- 1School of Transportation and Logistics Engineering, Wuhan University of Technology, Wuhan, China
- 2CCCC Second Harbor Consultants Co., Ltd., Wuhan, China
- 3Engineering Technology Research Center for Aquatic Plant Resources and Utilization of Hubei Province, Wuhan, China
- 4Oceanic Bureau of Qinzhou, Qinzhou, China
- 5Yangtze River Nanjing Waterway Engineering Bureau, Nanjing, China
Introduction: Mangroves are the main carbon sinks in tropical regions and have high capabilities for carbon sequestration. Protection and restoration of mangroves are necessary to reduce carbon emissions and fight climate change. While the Qinzhou Bay as the main area of national mangrove restoration plan in the future, studies on its carbon pools, especially assessment of the carbon sink enhancement effect of restored mangroves along forest chronosequence, are lacking.
Methods: This study aimed to quantify the changes in restored mangrove soil carbon stock, vegetation and root carbon stocks along the forest age sequence in Qinzhou Bay through field survey.
Results: The results revealed that the carbon stocks of vegetation and roots significantly increased with the developing forest age. Only in the soil layer above 30 cm, the soil carbon storage apparently increased with the developing forest age in non-cofferdam area, and then decreased slowly after reaching the peak (at 6 ~ 8 years). Moreover, the soil carbon storage of mangroves was greater in the cofferdam area than in the non-cofferdam area.
Discussion: This implied that the cofferdam restoration efforts may be more effective in enhancing blue carbon storage, during the initial stages of the restoration process. The results of this study suggested that mangrove restoration has substantial potential capacity in carbon storage and nutrient cycling, providing a reference for the protection and restoration efforts concerning mangroves.
1 Introduction
Mangrove forests are pivotal coastal ecosystems in subtropical and tropical regions, providing numerous environmental services and crucial ecological functions that affect both coastal and oceanic resources (Giesen et al., 2007; Zhu et al., 2014; Castillo et al., 2017). They coexist with adjacent ecosystems such as coral reefs, seagrass beds and estuaries through physical, biochemical, and biological activities while remaining isolated (Lugo and Snedaker, 1974; Cooray et al., 2021a). Mangroves have developed a distinctive habitat because of their unique evolutionary process, providing a range of ecosystem services for fish and crustaceans, e.g., serving as nursery, breeding or feeding grounds, as well as providing protection from storms and tsunamis (Donato et al., 2011; Hilmi et al., 2017; Satyanarayana et al., 2017; Cooray et al., 2021a). Moreover, mangroves are highly productive and significantly contribute to carbon (C)storage (Bouillon et al., 2008; Donato et al., 2011; Hapsari et al., 2020).
Mangrove forests are the main carbon sinks in tropical regions and have high capabilities for carbon sequestration (Nellemann et al., 2009; Donato et al., 2011; Kauffman et al., 2011), about 50 times greater than that of many other tropical forests (Sandilyan and Kathiresan, 2012). Research has confirmed that coastal mangroves represent only 0.7% of global tropical forest cover but can store as much as 20 Pg C, which is approximately 2.5 times of the current annual global greenhouse gas emissions (Kauffman et al., 2017; Zhu and Yan, 2022). This remarkable carbon storage capability is attributed to the high levels of belowground biomass and considerable organic carbon storage in mangrove sediment soils (Sandilyan and Kathiresan, 2012), which could potentially mitigate rising atmospheric CO2 concentrations. Unfortunately, this vital ecosystem is under threat from various human activities, land-use change and natural calamities. According to statistics, global mangrove forests disappeared at a rate of 0.66% per year during the period of 2000–2005 (FAO, 2007). About 35% of the world’s mangroves were lost during 1980–2000 [Millennium Assessment (MA), 2005]. Even worse, it is predicted that existing mangroves may be seriously threatened by the relative sea-level rise (Gilman et al., 2008). If the current situation persists, mangroves could vanish within the next 100 years (Duke et al., 2007; Sandilyan and Kathiresan, 2012).
The protection and restoration of mangroves is a necessary way to reduce carbon emissions and fight climate change. Therefore, the evaluation of carbon storage in restored mangroves plays a pivotal guiding role in the implementation of mangrove restoration and conservation actions, and can also serve as a key indicator for assessing the effectiveness of mangrove restoration. However, the majority of recent studies predominantly concentrate on evaluating carbon storage within various natural mangrove ecosystems (Kauffman et al., 2014; Marchand, 2017; Wang et al., 2020), with only limited attention directed towards restored mangrove ecosystems (Song et al., 2023). Besides, the researches on the total carbon stocks of mangroves are very absent. Previous field studies of mangrove carbon storage were mainly restricted to a specific carbon storage component (vegetation biomass carbon or soil carbon) (Meng et al., 2021). More monitoring was conducted on the carbon stocks of mangrove vegetation biomass due to the convenience of the field survey (Hutchison et al., 2014; Hu et al., 2020; Wang et al., 2020), the estimation of belowground carbon stock remains uncertain. Existing studies have used allometric growth model to estimate the aboveground biomass carbon (AGC) and belowground root carbon (BRC) pools (Gunawardena et al., 2016; Perera and Amarasinghe, 2017, 2018; Cooray et al., 2018, 2021b; Wang et al., 2020). Nevertheless, the application of allometric biomass assessments, especially for belowground root biomass, may lead to substantial errors when extrapolated to sites characterized by environmental conditions divergent from those for which the models were initially developed (Cooray et al., 2021b). This discrepancy introduces a notable degree of uncertainty in local mangrove carbon storage estimations (Adamea et al., 2017). So, it is necessary to quantitatively estimate mangrove belowground root biomass carbon through field sampling to enhance the accuracy and reliability of carbon storage evaluations in diverse mangrove ecosystems.
Previous studies have indicated that both abiotic and biotic factors interactively drive the restoration efficiency and carbon sequestration capacity of mangroves. These factors include forest origin, forest age (Alongi et al., 2004; Song et al., 2023), soil condition, forest type and position in the tidal level (Alongi et al., 2005; Bouillon et al., 2008; Cooray et al., 2021b). The community-based research demonstrated that land-use changes in Puttalam Lagoon brought about a net carbon loss of 191,584 t C, which accounted for 75.5% of total carbon loss, mainly due to mangroves being converted into shrimp farms (Bournazel et al., 2015). In the worldwide deforested mangrove regions, reforestation in all sites with feasible habitat conditions might promote global absorption of 671.5–688.8 Tg CO2-eq globally over a 40-year period (Song et al., 2023). Furthermore, studies have indicated an increase in organic carbon storage of mangroves in the pedogenetic horizon in direct proportion to forest age. The range observed was from 4 to 107 Mg C ha−1 in the pioneering and aging stages, respectively (Marchand, 2017). Whereas, a meta-analysis showed that the carbon stock of mangroves was nonlinear with forest age because the AGB carbon accumulation rate first increased with age, peaked around 10 to 15 years, and then decreased (Song et al., 2023). The factors affecting the maximum carbon sequestration potential of mangroves were explored broadly. Nevertheless, a few studies focused on the change in the carbon storage in monoculture mangroves along the forest age gradient, and there are few studies to explore the differences in the total carbon storage of mangroves under different restoration ways, especially in Qinzhou Bay.
In this study, we conducted a field survey to determine how the soil C stocks of restored mangroves compare with the breeding tidal flats, and quantified the different carbon pools, including aboveground biomass carbon (AGC), belowground root biomass carbon (BRC), and soil carbon to a 1 m depth from 5 restored mangroves along forest age sequence. This study aimed (1) to estimate aboveground vegetation biomass carbon, root biomass carbon, and soil carbon stocks of restored mangroves at different forest ages; and (2) to analyze the impact of restoration ways, vegetation and soil attributes on mangrove aboveground and belowground carbon storage.
2 Methods
2.1 Study site description
This study was conducted in Qinzhou Bay situated in the south of the Guangxi Province (Figure 1), facing the Beibu Gulf. The area of the Bay in 2020 was 380 km2, with beaches accounting for approximately 200 km2 of the total area (Li et al., 2022). The zone had an obvious maritime climate with annual average precipitation of 1,600 mm and annual average temperature of 22°C, ensuring warm and humid conditions throughout the year. The annual evaporation was 1,498 mm, the relative humidity was 79% ~ 84%, and the frost-free period was 329 ~ 354 days. The study sites were identified between latitudes of 21°65′45″ ~ 21°70′85″ N and longitudes of 108°69′85″ ~ 108°73′27″ E. All six sites were established across Qinzhou Bay (Figure 1), including one oyster-breeding tidal flat (there are no mangroves in this area) and five restored mangroves (a 3-year-old mangrove in cofferdam area and 4 mangroves of different ages in non-cofferdam areas) with Aegiceras corniculatum as the sole constructive species.
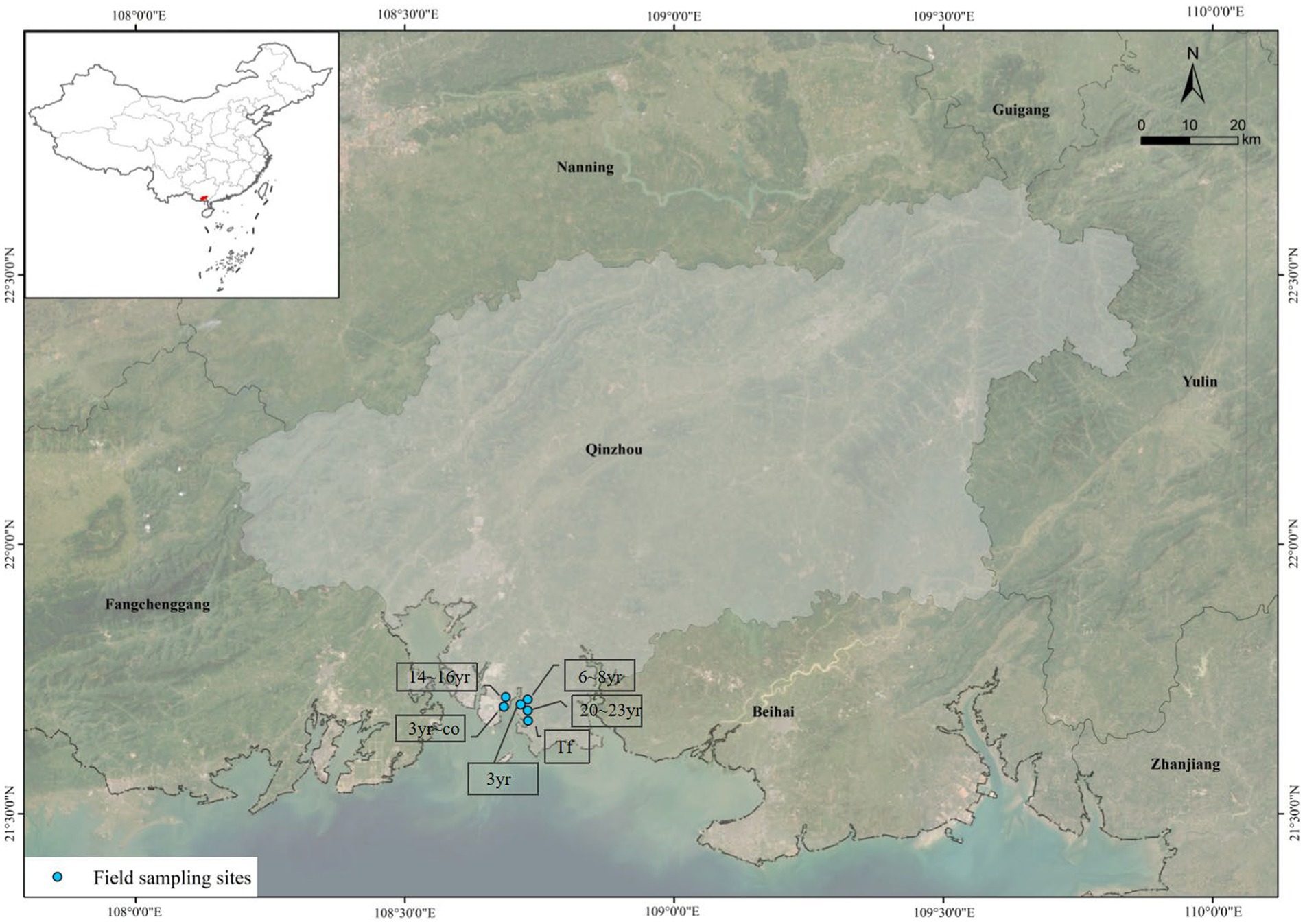
Figure 1. The location of sampling sites (Tf: Breeding tidal flat; 3 yr.: 3-year-old mangroves; 3 yr-co: 3-year-old mangroves in cofferdam area; 6 ~ 8 yr.: 6 ~ 8-year-old mangroves; 14 ~ 16 yr.: 14 ~ 16-year-old mangroves; 20 ~ 23 yr.: 20 ~ 23-year-old mangroves).
2.2 Field survey
Most of the mangrove forests in the identified study area were dominated by Aegiceras corniculatum. Besides, the mangroves were formed on tidal flats through natural and artificial regeneration. We estimated aboveground vegetation biomass and collected root and soil samples along a chronosequence of restored mangroves at various ages. Among the six sites where field sampling was conducted for carbon storage calculation, three 20 m × 20 m plots were established at each site. Banded quadrats located at high tide levels. The field survey and sampling were conducted in late May 2023. All the mangrove trees (Diameter at breast height (DBH) ≥2.5 cm), saplings (DBH < 2.5 cm and/or 30 cm < height < 130 cm) and seedlings (height ≤ 30 cm) in each plot were enumerated, and their respective species were recorded (Cooray et al., 2021a). Subsequently, the aboveground vegetation attributes, stand basal area (BA) and stand density index (SDI) were estimated for each plot.
2.3 Field sampling and analyses
We collected root and soil samples in each plot to analyze their physical–chemical properties in the laboratory. The soil samples were collected from four depth intervals: 0–10 (topsoil), 10–30 (midsoil), 30–60 (subsoil), and 60–100 cm (deepsoil) after removal of surface litter at each plot (Castillo et al., 2017) (Figure 2). Soil bulk density (SBD) was estimated by the cutting ring method consistent with the soil layers (Dai et al., 2018). Samples were taken from three separate 0.3 m × 0.3 m quadrats and homogenized according to the different soil layers at each plot. Soil layers were measured at the actual depth if the total soil depth was less than 100 cm. Similar to soil sampling, fine root samples were collected using a steel soil corer (3.8 cm in diameter) within a 1-m range from the main trunk.
Each fine root sample was washed through a 1-mm sieve and all dead and live roots were picked out. These fine roots were oven-dried at 65°C to attain a constant weight. Meanwhile, all soil samples were dried at 105°C until they reached a constant weight. Subsequently, animal residues were removed from the soil samples using a 2-mm sieve. Later, partial root and soil samples were ground with a grinder and passed through a 100-mesh sieve for laboratory analysis (Hatton et al., 2015). The organic C and total nitrogen content were determined using an elemental analyzer (Flash 2000, Thermo Fisher Scientific Inc., Waltham, MA, United States).
2.4 Aboveground biomass carbon
The AGB of mangroves was calculated by the following allometric Eq. (1) for Aegiceras corniculatum (Tam et al., 1995), and aboveground carbon stock (AGC) was estimated using the Eq. (2) (Cooray et al., 2021b).
Where, AGB and AGC is in kg, DBH is in cm, H (height) is in m.
2.5 Belowground carbon stocks
Soil bulk density was calculated as the mass of the oven-dried soil per volume of soil (Keller and Håkansson, 2010), and the soil organic carbon (SOC) stock of the four layers was estimated using the following Eq. (3):
Where, St is the soil organic carbon stocks at depth t (kg C ha−1), t is the tth layer; Dt is the SBD (g cm−3); Ct is the SOC concentration (g kg−1); and Ht is the thickness of the tth soil layer (cm).
The dried weights of mangrove roots were multiplied by 0.39 Eq. (4) (Kauffman and Donato, 2012; Cooray et al., 2021b) to obtain corresponding root carbon stocks.
Where, BRC is the belowground root carbon; ODW root is the oven-dried weight of mangrove roots.
2.6 Statistical analyses
Excel 2019, the SPSS 20, and R statistical software (R v3.6.1) were used for statistical analyses. The effect of mangrove restoration on blue carbon storage was analyzed by comparing the total carbon storage of breeding tidal flats and restored mangroves. By comparing the mangroves carbon stocks in non-cofferdam areas, the impacts of forest age on carbon storage from different component carbon pools were estimated. The mangrove carbon stocks in cofferdam areas were compared with those in non-cofferdam areas to analyze the impacts of restoration ways on carbon stocks of mangrove ecosystems. The relationship between forest age and carbon stock is analyzed by taking the middle value within the range of forest age. One-way ANOVA was used to test the significant differences among variables, followed by post hoc comparisons using the least significant difference (LSD). The average carbon stocks of belowground and total culms in all sampling sites were calculated. Spearman’s rank correlation analysis was employed from the R “corrplot” package to preliminarily examine the covarying environmental factors and the relationship between all environmental variables and carbon storage in different components (Huang et al., 2022). Linear regression analysis was used in this study to analyze the relationships between the environmental variables and carbon stocks in different components.
3 Results
3.1 Stand properties and aboveground carbon stock
Stand density index, stand basal area, AGB and AGC stock all increased with the developing forest age (Table 1). AGB of mangrove forests ranged from 481.35 to 8445.69 kg C ha−1, and AGC ranged from 231.05 to 4101.93 kg C ha−1 (excluding tidal flat). The aboveground vegetation biomass and carbon storage of mature mangroves (forest age ≥ 14 yr) were much greater than those of other sites.
3.2 Soil carbon stocks
The SOC stocks ranged from 8266.5 to 15,834.2, 21,756.0 to 40,932.4, 25,253.3 to 59,277.4, and 36,342.0 to 41,409.6 kg C ha−1 in the 0–10, 10–30, 30–60 and 60–100 cm soil layers, respectively (Figure 3; Table 2). In the soil layer above 30 cm, the soil carbon storage of mangroves restored in the non-cofferdam area increased slightly with the forest age, peaked in about 6–8 years, and then decreased. However, this trend was not significant in the soil layer below 30 cm (Figure 3). The SOC storage of 3-year-old mangroves restored in the cofferdam area was greater than that in other sites at all soil layers, with a total soil carbon storage of up to 188040.62 kg C ha−1 (Table 2). Overall, the SOC stocks were significantly different across the soil depth (F = 267.07, p < 0.0001) and increased with the soil depth at all sites (Table 2).
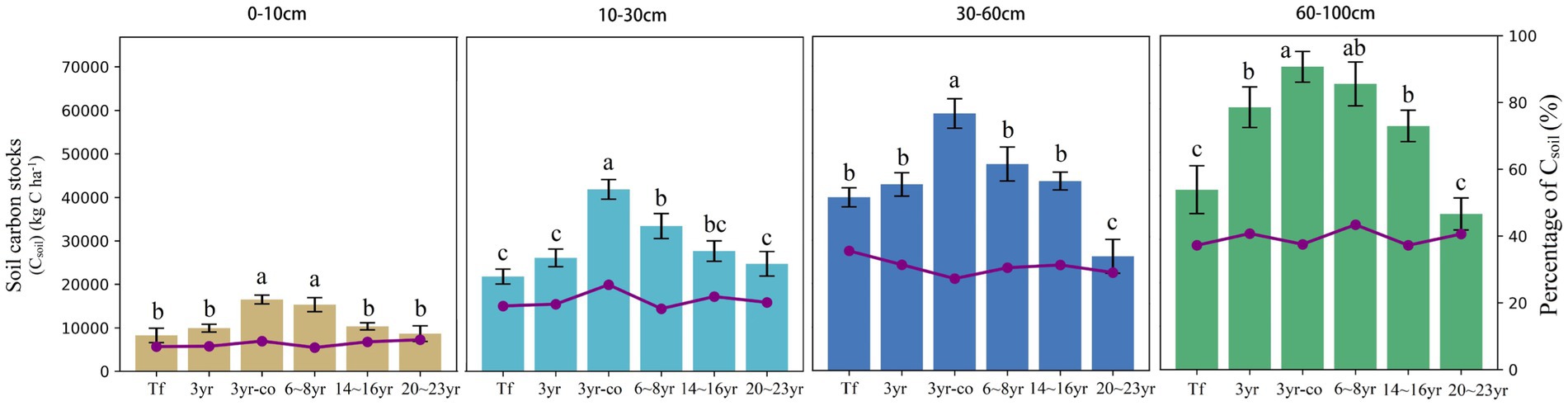
Figure 3. Soil carbon stocks of mangroves across four soil layers (column), and the percentage of soil carbon stock at each soil layer relative to the total soil carbon storage of all soil layers (polyline) in different sample sites. Different letters in the same soil layer are obviously different at different sample points (p < 0.05) (Tf: Breeding tidal flat; 3 yr.: 3-year-old mangroves; 3 yr-co: 3-year-old mangroves in cofferdam area; 6 ~ 8 yr.: 6 ~ 8-year-old mangroves; 14 ~ 16 yr.: 14 ~ 16-year-old mangroves; 20 ~ 23 yr.: 20 ~ 23-year-old mangroves) (Csoil) (kg C ha−1).
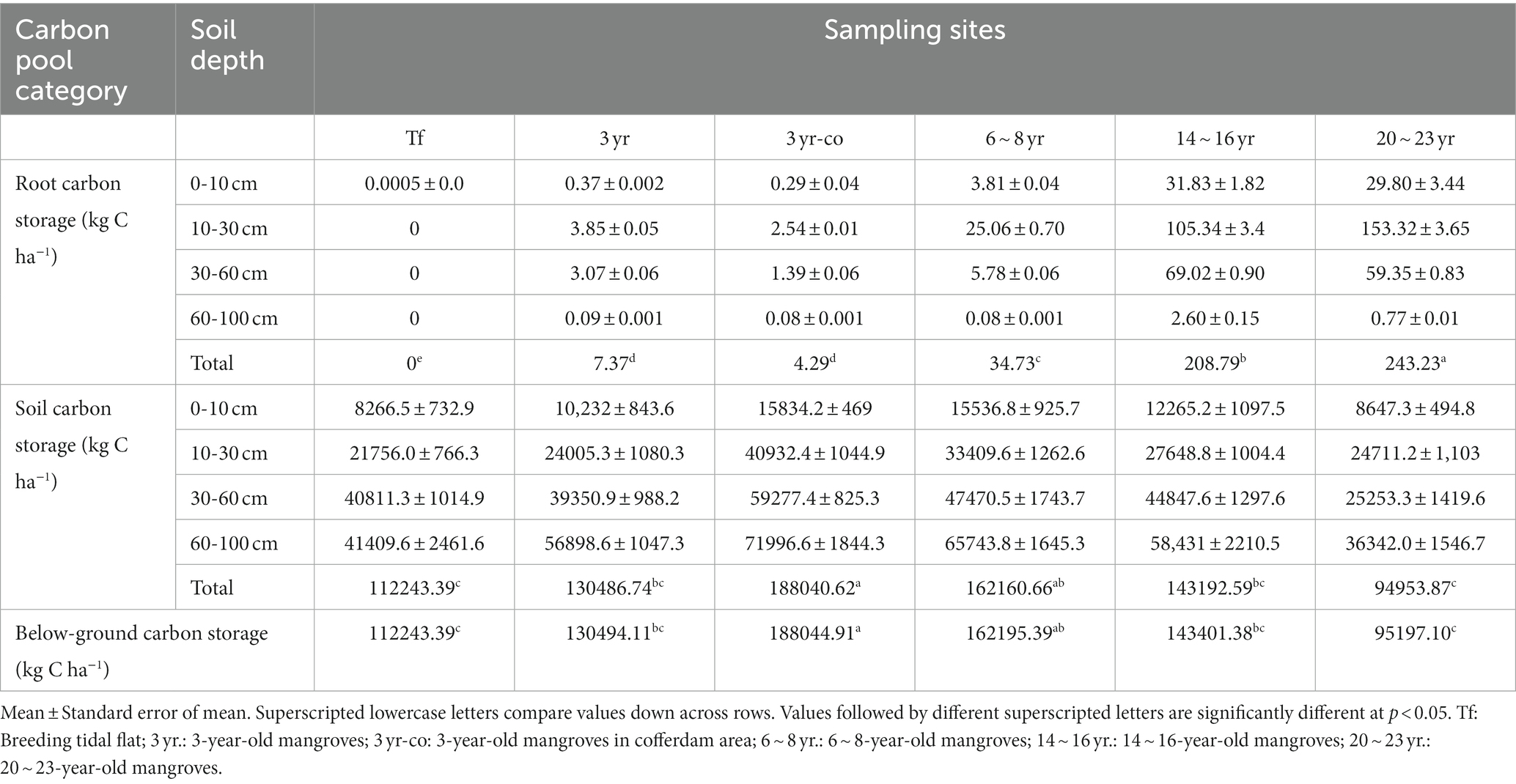
Table 2. The vertical distributions of belowground soil carbon and root carbon stocks of mangroves studied.
3.3 Belowground carbon stock
The total root carbon storage ranged from 4.29 kg C ha−1 in 3-year non-cofferdam area to 243.23 kg C ha−1 in 20 ~ 23-year area (Table 2). Total root carbon storage of 3-year-old mangroves (except for breeding tidal flat) was markedly lower than that at other sampling sites, with values of 4.29 and 7.37 kg C ha−1, respectively. In the vertical direction, combining all sampled sites, the soil depth was shown to be a highly conspicuous predictor of root carbon stock (F = 522.2, p < 0.0001). Almost 50.45% ~ 72.16% of the root carbon stock was concentrated in the 10–30 cm soil layer.
Carbon stock estimation for the whole belowground of mangroves (sum of root carbon and soil carbon) ranged from 95197.10 kg C ha−1 in 20 ~ 23 yr. area to 188044.91 kg C ha−1 in 3-yr cofferdam area (Table 2). At the same time, the total belowground carbon stock at 3-yr cofferdam zone, of 188044.91 kg C ha−1, was notably greater than that at other sites (p < 0.05), excluding 6 ~ 8 yr. site (p > 0.05) (Table 2).
3.4 Total carbon stock
For all sites, the total carbon stocks of mangroves were 112,243.70, 130,681.20, 188,308.29, 162,922.53, 145,837.51, and 99,392.85 kg C ha−1 in the tidal flat, 3-year, 3-year-cofferdam, 6 ~ 8 year, 14 ~ 16 year and 20 ~ 23 year sites, respectively (Figure 4). Besides, the total carbon stock of mangroves at 3-year cofferdam area was dramatically greater than that at other sites (p < 0.05), except in 6 ~ 8 year site (p > 0.05). The soil carbon pools dominated in their contribution to total carbon storage with 95.53 to 99.85% of the total ecosystem carbon storage of the sampled mangrove sites, while the contribution of aboveground vegetation and root biomass carbon stocks ranged from 0.14 to 4.22% and from 0.006 to 0.24%, respectively.
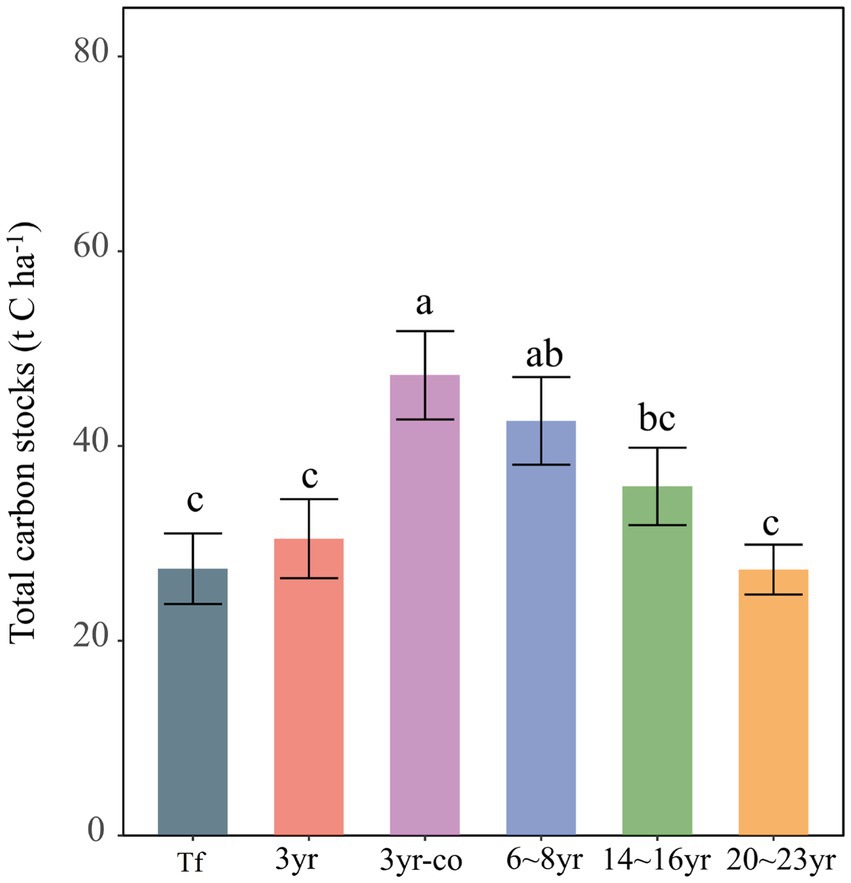
Figure 4. Total carbon stocks in six sampling sites. Different lowercase letters indicate significantly different at p < 0.05 (Tf: Breeding tidal flat; 3 yr.: 3-year-old mangroves; 3 yr-co: 3-year-old mangroves in cofferdam area; 6 ~ 8 yr.: 6 ~ 8-year-old mangroves; 14 ~ 16 yr.: 14 ~ 16-year-old mangroves; 20 ~ 23 yr.: 20 ~ 23-year-old mangroves).
3.5 Relationship between above- and below-ground carbon stock and various variables
Spearman’s rank correlation analysis showed that the stand attributes were positively correlated with AGC and RGC (Figure 5). Further linear regression analysis confirmed that the AGC was positively related to SDI (Radj2 = 0.97, p < 0.001; Figure 6A) and forest age (Radj2 = 0.98, p < 0.001; Figure 6B); meanwhile, root biomass carbon stocks significantly increased with the development of AGC (Radj2 = 0.41, p < 0.001; Figure 6C) and forest age (Radj2 = 0.37, p < 0.001; Figure 6D).
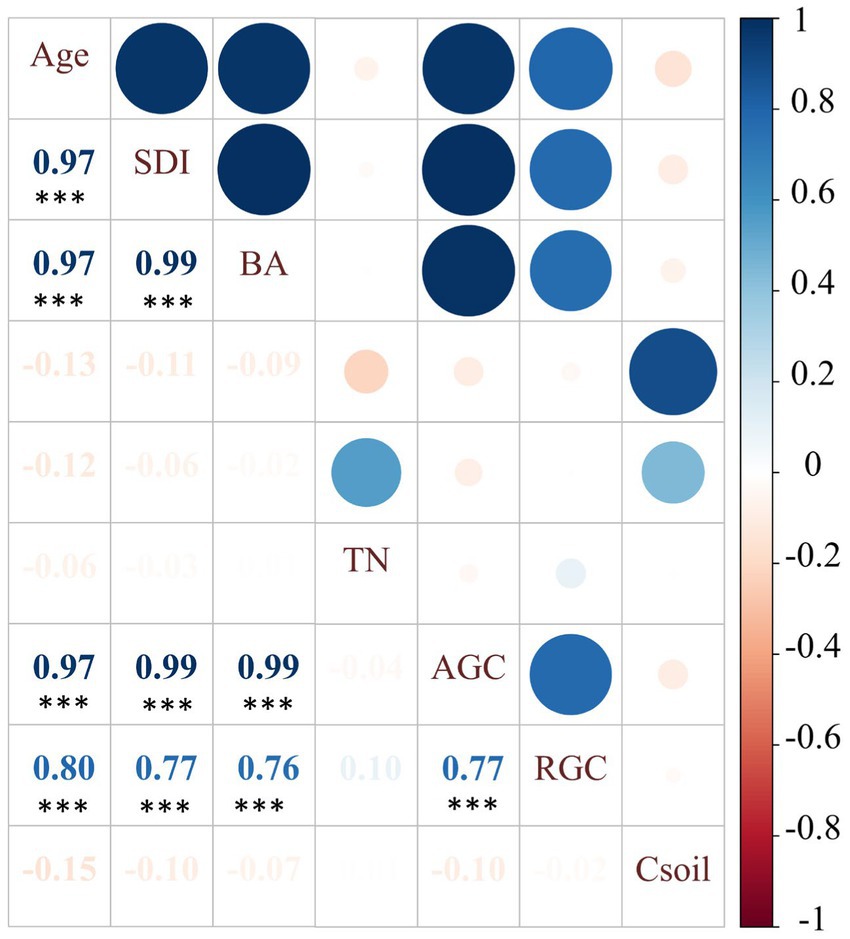
Figure 5. Pairwise correlations among all variables (Age: forest age; SDI: stand density index; BA: stand basal area; TN: soil total nitrogen content; AGC: aboveground carbon stock; RGC: root biomass carbon stock; Csoil: soil carbon stocks). Asterisks indicate that the correlations are significant, **p < 0.01, ***p < 0.001. The digit of the square is proportional to the correlation coefficient.
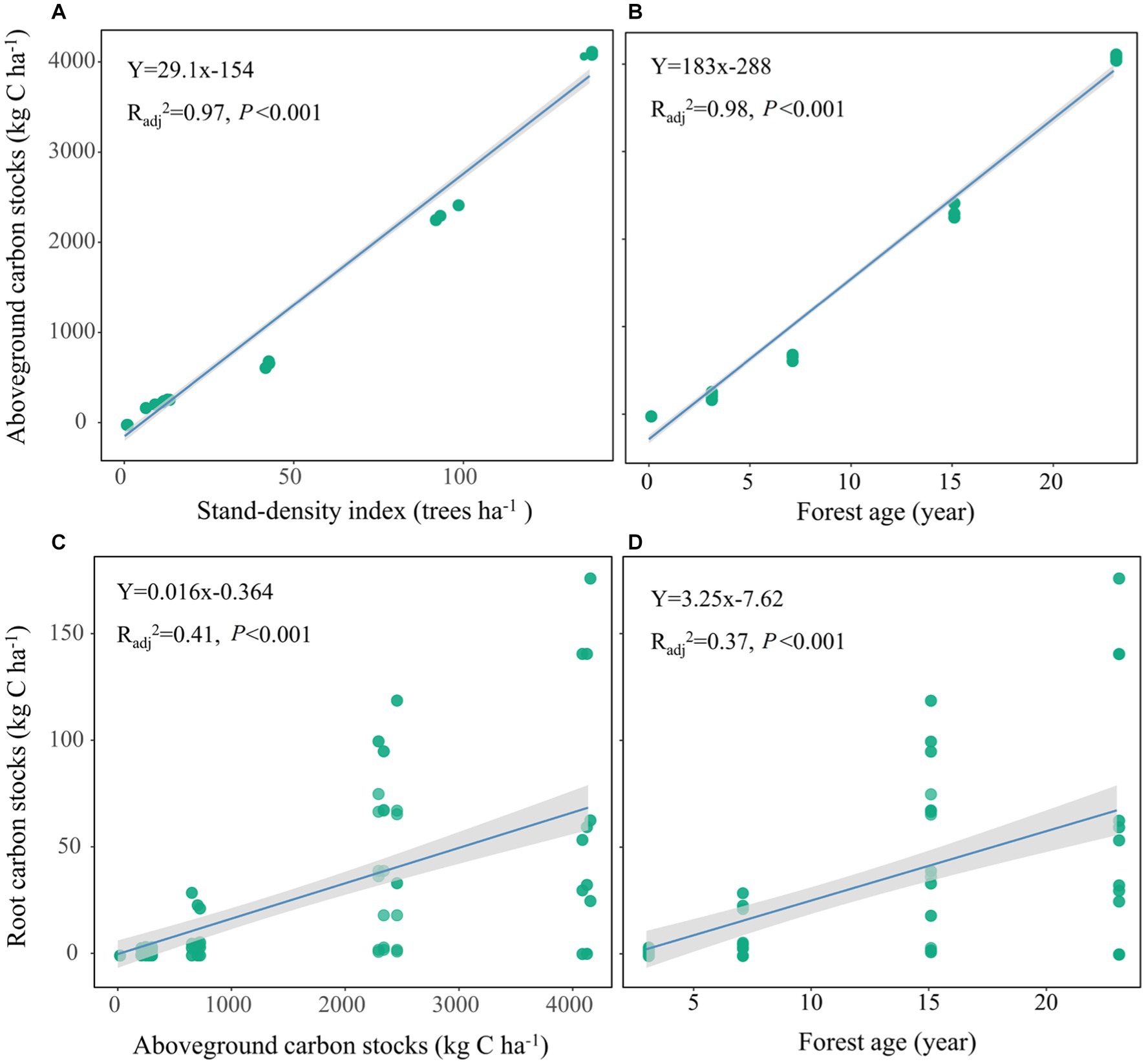
Figure 6. Relationships between aboveground vegetation attributes and aboveground carbon stocks (A,B) and root carbon stocks (C,D). Shaded areas show the 95% confidence intervals of the fitted lines.
4 Discussion
4.1 Aboveground biomass carbon stock increased with the development of forest age
Forest age was a primary factor in affecting the aboveground vegetation carbon stocks. AGB, AGC, SDI and BA all increased gradually with the developing forest age. This result was consistent with previous studies (Fonseca et al., 2011; Cheng et al., 2015; Adamea et al., 2017; Marchand, 2017; Song et al., 2023). Wang et al. (2017) suggested that the major determinant of vegetation carbon turnover times for all forest types was the forest age. Additionally, Xu et al. (2018) found that the second most important factor contributing to vegetation carbon storage in subtropical forests was forest age. Many previous studies supported this finding, attributing it to the increase in the aboveground vegetation biomass with forest age caused by the growth in individual tree mass (Alongi, 2002; Analuddin et al., 2009; Deshar et al., 2012; Walcker et al., 2018; Estrada-Villegas et al., 2020). Moreover, massive accumulation of AGB would contribute much more leaf litter to the sediment in reforestation sites (Song et al., 2023). Compared with the breeding tidal flat, mangrove reforestation thus obviously may be an effective strategy for carbon sequestration, supporting climate change mitigation actions.
4.2 Influencing factors and vertical distribution of root biomass carbon stock
The belowground root biomass carbon stock of mangroves dramatically increased with the development of the stand age, but decreased with soil depth. Alongi and Dixon (2000) reported that the root biomass of mangrove forest restored in 5-year-old was 23.1 t ha−1, which increased to 35.6 t ha−1 in 25-year-old. Similarly, the root biomass of 6-year-old plantation was 7.5 t ha−1, which increased to 24.9 t ha−1 in 12-year-old (Tamooh et al., 2008; Adamea et al., 2017). The potential for root biomass growth largely depends on the development of aboveground vegetation.
In northern Australian mangroves, 80% of the root biomass was concentrated in the upper layer of soil (Boto and Wellington, 1984). The findings of this study revealed that 57.30% ~ 83.13% of the fine root biomass carbon storage was mainly distributed at the 0–10 cm and 10–30 cm soil layer. This vertical distribution pattern of the fine root biomass carbon stock may be attributed to the higher soil nutrient concentration in the surface soil as opposed to the deeper soil layers (McKee, 2011; Adame et al., 2014). In this study, SOC and the total nitrogen content decreased memorably with depth at all sites (Supplementary Table S1). The distributions of fine root biomass in all sites were consistent with those of soil nitrogen across the soil depth, which was attributed to the fact that nitrogen had been implicated as the nutrient most likely to limit growth in anoxic soil conditions (Reef et al., 2010). Furthermore, we observed a predominant distribution of aerial roots and noted the presence of dense crab burrows in the topsoil during the field investigation. Arnaud et al. (2021) thought that the shallowest soil layer is more aerobic than the deeper layer due to the radial loss of oxygen by roots and oxygenation of the soil through animal burrows is another possible explanation for the variation in the soil depth of the mangrove root biomass carbon storage. It has also been suggested that an opportunistic distribution of mangrove fine roots might occur at shallow profiles to enhance the absorption of precipitation originating fresh water (Reef and Lovelock, 2015).
4.3 Impact of environmental factors on soil carbon storage
In the soil layer above 30 cm, the soil carbon storage of mangroves restored in the non-cofferdam area apparently increased with the developing forest age, and then decreased slowly after reaching the peak. However, this trend was not significant in the soil layer below 30 cm. Song et al. (2023) also found that the total soil carbon storage followed an increasing and then decreasing trend in a chronosequence of reforested mangroves. Perhaps due to the plant carbon sink capacity declines with stand age, and the soil carbon sequestration rate remains constant or even decreased over many years (Walcker et al., 2018). Besides, the soil pool size of most elements decreases with increasing stand age in the mature mangroves (Alongi et al., 2004). This trend mainly occurred at 0–30 cm soil layers, which could be due to the more aerobic surroundings in the shallow soil profiles (Kwon et al., 2019), the microbial activity was more sensitive to elemental changes. In general, this pattern must be considered with caution owing to the limited number of samples at the same age, although current studies on many other forest ecosystems showed that the concentration of elements in tree components decreased with the stand age at the mature stage (Folster and Khanna, 1997; Song et al., 2023). It is still necessary to further explore the internal mechanism of microbial community changes.
For land-use changes, mangrove restoration could promote the potential ability of carbon storage in tidal flats. After the tidal flats were converted into mangroves, the vegetation and root biomass carbon storage significantly increased with the developing mangrove forests (Figure 6). The decomposition of leaf and fine root litter constitutes the primary pathway for mangroves to return the C to soils (Schlesinger, 1977; Guo et al., 2021). Mangrove carbon accumulation occurred at both the tree and litter layers, bringing about more organic carbon storage in soils. Recent studies indicated that removing mangrove forests undoubtedly reduced future storage capacity and may cause the partial or total loss of carbon sink (Bournazel et al., 2015). Our study found that the total soil carbon stocks of 20 ~ 23-year-old restored mangroves were markedly lower than other sites in the soil layer below 30 cm. This difference can be mainly attributed to the obvious presence of calcified hardening in the soil below 30 cm layers (the phenomenon was visibly observed in field sampling).
The soil carbon storage of mangroves in the cofferdam restoration area was notably greater than that in other sites, especially the total soil carbon storage of mangroves in 3-year-old cofferdam area was almost 1.4 times higher than that in the 3-year-old non-cofferdam area, indicating that the cofferdam restoration would predominantly improve carbon storage capacity. Current study proved that the loss of soil dissolved organic carbon increased with the enhancement of inundation frequency, and the soil dissolved organic carbon loss in the surface was significantly greater than that in the subsurface (Li et al., 2020). Besides, the input of tidal organic carbon could accelerate the SOC mineralization in wetlands, thereby weakening carbon sequestration (Yan et al., 2023). However, soil nutrients in the cofferdam area remain relatively unaffected by tidal inundation (Hasan et al., 2022), which likely accounts for the higher soil carbon storage in the cofferdam area compared with other sites. This study recommends that future studies should combine the microbial community structure and older forests in the cofferdam mangrove restoration area to assess whether cofferdam restoration will promote long-term carbon sequestration capacity.
5 Conclusion
Overall, the distribution trend of the mangrove total carbon storage at various sites was consistent with the distribution of total soil carbon storage. The aboveground vegetation and belowground root carbon stocks significantly increased with the developing forest age. In the soil layer above 30 cm, the mangrove soil carbon storage restored in the non-cofferdam area apparently increased with the developing forest age, and then it decreased slowly after reaching the peak (at 6 ~ 8 years). But this trend was not significant in soil layer below 30 cm. Mangrove reforestation could obviously enhance carbon storage compared with maintaining breeding tidal flats. In addition, the soil carbon storage of mangroves in the cofferdam restoration area was greater than in the non-cofferdam restoration area, implying that cofferdam restoration may be more effective for improving blue carbon storage along the coastline at the initial stages of restoration. Therefore, it is recommended that future studies include older forests and cofferdam restoration sites of various ages to determine whether mangrove carbon storage will increase from a long-term perspective.
Data availability statement
The original contributions presented in the study are included in the article/Supplementary material, further inquiries can be directed to the corresponding author.
Author contributions
WS: Writing – original draft, Formal analysis. YH: Data curation, Writing – original draft. WZ: Resources, Validation, Writing – review & editing. YF: Methodology, Writing – review & editing. HX: Investigation, Writing – original draft. CC: Investigation, Writing – original draft. GL: Visualization, Writing – original draft. LH: Writing – review & editing, Supervision, Visualization.
Funding
The author(s) declare financial support was received for the research, authorship, and/or publication of this article. This study received funding from the CCCC Second Harbor Consultants Co., Ltd. The funder was not involved in the study design, collection, analysis, interpretation of data, the writing of this article or the decision to submit it for publication.
Acknowledgments
We are grateful for the valuable help of Oceanic Bureau of Qinzhou, Yangtze River Nanjing waterway engineering Bureau and other colleagues who assisted with field work and samples handling. All authors declare no other competing interests.
Conflict of interest
WS, YH, YF, GL, and LH were employed by CCCC Second Harbor Consultants Co., Ltd.
The remaining authors declare that the research was conducted in the absence of any commercial or financial relationships that could be construed as a potential conflict of interest.
Publisher’s note
All claims expressed in this article are solely those of the authors and do not necessarily represent those of their affiliated organizations, or those of the publisher, the editors and the reviewers. Any product that may be evaluated in this article, or claim that may be made by its manufacturer, is not guaranteed or endorsed by the publisher.
Supplementary material
The Supplementary material for this article can be found online at: https://www.frontiersin.org/articles/10.3389/ffgc.2024.1328783/full#supplementary-material
References
Adamea, M. F., Cherian, S., Reef, R., and Stewart-Kostera, B. (2017). Mangrove root biomass and the uncertainty of belowground carbon estimations. For. Ecol. Manag. 403, 52–60. doi: 10.1016/j.foreco.2017.08.016
Adame, M. F., Teutli, C., Santini, N. S., Caamal, J. P., Zaldívar-Jiménez, A., Hernández, R., et al. (2014). Root biomass and production of mangroves surrounding a karstic oligotrophic coastal lagoon. Wetlands 34, 479–488. doi: 10.1007/s13157-014-0514-5
Alongi, D. M. (2002). Present state and future of World's mangrove forests. Environ. Conserv. 29, 331–349. doi: 10.1017/S0376892902000231
Alongi, D. M., Clough, B. F., and Robertson, A. I. (2005). Nutrient-use efficiency in arid-zone forests of the mangroves Rhizophora stylosa and Avicennia marina. Aquat. Bot. 82, 121–131. doi: 10.1016/j.aquabot.2005.04.005
Alongi, D. M., and Dixon, P. (2000). Mangrove primary production and above- and below-ground biomass in Sawi Bay, southern Thailand. Phuket Marine Biology Centre Special Publication, 22, 31–38.
Alongi, D. M., Wattayakorn, G., Frank, T., and Paul, D. (2004). Nutrient capital in different aged forests of the mangrove Rhizophora apiculata. Bot. Mar. 47, 116–124. doi: 10.1515/BOT.2004.011
Analuddin, K., Suwa, R., and Hagihara, A. (2009). The self-thinning process in mangrove Kandelia obovate stands. J. Plant Res. 122, 53–59. doi: 10.1007/s10265-008-0190-8
Arnaud, M., Morris, P. J., Baird, A. J., Dang, H., and Nguyen, T. T. (2021). Fine root production in a chronosequence of mature reforested mangroves. New Phytol. 232, 1591–1602. doi: 10.1111/nph.17480
Boto, K. G., and Wellington, J. T. (1984). Soil characteristics and nutrient status in a northern Australian mangrove forest. Estuaries 7, 61–69. doi: 10.2307/1351957
Bouillon, S., Borges, A. V., Castañeda-Moya, E., Diele, K., Dittmar, T., Duke, N. C., et al. (2008). Mangrove production and carbon sinks: a revision of global budget estimates. Glob. Biogeochem. Cycl. 22. doi: 10.1029/2007GB003052
Bournazel, J., Marappullige, P. K., Jayatissa, L. P., Viergever, K., Morel, V., and Huxham, M. (2015). The impacts of shrimp farming on land-use and carbon storage around Puttalam lagoon, Sri Lanka. Ocean Coastal Manag. 113, 18–28. doi: 10.1016/j.ocecoaman.2015.05.009
Castillo, J. A. A., Apan, A. A., Maraseni, T. N., and Salmo, S. G. (2017). Soil C quantities of mangrove forests, their competing land uses, and their spatial distribution in the coast of Honda Bay. Philippines. Geoderma. 293, 82–90. doi: 10.1016/j.geoderma.2017.01.025
Cheng, J. Z., Lee, X. Q., Theng, B. K. G., Zhang, L. K., Fang, B., and Li, F. S. (2015). Biomass accumulation and carbon sequestration in an age-sequence ofZanthoxylum bungeanum plantations under the Grain for Green Program in karst regions, Guizhou province. Agric. For. Meteorol. 203, 88–95.
Cooray, P. L. I. G. M., Jayawardana, D. T., Gunathilake, B. M., and Pupulewatte, P. G. H. (2021a). Characteristics of tropical mangrove soils and relationships with forest structural attributes in the northern coast of Sri Lanka. Reg. Stud. Mar. Sci. 44, 101741–104855. doi: 10.1016/j.rsma.2021.101741
Cooray, P. L. I. G. M., Kodikara, K. A. S., Kumara, M. P., Jayasinghe, U. I., Madarasinghe, S. K., and Dahdouh-Guebas, F. (2021b). Climate and intertidal zonation drive variability in the carbon stocks of Sri Lankan mangrove forests. Geoderma 389, 114929–117061. doi: 10.1016/j.geoderma.2021.114929
Cooray, P. L. I. G. M., Marynathan, E., and Prasad, S. A. A. (2018). Species diversity and vegetation structure of mangroves in Vidattaltivu, Mannar, Sri Lanka, in: Subasinghe, S.M.C.U.P., Perera, P.K.P., Chandratilake, G.G.T., Jayasooriya, V. (Eds.), Proceedings of the 23rd international forestry and environment symposium, Sri Lanka: Department of forestry and environmental science. University of Sri Jayewardenepura. pp. 133.
Dai, W., Fu, W. J., Fu, W., Jiang, P., Zhao, K., Li, Y., et al. (2018). Spatial pattern ofcarbon stocks in forest ecosystems of a typical subtropical region of southeastern China. For. Ecol. Manag. 409, 288–297. doi: 10.1016/j.foreco.2017.11.036
Deshar, R., Sharma, S., Mouctar, K., Wu, M., Hoque, A. T. M. R., and Hagihara, A. (2012). Self-thinning exponents for partial organs in overcrowded mangrove Bruguiera gymnorrhiza stands on Okinawa Island, Japan. Forest Ecol. Manag. 278, 146–154. doi: 10.1016/j.foreco.2012.05.007
Donato, D. C., Kauffman, J. B., Murdiyarso, D., Kurnianto, S., Stidham, M., and Kanninen, M. (2011). Mangrove among the most carbon-rich forests in the tropics. Nat. Geosci. 4, 293–297. doi: 10.1038/ngeo1123
Duke, N. C., Meynecke, J. O., Dittmann, S., et al. (2007). A world without mangroves? Science 17, 41–42. doi: 10.1126/science.317.5834.41b
Estrada-Villegas, S., Bailón, M., Hall, J. S., Schnitzer, S. A., Turner, B. L., Caughlin, T., et al. (2020). Edaphic factors and initial conditions influence successional trajectories of early regenerating tropical dry forests. J. Ecol. 108, 160–174. doi: 10.1111/1365-2745.13263
Folster, H., and Khanna, P. K. (1997). “Dynamics of nutrient supply in plantation soils” in Management of soil, nutrients and water in tropical plantations. eds. E. K. S. Nambiar and A. G. Brown, vol. 43 (Canberra: ACIAR Monograph), 339–378.
Fonseca, W., Benayas, J. M. R., and Alice, F. E. (2011). Carbon accumulation in the biomass and soil of different aged secondary forests in the humid tropics of Costa Rica. For. Ecol. Manag. 262, 1400–1408.
Giesen, W., Wulffraat, S., Zieren, M., and Scholten, L. (2007). Mangrove guidebook for Southeast Asia. Food and Agricultural Organisation and Wetlands International, Bangkok, Thailand. 769p.
Gilman, E., Ellison, J., Duke, N. C., and Field, C. (2008). Threats to mangroves form climate change and adaptation options: a review. Aquat. Bot. 89, 237–250. doi: 10.1016/j.aquabot.2007.12.009
Gunawardena, A. R., Nissanka, S. P., Dayawansa, N. D. K., and Fernando, T. T. (2016). Above ground biomass estimation of mangroves located in Negombo-Muthurajawela wetland in Sri Lanka using ALOS PALSAR images. Tropical Agric. Res. 27, 137–146. doi: 10.4038/tar.v27i2.8162
Guo, L., Deng, M., Yang, S., Liu, W., Wang, X., Wang, J., et al. (2021). The coordination between leaf and fine root litter decomposition and the difference in their controlling factors. Glob. Ecol. Biogeogr. 30, 2286–2296. doi: 10.1111/geb.13384
Hapsari, K. A., Jennerjahn, T. C., Lukas, M. C., Karius, V., and Behling, H. (2020). Intertwined effects of climate and land use change on environmental dynamics and carbon accumulation in a mangrove-fringed coastal lagoon in Java, Indonesia. Glob. Change Biol. 26, 1414–1431.
Hasan, J., Chandra Shaha, D., Rani kundu, S., Ahmed, M., Haque, S. M., Haque, F., et al. (2022). Outwelling of nutrients into the Pasur River estuary from the Sundarbans mangrove creeks. Heliyon 8, e12270–e18440. doi: 10.1016/j.heliyon.2022.e12270
Hatton, P. J., Castanha, C., Torn, M. S., and Bird, J. A. (2015). Litter type control on soil C and N stabilization dynamics in a temperate forest. Glob. Chang. Biol. 21, 1358–1367. doi: 10.1111/gcb.12786
Hilmi, E., Kusmana, C., Suhendang, E., and Iskandar, I. (2017). Correlation analysis between seawater intrusion and mangrove greenbelt. Indonesian J. Forestry Res. 4, 151–168. doi: 10.20886/ijfr.2017.4.2.151-168
Huang, L., Zhao, R. D., Zhao, X. X., et al. (2022). Effects of stand condition and root density on fine root dynamics across root functional groups in a subtropical montane forest. J. For. Res. (Harbin) 34, 665–675. doi: 10.1007/s11676-022-01514-0
Hutchison, J., Manica, A., Swetnam, R. D., Balmford, A., and Spalding, M. (2014). Predicting global patterns in mangrove forest biomass. Conserv. Lett. 7, 233–240. doi: 10.1111/conl.12060
Hu, T. Z., Ying, Y., Yi, Z., Yanjun, S., Guanghui, L., and Qinghua, G. (2020). Mapping the global mangrove forest aboveground biomass using multisource remote sensing data. Remote Sens. 12:1690. doi: 10.3390/rs12101690
Kauffman, J. B., Arifanti, V. B., Hernandez Trejo, H., Del Carmen Jesús García, M., Norfolk, J., Cifuentes, M., et al. (2017). The jumbo carbon footprint of a shrimp: carbon losses from mangrove deforestation. Front. Ecol. Environ. 15, 183–188. doi: 10.1002/fee.1482
Kauffman, J. B, and Donato, D. C. (2012). Protocols for the measurement, monitoring and reporting of structure, biomass and carbon stocks in mangrove forests. Working Paper 86. CIFOR, Bogor, Indonesia.
Kauffman, J. B., Heider, C., Cole, T. G., Dwire, K. A., and Donato, D. C. (2011). Ecosystem carbon stocks of Micronesian mangrove forests. Wetlands 31, 343–352. doi: 10.1007/s13157-011-0148-9
Kauffman, J. B., Heider, C., Norfolk, J., and Payton, F. (2014). Carbon stocks of intact mangroves and carbon emissions arising from their conversion in the Dominican Republic. Ecol. Appl. 24, 518–527. doi: 10.1890/13-0640.1
Keller, T., and Håkansson, I. (2010). Estimation of reference bulk density from soil particle size distribution and soil organic matter content. Geoderma 154, 398–406. doi: 10.1016/j.geoderma.2009.11.013
Kwon, M. J., Natali, S. M., Hicks Pries, C. E., Schuur, E. A. G., Steinhof, A., Crummer, K. G., et al. (2019). Drainage enhances modern soil carbon contribution but reduces old soil carbon contribution to ecosystem respiration in tundra ecosystems glob. Change Biol. 25, 1315–1325. doi: 10.1111/gcb.14578
Li, J. Y., Han, G. X., Zhao, M. L., Qu, W. D., Nie, M., Song, W. M., et al. (2020). Nitrogen input weakens the control of inundation frequency on soil organic carbon loss in a tidal salt marsh. Estuarine. Coast. Shelf Sci. 243, 106878–107714. doi: 10.1016/j.ecss.2020.106878
Li, P., Du, J., Zhang, Z., and Xu, G. (2022). Sedimentological analysis of regional differentiation and sediment provenance in the Lu’erhuan River Sea area of Qinzhou Bay, Guangxi Province. J. Marine Sci. Eng. 10:1732. doi: 10.3390/jmse10111732
Lugo, A. E., and Snedaker, S. C. (1974). The ecology of mangroves. Annu. Rev. Ecol. Syst. 5, 39–64. doi: 10.1146/annurev.es.05.110174.000351
Marchand, C. (2017). Soil carbon stocks and burial rates along a mangrove forest chronosequence (French Guiana). For. Ecol. Manag. 384, 92–99. doi: 10.1016/j.foreco.2016.10.030
McKee, K. L. (2011). Biophysical controls on accretion and elevation change in Caribbean mangrove ecosystems. Estuar. Coast. Shelf Sci. 91, 475–483. doi: 10.1016/j.ecss.2010.05.001
Meng, Y., Bai, J., Gou, R., Cui, X., Feng, J., Dai, Z., et al. (2021). Relationships between above- and below-ground carbon stocks in mangrove forests facilitate better estimation of total mangrove blue carbon. Carbon Balance Manag. 16:8. doi: 10.1186/s13021-021-00172-9
Millennium Assessment (MA) (2005). Mille ecosystems and human well-being. Synthesis Island Press, Washington.
Nellemann, C., Corcoran, E., Duarte, C. M., Valdés, L., De Young, C., Fonseca, L., et al. (2009). Blue carbon - A UNEP (United Nations environment Programme and GRID-Arendal) rapid response assessment. Environment, 9.
Perera, K. A. R. S., and Amarasinghe, M. D. (2017). Partitioning of system total carbon pool of kala Oya mangrove ecosystem in Sri Lanka. Int. J. Adv. Sci. Eng. Technol. 5, 1–4.
Perera, K. A. R. S., and Amarasinghe, M. D. (2018). Ecosystem carbon stock of mangroves at the Batticaloa lagoon, Sri Lanka. OUSL J 13, 81–100. doi: 10.4038/ouslj.v13i2.7441
Reef, R., Feller, I. C., and Lovelock, C. E. (2010). Nutrition of mangroves. Tree Physiol. 30, 1148–1160. doi: 10.1093/treephys/tpq048
Reef, R., and Lovelock, C. E. (2015). Regulation of water balance in mangroves. Ann. Bot. 115, 385–395. doi: 10.1093/aob/mcu174
Sandilyan, S., and Kathiresan, K. (2012). Mangrove conservation: a global perspective. Biodivers. Conserv. 21, 3523–3542. doi: 10.1007/s10531-012-0388-x
Satyanarayana, B., Van der Stocken, T., Rans, G., Kodikara, K. A. S., Ronsmans, G., Jayatissa, L. P., et al. (2017). Islandwide coastal vulnerability assessment of Sri Lanka reveals that sand dunes, planted trees and natural vegetation may play a role as potential barriers against ocean surges. Global Ecol. Conserv. 12, 144–157. doi: 10.1016/j.gecco.2017.10.001
Schlesinger, W. H. (1977). Carbon balance in terrestrial detritus. Annu. Rev. Ecol. Syst. 8, 51–81. doi: 10.1146/annurev.es.08.110177.000411
Song, S., Ding, Y., Li, W., Meng, Y., Zhou, J., Gou, R., et al. (2023). Mangrove reforestation provides greater blue carbon benefit than afforestation for mitigating global climate change. Nat. Commun. 14:756. doi: 10.1038/s41467-023-36477-1
Tam, N. F. Y., Wong, Y. S., Lan, C. Y., and Chen, G. Z. (1995). Community structure and standing crop biomass of a mangrove forest in Futian nature reserve, Shenzhen, China. Hydrobiologia 295, 193–201. doi: 10.1007/BF00029126
Tamooh, F., Huxham, M., Karachi, M., Mencuccini, M., Kairo, J. G., and Kirui, B. (2008). Below-ground root yield and distribution in natural and replanted mangrove forests at Gazi bay, Kenya. For Ecol Manag. 256, 1290–1297.
Walcker, R., Gandois, L., Proisy, C., Corenblit, D., Mougin, É., Laplanche, C., et al. (2018). Control of “blue carbon” storage by mangrove ageing: evidence from a 66-year chronosequence in French Guiana. Glob. Chang. Biol. 24, 2325–2338. doi: 10.1111/gcb.14100
Wang, D., Wan, B., Liu, J., Su, Y., Guo, Q., Qiu, P., et al. (2020). Estimating aboveground biomass of the mangrove forests on Northeast Hainan Island in China using an upscaling method from field plots, UAV-LiDAR data and Sentinel-2 imagery. Int. J. Appl. Earth Obs. Geoinf. 85, 101986–108432. doi: 10.1016/j.jag.2019.101986
Wang, J., Sun, J., Xia, J., He, N., Li, M., and Niu, S. (2017). Soil and vegetation carbon turnover times from tropical to boreal forests. Funct. Ecol. 32:1. doi: 10.1111/1365-2435.12914
Xu, L., Shi, Y., Fang, H., Zhou, G., Xu, X., Zhou, Y., et al. (2018). Vegetation carbon stocks driven by canopy density and forest age in subtropical forest ecosystems. Sci. Total Environ., 631–632. doi: 10.1016/j.scitotenv.2018.03.080
Yan, J., Hu, X., Qian, L., Fu, X., and Wang, L. (2023). Tidal organic input restricts CO2 sequestration capacity of estuarine wetlands. Environ. Sci. Pollut. Res. 30, 63580–63591. doi: 10.1007/s11356-023-26642-w
Zhu, J.-J., and Yan, B. (2022). Blue carbon sink function and carbon neutrality potential of mangroves. Sci. Total Environ. 822, 153438–159697. doi: 10.1016/j.scitotenv.2022.153438
Keywords: mangrove restoration, carbon storage, forest age, soil depth, cofferdam restoration
Citation: Song W, Hou Y, Zhu W, Fan Y, Xu H, Cai C, Li G and Huang L (2024) Enhancement effects of mangrove restoration on blue carbon storage in Qinzhou Bay. Front. For. Glob. Change. 7:1328783. doi: 10.3389/ffgc.2024.1328783
Edited by:
Rudong Zhao, Chinese Academy of Sciences (CAS), ChinaReviewed by:
Chang Liao, Yunnan University, ChinaChenjun Du, Chinese Academy of Sciences (CAS), China
Copyright © 2024 Song, Hou, Zhu, Fan, Xu, Cai, Li and Huang. This is an open-access article distributed under the terms of the Creative Commons Attribution License (CC BY). The use, distribution or reproduction in other forums is permitted, provided the original author(s) and the copyright owner(s) are credited and that the original publication in this journal is cited, in accordance with accepted academic practice. No use, distribution or reproduction is permitted which does not comply with these terms.
*Correspondence: Lin Huang, aHVhbmdsaW4xNkBtYWlscy51Y2FzLmVkdS5jbg==