- 1USDA Forest Service, Pacific Southwest Research Station, Arcata, CA, United States
- 2California Department of Forestry and Fire Protection, Sacramento, CA, United States
- 3School of Forestry, Northern Arizona University, Flagstaff, AZ, United States
- 4USDA Forest Service, Pacific Southwest Research Station, Fort Bragg, CA, United States
- 5USDA Forest Service, Pacific Southwest Research Station, Coarsegold, CA, United States
Given the high costs of constructing, maintaining, monitoring, and sampling paired watersheds, it is prudent to ask “Are paired watershed studies still worth the effort?” We present a compilation of 90 North American paired watershed studies and use examples from the Caspar Creek Experimental Watersheds to contend that paired watershed studies are still worth the effort and will continue to remain relevant in an era of big data and short funding cycles. We offer three reasons to justify this assertion. First, paired watersheds allow for watershed-scale experiments that have produced insights into hydrologic processes, water quality, and nutrient cycling for over 100 years. Paired watersheds remain an important guide to inform best management practices for timber harvesting and other land-management concerns. Second, paired watersheds can produce long climate, streamflow, and water quality records because sites are frequently maintained over the course of multiple experiments or long post-treatment periods. Long-term datasets can reveal ecological surprises, such as changes in climate-streamflow relationships driven by slow successional processes. Having multiple watershed records helps identify the cause of these changes. Third, paired watersheds produce data that are ideal for developing and testing hydrologic models. Ultimately, the fate of paired watersheds is up to the scientific community and funding agencies. We hope that their importance continues to be recognized.
1. Introduction
Paired watershed designs have been used for over 100 years (Neary, 2016), but the number of active studies with recent treatments has trended downward in North America and elsewhere (Ziemer and Ryan, 2000). The general approach to paired watershed experiments is that one watershed serves as a control while one or more watersheds undergo a management treatment. A few years of calibration data are typically collected to develop a baseline before the treatment begins. In some locations, climate change or some other factor might affect one watershed differently than other watersheds. In these cases, the “control” watershed is more accurately referred to as a “reference.” We use the terms interchangeably in this paper.
Neary (2016) summarized common criticisms of paired watershed studies as “too costly, unrepresentative, used leaky watersheds, had questionable application of results, and did not contribute to scientific progress on hydrologic processes,” but also offered an argument originally made by Hewlett et al. (1969) in defense of paired watersheds. Hewlett et al.’s (1969) justification of paired watersheds focused on the substantial benefit gained from the paired watershed approach which enables investigators to separate vegetation cover effects from climate effects in a manner not possible with alternative empirical approaches. Ultimately, when considered across the large number of studies, paired watersheds have contributed to major hydrologic insights that have been critical for advancing the field of hydrology (Burt and McDonnell, 2015). Nonetheless, the criticisms summarized by Neary (2016) persist due to the high costs to establish and maintain paired watershed studies and potential weaknesses associated with poorly-paired control watersheds. However, even when these limitations are considered, no alternative approach currently exists that is capable of producing the same level of land-use knowledge.
Given the cost and effort required to maintain long-term study sites, we assessed their overall value and identified three key benefits to using the paired watershed approach for forest hydrology. We anecdotally draw from examples pertaining to the Caspar Creek Experimental Watersheds in the northern California Coast Ranges, a long-term paired watershed site, to highlight the importance of paired watershed experiments and continued monitoring. We also offer an overview of other experimental paired watersheds and present a compilation of existing and prior paired watershed studies in North America. To our knowledge, this is the largest compilation of North American paired watershed studies yet produced. We summarize data availability for these sites and include suggestions regarding how we might keep paired watershed studies relevant and improve data management for these studies and others.
2. Methods
We assembled a compilation of North American paired watersheds by completing an extensive literature search and including sites known to the authors that are undiscoverable in published literature. We focused on non-agricultural, wildland sites (forest, shrubland, grassland, and tundra) and extracted information about each study’s location, experimental watershed sizes, vegetation type, data availability, project description and design, funding sources, and experimental networks to which they belong. We also identified and contacted principal investigators or site managers when possible to verify the information.
Some experimental sites were designed as paired watershed studies while other sites have used paired watershed analytical techniques for some of their analyses. We focus our attention primarily on those sites that were designed as paired watershed experiments and include experimental control and treated paired watersheds designed to include measurements before and after treatment. When coordinates were not supplied, we estimated site locations from published maps or site location descriptions. If multiple paired watersheds were located in the same region and part of the same study, we typically report on the group of paired watershed studies as a single site unless otherwise stated. We reported the maximum range of years presented in cited literature, available online, or confirmed by lead scientist or site managers. If data were not referenced in publications, available online, or available from the lead authors, the data are reported as “data unavailable / availability unknown.” Some data types may have only been collected for a subset of reported years and additional data types may also be available.
3. Paired watershed studies in North America
Paired watersheds have been used to investigate both natural disturbances (e.g., wildfire, insect outbreaks, and extreme hydrologic events) and anthropogenic disturbances (Neary, 2016). Investigations of hydrologic and water quality response to anthropogenic disturbances are extensive and include controlled experiments on timber harvesting (e.g., Harr, 1980; Blackburn et al., 1985; Burton, 1997; Ziemer, 1998; Stubblefield et al., 2017), forest thinning (e.g., DeBano et al., 1999; Perry and Jones, 2017), road construction (e.g., King and Tennyson, 1984; Lewis, 1998; Troendle et al., 2001), salvage logging (e.g., Moore and Scott, 2005; Elliot and Glaza, 2009; Niemeyer et al., 2020), prescribed fire (e.g., Gottfried et al., 2007; Neary D. G. et al., 2012), use of fertilizers (e.g., Hetherington, 1985), rainfall acidification (e.g., Cosby et al., 1996), and vegetation conversion (e.g., Ingebo, 1972; Dunn et al., 1988; Zhong et al., 2020). With each of these investigations, it is possible to monitor a variety of hydrologic and ecological responses including changes to total streamflow, peak flow response, summer low flows, stream temperature, groundwater storage, recharge and discharge, sediment concentrations and loads, nutrient concentrations and loads, channel morphology, landslide frequency and size, and biological responses (algae, macroinvertebrates, fish, and amphibians). Our compilation (Figure 1; Supplementary Table S1) includes studies with a wide range of experimental designs and goals. Some studies have focused on a single control and treatment watershed while other studies have included multiple control and treatment watersheds. Studies that only include a single control and treatment watershed are more likely to receive criticism due to the lack of replication. Paired watershed studies that included multiple control and treatment watersheds have improved statistical power due to the increased number of measured responses, better assessment of variability for the controls, and having multiple replications of similar treatments or, in the case of different treatments, stronger hypotheses regarding predictive relationships for the treatment watersheds. As an example of the latter case, the third experiment at Caspar Creek utilized a gradient in harvest intensities across treatment watersheds (Dymond et al., 2021).
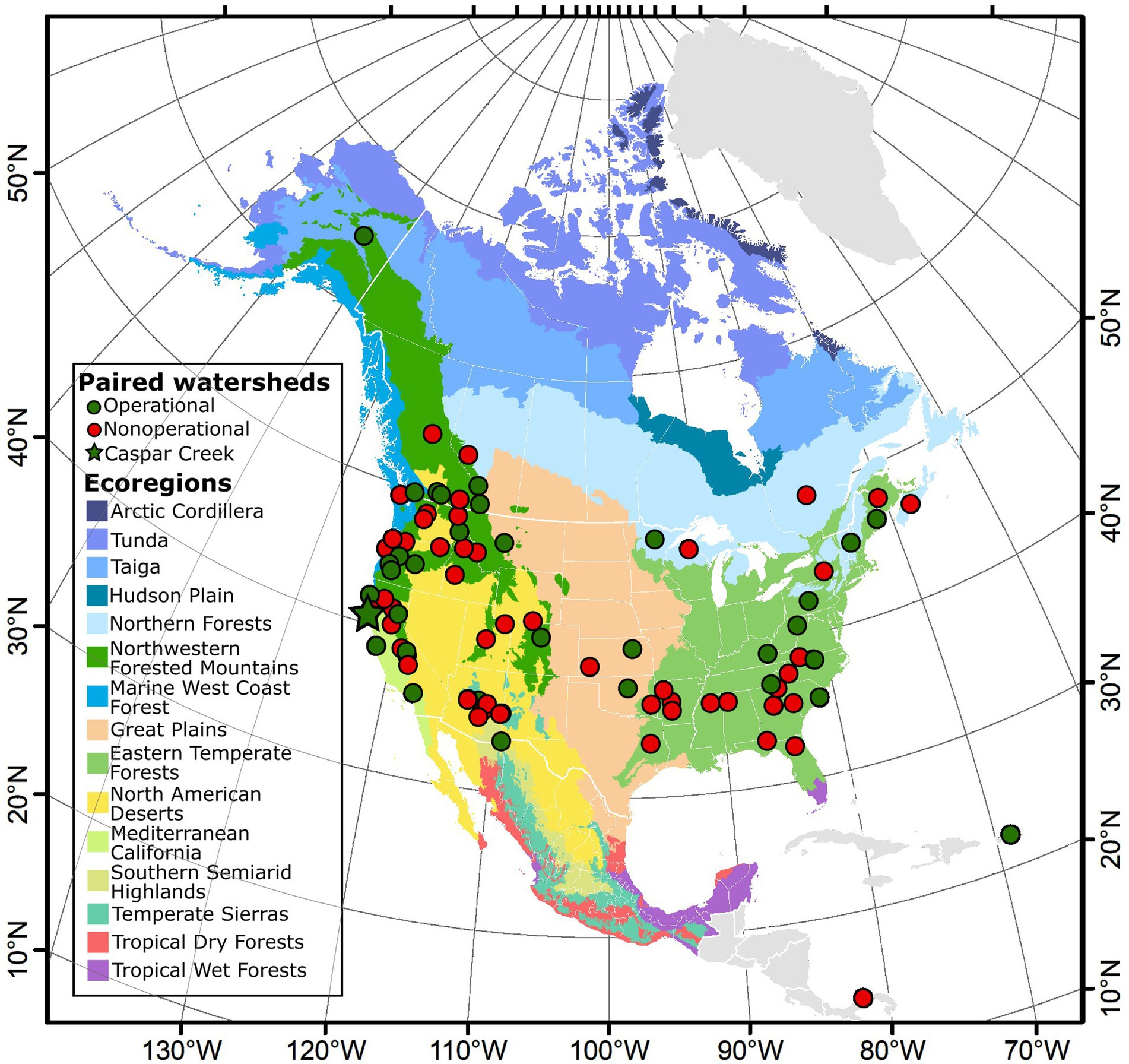
Figure 1. Map of North America showing operational (green) and nonoperational (red) paired watershed study sites. Colored shading shows EPA Level 1 Ecoregions for North America, which include the United States, Mexico, and Canada (Omernik, 1987). The Domain level (coarsest level) of the USDA Forest Service Ecoregions of the Continents categorizes Puerto Rico and Panama as Humid Tropical (Bailey, 1989). For our analysis, we consider Panama and Puerto Rico as Tropical Wet Forests in the EPA Level 1 Ecoregions system. Operational paired watersheds represent 10 of the 15 Environmental Protection Agency (EPA) Level 1 Ecoregions in North America. In some cases, nonoperational paired watershed sites are still operational experimental sites, but the paired watershed monitoring has ended. Please refer to Supplementary Table S1 for a description of each site.
The USDA Forest Service oversees a vast network of experimental forest and ranges, many of which include historic or active paired watersheds (Adams et al., 2004; Lugo et al., 2006; Neary D. et al., 2012; Hayes et al., 2014; Amatya et al., 2016). The first paired watershed study in North America was Wagon Wheel Gap, Colorado. The study began in 1910 to investigate how streamflow, erosion, and sediment transport respond to timber harvesting in the Rio Grande National Forest (Bates and Henry, 1928). Over 150 paired watersheds have been established globally (McDonnell et al., 2018). Like Wagon Wheel Gap, many of the original studies were developed to investigate the hydrologic response to timber harvesting. Overwhelmingly, these studies found that timber harvesting typically leads to moderate initial increases in water yield until vegetation regrows (Bosch and Hewlett, 1982; Stednick, 1996). Although we focus on paired watershed studies in North America, we acknowledge the substantial effort that has been made elsewhere to establish paired watersheds.
A substantial paired watershed network administered by the USDA Agricultural Research Service (Slaughter and Richardson, 2000; Goodrich et al., 2021) also exists to address agricultural land-use concerns in the United States and was implemented after the devastation caused by the Dust Bowl (Goodrich et al., 2021). However, we excluded USDA Agricultural Research Service paired watersheds from our compilation to limit the scope of this study to wildland sites as defined above.
We heavily draw from examples at the Caspar Creek Experimental Watersheds, which are located in the Jackson Demonstration State Forest in northern California and include both an experimental pair of watersheds (the North Fork and South Fork) and nested subwatersheds (Figure 2). Three experimental timber harvests have been completed at Caspar Creek during the 60-year research history (Dymond et al., 2021; Richardson et al., 2021c). We emphasize Caspar Creek examples because the authors of this manuscript have conducted research at Caspar Creek, and we believe that focusing on a single site helps to highlight the abundance and variety in scientific potential that commonly exists at a single site. Many of the research opportunities that exist at Caspar Creek also exist at other experimental paired watersheds.
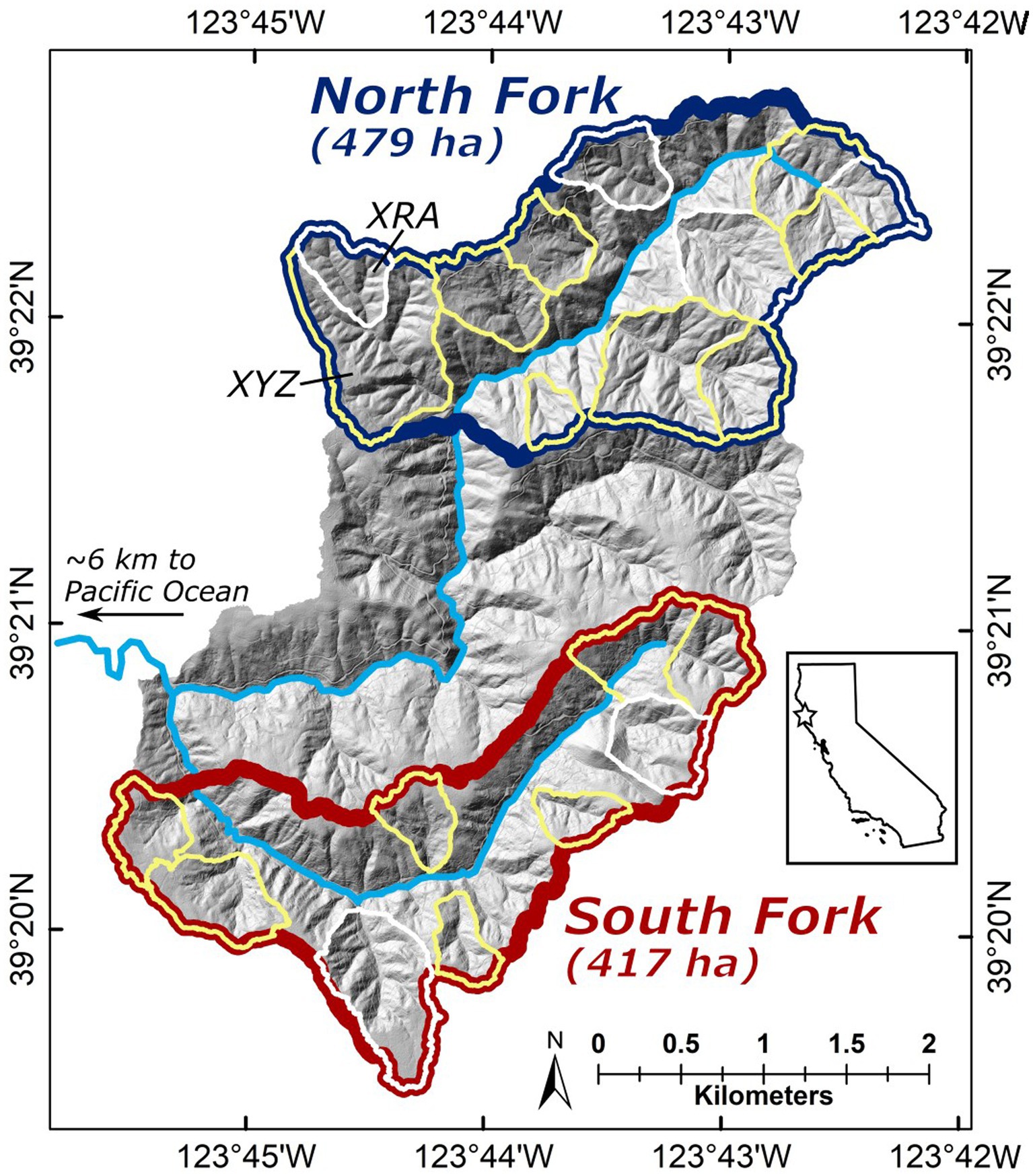
Figure 2. Shaded relief map showing the Caspar Creek Experimental Watersheds. The South Fork (red) and the North Fork (blue) watersheds are the primary pair. Nested paired subwatersheds are outlined (harvested subwatersheds outlined in yellow and control subwatersheds outlined in white). Some subwatersheds include a nested subwatershed [for example, XYZ includes the subwatershed XRA (labeled)]. All outlined subwatersheds have been gaged for a subset of the study period. Main waterways are shown in light blue. The location of Caspar Creek is marked with a star on the inset map of California.
4. Results and discussion
4.1. The strength of paired watershed studies
After over 100 years of paired watershed studies, it is reasonable to pose the question “Are paired watershed studies still worth the effort?” We believe they are for the reasons provided below.
4.1.1. Paired watersheds provide excellent opportunities for watershed-scale experiments
Paired watershed studies are the most rigorous field-based tools that hydrologists have to study the impacts of a treatment or disturbance on hydrologic processes at the watershed scale. While watershed-scale models can certainly help develop hypotheses and validate field results, field investigations are still the best way to discover new mechanisms by which watersheds function (Burt and McDonnell, 2015). For paired watershed studies to remain relevant, they must be able to address new and exciting research questions and move beyond investigations of streamflow response (McDonnell et al., 2018). For example, as part of the third experimental harvest at Caspar Creek, we are investigating how timber harvests influence physical, biological, and chemical mechanisms that impact hydrologic processes at the plot, hillslope, and watershed scales (Dymond et al., 2021).
The three experimental timber harvests at Caspar Creek have tested technological, operational, and regulatory innovations. The first experiment tested impacts of minimally restricted road construction and tractor yarding on streamflow and sediment delivery, which informed modifications to the California Forest Practice Rules (Cafferata and Reid, 2013). The second experimental harvest tested best management practices (BMPs) designed to reduce sediment delivery to streams that included placement of roads high on hillslopes with mandatory drainage structures, riparian buffer strips following the California Forest Practice Rules in place at the time, and cable yarding (Cafferata and Reid, 2013). The increase in suspended sediment yield following the North Fork harvest was typically less than the increase following the South Fork harvest, reflecting improvements in forest practices and in road network design (Lewis, 1998; Keppeler and Lewis, 2007).
The third experiment is assessing harvesting from 2017 to 2019 over a wide range of harvest intensities permitted under the current California Forest Practice Rules and includes updated riparian buffer requirements and additional watershed protections for anadromous salmonids. These updated BMPs, which have been influenced by the results from prior paired watershed experiments, include buffers with no harvesting within 10 meters of fish-bearing streams (with the exception of harvesting cable corridor trees where needed), very minimal harvesting within 10 to 30 meters of fish-bearing streams, increased protection measures for larger non-fish bearing and ephemeral streams, and maintaining hydrologic disconnection between roads and stream channels.
We anticipate that additional paired watershed experiments will be necessary as technology continues to advance. For example, future harvesting experiments may investigate watershed-scale impacts of shovel logging (i.e., where a log loader is used to incrementally swing logs from the forest to a road) or tethered logging (i.e., harvesting equipment is anchored by cable and used on steep hillslopes). Other experimental treatments may investigate fire resilience and fuel reduction management techniques, which are important topics in many forested communities. Even when new technologies are not being tested, paired watershed studies can help assess cumulative effects due to multiple harvesting cycles, serve as a check on current practices, and provide feedback for within-regulation adaptive management.
Paired watershed studies also help us assess restoration efforts. For example, logging roads are hotspots for nonpoint source pollution in the form of fine sediment (e.g., Reid and Dunne, 1984; Madej, 2001; MacDonald and Coe, 2008). Because roads often increase the delivery of fine sediment to streams, road decommissioning has been a major focus of restoration efforts at Caspar Creek (e.g., Keppeler et al., 2007). The long pre-decommissioning record of sediment transport coupled with annual erosion inventories provided the baseline for an assessment of the consequences of legacy erosion, road rehabilitation sediment delivery, and road-stream connectivity (Keppeler et al., 2007). The third Caspar Creek experiment is also assessing the effects of restoration treatments on erosion and sedimentation to help inform guidance as to whether legacy road restoration should be done before, coincidentally, or after timber harvest. These and other restoration and resource protection practices, such as adding large wood to fish-bearing channels, fuel reduction, and fuel break construction, may be combined with future timber harvests. Many watersheds have experienced substantial anthropogenic disturbance and restoring vulnerable watersheds to some pre-disturbance condition is often a major environmental goal. Paired watershed studies can help us quantify and rigorously assess whether the approaches used in restoration will help achieve these goals.
4.1.2. Paired watersheds make ideal, long-term, and detailed monitoring sites
Paired watersheds are ideal for developing long-term observational records. Such studies are often long-lived because it is necessary to collect pre-treatment, treatment, and post-treatment data. Also, because of the longevity of infrastructure, new or modified experiments can occur at the same site with less cost and effort relative to establishment at new locations. Although some paired watershed studies in our compilation lasted fewer than five years (Figure 3; Supplementary Table S1), the mean total duration for data collection was 27 years, with 15 of the 90 paired watersheds having continuous records longer than 50 years. The total number of paired watersheds in our compilation peaked at 44 in 1979 and 1980 and decreased to 25 in 1990 before increasing to a total of 43 paired watersheds in 2009 (Figure 3). The revival starting in the 1990s was due to new investigations of prior treatments (e.g., McBroom et al., 2003; Pomeroy et al., 2012; Perry and Jones, 2017; Niemeyer et al., 2020), new experimental treatments at previously operational sites (e.g., Neary D. G. et al., 2012; Hatten et al., 2018), and the establishment of new paired watershed sites (Figure 3B; Supplementary Table S1).
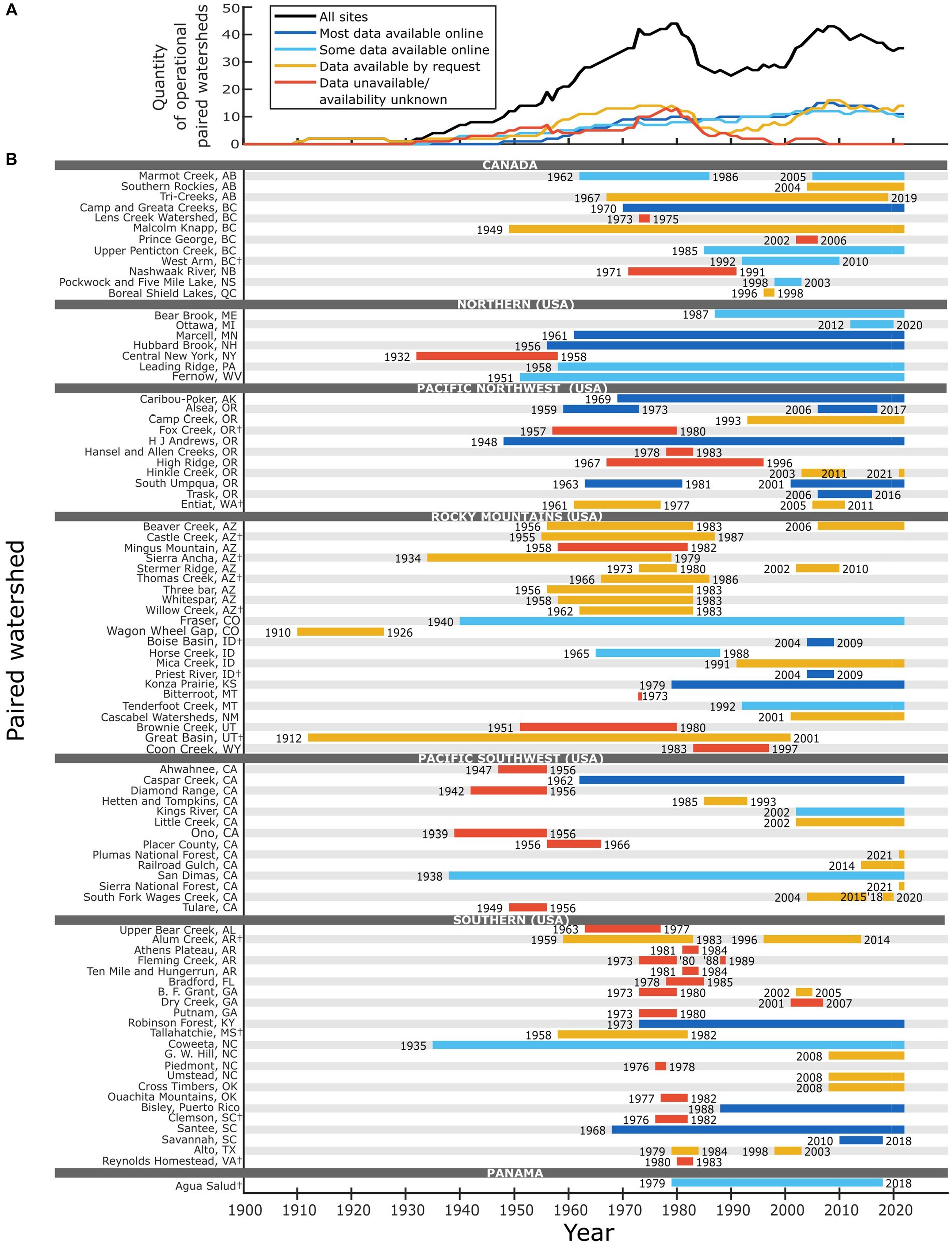
Figure 3. (A) The quantity of operational paired watershed sites and their current data availability and (B) timeline showing operational periods and data availability for paired watershed studies included in this compilation. (A,B) Share the same legend. Reported years for each site are the first and last year of known data collection during the paired watershed study. Figure 1 maps the location of each site and Supplementary Table S1 includes site summaries. A dagger symbol (†) after the study name denotes that the site is an operational experimental site, but no longer an operational paired watershed. We define the data availability categories as follows: “Most data available online” when the major components of the study are available at all intervals measured; “Some data available online” when we identified major components of the study that were not available at all time intervals measured; “Data available by request” when a statement was made by one of the investigators on a website or in a publication and the contact information for requests was valid; and “Data unavailable/availability unknown” when we could find no information about data availability publicly or through contact with the investigators or the contact information was not valid.
A major strength of the paired watershed approach relative to a single watershed approach is that paired watersheds allow treatment effects to be disentangled from multi-decadal climatic trends. Specifically, because the relationships between climatic inputs and watershed responses are determined for both control and treated watersheds prior to treatment, relative changes in these relationships during the post-treatment period can be attributed to the treatment and not climate. Perry and Jones (2017) present another example that highlights the utility of paired watershed studies. Using streamflow records from multiple paired watersheds in the Oregon Cascades, they found that conversion of old-growth forest to Douglas-fir plantations resulted in declining streamflow—a result not detectable in larger basins due to the confounding effects of climate change with past forest management. One potential limitation of the paired watershed approach is if the control watershed experiences conditions that vary from the treated watershed. Suitable watersheds that experience similar meteorological and geological conditions must be identified to limit differences that may occur between watersheds.
As the temporal record lengthens for a paired watershed study, each watershed record can be investigated independently and within the context of the treatment effects, and spatial variability across close proximity can be assessed more easily because two or more unique datasets exist for the same period. As hydrologic models improve, the opportunity for single watershed experimental treatments will increase (e.g., Zégre et al., 2010), but paired watershed studies will still prove advantageous for certain experiments, such as investigating the consequences of land-use on subsurface water storage (e.g., McDonnell et al., 2018).
Although in some cases we may be able to infer expected impacts of technological or operational advances on watershed resources without new paired watershed studies, complex ecosystem responses are likely to be missed without a long-term observational record. For example, Reid (2012) found that summer low flows following the South Fork harvesting at Caspar Creek increased for approximately seven years before decreasing for the next 20 years. Understanding the post-harvest summer low flow response is important because decreased low flows can be stressful to fish and other aquatic biota (e.g., Harvey et al., 2006; Bradford and Heinonen, 2008). Without continued, long-term post-harvest monitoring of the South Fork, this low flow response would likely have been missed. Another similar example is found at the Coweeta Hydrologic Laboratory in North Carolina, where continued monitoring captured an unexpected shift to reduced streamflow and increased dissolved inorganic nitrogen decades after harvesting and the initial observations of a return towards pre-disturbance conditions (Jackson et al., 2018). Research on the impacts of new harvest technology and practices is paramount for understanding the full effects of these changes, and paired watersheds are the most suitable method for measuring these impacts.
The long data records produced by paired watersheds may also aid us in reassessing older data. At Caspar Creek, the revaluation of previous data in the context of longer records has occasionally led to revised conclusions relative to former analyses. For example, Thomas (1990) suggested that by 1985 increases in South Fork Caspar Creek streamflow related to the early 1970s harvest experiment had ameliorated and sediment discharge had returned to pre-treatment conditions. Subsequent data indicated that both summer flows (Reid, 2012) and sediment production (Keppeler, 2012) had not yet entirely recovered. Additionally, Keppeler et al. (2009) reported that two-year recurrence interval peak flows in the South Fork had increased 14% for the eight-year period following the completion of harvesting, while earlier analyses had not found increases for larger winter peak flows (Ziemer, 1981). New analytical approaches may allow us to further explore older data and either confirm or better define conclusions.
4.1.3. Paired watershed data are well-suited for advancing hydrologic modeling
Burt and McDonnell (2015) called for “outrageous hydrologic hypotheses” within the context of field-based observations, but maybe the age of outrageous field-based observations has ended. Nearing et al. (2021) may have answered the call for outrageous hydrologic revelations by suggesting that the future of hydrologic predictions may require limited input from hydrologists and instead rely on machine learning algorithms that consider complex relationships between data and do not include hydrologic processes. Nearing et al.’s (2021) predictions mesh well with the transition to the “fourth paradigm of science (i.e., data-intensive scientific discovery)” (Silvello, 2018). Whether or not the input from hydrologists is critical for developing the next generation of predictive hydrologic models, we expect that the need for high-quality hydrologic data, such as the data collected at Caspar Creek and other paired watersheds, will remain strong and that it will continue to be collected by hydrologists. We also expect that machine learning will lead to substantial advances in hydrologic prediction and model formulation (e.g., Pate et al., 2020; Wilder et al., 2020), but we believe that there will still be a substantial need for field investigations that can deepen our knowledge of physical, biological, and chemical processes while also inspiring new hydrologic concepts and contributing to improved BMPs.
Machine learning offers a framework that allows for a high degree of model complexity (Gupta and Nearing, 2014). Although not unique to complex models, model results that are detached from well-understood physical mechanisms risk higher likelihood of equifinality. This is an important issue facing the hydrologic community, especially those who focus on hydrologic modeling (Beven, 2006); equifinality can be summarized as “getting the right answer for the wrong reasons.” In other words, if we think we understand how a watershed works in a modeling framework and then apply treatments, we may predict incorrect watershed responses when the model is applied to these new scenarios. In order to advance our understanding of hydrologic processes and reduce the likelihood of equifinality in hydrologic models of increasing complexity, measurements and observations must be made at experimental sites. Paired watersheds, in addition to other experimental watersheds (e.g., Goodrich et al., 2021), offer the scientific community a way to test these models and assess their ability to predict the consequences of land-use management decisions.
Treatments enable us to test hydrologic models under substantially different environmental conditions. Paired watershed studies that include treatments allow researchers to complete differential split-sample tests where different conditions and processes are represented. This is valuable because differential split-sample tests are considered superior to typical split-sample tests (Kirchner, 2006). In each type of test, a dataset is split into two periods, and one period is used to calibrate the model while the other period is used to verify the model. A differential split-sample test adds a level of rigor to the verification because it relies on a substantial change in conditions for the two periods, such as an experimental timber harvest.
In addition to advancing hydrologic modeling efforts, paired watershed data may also contribute to synthesis analysis between sites. Comparative analysis may help us achieve McDonnell’s (2003) goal of breaking the “mantra” of documenting experimental watershed idiosyncrasies and instead enable us to “seek commonality of response among watersheds”. Studies that analyze large compilations of paired watershed data may help us find commonality among watersheds and improve our understanding of the primary factors that influence a given treatment such as timber harvest at a more global scale. We expect that big data studies and machine learning techniques that are capable of analyzing large experimental watershed data compilations will become more prevalent in the future and contribute to this goal. We anticipate the compilation provided in Supplementary Table S1 will support additional cross-watershed meta-analysis and synthesis.
4.2. Good data management returns interest
In order for paired watershed studies to most benefit the scientific community, the resulting data must be high quality, understandable, and easily accessible, but this is not an easily achieved goal. In 1931, Horton lamented the inaccessibility of hydrologic data (Horton, 1931), and almost a century later we continue to struggle to consistently make data easily accessible. The challenges of data management are evident in our compilation: Of the 35 operational paired watersheds, only 11 sites have made most of their data publicly available in an online archive (Figure 3; Supplementary Table S1). The lack of accessible data suggests that over two-thirds of the operational paired-watershed studies in our compilation face scientific obsolescence because they may not have appropriate data available for reanalysis endeavors. To reduce the risk of irrelevance, data from long-term experiments should be maintained in periodically-updated, publicly-accessible formats.
Previous efforts to distribute Caspar Creek data included use of paper records, punch cards, reel tapes, DVDs, websites, and via email attachments. Recently, much of the data from the first and second experimental harvests at Caspar Creek were made publicly available online on the USDA Forest Service Research Data Archive (Richardson et al., 2021a,b) and are described in Richardson et al. (2021c). Improving access to data should be a major goal of those responsible for collecting and managing paired watershed data, and several other data repositories are supported by publishers and funding organizations to help meet this goal.
Many publications now require that all data used in their articles be archived in a public repository according to the FAIR (findable, accessible, interoperable, and reusable) Data Principles [see Wilkinson et al. (2016) for additional information about FAIR principles]. These data policies are important for guaranteeing transparency and increasing data availability, but they also place higher demands on data managers. In line with those policies, the USDA Forest Service strongly encourages archiving data and improving public access to data managed by the Forest Service, but much of previously collected Forest Service data are still not easily accessed online. Similarly, National Science Foundation (NSF)-funded projects require that data collected with NSF funds be made publicly available.
Since 2007, the NSF has funded Critical Zone Observatories (CZOs), which have often included a more in-depth scientific investigation of watersheds (e.g., Brantley et al., 2017) and have been paramount to natural process discovery. However, CZOs do not typically include treatments and are therefore unable to produce the same level of land-use information as using a manipulative experimental approach. A new wave of funding (Critical Zone Collaborative Network) began in 2020 that is intended to support new studies and includes support for a single Coordinating Hub, which is responsible for archiving data for the CZOs (NSF Program Solicitation 19–586). Those responsible for paired watershed studies would benefit from adopting a similar approach of centralized data management.
Until recently, the time-consuming efforts to improve data quality and accessibility have received limited recognition. Many authors have advocated for increased recognition of data publication in the form of citations (e.g., Callaghan et al., 2012; Kratz and Strasser, 2014; Candela et al., 2015), and some journals have developed publication types that are specifically intended to describe data. Receiving credit in the form of citations for published datasets without an accompanying manuscript would further incentivize proper archiving and documenting of data (Callaghan et al., 2012). As an example, the USDA Forest Service, among other organizations, has recently recognized published datasets as research products in its personnel evaluation standards.
Another issue facing scientists and data managers is that hydrologic analyses can only be as good as the data they are derived from, and this should encourage those of us responsible for archiving and disseminating data to do so with the utmost care. A careful balance must be struck to maintain high data quality while also making it available in a timely manner. A common protocol does not exist for data standards, although approaches have been suggested (e.g., Cai and Zhu, 2015; Gudivada et al., 2017). While it would be challenging to develop and implement, adopting a standard protocol for reviewing data would benefit the hydrologic community and encourage a minimum data review standard.
As with making data accessible, assessing data quality and maintaining consistent, high-quality data can be difficult, and developing an approach for assuring data quality and consistency throughout long records is an important consideration. Long-term studies often collect the same data using different protocols, which can complicate interpretation of results. For example, at Caspar Creek, suspended sediment samples have been collected using five different methods. Each method improved estimated suspended sediment loads but led to temporal changes in the record. Assessing and improving spatial and temporal consistency of data from long-term studies should be an important goal of the experimental watershed community. The use of machine learning techniques for data quality management is promising and may lead to a robust data quality assurance approach because machine learning can be used to identify questionable data and fill data gaps (e.g., Gudivada et al., 2017; Kim et al., 2020).
4.3. What does the future hold for paired watersheds?
Paired watershed studies are demanding. They operate best when scientists and funding agencies are committed to high-quality and consistent long-term management, including the data products. Short-term funding cycles and demanding publication timelines are unfortunately not conducive to maintaining long-term experimental sites. In recent years, when post-harvest monitoring duties are substantial, six to twelve USDA Forest Service employees are involved with the collection, processing, maintenance, and analysis of Caspar Creek data. The California Department of Forestry and Fire Protection (CAL FIRE) and other funding agencies and partners provide additional support. Since the project’s inception, hundreds of USDA Forest Service and CAL FIRE personnel, other state and federal employees and volunteers, university scientists, and students have been involved with collecting and analyzing Caspar Creek data. One of the goals for those involved with Caspar Creek is to make the data accessible for a wide range of studies (e.g., Keppeler et al., 2003; Ferrier et al., 2005; Keppeler, 2016; Coble et al., 2020; Richardson et al., 2020). This is not a small task and funds specifically allotted to data review and distribution have helped achieve this goal at Caspar Creek.
Neary (2016) suggested that although landscape-level monitoring (e.g., USGS monitoring of 7,200 gaging stations) is important, paired watershed studies are essential and more likely to identify changes in hydrologic processes under changing climate conditions. The next generation of paired watershed studies are likely to adopt analytical approaches that enable investigations of shallow groundwater movement and the ecohydrologic connections between streamflow, soil moisture, rock moisture, land use, and vegetation. Indeed, these analytical approaches are being applied to the third Caspar Creek experiment (Dymond et al., 2021). Acknowledging the importance of continuing paired watershed studies into the 21st century, Neary (2016) realized that a large-scale monitoring network of paired watersheds is cost prohibitive. Instead, Neary (2016) suggested that a balance must be struck between higher spatial coverage offered by monitoring networks such as the NSF-funded USA Long Term Ecological Research (LTER) network and the National Ecological Observatory Network (NEON), and less spatial coverage but higher detail offered at paired watersheds. Opportunities also exist to develop new paired watershed studies in North America where spatial gaps are present, such as the EPA Level 1 Ecoregions that have few or no paired watershed studies (Figure 1). Maintaining paired watershed studies in as many ecoregions of North America as possible is important for understanding how climate change may affect these areas differently and to address region-specific science questions and best management practices. We expect that paired watersheds will remain an essential research and monitoring tool throughout the 21st century. Moving forward, the critical decision may be deciding how many paired watersheds we can afford to monitor and which ones are maintained continuously.
5. Conclusion
Long-term studies like Caspar Creek and others included in our compilation of North American paired watersheds are invaluable for testing treatments, gaining insight into hydrologic and geomorphic processes, and developing and confirming hydrologic and climate models. We presented three main reasons why paired watershed experiments are still relevant, including: they allow for watershed-scale experiments that inform us about hydrologic processes, water quantity, water quality, and nutrient cycling; they produce long climate, streamflow, and water quality records which are essential for long-term trend assessments; and they produce data that are ideal for developing and testing hydrologic models. For paired watershed studies to maximize their continued relevance, new and important questions that are well-suited to the paired watershed approach will need to be tested and the effects of land-use management decisions will need to be continually assessed. In addition to Caspar Creek, we hope that many other paired watershed experiments will continue to operate into the next century, and that their past and future data are made accessible. These experiments continue to prove invaluable for addressing a range of questions regarding best management practices, offer insight into hydrologic processes, and serve as important bellwethers for climate change and cumulative effects.
Data availability statement
The original contributions presented in the study are included in the article/Supplementary material, further inquiries can be directed to the corresponding author.
Author contributions
PR: Conceptualization, Data curation, Formal analysis, Methodology, Visualization, Writing – original draft, Writing – review & editing, Investigation. PC: Conceptualization, Data curation, Investigation, Methodology, Writing – original draft, Writing – review & editing. SD: Conceptualization, Data curation, Investigation, Methodology, Writing – original draft, Writing – review & editing. EK: Conceptualization, Data curation, Investigation, Methodology, Writing – original draft, Writing – review & editing. JWW: Conceptualization, Data curation, Investigation, Methodology, Supervision, Writing – original draft, Writing – review & editing. JAW: Conceptualization, Data curation, Methodology, Writing – original draft, Writing – review & editing, Investigation.
Funding
The author(s) declare financial support was received for the research, authorship, and/or publication of this article. Funding was supplied by the USDA Forest Service Pacific Southwest Research Station and the California Department of Forestry and Fire Protection.
Acknowledgments
We thank the scientists, technicians, students, agency managers, and volunteers who have diligently contributed to the longevity of the Caspar Creek Experimental Watersheds and other paired watersheds. We also thank the scientists who oversee the paired watersheds included in our compilation for supplying us with site details and data availability information. In addition, we thank two reviewers for comments that improved this manuscript.
Conflict of interest
The authors declare that the research was conducted in the absence of any commercial or financial relationships that could be construed as a potential conflict of interest.
The author(s) declared that they were an editorial board member of Frontiers, at the time of submission. This had no impact on the peer review process and the final decision.
Publisher’s note
All claims expressed in this article are solely those of the authors and do not necessarily represent those of their affiliated organizations, or those of the publisher, the editors and the reviewers. Any product that may be evaluated in this article, or claim that may be made by its manufacturer, is not guaranteed or endorsed by the publisher.
Supplementary material
The Supplementary material for this article can be found online at: https://www.frontiersin.org/articles/10.3389/ffgc.2023.1275392/full#supplementary-material
References
Adams, M. B., Loughry, L. H., and Plaugher, L. L.. (2004). “Experimental forests and ranges of the USDA Forest Service.” General Technical Report NE-321, Newton Square, PA: USDA Forest Service, Northeastern Research Station.
Amatya, D. M., Campbell, J., Wohlgemuth, P., Elder, K., Sebestyen, S., Johnson, S., et al. (2016). “Hydrological processes of reference watersheds in Experimental Forests, USA” in Forest Hydrology: Processes, Management, and Applications., Eds. D. M. Amatya, T. M. Williams, L. Bren, and C. de Jong (Wallingford, UK: CABI Publishers), 219–239.
Bailey, R. G. (1989). Explanatory supplement to ecoregions map of the continents. Environ. Conserv. 16, 307–309. doi: 10.1017/S0376892900009711
Bates, C. G., and Henry, A. J. (1928). Forest and streamflow experiment at Wagon Wheel Gap, Colorado. Mon. Weather Rev. Suppl. 30:79.
Beven, K. (2006). A manifest for the equifinality thesis. J. Hydrol. 320, 18–36. doi: 10.1016/j.jhydrol.2005.07.007
Blackburn, W. H., Wood, J. C., and DeHaven, M. G. (1985). “Forest harvesting and site preparation impacts on stormflow and water quality in East Texas” in Proceedings of forestry and water quality: A mid-south symposium. ed. B. G. Blackmon (Monticello, AR: Department of Forestry Resources, University of Arkansas)
Bosch, J. M., and Hewlett, J. D. (1982). A review of catchment experiments to determine the effect of vegetation changes on water yield and evapotranspiration. J. Hydrol. 55, 3–23. doi: 10.1016/0022-1694(82)90117-2
Bradford, M. J., and Heinonen, J. S. (2008). Low flows, instream flow needs and fish ecology in small streams. Canadian Water Res. J. 33, 165–180. doi: 10.4296/cwrj3302165
Brantley, S. L., McDowell, W. H., Dietrich, W. E., White, T. S., Kumar, P., Anderson, S. P., et al. (2017). Designing a network of critical zone observatories to explore the living skin of the terrestrial earth. Earth Surf. Dyn. 5, 841–860. doi: 10.5194/esurf-5-841-2017
Burt, T. P., and McDonnell, J. J. (2015). Whither field hydrology? The need for discovery science and outrageous hydrological hypotheses. Water Resour. Res. 51, 5919–5928. doi: 10.1002/2014WR016839
Burton, T. A. (1997). Effects of basin-scale timber harvest on water yield and peak streamflow. J. Am. Water Resour. Assoc. 33, 1187–1196. doi: 10.1111/j.1752-1688.1997.tb03545.x
Cafferata, P. H., and Reid, L. M.. (2013). “Applications of long-term watershed research to forest management in California: 50 years of learning from the Caspar Creek experimental watersheds.” California Forestry Report No. 5. California Department of Forestry and Fire Protection.
Cai, L., and Zhu, Y. (2015). The challenges of data quality and data quality assessment in the big data era. Data Sci. J. 14, 1–10. doi: 10.5334/dsj-2015-002
Callaghan, S., Donegan, S., Pepler, S., Thorley, M., Cunningham, N., Kirsch, P., et al. (2012). Making data a first class scientific output: data citation and publication by NERC’s environmental data centres. Int. J. Digit. Curation 7, 107–113. doi: 10.2218/ijdc.v7i1.218
Candela, L., Castelli, D., Manghi, P., and Tani, A. (2015). Data journals: A survey. J. Assoc. Inf. Sci. Technol. 66, 1747–1762. doi: 10.1002/asi.23358
Coble, A. A., Barnard, H., Du, E., Johnson, S., Jones, J., Keppeler, E., et al. (2020). Long-term hydrological response to forest harvest during seasonal low flow: potential implications for current forest practices. Sci. Total Environ. 730:138926. doi: 10.1016/j.scitotenv.2020.138926
Cosby, B. J., Norton, S. A., and Kahl, J. S. (1996). Using a paired-catchment manipulation experiment to evaluate a catchment-scale biogeochemical model. Sci. Total Environ. 183, 49–66. doi: 10.1016/0048-9697(95)04965-7
DeBano, L. F., Baker, M. B., and Overby, S. T. (1999). “Managing chaparral in Yavapai County” in History of watershed research in the Central Arizona Highlands. ed. M. B. Baker Jr. (Fort Collins, CO: USDA Forest Service, Rocky Mountain Research Station), 19–26. General Technical Report RMRS-GTR-29.
Dunn, P. H., Barro, S. C., Wells, W. G., Poth, M. A., Wohlgemuth, P. M., and Colver, C. G.. (1988). “The San Dimas Experimental Forest: 50 years of research.” General Technical Report PSW-104. Berkeley, CA: USDA Forest Service, Pacific Southwest Forest and Range Experiment Station.
Dymond, S. F., Richardson, P. W., Webb, L. A., Keppeler, E. T., Arismendi, I., Bladon, K. D., et al. (2021). A field-based experiment on the influence of stand density reduction on watershed processes at the Caspar Creek Experimental Watersheds. Front. For. Glob. Change 4:691732. doi: 10.3389/ffgc.2021.691732
Elliot, W. J., and Glaza, B. D. (2009). “Impacts of forest management on runoff and erosion” in Planning for an uncertain future-monitoring, integration, and adaptation; proceedings of the third interagency conference on research in the watersheds. eds. R. M. T. Webb and D. J. Semmens, Scientific Investigations Report 2009–5049 (Estes Park, CO: US Geological Survey), 117–127.
Ferrier, K. L., Kirchner, J. W., and Finkel, R. C. (2005). Erosion rates over millennial and decadal timescales at Northern California Coast Ranges. Earth Surf. Process. Landf. 30, 1025–1038. doi: 10.1002/esp.1260
Goodrich, D., Heilman, P., Anderson, M., Baffaut, C., Bonta, J., Bosch, D., et al. (2021). The USDA-ARS experimental watershed network: evolution, lessons learned, societal benefits, and moving forward. Water Resour. Res. 57:e2019WR026473. doi: 10.1029/2019WR026473
Gottfried, G. J., Neary, D. G., and Ffolliott, P. F. (2007). “An ecosystem approach to determining effects of prescribed fire on southwestern borderlands oak savannas: a baseline study” in Proceedings of the 23rd tall timbers fire ecology conference; fire in grassland and shrubland ecosystems. eds. R. E. Masters and K. E. M. Galley (Tallahassee, FL: Tall Timbers Research Station), 140–146.
Gudivada, V., Apon, A., and Ding, J. (2017). Data quality considerations for big data and machine learning: going beyond data cleaning and transformations. Int. J. Adv. Softw. 10, 1–20.
Gupta, H. V., and Nearing, G. S. (2014). Debates—the future of hydrological sciences: A (common) path forward? Using models and data to learn: A systems theoretic perspective on the future of hydrological science. Water Resour. Res. 50, 5351–5359. doi: 10.1002/2013WR015096
Harr, R. D. (1980). “Streamflow after patch logging in small drainages within the Bull Run Municipal Watershed, Oregon” in USDA For. Ser. Res. Pap. PNW-268 16 p., illus., (Portland, Oregon: USDA Forest Service, Pacific Northwest Forest and Range Experiment Station).
Harvey, B. C., Nakamoto, R. J., and White, J. L. (2006). Reduced streamflow lowers dry-season growth of rainbow trout in a small stream. Trans. Am. Fish. Soc. 135, 998–1005. doi: 10.1577/T05-233.1
Hatten, J. A., Segura, C., Bladon, K. D., Hale, V. C., Ice, G. G., and Stednick, J. D. (2018). Effects of contemporary forest harvesting on suspended sediment in the Oregon Coast Range: Alsea watershed study revisited. For. Ecol. Manag. 408, 238–248. doi: 10.1016/j.foreco.2017.10.049
Hayes, D. C., Stout, S. L., Crawford, R. H., and Hoover, A. P. (Eds.). (2014). USDA Forest Service experimental forests and ranges: Research for the long term. New York: Springer.
Hetherington, E. D. (1985). Streamflow nitrogen loss following forest fertilization in a southern Vancouver Island watershed. Can. J. For. Res. 15, 34–41. doi: 10.1139/x85-008
Hewlett, J. D., Lull, H. W., and Reinhart, K. G. (1969). In defense of experimental watersheds. Water Resour. Res. 5, 306–316. doi: 10.1029/WR005i001p00306
Horton, R. E. (1931). The field, scope, and status of the science of hydrology. Trans. Am. Geophys. Union 12, 189–202. doi: 10.1029/TR012i001p00189-2
Ingebo, P. A. (1972). “Converting chaparral to grass to increase streamflow.” In: Hydrology and water resources in Arizona and the southwest. Proceedings of the 1972 meetings of the Arizona section, American Waters Resources Association and the Hydrology Section, Arizona Academy of Science, 181–192. Prescott. Arizona: Arizona Academy of Science. May 5–6, 1972.
Jackson, C. R., Webster, J. R., Knoepp, J. D., Elliott, K. J., Emanuel, R. E., Caldwell, P. V., et al. (2018). Unexpected ecological advances made possible by long-term data: A Coweeta example. Wiley Interdiscip. Rev. Water 39:e1273. doi: 10.1002/wat2.1273
Keppeler, E. T. (2012). “Sediment production in a coastal watershed: legacy, land use, recovery, and rehabilitation.” In: Proceedings of coast redwood forests in a changing California: A symposium for scientists and managers, edited by R.B. Standiford, T.J. Weller, D.D. Piirto, and J.D. Stuart, 69–77. General Technical Report PSW-GTR-238. Albany, CA: USDA Forest Service, Pacific Southwest Research Station.
Keppeler, E. T. (2016). “Hydrologic change in a coast redwood forest, Caspar Creek Experimental Watersheds: implications for salmonid survival” in Headwaters to estuaries: Advances in watershed science and management-proceedings of the fifth interagency conference on research in the watersheds. eds. C. E. Stringer, K. W. Krauss, and J. S. Latimer, e-General Technical Report SRS-211 (North Charleston, SC: USDA Forest Service, Southern Research Station. March 2–5, 2015)
Keppeler, E. T., Cafferata, P. H., and Baxter, W. T.. (2007). “State Forest road 600: a riparian road decommissioning case study in Jackson demonstration state Forest.” California Forestry Note no. 120, California Department of Forestry and Fire Protection.
Keppeler, E. T., and Lewis, J.. (2007). “Understanding the hydrologic consequences of timber-harvest and roading: four decades of streamflow and sediment results from the Caspar Creek Experimental Watersheds.” In: Advancing the fundamental sciences: Proceedings of the Forest Service National Earth Sciences Conference, San Diego, CA, 18–22 October 2004, edited by M.J. Furniss, C.F. Clifton, K.L. Ronnenberg, 191–196. General Technical Report PNW-GTR-689. Portland, OR: USDA Forest Service, Pacific Northwest Research Station.
Keppeler, E. T., Lewis, J., and Lisle, T. E. (2003). “Effects of forest management on streamflow, sediment yield, and erosion, Caspar Creek Experimental Watersheds” in First interagency conference on research in the watersheds. October 27–30, 2003; eds. K. G. Renard, S. A. McElroy, W. J. Gburek, H. E. Canfield, and R. L. Scott (USDA Agricultural Research Service), 77–82.
Keppeler, E., Reid, L., and Lisle, T. (2009). “Long-term patterns of hydrologic response after logging in a coastal redwood forest” in Planning for an uncertain future–monitoring, integration, and adaptation. Proceedings of the third interagency conference on research in the watersheds. eds. R. M. T. Webb and D. J. Semmens (Estes Park, CO: U.S. Geological Survey Scientific Investigations Report 2009–5049. September 8–11, 2008), 265–272.
Kim, Y., Johnson, M. S., Knox, S. H., Black, T. A., Dalmagro, H. J., Kang, M., et al. (2020). Gap-filling approaches for eddy covariance methane fluxes: A comparison of three machine learning algorithms and a traditional method with principal component analysis. Glob. Chang. Biol. 26, 1499–1518. doi: 10.1111/gcb.14845
King, J. G., and Tennyson, L. C. (1984). Alteration of streamflow characteristics following road construction in north central Idaho. Water Resour. Res. 20, 1159–1163. doi: 10.1029/WR020i008p01159
Kirchner, J. W. (2006). Getting the right answers for the right reasons: linking measurements, analyses, and models to advance the science of hydrology. Water Resour. Res. 42:W03S04. doi: 10.1029/2005WR004362
Kratz, J., and Strasser, C. (2014). Data publication consensus and controversies. F1000Res. 3:94. doi: 10.12688/f1000research.3979.3
Lewis, J. (1998). “Evaluating the impacts of logging activities on erosion and suspended sediment transport in the Caspar Creek watersheds” in Proceedings of the conference on coastal watersheds: The Caspar Creek story. ed. R. R. Ziemer , General Technical Report PSW GTR-168 (Albany, California: USDA Forest Service, Pacific Southwest Research Station. May 6, 1998), 55–69.
Lugo, A. E., Swanson, F. J., González, O. R., Adams, M. B., Palik, B., Thill, R. E., et al. (2006). Long-term research at the USDA Forest Service's experimental forests and ranges. Bioscience 56, 39–48. doi: 10.1641/0006-3568(2006)056[0039:LRATUF]2.0.CO;2
MacDonald, L. H., and Coe, D. R.. (2008). “Road sediment production and delivery: processes and management.” In: Proceedings of the first world landslide forum, international Programme on landslides and international strategy for disaster reduction, 381–384. Tokyo, Japan: United Nations University.
Madej, M. A. (2001). Erosion and sediment delivery following removal of forest roads. Earth Surf. Process. Landf. 26, 175–190. doi: 10.1002/1096-9837(200102)26:2<175::AID-ESP174>3.0.CO;2-N
McBroom, M. W., Beasley, R. S., Chang, M., Gowin, B., and Ice, G. G. (2003). Runoff and sediment losses from annual and unusual storm events from the Alto Experimental Watersheds, Texas: 23 years after silvicultural treatments, vol. 7. Nacogdoches, TX: Faculty Publications.
McDonnell, J. J. (2003). Where does water go when it rains? Moving beyond the variable source area concept of rainfall-runoff response. Hydrol. Process. 17, 1869–1875. doi: 10.1002/hyp.5132
McDonnell, J. J., Evaristo, J., Bladon, K. D., Buttle, J., Creed, I. F., Dymond, S. F., et al. (2018). Water sustainability and watershed storage. Nat. Sustain. 1, 378–379. doi: 10.1038/s41893-018-0099-8
Moore, R. D., and Scott, D. F. (2005). Camp Creek revisited: streamflow changes following salvage harvesting in a medium-sized, snowmelt-dominated catchment. Canadian Wat. Res. J. 30, 331–344. doi: 10.4296/cwrj3004331
Nearing, G. S., Kratzert, F., Sampson, A. K., Pelissier, C. S., Klotz, D., Frame, J. M., et al. (2021). What role does hydrological science play in the age of machine learning? Water Resour. Res. 57:e2020WR028091. doi: 10.1029/2020WR028091
Neary, D. G. (2016). Long-term forest paired catchment studies: what do they tell us that landscape-level monitoring does not? Forests 7:164. doi: 10.3390/f7080164
Neary, D. G., Gottfried, G. J., Ffolliott, P. F., and Poff, B. (2012). Long-term forest watershed studies in the southwest: recycled for wildfire and prescribed fire. Hydrol. Water Res. Arizona Southwest 42, 55–60.
Neary, D., Hayes, D., Rustad, L., Vose, J., Gottfried, G., Sebesteyn, S., et al. (2012). “US Forest Service experimental forests and ranges network: a continental research platform for catchment-scale research” in Revisiting experimental catchment studies in Forest hydrology. ed. A. A. Webb (Melbourne, Australia: The International Union of Geodesy and Geophysics. July 6–8, 2011), 49–57. IAHS Publication 353.
Niemeyer, R. J., Bladon, K. D., and Woodsmith, R. D. (2020). Long-term hydrologic recovery after wildfire and post-fire forest management in the interior Pacific northwest. Hydrol. Process. 34, 1182–1197. doi: 10.1002/hyp.13665
Omernik, J. M. (1987). Ecoregions of the conterminous United States. Map (scale 1:7,500,000). Ann. Assoc. Am. Geogr. 77, 118–125. doi: 10.1111/j.1467-8306.1987.tb00149.x
Pate, A. A., Segura, C., and Bladon, K. D. (2020). Streamflow permanence in headwater streams across four geomorphic provinces in Northern California. Hydrol. Process. 34, 4487–4504. doi: 10.1002/hyp.13889
Perry, T. D., and Jones, J. A. (2017). Summer streamflow deficits from regenerating Douglas-fir forest in the Pacific Northwest, USA. Ecohydrology 10:e1790. doi: 10.1002/eco.1790
Pomeroy, J., Fang, X., and Ellis, C. (2012). Sensitivity of snowmelt hydrology in Marmot Creek, Alberta, to forest cover disturbance. Hydrol. Process. 26, 1891–1904. doi: 10.1002/hyp.9248
Reid, L. M. (2012). “Comparing hydrologic responses to tractor-yarded selection and cable-yarded clearcut logging in a coast redwood forest” in Proceedings of coast redwood forests in a changing California: A symposium for scientists and managers. eds. R. B. Standiford, T. J. Weller, D. D. Piirto, and J. D. Stuart, General Technical Report PSW-GTR-238 (Albany, CA: USDA Forest Service, Pacific Southwest Research Station), 151–161.
Reid, L. M., and Dunne, T. (1984). Sediment production from forest road surfaces. Water Resour. Res. 20, 1753–1761. doi: 10.1029/WR020i011p01753
Richardson, P. W., Seehafer, J. E., Keppeler, E. T., Sutherland, D. G., and Wagenbrenner, J. W. (2021a). Caspar Creek Experimental Watersheds Phase 1 (1962–1985) data. 2nd Edn. Fort Collins, CO: USDA Forest Service research data archive.
Richardson, P. W., Seehafer, J. E., Keppeler, E. T., Sutherland, D. G., and Wagenbrenner, J. W. (2021c). Fifty-eight years and counting of watershed science at the Caspar Creek Experimental Watersheds in Northern California. Hydrol. Process. 35:e14207. doi: 10.1002/hyp.14207
Richardson, P. W., Seehafer, J. E., Keppeler, E. T., Sutherland, D. G., Wagenbrenner, J. W., Bladon, K. D., et al. (2021b). Caspar Creek Experimental Watersheds Phase 2 (1985–2017) data. 2nd Edn. Fort Collins, CO: USDA Forest Service research data archive.
Richardson, P. W., Wagenbrenner, J. W., Sutherland, D. G., and Lisle, T. E. (2020). Measuring and modeling gravel transport at Caspar Creek, CA to detect changes in sediment supply, storage, and transport efficiency. Water Resour. Res. 56:e2019WR026389. doi: 10.1029/2019WR026389
Silvello, G. (2018). Theory and practice of data citation. J. Assoc. Inf. Sci. Technol. 69, 6–20. doi: 10.1002/asi.23917
Slaughter, C. W., and Richardson, C. W. (2000). Long-term watershed research in USDA Agricultural Research Service. Water Res. Impact 2, 28–31.
Stednick, J. D. (1996). Monitoring the effects of timber harvest on annual water yield. J. Hydrol. 176, 79–95. doi: 10.1016/0022-1694(95)02780-7
Stubblefield, A., Beach, S., Harrison, N. M., and Haskins, M. (2017). “Watershed-scale evaluation of Humboldt Redwood Company’s habitat conservation plan timber harvest best management practices, Railroad Gulch, Elk River, California” in Coast Redwood Science Symposium—2016: Past successes and future direction. Proceedings of a workshop. eds. R. B. Standiford and Y. Valachovic (Albany, CA: USDA Forest Service, Pacific Southwest Research Station), 105–115. General Technical Report PSW-GTR-258.
Thomas, R. B. (1990). Problems in determining the return of a watershed to pretreatment conditions: techniques applied to a study at Caspar Creek, California. Water Resour. Res. 26, 2079–2087. doi: 10.1029/WR026i009p02079
Troendle, C. A., Wilcox, M. S., Bevenger, G. S., and Porth, L. S. (2001). The Coon Creek Water Yield Augmentation Project: implementation of timber harvesting technology to increase streamflow. For. Ecol. Manag. 143, 179–187. doi: 10.1016/S0378-1127(00)00516-8
Wilder, B. A., Lancaster, J. T., Cafferata, P. H., Coe, D. B. R., Swanson, B. J., Lindsay, D. N., et al. (2020). An analytical solution for rapidly predicting post-fire peak streamflow for small watersheds in southern California. Hydrol. Process. 35, 1–14. doi: 10.1002/hyp.13976
Wilkinson, M. D., Dumontier, M., Aalbersberg, I. J., Appleton, G., Axton, M., Baak, A., et al. (2016). The FAIR guiding principles for scientific data management and stewardship. Sci. Data 3, 1–9. doi: 10.1038/sdata.2016.18
Zégre, N., Skaugset, A. E., Som, N. A., McDonnell, J. J., and Ganio, L. M. (2010). In lieu of the paired catchment approach: hydrologic model change detection at the catchment scale. Water Resour. Res. 46:W11544. doi: 10.1029/2009WR008601
Zhong, Y., Zou, C. B., Saenz, A., Stebler, E., Kakani, G., and Will, R. E. (2020). Conversion of encroached juniper woodland back to native prairie and to switchgrass increases root zone soil moisture and watershed runoff. J. Hydrol. 584:124640. doi: 10.1016/j.jhydrol.2020.124640
Ziemer, R. R. (1981). Stormflow response to roadbuilding and partial cutting in small streams of northern California. Water Resour. Res. 17, 907–917. doi: 10.1029/WR017i004p00907
Ziemer, R. R. (1998). “Flooding and stormflows” in Proceedings of the Conference on Coastal Watersheds: The Caspar Creek Story. ed. R. R. Ziemer , General Technical Report PSW GTR-168 (Albany, California: USDA Forest Service, Pacific Southwest Research Station. May 6, 1998), 15–24.
Keywords: paired watershed, experimental watershed, hydrology, treatment, timber harvest, data management, water resources management, Caspar Creek
Citation: Richardson PW, Cafferata PH, Dymond SF, Keppeler ET, Wagenbrenner JW and Whiting JA (2023) Past and future roles of paired watersheds: a North American inventory and anecdotes from the Caspar Creek Experimental Watersheds. Front. For. Glob. Change. 6:1275392. doi: 10.3389/ffgc.2023.1275392
Edited by:
Taehee Hwang, Indiana University Bloomington, United StatesReviewed by:
Mark B. Green, Case Western Reserve University, United StatesLuis Andrés Guillén, Swedish University of Agricultural Sciences, Sweden
Copyright © 2023 Richardson, Cafferata, Dymond, Keppeler, Wagenbrenner and Whiting. This is an open-access article distributed under the terms of the Creative Commons Attribution License (CC BY). The use, distribution or reproduction in other forums is permitted, provided the original author(s) and the copyright owner(s) are credited and that the original publication in this journal is cited, in accordance with accepted academic practice. No use, distribution or reproduction is permitted which does not comply with these terms.
*Correspondence: Paul W. Richardson, cGF1bC5yaWNoYXJkc29uQGNvbnNlcnZhdGlvbi5jYS5nb3Y=
†Present address: Paul W. Richardson, California Geological Survey, Redding, CA, United States