- 1VCSGUUHF, College of Forestry, Tehri, India
- 2ICAR-Central Soil Salinity Research Institute, Karnal, India
- 3ICAR-National Institute of Abiotic Stress Management, Baramati, India
- 4ICAR- Central Coastal Agricultural Research Institute, Old Goa, India
- 5ICAR-Indian Institute of Soil and Water Conservation, Research Centre, Vasad, Gujarat, India
- 6Himalayan Forest Research Institute, Shimla, India
- 7ICAR-Central Agroforestry Research Institute, Jhansi, India
- 8Agriculture University, Jodhpur, India
Climate change and extreme weather events are threatening agricultural production worldwide. The anticipated increase in atmospheric temperature may reduce the potential yield of cultivated crops. Agroforestry is regarded as a climate-resilient system that is profitable, sustainable, and adaptable, and has strong potential to sequester atmospheric carbon. Agroforestry practices enhance agroecosystems’ resilience against adverse weather conditions via moderating extreme temperature fluctuations, provisioning buffers during heavy rainfall events, mitigating drought periods, and safeguarding land resources from cyclones and tsunamis-type events. Therefore, it was essential to comprehensively analyze and discuss the role of agroforestry in providing resilience during extreme weather situations. We hypothesized that integrating trees in to the agro-ecosystems could increase the resilience of crops against extreme weather events. The available literature showed that the over-story tree shade moderates the severe temperature (2–4°C) effects on understory crops, particularly in the wheat and coffee-based agroforestry as well as in the forage and livestock-based silvipasture systems. Studies have shown that intense rainstorms can harm agricultural production (40–70%) and cause waterlogging. The farmlands with agroforestry have been reported to be more resilient to heavy rainfall because of the decrease in runoff (20–50%) and increase in soil water infiltration. Studies have also suggested that drought-induced low rainfall damages many crops, but integrating trees can improve microclimate and maintain crop yield by providing shade, windshield, and prolonged soil moisture retention. The meta-analysis revealed that tree shelterbelts could mitigate the effects of high water and wind speeds associated with cyclones and tsunamis by creating a vegetation bio-shield along the coastlines. In general, existing literature indicates that implementing and designing agroforestry practices increases resilience of agronomic crops to extreme weather conditions increasing crop yield by 5–15%. Moreover, despite its widely recognized advantages in terms of resilience to extreme weather, the systematic documentation of agroforestry advantages is currently insufficient on a global scale. Consequently, we provide a synthesis of the existing data and its analysis to draw reasonable conclusions that can aid in the development of suitable strategies to achieve the worldwide goal of adapting to and mitigating the adverse impacts of climate change.
1 Background
Modern agriculture is greatly affected by a variety of factors, including meteorological conditions, agronomic practices, supply and demand dynamics, price fluctuations, government regulations, and socio-cultural influences (Simelton et al., 2015). The changing climate conditions, including the increasing intensity, frequency, and duration of extreme weather events, pose significant challenges to the both global agricultural production and economies of local communities (FAO, 2015). Recent studies have thoroughly investigated how shifting weather patterns affect the growth and economic output of important crops, offering insightful data analysis (Asseng et al., 2015; Challinor et al., 2016). These studies have shown that despite the general decrease in crop yield and livestock productivity due to changing climate conditions, there can be location-specific differences. Researchers have projected a decline in crop yields ranging from 2.5 to 10% with rising temperatures in the 21st century (Hatfield et al., 2011). Various crops can experience varying degrees of impact, even within the same area (Figure 1). For instance, Zhao et al. (2017) predicted a decrease in the yields of wheat, rice, maize, and soybean crops by 3.2, 7.4, 6.0, and 3.1%, respectively, for every degree rise in the earth’s mean temperature (Figure 2). Studies from sub-Saharan Africa show that shifting climate patterns have different but negative impacts on intensively cultivated crops. Further, Blanc (2012) modeled data on different crops from 37 countries for the period 1961–2002 and predicted that the yield changes under alternative climate scenarios relative to no climate change in 2100 would be −19 to +6% for maize, −38% to −13% for millets, and − 47% to −7% for sorghum. Similarly, Warsame et al. (2023) examined the impacts of climate change and non-climatic factors on maize production in Somalia between 1980 and 2018 and suggested that the mean temperature and rainfall had negative effects on crop yield in both the short and long term.
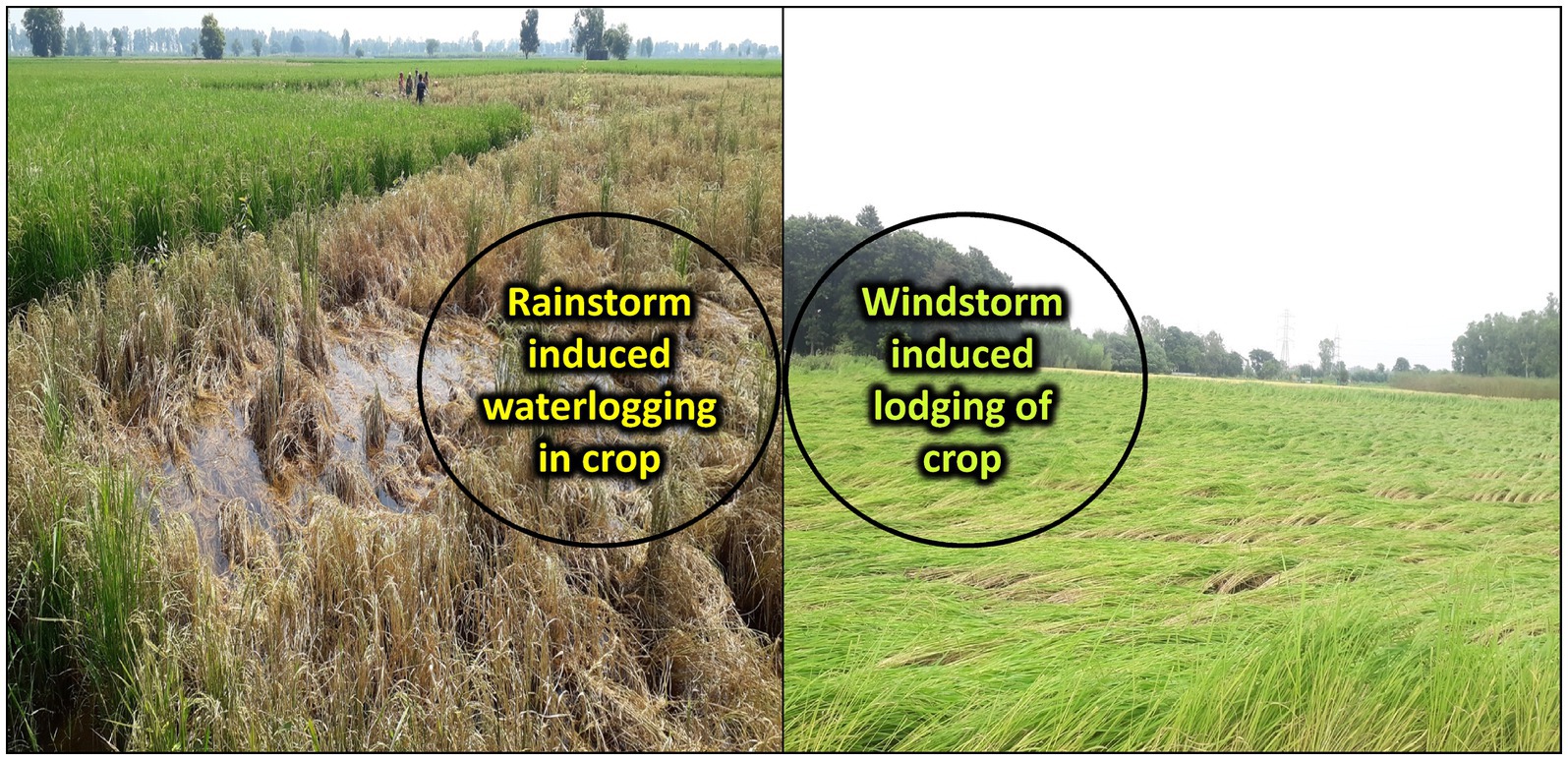
Figure 1. Extreme weather induced damage in rice crop (Photo courtesy: Raj Kumar; Sept, 2023, Karnal, India).
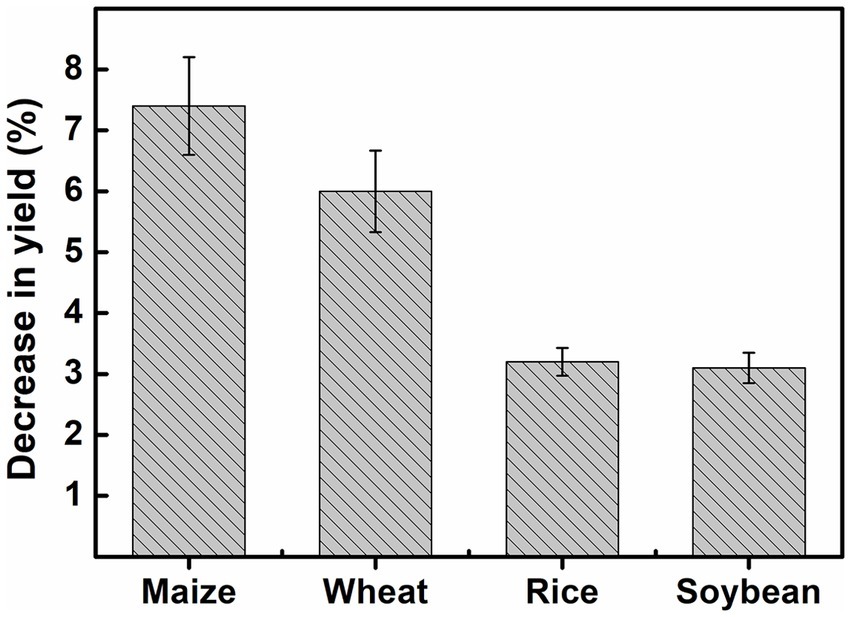
Figure 2. Impacts of a 1°C increase in global temperature on crop yields (adapted from Zhao et al., 2017).
Climate models predict a greater temperature rise in regions closer to the poles than in areas near the equator and in the middle latitudes (IPCC, 2021). As a result, the cultivation of cereal and winter crops would shift toward the north due to the formation of a more favorable climate for their growth (Maracchi et al., 2005; Tuck et al., 2006; Olesen et al., 2007). For example, in the Indian Himalayas, the effect of temperature on apple productivity revealed that rising temperatures at higher elevations create more favorable conditions for plant growth, resulting in greater fruit yields than in lower and mid-altitude regions (Rana et al., 2011). Furthermore, it is crucial to prioritize adaptation and risk mitigation strategies in order to minimize the adverse effects of extreme climatic conditions. Agroforestry, multilayer farming, and silvopastoral systems are a few examples of complex agro-ecosystems that exhibit significant potential for coping with and adapting to extreme weather (Altieri et al., 2015). As a result, it is critical to examine climate-resilient agricultural and agroforestry practices that are appropriate for specific regions and commodities in order to adapt to extreme climatic conditions.
The deliberate introduction of trees in agricultural systems in the form of block plantations, tree-crop combinations, and boundary plantations is widespread agroforestry practice across the globe (Nair et al., 2011). Tree plantation in cropping systems, under diverse management regimens and in various kinds of spatial and temporal arrangements, is essential for reducing vulnerability to uncertain and changing climates (Kumar et al., 2022). Numerous studies have reported the benefits of agroforestry in terms of improving the sustainability, productivity, and profitability of farmlands (Schoeneberger et al., 2012). However, the introduction of trees in croplands also offers a variety of climatic benefits, such as microclimate regulation (Singh et al., 2024), extreme climate tolerance (Noordwijk et al., 2011), and atmospheric carbon sequestration (Kumar et al., 2021). In particular, agroforestry systems have the capacity to enhance the resilience of agronomic crops in the face of a variety of climatic conditions, such as frequent droughts, infrequent floods, and extreme temperature and rainfall events (Altieri et al., 2015). This is accomplished by minimizing the intensity of the adverse effects of changing weather conditions. For instance, tree-crop combinations may be beneficial in regions where the negative effects of climate change are often observed, as crop damage resulting from different weather events can be countered by the output obtained from trees (Kumar et al., 2020a). A large number of farmers have asserted that the diverse tree species play an active role in the adaptation process of the farmers and that this constitutes advance preparation for the anticipated extreme events. For example, Pulhin et al. (2016) found that farmers prefer afforestation and tree protection in anticipation of climate change impacts across all extreme events, including typhoons (19%), drought (21%), and heavy rains (27%), over irrigation and embankment construction. This suggests that agricultural systems that are vulnerable to the adverse impact of climate change, particularly extreme weather events, should consider the implementation of agroforestry practices and tree plantations (Simelton et al., 2015). However, various countries implement a wide range of agroforestry practices, which play a pivotal role in ensuring the long-term sustainability of farming systems (Iizumi and Navin, 2015). Consequently, it was essential to collect factual information regarding the kinds of species and agroforestry systems that have clearly demonstrated a high level of resistance to extreme weather events in the climate change-vulnerable regions of the world (Tables 1, 2). We hypothesized that the integration of trees in agro-ecosystems could enhance crop resilience against extreme weather events under climate change scenarios. This compilation addresses two important issues: (i) What kinds of agroforestry systems are most effective in lessening the effects of extreme weather events? (ii) What types of trees and associated components, as well as their spatial and temporal design, can effectively mitigate the effects of harsh weather in various farming systems? The primary goal of this review was to identify species and propose agroforestry approaches that have demonstrated strong potential for enhancing the resilience of agroecosystems to extreme weather conditions. For the first time, we have made available a special compilation of the potential benefits of agroforestry practices during extreme events. This will enable the drawing of lessons and promote the adoption of climate-resilient practices, which will aid in the development of policies and programs at the regional and global level.
2 Methodology and literature search
The potential of agroforestry to respond and adapt to extreme weather events was analyzed by searching the keywords on the Google and Web of Science. The keywords used are based on (“Agroforestry”) and (“Climate change mitigation” or “extreme weather events” or “extreme temperature” or “extreme rainfall moderation” or “drought” or “tsunami mitigation” or “cyclone”). A dataset comprising 1,222 publications were first extracted which was screen out based on exclusion criteria and within scope of the study. The PRISMA framework is shown in Figure 3.
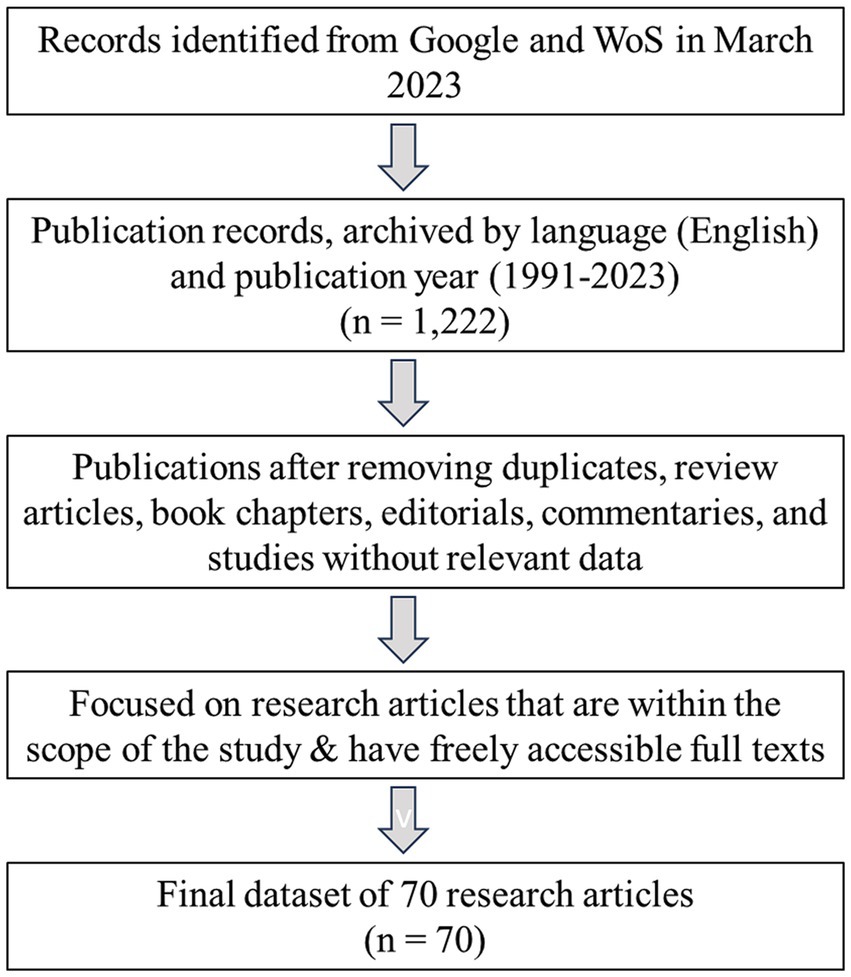
Figure 3. Flow diagram of identification, screening, eligibility, and inclusion of the research studies.
We retrieved 70 research publications from 1991 to 2023 based on these keywords, and collected the data from the databases. The extracted information was categorized into various sections, including the extreme temperature, high rainfall, drought, tsunami, and cyclone. Under each section, the information was organized both agroforestry system-wise and region-wise. Additionally, under each category, all the similar information was organized in a single paragraph and discussed in a chronological pattern based on their relatedness. This subject matter was then followed by other pertinent information on the same aspect. After analyzing the reviewed information, we identified various potential agroforestry models to counter the extreme temperature, high rainfall, drought, and tsunami/cyclone conditions. We conducted a comprehensive analysis after carefully considering the available evidence and information to address the challenges, develop future strategies, and identify the research needs of agroforestry under extreme climate conditions.
3 Agroforestry for mitigating the extreme weather events
3.1 Extreme temperature
The increasing maximum day and night temperatures have a significant impact on crop and animal productivity (Gowda et al., 2018). According to climate models, the earth’s average global temperature will increase by an additional 4°C (7.2°F) in the twenty-first century (IPCC, 2021). Similarly, simulations indicate that a 2°C increase in temperature could lead to a 1.7 times higher likelihood of heavy rain events, with a 14% increase in rainfall intensity (IPCC, 2021). Some of the studies have suggested that there could be a rise in temperature and rainfall (5–20%) during the months of December to February and a decrease in rainfall between June and August by the year 2050 in certain regions of the world (Hulme et al., 2005). The increase in temperatures is projected to have a detrimental impact on most crops, particularly monoculture crops, and the resulting drought could significantly lower crop productivity and create a famine-like situation (Gregory and Ingram, 2000). Smallholder farmers without financial or irrigation resources may use more natural systems to achieve ecological benefits to counteract climate change. Agroforestry systems are natural agro-ecosystems with diverse components that can greatly enhance the resilience of crops to temperature fluctuations (Lin, 2007). The presence of trees on agricultural lands reduces temperature change in a variety of ways (Tables 1, 2). The canopy of trees serves as a buffer against the rapid shift in maximum and minimum temperatures, sheltering the understory from the negative effects of high temperature conditions. The effect of trees on temperature varies not only from one place to another, but also according to the time of year and the day. During the daytime, the tree shade lowers the under-story temperature, protecting the crops from excessive heat during peak summer, whereas at night, the tree canopy maintains higher temperatures, protecting the crops from frost damage, especially during the winter (Gosme et al., 2016). For example, Karki and Goodman (2015) found lower temperatures in agroforestry systems (19.2°C) than in open areas (21.5°C) during the season (Figure 4). This temperature difference between agroforestry and open systems is more marked on clearer days (Gosme et al., 2016). This suggests that the shade of the tree canopy improves the microclimatic conditions for understory crops, increasing their growth and yield (Gregory and Ingram, 2000). The detailed agroforestry system-specific studies are outlined in Table 2.
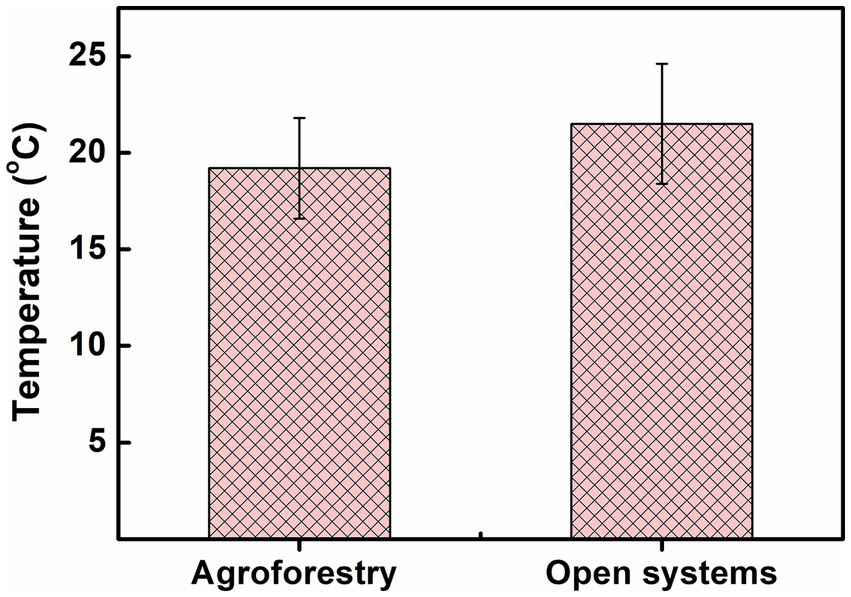
Figure 4. Temperature difference between agroforestry and open systems (adapted from Karki and Goodman, 2015).
3.1.1 Wheat based agroforestry systems
Across the globe, agroforestry systems widely cultivate wheat as an annual crop, which is particularly vulnerable to temperature fluctuations. High air temperatures during crucial developmental stages can have a negative impact on grain yield (Porter and Semenov, 2005). Specifically, temperatures above 30°C can lead to pollen sterility and decrease in seed yield (Gregory and Ingram, 2000). Kanzler et al. (2019) found that during hot summer days, the poplar tree-based alley cropping system had a 3.4°C lower temperature than a sole wheat system. Through the practice of agro-forestry, trees play a crucial role in improving water use efficiency and mitigating heat stress in agricultural crops, which ultimately results in the increase in crop yield. For example, Sida et al. (2018) found that agroforestry systems with Faidherbia albida trees cut down on the amount of photosynthetically active radiation that reached the wheat canopy. This made the wheat 6°C cooler than in open wheat systems. Additionally, temperature exhibited significant variability in open fields, whereas it showed less fluctuation under the tree canopy. This is due to the ability of trees to buffer heat, providing relief from heat stress during crucial reproductive stages. In India, Kohli and Saini (2003) reported more favorable microclimatic conditions for wheat crop development under Populus deltoids, leading to a lower heat load during the post-anthesis period (Figure 5). Such a decrease in heat load under an agroforestry system results in the increase in wheat grain yield by 6.8% (Singh et al., 2024). Similarly, Deswal et al. (2022) showed increase in wheat yield during extreme high temperature conditions with increasing tree density in a Melia dubia-based agroforestry system. Research has shown that windbreaks significantly increase wheat yields by 15 to 20% and are highly effective in protecting plants from the damaging effects of winter desiccation (Brandle et al., 1984; Kort, 1988). Thus, the existing research clearly shows the role of tree shade in improving extreme climatic conditions, highlighting the important role of agroforestry in enhancing the ability of the wheat crop to withstand extreme temperatures.
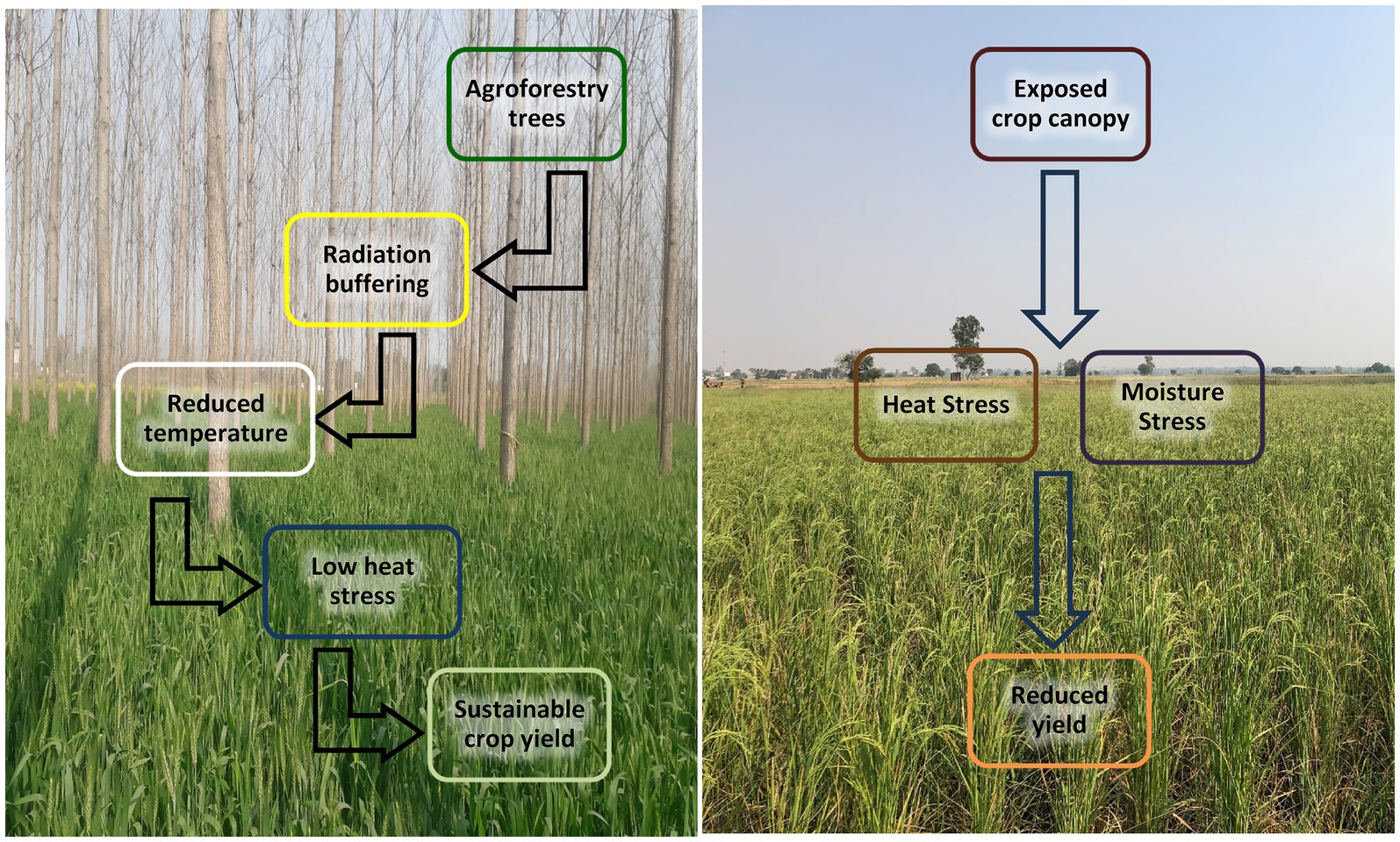
Figure 5. Popular (Populus deltiodes) based agri-silviculture systems enhances resilience of wheat crop against heat load during during extreme temperature events. (Photo courtesy: Raj Kumar; April, 2022, Yamunanager, India).
3.1.2 Coffee based agroforestry systems
Coffee is produced all over the world, with Brazil, Vietnam, Colombia, Indonesia, and Ethiopia being the top coffee-producing nations. Tree species such as Cordia, Albizia, Croton, Persea, Grevillea, and Mangifera are recommended for providing shade to the coffee plants (Wariyo and Negewo, 2023). According to projections, climate change in Brazil might result in a 60% reduction in the area suitable for coffee production (Gomes et al., 2020). However, studies suggest that agroforestry systems have the potential to buffer the negative effects of climate change, and it could preserve 75% of the land suitable for coffee production (Gomes et al., 2020). In Mozambique, the most critical variables that determine the suitability of coffee regions are annual precipitation and altitude. Full sun will be unsuitable for coffee production in Mozambique from 2040 onwards (Cassamo et al., 2023). Shade trees have been found to provide protection for coffee plants against extreme heat and intense sunlight, resulting in a significant decrease in evapo-transpiration loss by 41% during the hot and dry seasons (Lin, 2006). It has been observed that the leaf temperature of coffee plants grown in full sun exceeded the air temperature consistently, while the leaf temperature of shaded coffee plants was lower by 5°C. This difference in temperature could have a positive impact on the physiological processes of the plants (Siles et al., 2010). Further, reports also suggested a decline in the intensity and level of day-night temperature variation in coffee-based agroforestry compared to monoculture. Specifically, Campanha et al. (2004) recorded a 2.6°C decrease in the extreme high temperature in the agroforestry system. Furthermore, literature suggested that trees act as a natural barrier to shield coffee plants from both scorching heat and chilling winds, and they play a vital role in conserving water by reducing evapotranspiration. By mitigating sudden temperature changes between day and night, trees help to prevent thermal shocks that can harm the coffee plants. Thus, the presence of trees enhances the microclimatic conditions, creating a more favorable environment for the growth of coffee plants.
3.1.3 Agri-silviculture systems in tropical Hot-arid conditions
Agri-silviculture systems, which combine trees and crops, are the most common agroforestry practices in tropical hot arid climates. The increase in global temperatures negatively affect growth of agronomic crops, especially in the tropical arid and semi-arid regions of the world (Hatfield et al., 2011). In these areas, temperatures under tree canopies are usually 2–4°C lower compared to places exposed to direct sunlight (Cordeiro et al., 2015). The shade of the tree canopy maintains soil and air temperatures, and the row spacing of the trees has been observed to be inversely related to the atmospheric temperature (Chauhan and Dhiman, 2007). Tree species such as Prosopis and Faidherbia are commonly used in agroforestry systems in the tropical hot-arid regions. In India, Prosopis tree-based agroforestry showed a positive impact on the growth of various crops such as cow pea, cluster bean, Pearl millet, Mustard, Taramira, Wheat, and Mungbean in the hot arid conditions of India (Figure 6). They observed a 10–15% higher crop yield under the tree canopy than outside the canopy (Keerthika et al., 2015). This improvement can be attributed to the positive change in the microclimate, particularly the reduction in soil and air temperature. In Africa, studies have found that the Faidherbia albida systems increase crop yield by two to seven times compared to open systems (Table 3). Under such conditions, tree windbreaks can lead to a 15–30% decrease in crop water consumption because tree rows help shield the crops from intense and direct sunlight (Thevs et al., 2019). Furthermore, tree-based systems could shield understory crops from heat stress in the face of climate change, as the increase in atmospheric heat and soil moisture stress is expected in the near future. This would be accomplished primarily through microclimate modification, which is influenced by the particular species of trees used and their design (Pezzopane et al., 2015). As a result, tree-based systems and their ability to modify microclimates could play a critical role in addressing climate change in hot and arid environments.
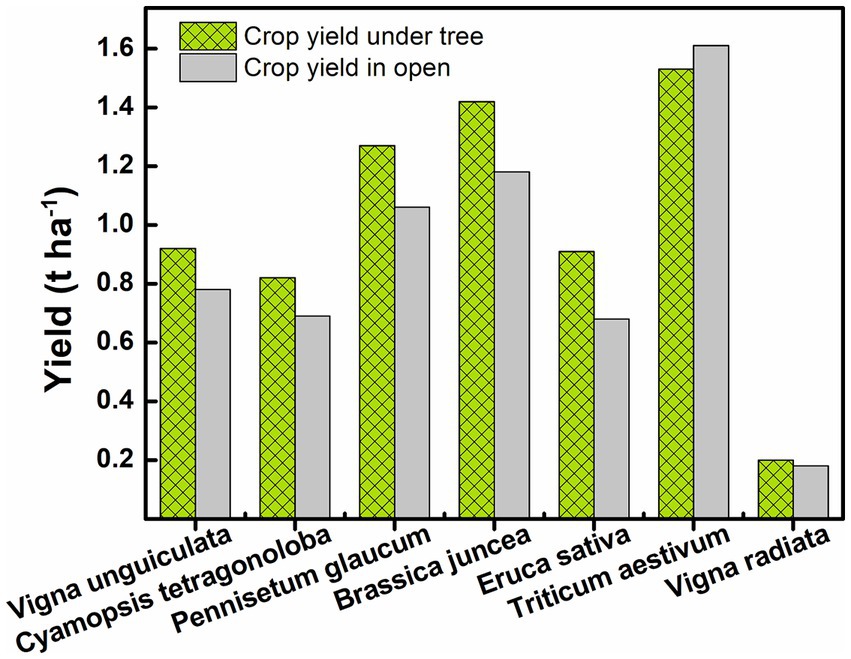
Figure 6. Crop yields under Prospis cineraria agroforestry in Rajasthan (modified from Keerthika et al., 2015).
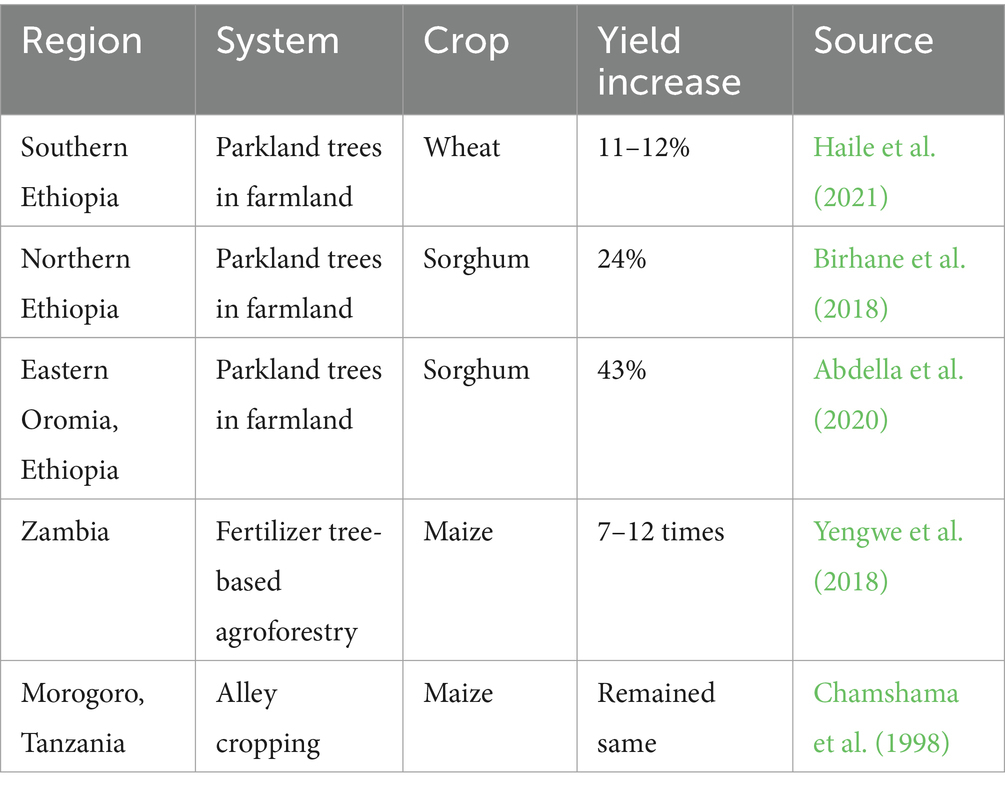
Table 3. Impact of Faidherbia albida agroforestry systems on crop yield under extreme temperatures in the African conditions.
3.1.4 Silvipasture systems
Silvopastoral systems (SPS), which are practiced across the globe, are a form of agroforestry system that combines trees and pastures to fulfill the fodder requirements of domestic animals. During the daytime, extreme high temperature can have a significant impact on the physiology of the livestock, leading to the decrease in their reproductive and milk production capabilities (Karki and Goodman, 2010). Under such circumstances, agroforestry systems like silvopasture and windbreaks can mitigate the heat stress of the animals as well as maintain the productivity and yield of the forage crops (Dosskey et al., 2017). For example, Mitlöhner et al. (2001) revealed that trees with shade increase cattle weight in less time (20 days early) than those without shade because of the lower air temperature under the silvo-pastoral system than in open pastures (Deniz et al., 2019). Silvipasture systems have the potential to help livestock adapt to the impacts of global warming by creating a more favorable thermal environment that is less restrictive to animal performance (Magalhaes et al., 2020). During spring and summer, the silvo-pastoral system offers a comfortable environment for the livestock (Pezzopane et al., 2019). However, livestock are able to thrive in the treeless pastures in the autumn and winter seasons. They further reported that pasture without trees experiences the unfavorable microclimate and can have a higher thermal comfort index (ranging from 79 to 85) during the early hours of the summer season, making it unsuitable for raising domestic animals (Magalhaes et al., 2020). During the winter season, windbreaks can reduce wind speed, which improves feeding efficiency, lessens animal stress, and increases lamb survival (Dosskey et al., 2017). The triple-row groves under agroforestry systems showed the most significant reductions in heat stress conditions, specifically the Black Globe temperature-humidity index and radiant thermal load for grazing animals compared to open pasture. Moreover, the impact of trees on temperature modification is specific to region, climate, season, and tree density. In Florida, United States, sylvopasture practices lower the wind movement, which leads to lower diurnal dew point and higher temperatures (air and soil) in March, June, and September (Karki and Goodman, 2010). In New Zealand, increasing tree stocking from 0 to 400 stems ha−1 in Pinus radiata-based sylvopasture system resulted in increase in the minimum temperature of the grass and decrease in the temperature of the soil (Hawke and Wedderburn, 1994). In temperate regions, tree stocking protects animals from sudden increases in the above-ground temperature (Hawke and Wedderburn, 1994). Therefore, silvopastoral systems can be used to lessen the negative effects of climate change because trees can shield pasture land and animals from strong winds, intense sun radiation, and extremely high or low temperatures. This keeps pasture biomass stable and lowers animal stress, which in turn increases the productivity of the livestock and forage resources (Bosi et al., 2020).
3.1.5 Riparian forestry buffers
A riparian forest buffer consists of a wide variety of trees and other perennial plants that are strategically planted alongside a stream, lake, or wetland. These buffers are intended to provide valuable conservation benefits. The increasing air temperature has a direct impact on the temperature of water resources, which in turn affects the habitats of aquatic fish and animal species (Bowler et al., 2012). According to Mugwanya et al. (2022), the rising temperatures of stream water may have a negative impact on the habitat of cold-water aquatic species. However, the shade provided by riparian forest buffers can help keep the water temperatures cooler (Bentrup and Dosskey, 2017). For instance, Cross et al. (2013) showed that increasing stream shade from 0 to 75 percent can lead to a 4.8°C decrease in maximum temperature. This highlights the crucial role that trees play in regulating and preventing fluctuations in stream temperature. A meta-analysis of 10 research studies reported that riparian forest buffers can reduce maximum and mean summer stream temperatures by 3.3 and 0.60°C, respectively, compared to streams without buffers (Bowler et al., 2012). In snow-covered areas, field windbreaks can impede snow and retain moisture by reducing wind speed and dispersing snow throughout the field. This demonstrates the immense potential of tree-based farming systems to regulate the maximum and minimum temperatures of water resources.
4 High rainfall
Rainfall is important to fulfill the water requirements of both cultivated annuals and perennials. However, high rainfall negatively affects agronomic production, and integrated crop-tree production can mitigate the damage from such rainfall events (Kumar et al., 2020b). For instance, Schoeneberger et al. (2012) found that trees and agroforestry-based farming systems are the most preferred farming practices to mitigate the adverse impact of high rainfall on agronomic production. Specifically, compared to agronomic practices such as no till, cover crops, cropping systems, and crop rotation, adding perennial trees to cropland increases rainfall water infiltration by 59% into the soil (Basche and DeLonge, 2019). In agroforestry, trees prevent the direct impact of raindrops, reduce runoff, and increase soil water infiltration, thereby enhancing crops resilience to extreme rainfall events. Furthermore, expanding tree plantations in agroforestry promotes greater rainwater interception and infiltration, which reduces the quantity, speed, and peak flows of runoff, thereby helping landowners adapt to the adverse impacts of extremely high precipitation events (Dosskey et al., 2017; Hernández-Morcillo et al., 2018). Several farmers in the United States reported increased flooding and landslides because of deforestation, which encouraged them to initiate tree plantations and avoid cutting of the existing trees (Philpott et al., 2008). The multi-story combinations of various perennial and annual crop-based agroforestry systems known as homegardens (Figure 7) are considered one of the most preferred and sustainable land use systems for extreme rainfall events in tropical humid conditions (Torquebiau, 1992; Nguyen et al., 2013). Moreover, crop diversification and tree plantations/agroforestry have been suggested as adaptation strategies for reducing the vulnerability of agronomic crops to high rainfall conditions (Altieri et al., 2015).
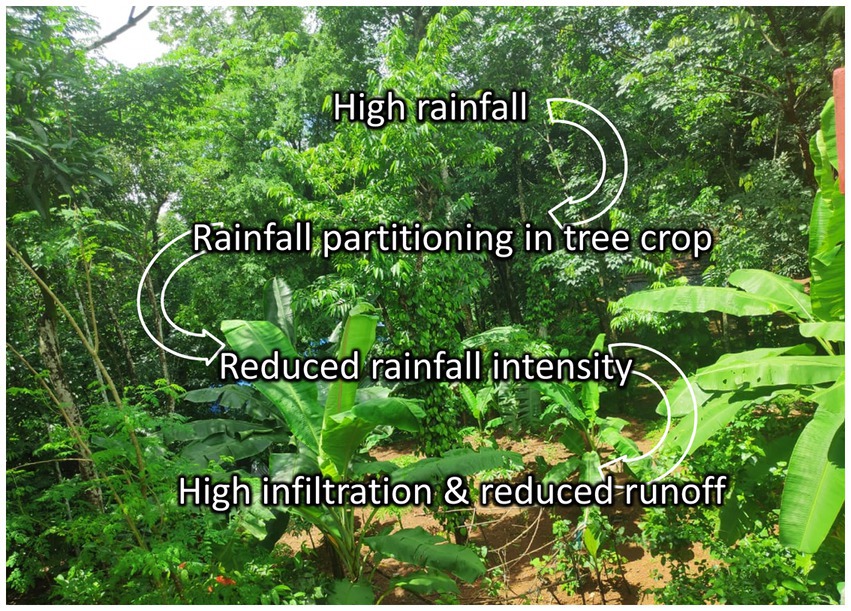
Figure 7. Tropical homegardens consists of multiple crops and trees are considered sustainable land use for extreme rainfall events in conditions. (Photo courtesy: A. R. Uttapha; August, 2022, Goa, India).
In the Indian subcontinent, high rainfall often creates water-logging conditions and salinity hazards, leading to an adverse impact on agronomic production. The cultivation of trees along with crops could transpire excess water into the atmosphere, reducing the adverse impact of waterlogging. For example, under waterlogging conditions in Punjab, India, the study findings showed higher evapo-transpiration rates in the agroforestry systems than sole crops, indicating greater removal of excess water from soils and the creation of a better soil environment for plant growth in the tree-dominated systems (Dhillon et al., 2010). In Sri Lanka, the high rainfall-induced rise in water tables increased the soil salinity of low-lying coastal areas, severely affecting the productivity of agricultural land. By designing and practicing agroforestry along the coastal areas, the high water table and salinity level can be reduced to a great extent (Gopalakrishnan et al., 2019). Bangladesh is also highly vulnerable to heavy rainfall events that occur frequently. The anticipated adverse effect includes increased seawater intrusion, which will inundate coastal agriculture lands and human settlements. One of the innovative interventions suggested by the policy planners is the fish, fruit, and forest model (FFF), an agroforestry practice that generates substantial economic return over short, medium, and long terms and, as well as offering diversified livelihood options, reduces salinity, increases protection, and provides resilience against climate change (Shah, 2014). In Vietnam, heavy rainfall-induced extreme flood conditions caused 40% yield loss of the regionally cultivated crops, while farms with trees were less affected (Nguyen et al., 2013). The various tree species performed satisfactorily during extreme weather conditions to provide multiple benefits, including income, food, feed, and other environmental benefits, to the local population. Likewise, in Uttarakhand state of India, an extremely heavy rainfall (338 mm in 1 day) occurred during 2013 that caused widespread flooding and the occurrence of landslides, leading to huge losses to agricultural crops, human property, and animal life. The crop production was severely affected, resulting in a huge loss to the farmers’ livelihoods and the state’s economy. In contrast, farms with tree-based land use systems were least affected, neutralizing the negative effects of heavy rainfall on agriculture production (Dobhal et al., 2020).
In the United States, high rainfall creates erosion and flooding situations, leading to the occurrence of landslides and the reduction in agricultural productivity. Trees and other permanent vegetation in agriculture systems in the form of agroforestry reduce the adverse impacts of extreme rainfall events, which lead to greater agricultural production (USDA, 2016). Specifically, riparian forest buffers, windbreaks, and alley cropping systems control flooding and soil erosion as well as contribute to sustaining crop production throughout the watershed. Silvopasture and forest farming provide climate-related benefits associated with the extent of forest cover, as well as contributing to diversifying and increasing economic productivity (USDA, 2016). Likewise, in Brazil, analysis showed that integrated systems comprising crops, trees, and animals increase the resilience of farms against extreme rainfall events, as well as control soil erosion, enhance productivity, and generate a large number of socio-economic benefits by increasing the number of products and services available to the farmers (Calmon and Feltran-Barbieri, 2019).
Agroforestry techniques have great potential to reduce farmers’ vulnerability to various climate-related challenges in African conditions. In Kenya, researchers identified that farmers are shifting from sole cropping to diverse agroforestry practices in order to protect their farms from future floods (Quandt et al., 2017). In Mesoamerica, coffee-based agroforestry can regulate various ecological services, such as mitigating drought conditions, improving soil health, and preventing flood occurrences that help reduce the adverse impact of extreme rainfall events (Eychène et al., 2014). Such agroforestry systems help reduce farm vulnerability to climate-related hazards by providing multiple ecosystem services (Thorlakson, 2011). Therefore, during high rainfall events, the tree canopy partitions the rainfall and reduces the rainfall intensity, resulting in decreased runoff, increased infiltration, and reduced waterlogging.
5 Intense drought
Drought is a prolonged dry period in the natural climate cycle, which can have a serious impact on society in general and agriculture in particular. The rise in surface temperature is also predicted to increase the frequency of heat waves and droughts, which can severely affect crop production (IPCC, 2021). In particular, arid and semi-arid regions experience prolonged drought conditions caused by low rainfall, resulting in severe damage to agricultural crops. Under drought conditions, the integration of trees into cropping systems provides numerous benefits, such as shade and protection from high winds that help retain soil moisture. This moderation of the harsh microclimate increases crop resilience against drought-induced risks. Specifically, agroforestry can reduce crop water requirements during hot and dry periods of the cropping cycle (Figure 8). One such example is windbreaks, which conserve soil moisture by reducing wind speed, a major factor controlling soil evaporation and plant transpiration (Brandle et al., 2009), and could be highly valuable under water-stressed conditions (Rivest et al., 2013). Such climatic and environmental co-benefits in a tree-based farming system have been proven highly effective in reducing the frequent drought-related risks in arid environments (Eitzinger et al., 2017). The longer and hotter drought periods reduce the vegetation biomass cover and increase the vulnerability of the soil to accelerated erosion (Smukler et al., 2010). Agroforestry practices involving drought-hardy trees can maintain soil by complementing cover crops, which may face establishment challenges in times of drought (Al-Kaisi et al., 2013; Dosskey et al., 2017). The tree canopy also creates a congenial environment for soil biological activities in water-stressed environments (Martius et al., 2004). Further, evidence has shown that low rainfall-induced drought adversely affects annual crops compared to perennial trees, especially in arid and semi-arid rain-fed conditions (Al-Kaisi et al., 2013; Eitzinger et al., 2017; Kumar et al., 2020a). Under normal rainfall conditions, tree shade could decrease the yield of understory crops, while during the drought, the yield could be higher than in an open system, even in intense shade. For example, Kumar et al. (2020a) reported that trees are more resilient to drought conditions than crops, implying that trees have better water and nutrient efficiency during drought conditions. They examined the performance of Sapota trees (Achras zapota) and regional crops (Cowpea and Castor) in agroforestry systems, demonstrating that the fruit (Sapota) could offset crop failure during drought conditions, indicating the increased resilience of tree-based farming systems in semi-arid climatic conditions. Furthermore, during the simultaneous production of trees and crops, the trees get water and nutrients from deeper layers, thereby helping to mitigate drought conditions. Blanchet et al. (2021) also reported higher pea yield under trees in drought conditions than normal rainfall. In India, some farmers adopt and promote tree planting to counter the impacts of extreme drought (9% farmers) and intense rains (17% farmers) on their agronomic crops (Udmale et al., 2014).
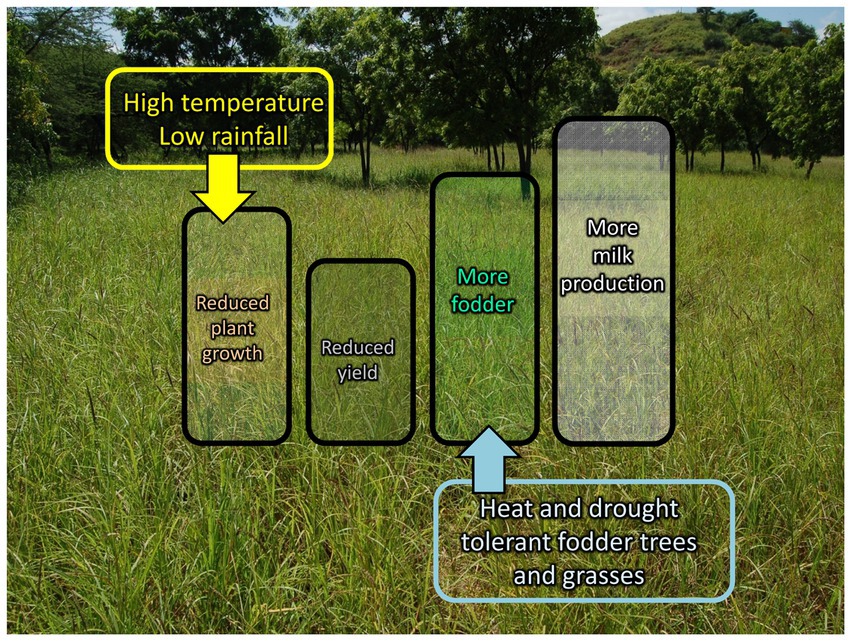
Figure 8. Silvipasture systems for extreme drought conditions in hot and arid climatic conditions (Photo courtesy: Arvind Kumar; September, 2022, Bhuj, India).
A silvipasture system (SPS) at the El Hatico farm in Colombia, composed of large trees, medium-sized trees, Leucaena shrubs, and a five-story layer of grasses, provided fodder throughout the year for sustainable milk production. In 2009, precipitation declined by 44% that resulted in a 25% decline in pasture biomass production, while the biomass of trees and shrubs was least affected, neutralizing the adverse impact of drought on overall fodder production. Other farmers also reported severe weight loss and reduced milk production in the animals because of starvation and thirst (Murgueitio et al., 2001). Similarly, the agro-pastoralists in Southern Zambia face a significant challenge in securing sufficient fodder for their livestock during droughts. This causes a disruption in their primary source of income because the majority of them does not engage in pasture or fodder management. Trees were the most important source of animal food and milk production during drought (Chibinga et al., 2012), demonstrating the tremendous potential of SPS as a sustainable intensification strategy for climate change adaptation.
In Southeast Asia, farmers who engaged in traditional tree-based agroforestry experienced a speedier recovery from natural disasters, which suggests that they have a greater ability to adapt to climate-related hazards (Burgess et al., 2022). In Peru, multiple weather hazards, viz., frost, floods, drought, and hailstorms, severely affect agriculture and farm animals. The households practicing agroforestry are able to counter the risks and cope with the damages from multiple hazards (Jost and Pretzsch, 2012). In Sri Lanka, farms that are rain-fed and devoid of irrigation are being replaced by drought-tolerant cashew tree plantations to reduce the risk of agricultural failure from severe drought conditions (Bantilan and Mohan, 2014). Recently in India, integrated farming systems, comprising a combination of diverse agricultural, horticultural, forestry, animal husbandry, and fishery practices on a piece of land, have been found quite promising, and these types of farming are also at the top agenda of the Indian government for enhancing climate resilience, in addition to increasing farm income and labor employment (Yadav et al., 2021).
African content is one of the most severely affected regions by frequent droughts, which have a significant impact on the agriculture and livelihoods of millions of individuals. In 2005, Niger experienced one of the worst droughts in its history, resulting in widespread food scarcity, and farmers exchanged timber trees in exchange for cereals. To lessen the effects of drought, some farmers were growing tree species for fruit, fuel wood, timber, and fodder in place of annual crops (Miyan, 2015). These studies demonstrate that establishing drought-tolerant trees and crops can help alleviate high-temperature and low-rainfall-induced drought conditions.
6 Tsunami
Tsunamis are waves that occur near or under the ocean. The rising sea levels induced flooding due to storms and/or tsunamis severely affect coastal communities. Climate-associated geological changes triggered the frequency of earthquakes as well as volcanic eruptions, which, in turn, intensify the threat of tsunamis (Latief et al., 2007). The intensity of damage by a tsunami strike in a coastal area is determined by its strength, coastal geomorphology, area topography, and land use-land cover (Chadha et al., 2005; Kurian et al., 2006). Using coastal vegetation for averting disaster incidences was discussed more than 100 years ago in Japan (Honda, 1898). The coastal vegetation can disperse the energy of the waves, check ingress and flooding debris to a significant level, and reduce the chances of severe consequences (ProAct, 2008). Furthermore, the shelterbelts can be designed to achieve a number of additional benefits, including economic (for provisioning food, fuel wood, timber, and household requirements) and environmental (moderating winds, checking sand dune expansion, improving esthetics, and checking erosion) (De Zoysa, 2008). In contrast, coastlines devoid of any vegetation are always susceptible to storms and tsunami disasters (Danielsen et al., 2005). In some countries, reports have outlined that the coastal communities situated at the back of tree cover are less affected compared to those coastal communities that are directly exposed to the sea (Danielsen et al., 2005; Stein and Okal, 2005; Latief et al., 2007).
The establishment and strengthening of greenbelts or coastal forests (including mangroves) can play an important role in minimizing the consequences of future extreme disaster events (Danielsen et al., 2005). To effectively check tsunami damage, the ProAct (2008) recommends a protective mangrove greenbelt width of 100 to 200 m. Parameters like bioshield width, tree height, and stem diameter have been noted to be the key characteristics influencing impact mitigation (Latief et al., 2007). In addition, multitier agroforestry systems, such as home gardens, have also been found effective in resisting the impacts of climate change. The people living along the coast have acknowledged the importance of these home gardens as protective instruments. Particularly, in Sri Lanka, home gardens have been recognized as future protection measures. In Tamil Nadu, India, areas with mangrove forests aid in protecting human life from tsunami like disasters (Kathiresan and Rajendran, 2005). Overall, most of the research studies have recommended an ‘integrated coastal vegetation management system’ (Figure 9) that could augment the vegetation bioshield for many years along the coastal areas (Tanaka, 2009).
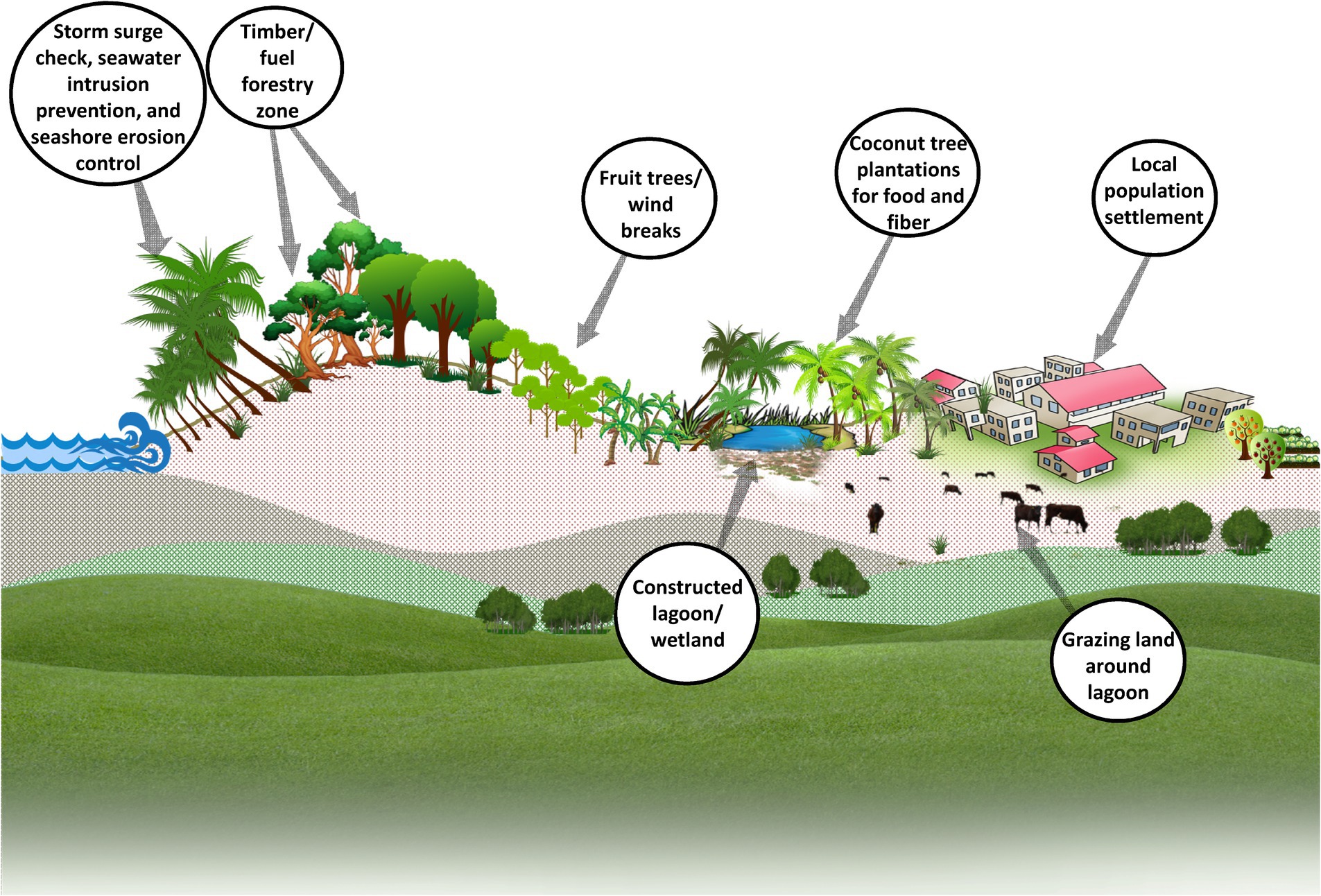
Figure 9. Schematic of a vegetation bioshield in the coastal region for storm protection (Adapted from: Tanaka, 2009).
The resistance to withstand the tsunami varies with the types of tree species and characteristics of the vegetation (Figure 10). For instance, during the 1998 Papua New Guinea tsunami disaster, Casuarina trees provided relatively higher resistance compared to palm trees (Dengler and Preuss, 2003). In contrast, the older belts of Casuarina equisetifolia trees were found ineffective to provide good quality protection in Sri Lanka and Thailand during the tsunami (Tanaka et al., 2006a,b, 2007). Casuarina shelterbelt also provided social and ecological impacts in a better way than the potential economic benefits (De Zoysa, 2008). The effect of the location of tree cover or vegetation indicated that man-made structures placed directly at the back of the most widespread mangroves were not much damaged (Dahdouh-Guebas et al., 2005), and the intense mangroves of Rhizophora spp. and Avicennia spp. reduced the damage by 96% in most cases (Danielsen et al., 2005). The directly exposed villages experienced maximum damage; those behind the mangroves had a medium level of damage, though the distance between the shoreline and the settlement is also a major factor determining the fatalities and damage. The vegetation in front of the settlement caused around 8% decline in casualties (Bayas et al., 2011). The thick vegetation at the back of settlements resulted in unfavorable effects, increasing structural damage and casualties. Thus, for future coastal planning, productive agroforestry belts should be developed in front of coastal communities, whereas cropping area must be allocated to the back of the villages. The relationship between the crown height and tsunami height is also important in terms of the drag characteristics of broad-leaved trees because they have large diameters sized branches (Tanaka et al., 2007). Moreover, active forest management is necessary to produce all-aged stands with different sizes as well as branches at all levels to improve the mitigation potential, mainly in smaller tsunami events (FAO, 2007). For example, the two layers of vegetation with P. odoratissimus and C. equisetifolia in a vertical direction demonstrated a strong ability to reduce the damage at the back of the vegetation cover (Tanaka et al., 2007). The coastal grasses (Spinifex littoreu and Ipomoea pes-caprae) in front of the coastal forest also played an important role in decreasing dune erosion during the low-level tsunami (Sasaki et al., 2007). The integration of diverse tree species has also been advocated along the coastal areas (Tanaka et al., 2007, 2008b). These coastal vegetation captures debris to help humans go up to escape as well as provide a soft landing place during tsunami events (Tanaka et al., 2006b, 2007).
Several studies on the tsunami that ravaged India in 2004 indicated the effectiveness of vegetation along the coastline in saving human lives and infrastructure (Dahdouh-Guebas et al., 2005; Danielsen et al., 2005; Harada and Kawata, 2005; Tanaka et al., 2006a,b, 2007; Nandasena et al., 2007; Sasaki et al., 2007; Tanaka and Sasaki, 2007). In Sri Lanka, the home gardens exhibited greater resilience and protection from the 2004 tsunami, and the houses in their vicinity of home gardens received less damage (Mattsson et al., 2009). The farmers of Central American hillsides have been using diversified practices like intercropping, cover crops, and agroforestry models. They reported less damage in diversified practices compared to their conventional monoculture system during Hurricane Mitch. It was observed that the diversified farms had 20 to 40% more topsoil due to less erosion and better soil moisture and experienced lesser economic losses than the monoculture farms (Holt-Giménez, 2002).
7 Cyclone
A cyclone is a large system of winds that circulates around a center of low atmospheric pressure in a counter clockwise direction. Researchers have predicted that high temperature-induced warming in oceans is expected to intensify storm events, and it is believed that there will be a higher proportion of the most powerful and destructive storms (IPCC, 2021). Tree species play an important role in reducing the severity of storms and other similar events. For instance, during or after typhoons, the top priority could be to use trees for consuming or selling fruits, fuelwood, charcoal, or timber to augment income loss from the damaged crops, besides providing fodder for the livestock (Udmale et al., 2014). Windbreaks can lower cyclone-type high wind speeds across the land surface through a number of physical mechanisms, which in turn reduce the mobilization and movement of dust and dust-related particles (Heisler and Dewalle, 1988; Tibke, 1988). Large agricultural landscapes frequently need multiple barriers to effectively control wind erosion because the zone of reduced wind speed typically extends 10 to 20 times the tree heights downwind of a windbreak (Tibke, 1988). Windbreak also provides additional benefits, such as reduced greenhouse emissions and adaptation to harsh weather (FAO, 2013). For instance, planting a windbreak to reduce the effect of cyclones also sequesters carbon, which in turn maintains the long-term health and productivity of the soil (Schoeneberger et al., 2012). Additionally, compared to monoculture farms, agroecologically managed farms have the potential for a faster productive recovery (80–90% within 40 days following the hurricane) (Rosset et al., 2011). For example, in Sotonusco, Chiapas, Philpott et al. (2008) reported less damage to more diverse coffee systems during hurricane events compared to less diverse coffee systems. Similarly, in Cuba, diversified farms showed 50% lower losses as opposed to 90–100% extensive losses in monocultures. In order to lessen the perceived risk brought on by the increasing floods and landslide incidents, many farmers grow trees and refrain from cutting them. Farmers may be able to reduce their exposure to major storm events by carefully maintaining their crops. Although unfavorable geographic elements of farms may be beyond farmers’ control, the increasing complexity and diversity of the flora within farms could be a successful tactic to lessen some sensitivity to hurricane disturbances (Philpott et al., 2008).
8 Future implications, challenges, and research needs
Across the globe, the increasing frequency and duration of extreme weather events like extreme seasonal temperatures, droughts, and high rainfall events, as well as extreme storms (cyclones and tsunamis), are increasingly posing major threats to agriculture and livestock in general and to humans in particular. The planning and practice of agroforestry have, time and again, demonstrated enormous potential in curtailing extreme weather-related damages to a great extent. Agri-silviculture for crop-tree production and silvi-pasture for pasture production have proven highly effective in countering the effects of extreme temperatures by maintaining crop yield. Under high rainfall conditions, multi-tiered/homestead agroforestry for generating multiple outputs, aqua-forestry for fish-tree production, and alley cropping for erosion control have been found highly effective and may be adopted in regions experiencing extreme rain events. In drought-prone areas, despite the success of large number of tree based farming systems, silvipasture for fodder production showed exceptional performance in terms of maintaining and increasing the livestock milk production. For large-scale catastrophic disturbances such as tsunamis and cyclones, the planning and designing of shelterbelts and windbreak-based bio-shields can largely reduce the damages as anticipated by the predicted climate change. Assessment based on current literature led to the conceptualization of various potential agroforestry systems suitable for obtaining greater economic and ecological benefits under different extreme weather events, as illustrated in Figure 11. However, the extent of climate change mitigation via agroforestry varies with the type of species and the intensification level of each tree component in the agriculture system. Specifically, the findings of the literature available until date explained that the climate resilience is low in a cropland, moderate in a boundary plantation, high in a strip plantation, and very high in a mixed species block plantation (Figure 12). Until now, little to moderate data on field-based evidence is available for countering extreme weather events through agroforestry, therefore, emphasis needs to be given to document precise information for designing the best possible practices to sustain agriculture and animal productivity in the climate change era.
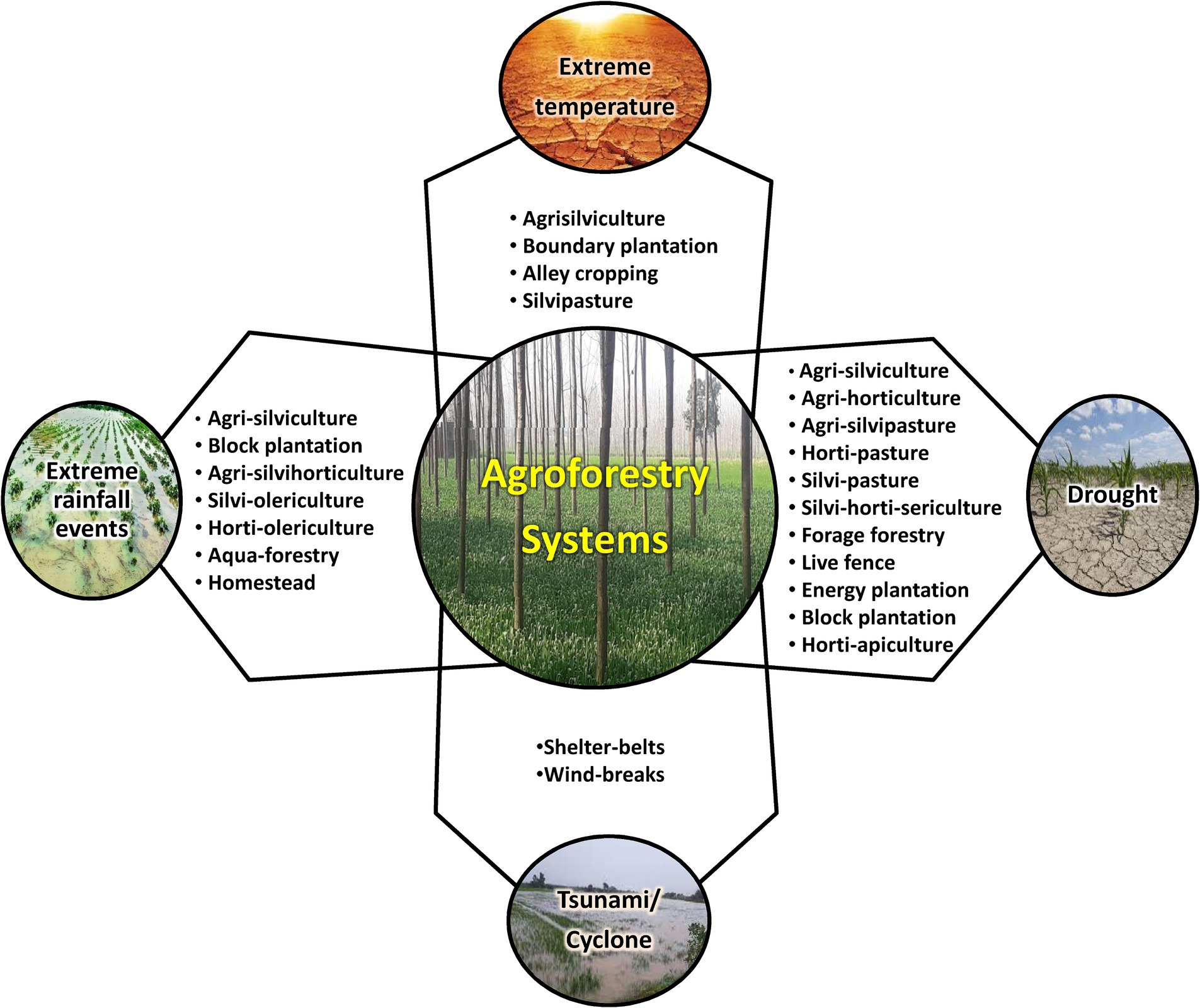
Figure 11. Conceptual diagram depicting suitability of various agroforestry systems for different extreme weather events.
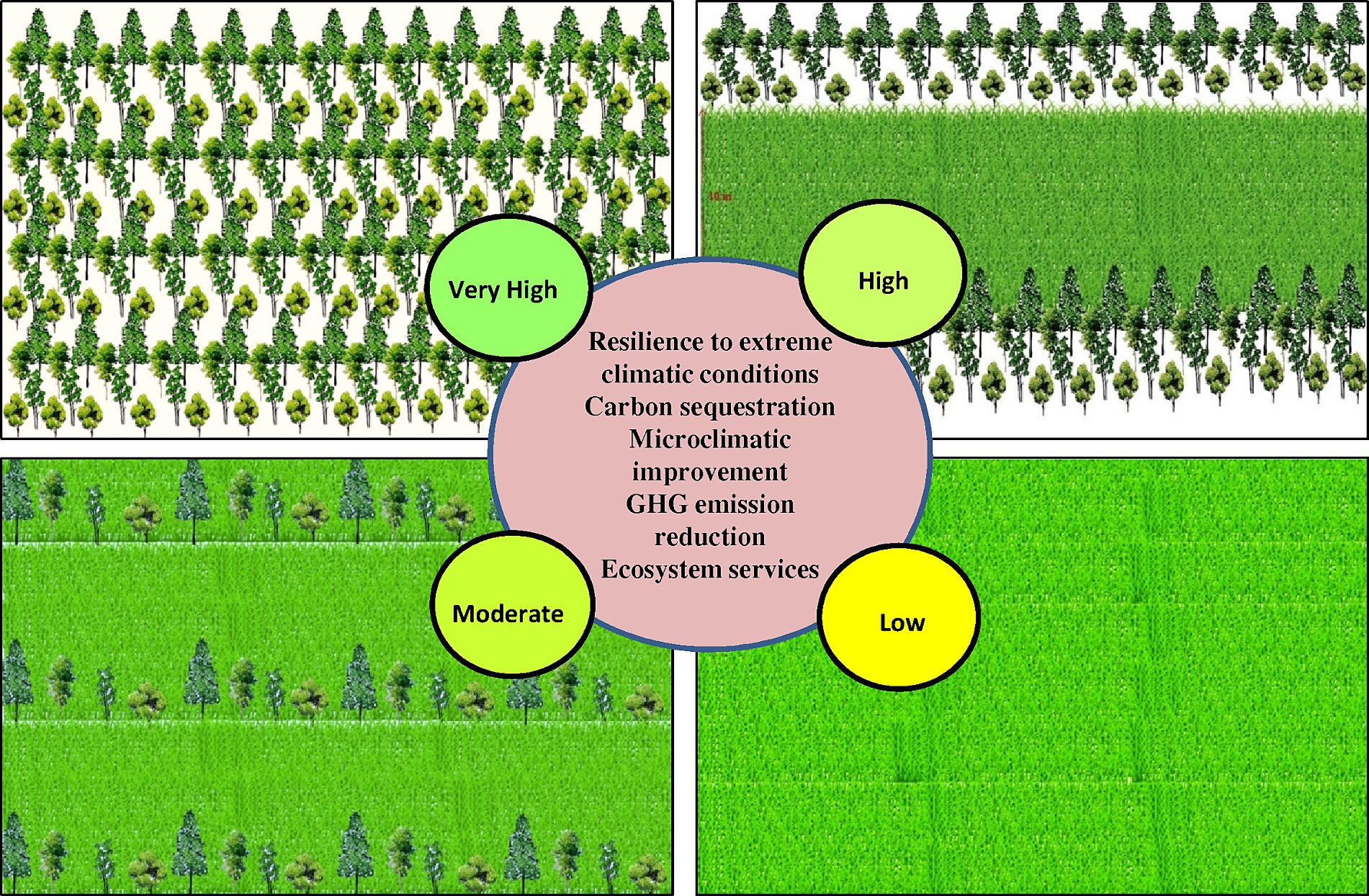
Figure 12. Conceptual framework depicting process of enhancing climate resilience of the agro-ecosystem.
Despite the good adaptation response of agroforestry to extreme weather events, several constraints and challenges at the national and global level have plagued the expansion of agroforestry in new areas. Some of the challenges that were sought were: lack of quality plating material, poor knowledge of local people on agroforestry benefits, poor extension strategies, existence of multiple abiotic stresses, lack of policy and program on promotion of agroforestry, limited best agroforestry practices and models, lack of high-value agroforestry models, and non-availability of data on the relative comparison of resilient agroforestry systems. In order to shift from a crop-based production system to an agroforestry-based farming system, several research, technological, extension, and policy interventions are needed to be addressed immediately and on priority. The most common agroforestry practices in world, include, improved fallow, multifunctional trees on farms and rangelands, alley cropping, home gardens, silvopastoral systems, windbreaks, shelterbelts, taungya farming, and shaded perennial-crop systems. However, the most successful agroforestry practice is the combination of poplar (Populus deltiodes) and wheat, which have successfully spread over a thousand hectares in different states in Northern India. Moreover, for successful promotion and upscaling of these types of agroforestry across different region of the globe, various interventions, such as the development of farmer-friendly agroforestry practices, the multiplication and upscaling of superior germplasm, the dissemination of knowledge on ecosystem services, the establishment of wood-based industries, the easy access to wood-based markets, the development of agroforestry based business models, the creation of insurance, credit and subsidy scheme for tree planting, and imparting training and capacity-building to the stakeholders, etc. could be very helpful in transforming crop based production systems to agroforestry based production systems.
Climate change, especially extreme weather events, is causing huge damage to agricultural crops and allied sectors across the globe. The high temperature, abnormal rainfall, and prolonged drought conditions are creating adverse effects on the crops, and in most cases, the failure of agricultural crops has been reported. The devising relationships between extreme weather, crop yield reduction, and agroforestry benefits could provide precise information about crop selection and the design of a mitigation strategy under such conditions. The prolonged exposure of some sensitive tree species to extreme temperatures, rainfall, and drought could adversely affect their growth and biomass production; hence, tree species should be identified those can withstand such harsh conditions. Arid and semi-arid regions are worst affected by extreme weather events, and soil water limitations are the main hindrance to the adoption of commercial agroforestry. Under such conditions, metabolite yielding tree species should be identified, as extreme environments might instigate higher metabolite production in the trees. Further, the complexity of the existence of multiple traits (timber, fuelwood, fodder, and fruit) in tree species makes it difficult to decide which type of trait will be more suitable under a particular type of extreme climate. The information on the level of spatial and temporal intensification of trees in agricultural lands also needs to be redesigned to combat and reduce the adverse effects of extreme weather. To consolidate the claims in the current knowledge further focus need to be exerted on issues that would strengthen adoption of agroforestry as a climate adoption practice.
• Although extreme weather events occur in every part of the world but their documentation is very poor with reference to the analyzing agroforestry response.
• Better analysis of the interaction between the various climate change and mitigation aspects like extreme weather, carbon sequestration, greenhouse gas emission and microclimate moderation, and regional climate amelioration with agroforestry.
• Developing programs for increasing understanding of climate issues and learning from the field experience of farmers on agroforestry designs and their potential in fulfilling adaptation and mitigation objectives, etc., should be prioritized.
• Agroforestry-based climate resilience should also meet productivity, profitability, and sustainability objectives at the farm level.
• Identification of agroforestry tree species for different agroecological and farming systems that meet both production and ecological objectives in general and for the domestication and promotion of tree species suitable for agroforestry in climate-vulnerable regions should be focused on.
• Extreme weather events can also have adverse effects on tree components; therefore, identifying climate-resilient species and their germplasm is important for climate change adaptation.
• The formulation and promotion of appropriate policies, programs, action plans, and institutional infrastructure for greater adoption of agroforestry are urgent needs.
9 Conclusion
The current literature suggests that maintenance and promotion of diverse agroforestry practices at the farm level, with high resilience and multiple benefits, can considerably contribute to the mitigation and adaptation to climate change. Under present conditions, agri-silviculture, home garden, and silvi-pasture are the best systems to cope with the extreme temperature, rainfall, and drought conditions, respectively. Despite the low evidence, the rest of the agroforestry systems can equally contribute to increase farm resilience to extreme weather events. A more complex ‘integrated farming system’ practice, which is an extension of tropical home gardens, is invariably receiving considerable attention recently among policymakers throughout the world. Though agroforestry practices are adopted by only a small percentage of farmers worldwide, the substantial economic benefits and mitigation-adaptation provided by trees during extreme weather events should be promoted to gather their interest in shifting from monoculture to an agroforestry-based polyculture farming system. Research needs to be focused on the type of tree species to best fulfill the area-specific needs, and the agroforestry package of practices best suited to attain the multiple objectives—climate mitigation, adaptation, productivity, and farm profitability—should be prioritized. A suitable policy framework for localized as well as regional-level adoption of agroforestry to meet the anticipated impacts under the predicted climate change scenarios needs to be urgently developed and implemented with medium- to long-term goals in sight.
Author contributions
SD: Writing – original draft. RK: Writing – review & editing, Formal analysis, Conceptualization, Writing – original draft. AB: Writing – review & editing, Visualization. SC: Writing – original draft. AU: Writing – original draft. MK: Writing – review & editing, Methodology. AS: Writing – review & editing, Validation. DJ: Writing – review & editing. PR: Writing – review & editing. AH: Writing – original draft, Conceptualization. NR: Writing – review & editing, Supervision.
Funding
The author(s) declare financial support was received for the research, authorship, and/or publication of this article. This work was supported by the Indian Council of Agricultural Research (ICAR), Central Soil Salinity Research Institute (CSSRI), and NICRA (National Innovations in Climate Resilient Agriculture) project through grant no. ICAR-DARE-NICRA-03.
Conflict of interest
The authors declare that the research was conducted in the absence of any commercial or financial relationships that could be construed as a potential conflict of interest.
Publisher’s note
All claims expressed in this article are solely those of the authors and do not necessarily represent those of their affiliated organizations, or those of the publisher, the editors and the reviewers. Any product that may be evaluated in this article, or claim that may be made by its manufacturer, is not guaranteed or endorsed by the publisher.
References
Abdella, M., Nigatu, L., and Akuma, A. (2020). Impact of parkland trees (Faidherbia albida Delile and Cordia africana Lam) on selected soil properties and sorghum yield in Eastern Oromia, Ethiopia. Agriculture, Forestry and Fisheries 9, 54–66. doi: 10.11648/j.aff.20200903.13
Al-Kaisi, M. M., Elmore, R. W., Guzman, J. G., Hanna, H. M., Hart, C. E., Helmers, M. J., et al. (2013). Drought impact on crop production and the soil environment: experiences from Iowa. J. Soil Water Conserv. 68, 19A–24A. doi: 10.2489/jswc.68.1.19A
Altieri, M. A., Nicholls, C. I., Henao, A., and Lana, M. A. (2015). Agroecology and the design of climate change-resilient farming systems. Agron. Sustain. Dev. 35, 869–890. doi: 10.1007/s13593-015-0285-2
Asseng, S., Ewert, F., Martre, P., Rötter, R. P., Lobell, D. B., Cammarano, D., et al. (2015). Rising temperatures reduce global wheat production. Nat. Clim. Chang. 5, 143–147. doi: 10.1038/nclimate2470
Bantilan, M. C. S., and Mohan, G. (2014). Adaptation to climate change in agriculture in selected Asian countries policy brief 25. Hyderabad: ICRISAT.
Basche, A. D., and DeLonge, M. S. (2019). Comparing infiltration rates in soils managed with conventional and alternative farming methods: a meta-analysis. PLoS One 14:e0215702. doi: 10.1371/journal.pone.0215702
Bayas, J. C. L., Marohn, C., Dercon, G., Dewi, S., Piepho, H. P., Joshi, L., et al. (2011). Influence of coastal vegetation on the 2004 tsunami wave impact in West Aceh. Proc. Natl. Acad. Sci. 108, 18612–18617. doi: 10.1073/pnas.1013516108
Bentrup, G., and Dosskey, M. G. (2017). “Appendix B. evaluation of agroforestry for protecting Coldwater fish habitat” in Agroforestry: Enhancing resiliency in U.S. agricultural landscapes under changing conditions. Gen. Tech. Report WO-96. eds. M. M. Schoeneberger, G. Bentrup, and T. Patel-Weynand (Washington, DC: U.S. Department of Agriculture, Forest Service), 207–209.
Birhane, E., Teklay, R., Gebrehiwet, K., and Solomon, N. (2018). Maintaining Acacia polyacantha trees in farmlands enhances soil fertility and income of farmers in North Western. Agroforestry Systems, 1. doi: 10.1007/s10457-018-0328-1
Blanc, E. (2012). The impact of climate change on crop yields in sub-Saharan Africa. Am. J. Clim. Chang. 1, 1–13. doi: 10.4236/ajcc.2012.11001
Blanchet, G., Barkaoui, K., Bradley, M., Dupraz, C., and Gosme, M. (2021). Interactions between drought and shade on the productivity of winter pea grown in a 25-year-old walnut-based alley cropping system. J. Agron. Crop Sci. 1–16. doi: 10.1111/jac.12488
Bosi, C., José, R. M., Pezzopane, P., and Sentelhas, P. C. (2020). Silvopastoral system with Eucalyptus as a strategy for mitigating the effects of climate change on Brazilian pasturelands. Ann. Braz. Acad. Sci. 92:e20180425. doi: 10.1590/0001-3765202020180425
Bowler, D. E., Mant, R., Orr, H., Hannah, D. M., and Pullin, A. S. (2012). What are the effects of wooded riparian zones on stream temperature? Environ. Evid. 1, 3–9. doi: 10.1186/2047-2382-1-3
Brandle, J. R., Hodges, L., Tyndall, J., and Sudmeyer, R. A. (2009). “Windbreak practices” in North American agroforestry: An integrated science and practice. ed. H. E. Garrett. 2nd ed (Madison, WI: American Society of Agronomy), 75–104.
Brandle, J. R., Johnson, B. B., and Dearmont, D. D. (1984). Windbreak economics: the case for wheat production in eastern Nebraska. J. Soil Water Conserv. 39, 339–343.
Burgess, A. J., Maria, E. C. C., and Ben, P. (2022). The deployment of intercropping and agroforestry as adaptation to climate change. Crop and Environment 1, 145–60. doi: 10.1016/j.crope.2022.05.001
Calmon, M., and Feltran-Barbieri, R. Four ways farmers can adapt to climate change and generate income. Commentary, world resource institute, United States office 10 G street, NE suite 800, Washington, DC 20002 United States (2019).
Campanha, M. M., Santos, R. H. S., De Freitas, G. B., Martinez, H. E. P., Garcia, S. L. R., and Finger, F. L. (2004). Growth and yield of coffee plants in agroforestry and monoculture systems in Minas Gerais, Brazil. Agrofor. Syst. 63, 75–82. doi: 10.1023/B:AGFO.0000049435.22512.2d
Cassamo, C. T., Draper, D., Romeiras, M. M., Marques, I., Chiulele, R., Rodrigues, M., et al. (2023). Impact of climate changes in the suitable areas for Coffea arabica L. production in Mozambique: agroforestry as an alternative management system to strengthen crop sustainability. Agric. Ecosyst. Environ. 346:108341. doi: 10.1016/j.agee.2022.108341
Chadha, R. K., Latha, G., Yeh, H., Peterson, C., and Katada, T. (2005). The tsunami of the great Sumatra earthquake of M 9.0 on 26 December 2004–impact on the east coast of India. Curr. Sci. 88, 1297–1301.
Challinor, A. J., Koehler, A.-K., Ramirez-Villegas, J., Whitfield, S., and Das, B. (2016). Current warming will reduce yields unless maize breeding and seed systems adapt immediately. Nat. Clim. Chang. 6, 954–958. doi: 10.1038/nclimate3061
Chamshama, S. A. O., Mugasha, A. G., Klovstad, A., Haveraaen, O., and Maliondo, S. M. S. (1998). Growth and yield of maize alley cropped with Leucaena leucocephala and Faidherbia albida in Morogoro Tanzanian. Agrofor. Syst. 40, 215–225.
Charles, R., Munishi, P., and Nzunda, E. (2013). Agroforestry as adaptation strategy under climate change in Mwanga District, Kilimanjaro, Tanzania. Int. J. Environ. Prot. 3, 29–38.
Chauhan, V. K., and Dhiman, R. C. (2007). Atmospheric humidity and air temperature studies in wheat-poplar based agroforestry system. Indian Forester 133, 73–78.
Chavan, S. B., Uthappa, A. R., Sridhar, K. B., Keerthika, A., Handa, A. K., Newaj, R., et al. (2016). Trees for life: creating sustainable livelihood in Bundelkhand region of Central India. Curr. Sci. 1, 994–1002. doi: 10.18520/cs/v111/i6/994-1002
Chibinga, O. C., Musimba, N. R. K., Nyangito, M. M., Simbaya, J., and Daura, M. T. (2012). Climate variability; enhancing adaptive utilization of browse trees for improved livestock production among agropastoralists communities in southern Zambia. Afr. J. Environ. Sci. Technol. 6, 267–274.
Cordeiro, N. J., Borghesio, L., Joho, M. P., Monoski, T. J., Mkongewa, V. J., and Damp, C. J. (2015). Forest fragmentation in an African biodiversity hotspot impacts mixed-species bird flocks. Biol. Conserv. 188, 61–71. doi: 10.1016/j.biocon.2014.09.050
Cross, B. K., Bozek, M. A., and Mitro, M. G. (2013). Influences of riparian vegetation on trout stream temperatures in Central Wisconsin. N. Am. J. Fish Manag. 33, 682–692. doi: 10.1080/02755947.2013.785989
Dahdouh-Guebas, F., Jayatissa, L. P., Di Nitto, D., Bosire, J. O., Lo Seen, D., and Koedam, N. (2005). How effective were mangroves as a defence against the recent tsunami? Curr. Biol. 15, R443–R447. doi: 10.1016/j.cub.2005.06.008
Danielsen, F., Sørensen, M. K., Olwig, M. F., Selvam, V., Parish, F., Burgess, N. D., et al. (2005). The Asian tsunami: a protective role for coastal vegetation. Science 310:643. doi: 10.1126/science.1118387
De Zoysa, M. (2008). Casuarina coastal forest shelterbelts in Hambantota City, Sri Lanka: assessment of impacts. Small Scale For. 7, 17–27. doi: 10.1007/s11842-008-9038-2
Dengler, L., and Preuss, J. (2003). Mitigation lessons from the July 17, 1998 Papua New Guinea tsunami. Pure Appl. Geophys. 160, 2001–2031. doi: 10.1007/s00024-003-2417-x
Deniz, M., Schmitt, F. A. L., Farley, J., de Quadros, S. F., and Hötzel, M. J. (2019). High biodiversity silvopastoral system as an alternative to improve the thermal environment in the dairy farms. Int. J. Biometeorol. 63, 83–92. doi: 10.1007/s00484-018-1638-8
Deswal, R. P. S., Jat, M. K., Kaushik, N. K., Singh, R., Yadav, P. K., and Satyajeet, S. (2022). Effect of density of Mahaneem (Ailanthus excelsa) plantation on biomass production, nutrient cycling, and yield of field crops. In: Mandal, S., Mukhopadhyay, R., Fagodiya, R. K., Singh, A., Thakur, R., and Kumar, A.. Salinity management for land degradation neutrality and livelihood security under changing climate, abstracts, (eds.) 6th National Conference of Indian Society of Soil Salinity and Water Quality, Karnal - 132001, Haryana, India, held at Tiruchirapalli during 11–13 October 2022, Tamil Nadu, pp. 25.
Dhillon, W. S., Srinidhi, H. V., Chauhan, S. K., Singh, C., Singh, N., and Jabeen, N. (2010). Micro-environment and physiology of turmeric cultivated under poplar tree canopy. Ind. J. Agrof. 12, 23–37.
Dobhal, S., Kumar, R., Chauhan, K., and Rawat, P. (2020). Soil and water conservation measures for sustainable agro ecosystem development of Uttrakhand. Van Sangyan 7, 1–4.
Dosskey, M. G., Brandle, J., and Bentrup, G. (2017). “Reducing threats and enhancing resiliency” in Agroforestry: Enhancing resiliency in U.S. agricultural landscapes under changing conditions. Gen. Tech. Report WO-96. Washington, DC: U.S. eds. M. M. Schoeneberger, G. Bentrup, and T. Patel-Weynand (Forest Service: Department of Agriculture), 7–42.
Eitzinger, J., Utset, A., Trnka, M., Zalud, Z., Nikolaev, M., and Uskov, I. (2017). “Weather and climate and optimization of farm technologies at different input levels” in Managing weather and climate risks in agriculture. eds. M. Sivakumar and R. Motha (Berlin: Springer), 141–170.
Eychène, C., Fallot, A., Meija, N., Rapidel, B., Le Coq, J. F., and Botta, A. (2014). Assessing the resilience of agroforestry systems in Central America: what do we learn about the transformability of coffee plantations? 3ème congrès international Resilience, Resilience and development: mobilising for transformation, Resilience Alliance, Montpellier, 4–8 mai 2014, Montpellier, France, pp. 60–61.
FAO (2007). The role of coastal forests in the mitigation of tsunami impacts–main report. Bangkok: Thammada Press Available at: http://www.fao.org/forestry/site/coastalprotection/en.
FAO (2013). Climate-smart agriculture–sourcebook. Rome: Food and agriculture Organization of the United Nations.
FAO. (2015). Climate change and food security: Risks and responses. Food and agricultural Organization of the United Nations, Rome, Italy. Available at: https://www.fao.org/3/i5188e/I5188E.pdf.
Gomes, L. C., Bianchi, F. J. J. A., Cardoso, I. M., Fernande, R. B. A., Fernandes Filho, E. I., and Schulte, R. P. O. (2020). Agroforestry systems can mitigate the impacts of climate change on coffee production: a spatially explicit assessment in Brazil. Agric. Ecosyst. Environ. 294:106858. doi: 10.1016/j.agee.2020.106858
Gopalakrishnan, T., Hasan, M. K., Haque, A. T. M. S., Jayasinghe, S. L., and Kumar, L. (2019). Sustainability of coastal agriculture under climate change. Sustain. For. 11:7200. doi: 10.3390/su11247200
Gosme, M., Dufour, L., Aguirre, H. D. I., and Dupraz, C. Microclimatic effect of agroforestry on diurnal temperature cycle. In: European agroforestry conference (EURAF 2016), p. 466.
Gowda, P., Steiner, J. L., Olson, C., Boggess, M., Farrigan, T., and Grusak, M. A. (2018). “Agriculture and rural communities” in Impacts, risks, and adaptation in the United States: Fourth National Climate Assessment. eds. D. R. Reidmiller, C. W. Avery, D. R. Easterling, K. E. Kunkel, K. L. M. Lewis, and T. K. Maycock, vol. 2 (Washington, DC: US Global Change Research Program), 391–437.
Gregory, P. J., and Ingram, J. S. I. (2000). Global change and food and forest production: future scientific challenges. Agric. Ecosyst. Environ. 82, 3–14. doi: 10.1016/S0167-8809(00)00212-7
Haile, G., Mulugeta, L., Fisseha, I., and Getachew, A. (2021). Comparative study on the effects of Acacia albida on yield and yield components of different cereal crops in Southern Ethiopia. Acta agric. Scand., B Soil plant sci. 71, 453–65. doi: 10.1080/09064710.2021.1918234
Hairiah, K., Widianto, W., Suprayogo, D., and Van Noordwijk, M. (2020). Tree roots anchoring and binding soil: reducing landslide risk in Indonesian agroforestry. Land 9:256. doi: 10.3390/land9080256
Harada, K., and Kawata, Y. (2005). Study on tsunami reduction effect of coastal forest due to forest growth, annuals of disaster prevention research institute. Kyoto Univ 48C, 161–165.
Hatfield, J. L., Boote, K. J., Kimball, B. A., Ziska, L. H., Izaurralde, R. C., Ort, D., et al. (2011). Climate impacts on agriculture: implications for crop production. Agron. J. 103, 351–370. doi: 10.2134/agronj2010.0303
Hawke, M. F., and Wedderburn, M. E. (1994). Microclimate changes under Pinus radiata agroforestry regimes in New Zealand. Agric. For. Meteorol. 71, 133–145. doi: 10.1016/0168-1923(94)90104-X
Heisler, G. M., and Dewalle, D. R. (1988). Effects of windbreak structure on wind flow. Agric. Ecosyst. Environ. 22-23, 41–69. doi: 10.1016/0167-8809(88)90007-2
Hernández-Morcillo, M., Burgess, P., Mirck, J., Pantera, A., and Plieninger, T. (2018). Scanning agroforestry-based solutions for climate change mitigation and adaptation in Europe. Environ Sci Policy 80, 44–52. doi: 10.1016/j.envsci.2017.11.013
Holt-Giménez, E. (2002). Measuring farmers’ agroecological resistance after Hurricane Mitch in Nicaragua: a case study in participatory, sustainable land management impact monitoring. Agric. Ecosyst. Environ. 93, 87–105. doi: 10.1016/S0167-8809(02)00006-3
Honda, S. (1898). Bulletin of the College of Agriculture 3(4). Tokyo: Imperial University, Komaba, 281–298.
Hulme, M., Doherty, R., Ngara, T., and New, M. (2005). “Global warming and African climate change” in Climate change and Africa. ed. P. S. Low (Cambridge: Cambridge University Press), 29–40.
Iizumi, T., and Navin, R. (2015). How do weather and climate influence cropping area and intensity? Glob. Food Sec. 1, 46–50. doi: 10.1016/j.gfs.2014.11.003
IPCC. (2021). Climate change 2021: the physical science basis. Contribution of Working Group I to the Sixth Assessment Report of the Intergovernmental Panel on Climate Change. Available at: https://www.ipcc.ch/report/ar6/wg1/.
Jost, F., and Pretzsch, J. (2012). Influence of agroforestry systems in risk reduction and climate change adaptation in the Peruvian Andes. Presented at Tropentag 2012: Resilience of agricultural systems against crises. September 19–21, Göttingen, Germany.
Kanzler, M., Böhm, C., Mirck, J., Schmitt, D., and Veste, M. (2019). Microclimate effects on evaporation and winter wheat (Triticum aestivum L.) yield within a temperate agroforestry system. Agrofor. Syst. 93, 1821–1841. doi: 10.1007/s10457-018-0289-4
Karki, U., and Goodman, M. S. (2010). Landscape use by cattle in silvopasture versus open pasture. Agrofor. Syst. 78, 159–168. doi: 10.1007/s10457-009-9250-x
Karki, U., and Goodman, M. S. (2015). Microclimatic differences between mature loblolly-pine silvopasture and open-pasture. Agrofor. Syst. 89, 319–325. doi: 10.1007/s10457-014-9768-4
Kathiresan, K., and Rajendran, N. (2005). Coastal mangrove forests mitigated tsunami. Estuar. Coast. Shelf Sci. 65, 601–606. doi: 10.1016/j.ecss.2005.06.022
Keerthika, A., Chavan, S. B., and Monika, S. (2015). Khejri agroforestry for addressing issues of soil health. Life Sci. Leaf. 64, 102–108.
Kohli, A., and Saini, B. (2003). Microclimate modification and response of wheat planted under trees in a fan design in northern India. Agrofor. Syst. 58, 109–117. doi: 10.1023/A:1026090918747
Kort, J. (1988). Benefits of windbreaks to field and forage crops. Agric. Ecosyst. Environ. 22-23, 165–190. doi: 10.1016/0167-8809(88)90017-5
Kumar, R., Banyal, R., Kumar, M., and Dobhal, S. (2020b). Agroforestry: A sustainable land use systems. New Delhi: Astral International Pvt. Ltd., 187.
Kumar, R., Bhatnagar, P. R., Kakade, V., and Dobhal, S. (2020a). Tree plantation and soil water conservation enhances climate resilience and carbon sequestration of agro ecosystem in semi-arid degraded ravine lands. Agric. For. Meteorol. 282-283:107857. doi: 10.1016/j.agrformet.2019.107857
Kumar, R., Mehta, H., Kumar, A., Bhardwaj, A. K., Kaushal, R., Dobhal, S., et al. (2021). Seed source variation affects the growth, biomass, carbon stock, and climate resilience potential: a case study of Celtis australis in Indian Himalayas. Glob. Ecol. Conserv. 26:e01469. doi: 10.1016/j.gecco.2021.e01469
Kumar, R., Pande, V. C., Bhardwaj, A. K., Dinesh, D., Bhatnagar, P. R., Dobhal, S., et al. (2022). Long-term impacts of afforestation on biomass production, carbon stock, and climate resilience in a degraded semi-arid ravine ecosystem of India. Ecol. Eng. 177:106559. doi: 10.1016/j.ecoleng.2022.106559
Kurian, N. P., Pillai, A. P., Rajith, K., Murali Krishnan, B. T., and Kalaiarasan, P. (2006). Inundation characteristics and geomorphological impacts of December 2004 tsunami on Kerala coast. Curr. Sci. 90, 240–249.
Latief, H., and Hadi, S. (2007). “The role of forests and trees in protecting coastal areas against tsunamis” in Regional technical workshop on coastal protection in the aftermath of the Indian Ocean tsunami what role for forests trees? eds. S. Braatz, S. Fortuna, and J. Broadhead (Rome: FAO), 5–32.
Lin, B. B. (2006). The role of agroforestry in water conservation: The effect of shade on evapotranspiration in coffee agroecosystems, mitigation of microclimate variation through agroforestry: Protecting coffee agriculture from the impacts of climate change. Ann Arbor, MI: University of Michigan.
Lin, B. B. (2007). Agroforestry management as an adaptive strategy against potential microclimate extremes in coffee agriculture. Agric. For. Meteorol. 144, 87–94.
Magalhaes, C. A. S., Zolin, C. A., Lulu, J., Lopes, L. B., Furtini, I. V., Vendrusculo, L. G., et al. (2020). Improvement of thermal comfort indices in agroforestry systems in the southern Brazilian Amazon. J. Therm. Biol. 91:102636. doi: 10.1016/j.jtherbio.2020.102636
Maracchi, G., Sirotenko, O., and Bindi, M. (2005). Impacts of present and future climate variability on agriculture and forestry in the temperate regions: Europe. Clim. Chang. 70, 117–135. doi: 10.1007/s10584-005-5939-7
Martius, C., Höfer, H., Garcia, M., Römbke, J., Förster, B., and Hanagarth, W. (2004). Microclimate in agroforestry systems in Central Amazonia: does canopy closure matter to soil organisms? Agrofor. Syst. 60, 291–304. doi: 10.1023/B:AGFO.0000024419.20709.6c
Mattsson, E., Ostwald, M., Nissanka, S. P., Holmer, B., and Palma, M. (2009). Recovery and protection of coastal ecosystems after tsunami event and potential for participatory forestry CDM—examples from Sri Lanka. Ocean Coast. Manag. 52, 1–9. doi: 10.1016/j.ocecoaman.2008.09.007
Mertia, R. S., Prasad, R., Gajja, B. L., Samara, J. S., and Narain, P. (2006). Impact of shelterbelt in arid region of western Rajasthan. Jaisalmer, Rajasthan: RRS, ICAR-CAZRI, 91.
Mitlöhner, F. M., Morrow, J. L., and Dailey, J. W. (2001). Shade and water misting effects on behavior, physiology, performance, and carcass traits of heat-stressed feedlot cattle. J. Anim. Sci. 79, 2327–2335. doi: 10.2527/2001.7992327x
Miyan, M. A. (2015). Droughts in Asian least developed countries: vulnerability and sustainability. Weather Clim. Extrem. 7, 8–23. doi: 10.1016/j.wace.2014.06.003
Mugwanya, M., Dawood, M. A., Kimera, F., and Sewilam, H. (2022). Anthropogenic temperature fluctuations and their effect on aquaculture: a comprehensive review. Aquac. Fish. 7, 223–243. doi: 10.1016/j.aaf.2021.12.005
Murgueitio, E., Calle, Z., Uribea, F., Calle, A., and Solorio, B. (2001). Native trees and shrubs for the productive rehabilitation of tropical cattle ranching lands. For. Ecol. Manag. 261, 1654–1166. doi: 10.1016/j.foreco.2010.09.027
Nair, P. K. R., Tonucci, R. G., Garcia, R., and Nair, V. D. (2011). “Silvopasture and carbon sequestration with special reference to the Brazilian savanna (Cerrado)” in Carbon sequestration potential of agroforestry systems. eds. B. M. Kumar and P. K. R. Nair (Netherlands: Springer), 145–162.
Nandasena, N. A. K., Tanaka, N., and Takagi, T. (2007). A new derivation of horizontal two dimensional depth averaged momentum equation and continuity equation which include total effect of porosity inside the vegetation. Proc. Hydraul. Eng. 51, 163–168. doi: 10.2208/prohe.51.163
Nguyen, Q., Hoang, M. H., Oborn, I., and Van Noordwijk, M. (2013). Multipurpose agroforestry as a climate change resiliency option for farmers: an example of local adaptation in Vietnam. Clim. Chang. 117, 241–257. doi: 10.1007/s10584-012-0550-1
Noordwijk, V. M., Hoang, M. H., Neufeldt, H., Oborn, I., and Yatich, T. (2011). How trees and people can co-adapt to climate change: Reducing vulnerability through multifunctional agroforestry landscapes. World Agroforestry Centre: Nairobi.
Olesen, J. E., Carter, T. R., Díaz-Ambrona, C. H., Fronzek, S., Heidmann, T., Hickler, T., et al. (2007). Uncertainties in projected impacts of climate change on European agriculture and terrestrial ecosystems based on scenarios from regional climate models. Clim. Chang. 81, 123–143. doi: 10.1007/s10584-006-9216-1
Pezzopane, J. R. M., Bosi, C., Nicodemo, M. L. F., Santos, P. M., Cruz, P. G., and Parmejiani, R. S. (2015). Microclimate and soil moisture in a silvopastoral system in southeastern Brazil. Bragantia 74, 110–119. doi: 10.1590/1678-4499.0334
Pezzopane, J. R. M., Nicodemo, M. L. F., Bosi, C., Garcia, A. R., and Lulu, J. (2019). Animal thermal comfort indexes in silvopastoral systems with different tree arrangements. J. Therm. Biol. 79, 103–111. doi: 10.1016/j.jtherbio.2018.12.015
Philpott, S. M., Lin, B. B., Jha, S., and Brines, S. J. (2008). A multi-scale assessment of hurricane impacts on agricultural landscapes based on land use and topographic features. Agric. Ecosyst. Environ. 128, 12–20. doi: 10.1016/j.agee.2008.04.016
Porter, J. R., and Semenov, M. A. (2005). Crop responses to climatic variation. Phil. Trans. R. Soc. 2005, B3602021–B3602035. doi: 10.1098/rstb.2005.1752
ProAct. (2008). The role of environmental management and eco-engineering in disaster risk reduction and climate change adaptation. ProAct Network, Nyon. Available at: http://www.proactnetwork.org/proactwebsite/policyresearchtoolsguidance/environmental.management-in-drr-a-cca.
Puri, S., and Bangarwa, K. S. (1992). Effects of trees on the yield of irrigated wheat crop in semi-arid regions. Agrofor. Syst. 20, 229–241. doi: 10.1007/BF00053141
Quandt, A. H., Neufeldt, H., and McCabe, J. T. (2017). The role of agroforestry in building livelihood resilience to floods and drought in semiarid Kenya. Ecol. Soc. 22:10. doi: 10.5751/ES-09461-220310
Rana, R. S., Bhagat, R. M., and Kali, V. (2011). Impact of climate change on apple crop in Himachal Pradesh. J. Agrometeorol. 13, 97–103. doi: 10.54386/jam.v13i2.1349
Rivest, D., Lorente, M., Olivier, A., and Messier, C. (2013). Soil biochemical properties and microbial resilience in agroforestry systems: effects on wheat growth under controlled drought and flooding conditions. Sci. Total Environ. 463-464, 51–60. doi: 10.1016/j.scitotenv.2013.05.071
Rosset, P. M., Sosa, B. M., Jaime, A. M. R., and Lozano, D. R. A. (2011). The campesino-to-campesino agroecology movement of ANAP in Cuba: social process methodology in the construction of sustainable peasant agriculture and food sovereignty. J. Peasant Stud. 38, 161–191. doi: 10.1080/03066150.2010.538584
Sasaki, Y., Homchuen, S., and Tanaka, N. (2007). The role of coastal vegetation in case of the Indian Ocean tsunami—coastal area of Thailand and Sri Lanka. Mangrove Sci. 4–5, 49–55.
Schoeneberger, M., Bentrup, G., de Gooijer, H., Soolanayakanahally, R., Sauer, T., Brandle, J., et al. (2012). Branching out: agroforestry as a climate change mitigation and adaptation tool for agriculture. J. Soil Water Conserv. 67, 128A–136A. doi: 10.2489/jswc.67.5.128A
Shah, M. (2014). Forest, fish and fruit: an innovative agroforestry practice in coastal Bangladesh to reduce vulnerability due to climate change. The Guardian.
Sida, T. S., Baudron, F., Kim, H., and Giller, K. E. (2018). Climate-smart agroforestry: Faidherbia albida trees buffer wheat against climatic extremes in the central Rift Valley of Ethiopia. Agric. For. Meteorol. 248, 339–347. doi: 10.1016/j.agrformet.2017.10.013
Siles, P., Harmand, J. M., and Vaast, P. (2010). Effects of Inga densiflora on the microclimate of coffee (Coffea arabica L.) and overall biomass under optimal growing conditions in Costa Rica. Agrofor. Syst. 78, 269–286. doi: 10.1007/s10457-009-9241-y
Simelton, E., Dam, B. V., and Catacutan, D. (2015). Trees and agroforestry for coping with extreme weather events: experiences from northern and Central Viet Nam. Agrofor. Syst. 89, 1065–1082. doi: 10.1007/s10457-015-9835-5
Singh, A., Singh, P., and Gill, R. I. S. (2024). Agroforestry could be one of the viable options to deal with terminal heat stress in wheat causing yield loss in Indo-Gangetic Plains. Environ. Dev. Sustain. doi: 10.1007/s10668-024-04731-1
Smukler, S. M., Sánchez-Moreno, S., Fonte, S. J., Ferris, H., Klonsky, K., O’Geen, A. T., et al. (2010). Biodiversity and multiple ecosystem functions in an organic farmscape. Agric. Ecosyst. Environ. 139, 80–97. doi: 10.1016/j.agee.2010.07.004
Stein, S., and Okal, E. (2005). Speed and size of the Sumatra earthquake. Nature 434, 581–582. doi: 10.1038/434581a
Tanaka, N. (2009). Vegetation bioshields for tsunami mitigation: review of effectiveness, limitations, construction, and sustainable management. Landsc. Ecol. Eng. 5, 71–79. doi: 10.1007/s11355-008-0058-z
Tanaka, N., Nandasena, N. A. K., Jinadasa, K. B. S., Sasaki, Y., Tanimoto, K., and Mowjood, M. I. M. (2008b). Developing effective vegetation bioshield for tsunami protection. Civil Environ. Eng. Syst. 26, 163–180. doi: 10.1080/10286600802435850
Tanaka, N., and Sasaki, Y. (2007). Limitations of coastal vegetation in the 2004 Indian Ocean tsunami and 2006 Java tsunami, in: Proceedings of IAHR 32nd congress, Venice (CDROM).
Tanaka, N., Sasaki, Y., and Mowjood, M. I. M. (2006b). Effects of sand dune and vegetation in the coastal area of Sri Lanka at the Indian Ocean tsunami, Namsik Park et al (eds) advances in geosciences 6. Singapore: World Scientific Publishing, Co.
Tanaka, N., Sasaki, Y., Mowjood, M. I. M., and Jinadasa, K. B. S. N. (2007). Coastal vegetation structures and their functions in tsunami protection: experience of the recent Indian Ocean tsunami. Landsc. Ecol. Eng. 3, 33–45. doi: 10.1007/s11355-006-0013-9
Tanaka, N., Sasaki, Y., Mowjood, M. I. M., Jinadasa, K. B. S., and Takemura, T. (2006a). Effective coastal vegetation species and structures with landform, sand dune and lagoon, for tsunami protection at the Indian Ocean tsunami. 15th APD-IAHR congress, Chennai, pp. 1279–1285.
Thevs, N., Gombert, A. J., Strenge, E., Lleshi, R., Aliev, K., and Emileva, B. (2019). Tree wind breaks in Central Asia and their effects on agricultural water consumption. Land 8:167. doi: 10.3390/land8110167
Thorlakson, T. (2011). Reducing subsistence farmers’ vulnerability to climate change: The potential contributions of agroforestry in Western Kenya, occasional paper 16. Nairobi: World Agroforestry Centre.
Thorlakson, T., and Neufeldt, H. (2012). Reducing subsistence farmers’ vulnerability to climate change: evaluating the potential contributions of agroforestry in western Kenya. Agric. Food Secur. 1:15. doi: 10.1186/2048-7010-1-15
Tibke, G. (1988). Basic principles of wind erosion control. Agric. Ecosyst. Environ. 22–23, 103–122.
Torquebiau, E. (1992). Are tropical agroforestry homegardens sustainable. Agric. Ecosyst. Environ. 41, 189–207. doi: 10.1016/0167-8809(92)90109-O
Tuck, G., Glendining, M. J., Smith, P., House, J. I., and Wattenbach, M. (2006). The potential distribution of bioenergy crops in Europe under present and future climate. Biomass Bioenergy 30, 183–197. doi: 10.1016/j.biombioe.2005.11.019
Udmale, P. D., Ichikawa, Y., Manandhar, S., Ishidaira, H., and Kiem, A. S. (2014). Farmers׳ perception of drought impacts, local adaptation and administrative mitigation measures in Maharashtra state, India. Int. J. Disaster Risk Reduct. 10, 250–269. doi: 10.1016/j.ijdrr.2014.09.011
University of the Philippines Los Banos Pulhin, J., Peras, R. J., Pulhin, F., and Gevaña, D. (2016). Farmers’ adaptation to climate variability: assessment of effectiveness and barriers based on local experience in southern Philippines. J. Environ. Sci. Manag. 1, 1–14. doi: 10.47125/jesam/2016_sp1/01
USDA (2016). How can agroforestry help landowners adapt to increased rain intensity? Working trees, USDA National Agroforestry Center. Lincoln, Nebraska: USDA, 68583–60822.
Van Noordwijk, M., Tanika, L., and Lusiana, B. (2017). Flood risk reduction and flow buffering as ecosystem services – part 2: land use and rainfall intensity effects in Southeast Asia. Hydrol. Earth Syst. Sci. 21, 2341–2360. doi: 10.5194/hess-21-2341-2017
Wariyo, Z., and Negewo, D. (2023). Coffee shade tree selection criteria and management techniques in smallholder coffee-based agroforestry system in Gomma woreda, southwest of Ethiopia. East Afr. J. Forest. Agroforest. 6, 84–97. doi: 10.37284/eajfa.6.1.1126
Warsame, A. A., Sheik-Ali, I. A., Barre, G. M., and Ahmed, A. (2023). Examining the effects of climate change and political instability on maize production in Somalia. Environ. Sci. Pollut. Res. 30, 3293–3306. doi: 10.1007/s11356-022-22227-1
Yadav, G., Jat, H. S., Raju, R., Yadav, R. K., Singh, S. K., Chaudhari, S. K., et al. (2021). Enterprise mix diversification: an option for ecologically sustainable food and nutritional security of small holders in Indo-Gangetic plains. Int. J. Agric. Sustain. :1912978. doi: 10.1080/14735903
Yengwe, J., Okky, A., Obed, I. L., and Stefaan, D. N. (2018). Quantifying nutrient deposition and yield levels of maize (Zea mays) under Faidherbia albida agroforestry system in Zambia. Eur. J. Agron. 99:148–55. doi: 10.1016/j.eja.2018.07.004
Keywords: agroforestry, extreme weather, climate change, resilience, adaptation
Citation: Dobhal S, Kumar R, Bhardwaj AK, Chavan SB, Uthappa AR, Kumar M, Singh A, Jinger D, Rawat P, Handa AK and Ramawat N (2024) Global assessment of production benefits and risk reduction in agroforestry during extreme weather events under climate change scenarios. Front. For. Glob. Change. 7:1379741. doi: 10.3389/ffgc.2024.1379741
Edited by:
Ronald C. Estoque, Forestry and Forest Products Research Institute, JapanReviewed by:
Sileshi Gudeta Weldesemayat, University of KwaZulu-Natal, South AfricaManoj Kumar Jhariya, Sant Gahira Guru Vishwavidyalaya, India
Copyright © 2024 Dobhal, Kumar, Bhardwaj, Chavan, Uthappa, Kumar, Singh, Jinger, Rawat, Handa and Ramawat. This is an open-access article distributed under the terms of the Creative Commons Attribution License (CC BY). The use, distribution or reproduction in other forums is permitted, provided the original author(s) and the copyright owner(s) are credited and that the original publication in this journal is cited, in accordance with accepted academic practice. No use, distribution or reproduction is permitted which does not comply with these terms.
*Correspondence: Raj Kumar, cmFqY3N3Y3J0aUBnbWFpbC5jb20=; Ajay Kumar Bhardwaj, YWpheWtiaGFyZHdhakBnbWFpbC5jb20=