenhauer, N., et al. (2023). Young mixed planted forests store more carbon than monocultures—a Meta-analysis. Front. Forests Global Change 6:1226514. doi: 10.3389/ffgc.2023.1226514
Weatherspoon, C. P., and Skinner, C. N. (1995). An assessment of factors associated with damage to tree crowns from the 1987 wildfires in northern California. For. Sci. 41, 430–451. doi: 10.1093/forestscience/41.3.430
Weiss, G., Emery, M. R., Corradini, G., and Živojinović, I. (2020). New values of non-Wood Forest products. Forests 11:165. doi: 10.3390/f11020165
Westfall, J. A., Coulston, J. W., Moisen, G. G., and Andersen, H. E. (2022). Sampling and estimation documentation for the enhanced Forest inventory and analysis program: 2022. Madison, WI: U.S. Department of Agriculture, Forest Service, Northern Research Station.
Westveld, R. H. (1939). Applied Silviculture in the United States. New York & London: John Wiley and Sons, Inc. & Chapman and Hall Ltd.
White, A. M., and Long, J. W. (2019). Understanding ecological contexts for active reforestation following wildfires. New For. 50, 41–56. doi: 10.1007/s11056-018-9675-z
Williams, A. P., and Abatzoglou, J. T. (2016). Recent advances and remaining uncertainties in resolving past and future climate effects on global fire activity. Curr. Clim. Chang. Rep. 2, 1–14. doi: 10.1007/s40641-016-0031-0
Williams, M. I., and Dumroese, R. K. (2013). Preparing for climate change: forestry and assisted migration. J. For. 111, 287–297. doi: 10.5849/jof.13-016
Williams, C. A., Gu, H., MacLean, R., Masek, J. G., and Collatz, G. J. (2016). Disturbance and the carbon balance of US forests: a quantitative review of impacts from harvests, fires, insects, and droughts. Glob. Planet. Chang. 143, 66–80. doi: 10.1016/j.gloplacha.2016.06.002
Williams, J. N., Quinn-Davidson, L., Safford, H. D., Grupenhoff, A., Middleton, B. R., Restaino, J., et al. (2024). Overcoming obstacles to prescribed fire in the North American Mediterranean climate zone. Front. Ecol. Environ. 22:e2687. doi: 10.1002/fee.2687
York, R. A., Levine, J., Russell, K., and Restaino, J. (2021). Opportunities for winter prescribed burning in mixed conifer plantations of the Sierra Nevada. Fire Ecol. 17:33. doi: 10.1186/s42408-021-00120-5
Yospin, G. I., Bridgham, S. D., Neilson, R. P., Bolte, J. P., Bachelet, D. M., Gould, P. J., et al. (2015). A new model to simulate climate-change impacts on Forest succession for local land management. Ecol. Appl. 25, 226–242. doi: 10.1890/13-0906.1
Zald, H. S. J., and Dunn, C. J. (2018). Severe fire weather and intensive Forest management increase fire severity in a multi-ownership landscape. Ecol. Appl. 28, 1068–1080. doi: 10.1002/eap.1710
Zald, H. S. J., Gray, A. N., North, M., and Kern, R. A. (2008). Initial tree regeneration responses to fire and thinning treatments in a Sierra Nevada mixed-conifer Forest, USA. For. Ecol. Manag. 256, 168–179. doi: 10.1016/j.foreco.2008.04.022
Ziegler, J. P., Hoffman, C. M., Collins, B. M., Knapp, E. E., and Mell, W. (2021). Pyric tree spatial patterning interactions in historical and contemporary mixed conifer forests, California, USA. Ecol. Evol. 11, 820–834. doi: 10.1002/ece3.7084
Keywords: reforestation, wildfire, tree planting, forests, natural climate solutions, conservation finance
Citation: Dobrowski SZ, Aghai MM, Chichilnisky du Lac A, Downer R, Fargione J, Haase DL, Hoecker T, Kildisheva OA, Murdoch A, Newman S, North M, Saksa P, Sjoholm M, Baribault T, Buonanduci MS, Chambers ME, Gonzales-Kramer L, Harvey BJ, Hurteau MD, Loevner J, Safford HD and Sloan J (2024) ‘Mind the Gap’—reforestation needs vs. reforestation capacity in the western United States. Front. For. Glob. Change. 7:1402124. doi: 10.3389/ffgc.2024.1402124
Edited by:
Jose G. Borges, University of Lisbon, PortugalReviewed by:
Pete Bettinger, University of Georgia, United StatesDavid Ellison, University of Bern, Switzerland
Copyright © 2024 Dobrowski, Aghai, Chichilnisky du Lac, Downer, Fargione, Haase, Hoecker, Kildisheva, Murdoch, Newman, North, Saksa, Sjoholm, Baribault, Buonanduci, Chambers, Gonzales-Kramer, Harvey, Hurteau, Loevner, Safford and Sloan. This is an open-access article distributed under the terms of the Creative Commons Attribution License (CC BY). The use, distribution or reproduction in other forums is permitted, provided the original author(s) and the copyright owner(s) are credited and that the original publication in this journal is cited, in accordance with accepted academic practice. No use, distribution or reproduction is permitted which does not comply with these terms.
*Correspondence: Solomon Z. Dobrowski, solomon.dobrowski@umontana.edu
†These authors have contributed equally to this work and share first authorship
‡These authors share secondary authorship
δThese authors share last authorship