- 1ICAR-Indian Institute of Soil and Water Conservation, Dehradun, Uttarakhand, India
- 2Department of Civil and Environmental Engineering, North Dakota State University, Fargo, ND, United States
- 3Govind Ballabh Pant University of Agriculture and Technology, Krishi Vigyan Kendra, Jyolikt, Uttarakhand, India
- 4ICAR-Indian Institute of Soil and Water Conservation, Research Centre, Vasad, India
- 5ICAR-Indian Grassland and Fodder Research Institute, Jhansi, Uttar Pradesh, India
- 6ICAR-Indian Institute of Soil and Water Conservation, Research Centre, Datia, Madhya Pradesh, India
- 7ICAR-Central Coastal Agricultural Research Institute, Ela, Goa, India
The 20-year study investigated the effects of conservation practices (CPs) and farmers' practices (FPs) on various soil quality parameters, yield, and economics of horticultural land use systems. CPs demonstrated significant improvements in soil organic carbon (SOC), available nitrogen (N), phosphorus (P), and potassium (K), compared to FPs. Horticultural systems exhibited higher SOC and available N and P contents than FPs, with substantial variations among different fruit species. CPs also enhanced soil quality index, functional diversity, culturable microbial populations, enzyme activity, and soil microbial biomass carbon (SMBC) compared to FPs. It was observed that the SMBC values were 25.0–36.6% and 4.12–25.7% higher in 0–15 cm and 15–30 cm, respectively, under CPs compared to FPs for all the land use systems. In CPs, dehydrogenase activities (DHAs) in surface soils were 9.30 and 7.50 times higher under mango- and citrus-based horticultural systems compared to FPs. The CPs adopted in aonla, guava, mango, litchi, and citrus-based horticultural systems increased SOC by ~27.6, 32.6, 24.4, 26.8, and 22.0%, respectively, over FPs. Canopy spread, fruit yield, litter yield, and soil moisture were significantly higher in fruit-based horticultural systems under CPs. Economic viability analysis indicated higher net present values (NPVs), benefit-cost ratio (BCR), and shorter payback periods (PBPs) for horticultural land use systems under CPs. Principal component analysis (PCA) revealed that CPs had a more positive influence on soil parameters, particularly DHA, acid and alkali phosphatase activity, available N, P, and K contents, soil microbial load, and organic carbon. The maximum ecosystem services were contributed through mango-based land uses among all land uses. Mango-based horticultural systems exhibited the least impact from both CPs and FPs, while peach-based systems were most affected by CPs. Overall, the findings highlight the benefits of conservation practices in improving soil quality, microbial populations, enzyme activity, and crop productivity in horticultural systems.
Introduction
The land is a vital and non-renewable natural resource of Mother Earth that provides essential resources such as food, shelter, and fiber. Land degradation is an emerging global issue that is caused by both anthropogenic and climatic factors (Singh et al., 2023). This phenomenon is negatively impacting agricultural productivity, with approximately 20% of agricultural land, 30% of forests, and 10% of savannas worldwide (Zhang et al., 2019). Moreover, it is projected that the percentage of degraded land will increase in future, particularly in low- and middle-income nations of tropical and subtropical regions (Chen et al., 2019). The loss of productive capacity due to natural processes or human activities is causing a decline in on-site and off-site native environmental services, which has long-term effects. Land degradation is a significant driver of food insecurity and climate change, resulting in a yearly loss of 6.0 million hectares (M ha) of productive land globally (Lal, 2015). Additionally, soil degradation is accelerating, causing a decline in SOC and fertility, which is promoting soil erosion problems, resulting in the loss of nutrients and SOC within the root zone, and reducing crop productivity (Singh et al., 2022; Jinger et al., 2023). Land degradation is affecting the lives of nearly 1.5 billion people, with approximately 15 BT of soil lost each year due to desertification and drought. Moreover, approximately 12 M ha area is lost annually owing to these phenomena. The biodiversity loss caused by land degradation amounts to approximately 27,000 species each year, with around 110 countries being under potential risk, affecting the lives of 250 million people and putting 1 billion individuals at risk. The global cost of desertification is estimated at USD 42 million (Hamdy and Aly, 2014). El-Swaify and Dangler (1982) noticed that the degradation of land reduces the availability of plant nutrients present in the soil along with the reduction in SOC, which is the cause of lowering crop productivity. Moreover, the reduction in crop productivity is due to the initiation of land degradation in the rooting zone of crops (El-Swaify and Cooley, 1981).
The United Nations Convention to Combat Desertification (UNCCD) at COP 14 in 2019 aimed to bring 350 M ha of degraded land worldwide into cultivation by 2030. India has approximately 120.7 M ha of degraded land, with a significant portion of it being physically degraded due to water erosion, characterized by poor soil physico-chemical properties that make it unsuitable for field crops (Gupta et al., 2021a,b; Jinger et al., 2022). Recently, India committed to the restoration of 26 M ha of degraded land in the country to achieve land degradation neutrality through prevention, mitigation, and rehabilitation techniques (Dhyani et al., 2023). The degraded lands in India can be restored through the application of various techniques, such as micro-site improvements, the addition of organic manures, forest litter, crop residues, and perennial deep-rooted fruit tree species (Rathore et al., 2014). Rainfed agriculture, which makes up approximately 80% of the world's produce and over 60% of the world's food, is particularly important for developing countries, as it is the backbone of marginal or subsistence farming (Singh et al., 2021). However, poor yields and high water losses are major issues in rainfed agriculture, exacerbated by climate change and monsoon variability. To improve productivity, in-situ moisture conservation strategies should be given more attention. Additionally, innovative interventions are needed to restore degraded or wastelands and enable them remunerative, ecologically benign for sustainable agriculture production systems, particularly in India, which supports a large proportion of the world's human and livestock populations on limited land area.
In India's Nationally Determined Contribution to the Paris COP 21 agreement in 2015, India committed to sequester an additional 2.5 to 3 billion tons of CO2eq through additional planting of trees or fruit trees by 2030 (MOEFCC, 2015). Therefore, growing fruit crops on degraded lands is a natural way to enhance soil fertility and promote biological activities by adding organic matter such as litterfall and root decay to the soil, leading to an increase in soil organic carbon, soil fertility, nutrient recycling, and biological transformations in the rhizosphere (Rathore et al., 2014). Commercial fruit species, such as mango, guava, aonla, bael, litchi, lemon, kagzi lime, pumelo, and grapefruit, are commonly cultivated, but there is limited information on utilizing degraded lands under rainfed conditions (Rathore et al., 2021). Thus, it is important to cultivate fruit crops with deep roots and low water requirements on degraded lands. Mango (Mangifera indica L., Anacardiaceae), litchi (Litchi chinensis Som.; Sapindaceae), peach (Prunus persica L.; Rosaceae), aonla (Emblica officinalis; Phyllanthaceae), and mandarin (Citrus reticulata L; Rutaceae) are commercially significant subtropical fruit crops that provide a good source of vitamins, minerals, and antioxidants. These crops cover an area of 3.6 M ha, accounting for 53.5% of the total area under fruits in India and producing approximately 36.7 MT of fruits, which is 37.0% of India's total fruit production. Their average productivity ranges from 7.60 to 15.0 t ha−1 (NHB, 2021). Fruit-based land use systems are economically viable for class V and VI soil types as they use various resources judiciously and cater to multiple needs simultaneously. These systems are most suitable for areas that require soil moisture conservation, soil erosion reduction, and sustainable production and income (Rathore et al., 2018). They provide a self-sustainable system where solar energy is harvested at different heights, resulting in higher economic returns even under stressed growing conditions than annual crops (Saroj et al., 2000). Furthermore, they offer opportunities for ancillary industries such as fruit processing (preserves, jam, jelly, etc.), essential oil extraction, employment generation, improved soil organic carbon, and enhanced biological activity for rhizospheric environment stability (Rathore et al., 2014). Cultivation of these fruit crops under conservation practices imparts good quality production of fruits and crops with higher productivity, ultimately leading to achieving the SDG of zero hunger (SDG no. 2) and no poverty (SDG no. 1). Moreover, employment generation through digging pits for planting of saplings, pruning, harvesting of fruits and crops, sustainable production of the fruit production system, and mitigation of GHGs would achieve SDG of decent work and economic growth (SDG no. 8), responsible consumption and production (SDG no. 12), and climate action (SDG no. 13), respectively.
Furthermore, the valuation of ecosystem services in different horticultural land use systems is important because it provides a quantifiable measure of the benefits to the farmers and human wellbeing. Ecosystem services include services such as water purification, climate regulation, and nutrient cycling, which are crucial for sustaining agricultural productivity and maintaining ecological balance (Orlandi et al., 2023). By assigning economic values to these services, policymakers, land managers, and stakeholders can make informed decisions regarding land management, conservation efforts, and resource allocation. Valuation helps in recognizing the contributions of ecosystems, guiding sustainable practices, and promoting the conservation and restoration of horticultural systems for long-term environmental and socio-economic benefits. After considering these things, the monetary value of regulating (carbon sequestration) and supporting ecosystem service (nutrient augmentation) of different horticultural land use systems has been estimated in this study (Pandey et al., 2021).
We put forth a hypothesis that by restoring degraded land and implementing an ecological approach that integrates deep-rooted fruit-based land uses, with or without intercropping, and applying crop residues for in-situ moisture retention, integrated nutrient management, we can substantially enhance fruit yields, soil fertility (including SOC and available N, P, and K contents), microbial populations, and enzyme activity, thereby improving nutrient recycling and valuation of ecosystem service. There is still a lack of comprehensive information on soil enzymatic activities, microbial biodiversity, and available nutrients in degraded lands restored through horticulture land use systems. To validate the above hypothesis, our objectives were to (a) assess the effect of CPs and farmers' practices (FPs) on long-term fruit productivity, (b) evaluate the changes in soil fertility parameters, including SOC and nutrient availability, (c) ascertain the soil microbial populations and soil enzyme activities, and (d) valuate ecosystem services under horticultural systems.
Materials and methods
Experimental site
The study was conducted at the research farm of the ICAR-Indian Institute of Soil and Water Conservation in Selakui, Dehradun, Uttarakhand, India from 1995 to 2015 under the subtropical climate of the Indian Himalayas. The site is located at 30° 21′ N latitude, 77° 52′ E longitude, and 517 m above mean sea level with an annual rainfall of 1,600 mm. The mean maximum and minimum temperatures ranged between 19.0 and 37.6°C and between 3.6 and 24.0°C in summer and winter, respectively. In general, May–June were the hottest (45°C), while December–January were the coldest (2°C). The experimental site was a bouldery riverbed situated at Asan River, a tributary of River Yamuna. Sieve analysis of 1 m3 soil profile conducted at soil working time indicated that 1.27% to 79.46% of the material was found to be <2 mm, and the remaining were gravels and boulders (weight basis). The soil gravel ratio observed in the 1 m3 pit is mentioned in Table 1. The soil was neutral in reaction (pH 6.5–7.0) with low organic carbon (0.5–0.6%), total N (0.06–0.065%), available P (24.49–25.00 kg ha−1), available K (116.42–117.56 kg ha−1), high Ca (0.195–0.197%), and Mg (0.14–0.15%).
Treatment details
Five horticulture species were used, viz. mango, litchi, peach, aonla, and mandarin, along with farmers' practices (control) for study. Two practices were selected for the study, viz. (1) conservation practices (CPs) which include microsite improvement (removal of boulders from the pit), integrated nutrient management (farm yard manure + inorganic fertilizers + NPK-consortia of biofertilizer), and mulching (crop residue and leaf litter of trees); and (2) farmers' practices (FPs) includes normal planting with fertilizers without mulching. The varieties of different fruit species and planting geometry are mentioned in Table 1. The methodology of how conservation practices in different horticulture land use improve the ecosystem services has been mentioned in a graphical format in Figure 1.
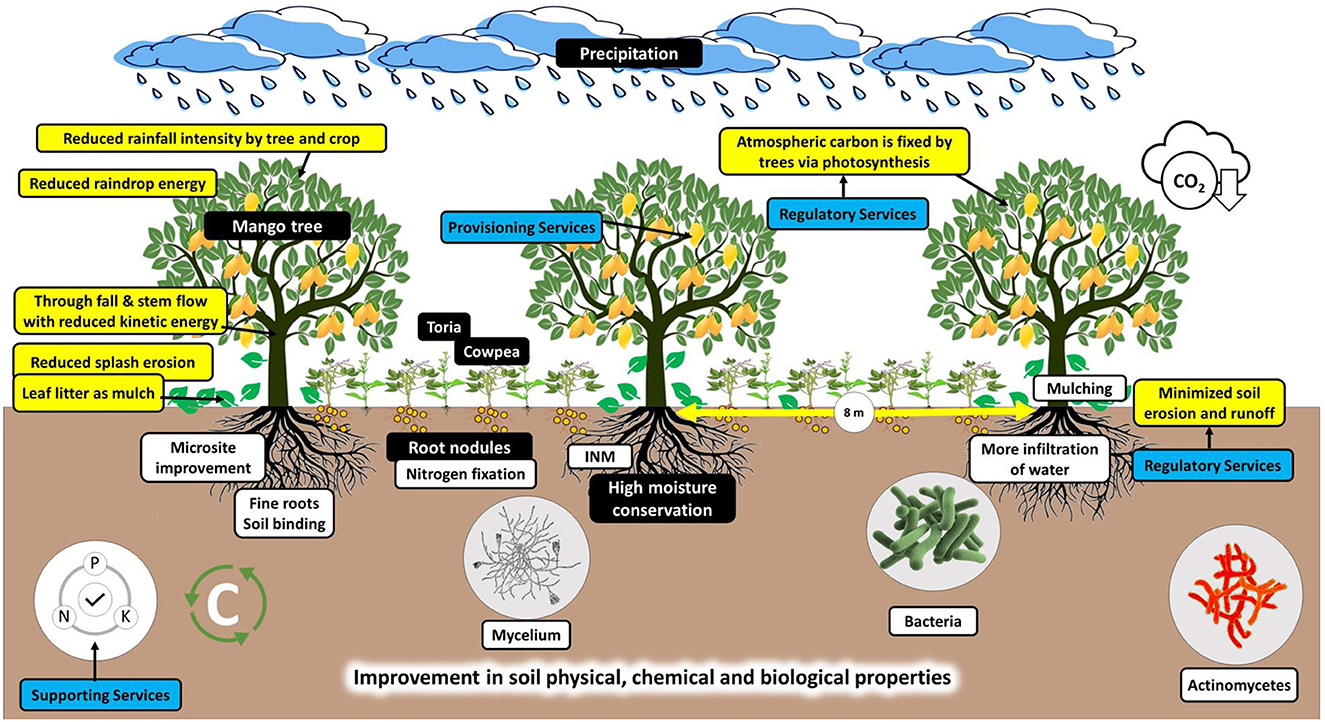
Figure 1. Methodology or conservation practices in mango-based land use improve the ecosystem services (This model is similar to other fruit crops of this experiment).
Soil sampling and analysis
Soil samples were collected using a randomized quadrat sampling experimental design from different land use types. Three quadrats, each of 10 × 10 m for CPs and FPs land use types, were taken as samples with two different soil depths (0–15 and 15–30 cm) in three replications from each quadrat. Pooling of samples was done, and a composite sample was created with a total of 12 samples. The samples were sieved with a 2-mm sieve and stored at ambient temperature for further analysis. SOC was determined using the K2Cr2O7-H2SO4 wet oxidation method (Walkley and Black, 1934). The soil moisture was determined using the gravimetric method (Reynolds, 1970). Soil mineral N and available P and K contents were measured using standard procedures of Hanway and Heidel (1952), Olsen et al. (1954), and Bremner and Keeney (1965), respectively.
Enzyme activity-based index calculation
Soil quality index
The geometric mean (GMea) of the assayed enzymes was calculated for each sample as:
where DHA, AP, ACP, BGD, and URE stand for dehydrogenase, alkali phosphatase, acid phosphatase, β-D-glucosidase, and urease, respectively. GMea is an integrative approach for combining a large number of enzyme activities related to different soil functions and nutrients. Hence, it can imitate soil quality index (Paz-Ferreiro et al., 2011). In addition, its sensitivity to soil management (García-Ruiz et al., 2008; Paz-Ferreiro et al., 2012) makes it an eligible early indicator of soil quality changes.
Soil functional diversity
It was determined using the
(i) Shannon's diversity index (H)
(ii) Simpson–Yule index (SYI)
where Pi is the ratio of each enzyme activity to the sum of all enzyme activities for a particular sample. In all cases, enzyme activities were expressed as microgram products formed per gram of soil per hour.
Microbial population count
The stored soil samples at 4°C were used for the analysis of microbial count, viz. bacteria, fungi, and actinomycetes population in the respective treatments along with control in three replications. The autoclaved readymade potato dextrose agar media (38 g l−1) with pH 7 was used for bacterial and fungal population count using the serial dilution method. The suspension of serially diluted samples from each treatment was spread in plates using a spreader and incubated at 25 ± 2°C and 32 ± 2°C for fungus and bacteria, respectively. The bacterial and fungal populations were recorded in 48 h and 72 h, respectively, after incubation. For actinomycetes, autoclaved actinomycetes isolation agar media with pH 7 was used to ascertain the actinomycetes population in treatments. The serially diluted samples were inoculated in plates, and reading was taken after 3 days of incubation (25 ± 2°C) (Vance et al., 1987). The population of fungi and bacteria was calculated in terms of colony forming units (CFU) per gram oven dry weight basis (Rolf and Bakken, 1987; Sepehri and Sarrafzadeh, 2018).
Soil enzyme activities
Five soil enzymes were estimated, which include β-glucosidase activity (BGD), urease activity, alkaline phosphatase (AP), acid phosphatase activity (ACP), and DHA. β-glucosidase activity was determined using a procedure adopted by Eivazi and Tabatabai (1977, 1988). In β-glucosidase activity, p-nitrophenyl-β-D-glucoside was used as the substrate. The activity was expressed as μg PNG g−1 dwt h−1 at 37°C. Similarly, urease activity and acid phosphatase activities were determined using the protocol described by Tabatabai and Bremner (1969). Urease activity was expressed as NH3-N g−1 h−1 at 37°C and AP was expressed as μg p-NPP g−1 h−1 at 30°C (Tabatabai and Bremner, 1969). DHA was determined using a spectrophotometer and expressed as μg TTC g−1 h−1.
Soil microbial biomass carbon
Soil microbial biomass carbon (SBMC) was calculated by fumigating fresh soil of known mass (35 g) with 2 mL of ethanol-free CHCl3, and the extract was taken with 140 ml of 0.5 M K2SO4 after 1-day ambient temperature incubation. However, unfumigated soil extract was taken directly. SBMC was calculated from the difference in the carbon of fumigated and unfumigated soil using a conversion factor (0.38) described by Vance et al. (1987).
Growth, yield, and litter production estimation
The canopy spread of all the fruit species was measured with the help of a measuring tape in north–south and east–west directions during the first year and final year. The values of canopy spread in both directions were averaged and expressed in meters (m). The four trees of all treatments were marked for recording data on fruit yield. The fruits of all five fruit species were harvested at maturity stages, weighed with the help of electronic balance every year after the initial fruit-bearing year, and expressed in t ha−1. Litterfall of all fruit species was collected annually with the help of litter traps of 1 × 1 m size placed in four directions under the tree during a litterfall period of 12 months each year (January–December) and weighed by electronic balance after drying in oven and expressed in t ha−1.
Economic analysis
The agriculture inputs such as fertilizers and manures, seeds, intercultural operations, etc., and fruit yields were recorded annually. The monetary values of these inputs and outputs were calculated based on current economic values. During the study, the average price of inputs and outputs was worked on based on yearly price fluctuations. Minimum support prices of fruits and intercrops for every year were taken into account for calculating the economy (DFPD, 2015; NHB, 2015). Total returns were calculated annually, and the benefit:cost ratio was inferred. Based on the economic life of 20 years for five fruit species utilized for estimating the benefit:cost ratio (BCR), net present value (NPV), and payback period (PBP) were calculated at a discounted rate of 8%.
The equivalent yield of crop X [t ha−1] =
Tree and soil carbon sequestration
The calculated tree biomass was multiplied with the constant factor of 0.50 for the computation of carbon stock (IPCC, 2003). The calculated carbon stock was used for calculating CO2 sequestration by multiplying the carbon stock with a constant factor of 3.67 (IPCC, 2003). Based on SOC and bulk density, the soil carbon sequestration was calculated (Lenka et al., 2013; Paul et al., 2016; Yadav et al., 2018). Equation 5 has been used for calculating soil carbon sequestration:
Ecosystem services valuation
The unit coefficients of these ecosystem services (carbon sequestration and nutrient augmentation) were calculated. After that, the avoided cost principle or the market price method was used to compute monetary values associated with these ecosystem services. The data requirements and valuation methodologies used for these services are summarized in Table 2. The per hectare quantity of nutrients augmented by the different land use was multiplied by the economical price (market price and subsidies) of chemical fertilizers, as shown in Equation 6. The data on prices of fertilizers in terms of nutrients were taken from the Department of Fertilizers, Ministry of Chemicals and Fertilizers, Government of India (GoI).
where MVNS is the monetary value (Rs ha−1) of nutrients saved due to retained soil that otherwise would have been lost; QN, QP, and QK are unit coefficients of saved N, P, and K, respectively, and PN, PP, and PK are the economical prices for N, P, and K, respectively. Similar to the nutrient augmentation service, the monetary value of the carbon sequestration was estimated by multiplying the avoided cost per ton of CO2 by the per hectare carbon sequestration potential of fruit trees and soil. The damage avoided cost of CO2 was taken from the published literature (Ricke et al., 2018; GOI, 2021). In most of the studies, carbon sequestration has been reported in the form of SOC; hence, it was converted into CO2 equivalent using the conversion coefficient of the Intergovernmental Panel on Climate Change (Mekuria et al., 2011).
Statistical analysis
To assess the impact of land use on various parameters, including soil moisture, soil N, P, K, SOC, organic carbon of bacteria, organic carbon of fungi, organic carbon of actinomycetes, β-glucosidases, ureases, acid phosphatase, and DHA, factorial (ANOVA) was carried out. Three factorial designs were used for conducting the experiment (tree × conservation practices × depth of soil). The statistical significance was determined and compared using Duncan's multiple range test (DMRT) at a significance level of P <0.05. The statistical analysis was performed using OPSTAT (Sheoran et al., 1989). Principal component analysis (PCA) was used to assess the relationship between multivariate data using R Studio (version 3.5.1).
Results
Soil organic carbon, total N, and P
The analysis of variance showed that most of the characters taken in the study showed significance. However, organic carbon of fungi (OCF), organic carbon of actinomycetes (OCA), SMBC, organic carbon, P content, N content, and dehydrogenase enzyme activity (DHA) showed non-significant effect of interactions (tree × conservation practices × depth of soil). However, other parameters showed no significant interaction. With respect to AP, CP has a non-significant effect, although tree species, soil depth, and interactions do have significant effects. In relation to DHA, the interaction between tree species and soil depth was non-significant. The total P content showed a non-significant interaction between tree species and CP. The SOC, available N, P, and K were significantly improved in CP among horticultural land use systems (Table 3). Significant improvements in the chemical composition of soil (0–15 and 15–30 cm) were observed after 20 years of horticultural system involvement (Table 3). Despite soil depth, marked improvement in SOC was noted in all fruit-based land use systems under CPs as against FPs. The peach-based land use system with CPs recorded the highest increment in SOC, i.e., 37.70% and 26.32% at 0–15 cm and 15–30 cm soil layer, respectively, as compared to FPs, while citrus-based land use noted the lowest increment (23.29%) in SOC at 0–15 cm soil layer. Interestingly, mango, litchi, and citrus-based land uses recorded the equivalent 20% increase in SOC at 15–20 cm soil layer in CPs as against FPs. In fallow land, SOC increment in CPs was 24.49% and 18.92% when compared to FPs.
Between practices, ~23.29–37.70% at 0–15 cm depth and 18.92–26.32% at 15–30 cm depth, more SOC was noticed among all horticultural land use systems and fallow land use. The CPs adopted in aonla, guava, mango, litchi, and citrus-based horticultural systems increased SOC by ~27.6, 32.6, 24.4, 26.8, and 22.0%, respectively, over FPs. Approximately 3.03–57.5% and 23.8–157.1% higher available N at 0–15 cm and 15–30 cm soil depth, respectively, were recorded under CPs in horticultural land use systems over fallow land. A similar trend was also found in FPs over control. Between practices, 20.4–29.8 and 11.1–23.4% more available N at 0–15 cm and 15–30 cm depth, respectively, were registered in CPs over FPs among land use systems. The mean plant available N observed was 15.6–27.2% higher in the case of CPs than FPs among horticultural land use systems. The horticultural systems improved P availability by ~1.36–4.47 times and K availability by 1.17 to 3.04 times over control at the 0–15 cm soil layer. A similar trend was also found in sub-surface (15–30 cm) soil.
Soil quality index and functional diversity
In surface soil, the geometric mean of enzyme activities (GMEa) of the horticultural systems of mango and aonla was 3.87 and 3.38 times higher than the control, respectively. The subsurface soil revealed similar findings (Table 4). Surface soil had a GMEa that was around 4% higher than subsurface soil. In both soil layers, Shannon's diversity index (H) was greater in mango- and aonla-based horticultural systems than in other treatments. Intriguingly, the H value in the 0–15 and 15–30 cm soil layers of the mango-based horticultural system was 2.29 and 2.52 times higher than the control, respectively. The H values of citrus- and litchi-based horticultural systems, however, were comparable. Mango- and aonla-based horticultural systems in both soil layers had SYI values that were noticeably greater than litchi- and citrus-based horticultural systems. SYI levels were greater in both soil layers than in H, in contrast. Notably, horti-pasture adoption produced more soil functional diversity in surface and sub-surface soil layers than in control land.
Culturable microbial population
The microbial populations of bacteria, fungi, and actinomycetes were significantly improved in CPs among horticultural systems (Table 5). The microbial counts of bacteria were 14.2–47.0% and 20.4–92.4% more in CPs than FPs at soil depths of 0–15 and 15–30 cm, respectively. Similarly, approximately 27.4–53.8 and 10.4–46.6% higher fungal population was recorded in CPs over FPs at a soil depth of 0–15 and 15–30 cm, respectively. Similarly, actinomycetes counts were also 20.4–51.5% and 15.5–40.4% higher under CPs compared to FPs at soil depths of 0–15 and 15–30 cm, respectively. Among the different horticultural land use systems, higher densities of bacteria, fungi, and actinomycetes were recorded in the aonla, mango, and citrus land use systems, respectively, over the rest of the land use systems under both CPs and FPs.
Soil enzyme activity
CPs across horticulture land use systems substantially increased the enzymatic activity (Table 6). The enzymatic activities of ACP and AP were 94.5–175.2% and 155.8–319.4% and 64.3–144.3% and 30.6–133.2% higher under CPs over FPs at 0–15 cm and 15–30 cm soil depth, respectively. CP and surface soil depth (0–15 cm) had more enzymatic activities compared to FPs and sub-surface soil depth (15–30 cm). Similarly, urease and BGD enzymatic activities were also 130–139.0% and 50.1–279.1% and 13.6–47.0% and 37.1–252.3% higher under CPs over FPs at 0–15 and 15–30 cm soil depth, respectively. DHA was also 128–1,090% and 118.7–1,076.5% more under CPs over FPs at 0–15 and 15–30 cm soil depth, respectively. Among the different horticultural land use systems, higher activity of ACP, AP, DHA, and BGD was observed in the kinnow land use system, respectively, over the rest of the land use system under both CPs and FPs. However, the mango land use system recorded the higher activity of urease both in CPs and FPs over other land use systems.
Soil microbial biomass carbon
The data show that the SMBC values for different land uses under CPs and FPs at two soil depths (0–15 cm and 15–30 cm) were significantly improved after 20 years of plantation (Table 7). Among horticultural land use systems, mango followed by litchi had the highest SMBC values with the lowest value in peach under both CPs and FPs and soil depths. Approximately 11.1–56.7% and 28.2–57.1% more SMBC values were recorded in horticultural land systems established under CPs compared to fallow land at 0–15 cm and 15–30 cm soil layers, respectively. Similarly, 1.69–43.9 and 8.54–32.0% higher SMBCs were observed in horticultural land systems established under FPs over fallow land at 0–15 cm and 15–30 cm layer, respectively, among horticultural land systems established under FPs. It was observed that the SMBC values were 25.0–36.6% and 4.12–25.7% higher in 0–15 cm and 15–30 cm, respectively, under CPs compared to FPs for all the land use systems. The data also revealed that 14.3–31.8% and 2.89–21.6% higher SMBCs were registered under CPs and FPs, respectively, in the surface soil layer (0–15 cm) in comparison to the sub-surface soil layer (15–30 cm). The average values of SMBC (0–30 cm) recorded were 15.0–31.4% more under CPs than FPs among all horticultural land use systems and fallow land. Finally, the fallow land use has the lowest SMBC values under both CPs and FPs, with a more considerable difference between the two practices in the 0–15 cm surface soil than in the 15–30 cm sub-surface soil.
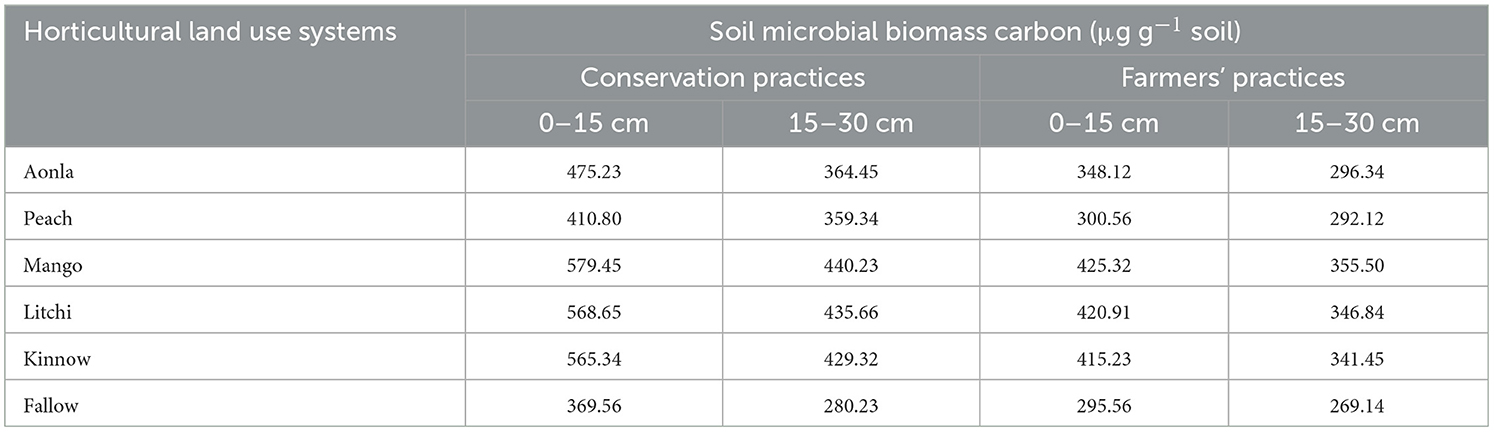
Table 7. Soil microbial biomass carbon (μg g−1 soil) in different fruit species established with conservation and farmers' practices.
Canopy spread, fruit yield, litter yield, and soil moisture
Canopy spread, fruit yield, litter yield, canopy spread, and soil moisture were observed significantly more in fruit-based horticultural systems established with CPs over FPs on degraded land (Table 8). The mean canopy spread of different fruit species observed was mango (6.05 m and 7.95 m), litchi (5.82 and 7.46), aonla (5.48 and 7.00), peach (5.56 and 7.00), and citrus (3.93 m and 5.00 m) in different fruit species established under FPs and CPs, respectively. The long-term data on fruit yield have shown an increasing trend in fruit yield with the progressive years except for litchi and kinnow. There was a declining trend in fruit yield of kinnow and litchi after 12 years of plantation in both CPs and FPs (Figure 2). The mango fruit yield and mango equivalent fruit yield (MEFY) of litchi, aonla, peach, and citrus were calculated for comparison of systems using Equation 4. Among the horticultural systems, the maximum mango fruit yield was recorded (10.1 and 15.0 t ha−1) followed by mango equivalent fruit yield (MEFY) of litchi (7.76 and 10.9 t ha−1), MEFY of peach (6.92 and 10.0 t ha−1), MEFY of aonla (6.23 and 8.41 t ha−1), and MEFY of citrus (6.11 and 8.13 tha−1) under FPs and CPs, respectively, of 20 years plantation. Similarly, mean litter yields of mango, litchi, aonla, peach, and citrus were observed at 3.02 and 4.05, 2.85 and 3.78, 5.46 and 7.48, 5.56 and 7.25, and 6.05 and 8.35 t ha−1 in FPs and CPs, respectively during 20 years of life span. Similarly, mean soil moisture in mango, litchi, aonla, peach, and citrus under FPs and with CPs were 10.5 and 13.6, 10.15 and 13.1, 10.12 and 13.5, 12.6 and 14.5, and 13.4% and 15.2%, respectively, during 20 years of life span in degraded lands.
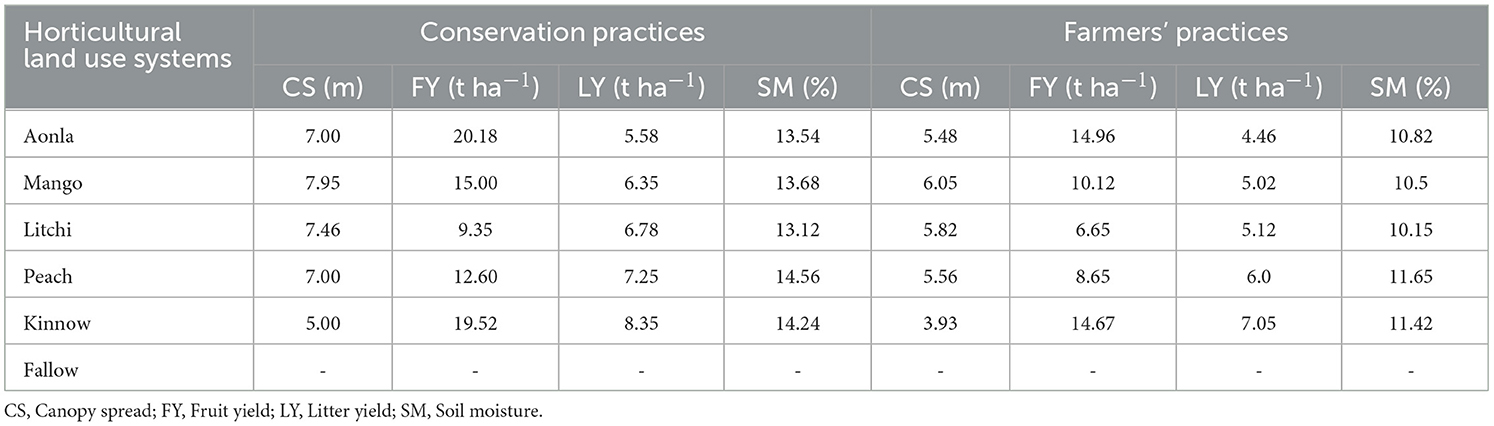
Table 8. Average growth parameter, fruit and litter yields, and soil moisture in different fruit species.
Economic viability
The net present values (NPVs), benefit-cost ratio (BCR), and payback period (PBP) were analyzed for mango, litchi, aonla, peach, and citrus fruit species based horticultural land use systems with CP and FP conditions (Table 9). The values of NPV, BCR, and PBP of all fruit species in the case of CPs were higher than FPs. The highest NPVs were realized in mango (607,201.8 and 900,032 Rs ha−1) followed by litchi (465,514 and 654,524 Rs ha−1), peach (415,166 and 604,832 Rs ha−1), aonla (374,002 and 504,464 Rs ha−1), and citrus (366,786 and 487,982 Rs ha−1) established under FPs and CPs, respectively. Similarly, the highest BCR was also realized in mango (2.93 and 3.90), followed by litchi (2.81 and 3.14), aonla (2.24 and 3.05), peach (2.11 and 2.95), and minimum in citrus (2.05 and 2.88) in FPs and with CPs, respectively. Similarly, the minimum PBP (4.0 and 3.2 years) was observed with peach, followed by citrus (4.2 and 3.5), mango (5.0 and 4.0), litchi (5.4 and 4.2), and the highest PBP with aonla (5.5 and 4.4 years) (Table 9).
Principal component and DMRT analysis
PCA biplots were compared for both CP and FP. The total variation was 72.6% and 74.5%, respectively. In the scree plot, the Y axis shows PC components while the X axis shows the variances. By comparing both FPs and CPs, more correlation in the values of BGD, DHA, ACP, AP, N, K, soil microbial load, and organic carbon was found in CPs compared to the FPs. However, urease, organic carbon fungi, P, organic carbon bacteria, and organic carbon actinomycetes were less related, which is similar in both cases. This showed that the CPs improved most of the soil enzymatic properties holistically when applied compared to FPs. The PCA biplot showed a better response by mango was least affected by both CPs and FPs; however, peach was most affected by CPs (Figure 3). The DMRT was also done to compare the different treatments (Table 10).
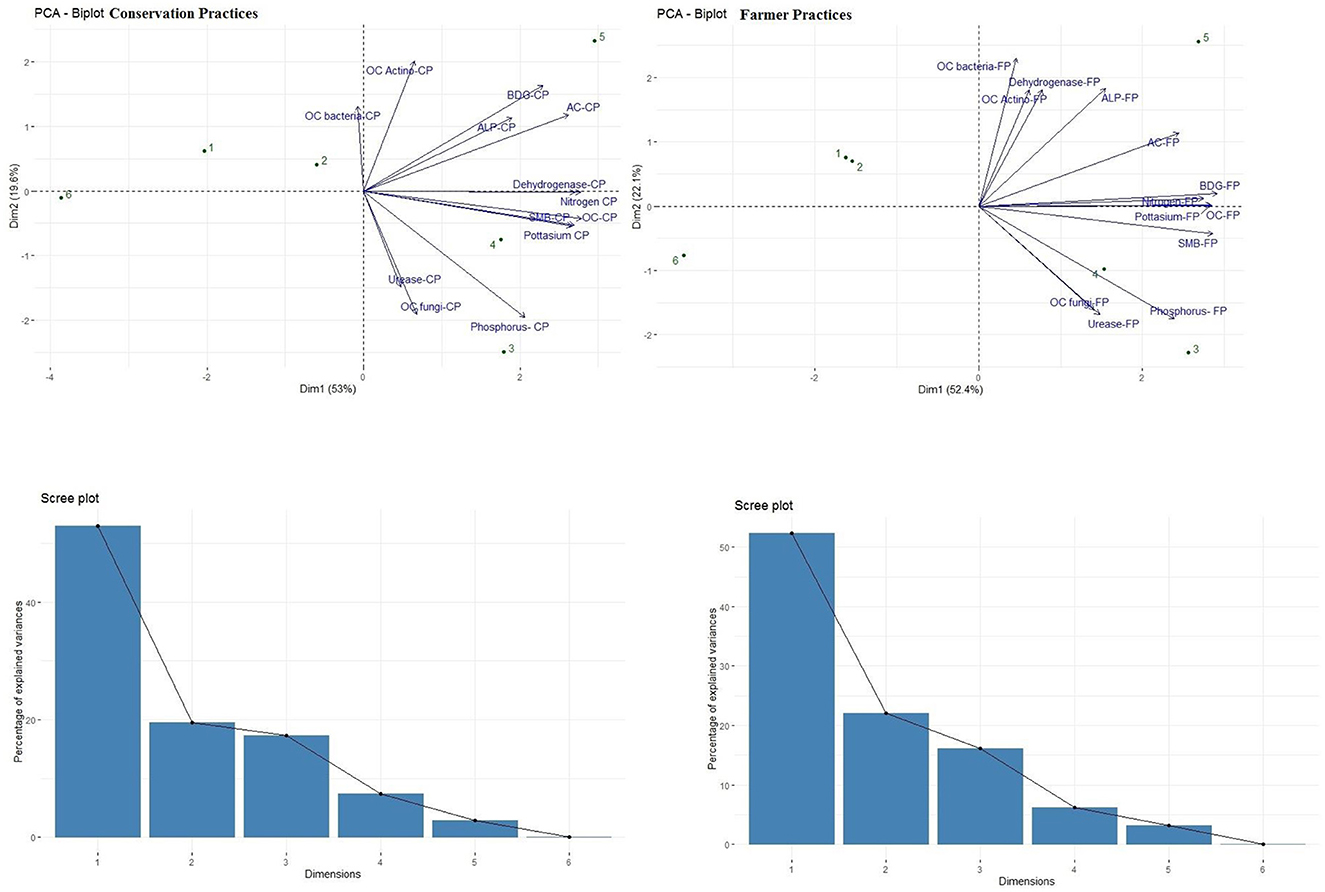
Figure 3. PCA biplot and screen plot for conservation practices (Left) and farmers' practice (Right).
Ecosystem services
The carbon sequestration (trees + soil), nutrient augmentation, and total ecosystem services of horticultural land use system under conservation and farmers' practices have been assessed in Tables 11–13. The monetary value of C-sequestration ranged from Rs. 29,291.99 to 45,508.45 ha−1 under conservation practice, which is 8.33% to 15.94% more than monetary values observed under farmer practice. Similarly, the monetary value of nutrient augmentation ranged from 808.6 to 11,414.5 and 377.4 to 9,411.1 in conservation practice and farmer practice, respectively (Table 12). The highest monetary valuation of the total ecosystem services (regulating service + supporting service) among different fruit-based land uses with conservation practice is Rs 56,907 ha−1 in the mango-based land use system among all land uses (Table 13). The conservation practice realized 9.6% to 16.4% of additional benefits in the form of ecosystem services in different horticultural land use systems.
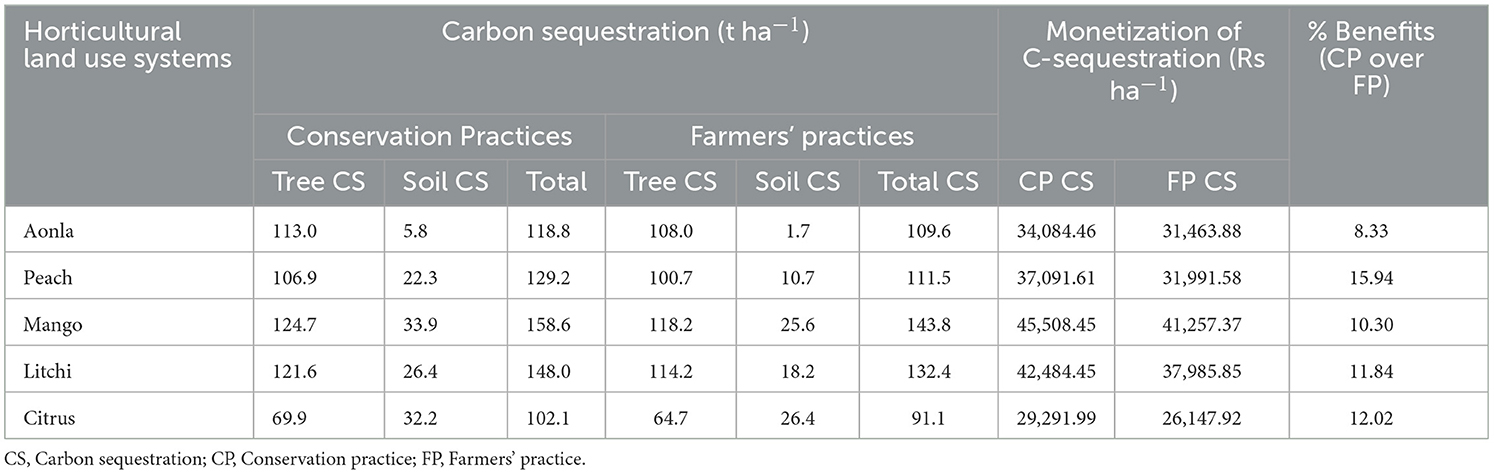
Table 11. Carbon sequestration regulatory ecosystem services (t ha−1) and monetization (Rs ha−1) under different horticultural land use systems.
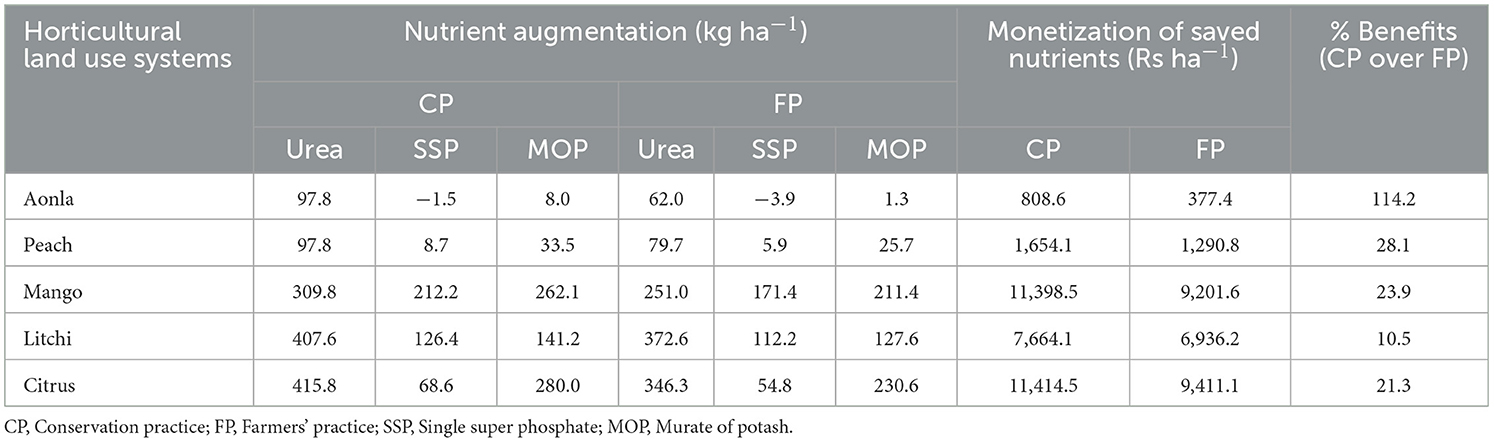
Table 12. Supportive ecosystem services (nutrient augmentation with fertilizer equivalent of N, P, and K) in different horticultural land use systems.
Discussion
C, N, and P in soils
The carbon, N, P, and soil quality index have been influenced significantly by various fruit-based land uses (Tables 3, 4). According to Ghosh et al. (2016), there may be a positive association between microbial population and SOC in both soil layers, which may account for the significantly higher DHA in the kinnow- and mango-based horticulture systems. According to Kumar et al. (2019), kinnow and mango-based agricultural systems with higher levels of glucosidase activity than others may have higher microbial biomass turnover and adequate C availability because of higher litterfall, root biomass, and rhizodeposition. Urea and similar compounds are hydrolyzed by urease. Due to increasing SOC and a microbial population that promoted urease secretion in mango- and litchi-based horticulture systems, its activity increased (Chang et al., 2007). According to Chakrabarti et al. (2004), the reduced urease activity in the control group may be caused by insufficient C and energy. Kinnow- and peach-based horticulture systems have much greater ACP than fallow. According to Dodor and Tabatabai (2003), SOC can specify phosphatase activity. It is possible that the presence of phospholipids and inositol phosphates in litters promoted phosphatase activity. Improved animal-based and horticulture-based system + conservation practices were found to reduce soil erosion (34%−48%) and loss of SOM (26%−51%), N (33%−45%), P (19%−54%), and K (27%−51%) compared to the traditional shifting cultivation system in Nagaland, India. It might be due to the improved physical, chemical, and biological properties of soil due to integrated nutrient management in this production system (Chatterjee et al., 2021).
Soil functional diversity is controlled by the kind, amount, and accessibility of the substrate to microbes (Ghosh et al., 2019). As a result, agroecosystems that get organic C, N, P, and S from various sources may have an impact on how microbial communities in soils operate and change organic matter (Hu et al., 2011; Ghosh et al., 2020). Horticultural systems based on kinnow and peach had the most litterfall. However, the larger Shanon's diversity index (H) in these plots than others was brought on by strong urease activity in mango and aonla (Table 4). Although some systems received varied amounts of litterfall, this measure was unable to distinguish between the functional varieties of the soil in those systems. We created the Simpson–Yule index (SYI), a dominance indicator that gives more weight to common or dominant species, to distinguish between them. Thus, a distinct distinction between litchi and citrus was discovered. SYI fared better in this investigation at detecting changes in soil functional diversity. The biggest C availability, in this case, was accounted for by mango- and aonla-based horticulture systems, which were notably different from the others due to catabolic diversity (Ghosh et al., 2023) and led to increased soil functional diversity. Mango- and aonla-based horticulture systems may deliver organically bound N, P, and S slowly but gradually thanks to equilibrium between labile and recalcitrant pools of SOM. It is evident from GMEa values that horticulture systems enhanced soil health. Utilizing horticulture methods ensured a larger above-ground biomass production, which in turn led to a higher litterfall and SOC status. In mango-based horticulture systems, these events increased microbial population and enzyme activity over control. Through increased root biomass and higher litterfall, horticultural systems also made sure that enzymes had access to the substrate. Improved nutrient cycling, availability, and soil carbon sequestration were all made possible by increased soil enzyme activity.
Culturable microbial population
Substrate quantity, quality, and microbial accessibility control soil functional diversity (Bending et al., 2002). Therefore, the agroecosystems receiving organic C, N, P, and S from different sources can affect the microbial transformation of organic matter and the functional diversity of microbial communities in soils (Sall et al., 2006). The microbial populations of bacteria, fungi, and actinomycetes were significantly improved in different horticulture land use systems under CPs over FPs (Table 5). The population of bacteria, fungi, and actinomycetes increased under all horticultural systems over control, with the mango- and aonla-based systems having the greatest population than others. Interestingly, topsoil has a substantially greater population of culturable microbes than sub-surface soil. In CPs, microsite improvement, mulching, and integrated nutrient management all together might have improved SOC and soil moisture regime, which served as a source of nutrients and supplied hydrogen and oxygen to the microorganisms, and it served as a solvent and carrier of other food nutrients to the microorganisms (Jat et al., 2023). Thus, soil moisture and SOC helped in improving the soil bacterial, fungal, and actinomycetes density. In turn, it might have influenced the nutrient dynamics to improve nutrient availability to fruit crops (Sahu et al., 2017). Soil microorganisms mineralize litter and facilitate the release of nutrient elements and their continual recycling (Kumar et al., 2019). Dead plant residues and plant nutrients become food for the microbes in the soil. The decomposition of SOM imparted energy for growth and provided carbon for the formation of new cells. In the process, N, P, and K were recycled and helped improve soil health (Smith, 2018).
Soil enzyme activity
Our study revealed that CPs had higher activity of AP, ACP, urease, and BGD over FPs both in surface and sub-surface soil depths (Table 6). In both soil layers, horticulture systems had considerably greater activities of C-cycling enzymes such as BGD. In both soil layers, horticulture systems based on mango and guava produced considerably greater levels of N and P cycling enzymes such as phosphatase and urease. Significantly higher activities of BGD, AP, ACP, and DHA under CPs might be due to greater SMBC and SOC over FPs. Mijangos et al. (2006) have reported that SMBC or SOC had a positive correlation (P <0.05) with soil enzyme activity in both soil layers. Mulching, microsite improvement, and INM in CPs contributed a good amount of litterfall, root biomass, and rhizodeposition and eventually enhanced carbon availability (Hu et al., 2011). Dodor and Tabatabai (2003) reported that SOC could stipulate AP and ACP activity. The presence of inositol phosphates and phospholipids in litters might have encouraged phosphatase activity. The decomposed part of the litterfall worked as the substrate for BGD and improved its activity (Ghosh et al., 2019).
Soil microbial biomass carbon
The SMBC significantly improved by different fruit-based land uses (Table 7). Rathore et al. (2021) also observed a higher soil microbial population in the upper layer of soil because of more SOC present in the layer. The results on SMBC values under CPs, FPs, and soil depths were significantly varied among various land uses. This indicates that mango followed by litchi had the highest SMBC values, whereas the lowest values of SMBC in peach were recorded under both CPs and FPs at two depths. The higher SMBC under CP as well as in the upper soil layer is due to differences in the root exudates of different plants, SOM content, litter yields, and microbial community composition as compared to the lower soil layer of degraded lands (Ghosh et al., 2019). The SMBC values decreased with increasing soil depth, which is in line with the fact that microbial biomass is highest in the topsoil layers, where the SOM content is highest. Additionally, the difference in SMBC values between CPs and FPs is more pronounced in the 0–15 cm soil layer than in the 15–30 cm soil layer. This finding suggests that CPs may have a more significant impact on microbial biomass in deeper soil layers. Additionally, better soil structure, stabilized microclimate, and higher nutrient recycling potential of CPs might have also enhanced SOC and SMBC (Moore et al., 2003).
The horticultural land use systems enhanced the SMBC because of the addition of leaf litter and root exudates, which increased SOM and created favorable conditions for microbial growth. The degradation of organic substances provides both energies for growth and carbon for the creation of new cells of soil microbial bacteria. SMBC and SOC in fruit-based land use systems had grown in the degraded land, with CP accumulating higher SMBC and SOC than farmer practice. Verma et al. (2010) also reported that conservation practice improved SOC in apple orchards with continuous application of farm yard manure. Furthermore, the difference between SMBC values under CPs and FPs is more prominent in the 15–30 cm soil layer than in the 0–15 cm soil layer, suggesting that conservation practices have a more significant impact on the microbial biomass in deeper soil layers. Finally, the fallow land use has the lowest SMBC values under both CPs and FPs, with a more considerable difference between the two practices in the 0–15 cm soil layer than in the 15–30 cm soil layer. Overall, the data highlight the positive impact of conservation practice on SMBC, which varies among different land uses and soil depths.
Canopy spread, fruit yield, litter yield, and soil moisture
The growth and fruit production have been significantly varied under different fruit-based land uses (Table 8). Among the fruit species planted with CPs and FPs, canopy spread was positively correlated with fruit yield (r = 0.95) and litter yield (r = 0.92). This indicated that higher plant spread would capture more sunlight and convert more solar energy into food material (photosynthates) for the production of more fruit yield under both situations on degraded lands. The mean canopy spread was recorded higher under CPs in mango followed by litchi, aonla, citrus, and peach as compared to FPs. Mango attained maximum canopy spread followed by litchi and aonla among all other fruit species because of its very deep rooting pattern and ability to draw more nutrients and moisture from deeper soil layer, which supplied the required amount of moisture along with nutrients to the plant under CPs as compared to FPs. Similarly, among the fruit species established with CPs, the mango produced higher MEFY, litter yield, and soil moisture than FPs on degraded lands, followed by litchi, peach, aonla, and citrus. Mango produced more canopy spread and fruit yield as compared to all other fruit species with CPs due to the mulching effect and its suitability to edapho-climatic conditions, which favored attaining more canopy spread, fruit yield, and soil moisture (Chavan et al., 2023). Melia dubia + dragon fruit and Melia dubia + lemon grass cultivation along with soil moisture conservation practices has resulted in better fruit yield of dragon fruit and biomass yield of Melia dubia and lemon grass compared to control besides conservation of soil and water in Mahi ravines of Central Gujarat (Jinger et al., 2020, 2021; Kakade et al., 2020).
Economic viability
The economic profitability was assessed in different fruit-based land uses (Table 9). Benefit–cost analysis of horticultural land use systems (mango, litchi, peach, aonla, and citrus) established with CPs and FPs for 20 years of life span indicated that fruit species established with CPs were more economically viable and profitable than fruit species planted under FPs on degraded land (Table 7). The NPVs of 900,032, 654,524, 504,464, 604,832, and 487,982 Rs ha−1 were observed in mango, litchi, aonla, peach, and citrus land use systems, respectively, planted with CP, whereas these NPVs were lower among fruit species planted in FP analyzed for 20 years of lifespan. Among horticultural land use systems, approximately 37.51–84.43% and 30.44–65.56% higher NPVs were observed in mango land use under CPs and FPs, respectively, over other land use systems. Similarly, the BCR of horticultural land use systems was maximum in mango, followed by litchi, aonla, peach, and lowest in citrus under CPs and FPs, respectively. The PBP observed in different horticultural land use systems planted with FPs and CPs was recorded minimum in mango followed by peach, citrus, and litchi and highest PBP in aonla for 20 years. A comparison of the horticultural land use systems under CPs with FPs indicated that NPV of horticultural land use systems with CP ranged from Rs 487,982 to 900032 ha−1, which were more profitable than FP (366,786–607,202 Rs ha−1) calculated for 20 years of lifespan. The benefit analysis of various fruit species for 20 years was observed beneficial, which indicated that practicing INM, organic mulching, and allowing natural grasses in different fruit species provided more economic benefits than without INM and organic mulching. Moreover, the fruit yield of various fruit species under CP was higher because of more canopy spread, the availability of more soil moisture in the soil profile helped in attaining more vegetative growth, and the adsorption of more moisture along with nutrients from deeper layers of soil helped in more fruit production under degraded land condition (Chavan et al., 2022). Between the two practices (CP and FP), fruit species established with CPs were the most profitable (487,982–900,032 Rs ha−1) in 20 years, and the difference of their respective NPVs was significantly 33.06–48.22% more of different fruit species over clean cultivation. Overall, fruit species established with CPs were a higher discounted profit earner over FPs. Thus, fruit species established with CPs observed higher BCRs than those established with FPs. In terms of PBP, fruit species established with CPs had the shortest and fruit species with FPs the longest (5.5–7.0 years). Thus, the utilization of inter-tree space by suitable crops can provide extra returns, leading to higher economic benefits even in a shorter period than sole litchi (Rathore et al., 2014).
Ecosystem services
The carbon sequestration in trees and soil under conservation and farmers' practices have been assessed in horticultural land use systems (Table 11). It is observed that additional monetary benefits accrued due to the adoption of CP over FP vary between 8.33% and 15.94% for carbon sequestration. This comparison highlights the advantage of adopting conservation practices in terms of enhanced carbon sequestration and the associated economic benefits. Similarly, the highest additional benefits due to the adoption of conservation practices are observed (114.2%) in the aonla-based horticultural land use system, whereas in the other systems, additional benefits vary between 10.5% and 28.1%. This can be inferred that conservation practices augment the nutrient content of soil and reduce the requirement for external fertilizer application (Table 12). The monetary quantification of the total ecosystem services (regulating service + supporting service) in different fruit-based land uses with conservation and farmers' practices has been computed, which revealed that the highest monetary value of total ecosystem services (56,907 Rs ha−1) assessed was in mango-based land use system among all land uses with CPs (Table 13). The total ecosystem services of mango under conservation practice is approximately 12.8% more than mango-based land use established with farmer practice (Pandey et al., 2021). By adopting CPs, farmers can gain additional benefits in the form of ecosystem services varying from 9.6% to 16.4% in different horticultural land use systems. The conservation practice used in fruit-based land uses attained more tree biomass and produced higher fruit yield, which realized more returns ha−1 as compared to farmer practice due to higher moisture availability and nutrition supplied through integrated nutrient management. Additionally, fruit-based land uses contributed to more litterfall into the soil, increasing porosity and preserving more soil moisture, which improved the vegetative growth of fruit trees (Kumar et al., 2018; Rathore et al., 2020). The higher ecosystem services or benefits of fruit trees have also been reported in the literature (Orlandi et al., 2023).
Conclusion
The study compared existing farmers with conservation practices based on deep-rooted fruit species in terms of the chemical and biological characteristics of the soil, including the organic carbon, N, and P contents, enzyme activities, and microbial population, as well as economic analyses. The results of the analysis of variance revealed that most of the parameters studied were significantly affected by the CPs and horticultural land use systems. The SOC and available N, P, and K contents were significantly improved in the CPs adopted in horticultural land use systems. The results showed that the CPs improved the soil enzymatic properties holistically when applied compared to FPs. Furthermore, the horticultural systems substantially increased the enzymatic activity and microbial population of bacteria, fungi, and actinomycetes in the soil. Moreover, SYI of mango- and aonla-based horticulture systems showed notable differences from the others due to higher catabolic diversity and led to increased soil functional diversity. Among the horticultural systems, the canopy spread, fruit, and litter yield was recorded highest in mango, followed by litchi. A similar trend was also observed for NPV, BCR, and PBP. Overall, the study provides evidence that CPs, such as the adoption of horticultural land use systems, have a positive effect and lead to improved SOC, nutrient availability, enzymatic activity, microbial population, yield, and BCR.
Constraints of the study area
The investigation site is situated in the North-Western Himalayas. The soil of the study site is prone to land degradation (soil erosion) owing to steep slopes. Cultivation of arable crops is very difficult due to high boulder content, low infiltration rate, and poor soil organic matter, leading to crop failure. Moreover, establishing fruit trees on these lands is also difficult as the root growth is seriously affected. However, CPs played a crucial role in enhancing soil fertility, survival, growth, and yield of the crops. Furthermore, wild animals also cause damage to food crops and fruit trees. However, spiny bamboo fencing reduced the damage caused by the wild animal.
Data availability statement
The data analyzed in this study is subject to the following licenses/restrictions: Data set will be made available on request. Requests to access these datasets should be directed to AR, cmF0aG9yZWFjQGdtYWlsLmNvbQ==.
Author contributions
AR: Conceptualization, Investigation, Methodology, Writing—original draft, Writing—review & editing. CS: Writing—original draft, Writing—review & editing. JJ: Investigation, Writing—review & editing. AGu: Methodology, Writing—original draft. VD: Conceptualization, Methodology, Writing—original draft. DJ: Formal analysis, Investigation, Methodology, Writing—review & editing. DS: Formal analysis, Methodology, Writing—original draft. DY: Formal analysis, Conceptualization, Writing—review & editing. AB: Methodology, Writing—review & editing. SI: Formal analysis, Writing—review & editing. AGh: Formal analysis, Methodology, Supervision, Writing—original draft. DK: Formal analysis, Investigation, Supervision, Writing—review & editing. VP: Formal analysis, Investigation, Methodology, Writing—review & editing. AJ: Formal analysis, Supervision, Writing—review & editing. VS: Investigation, Methodology, Writing—review & editing. RP: Investigation, Methodology, Writing—review & editing. MM: Writing—review & editing.
Funding
The author(s) declare that no financial support was received for the research, authorship, and/or publication of this article.
Conflict of interest
The authors declare that the research was conducted in the absence of any commercial or financial relationships that could be construed as a potential conflict of interest.
The handling editor SC declared a past authorship with one of the authors DJ.
Publisher's note
All claims expressed in this article are solely those of the authors and do not necessarily represent those of their affiliated organizations, or those of the publisher, the editors and the reviewers. Any product that may be evaluated in this article, or claim that may be made by its manufacturer, is not guaranteed or endorsed by the publisher.
References
Bending, G. D., Turner, M. K., and Jones, J. E. (2002). Interactions between crop residue and soil organic matter quality and the functional diversity of soil microbial communities. Soil Biol. Biochem. 34, 1073–1082. doi: 10.3390/w14162471
Bremner, J. M., and Keeney, D. R. (1965). Steam distillation methods for determination of ammonium, nitrate and nitrite. Anal. Chim. Acta 32, 485–495. doi: 10.1016/S0003-2670(00)88973-4
Chakrabarti, K., Sinha, N., Chakraborty, A., and Bhattacharyya, P. (2004). Influence of soil properties on urease activity under different agro-ecosystems. Arch. Agron. Soil Sci. 50, 477–483. doi: 10.1080/03650340410001692998
Chang, E. H., Chung, R. S., and Tsai, Y. H. (2007). Effect of different application rates of organic fertilizer on soil enzyme activity and microbial population. Soil Sci. Plant Nutr. 53, 132–140. doi: 10.1111/j.1747-0765.2007.00122.x
Chatterjee, D., Kuotsu, R., Ray, S. K., Patra, M. K., Thirugnanavel, A., Kumar, R., et al. (2021). Preventing soil degradation in shifting cultivation using integrated farming system models. Arch. Agron. Soil Sci. 68, 1841–1857. doi: 10.1080/03650340.2021.1937139
Chavan, S. B., Dhillon, R. S., Sirohi, C., Keerthika, A., Kumari, S., Bharadwaj, K. K., et al. (2022). Enhancing farm income through boundary plantation of poplar (Populus deltoids): an Economic analysis. Sustainability 14, 8663. doi: 10.3390/su14148663
Chavan, S. B., Dhillon, R. S., Sirohi, C., Uthappa, A. R., Jinger, D., Jatav, H. S., et al. (2023). Carbon sequestration potential of commercial agroforestry systems in indo-gangetic plains of india: poplar and eucalyptus-based agroforestry systems. Forests 14, 559. doi: 10.3390/f14030559
Chen, C., Liu, W., Wu, J., Jiang, X., and Zhu, X. (2019). Can intercropping with the cash crop help improve the soil physico-chemical properties of rubber plantations? Geoderma 335, 149–160. doi: 10.1016/j.geoderma.2018.08.023
DFPD (2015). Department of Food and Public Distribution, Ministry of Consumer Affairs, food and Public Distribution, Government of India, New Delhi. Available online at: https://dfpd.gov.in/minimum-support-prices.htm (accessed September 15, 2023).
Dhyani, S., Santhanam, H., Dasgupta, R., Bhaskar, D., Murthy, I. K., and Singh, K. (2023). Exploring synergies between India's climate change and land degradation targets: Lessons from the Glasgow Climate COP. Land Degrad. Dev. 34, 196–206. doi: 10.1002/ldr.4452
Dodor, D. E., and Tabatabai, M. A. (2003). Effect of cropping systems on phosphatases in soils. J. Plant Nutr. Soil Sci. 166, 7–13. doi: 10.1002/jpln.200390016
Eivazi, F., and Tabatabai, M. A. (1977). Phosphatases in soils. Soil Biol. Biochem. 9, 167–172. doi: 10.1016/0038-0717(77)90070-0
Eivazi, F., and Tabatabai, M. A. (1988). Glucosidases and galactosidases in soils. Soil Biol. Biochem. 20, 601–606. doi: 10.1016/0038-0717(88)90141-1
El-Swaify, S. A., and Cooley, K. R. (1981). “Soil loss from sugarcane and pineapple lands in Hawaii” in Assessment of Erosion, eds. De Boodt, M., and Gabriels, D. Kussow (New York: Wiley), 327–340.
El-Swaify, S. A., and Dangler, E. W. (1982). “Rainfall erosion in the tropic– A state-of- the art” in Soil Erosion and Conservation in the Tropics, eds. W. Kussow (New York: Wiley), 1–25. doi: 10.2134/asaspecpub43.c1
García-Ruiz, R., Ochoa, V., Hinojosa, M. B., and Carreira, J. A. (2008). Suitability of enzyme activities for the monitoring of soil quality improvement in organic agricultural systems. Soil Biol. Biochem. 40, 2137–2145. doi: 10.1016/j.soilbio.2008.03.023
Ghosh, A., Bhattacharyya, R., Dwivedi, B. S., Meena, M. C., Agarwal, B. K., Mahapatra, P., et al. (2016). Temperature sensitivity of soil organic carbon decomposition as affected by long-term fertilization under a soybean based cropping system in a sub-tropical Alfisol. Agric., Ecosyst. Environ. 233, 202–213. doi: 10.1016/j.agee.2016.09.010
Ghosh, A., Kumar, S., Manna, M. C., Singh, A. K., Sharma, P., Sarkar, A., et al. (2019). Long-term in situ moisture conservation in horti-pasture system improves biological health of degraded land. J. Environ. Manag. 248, 109339. doi: 10.1016/j.jenvman.2019.109339
Ghosh, A., Singh, A. K., Das, B., Modak, K., Kumar, R. V., Kumar, S., et al. (2023). Resiliencies of soil phosphorus fractions after natural summer fire are governed by microbial activity and cation availability in a semi-arid Inceptisol. Environ. Res. 216, 114583. doi: 10.1016/j.envres.2022.114583
Ghosh, A., Singh, A. B., Kumar, R. V., Manna, M. C., Bhattacharyya, R., Rahman, M. M., Sharma, P., et al. (2020). Soil enzymes and microbial elemental stoichiometry as bio-indicators of soil quality in diverse cropping systems and nutrient management practices of Indian Vertisols. Appl. Soil Ecol. 145, 103304. doi: 10.1016/j.apsoil.2019.06.007
GOI (2021). Report of the NCAVES project. Ministry of Statistics and Programme Implementation. New Delhi: Government of India
Gulati, A., and Rai, S. C. (2014). Cost estimation of soil erosion and nutrient loss from a watershed of the Chotanagpur Plateau, India. Curr. Sci. 107, 670–674.
Gupta, A. K., Kumar, P., Rathore, A. C., Kumar, P., Kaushal, R., Islam, S., et al. (2021a). Soil and water conservation techniques based land degradation neutrality: a need-based solution for degraded lands in Indian perspective. Curr. Sci. 121, 1343–1347 doi: 10.18520/cs/v121/i10/1343-1347
Gupta, A. K., Tomar, J. M. S., Kaushal, R., Kadam, D. M., Rathore, A. C., Mehta, H., et al. (2021b). Aromatic plants based environmental sustainability with special reference to degraded land management. J. App. Res. Med. Aromat. Plants 22, 100298. doi: 10.1016/j.jarmap.2021.100298
Hamdy, A., and Aly, A. (2014). “Land degradation, agriculture productivity and food security” in Proceedings of Fifth International Scientific Agricultural Symposium; 2014 October 23–26; Jahorina, Bosnia and Herzegovina, 708–717.
Hanway, J. J., and Heidel, H. (1952). Soil analysis methods as used in Iowa state college soil testing laboratory. Iowa Agric. 57, 1–31.
Hu, J., Lin, X., Wang, J., Dai, J., Chen, R., Zhang, J., et al. (2011). Microbial functional diversity, metabolic quotient, and invertase activity of a sandy loam soil as affected by long-term application of organic amendment and mineral fertilizer. J. Soils Sediments. 11, 271–280. doi: 10.1007/s11368-010-0308-1
IPCC (2003). Good Practice Guidance for Land Use, Land-Use Change and Forestry; the Intergovernmental Panel on Climate Change, Hayama, Japan. Geneva: Intergovernmental Panel on Climate Change
Jat, R. A., Jain, N. K., Yadav, R. S., Reddy, K. K., Choudhary, R. R., Zala, P. V., et al. (2023). System-Based integrated nutrient management improves productivity, profitability, energy use efficiency and soil quality in peanut-wheat cropping sequence in light black soils. Sustainability 15, 1361. doi: 10.3390/su15021361
Jinger, D., Kakade, V. D., Kaushal, R., Dinesh, D., Bhatnagar, P. R., and Singhal, V. (2021). Rehabilitation of degraded lands through different planting techniques in Melia dubia Cav. Food Sci. Rep. 2, 7−9.
Jinger, D., Kakade, V. D., Singhal, V., and Dinesh, D. (2020). Lemon grass: a lucrative crop for degraded lands of Central Gujarat. Agric. Environ. 1, 26–29.
Jinger, D., Kaushal, R., Kumar, R., Paramesh, V., Verma, A., Shukla, M., et al. (2023). Degraded land rehabilitation through agroforestry in India: Achievements, current understanding, and future prospectives. Front. Ecol. Evol. 11, 1088796. doi: 10.3389/fevo.2023.1088796
Jinger, D., Kumar, R., Kakade, V., Dinesh, D., Singh, G., Pande, V. C., et al. (2022). Agroforestry system for controlling soil erosion and enhancing system productivity in ravine lands of Western India under climate change scenarios. Environ. Monit. Assess. 194, 267. doi: 10.1007/s10661-022-09910-z
Kakade, V. D., Jinger, D., Dayal, V., Chavan, S., Nangare, D. D., Wakchaure, G. C., et al. (2020). Dragon fruit: wholesome and remunerative fruit crop for India. Food Sci. Rep. 1, 44–48.
Kumar, D., Srivastava, K. K., Singh, S. R., and Rathore, A. C. (2018). Fruit production and carbon sequestration potential of rainfed apple (Malus x domestica Borkh) under various moisture conservation techniques. Indian J. Soil Conser. 46, 109-117.
Kumar, S., Singh, A. K., Singh, R., Ghosh, A., Chaudhary, M., Shukla, A. K., et al. (2019). Degraded land restoration ecological way through horti-pasture systems and soil moisture conservation to sustain productive economic viability. Land Degrad. Dev. 30, 1516–1529. doi: 10.1002/ldr.3340
Lal, R. (2015). Restoring soil quality to mitigate soil degradation. Sustainability 7, 5875–5895. doi: 10.3390/su7055875
Lenka, N. K., Sudhishri, S., Dass, A., Choudhury, P., Lenka, S., and Patnaik, U. (2013). Soil carbon sequestration as affected by slope aspect under restoration treatments of a degraded alfisol in the Indian sub-tropics. Geoderma 204, 102–110. doi: 10.1016/j.geoderma.2013.04.009
Mekuria, W., Veldkamp, E., Tilahun, M., and Olschewski, R. (2011). Economic valuation of land restoration: the case of exclosures established on communal grazing lands in Tigray, Ethiopia. Land Degrad. Dev. 22, 334–344 doi: 10.1002/ldr.1001
Mijangos, I., Perez, R., Albizu, I., and Garbisu, C. (2006). Effects of fertilization and tillage on soil biological parameters. Enzym. Microb. Technol. 40, 100–106. doi: 10.1016/j.enzmictec.2005.10.043
MOEFCC (2015). India's Intended Nationally Determined Contribution: Working Towards Climate Justice. New Delhi: Ministry of Environment, Forests and Climate Change.
Moore, J. C., McCann, K., Setala, H., and de Ruiter, P. C. (2003). Top-down is bottom-up: does predation in the rhizosphere regulate aboveground dynamics? Ecology 84, 846–857. doi: 10.1890/0012-9658(2003)0840846:TIBDPI2.0.CO;2
NHB (2015). Indian Horticulture Database, Ministry of Agriculture and Farmer welfare, Government of India, Gurgaon: National Horticulture Board. Available online at: http://www.nhb.gov.in (accessed September 15, 2023).
NHB (2021). Indian Horticulture Database, Ministry of Agriculture and Farmer welfare, Government of India. Gurgaon: National Horticulture Board. Available at: http://www.nhb.gov.in (accessed April 10, 2022).
Olsen, S. R., Cole, C. V., Watanabe, F. S., and Dean, L. A. (1954). Estimation of Available Phosphorus in Soils by Extraction with Sodium Bicarbonate. Washington, DC: US Dept. Agric. Circ.
Orlandi, F., Marrapodi, S., Chiara Proietti, C., Ruga, L., and Fornaciari, M. (2023). Ecosystem functions of fruit woody species in an urban environment. Environ. Monit. Assess 195, 118. doi: 10.1007/s10661-022-10717-1
Pandey, V. C., Bhatnagar, P. R., Jinger, D., Kumar, R., and Kumar, G (2021). Ecosystem services from ravine agro-ecosystem and its management-Farmers' perceptions. Curr. Sci. 121 (10):1352-1357. doi: 10.18520/cs/v121/i10/1352-1357
Paul, J., Choudhary, A. K., Sharma, S., Bohra, M., Dixit, A., and Kumar, P. (2016). Potato production through bio-resources: long-term effects on tuber productivity, quality, carbon sequestration and soil health in temperate Himalayas. Sci. Hortic. 213, 152–163. doi: 10.1016/j.scienta.2016.10.022
Paz-Ferreiro, J., Gascó, G., Gutiérrez, B., and Méndez, A. (2012). Soil biochemical activities and the geometric mean of enzyme activities after application of sewage sludge and sewage sludge biochar to soil. Biol. Fertil. Soils 48, 511–517. doi: 10.1007/s00374-011-0644-3
Paz-Ferreiro, J., Trasar-Cepeda, C., del Carmen Leirós, M., Seoane, S., and Gil-Sotres, F. (2011). Intra-annual variation in biochemical properties and the biochemical equilibrium of different grassland soils under contrasting management and climate. Biol. Fertil. Soils 47, 633–645. doi: 10.1007/s00374-011-0570-4
Rathore, A. C., Kumar, A., Tomar, J. M. S., Jayaprakash, J., Mehta, H., Kaushal, R., et al. (2018). Predictive models for biomass and carbon stock estimation in Psidium guajava on bouldery riverbed lands in North-Western Himalayas, India. Agrofor. Syst. 92, 171–182. doi: 10.1007/s10457-016-0023-z
Rathore, A. C., Lal, H., Sharma, N. K., Jayaprakash, J., Mehta, H., and Chaturvedi, O. P. (2014). Livelihood security through Litchi (Litchi chinensis L.)-based agri-horticultural models for resource-poor communities of Indian Sub-Himalaya. Curr. Sci. 106, 1481–1484.
Rathore, A. C., Mehta, H., Islam, S., Saroj, P. L., Sharma, N. K., Gupta, A. K., et al. (2021). Biomass, carbon stocks estimation and predictive modelling in mango based land use on degraded lands in Indian Sub-Himalayas. Agrofor. Syst. 95, 1563–1575. doi: 10.1007/s10457-021-00660-4
Rathore, A. C., Mehta, H., Sharma, N. K., Gupta, A. K., Alam, N. M., Islam, S., et al. (2020). Performance of litchi (Litchi chinensis Sonn.) based agri-horticultural land uses in rainfed condition on degraded lands in North Western Himalayas, India. Agrofor. Syst. 94, 2225–2236. doi: 10.1007/s10457-020-00544-z
Reynolds, S. G. (1970). The gravimetric method of soil moisture determination Part I A study of equipment, and methodological problems. J. Hydrol. 11, 258–273. doi: 10.1016/0022-1694(70)90066-1
Ricke, K., Drouet, L., Caldeira, K., and Tavoni, M. (2018). Country-level social cost of carbon. Nature Climate Chang. 8, 895–900 doi: 10.1038/s41558-018-0282-y
Rolf, A. O., and Bakken, L.R. (1987). Viability of soil bacteria: optimization of plate counting technique and comparison between total counts and plate counts within different size groups. Microb. Ecol. 13, 59–74. doi: 10.1007/BF02014963
Sahu, N., Vasu, D., Sahu, A., Lal, N., and Singh, S.K. (2017). “Strength of microbes in nutrient cycling: a key to soil health,” in Agriculturally Important Microbes for Sustainable Agriculture (Singapore: Springer), 69–86.
Sall, S. N., Masse, D., Ndour, N. Y. B., and Chotte, J. L. (2006). Does cropping modify the decomposition function and the diversity of the soil microbial community of tropical fallow soil? Appl. Soil Ecol. 31, 211–219. doi: 10.1016/j.apsoil.2005.05.007
Saroj, P.L., Tomar, D. S., and Arora, Y. K. (2000). Peach based Agroforestry Systems in degraded foothills of north-western Himalayan region. J. Appl. Hort. 2, 21–24. doi: 10.37855/jah.2000.v02i01.06
Sepehri, A., and Sarrafzadeh, M. H. (2018). Effect of nitrifiers community on fouling mitigation and nitrification efficiency in a membrane bioreactor. Chem. Eng. Process 128, 10–18. doi: 10.1016/j.cep.2018.04.006
Sheoran, O. P., Tonk, D. S., Kaushik, L. S., Hasija, R. C., and Pannu, R. S. (1989). “Statistical software package for agricultural research workers” in Recent Advances in Information Theory. eds. D. S. Hooda and R.C. Hasija (Hisar: Chaudhary Charan Singh Hisar Agricultural University), 139–143.
Singh, D., Mishra, A. K., Patra, S., Mariappan, S., and Singh, N. (2021). Near-saturated soil hydraulic conductivity and pore characteristics as influenced by conventional and conservation tillage practices in North-West Himalayan region, India. Int. Soil Water Conserv. Res. 9, 249–259. doi: 10.1016/j.iswcr.2021.01.001
Singh, D., Mishra, A. K., Patra, S., Mariappan, S., Singh, N., and Kar, S.K. (2023). Spatial variability of soil hydraulic and physical properties in erosive sloping agricultural fields. Water Sci. Eng. 39, 590–91. doi: 10.1016/j.wse.2022.10.001
Singh, D., Patra, S., Mishra, A. K., Mariappan, S., and Singh, N. (2022). Temporal variation of saturated and near-saturated soil hydraulic conductivity and water-conducting macroporosity in a maize-wheat rotation under conventional and conservation tillage practices. Land Degrad. Dev. 33, 2208–19. doi: 10.1002/ldr.4251
Smith, J. L. (2018). “Cycling of nitrogen through microbial activity”. in Soil Biology: Effect on Soil Quality. (Boca Raton, Florida: CRC Press), 97–126.
Tabatabai, M. A., and Bremner, J. M. (1969). Use of p-nitrophenyl phosphate for assay of soil phosphatase activity. Soil Biol. Biochem. 1, 301–307. doi: 10.1016/0038-0717(69)90012-1
Vance, E. D., Brookes, P. C., and Jenkinson, D. S. (1987). An extraction method for measuring soil microbial biomass C. Soil Biol. Biochem. 19, 703–707. doi: 10.1016/0038-0717(87)90052-6
Verma, M. L., Rakesh, S., Charan, S., and Rathore, A. C. (2010). Influence of organic manuring on apple performance and soil properties in temperate zone of Himachal Pradesh. Indian J.Soil Conserv. 38, 212–216.
Walkley, A., and Black, I. A. (1934). An examination of the Degtjareff method for determining soil organic matter, and a proposed modification of the chromic acid titration method. Soil Sci. 37, 29–38. doi: 10.1097/00010694-193401000-00003
Wilson, M. A., and Carpenter, S. R. (1999). Economic valuation of freshwater services in the United States: 1971–1997. Ecol. Appl. 9, 772–783. doi: 10.1890/1051-0761(1999)009[0772:EVOFES]2.0.CO;2
Yadav, G. S., Das, A., Lal, R., Babu, S., Meena, R. S., Saha, P., et al. (2018). Energy budget and carbon footprint in a no-till and mulch based rice–mustard cropping system. J. Clean. Prod. 191, 144–157. doi: 10.1016/j.jclepro.2018.04.173
Keywords: fruit yield, land degradation, rehabilitation, soil organic carbon, soil quality index
Citation: Rathore AC, Singh C, Jayaprakash J, Gupta AK, Doharey VK, Jinger D, Singh D, Yadav D, Barh A, Islam S, Ghosh A, Kadam D, Paramesh V, Jhajhria A, Singhal V, Pal R and Madhu M (2023) Impact of conservation practices on soil quality and ecosystem services under diverse horticulture land use system. Front. For. Glob. Change 6:1289325. doi: 10.3389/ffgc.2023.1289325
Received: 05 September 2023; Accepted: 26 October 2023;
Published: 30 November 2023.
Edited by:
Sangram Bhanudas Chavan, National Institute of Abiotic Stress Management (ICAR), IndiaReviewed by:
Gopal Shankar Singh, Banaras Hindu University, IndiaKeerthika Arumugam, Central Arid Zone Research Institute (ICAR), India
Copyright © 2023 Rathore, Singh, Jayaprakash, Gupta, Doharey, Jinger, Singh, Yadav, Barh, Islam, Ghosh, Kadam, Paramesh, Jhajhria, Singhal, Pal and Madhu. This is an open-access article distributed under the terms of the Creative Commons Attribution License (CC BY). The use, distribution or reproduction in other forums is permitted, provided the original author(s) and the copyright owner(s) are credited and that the original publication in this journal is cited, in accordance with accepted academic practice. No use, distribution or reproduction is permitted which does not comply with these terms.
*Correspondence: Avinash Chandra Rathore, cmF0aG90cmVhY0BnbWFpbC5jb20=