- 1Swiss Federal Institute for Forest, Snow, and Landscape Research (WSL), Birmensdorf, Switzerland
- 2WSL Institute for Snow and Avalanche Research SLF, Davos, Switzerland
- 3Climate Change, Extremes and Natural Hazards in Alpine Regions Research Centre CERC, Davos, Switzerland
A warmer climate with drier summers will affect the suitability of tree species in their current range in most of Europe. To preserve ecosystem services in the future, many European countries are looking for tree species adapted to the expected future climate and are setting up trials to test them in different environments. In collaboration with forest practitioners and federal and regional authorities, we have established a network of 57 common gardens across large environmental gradients in Switzerland. Over a period of 30 to 50 years, the vitality, growth and survival of 18 tree species from 117 seed sources will be monitored to develop tree species recommendations for forest managers. In this article, we outline the considerations, challenges and trade-offs involved in designing this experiment, as well as the participatory process with a variety of stakeholders, from local foresters to the Federal Office for the Environment. Further, we list experiments testing multiple species on multiple sites in Europe and compare experimental designs, tree species and environmental gradients. The Swiss common garden network complements other European experiments and broadens the potential network by extending the covered environmental gradients to colder and moister conditions. Such targeted assisted migration trials are key to promoting tree species that can cope with the future climate and can help us to foster forest ecosystems that can adapt to rapidly changing climates.
1 Introduction
Global warming combined with more frequent and severe summer droughts and heat spells in large parts of the world, including Europe (Zscheischler et al., 2018; IPCC, 2021), is increasingly affecting forest ecosystems (Chen et al., 2011; McDowell et al., 2020). Changing disturbance regimes will likely result in major declines in forest ecosystem services, such as timber production, protection against natural hazards, biodiversity and recreation (Seidl et al., 2017; Forzieri et al., 2021), and will compromise sustainable forest management (Bolte et al., 2009; Sousa-Silva et al., 2018). Intense climatic drought can impair tree growth and reproduction and increase mortality rates (Allen et al., 2015; Clark et al., 2016; Cailleret et al., 2017; Choat et al., 2018; Brodribb et al., 2020; Schuldt et al., 2020; Senf et al., 2020), ultimately leading to changes in tree species composition and forest structure and to shifts in species distributions (Anderegg et al., 2013; Clark et al., 2016; Brodribb et al., 2020; McDowell et al., 2020; Senf et al., 2021). Since rapid climate change is likely to outpace the ability of many tree species to adapt and survive in their changing habitats (Jump and Peñuelas, 2005), tree species will be forced to colonize new habitats upward in elevation and poleward of their current distribution to track suitable climatic conditions, or they will face local extinction (Parmesan and Yohe, 2003; Aitken et al., 2008; Vitasse et al., 2012; Burrows et al., 2014). Tree species can accomplish part of this migration through natural propagation, but in many cases this process fails to keep pace with the rate of global warming (Zhu et al., 2012). This is especially true in fragmented landscapes, where migration barriers impede range shifts (Saltré et al., 2015).
Assisted migration of tree species (Williams and Dumroese, 2013; Dumroese et al., 2015) and assisted gene flow among populations (Aitken et al., 2008) are management strategies that aim to artificially introduce tree species or tree populations, to locations where growing conditions are expected to be suitable for them in the future climate. This may even save some tree species from extinction (Mc Lachlan et al., 2007). However, assisted migration to areas far outside the current range of a species can also bear some risks: ethical, economical, legal, political and ecological concerns have been brought forward (Spies et al., 2010; Vitt et al., 2010; Aubin et al., 2011; Sansilvestri et al., 2015; Hagerman and Kozak, 2021). Since past relocations have had severe negative ecological implications in some instances, best practices for assisted migration need to be developed (Schwartz et al., 2012; Klein and Arts, 2022). Further, while assisted gene flow has improved growth in certain cases (Aitken and Bemmels, 2016), large environmental distances between seed sources and planting sites have sometimes resulted in lower yield and survival, for example in a provenance trial where Pseudotsuga menziesii var. menziesii Mirb. (Douglas fir) was established in North America in 1912 (St Clair et al., 2020). In Europe, provenance trials started as early as 1745 (Langlet, 1971), including those with Abies alba Mill. (silver fir, Larsen, 1986) Larix decidua Mill. (European larch, Weisgerber and Šindelář, 1992), Picea abies (L.) Karst. (Norway spruce, Şofletea and Budeanu, 2015), Pinus sylvestris L. (Scots pine, Stephan and Liesebach, 1997) and Quercus petraea (sessile oak, Sáenz-Romero et al., 2017). In addition, assisted migration experiments in the form of biodiversity trials have been established in major forest types throughout the world to investigate ecosystem functioning with different tree species combinations and various levels of functional diversity (Paquette et al., 2018).
In Switzerland, close-to-nature silviculture is the prevailing forest management system. It favors the natural regeneration of trees (Brang et al., 2014; Brüllhardt et al., 2022), resulting in uneven-aged forests bearing a high species and structural diversity, which are generally more resilient to disturbance and climate change than even-aged forests (Bauhus et al., 2013; Hof et al., 2017). However, natural regeneration does not lead to climate adapted forests per se, as tree species presumably adapted to the future climate are often missing in these forests, for example because they are outcompeted by dominant tree species such as beech (Ligot et al., 2013; Dietz et al., 2022) or because they are preferentially consumed by browsing ungulates (Champagne et al., 2021; Angst and Kupferschmid, 2023). Recently, forest managers have started to implement adaptive measures, in particular through the introduction of tree species, i.e., assisted migration (Williams and Dumroese, 2013). Tree species or provenances are often introduced in single pilot plantations (Bürgi and Dietz, 1986; Pretzsch, 2005; Nyssen et al., 2016), which can provide information about tree performance under specific site conditions and the current climate but allow only limited inference to other sites. Therefore, a coordinated plantation approach is needed to generate well-founded tree species recommendations for future forestry practice.
Despite a large body of literature from forest inventories (Mina et al., 2018; Etzold et al., 2019; Abegg et al., 2020; Del Río et al., 2021), provenance studies (Sáenz-Romero et al., 2017), niche modeling (De Rigo et al., 2016), dendroecological studies (Bottero et al., 2021) and experiments (Frank et al., 2017; Fririon et al., 2023), there is still considerable uncertainty regarding the factors that determine the survival, vitality and growth of tree species and provenances under climate change and assisted migration. For over two centuries, scientists have conducted common garden (CG) experiments, also known as experimental plantations, to study the performance of trees from different genetic and/or geographic origins under identical environmental conditions (Langlet, 1971). The majority of early trials were designed to optimize timber production, established due to the growing awareness of the importance of geographic variation, which had earlier repeatedly resulted in plantation failure (Aitken and Bemmels, 2016). In light of the recent and expected climate-change-induced habitat shifts of tree species, the focus of CG experiments changed from provenance studies to networks that systematically incorporate a broad set of tree species along environmental gradients (Alizoti et al., 2022; Fady and Rihm, 2022). Such networks may help to identify species’ optima and limits, as well as species and seed sources that can cope with the expected climate of the future, thus enabling forest ecosystems to adapt to rapidly changing conditions. Such information is needed to build a solid scientific foundation for assisted migration.
Large CG networks offer opportunities to investigate research questions over broad environmental gradients. For forest managers, it is more attractive to join coordinated and subsidized CG networks, where they can benefit from insights gained throughout the network, rather than investing in stand-alone pilot plantations. To produce reliable results and use resources effectively, it is important to have a statistically sound and powerful design, as well as coordination with similar initiatives. A particular challenge is that the optimal scientific solution would involve numerous sites over large environmental gradients, ample replication and large tree populations observed over a long timeframe, yet these aspects collide with feasibility constraints, especially regarding available resources. Moreover, such experiments involve many stakeholders, and their planning, establishment and maintenance require major investments in personnel and equipment (Hatfield, 1998). Long-term commitments of the involved stakeholders are essential to secure the success of such experiments. However, reorganizations and financial constraints, which become increasingly likely with the longer duration of projects, can threaten the continuation of an experimental network (Pretzsch, 2005).
New CG networks have been and are currently being established in many places around the globe with the goal of finding tree species and seed sources that are suitable for the future climate (Fady and Rihm, 2022). In North America, similar considerations have resulted in a large number of publications on assisted migration experiments over the last three decades (Palik et al., 2022; Park and Rodgers, 2023). In Switzerland, we have recently established a CG network testing tree species in forests across a large gradient covering most of the climates that occur in the country and are suitable for tree growth. We planted seedlings grown from seeds of selected tree populations in CGs to improve our understanding of the species’ capacities to cope with climate change (Frei et al., 2018). The aim of the project is to derive recommendations that will help forest managers to promote suitable, climate-adapted species, either through natural regeneration or by planting. In this method paper, we present the new Swiss CG network. Specifically, we: (1) describe the participatory planning and decision process employed for designing the Swiss CG network and present its final design, (2) give an overview of the experiments testing multiple tree species on multiple sites in Europe and compare the designs, tree species and environmental gradients to point out the benefits of a European network, and (3) discuss the potential and limits of such trials. We expect this information to benefit others interested in designing similar CG networks and to encourage collaboration, especially across national borders throughout Europe.
2 Methods and comparison
2.1 The Swiss common garden network
2.1.1 Aims and scope
In anticipation of a warmer climate with drier summers, which will affect the climatic suitability of tree species in their current range, scientists, practitioners and authorities in Switzerland have recognized the need to identify suitable tree species that can secure the provisioning of ecosystem services in the future.
Through a joint initiative, involving scientists, federal and regional forest authorities, and forest practitioners from the public and private sectors, a network of 57 CGs has recently been established in forests across large environmental gradients in Switzerland. The network is designed to test and compare 18 tree species. By planting seedlings from seeds of the same populations under different climates, the aim is to improve our understanding of the species’ capacities to cope with the expected future climate. The CG network enables a comparison of the performance of different tree species under identical climatic and environmental conditions. Additionally, it makes it possible to study the performance of individual tree species along the environmental gradients. To assess the intraspecific variability of each species in its response to various climates, several seed sources are tested for each species.
The Swiss CG network is designed to serve as an infrastructure for fundamental and applied research in the field of adaptation of forests to future climate conditions over the next 30–50 years. In particular, the network is intended to address the following questions:
Which environmental factors determine the performance of tree species along environmental gradients, and how is their intraspecific variation affected by environmental factors?
How does the performance compare among tree species, how is the interspecific variation affected by environmental factors, and how do the tree species compare regarding intraspecific variation?
2.1.2 The participatory process used to develop the Swiss common garden network
The concept and design for the Swiss CG network were developed through a participatory process involving three main stakeholder groups: (1) the Swiss Federal Office for the Environment (FOEN); (2) the cantonal forest offices, local forest managers and forest owners; and (3) national and international researchers. These stakeholder groups have different roles: FOEN leads the political process toward sustainable forest services under climate change, funds projects that support evidence-based decision-making, and supports the scientific coordination of the Swiss CG network (FOEN, 2020). Land required for the CG sites, as well as the financial and personnel resources to establish and maintain the CGs, is provided by the cantonal forest offices and the forest owners. National and international researchers add value to the CG network by exploring their own research questions based on the CG infrastructure and securing additional third-party funding. They contribute scientific expertise to the development of the experimental design and to evaluations of the results. The Swiss CG network team at WSL coordinates interactions among the stakeholders and is responsible for the implementation of the CG network regarding the interests of the stakeholders.
All stakeholders were consulted throughout the project development phase, i.e., before important design and implementation decisions were made (Figure 1). The experimental design was discussed with all stakeholders in three workshops in 2017 and improved based on their input. The statistical power of the experimental design was validated by Biomathematics and Statistics Scotland (James Hutton Institute, Aberdeen). Statistical power calculations were based on tree height and stem diameter data from experimental forest management sites (Forrester et al., 2021) and from Abies alba provenance trials in Switzerland (Commarmot, 1995; Commarmot, 1997). The aim of these evaluations was to guarantee that the experiment has the necessary statistical power to derive inter- and intraspecific differences and relationships between environmental factors and tree performance. The tree species selection was discussed with the cantonal forest authorities via e-mail and in two workshops in 2017, and the seed source selection was discussed with researchers in a workshop in 2018. The experimental sites were selected in close cooperation with the cantonal authorities and the forest owners. The initiation of add-on projects was encouraged during a workshop with the research community in 2019.
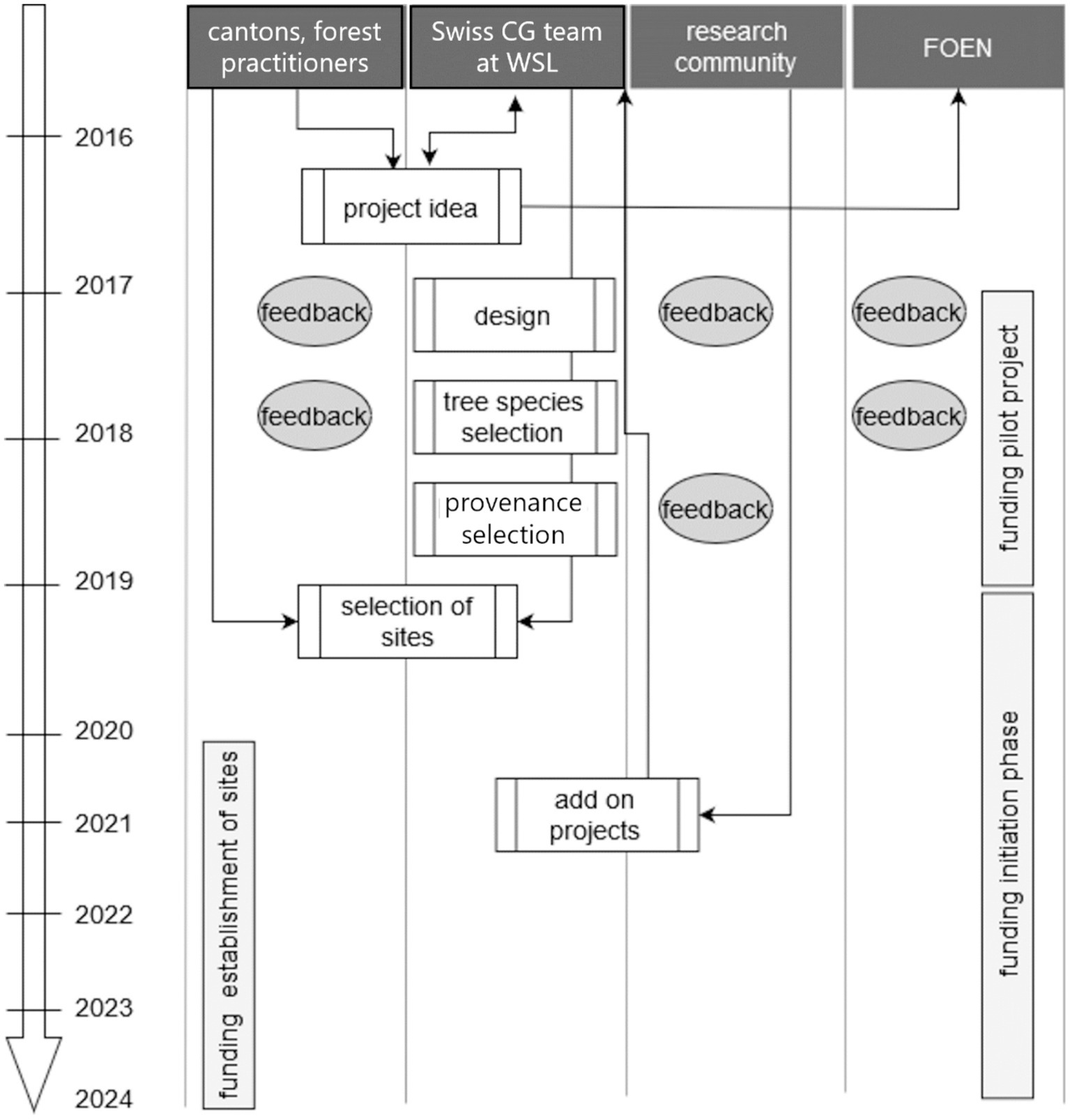
Figure 1. Collaboration of stakeholder groups during the project initiation phase of the Swiss common garden (CG) experiment.
2.1.3 Experimental design
To acquire statistically sound data, the experimental design needed to be consistent throughout the network. The statistical power analyses revealed that the most crucial design parameter was the number of sites on which a tree species was to be tested. For a high explanatory power of 80%, the number of sites per species had to exceed 30. In comparison, the number of trees per experimental unit was found to be of lower importance for analyzing growth parameters. However, for mortality analysis, the number of trees needed to be sufficient to be able to estimate the expected mortality at a site. As a minimum difference of 4% in mortality among seed sources on a site was considered relevant, at least 25 seedlings per seed source per site were needed. A block design was used to account for spatial variation within sites (Binkley, 2008). As great attention was paid to finding homogeneous sites, spatial variation within sites was expected to be relatively small compared with the site-to-site variation. Therefore, three instead of the initially recommended four blocks per site were ultimately used because of feasibility considerations.
All tree species were planted once in every block (Figure 2). They were grouped into slow- and fast-growing species. These groups were placed in alternating order in the blocks, and the tree species were assigned randomly to plots within each group. Each plot was split into four subplots to which four seed sources of the same tree species were assigned randomly. Nine individual trees were planted in each subplot. The number of seedlings of a specific seed source per site thus amounted to 27 (i.e., 108 seedlings per tree species; Figure 2).
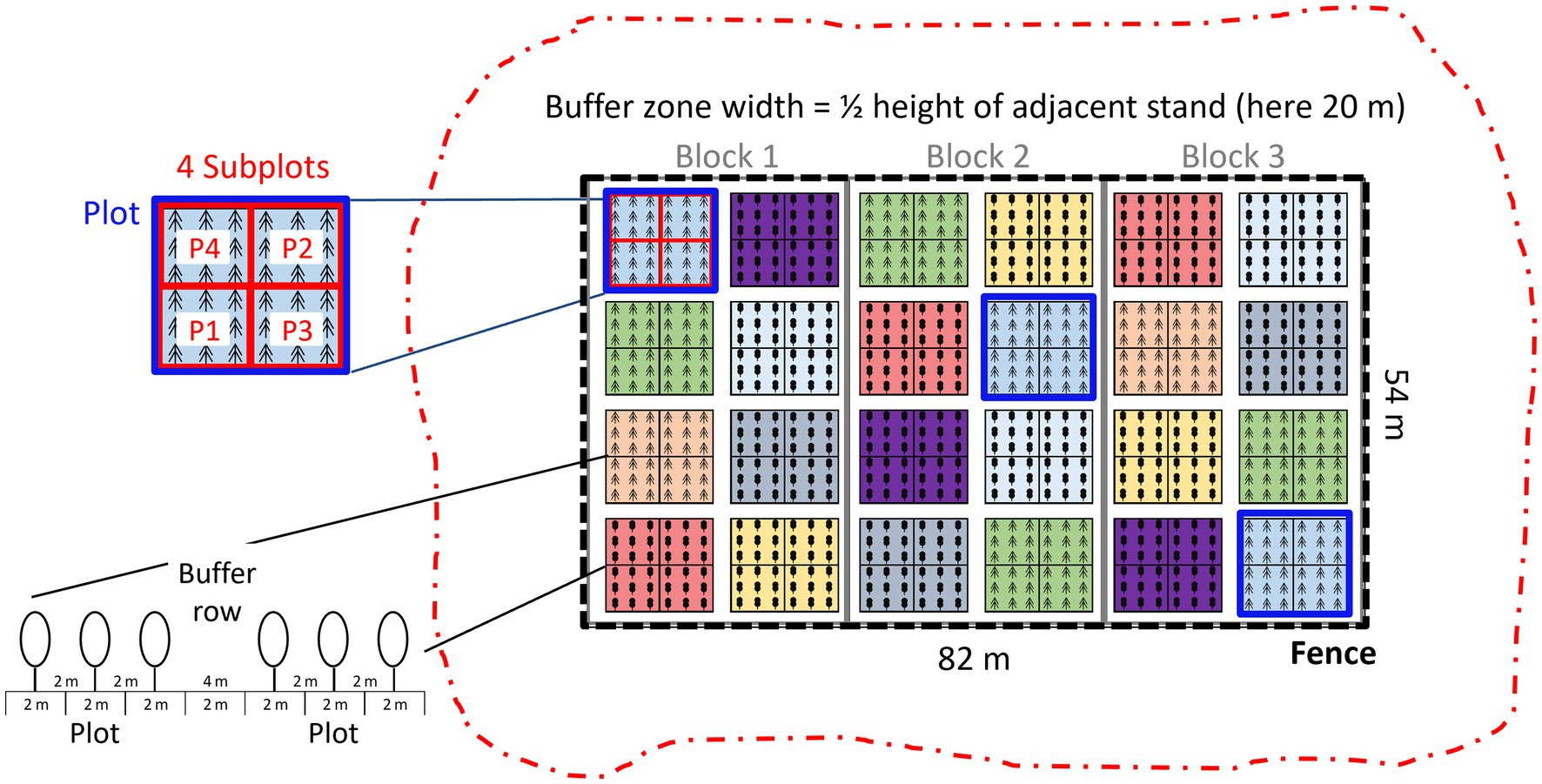
Figure 2. Idealized design of a common garden, with 8 tree species repeated in 3 blocks, leading to 24 plots. The plots are split in 4 subplots containing 4 seed sources per species. Each subplot contains 9 individual trees of a given species and seed source. Plots are separated by a buffer row to minimize competition among tree species. To prevent shading effects, the planting zone is surrounded by a buffer zone without trees of approximately half the height of the adjacent stand.
To minimize competition, trees were planted with 2 m spacing between individuals. Between plots, the spacing was doubled to 4 m (Figure 2). The planting zone was protected by a fence to exclude browsing ungulates. Around the fenced planting zone, a buffer zone with a width of approximately half the adjacent stand height was cut clear to reduce shading effects. The available area determined the number of tree species that could be studied at a specific CG site. As an example, the area required for a CG with eight tree species was approximately 1 ha, and nearly half of this area was assigned as buffer zone (47%).
2.1.4 Species selection
The tree species for the experiment were chosen based on several criteria that were considered of importance for future forestry in Switzerland. These included (1) the species’ current abundance in Switzerland (i.e., percentages of standing timber volume and basal area in the Swiss National Forest inventory ≥0.1% in at least one of the biogeographical regions; Brändli, 1998); (2) its potential for providing ecosystem services, such as timber production, natural hazard protection, and biodiversity; and (3) the width of its ecological amplitude. Invasive species and those highly susceptible to pathogens or drought were excluded, on the assumption that they will not play a major role in Swiss forestry in the future.
The original set of species included all those with standing timber volume data in the National Forest Inventory. The set was augmented with a number of less abundant species, as well as non-native species, which may have potential on the warmest and driest sites. Initially, 61 candidate tree species were evaluated (Supplementary Table S1). Based on feedback from stakeholders in the participatory process, the decision was made to carry out the experiment with two subsets of tree species (Table 1): a so-called ‘core set’ of 9 species, each planted on 35 sites, for which statistically sound data could be obtained; and (2) a so-called ‘extension set’ of 9 additional species tested on only 15 sites each, mainly at the warm and dry end of the environmental gradient (Figure 3). Abies alba was planted on all sites as a reference species.
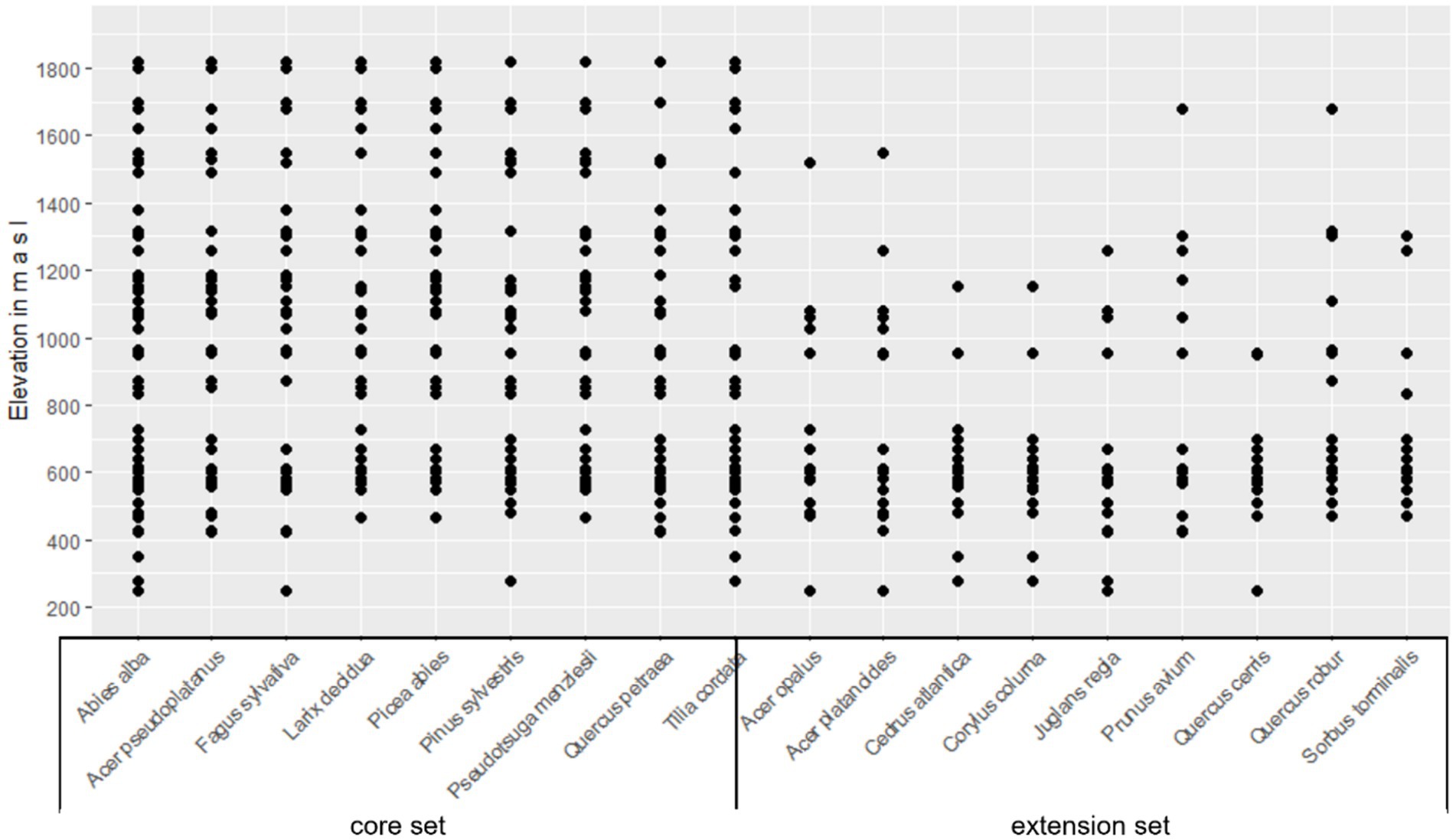
Figure 3. Elevations at which the tree species are tested in the Swiss common garden (CG) experiment.
The Swiss CG network was designed such that tree species are tested in their current range, as well as on sites where the climate is projected and assumed to be suitable for them toward the end of the century, i.e., on sites that are currently colder and/or wetter than their future habitat. To assign tree species to these sites, the following scenario was considered: between the periods 1981–2010 and 2070–2099, the emission scenario RCP 8.5 with the model combination CLMCOM-CCLM5_HADGEM_EUR44 (NCCS 2018) projects an average warming of 4.4°C for Switzerland and a reduction in precipitation of 17% (northern Switzerland) to 25% (southern Switzerland) for the months of April through August, which are considered most relevant for the climatic suitability of trees because this is the main period of growth (Zischg et al., 2021). The anticipated temperature increase of 4.4°C corresponds to an elevational shift of about 800 m considering a lapse rate of 0.55°C/100 m (Fairbridge and Oliver, 2005). The tree species were thus assigned to the sites such that they covered the elevation gradient of their current habitat and elevations up to 800 m higher (Figure 3). Sites below the current warm and dry habitat limit were not included because: (1) the climate will become warmer and drier during the lifetime of the trees, which will lead to a gradually increasing representation of the warm and dry edge over the planned experiment duration; and (2) this project is focused on laying a scientific foundation for assisted migration.
2.1.5 Seed source selection and plant provisioning
With the selected design, four seed sources could be planted per species on each site. However, four seed sources were not considered sufficient to adequately represent the genetic variation within the species (workshop with scientists in 2018, see section 3.2). Therefore, the decision was made to test seven seed sources for each species. One of them is planted as a within-species reference on all sites hosting the respective species, whereas the other six seed sources are planted randomly on half of the sites. This design will allow us to better catch effects of intraspecific variation but not to give recommendations for specific seed sources, because of the small number of seed sources tested per species and the limited number of sites on which individual seed sources are replicated.
The search for suitable seed sources started in summer 2018, based on the following criteria: (1) seed material should derive from autochthonous stands to ensure that it is adapted to the local climate, (2) seed sources should be OECD-certified (Organization for Economic Co-operation and Development; OECD) to ensure a certain quality level and allow comparisons with other trials, (3) the different seed sources of a species should cover large environmental gradients across the natural habitat and originate from different refugial areas in order to represent the within-species genetic variation, (4) truly marginal populations should be excluded to preclude a potential loss of genetic diversity in isolated populations and (5) sufficient quantities of high-quality seed material should be available. For native species, four to five Swiss seed sources were complemented by two to three seed sources from the warm and dry end of the habitat outside of Switzerland.
In total, planting material was procured from 117 seed sources (for a complete list of seed sources and suppliers, see Supplementary Table S2). For six tree species, the full set of seven seed sources was not found. In these cases, several seed sources were planted on all sites. Most seedlings were raised at the nursery of Emme Forstbaumschule SA in Wyler bei Utzensdorf, Switzerland. However, all seedlings of Quercus cerris were grown in Vivaio forestale di Lattecaldo in Morbio Superiore, Switzerland and all seedlings of Sorbus torminalis were grown at WSL in Birmensdorf, Switzerland, because these nurseries had specific experience with these two species. In addition, one Abies alba seed source, two Larix decidua seed sources, and one Picea abies seed source were grown at the Forstgarten Rodels nursery in Rodels, Switzerland. One seed source of Acer platanoides was grown at the Forstgarten Lobsigen nursery in Lobsigen, Switzerland and one seed source of Abies alba was grown at the nursery of Allasia Plant Magna Grecia S.S. in Soveria Mannelli, Italy.
2.1.6 Site selection
Forest sites suitable as potential test sites were suggested by the cantonal forest offices. The proposed sites were evaluated according to the following criteria: (1) homogeneous in aspect, slope and soil properties and (2) area of at least 0.6 ha. A total of 172 sites were proposed, covering 20 of the 26 Swiss cantons. The project team visited 125 sites in 2018, together with representatives of the cantonal forest offices and the local forest managers, to assess the suitability of the sites for the project. Sites that had failed to harbor regeneration for more than 15 years, due to being waterlogged or overgrown with competing vegetation (megaforbs), were excluded. Additional site selection criteria were: (3) interest in participating in the project on the part of the corresponding forest managers and owners and (4) commitment of the cantonal authorities to contributing funding for the establishment of the CG.
Since the gradients over which the species were to be tested varied among the species (Figure 4) and most of the available sites were too small to harbor more than 8–9 tree species, close to 60 sites were required to provide sufficient space for testing all 18 species. The final selection of 57 test sites (Figure 4) represents the major Swiss biogeographic regions, elevation belts, soil types and slope aspects. Six of the 57 sites are large enough to test all 18 tree species. These sites are geographically widespread but are all situated below 1,000 m a.s.l. They have been preselected for intensive monitoring. Currently, additional phytosanitary monitoring is carried out on these sites.
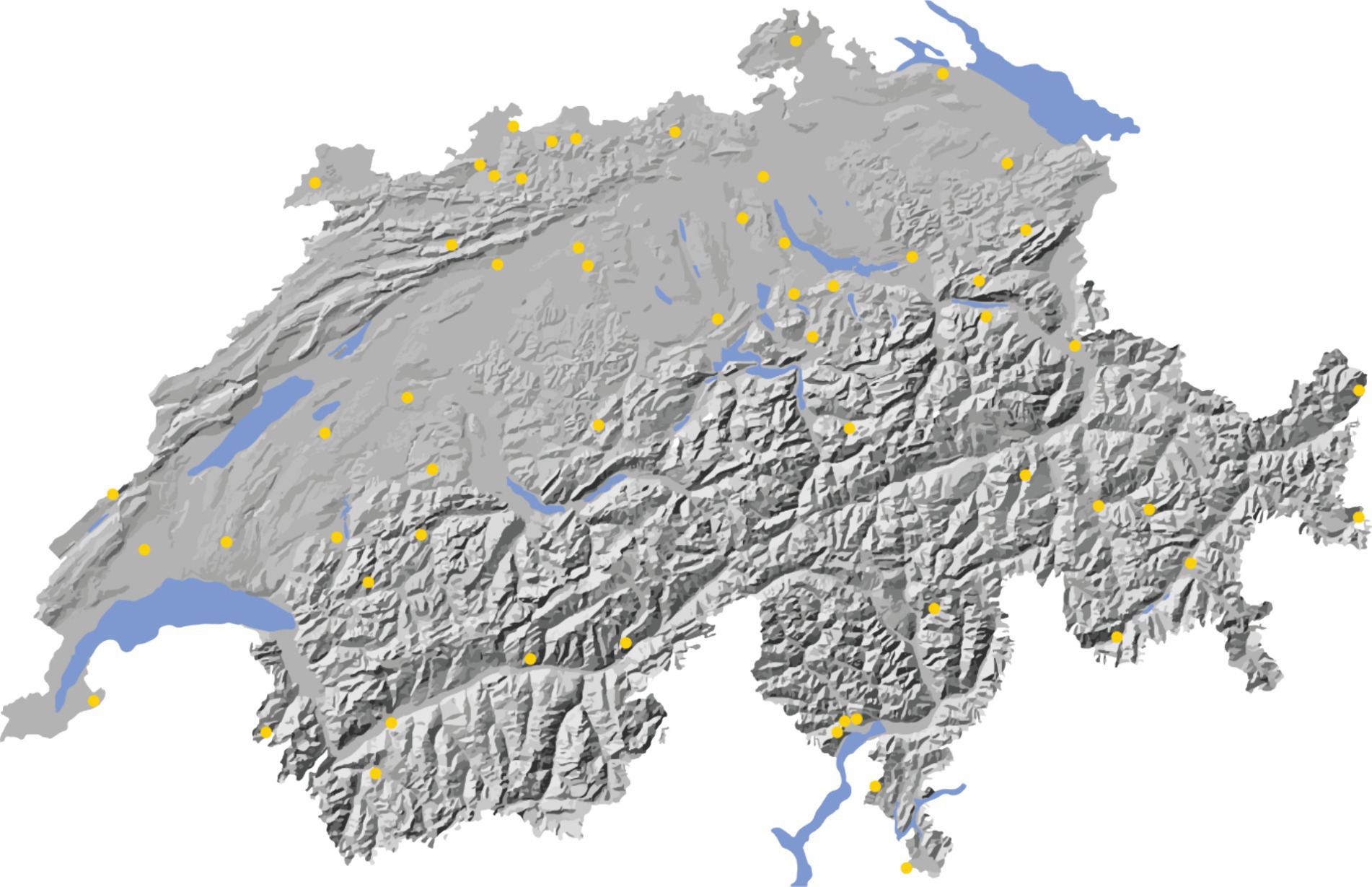
Figure 4. Map of Switzerland showing the locations of the 57 experimental sites of the Swiss common garden experiment (yellow dots). For site names, refer to Supplementary Table S3. For site-specific information on the tested tree species and the experimental design, refer to the website: www.testpflanzungen.ch.
2.1.7 Environmental measurements
On all sites, at least one soil profile was dug for detailed soil characterization, including morphology and classification, physical properties, organic matter content, acidity and nutrient availability. Additionally, each site is equipped with an automatic weather station, monitoring air temperature, soil temperature at 15 and 50 cm depth, soil suction at 15 and 50 cm depth, relative humidity, precipitation, wind velocity and direction, solar radiation and barometric pressure, all at 10-min intervals.
2.1.8 Measurements of plant performance
Shortly before planting, seedlings were individually labeled and their initial height (seedling length from root collar to terminal bud) and root collar diameter were measured. Establishment and planting of the 57 sites was distributed over three planting seasons between autumn 2020 and spring 2023. Seedlings that died or disappeared were replaced by replanting new seedlings, up to 2 years after site set-up. Seedling survival and damage were monitored for the first time in the summer after planting. For the 19 sites set up in autumn 2020 and spring 2021, this was done in summer 2021. In summer 2023, seedling survival and damage were monitored on all 57 sites, i.e., on a total of approximately 55,000 plants. Damage was assessed separately for terminal buds, bark and leaves. This survival and damage monitoring will be repeated annually for at least 5 years. Height and length of the seedlings will be measured for the first time after the end of the installation phase in summer 2024. These measurements will be continued annually for at least 5 years, after which the measurement interval will be increased to 2–3 years. The first results on mortality and damage will be published in 2025, whereas an observation time of about a decade is needed to gain valid information on tree growth.
2.2 Overview of common garden experiments in Europe
2.2.1 Approach
In this section, we list experiments conducted in Europe that test multiple tree species on multiple sites, and we acknowledge the potential offered by broadening the CG network. We conducted a systematic literature search using Scopus with the terms ‘common gardens’, ‘trees’, ‘tree species’, ‘Europe’ and ‘assisted migration’. In addition, we contacted colleagues in forest science in Germany, Austria, France, Italy, the Czech Republic and Great Britain to obtain information on more recent, yet unpublished, trials. In addition, we included CG networks in Belgium, France, Germany and Switzerland that are currently in their establishment phase. To ensure a consistent sample, we excluded all single species provenance trials and all experiments conducted on single sites. We further excluded experiments with a focus on the ecosystem functioning of tree species combinations since they offer limited insights on the performance of individual tree species along environmental gradients. Our comparison focuses on the climate space of the planting sites, considering annual mean temperature, annual precipitation sum, and the climatic water balance during the growing season, i.e., the difference between precipitation and potential evapotranspiration for the months with a mean temperature above 5°C. We derived climatic parameters from CHELSA (Climatologies at high resolution for the earth’s land surface areas; Karger et al., 2017) and used an approximation for potential evapotranspiration (Turc, 1961). In addition, we compared the sets of tree species tested and the designs of the various CG experiments.
2.2.2 Common garden experiments in Europe
We identified 16 CG experiments with tree species in Europe that matched the criteria defined above (Table 2). Already more than 50 years ago, 8 arboretums were set up in France testing 2,300 seed sources of 70 species (French arboretum; Ducatillion et al., 2022). Although the designs of the arboretums are not consistent, the planting dates are well documented and within-site replication information is available. The fact that the trees in these CGs are at least 50 years old, and were thus planted under different from current climate conditions, makes the data from this experiment very interesting for dendrochronological studies. The arboretums are part of the GEN4X Network (Forest Genetics Network for Research and Experimentation), which includes a total of 1,208 sites installed to study ecosystem functioning or to generate improved reforestation material (only arboretum sites are included in Table 2: Rihm and Fady, 2023).
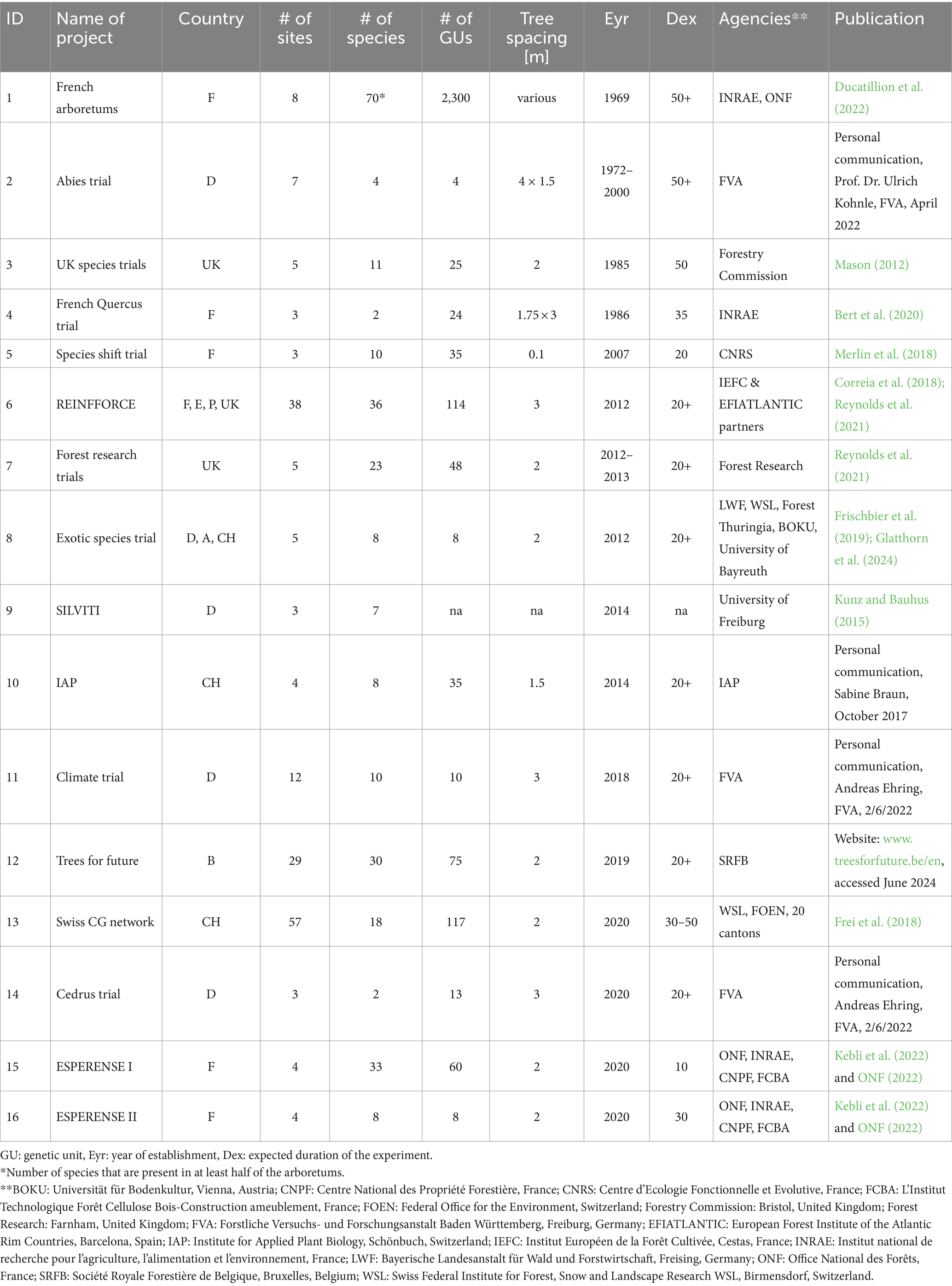
Table 2. Overview of common garden experiments in Europe that test the climatic suitability of tree species.
The Forest Research Institute of Baden-Württemberg (FVA) initiated the establishment of trials involving four non-native Abies species in 1972 to compare their performance with that of native Abies alba (personal communication, Prof. Dr. Ulrich Kohnle, FVA, April 2022).
In 1985, the Forestry Commission of the United Kingdom initiated a CG trial to find conifers other than Picea sitchensis (Bong.) Carrière (Sitka spruce) that could thrive under the climatic conditions in the UK (UK species trials; Mason, 2012). This trial included 11 conifer species (with 25 seed sources) that were planted at 5 sites.
A CG trial was established in France in 1986 to find suitable Quercus petraea and Q. robur seed sources under a range of climatic conditions. The trial includes 24 European Quercus seed sources planted on three sites (French Quercus trial; Bert et al., 2020).
In 2007, an assisted migration trial was started in France, in which three Mediterranean, three cold-adapted and two so-called ‘cosmopolitan’ tree species were tested on three sites in southern, central and northern France. The objective was to identify whether the projected northward shift of the species could already be observed in this experimental setting (species shift trial; Merlin et al., 2018).
REINFFORCE was the first large-scale European experiment to assess the performance of tree species across a large environmental gradient. The experiment, which was started in 2012, focuses on the climatic suitability of 33 native and non-native tree species (with 114 seed sources) along the Atlantic rim from Portugal to the British Isles (Prieto-Recio et al., 2011; Correia et al., 2018; Reynolds et al., 2021). In the UK, REINFFORCE was complemented by the Forest Research trial. This trial shares the three British REINFFORCE sites and includes two additional sites located further east. The two experiments share 13 species, but the Forest Research trial, which was also started in 2012, included 10 additional species of specific interest to the UK (Reynolds et al., 2021).
At the same time, a trial with eight non-native species (one seed source per species), the so-called ‘exotic species trial,’ was initiated at five sites spread over Germany, Austria and Switzerland to investigate whether the tested species thrive under central European conditions (Frischbier et al., 2019; Glatthorn et al., 2024).
In 2014, the University of Freiburg initiated a project to find out whether rare and drought-tolerant native tree species could be used for the afforestation of former vineyards in a way that would help preserve the typical cultural landscape structures and increase biodiversity, while serving as a forest genetic reserve for rare species (SILVITI project: Kunz and Bauhus, 2015).
In the same year, the Institute for Applied Plant Biology in Switzerland started a trial with seven native tree species and Pseudotsuga menziesii from a total of 35 seed sources on four sites in northern Switzerland, to find out whether seed sources from drier sites in Europe would outperform the native seed sources in the years to come (IAP trial; personal communication, Sabine Braun, October 2017).
The ‘climate trials’ were installed in Baden Württemberg by the Forest Research Institute of Baden-Württemberg (FVA) in 2018 to test native and non-native tree species and their adaptation to climate change. Further sites testing different seed sources of Cedrus species were established in 2020 (personal communication, Andreas Ehring, FVA, June 2022).
With ‘Trees for Future,’ the Royal Forest Society of Belgium started an innovative project in 2019 to facilitate assisted migration of native and non-native tree species, prevent further degradation of forests due to drought and pest attacks, and increase tree species diversity in forests throughout Belgium (website: www.treesforfutrure.be/en, accessed November 2022).
The aim of the Swiss CG network is to find suitable tree species and seed sources for the future climate along an elevation gradient in all regions of Switzerland. The establishment of the network, testing 117 seed sources of 18 species at 57 experimental sites, started in 2020. (see section 3 for details).
In France, several new CGs have been established recently within the project ESPERENSE with the goal of identifying climate-tolerant tree species. This project started in 2020 with two experiments at eight sites, following an analogue-climate approach, i.e., using four sites that are currently exposed to the climate predicted for the other four sites toward the end of the century. In one of the experiments (ESPERENSE I), the survival of 30 species over 10 years will be studied. In the other experiment (ESPERENSE II), the growth of 8 tree species over approximately 30 years will be tested (Kebli et al., 2019; Kebli et al., 2022; ONF, 2022).
Further experiments are planned across Europe in the framework of the OptForests project (https://www.optforests.eu), which will also include a site in Switzerland managed by the Swiss CG team (planting is planned for autumn 2025). The above list of CG experiments is likely incomplete since many European countries have recently begun to invest into projects to find suitable tree species to ensure important ecosystem services for the future.
2.2.3 Comparison of experiments
The 16 experiments listed in Table 2 show considerable variation in the number of sites (3–57), the number of species (2–70), the number of seed sources or genetic units (4–2,300), the spacing between trees at the time of planting (0.1–3 m), and the duration of the trials (10–50+ years; Table 2). Overall, the currently running or planned experiments in Europe include about 140 tree species from approximately 2,500 different seed sources on 185 sites. The most frequently selected tree species across all trials are Quercus petraea, Cedrus atlantica and Pseudotsuga menziesii (all tested in 9 of 16 experiments), followed by Quercus robur and Pinus sylvestris (both in 7 experiments) and Cedrus libani, Larix decidua and Pinus nigra (in 6 experiments). The 185 experimental sites cover gradients of mean annual temperatures between 3°C and 16°C (median 10°C) and of mean growing season temperatures (months with mean temperatures above 5°C) between 10°C and 16°C (median 13°C). The annual precipitation sums of the experimental sites range from 511 mm to 2,260 mm (median 972 mm), and average monthly precipitation during the growing season ranges from 43 mm to 187 mm (median 80 mm) (Figure 5A). The climatic water balance (CWB) during the growing season at the experimental sites ranges from −46 mm to +158 mm (median + 9 mm). Nearly one-third of the sites (53 of 185) have a negative CWB, meaning that the potential evapotranspiration is higher than the average precipitation sum (sites below the 1:1 line in Figure 5B). In comparison to the other experiments, the Swiss CG network covers the largest gradient of CWB, ranging from −29 mm to +158 mm (median + 26 mm), followed by REINFFORCE, with CWB ranging from −46 mm to +96 mm (median + 3 mm). While the Swiss CG network includes sites with humid and cold climates, REINFFORCE covers humid and warm climates along the European Atlantic coast (Figure 5B). The French Quercus trial, the species shift trial, the exotic species trial, SILVITI, and ESPERENSE I and II focus mainly on the dry end of the gradient by including sites with a CWB over the growing season ranging from −42 mm to +7 mm (median − 11 mm).
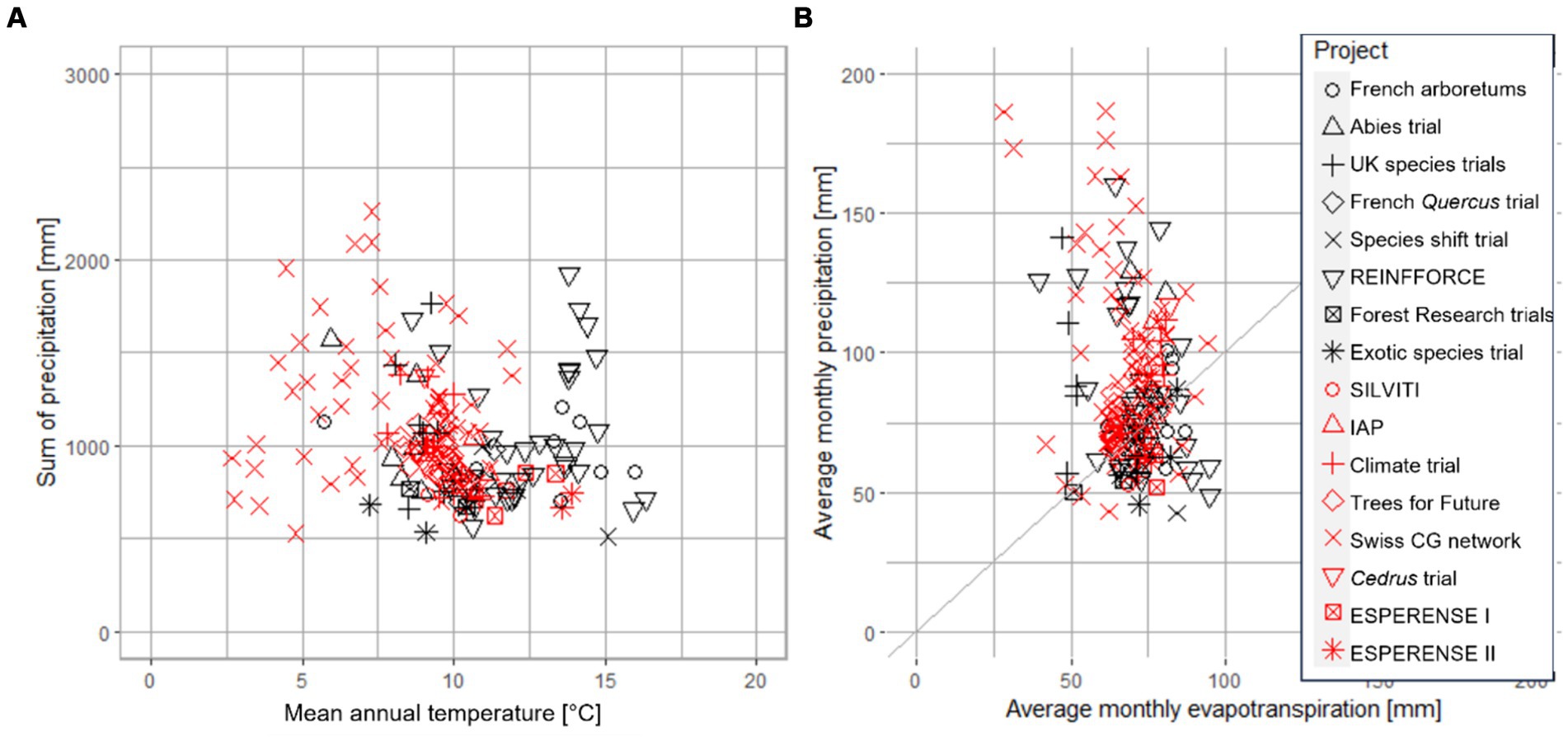
Figure 5. Annual precipitation sum [mm] vs. mean annual temperature [°C] at 185 experimental sites in 16 projects (A). Average monthly precipitation [mm] vs. average monthly potential evapotranspiration [mm] after Turc during the growing season at the same sites (B). Sites above the diagonal 1:1 line are wet and sites below are dry.
2.2.4 Filling a niche among European common garden experiments
Most of the currently existing and the planned CG experiments in Europe focus on warm and dry sites (Table 2; Figure 5A) because this is where most countries expect the most severe problems to occur, and hence they are urged to find suitable species for these climates. For Switzerland, however, it is not sufficient to limit trials to the warmest and driest sites, because important ecosystem services, such as the protection against natural hazards, are also required at higher elevations. The climatic suitability of the current tree species is likely to become an issue in these regions as well (Moos et al., 2023). Other tree species already present at lower elevations in Switzerland may be suitable candidates for replacing tree species at higher elevations. However, seed trees of these species are currently mostly absent in these regions.
To identify factors that determine the survival, vitality and growth of tree species, CG networks need to cover broad environmental gradients (Fady and Rihm, 2022). A gradient can be extended by broadening the network, thus meta-analyzing data from multiple European CG experiments together. The REINFFORCE project and the UK species trial offer especially high potential for collaboration, as they contain many sites and cover broad gradients in Atlantic climates (Correia et al., 2018). Whereas REINFFORCE expands to humid and warm climates, the Swiss CG network reaches to humid and cool climates thereby complementing the range covered by REINFFORCE (Figure 5B).
3 Discussion
3.1 The importance of assisted migration experiments for climate change adaptation in forestry
In an evolving climate with more frequent extreme events, forest services are at stake. Assisted migration is recognized as a key strategy for mitigating climate change and maintaining optimum forest health, productivity and ecosystem services (Aitken and Bemmels, 2016). However, to prevent negative ecological implications (Schwartz et al., 2012; Klein and Arts, 2022), assisted migration should be tested in a coordinated network rather than in individual pilot plantations. In-situ plantations in a forest environment have many benefits over experiments in controlled environments, such as greenhouses or growth chambers. First, in-situ trials can run for several decades, capturing the effects of a combination of hazards and extreme climatic events on trees and providing valuable results regarding mature trees. Second, these mature trees can serve as seed trees for the next generation of trees, providing a head start for forest adaptation.
Since the establishment of in-situ trials is labor-intensive, the recombination of existing planting trials created for other reasons, even including pilot plantations of practitioners, may alternatively be used to answer all kinds of research questions (e.g., for dendrochronological, genetic, pest resistance, or biodiversity studies). However, due to differing designs and other experimental variables, which must be accounted for in the analysis, the power to detect the factors that determine the survival, vitality and growth of tree species is lower than in assisted migration experiments with a coherent design. Still, the recombination approach is valuable and followed in several countries, e.g., France (Rihm and Fady, 2023) and Austria (Kristöfel, 2015). In Switzerland, an online application for the documentation of the occurrence of future tree species (from plantations and natural regeneration) was released in April 2024 (www.zukunftsbaumarten.ch, collaboration between the Education Center for Forest BZW in Maienfeld, School of Agricultural, Forest and Food Sciences HAFL and WSL). It will facilitate the exchange between practitioners.
To broaden the potential network of existing planting trials, these approaches should not be restricted to single countries but cover continents. Gaining an overview of experiments testing multiple species on multiple sites in Europe is therefore essential. European initiatives that aim to improve networking across country borders have recently started. The Swiss CG project team contributes expertise, data and research infrastructure to the initiatives OptForest, a Horizon project lead by INRAE, and In-Sylva (proposed for funding), to enlarge the potential network.
3.2 Experiences with the participatory approach for the Swiss common garden network
The objective of the participatory process for planning and designing the Swiss CG network was to ensure that the design of the initiative not only meets scientific requirements, but also integrates the questions and requirements of the various stakeholder groups (Figure 1). While it was not possible to accommodate all expectations of the stakeholders, the participatory process increased acceptance of design decisions and enhanced the support for the project. Nevertheless, the scientific team was aware that while stakeholder input was clearly valuable, it was also important not to compromise scientific rigor, in particular regarding the statistical power of the experimental design. An important drawback of the participatory process was that it increased the complexity of the decision processes, particularly when many stakeholders contributed to a discussion, and thus additional time was required to reach final decisions.
3.3 Trade-offs and challenges in designing the Swiss common garden network
When designing a large-scale experiment like the Swiss CG network, some compromises between statistical desirability and practical feasibility are inevitable, given limited resources. During the project planning phase, such conflicts of interest were negotiated between the stakeholders and the project’s scientific team. For instance, an important question that arose was the number of CGs in which a tree species should be tested: while it was desirable to test each tree species in many CGs to obtain statistically sound results, the number of suitable sites with the necessary size to establish a CG and the resources available for establishing and maintaining the sites were limited. A power analysis, which was conducted to find the minimum number of sites necessary to answer the research questions with statistical validity, revealed that a species should be tested on at least 30 sites.
Another point of discussion was the number of tree species to be included in the experiment. While the cantons were generally interested in testing a large number of species, a compromise had to be found because both the number and size of sites were limited. For each of the 18 species in the core and extension sets combined, we expect to obtain data on mortality, plant damage and growth performance across the environmental gradient. Where environmental gradients of tree species overlap, interspecific comparisons of species performance will also be possible. For the nine tree species of the core set, statistically sound results can be expected for at least five explanatory variables. The data generated for the species of the extension set are restricted to fewer explanatory variables, to avoid reducing explanatory power.
The number of seed sources to be tested per species presented a similar challenge. The initial intention to include four seed sources per species was discarded because of concerns that this number would not be sufficient to adequately represent the expected within-species genetic variation. Considering the limited size and number of CGs and the fact that reducing the number of species was not a suitable option, the number of seed sources per species was increased to seven, but with the constraint that only four seed sources would be planted per CG. This increased the genetic variation represented in the experiment at the cost of reducing the statistical power to analyze the performance of individual seed sources. However, since the primary aim of the Swiss CG network is to draw conclusions about the species rather than at the seed source level, this compromise was accepted.
For an optimal representation of within-species genetic variation, seed sources should represent large parts of a species’ habitat, which also requires taking postglacial migration history into consideration. Due to financial, technical and legal restrictions, it was not possible to harvest seed sources outside of Switzerland specifically for the project. Therefore, mostly commercially available seed sources were procured, but in some cases they were complemented with seed material obtained through research contacts in their respective regions of origin. Forest sanitary concerns also had to be considered. For example, the purchase of native Pseudotsuga menziesii seed sources had to be limited to a range from Washington to northern Oregon, while seeds from any more southern seed sources, e.g., California and southern Oregon, could not be imported because of restrictions due to the pathogen Gibberella circinata (Gordon et al., 2006), even though these sources might have been interesting for their adaptation to warmer and drier climates.
The cultivation of the reproductive material was challenging in this project. Even after the successful procurement of seeds, seed material from some seed sources did not germinate, or it was impossible to get seeds from the same seed sources again in the following year. Generally, 2020 was a bad seed year, leading to missing seed material from many seed sources in autumn 2021. These restrictions in plant availability from certain seed sources in certain years affected the optimal distribution of the seed sources across the sites and resulted, in some cases, in subplots that had to be left empty.
The selected CG sites in forests cover a large part of the environmental gradient in Switzerland. On average, the Swiss CG network contains one site per 21,000 ha of forested land in Switzerland. In comparison to the forested land area in the different elevation belts (forested land area without shrubs according to the Swiss National Forest Inventory, Abegg et al., 2020), lower sites (below 600 m a.s.l.) are overrepresented (1 site per 12,000 ha of forested land), while higher sites (above 1,000 m a.s.l.) are underrepresented (1 site per 26,000 ha of forested land). The likely reason for the underrepresentation of higher sites is that forests at higher elevations are often less intensively managed because of excessive costs due to difficult terrain and limited accessibility. Further, since the protection against natural hazards is usually the main management target in such forests, this prohibits the creation of larger canopy openings. Therefore, it was usually not possible to establish CG sites in mountain protective forests except in cases where there were pre-existing openings created by natural disturbances, mainly windthrows.
In our experiment, tree species are tested on forest sites under open conditions. Thus, shading and competition between different tree species and between individuals of the same species are excluded as factors influencing tree vitality and growth, leaving only the influence of environmental factors. This setting diminishes, however, the relevance of our results for smaller gaps within forests, where shading and competition play a central role.
3.4 Enhancing the value of the Swiss common garden network
The establishment of the Swiss CG network opens valuable opportunities to study additional research questions beyond the original project goals. The CG team welcomes add-on projects, as long as they do not interfere with the goals of the main project. In a workshop with interested researchers in March 2019, several add-on projects were initiated. In the Swiss Biomass project, the stocked stands on a subset of the experimental sites were investigated before clear-cutting. The data were used to improve the allometric functions, which are important to inform models of carbon storage in forests. The Polytunnel project involves using greenhouses to experimentally extend the climatic gradient beyond the warm and dry range edge of the CG network sites. Specifically, at three CG sites the effects of experimental warming and precipitation reduction on the phenology and growth of a subset of six tree species are being examined. This project was started in 2021 and is planned to run for 10 years. Further, phytopathological monitoring is being conducted on some CG sites to detect the introduction of pathogens, which is an important concern in assisted migration projects. For at least 5 years, this yearly monitoring will be conducted on two sites that each host all 18 tree species and complement each other to host all 117 seed sources. Some of the sites will be used to test new airborne or terrestrial laser-based monitoring techniques. Additional projects on plant–soil interactions and effects of leaf and root biomes on plant mortality are currently being proposed for funding. The initiation of further add-on projects would be desirable. The monitoring of additional parameters could make it possible to answer more questions. For instance, a comprehensive understanding of genetic variation within species and a thorough phenotypic characterization would enhance discussions on intraspecific variation in mortality and growth, as well as phenotypic plasticity. Additionally, the analysis of isotopic variation in leaf matter could shed light on tree species physiological responses to drought.
4 Conclusion
Climate change, with rapidly rising temperatures and an increasing frequency and severity of droughts, may question the continued existence of forests in many regions. Many tree species will cease to be viable in their current habitats. Science-based targeted interventions are therefore urgently needed to promote tree species that can cope with the climate of the future and thus enable forest ecosystems to continue to provide ecosystem services. With these aims, several initiatives have started in recent years across Europe. The recently established Swiss CG network is currently the largest network in Europe dedicated to the search for tree species that can cope with the future climate.
The Swiss CG network lays the foundation for a long-term research program that is tailored to the specific questions and requirements of forest stakeholders in Switzerland, such as the need for protection against natural hazards in mountain forests, but it also fits well within a potential network of European approaches. Therefore, it opens opportunities for common evaluations and meta-analyses. In addition, it offers possibilities for add-on projects, which answer additional research questions. With the findings from the Swiss CG network, we expect to provide politicians, forest authorities and practitioners with scientific evidence to make informed management decisions that secure the future of Swiss forests and the ecosystem services they provide.
Data availability statement
Publicly available datasets were analyzed in this study. This data can be found here: We only used approximate site locations as well as species and design information from European common garden networks. Climate data were derived from CHELSA (Karger et al., 2017).
Author contributions
KS: Conceptualization, Formal analysis, Funding acquisition, Methodology, Project administration, Visualization, Writing – original draft, Writing – review & editing. PB: Conceptualization, Funding acquisition, Methodology, Writing – original draft. EF: Conceptualization, Methodology, Validation, Writing – original draft, Writing – review & editing.
Funding
The authors declare that financial support was received for the research, authorship and/or publication of this article. Open access funding by Swiss Federal Institute for Forest, Snow and Landscape Research (WSL).
Acknowledgments
We are grateful to the numerous colleagues and students who helped during the conceptualization, planning and installation phases of the Swiss CG network. We also thank our international colleagues, especially Bruno Fady, Bill Mason, Brigitte Musch and Victoria Stokes, who supported the project with valuable advice. We further acknowledge the support of the federal authorities, the cantonal forest authorities, and all other associated stakeholders and institutions. We thank the landowners for the permission to establish common gardens and to work on their land. Finally, we are grateful to Claus-Dieter Meyer from the James Hutton Institute in Aberdeen for statistical advice.
Conflict of interest
The authors declare that the research was conducted in the absence of any commercial or financial relationships that could be construed as a potential conflict of interest.
Publisher’s note
All claims expressed in this article are solely those of the authors and do not necessarily represent those of their affiliated organizations, or those of the publisher, the editors and the reviewers. Any product that may be evaluated in this article, or claim that may be made by its manufacturer, is not guaranteed or endorsed by the publisher.
Supplementary material
The Supplementary material for this article can be found online at: https://www.frontiersin.org/articles/10.3389/ffgc.2024.1396798/full#supplementary-material
References
Abegg, M., Brändli, U.-B., Cioldi, F., Fischer, C., Herold, A., Meile, R., et al., (2020). Schweizerisches Landesforstinventar Lfi. Ergebnistabellen Und Karten Der Lfi-Erhebungen 1983–2017 (Lfi1, Lfi2, Lfi3, Lfi4) Im Internet. Available at: http://Www.Lfi.Ch/Resultate (Accessed June 17, 2024).
Aitken, S. N., and Bemmels, J. B. (2016). Time to get moving: assisted gene flow of Forest trees. Evol. Appl. 9, 271–290. doi: 10.1111/eva.12293
Aitken, S. N., Yeaman, S., Holliday, J. A., Wang, T., and Curtis-Mclane, S. (2008). Adaptation, migration or extirpation: climate change outcomes for tree populations. Evol. Appl. 1, 95–111. doi: 10.1111/j.1752-4571.2007.00013.x
Alizoti, P., Bastien, J. C., Chakraborty, D., Klisz, M. M., Kroon, J., Neophytou, C., et al. (2022). Non-native Forest tree species in Europe: the question of seed origin in afforestation. Forests 13:13. doi: 10.3390/f13020273
Allen, C. D., Breshears, D. D., and Mcdowell, N. G. (2015). On underestimation of global vulnerability to tree mortality and forest die-off from hotter drought in the Anthropocene. Ecosphere 6, 1–55. doi: 10.1890/ES15-00203.1
Anderegg, W. R. L., Kane, J. M., and Anderegg, L. D. L. (2013). Consequences of widespread tree mortality triggered by drought and temperature stress. Nat. Clim. Chang. 3, 30–36. doi: 10.1038/nclimate1635
Angst, J. K., and Kupferschmid, A. D. (2023). Assessing browsing impact in beech forests: the importance of tree responses after browsing. Diversity 15:262. doi: 10.3390/d15020262
Aubin, I., Garbe, C. M., Colombo, S., Drever, C. R., Mckenney, D. W., Messier, C., et al. (2011). Why we disagree about assisted migration: ethical implications of a key debate regarding the future of Canada's forests. For. Chron. 87, 755–765. doi: 10.5558/tfc2011-092
Bafu (2019). Nationaler Kataster Der Samenerntebestände Nks. Available at: https://www.nks.admin.ch (Accessed June 17th 2024).
Bauhus, J., Puettmann, K. J., and Kühne, C. (2013). Close-to-nature forest management in Europe: Does it support complexity and adaptability of forest ecosystems? In Managing forests as complex adaptive systems: Building resilience to the challenge of global change. eds. C. Messier, K. J. Puettmann, and K. D. Coates (London Routledge).
Bert, D., Lebourgeois, F., Ponton, S., Musch, B., and Ducousso, A. (2020). Which oak provenances for the 22nd century in Western Europe? Dendroclimatology in common gardens. PLoS One 15:E0234583. doi: 10.1371/journal.pone.0234583
Bertrand, R., Lenoir, J., Piedallu, C., Riofrío-Dillon, G., De Ruffray, P., Vidal, C., et al. (2011). Changes in plant community composition lag behind climate warming in lowland forests. Nature 479, 517–520. doi: 10.1038/nature10548
Binkley, D. (2008). Three key points in the design of Forest experiments. For. Ecol. Manag. 255, 2022–2023. doi: 10.1016/j.foreco.2008.01.047
Bolte, A., Ammer, C., Löf, M., Madsen, P., Nabuurs, G.-J., Schall, P., et al. (2009). Adaptive Forest management in Central Europe: climate change impacts, strategies and integrative concept. Scand. J. For. Res. 24, 473–482. doi: 10.1080/02827580903418224
Bottero, A., Forrester, D. I., Cailleret, M., Kohnle, U., Gessler, A., Michel, D., et al. (2021). Growth resistance and resilience of mixed silver fir and Norway spruce forests in Central Europe: contrasting responses to mild and severe droughts. Glob. Chang. Biol. 27, 4403–4419. doi: 10.1111/gcb.15737
Brändli, U.-B. (1998). Die Häufigsten Waldbäume Der Schweiz. Egebnisse Aus Dem Landesforstinventar 1983–85: Verbreitung, Standort Und Häufigkeit Von 30 Baumarten. Berichte Der Eidgenössischen Forschungsanstalt für Wald, Schnee und Landschaft. Birmensdorf: Swiss Federal Institut Wsl.
Brang, P., Spathelf, P., Larsen, J. B., Bauhus, J., Boncčìna, A., Chauvin, C., et al. (2014). Suitability of close-to-nature Silviculture for adapting temperate European forests to climate change. Forestry 87, 492–503. doi: 10.1093/forestry/cpu018
Brodribb, T. J., Powers, J., Cochard, H., and Choat, B. (2020). Hanging by a thread? Forests and drought. Science 368, 261–266. doi: 10.1126/science.aat7631
Brüllhardt, M., Rotach, P., Forrester, D. I., and Bugmann, H. (2022). Sustainable regeneration in uneven-aged mixed deciduous forests managed by selection Silviculture: the role of demographic structure. Forestry 95, 201–214. doi: 10.1093/forestry/cpab041
Bürgi, A., and Dietz, C. (1986). Übersicht Über Den Exotenanbau In Der Schweiz Aufgrund Einer Umfrage Vom Herbst/Winter 1984/85. Schweiz. Z. Forstwes. 137, 833–851.
Burrows, M. T., Schoeman, D. S., Richardson, A. J., Molinos, J. G., Hoffmann, A., Buckley, L. B., et al. (2014). Geographical limits to species-range shifts are suggested by climate velocity. Nature 507, 492–495. doi: 10.1038/nature12976
Cailleret, M., Jansen, S., Robert, E. M. R., Desoto, L., Aakala, T., Antos, J. A., et al. (2017). A synthesis of radial growth patterns preceding tree mortality. Glob. Chang. Biol. 23, 1675–1690. doi: 10.1111/gcb.13535
Champagne, E., Raymond, P., Royo, A. A., Speed, J. D. M., Tremblay, J. P., and Côté, S. D. (2021). A review of ungulate impacts on the success of climate-adapted Forest management strategies. Curr. For. Rep. 7, 305–320. doi: 10.1007/s40725-021-00148-5
Chen, I.-C., Hill, J. K., Ohlemüller, R., Roy, D. B., and Thomas, C. D. (2011). Rapid range shifts of species associated with high levels of climate warming. Science 333, 1024–1026. doi: 10.1126/science.1206432
Choat, B., Brodribb, T. J., Brodersen, C. R., Duursma, R. A., López, R., and Medlyn, B. E. (2018). Triggers of tree mortality under drought. Nature 558, 531–539. doi: 10.1038/s41586-018-0240-x
Clark, J. S., Iverson, L., Woodall, C. W., Allen, C. D., Bell, D. M., Bragg, D. C., et al. (2016). The impacts of increasing drought on Forest dynamics, structure, and biodiversity in the United States. Glob. Chang. Biol. 22, 2329–2352. doi: 10.1111/gcb.13160
Commarmot, B. (1995). Internationaler Weisstannen-Herkunftsversuch: Entwicklung Der Herkünfte Bis Zum Alter 12 Auf Der Versuchsfläche Bourrignon Im Schweizer Jura. Ergebnisse Des 7. Iufro Tannen-Symposium Der Wp S. 1.01-08, «Ökologie Und Waldbau Der Weisstanne» ed. W. EderIUFRO. 59–68.
Commarmot, B. (1997). Anbauversuch Mit Kalabrischen Tannen In Verschiedenen Höhenlagen – Erste Ergebnisse. Schweiz. Z. Forstwes. 148, 353–365.
Correia, A. H., Almeida, M. H., Branco, M., Tomé, M., Cordero Montoya, R., Di Lucchio, L., et al. (2018). Early survival and growth plasticity of 33 species planted in 38 arboreta across the European Atlantic area. Forests 9:630. doi: 10.3390/f9100630
De Rigo, D., Caudullo, G., Houston Durrant, T., and San-Miguel-Ayanz, J. (2016). “The European atlas of Forest tree species: modelling, data and information on Forest tree species” in European atlas of Forest tree species. eds. J. San-Miguel-Ayanz, D. De Rigo, G. Caudullo, T. Houston Durrant, and A. Mauri (Luxembourg: Publications Office of the European Union).
Del Río, M., Vergarechea, M., Hilmers, T., Alday, J. G., Avdagić, A., Binderh, F., et al. (2021). Effects of elevation-dependent climate warming on intra- and inter-specific growth synchrony in mixed mountain forests. For. Ecol. Manag. 479:118587. doi: 10.1016/j.foreco.2020.118587
Dietz, L., Gégout, J. C., Dupouey, J. L., Lacombe, E., Laurent, L., and Collet, C. (2022). Beech and hornbeam dominate oak 20 years after the creation of storm-induced gaps. For. Ecol. Manag. 503:119758. doi: 10.1016/j.foreco.2021.119758
Ducatillion, C., Lamant, T., Bellanger, R., Bouttier, V., Bastien, J.-C., Michotey, C., et al. (2022). Survival and growth of 711 Forest tree taxa in eight French arboretums from three different climate regions. Ann Silvicul Res. 47, 30–38. doi: 10.12899/asr-2325
Dumroese, R. K., Williams, M. I., Stanturf, J. A., and Clair, J. B. S. (2015). Considerations for restoring temperate forests of tomorrow: Forest restoration, assisted migration, and bioengineering. New For. 46, 947–964. doi: 10.1007/s11056-015-9504-6
Etzold, S., Ziemińska, K., Rohner, B., Bottero, A., Bose, A. K., Ruehr, N. K., et al. (2019). One century of Forest monitoring data in Switzerland reveals species- and site-specific trends of climate-induced tree mortality. Front. Plant Sci. 10:307. doi: 10.3389/fpls.2019.00307
Fady, B., and Rihm, G. (2022). Arboretums, common gardens and Forest tree resilience. New For. 53, 603–606. doi: 10.1007/s11056-022-09908-y
Fairbridge, R. W., and Oliver, J. E. (2005). “Lapse Rate” in Encyclopedia of world climatology. ed. J. E. Oliver (Dordrecht: Springer Netherlands).
Forrester, D. I., Schmid, H., and Nitzsche, J. (2021). Growth and structural changes in Swiss uneven-aged forests over 100 years, and comparisons between 15 uneven-aged Forest types of Europe, North America and Australia. Forestry 95, 166–186. doi: 10.1093/forestry/cpab042
Forzieri, G., Girardello, M., Ceccherini, G., Spinoni, J., Feyen, L., Hartmann, H., et al. (2021). Emergent vulnerability to climate-driven disturbances in European forests. Nat. Commun. 12, 1–12. doi: 10.1038/s41467-021-21399-7
Frank, A., Howe, G. T., Sperisen, C., Brang, P., Clair, J. B. S., Schmatz, D. R., et al. (2017). Risk of genetic maladaptation due to climate change in three major European tree species. Glob. Chang. Biol. 23, 5358–5371. doi: 10.1111/gcb.13802
Frei, E. R., Streit, K. E., and Brang, P. (2018). Testpflanzungen Zukunftsfähiger Baumarten: Auf Dem Weg Zu Einem Schweizweiten Netz. Schweizerische Zeitschrift Fur Forstwesen 169, 347–350. doi: 10.3188/szf.2018.0347
Fririon, V., Davi, H., Oddou-Muratorio, S., Lebourgeois, F., and Lefèvre, F. (2023). Within and between population phenotypic variation in growth vigor and sensitivity to drought stress in five temperate tree species. For. Ecol. Manag. 531:120754. doi: 10.1016/j.foreco.2022.120754
Frischbier, N., Nikolova, P. S., Brang, P., Klumpp, R., Aas, G., and Binder, F. (2019). Climate change adaptation with non-native tree species in central European forests: Early tree survival in a multi-site field trial. Eur. J. For. Res. 138, 1015–1032. doi: 10.1007/s10342-019-01222-1
Glatthorn, J., Maier, B., Binder, F., Brang, P., Frischbier, N., Horna, V., et al. (2024). Limited influence of air temperature and precipitation on six-year survival and growth of non-native tree species in a central European multi-site field trial. For. Ecol. Manag. 553:121645. doi: 10.1016/j.foreco.2023.121645
Gordon, T. R., Kirkpatrick, S. C., Aegerter, B. J., Wood, D. L., and Storer, A. J. (2006). Susceptibility of Douglas fir (Pseudotsuga Menziesii) to pitch canker, caused by Gibberella Circinata (anamorph = Fusarium Circinatum). Plant Pathol. 55, 231–237. doi: 10.1111/j.1365-3059.2006.01351.x
Hagerman, S., and Kozak, R. (2021). Disentangling the social complexities of assisted migration through deliberative methods. J. Ecol. 109, 2309–2316. doi: 10.1111/1365-2745.13667
Hatfield, J. L. (1998). Experiments in ecology: their logical design and interpretation using analysis of variance. J. Environ. Qual. 27:246. doi: 10.2134/jeq1998.00472425002700010038x
Hof, A. R., Dymond, C. C., and Mladenoff, D. J. (2017). Climate change mitigation through adaptation: the effectiveness of Forest diversification by novel tree planting regimes. Ecosphere 8:E01981. doi: 10.1002/ecs2.1981
Illés, G., and Móricz, N. (2022). Climate envelope analyses suggests significant rearrangements in the distribution ranges of central European tree species. Ann. For. Sci. 79:35. doi: 10.1186/s13595-022-01154-8
IPCC (2021). Summary for policymakers in The physical science basis. Contribution of Working Group I to the sixth assessment report of the intergovernmental panel on climate change. eds. V. Masson-Delmotte, P. Zhai, A. Pirani, S. L. Connors, C. Péan, and S. Berger, et al. (Cambridge University Press).
Jump, A. S., and Peñuelas, J. (2005). Running to stand still: adaptation and the response of plants to rapid climate change. Ecol. Lett. 8, 1010–1020. doi: 10.1111/j.1461-0248.2005.00796.x
Karger, D. N., Conrad, O., Böhner, J., Kawohl, T., Kreft, H., Soria-Auza, R. W., et al. (2017). Climatologies at high resolution for the Earth’s land surface areas. Scientific Data 4:170122. doi: 10.1038/sdata.2017.122
Kebli, H., Perrier, C., Riou-Nivert, P., Rousselle, Y., Legay, M., and Morneau, F. (2019). Un Réseau Collectif D’évaluation Du Potentiel D’adaptation Des Essences Et Provenances Pour Le Futur. Forêt-Entreprise 249, 24–26.
Kebli, H., Perrier, C., Riou-Nivert, P., Rousselle, Y., Legay, M., and Morneau, F. (2022). Comment Déterminer L'exposition Aux Changements Climatiques Des Zones De Production Forestière Française? Méthodologie Utilisée Dans Le Projet Esperense Pour Cibler Les Zones D’intérêt Pour L’installation D’essais De Comparaison D’essences Et De Provenances. Revue Forestière Française 73, 523–540. doi: 10.20870/revforfr.2021.7104
Klein, L., and Arts, K. (2022). Public participation in decision-making on conservation translocations: the importance and limitations of a legislative framework. Restor. Ecol. 30:E13505. doi: 10.1111/rec.13505
Kunz, J., and Bauhus, J. (2015). “Das Potenzial Seltener Und Trockentoleranter Laubbaumarten Zur Aufforstung Von Aufgelassenen Weinbergen” in Biodiversität Und Klima - Vernetzung Der Akteure In Deutschland Xi. eds. H. Korn, K. Bockmühl, and R. Schliep (Bundesamt Für Naturschutz: Bonn).
Larsen, J. B. (1986). Die Geographische Variation Der Weißtanne (Abies Alba Mill.) Wachstumsentwicklung Und Frostresistenz. Forstwissenschaftliches Centralblatt 105, 396–406. doi: 10.1007/BF02741748
Ligot, G., Balandier, P., Fayolle, A., Lejeune, P., and Claessens, H. (2013). Height competition between Quercus Petraea and Fagus Sylvatica natural regeneration in mixed and uneven-aged stands. For. Ecol. Manag. 304, 391–398. doi: 10.1016/j.foreco.2013.05.050
Mason, W. L. (2012). The role of true fir species in the Silviculture of British forests: past, present and future. J. For. Facul. Kastamonu Univ. 15–26.
Mc Lachlan, J. S., Hellmann, J. J., and Schwartz, M. W. (2007). A framework for debate of assisted migration in an era of climate change. Conserv. Biol. 21, 297–302. doi: 10.1111/j.1523-1739.2007.00676.x
Mcdowell, N. G., Allen, C. D., Anderson-Teixeira, K., Aukema, B. H., Bond-Lamberty, B., Chini, L., et al. (2020). Pervasive shifts in Forest dynamics in a changing world. Science 368:Eaaz9463. doi: 10.1126/science.aaz9463
Merlin, M., Duputié, A., and Chuine, I. (2018). Limited validation of forecasted northward range shift in ten European tree species from a common garden experiment. For. Ecol. Manag. 410, 144–156. doi: 10.1016/j.foreco.2018.01.001
Mina, M., Huber, M. O., Forrester, D. I., Thürig, E., and Rohner, B. (2018). Multiple factors modulate tree growth complementarity in central European mixed forests. J. Ecol. 106, 1106–1119. doi: 10.1111/1365-2745.12846
Moos, C., Stritih, A., Teich, M., and Bottero, A. (2023). Mountain protective forests under threat? An in-depth review of global change impacts on their protective effect against natural hazards. Front. Forests Glob. Change. 6:1223934. doi: 10.3389/ffgc.2023.1223934
Nyssen, B., Schmid, U. E., Muys, B., van der Lei, P. B.., and Pyttel, P. (2016). in The history of introduced tree species in Europe in a nutshell in Introduced tree species to European forests: challenges and opportunities. ed. F. Krumm (Freiburg: European Forest Institute), 44–55.
OECD. (2024). The OECD Scheme for the Certification of Forest Reproductive Material. Available at: http://www.oecd.org/Agriculture/Forest/. (Accessed June 17, 2024).
ONF. (2022). Esperense: L’expérimentation Au service de la forêt de demain. RenDez-vous Techniques, 74, 1–42.
Palik, B. J., Clark, P. W., D'amato, A. W., Swanston, C., and Nagel, L. (2022). Operationalizing Forest-assisted migration in the context of climate change adaptation: examples from the eastern Usa. Ecosphere 13:E4260. doi: 10.1002/ecs2.4260
Paquette, A., Hector, A., Castagneyrol, B., Vanhellemont, M., Koricheva, J., Scherer-Lorenzen, M., et al. (2018). A million and more trees for science. Nat. Ecol. Evol. 2, 763–766. doi: 10.1038/s41559-018-0544-0
Park, A., and Rodgers, J. L. (2023). Provenance trials in the service of forestry assisted migration: a review of north American field trials and experiments. For. Ecol. Manag. 537:120854. doi: 10.1016/j.foreco.2023.120854
Parmesan, C., and Yohe, G. (2003). A globally coherent fingerprint of climate change impacts across natural systems. Nature 421, 37–42. doi: 10.1038/nature01286
Pretzsch, H. (2005). “Diversity and productivity in forests: evidence from long-term experimental plots” in Forest diversity and function: Temperate and boreal systems. eds. M. Scherer-Lorenzen, C. Körner, and E.-D. Schulze (Berlin, Heidelberg: Springer Berlin Heidelberg).
Prieto-Recio, C., Bravo, F., and Diez, J. (2011). Resource infrastructure for monitoring and adapting European Atlantic forests under changing climate (REINFFORCE): Establishing a network of arboretums and demonstration sites to assess damages caused by biotic and abiotic factors. J. Agricul. Exten. Rural Develop. 4, 241–245.
Reynolds, C., Jinks, R., Kerr, G., Parratt, M., and Mason, B. (2021). Providing the evidence base to diversify Britain’s forests: initial results from a new generation of species trials. Q. J. For. 115, 26–37.
Rihm, G., and Fady, B. (2023). Caractériser L’enveloppe Climatique Future Des Jardins Communs Forestiers: Une Approche Essentielle Pour Mieux Raisonner L’adaptation Des Essences. Revue Forestière Française 74, 495–506. doi: 10.20870/revforfr.2023.7900
Sáenz-Romero, C., Lamy, J.-B., Ducousso, A., Musch, B., Ehrenmann, F., Delzon, S., et al. (2017). Adaptive and plastic responses of Quercus Petraea populations to climate across Europe. Glob. Chang. Biol. 23, 2831–2847. doi: 10.1111/gcb.13576
Saltré, F., Duputié, A., Gaucherel, C., and Chuine, I. (2015). How climate, migration ability and habitat fragmentation affect the projected future distribution of European beech. Glob. Chang. Biol. 21, 897–910. doi: 10.1111/gcb.12771
Sansilvestri, R., Frascaria-Lacoste, N., and Fernández-Manjarrés, J. F. (2015). Reconstructing a deconstructed concept: policy tools for implementing assisted migration for species and ecosystem management. Environ. Sci. Pol. 51, 192–201. doi: 10.1016/j.envsci.2015.04.005
Schuldt, B., Buras, A., Arend, M., Vitasse, Y., Beierkuhnlein, C., Damm, A., et al. (2020). A first assessment of the impact of the extreme 2018 summer drought on central European forests. Basic Appl. Ecol. 45, 86–103. doi: 10.1016/j.baae.2020.04.003
Schwartz, M. W., Hellmann, J. J., Mclachlan, J. M., Sax, D. F., Borevitz, J. O., Brennan, J., et al. (2012). Managed relocation: integrating the scientific, regulatory, and ethical challenges. Bioscience 62, 732–743. doi: 10.1525/bio.2012.62.8.6
Seidl, R., Thom, D., Kautz, M., Martin-Benito, D., Peltoniemi, M., Vacchiano, G., et al. (2017). Forest disturbances under climate change. Nat. Clim. Chang. 7, 395–402. doi: 10.1038/nclimate3303
Senf, C., Buras, A., Zang, C. S., Rammig, A., and Seidl, R. (2020). Excess Forest mortality is consistently linked to drought across Europe. Nat. Commun. 11:6200. doi: 10.1038/s41467-020-19924-1
Senf, C., Sebald, J., and Seidl, R. (2021). Increasing canopy mortality affects the future demographic structure of Europe's forests. One Earth 4, 749–755. doi: 10.1016/j.oneear.2021.04.008
Şofletea, N., and Budeanu, M. (2015). Response of Norway spruce (Picea Abies (L.) H. Karst) seed stand progenies tested und Er different site conditions. Sumarski List 139, 47–57.
Sousa-Silva, R., Verbist, B., Lomba, Â., Valent, P., Suškevičs, M., Picard, O., et al. (2018). Adapting Forest management to climate change in Europe: linking perceptions to adaptive responses. Forest Policy Econ. 90, 22–30. doi: 10.1016/j.forpol.2018.01.004
Spies, T. A., Giesen, T. W., Swanson, F. J., Franklin, J. F., Lach, D., and Johnson, K. N. (2010). Climate change adaptation strategies for Federal Forests of the Pacific Northwest, Usa: ecological, policy, and socio-economic perspectives. Landsc. Ecol. 25, 1185–1199. doi: 10.1007/s10980-010-9483-0
St Clair, J. B., Howe, G. T., and Kling, J. G. (2020). The 1912 Douglas-fir heredity study: long-term effects of climatic transfer distance on growth and survival. J. For. 118, 1–13. doi: 10.1093/jofore/fvz064
Stephan, B. R., and Liesebach, M. (1997). Results of the Iufro 1982 scots pine (Pinus Sylvestris L.) provenance experiment in southwestern Germany. Silvae Genetica 45, 342–349.
Turc, L. (1961). Evaluation Des Besoins En Eau D'irrigation, Évapotranspiration Potentielle. Ann. Agron. 12, 13–49.
Vitasse, Y., Hoch, G., Randin, C. F., Lenz, A., Kollas, C., and Körner, C. (2012). Tree recruitment of European tree species at their current upper Elevational limits in the Swiss Alps. J. Biogeogr. 39, 1439–1449. doi: 10.1111/j.1365-2699.2012.02697.x
Vitt, P., Havens, K., Kramer, A. T., Sollenberger, D., and Yates, E. (2010). Assisted migration of plants: changes in latitudes, changes in attitudes. Biol. Conserv. 143, 18–27. doi: 10.1016/j.biocon.2009.08.015
Weisgerber, H., and Šindelář, J. (1992). Iufro's role in coniferous tree improvement. History, results, and future of research and international cooperation with European larch (Larix Decidua mill.). Silvae Genetica 41, 150–161.
Williams, M. I., and Dumroese, R. K. (2013). Preparing for climate change: forestry and assisted migration. J. For. 111, 287–297. doi: 10.5849/jof.13-016
Zhu, K., Woodall, C. W., and Clark, J. S. (2012). Failure to migrate: lack of tree range expansion in response to climate change. Glob. Chang. Biol. 18, 1042–1052. doi: 10.1111/j.1365-2486.2011.02571.x
Zischg, A. P., Frehner, M., Gubelmann, P., Augustin, S., Brang, P., and Huber, B. (2021). Participatory modelling of upward shifts of altitudinal vegetation belts for assessing site type transformation in Swiss forests due to climate change. Appl. Veg. Sci. 24:E12621. doi: 10.1111/avsc.12621
Keywords: assisted migration, climate change adaptation, common garden, experimental plantation, future forest, seed source, tree species
Citation: Streit K, Brang P and Frei ER (2024) The Swiss common garden network: testing assisted migration of tree species in Europe. Front. For. Glob. Change. 7:1396798. doi: 10.3389/ffgc.2024.1396798
Edited by:
Kevin Boston, University of Arkansas at Monticello, United StatesReviewed by:
Emma Holmström, Swedish University of Agricultural Sciences, SwedenBrian J. Palik, Northern Research Station, Forest Service (USDA), United States
Copyright © 2024 Streit, Brang and Frei. This is an open-access article distributed under the terms of the Creative Commons Attribution License (CC BY). The use, distribution or reproduction in other forums is permitted, provided the original author(s) and the copyright owner(s) are credited and that the original publication in this journal is cited, in accordance with accepted academic practice. No use, distribution or reproduction is permitted which does not comply with these terms.
*Correspondence: Kathrin Streit, a2F0aHJpbi5zdHJlaXRAd3NsLmNo
†Deceased