- 1Natural Resources Canada, Canadian Forest Service, Pacific Forestry Centre, Victoria, BC, Canada
- 2Retired, Victoria, BC, Canada
- 3FPInnovations, Vancouver, BC, Canada
Research on reducing the movement of pests on wood products has led to several options for safer trade including heat treatment of wood to mitigate pests. In this study, pathogenic organisms commonly regulated in the trade of forest products were tested to determine the minimum heat dose (temperature and time) required to cause mortality. The mycelial stage of tree pathogens, Heterobasidion occidentale, Grosmannia clavigera, Bretziella fagacearum, Phytophthora cinnamomi, P. lateralis, P. ramorum and P. xmultiformis, which may be found in untreated wood products, were tested in vitro using the Humble water bath with parameters simulating the rate of heat applied to wood in a commercial kiln. RNA detection using reverse transcription real-time PCR was used to validate pathogen mortality following treatment for: P. ramorum, P. lateralis, P. cinnamomi, P. xmultiformis and G. clavigera. The lethal temperature for all pathogens ranged from 44 to 50°C for a 30-min treatment duration. Using this method to evaluate heat treatment for other forest product pests is recommended to accurately identify the minimum dose required to support phytosanitary trade. With more data potentially lower heat treatment applications may be recommended under specific conditions to produce more efficient and economical heat treatment schedules and reduce environmental impacts.
Introduction
Forests are facing unprecedented changes in climate, anthropogenic pressures, and exposure to new pests (Millar and Stephenson, 2015; Ramsfield et al., 2016; Seebens et al., 2017; Prăvălie, 2018). These changing conditions result in maladaptation in trees and an increasing frequency and scale of disease outbreaks (Sturrock et al., 2011; Weed et al., 2013). It is becoming increasingly important that the pressure on forests caused by exposure to invasive pests is reduced. The availability of effective phytosanitary measures for forest products can reduce the risk of pest introductions resulting in safer trade practices (Allen et al., 2017; Haack et al., 2022). Fungi and other inconspicuous organisms can be transported in untreated wood products and if introduced to new environments, can be detrimental to forest ecosystems (Brasier, 2001; Gonthier et al., 2004). Trade regulations that require phytosanitary measures are often implemented by importing countries to protect forest resources and reduce the impacts caused by the establishment of pathogens; these can be found on the websites of National Plant Protection Organizations (NPPO, e.g., APHIS, 2024). Costs for managing introduced species, and the loss of revenue caused by mortality as well as lost trading opportunities can be extensive. Several Phytophthora species have been added to quarantine and regulated pathogen lists in many countries due to recent introductions that have resulted in widespread damage to forest ecosystems [e.g., P. ramorum, P. alni, P. kernoviae (EPPO Global Database, 2023)]. For instance, P. ramorum is internationally regulated (EPPO Global Database, 2023). It causes sudden oak death, sudden larch death, and ramorum blight, and has a host range of more than 100 plant and tree species in Europe and North America where it is introduced (Brasier et al., 2004a,b; Grünwald et al., 2008, 2019). Phytophthora ramorum is estimated to have caused well over a hundred million dollars in losses in the United States in management costs, loss of nursery and ornamental crops, loss in property value and ecological impacts (Dart and Chastagner, 2007; Kovacs et al., 2011; Grünwald et al., 2019; LeBoldus et al., 2022). The root pathogen Phytophthora cinnamomi affects nearly 5,000 different host species, several of which are critical to agriculture, forestry, and horticulture (Hardham and Blackman, 2018). Phytophthora cinnamomi is notorious for its disastrous effects on the economy and biodiversity and is included in the list of Key Threatening Processes in the Commonwealth Environmental Protection & Biodiversity Conservation Act 1999 in Australia, where it has the potential to affect 50% of all plant species present in forests and heathlands, and it is listed among the top 100 worst invasive alien species by the Global Invasive Species Database (Lowe et al., 2000; Murphy and Van Leeuwen, 2021; Global Invasive Species Database, 2024). The economic impacts of P. cinnamomi are large; in California alone, avocado crop losses due to the disease are in excess of $40 M USD annually (Ploetz, 2013). The oak wilt pathogen, Bretziella fagacearum, formerly Ceratocystis fagacearum (De Beer et al., 2017) is a lethal pathogen of Quercus spp.(Juzwik et al., 2008). It is currently found in the eastern and midwestern states and Texas in the United States on oak trees and was recently reported on Castanea (Juzwik et al., 2011; Chahal et al., 2024). The removal and replacement of oak trees in urban areas alone has been estimated at $266–420 M CDN (Haight et al., 2011; Pedlar et al., 2020). Oak wilt was first reported in Canada in 2023, where it was found in three locations in Ontario (CFIA, 2024). Effective phytosanitary treatments not only prevent accidental introductions but can keep vital international trade pathways open and minimize economic losses due to lost trade opportunities.
The application of heat to treat plant products is a successful phytosanitary method used to reduce the spread of pests with traded commodities. Heat is used to treat agricultural products ensuring sanitary requirements for human health and phytosanitary requirements to reduce the movement of pests on this pathway are met (Usall et al., 2016). For example, hot water dipping (50°C for 5 min) and hot air treatment (40°C for 4 h) of mangoes effectively reduces the risk of moving fungal pests on fruit and reduces future contamination (Mansour et al., 2006). Vapor heat treatment applied to papaya fruits (47.2°C for 3 h) and mangoes (46.5°C for 40 min) effectively causes the complete mortality of fruit fly eggs (the most heat resistant life stage; Le et al., 2010; Hsu et al., 2018).
Similarly, heat treatment has been used for a century to mitigate wood product pests. Guidelines outlining effective methods and parameters for the application of heat to wood products are documented and shared internationally through International Standards for Phytosanitary Measures (ISPM 39 for movement of wood and ISPM 15 for wood packaging material) (IPPC, 2021a,b) and Regional Standards for Phytosanitary Measures (RSPM 41 for systems approaches for wood products) (NAPPO, 2018). These guidelines are implemented and described in detail in national programs outlined and monitored by National Plant Protection Organizations (NPPOs) and implemented by industry. For example, Canada’s NPPO, the Canadian Food Inspection Agency (CFIA), outlines specific heat treatment guidelines for wood exports in the Technical Heat Treatment Guidelines and Operating Conditions Manual (CFIA, 2023). This manual provides specific heat treatment schedules developed for different wood types based on material thickness (including combined thickness) and provides prescribed schedules including heat energy, temperatures, and time required for lethal treatment to ensure forestry products meet the phytosanitary requirements of trading partners.
Heat treatment parameters may vary according to the material being treated (e.g., type of plant tissue or soil) with consideration of economic and environmental implications and to the quality of the infected material, ensuring it will not be altered by the heating process (e.g., fruit quality, physical characteristics of wood). Heat treatments applied to eliminate a pathogen without harming nursery trees and fruit may require application of lower temperatures for longer periods of time (Horner et al., 2019). In some cases, combining lower heat treatment temperature with other biological control agents resulted in enhanced efficacy of the control agents and induced resistance of the plants to the pathogens (Sui et al., 2016). Horner et al. (2019) found soil should be heated to 50°C for 72 h, while plants could be treated at 40°C for 1–2 days or 35°C for 7 or more days to deactivate P. agathidicida. Furthermore, repeated daily heat treatment of 40°C for 8 h eradicated culturable foliar endophytes of Norway spruce (Picea abies) after 9 days of treatment (Müller and Hamberg, 2021). Lesions on live Citrus paradisi trees were heat treated with a blowtorch resulting in complete mortality of P. parasitica above 60°C with full recovery of the tree (Hough et al., 1979).
Wood products can tolerate higher temperatures without compromising the end product. Variability in heat treatment parameters (time, temperature, and kiln schedule) and wood characteristics (e.g., species, density, moisture content, etc.) make it difficult to identify the specific dose required to cause mortality of fungi inhabiting wood tissues. Different temperature–time combinations have been tested under various experimental conditions over the years for a range of different pathogens. For example, temperatures ranging from 49 to 57°C were tested over a 16-day period to treat decay fungi (Polyporus, Trametes, Alternaria, and Fomes) in logs (Hubert, 1924), while treatment required to kill decay fungi, blue stain fungi, and root and canker fungi in wood blocks was shown with slightly higher effective temperatures (55–60°C) at shorter treatment times (15–60 min) (Montgomery, 1936; Uzunovic and Khadempour, 2007; Uzunovic et al., 2008). In vitro applications for various fungi showed a range of treatments were effective, e.g., Coniophora (45–100°C), Gloeophyllum (65–100°C) with decreasing exposure times (Kurpik and Wazny, 1978), Ophiostoma (60°C for 10 min), Phellinus (50°C for 20 min) and Phytophthora (40°C for 40 min) (Hosking, 2007). More recent studies have shown temperatures in the range of 48–56°C for 30–35 min are effective for many important species (Phytophthora, Cladosporium, Geosmithia) while higher heat (61°C) or longer treatment times (60 min) were effective for some species (Lasiodiplodia and Ophiostoma) (Ramsfield et al., 2010; Mayfield III et al., 2014). A few species may require even higher temperatures such as Schizophyllum commune, a thermotolerant wood-decay fungus that showed complete mortality at 71°C for 30 min (Ramsfield et al., 2010).
Developing heat treatment protocols (schedules) for wood and wood products that will be accepted globally or bilaterally between trading partners is challenging. Scientific evidence is needed to demonstrate that the effectiveness of the treatment for traded wood commodities reduces pest risk to a level acceptable to the importing country. Differences in the physical characteristics of the wood to be treated, confound the challenge including, for example, presence of bark, wood density, moisture content, size and structure of the wood commodity (whole logs, sawn wood, chips, etc.). These physical differences affect how heat is transferred through the wood; how the “dose” of heat determined to be enough to kill the target pest is “delivered” unhindered to pest organisms inhabiting wood tissues.
Determining, proving and agreeing on the appropriate temperature to apply as a lethal dose is a challenge. Experiments can be carried out on pests either while they are in wood (in vivo), or on pests that are isolated from the wood (in vitro) where insect life stages, fungi or nematodes are exposed directly to heat. For testing fungi, fungus-like organisms (e.g., Phytophthora) and nematodes, samples are generally cultured on small pieces of wood, grain seeds, or on nutrient agar (plates or tubes) and then exposed to test conditions. Both in vivo and in vitro approaches have pros and cons that need to be considered in interpreting results for policy development, as summarized below.
In vivo (tests using infested wood)
Pros
• Test subjects are unaffected by handling procedures providing a close to a real-life scenario.
• The wood may be providing some form of physical or chemical protection; factors that would not show up in in vitro studies.
• The test results may be directly applicable to traded commodities (e.g., tests in wood chips for wood chip trade) and immediately adopted to be used providing that the test meets the required statistical reliability.
• In vivo tests may reveal interactions (e.g., competition) with other organisms that could affect overall treatment outcomes (Uzunovic and Khadempour, 2007).
Cons
• Physical characteristics of the wood can modify the rates of heating and cooling making it difficult to determine the precise lethal temperature and duration of exposure.
• The number of test organisms cannot be precisely determined which is a challenge for experimental design.
• The health status of organisms cannot be observed prior to treatment.
• For insects, the observation of treatment effects is limited to adult emergence counts or post-treatment dissection of wood to assess insects. Dissection can result in damage to insects, obscuring observation of treatment effects.
• Experiments are expensive and logistically challenging, limiting the number of species that can be tested.
• It may be a challenge to isolate pathogens from infested wood in either control or treated wood samples, which may confound results.
In vitro
Pros
• Sample organisms can be pre-screened for vitality, size, life stage, etc., in order to meet experimental design requirements.
• Tests are more affordable and easier to control and organize.
• Sample organisms are observed and assessed before and after treatment for a direct comparison of treatment effects.
• Pathogens are generally easy to re-isolate after treatment. Controls typically have 100% recovery.
• Replicate (ring) studies in different parts of the world allow for testing of “quarantine” organisms in their natural range.
• The precise lethal dose can be determined without the confounding physical factors introduced by the wood substrate. Understanding this physiologically-driven lethal temperature can then be used to develop cost- and energy-efficient heat treatment systems for any commodities.
Cons
• Handling of some pests may cause them to be stressed, damaged and more susceptible to treatment compared to pests in a natural environment (e.g., dissecting early instar insect larvae from wood).
• In the real world, test organisms may be affected by physical or chemical attributes of the substrate, possibly modifying response to treatment.
• In vitro results need to be validated through some form of in vivo testing.
The current study investigates specific dosage (temperature–time combination) to effectively cause mortality in a range of pathogens in the absence of the substrate with a tightly-controlled heat application system. Using the Humble water bath, both the treatment temperature, rate of heat applied, final treatment temperature and treatment time can be controlled with precision (Noseworthy et al., 2023). With the specific lethal temperature dose, heat treatment kiln schedules, which take into account how the heat moves through the woody material, can be designed with confidence to deliver the required dose in all parts of treated wood. We tested the specific lethal temperature dose for a range of forest pathogens (Heterobasidion occidentale, Grosmannia clavigera (=Ophiostoma clavigerum), Bretziella fagacearum, Phytophthora cinnamomi, P. lateralis, P. ramorum and P. xmultiformis) that have both an environmental and economic impact, occupy different niches in the tree including both heartwood and sapwood pathogens, and are from different taxonomic groups, the fungi and oomycetes (see Table 1 for species authorities). Treatment efficacy was validated using reverse transcription real-time PCR. This method was available for five of the species heat treated (P. ramorum, P. lateralis, P. cinnamomi, P. xmultiformis and G. clavigera). The technique and treatment were evaluated using three treatment and three control grains for each isolate.
Methods and materials
Seven taxonomic groups of forest pathogens were selected for testing, covering a range of hosts, host tissues normally infested and taxa, including both fungi and Phytophthora species (Table 1). Where possible, multiple isolates of each species were included in the study. The decay fungus, Gloeophyllum sepiarium, was included as a positive control as it is a known thermophile (Kurpik and Wazny, 1978; Xie et al., 1997; NAPPO, 2014). Barley (Hordeum vulgare) was used to grow and maintain individual isolates following the Sinden grain spawn method as follows (Sinden, 1932). Whole organic barley was washed and soaked in distilled water for 24 h, then simmered for 10 min, strained, and cooled. Once cool, the barley was rinsed for 15 min, distributed into Erlenmeyer flasks that were plugged with cotton wrapped in gauze to allow air-flow, and capped with aluminum foil. Flasks were autoclaved for 30 min at 121°C and once cooled, each flask was inoculated with several five-millimeter diameter pieces of agar containing mycelium. Flasks were incubated at 25°C for 2–4 weeks with occasional mixing by manual shaking. Colonized barley grains were transferred to sealed Falcon tubes and stored at 4–8°C.
To confirm the identity of the isolates used in the study, DNA was extracted using one of two methods: in the first, the DNeasy Ultraclean Microbial kit (QIAGEN Inc. Toronto, Canada) was used following the manufacturer’s protocol with the following modifications: mycelium was collected into PowerBead tubes, immersed in liquid nitrogen for 30 s, and shaken at maximum speed on a vortex adapter for 90 s before proceeding to step two of the protocol; after step three, samples were incubated at 70°C for 10 min and then processed two times on the FastPrep-24 5G Bead Beating grinder and lysis system (MP Biomedicals, Irvine, CA, United States) for 30 s at 4 m/s before proceeding to step five. In the second method, mycelium was placed into 2 mL MPBio tubes containing lysing matrix D (MP Biomedicals, Irvine, CA, United States), immersed in liquid nitrogen, and placed on a VWR mini bead mill (VWR International, Radnor, PA, United States) at maximum speed for 30 s. Samples were then heated at 95°C in a VWR heat block (VWR International, Radnor, PA, United States) for 1 min and diluted 1:10 in sterile water. The internal transcribed spacer (ITS) regions one and two of the nuclear ribosomal RNA genes (rDNA) were amplified in PCR using the primers ITS1F (Gardes and Bruns, 1993) and ITS4 (White et al., 1990) for fungi, and ITS6 (Cooke et al., 2000) with ITS4 for Phytophthora species. PCR reactions using DNA from the DNeasy kit were performed in 50 μL volumes and contained 1.25 units of Invitrogen Platinum Taq DNA polymerase (ThermoFisher Scientific, Waltham, MA, United States), 1X PCR buffer, 1.5 mM MgCl2, 0.2 mM Invitrogen dNTPs (ThermoFisher Scientific, Waltham, MA, United States), and 0.2 μM of each primer. The second set of reactions using the mechanical lyse and boil protocol were amplified in 25 μL volumes and contained 0.5 units of GoTaq Hot Start polymerase (Promega Corporation, Madison, WI, United States), 1X PCR buffer, 1.5 mM MgCl2, 0.2 mM of each dNTP (Promega Corporation, Madison, WI, United States) and 1 μM of each primer. All reactions were performed with an initial heating step at 94°C for 2 min, followed by a touchdown step with six cycles of 94°C for 30 s, 57°C for 30 s and reducing by 1°C/cycle to 52°C, and 72°C for 1 min, followed by 30 cycles at 94°C for 30 s, 55°C for 30 s, and 72°C for 1 min, finishing with 72°C for 10 min. PCR products were visualized on a 1% agarose gel stained with SafeGreen (Applied Biological Materials, Richmond, Canada). PCR products were sent to the Plateforme de Séquençage et de Génotypage des Genomes, Center de Recherche du CHUL (Quebec, Canada) for sequencing. Species identities were obtained by comparing the sample ITS sequences with sequence data in Genbank (United States Center for Biotechnology Information) using BLAST searches (Altschul et al., 1990).
Heat treatment
Isolates used for experimentation were transferred to room temperature (20°C) 24 h before the experiment. Prior to treatment, inoculated barley grains were placed in sterilized 1/2-dram flat-bottom glass vials sealed with sterilized stoppers (000 natural latex). Vials with stoppers were further sealed with PetriSEAL (Diversified Biotech, Dedham MA). For each isolate, six grains with actively growing mycelium were treated at each treatment temperature, and three grains with actively growing mycelium were plated as untreated controls. The G. sepiarium isolates (positive control) were treated at the same temperatures as the test isolates.
The vials were lowered into the Humble water bath at a start temperature of 20°C and then heated at a rate of 0.5°C/min, reduced to 0.2°C/min for the final 1°C, until reaching the treatment target temperature. Samples were held at that temperature for 30 min before removal and acclimation to room temperature (for water bath methods, photos and details see Noseworthy et al., 2023). In a pilot run, a subset of species was tested at 56°C, 52°C, and no treatment (control). In the second stage of the experiment, maximum survival temperatures and minimum lethal temperatures were established. To determine the maximum survival temperature, we started with a 52°C treatment, and subsequent treatments decreased by 2°C, descending until each species showed growth after treatment. The minimum lethal temperature was defined as 2°C above the maximum survival temperature.
Post-treatment
After heat treatment the grains were aseptically removed from the vials and plated on 20% V8 agar [V8 Juice 200 mL, agar 15 g, CaCO3 3 g, deionized water to 1 L (Miller, 1955)] for Phytophthora species, or 2% malt extract agar with yeast extract (malt extract 20 g, agar 15 g, yeast extract 1 g, deionized water 1 L) for fungal species. Plates were sealed with Parafilm, incubated at room temperature (20–25°C) and observed for the presence of mycelial growth from the grain to agar every week for 8 weeks to assess survival.
Design of molecular detection assays
Pathogen mortality was confirmed with RNA detection using reverse transcription real-time PCR for the species for which a molecular diagnostic method was developed. Three treated and three control grains for P. cinnamomi, P. lateralis, P. ramorum, P. xmultiformis, and G. clavigera isolates were placed in cryovials, held for 72 h after treatment to allow for the degradation of mRNA (see Wong et al., 2020) and placed in liquid nitrogen for RNA analysis. Assays for P. ramorum and G. clavigera were designed as per Wong et al. (2020), and for the other three Phytophthora species as per Leal et al. (in preparation).
DNA and RNA from mycelia samples in barley were simultaneously extracted from each sample using an AllPrep DNA/RNA mini kit (QIAGEN Inc. Toronto, Canada) as per the manufacturer protocol. Prior to adding the RLT plus lysis buffer the mycelia containing barley grains were transferred to a Lysing Matrix M tube (MP Biomedicals, Santa Ana, CA, United States) and frozen in liquid nitrogen. The samples were then ground into powder using three 20-s intervals at 5.5 m/s, placing the tube in liquid nitrogen between grinds. To preserve RNA integrity 1.5 μL of 20 U/L Superase-In (Fisher Scientific, Waltham, MA, United States) were added after elution from the column to each RNA sample. DNA and RNA concentrations were determined as per Wong et al. (2020) and Leal et al. (in preparation).
cDNA synthesis, qPCR, and RT-qPCR
Using 20 ng of RNA, cDNA synthesis was performed using the QuantiTect Reverse Transcription Kit (QIAGEN Inc. Toronto, Canada) including the additional steps as per Wong et al. (2020).
Real-time PCR mix included 0.8X QuantiTect Multiplex PCR Master Mix (QIAGEN Inc. Toronto, Canada), 500 nM for all forward primers, and for the reverse primer for P. ramorum and P. lateralis with the exception of 300 nM for P. cinnamomi, and 400 nM for P. xmultiformis, 150 nM of each TaqMan probe, 2.0 ng of template (gDNA or cDNA), and ROX dye for a final volume of 25 μL. Thermal cycling parameters were used as in Leal et al. (in preparation). Species-specific primer pairs and probes were designed and tested to confirm specificity and level of sensitivity for each of the Phytophthora species and G. clavigera as per Leal et al. (in preparation). TaqMan probes were designed to be able to distinguish between the gDNA and cDNA of a given target species as per Wong et al. (2020) and Leal et al. (in preparation). For the measure of mRNA stability post heat treatment, the real-time PCR reactions of each sample were performed in triplicate. The positive control consisted of RT-qPCR amplification from the corresponding nucleic acids from mycelia of all species and isolates. The negative control was a non-template control (NTC control).
Statistical analysis
The proportion of viable pathogen was estimated by using the ratio of mRNA over gDNA quantity [i.e., quantification cycle (Cq) ratio of the real-time PCR probe targeting cDNA over Cq value of the probe targeting gDNA]. This ratio was the unit of measurement used to compare the efficacy of heat treatment parameters by using Rcmdr v2.9 package (R Core Team, 2024). Average comparisons were carried out with ANOVA when the assumption for normality was respected, and a non-parametric Kruskal-Wallis test was used when normality of the data was not respected (i.e., cDNA control vs. cDNA heat-treatment).
Results
Sample identities were confirmed as listed in Table 1. In the first stage of the experiment, there was no survival in any of the six replicates of all pathogen isolates that were heat treated to 56°C for 30 min. For the heat tolerant G. sepiarium, used as a positive control, all replicates survived, as did all of the negative control isolates (three control grains per isolate) that received no heat treatment.
The effective treatment temperature for the pathogen species tested ranged from 44 to 50°C for the 30 min exposure (see Table 2; Figure 1). The only species able to survive above 50°C was the heat tolerant, positive control, G. sepiarium. Different isolates of the same species shared similar lethal temperatures or were within 2°C of the other isolates. Similarly different species of the same genus shared similar lethal temperatures, the minimum lethal temperature for all Phytophthora species isolates tested was 46°C or 44°C and all B. fagacearum isolates were killed at 48°C.
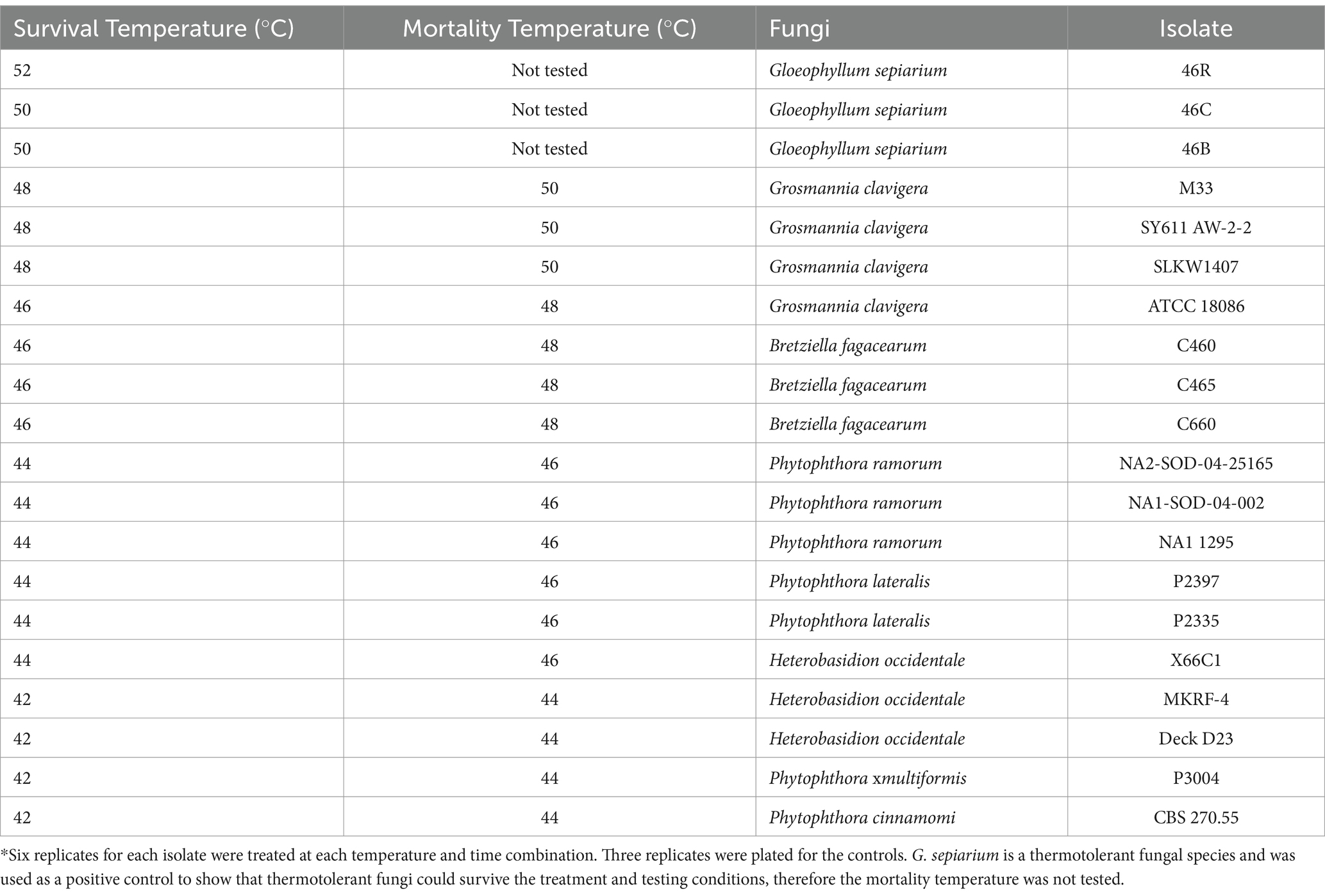
Table 2. Survival and mortality of heat-treated fungal species brought up to temperature with a ramp speed of 0.5°C/min (0.2°C/min for the final 1°C to reduce overshoot) and maintained for 30 min in a hot water bath.
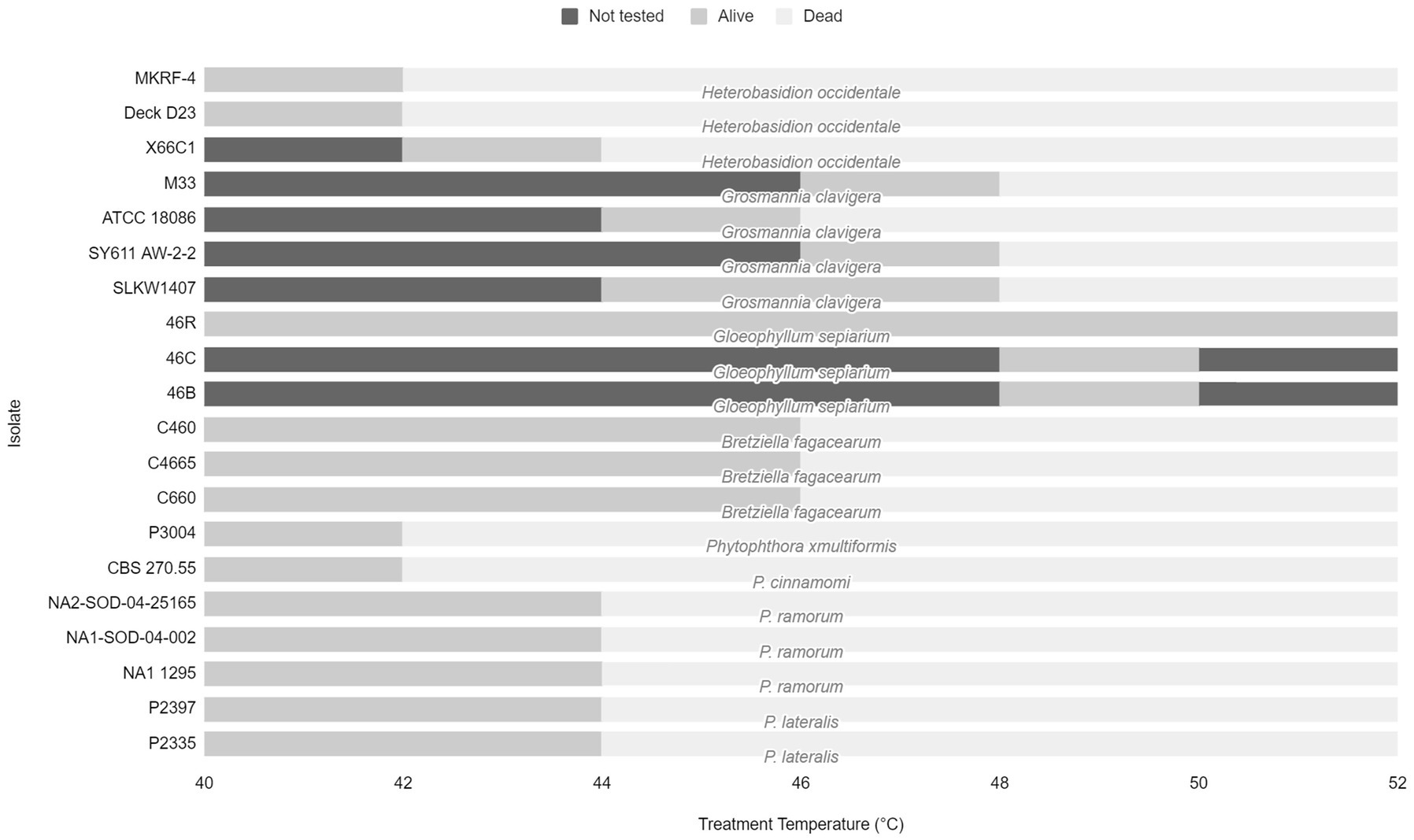
Figure 1. Range of treatment temperatures from 40 to 52°C tested for each fungal species and isolate to determine lethal temperatures. Dark gray bars represent temperature not tested. Light gray bars represent the treatment temperature that the pathogen survived. Pale gray bars represent the lethal temperature.
Use of molecular assays to assess viability of pathogens following heat treatment
Ratio of mRNA over gDNA quantity as measured by Cq values for cDNA and gDNA were compared following heat treatment. Genomic DNA (gDNA) was systematically detected for each species in controls and after heat treatment of the infected barley grains. However, we observed a significant difference in gDNA Cq values between the controls (mean Cq of 23.81 +/− 1.37) and heat-treated samples (27.18 +/− 1.20) indicating that gDNA is affected by heat for all species (F value of 245.2, p < 0.0001; Figure 2). For the cDNA (mRNA) assays, the Cq values obtained on controls (i.e., untreated grains; mean Cq of 29.53 +/− 0.88) were significantly higher than those for gDNA (mean Cq of 23.81 +/− 1.37) indicating later detections than the one observed for gDNA (F-value of 887.1, p < 0.0001; Figure 2). No cDNA was amplified for each species following their respective heat treatments (Cq values > = 40.0 or “undetermined”), suggesting that mRNA was completely degraded by these lethal temperatures.
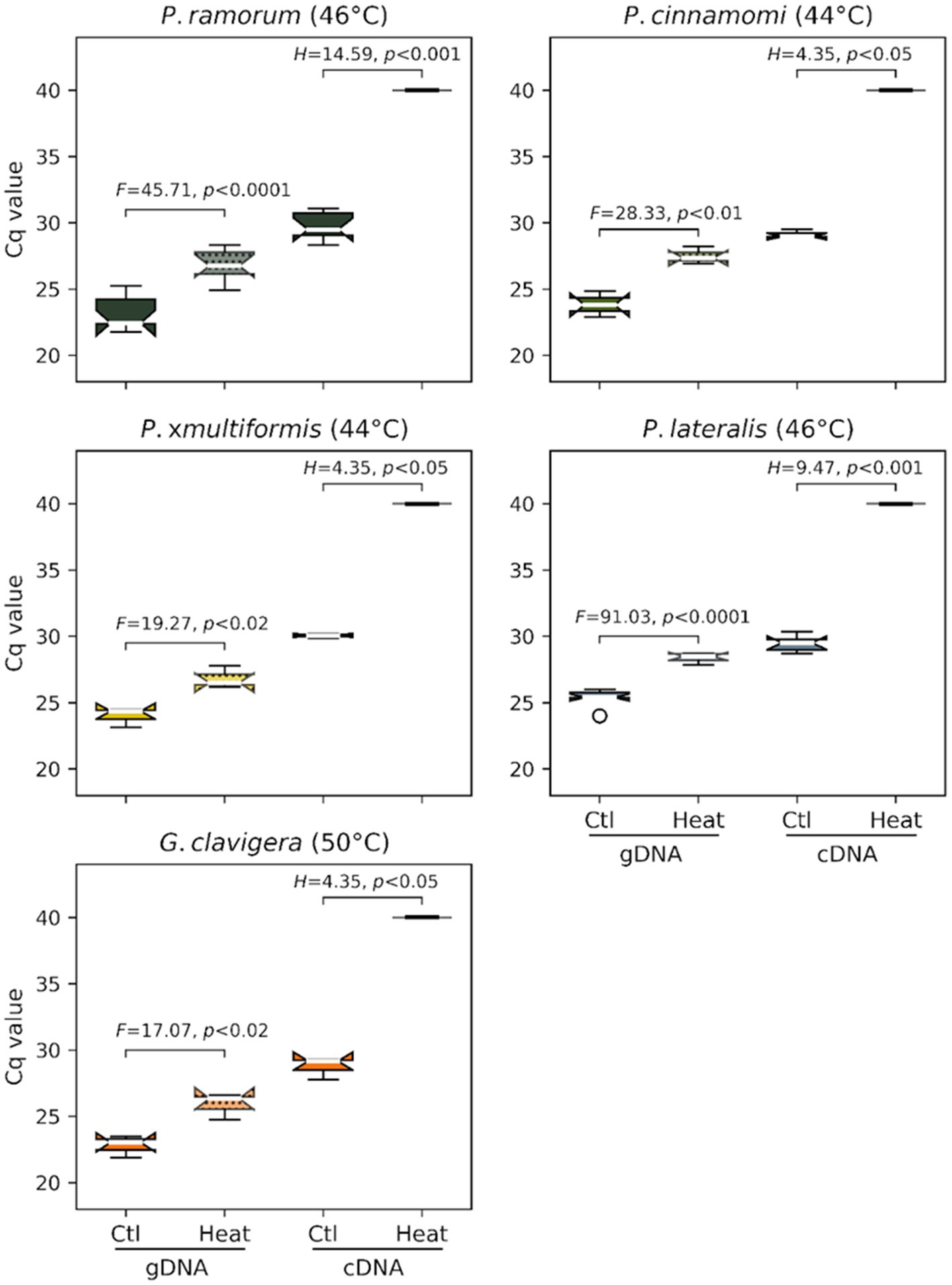
Figure 2. Efficacy of the minimum lethal temperature dose applied to four Phytophthora species and G. clavigera. Each graph represents distributions of cycle-threshold (Ct) values obtained by real-time PCR assays targeting these organisms from gDNA (left two box plots) or mRNA (cDNA) (right two box plots) extracted from barley grains sampled after heat treatment.
Discussion
While there are a large number of pathogens that may be associated with forest commodities, a small number have been reported to move with global forest product trade and establish in novel forest ecosystems. Well-known examples of introduced pathogens include Dutch elm disease caused by Ophiostoma ulmi and O. novo-ulmi in Europe and North America (Brasier, 2001), chestnut blight caused by Cryphonectria parasitica (Loo, 2009), ash dieback caused by Hymenoscyphus fraxineus in Europe (Landolt et al., 2016; Hultberg et al., 2020), sudden oak death caused by P. ramorum in North America (Grünwald et al., 2019), and Phytophthora dieback in Australia caused by P. cinnamomi (Hardham and Blackman, 2018). To ensure these and other forest pathogens are not introduced or spread through forest product trade, heat treatment of wood has been and continues to be used. Numerous studies investigating the effect of heat treatment on pests have been conducted specifically for insects and nematodes (Smith, 1991; Merkl and Tusnádi, 1992; Ikediala et al., 2000; Mushrow et al., 2004; Nzokou et al., 2008; Myers et al., 2009; Hoover et al., 2010; Ramsfield et al., 2010; Sobek et al., 2011; Mayfield et al., 2014; Dubey et al., 2016; Callan et al., 2018; Pawson et al., 2019; MacQuarrie et al., 2020; Noseworthy et al., 2023), however fewer have studied the effect of heat on tree pathogens (Hubert, 1924; Montgomery, 1936; Kurpik and Wazny, 1978; Uzunovic and Khadempour, 2007; Uzunovic et al., 2008; Ramsfield et al., 2010; Mayfield III et al., 2014). Fungal pathogens in wood are more cryptic in nature and less well-known and studied. Comparisons of heat treatment studies to kill forest pathogens are challenging due to a number of variables which are difficult to control (e.g., dimensions of infested material, heat application method, overshoot of the target temperature and time, initial moisture content, density, irregularity of wood fiber around knots and scars). The in vitro approach described here, using inoculated barley grains in a carefully controlled water bath, provided a unique method to study the effect of heat on the stage commonly found in wood products, mycelium. The use of small, inoculated samples allows for efficient delivery of the treatment across the medium without issues associated with physical penetration of heat. We suggest that these data reflect the physiological lethal temperature for the organisms tested. The physiological lethal temperature should not change, regardless of the substrate, as long as the target temperature can be delivered. With this information treatment schedules can be developed to deliver a lethal dose taking into consideration the variable characteristics of the wood being treated. The precision provided by this approach should allow more reliable, environmentally sound, and cost-effective heat treatment alternatives. By using RNA detection assays as a criterion of viability on a subset of samples, we corroborated the results of minimum lethal temperature for pathogen mortality obtained by conventional plating methods.
In the current study, the minimum lethal temperature, defined in this study as 2°C above the maximum tested temperature at which the organism was able to survive, for all of the tested species (except the known thermophile, G. sepiarium) was below 50°C for 30 min indicating none of the other isolates are heat tolerant (Kurpik and Wazny, 1978; Xie et al., 1997; NAPPO, 2014). This is below the required minimum treatment temperature and time of 56°C for 30 min using a conventional steam or dry kiln chamber specified in ISPM No. 15, which addresses the treatment requirements for wood packaging material in international trade (IPPC, 2021a). Within the species tested in our experiment, some isolates of G. clavigera survived treatment at 48°C and did not survive treatment at 50°C, still well below the recommended treatment conditions for wood packaging material. A range of pathogens important in wood trade and representative of several different types of disease were tested in this study. We tested both fungal and oomycete pathogens, both of which can be challenging to detect due to their cryptic nature.
Testing of more organisms using this methodology would increase our understanding of the thermal death point across a wide range of invasive pathogen species. In addition, experiments to correlate in vivo and in vitro testing should be carried out. Furthermore, given that many destructive invasive pathogens are unknown to cause disease in their endemic host range, testing diverse forest-associated pathogens (i.e., currently non-regulated, non-invasive species) may proactively identify the lethal temperature for species of potential phytosanitary concern. Working with indigenous species in their current range provides a safe environment for testing and avoids the need to move species to novel habitats where they might escape. Using the same methods and apparatus would provide comparable results (i.e., a ring study). Future studies should also consider fungal propagules such as chlamydospores, conidia, basidiospores, and ascospores, to compare heat treatment effectiveness for other life stages (Nguyen et al., 2014). The causes of variability in response to heat treatment among isolates of the same species could be an artifact of sub-culturing and should be pursued further using the Humble water bath (Ryan et al., 2001). Preliminary analysis suggested that both mycelium age and isolate source can affect response to temperature. Such effects should be studied further and incorporated into both in vivo and in vitro testing. Where possible we tested at least 3 isolates per species in order to address variation within a species. Currently there is no guidance provided on what the required number of isolates should be in pathogen heat treatment testing (Ormsby, 2022). Ideally three isolates from different geographical locations would be tested (Ramsfield et al., 2010). Further work on variability in heat tolerance within a population or species will be investigated by this group.
This current study shows heat treatment is effective for many invasive pathogens. These results provide support for current heat treatment regimes for forest products outlined in ISPM 15 provided that the target temperature/time is delivered throughout the treated wood. Pairing data on heat treatment dose with kiln schedules which apply heat throughout the profile of a wood product is essential for confidence in treatment parameters and delivery. Two recent studies have examined an insect pest in vitro and in vivo to show that the lethal temperature is conserved (e.g., EAB, MacQuarrie et al., 2020; Noseworthy et al., 2023). We propose pairing in vitro hot water bath experiments with core probe studies of heat transfer across wood or kiln schedules developed using core probes and wet and dry bulbs. The current research on tree pathogens has stimulated ideas for future studies. The Humble water bath offers an opportunity to precisely measure variability between isolates or populations, testing vectors and their associated organisms, mycelium age and condition, and different pathogen life stages.
With increasing global trade and consumer demand, effective phytosanitary treatments and a thorough understanding of lethal temperatures are important tools for regulators and countries to limit the spread of invasive forest pathogens. The importance of these tools is only expected to grow as the climate becomes more favorable for pathogen emergence and spread (Aguayo et al., 2014; Burgess et al., 2017).
Data availability statement
The data presented in the study are deposited in the Dryad repository, accession number https://doi.org/doi:10.5061/dryad.7m0cfxq3k.
Author contributions
MN: Conceptualization, Data curation, Funding acquisition, Investigation, Methodology, Project administration, Resources, Supervision, Validation, Writing – original draft, Writing – review & editing. EA: Conceptualization, Methodology, Writing – original draft, Writing – review & editing. AD: Data curation, Formal analysis, Methodology, Validation, Writing – original draft, Writing – review & editing. IL: Investigation, Methodology, Validation, Writing – original draft, Writing – review & editing. EJ: Data curation, Formal analysis, Investigation, Methodology, Writing – original draft, Writing – review & editing. TS: Investigation, Methodology, Data curation, Formal analysis, Writing – original draft, Writing – review & editing. JT: Investigation, Methodology, Validation, Writing – review & editing. AU: Conceptualization, Investigation, Methodology, Validation, Writing – review & editing.
Funding
The author(s) declare that financial support was received for the research, authorship, and/or publication of this article. This work was supported by Natural Resources Canada.
Acknowledgments
Leland M. Humble for the design of the water bath and first experiments, Forest Research (Research Agency of the Forestry Commission) for fungal isolates P. alni P3004, P. lateralis P2397 and P2335, FPInnovations for fungal isolates, Stacey Kus, Carmina Raposo, Brett Foord for technical assistance and Nicolas Feau for preparation of Figure 2.
Conflict of interest
The authors declare that the research was conducted in the absence of any commercial or financial relationships that could be construed as a potential conflict of interest.
Publisher’s note
All claims expressed in this article are solely those of the authors and do not necessarily represent those of their affiliated organizations, or those of the publisher, the editors and the reviewers. Any product that may be evaluated in this article, or claim that may be made by its manufacturer, is not guaranteed or endorsed by the publisher.
References
Aguayo, J., Elegbede, F., Husson, C., Saintonge, F. X., and Marcais, B. (2014). Modeling climate impact on an emerging disease, the Phytophthora alni-induced alder decline. Glob. Chang. Biol. 20, 3209–3221. doi: 10.1111/gcb.12601
Allen, E., Noseworthy, M., and Ormsby, M. (2017). Phytosanitary measures to reduce the movement of forest pests with the international trade of wood products. Biol. Invasions 19, 3365–3376. doi: 10.1007/s10530-017-1515-0
Altschul, S. F., Gish, W., Miller, W., Myers, E. W., and Lipman, D. J. (1990). Basic local alignment search tool. J. Mol. Biol. 215, 403–410. doi: 10.1016/s0022-2836(05)80360-2
APHIS. (2024). Animal and Plant Health Inspection Service U.S. Department of Agriculture. Available at: https://www.aphis.usda.gov/aphis/ourfocus/importexport. (Accessed May 27, 2024).
Brasier, C. M. (2001). Rapid evolution of introduced plant pathogens via interspecific hybridization: hybridization is leading to rapid evolution of Dutch elm disease and other fungal plant pathogens. Bioscience 51, 123–133. doi: 10.1641/0006-3568(2001)051[0123:REOIPP]2.0.CO;2
Brasier, C. M., Beales, P., Kirk, S. A., Barton, V., Giltrap, P., Griffin, R., et al. (2004b). A new Phytophthora species affecting European beech (Fagus sylvatica) and rhododendron in the UK. Proceedings of the California Oak Mortality Task Force Symposium, Monterey, California, pp. 9–11.
Brasier, C. M., Denman, S., Rose, J., Kirk, S. A., Hughes, K. J. D., Griffin, R. L., et al. (2004a). First report of ramorum bleeding canker on Quercus falcata caused by Phytophthora ramorum. Plant Pathol. 53:804. doi: 10.1111/j.1365-3059.2004.01079.x
Burgess, T. I., Scott, J. K., McDougall, K. L., Stukely, M. J. C., Crane, C., Dunstan, W. A., et al. (2017). Current and projected global distribution of Phytophthora cinnamomi, one of the world’s worst plant pathogens. Glob. Chang. Biol. 23, 1661–1674. doi: 10.1111/gcb.13492
Callan, B. E., Leal, I., Islam, A., and Foord, B. (2018). Analysis of Canadian wood pellets for the presence of living phytopathogenic fungi and pinewood nematode. EPPO Bull. 48, 245–253. doi: 10.1111/epp.12476
CFIA. (2023). Technical Heat Treatment Guidelines and Operating Conditions Manual. Canadian Food Inspection Agency. Available at: https://inspection.canada.ca/plant-health/forestry/exports/ht-program/pi-07/eng/1383841840107/1383841890825 (Accessed July 10, 2023).
CFIA. (2024). Canadian Food Inspection Agency. Oak wilt. Available at: https://inspection.canada.ca/plant-health/invasive-species/plant-diseases/oak-wilt/eng/1325624048625/1325624535106.
Chahal, K. S., Wachendorf, E. J., Miles, L. A., Stallmann, A., Lizotte, E. L., Mandujano, M., et al. (2024). First report of Bretziella fagacearum infecting chestnut in Michigan. Plant Dis. [ahead of print]. doi: 10.1094/PDIS-10-23-2267-PDN
Cooke, D. E. L., Drenth, A., Duncan, J. M., Wagels, G., and Brasier, C. M. (2000). A molecular phylogeny of Phytophthora and related oomycetes. Fungal Genet. Biol. 30, 17–32. doi: 10.1006/fgbi.2000.1202
Dart, N. L., and Chastagner, G. A. (2007). “Estimated economic losses associated with the destruction of plants due to Phytophthora ramorum quarantine efforts in Washington state” in Proceedings of the sudden oak death third science symposium. eds. S. J. Frankel, J. T. Kliejunas, and K. M. Palmieri (Albany, CA: U.S. Department of Agriculture, Forest Service, Pacific Southwest Research Station), 341–343.
De Beer, Z. W., Marincowitz, S., Duong, T. A., and Wingfield, M. J. (2017). Bretziella, a new genus to accommodate the oak wilt fungus, Ceratocystis fagacearum (Microascales, Ascomycota). MycoKeys 27, 1–19. doi: 10.3897/mycokeys.27.20657
Dubey, M. K., Janowiak, J., Mack, R., Elder, P., and Hoover, K. (2016). Comparative study of radio-frequency and microwave heating for phytosanitary treatment of wood. Eur. J. Wood. Prod. 74, 491–500. doi: 10.1007/s00107-016-1025-2
EPPO Global Database. (2023). Phytophthora ramorum (PHYTRA). Available at: https://gd.eppo.int/taxon/PHYTRA/categorization (Accessed Dec 20, 2023).
Gardes, M., and Bruns, T. D. (1993). ITS primers with enhanced specificity for basidiomycetes–application to the identification of mycorrhizae and rusts. Mol. Ecol. 2, 113–118. doi: 10.1111/j.1365-294X.1993.tb00005.x
Global Invasive Species Database. (2024). 100 of the World's worst invasive alien species. Available at: https://www.iucngisd.org/gisd/100_worst.php.
Gonthier, P., Warner, R., Nicolotti, G., Mazzaglia, A., and Garbelotto, M. (2004). Pathogen introduction as a collateral effect of military activity. Mycol. Res. 108, 468–470. doi: 10.1017/S0953756204240369
Grünwald, N. J., Goss, E. M., and Press, C. M. (2008). Phytophthora ramorum: a pathogen with a remarkably wide host range causing sudden oak death on oaks and ramorum blight on woody ornamentals. Mol. Plant Pathol. 9, 729–740. doi: 10.1111/j.1364-3703.2008.00500.x
Grünwald, N. J., LeBoldus, J. M., and Hamelin, R. C. (2019). Ecology and evolution of the sudden oak death pathogen Phytophthora ramorum. Annu. Rev. Phytopathol. 57, 301–321. doi: 10.1146/annurev-phyto-082718-100117
Haack, R. A., Hardin, J. A., Caton, B. P., and Petrice, T. R. (2022). Wood borer detection rates on wood packaging materials entering the United States during different phases of ISPM 15 implementation and regulatory changes. Front. Forests Glob. Change 5:1069117. doi: 10.3389/ffgc.2022.1069117
Haight, R. G., Homans, F. R., Horie, T., Mehta, S. V., Smith, D. J., and Venette, R. C. (2011). Assessing the cost of an invasive forest pathogen: a case study with oak wilt. Environ. Manag. 47, 506–517. doi: 10.1007/s00267-011-9624-5
Hardham, A. R., and Blackman, L. M. (2018). Pathogen profile update Phytophthora cinnamomi. Mol. Plant Pathol. 19, 260–285. doi: 10.1111/mpp.12568
Hoover, K., Uzunovic, A., Gething, B., Dale, A., Leung, K., Ostiguy, N., et al. (2010). Lethal temperature for pinewood nematode, Bursaphelenchus xylophilus, in infested wood using microwave energy. J. Nematol. 42, 101–110. doi: 10.1007/s10086-012-1306-2
Horner, I., Arnet, M., Bellgard, S., Probst, C., Paynter, Q., and Claydon, J. (2019). 17748 temperature treatment protocol for deactivating oospores of Phytophthora agathidicida. Final report. Biosecurity New Zealand technical report paper no: 2020/06. Wellington, New Zealand. pp. 1–39.
Hosking, G. (2007). Temperature mortality thresholds for insects and fungi in New Zealand. Unpublished report. pp. 1–10.
Hough, A., Mulder, N. J., and La Grange, J. M. (1979). Heat treatment for the control of Phytophthora gummosis in citrus. Plant Dis. Rep. 63, 40–43,
Hsu, Y., Chen, S., Lin, K., Shiesh, C., Lin, C., and Yeh, W. (2018). Quarantine vapor heat treatment of papaya fruit for Bactrocera dorsalis and Bactrocera cucurbitae (Diptera: Tephritidae). J. Econ. Entomol. 111, 2101–2109. doi: 10.1093/jee/toy170
Hubert, E. E. (1924). Effect of kiln drying, steaming and air seasoning on certain fungi in wood. USDA, Rep. USDA Dept. Bull. No. 1262.
Hultberg, T., Sandström, J., Felton, A., Öhman, K., Rönnberg, J., Witzell, J., et al. (2020). Ash dieback risks an extinction cascade. Biol. Conserv. 244:108516. doi: 10.1016/j.biocon.2020.108516
Ikediala, J. N., Tang, J., and Wig, T. (2000). A heating block system for studying thermal death kinetics of insect pests. Trans. ASAE 43, 351–358. doi: 10.13031/2013.2711
IPPC (2021a). International standard for phytosanitary measures no. 15: Regulation of wood packaging material in international trade. Published by FAO on behalf of the secretariat of the international plant protection convention. Rome: International Plant Protection Convention, 24.
IPPC (2021b). International standard for phytosanitary measures no. 39: International movement of wood. Published by FAO on behalf of the secretariat of the international plant protection convention (IPPC). Rome: International Plant Protection Convention, 20.
Juzwik, J., Appel, D. N., MacDonald, W. L., and Burks, S. (2011). Challenges and successes in managing oak wilt in the United States. Plant Dis. 95, 888–900. doi: 10.1094/PDIS-12-10-0944
Juzwik, J., Harrington, T. C., MacDonald, W. L., and Appel, D. N. (2008). The origin of Ceratocystis fagacearum, the oak wilt fungus. Annu. Rev. Phytopathol. 46, 13–26. doi: 10.1146/annurev.phyto.45.062806.094406
Kovacs, K., Václavík, T., Haight, R. G., Pang, A., Cunniffe, N. J., Gilligan, C. A., et al. (2011). Predicting the economic costs and property value losses attributed to sudden oak death damage in California (2010–2020). J. Environ. Manag. 92, 1292–1302. doi: 10.1016/j.jenvman.2010.12.018
Kurpik, V. W., and Wazny, J. (1978). Lethal temperatures for the wood-destroying fungi Coniophora putanea Fr. and Gloeophyllum sepiarium (Wulf.) karst. Material und Organismen 13, 1–12,
Landolt, J., Gross, A., Holdenrieder, O., and Pautasso, M. (2016). Ash dieback due to Hymenoscyphus fraxineus: what can be learnt from evolutionary ecology? Plant Pathol. 65, 1056–1070. doi: 10.1111/ppa.12539
Le, T. N., Shiesh, C. C., Lin, H. L., and Lee, E. (2010). Vapour heat quarantine treatment for Taiwan native mango variety fruits infested with fruit fly. J. Appl. Hort. 12, 107–112. doi: 10.37855/jah.2010.v12i02.23
LeBoldus, J. M., Navarro, S. M., Kline, N., Ritokova, G., and Grünwald, N. J. (2022). Repeated emergence of sudden oak death in Oregon: chronology, impact, and management. Plant Dis. 106, 3013–3021. doi: 10.1094/PDIS-02-22-0294-FE
Loo, J. A. (2009). “Ecological impacts of non-indigenous invasive fungi as forest pathogens” in Ecological impacts of non-native invertebrates and fungi on terrestrial ecosystems (Berlin: Springer Science and Business Media), 81–96.
Lowe, S., Browne, M., Boudjelas, S., and De Poorter, M. (2000). 100 of the world’s worst invasive alien species: A selection from the global invasive species database. New Zealand: The invasive species specialist group (ISSG), a specialist group of the species survival commission (SSC) of the world conservation union (IUCN)
MacQuarrie, C. J., Gray, M., Lavallée, R., Noseworthy, M. K., Savard, M., and Humble, L. M. (2020). Assessment of the systems approach for the phytosanitary treatment of wood infested with wood-boring insects. J. Econ. Entomol. 113, 679–694. doi: 10.1093/jee/toz331
Mansour, F. S., Abd-El-Aziz, S. A., and Helal, G. A. (2006). Effect of fruit heat treatment in three mango varieties on incidence of postharvest fungal disease. J. Plant Pathol. 88, 141–148. doi: 10.4454/JPP.V88I2.856
Mayfield, A. E., Fraedrich, S. W., Taylor, A., Merten, P., and Myers, S. W. (2014). Efficacy of heat treatment for the thousand cankers disease vector and pathogen in small black walnut logs. J. Econ. Entomol. 107, 174–184. doi: 10.1603/ec13390
Merkl, O., and Tusnádi, C. K. (1992). First introduction of Xyleborus affinis (Coleoptera Scolytidae), a pest of Dracaena fragans ‘Massangeana’ to Hungary. Folia Entomol. Hung. 52, 67–72,
Millar, C. I., and Stephenson, N. L. (2015). Temperate forest health in an era of emerging megadisturbance. Science 349, 823–826. doi: 10.1126/science.aaa9933
Miller, P. M. (1955). V-8 juice agar as a general purpose medium for fungi and bacteria. Phytopathology 45, 461–462,
Montgomery, H. B. S. (1936). An investigation of the temperatures lethal to some wood-decaying fungi. Trans. Brit. Mycol. Soc. 20, 293–298. doi: 10.1016/S0007-1536(36)80018-7
Müller, M. M., and Hamberg, L. (2021). Eradication of endophytes from needles, their inoculation with Lophodermium piceae and persistence of this fungus in needles of Norway spruce. Mycol. Prog. 20, 981–992. doi: 10.1007/s11557-021-01714-8
Murphy, H., and Van Leeuwen, S. (2021). Australia state of the environment 2021: biodiversity, independent report to the Australian government minister for the Environment, Commonwealth of Australia, Canberra.
Mushrow, L., Morrison, A., Sweeney, J., and Quiring, D. (2004). Heat as a phytosanitary treatment for the brown spruce longhorn beetle. For. Chron. 80, 224–228. doi: 10.5558/tfc80224-2
Myers, S. W., Fraser, I., and Mastro, V. C. (2009). Evaluation of heat treatment schedules for emerald ash borer (Coleoptera: Buprestidae). J. Econ. Entomol. 102, 2048–2055. doi: 10.1603/029.102.0605
NAPPO. (2014). ST 05: Review of heat treatment of wood and wood packaging. North American Plant Protection Organization, Ottawa, Canada, Available at: http://www.nappo.org/files/7714/6013/3041/NAPPO_ST_Heat_treatment_of_wood_woodpkg-e.pdf.
NAPPO (2018). North American plant protection organization RSPM 41: Use of systems approaches to manage pest risks associated with the movement of forest products. Raleigh, North Carolina: The Secretariat of the North American Plant Protection Organization.
Nguyen, H. D., Tanney, J. B., Chabot, D., Nickerson, N. L., and Seifert, K. A. (2014). Paratritirachium curvibasidium, a new heat-resistant basidiomycete from flare pit soils in Alberta, Canada. Mycol. Prog. 13, 575–587. doi: 10.1007/s11557-013-0941-3
Noseworthy, M. K., Humble, L. M., Souque, T. J., John, E. P., Roberts, J., Lloyd, C. R., et al. (2023). Determination of specific lethal heat treatment parameters for pests associated with wood products using the Humble water bath. J. Pest. Sci. 96, 1187–1197. doi: 10.1007/s10340-022-01567-4
Nzokou, P., Tourtellot, S., and Kamdem, D. P. (2008). Kiln and microwave heat treatment of logs infested by the emerald ash borer (Agrilus planipennis Fairmaire) (Coleoptera: Buprestidae). For. Prod. J. 58, 68–72,
Ormsby, M. D. (2022). Elucidating the efficacy of phytosanitary measures for invasive alien species moving in wood packaging material. J. Plant Dis. Protection. 129, 339–348. doi: 10.1007/s41348-022-00571-1
Pawson, S. M., Bader, M. F., Brockerhoff, E. G., Heffernan, W. J., Kerr, J. L., and O’Connor, B. (2019). Quantifying the thermal tolerance of wood borers and bark beetles for the development of joule heating as a novel phytosanitary treatment of pine logs. J. Pest. Sci. 92, 157–171. doi: 10.1007/s10340-018-1015-8
Pedlar, J. H., McKenney, D. W., Hope, E., Reed, S., and Sweeney, J. (2020). Assessing the climate suitability and potential economic impacts of oak wilt in Canada. Sci. Rep. 10:19391. doi: 10.1038/s41598-020-75549-w
Ploetz, R. C. (2013). “Phytophthora root of avocado” in Phytophthora: A global perspective. ed. K. Lamouir (Wallingford, UK: CABI), 197–203.
Prăvălie, R. (2018). Major perturbations in the Earth's forest ecosystems. Possible implications for global warming. Earth Sci. Rev. 185, 544–571. doi: 10.1016/j.earscirev.2018.06.010
R Core Team (2024). R: A language and environment for statistical computing. Vienna, Austria: R Foundation for Statistical Computing.
Ramsfield, T. D., Ball, R. D., Gardner, J. F., and Dick, M. A. (2010). Temperature and time combinations required to cause mortality of a range of fungi colonizing wood. Can. J. Plant. Pathol. 32, 368–375. doi: 10.1080/07060661.2010.499269
Ramsfield, T. D., Bentz, B. J., Faccoli, M., Jactel, H., and Brockerhoff, E. G. (2016). Forest health in a changing world: effects of globalization and climate change on forest insect and pathogen impacts. Forestry 89, 245–252. doi: 10.1093/forestry/cpw018
Ryan, M. J., Jeffries, P., Bridge, P. D., and Smith, D. (2001). Developing cryopreservation protocols to secure fungal gene function. Cryo Letters 22, 115–124
Seebens, H., Blackburn, T. M., Dyer, E. E., Genovesi, P., Hulme, P. E., Jeschke, J. M., et al. (2017). No saturation in the accumulation of alien species worldwide. Nat. Commun. 8:14435. doi: 10.1038/ncomms14435
Sinden, J. (1932). Mushroom spawn and method of making same. (U.S. patent no. US1869517A). U.S. patent and trademark office. Available at: https://patents.google.com/patent/US1869517A/en.
Smith, R. S. (1991). The use of heat treatment in the eradication of the pinewood nematode and its vectors in softwood lumber. Report to the task force on pasteurization of softwood lumber. Forintek Canada Corporation, Vancouver.
Sobek, S., Rajamohan, A., Dillon, D., Cumming, R. C., and Sinclair, B. J. (2011). High temperature tolerance and thermal plasticity in emerald ash borer Agrilus planipennis. Agric. For. Entomol. 13, 333–340. doi: 10.1111/j.1461-9563.2011.00523.x
Sturrock, R. N., Frankel, S. J., Brown, A. V., Hennon, P. E., Kliejunas, J. T., Lewis, K. J., et al. (2011). Climate change and forest diseases. Plant Pathol. 60, 133–149. doi: 10.1111/j.1365-3059.2010.02406.x
Sui, Y., Wisniewski, M., Droby, S., Norelli, J., and Liu, J. (2016). Recent advances and current status of the use of heat treatments in postharvest disease management systems: is it time to turn up the heat? Trends Food Sci. 51, 34–40. doi: 10.1016/j.tifs.2016.03.004
Usall, J., Ippolito, A., Sisquella, M., and Neri, F. (2016). Physical treatments to control postharvest diseases of fresh fruits and vegetables. Postharvest Biol. Technol. 122, 30–40. doi: 10.1016/j.postharvbio.2016.05.002
Uzunovic, A., and Khadempour, L. (2007). Heat disinfestation of mountain pine beetle-affected wood. In: Mountain pine beetle initiative working paper 2007–14, Natural Resources Canada, Canadian Forest Service, Pacific Forestry Centre, Victoria BC. Report Fo143-3/2007-14-E, pp. 1–24
Uzunovic, A., Khadempour, L., and Leung, K. (2008). Heat disinfestation of decay fungi found in post-mountain pine beetle wood. Natural Resources Canada, Canadian Forest Service, Pacific Forestry Centre, Victoria BC. Report Fo144-7/2010E-PDF, pp. 1–12.
Weed, A. S., Ayres, M. P., and Hicke, J. A. (2013). Consequences of climate change for biotic disturbances in north American forests. Ecol. Monogr. 83, 441–470. doi: 10.1890/13-0160.1
White, T. J., Bruns, T., Lee, S., and Taylor, J. W. (1990). “Amplification and direct sequencing of fungal ribosomal RNA genes for phylogenetics” in PCR protocols: A guide to methods and applications. eds. M. A. Innis, D. H. Gelfand, J. J. Sninsky, and T. J. White (New York: Academic Press, Inc.), 315–322.
Wong, B., Leal, I., Feau, N., Dale, A., Uzunovic, A., and Hamelin, R. C. (2020). Molecular assays to detect the presence and viability of Phytophthora ramorum and Grosmannia clavigera. PLoS One 15:e0221742. doi: 10.1371/journal.pone.0221742
Keywords: Humble water bath, forestry pests, sudden oak death, oak wilt, root disease, blue stain, Phytophthora
Citation: Noseworthy MK, Allen EA, Dale AL, Leal I, John EP, Souque TJ, Tanney JB and Uzunovic A (2024) Evidence to support phytosanitary policies–the minimum effective heat treatment parameters for pathogens associated with forest products. Front. For. Glob. Change. 7:1380040. doi: 10.3389/ffgc.2024.1380040
Edited by:
Juan A. Martin, Polytechnic University of Madrid, SpainReviewed by:
Susan Frankel, Forest Service (USDA), United StatesDenita Hadziabdic, The University of Tennessee, United States
Copyright © 2024 His Majesty the King in Right of Canada as represented by the Minister of Natural Resources. This is an open-access article distributed under the terms of the Creative Commons Attribution License (CC BY). The use, distribution or reproduction in other forums is permitted, provided the original author(s) and the copyright owner(s) are credited and that the original publication in this journal is cited, in accordance with accepted academic practice. No use, distribution or reproduction is permitted which does not comply with these terms.
*Correspondence: Meghan K. Noseworthy, meghan.noseworthy@nrcan-rncan.gc.ca