- 1Department of Biological Sciences, California State University, San Marcos, CA, United States
- 2Programa de Pós-Graduação em Ciências Ambiental, Universidade de Cuiabá (UNIC), Cuiabá, Brazil
- 3Programa de Pós-Graduação em Física Ambiental, Universidade Federal de Mato Grosso (UFMT), Cuiabá, Cuiabá, Brazil
Productivity and ecosystem carbon (C) storage are often positively associated with species and/or structural diversity; however, positive relationships in tropical forests and woodlands are not universal and the strength of this relationship may be affected by climate. Diversity-productivity relationships were evaluated in upland and seasonally flooded savanna (Cerrado) of the Cuiaba Basin and Northern Pantanal in southern Mato Grosso, Brazil. Data on wood C increment, tree species composition, and alpha diversity were measured over a 10-year period in nine communities located in the Cerrado-Pantanal transition zone. Communities were composed of a wide spectrum of tropical savanna physiognomies, including mixed grassland (campo sujo), typical wooded savanna (stricto sensu), dense wooded savanna (cerrado denso), seasonal forest (mata seca and mata ciliar), and riparian forest (mata de galeria). We hypothesized that tree species richness and diversity would increase from grassland to forest. We further hypothesized that there would be a positive relationship between woody C storage and diversity, but the strength of this relationship would be higher in wet years and wetter environments, such as the Pantanal, due to an increase in water availability. We found that tree species richness and diversity did not increase from mixed grasslands to forests, as mixed grasslands and riparian forests had similarly low levels of tree species richness and diversity compared to the other physiognomies. However, the rate of annual aboveground wood C increment was positively related to species richness and alpha diversity, and the positive relationship was primarily observed during wet years when the annual precipitation was at, or above, the long-term average for the region. Presumably, the impact of structural and species diversity on productivity declines during dry years when water availability becomes a more important control on stem C increment for tree species in the Cerrado-Pantanal transition. These data suggest that maintenance of diversity in these Cerrado woodlands and forests is important for maximizing aboveground C gain. However, climate change, which is causing warming and drying for the region, may limit the importance of diversity on wood C storage.
1 Introduction
Brazilian savanna (Cerrado) is a “hotspot” for plant species diversity, with an estimated 10,000 plant species, approximately 45% of which are endemic to the Cerrado biome, but rapid deforestation has led to the destruction of nearly 45% of the original Cerrado vegetation (Alencar et al., 2020; Colli et al., 2020). The high biodiversity of Cerrado is thought to be due to a variety of factors, including spatial variation in climate, topography, water availability, soil type and fertility, and disturbance (Eiten, 1972; Lopes and Cox, 1977; Furley and Ratter, 1988; Ruggiero et al., 2002; Felfili et al., 2004; Vourlitis et al., 2013, 2019). These same environmental factors also introduce a high level of structural diversity, for example, Cerrado can consist of grasslands (campos), woodlands (stricto sensu), or forests (matas) (Eiten, 1972; Lopes and Cox, 1977; Furley and Ratter, 1988; Ribeiro and Walter, 2008), and high beta-diversity due to species turnover that occurs across these climatic, edaphic, or disturbance gradients (Pellegrini et al., 2016; Silva-Souza et al., 2023).
Biodiversity is a major determinant of ecosystem carbon (C) storage (Paquette and Messier, 2011; Tilman et al., 2014), and in tropical forests, there is often a positive relationship between C storage and species diversity (Gilroy et al., 2014; Poorter et al., 2015; Pellegrini et al., 2016). Given the high biodiversity of Cerrado, and the large structural diversity associated with the various landforms, a positive relationship between diversity and productivity presumably occurs in Cerrado as well. However, diversity-productivity relationships in Cerrado and more complex due to the same variables that promote the high structural diversity. Several studies reported that tree species richness increased from grass-dominated to forested Cerrado (Goodland and Pollard, 1973; Vourlitis et al., 2013), but these relationships may not be universal. For example, Cerrado woodlands (stricto sensu) may have higher species richness than Cerrado forests because woodlands contain species that represent extremes of the Cerrado physiognomic gradient (Batalha et al., 2001). Rupestrian grasslands, which are dominated by grasses and shrubs that are typically on rocky, nutrient-poor soils (Ribeiro and Walter, 2008), reportedly have high species diversity and some of the highest levels of plant endemism in the world (Fernandes et al., 2018). Furthermore, Cerrado areas that are under afforestation typically exhibit an increase in total tree species richness; however, richness of Cerrado endemics declines with forest encroachment because many of these endemics are understory or herbaceous species that are more common in grass dominated Cerrado (Pellegrini et al., 2016). Across Cerrado and Amazon Forest transitions, Santos et al. (2015) and Morandi et al. (2020) found that Cerrado forest (Cerradão), typical woodlands (Cerrado sensu stricto), and dense woodlands (Cerrado denso) were floristically similar and had similar alpha-diversity. Thus, even though Cerrado landforms have large differences in structure, trends in alpha-diversity along this structural gradient are less conclusive.
Variations in Cerrado structure also have implications for above- and belowground carbon (C) storage. Several studies reported an increase in stem density, basal area, diameter, and tree height along the grassland-forest structural gradient (Goodland and Pollard, 1973; Vourlitis et al., 2013), causing an increase in aboveground C storage (Ribeiro et al., 2011; Schöngart et al., 2011; Miranda et al., 2014; Vourlitis et al., 2015, 2019; Morandi et al., 2020; Godlee et al., 2021). Similarly, Cerrado areas that are experiencing forest encroachment typically have an increase in ecosystem C storage due to the rapid colonization of forest trees (Pellegrini et al., 2016). Rates of net primary production (NPP) in grass-dominated savanna are often lower than in woodlands or tree-dominated savanna (Grace et al., 2006; Vourlitis et al., 2022); however, Cerrado grasslands often store substantially more C belowground than other Cerrado structural variants (Terra et al., 2023).
Diversity-productivity relationships in tropical savanna, including Cerrado, are also complicated by interactions with other environmental variables such as climate, disturbance, and/or soil properties. For example, aboveground woody C storage in Cerrado was found to be strongly affected by species diversity, but this relationship was modified by variations in soil fertility (Terra et al., 2023). In African savanna, aboveground C storage was positively linked to species richness, but the strength of this relationship was stronger in wetter areas (Mensah et al., 2023). As mentioned above, tree encroachment typically increases both total tree species richness and C storage; however, fire alters aboveground C storage and helps maintain species richness of Cerrado endemics, which fundamentally alters the diversity-productivity relationships in stands exposed to fire (Pellegrini et al., 2016).
Given the uncertainty in the diversity-productivity relationship in Cerrado we evaluated this relationship in Cerrado stands in the Cuiaba Basin and Northern Pantanal located in southern Mato Grosso, Brazil. This area spans a hydrological gradient from the drier upland Cerrado of the Cuiaba Basin to the seasonally flooded (hyperseasonal) Cerrado of the Pantanal. Here, we looked for evidence of the diversity-productivity relationship both within and between these areas, and asked whether annual rates of aboveground woody C increment were related to tree species richness and alpha-diversity. If tree species richness increases from grass-dominated to forested Cerrado (Goodland and Pollard, 1973; Vourlitis et al., 2013), we hypothesize that there will be an increase in tree species richness, alpha diversity, and stand structural diversity from mixed-grasslands to Cerrado forests in both upland (Cuiaba Basin) and hyperseasonal (Pantanal) Cerrado. If C storage is related to community structure (Grace et al., 2006; Ribeiro et al., 2011; Miranda et al., 2014; Vourlitis et al., 2019), then there should be a positive relationship between woody C storage and tree species diversity in both upland and hyperseasonal Cerrado. If the diversity-productivity relationship is affected by water availability (Hofhansl et al., 2020; Godlee et al., 2021; Mensah et al., 2023), then the strength of the relationship will likely be different depending on rainfall variation and/or hydrological differences between the Cuiaba Basin and the Pantanal. Thus, we hypothesize that there will be a positive relationship between woody C storage and diversity but that the strength of this relationship will vary between the upland and seasonally flooded Cerrado and during times of above- vs. below-average annual precipitation.
Evaluating the diversity-productivity relationship, and the potential for this relationship to vary due to water availability, has important implications for Cerrado NPP and ecosystem C storage as the region becomes warmer and drier with climate change. Climate observations indicate warming and drying trends in the Cerrado-Pantanal transition (Lazaro et al., 2020; Marengo et al., 2021; Hofmann et al., 2021), and because some tree species may be more susceptible to warming and drying than others (Esquivel-Muelbert et al., 2019; Vourlitis et al., 2022), changes in climate will undoubtedly cause changes in community composition, diversity, and presumably, productivity.
2 Materials and methods
2.1 Study area
Field measurements were conducted between 2011 and 2021 in five Cerrado stands in the Cuiaba Basin (hereafter referred to as Cerrado) and four Cerrado stands in the Pantanal (hereafter referred to as Pantanal) located in the Cerrado-Pantanal transition of southern Mato Grosso, Brazil. These study areas have been thoroughly described in several papers (see Arruda et al., 2016; Pinto et al., 2018; Pinto et al., 2020; Vourlitis et al., 2013, 2015; Vourlitis et al., 2014; Vourlitis et al., 2023); however, a brief discussion is provided below.
Communities studied comprised a wide spectrum of tropical savanna physiognomies (nomenclature according to Ribeiro and Walter, 2008), including mixed grassland (campo sujo; Cerrado only), typical wooded savanna (stricto sensu: Cerrado and Pantanal), dense wooded savanna (cerrado denso: Cerrado and Pantanal), seasonal forest (mata seca, Cerrado; mata ciliar, Pantanal), and riparian forest (mata de galeria; Cerrado and Pantanal). Mixed grassland was dominated by native and non-native grasses with scattered short (2–3 m) trees and shrubs. Woodlands consisted of trees and shrubs that were taller (ca. 4–5 m) and denser but with a lower understory cover than the mixed grassland, while dense woodlands had a sparser understory and taller, denser trees than the typical wooded savanna. Seasonal forests had a similar stand density as dense wooded savanna; however, trees were taller (ca. 6–8 m) and the understory vegetation was sparser. Finally, riparian forests were seasonally flooded between December and May and were dominated by Vochysia divergens Pohl; however, other sites such as the typical wooded savanna and seasonal forest in the Pantanal also experienced seasonal flooding between December and May.
Soils underlying the Cerrado sites consisted of Oxisols (Latossolos vermelho-amarelos, according to the Brazilian soil classification system) that were shallow (ca. 50 cm) and stony (Radambrasil, 1982; Gonçalves dos Santos et al., 2018), while soils associated with the Pantanal sites were Oxisols (Plintossolos, according to the Brazilian soil classification system) with higher clay content than the Cerrado soils (Couto and Oliveira, 2011; Gonçalves dos Santos et al., 2018). The long-term (ca. 30 year) annual precipitation of the study region is 1,420 mm, with a dry season occurring from May–September when <5% of the annual precipitation is recorded (Nunes da Cunha and Junk, 2001; Vourlitis and Rocha, 2011). Mean annual temperature the region is 26.5°C, with warmer temperatures during the wet season (Vourlitis and Rocha, 2011). All of the study sites have been protected from fire over 35–40 years.
2.2 Data collection
Data were collected along a permanent 100 m long transect that was randomly located within each community (n = 5 transects in the Cerrado of the Cuiaba Basin and 4 transects in the Pantanal, one transect in each community). We used permanent transects instead of large (0.5–1 ha) plots because our sites were in Cerrado fragments, which are common in southern Mato Grosso (Jasinski et al., 2005). Field sampling was conducted during the dry season when all sites were accessible.
Tree inventories were conducted periodically beginning in 2011 (Cerrado) and 2013 (Pantanal) and ending in 2021. The density and basal area of trees >3 cm in diameter was measured every 5 m on each 100-m transect using the point-centered quarter method (Goldsmith and Harrison, 1976). This technique has been used in temperate forestry for several decades and has the advantage of requiring fewer measurement points to obtain an accurate estimate of tree density (Cottam and Curtis, 1956). For this method, each measurement point was divided into four quadrants, and the distance between the measurement point and the nearest tree in each quadrant was measured. Each tree encountered was tagged and identified to species. Trunk circumference at breast height (1.3 m aboveground) was measured using a metric tape for all tagged trees between 2011 and 2013 (Cerrado) and 2013–15 (Pantanal), but after 2016, changes in trunk circumference were measured annually using plastic, spring-loaded dendrometer bands (Marthews et al., 2012) that were installed on all tagged trees (Vourlitis et al., 2019).
Wood cores were extracted in 2013 using an increment borer from 261 trees in the Cerrado and 350 trees in the Pantanal. Tree cores were measured for volume at the time of extraction and dried at 70°C for approximately 1 week to calculate wood density (ρ = g dry wood/cm3). Wood cores were analyzed for C concentration using the Walkley-Black technique at a local commercial laboratory (AgroAnalise, Cuiaba, Mato Grosso, Brazil).
Leaf area index (LAI) was measured in 2011 and 2012 in Cerrado and in 2013 in the Pantanal. Measurements were made at each sample point along the permanent 100 m transects (n = 20 points/transect) using a portable PAR-ceptometer (Vourlitis et al., 2013, 2015). Understory cover was measured along each permanent transect in 2011 and 2013 in the Cerrado and Pantanal stands, respectively using the line-intercept method, and percent cover was calculated as the sum of the intercepted distances divided by the total transect length (Goldsmith and Harrison, 1976).
Precipitation was measured at meteorological towers that were installed at both sites, but gaps in data coverage rendered these data unreliable. Thus, precipitation data were obtained from the Brazilian Instituto Nacional de Meteorologia (INMET; https://bdmep.inmet.gov.br/; accessed on December 1, 2021) for the Cuiabá station (Station no. 83361), which is located between both research sites.
2.3 Data treatment and statistical analysis
The density of trees for each sample point along a 100 m long transect was calculated as 1/dave2, where dave is the average distance between the sample point and the closest tree in each quadrant (Goldsmith and Harrison, 1976; Barbour et al., 1987). Tree basal area for each sample point was calculated as the mean basal area of the four trees sampled at each point multiplied by the tree density at each point. Mean basal area for each tree was calculated from trunk diameter converted from measurements of circumference at breast height, and trunk diameter at breast height (DBH) was converted to basal area as πDBH2/4. Estimates of tree density and basal area at each sample point were averaged for the transect to provide estimates of density and basal area for the entire stand.
Tree density per species i (Di) was estimated from the stand tree density × the proportion of individuals of species i in the stand, pi = ni/N, where ni is the number of individuals of species i and N is the total number of individuals encountered on each 100 m long transect. Absolute cover of each species (Ci) along each transect was calculated as the sum of the basal area of each individual of species i × Di. The frequency of occurrence (Fi) for each species i was calculated as the number of sample points that species i was encountered along each 100 m long transect divided by the total number of sample points (n = 20/transect). Tree species abundances were calculated as importance values (IVs), which equal the relative density (Di/ΣDtotal) + relative cover (Ci/ΣCtotal) + relative frequency (Fi/ΣFtotal). IVs for each species range from 0 (zero abundance) and 3 (maximum abundance).
We calculated a variety of different indices of species richness and diversity because these indices are often differentially affected by species numbers, dominance, evenness, or rare species (Magurran, 1988). Thus, using only one richness or diversity index may bias our conclusion of how diversity varies between stands and limit the ability to assess potential diversity-productivity relationships.
Species richness for each site was defined as the number of species encountered on each 100 m long permanent transect. Species-area curves were generated by randomly resampling each transect 100 times and counting the mean number of species encountered at each sampling point. Coefficients of the species area curve were estimated from linear regression of the log-power model (referred to as the log-linear model), Log (S) = Log (c) + z Log (T), where S is the number of species encountered on the 100 m long transect, T is a consecutive point on the transect (n = 20 points), and Log (c) and z are the y-intercept and slope, respectively of the linear model describing how Log (S) changes with Log (T) (Gould, 1979; Desilets and Houle, 2005). These coefficients have additional information of how species richness varies with spatial scale. For example, the slope (z) has information on the rate of species addition with an increase in sampling scale (Sagar et al., 2003; Desilets and Houle, 2005), in this case the length of the transect. The y-intercept Log (c) is more difficult to interpret but may indicate a relative difference in the number of taxa between regions that have similar values of z (Gould, 1979).
Two different indices were used to estimate alpha diversity, or the diversity of species within each community. The first index, the Shannon-Weiner H′, is an “information statistic index” that considers the evenness of the species relative abundances (Magurran, 1988) and is calculated as H′ =-Σ pi × LN(pi), where LN is the natural log, and pi calculated as ni/N as described above. H′ values were bootstrapped over 1,000 iterations to estimate the mean H′ (± 95% confidence interval) for each transect (Christie, 2004). The second index was the inverse Simpson’s index, 1/D = 1/Σ[(ni × (ni − 1))/(N × (N-1))], which is an index that is weighed toward the most abundant species in the community (Magurran, 1988).
We calculated an index of structural diversity (ΣV) according to Storch et al. (2018) using 10 different structural variables that were measured from 20 points along each permanent 100 m transect. Structural variables included the mean stem density, the standard deviation (SD) of mean stem density, mean stem diameter, the SD of mean stem diameter, mean stem basal area, the SD of mean stem basal area, leaf area index (LAI), the SD of LAI, understory cover, and the SD of understory cover. These variables were then converted to a variable score (Vi) for each variable i as (X-Xmin)/(Xmax-Xmin), where X = the variable of interest (e.g., mean density for a given site) and Xmin and Xmax were the minimum and maximum values, respectively of variable X for all vegetation types. Variable scores for each variable were summed for each site, and the sum was divided by the number of variables (n = 10) to estimate ΣV for each vegetation type.
Aboveground biomass (AGB; kg/tree) was estimated for each tree using Equation 1 for tropical forest trees described by Chave et al. (2014).
where DBH is the diameter at breast height (1.3 m aboveground) calculated from measurements of trunk circumference, h is an estimate of tree height estimated from measurements of DBH (Schöngart et al., 2011; Vourlitis et al., 2019), and ρ is wood density. AGB was converted to C units (AGC; kgC/tree) by multiplying AGB by the wood C concentration, and the aboveground wood C pool for each site (MgC/ha) was calculated by multiplying AGC by the average stand density (Vourlitis et al., 2019). The annual change in the wood C increment for each stand (MgC ha−1 y−1) was calculated as the difference in the wood C pool over consecutive years (Vourlitis et al., 2019).
Principal components analysis (PCA) was used to characterize relationships between the study sites and their structural characteristics. PCA was run on a correlation matrix using varimax factor rotation, and only factors with eigenvalues >1 were retained for analysis. Repeated-measures ANOVA (RM-ANOVA) was used to assess whether estimates of wood C pool size and annual increment varied as a function of site (Cerrado vs. Pantanal) and time. Data were tested for equality using Box’s M test and compound-symmetry (sphericity) using Mauchly’s test of the between-group covariance matrices, and Greenhouse–Geisser corrections were used for data that failed these assumptions. Analysis of covariance (ANCOVA) was used to quantify linear relationships between indices of species richness, alpha diversity, and structural diversity as co-variates and wood C pool size and annual increment. To assess if diversity-productivity relationships were affected by interannual variations in rainfall (see hypotheses), ANCOVA was also run separately on data collected during wet years (precipitation >1,420 mm, which is the long-term mean precipitation for the region) and dry years (precipitation <1,420 mm). Linear (Pearson-product) correlation was used to determine if correlations between species richness and diversity indices, and correlations between these indices and productivity (wood C storage and increment), were statistically significant. For these analyses we accepted correlations with p < 0.10 to be statistically significant given the low statistical power associated with our sample sizes (n = 5 and 4 for Cerrado and Pantanal, respectively).
Bootstrapping (Christie, 2004) and correlation analyses were conducted using MS Excel V2208. All other statistical tests were conducted using NCSS (version 12.0.16).
3 Results
3.1 Community variations in species richness and diversity
Principal components analysis (PCA) explained 95.3% of the structural variation in the different communities (Figure 1). PCA axis 1 explained 68.6% of the variance and primarily separated the stands according to basal area, stem diameter, and leaf area index (LAI) on the right-hand side of the biplot and understory cover on the left-hand side of the biplot (Figure 1). All of the Cerrado stands in the Cuiaba basin were on the left-side of the PCA biplot reflecting the higher understory cover and lower stem diameter, basal area, and LAI than similar stands in the Pantanal. PCA axis 2 explained an additional 26.7% of the variance and separated stands based on stem density, with woodlands, seasonal forests, and dense woodlands having higher stem densities than mixed grassland and riparian forests (Figure 1).
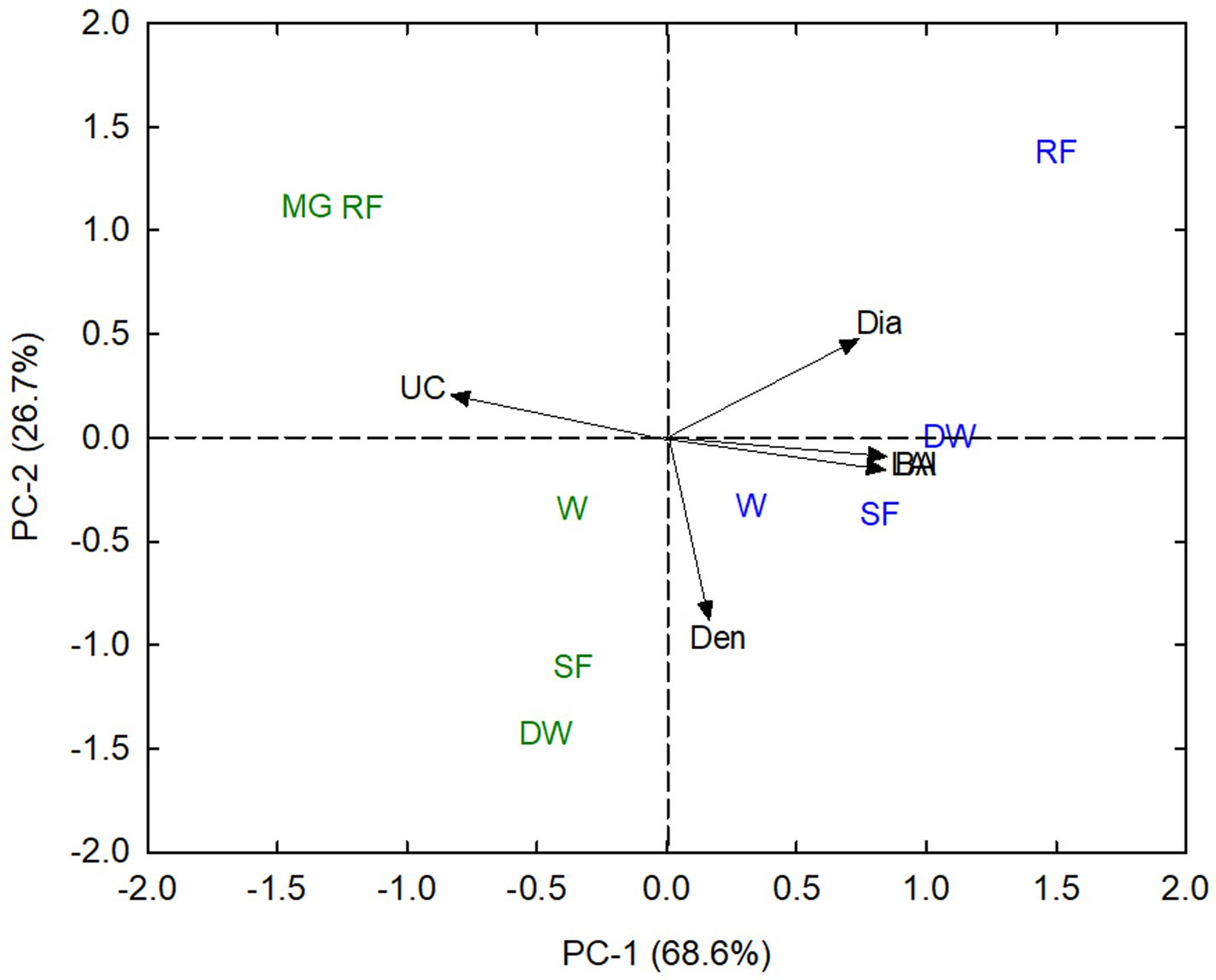
Figure 1. Principal components analysis (PCA) of the structural attributes of Cerrado (green symbols) and Pantanal (blue symbols) ecosystems, including understory cover (UC), stem density (Den), stem diameter (Dia), stem basal area (BA), and leaf area index (LAI), for MG, mixed grassland (Cerrado only); W, typical savanna woodlands; DW, dense savanna woodlands; SF, seasonal forest; RF, riparian forests. The percentages in the parentheses of each axis refer to the percent variation explained by each axis.
There was a total of 78 tree species identified at all of our research sites in the Cerrado and Pantanal (Supplementary Table S1). The log-linear model of the species area-curve performed well in characterizing the increase in species encountered per unit transect length (Figure 2), with the coefficient of the determination (R2) > 0.96 for all regression models. Based on the 95% confidence intervals calculated from linear regression, the y-intercept of the log-linear model [Log (c)] varied significantly between the Cerrado and Pantanal and between each vegetation type except mixed grassland, dense woodland, and riparian forest in the Cerrado (Table 1). Similar patterns were observed for the slope of the species area curve (z), however, only dense woodland and seasonal forest in Cerrado had similar values (Table 1). There were also significant (p < 0.05) differences in the Shannon-Weiner index (H′) between vegetation types, based on bootstrapped 95% confidence intervals (Table 1). Mixed grassland and riparian forests in the Cerrado and Pantanal had the lowest values, while H′ values for the other stands ranged between 2.1 (Pantanal woodland) and 3.0 (Cerrado seasonal forest). Many of the richness and diversity indices were positively correlated and had similar patterns across the grassland-forest gradient. H′ was positively correlated with S, 1/D, z, and ΣV in the Pantanal and when for all sites were analyzed together, but in Cerrado, H′ was only significantly correlated with S and z (Table 2).
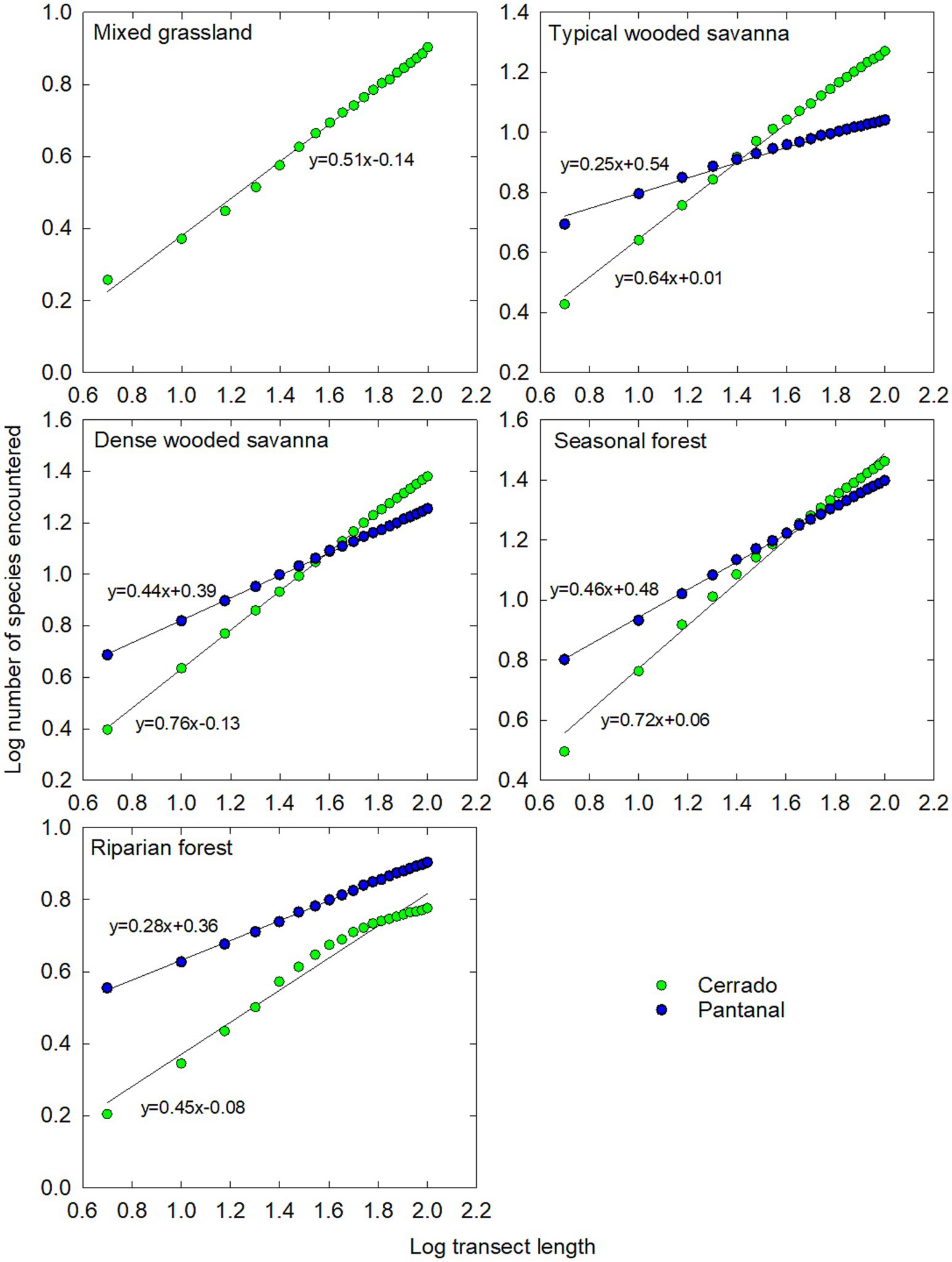
Figure 2. Log-number of species vs. Log-transect length for Cerrado (green symbols) and Pantanal (blue symbols) ecosystems. Regression lines represent straight lines (y = mx + b) where b is the y-intercept [Log (c)] and m is the slope (z). All regression lines have r2 > 0.96.
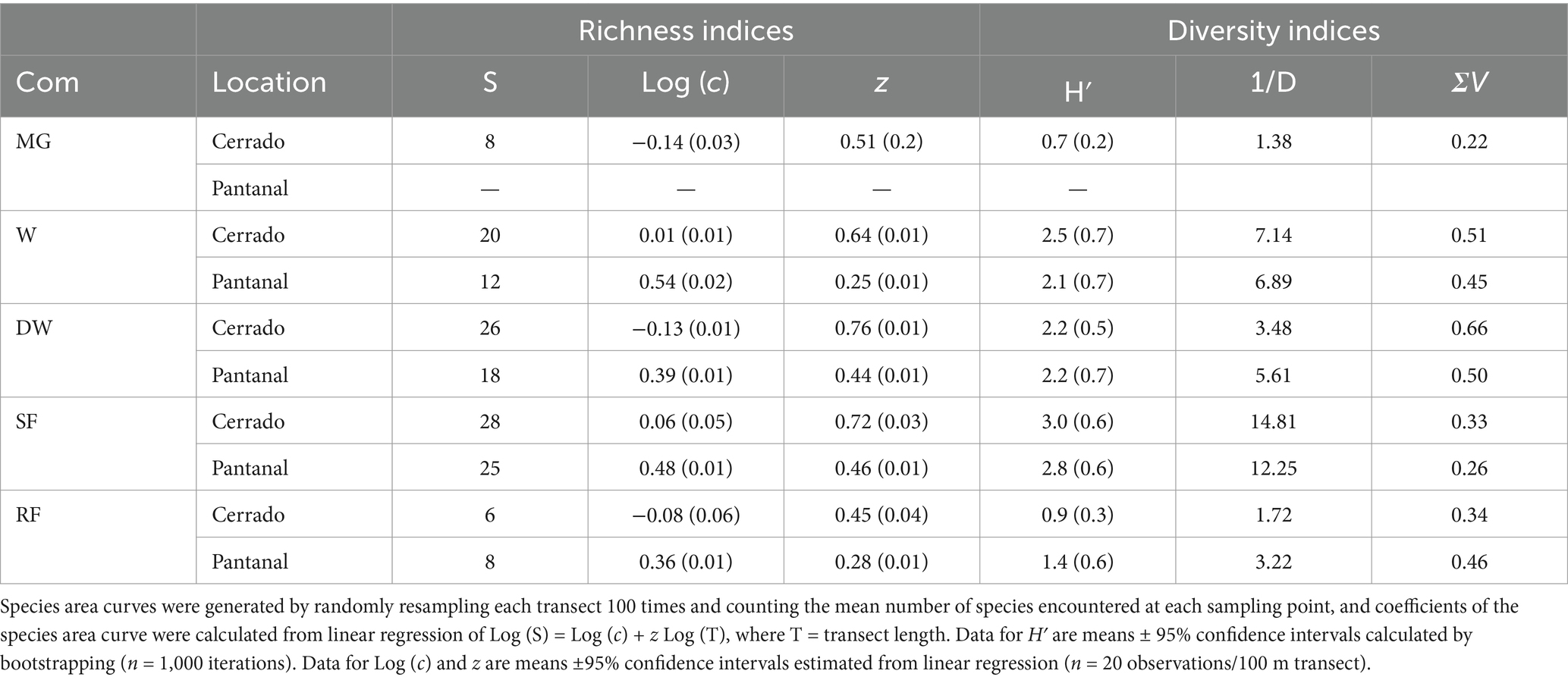
Table 1. Tree species richness, including species richness (S) and the y-intercept [Log (c)] and slope (z) calculated from the species-area curve, alpha diversity indices, including the Shannon-Weiner (H′) and inverse Simpson’s index (1/D), and estimated structural diversity (ΣV) for 100 m long permanent transects located in mixed grasslands (MG), typical (W) and dense (DW) woodlands, and seasonal (SF) and riparian (RF) forests of the Cerrado and Pantanal.
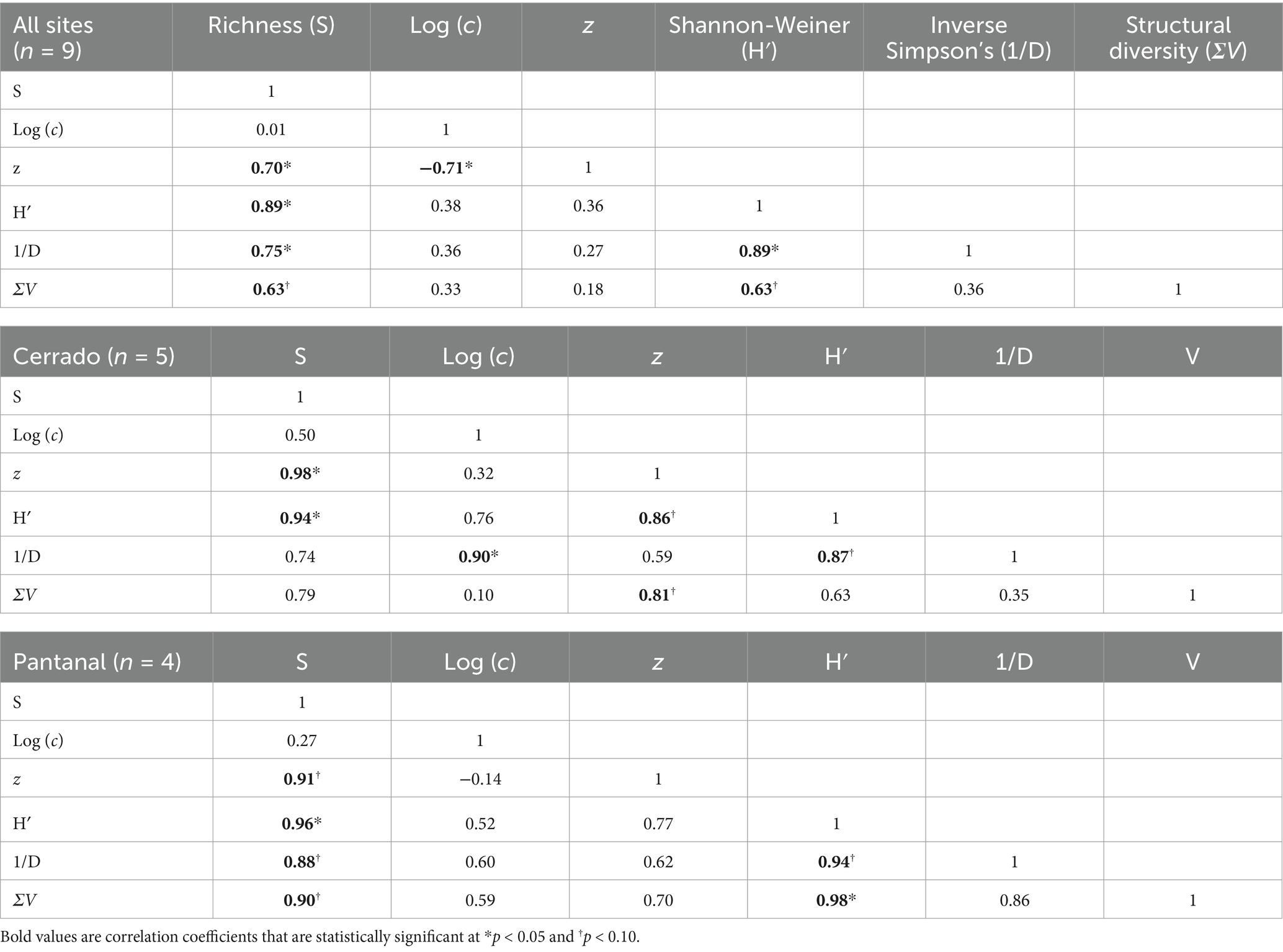
Table 2. Pearson-product correlation matrix for indices of species richness [S, log (c), and z], alpha diversity (H′, and 1/D), and structural diversity (ΣV) for mixed grassland, woodland, dense woodland, seasonal forest, and riparian forest communities of the Cerrado of the Cuiaba Basin and Pantanal.
3.2 Links between community diversity and wood C storage and production
Average aboveground wood C storage of woodlands and forests were nearly 3-times higher in the Pantanal than in the Cerrado of the Cuiaba Basin, resulting in a statistically significant difference in wood C storage between the two sites (F1,7 = 20.4; p < 0.01; Supplementary Figure S1). Temporal variations in the wood C pool size were small and not statistically significant (F7,7 = 0.9; p > 0.05) and there was no site × time (S × T) interaction (F7,7 = 0.6; p > 0.05) in wood C pool size (Supplementary Figure S1). In contrast, aboveground annual wood C increment varied significantly (F1,7 = 34.9; p < 0.001) between sites, and interannual variations were large enough to cause a statistically significant (F7,7 = 7.3; p < 0.01) temporal variation in wood C increment (Figure 3). Differences in mean annual C increments between the different sites were larger during wetter years, with many of the differences were statistically significantly (p < 0.05; Figure 3).
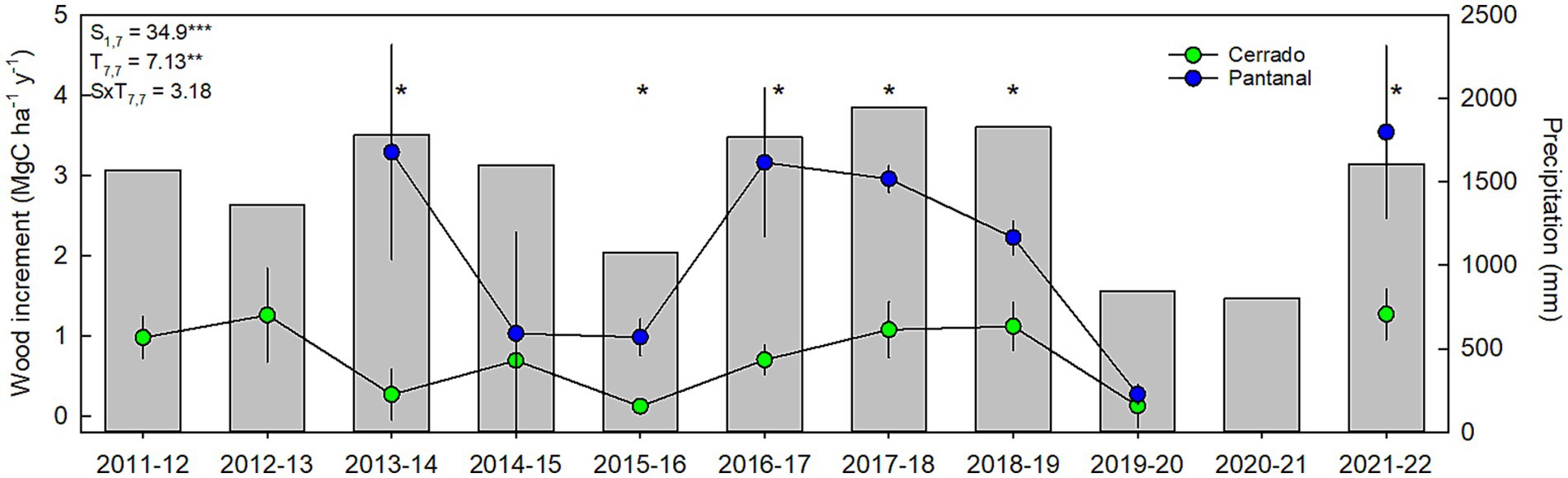
Figure 3. Mean (±se) annual wood increment for Cerrado (green symbols; n = 5) and Pantanal (blue symbols; n = 4) ecosystems (left-hand y-axis) and annual precipitation (grey bars, right-hand y-axis). Repeated measures ANOVA results (F-statistic, degrees of freedom) for site (S), time (T), and the site × time (S × T) interaction are displayed in the upper right-hand corner of the figure (**p < 0.01; ***p < 0.001). Wood increment means with an asterisk (*) above are significantly different (p < 0.05).
Analysis of covariance (ANCOVA) revealed that aboveground wood C storage and production were differentially related to measures of community richness or diversity (Table 3). Aboveground wood C storage was significantly higher in the Pantanal than the Cerrado, but there was no statistically significant relationship between wood C storage and any of the richness or diversity covariates (Table 3). However, wood C storage was significantly positively correlated with S, z, H′, and ΣV in Cerrado, regardless of the annual precipitation regime, and when all sites were included, wood increment was significantly positively correlated with Log (c) regardless of the annual precipitation (Figure 4).
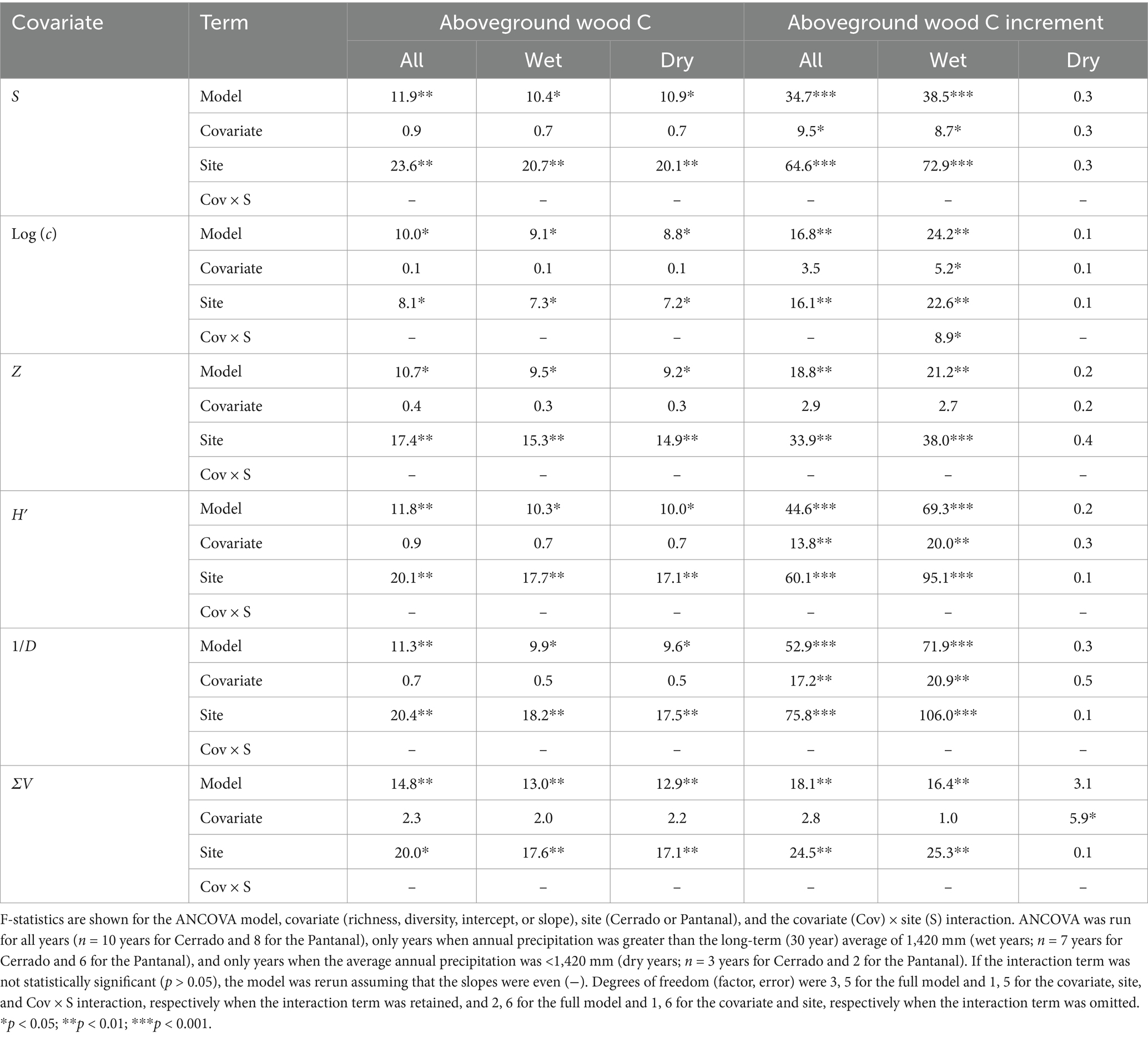
Table 3. Analysis of covariance (ANCOVA) F-statistics for aboveground wood carbon (C) and annual aboveground wood C increment as a function of species richness indices, including species richness (S) and the slope and intercept of the species area curves, and diversity indices, including the Shannon-Wiener (H′), inverse Simpson’s index (1/D), and the index of structural diversity (SV), for woodlands and forests in the Cerrado (n = 5 sites) and Pantanal (n = 4 sites).
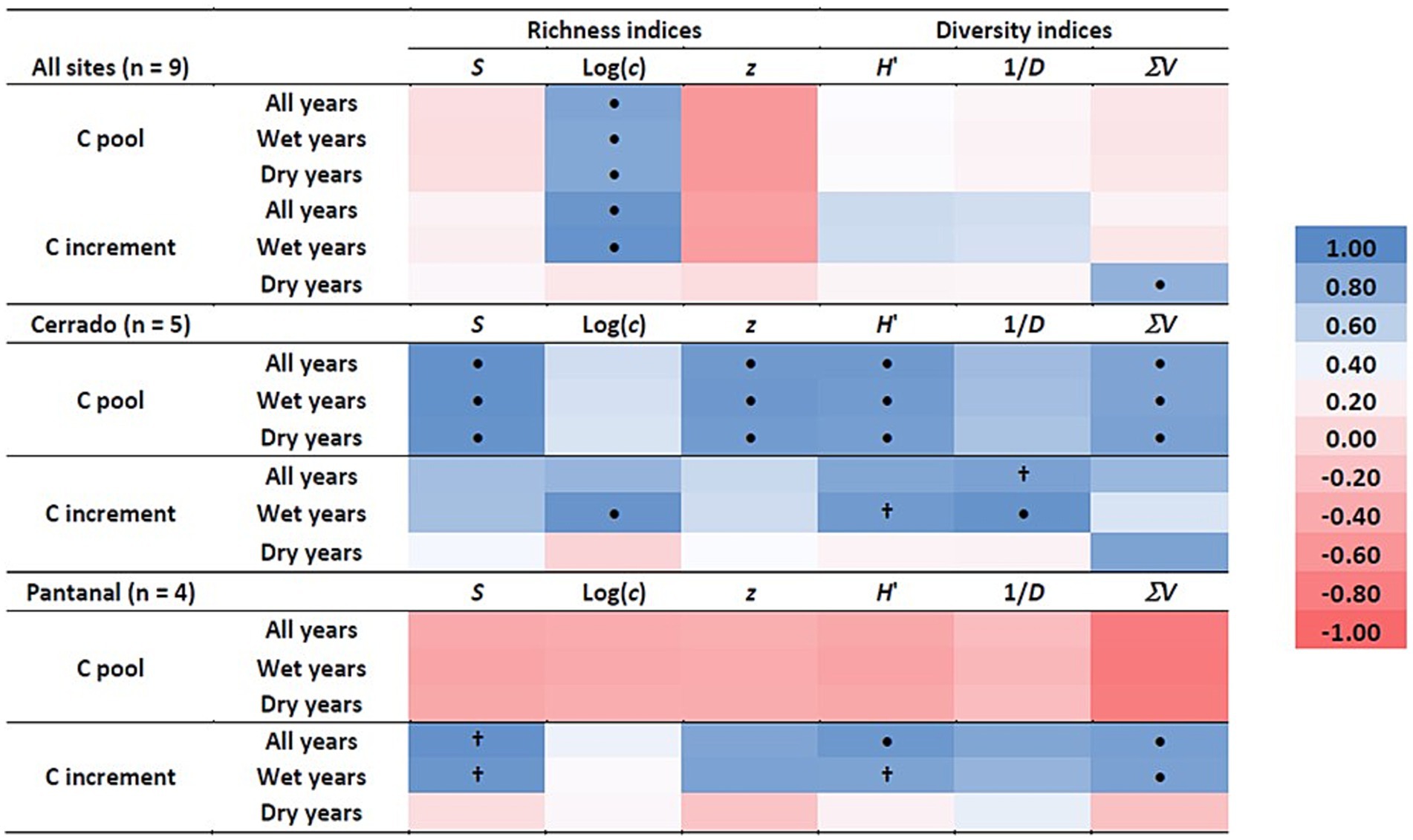
Figure 4. Pearson correlation coefficient heat map for mean aboveground wood C pool size and the mean annual wood C increment as a function of species richness indices (species richness (S) and the intercept [Log (c)] and slope (z) of the log-transformed species area curve) and diversity indices [Shannon-Weiner H′, inverse Simpson’s index (1/D), and the index of structural diversity (V)] for all sites together (top panel), Cerrado sites only (middle panel), and Pantanal sites only (bottom panel) for all years, years with annual precipitation >1,420 mm (Wet years), and years with precipitation <1,420 mm (Dry years). The precipitation threshold of 1,420 mm is based in the long-term (30 year) mean precipitation for the region. •p < 0.05; †p < 0.1.
ANCOVA revealed that annual wood C increment was significantly affected by a variety of richness and diversity indices in the wet season and when all years were analyzed together, but except for ΣV, none of the covariates were statistically significant during the dry season (Table 3). In Cerrado, wood C increment was correlated with Log (c), 1/D, and H′ during wet years and 1/D when all years were combined (Table 2). In the Pantanal, wood increment was significantly positively correlated S, H′, and ΣV during wet years and with all years combined, and when all sites were together wood increment was positively correlated with Log (c) during wet years and with all years combined (Figure 4).
4 Discussion
4.1 Community variations in species richness and diversity
We hypothesized that there would be an increase in species richness, alpha diversity, and structural diversity from mixed grasslands to Cerrado forests in both upland (Cuiaba Basin) and hyperseasonal (Pantanal) Cerrado based on previous research that reported similar trends (Goodland and Pollard, 1973; Vourlitis et al., 2013). However, our data did not support our hypothesis. We found that mixed grasslands and riparian forests had lower species richness and diversity than typical woodlands, dense woodlands, and seasonal forests. Furthermore, our index of structural diversity (ΣV) did not exhibit a systematic increase from the mixed grassland to forests as expected (Storch et al., 2018), suggesting that structural diversity encompasses more than differences in tree height and density that occur across the grassland to forest structural gradient.
Riparian forests had lower values of species richness and diversity than the other forests and woodlands due to the dominance of Vochysia divergens Pohl, locally known as cambara. These types of riparian forests are locally recognized as cambarazais due to the monodominance of V. divergens and are very common in the Cerrado-Pantanal transition of southern Mato Grosso (Nunes da Cunha and Junk, 2001). In contrast, woodlands, dense woodlands, and seasonal forests had similar richness and diversity values because these vegetation types share many of the same tree species (Supplementary Table S1) and have similar soil physical and chemical properties (Vourlitis et al., 2013, 2015, 2019). These results are similar to those reported for other transition zones. In the Cerrado-Amazon Forest transition in northern Mato Grosso (Santos et al., 2015) and across several states in Brazil (Morandi et al., 2020), Cerrado forests (Cerradão) and typical (stricto sensu) and dense (denso) woodlands were found to be floristically similar and have similar alpha-diversity. This may be especially true in woodlands and forests where fire has been suppressed because forest taxa are recruiting in these stands (Pellegrini et al., 2016).
There were also regional and community differences in the coefficients of the log species-area curve, implying that the impact of spatial variation on species assemblage varied both within and between the Cerrado and Pantanal (Desilets and Houle, 2005). The slope of the regression (z) is reportedly positively related to spatial heterogeneity causing S to increase in more heterogenous environments (Lomolino, 1989; Sagar et al., 2003; Desilets and Houle, 2005). This suggests that the change in species richness was higher in Cerrado of the Cuiaba Basin than in the Pantanal, which is plausible because Cerrado fragments measured in the Cuiaba basin are smaller and more heterogeneous than those studied in the Pantanal, and variations in substrate are larger in the Cuiaba Basin (Vourlitis et al., 2013; Couto and Oliveira, 2011; Gonçalves dos Santos et al., 2018). However, the y-intercept [Log (c)] also varied significantly both within and between vegetation types so it is difficult to interpret the impact of spatial variability on z (Lomolino, 1989).
In general, many of the richness and diversity indices were positively correlated even though they have different computational sensitivities and biases (Magurran, 1988). While this result is perhaps not surprising, it is interesting to speculate why certain indices were more highly correlated with the structural index (ΣV) than others. For example, S and H′ were highly correlated with ΣV in the Pantanal presumably because all of the sites were woodlands and forests which had similar floristic and structural properties, and they all lacked a significant understory component (Vallejo, 2020). Thus, ΣV was presumably related more to the structural elements (i.e., stem density, basal area) that tend to cause an increase in species diversity as they increase from woodlands to forests (Gilroy et al., 2014; Pellegrini et al., 2016). In the Cuiaba basin, only z was correlated with ΣV, suggesting that higher spatial patchiness in S was important for increasing ΣV.
4.2 Links between community diversity and wood C storage and production
We predicted there would be a positive relationship between woody C storage and species richness and/or diversity in both upland and hyperseasonal Cerrado based on observations that C storage was related to community structure (Grace et al., 2006; Ribeiro et al., 2011; Miranda et al., 2014; Vourlitis et al., 2019; Godlee et al., 2021). We found that aboveground wood C storage was positively correlated with several indices of species richness or diversity in Cerrado but not in the Pantanal, while annual wood C increment was positively correlated with richness or diversity in both regions. There are likely two reasons why wood C storage was positively related to richness and diversity in Cerrado. First, differences in stand species richness (S and z), diversity (H′ and ΣV), and wood C pool size were much larger in the Cuiaba Basin, which presumably increased the potential for observing positive relationships between richness, diversity, and wood C storage, especially with the low sample sizes that were inherent to our study. This was also probably the reason that wood C storage was positively related to Log (c) when all sites were included. Similar results have been observed in the Cerrado-tropical forest transition in the Amazon Basin where spatial differences in diversity were too small to explain variations in aboveground biomass (Morandi et al., 2020). Second, the species-poor riparian forest in the Pantanal had more than 1.3 times higher wood C storage than the next highest community in the Pantanal, and forests dominated by V. divergens, which is a species that can reach a diameter in excess of 35 cm and a height of 17 m, have the capacity to store substantial amounts of C in wood (Schöngart et al., 2011; Vourlitis et al., 2019). While the riparian forest in the Cuiaba Basin was also dominated by V. divergens, individuals were smaller than those in the Pantanal, which might have been due to the shallower, nutrient poor soils associated with the Cuiaba Basin (Vourlitis et al., 2023). These size differences caused aboveground C storage to be significantly lower in the riparian forest in the Cuiaba basin (Vourlitis et al., 2019).
We also hypothesized that if the diversity-productivity relationship is affected by water availability (Hofhansl et al., 2020; Godlee et al., 2021; Mensah et al., 2023), and our data on stem C increment generally supported this hypothesis as the effect of the richness and diversity covariates on wood C increment was statistically significant during years when average annual precipitation was greater than the long-term average (Table 2). However, we did not see any covariate × site interaction, indicating that the positive diversity-productivity relationship was similar for the Cerrado and Pantanal.
Water availability has been found to be a key control on leaf gas exchange in seasonal systems such as Cerrado, and leaf photosynthesis of many of the tree species in our stands is significantly higher during the wet season or during wetter years (Dalmagro et al., 2014; Dalmolin et al., 2018). This pattern even holds for trees in hyperseasonal stands of the Pantanal, which are flooded for up to 6 months of the year, and higher soil moisture during the wet-dry season transition allows leaf gas exchange to be elevated well into the dry season (Dalmagro et al., 2014, 2016). Higher rates of leaf gas exchange during wetter years also increases tree growth (Dalmolin et al., 2015; Sallo et al., 2017; Vourlitis et al., 2022) and reduces mortality (Reis et al., 2022), which increases stand cover, wood C increment (Sankaran et al., 2005; Vourlitis et al., 2019; Godlee et al., 2021; Mensah et al., 2023), and ecosystem C storage (Arruda et al., 2016; Dalmagro et al., 2019). Increases in water availability also increase nutrient availability in these seasonal communities (Bustamante et al., 2006; Vourlitis et al., 2017), which will increase wood C increment (Viani et al., 2011; Morandi et al., 2020; Vourlitis et al., 2019; Godlee et al., 2021; Mensah et al., 2023).
The impact of precipitation on the strength of the diversity-productivity relationship has implications on how climate change may alter future productivity and/or diversity in this region. Climate models predict an intensification of the dry season resulting a longer drought period (Boisier et al., 2015), and climate observations indicate that warming and drying is already occurring in the Cerrado-Pantanal transition (Lazaro et al., 2020; Hofmann et al., 2021; Marengo et al., 2021). Because not all Cerrado tree species have similar sensitivities to warming and drying (Esquivel-Muelbert et al., 2019; Vourlitis et al., 2022), an intensification in the dry season will undoubtedly cause changes in community composition through differential survival/mortality, and as a result, community richness and diversity. Our data suggest changes in diversity will affect rates of wood C increment, but if warming and drying continue, the impact of prolonged drought will likely become more important than diversity in affecting wood C increment in our Cerrado stands.
4.3 Sources of uncertainty
There are several sources of uncertainty that must be considered for interpreting the data presented here. First, while the point-centered quarter method has been used for decades for measuring density and/or basal area in forests (Cottam and Curtis, 1956; Goldsmith and Harrison, 1976; Notarangelo et al., 2023), some authors have cautioned the use of this method when the underlying distribution of trees in the stand are non-random (Goldsmith and Harrison, 1976; Hijbeek et al., 2013). Unfortunately, we do not know the distribution of trees in our stands; however, there is little consensus as to whether the point-center quarter method provides biased estimates of tree density or basal area when the distribution of trees is non-random. For example, Goldsmith and Harrison (1976) warn of the potential for bias in non-random stands but provide no evidence to support this claim, while Hijbeek et al. (2013) demonstrate errors on the order of −31 to −37% using the point-centered quarter method for species that are clumped. However, in other studies Cottam and Curtis (1956) found that the point-quarter method did not suffer from bias in stands that had varying tree dispersion patterns, while Notarangelo et al. (2023) found that the point quarter method accurately estimated density in Italian forests where tree distributions varied from highly clumped to highly uniform. Thus, while all estimated of density and basal are are likely to have some uncertainty, it is unknown whether the use of the point-centered quarter method resulted in biased estimates for our woodlands and forests.
We found that several richness and diversity indices were positively correlated with productivity, but results from the species area-curve indicate that there were likely additional tree species that were not encountered along the 100 m transect. Thus, our estimates of S, H′, and 1/D are probably only appropriate for the area directly surrounding our permanent transects. Furthermore, it is likely that our stands would have higher richness and diversity if the understory component was included (Pellegrini et al., 2016), especially in the mixed-grassland and riparian forest in the Cuiaba basin, which had high understory plant cover.
Finally, our sample size was very low (n = 5 stands in the Cerrado of the Cuiaba Basin and 4 stands in the Pantanal), which caused our statistical power to be very low and caused us to adopt a higher critical p-value (0.10) for some of our analysis. It is also unclear whether our results are applicable to other Cerrado areas. Clearly, given that fire has been suppressed at our study sites for several decades, our results are probably not applicable to Cerrado that is exposed to fire.
5 Conclusion
We used several indices of species richness and diversity and utilized an index of structural diversity to characterize the composition of Cerrado stands in the Cerrado-Pantanal transition of southern Mato Grosso Brazil. We hypothesized that species richness, alpha diversity, and structural diversity would increase from mixed grasslands to Cerrado forests; however, our data did not support our hypothesis as mixed grasslands and riparian forests had similar values of species richness and diversity. Furthermore, although the species richness and diversity indices used here are affected differently by species dominance and/or evenness, many were highly correlated indicating that they shared similar information on stand species composition and/or structure. We also hypothesized that aboveground wood C stocks and wood increment would be positively correlated with richness and diversity, especially during wetter years. In terms of wood C pool size, many of the indices were positively correlated with wood C storage in the Cerrado, where stand differences in wood C storage and stand richness and diversity were larger. However, for wood increment we observed several positive diversity-productivity relationships during years when the annual precipitation was at or above the long-term average for the region. This suggests that positive diversity-productivity relationships for these seasonal biomes only hold for periods when adequate water is available for growth, a result that has been observed for other tropical savannas (Hofhansl et al., 2020; Godlee et al., 2021; Mensah et al., 2023). More research needs to be done across this important transition zone, similar to what has been done across the Amazon Forest-Cerrado transition, to see if the patterns that we observed here are applicable to the region as a whole.
Data availability statement
The datasets presented in this study can be found in online repositories. The names of the repository/repositories and accession number(s) can be found at: https://data.mendeley.com, https://10.17632/kcwwktkd85.1, and https://data.mendeley.com.
Author contributions
GV: Conceptualization, Data curation, Formal analysis, Funding acquisition, Investigation, Methodology, Project administration, Resources, Visualization, Writing – original draft, Writing – review & editing. OP: Data curation, Formal analysis, Funding acquisition, Investigation, Methodology, Project administration, Resources, Writing – review & editing. HD: Data curation, Formal analysis, Investigation, Methodology, Project administration, Writing – review & editing. PA: Data curation, Formal analysis, Investigation, Methodology, Project administration, Resources, Writing – review & editing. FA: Investigation, Writing – review & editing.
Funding
The author(s) declare that financial support was received for the research, authorship, and/or publication of this article. This study was supported by the Coordination of Improvement of Higher Education Personnel (CAPES) Science without Borders Program to GLV (n. 401908/2012-7), a productivity scholarship funded by the National Council for Scientific and Technological Development and the Ministry of Science and Technology to GLV (CNPq n. 304575/2017-8), and National Science Foundation–Office of International Sciences and Engineering grant to GV (NSF-OISE 0968245). All funding sources were used to support GV travel between Brazil and the U.S., travel to field sites, sample laboratory analyses, and purchase of field supplies.
Acknowledgments
The authors thank the Federal University of Mato Grosso (UFMT - PGFA) and University of Cuiabá (UNIC) for laboratory and office facilities and vehicle access, SESC-Pantanal and Dr. Clovis Miranda and his family for access to the field sites and logistical support, and the Xomanoflux group for the field support.
Conflict of interest
The authors declare that the research was conducted in the absence of any commercial or financial relationships that could be construed as a potential conflict of interest.
Publisher’s note
All claims expressed in this article are solely those of the authors and do not necessarily represent those of their affiliated organizations, or those of the publisher, the editors and the reviewers. Any product that may be evaluated in this article, or claim that may be made by its manufacturer, is not guaranteed or endorsed by the publisher.
Supplementary material
The Supplementary material for this article can be found online at: https://www.frontiersin.org/articles/10.3389/ffgc.2024.1380600/full#supplementary-material
References
Alencar, A., Shimbo, J. Z., Lenti, F., Marques, C. B., Zimbres, B., Rosa, M., et al. (2020). Mapping three decades of changes in the Brazilian savanna native vegetation using Landsat data processed in the google earth engine platform. Remote Sens. 12:924. doi: 10.3390/rs12060924
Arruda, P. H. Z., Vourlitis, G. L., Pinto, O. B. Jr., Lobo, F. A., Santanna, F. N., and Nogueira, J. S. (2016). Large net CO2 loss from a grass-dominated tropical savanna in south-Central Brazil in response to seasonal and interannual drought. J. Geophys. Res. Biogeosci. 121, 2110–2124. doi: 10.1002/2016JG003404
Barbour, M. G., Burk, J. H., and Pitts, W. D. (1987). Terrestrial plant ecology. Menlo Park, CA: Benjamin Cummings Publishing Company.
Batalha, M. A., Mantovani, W., and de Mesquita Júnior, H. N. (2001). Vegetation structure in cerrado physiognomies in South-Eastern Brazil. Braz. J. Biol. 61, 475–483. doi: 10.1590/S1519-69842001000300018
Boisier, J. P., Ciais, P., Ducharne, A., and Guimberteau, M. (2015). Projected strengthening of Amazonian dry season by constrained climate model simulations. Nat. Clim. Chang. 5, 656–660. doi: 10.1038/nclimate2658
Bustamante, M. M. C., Medina, E., Asner, G. P., Nardoto, G. B., and Garcia-Montiel, D. C. (2006). Nitrogen cycling in tropical and temperate savannas. Biogeochemistry 79, 209–237. doi: 10.1007/s10533-006-9006-x
Chave, J., Rejou-Mechain, M., Burquez, A., Chidumayo, E., Colgan, M. S., Deltiti, W. B. C., et al. (2014). Improved allometric models to estimate the aboveground biomass of tropical trees. Glob. Chang. Biol. 20, 3177–3190. doi: 10.1111/gcb.12629
Christie, D. (2004). Resampling with excel. Teach. Stat. 26, 9–14. doi: 10.1111/j.1467-9639.2004.00136.x
Colli, G. R., Vieira, C. R., and Dianese, J. C. (2020). Biodiversity and conservation of the Cerrado: recent advances and old challenges. Biodivers. Conserv. 29, 1465–1475. doi: 10.1007/s10531-020-01967-x
Cottam, G., and Curtis, J. T. (1956). The use of distance measures in phytosociological sampling. Ecology 37, 451–460. doi: 10.2307/1930167
Couto, E. G., and Oliveira, V. A. (2011). The soil diversity of the Pantanal. In W. J. Junk, C. J. Silvada, C. Nunes da Cunha, and K. M. Wantzen (Eds.), The Pantanal: Ecology, biodiversity, and sustainable management of a large neotropical seasonal wetland, (pp. 71–102). Sofia-Moscow: Pensoft Publishers.
Dalmagro, H. J., de Arruda, P. H. Z., Vourlitis, G. L., Lathuillière, M. J., Nogueira, J. S., Couto, E. G., et al. (2019). Radiative forcing of methane fluxes offsets net carbon dioxide uptake for a tropical flooded forest. Glob. Chang. Biol. 25, 1967–1981. doi: 10.1111/gcb.14615
Dalmagro, H. J., Lathuillière, M. J., Vourlitis, G. L., Campos, R. C., Pinto, O. B. Jr., Johnson, M. S., et al. (2016). Physiological responses to extreme hydrological events in the Pantanal wetland: heterogeneity of a plant community containing super-dominant species. J. Veg. Sci. 27, 568–577. doi: 10.1111/jvs.12379
Dalmagro, H. J., Lobo, F. A., Vourlitis, G. L., Dalmolin, Â. C., Antunes, M. Z. Jr., Ortíz, C. E. R., et al. (2014). The physiological light response of two tree species across a hydrologic gradient in Brazilian savanna (Cerrado). Photosynthetica 52, 22–35. doi: 10.1007/s11099-014-0001-5
Dalmolin, A. C., Lobo, F. A., Vourlitis, G. L., Dalmagro, H. J., Antunes Junior, M. Z., and Rodriguez-Ortiz, C. E. (2018). Physiological adjustments of an invasive tree species to extreme hydrological events in a tropical seasonal wetland. Trees 32, 1365–1375. doi: 10.1007/s00468-018-1718-8
Dalmolin, Â. C., Lobo, F. A., Vourlitis, G., Silva, P. R., Dalmagro, H. J., Antunes, M. Z. Jr., et al. (2015). Is the dry season an important driver of phenology and growth for two Brazilian savanna tree species with contrasting leaf habits? Plant Ecol. 216, 407–417. doi: 10.1007/s11258-014-0445-5
Desilets, P., and Houle, G. (2005). Effects of resource availability and heterogeneity on the slope of the species-area curve along a floodplain-upland gradient. J. Veg. Sci. 16, 487–496. doi: 10.1111/j.1654-1103.2005.tb02389.x
Esquivel-Muelbert, A., Baker, T. R., Dexter, K. G., Lewis, S. L., Brienen, R. J., Feldpausch, T. R., et al. (2019). Compositional response of Amazon forests to climate change. Glob. Chang. Biol. 25, 39–56. doi: 10.1111/gcb.14413
Felfili, J. M., da Silva Júnior, M. C., Sevilha, A. C., Fagg, C. W., Walter, B. M. T., Nogueira, P. E., et al. (2004). Diversity, floristic and structural patterns of cerrado vegetation in Central Brazil. Plant Ecol. 175, 37–46. doi: 10.1023/B:VEGE.0000048090.07022.02
Fernandes, G. W., Barbosa, N. P. U., Alberton, B., Barbieri, A., Dirzo, R., Goulart, F., et al. (2018). The deadly route to collapse and the uncertain fate of Brazilian rupestrian grasslands. Biodivers. Conserv. 27, 2587–2603. doi: 10.1007/s10531-018-1556-4
Furley, P. A., and Ratter, J. A. (1988). Soil resources and plant communities of the central Brazilian cerrado and their development. J. Biogeogr. 15, 97–108. doi: 10.2307/2845050
Gilroy, J. J., Woodcock, P., Edwards, F. A., Wheeler, C., Baptiste, B. L., Medina Uribe, C. A., et al. (2014). Cheap carbon and biodiversity co-benefits from forest regeneration in a hotspot of endemism. Nat. Clim. Chang. 4, 503–507. doi: 10.1038/nclimate2200
Godlee, J. L., Ryan, C. M., Bauman, D., Bowers, S. J., and Carreiras, J. M. B. (2021). Structural diversity and tree density drives variation in the biodiversity–ecosystem function relationship of woodlands and savannas. New Phytol. 232, 579–594. doi: 10.1111/nph.17639
Goldsmith, F. B., and Harrison, C. M. (1976). “Description and analysis of vegetation” in Methods in plant ecology. ed. S. B. Chapman (New York, NY: Halsted press), 85–156.
Gonçalves dos Santos, H., Jacomine, P. T., Dos Anjos, L. H. C., De Oliveira, V. Á., Lumbreras, J. F., and Coelho, M. R. (2018). Brazilian soil classification system. Brasília: Brazilian Agricultural Research Corporation (Embrapa soils).
Goodland, R. J., and Pollard, R. (1973). The Brazilian cerrado vegetation: a fertility gradient. Ecology 61, 219–224. doi: 10.2307/2258929
Gould, S. J. (1979). An allometric interpretation of species-area curves: the meaning of the coefficients. Am. Nat. 114, 335–343. doi: 10.1086/283482
Grace, J., Jose, J. S., Meir, P., Miranda, H. S., and Montes, R. A. (2006). Productivity and carbon fluxes of tropical savannas. J. Biogeogr. 33, 387–400. doi: 10.1111/j.1365-2699.2005.01448.x
Hijbeek, R., Koedam, N., Khan, M. N. I., Kairo, J. G., Schoukens, J., and Dahdouh-Guebas, F. (2013). An evaluation of plotless sampling using vegetation simulations and field data from a mangrove forest. PloS One 8:e67201. doi: 10.1371/journal.pone.0067201
Hofhansl, F., Chacon-Madrigal, E., Fuchslueger, L., Jenking, D., and Morea-Beita, A. (2020). Climatic and edaphic controls over tropical forest diversity and vegetation carbon storage. Sci. Rep. 10, 1–12. doi: 10.1038/s41598-020-61868-5
Hofmann, G. S., Cardoso, M. F., Alves, R. J., Weber, E. J., Barbosa, A. A., deToledo, P. M., et al. (2021). The Brazilian Cerrado is becoming hotter and drier. Glob. Change Biol. 27, 4060–4073. doi: 10.1111/gcb.15712
Jasinski, E., Morton, D., DeFries, R., Shimabukuro, Y., Anderson, L., and Hansen, M. (2005). Physical landscape correlates of the expansion of mechanized agriculture in Mato Grosso, Brazil. Earth Interact. 9, 1–18. doi: 10.1175/EI143.1
Lazaro, W., Oliveira-Júnior, E. S., Silva, C. J. D., Castrillon, S. K. I., and Muniz, C. C. (2020). Climate change reflected in one of the largest wetlands in the world: an overview of the northern Pantanal water regime. Acta Limnol. Bras. 32:104. doi: 10.1590/s2179-975x7619
Lomolino, M. V. (1989). Interpretations and comparisons of constants in the species-area relationship: an additional caution. Am. Nat. 133, 277–280. doi: 10.1086/284917
Lopes, A. S., and Cox, F. R. (1977). Cerrado vegetation in Brazil: An edaphic gradient. Agron. J. 69, 828–831. doi: 10.2134/agronj1977.00021962006900050025x
Magurran, A. E. (1988). Diversity indices and species abundance models. Ecol. Div. Measur. 15, 7–45. doi: 10.1007/978-94-015-7358-0_2
Marengo, J. A., Cunha, A. P., Cuartas, L. A., Deusdara Leal, K. R., Broedel, E., Seluchi, M. E., et al. (2021). Extreme drought in the Brazilian Pantanal in 20 19–20 20: characterization, causes, and impacts. Front. Water 3:639204. doi: 10.3389/frwa.2021.639204
Marthews, T. R., Metcalfe, D., Malhi, Y., Phillips, O., Huaraca Huasco, W., Riutta, T., et al. (2012). Measuring tropical Forest carbon allocation and cycling: a RAINFOR-GEM field manual for intensive census plots (v2.2). Manual, Global Ecosystems Monitoring network. Available at: http://gem.tropicalforests.ox.ac.uk/.
Mensah, S., Noulèkoun, F., Dimobe, K., Seifert, T., and Glèlè Kakaï, R. (2023). Climate and soil effects on tree species diversity and aboveground carbon patterns in semi-arid tree savannas. Sci. Rep. 13:11509. doi: 10.1038/s41598-023-38225-3
Miranda, S. C., Bustamante, M., Palace, M., Hagen, S., Keller, M., and Ferreira, L. G. (2014). Regional variations in biomass distribution in Brazilian savanna woodland. Biotropica 46, 125–138. doi: 10.1111/btp.12095
Morandi, P. S., Marimon, B. S., Marimon-Junior, B. H., Ratter, J. A., Feldpausch, T. R., Colli, G. R., et al. (2020). Tree diversity and above-ground biomass in the South America Cerrado biome and their conservation implications. Biodivers. Conserv. 29, 1519–1536. doi: 10.1007/s10531-018-1589-8
Notarangelo, M., Carrer, M., Lingua, E., Puletti, N., and Torresan, C. (2023). Performance assessment of two plotless sampling methods for density estimation applied to some alpine forests of northeastern Italy. iForest-Biogeosci. Forestry 16, 385–391. doi: 10.3832/ifor4335-016
Nunes da Cunha, C., and Junk, W. J. (2001). Distribution of woody plant communities along the flood gradient in the Pantanal of Pocone, Mato Grosso, Brazil. Int. J. Ecol. Environ. Sci. 27, 63–70.
Paquette, A., and Messier, C. (2011). The effect of biodiversity on tree productivity: from temperate to boreal forests. Glob. Ecol. Biogeogr. 20, 170–180. doi: 10.1111/j.1466-8238.2010.00592.x
Pellegrini, A. F., Socolar, J. B., Elsen, P. R., and Giam, X. (2016). Trade-offs between savanna woody plant diversity and carbon storage in the Brazilian Cerrado. Glob. Chang. Biol. 22, 3373–3382. doi: 10.1111/gcb.13259
Pinto, O. B., Marques, A. C. A., and Vourlitis, G. L. (2020). Aboveground carbon storage and cycling of flooded and upland forests of the Brazilian Pantanal. Forests 11:665. doi: 10.3390/f11060665
Pinto, O. B., Vourlitis, G. L., Carneiro, E. M. S., Dias, M. F., Hentz, C., and Nogueira, J. S. (2018). Interactions between vegetation, hydrology, and litter inputs on decomposition and soil CO2 efflux of tropical forests in the Brazilian Pantanal. Forests 9:281. doi: 10.3390/f9050281
Poorter, L., Thompson, J., Arets, E. J. M. M., Alarcon, A., Alvarez-Sanchez, J., Ascarrunz, N., et al. (2015). Diversity enhances carbon storage in tropical forests. Glob. Ecol. Biogeogr. 24, 1314–1328. doi: 10.1111/geb.12364
Radambrasil (1982, 1982). Levantamentos dos Recursos Naturais Ministério das Minas de Energia. Rio de Janeiro: Secretaria Geral. Projeto RADAMBRASIL.
Reis, S. M., Marimon, B. S., Esquivel‐Muelbert, A., Jr Marimon, B. H., Morandi, P. S., Elias, F., et al. (2022). Climate and crown damage drive tree mortality in southern Amazonian edge forests. J. Ecol. 110, 876–888. doi: 10.1111/1365-2745.13849
Ribeiro, J. F., and Walter, B. M. T. (2008). “As principais fitofisionomias do bioma Cerrado” in Cerrado Ecologia e Flora, Vol. 1. Emprapa Informacao Technologica, Ministerio da Agricultura, Pecuaria e Abastecimento, Brasilia. eds. S. P. de Almeida and J. F. Ribeiro (Brasília: Districto Federal), 153–212.
Ribeiro, S. C., Fehrmann, L., Soares, C. P. B., Jacovine, L. A. G., Kleinn, C., and Gaspar, R. O. (2011). Above- and belowground biomass in a Brazilian Cerrado. For. Ecol. Manag. 262, 491–499. doi: 10.1016/j.foreco.2011.04.017
Ruggiero, P. G. C., Batalha, M. A., Pivello, V. R., and Meirelles, S. T. (2002). Vegetation-soil relationships in cerrado (Brazilian savanna) and semideciduous forest, southeastern Brazil. Plant Ecol. 160, 1–16. doi: 10.1023/A:1015819219386
Sagar, R., Raghubanshi, A. S., and Singh, J. S. (2003). Asymptotic models of species–area curve for measuring diversity of dry tropical forest tree species. Curr. Sci. 84, 1555–1560.
Sallo, F. S., Sanches, L., Dias, V. R. M., Palácios, R. S., and Nogueira, J. S. (2017). Stem water storage dynamics of Vochysia divergens in a seasonally flooded environment. Agric. For. Meteorol. 232, 566–575. doi: 10.1016/j.agrformet.2016.10.015
Sankaran, M., Hanan, N. P., Scholes, R. J., Ratnam, J., Auguistine, D. J., et al. (2005). Determinants of woody cover in African savannas. Nature 438, 846–849. doi: 10.1038/nature04070
Santos, L. M., Lenza, E., dos Santos, J. O., Marimon, B. S., Eisenlohr, P. V., Marimon Junior, B. H., et al. (2015). Diversity, floristic composition, and structure of the woody vegetation of the Cerrado in the Cerrado–Amazon transition zone in Mato Grosso, Brazil. Rev. Bras. Bot. 38, 877–887. doi: 10.1007/s40415-015-0186-2
Schöngart, J., Arieira, J., Felfili Fortes, C., de Arruda, E. C., and Nunes da Cunha, C. N. (2011). Age-related and stand-wise estimates of carbon stocks and sequestration in the aboveground coarse wood biomass of wetland forests in the northern Pantanal, Brazil. Biogeoscinces 8, 3407–3421. doi: 10.5194/bg-8-3407-2011
Silva-Souza, K. J. P., Pivato, M. G., Silva, V. C., Haidar, R. F., and Souza, A. F. (2023). New patterns of the tree beta diversity and its determinants in the largest savanna and wetland biomes of South America. Plant Div 45, 369–384. doi: 10.1016/j.pld.2022.09.006
Storch, F., Dormann, C. F., and Bauhus, J. (2018). Quantifying forest structural diversity based on large-scale inventory data: a new approach to support biodiversity monitoring. Forest Ecosyst 5, 1–14. doi: 10.1186/s40663-018-0151-1
Terra, M. C., Nunes, M. H., Souza, C. R., Ferreira, G. W., Prado-Junior, J. A., Rezende, V. L., et al. (2023). The inverted forest: aboveground and notably large belowground carbon stocks and their drivers in Brazilian savannas. Sci. Total Environ. 867:161320. doi: 10.1016/j.scitotenv.2022.161320
Tilman, D., Isbell, F., and Cowles, J. M. (2014). Biodiversity and ecosystem functioning. Annu. Rev. Ecol. Evol. Syst. 45, 471–493. doi: 10.1146/annurev-ecolsys-120213-091917
Vallejo, I. (2020). Estimates of, and Influences on, Aboveground Biomass Production of Tropical Savanna (Cerrado) in the Cuiabá Basin and Pantanal of Mato Grosso, Brazil. MS Thesis. California State University. 37.
Viani, R. A. G., Rodrigues, R. R., Dawson, T. E., and Oliveira, R. S. (2011). Savanna soil fertility limits growth but not survival of tropical forest tree seedlings. Plant Soil 349, 341–353. doi: 10.1007/s11104-011-0879-7
Vourlitis, G. L., Hentz, C. S., Pinto, O. B. Jr., Carneiro, E., and Nogueira, J. S. (2017). Soil N, P, and C dynamics of upland and seasonally flooded forests of the Brazilian Pantanal. Global Ecol. Conserv. 12, 227–240. doi: 10.1016/j.gecco.2017.11.004
Vourlitis, G. L., Lobo, F. A., Lawrence, S., Holt, K., Zappia, A., Pinto, O. B. Jr., et al. (2014). Nutrient resorption in tropical savanna forests and woodlands of Central Brazil. Plant Ecol. 215, 963–975. doi: 10.1007/s11258-014-0348-5
Vourlitis, G. L., Lobo, F. A., Lawrence, S., Lucena, I. C., Borges, O. P. Jr., Dalmagro, H. J., et al. (2013). Variations in stand structure and diversity along a soil fertility gradient in a Brazilian savanna (Cerrado) in southern Mato Grosso. Soil Sci. Soc. Am. J. 77, 1370–1379. doi: 10.2136/sssaj2012.0336
Vourlitis, G. L., Lobo, F. A., Pinto, O. B. Jr., Dalmagro, H. J., Arruda, P. H. Z., and Nogueira, J. S. (2015). Variations in aboveground vegetation structure along a nutrient availability gradient in the Brazilian Pantanal. Plant Soil 389, 307–321. doi: 10.1007/s11104-014-2364-6
Vourlitis, G. L., Pinto, O. B. Jr., Dalmagro, H. J., Arruda, P. E. Z., Lobo, F. A., and Nogueira, J. S. (2022). Tree growth responses to climate variation in upland and seasonally flooded forests and woodlands of the Cerrado-Pantanal transition of Brazil. For. Ecol. Manag. 505:119917. doi: 10.1016/j.foreco.2021.119917
Vourlitis, G. L., Pinto, O. B. Jr., Dalmagro, H. J., de Arruda, P. E. Z., and de Almeida Lobo, F. (2023). Nitrogen and phosphorus pools and fluxes in upland and seasonally flooded forests and woodlands of the Cerrado-Pantanal transition of Brazil. Trees Forests People 12:100383. doi: 10.1016/j.tfp.2023.100383
Vourlitis, G. L., and Rocha, H. R. (2011). “Flux dynamics in the cerrado and cerrado–forest transition of Brazil” in Ecosystem function in global savannas: Measurement and modeling at landscape to global scales. eds. M. J. Hill and N. P. Hanan (Boca Raton, FL: CRC, Inc), 97–116.
Vourlitis, G. L., Zappia, A., Pinto, O. B. Jr., Arruda, P. H. Z., Santanna, F. B., Dalmagro, H. J., et al. (2019). Spatial and temporal variations in aboveground woody carbon storage for cerrado forests and woodlands of Mato Grosso, Brazil. J. Geophys. Res. Biogeosci. 124, 3252–3268. doi: 10.1029/2019JG005201
Keywords: alpha diversity, carbon storage, ecotone, tree growth, Shannon Weiner index, Simpson’s index, species-area curves, structural diversity
Citation: Vourlitis GL, Pinto OB Jr, Dalmagro HJ, Arruda PH and de Almeida Lobo F (2024) Diversity-productivity relationships of savanna ecosystems in the Cerrado-Pantanal transition of southern Mato Grosso, Brazil. Front. For. Glob. Change. 7:1380600. doi: 10.3389/ffgc.2024.1380600
Edited by:
Yule Roberta Ferreira Nunes, State University of Montes Claros, BrazilReviewed by:
Natalia Mesa-Sierra, Western Institute of Technology and Higher Education, MexicoNathalle Fagundes, Minas Gerais State University, Brazil
MArly Antonielle Ávila, State University of Montes Claros, Brazil
Copyright © 2024 Vourlitis, Pinto, Dalmagro, Arruda and de Almeida Lobo. This is an open-access article distributed under the terms of the Creative Commons Attribution License (CC BY). The use, distribution or reproduction in other forums is permitted, provided the original author(s) and the copyright owner(s) are credited and that the original publication in this journal is cited, in accordance with accepted academic practice. No use, distribution or reproduction is permitted which does not comply with these terms.
*Correspondence: George L. Vourlitis, Z2VvcmdldkBjc3VzbS5lZHU=