- 1Faculty of Forestry and Wood Sciences, Czech University of Life Sciences Prague, Prague, Czechia
- 2Faculty of the Environment, Jan Evangelista Purkyně University in Ústí nad Labem, Ústí nad Labem, Czechia
Compared to other sectors, the long rotation period in forest management makes it difficult to carry out a life cycle assessment (LCA). Data collection is not possible in a short time frame but would require decades. For this reason, forestry is often forced to rely on secondary data. To address this challenge, environmental impacts of the forestry sector in the Czech Republic were investigated according to the value chain corresponding to silvicultural and harvesting processes, without any further wood use options. The methodological procedure is based on the ISO 14040 and 14,044 standards, using SimaPro, an LCA software. The study was carried out in four phases of LCA in the scope of cradle-to-gate and focused on the early stages of the product life cycle. The system boundaries were set up to include seed collection, seedling production, establishment and tending of young forest stands up to 20 years of age, thinning, harvesting, maintenance of roads and extraction trails, with the endpoint is the hauling place. Three scenarios have been defined that differ in the method of harvesting. Scenario I– chainsaw felling, horse extraction. Scenario II– chainsaw (90% of hours worked) and harvester (10% of hour-worked), tractor extraction. Scenario III– chainsaw (23% of hour-worked) and harvester (77% of hour-worked), extraction by a tractor with a winch. The results show that the maintenance of the forest road network holds a significant share of the overall environmental impact of forest management. Other significant consequences are associated with timber harvesting. These impacts vary considerably depending on the mechanical equipment used. The highest impact was recorded for the third scenario, still, it is practically the most often applied, as it is the most cost-effective. The results show the need to optimize forest management practices in the future both from an economic and environmental point of view. The work on the life cycle assessment was particularly challenging because the silviculture and harvesting of raw timber involve 20 production operations. This research was based on long-term knowledge of forest typology, forest management planning, forest economics, experience with forest technologies, and regulatory measures. Thus, the findings serve for further analysis of LCA in timber production, and future analysis for other forest ecosystem services.
1 Introduction
Forests produce an array of forest ecosystem services (Patterson and Coelho, 2009; Ciccarese et al., 2012; Miura et al., 2015; Vauhkonen and Ruotsalainen, 2017), but also, like in other sectors, causes negative environmental impact (Lundström et al., 2018). Research on the environmental impacts of the forestry sector is often limited to the negative effects of harvesting on the ecosystem (Martin et al., 2015; Kļaviņš et al., 2019; Ugawa et al., 2020). In terms of the magnitude of the environmental impact of the forestry sector, assessment often only focuses on certain aspects, such as biodiversity (Michelsen et al., 2012; Seedre et al., 2018), water (Allen and Chapman, 2001; Brown et al., 2007; Sundnes et al., 2020), soil (Piirainen et al., 2015), and air emissions (Virbickas and Galickaja, 2017).
Life Cycle Assessment (LCA) is among the methodologies utilized in environmental accounting, alongside Environmental Input–Output Analysis, Ecological Footprinting, Carbon Footprinting, and Ecological Cost (Patterson et al., 2017). These methodologies rely on input–output analysis and assess adverse environmental effects within the physical realm, among other aspects (Hayati et al., 2013; Latan et al., 2018; Burritt et al., 2019). A holistic view can be expected when applying the Life Cycle Assessment (LCA) method. However, its use on the forestry sector is connected to a number of methodological problems along with the risk of misapplication (Heinimann, 2012). Because, unlike the industry sector, forestry operates in a natural environment that is characterized by a complex set of internal and external ecosystem links. The function of this ecological system and its resilience guarantee the fulfillment of an array of ecosystem services that are important to human beings (Stohr, 2013). Ecosystem services provided by forests do not only include timber production, but also water management, soil conservation, climate, biodiversity conservation, hunting, forest fruit picking, and last but not least, recreational and spiritual services. From this point of view, the very definition of the scope of the study in the context of the LCA method is already complex, not to mention that the different forest functions may significantly interact (Sacchelli and Bernetti, 2019). For instance, monoculture timber production forests can have a negative impact on biodiversity and on the fulfillment of many other services, such as water, esthetic, recreation value and erosion control (Felton et al., 2016; Wan et al., 2017). Similarly, the timber-production ecosystem service of forests can be negatively affected by, for example, excessive of game animals for hunting (Ward and Williams, 2020), overabundant forest fruit picking, and intensive forest tourism (Price, 1987; Font and Tribe, 2000) can destruct the quantity and quality of woody tree regeneration.
Among the most common methodological problems in LCA is wrong understanding and inclusion processes of the silvicultural and harvesting into the product system. From an LCA perspective it is not about the “production” stage, but the raw material excavation stage, in this case, excavation of biotic raw material. Incorrect understanding and inclusion of the silviculture and logging processes within the life cycle stages then naturally entails an incorrectly defined functional unit. We often encounter a functional unit defined at the raw material excavation stage as 1 ha, 1 m3, or 1 t of timber (Bosner et al., 2012; Ferro et al., 2018). The problem is that it is not a functional unit, but only a declared unit, because at this stage a functional unit cannot be defined. Some studies include seed collection and subsequent operations up to the harvesting of timber, while others start with the felling of mature trees (Klein et al., 2015). Additionally, due to the length of rotation (100 years in this study), there is also a problem with the primary data collection (Sahoo et al., 2019). They often tend to be replaced by so-called secondary data, i.e., data from literature. This practice leads to a simplistic and often erroneous application of the LCA method. The shortcomings often reflect the fact that the LCA method has been primarily concerned with the assessment of industrial systems since its inception. The methodology should therefore be complemented by procedures related to living systems, and in particular by appropriate impact categories, or at least characterization factors, within the existing ones, to reflect the issues of human activity associated with living ecosystems.
These reasons led to the development of an improved approach from LCA. evaluating the environmental impacts of forestry activities in the Czech Republic. This methodology aims to provide a standardized framework for such assessments by prioritizing the collection of primary data over reliance on secondary sources, which would be a model methodological procedure for assessing the environmental impacts of the forestry sector throughout the Czech Republic. The first part of the methodology described in this article concerns forest production services. The methodology defines the scope of the study to avoid inaccuracies and methodological errors on the part of the LCA study developers regarding the determination of the appropriate reference unit, the scope of the system, the method of collection, and the quality of the data. It follows from our practical experience and literature research.
This paper aims to assess the environmental impacts of the forestry sector in the entire value chain corresponding to silviculture and harvesting, using the LCA for assessing the environmental impact of the forestry sector. Unlike the approach to LCA in the forestry sector in other publications (Heinimann, 2012; Klein et al., 2015; Đuka et al., 2017), our methodological approach focuses mainly on the qualitative aspect of primary data collection. The data are as detailed as possible, based on long-term research in forest typology, forest management planning, economics, standardization, and forestry sector technology. The validation of the proposed methodology is based on concrete data obtained at a company’s accounting statements. The paper also presents the findings of an environmental impact assessment conducted on three forest management scenarios, conducted at the scale corresponding to silviculture and harvesting. These scenarios were developed using the methodology outlined in the study. The methodology and findings in this study also serve as the basis of future research focusing on assessing the environmental impacts of other forest services.
2 Methods
2.1 Study location
Czech Republic is located in the Central Europe, of which about 34% of its land areas are covered by forests. About 75% of the Czech forests are intended for production, which made the country as one of the leading global exporters of roundwood. Among Central European nations, the Czech Republic holds the top position in exporting roundwood, followed by Slovakia (Ministry of Agriculture of the Czech Republic, 2020; FAO, 2021). Over a century ago, extensive monoculture coniferous plantations replaced the original mix of broadleaved and mixed trees in the Czech lands, all aimed at boosting wood production (Ardö, 1998). As a result, among the diverse forest vegetation present, the Norway spruce (Picea abies L. Karst) currently stands as the prevailing tree species, accounting for 50.7% of the entire forest composition, followed by the Scots pine (13.3%), larch (4.2%), and fir (1.6%), along with other coniferous varieties contributing 0.8%. Beech emerges as the primary broadleaved species, representing 9.5% of the forest makeup. Additional prominent broadleaved tree species encompass oak, alder, and maple, constituting 6.8, 2.3, and 2.1%, respectively. The rest of deciduous trees (8.7%) include, e.g., birch and hornbill. The state is the majority owner (53.7%) of the Czech forests The subsequent major proprietors consist of individuals (approximately 19%) and municipal and urban authorities (around 17%) (Ministry of Agriculture of the Czech Republic, 2020).
Primary data collected for the LCA were gathered from company’s accounting statement at the School Forest Enterprise Kostelec nad Černými lesy, located east of Prague, the capital of the Czech Republic, which is owned by the Czech University of Life Sciences Prague. Thereafter in this article the study area is called “CZU forests.” The current size of the managed forest property, which serves as a model for life cycle assessment, is approximately 7,000 ha.
2.2 Procedure of data collection
The methodological procedure is based on the defined objective and the ISO 14040 and 14,044 standards (ISO 14040:2006 Environmental management – Life cycle assessment – Principles and framework and ISO), using SimaPro, a specialized LCA computer software (PRé Consultants B.V., Amersfoort, The Netherlands.). The Centrum voor Milieuwetenschappen Leiden-Industrial Assessment (CML-IA) baseline method was used to calculate the results of the impact category indicators, because it represents the most common impact assessment model (Center of Environmental Sciences-Leiden University, 2016). The LCA study on the environmental impacts of silviculture and harvesting was carried out in four stages of data collection framework modified from Hellweg and Milà i Canals (Hellweg and Milà i Canals L., 2014; Figure 1). Primary data from the company’s financial statements for year 2016 at CZU forests up to the level of raw material excavation were supplemented with secondary information mainly from Ecoinvent 3, AGRIBALYSE 3.0, and ELCD databases. In the third stage of LCIA (Life Cycle Impact assessment), the result of the inventory analysis was converted into the following impact categories: abiotic depletion (AD), abiotic depletion - fossil fuels (FF), global warming (GWP 100a), ozone depletion (ODP), human toxicity (HT), terrestrial ecotoxicity (TE), photooxidant formation (PF), acidification (AP), eutrophication (EP), cumulative energy demand (CED). The calculation of the results of the indicator categories was based on CML-IA provided by Simapro. Lastly, the results were interpreted in an iterative procedure, incorporating the results of all previous phases.
2.2.1 Defining the boundaries of the system
The study was conducted only for the upper stream of the product life cycle, i.e., harvesting of the raw material (Figure 1). The other stages of timber manufacturing, −use and end-of-life were excluded. The functions of the future product system, in which the raw timber material will be used, therefore, may vary considerably, such as paper manufacturing, wood biomass, or wood construction, of which findings of the LCA study have been reported (Schmidt et al., 2007; M’hamdi et al., 2017; Gergel et al., 2020; Sgarbossa et al., 2020; Sadaghiani, 2023). For this reason, the functional unit has not been defined, as it is a quantification of the function of the system and is therefore linked exclusively to the “use” stage in the product life cycle structure. All calculations of the inventory analysis and impact category indicators were, therefore, based on the declared unit (DU) of 1 ha of forest stand. The boundaries of the silviculture and harvesting system were set up to include seed collection, seedling production, establishment and tending of young forest stands up to 20 years of age, thinning, harvesting, and maintenance of roads and extraction trails (Figure 1). The endpoint of the system is the hauling place. Furthermore, three scenarios of the harvesting methods that are practiced in the country were selected for the LCA study (Figure 2).
2.2.2 Methodological approach to primary data collection
Primary data collected within the assessed boundary of the product system include energy and raw material consumption, but exclude damage to the forest ecosystem from activities related to silviculture and harvesting of raw timber, such as alteration or damage to biodiversity, water management, erosion potential, etc. Methodologically, these procedures are highly challenging and are not yet adequately addressed in the LCA methodology (Klein et al., 2015).
2.2.3 Selection of the spatial unit
Due to the extremely diverse site conditions in which forest stands are cultivated in the Czech Republic and the difficulty of obtaining primary data, it was necessary to a priori narrow the selection of the spatial unit on which the analysis was performed. The unit defined by the forest typology as the “Group of Forest Habitat Types” (GFHT) was set as optimal. Forest habitat type is a set of forest biocenoses, both native and modified, and their developmental stages, including their habitats, i.e., geobiocenoses belonging together. In forestry sector practice in the Czech Republic, a forest habitat type is characterized by a distinctive species combination of the relevant phytocenosis, soil characteristics, occurrence in the landscape, and potential yield class.
GFHT links forest habitat types according to their ecological affinities, expressed in terms of economically important habitat characteristics. In the ecological network of the approved and utilized typological system, GFHTs are defined by altitudinal vegetation zones (vertically) and edaphic (soil) categories (horizontally). A GFHT is a complex of phytocenologically similar, native forest types, including all their altered and developmental stages in a particular altitudinal vegetation zone, ecological series, and edaphic (soil) category. Within each group of forest habitat types are defined as follows:
1. Production and economic parameters of forest stand management (species composition, rotation period, proportion of soil-improving tree species, optimal management measures, optimal costs, and yields).
2. Ecological effects of stands. Inclusion in a particular GFHT is limited by ecological functions:
– infiltration – plains and slopes up to 40% not threatened by erosion (mainly zonal soil cat.),
– erosion control – slopes above 40%, or less when threatened by erosion (extreme, exposed cat.),
– desiccating – permanently and temporarily waterlogged soils (gleyed, waterlogged cat.), precipitation-forming (climatic) – additional function, water-protection – strips of coastal stands.
GFHTs were analyzed for the company based on the tree species and their area. A total of 45 tree species and 54 forest types were evaluated, all of which were located in this area. Based on this analysis, the most numerous forest type for the spruce tree species was selected for the purposes of the study as representative.
The most commonly represented GFHTs are 3H (oak-beech forests on deep loamy soils and loess), 3 K (oak-beech forest on acidic soils), 3S (oak-beech forest on medium-nutrient soils), 3I (oak-beech forest on compacted acid luvisols), and 3 V (oak-beech forest on moist to wet soils). Our case study was performed for the 3H group of forest habitat types, as a cardinal representative of forest stand silviculture within the CZU forests. The altitude ranges from 210 to 528 meters above sea level. The average annual temperature is 7.0–7.5°C, with an average annual precipitation of 600–650 mm (of which 65% falls in the vegetation period), and an average vegetation period of 153 days. Climatically, the area is classified as warm to moderately warm. Therefore, if the proposed LCA assessment method is applied to another territory, only the GFHT can be changed.
2.2.4 Timing and species composition
In terms of time assessment, the LCA was delimited by 1 year. Based on long-term balanced economic and harvest parameters, the year 2016 was chosen. Regarding tree species composition in the forest management plan area, the most common species is Norway spruce (48.92%). It is also the most common species in the territory of the Czech Republic. Thus, it was selected for this study.
2.2.5 Identification of operations included in the analysis
Within the defined boundaries of the system, i.e., silviculture and harvesting, various operations are performed. A brief definition of these operations included in the analysis is given below.
The “sowing” operation is based on standard and recommended values of sowing per technical unit, it is calculated to the degree of seed purity. For application in forest stands, hand sowing is primarily used, either in large areas, or in patches. If preliminary mechanical soil disturbance or chemical preparation is necessary before the actual sowing and planting, these conditions are dealt with as mechanical or chemical in the “soil preparation” operations.
The mechanical “soil preparation” operation for natural and artificial regeneration is based on mechanical soil disturbance, usually by a universal wheel tractor (UWT). Furthermore, the operation of “artificial regeneration” is determined by the technology of implementation, type and number of seedlings, and the share of auxiliary operations.
The operation of “Plantation protection against wild game – chemical” is based on the number and nature of the planting material, the frequency of repeated interventions, and the age of the established plantation. The operation of “protection of plantations against wild game by fencing” is determined mainly by the proportion of tree species such as beech, fir, and others. The consumption of timber, netting, and transport of material and workers is included. The “protection of young plantations against forest weed” operation is based on the proportion of the area treated and the frequency of the intervention up to the stage of an established plantation. The “protection of seedlings against pine weevil” production operation is based on the proportion of seedlings attractive for infestation. In particular, the consumption of the protective substance, including water per application, is included.
The operation called “juvenile thinning” is divided according to the tree height into two categories, namely up to 4-m, and above 4-m height. The silvicultural tariff is multiplied by the number of trunks harvested.
The data for harvesting operations depends on the combination of the different technologies used. A similar approach has been taken to the performance of “timber extraction” (from horses to harvester nodes or cableways). The cut-to-length operation is calculated per m3 in the case of motor-manual production. Otherwise, it is usually part of the technological production of timber harvesting, e.g., harvester technology, etc. The “after-harvest treatment and clearing of brushwood” operation (manual or mechanical) depends on the quantity of timber harvested.
The “timber transport” operation is linked to the technical unit of m3.km and m3 to match the transport distance, density, and type of road network. The unit m3.km was also used in the CZU forest company’s accounting statements (1 m3 of wood corresponds to approximately 0.55 tons). Only forest road maintenance is considered here, not the construction of a new road network.
If we look in greater detail at the forest road network, we find that it includes four categories labeled L1 to L4 (according to the technical standard for forest roads), with forest paths and trails recorded separately. The forest road network consists of L1 and L2 backbone paved roads. The L3 and L4 roads are mainly unpaved transport routes. The share of the first two categories is 13.4% for L1, and 86.6% for L2. L1 and L2 categories are used for wood transport, therefore these categories were included in our analysis. Meanwhile, L3 and L4 categories are maintenance-free.
2.2.6 Primary data from accounting statements
Primary data sourced from the CZU forests’ accounting statements for 2016 was assessed and calculated according to the following production operations, seedling production that included seed collection, tending young stands, harvesting, and road maintenance. Tending young stands comprised of various activities, such as, soil preparation, natural regeneration, artificial regeneration and its repetition, protection of plantations, other silvicultural operations (e.g., disposal of harvest residue, cutting of non-target trees, making forest stand accessible), forest protection, and cleaning (juvenile thinning). Furthermore, tree felling and extraction were the main activities in harvesting. In this paper, we included the road maintenance operation, since this infrastructure is profoundly utilized by the forest owners to attend the silviculture and timber harvesting activities.
Detailed data were obtained mainly from the following financial statements and norms, which included costs and revenues of centers according to performance, costs and revenues of the center according to purposes/resources, logbook of auxiliary operations, costs and revenues per plant, silviculture equipment, harvesting equipment, yield tables by tree species, and catalog of permitted chemicals for the given period.
The primary inputs related to the production operations within the production phases are gathered and analyzed, including forest tree species seed collection (Table 1), seedling production (Table 2), establishment and tending of young forest stands up to 20 years of age (Table 3), three scenarios of timber harvesting (Table 4), extraction (Table 5) and road and extraction trail maintenance (Table 6), which correspond to the data from the aforementioned documents. The data in the tables are calculated based on 1 ha of spruce forest over a period of 100 years.
The data collected formed the basis for the calculation of the inventory analysis, which then became the grounds for the calculation of the potential environmental impacts of the assessment part of the life cycle, specifically raw timber production. In this phase of the LCA, the results of the inventory analysis were assigned to specific impact categories through category indicators. Then the results of the impact category indicators were calculated, using characterizing factors via CML IA baseline methodology.
Mechanical equipment used in silviculture and harvesting is included in the calculations to the extent of consumption of the machinery itself, its maintenance, repair, fuel consumption/combustion, and lubricant consumption. Each of the input from Tables 1–6 is supplemented by SimaPro for its consumption level by all previous unit processes from extraction of raw materials (energy, non-energy), through their treatment, and production of materials/fuel, to production of the specific input.
3 Results
3.1 Scenario-based impact assessment
In most cases, the greatest influence to environment of each category is associated with Scenario III, and the lowest with Scenario I. The relatively high difference between the scenarios is shown primarily by the impact categories of Global warming, Acidification, Eutrophication, Ozone layer depletion, Abiotic depletion – fossil fuels, and others (Figure 3). These scenarios mainly reflect the consumption of fossil resources, particularly related to gasoline and diesel use for transportation, and the operation of machinery and equipment during the silviculture and harvesting of raw timber.
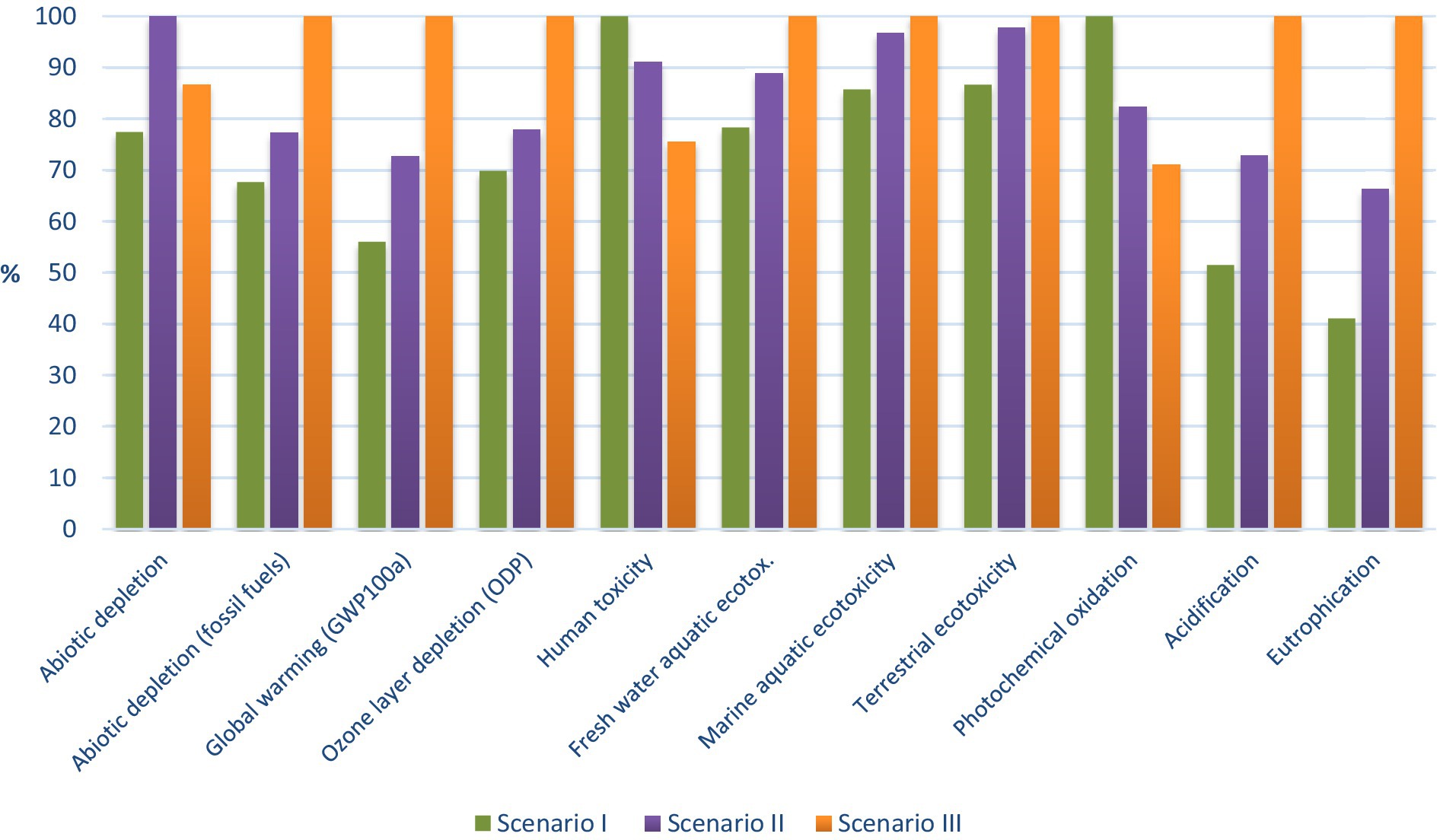
Figure 3. Results of selected impact categories of Scenarios I, II, and III in the range of unit processes analyzed in this paper.
The opposite results were found for two categories – Human toxicity and Photochemical oxidation. Scenario I showed the highest impact, while Scenario III the lowest. The main impacts here are the gasoline and diesel combustion, the wear and tear on equipment and the use of bitumen for road repairs. The highest impact of Scenario II relates to a single impact category – Abiotic Depletion. This effect is mainly related to the manufacture and maintenance of machinery and equipment from timber extraction.
The Cumulative energy demand (CED, in Mega Joule per Declared Unit (1 ha) or MJ/DU), which is 30% lower in Scenario I than in Scenario III, also shows a similar result pattern to most of the impact categories developed by the CML-IA method. If we separate the processes related to the silviculture and harvesting of timber (processes A to C) from those related to the maintenance of forest roads (process D), we find that the main cause of impact in this category is the maintenance of the road network (Figure 4), which is exacting both in terms of energy demand and the energy contained in the bitumen used to resurface the roads. For example, the maintenance of the road network of Scenario I accounts for up to 76.16% of the cumulative energy demand. For Scenario II, it is 67%, and for Scenario III, it is 53.32%, which is still a significant part of the effect of the silvicultural phase.
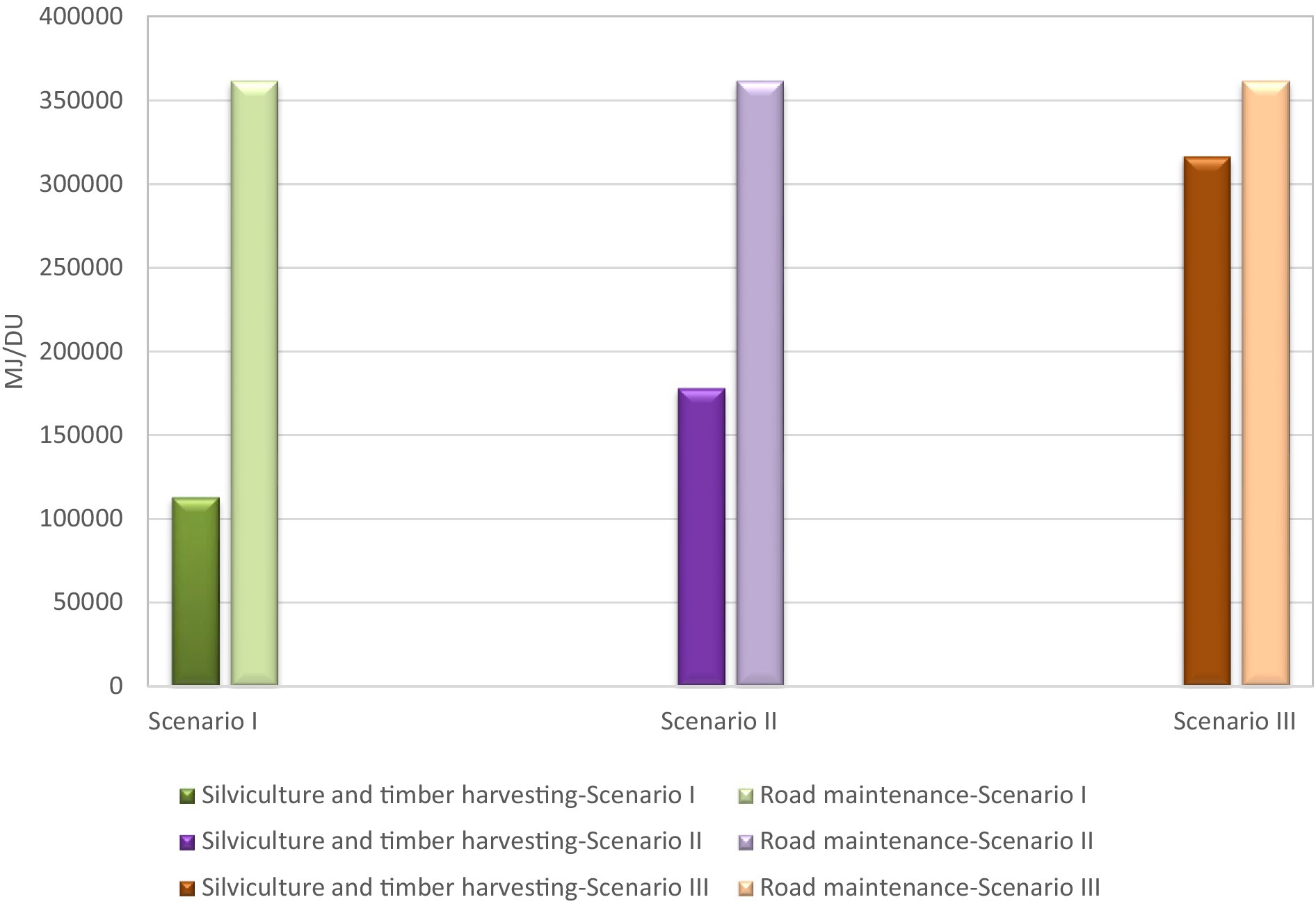
Figure 4. Cumulative energy demand of silviculture and timber harvesting and road maintenance processes, Scenarios I, II, and III (in MJ/DU).
The cumulative energy demand in processes A to C, i. e. silviculture and harvesting without road maintenance is shown in Figure 5. The differences between Scenario I and III without road maintenance are 64.26%. This is again due to the different harvesting and extraction technologies, while the data for the other process groups (seedling production and tending of young stands) are the same in all three scenarios.
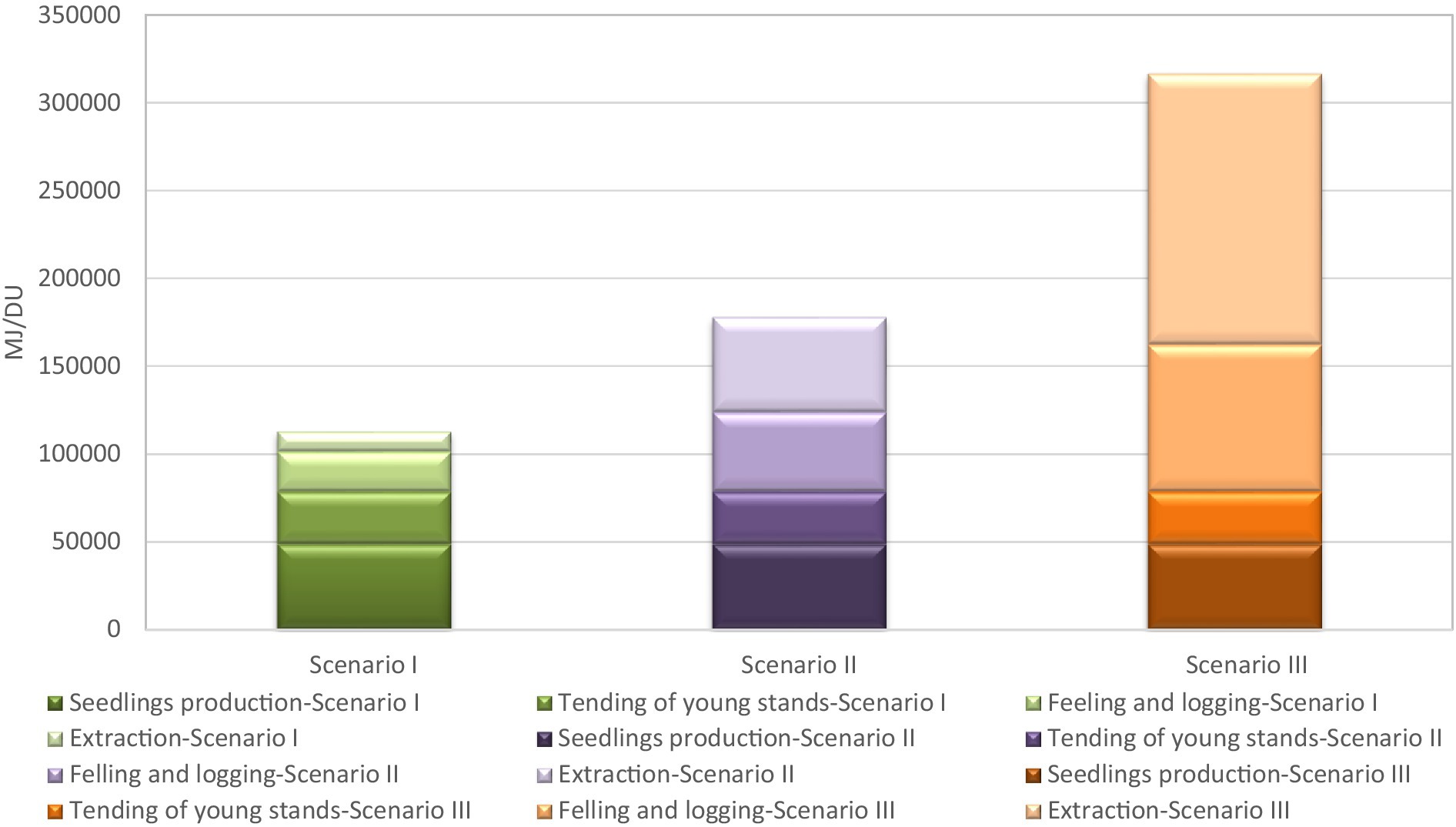
Figure 5. Cumulative energy demand in processes: seedling production, tending of young stands, felling and logging, and extraction of Scenarios I, II, and III (in MJ/DU).
However, a different pattern is shown for the results of the Global Warming impact category (Figure 6), where silviculture and harvesting of Scenario II, and especially III, exceed the Global warming impact from road maintenance. The reasons for these differences are the same as in the CED case. Once again, they can be found in the technology used for harvesting and extraction of trees.
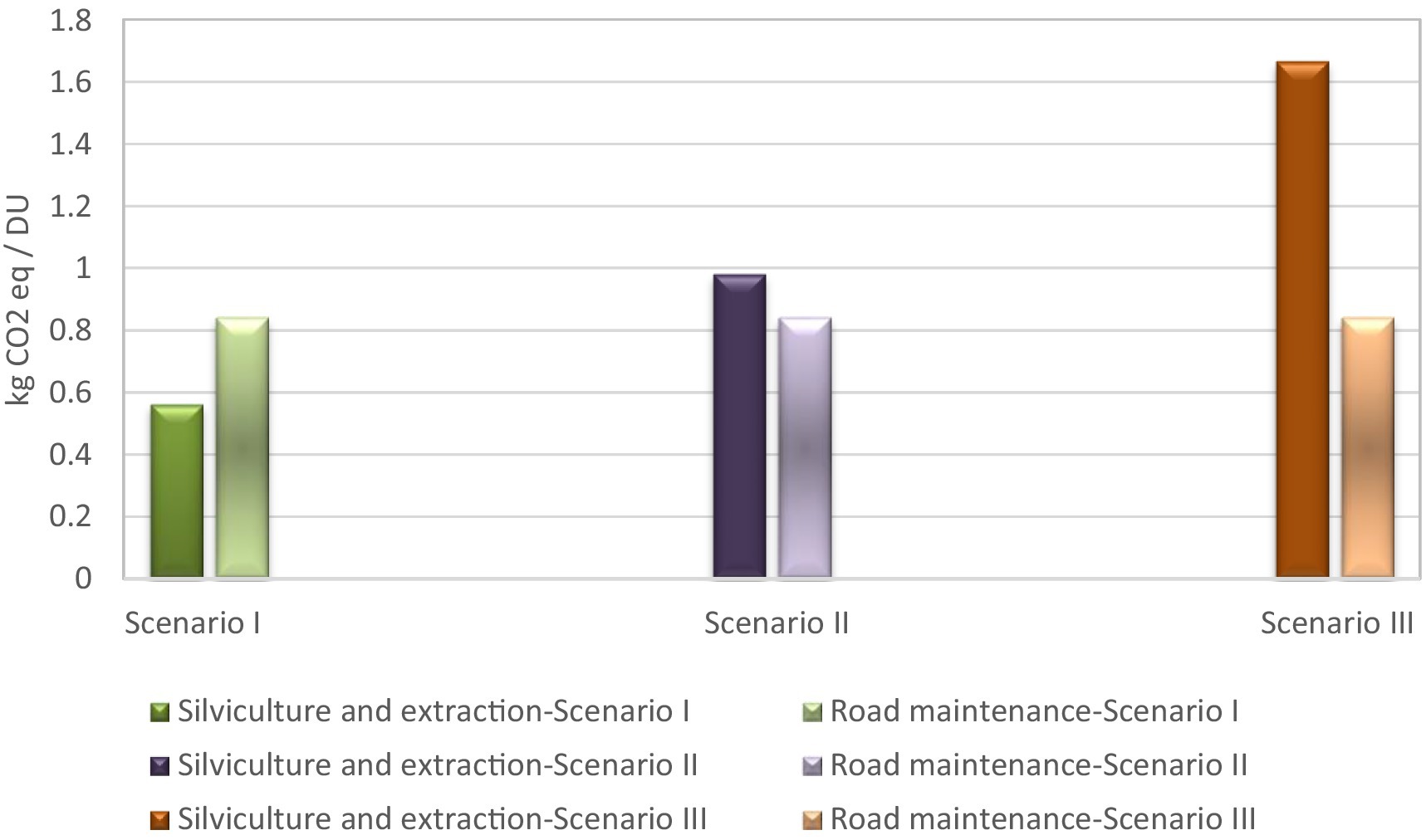
Figure 6. Global warming impact – silviculture and extraction, and road maintenance, Scenarios I, II, and III (in kg CO2 equivalent/DU).
In order to avoid result bias due to the impact of road network maintenance, we focused only on the results of silviculture and harvesting raw timber in the next step. We have found that the tending of young stands has the lowest impact in the indicators of the global warming impact categories. Relatively high impacts are related to two groups of activities, tree felling, and timber extraction. The environmental effects of the three scenarios differ significantly depending on the mechanisms used. The highest contribution to global warming is associated with tree felling using a harvester, while the lowest impact in this respect is associated with chainsaw logging. Extraction, like harvesting, is carried out in three variants. Option 1 uses horses to skid the timber, Option 2 uses a tractor, and Option 3 uses a crane-equipped tractor and a truck. Previous findings have shown that GWP is mainly associated with the combustion of gasoline and diesel. Thus, it is clear, that the lowest GWP impacts are associated with Option 1, i.e., horse-extraction. The highest GHG emissions are associated with the production and operation of the tractor and truck used in the extraction process of Scenario III. The results of the global warming impact category are shown in greater detail in Figure 7.
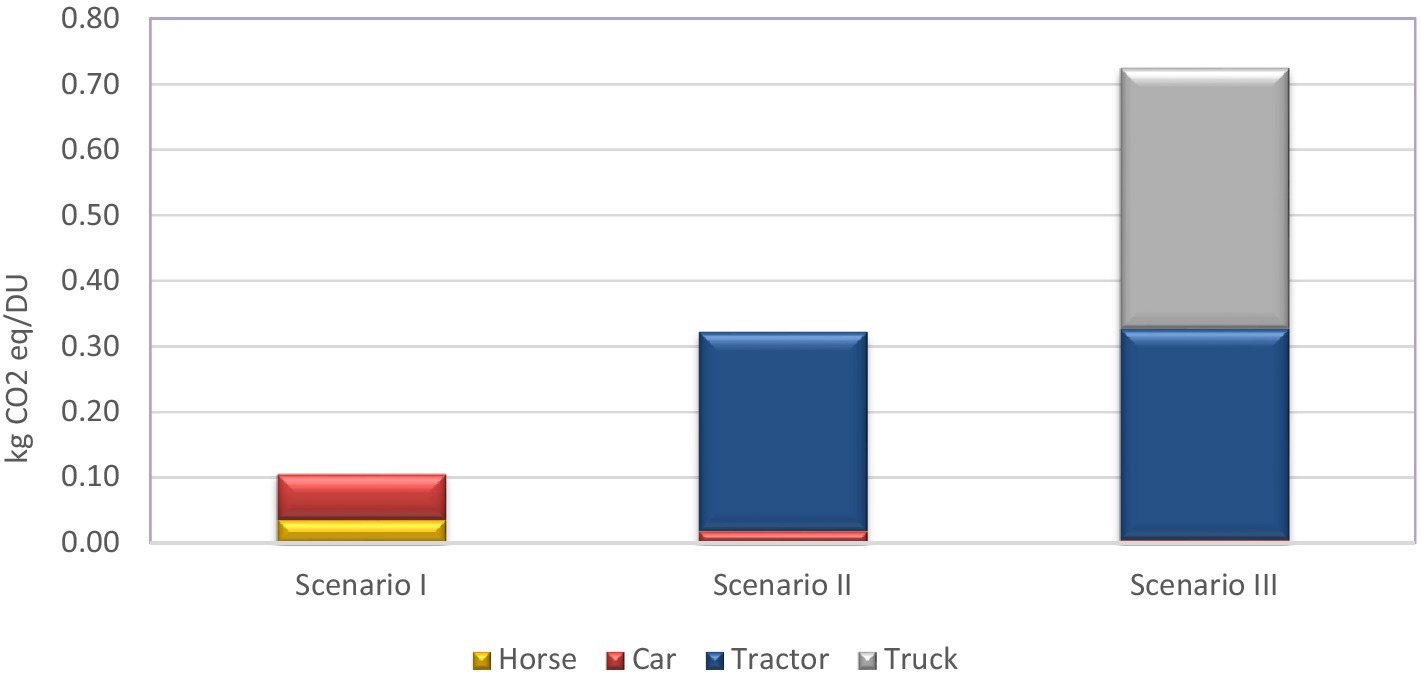
Figure 7. Global warming impact from extraction activity, comparison of Scenarios I, II, and III (in kg CO2 equivalent/DU).
All assessed impact categories of the Tending of young stands process group are shown in Figure 8. They show that in the vast majority, of the impact categories, plantation protection has a negative effect, accounting for more than 70% of the impact category indicator scores. The indicator results for these categories are mainly driven up by chemicals used in the protection of young stands against pests and browsing by wildlife, fence netting, and gasoline combustion emissions. Only in the case of Human Toxicity and Photochemical Oxidation is thinning more prominent, where gasoline combustion is the main cause of impact.
3.2 Road maintenance
Due to the relatively high impact from road network maintenance, these processes have been considered separately. A more detailed assessment of these processes presented in Figure 9 shows that the largest impactor in the CED category is the consumption of bitumen for road resurfacing. Its influence significantly exceeds that of all other inputs. The use of bitumen for road resurfacing is also the largest contributor to the global warming impact category. The consumption of gravel is also significant, where the environmental impact is mainly related to its extraction and crushing, i.e., the consumption of fossil fuels in these activities (Figure 9).
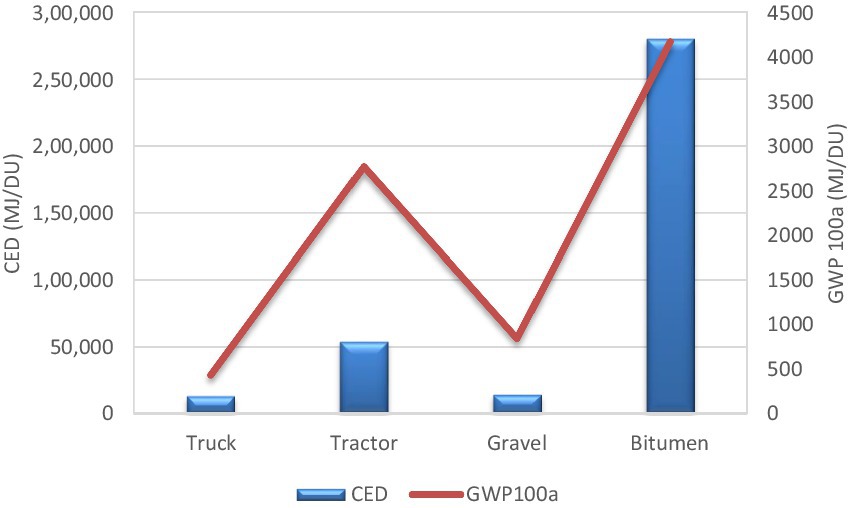
Figure 9. Cumulative energy demand (CED) on road maintenance and Global warming potential (100a) (in MJ/DU).
4 Discussion
The results are based on a detailed LCA approach applied to the forest management context using Simapro. All LCA tools (SimaPro, GABI, Umberto, OpenLCA) were developed for general purposes across multiple sectors, including agriculture. SimaPro and GABI have been used to investigate LCA in biomass, wood production, and industries (Goglio et al., 2018; Lopes Silva et al., 2019). However, none of these tools were specifically designed to assess and readily consider the environmental effects of selected forest management practices in the earlier stages of forestry, such as seedling and timber cutting. Hence, the methodology and findings presented in this paper can serve as a foundational framework for future research of LCA in forestry aiming at evaluating the environmental impacts of various forest ecosystem services such as non-wood production, recreation, and hunting.
An average/typical year was chosen for the assessment. Specifically, we chose the year 2016, which was a balanced year in terms of supply and demand, including a balanced level of timber harvest. Compared to other LCA studies in forest management, there are more options for setting a time range, e.g., a “whole rotation approach” and a “single moment approach” (Price, 1987; Klein et al., 2015), however, it is practically unfeasible to include the entire production or rotation period, given the length of time (e.g., 100 years).
Another important methodological starting point was the establishment of system boundaries for the LCA. First of all, we consider it crucial to point out that this is not the production phase of the life cycle, as reported in some LCA studies (Ferro et al., 2018), but the first phase, i.e., the biotic harvesting phase. This study’s boundary system was set from the seed collection to timber extraction so that the forest management operations were assessed across the entirety of the first life cycle phase. It is clear from the published LCA results that the choice of system boundaries has a significant effect on the LCA results (Peñaloza et al., 2019).
In the context of forestry in the Czech Republic, seedling activities exhibited similarities. In contrast, three scenarios of the harvesting methods that are practiced in the country were selected for the LCA study (Figure 2). The first scenario depicted complete tree felling and processing carried out with a chainsaw, alongside timber extraction utilizing a horse. This practice took place in forested areas surrounded by waterlogged terrain or muddy soil, making it difficult for a tractor or other vehicle to access. In the second scenario, the process of tree felling and processing was accomplished through a combination of chainsaw work (constituting 90% of the total hours worked) and harvester operation (accounting for the remaining 10% of hours). The extraction phase involved the use of a tractor. In the third scenario, a different approach was taken, with chainsaw usage reduced to 23% of the hours worked, while the majority of time (77% of hours worked) was dedicated to utilizing the harvester. For extraction, a tractor equipped with a winch was employed. In the third scenario, a small truck played a crucial role by providing essential spare parts, materials, and fuel to support the efficient operation of the forestry machinery.
The results of the LCA on silvicultural and harvesting operations show a significant contribution from the maintenance of the forest road network to the environmental impacts of this phase. Depending on the different harvesting scenarios, the share of the impacts from the maintenance of road network ranges from 53.3 to 76.2%. Similarly, Đuka et al. (2017) reported a share of 60% from this activity. In other articles, this ratio is quite variable. For example, Heinimann and Maeda-Inaba (2003) stated that in Switzerland, three factors are decisive for environmental impact: slope, transport distance for base course materials, and roadbed width. However, based on the described models, the impact can be very different. Dodson (2021) points to the effects of climate change on increasing forest road maintenance costs, as water quality could deteriorate. In addition, it draws attention to the higher maintenance costs due to the frequent bridges on forest roads and thus the higher environmental impact. On the other hand, forest road maintenance may be less energy intensive, as Whittaker et al. (2011), due to the smaller quantities of aggregate used per km, and fewer machinery operations.
Forest roads are an essential technical infrastructure within forest ecosystems (Drosos et al., 2013), serving a whole array of harvesting and silvicultural operations. In addition to these activities, forest roads serve other purposes such as hunting, hiking, fishing, forest fruit picking, and others that were not included in the LCA calculation. Their share of the environmental impact on the road network will be the subject of further research.
The high environmental burden is primarily due to the high proportion of gravel and bitumen for the maintenance of the L1 category roads. An option to eliminate this aspect is to move the roads from the L1 category and subsequent categories to the lower ones, i.e., to create a high-quality backbone network, followed by the lower categories where appropriate. It is also possible to use different technology, e.g., compacted aggregate can be used instead of bitumen, as gravel performs significantly better in terms of global warming and overall energy consumption. Another option is to use a variant with the lowest environmental impact when designing forest roads (Hayati et al., 2013; Picchio et al., 2018). It is also a question of making the forest accessible through an optimal forest road network, both from a technological and environmental perspective (Drosos et al., 2013), as well as, for example, fire protection (Majlingová, 2012).
Other significant impactors are associated with felling, and in particular, with timber extraction. These impacts vary considerably according to the machinery used (Đuka et al., 2017). These activities are mirrored in all impact categories, especially in cumulative energy demand and global warming. From this analysis, it can be seen, that the aforementioned level of impact categories is primarily influenced by two production operations, i.e., felling and extraction. Also, seedling production in forest nurseries, including seed collection and the tending of young stands, is not negligible. Nurseries have a higher effect than the tending of young stands in both categories. Global warming is 20% higher for nursery seedling production, and the cumulative energy demand is 39% higher. In silviculture, plantation protection is strongly reflected in almost all impact categories within this production operation. The electricity used for irrigation and fossil fuels used for growing seedling and transportation were the major sources of emission. In contrast, Aldentun (2002) stated that the major sources of emissions were the fossil fuels used for heating the greenhouses and for seedling transportation. The difference is due to the different cultivation technology.
The choice of harvesting technology is a challenging problem where several inputs are taken into account. In particular, site conditions (terrain configuration, slope, forest weeds), harvested assortments (their stem volume and length), extraction distance, and the volume of transported timber. A comparison of the three scenarios shows that the leading environmental impacts are associated with the use of the harvester. These results fly in the face of the economic intensity, where, contrarily, the best results are achieved when a harvester is employed. Additionally, although we did not analyze the biodiversity implication of the three-harvesting technology, horse-powered prevented soil compaction (García-Tomillo et al., 2017), compared to heavy machinery. Soil compaction can degrade environmental quality (bulk density and porosity) of various soil microorganisms and biota, thereby limiting their growth (Kretzschmar, 1991; Tan et al., 2008; Frey et al., 2009; Ugawa et al., 2020). The economic effect and the working safety are currently the criteria for evaluating economic activity, and this fact explains the rapidly increasing share of harvesting technologies used in forestry sector (Dvořák et al., 2019). However, the emphasis on the economic effect of production operations is in contrast to the impact on energy consumption and the global warming criterion. This was also confirmed by Kühmaier et al. (2022). The solution is to seek the optimum eco-economic efficiency in forest management. The innovation of newly designed mechanisms can be a promising solution.
5 Conclusion
Our research aimed to propose a methodological procedure for the LCA in the forestry sector. The work on the LCA was particularly challenging because the silviculture and harvesting of raw timber involve 20 production operations. The analysis was carried out in as much detail as possible while complying with all legislative, technical, technological, and material standards. The model was developed for a spatial unit defined by the forest typology in the Czech Republic (a group of forest habitat types) and for the most common tree species, i.e., spruce. Processed methodology can be applied to other tree species and forest habitat type groups, possibly to higher territorial units, forest estates, and also to the entire forestry sector at the national level.
The LCA was based on long-term knowledge of forest typology, forest management planning, forest economics, experience with forest technologies, and regulatory measures. In terms of the use of detailed data on forest management and knowledge of the forest ecosystem, this is an original approach based on many years of analysis and knowledge, which are used in practice by the government and forest owners. Three scenarios were selected, differing in the method of harvesting. The highest impact was recorded for the third scenario, i.e., chainsaw felling (23% of hours worked) and harvester operation (77% of hours worked) and extraction by a tractor (Supplementary Table S8).
The strength of our study was that we used primary data, unlike many other studies that use secondary data. The primary data are based on detailed conditions given by a specific forest ecosystem characterized by Group of Forest Habitat Types. Furthermore, these are the basic approaches used in life cycle assessment, which are often simplified in the case of the forestry sector at the expense of meaningful results. The results can be used in other regions or throughout the Czech Republic.
The boundaries of the silviculture and harvesting system can be extended to other stages of timber utilization in additional research. Further analysis of other forest ecosystem services, i.e., not only timber production but also non-timber and non-market ecosystem services, can be pursued.
Data availability statement
The original contributions presented in the study are included in the article/supplementary material, further inquiries can be directed to the corresponding authors.
Author contributions
MH: Conceptualization, Investigation, Resources, Supervision, Writing – original draft, Writing – review & editing. KP: Conceptualization, Investigation, Methodology, Resources, Visualization, Writing – original draft, Writing – review & editing. RP: Resources, Visualization, Writing – original draft, Writing – review & editing. MT: Conceptualization, Formal analysis, Investigation, Methodology, Resources, Visualization, Writing – original draft, Writing – review & editing. MP: Data curation, Resources, Writing – review & editing.
Funding
The author(s) declare that financial support was received for the research, authorship, and/or publication of this article. This work was supported by the Operational Program Research, Development and Education, the Ministry of Education, Youth and Sports of the Czech Republic [EVA4.0, No. CZ.02.1.01/0.0/0.0/16_019/0000803] and by the non-project research of the Department of Forestry and Wood Economics, Faculty of Forestry and Wood Sciences, Czech University of Life Sciences Prague.
Conflict of interest
The authors declare that the research was conducted in the absence of any commercial or financial relationships that could be construed as a potential conflict of interest.
Publisher’s note
All claims expressed in this article are solely those of the authors and do not necessarily represent those of their affiliated organizations, or those of the publisher, the editors and the reviewers. Any product that may be evaluated in this article, or claim that may be made by its manufacturer, is not guaranteed or endorsed by the publisher.
References
Aldentun, Y. (2002). Life cycle inventory of forest seedling production–from seed to regeneration site. J. Clean. Prod. 10, 47–55. doi: 10.1016/S0959-6526(01)00012-9
Allen, A., and Chapman, D. (2001). Impacts of afforestation on groundwater resources and quality. Hydrogeol. J. 9, 390–400. doi: 10.1007/s100400100148
Ardö, J. (1998). Remote sensing of forest decline in the Czech Republic (master thesis). Sweden: Lund University, Dept. of Physical Geography.
Bosner, A., Poršinsky, T., and Stankić, I. (2012). Forestry and life cycle assessment. London: InTech Rijeka.
Brown, A. E., Podger, G. M., Davidson, A. J., Dowling, T. I., and Zhang, L. (2007). Predicting the impact of plantation forestry on water users at local and regional scales: an example for the Murrumbidgee River basin, Australia. For. Ecol. Manag. 251, 82–93. doi: 10.1016/j.foreco.2007.06.011
Burritt, R. L., Herzig, C., Schaltegger, S., and Viere, T. (2019). Diffusion of environmental management accounting for cleaner production: evidence from some case studies. J. Clean. Prod. 224, 479–491. doi: 10.1016/j.jclepro.2019.03.227
Center of Environmental Sciences-Leiden University. (2016). Database. CML-IA characterisation factors. Leiden, the Netherlands: CML-Department of Indiustrial Ecology, University of Leiden.
Ciccarese, L., Mattsson, A., and Pettenella, D. (2012). Ecosystem services from forest restoration: thinking ahead. New For. 43, 543–560. doi: 10.1007/s11056-012-9350-8
Dodson, E. M. (2021). Challenges in forest road maintenance in North America. Croat. J. Forest Eng. 42, 107–116. doi: 10.5552/crojfe.2021.777
Drosos, V. C., Liampas, A.-S. G., and Giannoulas, V. (2013). Assessment of forest opening up with multi-criteria analysis. Proc. Technol. 8, 144–151. doi: 10.1016/j.protcy.2013.11.020
Đuka, A., Vusić, D., Horvat, D., Šušnja, M., Pandur, Z., Papa, I., et al. (2017). LCA studies in forestry–stagnation or Progress? Croat. J. Forest Eng. 38, 311–326.
Dvořák, J., Chytrỳ, M., Natov, P., Jankovský, M., Beljan, K., et al. (2019). Long-term cost analysis of mid-performance harvesters in Czech conditions. Aust. J. Forest Sci. 136, 351–372.
Felton, A., Nilsson, U., Sonesson, J., Felton, A. M., Roberge, J. M., Ranius, T., et al. (2016). Replacing monocultures with mixed-species stands: ecosystem service implications of two production forest alternatives in Sweden. Ambio 45, 124–139. doi: 10.1007/s13280-015-0749-2
Ferro, F. S., Silva, D. A. L., Icimoto, F. H., Lahr, F. A., and González-García, S. (2018). Environmental life cycle assessment of industrial pine roundwood production in Brazilian forests. Sci. Total Environ. 640-641, 599–608. doi: 10.1016/j.scitotenv.2018.05.262
Font, X., and Tribe, J. (2000). Recreation, conservation and timber production: a sustainable relationship? Forest tourism and recreation: case studies in environmental management, pp. 1–22.
Frey, B., Kremer, J., Rüdt, A., Sciacca, S., Matthies, D., and Lüscher, P. (2009). Compaction of forest soils with heavy logging machinery affects soil bacterial community structure. Eur. J. Soil Biol. 45, 312–320. doi: 10.1016/j.ejsobi.2009.05.006
García-Tomillo, A., Figueiredo, T. d., Almeida, A., Rodrigues, J., Dafonte Dafonte, J., Paz-González, A., et al. (2017). Comparing effects of tillage treatments performed with animal traction on soil physical properties and soil electrical resistivity: preliminary experimental results. Open Agric. 2, 317–328. doi: 10.1515/opag-2017-0036
Gergel, S. E., Powell, B., Baudron, F., Wood, S. L. R., Rhemtulla, J. M., Kennedy, G., et al. (2020). Conceptual links between landscape diversity and diet diversity: a roadmap for transdisciplinary research. Bioscience 70, 563–575. doi: 10.1093/biosci/biaa048
Goglio, P., Smith, W. N., Worth, D. E., Grant, B. B., Desjardins, R. L., Chen, W., et al. (2018). Development of Crop.LCA, an adaptable screening life cycle assessment tool for agricultural systems: a Canadian scenario assessment. J. Clean. Prod. 172, 3770–3780. doi: 10.1016/j.jclepro.2017.06.175
Hayati, E., Majnounian, B., Abdi, E., Sessions, J., and Makhdoum, M. (2013). An expert-based approach to forest road network planning by combining Delphi and spatial multi-criteria evaluation. Environ. Monit. Assess. 185, 1767–1776. doi: 10.1007/s10661-012-2666-1
Heinimann, H. R. (2012). Life cycle assessment (LCA) in forestry–state and perspectives. Croat. J. Forest Eng. 33, 357–372.
Heinimann, H. R., and Maeda-Inaba, S. (2003). Quantification of environmental performance indicators EPIS for forest roads. Proceedings of the Austro2003 meeting: High Tech Forest Operations for Mountainous Terrain, October.
Hellweg, S., and Milà i Canals, L. (2014). Emerging approaches, challenges, and opportunities in life cycle assessment. Science 344, 1109–1113. doi: 10.1126/science.1248361
Kļaviņš, I., Bārdule, A., Lībiete, Z., Lazdiņa, D., and Lazdiņš, A. (2019). Impact of biomass harvesting on nitrogen concentration in the soil solution in hemiboreal woody ecosystems. Silva Fennica 53:16. doi: 10.14214/sf.10016
Klein, D., Wolf, C., Schulz, C., and Weber-Blaschke, G. (2015). 20 years of life cycle assessment (LCA) in the forestry sector: state of the art and a methodical proposal for the LCA of forest production. Int. J. Life Cycle Assess. 20, 556–575. doi: 10.1007/s11367-015-0847-1
Kretzschmar, A. (1991). Burrowing ability of the earthworm Aporrectodea longa limited by soil compaction and water potential. Biol. Fertil. Soils 11, 48–51. doi: 10.1007/BF00335834
Kühmaier, M., Kral, I., and Kanzian, C. (2022). Greenhouse gas emissions of the Forest supply chain in Austria in the year 2018. Sustain. For. 14:792. doi: 10.3390/su14020792
Latan, H., Chiappetta Jabbour, C. J., Lopes de Sousa Jabbour, A. B., Wamba, S. F., and Shahbaz, M. (2018). Effects of environmental strategy, environmental uncertainty and top management’s commitment on corporate environmental performance: the role of environmental management accounting. J. Clean. Prod. 180, 297–306. doi: 10.1016/j.jclepro.2018.01.106
Lopes Silva, D. A., Nunes, A. O., Piekarski, C. M., da Silva Moris, V. A., de Souza, L. S. M., and Rodrigues, T. O. (2019). Why using different life cycle assessment software tools can generate different results for the same product system? A cause–effect analysis of the problem. Sustain. Prod. Consum. 20, 304–315. doi: 10.1016/j.spc.2019.07.005
Lundström, J., Öhman, K., and Laudon, H. (2018). Comparing buffer zone alternatives in forest planning using a decision support system. Scand. J. For. Res. 33, 493–501. doi: 10.1080/02827581.2018.1441900
M’hamdi, A. I., Kandri, N. I., Zerouale, A., Blumberga, D., and Gusca, J. (2017). Life cycle assessment of paper production from treated wood. Energy Procedia 128, 461–468. doi: 10.1016/j.egypro.2017.09.031
Majlingová, A. (2012). Opening-up of forests for fire extinguishing purposes. Croat. J. Forest Eng. 33, 159–168.
Martin, P. A., Newton, A. C., Pfeifer, M., Khoo, M. S., and Bullock, J. M. (2015). Impacts of tropical selective logging on carbon storage and tree species richness: a meta-analysis. For. Ecol. Manag. 356, 224–233. doi: 10.1016/j.foreco.2015.07.010
Michelsen, O., Cherubini, F., and Strømman, A. H. (2012). Impact assessment of biodiversity and carbon pools from land use and land use changes in life cycle assessment, exemplified with forestry operations in Norway. J. Ind. Ecol. 16, 231–242. doi: 10.1111/j.1530-9290.2011.00409.x
Ministry of Agriculture of the Czech Republic. (2020). Zpráva o stavu lesa a lesního hospodářství České Republiky v roce 2019. Prague, the Czech Republic: Ministerstvo zemědělství (Ministry of Agriculture the Czech Republic).
Miura, S., Amacher, M., Hofer, T., San-Miguel-Ayanz, J., Ernawati, E., and Thackway, R. (2015). Protective functions and ecosystem services of global forests in the past quarter-century. For. Ecol. Manag. 352, 35–46. doi: 10.1016/j.foreco.2015.03.039
Patterson, T. M., and Coelho, D. L. (2009). Ecosystem services: foundations, opportunities, and challenges for the forest products sector. For. Ecol. Manag. 257, 1637–1646. doi: 10.1016/j.foreco.2008.11.010
Patterson, M., McDonald, G., and Hardy, D. (2017). Is there more in common than we think? Convergence of ecological footprinting, emergy analysis, life cycle assessment and other methods of environmental accounting. Ecol. Model. 362, 19–36. doi: 10.1016/j.ecolmodel.2017.07.022
Peñaloza, D., Røyne, F., Sandin, G., Svanström, M., and Erlandsson, M. (2019). The influence of system boundaries and baseline in climate impact assessment of forest products. Int. J. Life Cycle Assess. 24, 160–176. doi: 10.1007/s11367-018-1495-z
Picchio, R., Pignatti, G., Marchi, E., Latterini, F., Benanchi, M., Foderi, C., et al. (2018). The application of two approaches using GIS technology implementation in Forest road network planning in an Italian Mountain setting. Forests 9:277. doi: 10.3390/f9050277
Piirainen, S., Finér, L., and Starr, M. (2015). Changes in forest floor and mineral soil carbon and nitrogen stocks in a boreal forest after clear-cutting and mechanical site preparation. Eur. J. Soil Sci. 66, 735–743. doi: 10.1111/ejss.12264
Price, M. (1987). Tourism and forestry in the Swiss Alps: parasitism or Symbiosis? Mt. Res. Dev. 7, 1–12. doi: 10.2307/3673320
Sacchelli, S., and Bernetti, I. (2019). Integrated Management of Forest Ecosystem Services: an optimization model based on multi-objective analysis and metaheuristic approach. Nat. Resour. Res. 28, 5–14. doi: 10.1007/s11053-018-9413-4
Sadaghiani, S. (2023). Life cycle assessment of bioenergy production using wood pellets: The case of remote communities in Canada (PhD Thesis). 16. Canada: Concordia University.
Sahoo, K., Bergman, R., Alanya-Rosenbaum, S., Gu, H., and Liang, S. (2019). Life cycle assessment of Forest-based products: a review. Sustain. For. 11:4722. doi: 10.3390/su11174722
Schmidt, J. H., Holm, P., Merrild, A., and Christensen, P. (2007). Life cycle assessment of the waste hierarchy–a Danish case study on waste paper. Waste Manag. 27, 1519–1530. doi: 10.1016/j.wasman.2006.09.004
Seedre, M., Felton, A., and Lindbladh, M. (2018). What is the impact of continuous cover forestry compared to clearcut forestry on stand-level biodiversity in boreal and temperate forests? A systematic review protocol. Environ. Evid. 7:28. doi: 10.1186/s13750-018-0138-y
Sgarbossa, A., Boschiero, M., Pierobon, F., Cavalli, R., and Zanetti, M. (2020). Comparative life cycle assessment of bioenergy production from different wood pellet supply chains. Forests 11:1127. doi: 10.3390/f11111127
Stohr, W. G. (2013). Belowground ecosystems: the foundation for forest health, restoration and sustainable management. J. Environ. Assmt Pol Mgmt 15:1350019. doi: 10.1142/S1464333213500191
Sundnes, F., Karlsson, M., Platjouw, F. M., Clarke, N., Kaste, Ø., and Valinia, S. (2020). Climate mitigation and intensified forest management in Norway: to what extent are surface waters safeguarded? Ambio 49, 1736–1746. doi: 10.1007/s13280-020-01357-1
Tan, X., Chang, S. X., and Kabzems, R. (2008). Soil compaction and forest floor removal reduced microbial biomass and enzyme activities in a boreal aspen forest soil. Biol. Fertil. Soils 44, 471–479. doi: 10.1007/s00374-007-0229-3
Ugawa, S., Inagaki, Y., Karibu, F., and Tateno, R. (2020). Effects of soil compaction by a forestry machine and slash dispersal on soil N mineralization in Cryptomeria japonica plantations under high precipitation. New For. 51, 887–907. doi: 10.1007/s11056-019-09768-z
Vauhkonen, J., and Ruotsalainen, R. (2017). Assessing the provisioning potential of ecosystem services in a Scandinavian boreal forest: suitability and tradeoff analyses on grid-based wall-to-wall forest inventory data. For. Ecol. Manag. 389, 272–284. doi: 10.1016/j.foreco.2016.12.005
Virbickas, L., and Galickaja, I. K. (2017). Analysis of air emissions and greenhouse gases in woody biomass production chain. Environ. Res. Eng. Manag. 73, 41–51. doi: 10.5755/j01.erem.73.2.18806
Wan, M., D’Amato, D., Toppinen, A., and Rekola, M. (2017). Forest company dependencies and impacts on ecosystem services: expert perceptions from China. Forests 8:134. doi: 10.3390/f8040134
Ward, J. S., and Williams, S. C. (2020). Influence of deer hunting and residual stand structure on tree regeneration in deciduous forests. Wildl. Soc. Bull. 44, 519–530. doi: 10.1002/wsb.1120
Keywords: production chain, silviculture, harvesting technology, life cycle assessment, environmental impact assessment
Citation: Hájek M, Pulkrab K, Purwestri RC, Tichá M and Paduchová M (2024) Life cycle assessment approach of silviculture and timber harvesting of Norway spruce – a case study in the Czech Republic. Front. For. Glob. Change. 7:1276740. doi: 10.3389/ffgc.2024.1276740
Edited by:
Enrico Tomelleri, Free University of Bozen-Bolzano, ItalyReviewed by:
Leonardo Bianchini, University of Tuscia, ItalyTobias Viere, Pforzheim University of Applied Sciences, Germany
Martin Kühmaier, University of Natural Resources and Life Sciences Vienna, Austria
Copyright © 2024 Hájek, Pulkrab, Purwestri, Tichá and Paduchová. This is an open-access article distributed under the terms of the Creative Commons Attribution License (CC BY). The use, distribution or reproduction in other forums is permitted, provided the original author(s) and the copyright owner(s) are credited and that the original publication in this journal is cited, in accordance with accepted academic practice. No use, distribution or reproduction is permitted which does not comply with these terms.
*Correspondence: Miroslav Hájek, hajek@fld.czu.cz; Ratna Chrismiari Purwestri, purwestri@fld.czu.cz