- 1College of Desert Control Science and Engineering, Inner Mongolia Agricultural University, Hohhot, China
- 2Inner Mongolia Forestry Research Institute, Hohhot, China
- 3College of Forestry, Inner Mongolia Agricultural University, Hohhot, China
The Genhe River Basin is an ecological barrier and water conservation area in northern China, but its hydrological process has undergone significant changes due to climate change and human activities, endangering ecosystem functions and water resource security. Systematic research on the influencing mechanisms and laws of hydrological processes in different ecosystems in this region remains lacking. Therefore, this study analyzed the effects of different anthropogenic factors on the hydrological processes of typical ecosystems in the Genhe River Basin. The Soil and Water Assessment Tool distributed hydrological model was used to simulate the surface runoff, evapotranspiration, and soil water content of the three ecosystems of forest, grassland, and farmland in four different periods of 1980, 1990, 2000, and 2010. The spatial and temporal changes in water resources in typical ecosystems under the influence of historical climate change were demonstrated. Results showed that under different land use scenarios, the surface runoff of the farmland ecosystem increased, the evapotranspiration remained unchanged, and the soil water content decreased. The surface runoff of forest and grassland ecosystems did not change significantly, the evapotranspiration increased, and the soil water content decreased. This study reveals the influence of different human factors on the hydrological processes of typical ecosystems in the Genhe River Basin and provides a scientific basis for water resources management and ecological protection in the region.
1 Introduction
Global climate change and human activities have caused great impacts on the structure and functioning of ecosystems, as well as negative consequences for human life and the land surface (Rehana and Mujumdar, 2011; Hu et al., 2015; Ostad-Ali-Askari et al., 2019). The hydrological cycle is one of the most important processes in the Earth’s surface system, which determines the distribution and exchange of water and energy in terrestrial ecosystems (He et al., 2013; Xiao-meng, 2013; Zhou, 2018; Liu et al., 2019). Moisture in nature is transformed into each other through different forms, and the water cycle that occurs on land or within a watershed is a complex process of precipitation-surface and subsurface runoff-evaporation (Margulis et al., 2006; Tang, 2020). The hydrological cycle shows great variability and complexity in different regions and different ecosystems, especially in the cold-temperate region, where there are significant differences in water cycle parameters (e.g., evapotranspiration, surface runoff, and soil water content) in different ecosystems (Su et al., 2016; Liu et al., 2019), which not only reflect the ecosystem vegetation growth status and water income and expenditure, but also reflect the impacts of climate change and human activities on ecosystems, and are influenced by factors such as precipitation, solar radiation, air temperature, vegetation and soil texture (Panigrahy et al., 2014; Ling et al., 2016).
At present, hydrological models commonly used by scholars include the following three categories: (1) Conceptual hydrological models are easy to understand and operate, can reflect the main hydrological characteristics of the watershed, and are suitable for long-term or seasonal hydrological prediction. However, they cannot describe the physical mechanisms of hydrological processes, the physical meanings of the parameters are not clear, the applicability and portability are limited, and the parameter rates and validation for different watersheds require a lot of work (Sun et al., 2023). Commonly used conceptual hydrological models include TOPMODEL (Deng et al., 2022; Januário et al., 2022), HBV (Burhan et al., 2020; Seibert and Bergström, 2022), XAJ (Shi et al., 2011; Chen et al., 2019), and so on. (2) Physical hydrological models are able to describe the physical mechanisms of hydrological processes, the physical meaning of parameters is clear, the applicability and portability of the model is strong, and it is suitable for short-term or event-based hydrological prediction. However, they are difficult to understand and operate, require a large amount of computational resources, are difficult to obtain and calibrate parameters, and the stability and accuracy of the model are affected by numerical methods and boundary conditions. Commonly used physical hydrological models are MIKE SHE (Aredo et al., 2021; Zhang et al., 2021), WASH123D (Richards et al., 2005; Hussain et al., 2022), SHETRAN (Op de Hipt et al., 2019; Đukić and Erić, 2021), etc. (3) Statistical hydrological models have a simple structure, a small number of parameters, and high computational efficiency, and are suitable for watersheds with good data quality or regularity. However, the physical meaning of the model is not clear, the applicability and portability of the model is poor, the quality and quantity of data is demanding, and it cannot adapt to the changes and abnormalities of the watershed. Commonly used statistical hydrological models are ARIMA (Wang et al., 2015; Dimri et al., 2020), ANN (Kouadri et al., 2021; Elbeltagi et al., 2022), etc.
The SWAT (Soil and Water Assessment Tool) model is a distributed, long-term, continuous watershed hydrologic model that is primarily used to assess the long-term impacts of watershed hydrology, water quality, agriculture, and chemicals, as well as the effectiveness of management practices (Arnold et al., 2012; Abbaspour et al., 2015; Aawar and Khare, 2020). The SWAT model is able to simulate a wide range of hydrological-physical-chemical processes such as water quantity, water quality, sediment, nitrogen-phosphorus, pesticides, etc., taking into account multiple factors such as soils, land use, meteorology, and management of the watershed, and is suitable for long-term simulation of hydrological and related processes, and is an effective tool for the current study of hydrological and other issues (Golmohammadi et al., 2014; Ostad Ali Askari, 2022). Du evaluated the accuracy of CFSR, CMADS, and OBS in runoff simulation using SWAT model combined with spatial interpolation technique and configured different scenarios to analyze the effects of climate and land use changes on runoff in the King River Basin from 1999–2018 using PLUS model. The results showed that the CMADS+SWAT model outperformed the CFSR+SWAT model, which underestimated peak runoff. Precipitation changes had a greater effect on runoff than temperature changes, agricultural land increased runoff, and forests had a stronger retention effect (Du et al., 2023). Li simulated the runoff in the Jiyun River Basin with the improved SWAT model, analyzed its relationship with land use changes and human activities, and revealed the process of reclassifying water in different ecosystems of the Jiyun River Basin. Climate change and land use change were found to have significant impacts on runoff in the Ji-Canal River Basin, with climate change having a greater impact (Li et al., 2023). Oo used the SWAT model to analyze the impacts of climate change on the runoff response of the Upper Ayeyarwady River Basin in Myanmar, which provides a reference for the management of water resources in the region. The results showed that future climate change will lead to a decrease in the mean annual runoff in the basin, an increase in low flows in the dry season and a decrease in high flows in the wet season. Meaning that the basin will be exposed to more water scarcity and drought problems, as well as more risk of floods and water-related disasters. It is recommended to consider the impacts of climate change in water resources management to improve water use efficiency and reduce water stress (Oo et al., 2020). Guiamel conducted a watershed simulation of the Mindanao River Basin in the Philippines using the SWAT model to provide a basis for water resources management for potential hydropower development in the region. Due to the lack of precipitation data in the Mindanao River Basin, the precipitation record was examined by comparing the observed data with globally gridded precipitation data (NCDC-CPC and GPCC) (Guiamel and Lee, 2020). In conclusion, the SWAT model can better reflect the complexity and heterogeneity of a watershed by considering the spatial variability of different land use types, soil types, and slope classes within the watershed, and by dividing the watershed into multiple hydrologic response units compared to other models (Bieger et al., 2017; Akoko et al., 2021).
The Genhe watershed is located in the Daxinganling region, which belongs to China’s cold-temperate bright coniferous forest area, and distributes a large number of seasonal permafrost and permafrost layers, which are more sensitive to the response of climate. The Genhe watershed is a typical river valley wetland ecosystem with rich biodiversity and ecological services. Forest, grassland and farmland are the three most dominant ecosystem types in the Genhe Basin, and they play a crucial role in the hydrological cycle. Studying the spatial and temporal changes of water resources in the Genhe River Basin can not only reflect the health of the wetland ecosystem and change trends, but also provide a reference basis for wetland protection and restoration (Li et al., 2008; Sang et al., 2014). In addition, water resources in the Genhe River Basin are also affected by climate change and human activities, which may lead to changes in water quantity and quality, affecting the balance between supply and demand of water resources within and outside the basin and water ecological security (Vörösmarty and Sahagian, 2000; Foley et al., 2005; Foley et al., 2011). It can also identify the vulnerability and adaptability of water resources and provide a supportive basis for the optimal allocation of water resources and risk prevention (Chase et al., 2000; Lambin and Geist, 2008; Chen et al., 2022). Therefore, based on the SWAT model, this study analyzed the water cycling parameters of different ecosystems in the Genhe River Basin, and analyzed their relationship with land use change and human activities, revealing the water reclassification process of different ecosystems in the Genhe River Basin. This study focuses on the characteristics and mechanisms of water cycling in the region and its relationship with climate change and human activities.
2 Materials and methods
2.1 Site description
The Genhe River Basin is a tributary of the Erguna River, located in the northeast of Inner Mongolia Autonomous Region. The watershed area is 15,837 km2, and the soil types are common leaching soil, common gray forest soil and common chernozem. The Genhe River Basin is located in the cold temperate continental monsoon climate zone, with a large number of permafrost and seasonal frozen soil. In the past 40 years, the annual average temperature was −4.9°C, the extreme maximum temperature was 39.1°C (2010), the extreme minimum temperature was −49.6°C (2001), and the annual average precipitation was 411.75 mm. The average annual temperature and precipitation are the highest and lowest in July and January. The frost-free period is 80–90 days, and the annual average wind speed is 2.1 m/s (Figure 1).
The typical ecosystems in the Genhe River Basin mainly include three types: forest, grassland, and farmland. The forest is mainly distributed in the western and northern mountainous areas of the Genhe River Basin, and the main types are temperate deciduous broad-leaved forest and coniferous and broad-leaved mixed forest. The forest ecosystem has high water conservation capacity and biodiversity, and is an important ecological protection area in the basin. Grassland is mainly distributed in the central and eastern plains and hilly areas of the Genhe River Basin, and the main types are typical steppe and desert steppe. Grassland ecosystem has strong soil and water conservation capacity and animal husbandry production capacity, which is an important economic development area of the basin. Farmland is mainly distributed in the eastern plains of the Genhe River Basin. The main types are dry land and irrigated land. The farmland ecosystem has high grain production capacity and water consumption capacity, and is an important grain supply area in the basin. There are hydrological links and ecological interactions among forest, grassland and farmland ecosystems in the Genhe River Basin, which together constitute the water resources cycle and ecological balance of the basin.
2.2 Data sources
In this study, the SWAT model was used to simulate the hydrological process of the Genhe River Basin, and the following data were collected:
Daily-scale meteorological data (including precipitation, temperature, humidity, wind speed, and radiation data) of national meteorological stations, in the basin from 1980 to 2017 were derived from the digital elevation model (DEM) data of the China Meteorological Data Network.1
With a spatial resolution of 30 m, data were derived from the spatial data geographic cloud,2 which was based on the data of the first version (V1) of ASTER GDEM.
The raster data of land use types in 1980, 1990, 2000, and 2010 were from the Resource and Environmental Science Center of the Chinese Academy of Sciences.3 The data set was generated by manual visual interpretation based on Landsat TM/ETM remote sensing images of each period, and the spatial resolution was 1 km.
The Chinese soil data set comprised data from the world soil database (HWSD); the database included China’s data source for the second national land survey of Nanjing soil provided by 1:1 million soil data; the data format was grid; the projection for WGS84, using the soil classification system, was mainly FAO-90, which was acquired from the cold and arid areas of the scientific data center.4
2.3 Research method
In this study, three typical ecosystems, namely, forest, grassland, and farmland, were divided in accordance with the land use type map and the field survey data of the basin. The SWAT distributed hydrological model and the land use type map of the four periods (1980LUCC, 1990LUCC, 2000LUCC, 2010LUCC) were used to study the spatial and temporal variation characteristics of water resources under the influence of human activities in the Genhe River Basin.
2.3.1 Establishment of SWAT model
The establishment of SWAT model requires the following data: DEM map, spatial data of land use type, spatial data of soil type, attribute data, and climate generator. DEM data can be utilized for extracting basin water system data, soil attribute data for establishing a soil type database, remote sensing monitoring data for creating a land use database, and the measured meteorological data of the basin meteorological station for developing the basin meteorological database. The soil database and land use database were established in combination with ARCGIS and SPAW software. Some parameters of the basin meteorological database were calculated using SWAT Weather software to improve the calculation speed and accuracy.
In this study, the data were input into the model to simulate the monthly runoff value in 1980, and the sub-basin output file (SUB), the main channel output file (RCH), and the hydrological response unit (HRU) output file were output for parameter sensitivity analysis and model calibration. The SWAT-CUP program was used to analyze and verify the parameter sensitivity of the model. The program is a public program that connects the maximum likelihood method (GLUE), SUFI-2 optimization algorithm (SUFI2), MCMC, ParaSol, and SWAT and can be used freely. This program can complete the parameter sensitivity analysis, calibration, verification, and uncertainty analysis of the SWAT model. After selection, this study used the SUFI2 method to obtain the parameters with greater sensitivity in the study area, and the model was calibrated and verified. The measured data of the watershed outlet hydrological station with relatively complete and accurate time series data were selected. The model preheating period from 1990 to 1994 was selected, the model calibration period was 1995 to 2009, and the model validation period was 2012 to 2016.
2.3.2 Statistical methods for model output data
(1) Mann–Kendall (M–K) nonparametric trend test.
In this study, the M–K trend test was used to assess the trend of hydrometeorology. It is a nonparametric test method, which does not need to assume that the data obey a specific distribution, and is suitable for small sample and non-normal distribution data. The null hypothesis H0 of the M–K test is time series data (x1, x2, …, xn), with N samples having independent random distribution and no trend; the alternative hypothesis H1 is a two-sided test, and the time series data have an upward or downward trend. The calculation formula of the nonstandardized test statistic Z of the M–K test is as follows:
where S is a sign function. When (xi − xj) is less than, equal to, and greater than zero, S is equal to −1, 0, and 1, respectively. S obeys a normal distribution, and the mean value is 0.
where n is the number of data points.
where Z represents the trend of change; a positive value indicates an upward trend, and a negative value indicates a downward trend. If the calculated absolute Z statistic is greater than the critical value of Z statistic (1.28, 1.64, 2.32) in the normal distribution table, the trend passes the significance level test of 90, 95, and 99%, respectively.
(2) Sen’s estimator (S-E) test.
To estimate the true slope of the existing trend, expressed by Q (as an annual change), Sen’s nonparametric method was used. The Sen method can be employed to estimate the magnitude of N pairs of data when the trend is assumed to be linear. Qi can be calculated using the following formula:
where xj and xk denote the values of j and k (j > k) at a certain time, respectively. The driving value of the N value of the S-E amplitude change Qi is equal to the median. If N is an odd number, then the amplitude calculation formula of S-E is
If N is even, then the formula for calculating the amplitude of S-E is
Qmed is a nonparametric two-tailed test under a confidence interval.
3 Result
The data of surface runoff, evapotranspiration, and soil water content were derived from the simulation results of the SWAT model. In this study, the basin was divided into 28 sub-basins, which were further divided into 1,356 HRUs, and the runoff of the basin was simulated. On the basis of SWAT’s built-in sensitivity analysis tool, 13 sensitive parameters were determined, calibrated, and verified using hydrological data from 1985 to 2017. The correlation coefficient (R2) between simulated and measured runoff was 0.82, and the efficiency coefficient (ENS) of the model was 0.80. The R2 and ENS in the validation period were 0.88 and 0.86, respectively. The SWAT model is suitable for hydrological simulation in the Genhe River Basin. In the map of this study, (A) are all land use types in 1980, (B) are all land use types in 1990, (C) are all land use types in 2000, and (D) are all land use types in 2010.
3.1 Spatial and temporal changes in surface runoff in typical ecosystems under different scenarios
To analyze the impact of different human factors on surface runoff, we selected four periods of land use types as representatives. Table 1 shows the surface runoff changes of forest ecosystems under various land use types from 1980 to 2017. Figure 2 depicts the change in total annual surface runoff of the forest ecosystem in the Genhe River Basin under different land use types. The results showed an insignificant change trend of surface runoff in the forest ecosystem. Except for the scenario of land use type in 2000, the other three scenarios reached the highest value in 2013, which were 23,600, 24,600, and 27,000 mm. We simulated the surface runoff under four land use types by using time change instead of spatial change. The simulation results showed that from 1980 to 2017, the largest total surface runoff belonged to the land use type scenario in 2000, which was 5,471,400 mm, followed by those in 2010 and 1980, which were 160,600 and 142,100 mm, respectively. The smallest total surface runoff was also from the land use type scenario in 1990, which was 131,100 mm.
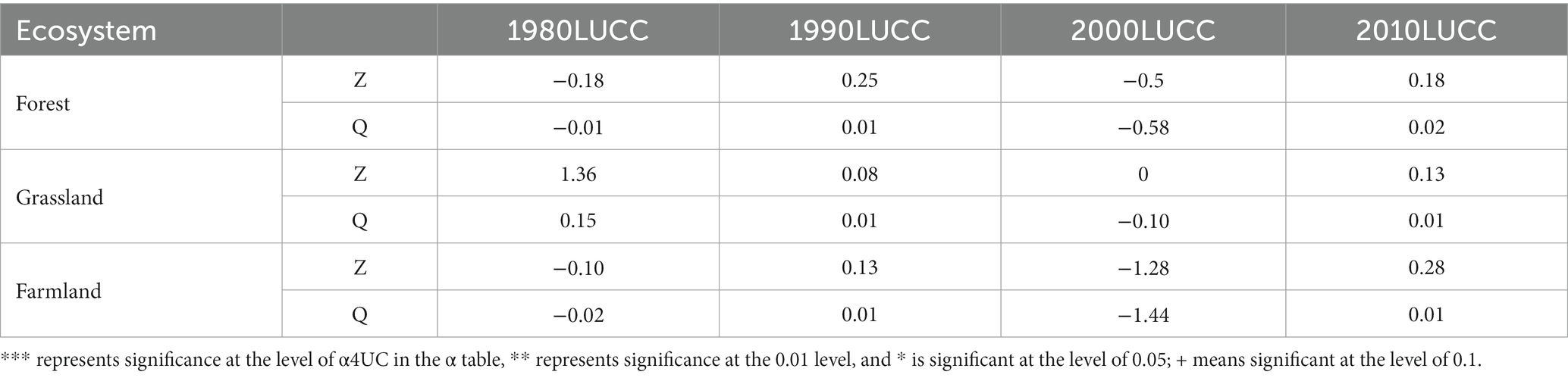
Table 1. Surface runoff of typical ecosystems in the Genhe River Basin under different land use (mm).
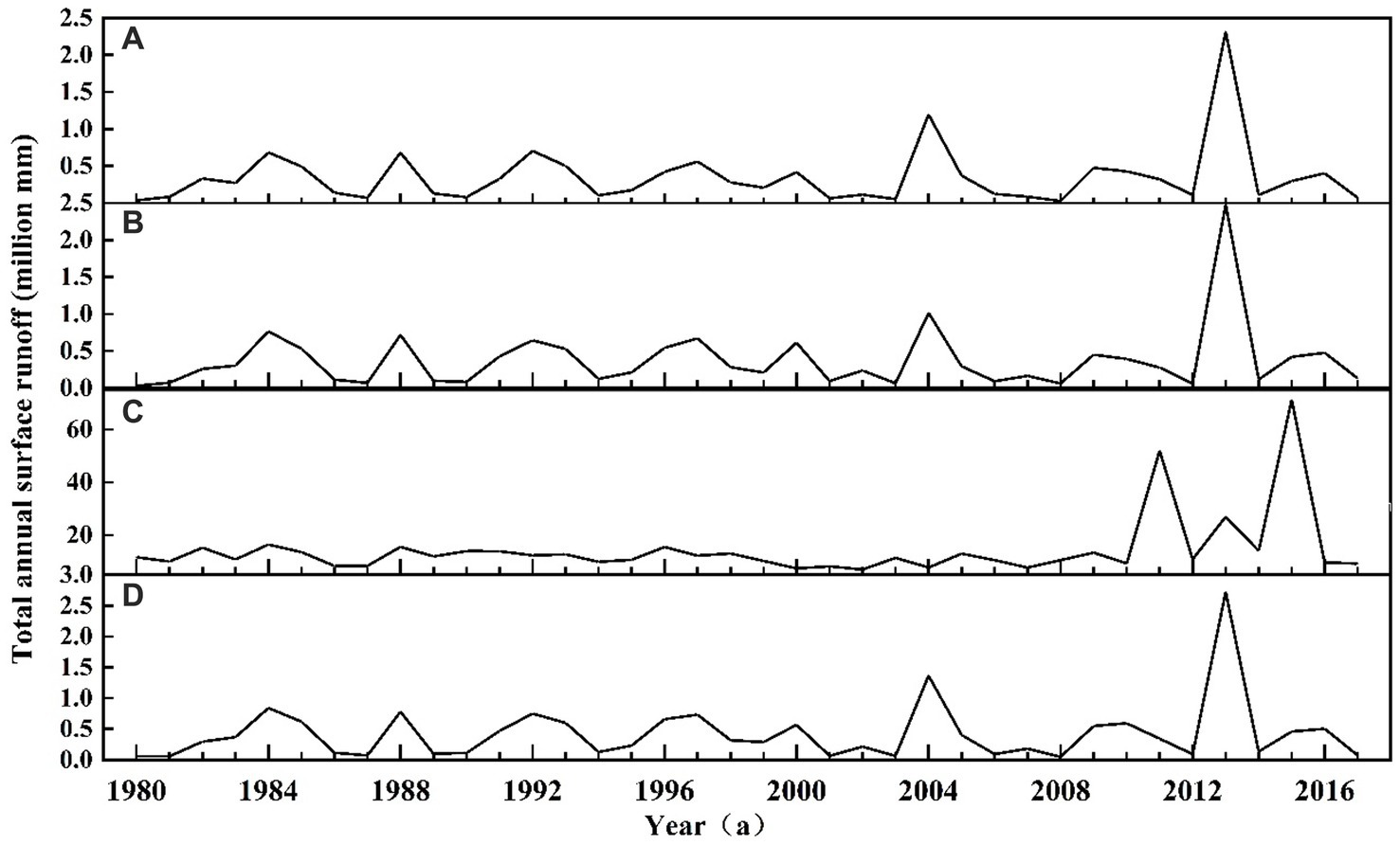
Figure 2. Surface runoff of the forest ecosystem under the influence of different human activities (mm).
Table 1 and Figure 3 show the surface runoff of grassland ecosystems under four land use types in four periods from 1980 to 2017. The results indicated an insignificant change in the surface runoff of the grassland ecosystem during the whole period, but it showed an upward trend under the four scenarios. Specifically, the total surface runoff under the land use scenario in 1980 increased the fastest, with a rate of 0.15 mm/a. Similar to the forest ecosystem, the grassland ecosystem reached the highest value in 2013 under all three scenarios, except the land use scenario in 2000, which were 44,100, 25,500, and 275,000 mm. We compared the total surface runoff under the four land use scenarios from 1980 to 2017 and found that the order was the same as that of the forest ecosystem, that is, 2000 > 1980 > 2010 > 1990, with specific values of 6,158,000, 300,500, 162,300, and 1,391,000 mm, respectively.
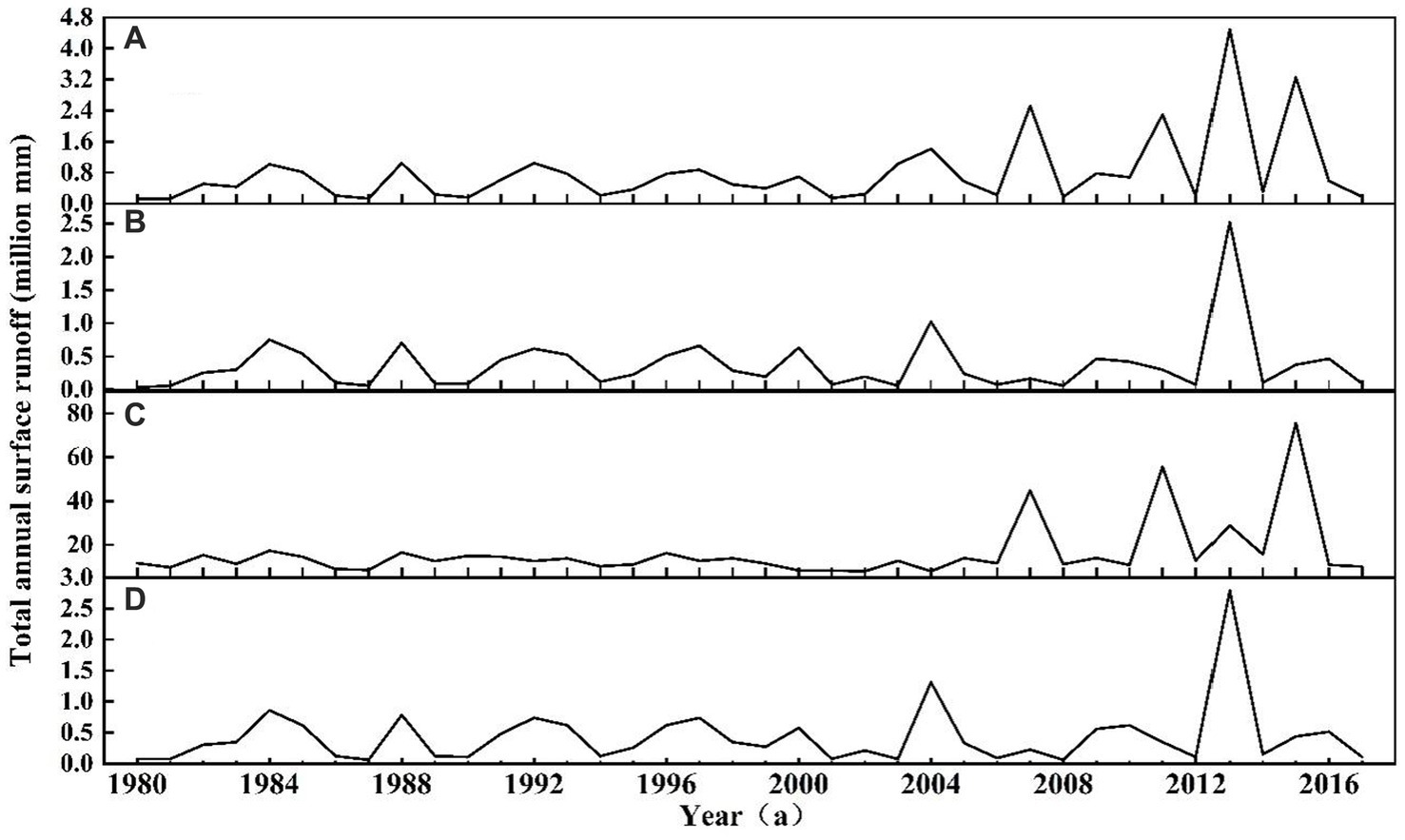
Figure 3. Surface runoff (mm) of the grassland ecosystem under the influence of different human activities.
Table 1 and Figure 4 present the surface runoff of farmland ecosystems under four land use types in four periods from 1980 to 2017. The results showed that the surface runoff of the farmland ecosystem had no significant change trend during the whole period, but the highest values appeared in 2013 under all scenarios, which were 13,800, 84,000, 122,300, and 88,000 mm. Compared with those of the forest and grassland ecosystems, the surface runoff of the farmland ecosystem exhibited a larger interannual variation, which might be related to agricultural activities and precipitation distribution. We compared the total surface runoff under four land use scenarios from 1980 to 2017 and found that the order was the same as that of the forest and grassland ecosystems, that is, 2000 > 1980 > 2010 > 1990, with specific values of 1,975,900, 101,900, 59,200, and 52,400 mm, respectively.
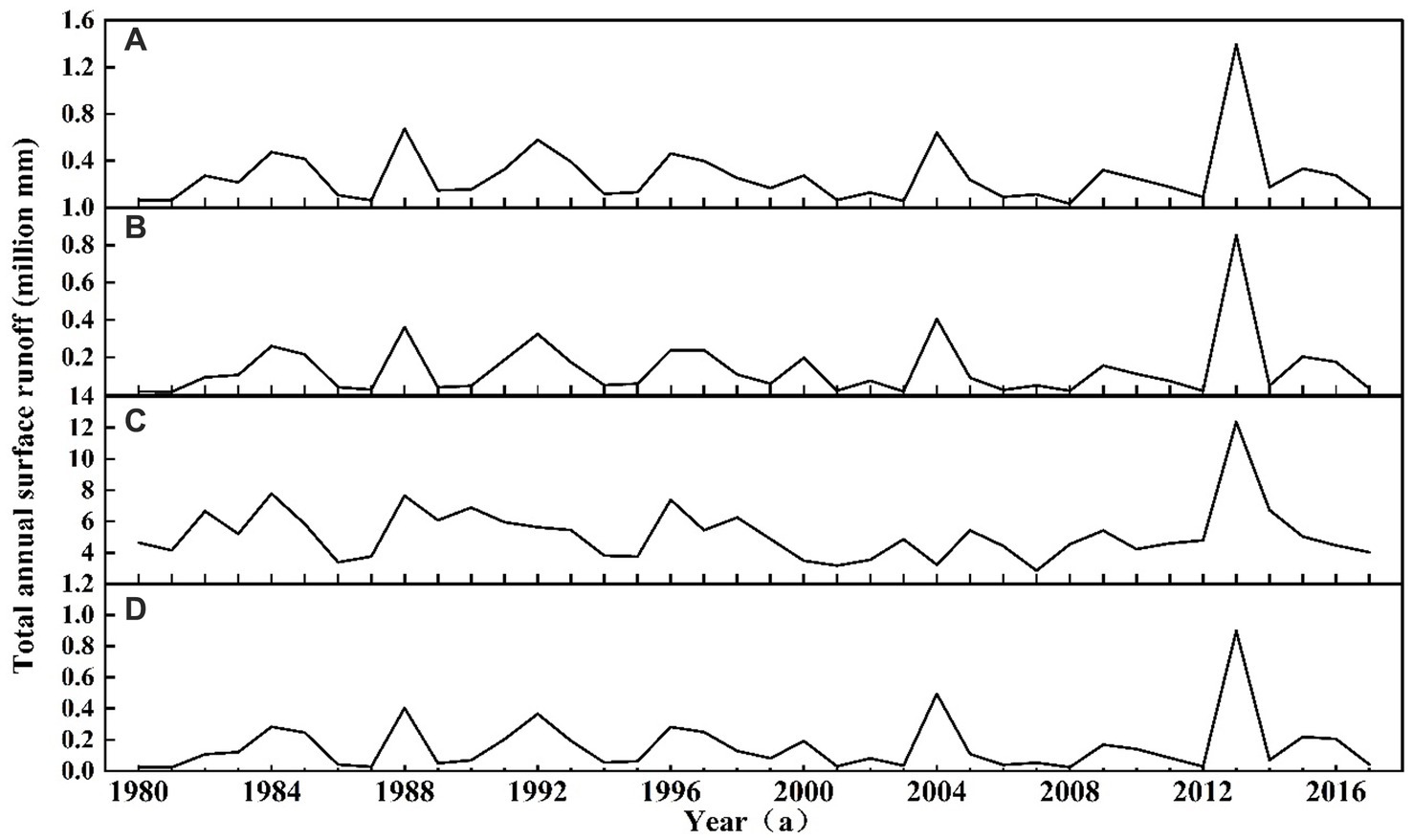
Figure 4. Surface runoff of the farmland ecosystem under the influence of different human activities (mm).
3.2 Spatiotemporal changes in evapotranspiration in typical ecosystems under different scenarios
We also analyzed the changes in evapotranspiration in typical ecosystems in the Genhe River Basin. Table 2 and Figure 5 show the annual evapotranspiration in forest ecosystems under four land use types in four periods from 1980 to 2017. The results showed that the evapotranspiration in the forest ecosystem showed an upward trend under the four scenarios. In particular, the evapotranspiration under the land use scenario in 1980 increased the fastest, with a rate of 0.63 mm/a, and was significant at the level of α = 0.05. From 1980 to 2017, the maximum evapotranspiration under the four land use scenarios appeared at different times. The maximum value under the land use scenario appeared in 2011, which was 352,900 mm, while those for the other three scenarios occurred in 2013, which were 142,400, 157,600, and 159,200 mm. We compared the total evapotranspiration between 1980 and 2017 under the four land use scenarios and determined that the order was 2010 > 1990 > 1980 > 2000, with specific values of 5.1656, 5.1288, 4.8167, and 3.1437 million mm, respectively.
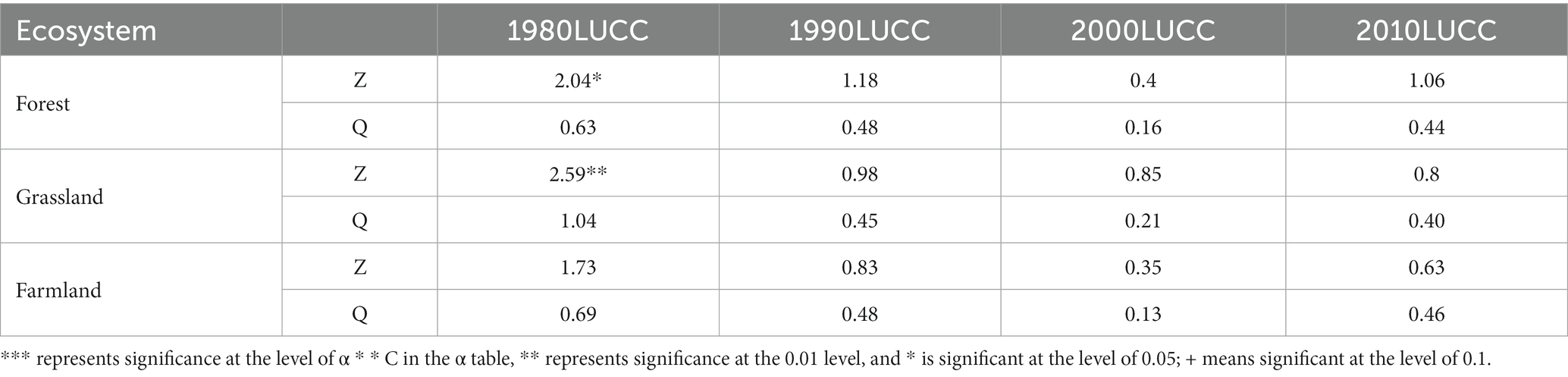
Table 2. Evapotranspiration in typical ecosystems in the Genhe River Basin under different land use types (mm).
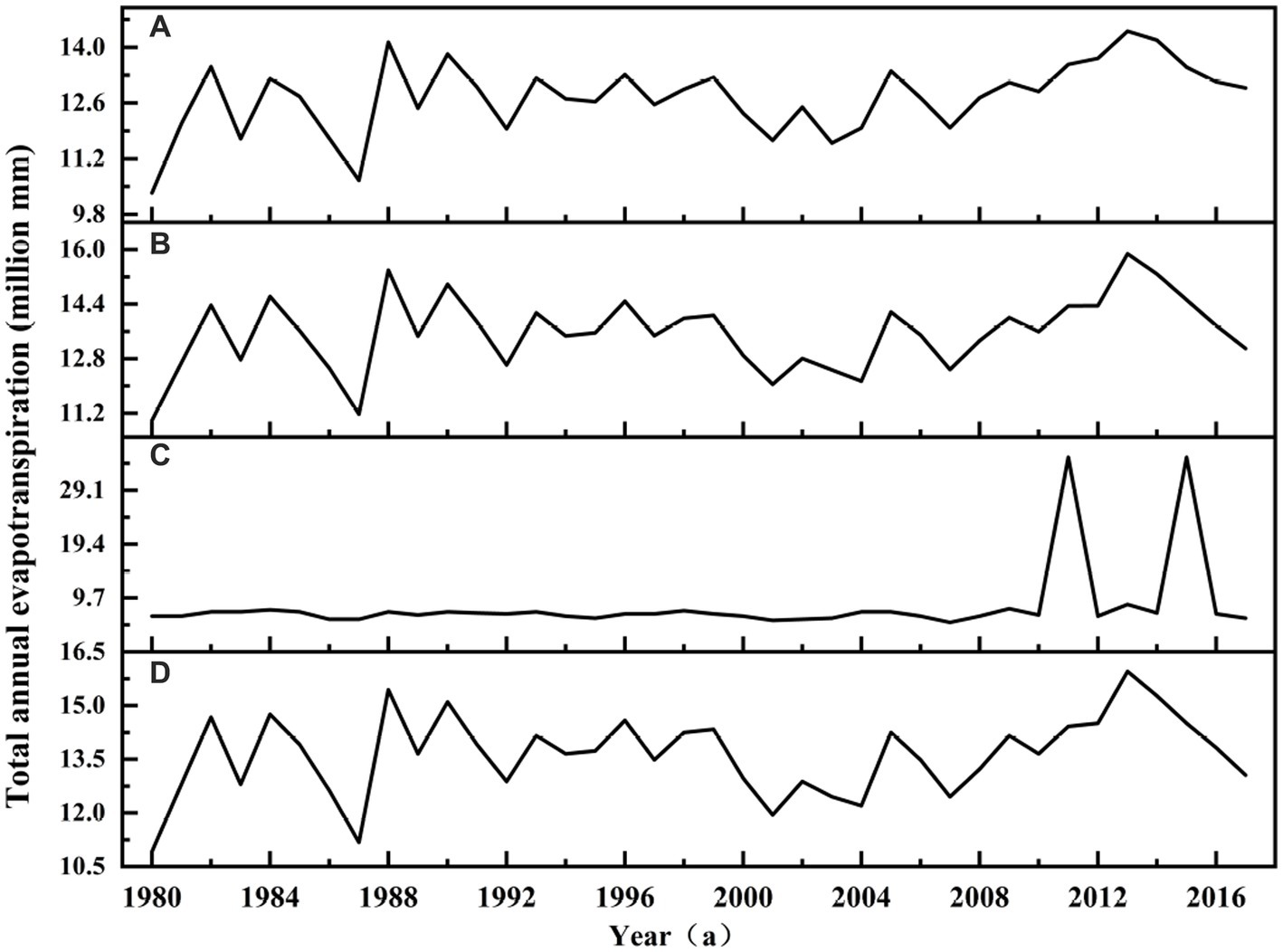
Figure 5. Evapotranspiration in the forest ecosystem under the influence of different human activities (mm).
Table 2 and Figure 6 indicate the annual evapotranspiration in grassland ecosystems under four land use types in four periods from 1980 to 2017. The results showed that the evapotranspiration in the grassland ecosystem showed an upward trend under the four scenarios. The evapotranspiration under the land use scenario in 1980 increased the fastest, with a rate of 1.04 mm/a, and was significant at the level of α = 0.01. We compared the total evapotranspiration between 1980 and 2017 under the four land use scenarios and found that the order was 1980 > 2010 > 1990 > 2000, with specific values of 7.3598, 5.5129, 5.4768, and 3.7427 million mm, respectively.
Based on the data in Table 2 and Figure 7, during the period 1980–2017, the annual evapotranspiration in the farmland ecosystem of the Genhe River Basin did not increase or decrease significantly under the four land use type scenarios. The annual evapotranspiration in these four land use types reached the highest value in 2013, which were 77,100, 85,200, 44,600, and 85,900 mm. Comparison of the total evapotranspiration under these four land use types in the same period showed that the total evapotranspiration under the land use type scenario in 2000 was the largest, reaching 2.647 million mm, followed by that in 1990 (2.5914 million mm) and that in 1980 (2.4589 million mm). By contrast, the total evapotranspiration under the land use type scenario in 2000 was the smallest, only 1.327 million mm.
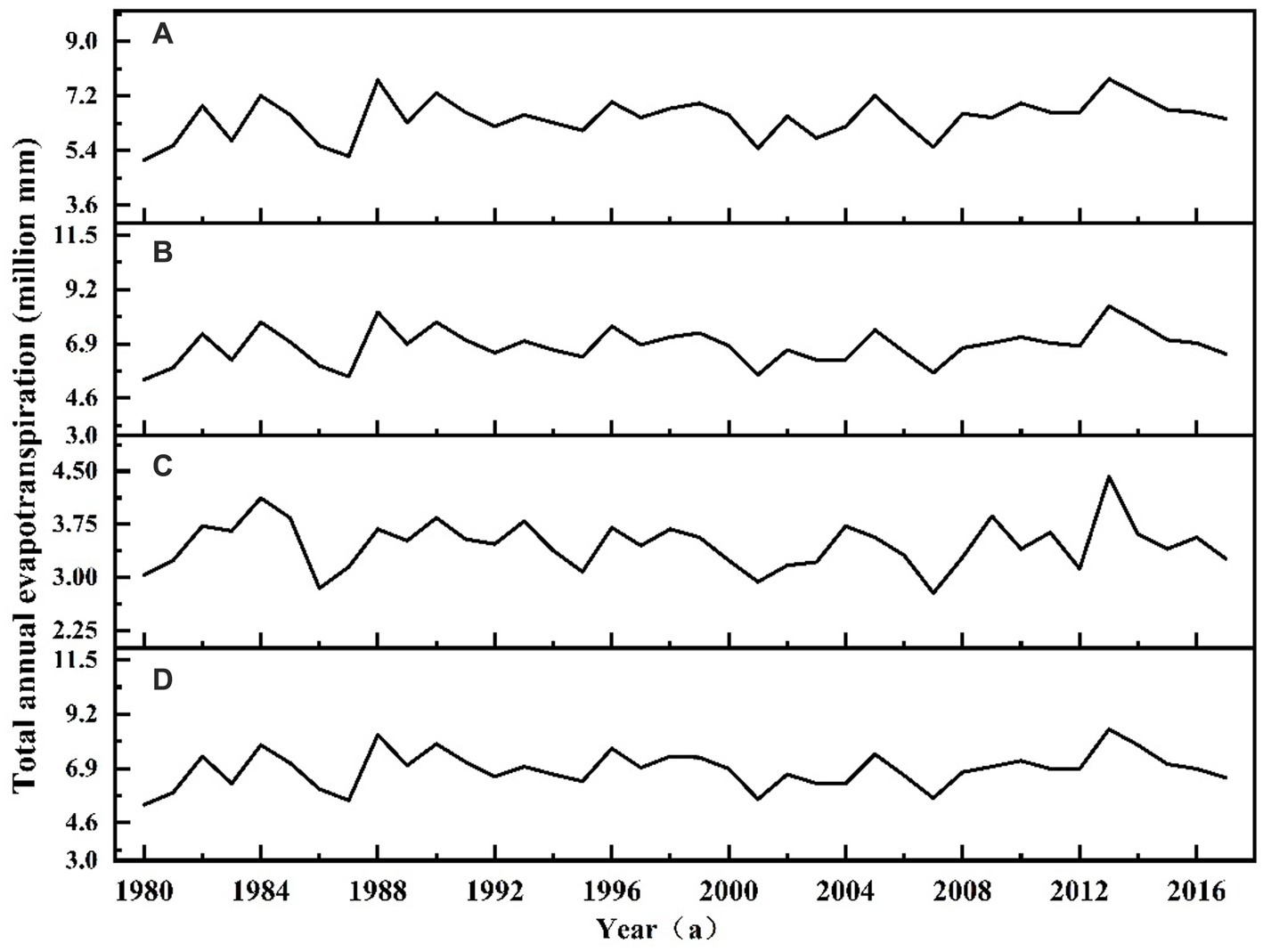
Figure 7. Evapotranspiration in the farmland ecosystem under the influence of different human activities (mm).
3.3 Spatial and temporal changes in soil water content in typical ecosystems under different scenarios
Based on the data in Table 3 and Figure 8, during the period 1980–2017, the soil moisture content in the forest ecosystem of the Genhe River Basin decreased under four land use type scenarios. The soil water content under these four land use types decreased significantly in 1980, 1990, and 2010 by 0.45, 0.49, and 0.52 mm/a, respectively, reaching the significant level of α = 0.05. It reached the highest values in 1985, which were 139.58, 132.74, and 131.45 mm. Comparison of the average soil water content under these four land use types in the same period demonstrated that the average soil water content under the land use type scenario in 1980 was the largest, reaching 113.23 mm, followed by that in 1990 (105.54 mm) and that in 2010 (104.49 mm). On the contrary, the average soil water content under the land use type scenario in 2000 was the smallest, only 14.25 mm.
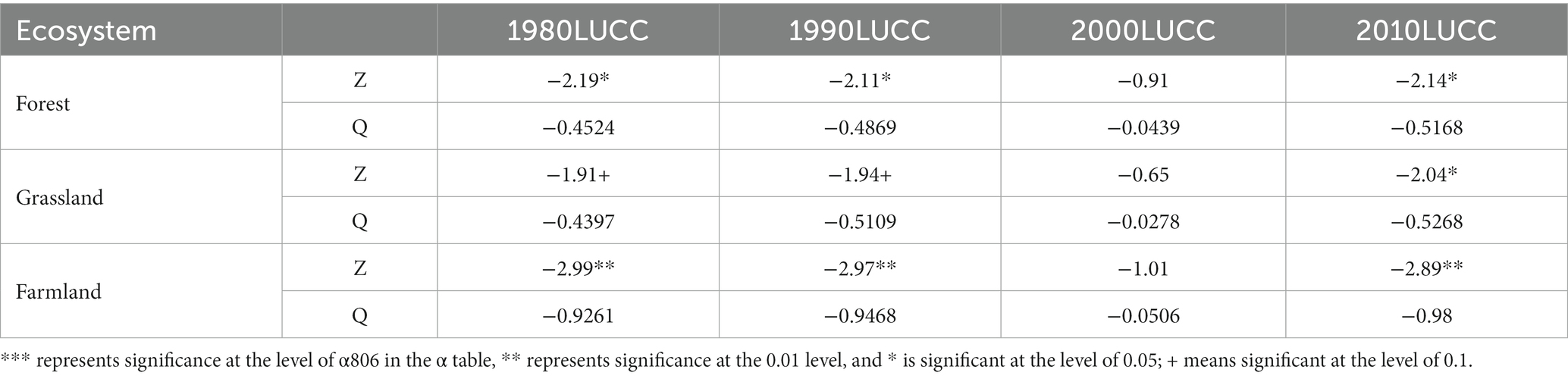
Table 3. Soil water content (mm) of typical ecosystems in the Genhe River Basin under different land use types.
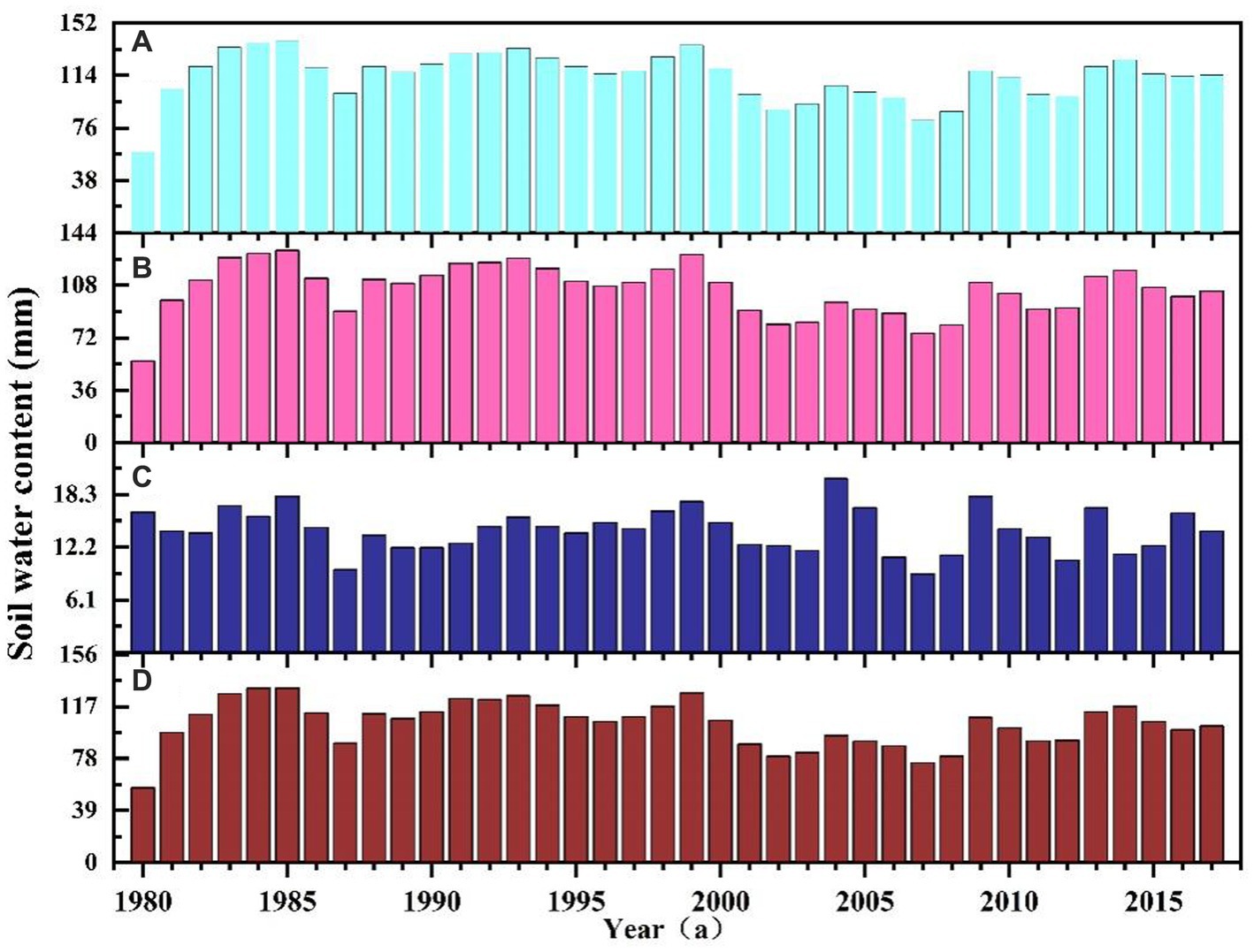
Figure 8. Soil water content (mm) of the forest ecosystem under the influence of different human activities.
The data in Table 3 and Figure 9 indicated that in 1980–2017, the soil moisture content in the grassland ecosystem of the Genhe River Basin decreased under four land use type scenarios. The soil water content decreased significantly in 1980, 1990, and 2010 by 0.44, 0.51, and 0.53 mm/a, respectively, reaching the significant level of α = 0.1. It reached the highest values in 1985, which were 136.23, 131.81, and 130.76 mm. The average soil water content under the land use type scenario in 1980 was the largest, reaching 110.40 mm, followed by that in 1990 (102.60 mm) and that in 2010 (101.78 mm). The smallest average soil water content, only 14.41 mm, belonged to the land use type scenario in 2000.
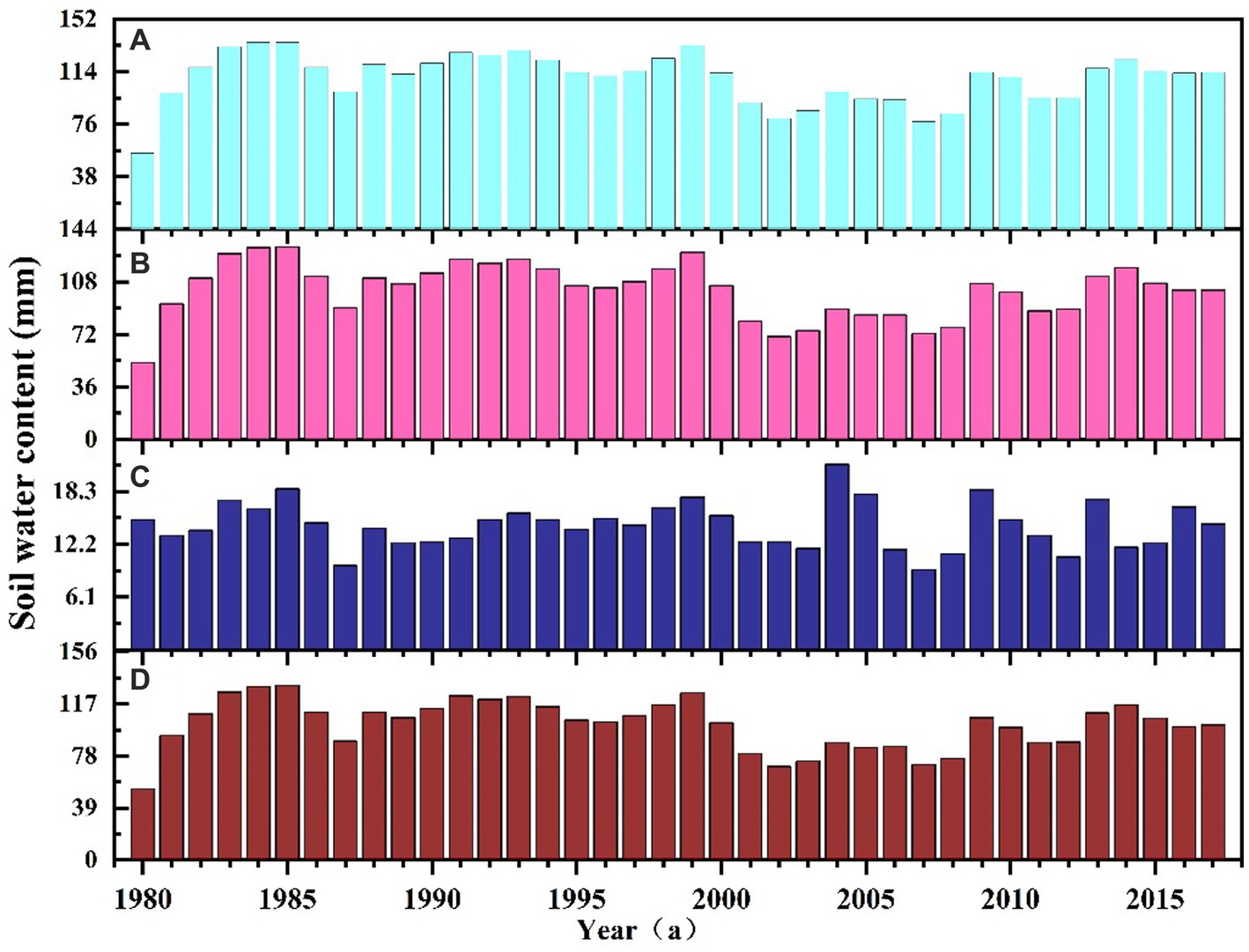
Figure 9. Soil water content (mm) of the grassland ecosystem under the influence of different human activities.
From Table 3 and Figure 10, the soil water content under the four land use types decreased significantly in 1980, 1990, and 2010, with reduction rates of 0.93, 0.95, and 0.98 mm/a, respectively, reaching a significant level of α = 0.01. The highest values were achieved in 1984, which were 128.02, 121.95, and 120.60 mm. The average soil water content under the land use type scenario in 1980 was the largest, reaching 93.37 mm, followed by that in 1990 (85.72 mm) and that in 2010 (83.21 mm). The average soil water content under the land use type scenario in 2000 was the smallest, only 13.20 mm.
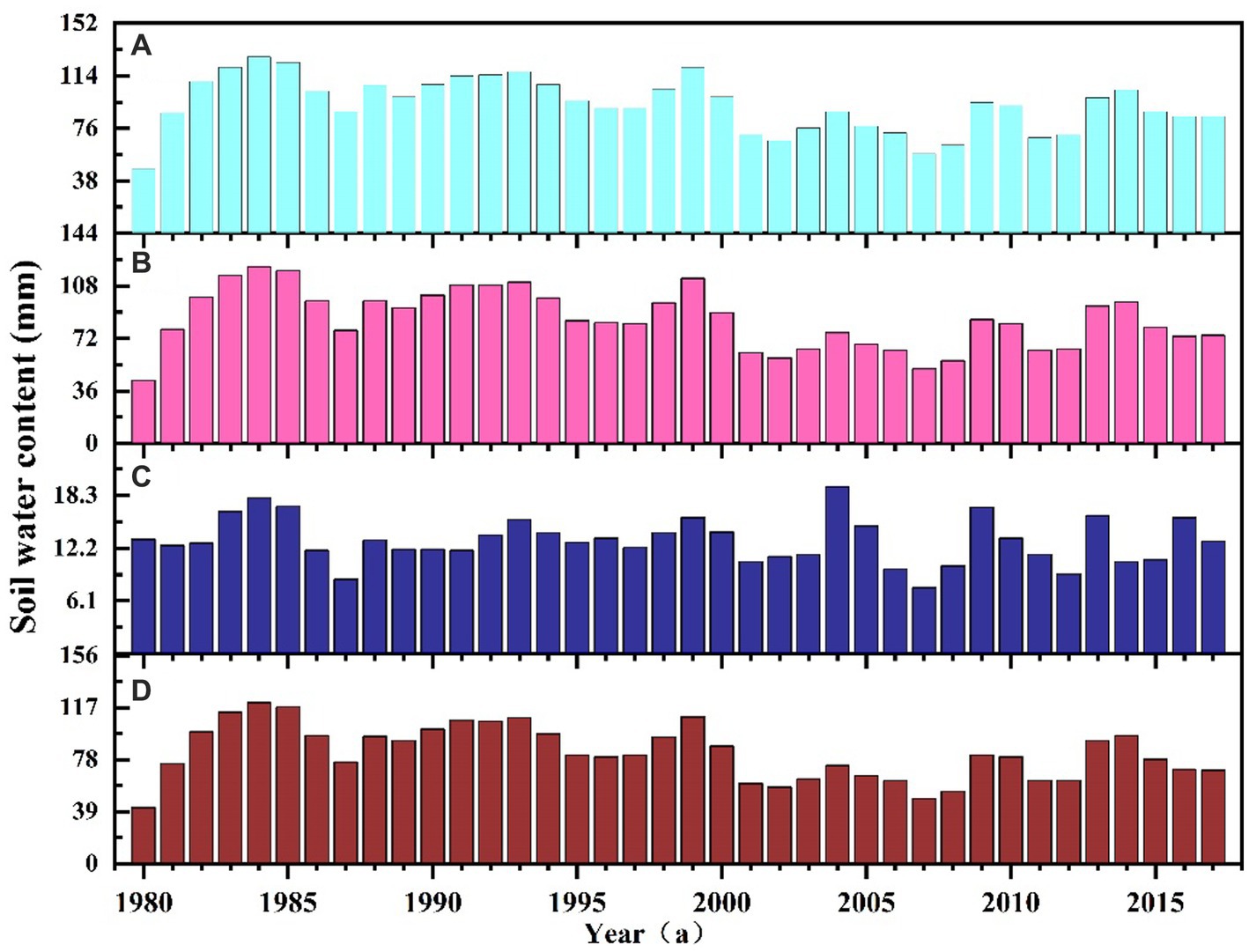
Figure 10. Soil water content (mm) of the farmland ecosystem under the influence of different human activities.
4 Discussion
4.1 Analysis of surface runoff in typical ecosystems
In this study, we simulated the hydrological processes under four different land use type scenarios for the period 1980–2017 in the Genhe River Basin, and analyzed the trends and influencing factors of surface runoff. We found that surface runoff in the Genhe River Basin did not have a significant increasing or decreasing trend during the study period, but showed fluctuating changes, which may be related to the seasonal changes in temperature and precipitation. It is noteworthy that the surface runoff under all four land use type scenarios reached peak levels in 2013, which may be attributed to the higher spring temperatures and precipitation in 2013, which led to an acceleration of the freeze–thaw process in the watershed and increased surface runoff. Further comparison of the ecosystems revealed that forest ecosystems had the highest surface runoff, followed by grassland ecosystems, while agricultural ecosystems had the lowest surface runoff. This difference can be attributed to the difference in the area occupied by the different ecosystems: forest ecosystems occupied the largest area, grassland ecosystems the second largest, and farmland ecosystems the smallest. In addition, this study revealed that surface runoff from the same ecosystems under different land use type scenarios had similar trends, but the total amount was significantly different. This suggests that both climate change and land use type changes have some influence on surface runoff in the Genhe River Basin.
In order to explore more deeply the effects of climate change and land use type changes on surface runoff, we combined data from previous studies (Yue et al., 2020) and in this study (Table 4) to analyze the changes in the area of the three main ecosystems, namely forests, grasslands, and agricultural lands, in the Genhe River Basin in the past few decades. The results of the study showed that the areas of both forests and agricultural lands showed an increasing trend between 1980 and 2017, while the area of grasslands was decreasing and gradually transformed into drylands. These changes in land use types may affect factors such as vegetation cover, vegetation type, and vegetation structure, which may have an impact on hydrological processes. We also analyzed the relationship between the total surface runoff of each ecosystem and the area they occupy. We found that the total amount of surface runoff from forest and grassland ecosystems was positively correlated with the area they occupied: the larger the area, the more surface runoff. This may be due to the fact that forest and grassland ecosystems have higher vegetation cover and stronger retention capacity, which can reduce water evaporation and infiltration and thus increase surface runoff. However, farmland ecosystems did not show this relationship: despite the increase in farmland area, the total surface runoff did not increase significantly. This may be due to the presence of anthropogenic irrigation activities in the agro-ecosystem, which can alter soil moisture conditions and thus affect surface runoff generation (Mehnaza et al., 2022). In summary, surface runoff in the Genhe River Basin is influenced by both climatic and anthropogenic factors, with the combination of climatic conditions and anthropogenic factors leading to the peak in surface runoff in 2013.
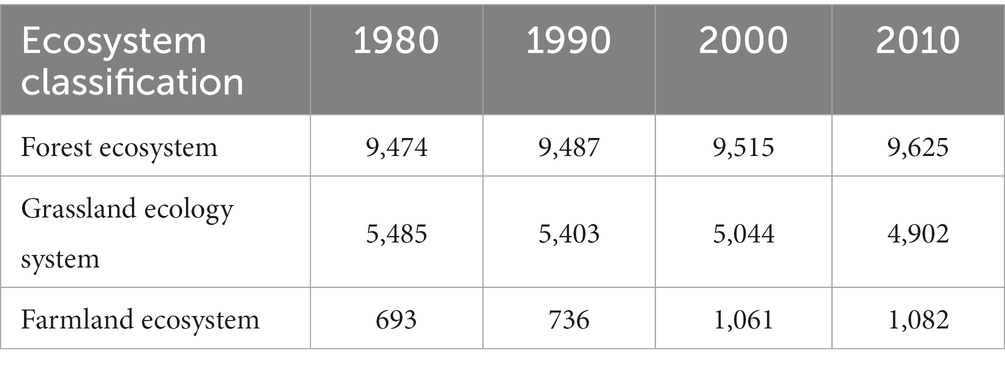
Table 4. Typical ecosystem area of land use types in the Genhe River Basin in 1980, 1990, 2000, and 2010 (km2).
4.2 Evapotranspiration analysis of typical ecosystems
The trend of evapotranspiration in typical ecosystems in the Genhe River Basin was discussed by analyzing the data under four land use scenarios from 1980 to 2017. The evapotranspiration showed an upward trend during the study period. This finding is consistent with the results of Zhang (Zhang et al., 2020) and others in their study of evapotranspiration in the Hulunbeier region from 2008 to 2017. They found that evapotranspiration in the Hulunbeier region increased overall, especially in the northern region. Because the Genhe River Basin is located in the northern part of Hulun Buir, the results of this study can reflect the hydrological changes in the region. In addition, we observed an upward trend in evapotranspiration under different land use types, although the specific values differed. Thus, in addition to climatic factors (e.g., precipitation, relative humidity, and atmospheric temperature), changes in land use types (e.g., changes in the area occupied by each ecosystem and changes in geographical location) also play a significant role in watershed evapotranspiration. In particular, precipitation is one of the factors that directly affect evapotranspiration because an increase in precipitation will lead to sufficient soil moisture, which will promote increased evapotranspiration. With regard to relative humidity, a lower value will lead to an increase in water vapor pressure difference, which in turn increases evapotranspiration. Meanwhile, a high atmospheric temperature will increase the water vapor saturation pressure, thus promoting evapotranspiration increase.
The influence of land use change on evapotranspiration is mainly reflected by factors such as vegetation coverage, type, and structure. High vegetation coverage usually means larger evapotranspiration area and higher evapotranspiration. In the regulation of evapotranspiration, different vegetation types have various physiological characteristics and ecological functions, which affect the absorption, transmission, and release of water. Furthermore, different vegetation structures show diverse morphological characteristics and spatial distribution, which affect the process of water exchange between soil, vegetation, and atmosphere. The data in this study (Table 4) demonstrated that during the period from 1980 to 2017, the area of forests and farmland in the Genhe River Basin increased, while the area of grassland decreased and gradually transformed into dry land. These changes in land use types may alter the vegetation coverage, type, and structure of each ecosystem, thus affecting the evapotranspiration in the basin.
4.3 Analysis of soil water content in typical ecosystems
In this study, the hydrological processes of three typical ecosystems, forest, grassland and farmland, were analyzed in the period of 1980–2017, focusing on the changing law of soil water content, using data from four different land-use scenarios in the Genhe River watershed as the research object. Soil water content is an important indicator reflecting the hydrological condition of a watershed, which determines the distribution and exchange of water and energy in terrestrial ecosystems. However, soil water content shows great variability and complexity in different regions and different ecosystems, and is influenced by a variety of factors.
We found that soil water content in the Genhe watershed showed a significant decreasing trend during the study period, which is consistent with the results of a previous study of soil water content in temperate meadow grasslands in Ewenke Autonomous Banner, Inner Mongolia (Zhang and Lei, 2019). We suggest that this decreasing trend may be related to the degradation of permanent and seasonal permafrost, which is widely distributed within the Genhe River basin. Permafrost in the Daxinganling region is gradually degrading due to climate warming, a phenomenon that affects groundwater and runoff, and thus has far-reaching effects on the soil environment. Our study also found that there were significant differences in soil water content under different land use scenarios, with the soil water content under the 2010 land use scenario being higher than that under the 2000 land use scenario. This may be due to the fact that the 2010 land use scenario is more conducive to maintaining soil moisture. Specifically, the 2010 scenario shows an increase in forest cover, a decrease in grassland, and a decrease in cropland, along with an increase in water area. These changes may affect the vegetation characteristics of individual ecosystems and thus soil water content.
In order to explore the factors affecting soil water content in more depth, we combined data from a previous study (Yue et al., 2020) as well as those from the present study (Table 4) to analyze the changes in the area of the three main ecosystems, namely, forests, grasslands, and agricultural lands, in the Genhe River Basin in the past few decades. The results of the study showed that the areas of both forests and agricultural lands showed an increasing trend between 1980 and 2017, whereas the area of grasslands was decreasing and gradually converted into drylands. These land use changes may affect factors such as vegetation cover, vegetation type, and vegetation structure in each ecosystem, which may have an impact on hydrological processes. We also analyzed the relationship between the soil water content of each ecosystem and the area they occupy. We found that the soil water content of forest and grassland ecosystems is positively correlated with the area they occupy: the larger the area, the higher the soil water content. This may be due to the fact that forest and grassland ecosystems have higher vegetation cover and stronger retention capacity, which can reduce water evaporation and infiltration, thus increasing soil water content (Liang, 2016; Liu, 2019). However, soil water content in farmland ecosystems did not show this relationship: despite the increase in farmland area, soil water content did not increase significantly. This may be due to the presence of anthropogenic irrigation activities in farmland ecosystems, which can change soil moisture conditions and thus affect soil water content (Metcalfe and Buttle, 2001; Chang et al., 2016). In addition, we found that the sudden decrease in total soil water content in the Genhe River Basin in 2000 could be attributed to the following: climate change led to higher temperatures and lower precipitation, which increased evapotranspiration from the watershed and lowered the water balance in the watershed, leading to a decrease in the soil water content (Tong et al., 2020); land-use changes led to a decrease in the area of forests and grasslands, and an agricultural land area increases, which affects the vegetation characteristics of the watershed and reduces the water-holding capacity of the watershed, leading to a decrease in soil water content; and anthropogenic disturbances lead to changes in hydraulic engineering and water resource management, which alter the water quantity and quality of the watershed, affecting the hydrological balance of the watershed and leading to a decrease in soil water content (Oki et al., 2021). The combined effect of these factors may have contributed to the sudden decline in total soil water content in the Genhe River Basin in 2000. In summary, the soil water content in the Genhe River Basin is subject to the combined effects of many factors, such as climate change, land use change and anthropogenic disturbances, and has a certain degree of complexity and uncertainty.
Land use types in the Genhe River Basin have changed significantly over the past few decades, mainly in the form of an increase in the area of agricultural land and a decrease in the area of forests and grasslands. This change may lead to a continued increase in surface runoff and a continued decrease in soil water content in farmland ecosystems, while surface runoff in forest and grassland ecosystems may decrease and soil water content may increase. Climate change in the Genhe River Basin is characterized mainly by increasing temperatures and decreasing precipitation. This change may lead to an increase in evapotranspiration from the watershed, a decrease in soil water content, and a decrease in surface runoff. At the same time, climate change may also affect the freezing and thawing processes in the basin, changing the runoff formation mechanism in the basin and leading to seasonal changes in surface runoff. Human activities in the Genhe River Basin mainly include agricultural production, water conservancy projects, and tourism development. These activities may affect the utilization and management of water resources in the basin, change the water quantity and quality of the basin, and affect the ecosystem service function of the basin. For example, water conservancy projects may regulate the runoff volume of the basin and reduce the risk of floods and droughts, but they may also disrupt the natural hydrological processes of the basin and affect the hydrological balance of the basin. In summary, the future trends of surface runoff, evapotranspiration and soil water content in the Genhe River Basin may be affected by a combination of factors, such as land use type, climate change and human activities, with a certain degree of uncertainty and complexity. Therefore, further research on hydrological modeling and prediction in the basin is needed to provide a scientific basis for the protection and management of water resources in the basin.
5 Conclusion
In this study, the Genhe River Basin was used as the research area, and four different land use types scenarios (1980, 1990, 2000, and 2010) were used to simulate the surface runoff, evapotranspiration and soil water content of each ecosystem. The effects of different human factors on the hydrological processes of typical ecosystems (forest, grassland, farmland) during 1980–2017 were analyzed. The results show that: (1) In terms of surface runoff, there was no significant change trend in forest and farmland ecosystems under different land use types, while grassland ecosystems showed an upward trend. The total amount of surface runoff under the four scenarios is 2000 > 1980 > 2010 > 1990. (2) In terms of evapotranspiration, forest and grassland ecosystems showed an upward trend under different land use types scenarios, among which grassland ecosystems were the most significant, and the fastest increase was under the 1980 scenario, while farmland ecosystems did not change significantly. The sum of evapotranspiration under the four scenarios was 2010 > 1990 > 1980 > 2000. (3) In terms of soil water content, the forest, grassland and farmland ecosystems showed a downward trend under different land use types, among which the farmland ecosystem was the most significant, and the decline was the fastest in the 1980 scenario. The average soil water content under the four scenarios was 1980 > 1990 > 2010 > 2000. In the future, the monitoring and evaluation of different land use types should be strengthened in the Genhe River Basin, and its impact mechanism on hydrological processes should be analyzed. The structure and layout of land use should be optimized to reduce the consumption of water resources by farmland, improve the water conservation capacity of forests and grasslands, and achieve efficient utilization and protection of water resources in the basin.
This study reveals the influence of different human factors on the hydrological processes of typical ecosystems in the Genhe River Basin and provides a scientific basis for water resource management and ecological protection in the region. However, this study also has some limitations, such as not considering the impact of natural factors such as climate change, not analyzing the hydrological links between different ecosystems, and not predicting future scenarios. These problems need to be discussed and addressed in further research.
Data availability statement
The original contributions presented in the study are included in the article/supplementary material, further inquiries can be directed to the corresponding author.
Author contributions
KZ: Methodology, Visualization, Writing – original draft. FQ: Writing – review & editing. YY: Methodology, Writing – review & editing. LoL: Methodology, Supervision, Writing – review & editing. XD: Visualization, Writing – review & editing. LiL: Formal analysis, Writing – review & editing. YL: Formal analysis, Writing – review & editing. YiW: Formal analysis, Writing – review & editing. RH: Data curation, Writing – review & editing. YaW: Software, Writing – review & editing. YX: Methodology, Writing – review & editing. YuW: Software, Writing – review & editing.
Funding
The author(s) declare financial support was received for the research, authorship, and/or publication of this article. This research was funded by the Inner Mongolia Autonomous Region directly under the basic scientific research business costs of colleges and universities “Inner Mongolia Yellow River Basin sandy coarse sand area of forest and grass vegetation quality and efficiency of technological innovation team project” (BR22-13-10); The basic scientific research project of university “Study on the spatial and temporal variation characteristics of hydraulic erosion under different slope vegetation patterns in the coarse sand area of the Yellow River Basin” (BR220109); National Natural Science Foundation of China (31660233) and Inner Mongolia Autonomous Region Science and Technology Major Project (2019ZD0070402).
Acknowledgments
Throughout the writing of this dissertation I have received a great deal of support and assistance. I would first like to thank my supervisor, FQ, whose expertise was invaluable in formulating the research questions and methodology. Your insightful feedback pushed me to sharpen my thinking and brought my work to a higher level. I would particularly like to acknowledge my teammate/group mate/team members, LiL, YL, YiW, YaW, YX, YuW, for their wonderful collaboration and patient support I would also like to thank my tutors, YY, LoL, and XD, for their valuable guidance throughout my studies. You provided me with the tools that I needed to choose the right direction and successfully complete my dissertation. Finally, I could not have completed this dissertation without the support of my girlfriend, RH, who provided care and support to rest my mind outside of my research.
Conflict of interest
The authors declare that the research was conducted in the absence of any commercial or financial relationships that could be construed as a potential conflict of interest.
Publisher’s note
All claims expressed in this article are solely those of the authors and do not necessarily represent those of their affiliated organizations, or those of the publisher, the editors and the reviewers. Any product that may be evaluated in this article, or claim that may be made by its manufacturer, is not guaranteed or endorsed by the publisher.
Footnotes
References
Aawar, T., and Khare, D. (2020). Assessment of climate change impacts on streamflow through hydrological model using SWAT model: a case study of Afghanistan. Model. Earth Syst. Environ. 6, 1427–1437. doi: 10.1007/s40808-020-00759-0
Abbaspour, K. C., Rouholahnejad, E., Vaghefi, S. A., Srinivasan, R., Yang, H., and Kløve, B. (2015). A continental-scale hydrology and water quality model for Europe: calibration and uncertainty of a high-resolution large-scale SWAT model. J. Hydrol. 524, 733–752. doi: 10.1016/j.jhydrol.2015.03.027
Akoko, G., Le, T. H., Gomi, T., and Kato, T. (2021). A review of SWAT model application in Africa. Water 13:1313. doi: 10.3390/w13091313
Aredo, M. R., Hatiye, S. D., and Pingale, S. M. (2021). Impact of land use/land cover change on stream flow in the Shaya catchment of Ethiopia using the MIKE SHE model. Arab. J. Geosci. 14:114. doi: 10.1007/s12517-021-06447-2
Arnold, J. G., Moriasi, D. N., Gassman, P. W., Abbaspour, K. C., White, M. J., and Raghavan, S. (2012). SWAT: model use, calibration, and validation. Trans. ASABE 55, 1491–1508. doi: 10.13031/2013.42256
Bieger, K., Arnold, J. G., Rathjens, H., White, M. J., Bosch, D. D., Allen, P. M., et al. (2017). Introduction to SWAT+, a completely restructured version of the soil and water assessment tool. JAWRA J. Am. Water Resourc. Assoc. 53, 115–130. doi: 10.1111/1752-1688.12482
Burhan, A. K., Usman, M., Bukhari, S., Khan, M. T., and Malik, K. M. (2020). Prognosis of hydro-meteorological attributes based on simulation and projection of streamflow in a high-Altitude Basin using Hydrologiska Byråns Vattenbalansavdelning (HBV) model. Aqua 4:ep20015. doi: 10.29333/aquademia/8226
Chang, Q. X., Sun, Z. Y., and Ma, R. (2016). Progress of research on groundwater flow process and its relationship with surface water transformation in permafrost zone. Advances in water resources and hydropower. Sci. Technol. 36:8. doi: 10.3880/j.issn.1006-7647.2016.05.016
Chase, T. N., Pielke Sr, R. A., Kittel, T. G. F., Nemani, R. R., Running, S. W., and Kittel, T. G. F. (2000). Simulated impacts of historical land cover changes on global climate in northern winter. Clim. Dyn. 16, 93–105. doi: 10.1007/s003820050007
Chen, Y., Li, Z., Li, P., Zhang, Y., Liu, H., Pan, J., et al. (2022). Impacts and projections of land use and demographic changes on ecosystem services: A case study in the guanzhong region, China
Chen, Y., Shi, P., Qu, S., Ji, X., Zhao, L., Gou, J., et al. (2019). Integrating XAJ model with GIUH based on Nash model for rainfall-runoff modelling. Water 11:772. doi: 10.3390/W11040772
Deng, H., Dan, L., Deng, H., Xiao, Y., and Wang, Q. (2022). Use of the SSIB4/TRIFFID model coupled with TOPMODEL to investigate the effects of vegetation and climate on evapotranspiration and runoff in a subalpine basin of southwestern China. J. Environ. Eng. Landsc. Manag. 30, 43–55. doi: 10.3846/jeelm.2022.15227
Dimri, T., Ahmad, S., and Sharif, M. (2020). Time series analysis of climate variables using seasonal ARIMA approach. J. Earth System Sci. 129, 1–16. doi: 10.1007/s12040-020-01408-x
Du, B., Wu, L., Ruan, B., Xu, L., and Liu, S. (2023). CMADS and CFSR data-driven SWAT modeling for impacts of climate and land-use change on runoff. Water 15:83240. doi: 10.3390/w15183240
Đukić, V., and Erić, R. (2021). SHETRAN and HEC HMS model evaluation for runoff and soil moisture simulation in the Jičinka River catchment (Czech Republic). Water 13:872. doi: 10.3390/w13060872
Elbeltagi, A., Kushwaha, N. L., Rajput, J., Vishwakarma, D. K., Kulimushi, L. C., and Kumar, M. (2022). Modelling daily reference evapotranspiration based on stacking hybridization of ANN with meta-heuristic algorithms under diverse agro-climatic conditions. Stoch. Env. Res. Risk 36, 3311–3334. doi: 10.1007/s00477-022-02196-0
Foley, J. A., Defries, R., Asner, G. P., Barford, C., Bonan, G., Carpenter, S. R., et al. (2005). Global consequences of land use. Science (New York, N.Y.) 309, 570–574. doi: 10.1126/science.1111772
Foley, J. A., Ramankutty, N., Brauman, K. A., Cassidy, E. S., Gerber, J. S., Johnston, M., et al. (2011). Solutions for a cultivated planet. Nature 478, 337–342. doi: 10.1038/nature10452
Golmohammadi, G., Prasher, S. O., Madani, A. B., and Rudra, R. P. (2014). Evaluating three hydrological distributed watershed models: MIKE-SHE, APEX, SWAT. Hydrology 1, 20–39. doi: 10.3390/hydrology1010020
Guiamel, I. A., and Lee, H. S. (2020). Watershed modelling of the Mindanao River basin in the Philippines using the SWAT for water resource management. Civil Eng. J. 6, 626–648. doi: 10.28991/cej-2020-03091496
He, Z., Wang, Z., Suen, C. J., and Ma, X. (2013). Hydrologic sensitivity of the upper San Joaquin river watershed in California to climate change scenarios. Hydrol. Res. 44, 723–736. doi: 10.2166/nh.2012.441
Hu, Z., Wang, L., Wang, Z., Hong, Y., Zheng, H., and Hu, Z. (2015). Quantitative assessment of climate and human impacts on surface water resources in a typical semi-arid watershed in the middle reaches of the yellow river from 1985 to 2006. Int. J. Climatol. 35, 97–113. doi: 10.1002/joc.3965
Hussain, F., Wu, R., and Shih, D. (2022). Water table response to rainfall and groundwater simulation using physics-based numerical model: WASH123D. J. Hydrol. 39:100988. doi: 10.1016/j.ejrh.2022.100988
Januário, T. E., Pereira Filho, A. J., and Salviano, M. F. (2022). Hydrometeorological modeling of Limpopo River basin in Mozambique with TOPMODEL and remote sensing. Open J. Mod. Hydrol. 12, 55–73. doi: 10.4236/ojmh.2022.122004
Kouadri, S., Pande, C. B., Panneerselvam, B., Moharir, K. N., and Elbeltagi, A. (2021). Prediction of irrigation groundwater quality parameters using ANN, LSTM, and MLR models. Environ. Sci. Pollut. Res. 29, 21067–21091. doi: 10.1007/s11356-021-17084-3
Lambin, E F, and Geist, H J. (2008) Land-use and land-cover change: Local processes and global impacts. Berlin: Springer Science & Business Media
Li, X., Cheng, G., Jin, H., Kang, E., Che, T., and Jin, R. (2008). Cryospheric change in China. Glob. Planet. Chang. 62, 210–218. doi: 10.1016/j.gloplacha.2008.02.001
Li, Z., Jian, S., Gu, R., and Sun, J. (2023). Runoff simulation under the effects of the modified soil water assessment tool (SWAT) model in the Jiyun River basin. Water 15:2110. doi: 10.3390/w15112110
Liang, M. (2016). Remote sensing study on the temporal and spatial variation of ground temperature in the permafrost region of Daxing ‘anling. (Doctoral dissertation, Jilin University)
Ling, Z., Zhuotong, N., Yi, X., Shuo, L., and Maite, D. (2016). Hydrological impacts of land use change and climate variability in the headwater region of the Heihe river basin, Northwest China. PLoS One 11:e0158394. doi: 10.1371/journal.pone.0158394
Liu, X. (2019). Impacts of climate change on permafrost and hydrological processes in Northeast China. (Doctoral dissertation, Hunan University of Science and Technology).
Liu, X., Feng, X., and Fu, B. (2019). Changes in global terrestrial ecosystem water use efficiency are closely related to soil moisture. Sci. Total Environ. 698:134165. doi: 10.1016/j.scitotenv.2019.134165
Liu, Y., Yang, Z., Lin, P., Zheng, Z., and Xie, S. (2019). Comparison and evaluation of multiple land surface products for the water budget in the yellow river basin. J. Hydrol. 584:124534. doi: 10.1016/j.jhydrol.2019.124534
Margulis, S. A., Wood, E. F., and Troch, P. A. (2006). The terrestrial water cycle: modeling and data assimilation across catchment scales. J. Hydrometeorol. 7, 309–311. doi: 10.1175/JHM999.1
Mehnaza, A., Malik, M. I., Mehraj, T., Shah, A. F., Ahmad, S., and Bhat, W. A. (2022). Runoff modelling of Aripal watershed using SWAT model. Arab. J. Geosci. 15:1419. doi: 10.1007/s12517-022-10708-z
Metcalfe, R. A., and Buttle, J. M. (2001). Soil partitioning and surface store controls on spring runoff from a boreal forest peatland basin in north-Central Manitoba, Canada. Hydrol. Proc. 15, 2305–2324. doi: 10.1002/hyp.262
Oki, J., Zhang, Y., Bing, H., Peng, J., Dong, F., Gao, J., et al. (2021). Characterizing the river water quality in China: recent progress and on-going challenges. Water Res. 201:117309. doi: 10.1016/j.watres.2021.117309
Oo, H. T., Zin, W. W., and Kyi, C. C. (2020). Analysis of streamflow response to changing climate conditions using SWAT model. Civil Eng. J. 6, 194–209. doi: 10.28991/cej-2020-03091464
Op de Hipt, F., Diekkrüger, B., Steup, G., Yira, Y., Hoffmann, T. O., and Rode, M. (2019). Modeling the effect of land use and climate change on water resources and soil erosion in a tropical west African catch-ment (Dano, Burkina Faso) using SHETRAN. Sci. Total Environ. 653, 431–445. doi: 10.1016/j.scitotenv.2018.10.351
Ostad Ali Askari, K. (2022). Investigation of meteorological variables on runoff archetypal using SWAT: basic concepts and fundamentals. Appl. Water Sci. 12:e8. doi: 10.1007/s13201-022-01701-8
Ostad-Ali-Askari, K., Hannah, D. M., Ostad-Ali-Askari, K., Krause, S., Zalewski, M., and Boogaard, F. C. (2019). The impact of future climate change and human activities on hydro-climatological drought, analysis and projections: using CMIP5 climate model simulations. Water Conser. Sci. Eng. 4, 71–88. doi: 10.1007/s41101-019-00069-2
Panigrahy, B.P., Singh, P.K., Tiwari, A.K., and Kumar, B. (2014). Impact of climate change on groundwater resources
Rehana, S., and Mujumdar, P. P. (2011). River water quality response under hypothetical climate change scenarios in tunga-bhadra river, India. Hydrol. Process. 25, 3373–3386. doi: 10.1002/hyp.8057
Richards, D.R., Lin, H.J., Cheng, H., Zhang, F., Huang, G., and Edris, E.V., (2005). A first-principle, physics-based watershed model: WASH123D
Sang, Y. F., Wang, Z., and Liu, C. (2014). The impact of changing environments on the runoff regimes of the arid Heihe river basin, China. Theor. Appl. Climatol. 115, 187–195. doi: 10.1007/s00704-013-0888-y
Seibert, J., and Bergström, S. (2022). A retrospective on hydrological catchment modelling based on half a century with the HBV model. Hydrol. Earth Syst. Sci. 26, 1371–1388. doi: 10.5194/hess-26-1371-2022
Shi, P., Chen, C., Srinivasan, R., Zhang, X., Cai, T., and Fang, X. (2011). Evaluating the SWAT model for hydrological modeling in the Xixian watershed and a comparison with the XAJ model. Water Resour. Manag. 25, 2595–2612. doi: 10.1007/s11269-011-9828-8
Su, B., Wang, A., Wang, G., Wang, Y., and Jiang, T. (2016). Spatiotemporal variations of soil moisture in the tarim river basin, China. Int. J. Appl. Earth Obs. Geoinf. 48, 122–130. doi: 10.1016/j.jag.2015.06.012
Sun, G., Wei, X., Hao, L., Sanchis, M. G., Hou, Y., and Yousefpour, R. (2023). Forest hydrology modeling tools for watershed management: a review. For. Ecol. Manag. 530:120755. doi: 10.1016/j.foreco.2022.120755
Tang, Q. (2020). Global change hydrology: terrestrial water cycle and global change. Science China Press 63, 459–462. doi: 10.1007/s11430-019-9559-9
Tong, Y., Wang, Y., Song, Y., Sun, H., and Xu, Y. (2020). Spatiotemporal variations in deep soil moisture and its response to land-use shifts in the wind–water Erosion crisscross region in the critical zone of the loess plateau (2011–2015), China. Catena 193:104643. doi: 10.1016/j.catena.2020.104643
Vörösmarty, C. J., and Sahagian, D. (2000). Anthropogenic disturbance of the terrestrial water cycle. Bioscience 50:753. doi: 10.1641/0006-3568(2000)050[0753:ADOTTW]2.0.CO;2
Wang, W., Chau, K., Xu, D., and Chen, X. (2015). Improving forecasting accuracy of annual runoff time series using ARIMA based on EEMD decomposition. Water Resour. Manag. 29, 2655–2675. doi: 10.1007/s11269-015-0962-6
Xiao-meng, S. (2013). Review for impacts of climate change and human activities on water cycle. J. Hydraul. Eng. 44:779. doi: 10.13243/j.cnki.slxb.2014.04.001
Yue, Y. J., Wu, Y. Z. L., Li, X., Wang, Y. Q., and Elizabeth, A. (2020). Analysis of the characteristics of climate and runoff changes in the Genhe River basin from 1980 to 2017. J. Irrig. Drain. 04, 96–105. doi: 10.13522/j.cnki.ggps.2019255
Zhang, J. C., and Lei, J. I. N. (2019). The relationship between dynamic changes of soil water content and climatic factors in temperate meadow steppe of Ewenki autonomous banner, Inner Mongolia. Animal Husbandr. Feed Sci. 40:5.
Zhang, J., Zhang, M., Song, Y., and Lai, Y. (2021). Hydrological simulation of the Jialing River basin using the MIKE SHE model in changing climate. J. Water Climate Change. 12, 2495–2514. doi: 10.2166/wcc.2021.253
Zhang, X., Zhu, X. Y., Beibei, S., Yuhai, B., Quansheng, H., and Xiaoping, X. (2020). Study on spatial and temporal variation of vegetation evapotranspiration in Hulun Buir. China Agric. Resour. Regional. 4, 308–316.
Keywords: Genhe River Basin, hydrological process, M–K test, typical ecosystems, land use scenarios, human factors
Citation: Zhao K, Qin F, Yue Y, Li L, Dong X, Liu L, Li Y, Wu Y, He R, Wang Y, Xu Y and Wu Y (2024) Analysis of temporal variation characteristics in water resources in typical ecosystems of the Genhe River Basin. Front. For. Glob. Change. 7:1345207. doi: 10.3389/ffgc.2024.1345207
Edited by:
Quanhou Dai, Guizhou University, ChinaReviewed by:
Fengling Gan, Chongqing Normal University, ChinaPingzong Zhu, Southwest University, China
Copyright © 2024 Zhao, Qin, Yue, Li, Dong, Liu, Li, Wu, He, Wang, Xu and Wu. This is an open-access article distributed under the terms of the Creative Commons Attribution License (CC BY). The use, distribution or reproduction in other forums is permitted, provided the original author(s) and the copyright owner(s) are credited and that the original publication in this journal is cited, in accordance with accepted academic practice. No use, distribution or reproduction is permitted which does not comply with these terms.
*Correspondence: Long Li, bGlsb25nZGhyQDEyNi5jb20=