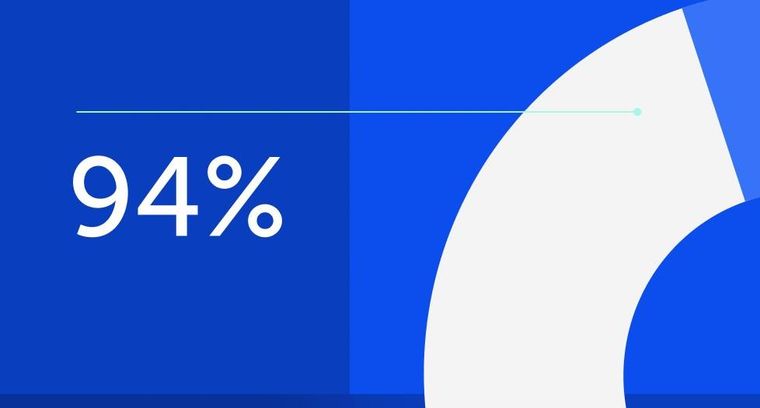
94% of researchers rate our articles as excellent or good
Learn more about the work of our research integrity team to safeguard the quality of each article we publish.
Find out more
ORIGINAL RESEARCH article
Front. For. Glob. Change, 11 January 2024
Sec. Forest Soils
Volume 6 - 2023 | https://doi.org/10.3389/ffgc.2023.1304897
Introduction: Burgeoning mycorrhizal research has focused on identifying the various diverse mycorrhizal strategies of forest communities. Mounting evidence suggests that mycorrhizae play important roles in regulating forest community structure and composition. However, research into the manifestation of this influence in the seedling stage is lacking, especially in small-scale plots.
Methods: Our research utilized structural equation models parameterized using data from a subtropical monsoon broad-leaved evergreen forest situated in Yunnan Province, China.
Results: We noted that seedlings included plants that utilized both arbuscular mycorrhizal (AM) and ectomycorrhizal (EM) fungi. More seedling plots with the relatively higher species diversity were the product of the mixed mycorrhizal strategy, meaning the coexistence of AM and EM seedlings in a small-scale plot rather than the dominance of one type of strategies. These mixed communities were primarily found on slopes and mountain ridges. The abundance of AM or EM trees indirectly affected seedling diversity by influencing the abundance of different mycorrhizal seedlings. In this case, the diversity of the mixed mycorrhizal community was also restricted by the level of leaf thickness in the community, followed by abiotic factors such as elevation, convexity, soil pH and soil available phosphorus.
Discussion: The effect of leaf thickness on mycorrhizal seedling abundance reflected the importance of water conditions in shaping seedling communities. The effect of convexity on seedling diversity also reflected the limitation of seedling communities by the distribution of humidity and heat conditions during topographic change. The results suggested that it is crucial to preserve seedling diversity via the mixed mycorrhizal strategy of communities in the subtropical forest.
Mycorrhizae are symbionts that facilitate nutrient exchange between mycorrhizal fungi and host plants (Tedersoo et al., 2020). Most plant families or species form these symbioses in forests (Genre et al., 2020). The two most common types of mycorrhizae found in woody plants are arbuscular mycorrhizae (AM) and ectomycorrhizae (EM), which possess similarities and differences in their functional roles (Corrales et al., 2018; Lanfranco et al., 2018; Dreyer et al., 2019; Genre et al., 2020; Wasyliw and Karst, 2020; Liang et al., 2021; Averill et al., 2022). Interestingly, in mixed forests, EM tree species tend to be overall larger, and the majority of biomass is allocated to EM tree species within the community (Ryberg and Matheny, 2012; Corrales et al., 2016; Mao et al., 2019). However, this is due largely to the preponderance of several large tree species, as most species (75% of terrestrial plants) do not form EM associations and instead associate with AM fungi (Mao et al., 2019; Genre et al., 2020). Plants associated with different mycorrhizal types differ in environmental or climatic adaptation (Soudzilovskaia et al., 2015). AM plants are more resilient in short-term dry environments (Kilpeläinen et al., 2020), whereas EM plants are more dependent on relatively constant wet environments (Soudzilovskaia et al., 2015). Globally, tropical to subtropical regions are predominantly composed of AM-associated plants, whereas EM plant species dominate temperate and boreal regions (Soudzilovskaia et al., 2019). It is worth noting that Fagaceae plants are one of the major components in subtropical evergreen broad-leaved forests in East Asia (Yu et al., 2017), which form EM associations more commonly (Kong et al., 2014; Li et al., 2021). Moreover, EM plant species are still widespread in tropical forests (Corrales et al., 2018). Hence, in mixed forests from subtropical or tropical regions, it appears unsuitable to solely ascribe the formation of forest diversity to richer AM-associated plants.
Mycorrhizae may influence plant diversity in different geographic regions (Karst et al., 2018; Zhong et al., 2021), and the tendency of AM and EM tree species to segregate in spatial distribution may be detrimental to forest diversity. This means that the occurrence of many tree species associated with only one mycorrhizal type may limit forest diversity (Carteron et al., 2022). Because trees and mycorrhizal fungi establish symbiotic relationships that facilitate mutual resource transfer and recruitment, forest community regeneration may produce more trees associated with the same mycorrhizal type (i.e., the single mycorrhizal strategy with AM or EM) than with alternative mycorrhizal types (Averill et al., 2022). In contrast, there are also mixed mycorrhizal forests. These forests with different mycorrhizal tree species tend to be more diverse than forests with single mycorrhizal tree species (Carteron et al., 2022). The coexistence of AM and EM mycorrhizal tree species is a mixed mycorrhizal strategy of communities that is beneficial for improving forest productivity (Luo et al., 2023). Mixed mycorrhizal strategies in more productive subtropical and tropical forests should then be emphasized (Liu et al., 2018). At the same time, there are different effects of mycorrhizal tree species on mycorrhizal seedling species. In small-scale spatial patterns, EM seedling species are more clustered around adult EM trees, while AM seedling species are more distant from adult AM trees (Johnson et al., 2018; Liang et al., 2020). Then, the mycorrhizal strategy of the seedling community is also intriguing.
The seedling stage is incredibly important for determining ecosystem demography and community composition (McDowell et al., 2020). For obvious reasons, seedlings play an essential function in short and long-term forest ecosystem processes (Wills et al., 2021). Mycorrhizae are closely associated with seedlings and their diversity. Plant–soil feedback studies are concerned with the effect of seedlings on the accumulation of soil pathogens, which feeds back into changes in seedling density (Chen et al., 2019; Kadowaki et al., 2021; Liang et al., 2021). Research has connected negative plant–soil feedback induced by the buildup of soil pathogens to the formation of community diversity in several studies of AM plants (Mangan et al., 2010; Bennett et al., 2017; Chen et al., 2019; Mao et al., 2019; Eagar et al., 2020; Segnitz et al., 2020; Tedersoo et al., 2020; Zhong et al., 2021). Associations with either AM and EM have been shown to protect against this pathogen damage, potentially influencing seedling success and therefore impacting forest community dynamics. One study suggests EM seedlings do not appear as susceptible to the conspecific negative density dependence caused by pathogens and in general tend to exhibit positive plant–soil feedback (Chen et al., 2019). The faster colonization rate of EM fungi on seedling hosts is thought to be the reason for the higher resistance of EM seedlings to pathogens compared to AM seedlings (Liang et al., 2021). In mediating the success of seedlings, fungi can impact the relative rarity of species, and it has been proposed that the strength of this impact leads to patterns of rareness observed in tree communities (Liang et al., 2020; Tedersoo et al., 2020). The response of common seedling hosts of fungi to the negative density dependence is weak, and its abundance is associated with net plant–soil feedbacks (Schroeder et al., 2020). Therefore, if EM seedlings are more abundant, their coexistence with other relatively rare AM seedlings in the community is likely to be compatible.
In the workings of the symbiosis, plants have easier access to carbon, while mycorrhizae have easier access to phosphorus and nitrogen (Dreyer et al., 2019; Genre et al., 2020). Coexistence of different mycorrhizal species in communities may result in complementary nutrient utilization (Luo et al., 2023). As we know, AM fungi can transform the occluded phosphorus in the rhizosphere and improve the availability of soil phosphorus (Liu Y. et al., 2021), and also can induce local and systemic resistance to pathogens and pests by triggering an increase in phenols (Zhu et al., 2015). While EM fungi has been shown to facilitate soil nitrogen cycling (Corrales et al., 2016). The nutrient acquisition capacity of plants can vary with their mycorrhizal types. AM plant species can produce more roots to draw underground nutrients, while EM fungi can produce more mycelium to help EM plant species access nutrients (Chen et al., 2016). EM plant species are more conserved in their use of nitrogen than AM plant species in both temperate and tropical forests (Averill et al., 2019). In subtropical forests, the net primary production of AM plant species is superior to that of EM plant species due to stronger nutrient resorption and litter decomposition (Deng et al., 2023). In addition, symbiotic mycorrhizal traits such as mycorrhizal type, exchange rates for resources and shifts in mycorrhizal type over plant lifespan are closely related to plant nutrient acquisition, survival and growth (Chaudhary et al., 2022). Functional traits can reflect the performance of all plants in a community habitat in terms of survival and growth (Muscarella and Uriarte, 2016). There may also be combined effects of different functional traits on seedling growth (Worthy et al., 2020). Mycorrhizal traits, then, may also have the opportunity to interact with functional traits (Wasyliw and Karst, 2020).
Previous studies of mycorrhizal associations in plants have focused on uncovering the physiological and physical effects that mycorrhizal fungi or mycelial networks produce with tree species (Corrales et al., 2018; Genre et al., 2020; Tedersoo et al., 2020). The reason for the slow development of the research is the difficulty of the techniques for investigating plant roots in the field and for simulating mycorrhizal colonization experiments (Karst et al., 2023). Nowadays, with the accumulated knowledge of scientists about plant-mycorrhizal associations and other environmental factors, it is necessary to elaborate the reasons for the formation of community diversity in more dimensions (Soudzilovskaia et al., 2020). A new dimension of studying mycorrhizal strategies in communities has emerged (Averill et al., 2022). Currently, in addition to single mycorrhizal strategies, the mixed mycorrhizal strategy of AM and EM has also been further validated in adult tree communities (Luo et al., 2023). However, few mycorrhizal strategies have been explored in seedling stages of tree communities. It was also shown that the survival of AM seedlings also benefited from EM fungi carried by seedling neighbors (Chen et al., 2019; Liang et al., 2021), suggesting at the possibility of a mixed mycorrhizal strategy forming in seedling communities. Therefore, we focus our efforts on the effect of mycorrhizal strategies on seedling diversity in this study. We have selected a subtropical monsoon broad-leaved evergreen forest, which accommodates a variety of flora and soil fungi with both types of mycorrhizal association (Li et al., 2021; Wang et al., 2022). We sought to explore (1): do mixed-mycorrhizal seedling communities remain mixed, or do they tend to dominance of AM or EM? (2): In seedling communities mixing both mycorrhizal types, what is their spatial autocorrelation? Better stated, how do the two types distribute themselves in uneven topographies? And finally, (3): Could either the environment or the relative distribution of AM and EM associated seedlings be used to predict community composition and diversity?
The investigation was carried out in the Pu’er 30 hm2 forest dynamics plot. This is an experimental plot established in a monsoon broad-leaved evergreen forest in Taiyanghe Provincial Nature Reserve, located at 22° 50′–22° 63′ N, 101° 12′–101° 25′ E in Pu’er City, Yunnan Province, China (Figure 1). We used a total station electronic surveying device to partition our 30 hm2 forest dynamics plot into 750 tree plots, each measuring 20 m × 20 m. This division left us with 30 plots in each east–west row and 25 plots in each north–south column. The corners of each tree plot were flagged using piles of cement, and marked with plot numbers. Within each of the 750 tree plots, a 1 m × 1 m seedling plot was established, each with a corresponding plot number. The plot spans 30 hm2 with an average elevation of 1,523 m, ranging from 1,467 m to 1,587 m. The mean annual temperature at the plot is 17.7°C, and precipitation is concentrated during the rainy season from May to October at an average annual rate of 1547.6 mm.
The elevation, slope, aspect, tree abundance, species richness, and diameter at breast height, as well as soil pH, total potassium, total nitrogen, total phosphorus, organic carbon, available potassium, available phosphorus, and hydrolyzable nitrogen data for all of the 750 tree plots was gathered in 2019 (Li et al., 2020; Wang et al., 2020). In this sampling effort, we chose 375 tree plots, evenly distributed throughout the Pu’er 30 hm2 forest dynamics plot. We collected topsoil from five uniformly dispersed locations within each tree plot for microbial analysis. The samples were combined to create one soil microbial sample (to have a total of 375 microbial samples, one per plot). The soil sample was then screened through a 2 mm wire mesh. All 375 soil microbial samples were preserved in a refrigerator at −20°C. Using 16S/ITS amplicon sequencing techniques at Biomarker Technologies Corporation in Beijing, China, we identified the abundance and species of Operational Taxonomic Units (OTUs) identified for bacteria and fungi in each soil sample (Edgar, 2013; Wang et al., 2022). The bacterial 16S database used was SILVA, and the fungal ITS database was UNITE (Kõljalg et al., 2013; Quast et al., 2013).
We defined a seedling as a woody plant with a diameter at breast height of less than 1 cm. Raw data on seedling abundance and seedling richness were collected through a census of all 750 seedling plots in 2020, and every seedling was identified to the species level using the Flora of China website.1 Samples for functional traits were obtained from healthy seedlings based on the standard of Cornelissen et al. (2003), which entails collecting five seedlings of each species, and two full leaves from each seedling. Functional traits of rare or cryptic species for which we could not collect the data, were obtained by interpolating from the data we could collect. Functional trait data were measured using a tape measure, vernier calipers, and a LI-3000C leaf area meter. We measured the specific leaf area (cm2/g), leaf thickness (mm), and root mass fraction of seedling species. Specific leaf area is the ratio of the area of a single leaf to its dry mass. Root mass fraction is the ratio of the dry mass of roots to the dry mass of the whole seedling. Community-weighted mean trait values (i.e., reflecting the central behavior of species within a community using plot-level trait values weighted by species abundances) for function traits were computed through the use of the R package “FD” (Muscarella and Uriarte, 2016).
Based on the census, we could estimate the species diversity of seedlings and trees, which was quantified using the Shannon-Wiener index (Spellerberg, 2008). This index contains information on species richness and evenness. The coefficient of variation was calculated as the ratio of the standard deviation of all measurements to the mean measurement (Zhang and Chen, 2015). We calculated the coefficient of variation of tree diameter at breast height within each tree plot.
Topography can influence plant richness and soil properties and thus soil fungal diversity (Wang et al., 2022). Convexity is one of the topographic factors that affects the distribution patterns of tree species in the plot (Lan et al., 2011). Convexity, as in the relative curve of the plot surface, was determined by subtracting the average elevation of eight adjacent tree plots from the elevation of a center tree plot (Lan et al., 2011). To calculate the convexity of the boundary plot in the 30 hm2 forest dynamics plot, the elevation at the center was subtracted from the average elevation of the four corners. To ensure linear meaning for the aspect data, we transformed the aspect compass data into a continuous variable for our 750 tree plots in the northern hemisphere using the aspect transformation method (Yu and Sun, 2013). This method usually reflects changes in humidity and heat.
We computed the Shannon-Wiener index for both bacteria and fungi using the data of OTUs for the 375 tree plots. To confirm normality of bacterial and fungal diversity data, we employed the Kolmogorov–Smirnov test with a p - value of greater than 0.05: and both were normally distributed (bacterial diversity with a p - value of 0.14 and fungal diversity with a p - value of 0.95), both tested via the function “ks.test” in R software (Marsaglia et al., 2003). We then conducted spatial autocorrelation tests on the bacterial and fungal diversity data by utilizing the latitude and longitude coordinates. These coordinates represent the cement piles which simultaneously marked the corner of both seedling and tree plots. We utilized the R function “Moran. I” from the R package “ape” to calculate the Moran’s index that is one of spatial autocorrelation test parameters (Gittleman and Kot, 1990; Paradis and Schliep, 2019). Moran’s index shows that both bacterial and fungal diversity exhibit spatial autocorrelation, indicated by p < 0.05. We interpolated the bacterial and fungal diversity from our 375 plots up to the scale of all 750 plots based on the coordinates of all shared cement piles. This was carried out using the ordinary Kriging method by the R packages “sp” and “gstat” (Pebesma, 2004).
We searched for the mycorrhizal type corresponding to the plant species in the published FungalRoot database and associated data from the GBIF website (Soudzilovskaia et al., 2020). We could identify the mycorrhizal type in 61 and 49% of seedlings and trees at the species level, respectively. At the genus level, mycorrhizal types were identified in 98% of seedlings and 96% of trees. For the other remaining species, we retrieved and estimated their mycorrhizal types at the family level and referred to the relevant records on the Angiosperm Phylogeny Website2 for confirmation. To evaluate the effects of different associations between mycorrhizal fungi and plants, we utilized the abundance of different mycorrhizal trees and seedlings.
We define a community that contains only one type of plant-mycorrhizal association as a single mycorrhizal community, and the mixed mycorrhizal community that contain more than one. The most important abiotic factors were identified using principal component analysis (PCA). The PCA result data obtained from PCA analysis of soil and topology were used for subsequent analysis. The linear transformation coefficients for the conversion of soil and topography raw data into PCA result data are the rotation parameter, which indicate the relationship of the PCA result data to the raw data. The correlation between the abundance of diverse mycorrhizal trees and seedlings was examined. We standardized all variables using the basic function “scale()” of the R software to eliminate differences in data units and to ensure normality of the variables. Multiple regression models were constructed using seedling species diversity as the dependent variable and the remaining observed variables as independent variables. The optimal regression model was selected utilizing the R package “MASS” via stepwise regression. The Akaike information criterion served as the basis for selection. We screened out potential factors based on the magnitude of the variance inflation factor to avoid multicollinearity among the independent variables in the optimal regression model. Using a threshold for multicollinearity of a Pearson’s correlation coefficient greater than 0.60 between factors, the most representative factors were chosen to replace those causing multicollinearity.
The independent variables (i.e., the biotic and abiotic factors) affecting seedling species diversity that were retained after screening were used for further analysis. Comparisons were made between the single mycorrhizal community and the mixed mycorrhizal community for the independent variables using the “T-test” or “Wilcoxon test” approach on R software. To demonstrate the trend of large datasets objectively, we used the box-plot to illustrate the comparison findings. Among the results of the comparative analyzes, we picked out noteworthy differences among communities to use as input parameters for training and predicting support vector machines (SVM) implemented using the R package “e1071.” The SVM is a supervised learning algorithm that classifies and predicts based on high-dimensional feature data. We use the validation set approach to evaluate SVM models. Approximately 70% of the plot data was designated for SVM training, whereas the remaining 30% (i.e., the validation set) was set aside for evaluating accuracy. We illustrated the spatial distribution of various mycorrhizal plants and the SVM forecasts of mycorrhizal strategies in seedling communities in a series of spatial grid maps.
The structural equation model (SEM) is a statistical method for analyzing the relationship between variables based on their covariance matrices, and is an important tool for multivariate data analysis. The SEM includes latent variables that are difficult to measure, as well as observed variables that can be directly measured and used to represent these latent variables. Based on the research experience detailed in the introduction of this paper, a sequential left-to-right chain of relationships between latent variables was hypothesized: “Topography–soil nutrients – soil microbial diversity – tree diversity – functional traits – ectomycorrhizae – arbuscular mycorrhizae – seedling diversity.” For each latent variable we chose one or more appropriate observed variables to measure and quantify, as detailed in the section “2.2 Data collection” of the article. After the data dimensionality reduction of observed variables in section “2.3 Analysis of mycorrhizal strategies,” the interrelationships among the latent variables were explored using a series of SEMs and the observed variables.
The mixed mycorrhizal and the single mycorrhizal communities were represented by a total of 473 plots with two or more mycorrhizal plant species and an additional 166 plots with only one mycorrhizal plant species, respectively. The variables that were ultimately kept from the multiple regression model were used as observed variables to create the SEM with the R package “lavaan” (Rosseel, 2012). The Chi-squared test was used to test the extent to which the model fit matched the observed data. The null hypothesis is that the model fit is significantly different from the observed data. The Chi-squared test’s p > 0.05 indicates that there is no significant difference between the model fit and the observed data (i.e., the model fits well), so the null hypothesis is rejected. The assessment metrics for the SEM can also indicate good fit for the model: Comparative fit index (CFI > 0.95), root mean square error of approximation (RSEMA <0.05), goodness-of-fit index (GFI > 0.95), and standardized root mean square residual (SRMR <0.05).
Both direct and indirect paths showed statistically significant correlations between variables, as demonstrated by the SEM. The standardized coefficient of the direct path can be interpreted as a measure of the direction and magnitude of the direct contribution, whereas, the indirect standardized coefficients can be obtained by multiplying all standardized coefficients in each complete pathway of a variable connected to the seedling diversity. Finally, the indirect standardized coefficients are combined to calculate the net indirect standardized coefficient of the variable in question (Yuan et al., 2020).
In the monsoon broad-leaved evergreen forest, EM seedlings were the most abundant, though AM seedlings displayed higher species richness (Table 1). In the mixed mycorrhizal community, EM seedlings had the greatest abundance, though AM seedlings were in a greater number of plots and we were more likely to find single mycorrhizal communities dominated by AM than by EM (Table 1).
In the results of principal component analysis of the mixed mycorrhizal community, soil principal components 1, 2, and 3 together explained 66.59% of the variance in soil nutrient elements, and topography principal components 1 and 2 together explained 60.70% of the variance in topography elements. The rotational parameters are the linear transformation coefficients between elements with principal components. The soil principal component 3 (SPC3) was retained in the stepwise regression process, mainly contributed by soil available phosphorus (rotational parameter: 0.50) and soil pH (rotational parameter: −0.68). The topography principal component 1 (TPC1) was retained in the stepwise regression process, mainly contributed by elevation (rotational parameter: 0.72) and convexity (rotational parameter: 0.65).
For the mixed mycorrhizal community, Pearson correlation analyzes showed a significant negative correlation between EM tree abundance and tree species diversity (R = −0.74) and a significant positive correlation with tree abundance (R = 0.79) (Supplementary Figure S1). Therefore, we chose EM tree abundance instead of tree species diversity and abundance. Based on the above experience, the abundance of AM and EM trees was retained to represent tree diversity. Similarly, in the subsequent analysis of the single mycorrhizal community (Supplementary Figure S2), we replaced tree species diversity and tree abundance with EM tree abundance and tree species richness to represent tree diversity.
The slopes of the fitted lines and the adjusted R-squared values suggest that seedling abundance in the mixed mycorrhizal community primarily increased due to the rise of EM seedling abundance (Figure 2A). In contrast, the rise in seedling abundance in the single mycorrhizal community depended mainly on the increase in AM seedling abundance (Figure 2B). The linear relationship between the abundance of different mycorrhizal tree species on seedling abundance was not significant. The quantitative relationship between AM seedlings and EM seedlings was either positive or neutral.
Figure 2. The quantitative relationship between seedlings and mycorrhizal plants. (A) Mixed mycorrhizal community, (B) Single mycorrhizal community. The shaded areas represent 95% confidence interval.
There was a positive relationship between seedling species diversity and AM seedling abundance, whereas as the abundance of EM seedlings increased, diversity tended to decrease (Figure 3). The emergent soil principal component 3 was negatively correlated with seedling species diversity in the mixed mycorrhizal community (Figure 3A), which we interpret as the positive effect of increasing soil available phosphorus on seedling species diversity and the negative effect of increasing soil pH on seedling species diversity. Topography principal component 1 was positively correlated with increasing seedling species diversity in the mixed mycorrhizal community (Figure 3A), that is to say that increases in either elevation or convexity tended to be associated with increases in seedling species diversity. Furthermore, the seedling species diversity was higher in plots with an overall higher tree species richness, though only in the single mycorrhizal community (Figure 3B).
Figure 3. Multiple linear regression results. (A) Mixed mycorrhizal community, (B) Single mycorrhizal community, SPC3, Soil principal component 3, TPC1, Topography principal component 1. Black squares represent variables that have a significant effect on seedling diversity (p < 0.05), while gray squares are the opposite.
The mixed mycorrhizal community tended to have overall higher seedling species diversity, EM seedling abundance, EM tree abundance, and tended to be at higher elevation with greater convexity when compared to the community dominated only by a single mycorrhizal type (Figures 4A,B,D,G,H). On the other hand, leaf thickness, tree species richness, and soil pH were found to be lower in the mixed community than in the single mycorrhizal community (Figures 4C,E,F). No significant differences were observed in soil available phosphorus and the abundance of AM trees and AM seedlings in seedling communities with different mycorrhizal strategies.
Figure 4. Comparison of key factors affecting seedling species diversity between two mycorrhizal communities. MMC, Mixed mycorrhizal community, SMC, Single mycorrhizal community, (A) Seedling species diversity, (B) EM seedling abundance, (C) Leaf thickness (mm), (D) EM tree abundance, (E) Tree species richness, (F) Soil pH, (G) Elevation (m), (H) Convexity. The box-plot of SMC in panel B cannot be displayed due to too many 0 values. The level of significance: ***p < 0.001.
The prediction results for the validation set data showed that SVM is 91.15% accurate. The accuracy of the prediction results for all seedling plots was 87.79%. Single mycorrhizal plots were primarily found in concave habitats, such as gullies and ravines (Figure 5A). In contrast, mixed mycorrhizal plots were more common and widely distributed on slopes and ridges (Figure 5A). It is worth noting that the seedling communities’ actual distribution corresponds well with the distribution predicted by the SVM (Figure 5B).
Figure 5. Spatial grid maps of different mycorrhizal communities. (A) The reality of seedling plot locations, (B) The prediction of seedling plot locations. We used tree plots to represent seedling plot locations because tree and seedling plots correspond in plot numbers.
The study found that AM and EM have positive and negative effects on seedling diversity, respectively (Figure 6). A positive correlation was identified between EM and AM in the mixed mycorrhizal community (Figure 6A). With respect to functional traits in the mixed mycorrhizal community, leaf thickness had a positive impact on both seedling species diversity and AM seedling abundance, was negatively associated with EM seedling abundance (Figure 6A). Tree diversity, which encompasses AM tree abundance (standardized coefficient = 0.38) and EM tree abundance (standardized coefficient = −0.65), had an indirect impact on seedling diversity within the mixed mycorrhizal community, due to its influence on AM and functional traits (Figure 6A). Of the abiotic factors, topography and soil nutrients had both direct and indirect effects on seedling diversity within the mixed mycorrhizal community (Figure 6A), but neither had a direct impact on seedling diversity or the abundance of mycorrhizal plants in the single mycorrhizal community (Figure 6B). Tree diversity, which encompasses tree species richness (standardized coefficient = 0.60) and EM tree abundance (standardized coefficient = −0.61), had an indirect effect on seedling diversity in the single mycorrhizal community by influencing EM seedling abundance (Figure 6B).
Figure 6. The paths of factors affecting seedling diversity. (A) Mixed mycorrhizal community, (B) Single mycorrhizal community. R2 values indicate the proportion of variation in seedling diversity explained by the model. Bolded text is the latent variable. Below the latent variables are the observed variables. Path coefficients next to the arrows indicate significant standardized prediction coefficients. SEM’s evaluation metrics include the value of p of the Chi-squared test (p > 0.05), CFI, comparative fit index (CFI > 0.95), RSEMA, root mean square error of approximation (RSEMA < 0.05), GFI, goodness-of-fit index (GFI > 0.95), and SRMR, standardized root mean square residual (SRMR < 0.05). The level of significance: ***p < 0.001, **p < 0.01, *p < 0.05.
Topography, soil nutrients, functional traits, and EM directly and indirectly influenced seedling diversity (Figure 7). Tree diversity had the largest indirect contribution (Figure 7). In the mixed mycorrhizal community, functional traits contributed most to seedling diversity, followed by AM, EM, topography, and soil nutrients (Figure 7A). AM had largest direct contribution on seedling diversity (Figure 7A). For seedling diversity within the single mycorrhizal community, EM abundance had the most significant impact, followed by AM and tree diversity (Figure 7B). Furthermore, EM had the largest direct impact (Figure 7B). The seedling communities with different mycorrhizal strategies were influenced by the abundance of mycorrhizal seedlings and trees (Figure 7).
Figure 7. Relative contributions of biotic and abiotic factors to seedling diversity in SEM results. (A) Mixed mycorrhizal community, (B) Single mycorrhizal community. The direct contribution is represented by the direct path coefficient from the variable to seedling diversity, e.g., the path coefficient (0.368) from arbuscular mycorrhizae to seedling diversity, which corresponds to Figure 6A. Similarly, total indirect contributions require an additional calculation to summarize all indirect path coefficients from variables to seedling diversity, such as in the indirect contribution of functional traits to species diversity, which is calculated as “0.342 × 0.368 + (−0.443) × 0.145 × 0.368 + (−0.443) × (−0.190) = 0.186”.
This study examined how seedling diversity may be predicted or estimated by the relative makeup of AM and EM tree species in a subtropical monsoon evergreen broad-leaved forest. Statistical analysis indicated that the seedling community was, unsurprisingly, mainly composed of AM and EM seedlings. Interestingly, there was some obvious geospatial autocorrelation determining whether a given seedling plot was dominated by a single or a mixed mycorrhizal community. Mycorrhizal communities found in concave habitats, such as gullies and ravines, are typically comprised of the single mycorrhizal community. On the other hand, slopes and ridges usually host mixed mycorrhizal communities. The primary reason for this distinction lies in the varying levels of mycorrhizal plant abundance, particularly among EM seedlings and trees. Other factors influencing the ultimate relative contribution of each mycorrhizal type could be linked to both specific leaf thickness and to soil nutrient content. The abundance of AM trees or seedlings did not significantly vary among different mycorrhizal communities and their corresponding tree communities on small-scale plots. Although there are more plots with only AM-associated seedlings, the abundance of EM seedlings is higher than that of AM seedlings, and the mixed community diversity was consequently affected by the abundance of both AM and EM seedlings. In the path of SEM results, AM and EM tree abundance, representing tree diversity, were positively correlated with AM and EM seedling abundance, respectively, indirectly affecting seedling diversity. Thus, trees could potentially affect the seedling diversity by increasing the presence of seedlings belonging to the same mycorrhizal type.
In the mixed mycorrhizal community, the abundance of AM seedlings had a greater influence on diversity than the abundance of EM seedlings, and as there are overall fewer EM seedling species, increases in the number of EM seedlings reduced seedling diversity. This, and many of our results, are due to the increased number of AM plant species relative to EM plants (Soudzilovskaia et al., 2015). Furthermore, a pair of recent papers suggest that forests with multiple mycorrhizal types can be more productive than those of a single mycorrhizal type (Carteron et al., 2022; Luo et al., 2023). We observed that diversity was highest in seedling communities of mixed mycorrhizal type, and as diversity and productivity are often positively associated, we find our results consistent with their findings.
Our results also suggested that functional traits also contributed to seedling diversity. Greater leaf thickness in communities limited by habitat filtering may lead to increased AM seedling abundance and reduced EM seedling abundance – simply as a consequence of the increased likelihood that an AM seedling species will be able to exist in that habitat as a result of the higher number and more diverse body of AM seedling species. Leaf thickness corresponds to the ability of a plant or community to cope with water stress (Onoda et al., 2011). AM plants dominate in dry climates, whereas EM plants prefer wet climates that have lower selection pressure (Jo et al., 2019; Kilpeläinen et al., 2020). The EM fungal colonization is known to be strongly influenced by seasonal precipitation and significantly inhibited by drought (Soudzilovskaia et al., 2015; Patterson et al., 2019; Lagueux et al., 2021). Thus, the dry season in the monsoon broad-leaved evergreen forest may be an important factor in weakening the dominance of EM seedlings (Soudzilovskaia et al., 2015). Moreover, it has been suggested that weak competition for resources among coexisting species that jointly experience drought stress favors the recovery of plant communities after drought periods, while the opposite is true for single-species communities (Chen et al., 2022). Then, the coexistence of different mycorrhizal seedling species to reduce interspecific competition may also be a continuation strategy developed by the community after long-term adaptation to drought stress. It is worth noting that more EM plants producing EM fungi to resist pathogens is beneficial for the survival of EM seedlings as well as surrounding AM seedlings (Chen et al., 2019; Liang et al., 2021). However, in a high diversity subtropical forest, AM plants are more efficient at nutrient resorption and litter decomposition, thereby increasing net primary productivity and ultimately dominating the forest plot (Deng et al., 2023). At the same time, the inhibition of AM fungi to EM fungi and mycelial networks leads to decreased nutrient acquisition and reduced competitive ability of EM plants (Chen et al., 2016; Deng et al., 2023). Therefore, we suggest that the benefits and drawbacks of coexisting AM and EM seedlings have the potential to reconcile their quantitative relationships, leading to a mixed mycorrhizal strategy for seedling communities. In addition, specific leaf area and root mass fraction, which represent the nutrient uptake capacity of the seedling community (Cornelissen et al., 2003), did not significantly affect seedling abundance as well as the distribution of mycorrhizal communities. This also means that the nutrient uptake capacity of AM and EM seedlings within the subtropical monsoon broad-leaved evergreen forest is not constitute a limitation to the formation of seedling diversity.
Our results indicate that EM and AM seedling composition was consistent with the relative abundance of overstory EM and AM trees in terms of quantitative variation, respectively. A considerable body of evidence suggests adult trees regulate their local soil microbiome, which would suggest they play both a direct and indirect role in the establishment of new trees of the same mycorrhizal type (Fort et al., 2021). The strong interaction between EM seedlings and EM fungi is related to the small range size of EM trees (Karst et al., 2018). Studies at the species level have previously demonstrated the negative density dependence on seedlings by conspecific parent trees (Liu H. et al., 2021). Although it has been argued that the dominant AM trees in forests inhibit the formation of EM networks (Deng et al., 2023), the soil in our study area contains a large number of EM fungi (Wang et al., 2022), proving that the EM networks are not sufficiently influence (Karst et al., 2023). More EM fungi also predicted that the single mycorrhizal strategy is more likely to occur in EM plant communities (Averill et al., 2022). In contrast, the fact that the single mycorrhizal community diversity was dominated by more AM seedling plots is a result of the increased number of AM plants. The contradictory results imply that the genesis of the single mycorrhizal community may not have been captured in our study. On the one hand, fewer seedlings in this environment happen to be captured by the small survey scale, resulting in single mycorrhizal plots appearing. On the other hand, there may be more potential disturbances in the concave environment, such as drinking and feeding activities of animals in gullies or ravines (Krishnadas et al., 2018; Williams et al., 2021), the unfavorable effect of wet conditions on seed germination (Fort et al., 2021), the shade stress of large herbs (Li et al., 2020) and so on.
Survival of mycorrhizal plants can be enhanced by the ability of mycorrhizae to facilitate nutrient uptake (Averill et al., 2022). In subtropical forests, AM fungi have been shown to promote soil available phosphorus (P) production and alleviate P limitation, allowing forests to maintain high biomass and community diversity despite intense soil weathering that has left these ecosystems P-limited (Liu Y. et al., 2021). Our results also suggest that the soil available phosphorus does promote tree diversity, though it had a negative influence on seedling diversity (perhaps through increasing competition) and the overall contribution of P to diversity is relatively small. The positive effect of soil available phosphorus on AM tree abundance also suggests a potential positive association of AM fungi with soil available phosphorus. And the negative association of soil available phosphorus with EM tree abundance further suggested that mycorrhizal-tree interaction may drive the changes in forest community structure across soil fertility gradients (Mao et al., 2019). Existing work has shown that EM fungi promote the growth and survival of EM plants by influencing the soil nitrogen cycle (Corrales et al., 2016). Furthermore, it has been demonstrated that soil acidification caused by high soil nitrogen concentrations may inhibit the survival of AM fungi (Pan et al., 2020). Despite this, we did not observe any interactions between soil total nitrogen or hydrolyzable nitrogen and plant diversity in our study. Moreover, soil pH was negatively correlated with the abundance of AM trees, perhaps, suggesting that the soil nitrogen may not limiting the abundance of AM fungi. The previous study had also tentatively failed to identify the effects of soil nutrients on endophytic in the monsoon broad-leaved evergreen forest (Wang et al., 2022). Therefore, differences in the interaction of EM and AM with soil nutrients may also be one of the conditions for the formation of mixed mycorrhizal strategy communities. It is worth noting that altitudinal gradients in our study sites were small, but there was some contribution of topography (i.e., elevation and convexity) to seedling diversity. This phenomenon can be attributed to changes in species richness associated with environmental dissimilarity along the altitudinal gradient (Barczyk et al., 2023). In addition, changes in elevation can also lead to changes in seedling species composition due to the distribution of adult species along slopes (Xu et al., 2019).
Overall, we found that the coexistence of EM and AM seedlings is a mixed mycorrhizal strategy in seedling communities, and a larger seedling diversity in the mixed mycorrhizal community. This strategy is reflected in the fact that changes in AM and EM seedling abundance act directly on seedling diversity and mediate a range of effects of other biotic and abiotic factors. Good quantitative relationships between EM and AM mycorrhizal plants at a small scale (i.e., 1 m × 1 m seedling plots) are key to the formation of mixed mycorrhizal strategies in seedling communities. If the abundance of mycorrhizal plants restricted the formation of forest diversity, then it is possible to gain a fresh insight into the stochastic process of community assembly in subtropical or even tropical regions. That is, community species composition may not primarily, and instead community assembly is only required to maintain a certain number of mycorrhizal plants, which will hopefully be further demonstrated in future studies of species turnover.
The datasets presented in this study can be found in online repositories. The names of the repository/repositories and accession number(s) can be found in the article/Supplementary material.
ZC: Conceptualization, Data curation, Investigation, Software, Writing – original draft, Writing – review & editing. WL: Data curation, Formal analysis, Methodology, Project administration, Writing – original draft, Writing – review & editing. XL: Data curation, Formal analysis, Investigation, Writing – original draft, Writing – review & editing. MW: Writing – original draft, Data curation, Investigation. JL: Investigation, Software, Writing – original draft. CX: Investigation, Writing – original draft.
The author(s) declare financial support was received for the research, authorship, and/or publication of this article. This work was supported by Yunnan Fundamental Research Projects (grant no. 202001AS070005).
We sincerely thank the staff of Taiyanghe Provincial Nature Reserve for their help in the fieldwork. We would like to thank Daniel Petticord at the University of Cornell for his assistance with English language and grammatical editing of the manuscript.
The authors declare that the research was conducted in the absence of any commercial or financial relationships that could be construed as a potential conflict of interest.
All claims expressed in this article are solely those of the authors and do not necessarily represent those of their affiliated organizations, or those of the publisher, the editors and the reviewers. Any product that may be evaluated in this article, or claim that may be made by its manufacturer, is not guaranteed or endorsed by the publisher.
The Supplementary material for this article can be found online at: https://www.frontiersin.org/articles/10.3389/ffgc.2023.1304897/full#supplementary-material
SUPPLEMENTARY FIGURE S1 | Pearson correlation analysis of the mixed mycorrhizal community. Note: DBHCV - The coefficient of variation of tree diameter at breast height. The results of principal component analysis of soil nutrient and topography elements included SPC1 - Soil principal component 1, SPC2 - Soil principal component 2, SPC3 - Soil principal component 3, TPC1 - Topography principal component 1, TPC2 - Topography principal component 2. The level of significance: ***p < 0.001, **p < 0.01 and *p < 0.05.
SUPPLEMENTARY FIGURE S2 | Pearson correlation analysis of the single mycorrhizal community. Note: DBHCV - The coefficient of variation of tree diameter at breast height. The results of principal component analysis of soil nutrient and topography elements included SPC1 - Soil principal component 1, SPC2 - Soil principal component 2, SPC3 - Soil principal component 3, TPC1 - Topography principal component 1, TPC2 - Topography principal component 2. The level of significance: ***p < 0.001, **p < 0.01 and *p < 0.05.
Averill, C., Bhatnagar, J. M., Dietze, M. C., Pearse, W. D., and Kivlin, S. N. (2019). Global imprint of mycorrhizal fungi on whole-plant nutrient economics. Proc. Natl. Acad. Sci. U. S. A. 116, 23163–23168. doi: 10.1073/pnas.1906655116
Averill, C., Fortunel, C., Maynard, D. S., van den Hoogen, J., Dietze, M. C., Bhatnagar, J. M., et al. (2022). Alternative stable states of the forest mycobiome are maintained through positive feedbacks. Nat. Ecol. Evol. 6, 375–382. doi: 10.1038/s41559-022-01663-9
Barczyk, M. K., Acosta-Rojas, D. C., Espinosa, C. I., Schleuning, M., and Neuschulz, E. L. (2023). Biotic pressures and environmental heterogeneity shape beta-diversity of seedling communities in tropical montane forests. Ecography 2023:e06538. doi: 10.1111/ecog.06538
Bennett, J. A., Maherali, H., Reinhart, K. O., Lekberg, Y., Hart, M. M., and Klironomos, J. (2017). Plant-soil feedbacks and mycorrhizal type influence temperate forest population dynamics. Science 355, 181–184. doi: 10.1126/science.aai8212
Carteron, A., Vellend, M., and Laliberté, E. (2022). Mycorrhizal dominance reduces local tree species diversity across US forests. Nat. Ecol. Evol. 6, 370–374. doi: 10.1038/s41559-021-01634-6
Chaudhary, V. B., Holland, E. P., Charman-Anderson, S., Guzman, A., Bell-Dereske, L., Cheeke, T. E., et al. (2022). What are mycorrhizal traits? Ecol. Evol. 37, 573–581. doi: 10.1016/j.tree.2022.04.003
Chen, W. L., Koide, R. T., Adams, T. S., DeForest, J. L., Cheng, L., and Eissenstat, D. M. (2016). Root morphology and mycorrhizal symbioses together shape nutrient foraging strategies of temperate trees. Proc. Natl. Acad. Sci. U. S. A. 113, 8741–8746. doi: 10.1073/pnas.1601006113
Chen, L., Swenson, N. G., Ji, N. N., Mi, X. C., Ren, H. B., Guo, L. D., et al. (2019). Differential soil fungus accumulation and density dependence of trees in a subtropical forest. Science 366, 124–128. doi: 10.1126/science.aau1361
Chen, Y. X., Vogel, A., Wagg, C., Xu, T. Y., Iturrate-Garcia, M., Scherer-Lorenzen, M., et al. (2022). Drought-exposure history increases complementarity between plant species in response to a subsequent drought. Nat. Commun. 13:3217. doi: 10.1038/s41467-022-30954-9
Cornelissen, J. H. C., Lavorel, S., Garnier, E., Díaz, S., Buchmann, N., Gurvich, D. E., et al. (2003). A handbook of protocols for standardised and easy measurement of plant functional traits worldwide. Aust. J. Bot. 51, 335–380. doi: 10.1071/BT02124
Corrales, A., Henkel, T. W., and Smith, M. E. (2018). Ectomycorrhizal associations in the tropics-biogeography, diversity patterns and ecosystem roles. New Phytol. 220, 1076–1091. doi: 10.1111/nph.15151
Corrales, A., Mangan, S. A., Turner, B. L., and Dalling, J. W. (2016). An ectomycorrhizal nitrogen economy facilitates monodominance in a neotropical forest. Ecol. Lett. 19, 383–392. doi: 10.1111/ele.12570
Deng, M. F., Hu, S. J., Guo, L. L., Jiang, L., Huang, Y. Y., Schmid, B., et al. (2023). Tree mycorrhizal association types control biodiversity-productivity relationship in a subtropical forest. Sci. Adv. 9:eadd4468. doi: 10.1126/sciadv.add4468
Dreyer, I., Spitz, O., Kanonenberg, K., Montag, K., Handrich, M. R., Ahmad, S., et al. (2019). Nutrient exchange in arbuscular mycorrhizal symbiosis from a thermodynamic point of view. New Phytol. 222, 1043–1053. doi: 10.1111/nph.15646
Eagar, A. C., Cosgrove, C. R., Kershner, M. W., and Blackwood, C. B. (2020). Dominant community mycorrhizal types influence local spatial structure between adult and juvenile temperate forest tree communities. Funct. Ecol. 34, 2571–2583. doi: 10.1111/1365-2435.13674
Edgar, R. C. (2013). UPARSE: highly accurate OTU sequences from microbial amplicon reads. Nat. Methods 10, 996–998. doi: 10.1038/nmeth.2604
Fort, T., Pauvert, C., Zanne, A. E., Ovaskainen, O., Caignard, T., Barret, M., et al. (2021). Maternal effects shape the seed mycobiome in Quercus petraea. New Phytol. 230, 1594–1608. doi: 10.1111/nph.17153
Genre, A., Lanfranco, L., Perotto, S., and Bonfante, P. (2020). Unique and common traits in mycorrhizal symbioses. Nat. Rev. Microbiol. 18, 649–660. doi: 10.1038/s41579-020-0402-3
Gittleman, J. L., and Kot, M. (1990). Adaptation: statistics and a null model for estimating phylogenetic effects. Syst. Zool. 39:227. doi: 10.2307/2992183
Jo, I., Fei, S. L., Oswalt, C. M., Domke, G. M., and Phillips, R. P. (2019). Shifts in dominant tree mycorrhizal associations in response to anthropogenic impacts. Sci. Adv. 5:eaav6358. doi: 10.1126/sciadv.aav6358
Johnson, D. J., Clay, K., and Phillips, R. P. (2018). Mycorrhizal associations and the spatial structure of an old-growth forest community. Oecologia 186, 195–204. doi: 10.1007/s00442-017-3987-0
Kadowaki, K., Yamamoto, S., Sato, H., Tanabe, A. S., and Toju, H. (2021). Aboveground herbivores drive stronger plant species-specific feedback than belowground fungi to regulate tree community assembly. Oecologia 195, 773–784. doi: 10.1007/s00442-021-04868-0
Karst, J., Burns, C., Cale, J. A., Antunes, P. M., Woods, M., Lamit, L. J., et al. (2018). Tree species with limited geographical ranges show extreme responses to ectomycorrhizas. Glob. Ecol. Biogeogr. 27, 839–848. doi: 10.1111/geb.12745
Karst, J., Jones, M. D., and Hoeksema, J. D. (2023). Positive citation bias and overinterpreted results lead to misinformation on common mycorrhizal networks in forests. Nat. Ecol. Evol. 7, 501–511. doi: 10.1038/s41559-023-01986-1
Kilpeläinen, J., Aphalo, P. J., Barbero-López, A., Adamczyk, B., Nipu, S. A., and Lehto, T. (2020). Are arbuscular-mycorrhizal Alnus incana seedlings more resistant to drought than ectomycorrhizal and nonmycorrhizal ones? Tree Physiol. 40, 782–795. doi: 10.1093/treephys/tpaa035
Kõljalg, U., Nilsson, R. H., Abarenkov, K., Tedersoo, L., Taylor, A. F. S., Bahram, M., et al. (2013). Towards a unified paradigm for sequence-based identification of fungi. Mol. Ecol. 22, 5271–5277. doi: 10.1111/mec.12481
Kong, D. L., Ma, C. G., Zhang, Q., Li, L., Chen, X. Y., Zeng, H., et al. (2014). Leading dimensions in absorptive root trait variation across 96 subtropical forest species. New Phytol. 203, 863–872. doi: 10.1111/nph.12842
Krishnadas, M., Bagchi, R., Sridhara, S., and Comita, L. S. (2018). Weaker plant-enemy interactions decrease tree seedling diversity with edge-effects in a fragmented tropical forest. Nat. Commun. 9:4523. doi: 10.1038/s41467-018-06997-2
Lagueux, D., Jumpponen, A., Porras-Alfaro, A., Herrera, J., Chung, Y. A., Baur, L. E., et al. (2021). Experimental drought re-ordered assemblages of root-associated fungi across north American grasslands. J. Ecol. 109, 776–792. doi: 10.1111/1365-2745.13505
Lan, G. Y., Hu, Y. H., Cao, M., and Zhu, H. (2011). Topography related spatial distribution of dominant tree species in a tropical seasonal rain forest in China. For. Ecol. Manag. 262, 1507–1513. doi: 10.1016/j.foreco.2011.06.052
Lanfranco, L., Fiorilli, V., and Gutjahr, C. (2018). Partner communication and role of nutrients in the arbuscular mycorrhizal symbiosis. New Phytol. 220, 1031–1046. doi: 10.1111/nph.15230
Li, S. F., Lang, X. D., Huang, X. B., Wang, Y. H., Liu, W. D., Xu, C. H., et al. (2020). Association classification of a 30 hm2 dynamics plot in the monsoon broad-leaved evergreen forest in Pu’er, Yunnan, China. Chinese J. Plant Ecol. 44, 236–247. doi: 10.17521/cjpe.2019.0268 (in Chinese)
Li, M. X., Wu, G., and Yang, Z. L. (2021). Four new species of Hemileccinum (Xerocomoideae, Boletaceae) from southwestern China. J. Fungi 7:823. doi: 10.3390/jof7100823
Liang, M. X., Johnson, D., Burslem, D. F. R. P., Yu, S. X., Fang, M., Taylor, J. D., et al. (2020). Soil fungal networks maintain local dominance of ectomycorrhizal trees. Nat. Commun. 11:2636. doi: 10.1038/s41467-020-16507-y
Liang, M. X., Shi, L. Q., Burslem, D. F. R. P., Johnson, D., Fang, M., Zhang, X. Y., et al. (2021). Soil fungal networks moderate density-dependent survival and growth of seedlings. New Phytol. 230, 2061–2071. doi: 10.1111/nph.17237
Liu, X. B., Burslem, D. F. R. P., Taylor, J. D., Taylor, A. F. S., Khoo, E., Majalap-Lee, N., et al. (2018). Partitioning of soil phosphorus among arbuscular and ectomycorrhizal trees in tropical and subtropical forests. Ecol. Lett. 21, 713–723. doi: 10.1111/ele.12939
Liu, H. M., Johnson, D. J., Yang, Q. S., Xu, M. J., Ma, Z. P., Fang, X. F., et al. (2021). The dynamics of conspecific tree and seedling neighbors on seedling survival in a subtropical forest. For. Ecol. Manag. 483:118924. doi: 10.1016/j.foreco.2021.118924
Liu, Y., Zhang, G. H., Luo, X. Z., Hou, E. Q., Zheng, M. H., Zhang, L. L., et al. (2021). Mycorrhizal fungi and phosphatase involvement in rhizosphere phosphorus transformations improves plant nutrition during subtropical forest succession. Soil Biol. Biochem. 153:108099. doi: 10.1016/j.soilbio.2020.108099
Luo, S., Phillips, R. P., Jo, I., Fei, S. L., Liang, J. J., Schmid, B., et al. (2023). Higher productivity in forests with mixed mycorrhizal strategies. Nat. Commun. 14:1377. doi: 10.1038/s41467-023-36888-0
Mangan, S. A., Schnitzer, S. A., Herre, E. A., Mack, K. M. L., Valencia, M. C., Sanchez, E. I., et al. (2010). Negative plant-soil feedback predicts tree-species relative abundance in a tropical forest. Nature 466, 752–755. doi: 10.1038/nature09273
Mao, Z. K., Corrales, A., Zhu, K., Yuan, Z. Q., Lin, F., Ye, J., et al. (2019). Tree mycorrhizal associations mediate soil fertility effects on forest community structure in a temperate forest. New Phytol. 223, 475–486. doi: 10.1111/nph.15742
Marsaglia, G., Tsang, W. W., and Wang, J. (2003). Evaluating Kolmogorov’s distribution. J. Stat. Soft. 8, 1–4. doi: 10.18637/jss.v008.i18
McDowell, N. G., Allen, C. D., Anderson-Teixeira, K., Aukema, B. H., Bond-Lamberty, B., Chini, L., et al. (2020). Pervasive shifts in forest dynamics in a changing world. Science 368:eaaz9463. doi: 10.1126/science.aaz9463
Muscarella, R., and Uriarte, M. (2016). Do community-weighted mean functional traits reflect optimal strategies? Proc. R. Soc. B 283:20152434. doi: 10.1098/rspb.2015.2434
Onoda, Y., Westoby, M., Adler, P. B., Choong, A. M. F., Clissold, F. J., Cornelissen, J. H. C., et al. (2011). Global patterns of leaf mechanical properties. Ecol. Lett. 14, 301–312. doi: 10.1111/j.1461-0248.2010.01582.x
Pan, S., Wang, Y., Qiu, Y. P., Chen, D., Zhang, L., Ye, C. L., et al. (2020). Nitrogen-induced acidification, not N-nutrient, dominates suppressive N effects on arbuscular mycorrhizal fungi. Glob. Chang. Bio. 26, 6568–6580. doi: 10.1111/gcb.15311
Paradis, E., and Schliep, K. (2019). Ape 5.0: an environment for modern phylogenetics and evolutionary analyses in R. Bioinformatics 35, 526–528. doi: 10.1093/bioinformatics/bty633
Patterson, A., Flores-Rentería, L., Whipple, A., Whitham, T., and Gehring, C. (2019). Common garden experiments disentangle plant genetic and environmental contributions to ectomycorrhizal fungal community structure. New Phytol. 221, 493–502. doi: 10.1111/nph.15352
Pebesma, E. J. (2004). Multivariable geostatistics in S: the gstat package. Comput. Geosci. 30, 683–691. doi: 10.1016/j.cageo.2004.03.012
Quast, C., Pruesse, E., Yilmaz, P., Gerken, J., Schweer, T., Yarza, P., et al. (2013). The SILVA ribosomal RNA gene database project: improved data processing and web-based tools. Nucleic Acids Res. 41, D590–D596. doi: 10.1093/nar/gks1219
Rosseel, Y. (2012). Lavaan: an R package for structural equation modeling. J. Stat. Soft. 48, 1–36. doi: 10.18637/jss.v048.i02
Ryberg, M., and Matheny, P. B. (2012). Asynchronous origins of ectomycorrhizal clades of Agaricales. Proc. R. Soc. B 279, 2003–2011. doi: 10.1098/rspb.2011.2428
Schroeder, J. W., Dobson, A., Mangan, S. A., Petticord, D. F., and Herre, E. A. (2020). Mutualist and pathogen traits interact to affect plant community structure in a spatially explicit model. Nat. Commun. 11:2204. doi: 10.1038/s41467-020-16047-5
Segnitz, R. M., Russo, S. E., Davies, S. J., and Peay, K. G. (2020). Ectomycorrhizal fungi drive positive phylogenetic plant–soil feedbacks in a regionally dominant tropical plant family. Ecology 101:e03083. doi: 10.1002/ecy.3083
Soudzilovskaia, N. A., Douma, J. C., Akhmetzhanova, A. A., van Bodegom, P. M., Cornwell, W. K., Moens, E. J., et al. (2015). Global patterns of plant root colonization intensity by mycorrhizal fungi explained by climate and soil chemistry. Glob. Ecol. Biogeogr. 24, 371–382. doi: 10.1111/geb.12272
Soudzilovskaia, N. A., Vaessen, S., Barcelo, M., He, J., Rahimlou, S., Abarenkov, K., et al. (2020). FungalRoot: global online database of plant mycorrhizal associations. New Phytol. 227, 955–966. doi: 10.1111/nph.16569
Soudzilovskaia, N. A., van Bodegom, P. M., Terrer, C., Zelfde, M., McCallum, I., Luke McCormack, M., et al. (2019). Global mycorrhizal plant distribution linked to terrestrial carbon stocks. Nat. Commun. 10:5077. doi: 10.1038/s41467-019-13019-2
Spellerberg, I. F. (2008). “Shannon–Wiener Index” in Encyclopedia of ecology. eds. S. E. Jørgensen and B. D. Fath (Amsterdam, Netherlands: Elsevier B.V), 3249–3252.
Tedersoo, L., Bahram, M., and Zobel, M. (2020). How mycorrhizal associations drive plant population and community biology. Science 367:eaba1223. doi: 10.1126/science.aba1223
Wang, Y. H., Li, S. F., Lang, X. D., Huang, X. B., Liu, W. D., Xu, C. H., et al. (2020). Effects of topographic heterogeneity on species diversity in a monsoon evergreen broad-leaved forest in Puʼer, Yunnan, China. Chinese J. Plant Ecol. 44, 1015–1027. doi: 10.17521/cjpe.2020.0148
Wang, Y. H., Li, S. F., Lang, X. D., Huang, X. B., and Su, J. R. (2022). Effects of microtopography on soil fungal community diversity, composition, and assembly in a subtropical monsoon evergreen broadleaf forest of Southwest China. Catena (Amst) 211:106025. doi: 10.1016/j.catena.2022.106025
Wasyliw, J., and Karst, J. (2020). Shifts in ectomycorrhizal exploration types parallel leaf and fine root area with forest age. J. Ecol. 108, 2270–2282. doi: 10.1111/1365-2745.13484
Williams, P. J., Ong, R. C., Brodie, J. F., and Luskin, M. S. (2021). Fungi and insects compensate for lost vertebrate seed predation in an experimentally defaunated tropical forest. Nat. Commun. 12:1650. doi: 10.1038/s41467-021-21978-8
Wills, J., Herbohn, J., Wells, J., Maranguit Moreno, M. O., Ferraren, A., and Firn, J. (2021). Seedling diversity in actively and passively restored tropical forest understories. Ecol. Appl. 31:e02286. doi: 10.1002/eap.2286
Worthy, S. J., Laughlin, D. C., Zambrano, J., Umaña, M. N., Zhang, C. C., Lin, L. X., et al. (2020). Alternative designs and tropical tree seedling growth performance landscapes. Ecology 101:e03007. doi: 10.1002/ecy.3007
Xu, Y. Z., Wan, D., Xiao, Z. Q., Wu, H., and Jiang, M. X. (2019). Spatio-temporal dynamics of seedling communities are determined by seed input and habitat filtering in a subtropical montane forest. For. Ecol. Manag. 449:117475. doi: 10.1016/j.foreco.2019.117475
Yu, X. Q., Gao, L. M., Soltis, D. E., Soltis, P. S., Yang, J. B., Fang, L., et al. (2017). Insights into the historical assembly of east Asian subtropical evergreen broadleaved forests revealed by the temporal history of the tea family. New Phytol. 215, 1235–1248. doi: 10.1111/nph.14683
Yu, M., and Sun, O. J. (2013). Effects of forest patch type and site on herb-layer vegetation in a temperate forest ecosystem. For. Ecol. Manag. 300, 14–20. doi: 10.1016/j.foreco.2012.12.039
Yuan, Z. Q., Ali, A., Ruiz-Benito, P., Jucker, T., Mori, A. S., Wang, S. P., et al. (2020). Above- and below-ground biodiversity jointly regulate temperate forest multifunctionality along a local-scale environmental gradient. J. Ecol. 108, 2012–2024. doi: 10.1111/1365-2745.13378
Zhang, Y., and Chen, H. Y. H. (2015). Individual size inequality links forest diversity and above-ground biomass. J. Ecol. 103, 1245–1252. doi: 10.1111/1365-2745.12425
Zhong, Y., Chu, C., Myers, J. A., Gilbert, G. S., Lutz, J. A., Stillhard, J., et al. (2021). Arbuscular mycorrhizal trees influence the latitudinal beta-diversity gradient of tree communities in forests worldwide. Nat. Commun. 12:3137. doi: 10.1038/s41467-021-23236-3
Zhu, H., Zhang, R., Chen, W., Gu, Z., Xie, X., Zhao, H., et al. (2015). The possible involvement of salicylic acid and hydrogen peroxide in the systemic promotion of phenolic biosynthesis in clover roots colonized by arbuscular mycorrhizal fungus. J. Plant Physiol. 178, 27–34. doi: 10.1016/j.jplph.2015.01.016
Keywords: mycorrhizal strategy, seedling diversity, community assembly, habitat, subtropical forest
Citation: Chen Z, Liu W, Lang X, Wang M, Liu J and Xu C (2024) Arbuscular mycorrhizal and ectomycorrhizal plants together shape seedling diversity in a subtropical forest. Front. For. Glob. Change. 6:1304897. doi: 10.3389/ffgc.2023.1304897
Received: 30 September 2023; Accepted: 26 December 2023;
Published: 11 January 2024.
Edited by:
Azade Deljouei, University of Florida, United StatesReviewed by:
Shuai Ouyang, Central South University of Forestry and Technology, ChinaCopyright © 2024 Chen, Liu, Lang, Wang, Liu and Xu. This is an open-access article distributed under the terms of the Creative Commons Attribution License (CC BY). The use, distribution or reproduction in other forums is permitted, provided the original author(s) and the copyright owner(s) are credited and that the original publication in this journal is cited, in accordance with accepted academic practice. No use, distribution or reproduction is permitted which does not comply with these terms.
*Correspondence: Wande Liu, bGl1d2FuZGVAMTI2LmNvbQ==
Disclaimer: All claims expressed in this article are solely those of the authors and do not necessarily represent those of their affiliated organizations, or those of the publisher, the editors and the reviewers. Any product that may be evaluated in this article or claim that may be made by its manufacturer is not guaranteed or endorsed by the publisher.
Research integrity at Frontiers
Learn more about the work of our research integrity team to safeguard the quality of each article we publish.