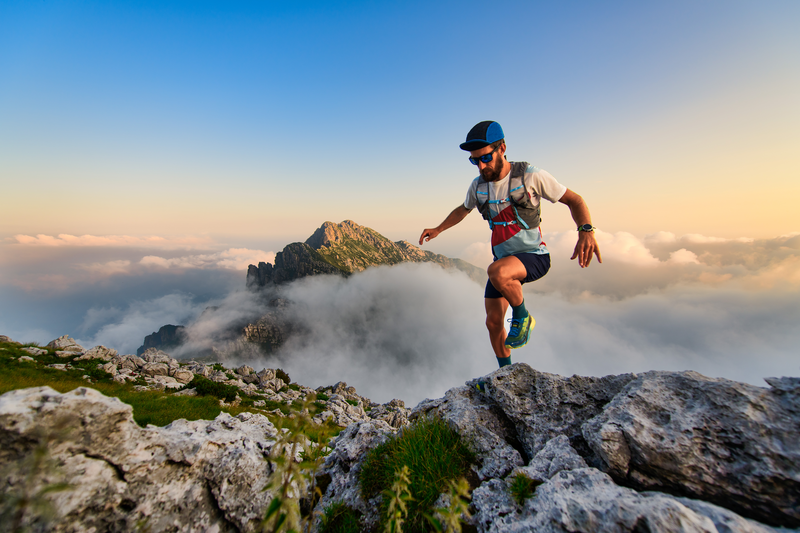
94% of researchers rate our articles as excellent or good
Learn more about the work of our research integrity team to safeguard the quality of each article we publish.
Find out more
ORIGINAL RESEARCH article
Front. For. Glob. Change , 28 March 2024
Sec. Forest Growth
Volume 7 - 2024 | https://doi.org/10.3389/ffgc.2024.1343206
This article is part of the Research Topic Response of Plant Functional Traits to Environments and Their Roles in Ecosystem Processes View all 8 articles
Introduction: Transpiration is the driving force of water transport, which plays a crucial role in the rapid growth of bamboo shoots. Nonetheless, the transpiration changes that occur in the shoot sheath of Moso bamboo during the leafless stage and the physiological processes involved in water transport from the mother bamboo to bamboo shoots are not completely understood.
Methods: This study investigated the temporal dynamics of the transpiration rate (Tr) and stomatal conductance (gs) of the sheaths from bamboo shoots to culms and diurnal variations in Tr and gs as well as the spatial dynamics of Tr and gs in various parts of the shoot sheaths. Water distribution patterns in bamboo shoots were analyzed using the isotope tracer method, and the water transportation path in bamboo shoots was determined by soaking the shoots in fuchsine dye solution.
Results: We observed that the Tr was higher in bamboo shoots at heights of 4 and 7 m compared to that at 0.5, 1, 2, and 13 m, with rates ranging from 6.8 mmol/m2/s1 to 8.3 mmol/m2/s. Additionally, the Tr and gs of the shoot sheath were lower at noon, but higher in the morning and evening. The Tr in the lower parts of the culm sheath was higher than that that in the upper part (height: 0.5–4 m). However, when injected into the mother bamboo, D2O was not immediately transported to the shoots via the mother culms but was transported upward through the vascular bundle, with a larger vascular bundle near the pulp cavity transporting high volumes of water.
Discussion: These findings provide the foundation for further studies on the rapid growth of Moso bamboo and establish a theoretical basis for water management during its shoot developmental period.
Moso bamboo (Phyllostachys edulis), a native of China, is widely distributed in the tropical and subtropical areas of East and Southeast Asia and plays important roles in regional water and soil loss management, water restoration, and soil conservation (Song et al., 2017; Zhang et al., 2019). The species is known for the rapid growth of emerging shoots (Liese and Köhl, 2015), with growth rates reaching up to 10–80 cm per day (Liese and Köhl, 2015; Song et al., 2016). Furthermore, it takes only 1–2 months for this bamboo species to grow from shoot to culm through a stage known as the “explosive growth” stage (Song et al., 2016).
Water is an important solvent for plant cells as it affects various life activities and regulates plant growth rate. It is an important factor that not only directly affects plant growth and development but is also an essential component of physiological metabolism. In bamboo species, water supply is thus a determinant of shoot differentiation, the number of shoots available for harvesting, and the quality of new growth (Fu et al., 2005). Moso bamboo is connected by a subterranean rhizome system that serves as a conduit for water and nutrient exchange between different culms within the same clone (Wilsey, 2002; Xu et al., 2013). Studies have found that during the rapid growth phase of Moso bamboo, water is transferred from the stem through the root, and thus, the rapid growth of bamboo shoots is related to water transport from the mother culms to the shoots (Dierick et al., 2010; Zhang R. et al., 2017; Zhang Z. Z. et al., 2017).
Transpiration is commonly used to indicate the water status of plants as well as the interactions between plants and environmental factors (Ruiz-Pdrez et al., 2001). In this regard, the evaporative surface of the sheath is considered crucial in gramineous plants, particularly as the plant approaches a critical stage. Sheath transpiration significantly contributes to overall water loss in plants, particularly during the night (Sadok et al., 2020). When bamboo shoots and young culms are branchless and leafless, the shoot sheath serves as the primary transpiratory organ (Wang et al., 2018). The culm sheath is also directly connected to the outer vascular bundle, and its transpiration rate (Tr) and stomatal conductance (gs) are significantly higher than those of the leaf sheath (Wang et al., 2018). Moreover, the removal of the culm sheath affects the height of bamboo shoots (Shang et al., 2013; Wang et al., 2017). However, despite the multiple functions of the shoot sheath in the rapid growth phase of Moso bamboo, studies on the spatiotemporal dynamics of the Tr of the shoot sheath are limited.
Deuterium oxide (D2O) tracing is an effective method for studying water transport among interconnected plants such as those connected by bamboo culms and rhizomes. In Bambusa blumeana, increased deuterium concentrations were detected in the leaves of culms of neighboring bamboos that had been treated with D2O (Dierick et al., 2010). Thermal dissipation probes (Granier, 1985) in the clumps of Bambusa vulgaris also indicated water transport from mature culms to newly sprouted culms during the sprouting stage (Fang et al., 2019). Similarly, a 20% decrease in water use rates was noted after cutting rhizomes between neighboring culms (Zhao et al., 2017). Nevertheless, the relationship between water transport among culms of Moso bamboo and its effect on shoot sheath transpiration during the explosive growth stage has not been adequately explored.
In this study, we determined the spatiotemporal dynamics of Tr and gs in the shoot sheaths and analyzed the water distribution patterns in the shoots of Moso bamboo using the isotope tracer method. An acidic magenta aqueous solution was used to trace the water transport route. The objectives of this study were (1) to determine the spatiotemporal variations in shoot sheath transpiration and its effect on the rapid growth of bamboo shoots during the branchless, leafless stage and (2) to determine the water transport pattern and its relationship with shoot sheath transpiration. The findings of this study will provide a theoretical basis for water management during the explosive growth stage of Moso bamboo shoots.
The experiment was conducted in a Moso bamboo forest in Jing County, Anhui Province, China. We established three plots (10 × 10 m2), containing a total of 41 bamboo culms. Plant height was measured from the tip of the shoot to the culm using a CGQ-1 direct reading height gauge (Harbin Optical Instrument Factory Co., Ltd., China) between March 29 and April 28. Measurements were taken in the morning (06:00–08:00) and evening (18:00–20:00), ensuring a 12-h interval between successive measurements and two measurements every 24 h. Once the bamboo shoots attained a height of 4 m, three plants were randomly selected, and their heights were recorded every hour for 48 h.
The light intensity (Lx), air temperature (T, °C), and relative humidity (RH, %) were recorded every hour during the study period using Microclimate meters (Pocket Weather Tracker 4000, Kestrel Corp., USA), which were installed in the center of each plot. Thereafter, the vapor pressure deficit (VPD) was calculated using RH and T.
Tr and gs were measured using a Li-COR 6400 photosynthesis apparatus (LI-COR, USA), with a light intensity of 800 mol/m2/s, CO2 concentration of 400 μmol/mol, and temperature of 25°C. When the bamboo shoots attained a height of more than 4 m, a researcher needed to climb a ladder and record the measurements.
A total of 18 (out of 41) bamboo plants were selected from the three plots to measure the changes in Tr and gs in the shoot sheaths and culms of plants with an average height of 0.5, 1, 2, 4, 7, and 13 m (Figures 1b–d, f, g) and average diameter of 4–5 cm. The Tr and growth rate were measured for different bamboo plants. Nonetheless, the Tr and gs of three plants with similar heights were determined simultaneously from 08:00 to 10:00.
Figure 1. (a, e) Peeled-off sheath of 0.5-m bamboo shoot, the number (s1, s2, s3…s30) represents the location of the sheath in the bamboo shoots, the same as (c). The s-upper, s-middle, and s-lower parts of the culm sheath are marked, I represents the innermost layer of the culm sheath. (b–d, f, g) Photographs of 1-, 2-, 4-, 7-, and 13-m bamboo shoots. The number (s1, s2, s3…s15) represents the location of the sheath, the same as (a, e). S represents the top of the culm sheath; W stands for culm sheath of internode 13; T represents the upper part of the outermost-layer culm sheath; M represents the middle part of the outmost-layer culm sheath; B represents the bottom part of the outermost-layer culm sheath.
It was difficult to determine the Tr and gs in the interior of the sheath; hence, these parameters were measured for the exterior of the sheath, which was then peeled immediately to rapidly determine the values of these parameters in the interior of the sheath. In the process, bamboo shoots were damaged, and thus, another set of shoots was used for further estimations. Each sheath of the bamboo shoots was numbered (at different heights) as follows: 0.5 m (sheath number: 30, 21, and 23), 1 m (sheath number: 31, 31, and 23), 2 m (sheath number: 31, 31, and 31), 4 m (sheath number: 30, 31, and 31), 7 m (sheath number: 30, 21, and 23), and 13 m (sheath number: 37, 38, and 39). The peeled-off sheath is shown in Figures 1a, e. We measured the Tr and gs of the S-upper, S-middle, and S-lower parts of all the culm sheaths (s1 to sn; Figure 1a), and the area of each culm sheath was measured using the grid method (Zhuang, 1979). Then, average values of the Tr and gs of three bamboo shoots of similar height were analyzed.
As the unit of Tr was mmol/m2/s, the unit of transpiration water consumption (Tc) was converted to kilogram per meter square per hour to facilitate its estimation at different heights using the following formula: Tc = 0.0648Tr. The formula used to calculate the Tc of bamboo shoots over 8 h during the day (Ts, kg) is as follows.
where Tci is the transpiration rate of the sheath i (kg/m2/h), Ai is the area (m2) of the sheath i, t (8 h) is the time interval, and n is the number of culm sheaths.
Three bamboo shoots were then selected to measure the Tr and gs at 09:00, 12:00, 14:00, 15:00, 17:00, and 18:00. The values for the S-upper part of bamboo sheaths of s1–s5, s10–s15, and s15–s20 culms were measured without any damage to the plants. Thereafter, we calculated the average of the values obtained from different sheaths of a bamboo shoot and the values obtained for the same period.
We found that the length of the internode of the shoots of heights 0.5, 1, 2, and 4 m was extremely reduced, and the amount of tissue fluid was low. Additionally, as lignification was severe in the internode of bamboo shoots with a height of over 7 m, the tissue fluid could not be extracted. Moreover, the Tr of 7-m-tall bamboo shoots was the highest; hence, they were selected for further estimations. Furthermore, the growth rate of bamboo shoots was not uniform. Therefore, three bamboo shoots with a height of 7 m (collected from 24 April 2019 to 30 April 2019) and another set of three shoots (collected between 30 April 2019 and 11 May 2019) were used for isotopic tracer experiments.
The mother bamboo of each shoot was identified using the rhizome. The surface soil of the sample plant was removed, and 10 mL of D2O (99.9% D, Shanghai Research Institute of Chemical Industry, China) was injected into the base of the mother bamboo. Following Meinzer et al.'s (2006) method, fluid from shoot guttation was collected after injection. Figure 2 shows three white water-receiving devices (top, middle, and bottom) around each bamboo shoot. Fluid from guttation before sunrise was collected, and the Tr of the sheath was measured between 08:00 and 09:00 from the second day to the seventh day of the two experiments. The s1–s5, s10–s15, and s15–s20 culm sheaths (S-upper part) were selected to measure the Tr, and their average was determined. After 7 days, tissue fluid was collected from 5, 10, 15, 20, 25, 30, and 35 internodes of the shoots. All the samples were tested at the Shanghai Chemical Research Institute, and the relative isotope ratio (δD) in the guttation and tissue fluid was measured at the Shanghai Research Institute of Chemical Industry. During the study period, the annual temperatures were 25.6 ± 5.3°C and 25.5 ± 5.2°C, the average soil moisture was 28.02 and 29.5%, and the average RH was 93.58 and 88%.
Figure 2. Schematic drawing of isotopic tracing experiment; the green bamboo culm is the mother bamboo; next to the three bamboo shoots, the top, middle, and bottom, three parts of the bamboo shoots are equipped with the device of guttation reception.
The δD of guttation before injection was used as the baseline, and the isotope data were standardized according to the maximum value of δD. After standardization, the baseline value was 0, and the maximum value was 1. When the δD increased significantly (>10% from the baseline), it was recorded as time “h”. Simultaneously, the distance (D) between the bamboo shoot and the mother bamboo was measured to determine tracer velocity (m/h) as D/h.
Six bamboo shoots (height: 2 m) were treated with 1% (m/V) acidic magenta aqueous solution for tracing, according to the protocol reported by Xie et al. (2011). The bamboo shoots were randomly divided into two groups. The cavity at the bottom of the shoots in the first group was injected with a magenta solution at 06:00. After 24 h, the shoots were excised at the bottom to observe whether the magenta solution was transported upward along the bamboo cavity. The bamboo shoots in the other group were excised at the base and then immersed in the magenta solution for 24 h. Subsequently, the 10th internode was manually sliced to observe and record the transportation path of the dye. Each experiment was performed in triplicate.
Following Li et al. (2017), paraffin sections were cut from the middle part of the 10th internode at a height of 0.5, 1, 2, 4, 7, and 13 m. The middle part of each shoot was sliced (~1 × 0.5 cm2), fixed in formaldehyde: acetic acid: ethanol (5: 5: 90%) solution, and placed in a container equipped with a vacuum pump to extract air. Each section was dehydrated using a series of gradient alcohol solutions and embedded in paraffin. Then, 8-μm-thick transverse slices were stained with Tibetan Red for 10 min and Fast Green for 5–10 s. Thereafter, the slices were sealed with a neutral adhesive and observed under an Olympus BX51 microscope (Olympus Medical Systems Corp., Japan) equipped with a digital image acquisition system.
Data are presented as mean ± standard deviation. SPSS 26.0 was used for one-way analysis of variance (ANOVA), least significant difference test, and multiple comparisons (α = 0.05), and SPSS 26.0 and Excel 2016 were used to create the linear model and correlation graphs. We determined the coefficient of variation (CV) using the following formula: CV = standard deviation/mean. Pearson's correlation coefficients are presented in tables.
The average T, RH, VPD, and light intensity during the daytime were 21.21 ± 5.38°C, 64.56 ± 22.60%, 1.05 ± 0.91, and 3,455.25 ± 6,053.22 Lx, respectively. The average T, RH, and VPD values at night were 15.39 ± 4.22°C, 88.01 ± 12.03%, and 0.22 ± 0.28, respectively. Between 10 April 2019 and 12 April 2019, the lowest temperature was ~10°C, and RH at night increased by 40%. On 20 April 2019, the temperature peaked at 33°C, and on 13 April 2019, light intensity reached its maxima at noon (~39,349 Lx) (Figures 3A–D).
Figure 3. (A–D) Changes in light intensity, VPD, temperature (T), and relative humidity (RH) during the period of the rapid growth of bamboo shoots. (E) Height growth rate of bamboo shoots during the rapid growth period. (F, G) Height change of bamboo shoot and Tr and gs changes of shoot sheath during the rapid growth period. The curves represent the change in height of the bamboo shoots; the columns represent the Tr and gs of shoot sheath in 0.5, 1, 2, 4, 7, and 13 m.
The height of the 41 bamboo shoots was measured daily from 30 March 2019 to 27 April 2019. The bamboo shoots grew from 15.17 ± 11.19 cm to 1,310 ± 19.89 cm (Figures 3F, G). The growth rate of the bamboo shoots was low during the early stages when the average plant height was 1 m. Thereafter, the shoots grew more rapidly, reaching an average plant height of 2 m. After 19 April 2019, the growth rate of the bamboo shoots decreased, with an average plant height of 5 m. On 26 April 2019, the growth rate of the bamboo shoots, with an average plant height of 10 m, decreased significantly during the late stages (Figure 3E).
Tr and gs of the shoot sheath were the lowest when the shoot height was 1 m and the highest when the shoot height was 4 and 7 m, respectively (Figures 3F, G). The Tr of the lower parts (s1–s10) of bamboo culm sheaths was higher than that of the upper parts (s21–s31) when the shoot height was 0.5–7 m, but the trend was opposite in taller plants, with a height of 7 m (Figures 4A, B). However, the variations in Tr and gs in different parts of the shoot sheaths were not significant, although gs in the lower parts (Figure 1c) of the culm sheaths was significantly higher than those in other parts (Figures 4C, D). The Ts of bamboo shoots was the highest in plants of height 4 m (Figure 4E). Additionally, Ts was related to T and RH of the microclimate (P < 0.05) (Table 1).
Figure 4. (A, B) Tr and gs of shoot sheaths at different growth heights, lower was s1–s10, mid was s11–s20, and upper was s21–s31 bamboo sheaths. (C, D) Tr and gs of bamboo shoots in different sheath parts, blue and yellow represent day and night values, respectively. (E) Transpiration water consumption of shoots sheaths over 8 h a day of different heights; means with different letters are significantly different (LSD test, P < 0.05). 0.05). *indicates a significant difference (P < 0.05).
Table 1. Correlation coefficient of microclimate change, transpiration, and plant height growth rate during the period of rapid growth of bamboo shoots.
From 11:00 on 18 April 2019 to 11:00 on 20 April 2019, the height of bamboo shoots, T, and RH were continuously monitored (Figures 5A, B). The height of bamboo shoots increased from 369.03 ± 13.69 cm to 469.3 ± 15.4 cm, and the growth rate was higher during the daytime and lower at night, with the highest growth rate occurring from 14:00 to 20:00 (Figure 5C). There was a diurnal variation in Tr and gs in 4-m-tall bamboo shoots; the values were significantly lower at noon and higher in the morning and evening (Figures 5D, E). The CV of the growth rate was significantly correlated with Tr (P < 0.05) (Figure 5F). However, there was no significant correlation between CV of the growth rate and gs (Figure 5G). Moreover, instantaneous Tr was significantly correlated with T, RH, and VPD (P < 0.01) (Table 1). The growth rate was influenced by light intensity (P < 0.01) and correlated with instantaneous Tr (P < 0.05) (Table 2).
Figure 5. (A, B) Diurnal variation of light intensity, T, RH, and VPD in 4-m bamboo shoots. (C) Diurnal variation of height growth rate of 4-m bamboo shoots. (D, E) Diurnal variation of Tr and gs of 4-m bamboo shoots, where L represents leaf sheath and S represents culm sheath. (F, G) Relationship between growth rate of plant height per hour and Tr and gs. Means with different letters are significantly different (LSD test, P < 0.05).
Table 2. Correlation coefficient of diurnal microclimate, dural transpiration rate, and plant height growth rate in 4-m bamboo shoots.
D2O was injected into the mother bamboo connected to 7-m-tall bamboo shoots; guttation and shoot tissue fluids were collected; and the path of the isotope tracer was determined (Figure 6). The results showed that the δD of the two experiments was consistent. On days 3 and 7 after injection, the isotope was transported to the top of bamboo shoots from the mother bamboo through rhizomes, indicating that the injected isotope was not transported to the bamboo shoots by the mother bamboo at once. On day 12, δD was high in all internodes of the shoots, indicating that the isotope was completely transported from the mother bamboo (Figures 6A–D). The δD of guttation increased significantly with an increase in Tr (P > 0.05) (Figure 6E), whereas tracer velocity increased with an increase in Tr (P > 0.05) (Figure 6F).
Figure 6. (A) δD ratio changes in guttation of bamboo shoots in the first test. (B) δD ratio changes in guttation of bamboo shoots in the second test. (C) Trace results of δD ratio in tissue fluid of different stem heights at 3rd day and 7th day after D2O injection in the first test. (D) Tracer results of δD ratio in tissue fluid of different stem heights at 7th and 11th days after D2O injection in the second test. (E) Relationship between isotope δD ratio in guttation and Tr of shoot sheath. (F) Relationship between δD tracer velocity and Tr of shoot sheath; means with different letters are significantly different (LSD test, P < 0.05).
A preliminary study was conducted on the water transportation pathway in bamboo shoots by soaking them in a fuchsine dye solution as well as injecting the fuchsine dye solution in situ (Figures 7A, E). The results revealed that the fuchsine dye solution was transported upward along the vascular bundles, and the staining was deeper in areas with larger conduits, near the medullary cavity (Figures 7B, C). A transportation pathway was thus observed along the transverse wall of the bamboo in the segments (Figure 7D). However, when the magenta solution was injected into the pulp cavity of the bamboo shoots, the dye was not transported upward through the pulp cavity (Figure 7A).
Figure 7. (A) Cross section of medullary cavity 24 h after injection to the bamboo cavity with a magenta solution. (B) Cross section of bamboo shoots after soaking for 24 h. (C, D) Longitudinal section of internode of bamboo shoots after soaking for 24 h. (E) Bamboo shoot is soaked in a magenta solution. Mc, medullary cavity; v, cross section of vascular bundle; lc, longitudinal conduit; ts, the transverse septum of the internode; c, culm of sheaths stripped off; s, sheaths.
This study verified the relationship between the δD of bamboo shoot guttation and the Tr of shoot sheaths. In this study, the δD of guttation increased with an increase in Tr (Figure 6E). Previous studies have also shown that plants exhibiting high guttation show enhanced metabolism and rapid growth (Singh et al., 2013; Chen et al., 2019).
In this study, the Tr of the shoot sheaths varied with shoot height (Figure 4A). As the vascular bundle matured, it extended outward from the wall, thereby enhancing the water-carrying capacity and transpiration in the bamboo culm (Figure 8). Paraffin sectioning of bamboo shoots at various heights revealed the gradual development of vascular bundle cells as the shoots matured (Figure 8), which developed completely with duct sieves when bamboo shoots reached a height of 4 m (Figure 8D). Furthermore, the Tr values were higher when the shoot height was 4 and 7 m (Figure 3F).
Figure 8. (A) Structure of vascular bundle at 10th internode in 0.5-m bamboo shoots. (B) Structure of vascular bundle at 10th internode in 1-m bamboo shoots. (C) Structure of vascular bundle at 10th internode in 2-m bamboo shoots. (D) Structure of vascular bundle at 10th internode in 4-m bamboo shoots. (E) Structure of vascular bundle at 10th internode in 7-m bamboo shoots. (F) Structure of vascular bundle at 10th internode in 13-m bamboo shoots. pa, parenchyma; v, vessel element; ph, phloem.
The Tr of bamboo sheaths decreased at noon (Figure 5D) because of the variations in water vapor pressure that may have led to daily fluctuations in water use (O'brien et al., 2004; Kume et al., 2007) (Table 2). This was consistent with the findings of previous studies (Wang et al., 2018). However, the growth rate of bamboo shoots was the highest from 16:00 to 18:00 (Figure 5C), which was different from the findings of previous studies (Thapa et al., 2015; Fang et al., 2019) in which tropical bamboo was observed to grow rapidly at night. This could be attributed to the micrometeorological drivers that exhibited greater control over the bamboo forests in this study. The growth of bamboo shoots and daily height variations in Moso bamboo shoots correlated with instantaneous variations in Tr and light intensity (Figure 5F; Table 2). Previous studies found that the increase in liquid flow density was likely driven by enhanced solar radiation and ventilation, leading to increased transpirational demand in the canopies of the remaining culms (Whitehead et al., 1984; Teklehaimanot et al., 1991; Bréda et al., 1995; Sun et al., 2014).
In this study, the Tr of the lower culm sheaths (s1–s10) was higher than that of the upper culm sheaths (s21–s31) at shoot heights ranging from 0.5 to 4 m (Figure 4A). Previous studies have suggested that the rate of daytime photosynthesis in the upper part of the culm sheaths was consistently higher than that in the lower parts (Wang et al., 2018). Vertessy et al. (1995) suggested that Tr is influenced by the ecological structure of the plants and environmental factors. Additionally, the upper part of bamboo culm sheaths (s21–s31) was purplish-brown and densely covered with brown spines, whereas the lower part (s1–s10) contained scattered purple spots. Zheng et al. (2022) observed numerous vascular bundles in the lower part of culm sheaths, where a greater number of xylem ducts were apparent in a vascular bundle and their cross-sectional area exceeded that of the phloem. This suggested that lower parts of the culm sheaths (s1–s10) exhibit a high capacity for water transport. However, further studies are required to understand the relationship between Tr and these morphological characteristics.
Bamboo shoots and culms maintain water balance through an interconnected network of roots (Marshall, 1996; Hutchings and Wijesinghe, 1997; Wang et al., 2011; Zhang et al., 2012). In this study, the isotope tracer revealed that water was transported from the culm to the shoots through rhizomes during the explosive growth phase (Figures 6A–D), which was consistent with the findings of previous studies (Li et al., 1998; Song et al., 2016; Cao et al., 2018). However, the transported water did not reach all the shoots in equal quantities (Figures 6C, D). A likely explanation is that water was transported by diffusion from adjoining cells, and the amount of water transferred was associated with the leaf water potential or leaf area of the connected plants (Kroon et al., 1996; Adonsou et al., 2016). Nonetheless, this hypothesis requires further investigation.
Bamboo sheaths play a crucial role in shoot transpiration and respiration (Wang et al., 2018) as they regulate dynamic changes in water pressure and transport through guttation at night and transpiration during the day (Wang et al., 2020). We observed a significant increase in the δD of guttation with an increase in Tr (Figure 6E). This was attributed to the increased water transport velocities owing to transpiration (Figure 6F), which led to decreased water potentials and the generation of negative water pressures and potentials in the sink cells (Deng et al., 2020). According to the cohesion–tension theory, guttation is the process by which water is pumped out of parenchyma cells and phloem under tension (Fichtner and Schulze, 1990; Zhang et al., 2013; Savage et al., 2016). The shoots of Moso bamboo exhibited high transpiration rates, which could not be completely satisfied by the water absorbed through the rhizome system. Thus, mother bamboo supplemented the water supply through the rhizome system (Figures 6A–D), indicating that transpiration is a significant factor that influences water transport.
This study found that the pulp cavity of the bamboo shoots was unable to transport water (Figure 7A), and the vascular bundle adjacent to the pulp cavity of the bamboo wall served as the primary pathway for water transport (Figures 7B–D). Staining was also observed in the ducts of the bamboo nodal diaphragm (Figure 7D), Indicating that these interconnecting ducts played a crucial role in facilitating an efficient water-transport system in bamboo shoots (Zheng et al., 2022).
The instantaneous shoot height growth was associated with the Tr of the shoot sheath. The isotopic tracer experiment revealed that water transport from the mother bamboo to bamboo shoots occurred at a slow rate. However, water transport was a systematic and continuous process in Moso bamboo shoots. The continuous monitoring of transpiration and stem flow at different shoot heights were valuable contributions to this study. However, it was difficult to analyze water transportation in bamboo shoots without branches and leaves using traditional methods. Nonetheless, this study provides reliable data and a method to study water transportation in bamboo shoots.
The original contributions presented in the study are included in the article/supplementary material, further inquiries can be directed to the corresponding author.
JL: Conceptualization, Data curation, Formal analysis, Funding acquisition, Investigation, Methodology, Project administration, Resources, Software, Supervision, Validation, Visualization, Writing – original draft, Writing – review & editing. LC: Conceptualization, Writing – review & editing. JW: Investigation, Writing – review & editing. JX: Investigation, Writing – review & editing. HZ: Investigation, Writing – review & editing. YB: Investigation, Writing – review & editing. ZC: Investigation, Writing – review & editing. SM: Investigation, Writing – review & editing. JG: Conceptualization, Writing – review & editing.
The author(s) declare financial support was received for the research, authorship, and/or publication of this article. This study was supported by the National Natural Science Foundation of China (Grant No. 32001379), the National Natural Science Foundation of China (Grant No. 31971737), and the Basic Scientific Research Fund Program of ICBR (Grant No. 1632021016).
The authors declare that the research was conducted in the absence of any commercial or financial relationships that could be construed as a potential conflict of interest.
All claims expressed in this article are solely those of the authors and do not necessarily represent those of their affiliated organizations, or those of the publisher, the editors and the reviewers. Any product that may be evaluated in this article, or claim that may be made by its manufacturer, is not guaranteed or endorsed by the publisher.
Adonsou, K. E., Desrochers, A., Tremblay, F., and Cernusak, L. (2016). Physiological integration of connected balsam poplar ramets. Tree Physiol. 36, 797–806. doi: 10.1093/treephys/tpv142
Bréda, N., Granier, A., and Aussenac, G. (1995). Effects of thinning on soil and tree water relation and growth in an oak forest (Quercus petraea (Matt.) Liebl.). Tree Physiol. 15, 295–306. doi: 10.1093/treephys/15.5.295
Cao, Y. H., Zhou, B. Z., Ni, X., Ge, X. G., and Wang, X. M. (2018). The Dynamic change of leaf water potential for Moso bamboo under throughfall exclusion. Forest. Res. 31, 183–191. doi: 10.13275/j.cnki.lykxyj.2018.04.025
Chen, A. L., Zhao, W. Q., Ruan, Y. Q., Guo, C. C., Zhang, W. G., Shi, J. M., et al. (2019). Regulation of shoot emergence and degradation and the changes of nutrient composition during degradation of Phyllostachys edulis ‘Pachyloen'. Sci. Silv. Sin. 55, 32–40. doi: 10.11707/j.1001-7488.20191204
Deng, L., Li, P. C., Chu, C. H., Ding, Y. L., and Wang, S. G. (2020). Symplasmic phloem unloading and post-phloem transport during bamboo internode elongation. Tree. Phys. 40, 391–412. doi: 10.1093/treephys/tpz140
Dierick, D., Hölscher, D., and Schwendenmann, L. (2010). Water use characteristics of a bamboo species (Bambusa blumeana) in the Philippines. Agric. For. Meteorol. 150, 1568–1578. doi: 10.1016/j.agrformet.2010.08.006
Fang, D., Mei, T., Röll, A., and Hölscher, D. (2019). Water transfer between bamboo culms in the period of sprouting. Front. Plant Sci. 10:786. doi: 10.3389/fpls.2019.00786
Fichtner, K., and Schulze, E. D. (1990). Xylem water flow in tropical vines as measured by a steady state heating method. Oecologia 82, 355–361. doi: 10.1007/BF00317483
Fu, A. H., Chen, Y. N., Li, W. H., and Zhang, H. F. (2005). Research advances on plant water potential under drought and salt stress. J. Desert Res. 25, 744–749. doi: 10.3321/j.issn:1000-694X.2005.05.021
Granier, A. (1985). A new method of sapflow measurement in tree stems. Ann. Sci. For. 42, 193–200. doi: 10.1051/forest:19850204
Hutchings, M. J., and Wijesinghe, D. K. (1997). Patchy habitats, division of labour and growth dividends in clonal plants. Trends Ecol. Evol. 12, 390–394. doi: 10.1016/S0169-5347(97)87382-X
Kroon, H., Fransen, B., Rheenen, J. W., Dijk, A., and Kreulen, R. (1996). High levels of inter-ramet water translocation in two rhizomatous Carex species, as quantified by deuterium labelling. Oecologia 106, 73–84. doi: 10.1007/BF00334409
Kume, T., Takizawa, H., Yoshifuji, N., Tanaka, K., Tantasirin, C., Tanaka, N., et al. (2007). Impact of soil drought on sap flow and water status of evergreen trees in a tropical monsoon forest in northern Thailand. For. Ecol. Manag. 238, 220–230. doi: 10.1016/j.foreco.2006.10.019
Li, L., Cheng, Z., Ma, Y., Bai, Q., Li, X., Cao, Z., et al. (2017). The association of hormone signalling genes, transcription and changes in shoot anatomy during moso bamboo growth. Plant Biotechnol. J. 16, 72–85. doi: 10.1111/pbi.12750
Li, R., Werger, M. J. A., During, H. J., and Zhong, Z. C. (1998). Carbon and nutrient dynamics in relation to growth rhythm in the giant bamboo (Phyllostachys pubescens). Plant Soil 201:113e123. doi: 10.1023/a:1004322812651
Marshall, C. (1996). Sectoriality and physiological organisation in herbaceous plants: an overview. Vegetatio 127, 9–16. doi: 10.1007/BF00054842
Meinzer, F. C., Brooks, J. R., Domec, J. C., Gartner, B. L., Warren, J. M., Woodruff, D. R., et al. (2006). Dynamics of water transport and storage in conifers studied with deuterium and heat tracing techniques. Plant Cell Environ. 29, 105–114. doi: 10.1111/j.1365-3040.2005.01404.x
O'brien, J. J., Oberbauer, S. F., and Clark, D. B. (2004). Whole tree xylem sap flow responses to multiple environmental variables in a wet tropical forest. Plant Cell Environ. 27, 551–567. doi: 10.1111/j.1365-3040.2003.01160.x
Ruiz-Pdrez, M., Fu, M. Y., Yang, X. S., and Belcher, B. (2001). Bamboo forestry in China: toward environmentally friendly expansion. J. For. Res. 99, 14–20. doi: 10.1016/S1389-9341(01)00037-5
Sadok, W., Lopez, J. R., Zhang, Y. Y., Tamang, B. G., and Muehlbauer, G. J. (2020). Sheathing the blade: Significant contribution of sheaths to daytime and nighttime gas exchange in a grass crop. Plant Cell Environ. 43, 1844–1861. doi: 10.1111/pce.13808
Savage, J. A., Clearwater, M. J., Haines, D. F., Klein, T., Mencuccini, M., Sevanto, S., et al. (2016). Allocation, stress tolerance and carbon transport in plants: how does phloem physiology affect plant ecology? Plant Cell Environ. 39, 709–725. doi: 10.1111/pce.12602
Shang, J. D., Li, C., Sun, J., Tao, H. F., and Wu, L. D. (2013). Effects of stripping sheath on bamboo shoot and height growth of Phyllostachys edulis. J. Northeast. For. Univ. 41, 10–12. doi: 10.3969/j.issn.1000-5382.2013.11.003
Singh, K., Gupta, I., and Gupta, S. (2013). Classification of bamboo species by fourier and legendre moment. Int. J. Adv. Sci. Tech. 50, 61–70. Available online at: http://article.nadiapub.com/IJAST/vol50/6.pdf
Song, X., Li, Q., and Gu, H. (2017). Effect of nitrogen deposition and management practices on fine root decomposition in Moso bamboo plantations. Plant Soil 410:207e215. doi: 10.1007/s11104-016-2997-8
Song, X., Peng, C., Zhou, G., Gu, H., Li, Q., and Zhang, C. (2016). Dynamic allocation and transfer of non-structural carbohydrates, a possible mechanism for the explosive growth of Moso bamboo. Sci. Rep. 6:25908. doi: 10.1038/srep25908
Sun, X., Onda, Y., Otsuki, K., Kato, H., Hirata, A., and Gomi, T. (2014). The effect of strip thinning on tree transpiration in a Japanese cypress (Chamaecyparis obtusa Endl.) plantation. Agric. For. Meteorol. 197, 123–135. doi: 10.1016/j.agrformet.2014.06.011
Teklehaimanot, Z., Jarvis, P. G., and Ledger, D. C. (1991). Rainfall interception and boundary layer conductance in relation to tree spacing. J. Hydrol. 123, 261–278. doi: 10.1016/0022-1694(91)90094-X
Thapa, P., Kaur, D., Sood, P., and Mehta, R. (2015). Bamboos in India. ENVIS Centre on Forestry, National Forest Library and Information Centre, Dehradun, Forest Research Institute.
Vertessy, R. A., Benyon, R. G., O'Sullivan, S. K., and Gribben, P. R. (1995). Relationships between stem diameter, sapwood area, leaf area and transpiration in a young mountain ash forest. Tree Physiol. 15, 559–567. doi: 10.1093/treephys/15.9.559
Wang, S. G., He, W. Z., and Zhan, H. (2018). Culm sheaths affect height growth of bamboo shoots in Fargesia yunnanensis. Braz. J. Bot. 41, 255–266. doi: 10.1007/s40415-018-0446-z
Wang, S. G., Zhan, H., Li, P. C., Chu, C. H., Li, J., and Wang, C. M. (2020). Physiological mechanism of internod bending growth after the excision of shoot sheath in Fargesia yunnanensisand its implications for understanding the rapid growth of bamboos. Front. Plant Sci. 11:418. doi: 10.3389/fpls.2020.00418
Wang, S. G., Zhan, H., Wan, C. B., and Lin, S. Y. (2017). Developmental characteristics of parenchyma and fiber cells and their secondary wall deposition in Fargesia yunnanensis. Pak. J. Bot. 49, 187–200.
Wang, Y, Dong, M., Yu, F., Jiang, H., Yu, S., Lin, X., et al. (2011). Mechanical shaking and soil water affect the growth of in the Mu Us Sandland. J. Arid Environ. 75, 974–977. doi: 10.1016/j.jaridenv.2011.04.019
Whitehead, D., Jarvis, P. G., and Waring, R. H. (1984). Stomatal conductance, transpiration, and resistance to water up take in a Pinus sylvestris spacing experiment. Can. J. For. Res. 14, 692–700. doi: 10.1139/x84-124
Wilsey, B. (2002). Clonal plants in a spatially heterogeneous environment: effects of integration on Serengeti grassland response to defoliation and urine-hits from grazing mammals. Plant. Ecol. 159, 15–22. doi: 10.1023/A:1015556816307
Xie, Z. S., Cao, H. M., Li, B., Li, W. F., Xu, W. P, and Wang, S.P. (2011). The effects of root restriction on water transport in the vascular bundle of grape fruit during different stages of development. Plant Physiol. J. 47, 899–903. doi: 10.3724/SP.J.1011.2011.00093
Xu, X. J., Zhou, G. M., Liu, S. G., Du, H. Q., Mo, L. F., Shi, Y. J., et al. (2013). Implications of ice storm damages on the water and carbon cycle of bamboo forests in southeastern China. Agr. Forest. Meteorol. 177, 35–45. doi: 10.1016/j.agrformet.2013.04.005
Zhang, M., Chen, S., Jiang, H., Lin, Y., Zhang, J., Song, X., et al. (2019). Water-use characteristics and physiological response of Moso bamboo to flash droughts. Int. J. Environ. Res. Publ. Health. 16:1e18. doi: 10.3390/ijerph16122174
Zhang, R., Wu, J. S., Li, Q., Hänninen, H., Peng, C. J., Yao, H., et al. (2017). Nitrogen deposition enhances photosynthesis in moso bamboo but increases susceptibility to other stress factors. Front. Plant Sci. 8:1975. doi: 10.3389/fpls.2017.01975
Zhang, Y., Zhang, Q., and Sammul, M. (2012). Physiological integration ameliorates Negative effects of drought stress in the clonal herb. PLoS ONE 7:e44221. doi: 10.1371/journal.pone.0044221
Zhang, Y. J., Meinzer, F. C., Qi, J. H., Goldstein, G., and Cao, K. F. (2013). Midday stomatal conductance is more related to stem rather than leaf water status in subtropical deciduous and evergreen broad leaf trees. Plant Cell Environ. 36, 149–158. doi: 10.1111/j.1365-3040.2012.02563.x
Zhang, Z. Z., Zhou, J., Zhao, X. H., Zhao, P., Zhu, L. W., Ouyang, L., et al. (2017). Maximised photosynthetic capacity and decreased hydraulic failure risk during aging in the clump bamboo, Bambusa chungii. Funct. Plant Biol. 44, 785–794. doi: 10.1071/FP16381
Zhao, X., Zhao, P., Zhang, Z., Zhu, L., Niu, J., Ni, G., et al. (2017). Sap flow-based transpiration in Phyllostachys pubescens: applicability of the TDP methodology, age effect and rhizome role. Trees Struct. Funct. 31, 765–779. doi: 10.1007/s00468-016-1407-4
Zheng, H. F., Cai, M. M., Bai, Y. C., Xu, J. L., Xie, Y., Song, H. J., et al. (2022). The effect of guttation on the growth of bamboo shoots. Forests 13:, 31. doi: 10.3390/f13010031
Keywords: shoot sheath, spatio-temporal, transpiration rate, isotopic tracing, water transportation path, shoot rapid growth
Citation: Li J, Chen L, Wang J, Xu J, Zheng H, Bai Y, Cheng Z, Mu S and Gao J (2024) Spatiotemporal dynamic changes in transpiration in the shoot sheath and its relation to water transportation during rapid growth of Moso bamboo. Front. For. Glob. Change 7:1343206. doi: 10.3389/ffgc.2024.1343206
Received: 23 November 2023; Accepted: 12 March 2024;
Published: 28 March 2024.
Edited by:
Wensheng Bu, Jiangxi Agricultural University, ChinaReviewed by:
Fen Yu, Jiangxi Agricultural University, ChinaCopyright © 2024 Li, Chen, Wang, Xu, Zheng, Bai, Cheng, Mu and Gao. This is an open-access article distributed under the terms of the Creative Commons Attribution License (CC BY). The use, distribution or reproduction in other forums is permitted, provided the original author(s) and the copyright owner(s) are credited and that the original publication in this journal is cited, in accordance with accepted academic practice. No use, distribution or reproduction is permitted which does not comply with these terms.
*Correspondence: Jian Gao, Z2FvamlhbmljYnJAMTYzLmNvbQ==
Disclaimer: All claims expressed in this article are solely those of the authors and do not necessarily represent those of their affiliated organizations, or those of the publisher, the editors and the reviewers. Any product that may be evaluated in this article or claim that may be made by its manufacturer is not guaranteed or endorsed by the publisher.
Research integrity at Frontiers
Learn more about the work of our research integrity team to safeguard the quality of each article we publish.