- Smithsonian Tropical Research Institute, Balboa, Ancón, Panama
Bark conductance to water vapor (gbark) is an important determinant of drought tolerance in tropical plants. Examining species differences in bark conductance can provide useful information about the resilience of tropical trees to ongoing climate change. Values of gbark are positively related to stem photosynthetic rate in desert species, showing that increased stem photosynthesis capacity is associated with increased bark conductance to water vapor. We determined whether stem morphometric traits, bioclimatic variables (e.g., temperature and precipitation), and shared evolutionary history help explain variation in gbark among tropical plant species. We surveyed 94 species (90 trees and 4 liana species) from lowland and mid-elevation forests in Panama and estimated gbark, stem diameter, specific stem area, bark thickness, sapwood area, and bark and wood density. Climate data were extracted from CHELSA 2.1 and Instituto de Meteorología e Hidrología de Panamá. Phylogenetic signal was estimated using Blomberg’s K statistic and Pagel’s λ. Bark conductance decreased with an increase in bark thickness and relative bark thickness, and was positively related to mean annual precipitation and mean annual temperature. We also determined the temperature response of gbark of six plant species (five trees and one liana) from a lowland forest. In all six species, gbark decreased as air temperature increased from 20 to 50°C. There was a significant phylogenetic signal in gbark, with closely related species resembling each other more than distantly related species. We conclude that interspecific differences in gbark of Neotropical tree species depend on all three factors studied: stem morphometry, climate, and evolutionary history.
Introduction
Water is the most limiting resource to plant productivity in many terrestrial ecosystems (Schulze et al., 1987; Chaves and Pereira, 1992). With anthropogenic climate change, we expect alterations in water availability in many regions of the world (IPCC, 2023), even in currently mesic ecosystems. The way plants acquire, use, and conserve water is important for their survival, especially in seasonally dry ecosystems. For some time, leaf minimum conductance (gmin, leaf) has been considered the last barrier to water lost in plants experiencing drought. For example, it has been proposed that when all other drought survival traits are exhausted, the rate at which water is lost from the leaves operating at minimum conductance should determine when leaves and plants die (Santiago et al., 2016). However, some plants lose their leaves when faced with water deficit stress (e.g., drought-deciduous species), and many species are able to hydraulically isolate themselves from drying soil by root shrinkage (Nobel and Cui, 1992). Furthermore, the outer surface of stems can continue transpiring as it is a semi-permeable barrier to water vapor, CO2, and O2 movement, and the rate of water loss through this outer bark may ultimately determine mortality. The bark is typically defined as all the tissues outside of the vascular cambium of trees, shrubs, and lianas and includes secondary and primary phloem, cortex, and the periderm, which is made up of phelloderm, phellogen (cork cambium), and phellem (cork) (Angyalossy et al., 2016). The periderm and rhytidome (successive development of periderms) are also called outer bark (Angyalossy et al., 2016). Wolfe (2020) showed that bark conductance (gbark) is related to drought performance in Neotropical trees, as stem water deficit and mortality during drought were positively associated with gbark in tree saplings growing in a greenhouse in central Panama.
The rate at which leaves desiccate after stomatal closure (gmin, leaf) has been of interest in recent years, with a meta-analysis showing no clear link between gmin, leaf and taxonomic associations, climate of origin, or leaf type, but rather that gmin, leaf was linked to growth conditions (Duursma et al., 2019). Less effort has focused on the minimum conductance of stem bark. Values of gbark are usually in the same order of magnitude as gmin, leaf (Ávila-Lovera et al., 2017, 2019); hence, the contribution of gbark to total plant water loss can be considerable, especially when leaves are absent. However, the lack of large data sets on gbark deters us from understanding the variability in gbark among plants and its contribution to whole-plant water loss. In this study, we seek to understand the drivers of gbark variation in Neotropical plant species.
Traits that determine gmin, leaf include the structure and composition of the cuticle. For example, the cutin matrix and the cuticular waxes (intra- and epicuticular) are known to influence gmin, leaf in desert plants (Bueno et al., 2019). In stem bark, multiple cells make up the limiting barrier for water movement, the multilayered periderm with live and dead tissues. Bark conductance was recently reviewed in the context of stem photosynthesis of woody plants (Berry et al., 2021), where the authors suggested that properties that make stem periderms more permeable to CO2 movement for photosynthesis also make periderms more permeable to water vapor movement. Indeed, two studies have linked the ability to perform stem photosynthesis with properties of the bark: either gbark in desert ecosystems (Ávila-Lovera et al., 2017) or outer bark (defined as the rhytidome or the phellem by the authors) thickness in species ranging from xerophytic shrublands to tropical rainforests (Rosell et al., 2015). A recent study in a Brazilian savanna showed that morphoanatomical traits of the outer bark influence variation in gbark, that high gbark is associated with a fast-resource-acquisitive strategy, and that the effect of gbark on whole-plant water balance depends on the hydraulic vulnerability of leaves (Loram-Lourenço et al., 2022). These studies highlight the need to include gbark in future studies of plant functional strategies.
Even though interest in understanding the cost of water loss through the bark has increased in recent years, we are still far from completely understanding the drivers of gbark variation among species from Neotropical forests. For example, climate of origin may influence gbark, as sites with lower precipitation, higher temperature, and drier atmospheres may select for species with low gbark. If communities are phylogenetically structured in these sites, the shared evolutionary history of species may also contribute to variation in gbark, as some families are known to share certain traits regarding their morphology and anatomy. For example, peeling outer bark is common in families such as Burseraceae and Myrtaceae. Furthermore, a recent review showed that plant-water relation traits that are considered to be structural were more likely to exhibit phylogenetic signal than physiological traits (Ávila-Lovera et al., 2023), suggesting that gbark could exhibit phylogenetic signal. This would indicate that similar drivers of gbark are acting similarly in closely related species.
In this study, we asked what are the factors that underlie the variation of gbark among Neotropical woody species. We tested whether stem morphometric traits, climate of origin, and/or shared evolutionary history play a role in explaining variation in gbark. We expected that increases in bark thickness or density would negatively influence gbark by increasing the path resistance for water movement. We also expected that species from warmer and drier sites, more seasonal sites, or sites with low precipitation in the driest month, would have lower gbark. Finally, we expected that there would be phylogenetic signals in gbark and other morphometric traits if common drivers of variation in gbark act similarly in closely related species. We also explored whether gbark would differ in different functional groups of differing leaf habits, as gbark has been previously found to be associated with different growth strategies.
Materials and methods
Study species and sites
We sampled 94 plant species from 27 families at six Neotropical sites in Central Panama. Since some species were present at more than one site, we have 131 species-at-site combinations (Supplementary Table S1). The sites included: (1) a seasonally dry secondary forest in Parque Natural Metropolitano (PNM), Panama City; (2) natural spaces in Panama City (PC); (3) a moist forest in Parque Nacional Soberanía (PNS), Gamboa; (4) a common garden in Gamboa (GCG); (5) a mid-elevation forest in Parque Nacional Altos de Campana (PNAC), Panama Oeste; and (6) a mid-elevation forest in Nueva Arenosa (NAR), Panama Oeste. More information about the study sites can be found in Table 1.
To test whether site climate influences gbark variation, we downloaded climate data (mean annual temperature and monthly precipitation and temperature) from CHELSA 2.1 (Karger et al., 2017, 2021). We also used mean annual precipitation (MAP) data from the Instituto de Meteorología e Hidrología de Panamá (IMHPA), which has meteorological stations in the country (data can be accessed).1 We were interested in testing the effect of mean annual temperature (MAT), MAP, the precipitation of the driest month, which was February at all sites, and precipitation seasonality, which we calculated from the monthly precipitation as the coefficient of variation.
Bark conductance measurements
During the dry season of 2021 from January to April, we sampled one to 10 individuals per species (mean = 3.81, median = 4, and SD = 1.55) from 94 plant species, for a total of 354 branches, to measure gbark following the bench dehydration and gravimetric method (Sack and Scoffoni, 2011). We collected 1 m long branches from each individual, placed them in a dark plastic bag with wet paper towels, and transported them to a laboratory in Gamboa, Panama. A 10-cm long segment was cut at 10 cm from the apex, i.e., a segment between 10 and 20 cm from the apex was excised. In most cases, these segments corresponded to stems that had both a secondary xylem and a thin periderm. In some species, for example, Lindackeria laurina (Achariaceae), Hirtella racemosa (Chrysobalanaceae), and Aiouea montana (Lauraceae), secondary xylem was present, but the epidermis with stomata had not yet been shed; hence, the stems were covered by an epidermis and not by a periderm. Delayed periderm formation is common in species with green photosynthetic stems (Gibson, 1983; Lindorf et al., 2006). Nonetheless, for all species studied, we use the term ‘bark conductance’ to describe the stem surface conductance to water vapor, as this term has been used consistently by others (Cernusak and Marshall, 2000; Wolfe, 2020; Loram-Lourenço et al., 2022). The cut ends were covered with two layers of melted candle wax and taped to a clothesline. When leaves were present, they were removed, and the wounds were covered with fast-drying glue (ethyl cyanoacrylate, Krazy Glue, Elmer’s Products, Westerville, OH, USA). Three fans were directed at the segments so that the segments slightly swayed in the breeze, and a thermohygrometer (ID-16S Casio, US) was placed close to the segments within the breeze (Supplementary Figure S1). The stem segments were allowed to dehydrate for ~1 h before starting recordings to ensure complete stomatal closure in those segments with stomata (most of the stem segments had lenticels instead). Once the hour had elapsed, stems were weighed at regular intervals using a balance (0.0001 g, H110, Sartorius, Göttingen, Germany), and air temperature and relative humidity (RH) were monitored. The stem segment fresh mass loss was plotted versus time, and the slope of the curve was used to estimate the transpiration rate standardized by stem surface area. Transpiration rate and the mole fraction air vapor pressure deficit [the driving force for gas phase diffusion (Jones, 1983)], calculated from air temperature and RH, were used to estimate gbark.
As in other studies using the gravimetric method to determine stem surface conductance (Wolfe, 2020; Loram-Lourenço et al., 2022; references therein), the internal air spaces of stems were assumed to be water-vapor saturated. We did not determine the water potential of stems under investigation. The reported midday minimum values of stem water potential for several species at two of our study sites were − 1.5 to −0.6 MPa (Meinzer et al., 2008). These values are expected to be closer to zero during the early morning hours when stems were sampled for the study presented here. Under our experimental conditions, the water potential of air was ca. –71 MPa (calculated for 28.2°C and 60% RH, see Supplementary Figure S1), vastly more negative than the likely water potential of stems. This suggests that the water potential of stems had little influence on the rate of stem water loss and that the stem-air vapor pressure difference was the principal driving force for stem water loss. However, it needs to be mentioned that the idea that internal spaces of leaves are always water-vapor saturated has recently been challenged (Buckley and Sack, 2019) and that in instances where unsaturation would occur, leaf conductance to water vapor transfer would be underestimated. Similar arguments could be made for gbark. Values of gbark are presented in Supplementary Table S1.
Bark conductance responses to temperature
After the field survey and given the potential role of air temperature on gbark, we ran temperature response curves for six species from PNS. The species were: Amaioua glomerulata (Lam. Ex Poir.) Delprete & C.H.Perss. (Rubiaceae), Coccoloba acuminata Kunth (Polygonaceae), Lindackeria laurina C.Presl (Achariaceae), Mabea occidentalis Benth. (Euphorbiaceae), Piper reticulatum L. (Piperaceae), and Serjania mexicana (L.) Willd. (Sapindaceae). These species were selected because of their size and abundance, ensuring that we could sample multiple stems from multiple individuals across various days. The temperatures used were: 20, 30, 35, 40, 45, and 50°C. Different stem segments from three individuals were used for each temperature. The gbark values at 20°C were obtained by following the protocol mentioned above in the laboratory at STRI’s Tupper Center in Panama City. The range of temperatures from 30 to 50°C was achieved using drying ovens (DKN816, Yamato Scientific America, USA).
Morphometric traits
From each stem segment used for gbark measurements, we determined the stem surface area and stem diameter using photographs analyzed with ImageJ (Abràmoff, 2004; Schneider et al., 2012). The following traits were measured in a subset of 61 species: bark thickness (mm) using a digital caliper (IP67, Mitutoyo, Aurora, IL, USA), by calculating the difference between whole stem diameter and wood diameter divided by two; relative bark thickness (%) as the ratio between stem bark thickness and stem radius multiplied by 100; sapwood area (cm2) by measuring the diameter of the wood to calculate the area of a circle and subtracting from it the pith area; bark density (g cm−3) and wood density (g cm−3) were estimated by calculating the volume of a cylinder using the diameter and length of a 1-cm long stem segment (wood) and the volume of a hollow cylinder (bark) and the dry mass of the wood and bark sections, respectively, after drying at 70°C for 72 h. From this 1-cm stem segment, we also estimated the ratio between stem surface area and stem bark dry mass (specific bark area, cm2 g−1) and the ratio between stem surface area and stem dry mass (specific stem area, cm2 g−1) (Cernusak and Marshall, 2000; Ávila-Lovera et al., 2017). We also calculated the ratio between sapwood area and stem surface area as an analog for the Huber value. The Huber value is the ratio of sapwood area to distal leaf area, i.e., the leaf area supplied by the sapwood area (Tyree and Zimmermann, 2002). For methodological reasons, bark thickness and bark density determined by us include the vascular cambium, which is difficult to separate from the phloem and the rest of the cortex in fresh samples.
Phylogenetic signal
We first built a phylogenetic tree using the ‘phylo.maker’ function in the ‘PhyloMaker’ R package (Jin and Qian, 2019). The tree had 94 species. We then estimated the phylogenetic signal of bark conductance and other morphometric traits as Blomberg’s K using the ‘phylosig’ function of the ‘phytools’ package (Revell, 2012), and Pagel’s λ by changing the method in the phylosig function to “lambda.” We also used the phylogenetic tree to map gbark onto it using the ‘contMap’ function of the ‘phytools’ package (Revell, 2012). All analyses were done in R v.4.3.0 (R Core Team, 2023).
Statistical analyses
Before performing statistical analyses, we removed outliers above 25 mmol m−2 s−1 as most gmin, leaf reported in the literature range from 0 to 25 (Duursma et al., 2019). These were five values above 30 mmol m−2 s−1, with two being between 55 and 60 mmol m−2 s−1, and one surpassing 80 mmol m−2 s−1. The outliers corresponded to few species-at-site means that did not affect the total number of species studied (94 species). These abnormally high values may have resulted from damage to the outer bark, incomplete sealing of leaf petiole wounds, or other causes not accounted for in this study.
Statistical analyses and plots were performed using R v.4.3.0 (R Core Team, 2023). We ran multiple linear mixed models using the function ‘lmer’ from the ‘lmerTest’ R package (Kuznetsova et al., 2017) to estimate the effect of morphometric traits or climatic variables on gbark, using species as a random effect (random intercept only) and the species-at-site means. For morphometric traits, we evaluated the significance of a model with all the traits as fixed factors and models with one trait at a time. We compared significant models using the ‘compare_performance’ function in the ‘performance’ package (Lüdecke et al., 2021) to select the best model based on r2 (conditional and marginal), intraclass correlation coefficient (ICC), root mean squared error (RMSE), Sigma, and Akaike information criterion (AIC), corrected AIC (AICc), and Bayesian information criterion (BIC) weights. The rank argument of the ‘compare_performance’ function provides a ranking of models based on normalizing all the indices (i.e., rescaling them to a range from 0 to 1) and taking the mean value of all indices for each model. We used this ranking to select the best model (Lüdecke et al., 2021). We made the same type of comparison for the models with climatic variables. Furthermore, for the climate models, we used log(gbark) instead of gbark because of heteroscedasticity in residual variance. Mixed models with one or multiple explanatory variables took the following form:
Linear models using temperature as a fixed factor were used to test the relationship between gbark and air temperature for the temperature response curves for each species.
Results
Across all species-at-site combinations, gbark varied from 0.94 to 24.60 mmol m−2 s−1 with a slightly skewed distribution toward low values (Supplementary Figure S2). Some families were more variable than others (F26,291 = 2.33, p = 3.85 × 10−4). For example, Anacardiaceae, Euphorbiaceae, Fabaceae, and Meliaceae showed the largest variation in gbark (Supplementary Figure S3). We found no difference in gbark between evergreen and deciduous species (F1,124 = 0.917, p = 0.340, Supplementary Figure S4).
From the morphometric traits measured and linear mixed models run, one was selected as the best model, a model with relative bark thickness as the fixed effect (conditional r2 = 0.784, p = 0.0002), with the highest gbark values at the lowest relative bark thickness (Figure 1A). Other models with relatively high ranking indicated that stem diameter and bark thickness were also related to gbark (stem diameter: conditional r2 = 0.535, p = 0.011; bark thickness: conditional r2 = 0.655, p = 0.040, Figure 1B), but these two traits are highly correlated (r = 0.623, p = 8.388 × 10−8). In the following, we primarily discuss relative bark thickness.
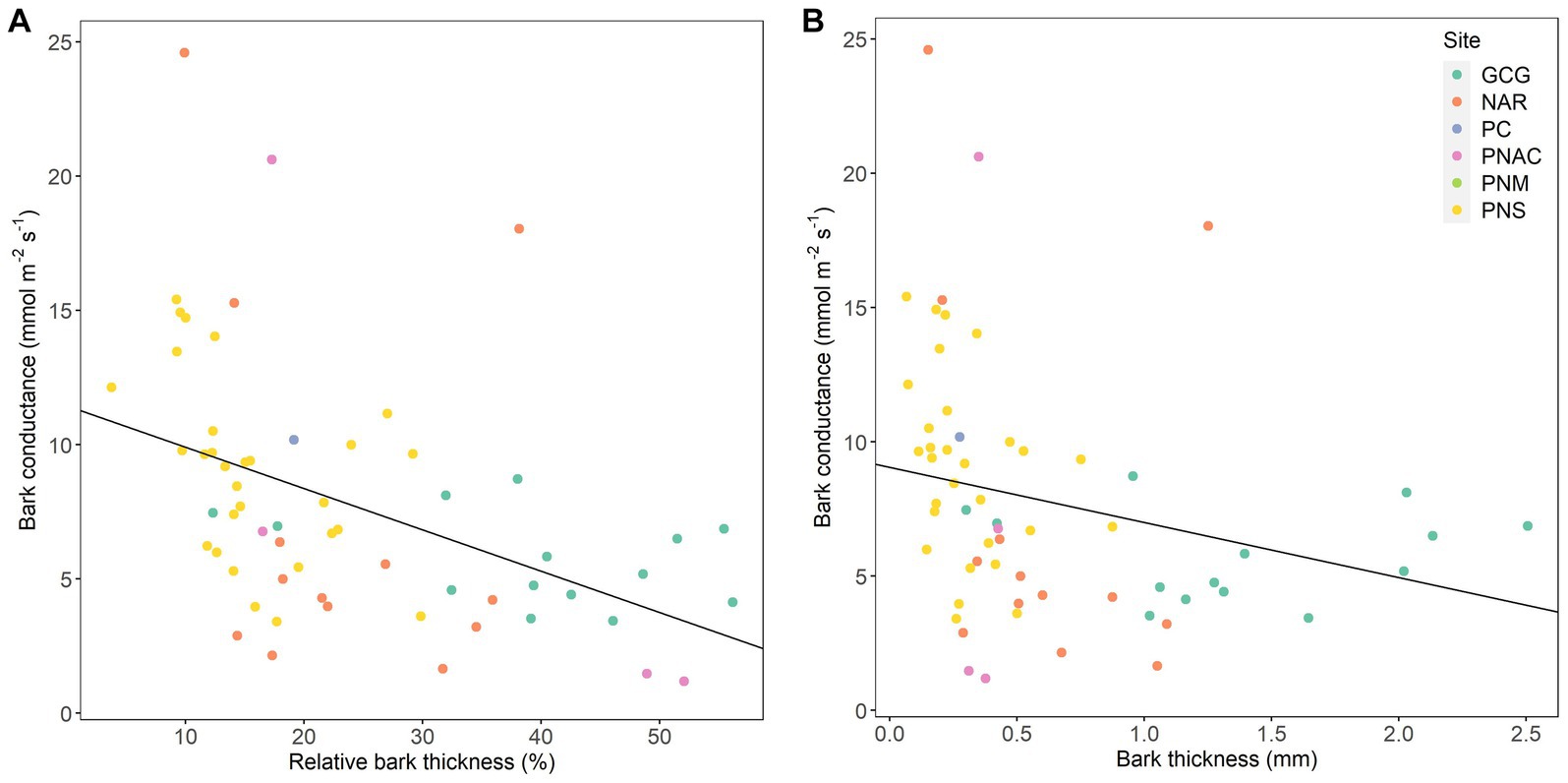
Figure 1. Bark conductance as a function of relative bark thickness (A) and bark thickness (B). Each point represents a species-at-site combination. Different colors indicate different forests: Gamboa Common Garden (GCG), Parque Nacional Soberanía (PNS), Parque Nacional Altos de Campana (PNAC), Nueva Arenosa (NAR), Panama City (PC), and Parque Natural Metropolitano (PNM). Regression lines are derived from a linear mixed model across all the data points ((A): conditional r2 = 0.784 p = 0.0002, (B): conditional r2 = 0.655, p = 0.040).
Bark conductance was also highly influenced by site, MAP and MAT (Figure 2). Values of log(gbark) increased with both MAP (conditional r2 = 0.391, p = 0.008) and MAT (conditional r2 = 0.440, p = 0.002), but there was no relationship with the precipitation of the driest month (p = 0.516) or precipitation seasonality (p = 0.977), as these two variables were very similar across sites (Supplementary Figure S5).
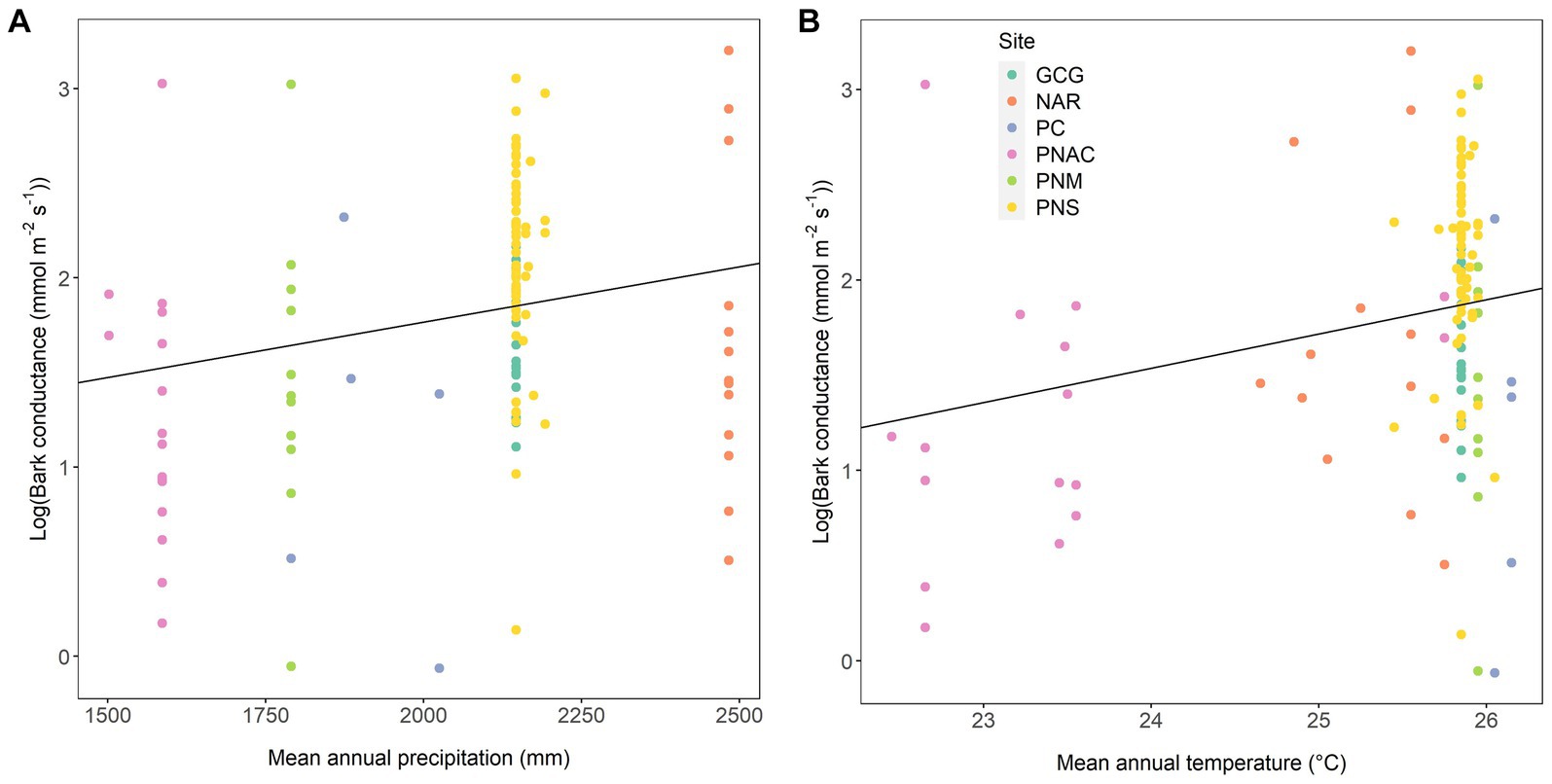
Figure 2. Bark conductance as a function of mean annual precipitation (A) and mean annual temperature (B). Each point represents a species-at-site combination. Different colors indicate different forests: Gamboa Common Garden (GCG), Parque Nacional Soberanía (PNS), Parque Nacional Altos de Campana (PNAC), Nueva Arenosa (NAR), Panama City (PC), and Parque Natural Metropolitano (PNM). Regression lines are derived from mixed models across all the data points. Conditional r2 = 0.391 and p = 0.008 for mean annual precipitation, and conditional r2 = 0.440 and p = 0.002 for mean annual temperature.
In addition to morphometric traits having an impact on gbark, as well as site climate, there was also a significant phylogenetic signal in gbark, evidenced by both Blomberg’s K of 0.19 (p = 0.006) and Pagel’s λ of 0.25 (p = 0.014) (Table 2) indicating that closely related species are more similar in terms of gbark than distantly related species. We also found a significant phylogenetic signal, as evidenced by both Blomberg’s K and Pagel’s λ indexes, in relative bark thickness and bark density (Table 2) and, as evidenced by Pagel’s λ only, in bark-specific area (Table 2). The phylogenetic signal in gbark can also be observed when mapping the trait onto a phylogenetic tree of the study species (Figure 3), where species in the Bixaceae, Salicaceae, and Sapindaceae show the highest gbark values and species in the Clusiaceae the lowest.
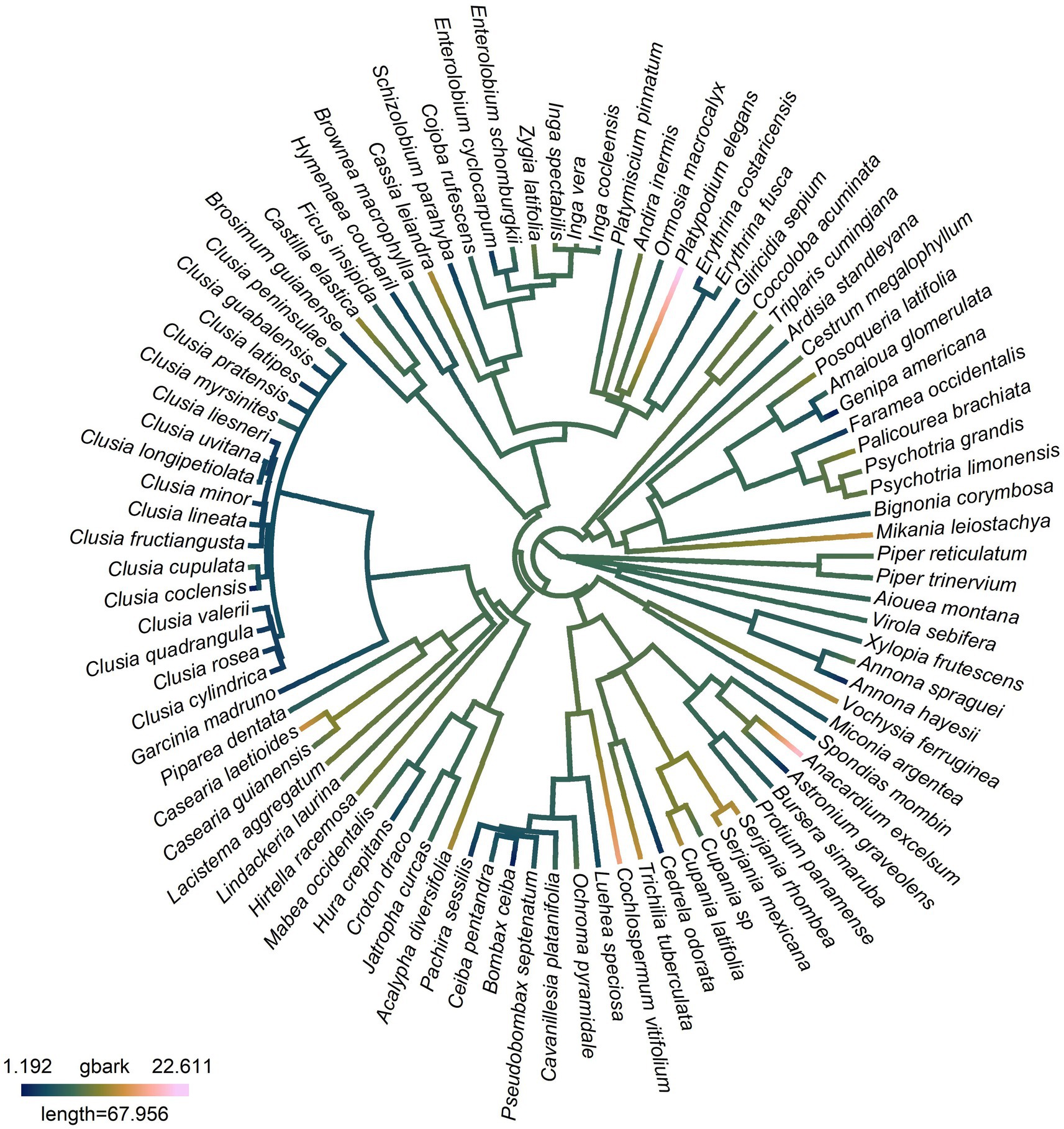
Figure 3. Bark conductance (gbark) mapped onto a phylogenetic tree of 94 Neotropical plant species. Darker colors along the branches of the tree indicate lower values of gbark, whereas lighter colors indicate higher values of gbark.
In all six species studied for temperature response, gbark decreased as air temperature increased (Figure 4).
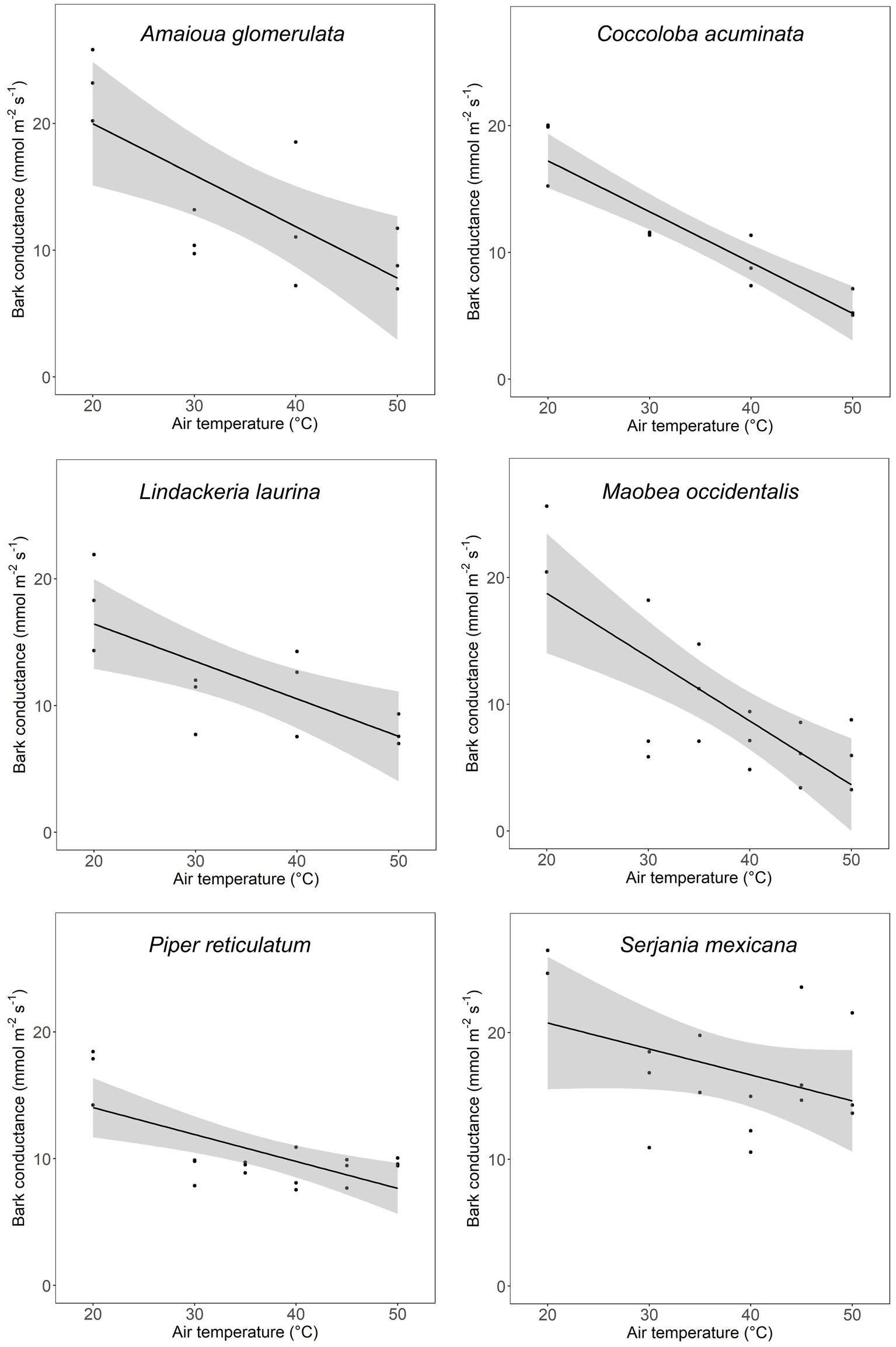
Figure 4. Temperature response curves of bark conductance in six plant species from Parque Nacional Soberanía. Each point is a different stem subjected to the specified temperature. Lines are from linear models fit to every species. Amaioua glomerulata (r2 = 0.547, p = 0.006), Coccoloba acuminata (r2 = 0.858, p = 1.50 × 10−5), Lindackeria laurina (r2 = 0.548, p = 0.006), Mabea occidentalis (r2 = 0.553, p = 4.07 × 10−4), Piper reticulatum (r2 = 0.465, p = 0.002), and Serjania mexicana (r2 = 0.275, p = 0.026).
Discussion
We studied the possible factors that underlie variation in gbark among Neotropical plant species from six forest sites in Panama. Bark conductance was strongly influenced by relative bark thickness. We also found that site climate, mainly MAP and MAT, influenced gbark. Finally, closely related species were more similar regarding gbark than distantly related species, i.e., there was phylogenetic signal in gbark evidenced by both Blomberg’s K and Pagel’s λ.
As reported for many other species (Poorter et al., 2014; Rosell, 2016; Rosell et al., 2017), bark thickness was positively related to stem diameter. We found that relative bark thickness was a key morphometric trait related to gbark. Even though we did not measure inner and outer bark thickness separately, we expect the outer bark to be more limiting to gbark than the inner bark as has been reported for Cerrado plants (Loram-Lourenço et al., 2022). Rosell et al. (2017) demonstrated that the metabolically active inner bark is not related to gbark. Our observations of the relationship between relative bark thickness and bark thickness and gbark (Figures 1A,B), respectively, are consistent with a previous study by Wolfe (2020) on Panamanian tree species and other studies that have demonstrated that low investment in structural carbon facilitates both stem CO2 exchange (photosynthetic and respiratory) and water loss in thin stems with thin bark in tropical and non-tropical species (Ávila-Lovera et al., 2017; Berry et al., 2021; Loram-Lourenço et al., 2022). A small relative bark thickness shortens the path length that water must travel from the xylem to the surface of the stem during transpiration. Similarly, in a thin bark, it is expected to find fewer cell walls and cell membranes that water must pass through. These may be two reasons why we found a negative relationship between gbark and relative bark thickness. In a comparison of photosynthetic vs. non-photosynthetic stems from six different ecosystems, the relative bark thickness of branches and the outer bark thickness of the main stem were smaller in photosynthetic than in non-photosynthetic stems (Rosell et al., 2014, 2015), further supporting the fact that thin bark, especially a thin outer bark, increases photosynthetic capacity and facilitates water movement. Overall, it seems that both CO2 and water vapor movement are not only linked in organs with stomata but also in organs without stomata, such as stems with periderm and lenticels.
Species from wetter and warmer forests, i.e., lowland forests, in Panama had greater gbark values than species from drier and slightly cooler forests supporting the idea of plant trait variation along climatic gradients (Wright et al., 2004; Moles et al., 2009, 2014; Blonder et al., 2017), which may be an indication of the adaptive significance of the trait. While it is unclear what the advantage would be of having a relatively high gbark in a wet and warm tropical forest, the adaptive value of having a low gbark in a dry tropical forest seems obvious. The situation changes completely when the precipitation gradient includes woody shrubs of Mediterranean-type drylands and deserts characterized by highly active green stems with an epidermis instead of a periderm (Gibson, 1983; Yiotis et al., 2006; Ávila et al., 2014); these stems attain high rates of net photosynthesis and transpiration (Ávila-Lovera et al., 2017).
As more phylogenetic information becomes available, the usage of phylogenetic trees to answer questions about the role of evolutionary relatedness on trait variation has become more relevant (for example, Ackerly and Reich, 1999; Maherali et al., 2004; Ávila-Lovera et al., 2023). Despite the strong effect of climate on many plant-water relations traits, phylogenetic signal is also common among these traits (Sanchez-Martinez et al., 2020; Ávila-Lovera et al., 2023), especially structural traits. The fact that we found the phylogenetic signal in gbark and morphometric traits, such as relative bark thickness and bark density, indicates that bark traits are influenced by the evolutionary history of closely related species. For example, the Clusiaceae species had the lowest gbark values measured. Consistent with observations of low gmin of leaves of Clusia rosea, Clusia pratensis, and Garcinia intermedia, all species of Clusiaceae from Panamanian forests (Slot et al., 2021), the stems of Clusiaceae species in our study also had relatively low values of gbark. Even though the photosynthetic physiology of Clusia stems is quite well understood (Kocurek et al., 2015, 2020), detailed studies of the structure and chemistry of Clusia stems are required to fully assess the reasons for their relatively low gbark, although it is clear that members of Clusiaceae we examined were those with the thickest barks (Figure 1).
Contrary to our expectations and the results of the field survey, in the laboratory, gbark decreased as air temperature increased. In leaves, an increase in air temperature leads to an increase in air temperature can lead to an increase in cuticular conductance (Schuster et al., 2016; Bueno et al., 2019; but see Slot et al., 2021), due to the fact that the cutin matrix and epicuticular waxes lose their stability at high temperatures, except in the highly thermostable cuticles of some desert plants (Schuster et al., 2016; Bueno et al., 2019). In stems, the barrier that water needs to pass through is a suberized periderm. We do not know whether higher air temperatures and greater VPD caused dehydration and shrinkage of the periderm leading to lower gbark values at high temperatures, although at all temperatures we observed linear decreases of stem fresh mass with time. Decreasing gbark with increasing air temperature and VPD has also been found in the study of Wolfe (2020), although the temperature range was narrower in that study compared with ours. More research is needed to disentangle the effect of air temperature and VPD on gbark.
Taken together, these results indicate that several factors contribute to the variation in gbark in Neotropical woody species. In a warmer and partly drier world with increased frequency of extreme weather events, including heat waves, gbark may determine the ability of plants to survive drought periods, as water loss through the stem bark is the remaining path for whole-plant dehydration after stomatal closure and loss of leaves and fine roots.
Data availability statement
The original contributions presented in the study are included in the article/Supplementary material, further inquiries can be directed to the corresponding author.
Author contributions
EÁ-L: Conceptualization, Formal analysis, Funding acquisition, Investigation, Methodology, Project administration, Resources, Writing – original draft. KW: Supervision, Writing – review & editing.
Funding
The author(s) declare financial support was received for the research, authorship, and/or publication of this article. The study was supported by the Smithsonian Tropical Research Institute. EÁ-L was a recipient of a Smithsonian Tropical Research Institute Earl S. Tupper Postdoctoral Fellowship.
Acknowledgments
We thank Jorge Aranda for identifying the species in the field. We also thank German Vargas G. and Joe Wright for providing leaf phenology data. Finally, we thank Helene Muller-Landau for discussions on climate data, and Vicente Vasquez for help with the CHELSA 2.1 climate dataset.
Conflict of interest
The authors declare that the research was conducted in the absence of any commercial or financial relationships that could be construed as a potential conflict of interest.
Publisher’s note
All claims expressed in this article are solely those of the authors and do not necessarily represent those of their affiliated organizations, or those of the publisher, the editors and the reviewers. Any product that may be evaluated in this article, or claim that may be made by its manufacturer, is not guaranteed or endorsed by the publisher.
Supplementary material
The Supplementary material for this article can be found online at: https://www.frontiersin.org/articles/10.3389/ffgc.2023.1278803/full#supplementary-material
Footnotes
References
Abràmoff, D. M. D. (2004). Image processing with image. J. Biophoton. Intenation. 11, 36–42. doi: 10.1007/s11099-014-0004-2
Ackerly, D. D., and Reich, P. B. (1999). Convergence and correlations among leaf size and function in seed plants: a comparative test using independent contrasts. Am. J. Bot. 86, 1272–1281. doi: 10.2307/2656775
Angyalossy, V., Pace, M. R., Evert, R. F., Marcati, C. R., Oskolski, A. A., Terrazas, T., et al. (2016). IAWA list of microscopic bark features. IAWA J. 37, 517–615. doi: 10.1163/22941932-20160151
Ávila, E., De Almeida, J., and Tezara, W. (2014). Comparación ecofisiológica y anatómica de los tejidos fotosintéticos de Cercidium praecox (Ruiz & Pav. ex Hook.) Harms (Fabaceae, Caesalpinioideae). Acta Botánica Venezuelica 37, 59–76.
Ávila-Lovera, E., Haro, R., Ezcurra, E., and Santiago, L. S. (2019). Costs and benefits of photosynthetic stems in desert species from southern California. Funct. Plant Biol. 46, 175–186. doi: 10.1071/FP18203
Ávila-Lovera, E., Winter, K., and Goldsmith, G. R. (2023). Evidence for phylogenetic signal and correlated evolution in plant-water relations traits. New Phytol. 237, 392–407. doi: 10.1111/nph.18565
Ávila-Lovera, E., Zerpa, A. J., and Santiago, L. S. (2017). Stem photosynthesis and hydraulics are coordinated in desert plant species. New Phytol. 216, 1119–1129. doi: 10.1111/nph.14737
Berry, Z. C., Ávila-Lovera, E., De Guzman, M. E., O’Keefe, K., and Emery, N. C. (2021). Beneath the bark: assessing woody stem water and carbon fluxes and its prevalence across climates and the woody plant phylogeny. Front. For. Global Change 4:675299. doi: 10.3389/ffgc.2021.675299
Blonder, B., Salinas, N., Bentley, L. P., Shenkin, A., Porroa, P. O. C., Tejeira, Y. V., et al. (2017). Predicting trait-environment relationships for venation networks along an Andes-Amazon elevation gradient. Ecology 98, 1239–1255. doi: 10.1002/ecy.1747
Buckley, T. N., and Sack, L. (2019). The humidity inside leaves and why you should care: implications of unsaturation of leaf intercellular airspaces. Am. J. Bot. 106, 618–621. doi: 10.1002/ajb2.1282
Bueno, A., Alfarhan, A., Arand, K., Burghardt, M., Deininger, A.-C., Hedrich, R., et al. (2019). Effects of temperature on the cuticular transpiration barrier of two desert plants with water-spender and water-saver strategies. J. Exp. Bot. 70, 1613–1625. doi: 10.1093/jxb/erz018
Cernusak, L., and Marshall, J. (2000). Photosynthetic refixation in branches of western white pine. Funct. Ecol. 14, 300–311. doi: 10.1046/j.1365-2435.2000.00436.x
Chaves, M. M., and Pereira, J. S. (1992). Water stress, CO2 and climate change. J. Exp. Bot. 43, 1131–1139. doi: 10.1093/jxb/43.8.1131
Duursma, R. A., Blackman, C. J., Lopéz, R., Martin-StPaul, N. K., Cochard, H., and Medlyn, B. E. (2019). On the minimum leaf conductance: its role in models of plant water use, and ecological and environmental controls. New Phytol. 221, 693–705. doi: 10.1111/nph.15395
Gibson, A. C. (1983). Anatomy of photosynthetic old stems of nonsucculent dicotyledons from north American deserts. Bot. Gaz. 144, 347–362. doi: 10.1086/337383
IPCC. (2023) Climate change 2023: synthesis report of the IPPC sixth assessment report (AR6). IPCC: Interlaken, Switzerland
Jin, Y., and Qian, H. (2019). V.PhyloMaker: an R package that can generate very large phylogenies for vascular plants. Ecography 42, 1353–1359. doi: 10.1111/ecog.04434
Karger, D. N., Conrad, O., Böhner, J., Kawohl, T., Kreft, H., Soria-Auza, R. W., et al. (2017). Climatologies at high resolution for the earth’s land surface areas. Sci. Data 4:170122. doi: 10.1038/sdata.2017.122
Karger, D. N., Conrad, O., Böhner, J., Kawohl, T., Kreft, H., Soria-Auza, R. W., et al. (2021). Climatologies at high resolution for the earth’s land surface areas. EnviDat. doi: 10.16904/envidat.228
Kocurek, M., Kornas, A., Pilarski, J., Tokarz, K., Lüttge, U., and Miszalski, Z. (2015). Photosynthetic activity of stems in two Clusia species. Trees 29, 1029–1040. doi: 10.1007/s00468-015-1182-7
Kocurek, M., Kornas, A., Wierzchnicki, R., Lüttge, U., and Miszalski, Z. (2020). Importance of stem photosynthesis in plant carbon allocation of Clusia minor. Trees 34, 1009–1020. doi: 10.1007/s00468-020-01977-w
Kuznetsova, A., Brockhoff, P. B., and Christensen, R. H. B. (2017). lmerTest package: tests in linear mixed effects models. J. Stat. Softw. 82, 1–26. doi: 10.18637/jss.v082.i13
Lindorf, H., de Parisca, L., and Rodríguez, P. (2006). Botánica: clasificación, estructura, reproducción. 2nd Edn Caracas, Venezuela: Ediciones de la Biblioteca de la Universidad Central de Venezuela.
Loram-Lourenço, L., Farnese, F. S., Alves, R. D. F. B., Dario, B. M. M., Martins, A. C., Aun, M. A., et al. (2022). Variations in bark structural properties affect both water loss and carbon economics in neotropical savanna trees in the Cerrado region of Brazil. J. Ecol. 110, 1826–1843. doi: 10.1111/1365-2745.13908
Lüdecke, D., Ben-Shachar, M., Patil, I., Waggoner, P., and Makowski, D. (2021). Performance: an R package for assessment, comparison and testing of statistical models. J. Open Source Softw. 6:3139. doi: 10.21105/joss.03139
Maherali, H., Pockman, W. T., and Jackson, R. B. (2004). Adaptive variation in the vulnerability of woody plants to xylem cavitation. Ecology 85, 2184–2199. doi: 10.1890/02-0538
Meinzer, F. C., Woodruff, D. R., Domec, J.-C., Goldstein, G., Campanello, P. I., Gatti, M. G., et al. (2008). Coordination of leaf and stem water transport properties in tropical forest trees. Oecologia 156, 31–41. doi: 10.1007/s00442-008-0974-5
Moles, A. T., Perkins, S. E., Laffan, S. W., Flores-Moreno, H., Awasthy, M., Tindall, M. L., et al. (2014). Which is a better predictor of plant traits: temperature or precipitation? J. Veg. Sci. 25, 1167–1180. doi: 10.1111/jvs.12190
Moles, A. T., Warton, D. I., Warman, L., Swenson, N. G., Laffan, S. W., Zanne, A. E., et al. (2009). Global patterns in plant height. J. Ecol. 97, 923–932. doi: 10.1111/j.1365-2745.2009.01526.x
Nobel, P. S., and Cui, M. (1992). Hydraulic conductances of the soil, the root-soil air gap, and the root: changes for desert succulents in drying soil. J. Exp. Bot. 43, 319–326. doi: 10.1093/jxb/43.3.319
Poorter, L., McNeil, A., Hurtado, V.-H., Prins, H. H. T., and Putz, F. E. (2014). Bark traits and life-history strategies of tropical dry- and moist forest trees. Funct. Ecol. 28, 232–242. doi: 10.1111/1365-2435.12158
R Core Team (2023) R: a language and environment for statistical computing. R Foundation for Statistical Computing, Vienna, Austria
Revell, L. J. (2012). Phytools: an R package for phylogenetic comparative biology (and other things). Methods Ecol. Evol. 3, 217–223. doi: 10.1111/j.2041-210X.2011.00169.x
Rosell, J. A. (2016). Bark thickness across the angiosperms: more than just fire. New Phytol. 211, 90–102. doi: 10.1111/nph.13889
Rosell, J. A., Castorena, M., Laws, C. A., and Westoby, M. (2015). Bark ecology of twigs vs. main stems: functional traits across eighty-five species of angiosperms. Oecologia 178, 1033–1043. doi: 10.1007/s00442-015-3307-5
Rosell, J. A., Gleason, S., Méndez-Alonzo, R., Chang, Y., and Westoby, M. (2014). Bark functional ecology: evidence for tradeoffs, functional coordination, and environment producing bark diversity. New Phytol. 201, 486–497. doi: 10.1111/nph.12541
Rosell, J. A., Olson, M. E., Anfodillo, T., and Martínez-Méndez, N. (2017). Exploring the bark thickness–stem diameter relationship: clues from lianas, successive cambia, monocots and gymnosperms. New Phytol. 215, 569–581. doi: 10.1111/nph.14628
Sack, L, and Scoffoni, C (2011). PrometheusWiki | Minimum epidermal conductance (gmin, a.k.a. cuticular conductance). Available at: https://prometheusprotocols.net/function/gas-exchange-and-chlorophyll-fluorescence/stomatal-and-non-stomatal-conductance-and-transpiration/minimum-epidermal-conductance-gmin-a-k-a-cuticular-conductance/ (Accessed March 28, 2016)
Sanchez-Martinez, P., Martínez-Vilalta, J., Dexter, K. G., Segovia, R. A., and Mencuccini, M. (2020). Adaptation and coordinated evolution of plant hydraulic traits. Ecol. Lett. 23, 1599–1610. doi: 10.1111/ele.13584
Santiago, L. S., Bonal, D., De Guzman, M. E., and Ávila-Lovera, E. (2016). “Drought survival strategies of tropical trees” in Tropical tree physiology. eds. G. Goldstein and L. S. Santiago (Cham: Springer International Publishing), 243–258.
Schneider, C. A., Rasband, W. S., and Eliceiri, K. W. (2012). NIH image to ImageJ: 25 years of image analysis. Nat. Methods 9, 671–675. doi: 10.1038/nmeth.2089
Schulze, E.-D., Robichaux, R. H., Grace, J., Rundel, P. W., and Ehleringer, J. R. (1987). Plant water balance. Bioscience 37, 30–37. doi: 10.2307/1310175
Schuster, A.-C., Burghardt, M., Alfarhan, A., Bueno, A., Hedrich, R., Leide, J., et al. (2016). Effectiveness of cuticular transpiration barriers in a desert plant at controlling water loss at high temperatures. AoB PLANTS 8:plw027. doi: 10.1093/aobpla/plw027
Slot, M., Nardwattanawong, T., Hernández, G. G., Bueno, A., Riederer, M., and Winter, K. (2021). Large differences in leaf cuticle conductance and its temperature response among 24 tropical tree species from across a rainfall gradient. New Phytol. 232, 1618–1631. doi: 10.1111/nph.17626
Tyree, MT, and Zimmermann, MH (2002) Xylem structure and the ascent of sap. Springer Berlin Heidelberg, Berlin, Heidelberg
Wolfe, B. T. (2020). Bark water vapour conductance is associated with drought performance in tropical trees. Biol. Lett. 16:20200263. doi: 10.1098/rsbl.2020.0263
Wright, I. J., Reich, P. B., Westoby, M., Ackerly, D. D., Baruch, Z., Bongers, F., et al. (2004). The worldwide leaf economics spectrum. Nature 428, 821–827. doi: 10.1038/nature02403
Keywords: bark traits, climate change, functional traits, morphoanatomy, tropical forest, water loss
Citation: Ávila-Lovera E and Winter K (2024) Variation in stem bark conductance to water vapor in Neotropical plant species. Front. For. Glob. Change. 6:1278803. doi: 10.3389/ffgc.2023.1278803
Edited by:
Julieta A. Rosell, National Autonomous University of Mexico, MexicoReviewed by:
Rodrigo Mendez Alonzo, Center for Scientific Research and Higher Education in Ensenada (CICESE), MexicoJohn T. Van Stan, Cleveland State University, United States
Copyright © 2024 Ávila-Lovera and Winter. This is an open-access article distributed under the terms of the Creative Commons Attribution License (CC BY). The use, distribution or reproduction in other forums is permitted, provided the original author(s) and the copyright owner(s) are credited and that the original publication in this journal is cited, in accordance with accepted academic practice. No use, distribution or reproduction is permitted which does not comply with these terms.
*Correspondence: Eleinis Ávila-Lovera, ZWxlaW5pcy5hdmlsYUBiaW9sb2d5LnV0YWguZWR1
†Present address: Eleinis Ávila-Lovera, School of Biological Sciences, University of Utah, Salt Lake City, UT, United States