- 1Faculty of Forestry and Wood Sciences, Czech University of Life Sciences Prague, Prague, Czechia
- 2Department of Geosciences and Natural Resources Management, University of Copenhagen, Copenhagen, Denmark
European beech (Fagus sylvatica L.) is becoming one of the go-to species in reconstructing declining conifer stands in Europe under climate change. Assisted migration may be considered when looking for suitable beech seedlings. Knowledge about the photosynthetic performance of beech seedlings is fundamental to understanding an essential part of their growth and survival potential in different planting conditions. We investigated the within-provenance variation in photosynthetic performance driven by altitude in contrast to inter-provenance variation given by geographical distance. The experiment was conducted on seedlings replanted in a Danish common garden comprising a cluster of provenances with various altitudinal subgroups transplanted from the Calabria region (Italy) and two local Danish provenances. Provenance and within-provenance variation in chlorophyll fluorescence (ChlF) kinetics, gas exchange (GE), flushing, and senescence were assessed. ChlF measurements revealed within-provenance differences based on altitude of origin and could distinguish between the two Danish provenances. In contrast, GE parameters detected variation in the geographical distance among Italian provenances. High-elevation subgroups of Italian provenances showed the best leaf-level photosynthetic performance in Danish weather conditions with high precipitation levels. Altitude of origin can be a significant source of within-provenance variation. We demonstrated that assessing this variation in young trees may be instrumental in maximizing the potential of provenance variation across diverse planting sites.
1 Introduction
Pure conifer forests in most of Europe are experiencing a decline due to ongoing climate change (Ols and Bontemps, 2021). Introducing broadleaved tree species into pure conifer stands to increase the resistance of forests to windthrow and bark beetle attacks is becoming the main objective of European forestry management (Griess et al., 2012). This effort has increased demand for seedlings of broadleaved species from suitable provenances and ecotypes (Ammer et al., 2008; Hazarika et al., 2021), such as European beech (Fagus sylvatica L.).
However, the survival of beech forests has become a great concern. A decline in the growth of most European beech populations is predicted for the following decades, with the only exception being the northern limit range of distribution (Martinez del Castillo et al., 2022). The rapidly changing climatic conditions might pose a challenge, primarily due to the increased risk of more severe drought events (Leuschner et al., 2001; Bolte et al., 2007; Geßler et al., 2007; Fotelli et al., 2009; Aranda et al., 2015). Populations at the southern margin of beech’s natural distribution are at the highest risk due to constantly increasing temperatures and prolonged periods of drought (Jump et al., 2006). On the other hand, xeric beech populations exhibit specific morphological and physiological adaptations to overcome unfavorable conditions, such as thicker leaves and increased drought tolerance (García-Plazaola and Becerril, 2001; Aranda et al., 2015). South-eastern European populations also show higher adaptability to different soil water contents and faster growth than north-western provenances (Nielsen and Jørgensen 2003), and higher net assimilation of CO2 rates (Čater and Levanič, 2019). This geographical trend is apparent in the phenology of European beech as north-western populations tend to flush later during Spring (Robson et al., 2013); the same study also stated that lower elevation populations flush earlier but could not fully separate the effect of elevation from latitude and longitude because most of the south-eastern provenances also originated from high altitudes. Beech displays a limited sensitivity to temperature along the altitudinal and temporal gradient in a study by Vitasse et al. (2009). However, long photoperiods tend to increase their bud growth rates, as reported by Basler and Körner (2014). Additionally, beech is known to maintain flushing patterns after replanting (Robson et al., 2013). Given the variation across the large distribution area, the full potential of provenances should be thoroughly assessed to ensure healthy and prosperous beech forests in the future.
Marginal populations are of great importance, which is well acknowledged and documented (e.g., Hampe and Petit, 2005). In the case of European ash (Fraxinus excelsior L.), the populations from the south-eastern edge of the distribution range are of particularly high value because they hold higher genetic diversity (Erichsen et al., 2018). Identifying and preserving such populations in beech might provide a potential source of stress-tolerant planting material suitable for assisted migration to regions experiencing beech decline (Aranda et al., 2015; Dounavi et al., 2016). Most of these diverse populations in Europe persist in the locations of former glacial refugia. The Italian peninsula served as one of the Mediterranean refugia for European beech during the glacial periods (Pott, 1997; Magri et al., 2006). However, new evidence suggests that the beech from the South Italian refugia did not spread much into central and northern Europe. The populations that mainly colonized central and northern Europe after the last glacial period spread likely from relatively northern refugia in southern France, eastern Alps, and southern Moravia – southern Bohemia (Magri et al., 2006). Therefore, the Mediterranean refugia could provide highly contrasting local phenology and growth adaptations reflected by different photosynthetic rates.
Several physiological parameters, such as photosynthetic assimilation measured by gas exchange (GE) and chlorophyll fluorescence (ChlF), can be used to estimate a tree’s leaf-level performance and offer a quick assessment of the physiological status (Bussotti et al., 2010; Niinemets, 2010). ChlF induction (OJIP transient) kinetics indirectly represent the photosynthetic process (Jansen et al., 2009; Jalink and Schoor, 2015) and the adaptive ability to tolerate variable environmental conditions (Baker and Rosenqvist, 2004). Calculated ChlF parameters and the shape of the OJIP curve have shown a high sensitivity to various stress factors (Strasser et al., 2004; Perboni et al., 2012; Kalaji et al., 2016). Each O-J-I-P phase corresponds to different processes in the photosynthetic apparatus – the O-J rise represents the reductions of the acceptor side of PSII, the J-I phase is connected to the oxidation or reduction of the plastoquinone pool, and the I-P phase has to do with re-reduction of plastocynin (PC)+ and P700+ in PSI (Oukarroum et al., 2009). Therefore, the calculated parameters have a different sensitivity to different stress factors and environmental conditions (Bussotti et al., 2010, 2020). ChlF kinetics can assess photosynthesis efficiency, responses to changing environments, genetic variation, and ecological diversity (Murchie and Lawson, 2013; Čepl et al., 2016; Mishra, 2018; Akinyemi et al., 2023) Specific studies of the photosynthesis performance of beech provenances under variable environmental conditions confirmed differences depending on the altitude of origin (Pšidová et al., 2018) or climate of origin (Robson et al., 2012; Kučerová et al., 2018; Kurjak et al., 2019). However, studies comparing ChlF kinetics with GE concerning adaptation across altitudes, and thus climatic gradients within provenances, are, to our knowledge, missing.
Our study, based on a common garden experiment, evaluated the differences in ChlF kinetics and GE among beech provenances and provenances’ altitudinal subgroups. Specifically, we aimed to investigate the parameters/indexes of each method (ChlF and GE), which could be used to distinguish provenances and their altitudinal subgroups. Further, we assessed leaf flushing and senescence as supporting measurements in the same vegetation season and two years later.
We hypothesized that the leaf-level photosynthetic performance in chosen parameters—PN (GE method) and PITOTAL (ChlF method) of Italian provenances would be higher than that of the Danish provenances. In addition, we investigated leaf-level photosynthetic performance among altitudinal subgroups of Italian provenances.
2 Materials and methods
2.1 Plant material
European beech (Fagus sylvatica L.) seedlings (1–2 years old) were replanted from Denmark and Italy (Calabria). The established common garden experiment comprised three Italian provenances (distinct mountain ranges) with various altitudinal subgroups and two Danish provenances representing local populations. The seedlings were planted into HerkuPlast boxes—model Quick Pot 12 T/18 (plastic tray made by Kubern GmbH, Germany) in September 2016 and placed in an outdoor common garden experiment in the nursery of the University of Copenhagen. Plants from the same provenance subgroup were planted in each box (3×4 plants initially). Each block within the original common garden comprised at least nine Italian provenance subgroups (from three provenances), retaining an incomplete randomized block design (9 boxes in each of 20 incomplete blocks). The Italian provenances were replicated in 9 to 15 boxes. The Danish material was replicated within two blocks in 3 and 4 boxes of 12 seedlings each. Seedling mortality occurred after replanting, which violated the original design before the measurement date. The spatial design of the common garden experiment in September 2018 is visualized in Supplementary Figure S1. The imbalances due to this mortality were accounted for in the REML analysis (Butler et al., 2018), and the model details are specified in the description of the statistical analysis.
For our study, we selected Italian plant material from provenances Aspromonte and Sila Grande, each containing two within-provenance subgroups: Aspromonte (1,270 and 1,900 m.a.s.l.) and Sila Grande (1,300 and 1,900 m.a.s.l.) and Danish plant material originating from two different seed stands, F13—Stenderup Midtskov and F419—Holstenshuus (Table 1). The climate data in Table 1 are a selection of the bioclimatic variables from WorldClim version 2.1 climate data for 1970–2000 (Fick and Hijmans, 2017). All provenance origins and the experimental site are shown in Figure 1.
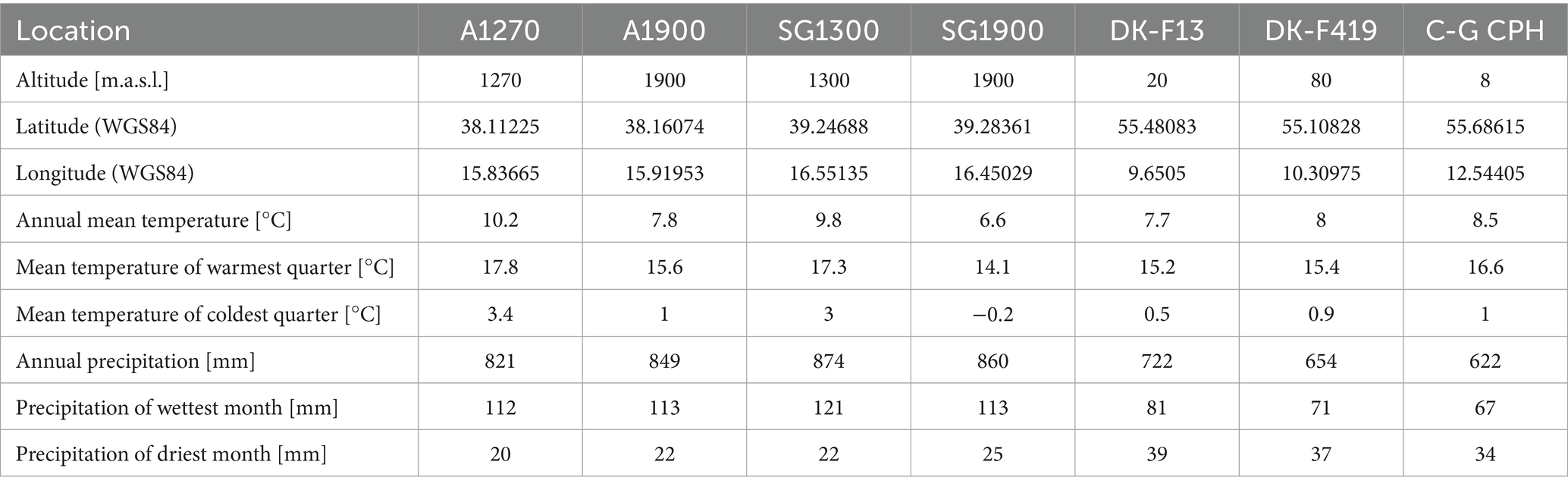
Table 1. Characteristics of locations of origin and the common garden experimental plot in Copenhagen (C-G CPH), where the study was conducted.
The main measurements (ChlF and GE) were done at the end of the second growing season of the trees in the common garden setting in September 2018 (10th–14th). There was sufficient precipitation at the end of August 2018 in Copenhagen, and during the measurement week, it was cloudy with occasional rainfall. The trees were not under any apparent stress during that time.
2.2 Chlorophyll fluorescence kinetics
We measured ChlF kinetics using FluorPen FP110 (PSI, Brno, Czech Republic). We included all surviving (320) seedlings of the six provenance subgroups in the ChlF measurements (Table 2). ChlF induction (OJIP) parameters were measured after dark adaptation (detachable leaf clips placed on leaves 20 min before the measurement). After attaching the instrument to a leaf clip, a saturating light pulse (blue light, 470 nm) of 3,000 μmol·m−2·s−1 intensity was applied to the leaf. Rapid fluorescence transient was captured within the first second of the measurement. We calculated the OJIP parameters according to Strasser et al. (2010) using the FluorPen software (Photon Systems Instruments, Brno, Czechia) and additional parameters from the I-P phase calculated using the raw data according to Oukarroum et al. (2009). All measured and calculated parameters are listed in Table 3.
2.3 Gas exchange
We measured GE (representing net photosynthesis parameters) on randomly selected 84 individuals from all six provenance subgroups: A1270 (14), A1900 (17), SG1300 (19), SG1900 (19), DK-F13 (7), DK-F419 (8). We monitored the light curve using the gas exchange system LI-6400XT (LICOR, NE, United States), where a diode (6400-02B-LED) maintained uniform and stable light inside the chamber. The temperature was adjusted to the external conditions. We sampled leaves from the crown’s upper part for light curve measurement. The reference CO2 concentration was set to 415 μmol·m−2·s−1. We monitored the decreasing light as follows: 1,500-1,000-500-250-120-60-40-20-10-0 μmol·m−2·s−1. The assimilation rate was displayed against the applied light, and the measured points were fitted according to the equation by Prado and De Moraes (1997) designed for woody species:
where PN is the net photosynthesis rate [μmol (CO2) m−2·s−1]; Pgmax is the maximum gross photosynthesis rate [μmol (CO2) m−2·s−1] k is the adjusting factor; I is the photosynthetic photon flux density [μmol (photons) m−2·s−1]; Icomp is the light compensation point [μmol (photons) m−2·s−1]; and RD is the dark respiration rate [μmol (CO2) m−2·s−1] derived from the curve according to Prado and De Moraes (1997). The constants were optimized by minimizing the residual sum of squares using the Solver function in MS Excel (Lobo et al., 2013). The GE parameters used in the analyses are listed in Table 4.
2.4 Flushing scores
The first flushing assessment was done in May 2018 at the beginning of the second vegetation season. The second flushing assessment took place in April 2020 at a permanent site in a nearby forest where the seedlings were replanted in 2019. The flushing was scored only on the Italian provenances in 2018 and on all provenances in 2020. In total, 361 individuals were included in the first flushing assessment and 247 in the second. The flushing scores scale is based on a 7-point scale by Robson et al. (2013):
1. Dormant buds
2. Buds swollen and elongated
3. First green visible from bud scales, buds adopt a silver-grey sheen
4. First folded hairy leaves visible but remain partially held by the bud
5. Entire leaves cascade from the bud; the leaves are still folded and flaccid
6. Leaves unfolded but still corrugated, fan-shaped and hairy
7. Leaves fully unfolded, smooth, and flat.
2.5 Senescence scores
Leaf senescence was assessed at the beginning of November 2020 for all 244 individuals in the permanent experimental site. The senescence assessment was based on a 0–9 point scale assessing the majority of leaves: 0 represents leaves with no visible signs of senescence; 1–4 represents leaves with increasing levels of yellowing; 4–8 represents leaves with increasing amounts of necroses, and 9 represents dry and dead leaves.
2.6 Statistical analysis
The statistical analysis was conducted using the R software, version 4.2.1 (R Core Team, 2022).
A linear mixed model (LMM) was fitted to evaluate the photosynthetic parameters with the terms:
where Y corresponds to the data vector; μ is the overall mean effect; X is an incidence matrix of a fixed effect; a is the fixed vector of individual provenance; Z1 and Z2 are incidence matrices of random effects; Si is the random effect of a block; Si:Sj is the random effect of a box (Sj) nested in a block (Si); e is the random vector of errors that were normally and independently distributed. The model was analyzed using the ASReml-R software (Butler et al., 2018). After fitting the model, outliers (0–10 per parameter) caused by error measurements were removed from the dataset. Custom R code for pairwise comparisons (least significant difference—LSD) was used for GE and ChlF parameters. We did not use any control for multiple testing.
Wald test (W-value) was implemented to evaluate the fixed effects of country of origin (a is the fixed vector of the country of origin in the model above for this analysis, replacing the fixed vector of individual provenance) when comparing their interaction on all measured traits between pooled Italian and Danish provenances.
For radar chart visualization, the following formula was used to perform a z-score normalization of the model-predicted mean values of photosynthetic parameters:
where X is the mean value of provenance in a given photosynthetic parameter predicted by the linear mixed model; μ is the mean of the data in a given photosynthetic parameter; σ is the standard deviation of the data in a given photosynthetic parameter.
The flushing scores were analyzed using a two-sided Wilcoxon signed-rank test 4.2.1 (R Core Team, 2022).
3 Results
3.1 Photosynthetic performance
The Italian provenances had significantly higher average values compared to the Danish ones in ChlF parameters Fm/F0 (W = 1.55e-06), Fv/Fm (W = 3.93e-07), ψ0 (W = 1.53e-05), φE0 (W = 7.27e-08), δR0 (W = 0.001), φR0 (W = 2.76–07), PIABS (W = 5.16e-08), PITOTAL (W = 6.58e-07), ΔVIP (W = 3.75e-06), and significantly lower average values than the Danish ones in parameters φD0 (W = 3.76e-07), ABS/RC (W = 4.24e-07), TR0/RC (W = 1.47e-05), DI0/RC (W = 8.81e-09). There was no clear observable difference in parameters ET0/RC (W = 0.721), φPav (W = 0.259). The model-predicted means of ChlF parameters for each provenance subgroup are outlined in Table 5. The Italian provenances manifested higher average values than the Danish ones in GE parameters LSP95 (W = 7.90e-08) and PN (W = 2.64e-05). Under the same irradiation, Italian provenances reached the maximum assimilation rate faster than the Danish ones, and their net assimilation was higher. There was no clear trend or difference in the light compensation point (LCP) (W = 0.764) or dark respiration (RD) (W = 0.309). All provenances needed the same photon flux density to assimilate more CO2 than they respire. No difference was observed in the quantum yield of photosynthesis (ϕI0) (W = 0.701), which signifies that the molar ratio between carbon assimilated to photons absorbed in the process does not differ among provenances. The model-predicted means of GE parameters for each provenance subgroup are shown in Table 6. The performance of individual provenance subgroups for each chosen parameter from both types of measurement is visualized in Figure 2.
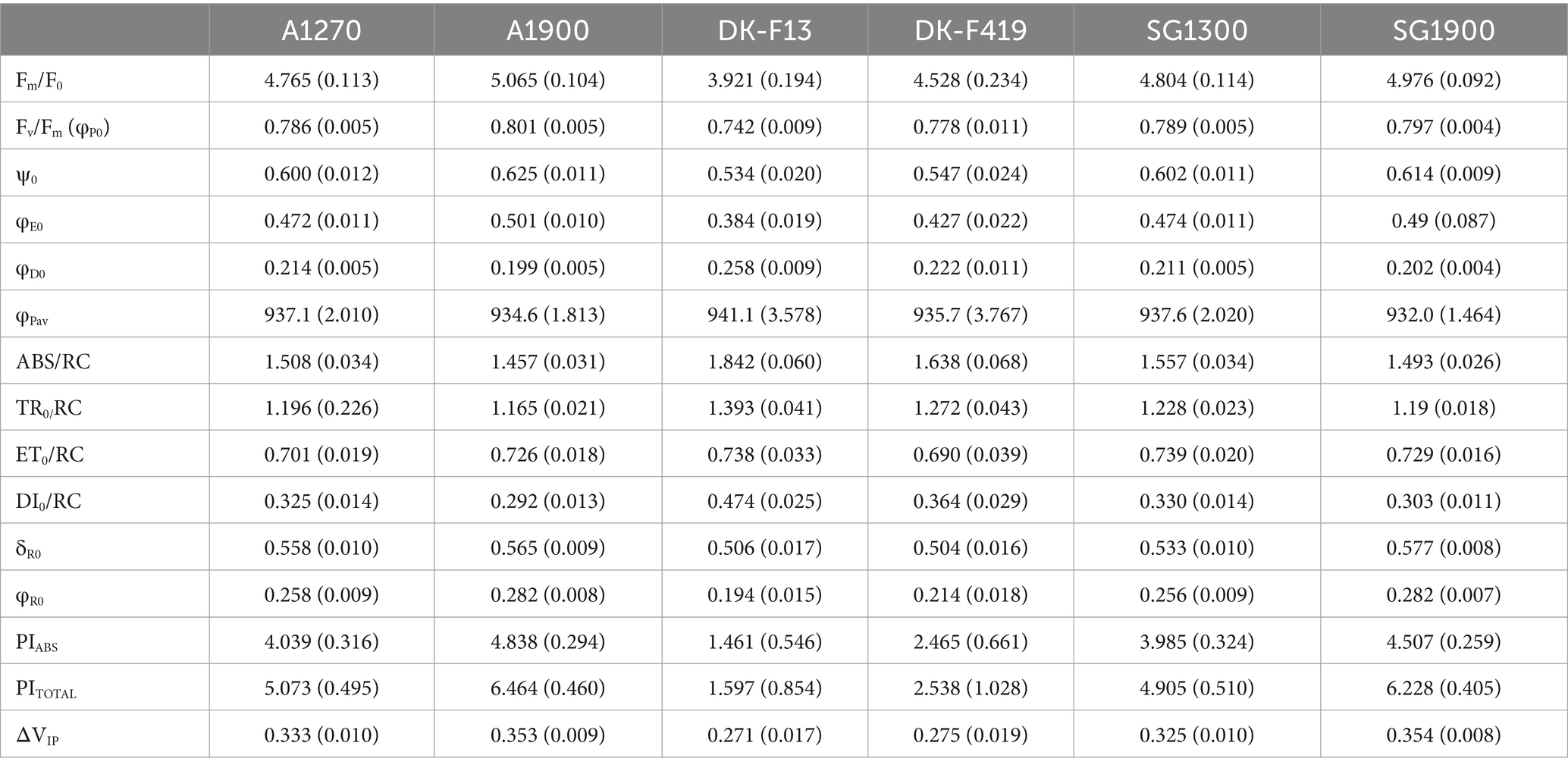
Table 5. Predicted means of ChlF parameters with standard errors (SE) of each provenance subgroup (based on LMM).
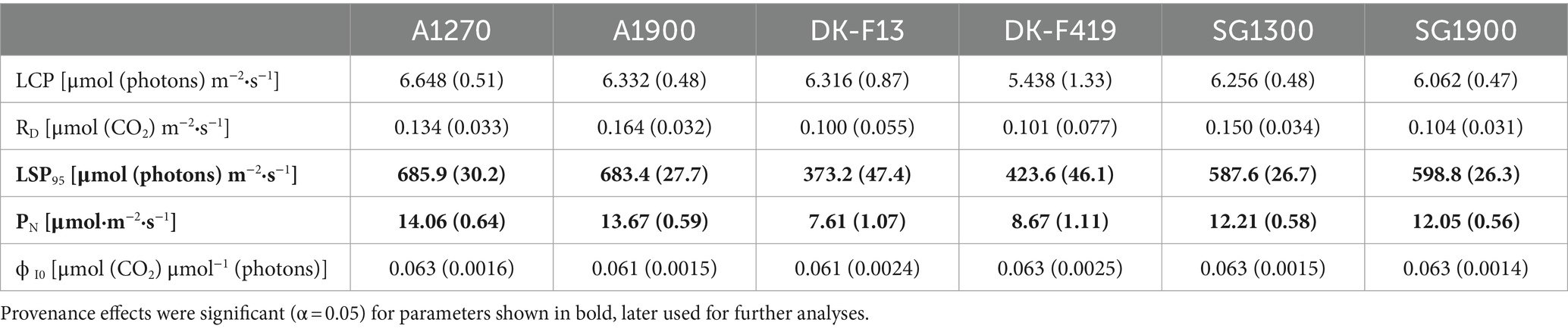
Table 6. Predicted means of GE parameters with standard errors (SE) of each provenance subgroup (based on LMM).
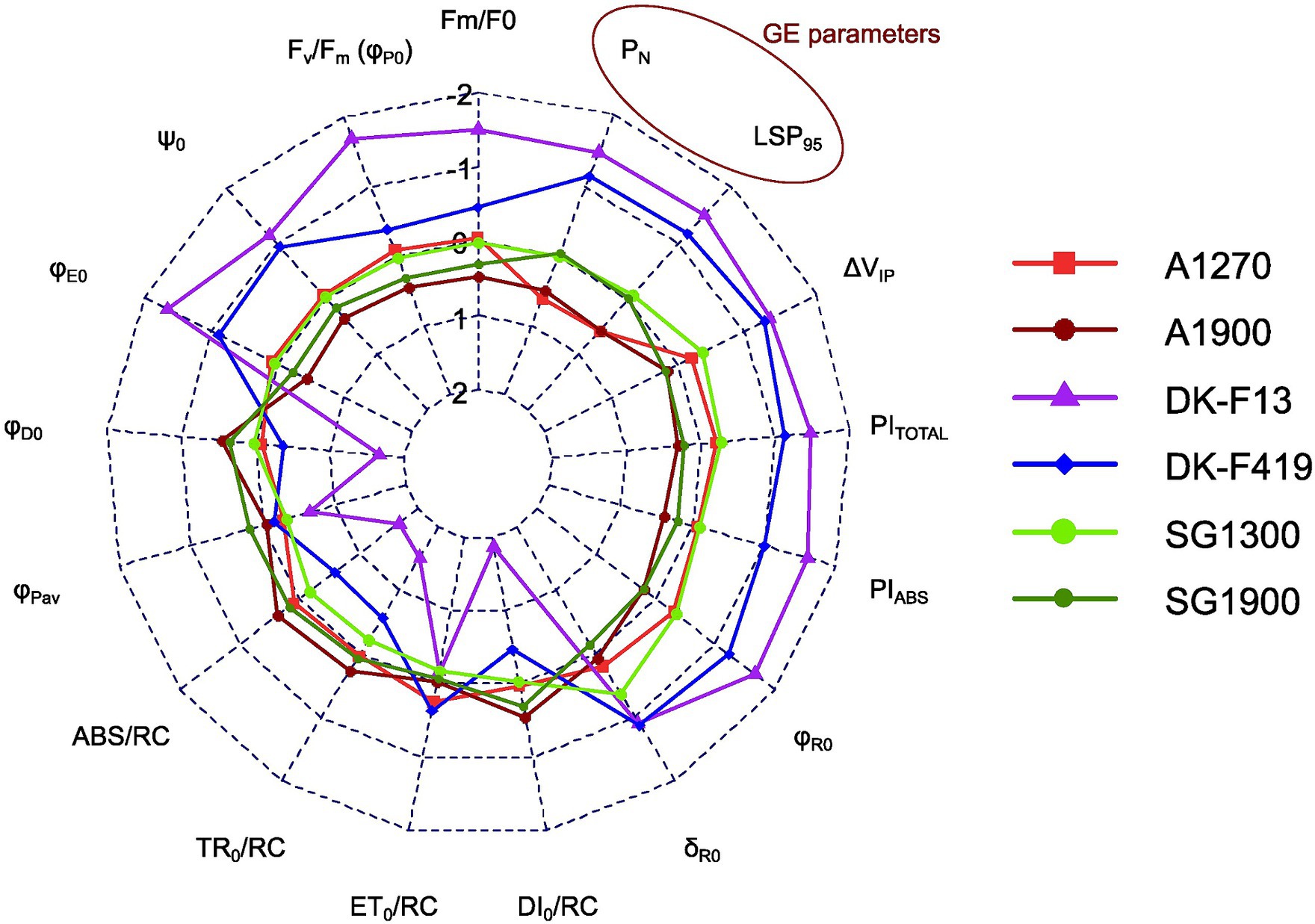
Figure 2. Radar chart of means of different provenances subgroups normalized into Z scores in each ChlF (Fm/F0, Fv/Fm, ψ0, φE0, φD0, φPav, ABS/RC, TR0/RC, ET0/RC, DI0/RC, δR0, φR0, PIABS, PITOTAL, ΔVIP) and GE (PN, LSP95) parameter (based on LMM).
3.2 Provenance pairwise comparison
All provenance subgroup pairs were compared in photosynthetic parameters of ChlF and GE (Figure 3). Both ChlF and GE parameters distinguished Italian and Danish provenances. ChlF parameters DI0/RC, φR0, PITOTAL, and ΔVIP differed among Italian provenance subgroups based on altitude (Figure 3–pink). The GE parameters LSP95 and PN showed differentiation between Italian provenances from Aspromonte and Sila Grande, i.e., different geographical locations (Figure 3–gray and red). In contrast, no altitudinal trend was visible from the GE data, while ChlF measurements could not separate all geographically distant provenances. A different pattern occurred when comparing the two local Danish provenances. The ChlF measurements yielded seven usable parameters (Fm/F0, Fv/Fm, φE0, φD0, ABS/RC, TR0/RC, DI0/RC) that capture differences between the two Danish provenances (Figure 3–light green). The two Danish provenances could not be separated based on the GE parameters. The only parameter that distinguished the Italian within-provenance variation in altitude of origin and also the Danish provenances was DI0/RC (Figure 3–pink and light green).
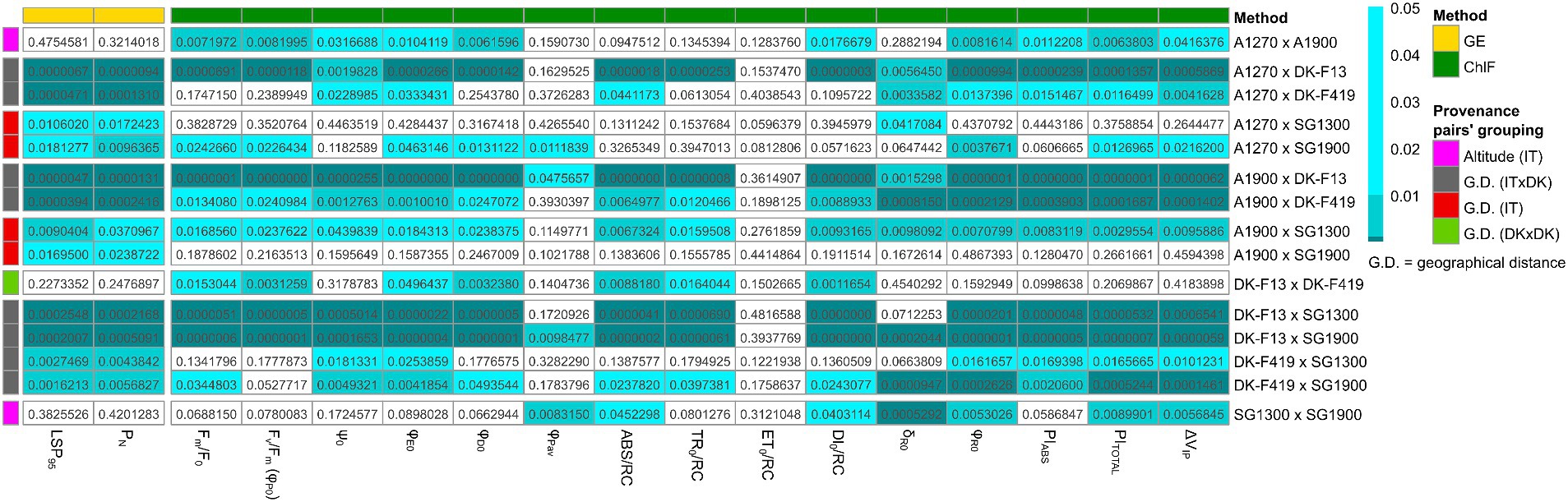
Figure 3. Heatmap of LSD test p-values comparing all pairs of provenance subgroups based on ChlF and GE parameters (without correction for multiple testing) (based on LMM).
3.3 Bud flushing
In May 2018, provenances from the two mountain ranges, Sila Grande (SG) and Aspromonte (A), varied significantly (p = 1.92E-15) in bud flushing scoring. The more southern Aspromonte showed more developed buds at the time of assessment. An altitudinal difference could be seen within the Sila Grande provenance, where SG1900 showed significantly less (p = 2.87E-14) developed buds than SG1300. No such difference could be observed within the Aspromonte provenance. The differences from May 2018 between all individual pairs are outlined in Table 7. The distribution of flushing scores illustrating the magnitude of difference between the provenance subgroups in May 2018 is shown in Figure 4A. The flushing assessment from April 2020 on the surviving trees revealed the same persisting trend (visualized in Figure 4B), although the flushing was in an earlier stage during this assessment. The differences between the provenances and their subgroups in April 2020 (Table 7) remained significant for the same pairs except for SG1300 and A1270. The overall difference between Aspromonte and Sila Grande remained substantial (Figure 4–red vs. green). In addition, in 2020, the Danish provenances were also scored. Both Danish provenances were in a later flushing stage than the Italian ones, except for SG1300, which showed a late flushing pattern more similar to the Danish beech. The significance of the differences is shown in Table 7.
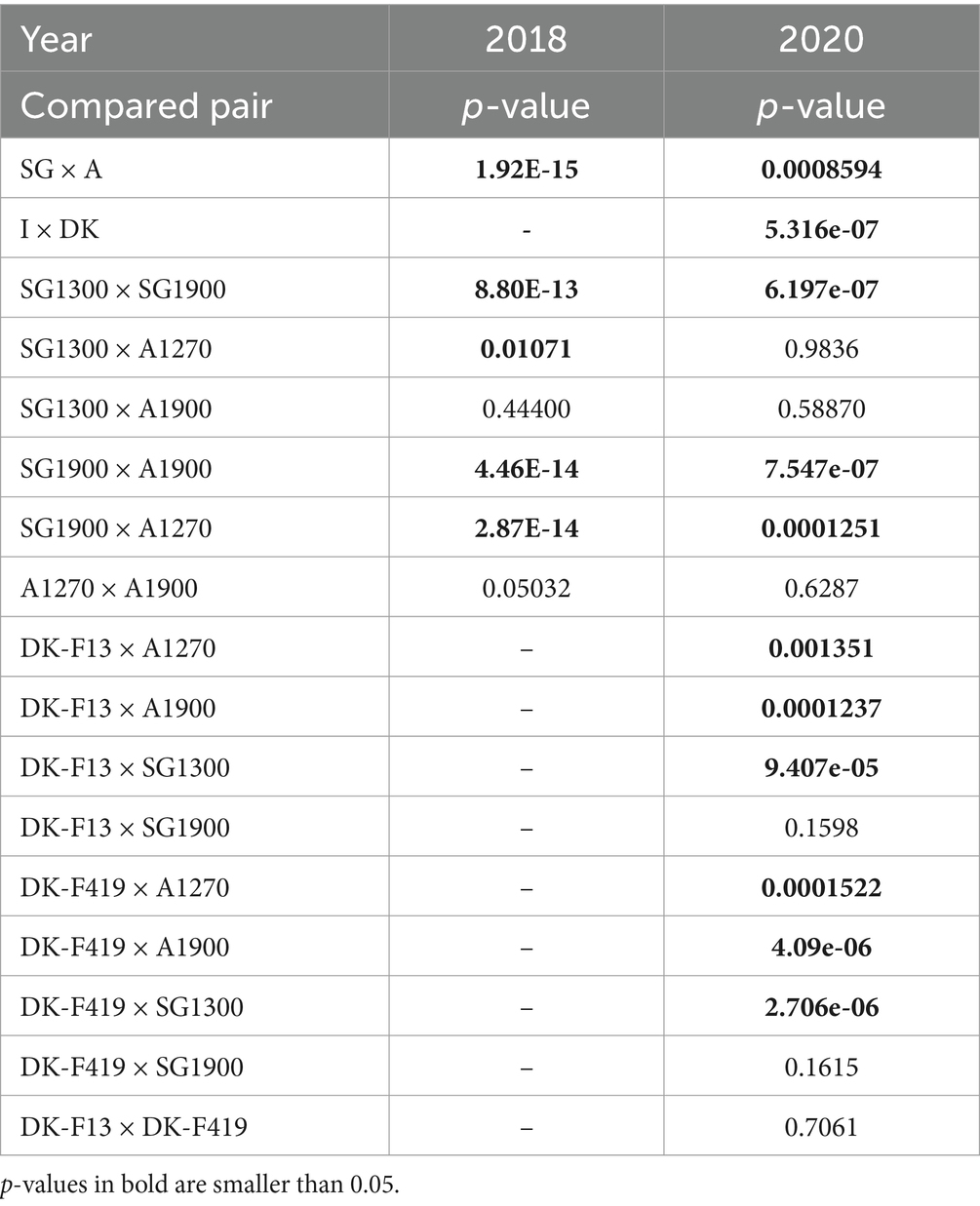
Table 7. Two-sided Wilcoxon signed-rank test of flushing scores between pairs of provenances and provenance subgroups scored in May 2018 and April 2020.
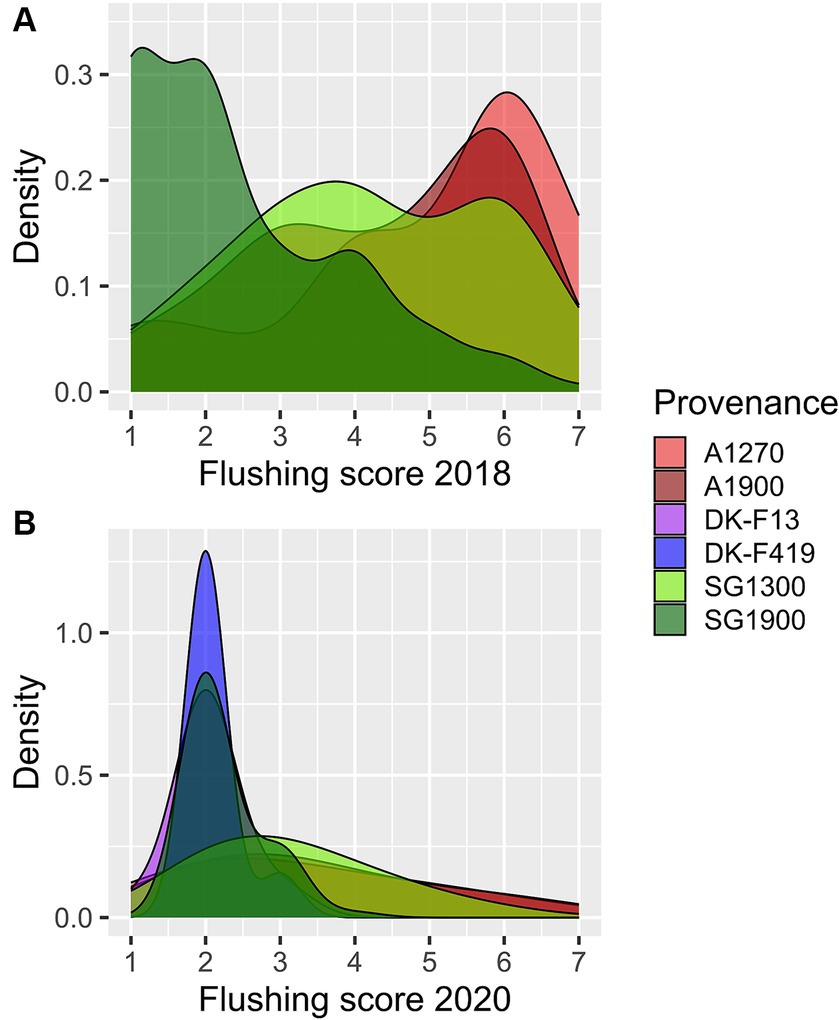
Figure 4. Density plots of flushing scores among provenance subgroups scored in May 2018 (A) and April 2020 (B).
3.4 Senescence
At the beginning of November 2020, all the provenances showed signs of senescence. The majority of leaves were yellowing on almost all of the assessed trees. No significant differences were captured between the provenance subgroups. The senescence scores for individual provenance subgroups are visualized in Figure 5.
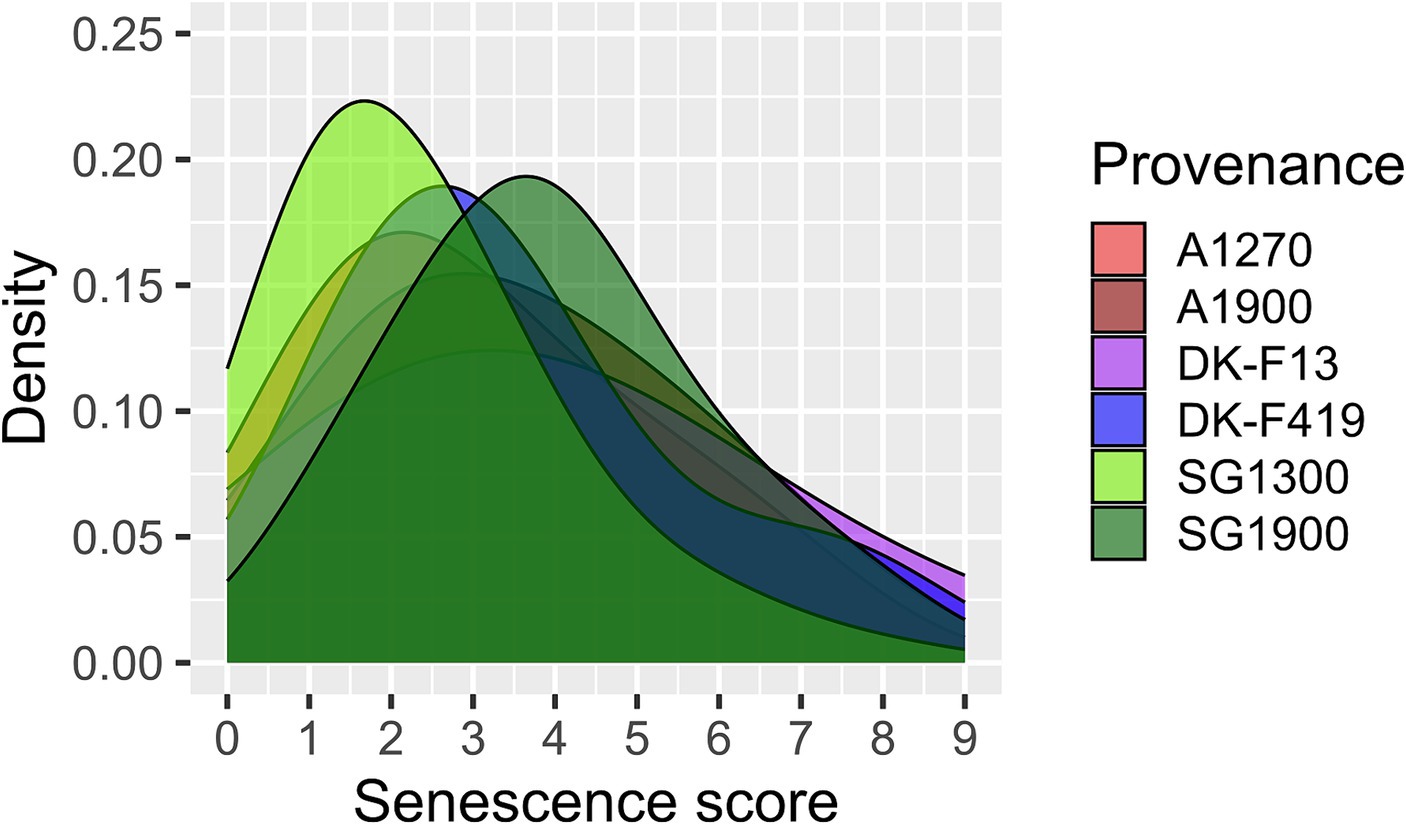
Figure 5. Density plots of leaf senescence scores of individual provenances and the altitudinal subgroups assessed at the beginning of November 2020.
3.5 Correlations between chlorophyll fluorescence parameters and gas exchange parameters
We highlighted the significant correlations (α = 0.05) parameters obtained from ChlF and GE methods in a heat map (Supplementary Figure S2). There were mostly weak correlations between ChlF and GE parameters, with the highest positive being 0.41 between PITOTAL and PN and the highest negative being −0.40 between TR0/RC and PN.
4 Discussion
ChlF and GE can estimate a tree’s physiological status based on photosynthetic assimilation rates obtained at the leaf level (Niinemets, 2010). We found significant differences between Italian and Danish provenances in almost all photosynthetic parameters. We confirmed our hypothesis that Italian provenances will perform better in chosen parameters PN (GE method) and PITOTAL (ChlF method). Furthermore, among the Italian provenances, the provenance subgroups from higher altitudes perform better in PN and PITOTAL in the Danish climate. Italian trees from higher altitudes react better to the wetter and colder Danish weather, possibly due to adaptation to harsher conditions. This is further supported by a higher survival rate of Italian provenances from higher elevations than the ones from lower elevations, especially in the case of SG1900, which had the highest survival rate in 2018, even surpassing the local provenances. The higher survival rate of SG 1900 could be connected to later bud flushing of this provenance in 2018, which could have protected it from spring frost damage. On the contrary, both Aspromonte provenances’ survival was relatively low at the end of 2018. Between 2018 and 2020, the survival rate of all provenance subgroups remained stable, with only a few trees not surviving (Table 2). The higher initial survival rate of Danish provenances can possibly be explained by adaptation to local conditions and shorter transport time before replanting than in the case of Italian ones.
Comparable studies focused on altitudinal differences within similar origins are lacking. However, several studies have tackled latitudinal differences in photosynthetic performance. In a beech common garden study, similar by design to ours, southern provenances maintained higher net photosynthesis rates (PN), water use efficiency, and growth rate throughout the summer (Robson et al., 2012). In contrast, a Romanian provenance of beech showed under different CO2 treatments lower absolute PN values than the Danish one in a study by Leverenz et al. (1999). Additionally, xeric provenances are reported to have a faster net photosynthesis rate (PN) recovery after drought treatment (Arend et al., 2016), which confirms that the differences in GE and ChlF parameters between provenances are magnified when exposed to drought stress (Aranda et al., 2015). In our case, the higher absolute values of photosynthetic parameters in Italian provenances were probably caused by differences in phenology and reflected adaptation. Nielsen and Jørgensen (2003) reported that Danish beech provenances ceased diameter growth sooner than southern European provenances in similar climate conditions as our experiment had.
4.1 Chlorophyll fluorescence kinetics and gas exchange variation of the replanted provenance subgroups
Other studies comparing the photosynthetic performance of European beech that originates from different altitudes include provenances where the trend of elevation is confounded in a shared cardinal direction (e.g., Robson et al., 2012, 2013; Kučerová et al., 2018; Pšidová et al., 2018). Therefore, the latitude and longitude of origin could also drive the differences and cannot be effectively separated. In our study, the geographical distances between provenance subgroups occupying a single mountain range were minimal, meaning that physiological differences within these provenances reflect mainly altitude.
The most common use of ChlF kinetics is to diagnose early stress responses to biotic or abiotic factors before any decline in growth can be observed (Fiorani and Schurr, 2013; Faseela et al., 2020). DI0/RC and Fv/Fm are often used as drought or temperature stress indicators (Mathur et al., 2013; Yuan et al., 2013; Fghire et al., 2015). However, the most commonly used parameter, Fv/Fm, is quite robust and sometimes cannot be as sensitive as other ChlF parameters (Bussotti et al., 2020). As the trees in our experiment were under no apparent stress at the time of measurements, these parameters instead reflected provenance variation and overall vitality after replanting. When comparing the subgroups of Italian provenances in ChlF, four parameters could make a distinction based on the altitude (DI0/RC, φR0, PITOTAL, ΔVIP). Higher φR0, PITOTAL, and ΔVIP values were estimated for the high-altitude provenance subgroups in Aspromonte and Sila Grande, and higher DI0/RC values for the low-altitude provenance subgroups in Aspromonte and Sila Grande (Table 5). ΔVIP is the parameter we found especially interesting in which the relative loss of the I-P phase is related to a loss of PSI reaction centers (Oukarroum et al., 2009), as indices connected to the I-P phase were not much previously explored in European beech. Furthermore, Fv/Fm and ΔVIP are suggested to be the two most suitable OJIP parameters to capture the variability of plant photosynthetic efficiency and their responses to environmental pressures (Bussotti et al., 2020). The ChlF parameter we found most indicative was DI0/RC, as it was the only one to differentiate between the two Danish provenances as well as between the Italian provenance subgroups on an altitudinal basis. However, in our study, ChlF parameters could not distinguish provenances based on their geographical origin.
Findings from two studies conducted in a single common garden experiment in Slovakia by Kučerová et al. (2018) and Pšidová et al. (2018) reported mechanistic differences in ChlF kinetics among beech provenances. Kučerová et al. (2018) observed that Austrian and Polish provenances differed from others in the Fv/Fm ratio, although this trend was inconsistent along the altitudinal gradient. Pšidová et al. (2018), focused on provenance performance during heatwaves, finding that ABS/RC and ψ0 were more limited in lower altitude provenances. Higher altitude provenances showed better PSII photochemistry performance, indicated by PIABS, under both dry and wet conditions, which aligns with our findings. Kurjak et al. (2019) found significant associations between ChlF parameters (mainly Fv/Fm and PIABS) and the climate of origin. Provenances from colder, wetter regions exhibited higher PSII performance compared to those from warmer, drier climates. This trend was also linked to the geographical distance from the Slovenian refugium and the common garden site, with closer proximity resulting in better PSII performance. PSII performance decreased towards the north-western margins, supporting our results regarding latitude-related variations in ChlF.
On the other hand, two GE parameters (LSP95 and PN) could reliably distinguish geographical origin (the different Italian mountain ranges, Aspromonte and Sila Grande). We observed no trend in altitude in the GE data. In contrast, Kučerová et al. (2018) reported a significant linear altitudinal trend for the CO2 assimilation rate between Central European provenances of European beech. Although the provenances used in Kučerová et al. (2018) study originated from different altitudes, they were also geographically distant; therefore, the latitude and longitude of origin could also drive the differences. The GE measurements revealed higher LSP95 and PN values for more southern Aspromonte than Sila Grande. The higher LSP95 values from Aspromonte suggest that Sila Grande beech may be more shade tolerant. A study by Meng et al. (2014) showed that shade-adapted plants have lower light saturation levels. However, in beech, the release of sunlight after a windstorm tends to diminish the differences in LSP between fully released (formerly shade-grown) and sun-grown seedlings (Reynolds et al., 2003). In our study, the different LSP95 values of Aspromonte and Sila Grande could most likely be attributed to the local adaptation. For example, Čater and Levanič (2019) report a reduced shade tolerance and higher efficiency of beech towards the south, which supports our results and may correspond to a boost in regeneration.
4.2 Flushing scores related to provenance subgroups’ variation
In 2018 and 2020, the southern provenance from Aspromonte exhibited significantly higher flushing scores compared to Sila Grande’s provenance. Additionally, in 2020, the Danish provenances showed lower flushing scores than the Italian ones. Previous studies have found that flushing is significantly correlated with latitude, longitude, and altitude, influenced by factors such as chilling, temperature sums, and day length (Nielsen and Jørgensen, 2003). The duration of phenological phases varies annually and mainly depends on temperature (Bednářová and Merklová, 2007; Slovíková and Bednářová, 2014). Similarly, research from Slovakia (Schieber et al., 2013), southern Germany (Dittmar and Elling, 2006), and Slovenia (Čufar et al., 2012) showed phenological variation in beech along the altitudinal gradient. In southern Germany, beech leaf unfolding was delayed by 2–3 days for every 100 m of altitudinal increase (Dittmar and Elling, 2006). While the Sila Grande provenance showed variation in flushing along the elevation gradient in 2018 and 2020, this was not observed within Aspromonte. However, our consistent results from 2018 and 2020 suggest that the adaptation of the provenances is linked to flushing variation, as the provenance flushing scores remained stable in Danish climatic conditions over two seasons. Vitasse et al. (2009) noted that beech leaf unfolding is less temperature-sensitive than other European tree species, indicating that other factors are more influential.
Flushing scores did not correlate significantly with photosynthetic parameters. The two data collections in 2018 (flushing vs. GE and ChlF) were conducted at the beginning and end of the vegetation season, respectively, so the expected correlation pattern may have been disrupted due to the various factors influencing the trees during the vegetation season. The weather fluctuations influenced the seedlings’ performance in the common garden, and some mortality occurred during the vegetation season. However, both GE and flushing similarly cluster the Italian provenances and their subgroups (Figures 2, 4). In 2020, the Danish provenances were also scored. Both Danish provenances were in a later flushing stage than the Italian ones. The only exception was SG1300, which showed a late flushing pattern similar to the Danish beech. It still had a slightly earlier flushing pattern than the Danish provenances, although not significantly.
4.3 Senescence
There were no visible differences in the senescence of leaves between the provenances and provenance subgroups at the beginning of November 2020 (Figure 5). We conclude that as there were no observable differences in November senescence, the photosynthetic measurements conducted at the beginning of September 2018 were not influenced by the direct onset of leaf senescence. It is widely known that senescence is connected to chlorophyll content. As leaves in deciduous trees lose some chlorophyll content during senescence, the photosynthetic capacity decreases on a leaf area basis (Adams et al., 1990). Leaves that fall later in the season have a better translocation of nutrients, and the translocation is species-dependent (Niinemets and Tamm, 2005). A study in another beech species (Fagus grandifolia Ehrh.) documented chlorophyll degradation that was not sudden but continuous, as the slower formation of the abscission layer during senescence allowed beech to translocate more nutrients out of leaves (Primka and Smith, 2019). However, ChlF does not seem to be affected to such a degree by large decreases in chlorophyll content as the yellowing leaves of deciduous trees are still efficiently used in photosynthesis because the photosynthetic capacity on a chlorophyll basis and photosynthetic energy conversion efficiency do not show a decline (Adams et al., 1990). Therefore, we believe that even at the beginning of the senescence process, the ChlF method could still yield relevant results.
4.4 Limitations
This study has potential limitations that need to be stated. Firstly, we only used two provenances from Calabria representing beech refugia, which we separated into two distinct altitudinal subgroups and two local Danish provenances. Secondly, the sample size of GE measurements was smaller than that of ChlF measurements due to the extensive time consumption of the GE method. The main measurements (GE and ChlF) were conducted during one week at the beginning of September, while flushing had to be scored in the Spring (2018 and 2020). Our study did not involve any stress treatment or biomass measurements, which would also be beneficial in understanding the well-being of replanted trees in new conditions. Flushing was not scored for the Danish plant material in 2018. Senescence was only assessed in 2020; however, this secondary evaluation provided more insight into the potential influence of different onsets of senescence on the measurements taken in September 2018. Our results and conclusions are based on methods applied at various times, as we aimed to observe the differences in spring and autumn phenology. This combined approach helped to verify our main conclusion connected to the variance of photosynthetic performance due to phenology.
5 Conclusion
We found significant differences between Italian and Danish provenances of European beech in almost all photosynthetic parameters. More interestingly, we also detected minor differences among and within Italian provenances. The high-throughput ChlF measurements revealed altitude-based differences within provenances and distinguished the two Danish provenances. As hypothesized, leaf-level photosynthetic performance, indicated by PN (GE method) and PITOTAL (ChlF method), was higher in Italian provenances than in Danish ones. Significant differences in photosynthetic performance were also found between altitudinal subgroups within Italian provenances. We conclude that photosynthetic within-provenance altitudinal variation is of similar importance as inter-provenance variation driven by geographical distance and that the sources of variation should be separately addressed. In our study, the photosynthetic parameters reflected phenology connected to broad genetic variation among provenances. We further conclude that the ChlF can uncover variability on a finer scale than GE as it revealed the provenance subgroups’ altitudinal differences. Moreover, spring flushing scores repeatedly clustered the Italian provenance subgroups similarly to GE. As the Italian provenances exhibited generally higher photosynthetic rates in Danish conditions than local provenances, we recommend further exploring the beech refugia when assisted migration is of interest.
Data availability statement
The datasets presented in this study can be found in online repositories. The names of the repository/repositories and accession number(s) can be found in the article/Supplementary material; and in Figshare: dx.doi.org/10.6084/m9.figshare.23736336.
Author contributions
DP: Conceptualization, Formal analysis, Investigation, Methodology, Validation, Visualization, Writing – original draft, Writing – review & editing. JS: Conceptualization, Data curation, Formal analysis, Investigation, Methodology, Supervision, Validation, Writing – original draft, Writing – review & editing. OH: Conceptualization, Data curation, Funding acquisition, Methodology, Project administration, Resources, Writing – review & editing. JČ: Formal analysis, Investigation, Methodology, Validation, Writing – review & editing. EE: Data curation, Funding acquisition, Project administration, Resources, Writing – review & editing. JH: Supervision, Validation, Writing – review & editing. DZ: Formal analysis, Investigation, Writing – original draft. IT: Conceptualization, Data curation, Formal analysis, Methodology, Supervision, Validation, Writing – review & editing.
Funding
The author(s) declare financial support was received for the research, authorship, and/or publication of this article. The plant material was collected and grown under the “Genetic diversity and adaptability of Calabrian forest trees” project funded by the Godfred Birkedal Hartmanns Family Foundation.
Acknowledgments
DP would like to thank Lance Parker Moore for his constructive feedback and valuable suggestions that helped to improve and finalize the manuscript.
Conflict of interest
The authors declare that the research was conducted in the absence of any commercial or financial relationships that could be construed as a potential conflict of interest.
Publisher’s note
All claims expressed in this article are solely those of the authors and do not necessarily represent those of their affiliated organizations, or those of the publisher, the editors and the reviewers. Any product that may be evaluated in this article, or claim that may be made by its manufacturer, is not guaranteed or endorsed by the publisher.
Supplementary material
The Supplementary material for this article can be found online at: https://www.frontiersin.org/articles/10.3389/ffgc.2024.1369464/full#supplementary-material
Supplementary Figure 1 | Spatial design of the common garden experiment in September 2018.
Supplementary Figure 2 | Heatmap of Pearson correlation coefficient between parameters obtained from ChlF and GE. Only significant (α = 0.05) correlations are shown.
References
Adams, W. W., Winter, K., Schreiber, U., and Schramel, P. (1990). Photosynthesis and chlorophyll fluorescence characteristics in relationship to changes in pigment and element composition of leaves of Platanus occidentalis L. during autumnal leaf senescence. Plant Physiol. 92, 1184–1190. doi: 10.1104/pp.92.4.1184
Akinyemi, O. O., Čepl, J., Keski-Saari, S., Tomášková, I., Stejskal, J., Kontunen-Soppela, S., et al. (2023). Derivative-based time-adjusted analysis of diurnal and within-tree variation in the OJIP fluorescence transient of silver birch. Photosynth. Res. 157, 133–146. doi: 10.1007/s11120-023-01033-x
Ammer, C., Bickel, E., and Kölling, C. (2008). Converting Norway spruce stands with beech – a review of arguments and techniques. Austrian J. For. Sci. 125, 3–26.
Aranda, I., Cano, F. J., Gasco, A., Cochard, H., Nardini, A., Mancha, J. A., et al. (2015). Variation in photosynthetic performance and hydraulic architecture across European beech (Fagus sylvatica L.) populations supports the case for local adaptation to water stress. Tree Physiol. 35, 34–46. doi: 10.1093/treephys/tpu101
Arend, M., Sever, K., Pflug, E., Gessler, A., and Schaub, M. (2016). Seasonal photosynthetic response of European beech to severe summer drought: limitation, recovery and post-drought stimulation. Agric. For. Meteorol. 220, 83–89. doi: 10.1016/j.agrformet.2016.01.011
Baker, N. R., and Rosenqvist, E. (2004). Applications of chlorophyll fluorescence can improve crop production strategies: an examination of future possibilities. J. Exp. Bot. 55, 1607–1621. doi: 10.1093/jxb/erh196
Basler, D., and Körner, C. (2014). Photoperiod and temperature responses of bud swelling and bud burst in four temperate forest tree species. Tree Physiol. 34, 377–388. doi: 10.1093/treephys/tpu021
Bednářová, E., and Merklová, L. (2007). Results of monitoring the vegetative phenological phases of European beech (Fagus sylvatica L.) in 1991-2006. Folia Oecol. 34, 77–85.
Bolte, A., Czajkowski, T., and Kompa, T. (2007). The north-eastern distribution range of European beech – a review. Forestry 80, 413–429. doi: 10.1093/forestry/cpm028
Bussotti, F., Desotgiu, R., Pollastrini, M., and Cascio, C. (2010). The JIP test: a tool to screen the capacity of plant adaptation to climate change. Scand. J. For. Res. 25, 43–50. doi: 10.1080/02827581.2010.485777
Bussotti, F., Gerosa, G., Digrado, A., and Pollastrini, M. (2020). Selection of chlorophyll fluorescence parameters as indicators of photosynthetic efficiency in large scale plant ecological studies. Ecol. Indic. 108:105686. doi: 10.1016/j.ecolind.2019.105686
Butler, D. G., Cullis, B. R., Gilmour, A. R., Gogel, B. J., and Thompson, R. (2018). ASReml-R reference manual version 4 ASReml estimates variance components under a general linear mixed model by residual maximum likelihood (REML), VSN International Ltd.
Čater, M., and Levanič, T. (2019). Beech and silver fir’s response along the Balkan’s latitudinal gradient. Sci. Rep. 9:16269. doi: 10.1038/s41598-019-52670-z
Čepl, J., Holá, D., Stejskal, J., Korecký, J., Kočová, M., Lhotáková, Z., et al. (2016). Genetic variability and heritability of chlorophyll a fuorescence parameters in scots pine (Pinus sylvestris L.). Tree Physiol. 36, 883–895. doi: 10.1093/treephys/tpw028
Čufar, K., de Luis, M., Saz, M. A., Črepinšek, Z., and Kajfež-Bogataj, L. (2012). Temporal shifts in leaf phenology of beech (Fagus sylvatica) depend on elevation. Trees 26, 1091–1100. doi: 10.1007/s00468-012-0686-7
Dittmar, C., and Elling, W. (2006). Phenological phases of common beech (Fagus sylvatica L.) and their dependence on region and altitude in southern Germany. Eur. J. For. Res. 125, 181–188. doi: 10.1007/s10342-005-0099-x
Dounavi, A., Netzer, F., Celepirovic, N., Ivanković, M., Burger, J., Figueroa, A. G., et al. (2016). Genetic and physiological differences of European beech provenances (F. sylvatica L.) exposed to drought stress. For. Ecol. Manag. 361, 226–236. doi: 10.1016/j.foreco.2015.11.014
Erichsen, E. O., Budde, K. B., Sagheb-Talebi, K., Bagnoli, F., Vendramin, G. G., and Hansen, O. K. (2018). Hyrcanian forests-stable rear-edge populations harbouring high genetic diversity of Fraxinus excelsior, a common European tree species. Distribution 24, 1521–1533. doi: 10.1111/ddi.12783
Faseela, P., Sinisha, A. K., Brestic, M., and Puthur, J. (2020). Special issue in honour of prof. Reto J. Strasser – chlorophyll a fluorescence parameters as indicators of a particular abiotic stress in rice. Photosynthetica 58, 293–300. doi: 10.32615/ps.2019.147
Fghire, R., Anaya, F., Ali, O. I., Benlhabib, O., Ragab, R., and Wahbi, S. (2015). Physiological and photosynthetic response of quinoa to drought stress. Chilean J. Agric. Res. 75, 174–183. doi: 10.4067/S0718-58392015000200006
Fick, S. E., and Hijmans, R. J. (2017). WorldClim 2: new 1-km spatial resolution climate surfaces for global land areas. Int. J. Climatol. 37, 4302–4315. doi: 10.1002/joc.5086
Fiorani, F., and Schurr, U. (2013). Future scenarios for plant phenotyping. Annu. Rev. Plant Biol. 64, 267–291. doi: 10.1146/annurev-arplant-050312-120137
Fotelli, M. N., Nahm, M., Radoglou, K., Rennenberg, H., Halyvopoulos, G., and Matzarakis, A. (2009). Seasonal and interannual ecophysiological responses of beech (Fagus sylvatica) at its south-eastern distribution limit in Europe. For. Ecol. Manag. 257, 1157–1164. doi: 10.1016/j.foreco.2008.11.026
García-Plazaola, J. I., and Becerril, J. M. (2001). Seasonal changes in photosynthetic pigments and antioxidants in beech (Fagus sylvatica) in a Mediterranean climate: implications for tree decline diagnosis. Aust. J. Plant Physiol. 28, 225–232. doi: 10.1071/pp00119
Geßler, A., Keitel, C., Kreuzwieser, J., Matyssek, R., Seiler, W., and Rennenberg, H. (2007). Potential risks for European beech (Fagus sylvatica L.) in a changing climate. Trees 21, 1–11. doi: 10.1007/s00468-006-0107-x
Griess, V. C., Acevedo, R., Härtl, F., Staupendahl, K., and Knoke, T. (2012). Does mixing tree species enhance stand resistance against natural hazards? A case study for spruce. For. Ecol. Manag. 267, 284–296. doi: 10.1016/j.foreco.2011.11.035
Hampe, A., and Petit, R. J. (2005). Conserving biodiversity under climate change: the rear edge matters. Ecol. Lett. 8, 461–467. doi: 10.1111/j.1461-0248.2005.00739.x
Hazarika, R., Bolte, A., Bednarova, D., Chakraborty, D., Gaviria, J., Kanzian, M., et al. (2021). Multi-actor perspectives on afforestation and reforestation strategies in Central Europe under climate change. Ann. For. Sci. 78:60. doi: 10.1007/s13595-021-01044-5
Jalink, H., and Schoor, R. (2015). “Role of fluorescence approaches to understand functional traits of photosynthesis” in Phenomics in crop plants: trends, options and limitations. eds. J. Kumar, A. Pratap, and S. Kumar (New Delhi: Springer), 181–194.
Jansen, M., Gilmer, F., Biskup, B., Nagel, K., Fischbach, A., Briem, S., et al. (2009). Simultaneous phenotyping of leaf growth and chlorophyll fluorescence via growscreen fluoro allows detection of stress tolerance in. Funct. Plant Biol. 36, 902–914. doi: 10.1071/FP09095
Jump, A. S., Hunt, J. M., and Pen̈uelas, J. (2006). Rapid climate change-related growth decline at the southern range edge of Fagus sylvatica. Glob. Chang. Biol. 12, 2163–2174. doi: 10.1111/j.1365-2486.2006.01250.x
Kalaji, H. M., Jajoo, A., Oukarroum, A., Brestic, M., Zivcak, M., Samborska, I. A., et al. (2016). Chlorophyll a fluorescence as a tool to monitor physiological status of plants under abiotic stress conditions. Acta Physiol. Plant. 38:102. doi: 10.1007/s11738-016-2113-y
Kučerová, J., Konôpková, A., Pšidová, E., Kurjak, D., Jamnická, G., Slugenová, K., et al. (2018). Adaptive variation in physiological traits of beech provenances in Central Europe. IForest 11, 24–31. doi: 10.3832/ifor2291-010
Kurjak, D., Konôpková, A., Kmeť, J., Macková, M., Frýdl, J., Živčák, M., et al. (2019). Variation in the performance and thermostability of photosystem II in European beech (Fagus sylvatica L.) provenances is influenced more by acclimation than by adaptation. Eur. J. For. Res. 138, 79–92. doi: 10.1007/s10342-018-1155-7
Leuschner, C., Backes, K., Hertel, D., Schipka, F., Schmitt, U., Terborg, O., et al. (2001). Drought responses at leaf, stem and fine root levels of competitive Fagus sylvatica L. and Quercus petraea (Matt.) Liebl. Trees in dry and wet years. For. Ecol. Manag. 149, 33–46. doi: 10.1016/S0378-1127(00)00543-0
Leverenz, J. W., Bruhn, D., and Saxe, H. (1999). Responses of two provenances of Fagus sylvatica seedlings to a combination of four temperature and two CO2 treatments during their first growing season: gas exchange of leaves and roots. New Phytol. 144, 437–454. doi: 10.1046/j.1469-8137.1999.00541.x
Lobo, F. D. A., De Barros, M. P., Dalmagro, H. J., Dalmolin, Â. C., Pereira, W. E., De Souza, É. C., et al. (2013). Fitting net photosynthetic light-response curves with Microsoft excel – a critical look at the models. Photosynthetica 51, 445–456. doi: 10.1007/s11099-013-0045-y
Magri, D., Vendramin, G. G., Comps, B., Dupanloup, I., Geburek, T., Gömöry, D., et al. (2006). A new scenario for the quaternary history of European beech populations: palaeobotanical evidence and genetic consequences. New Phytol. 171, 199–221. doi: 10.1111/j.1469-8137.2006.01740.x
Martinez del Castillo, E., Zang, C. S., Buras, A., Hacket-Pain, A., Esper, J., Serrano-Notivoli, R., et al. (2022). Climate-change-driven growth decline of European beech forests. Commun. Biol. 5:163. doi: 10.1038/s42003-022-03107-3
Mathur, S., Mehta, P., and Jajoo, A. (2013). Effects of dual stress (high salt and high temperature) on the photochemical efficiency of wheat leaves (Triticum aestivum). Physiol. Mol. Biol. Plants 19, 179–188. doi: 10.1007/s12298-012-0151-5
Meng, F., Cao, R., Yang, D., Niklas, K. J., and Sun, S. (2014). Trade-offs between light interception and leaf water shedding: A comparison of shade- and sun-adapted species in a subtropical rainforest. Oecol. 174, 13–22. doi: 10.1007/s00442-013-2746-0
Mishra, A. (2018). “Chlorophyll fluorescence: a practical approach to study ecophysiology of green plants” in Advances in plant ecophysiology techniques. eds. A. Sánchez-Moreiras and M. Reigosa (Cham: Springer), 77–97.
Murchie, E., and Lawson, T. (2013). Chlorophyll fluorescence analysis: a guide to good practice and understanding some new applications. J. Exp. Bot. 64, 3983–3998. doi: 10.1093/jxb/ert208
Nielsen, C. N., and Jørgensen, F. V. (2003). Phenology and diameter increment in seedlings of European beech (Fagus sylvatica L.) as affected by different soil water contents: variation between and within provenances. For. Ecol. Manag. 174, 233–249. doi: 10.1016/S0378-1127(02)00042-7
Niinemets, Ü. (2010). Responses of forest trees to single and multiple environmental stresses from seedlings to mature plants: past stress history, stress interactions, tolerance and acclimation. For. Ecol. Manag. 260, 1623–1639. doi: 10.1016/j.foreco.2010.07.054
Niinemets, Ü., and Tamm, Ü. (2005). Species differences in timing of leaf fall and foliage chemistry modify nutrient resorption efficiency in deciduous temperate forest stands. Tree Physiol. 25, 1001–1014. doi: 10.1093/treephys/25.8.1001
Ols, C., and Bontemps, J. D. (2021). Pure and even-aged forestry of fast-growing conifers under climate change: on the need for a silvicultural paradigm shift. Environ. Res. Lett. 16, 1–5. doi: 10.1088/1748-9326/abd6a7
Oukarroum, A., Schansker, G., and Strasser, R. J. (2009). Drought stress effects on photosystem i content and photosystem II thermotolerance analyzed using Chl a fluorescence kinetics in barley varieties differing in their drought tolerance. Physiol. Plant. 137, 188–199. doi: 10.1111/j.1399-3054.2009.01273.x
Perboni, A. T., Cassol, D., da Silva, F. S. P., Silva, D. M., and Bacarin, M. A. (2012). Chlorophyll a fluorescence study revealing effects of flooding in canola hybrids. Biologia (Bratisl) 67, 338–346. doi: 10.2478/s11756-012-0006-0
Pott, R. (1997). Invasion of beech and establishment of beech forests in Europe. Ann. Bot. 55, 27–58. doi: 10.4462/annbotrm-9024
Prado, C. H. B. A., and De Moraes, J. A. P. V. (1997). Photosynthetic capacity and specific leaf mass in twenty woody species of Cerrado vegetation under field conditions. Photosynthetica 33, 103–112. doi: 10.1023/A:1022183423630
Primka, E. J., and Smith, W. K. (2019). Synchrony in fall leaf drop: chlorophyll degradation, color change, and abscission layer formation in three temperate deciduous tree species. Am. J. Bot. 106, 377–388. doi: 10.1002/ajb2.1247
Pšidová, E., Živčák, M., Stojnić, S., Orlović, S., Gömöry, D., Kučerová, J., et al. (2018). Altitude of origin influences the responses of PSII photochemistry to heat waves in European beech (Fagus sylvatica L.). Environ. Exp. Bot. 152, 97–106. doi: 10.1016/j.envexpbot.2017.12.001
R Core Team (2022). R: A language and environment for statistica computing. R Foundation for Statistical Computing, Vienna, Austria. Available at: https://www.R-project.org/
Reynolds, P., Frochot, H., and Reynolds, P. E. (2003). Photosynthetic acclimation of beech seedlings to full sunlight following a major windstorm event in France. Ann. For. Sci. 60, 701–709. doi: 10.1051/forest:2003064
Robson, T. M., Rasztovits, E., Aphalo, P. J., Alia, R., and Aranda, I. (2013). Flushing phenology and fitness of European beech (Fagus sylvatica L.) provenances from a trial in La Rioja, Spain, segregate according to their climate of origin. Agric. For. Meteorol. 180, 76–85. doi: 10.1016/j.agrformet.2013.05.008
Robson, T. M., Sánchez-Gómez, D., Cano, F. J., and Aranda, I. (2012). Variation in functional leaf traits among beech provenances during a Spanish summer reflects the differences in their origin. Tree Genet. Genomes 8, 1111–1121. doi: 10.1007/s11295-012-0496-5
Schieber, B., Janík, R., and Snopková, Z. (2013). Phenology of common beech (Fagus sylvatica L.) along the altitudinal gradient in Slovak Republic (inner Western Carpathians). J. For. Sci. (Prague) 59, 176–184. doi: 10.17221/82/2012-JFS
Slovíková, K., and Bednářová, E. (2014). Monitoring of vegetative phenological stages in European beech (Fagus sylvatica l.) growing in a mixed stand. Acta Univ. Agric. Silvic. Mendel. Brun. 62, 1109–1115. doi: 10.11118/actaun201462051109
Strasser, R. J., Tsimilli-Michael, M., Qiang, S., and Goltsev, V. (2010). Simultaneous in vivo recording of prompt and delayed fluorescence and 820-nm reflection changes during drying and after rehydration of the resurrection plant Haberlea rhodopensis. Biochim. Biophys. Acta 1797, 1313–1326. doi: 10.1016/j.bbabio.2010.03.008
Strasser, R. J., Tsimilli-Michael, M., and Srivastava, A. (2004). “Analysis of the chlorophyll a fluorescence transient” in Chlorophyll a fluorescence: A signature of photosynthesis. eds. G. C. Papageorgiou and Govindjee (Dordrecht: Springer Netherlands), 321–362.
Vitasse, Y., Delzon, S., Dufrêne, E., Pontailler, J. Y., Louvet, J. M., Kremer, A., et al. (2009). Leaf phenology sensitivity to temperature in European trees: do within-species populations exhibit similar responses? Agric. For. Meteorol. 149, 735–744. doi: 10.1016/j.agrformet.2008.10.019
Keywords: OJIP transient, photosynthesis, common garden, beech refugia, phenology
Citation: Provazník D, Stejskal J, Hansen OK, Čepl J, Erichsen ER, Hansen JK, Zádrapová D and Tomášková I (2024) Addressing the altitudinal and geographical gradient in European beech via photosynthetic parameters: a case study on Calabrian beech transplanted to Denmark. Front. For. Glob. Change. 7:1369464. doi: 10.3389/ffgc.2024.1369464
Edited by:
Martin D. Venturas, Universidad Politécnica de Madrid, SpainReviewed by:
Jianfeng Liu, Chinese Academy of Forestry, ChinaRoman Gebauer, Mendel University in Brno, Czechia
Copyright © 2024 Provazník, Stejskal, Hansen, Čepl, Erichsen, Hansen, Zádrapová and Tomášková. This is an open-access article distributed under the terms of the Creative Commons Attribution License (CC BY). The use, distribution or reproduction in other forums is permitted, provided the original author(s) and the copyright owner(s) are credited and that the original publication in this journal is cited, in accordance with accepted academic practice. No use, distribution or reproduction is permitted which does not comply with these terms.
*Correspondence: Daniel Provazník, provaznik@fld.czu.cz