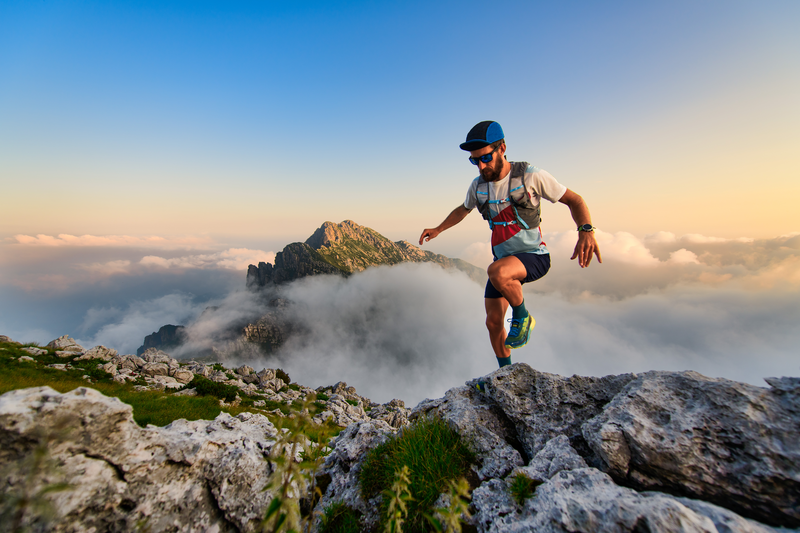
95% of researchers rate our articles as excellent or good
Learn more about the work of our research integrity team to safeguard the quality of each article we publish.
Find out more
ORIGINAL RESEARCH article
Front. For. Glob. Change , 27 October 2023
Sec. Forest Ecophysiology
Volume 6 - 2023 | https://doi.org/10.3389/ffgc.2023.1303886
Global change is affecting the frequency and temporal distribution of frost events. We can expect damage caused by low temperatures to increase specially at the upper limit of a species’ elevation range. Here, we assessed how Pinus canariensis two-year old seedlings from different islands and elevations responded differently to chilling and freezing temperatures. Seedlings from six populations were grown either in a greenhouse (tmin = 5 ̊C, chilling conditions) or outdoors (tmin = −3.3 ̊C, freezing conditions) for 104 days in winter. Seedlings from one of these populations belonged to three half-sib families. Maximum quantum yield of Photosystem II and evapotranspiration (ETP) were determined four times throughout the study. All populations suffered photoinhibition under freezing but not under chilling conditions. We measured the lowest ETP in the population from the warmest and driest environment and lowest elevation (250 m) both under chilling and freezing conditions. The three populations from the higher elevations reached the highest ETP under chilling, a likely adaptation to the shortening of the vegetative period. The effect of elevation remained elusive for other parameters, which could be partly attributed to high intrapopulation variability. Epicuticular wax concentration increased and needle water content decreased in plants growing outdoors. All populations except the one most affected by cold dehydration showed osmotic adjustment and a simultaneous decrease in tissue elasticity under freezing. This response was stronger in the population from the highest elevation (2,241 m). Our results highlight the coexistence of adaptive processes, genetic variation and plasticity conditioning the response to low temperatures in this species.
The Canary Island archipelago, located between 27° and 29° N and 13° and 18° W, is a natural laboratory for studying plant physiology and environmental plant responses and adaptations, due to the strong climate variability across each island. Dry, temperate, and cold climates can be found in the archipelago. Moreover, all these climates coexist in transect distances as short as 14 km in the island of Tenerife (AEMET-IM, 2012). This is caused by the mixed effect of the wide altitudinal range of these volcanic islands (0–3,715 m above sea level [a.s.l.]) and the influence of the humid trade winds, both triggering an altitudinal-belt structured flora (Aguilar et al., 2010). Northeastern trade winds influence the windward slopes, increasing air humidity that may condense below the inversion layer forming stratocumulus, locally called ‘sea of clouds’ (Máyer and Marzol, 2013; Ritter et al., 2019).
Among the flora species in the Canary Islands, Pinus canariensis Chr. Sm. ex DC. is the main tree species (10.45% of the archipelago surface) spreading over several flora altitudinal-belts on both windward and leeward slopes (200 to 2,200 m a.s.l.), with precipitation ranging from 300 to 3,000 mm year−1 and annual average temperatures from 10 to 20°C (Pérez-de-Paz et al., 1994; Climent et al., 1996). Therefore, P. canariensis represents an excellent case study species to broaden our understanding of tree physiological responses and adaptations to drought (López et al., 2013), altitudinal climate-gradients (Weigel et al., 2018; Miranda et al., 2021), volcanic eruptions (Rodríguez Martín et al., 2013; López de Heredia et al., 2014; Miranda et al., 2020), and wildfires (Rozas et al., 2013). Some previous studies highlight the plasticity of this species. For instance, López et al. (2013) and López de Heredia et al. (2014) studied hydraulic traits and adaptation to aridity, concluding that the ability of this species to inhabit a wide climatic range was associated with high phenotypic plasticity and to some degree to local adaptations.
Low temperatures are a limiting factor for Canary Island pine seedlings (Climent et al., 1996). Pinus canariensis tree-line populations held an average of 22.6 days with frost events a year, whereas the minimum recorded temperature in mid-altitude areas (632 m a.s.l.) within the species distribution is 4.0°C (AEMET-IM, 2012). There are some studies assessing Canary Island pine responses to chilling and freezing temperatures (Peters et al., 1999; Luis et al., 2007; González-Rodríguez et al., 2019; Fernández-Marín et al., 2021). However, none of those studies has taken advantage of the genetic variability across populations. Inter-population genetic variation could lead to phenotypic plasticity also in terms of chilling and frost endurance.
Chilling (0–15°C) and freezing (<0°C) temperatures can cause several dysfunctions in tree organs (Lambers and Oliveira, 2019). Compared to deciduous species, in evergreen plants such as Canary Island pine the photosynthetic tissues are exposed to low winter temperatures. Suboptimal chilling temperatures slow down enzymatic processes causing a winter depression of photosynthetic function (Larcher, 2000). Consequently, an excess of absorbed energy needs to be dissipated to avoid damages to photosystem II (PSII; Takahashi and Badger, 2011) during winter sunny days. Leaf chlorophyll fluorescence is widely accepted as a sensitive indicator of several kinds of plant stress, such as flooding (Pita et al., 2023), heat (Sharma et al., 2015), stem girdling (López et al., 2015) or pathogens (Castillo-Argaez et al., 2020), and has proven particularly worthy to analyze the effect of low-temperature stress (Fracheboud et al., 1999; Ogaya et al., 2011). Among the photo-protective mechanisms of leaves, epicuticular waxes have been proposed to modulate the absorption of photosynthetically active radiation, thus protecting against photoinhibition (Robinson et al., 1993; Shepherd and Griffiths, 2006; Mohammadian et al., 2007). Epicuticular wax deposition and its photo-protective function vary seasonally in pines (Olascoaga et al., 2014) and may also present variation between populations. In this regard, differences in epicuticular wax topography have been described for P. canariensis needles, with ω-hydroxy-n-alkanoic acids being the most abundant wax kind followed by 10-nonacosanol and n-alkanoic acids (Stabentheiner et al., 2004). Leaf epicuticular wax topography may influence the process of ice formation and prevent the direct contact of ice crystals with the underlying cuticle (Gorb and Gorb, 2022).
Under freezing temperatures, sharp ice crystals can cause mechanical damage to cells and tissues. In addition, extracellular ice formation promotes symplast dehydration, which may cause cell death (Larcher, 2005). Beside anatomical and phenological adaptations, plant freezing resistance depends on minimizing dehydration damages derived from ice formation in the apoplast by means of increasing the concentration of protective compounds, such as proteins and sugars (Gusta and Wisniewski, 2013; Körner, 2016) and therefore decreasing tissue osmotic potential. On the other hand, cell wall stiffening increases cells’ resistance to collapse (Scholz et al., 2012) and could therefore improve tolerance to dehydration during extracellular freezing (Zhang et al., 2016). Moreover, the extent of freeze-dehydration in the mesophyll can be reduced by cell wall rigidity, as reported in three conifer species adapted to low temperatures (Stegner et al., 2022).
The aim of this study was to analyze the response to freezing and chilling temperatures in P. canariensis seedlings from different populations, including one at the species’ upper elevation range. We hypothesized that: (1) seedlings will show both inter and intrapopulation variability in terms of their physiological responses to low temperatures (2) populations from higher elevations would be less affected by low temperatures; and (3) tissue osmotic potential and elasticity would decrease under freezing conditions while needle wax concentration would increase.
The experiment was carried out at the facilities of the School of Forest Engineering and Natural Resources, Universidad Politécnica de Madrid, Spain. Two-year old Pinus canariensis seedlings were grown in five-liter pots filled with a mixture of weakly fertilized fine peat moss and perlite (3:2 v:v). Seedlings were obtained from seed collected from six different populations in the Canary Islands: Arguineguín, Colada, Fayal, Hierro, Taburiente and Teide, differing in altitude, rainfall and thermal conditions (Table 1; Supplementary Figure 1). In the case of Arguineguín, seed was collected separately from three different trees, therefore constituting three half-sib families: A54, A79 and A80. For simplicity, the rest of populations will be named by their initials in some figures, except Taburiente (Tab). The plants never experienced chilling temperatures before the study.
Plants were kept in a greenhouse under natural sunlight and watered once/twice a week from September 20th to November 16th. On October 11th all plants were fertilized with 0.37 g of Peters® 20:20:20. On November 16th (d1) four plants per group except Taburiente with three were moved outdoors (outdoor plants – freezing temperatures) and placed under the same light conditions as the 3–6 plants per group kept inside the greenhouse (greenhouse plants – chilling temperatures) until the end of the experiment on February 27th (d104). The pots of the outdoor plants were placed in containers filled with paper balls to minimize the effect of low temperatures on their root systems. Top-soil temperature (~3 cm depth) was measured in outdoor plants eight times throughout the experiment, being always above 1°C. Air temperature was continuously recorded by means of Lambrecht thermo-hygrographs (Lambrecht Meteo GmbH, Göttingen, Germany). Maximum and minimum air temperatures were also measured with max min thermometers. Minimum temperatures remained above 5°C inside the greenhouse and fell below 0°C several times outdoors (Figure 1). Light snow fell on d82. Plants were watered once/twice a week, and soil water content remained between 10 and 40% both for inside and outdoor plants, as determined by time domain reflectometry (TRIME TDR IMKO Gmbh, Ettlingen, Germany).
Figure 1. Maximum (solid symbols) and minimum (empty symbols) temperatures measured inside (triangles) and outside (circles) the greenhouse throughout the experiment. Vertical dashed lines signal the days when chlorophyll fluorescence was measured. The thick vertical line signals needle collection for determining wax concentration. Dotted lines show the start and end of pressure-volume curves construction.
We calculated daily evapotranspiration (ETP) by weighing the potted plants at 24-h time intervals on four occasions throughout the experiment (d12, d35, d64, d85). These measurements were carried out along two or three consecutive rain-free days.
Maximum quantum yield of Photosystem II (Fv/Fm) was measured with a Hansatech FMS2 fluorometer (Hansatech Instruments Ltd., Norfolk, England) in a portion of needles that was previously kept in the dark for a minimum of 20 min. Plants were measured in batches, with one plant from each seed source in each batch and alternating batches of greenhouse and outdoor plants, between 9:00 and 11:00 (GMT + 1). Measurements were carried out on d18, d36, d65 and d86. All outdoor plants and a sample of 2–4 greenhouse plants per seed source were measured on every occasion except on d18, when greenhouse plants were not measured.
On d77 juvenile needles were sampled from 12 plants belonging to the populations Teide, Fayal and Arguineguín (A54) to analyze the amount of epicuticular waxes (soluble cuticular lipids). For this purpose, 130–170 needles were collected per population, measuring the corresponding fresh weight (FW) of the samples which varied between 2.5 and 4 g. Needle soluble cuticular lipids (waxes) were extracted by immersing them in 100 mL of chloroform for 1 min, using two replicates per sample. Extracts were first evaporated in a glass beaker and then in a watch glass until dryness in a laboratory fume cupboard. The amount of soluble cuticular lipids was expressed gravimetrically on a leaf dry weight (DW) basis, after having determined the FW to DW ratio for each provenance. For analyzing surface topography, healthy leaves were selected and approximately 1 cm long sections were cut from the central needle part with a scalpel. Needle sections were then placed in the stands for gold-sputtering (Q150T-S, Quorum Technologies, West Sussex, United Kingdom) prior to observation with a variable-pressure scanning electron microscope (SEM, Hitachi S-3400 N, Tokyo, Japan; acceleration potential, 20 kV; working distance, 15–17 mm).
From d90 to d98 pressure-volume (P-V) curves were established using the free transpiration method (Hinckley et al., 1980; Dreyer et al., 1990). We used one basal shoot per plant to construct the curves (n = 3–4 plants per population or family and growing location). Once excised from the plant, the shoots were placed with the base immersed in water and kept in the dark for 3–4 h. This was enough to fully rehydrate the tissue with no significant sign of infiltration. Shoot water potential (Ψ) was measured with a Scholander-type pressure chamber (PMS Instrument Co., Albany, OR, United States). Relative water deficit (RWD) was calculated as RWD = (SW-FW) (SW-DW)−1, where SW, FW and DW stand for saturated, fresh and dry weight of each shoot. Relative water content was calculated as RWC = 1-RWD. On average, 7 ± 0.2 pair of data (RWD, Ψ) were used to draw each curve.
We fitted the linear portion of each curve by linear regression and used the equations to calculate osmotic potential at full turgor (Ψs100) for each plant. The average osmotic adjustment was calculated afterwards as the difference between the average value of Ψs100 measured in plants growing inside the greenhouse and outdoors. Then, we pooled together the values of RWD and Ψ measured in all plants from each seed source × growing location and fitted these data to logarithmic equations to obtain the average P-V curve for each seed source × growing location: . Similarly, we fitted data measured beyond turgor loss to linear functions: . We also included the pair of values (0, −Ψav−1) to adjust the linear regression, where Ψav−1 was calculated as the average value of Ψs100−1 measured in greenhouse and outdoor plants for each population. We used these equations to calculate the average values of Ψ and Ψs and RWC at Ψ = -1 MPa. We chose Ψ = -1 MPa as a reference value because it was slightly higher than the average water potential at turgor loss. We calculated pressure potential (Ψp) as for all the values of RWD measured before reaching turgor loss and then plotted Ψp against RWD. We fitted the pair of values (RWD, Ψp) to second grade polynomials and calculated the maximum modulus of elasticity (εmax) as the initial slope of the curve (Stegner et al., 2022).
On d104 all plants were harvested, all needles were pulled out from the stem and weighed (FW). Needle dry weight (DW) was determined after oven-drying at 60°C for 48 h and re-weighing. Needle water content (NWC) was calculated on a dry weight basis as NWC = (FW-DW) DW−1.
Factorial ANOVA was used to analyze the effect of the population and growing location (inside the greenhouse/outdoors) together with their interaction on needle wax concentration, needle water content and Ψs100. We could not analyze the effect of the growing location together with the date and population on Fv/Fm because the assumption of homogeneity of variances failed, so we analyzed the effect of date and population in each growing location separately, by means of factorial ANOVA.
Differences in ETP were analyzed using analysis of covariance (ANCOVA), taking population, growing location and date as categorical variables and needle biomass as continuous predictor. We further analyzed differences between populations separately for each growing location by means of one-way ANOVA (needle water content) or factorial ANCOVA (ETP).
We used one-way ANOVA to analyze the effect of the growing location on the average values of εmax and shoot RWC calculated for each population. Post-hoc comparisons were carried out by means of unequal N HSD test and the Tukey’s HSD Test. A linear regression was performed confronting the average osmotic adjustment and the percentage of increase in εmax. We used the 6.0 software version STATISTICA (Stat Soft Inc.) to carry on all analyses. All statistical comparisons were considered significantly different at p < 0.05.
Evapotranspiration was 45–85% lower in outdoor compared to greenhouse plants, differences being highly significant (F1,195 = 963.8, p < 0.001; Figure 2). The effect of the day of measurement (F1,195 = 86.8, p < 0.0001) and population (F7,195 = 2.19, p = 0.037) were also significant, as the interactions between population and growing location (F7,195 = 6.7, p < 0.001) and growing location and date (F7,195 = 28.0, p < 0.001). When analyzed separately, we found a significant effect of the population both in plants growing outdoors (F7,91 = 2.76, p = 0.012) and inside the greenhouse (F7,103 = 4.0, p < 0.001). Inside the greenhouse, ETP was highest in the population from the highest elevation (Teide). Differences between Teide and the rest of populations were significant (Unequal N HSD test) except for those populations from the second and third higher elevations (Hierro and Colada). Plants from Fayal reached the highest ETP outdoors. The lowest values were always measured in Arguineguín families (Figure 2).
Figure 2. Mean ± SE evapotranspiration measured on different days in Pinus canariensis seedlings from different seed sources growing outdoors and inside the greenhouse. The average percentage of decrease in outdoors plants compared to plants growing inside the greenhouse is given in a box (n = 3–6 plants per population or family and growing location).
Values of Fv/Fm measured in outdoor plants were lower than 0.8 in all measurements, except for A80 on d65, while values measured in greenhouse plants were always higher than 0.8 (Figure 3). The lowest Fv/Fm values were measured on d86 (Figure 3), 4 days after the snowfall and after three consecutive days of minimum temperatures falling below −1.5°C (Figure 1). We found a highly significant effect of the day of measurement on Fv/Fm values measured outdoors (F3,109 = 29.5, p < 0.0001). On the other hand, neither the effect of the population nor the interaction between population and day of measurement were significant.
Figure 3. Mean ± SE maximum quantum yield of PSII (Fv/Fm) measured on different days in P. canariensis seedlings from several seed sources growing outdoors and inside the greenhouse (n = 3–4 plants per population or family and growing location).
Values of needle epicuticular wax concentration were significantly higher in plants growing outdoors (F1,6 = 110.5, p < 0.001). The effect of the population (F2.6 = 17.97, p = 0.0029) and the interaction between population and growing location (F2,6 = 49.68, p < 0.001) were also significant. The concentration of waxes increased by 26 and 54% in outdoor plants for A54 and Teide respectively; but did not change in Fayal (Figure 4). Pine needles of the three populations sampled had rows of stomata surrounded by tubular wax crystals which may appear as bands of variable thickness (Supplementary Figures 2A,B,C) Most of the needle surfaces in areas lacking stomata were covered with amorphous waxes (Supplementary Figures 3A,B,C).
Figure 4. Soluble cuticular lipid (wax) concentrations (mg g−1 D.W.) of P. canariensis needles collected from seedlings grown outdoors or in a greenhouse during winter. Different letters indicate significant differences between means according to Tukey’s HSD test. Mean values ± SE (n = 2).
Figure 6. Pressure-volume curves obtained for several populations of P. canariensis using the free-transpiration method. Data were collected from basal shoots cut from 2-year-old seedlings growing outdoors (solid symbols) or inside a greenhouse (empty symbols) in winter. Dashed lines show the logarithmic and linear regressions fitted to data obtained before and after reaching the turgor loss point in plants growing outdoors. Solid lines show the logarithmic and linear regressions for plants growing inside the greenhouse. Note the different scale in the Y-axis for the population Teide (T), n = 3–4 plants per population or family and growing location.
At the end of the experiment needle water content was significantly lower in plants growing outdoors (F1,51 = 176.5, p = 0.0000). The effect of the population was not significant and there was a significant population x growing location interaction (F7,51 = 4.8, p = 0.003). When analyzed separately, we found a significant effect of the population both in plants growing outdoors (F7,23 = 3.7, p = 0.008) and inside the greenhouse (F7,28 = 3.1, p = 0.016). Differences in needle water content between outdoors and greenhouse plants were highest in Fayal (37.8%) and lowest in Teide (15.7%; Figure 5).
Figure 5. Average ± SE values of needle water content calculated on a dry weight basis (NWC). Values were measured in two-year old P. canariensis seedlings from different populations growing outdoors (freezing temperatures) or inside a greenhouse (chilling temperatures) during winter. Different letters indicate significant differences between means for plants growing outdoors (upper-case) or inside the greenhouse (lower-case) according to the unequal N HSD test (n = 3–6 plants per population or family and growing location).
We found a significant effect of both the population (F7,45 = 3.76, p = 0.003) and growing location (F1,45 = 15.55, p = 0.0003) on Ψs100, with no significant interaction. The average degree of osmotic adjustment was highest in Teide and lowest in A79 (Table 2, Figure 6). Plants from Fayal showed an atypical response, by reaching higher values of Ψs100 outdoors than inside the greenhouse (Table 2, Figure 6). Therefore, plants from Fayal showed no osmotic adjustment at all in response to decreasing temperatures.
Table 2. Average ± SE values of osmotic potential at full turgor (Ψs100, MPa) measured in P. canariensis seedlings growing inside the greenhouse (IN) and outdoors (OUT) and the average degree of osmotic adjustment (OA) calculated as the difference between them for each seed source (n = 3–4 data per population or family and growing location).
There was a significant effect of the growing location on εmax values averaged across populations (F1,14 = 13.4, p = 0.003). Differences were highest in Teide, in which the average εmax was 285% higher for plants growing outdoors compared to those inside the greenhouse. Fayal was the only population in which εmax did not increase outdoors. The three Arguineguín families showed quite different responses, ranging from the 16% increase in εmax measured in A79 to the 127% increase measured in A54 (Table 2). We found a positive, linear relationship (F1,6 = 8.59, p = 0.026) between the average degree of osmotic adjustment and the percentage increase in εmax across populations (Figure 7).
Figure 7. Relationship between the average osmotic adjustment and the increase in the maximum modulus of elasticity for P. canariensis basal shoots from different populations.
Average values of RWC calculated for a shoot water potential of −1 MPa were higher (F1,14 = 6.6, p = 0.022) in plants growing outdoors. Once more, Fayal showed an atypical response, being the only population in which RWC was higher inside the greenhouse (Table 2).
Both chilling and freezing temperatures may decrease the activity of enzymes participating in the Calvin-Benson cycle and cause damage to the chloroplast (Liu et al., 2018), therefore hampering the ability of plants to use light energy in photosynthesis and leading to photoinhibition (Close and Beadle, 2003; Martínez-Ferri et al., 2004; Corcuera et al., 2011). These effects would be particularly relevant under the high-irradiance conditions typical of low latitudes and/or high altitudes, like those found in P. canariensis native habitat. According to our results, Canary Island pine seedlings were only affected by photoinhibition when submitted to freezing temperatures, but not under chilling conditions. Interestingly, we found no significant differences between populations in Fv/Fm values, despite the striking differences in altitude and thermal conditions between Teide and the rest of seed sources. This may be partly a consequence of the higher Fv/Fm intrapopulation variability compared to the interpopulation variability. We must recall that the largest difference in Fv/Fm between Arguineguín families was higher than the largest difference between populations measured throughout the experiment. Corcuera et al. (2011) reported similar results in three Pinus pinaster provenances. In addition, values of Fv/Fm may vary considerably under photoinhibitory stress (see for example Aranda et al., 2005), making it more difficult to find differences between closely related genotypes.
Photoinhibition affects the capacity to sustain photosynthesis but may also provide photo-protection in winter (Robakowski, 2005), acting as a mechanism in the chloroplast to reduce ROS production under low temperatures (Liu et al., 2018). The onset of photoinhibition may therefore be not so much important as the ability to recover from photoinhibitory stress. In a recent study, Döweler et al. (2021) concluded that factors other than the observed photoinhibition may be the cause of establishment failure in the tree-line-forming Fuscospora cliffortioides. Similar results were reported by Oliveira and Peñuelas (2004), who measured lower Fv/Fm values but greater leaf and plant survival in Quercus ilex than in Cistus albidus under cold stress.
Evapotranspiration was drastically reduced in plants growing outdoors. This could be expected by means of the effect of temperature on vapor pressure deficit. In addition, the range of evapotranspiration values across populations strongly support a relevant role of stomata in controlling water loss under chilling and freezing temperatures in this species. In this regard, keeping comparatively high stomatal conductances during acclimation to low temperatures was suggested to improve freezing avoidance by supercooling in Olea europaea (Arias et al., 2017). Similarly, maintaining high transpiration rates at the end of the growing season would provide the pioneer Larix decidua with carbon resources for maintenance respiration and protection against freezing damage (Peters et al., 2019). In 50-yr old P. canariensis trees growing 1,650 m a.s.l. maximum daily net photosynthesis, transpiration and stomatal conductance were generally higher during the cold season as compared to the dry and hot summer (Peters et al., 2008). On the other hand, a strategy of stomatal closure would reduce the risk of runaway embolism when water absorption from the soil is hampered by low temperatures (Peguero-Pina et al., 2011).
Decreasing cuticular conductance would be particularly relevant for controlling water loss when stomatal conductance is low. However, minimum transpiration, a proxy for cuticular transpiration, was found to increase with altitude in P. uncinata (Bueno et al., 2022). Such an increase has been reported in other species and can be addressed to an inadequate development of the cuticle before freezing temperatures are reached, resulting in decreased cuticle thickness; or the extreme abrasion of the cuticle under freezing (Bueno et al., 2022). The second factor would be more relevant under temperatures lower than those reached in the present study. As for an inadequate ‘ripening’ of the cuticle caused by the shortening of the vegetative period at high altitudes, it would have a lesser effect on species that store large amounts of starch in the stem like is the case of P. canariensis, a likely consequence of evolving in a volcanic environment (Miranda et al., 2020).
Our results show a plastic response of epicuticular wax concentration to low temperatures in P canariensis needles, highlighting the dynamic nature of the leaf surface. Indeed, the surface topography of P. canariensis needles was found to change from being completely covered with nano-tubes, which proved a glaucous appearance in 1-year old primary needles to becoming smooth owing to the occurrence of amorphous epicuticular waxes in secondary needles (Stabentheiner et al., 2004). Both surface typologies could be observed in the same needles in our study.
Changes in wax concentration in response to several kinds of stress have been previously documented in herbaceous and tree species (Sánchez et al., 2001; Samdur et al., 2003; Shepherd and Griffiths, 2006; Harrington and Carlson, 2015). Increasing cuticle thickness through wax deposition could improve thermal insulation (Huggins et al., 2018), which would be relevant to restrain ice formation in the apoplast under moderate freezing temperatures and reduce the extent of cold dehydration. In agreement with this, we measured the largest decrease in needle water content in the only population in which wax concentration did not increase under freezing temperatures (Fayal). On the other hand, changes in needle surface roughness mediated by wax deposition would affect water film formation (Fernández et al., 2017), destructive ice penetration into leaf cells (Rahman et al., 2021) and leaf reflectance patterns (Huggins et al., 2018; Ustin and Jacquemoud, 2020). In this regard, needle surface SEM images showed both rough and smooth surfaces in our study. Changing this pattern would affect the amount of light scattered in all directions or reflected off the leaf surface by rough and smooth leaf surfaces, respectively, (Ustin and Jacquemoud, 2020) and thus the amount of energy that reaches the chloroplast.
Freezing temperatures decreased needle water content and lead to osmotic adjustment by decreasing Ψs100 as previously reported in several species (Thomas and James, 1993; Rinne et al., 2015; Hajihashemi et al., 2020; Wang et al., 2020). High concentration of solutes combined with less extracellular water to freeze reduce the probability of ice nucleation (Charrier et al., 2015) and contribute to cold acclimation. Both water exclusion and solute accumulation lower the freezing point and, more importantly, limit the extent of symplast desiccation due to extracellular ice formation. Interestingly, despite the significant decrease in needle water content expressed on a dry weight basis, basal shoots collected from outdoor plants had a higher relative water content than greenhouse plants in most populations. Both results are compatible with a strategy based on solute accumulation and wall stiffening, aimed at excluding water from plant tissues. Plants growing outdoors had almost all the water their needles could hold, which was less than the water held by the more elastic tissue of plants growing inside the greenhouse.
In agreement with our results, Scholz et al. (2012) found that the bulk leaf tissue elastic modulus increased during winter in cold desert shrubs. Similar results have been reported for tree species like Olea europaea (Arias et al., 2017) and Eucalyptus platypus (White et al., 2000). Osmotic and elastic adjustment are considered non-simultaneous strategies to cope with tissue dehydration under drought (Lambers and Oliveira, 2019; Ghouil et al., 2020). Both increasing the concentration of solutes and increasing cell wall elasticity can help to maintain a positive turgor pressure in a dehydrating environment. By increasing solute concentration inside the cell water can be taken from the apoplast instead of being lost from inside the cell to the apoplast, to avoid reaching the turgor loss point. However, if the cell walls are very elastic, water absorption would result in an increase in cell volume, which in turn would increase water potential and promote water moving back to the apoplast. In fact, cell wall stiffening under drought has been reported in Damask rose (Al-Yasi et al., 2020). Similarly, Leuschner et al. (2019) reported a tendency for εmax to increase from early to late summer in several broadleaf tree species. Our results show a simultaneous decrease in the osmotic potential and cell wall elasticity. The positive relationship between the increase in εmax and the osmotic adjustment suggests that both mechanisms may act synergistically to improve tolerance to freezing dehydration.
We found significant differences between P. canariensis populations in evapotranspiration, needle water content, Ψs100 and the content of epicuticular waxes. Interestingly, the response to chilling and freezing temperatures differed between populations, as shown by the interactions between population and growing location found in three of these parameters. These results show some degree of adaptation to low temperatures, in agreement with the results reported by López et al. (2013) for xylem and leaf traits. The extensive, long-distance gene flow widely documented in the species (Navascués et al., 2006; Vaxevanidou et al., 2006; Navascués and Emerson, 2007; López de Heredia et al., 2014) can promote adaptive evolution by increasing genetic variation and enhancing plastic responses (López et al., 2013) even in the harshest environments. The extreme aridity of their natural habitat did not prevent Arguineguín families to show different degrees of plasticity in response to low temperatures in our study. Though populations from extreme environments tend to show lower levels of plasticity than those that evolve in variable environments, the response to extreme conditions would depend on genetic variation (Chevin and Hoffmann, 2017).
Among the populations tested, Fayal showed the lowest plasticity in the parameters derived from the P-V curves, together with some atypical responses. Interestingly, this population belongs to the same ecological region than the only population that showed no plasticity in the vulnerability to cavitation in a previous study (López et al., 2013). These results could be related to a stronger influence of the humid trade winds, being Fayal the most septentrional of the populations tested, located in a north-eastern facing slope and close to the sea. The abundance of clouds, typical of such locations, decreases the temperature range and needle transpiration, which would affect the adaptive response to low temperatures and needle dehydration.
Evapotranspiration under chilling conditions was the only parameter that grouped the three populations from the highest elevations. This response was particularly relevant in Teide, most probably as an adaptation to the rather low maximum temperatures and high radiation experienced at such high altitudes throughout a significant part of the year. Keeping the stomata opened may allow to maintain some photosynthesis and provide the plant with carbohydrates that may be used for storage or to protect cells and tissues from chilling and freezing damage. This effect would be reinforced by the displacement of the optimum temperature for photosynthesis toward lower temperatures during the cold season, as was reported for P. canariensis trees growing at high elevations (Peters et al., 2008). We measured the lowest evapotranspiration in the warmest population tested (Arguineguín), both under chilling and freezing conditions, supporting the stronger impact of chilling and freezing temperatures on plant metabolism previously reported in plants adapted to limiting environments (Smith et al., 2014). The effect of elevation remained elusive for the rest of parameters tested, which could be partially explained by the high microclimatic variability that characterizes the Canary Islands.
Our results show that freezing and chilling temperatures elicit heterogeneous plastic responses in P. canariensis seedlings from populations covering the elevation range of the species and contrasting climates. We measured the lowest ETP in the population from an extremely arid environment located at the lowest elevation both under chilling and freezing temperatures. Plants from the highest elevations kept a higher ETP under chilling, which probably is an adaptation to the shortening of the vegetative period with increasing altitude. Moderately cold temperatures (above −3.5°C) caused photoinhibition, with no significant differences in Fv/Fm values between populations despite the disparity of responses in terms of needle wax concentration, osmotic adjustment and tissue elasticity, both between and within populations. Lack of plasticity in the aforementioned traits contributes to explain the highest impact of cold dehydration measured in a population from an intermediate altitude.
The datasets presented in this article are not readily available because the datasets generated during and/or analyzed during the current study are available from the corresponding author on reasonable request. Requests to access the datasets should be directed to PP, cGlsYXIucGl0YUB1cG0uZXM=.
JM: Funding acquisition, Validation, Writing – original draft, Writing – review & editing. UL: Data curation, Funding acquisition, Validation, Writing – review & editing. VF: Data curation, Validation, Writing – review & editing. PP: Conceptualization, Data curation, Formal analysis, Funding acquisition, Supervision, Validation, Writing – original draft, Writing – review & editing.
The author(s) declare financial support was received for the research, authorship, and/or publication of this article. This work has been funded through the Project SPIP2014-01093 (OAPN, Spanish Ministry of Ecological Transition). JM was supported by the Maria Zambrano fellowship (reference: RCMZ-21-DJVHZ3-7-90M8PD) from the Spanish Ministry of Universities with European Union-Next Generation EU funds.
We are grateful to the Canary Islands Government and the Cabildos of Tenerife and Gran Canaria for long standing support in the study of Canary Island pine.
The authors declare that the research was conducted in the absence of any commercial or financial relationships that could be construed as a potential conflict of interest.
The author(s) declared that they were an editorial board member of Frontiers, at the time of submission. This had no impact on the peer review process and the final decision.
All claims expressed in this article are solely those of the authors and do not necessarily represent those of their affiliated organizations, or those of the publisher, the editors and the reviewers. Any product that may be evaluated in this article, or claim that may be made by its manufacturer, is not guaranteed or endorsed by the publisher.
The Supplementary material for this article can be found online at: https://www.frontiersin.org/articles/10.3389/ffgc.2023.1303886/full#supplementary-material
AEMET-IM (2012). Atlas climático de los archipiélagos de Canarias, Madeira y Azores. Agencia estatal de meteorología (MAGAMA) e Instituto de meteorologia de Portugal :80. doi: 10.31978/281-12-006-X
Aguilar, M. J. A., González-González, R., Garzón-Machado, V., and Pizarro-Hernández, B. (2010). Actual and potential natural vegetation on the Canary Islands and its conservation status. Biodivers. Conserv. 19, 3089–3140. doi: 10.1007/s10531-010-9881-2
Al-Yasi, H., Attia, H., Alamer, K., Hassan, F., Ali, E., Elshazly, S., et al. (2020). Impact of drought on growth, photosynthesis, osmotic adjustment, and cell wall elasticity in damask rose. Plant Physiol. Biochem. 150, 133–139. doi: 10.1016/j.plaphy.2020.02.038
Aranda, I., Castro, L., Alía, R., Pardos, J. A., and Gil, L. (2005). Low temperature during winter elicits differential responses among populations of the Mediterranean evergreen cork oak (Quercus suber). Tree Physiol. 25, 1085–1090. doi: 10.1093/treephys/25.8.1085
Arias, N. S., Scholz, F. G., Goldstein, G., and Bucci, S. J. (2017). The cost of avoiding freezing in stems: trade-off between xylem resistance to cavitation and supercooling capacity in woody plants. Tree Physiol. 37, 1251–1262. doi: 10.1093/treephys/tpx071
Bueno, A., Alonso-Forn, D., Peguero-Pina, J. J., de Souza, A. X., Ferrio, J. P., Sancho-Knapik, D., et al. (2022). Minimum leaf conductance (g(min)) is higher in the Treeline of Pinus uncinata ram. In the Pyrenees: Michaelis' hypothesis revisited. Front. Plant Sci. 12:8. doi: 10.3389/fpls.2021.786933
Castillo-Argaez, R., Schaffer, B., Vazquez, A., and Sternberg, L. (2020). Leaf gas exchange and stable carbon isotope composition of redbay and avocado trees in response to laurel wilt or drought stress. Environ. Exp. Bot. 171:103948. doi: 10.1016/j.envexpbot.2019.103948
Charrier, G., Ngao, J., Saudreau, M., and Améglio, T. (2015). Effects of environmental factors and management practices on microclimate, winter physiology, and frost resistance in trees. Front. Plant Sci. 6:259. doi: 10.3389/fpls.2015.00259
Chevin, L. M., and Hoffmann, A. A. (2017). Evolution of phenotypic plasticity in extreme environments. Philos. Trans. Royal Society B-Biolog. Sci. 372:20160138. doi: 10.1098/rstb.2016.0138
Climent, J., Gil, L., and Tuero, M. (1996). Las regiones de procedencia de Pinus canariensis Chr Sm. ex DC. ICONA, Madrid. 49 p.
Close, D. C., and Beadle, C. L. (2003). Chilling-dependent photoinhibition, nutrition and growth analysis of Eucalyptus nitens seedlings during establishment. Tree Physiol. 23, 217–226. doi: 10.1093/treephys/23.4.217
Corcuera, L., Gil-Pelegrín, E., and Notivol, E. (2011). Intraspecific variation in Pinus Pinaster PSII photochemical efficiency in response to winter stress and freezing temperatures. PLoS One 6:e28772. doi: 10.1371/journal.pone.0028772
del Arco, M.J., Wildpret, W., Pérez, P.L., Rodríguez, O., Acebes, J.R., García, A., et al. (2006). Mapa de vegetaciónn de Canarias. GRAFCAN, Santa Cruz de Tenerife.
Döweler, F., Case, B. S., Buckley, H. L., and Bader, M. K. F. (2021). High light-induced photoinhibition is not limiting seedling establishment at abrupt treeline ecotones in New Zealand. Tree Physiol. 41, 2034–2045. doi: 10.1093/treephys/tpab061
Dreyer, E., Bousquet, F., and Ducrey, M. (1990). Use of pressure volume curves in water relation analysis on woody shoots: influence of rehydration and comparison of 4 European oak species. Annales Des Sci. Forestieres. 47, 285–297. doi: 10.1051/forest:19900401
Fernández, V., Bahamonde, H. A., Peguero-Pina, J. J., Gil-Pelegrín, E., Sancho-Knapik, D., Gil, L., et al. (2017). Physico-chemical properties of plant cuticles and their functional and ecological significance. J. Exp. Bot. 68, 5293–5306. doi: 10.1093/jxb/erx302
Fernández-Marín, B., Ruiz-Medina, M. A., Miranda, J. C., and González-Rodríguez, A. M. (2021). Coexistent Heteroblastic needles of adult Pinus canariensis c.Sm. Ex DC. In Buch trees differ structurally and physiologically. Forests 12:341. doi: 10.3390/f12030341
Fracheboud, Y., Haldimann, P., Leipner, J., and Stamp, P. (1999). Chlorophyll fluorescence as a selection tool for cold tolerance of photosynthesis in maize (Zea mays L.). J. Exp. Bot. 50, 1533–1540. doi: 10.1093/jxb/50.338.1533
Ghouil, H., Sancho-Knapik, D., Ben, A., Amimi, N., Ammari, Y., Escribano, R., et al. (2020). Southeastern rear edge populations of Quercus suber L. showed two alternative strategies to cope with water stress. Forests 11:11. doi: 10.3390/f11121344
González-Rodríguez, A. M., Brito, P., Lorenzo, J. R., and Jiménez, M. S. (2019). Photosynthetic performance in Pinus canariensis at semiarid Treeline: phenotype variability to cope with stressful environment. Forests 10:845. doi: 10.3390/f10100845
Gorb, S. N., and Gorb, E. V. (2022). Anti-icing strategies of plant surfaces: the ice formation on leaves visualized by Cryo-SEM experiments. Sci. Nature 109:24. doi: 10.1007/s00114-022-01789-7
Gusta, L. V., and Wisniewski, M. (2013). Understanding plant cold hardiness: an opinion. Physiol. Plant. 147, 4–14. doi: 10.1111/j.1399-3054.2012.01611.x
Hajihashemi, S., Brestic, M., Landi, M., and Skalicky, M. (2020). Resistance of Fritillaria imperialis to freezing stress through gene expression, osmotic adjustment and antioxidants. Sci. Rep. 10:10427. doi: 10.1038/s41598-020-63006-7
Harrington, C. A., and Carlson, W. C. (2015). Morphology and accumulation of Epicuticular wax on needles of Douglas-fir (Pseudotsuga menziesii var. menziesii). Northwest Sci. 89, 401–408. doi: 10.3955/046.089.0409
Hinckley, T. M., Duhme, F., Hinckley, A. R., and Richter, H. (1980). Water relations of drought hardy shrubs: osmotic potential and stomatal reactivity. Plant Cell Environ. 3, 131–140.
Huggins, T. D., Mohammed, S., Sengodon, P., Ibrahim, A. M. H., Tilley, M., and Hays, D. B. (2018). Changes in leaf epicuticular wax load and its effect on leaf temperature and physiological traits in wheat cultivars (Triticum aestivum L.) exposed to high temperatures during anthesis. J. Agron. Crop Sci. 204, 49–61. doi: 10.1111/jac.12227
Körner, C. (2016). Plant adaptation to cold climates. F1000Research. 5:2769. doi: 10.12688/f1000research.9107.1
Lambers, H., and Oliveira, R.S. (2019). Plant physiological ecology, 3rd Springer, Cham, Switzerland.
Larcher, W. (2000). Temperature stress and survival ability of Mediterranean sclerophyllous plants. Plant Biosystems. 134, 279–295. doi: 10.1080/11263500012331350455
Larcher, W. (2005). Climatic constraints drive the evolution of low temperature resistance in Woody plants. J. Agricul. Meteorol. 61, 189–202. doi: 10.2480/agrmet.61.189
Leuschner, C., Wedde, P., and Lubbe, T. (2019). The relation between pressure-volume curve traits and stomatal regulation of water potential in five temperate broadleaf tree species. Ann. For. Sci. 76:60. doi: 10.1007/s13595-019-0838-7
Liu, X. M., Zhou, Y. L., Xiao, J. W., and Bao, F. (2018). Effects of chilling on the structure, function and development of chloroplasts. Front. Plant Sci. 9:1715. doi: 10.3389/fpls.2018.01715
López, R., Brossa, R., Gill, L., and Pita, P. (2015). Stem girdling evidences a trade-off between cambial activity and sprouting and dramatically reduces plant transpiration due to feedback inhibition of photosynthesis and hormone signaling. Front. Plant Sci. 6:285. doi: 10.3389/fpls.2015.00285
López de Heredia, U., López, R., Collada, C., Emerson, B. C., and Gil, L. (2014). Signatures of volcanism and aridity in the evolution of an insular pine (Pinus canariensis Chr. Sm. Ex DC in Buch). Heredity 113, 240–249. doi: 10.1038/hdy.2014.22
López, R., López de Heredia, U., Collada, C., Javier Cano, F., Emerson, B. C., Cochard, H., et al. (2013). Vulnerability to cavitation, hydraulic efficiency, growth and survival in an insular pine (Pinus canariensis). Ann. Bot. 111, 1167–1179. doi: 10.1093/aob/mct084
Luis, V. C., Taschler, D., Hacker, J., Jimenez, M. S., Wieser, G., and Neuner, G. (2007). Ice nucleation and frost resistance of Pinus canariensis seedlings bearing needles in three different developmental states. Ann. For. Sci. 64, 177–182. doi: 10.1051/forest:2006102
Martínez-Ferri, E., Manrique, E., Valladares, F., and Balaguer, L. (2004). Winter photoinhibition in the field involves different processes in four co-occurring Mediterranean tree species. Tree Physiol. 24, 981–990. doi: 10.1093/treephys/24.9.981
Máyer, P., and Marzol, M. V. (2013). “Análisis de la pluviosidad en las islas canarias mediante la elaboración de gradientes” in XXIII Congreso de Geógrafos Españoles (Islas Baleares, Spain: Universitat de les Illes Balears), 145–154.
Miranda, J. C., Lehmann, M. M., Saurer, M., Altman, J., and Treydte, K. (2021). Insight into Canary Island pine physiology provided by stable isotope patterns of water and plant tissues along an altitudinal gradient. Tree Physiol. 41, 1611–1626. doi: 10.1093/treephys/tpab046
Miranda, J. C., Rodríguez-Calcerrada, J., Pita, P., Saurer, M., Oleksyn, J., and Gil, L. (2020). Carbohydrate dynamics in a resprouting species after severe aboveground perturbations. Eur. J. For. Res. 139, 841–852. doi: 10.1007/s10342-020-01288-2
Mohammadian, M. A., Watling, J. R., and Hill, R. S. (2007). The impact of epicuticular wax on gas-exchange and photoinhibition in Leucadendron lanigerum (Proteaceae). Acta Oecologica-Int. J. Ecol. 31, 93–101. doi: 10.1016/j.actao.2006.10.005
Navascués, M., and Emerson, B. C. (2007). Natural recovery of genetic diversity by gene flow in reforested areas of the endemic Canary Island pine, Pinus canariensis. For. Ecol. Manag. 244, 122–128. doi: 10.1016/j.foreco.2007.04.009
Navascués, M., Vaxevanidou, Z., Gonzalez-Martinez, S. C., Climent, J., Gil, L., and Emerson, B. C. (2006). Chloroplast microsatellites reveal colonization and metapopulation dynamics in the Canary Island pine. Mol. Ecol. 15, 2691–2698. doi: 10.1111/j.1365-294X.2006.02960.x
Ogaya, R., Penuelas, J., Asensio, D., and Llusia, J. (2011). Chlorophyll fluorescence responses to temperature and water availability in two co-dominant Mediterranean shrub and tree species in a long-term field experiment simulating climate change. Environ. Exp. Bot. 71, 123–127. doi: 10.1016/j.envexpbot.2010.10.016
Olascoaga, B., Juurola, E., Pinho, P., Lukes, P., Halonen, L., Nikinmaa, E., et al. (2014). Seasonal variation in the reflectance of photosynthetically active radiation from epicuticular waxes of scots pine (Pinus sylvestris) needles. Boreal Environ. Res. 19, 132–141.
Oliveira, G., and Peñuelas, J. (2004). Effects of winter cold stress on photosynthesis and photochemical efficiency of PSII of the Mediterranean Cistus albidus L. and Quercus ilex L. Plant Ecol. 175, 179–191. doi: 10.1007/s11258-005-4876-x
Peguero-Pina, J. J., Alquézar-Alquezar, J. M., Mayr, S., Cochard, H., and Gil-Pelegrín, E. (2011). Embolism induced by winter drought may be critical for the survival of Pinus sylvestris L. near its southern distribution limit. Ann. For. Sci. 68, 565–574. doi: 10.1007/s13595-011-0068-0
Pérez-de-Paz, P., Salas, M., Rodríguez, O., Acebes, J., Del-Arco, M., and Wildpret, W. (1994). “Atlas Cartográfico de los Pinares Canarios IV: Gran Canaria y plantaciones de Fuerteventura y Lanzarote” in Viceconsejería de Medio Ambiente, Consejería de Política Territorial, Gobierno de Canarias (Spain: Santa Cruz de Tenerife).
Peters, J., Gonzalez-Rodriguez, A. M., Jimenez, M. S., Morales, D., and Wieser, G. (2008). Influence of canopy position, needle age and season on the foliar gas exchange of Pinus canariensis. Eur. J. For. Res. 127, 293–299. doi: 10.1007/s10342-008-0205-y
Peters, J., Jimenez, M. S., and Morales, D. (1999). Effect of extreme temperature on quantum yield of fluorescence and membrane leakage of the Canarian endemic pine (Pinus canariensis). Zeitschrift Fur Naturforschung Section C-a J. Biosci. 54, 681–687. doi: 10.1515/znc-1999-9-1010
Peters, R. L., Speich, M., Pappas, C., Kahmen, A., von Arx, G., Pannatier, E. G., et al. (2019). Contrasting stomatal sensitivity to temperature and soil drought in mature alpine conifers. Plant Cell Environ. 42, 1674–1689. doi: 10.1111/pce.13500
Pita, P., Hernández, M. J., and Pardos, M. (2023). Contrasting ethylene-mediated responses to waterlogging in four Eucalyptus globulus Labill. Clones. Environ. Exp. Bot. 215:105503. doi: 10.1016/j.envexpbot.2023.105503
Rahman, T., Shao, M. X., Pahari, S., Venglat, P., Soolanayakanahally, R., Qiu, X., et al. (2021). Dissecting the roles of Cuticular wax in plant resistance to shoot dehydration and low-temperature stress in Arabidopsis. Int. J. Mol. Sci. 22:1554. doi: 10.3390/ijms22041554
Rinne, K. T., Saurer, M., Kirdyanov, A. V., Bryukhanova, M. V., Prokushkin, A. S., Churakova, O. V., et al. (2015). Examining the response of needle carbohydrates from Siberian larch trees to climate using compound-specific C-13 and concentration analyses. Plant Cell Environ. 38, 2340–2352. doi: 10.1111/pce.12554
Ritter, A., Regalado, C. M., and Guerra, J. C. (2019). The impact of climate change on water fluxes in a Macaronesian cloud forest. Hydrol. Process. 33, 2828–2846. doi: 10.1002/hyp.13523
Robakowski, P. (2005). Susceptibility to low-temperature photoinhibition in three conifers differing in successional status. Tree Physiol. 25, 1151–1160. doi: 10.1093/treephys/25.9.1151
Robinson, S. A., Lovelock, C. E., and Osmond, C. B. (1993). Wax as a mechanism for protection against Photoinhibition - a study of Cotyledon orbiculata. Botanica Acta. 106, 307–312. doi: 10.1111/j.1438-8677.1993.tb00753.x
Rodríguez Martín, J. A., Nanos, N., Miranda, J. C., Carbonell, G., and Gil, L. (2013). Volcanic mercury in Pinus canariensis. Naturwissenschaften 100, 739–747. doi: 10.1007/s00114-013-1070-1
Rozas, V., García-González, I., Pérez-de-Lis, G., and Arévalo, J. R. (2013). Local and large-scale climatic factors controlling tree-ring growth of Pinus canariensis on an oceanic island. Clim. Res. 56, 197–207. doi: 10.3354/cr01158
Samdur, M. Y., Manivel, P., Jain, V. K., Chikani, B. M., Gor, H. K., Desai, S., et al. (2003). Genotypic differences and water-deficit induced enhancement in epicuticular wax load in peanut. Crop Sci. 43, 1294–1299. doi: 10.2135/cropsci2003.1294
Sánchez, F. J., Manzanares, M., de Andrés, E. F., Tenorio, J. L., and Ayerbe, L. (2001). Residual transpiration rate, epicuticular wax load and leaf colour of pea plants in drought conditions. Influence on harvest index and canopy temperature. Eur. J. Agron. 15, 57–70. doi: 10.1016/S1161-0301(01)00094-6
Scholz, F. G., Bucci, S. J., Arias, N., Meinzer, F. C., and Goldstein, G. (2012). Osmotic and elastic adjustments in cold desert shrubs differing in rooting depth: coping with drought and subzero temperatures. Oecologia 170, 885–897. doi: 10.1007/s00442-012-2368-y
Sharma, D. K., Andersen, S. B., Ottosen, C. O., and Rosenqvist, E. (2015). Wheat cultivars selected for high F-v/F-m under heat stress maintain high photosynthesis, total chlorophyll, stomatal conductance, transpiration and dry matter. Physiol. Plant. 153, 284–298. doi: 10.1111/ppl.12245
Shepherd, T., and Griffiths, D. W. (2006). The effects of stress on plant cuticular waxes. New Phytol. 171, 469–499. doi: 10.1111/j.1469-8137.2006.01826.x
Smith, B. C., Bullock, B. P., Isik, F., and McKeand, S. E. (2014). Modeling genetic effects on growth of diverse provenances and families of loblolly pine across optimum and deficient nutrient regimes. Can. J. For. Res. 44, 1453–1461. doi: 10.1139/cjfr-2013-0379
Stabentheiner, E., Pfeifhofer, H. W., Peters, J., Jimenez, M. S., Morales, D., and Grill, D. (2004). Different surface characteristics of primary and secondary needles of Pinus canariensis. Flora 199, 90–99. doi: 10.1078/0367-2530-00138
Stegner, M., Florl, A., Lindner, J., Plangger, S., Schaefernolte, T., Strasser, A. L., et al. (2022). Freeze dehydration vs. supercooling of mesophyll cells: impact of cell wall, cellular and tissue traits on the extent of water displacement. Physiol. Plant. 174:e13793. doi: 10.1111/ppl.13793
Takahashi, S., and Badger, M. R. (2011). Photoprotection in plants: a new light on photosystem II damage. Trends Plant Sci. 16, 53–60. doi: 10.1016/j.tplants.2010.10.001
Thomas, H., and James, A. R. (1993). Freezing tolerance and solute changes in contrasting genotypes of Lolium perenne L acclimated to cold and drought. Ann. Bot. 72, 249–254. doi: 10.1006/anbo.1993.1105
Ustin, S. L., and Jacquemoud, S. (2020). “How the optical properties of leaves modify the absorption and scattering of energy and enhance leaf functionality” in Remote sensing of plant biodiversity. eds. J. Cavender-Bares, J. A. Gamon, and P. A. Townsend (Cham: Springer International Publishing), 349–384.
Vaxevanidou, Z., Gonzalez-Martinez, S. C., Climent, J., and Gil, L. (2006). Tree populations bordering on extinction: a case study in the endemic Canary Island pine. Biol. Conserv. 129, 451–460. doi: 10.1016/j.biocon.2005.11.012
Wang, M. Q., Huang, Q. X., Lin, P., Zeng, Q. H., Li, Y., Liu, Q. L., et al. (2020). The overexpression of a transcription factor gene VbWRKY32 enhances the cold tolerance in Verbena bonariensis. Front. Plant Sci. 10:1746. doi: 10.3389/fpls.2019.01746
Weigel, R., Irl, S. D. H., Treydte, K., Beierkuhnlein, C., Berels, J., Field, R., et al. (2018). A novel dendroecological method finds a non-linear relationship between elevation and seasonal growth continuity on an island with trade wind-influenced water availability. Aob Plants. 10:ply070. doi: 10.1093/aobpla/ply070
White, D. A., Turner, N. C., and Galbraith, J. H. (2000). Leaf water relations and stomatal behavior of four allopatric Eucalyptus species planted in Mediterranean southwestern Australia. Tree Physiol. 20, 1157–1165. doi: 10.1093/treephys/20.17.1157
Keywords: chilling, freezing, Pinus canariensis , photoinhibition, cell wall stiffness, epicuticular waxes, cold dehydration, osmotic adjustment
Citation: Miranda JC, López de Heredia U, Fernández V and Pita P (2023) Phenotypic plasticity in Pinus canariensis seedlings growing at chilling and freezing temperatures. Front. For. Glob. Change. 6:1303886. doi: 10.3389/ffgc.2023.1303886
Received: 28 September 2023; Accepted: 12 October 2023;
Published: 27 October 2023.
Edited by:
José Javier Peguero-Pina, Aragon Agrifood Research and Technology Center (CITA), SpainReviewed by:
Georgios Liakopoulos, Agricultural University of Athens, GreeceCopyright © 2023 Miranda, López de Heredia, Fernández and Pita. This is an open-access article distributed under the terms of the Creative Commons Attribution License (CC BY). The use, distribution or reproduction in other forums is permitted, provided the original author(s) and the copyright owner(s) are credited and that the original publication in this journal is cited, in accordance with accepted academic practice. No use, distribution or reproduction is permitted which does not comply with these terms.
*Correspondence: Pilar Pita, cGlsYXIucGl0YUB1cG0uZXM=
Disclaimer: All claims expressed in this article are solely those of the authors and do not necessarily represent those of their affiliated organizations, or those of the publisher, the editors and the reviewers. Any product that may be evaluated in this article or claim that may be made by its manufacturer is not guaranteed or endorsed by the publisher.
Research integrity at Frontiers
Learn more about the work of our research integrity team to safeguard the quality of each article we publish.