- 1Key Laboratory of State Forestry Administration on Forest Ecosystem Protection and Restoration of Poyang Lake Watershed, College of Forestry, Jiangxi Agricultural University, Nanchang, China
- 2Jiulianshan National Observation and Research Station of Chinese Forest Ecosystem, Jiangxi Agricultural University, Nanchang, China
- 3Administration of Jiulianshan National Nature Reserve, Ganzhou, China
- 4Department of Health and Environmental Sciences, Xi’an Jiaotong-Liverpool University, Suzhou, China
Soil C:N:P stoichiometry can regulate plant survival and reflect soil fertility and nutrient utilization. Despite the widespread conversion of old-growth forests to plantations or secondary forests, there is little knowledge about how these conversions affect the relation between leaf and soil stoichiometries. We examined the topography, leaf, and soil stoichiometries of 75 plots (20 m × 20 m) across Chinese fir plantations, secondary forests, and old-growth forests in subtropical China. We found that: (1) There were significant differences in leaf carbon, nitrogen, phosphorus, and their stoichiometry ratios among different stand types (2) stand type significantly affected soil SOC, TP, C:N, C:P, and N:P, except TN and (3) the explanation percentage of leaf stoichiometry on soil stoichiometry doubled with the conversion of old-growth forest to Chinese fir plantation, whereas it was twofold decreased with the conversion of old-growth forest to secondary forest. The explanation percentage of topography on soil stoichiometry decreased onefold at a minimum with the conversion of the old-growth forest to the Chinese fir plantation or the secondary forest. Our results show the shortages of soil nutrients from transforming old-growth forests into plantations or secondary forests and indicate the urgent need to preserve the remaining old-growth forests and increase stand ages by reducing forest disturbances. Therefore, determining the optimal stand type and slope location can effectively promote the accumulation of carbon, nitrogen, and phosphorus nutrients in the topsoil, which is essential for improving the planning and implementation of appropriate forest restoration and ecosystem management strategies.
1 Introduction
Three of the essential elements for plant organisms on earth are carbon (C), nitrogen (N), and phosphorus (P) (Chen and Chen, 2021) and they represent the main research focus of ecological stoichiometry. Ecological stoichiometry is a useful method for examining the balance and circulation of coupled elements (e.g., carbon, nitrogen, and phosphorus), connecting different levels of biology, from the gene to the globe, by scaling up elemental ratios (Zhang et al., 2018; Li et al., 2022). Indicators of soil quality, such as soil organic carbon (SOC), total nitrogen (TN), and total phosphorus (TP), can be used to assess the effectiveness of soil remediation. As the three major nutrients in the soil have the greatest impact on the ecosystem structure and function (Tian et al., 2010), the C:N:P ratio can be used to predict growth rates, identify limiting elements, and show how mineral elements distribute with the process of allometric growth (Zhao et al., 2016; Zhang et al., 2018). The interactions between these elements regulate ecological processes including energy transmission and the biological elemental cycle (Ladanai et al., 2010). Soil C:N:P stoichiometry can regulate plant survival and nutrient status and reflects soil fertility and nutrient availability (Elisabeth and Henderson, 2013). A meta-analysis shows that the average soil C:N:P ratio at the global scale is 186:13:1 (Cleveland and Liptzin, 2007), while the average soil C:N:P ratio in China is 60:5:1 (Tian et al., 2010; Zhang et al., 2013). Climate, topography, vegetation composition, and plant physiological constraints were used to explain the inconsistent C:N:P results between plants and soils in different regions (Ågren and Weih, 2012).
Topography is the major environmental factor affecting the distribution of soil nutrients on a small scale (Jiang et al., 2019). A key driver of vegetation patterns, species distribution, and ecosystem processes is spatial variation in slope and aspect (Bennie et al., 2008; Wang et al., 2022). The slope gradient is an essential local parameter that affects the erosion and deposition process and the intensity of soil, organic components, and nutrients (Zhu et al., 2014). Slope gradient impacts soil nutrients by altering soil erosion processes and their spatial pattern, and N and P contents are lower in steep slopes than those in gentle slopes (Wang et al., 2022). A study showed that slope had a significant positive linear correlation with the erosion intensity, due to an increase of slope runoff (Wang et al., 2023). Elevation gradient and slope aspect determined plant survival and leaf carbon: nitrogen: phosphorus (C:N:P) by controlling local water and energy balances (Cao et al., 2020). The slope aspect can regulate soil moisture and temperature by altering solar radiation and wind direction, affecting C, N, and P contents and their stoichiometries of plant and soil (Bennie et al., 2008). The aspect significantly affects the concentration of organic matter, total nitrogen, and total phosphorus in topsoil and also causes different element restrictions on plant growth on different slopes (Qin et al., 2021). As elevation increases, environmental factors such as hydrothermal conditions in alpine ecosystems will change significantly (Hu et al., 2019). These changes strongly impact soil and plant nutrients and plant stoichiometry (Mooshammer et al., 2017).
Plants and soils are inextricably intertwined and interact as distinct components of the biogeochemical cycle. Plants sequester C through photosynthesis and progressively add it to the soil in the form of litter, whereas soil offers all plant nutrients as the medium of plant growth (Wang and Zheng, 2021). Plant stoichiometry plays a critical role in predicting species’ response to the environment (Li et al., 2021). The C:N:P stoichiometric relationship between plants and soil can be a major ecological indicator for understanding ecosystem functions and processes (Chen and Chen, 2021). The C:N:P stoichiometry in leaf and soil can effectively explore the nutrient restriction, nutrient cycling, and plant response to climate change and environmental conditions in plants and can also be used to investigate nutrient regulators in plant-soil interactions (Liu et al., 2015; Cao et al., 2020). For example, a study in Taibai Mountain showed that except for the C:N ratio, soil C:N:P stoichiometry was significantly correlated with leaf C:N:P stoichiometry, and both had a close relationship with soil temperature and soil moisture (Zhang et al., 2019a). The dynamics of the leaf N:P ratio reflect soil N or P limitations across altitudinal gradients, causing by temperature-plant physiological hypotheses relating to C:N, C:P, and N:P (Reich and Oleksyn, 2004). A study of vegetation communities on the Loess Plateau proved that the stoichiometry of herbaceous communities was correlated with soil properties (except soil P) (Fang et al., 2019). However, some studies suggest that forest plants and soil have no relationship in C, N, and P concentrations (Ladanai et al., 2010). Therefore, it is necessary to further explore whether there is a strong association between leaf and soil stoichiometry in field experiment, and how stand type changes the correlation between leaf and soil stoichiometry.
As a result of economic development, vast tracts of old-growth forests all over the world have been transformed into plantations and secondary forests. It is critical to gain more knowledge on how soil stoichiometry reacts to these forest conversions (Chen et al., 2023). A study shows that soil nutrients are lost when old-growth forests are converted to secondary forests or plantations (Chen et al., 2023). The variations in soil stoichiometry and nutrient concentration are generally related to vegetation type (Zhang et al., 2019b). Many studies have shown that vegetation succession significantly affects the soil organic carbon, total N contents, and soil C:P and N:P ratios. The C:N:P in the soil served as a direct indicator of soil fertility, controlled vegetation patterns, and provided information about the plant’s nutritional status (Fan et al., 2015; Guan et al., 2015). Some studies have shown that old-growth forests have greater carbon storage capacity than plantations (Cao and Chen, 2017), and the C:N ratio of secondary forest plants increases significantly with increasing forest age. Vegetation restoration can increase soil nutrient cycling and sustain soil quality, by modifying plant species and community composition, litter quality, and quantity. Understanding the relationship between vegetation succession and changes in soil ecological function requires some knowledge of forest conversions (Ma et al., 2020). Understanding the variation of C:N:P stoichiometry in the post-afforestation can help us better understand the nutrient coupling between above- and below-ground ecological components and show the response of biogeochemical cycles in the progress of forest succession.
It is a severe threat to convert forests into other land use for subtropical forests, more than half of natural old-growth forests have been converted to secondary forests and plantations. In many areas of South China, the forest land has been slashed, burned, and planted with economic conifer species, mainly Chinese fir [Cunninghamia lanceolata (Lamb.) Hook.] (Jin et al., 2019; Yang et al., 2021). Chinese fir plantation make up 25% of all plantation stocks (0.625 billion m3) and 19% of China’s total forest area (8.93 million ha) (Chang et al., 2022). In this study, we aimed to assess how forest conversion (changes in stand type) affects topsoil stoichiometry. We examined topography factors, leaf and soil stoichiometry in Chinese fir plantations, natural secondary forests, old-growth forests (>100 years old) in subtropical China. The main objectives of this study were: (i) to explore whether the stoichiometry of soil topsoil could be predicted by leaf stoichiometry; and (ii) to explore whether different forest conversions affect the relationship between leaf and soil stoichiometry.
2 Materials and methods
2.1 Study site
The study area has a subtropical monsoon climate with an average annual precipitation (2155.6 mm) average annual temperature (16.4°C), located in Jiulianshan of Longnan County, Ganzhou City, Jiangxi Province, China (24° 30′ to 24° 39′ N, 114° 22′ to 114° 30′ E). This area is a low hilly landscape with the slope ranging from 10 to 50° and elevations between 500 and 790 m. The soil types in this area included red soil, loess, and meadow soil, and the soil type of this sampling was red soil. The vegetation types mainly include evergreen broad-leaved forest, coniferous forest, bamboo forest, mountain dwarf forest, shrub and grass, and wetland vegetation.
2.2 Sample plot setting
There were two ways of regeneration after clear-cutting 42 years ago. Competitive vegetation was manually removed in the first 3 years after planting for Chinese fir plantations and then the natural recovery of forests sustained without human intervention. The secondary forests recovered naturally after clear-cutting without human intervention and formed by seeds of local tree species in the soil. In addition, there were some old-growth forests without human activities for more than a century in our research region. Forest ages were derived from the records of forest management, provided by Local Forestry Bureau.
We established 75 plots of three stand types (Chinese fir plantations, secondary forests, and old-growth forests) in 2018 and selected those spatially interspersed plots, with a minimum distance of 100 between plots, in order to reduce spatial autocorrelation (Figure 1). Twenty-five plots with an area of 20 m × 20 m were randomly set up within each stand, and the diameter at breast height (DBH ≥ 1 cm) and heights of all trees in each plot were measured. The results of the vegetation investigation indicate that tree species of Chinese fir plantations primarily included Cunninghamia lanceolata, Castanopsis carlesii, Machilus nanmu, Diospyros morrisiana, Rhododendron moulmainense, Eurya japonica, Daphniphyllum oldhamii, Schima superba, Castanopsis fargesii, Pinus massoniana. The dominant species of natural secondary forests primarily included Ormosia xylocarpa, Photinia prunifolia, Styrax confusus, Diospyros morrisiana, Eurya nitida, Symplocos congesta, Schoepfia jasminodora, Dendropanax dentiger, Pinus massoniana, Micromelum falcatum. The tree species of the old-growth forests mainly included Rhododendron moulmainense, Eurya nitida, Neolitsea chui, Litsea elongata, Adinandra millettii, Neolitsea aurata, Cinnamomum subavenium, Alniphyllum fortunei, Eurya japonica, Diplospora dubia.
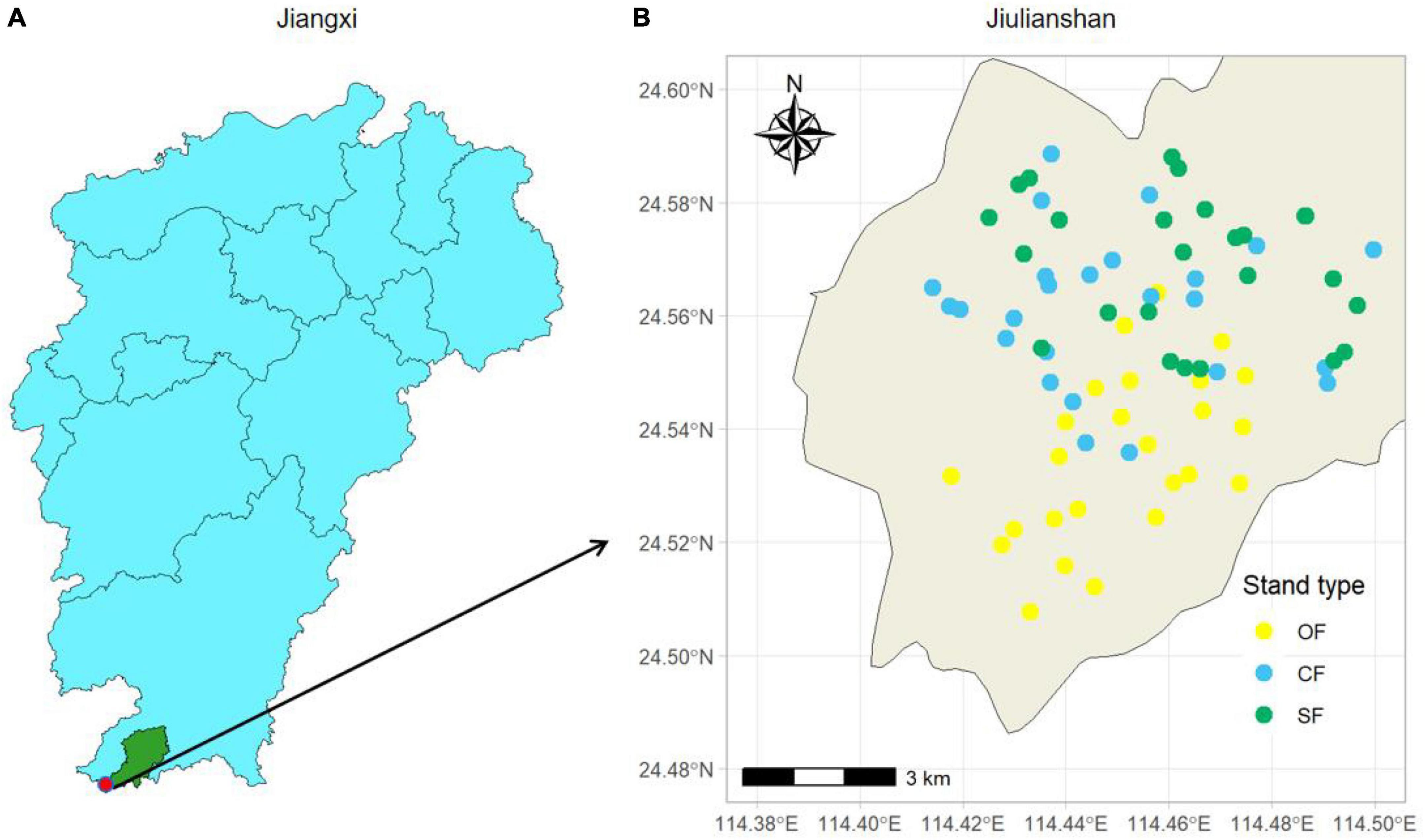
Figure 1. Location of study area in the Jiangxi Province (A) and the sample plots in Jiulianshan (B). OF, old-growth forest; CF: Chinese fir plantation; SF, secondary forest.
2.3 Data collection
Using standardized protocols for measuring plant functional traits, the leaf traits of all of these species were determined in at least ten well-growth individuals per species (4,203 individuals in total) in the old-growth forests, Chinese fir plantations, and secondary forests from July to August (growing season), 2018 (Cornelissen et al., 2003). For each individual, three to ten recently expanded and healthy leaves were collected and put into a self-sealing bag, including petioles and rachises of compound leaves. All leaf samples were oven-dried at 70°C for over 48 h to a constant mass and retained for subsequent chemical analysis.
In each plot, the soil sampling method is a fixed random sampling method; the 20 m × 20 m plot is divided into four 10 m × 10 m subplots, and soil samples (0–20 cm) are collected from the diagonal center of each 10 m × 10 m small plot, and the diagonal center of the 20 m × 20 m plot in July 2018, and after removed the litter and moss in the topsoil. Five parts of equal-depth soil were collected with a 5 cm diameter soil drill, and 375 soil samples were collected from 75 plots, sealed with a self-sealing bag, and back to the laboratory. Thegravel, visible roots, and plant debris were selected from soil samples in the lab. Then, samples of air-dried soil and dried leaves were ground into a fine powder. The total C and N concentrations in the soil and leaf samples were determined using an Elemental Analyser (Elementar Vario EL III; Elementar, Langenselbold, Germany). Using the molybdenum-antimony anti-colorimetric method, the total P concentrations of leaves and soil samples were examined and the nitrogen and phosphorus ratio was calculated (Chang et al., 2022); mass concentrations were used to express the levels of C, N, and P in the samples (Yang et al., 2018).
In this study, a total of 9 topography and leaf stoichiometry factors were selected, including three topography factors, including elevation (ELE), slope gradient (SLO), slope aspect (ASP), and six leaf stoichiometry factors, including community leaf carbon (LCC), leaf nitrogen (LN), leaf phosphorus (LP), leaf carbon-nitrogen ratio (C:N), leaf carbon-phosphorus ratio (C:P), and leaf nitrogen-phosphorus ratio (N:P). The elevation data were measured using GPS, and the slope and aspect were calculated using the standard method of plot data analysis (Legendre et al., 2009).
The community-level functional trait value (Community weighted mean, CWM) is calculated by weighting the functional trait value of the species in the plot and the abundance of each species in the plot. The specific formula is:
Where S is the species richness, Wi is the relative abundance of the ith species, and Ti is the trait value. CWM is calculated from the FD package in R software (Villéger et al., 2008).
2.4 Data analysis
Testing for normality indicated that all data met the assumptions of normality and homogeneity. To identify differences in the leaf and soil C, N, and P stoichiometric characteristics and topography among the stand types (including Chinese fir plantation, secondary forest, and old-growth forest), one-way ANOVA followed by multiple comparisons were performed. Significance was set at P < 0.05. Redundancy analysis was performed to assess the effects of leaf stoichiometry and topography on soil stoichiometry. The package “rdacca.hp” was used to quantify the single effect of factors and to obtain the contribution of leaf stoichiometry and topography to soil stoichiometry across different stand types in the redundancy analysis (Lai et al., 2022). All statistical analyses and plotting were calculated using the statistical software R4.2.2 (R Core Team, 2022).
3 Results
3.1 Characteristics of leaf stoichiometry and topography
There were significant differences in leaf carbon, nitrogen, phosphorus, and stoichiometry ratios among different stand types (Table 1). Leaf C content was the highest in the Chinese fir plantations (452.26 g kg–1), followed by the old-growth forests (445.77 g kg–1), and it was the lowest in the secondary forests (438.72 g kg–1). The leaf N content ranged from 14.51 to 20.29 g kg–1, with the highest content in old-growth forests and the lowest in Chinese fir plantations. The leaf P content of the Chinese fir plantations (0.79 g kg–1) was significantly lower than that of the other two stand types. The leaf C:N of the Chinese fir plantations (31.21) was significantly greater than that of the secondary forests, and there was also a significant difference between the secondary forests and the old-growth forests. The leaf C:P (574.57) of the Chinese fir plantations was significantly higher than that of the other two stand types. The N:P of secondary forests (17.60) was significantly lower than that of the other two stand types. The elevation ranged from 528.89 to 757.00 m, and it was higher in the secondary forests than that of the Chinese fir plantations and old-growth forests. The slope gradient ranged from 25.28 to 37.7, the steepest in the Chinese fir plantation and the slowest in the old-growth forest. There were no significant differences in the slope aspects among these stand types.
3.2 Variations in the soil stoichiometry under different stand types
There were significant differences of soil SOC, TP, C:N, C:P, and N:P, except TN, among different stand types (Figure 2). SOC was the highest in the old-growth forests, followed by Chinese fir plantations, and it was the lowest in the secondary forests. There was a non-significant difference in soil TP between the old-growth forests and secondary forest. Still, the soil TP of the Chinese fir plantations was significantly lower than that of the other two stand types. Soil C:N of the old-growth forests was significantly higher than that of the secondary forests, while there is no significant difference between the Chinese fir plantations and the other two stand types. Soil C:P of the secondary forests was significantly lower than that of old-growth forests and Chinese fir plantations. Soil N:P of Chinese fir plantations was significantly higher than that of old-growth forests and secondary forests, although it was a non-significant difference between the old-growth forests and secondary forests.
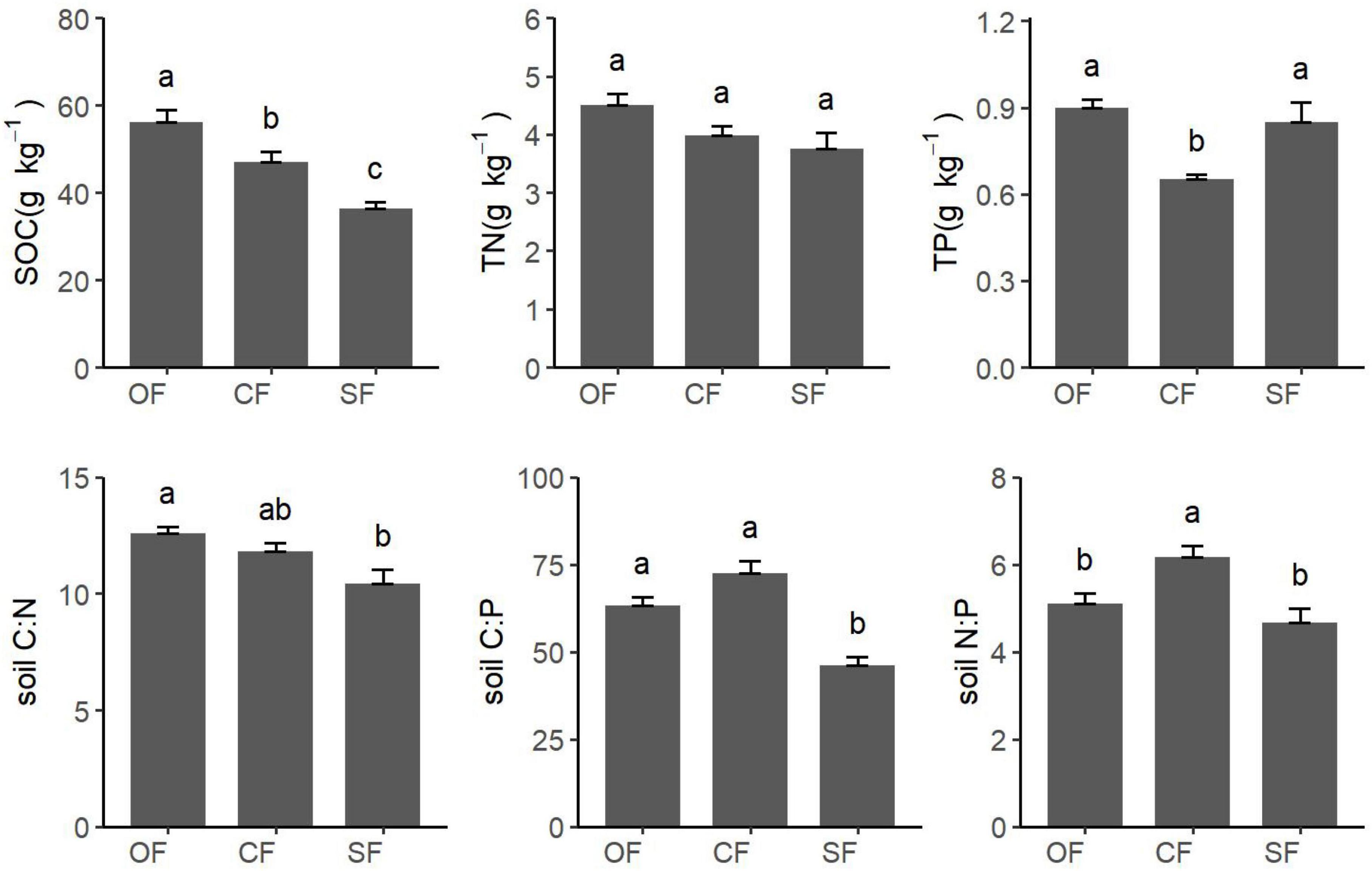
Figure 2. Variations in soil stoichiometry across different stand types. The lowercase letters mean the difference between different stand types (P < 0.05). SOC, community soil carbon; TN, community soil nitrogen; TP, community soil phosphorus; soil C:N, community soil carbon-nitrogen ratio; soil C:P, community soil carbon-phosphorus ratio; soil N:Pcommunity soil nitrogen-phosphorus ratio.
3.3 Effects of leaf stoichiometry and topography on soil stoichiometry
The top four important explanatory variables of the old-growth forests and Chinese fir plantations were slope aspect, slope gradient, leaf C:P, and leaf P for soil stoichiometry. In the old-growth forests, the slope aspect and slope gradient of topography were the most important factors, followed by leaf C:P, leaf P content, leaf C:N, and leaf N content (Figure 3A). In the Chinese fir plantations, leaf C:P and leaf P content of leaf stoichiometry are the most important factors, followed by the slope gradient, solpe aspect, leaf N:P, and C:N (Figure 3B). The elevation is the most important explanatory factor for soil stoichiometry in secondary forests, followed by leaf N:P, leaf N, and leaf C content (Figure 3C). The total explanation percentages of leaf stoichiometry and topography on soil stoichiometry were 51.72% for old-growth forests, 46.04% for Chinese fir plantations, and 15.27% for secondary forests (Figure 3D). The explanation percentage of leaf stoichiometry on soil stoichiometry doubled with the conversion of old-growth forests to Chinese fir plantations, whereas it was twofold decreased with the conversion of old-growth forests to secondary forests. The explanation percentage of topography on soil stoichiometry decreased onefold at a minimum with the conversion of the old-growth forests to the Chinese fir plantations or the secondary forests.
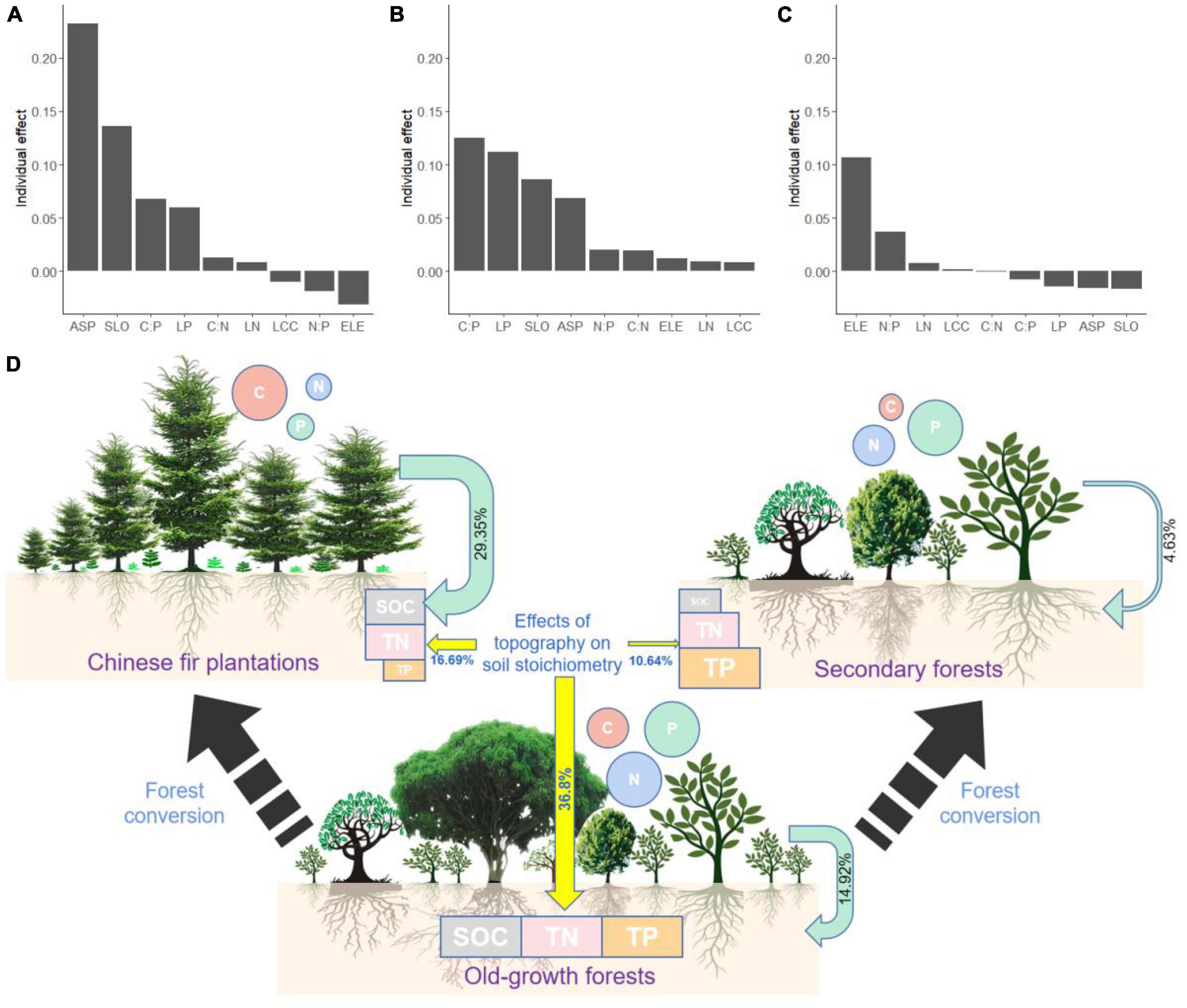
Figure 3. Effects of leaf stoichiometry and topography on soil stoichiometry among old-growth forests (A), Chinese fir plantations (B), secondary forests (C), and total effects through forest conversion (D). The size of the circle represents the content of leaf carbon, nitrogen and phosphorus, and the size of the rectangle represents the content of soil carbon, nitrogen and phosphorus. The green arrow indicates the effect of leaf stoichiometry on soil stoichiometry, the yellow arrow indicates the effect of topography on soil stoichiometry, and the arrow’s thickness indicates the magnitude of the effect.
4 Discussion
4.1 Responses of leaf stoichiometry to stand type
Our results show significant differences in leaf carbon, nitrogen, phosphorus and stoichiometry ratios among different stand types. The stoichiometry ratio of C, N and P is a key indicator of plant nutrient utilization and distribution, and the distribution ratio of C, N, and P in plants varies with the stand type (Wang et al., 2017). Different life forms of plants frequently exhibit varying stoichiometry in ecosystems, with different C, N, and P utilization strategies (Chang et al., 2022). Chinese fir plantations had the highest LCC content (452.26 g kg–1), which indicated that the leaves of Chinese fir plantations had higher organic compound concentration and higher carbon storage capacity than other stand types (Zhang et al., 2020). As with the results of a study in the Pearl River Delta in South China, coniferous forests have higher LCC than evergreen broadleaved forests (Wu et al., 2010). The LN and LP content and LN:LP of evergreen broadleaf forests were higher than those in coniferous forests (Ren et al., 2007). Evergreen trees have a long leaf lifespan, need to accumulate more organic substances (such as lignin, etc.) to build a defensive structure (Chen et al., 2020). The plant body maintains high N and P content, which is conducive to maintaining the growth and metabolism of vegetation (Zeng et al., 2016). The highest LN content was in the old-growth forests, whereas it was the lowest in the Chinese fir plantations (14.51∼20.29 g kg–1). This may be caused by conifer green leaves having less N, P content and nutrient uptake than other plant functional groups (Tong et al., 2021). Soil nitrogen deficiency affects LN content, photosynthesis, and LN distribution in many species (Tang et al., 2019). Secondary forests may have lower leaf N content than old-growth forests due to lower soil N supply than old-growth forests. The leaf P content and N:P value of all stand types in this study area were lower than the Chinese average (18.6 mg g–1) (Han et al., 2005). The main reason for the low leaf P content of plants in this area may be that the soil P content is generally low in southern China, resulting in low leaf P content. The LP content (0.79 g kg–1) of the Chinese fir plantations was significantly lower than that of the other two forest stands, which was due to the adaptation of nutrient-deficient habitat on the one hand and the strong coupling relationship between LP content and litter P content on the other hand (Tong et al., 2021).
Our study showed that leaf C:N and C:P ratios in the Chinese fir plantations were higher than that of other stand types. The C:N and C:P ratios of leaves are important physiological indicators related to plant growth rate and plant carbon assimilation capacity (Wang et al., 2018). Higher Leaf C:N and C:P in Chinese fir plantations indicates that the carbon assimilation capacity increases after being converted into Chinese fir plantations. The leaf N:P ratio can be used as an indicator for diagnosing nitrogen saturation and a threshold for determining nutrient limitations (Wang et al., 2018). The leaf N:P ratio in the three stands was larger than 16, indicating a general phosphorus limitation in the Jiulianshan subtropical forests. The N:P of the secondary forests was significantly lower than that of the other two stand types. The leaf N, P content, and N:P ratio of evergreen broadleaf forests were generally higher than those in coniferous forests (Ren et al., 2007).
4.2 Responses of soil stoichiometry to stand type
Our study significantly affects soil SOC, TP, C:N, C:P, and N:P, except TN among different stand types. Soil is a key environmental factor for plant survival, as it is an important source of plant nutrition in forest ecosystems. To support plant growth and development, plants absorb N and P from the soil, fix C through photosynthesis, and form litter at the surface after completing their life history, and some C, N, and P can gradually return to the soil after litter decomposition (Hobbie, 2015). The SOC, TN, and TP contents of old-growth forests were higher than those of Chinese fir plantations and secondary forests, possibly due to the high level of species richness and the large types and quantities of litter in the old-growth forests, which increased the input of SOC. The TP content of old-growth forests and secondary forests was higher than that of Chinese fir plantations, indicating a poor TP supply capacity in Chinese fir plantations. This may be due to the serious consumption of TP nutrient pool in the early stage of community formation after deforestation and the lack of fallen species and low litter decomposition rate in the Chinese fir plantations, the nutrient bank cannot be replenished in time, resulting in a low TP content in the Chinese fir plantations (Lambers et al., 2009).
To adapt to the microclimate environment produced by different stand types, plant communities adjust their nutrient distribution mechanisms, which in turn affects the balance strategy of soil nutrients (Sardans et al., 2012). The major forces behind soil biogeochemical processes are plant functional groups, which alter soil C:N:P stoichiometry through nutrient uptake, root exudates, and litter inputs (Zhou et al., 2018). An important indicator of the rate at which soil organic matter is mineralized and decomposed is soil C:N (Li et al., 2015). The soil C:N of old-growth forests was significantly higher than that of secondary forests, indicating that there is a high rate of mineralization and decomposition rate of soil organic matter in secondary forests. Plant residue input alters native SOC mineralization through the priming effect, controlling C sequestration during the process of vegetation restoration. A recent study shows that there is a higher SOC priming in the secondary forests of Southwest China (Cheng et al., 2022). Soil C:P is inversely proportional to soil P’s mineralization rate, reflecting soil P’s effectiveness (Tian et al., 2010). We found that soil C:P in secondary forests was significantly lower than that of old-growth forests and Chinese fir plantations, indicating a higher mineralization rate of soil P in the secondary forests. A study in the same region has also found that the Soil C:P increased from conversion of secondary forests to slash pine plantations in south China (Ding et al., 2021). In this study, the soil N:P of the Chinese fir plantations was significantly higher than that of the old-growth forest and secondary forest. This result indicates that an increase in soil N:P ratio can occur because of the greater solubility and loss of P from converting natural forests to Chinese fir plantations. A study in Fenyi County, Jiangxi Province, China, also shows that compared to natural forests, there is a relatively similar soil N but low soil P in Chinese fir plantations from 6 to 50 years old (Hou et al., 2021).
4.3 Effects of leaf stoichiometry and topography on soil stoichiometry across different forest conversions
Our results reported that the top four important explanatory variables of the old-growth forests and Chinese fir plantations were slope aspect, slope gradient, leaf C:P, and LP for soil stoichiometry. A key factor affecting vegetation patterns, species distribution, and ecosystem processes is spatial variation in slope gradient and slope aspect. The slope gradient mainly reflects water and light, thereby affecting soil microorganisms and leaching degree, indirectly affecting soil nutrients, and the underground diameter flow degree of different slopes after rainfall is different, resulting in different decomposition and nutrient return of litter under the forests (Bennie et al., 2008). Slope aspect also strongly influences plant growth and soil stoichiometry. The north-facing shady slope vegetation is thick and dense, and the soil is rich in nutrients, while the south-facing sunny slope vegetation is thin and scattered, the soil development is weak, and the erosion rate is high (Yang et al., 2020). Slope aspect was an important explanatory factor in soil stoichiometry due to south-facing sunny slope, sparse vegetation and high erosion rate, which amplified the influence of slope gradient on soil stoichiometry (Yang et al., 2020). Moreover, topography is a long-term influencing factor, slope gradient and slope aspect can comprehensively affect the redistribution of light, water and nutrients, including the processes of infiltration runoff, soil erosion and nutrient transport with the high annual average precipitation in Jiulianshan (Zhou et al., 2023). The cumulative effect of time continuously affects the spatial variation of soil nutrients, which is supported by the more stronger effect of topography of old-growth forests with more than 100 years on soil stoichiometry than that of Chinese fir plantations with 42 years. Vegetation cover alters ecosystem biogeochemical cycles (C, N, P) through plant residues and organic matter input, affecting soil physicochemical characteristics and microclimatic conditions (Zhou et al., 2023). In our study, there was the highest C:P ratio and lowest leaf P in the Chinese fir plantations, in contrast with the old-growth forest. The amount of understory litter in needle and broadleaf forests is different. The richer litter amount and faster decomposition rate of broadleaf forests accelerate the input of phosphorus from litter to soil. Those factors have a relatively large impact on soil P reservoir, as plants will rapidly absorb the available phosphorus produced by litter decomposition (Tang et al., 2013).
The total interpretation rate of leaf stoichiometry to soil stoichiometry was 14.92% for old-growth forests, 29.35% for Chinese fir plantations, and 4.63% for secondary forests (Figure 3D). This result shows tight relationships between leaf and topsoil stoichiometries after 42 years of forest conversion from old-growth forests to Chinese fir plantations and confirms the hypothesis (i) the stoichiometry of soil topsoil can be predicted by leaf stoichiometry. Furthermore, the results of our study also support the hypothesis that (ii) different forest conversions affect the relationship between leaf and soil stoichiometry. The explanation percentage of leaf stoichiometry on soil stoichiometry doubled with the conversion of old-growth forests to Chinese fir plantations, whereas it was twofold decreased with the conversion of old-growth forests to secondary forests. There is growing evidence that plant stoichiometry is an important driver of soil bioprocesses, and these results enhance our understanding of biogeographic scales in plant and soil states and ecosystem functions (Luo et al., 2015). In old-growth forests, species composition is usually relatively stable because of the complex ecological interactions between individual species (Liu et al., 2019). Since there is no human disturbance, nutrient internal cycling has been continuously running in old-growth forests, and it is not surprising that the correlation between leaf and soil stoichiometry has been observed. We found that forest conversion from old-growth forests to secondary forests and Chinese fir plantations had strongly decreased soil SOC, TN, and TP. The alteration of C:N:P stoichiometry in plant tissues during vegetation change is expected to have corresponding effects on soil C:N:P stoichiometry (Cao et al., 2018). Our results showed that leaf stoichiometry had tightly related to the stoichiometry of the soil after clear cutting 42 years ago, and the leaf C:P ratio and LP content are the most important explanatory factors in the Chinese fir plantations. P mainly depends on the decomposition of soil parent material, and the natural decomposition cycle of soil parent material is longer, with fewer influencing factors and less spatial variability (Schillereff et al., 2021). After converting the old-growth forests to a Chinese fir plantations, the low LP content of Chinese fir results in a low TP input and nutrient return rate to the soil through litter composition. Studies have revealed that compared with Chinese woody trees and global woody trees, there are low LN and LP contents in Chinese fir litter with low nutrient uptake efficiency (Tong et al., 2021). Furthermore, our results indicate a quickly decreased relationship between leaf stoichiometry and soil stoichiometry from the conversion of old-growth forests to secondary forests. The secondary forest was mainly pioneer species in the early stage, and some shade-tolerant tree species begin to colonize in the middle stage and initiate the process of species turnover (Dent et al., 2013). After 42 years, the structure of the secondary forests is mainly composed of a small number of pioneer tree species and a lot of shade-tolerant tree species. But soil nutrients in the secondary forest may mainly come from the litter decomposition of early pioneer species, and shade-tolerant tree species gradually replaced the position of pioneer species, resulting in a weak relationship between leaf and soil stoichiometry. A degraded tropical region of South China has undergone forest regeneration for more than 50 years, the biomass of secondary forests recovered quickly, but soil C stocks recovered slowly, compared with old-growth forests (Wang et al., 2017).
The explanation percentage of topography on soil stoichiometry decreased onefold at a minimum with the conversion of the old-growth forests to the Chinese fir plantations or the secondary forests. In general, nutrient stores in natural systems are higher than those in artificial systems, because there is less surface soil disturbance and more soil microbial activity in natural systems (Zhang et al., 2015). With the transformation of old-growth forests to Chinese fir plantations and secondary forests, surface disturbance increased, SOC, TN, and TP were gradually lost, and soil fertility decreased (Wang et al., 2014). In addition, preventing soil erosion and soil nutrient loss from runoff and sediment transport can alter the distribution of soil nutrients on hillsides by increasing surface vegetation cover and long-term vegetation restoration (Zhou et al., 2023).
5 Conclusion
Overall, our work sheds a crucial light on how forest conversions affect leaf and soil stoichiometries. According to our research, SOC, TN, and TP in the topsoil are significantly impacted when old-growth forests are converted to plantations and secondary forests. In Chinese fir plantations, the leaf traits (mainly LP) of the planted species proved to be a key factor affecting surface soil stoichiometry through the conversion from old-growth forests to Chinese fir plantations in the phosphorus-deficient subtropical forests. We should better understand the elemental coupling above and below ground to predict topsoil stoichiometry through leaf stoichiometry. Our findings highlight how important it is to preserve old-growth forests and maintain soil stoichiometry in subtropical forests. For more than 40 years, natural restoration alone cannot convert plantations or secondary forests into old-growth forests, and the soil nutrients are significantly lower than those of old-growth forests, indicating that the consequences of forest destruction are severe and long-term. Therefore, our study suggests promoting the accumulation of carbon, nitrogen, and phosphorus nutrients in soil topsoil by determining optimal stand types and slope locations. Understanding these knowledge is essential for planning and implementing appropriate forest restoration and ecosystem management strategies.
Data availability statement
The original contributions presented in the study are included in the article/Supplementary material, further inquiries can be directed to the corresponding author.
Author contributions
WB: Conceptualization, Data curation, Funding acquisition, Methodology, Project administration, Supervision, Writing – review and editing. CZ: Conceptualization, Formal analysis, Methodology, Writing – original draft. YuL: Conceptualization, Formal analysis, Methodology, Writing – original draft. XL: Investigation, Writing – original draft. FC: Methodology, Resources, Writing – review and editing. ZJ: Resources, Supervision, Writing – review and editing. YaL: Investigation, Visualization, Writing – original draft. YM: Investigation, Validation, Writing – original draft. SZ: Investigation, Validation, Writing – original draft. SY: Investigation, Validation, Writing – original draft.
Funding
The author(s) declare financial support was received for the research, authorship, and/or publication of this article. This work was supported by the National Natural Science Foundation of China (32360382), a special project of Forestry Science and Technology Innovation of Jiangxi Forestry Bureau and the Natural Science Foundation of Jiangxi Provincial Department of Science and Technology (20202ACBL215005).
Acknowledgments
We wish to express their thanks for the support received from the Jiulianshan Mountain Nature Reserve to allow us to collect samples. We would like to thank them for their help with field sampling and laboratory measurement.
Conflict of interest
The authors declare that the research was conducted in the absence of any commercial or financial relationships that could be construed as a potential conflict of interest.
Publisher’s note
All claims expressed in this article are solely those of the authors and do not necessarily represent those of their affiliated organizations, or those of the publisher, the editors and the reviewers. Any product that may be evaluated in this article, or claim that may be made by its manufacturer, is not guaranteed or endorsed by the publisher.
Supplementary material
The Supplementary Material for this article can be found online at: https://www.frontiersin.org/articles/10.3389/ffgc.2023.1268262/full#supplementary-material
References
Ågren, G. I., and Weih, M. (2012). Plant stoichiometry at different scales: element concentration patterns reflect environment more than genotype. New Phytol. 194, 944–952. doi: 10.1111/j.1469-8137.2012.04114.x
Bennie, J., Huntley, B., Wiltshire, A., Hill, M. O., and Baxter, R. (2008). Slope, aspect and climate: Spatially explicit and implicit models of topographic microclimate in chalk grassland. Ecol. Modell. 216, 47–59. doi: 10.1016/j.ecolmodel.2008.04.010
Cao, J., Wang, X., Adamowski, J. F., Biswas, A., Liu, C., Chang, Z., et al. (2020). Response of leaf stoichiometry of Oxytropis ochrocephala to elevation and slope aspect. Catena 194:104772. doi: 10.1016/j.catena.2020.104772
Cao, Y., and Chen, Y. (2017). Coupling of plant and soil C:N:P stoichiometry in black locust (Robinia pseudoacacia) plantations on the Loess Plateau, China. Trees 31, 1559–1570. doi: 10.1007/s00468-017-1569-8
Cao, Y., Zhang, P., and Chen, Y. (2018). Soil C:N:P stoichiometry in plantations of N-fixing black locust and indigenous pine, and secondary oak forests in Northwest China. J. Soils Sediments 18, 1478–1489. doi: 10.1007/s11368-017-1884-0
Chang, Y., Zhong, Q., Yang, H., Xu, C., Hua, W., and Li, B. (2022). Patterns and driving factors of leaf C, N, and P stoichiometry in two forest types with different stand ages in a mid-subtropical zone. For. Ecosyst. 9:100005. doi: 10.1016/j.fecs.2022.100005
Chen, C., Fang, X., Xiang, W., Lei, P., Ouyang, S., and Kuzyakov, Y. (2020). Soil-plant co-stimulation during forest vegetation restoration in a subtropical area of southern China. For. Ecosyst. 7, 1–17. doi: 10.1186/s40663-020-00242-3
Chen, X., and Chen, H. Y. H. (2021). Plant mixture balances terrestrial ecosystem C:N:P stoichiometry. Nat. Commun. 12:4562. doi: 10.1038/s41467-021-24889-w
Chen, X., Hu, M., Zheng, G., and Chen, H. Y. H. (2023). Persistent soil organic carbon deficits from converting primary forests to plantations and secondary forests in subtropical China. Glob. Ecol. Conserv. 45, e02530. doi: 10.1016/j.gecco.2023.e02530
Cheng, H., Zhou, X., Dong, R., Wang, X., Liu, G., and Li, Q. (2022). Priming of soil organic carbon mineralization and its temperature sensitivity in response to vegetation restoration in a karst area of Southwest China. Sci. Total Environ. 851:158400. doi: 10.1016/j.scitotenv.2022.158400
Cleveland, C. C., and Liptzin, D. (2007). C:N:P stoichiometry in soil: is there a “Redfield ratio” for the microbial biomass? Biogeochemistry 85, 235–252. doi: 10.1007/s10533-007-9132-0
Cornelissen, J. H. C., Lavorel, S., Garnier, E., Díaz, S., Buchmann, N., Gurvich, D. E., et al. (2003). A handbook of protocols for standardised and easy measurement of plant functional traits worldwide. Aust. J. Bot. 51:335. doi: 10.1071/BT02124
Dent, D. H., DeWalt, S. J., and Denslow, J. S. (2013). Secondary forests of central P anama increase in similarity to old-growth forest over time in shade tolerance but not species composition. J. Vegetation Sci. 24, 530–542. doi: 10.1111/j.1654-1103.2012.01482.x
Ding, X., Li, X., Qi, Y., Zhao, Z., Sun, D., and Wei, H. (2021). Depth-dependent C-N-P stocks and stoichiometry in ultisols resulting from conversion of secondary forests to plantations and driving forces. Forests 12:1300. doi: 10.3390/f12101300
Elisabeth, N. B., and Henderson, B. L. (2013). C:N:P stoichiometry in Australian soils with respect to vegetation and environmental factors. Plant Soil 373, 553–568. doi: 10.1007/s11104-013-1823-9
Fan, H., Wu, J., Liu, W., Yuan, Y., Hu, L., and Cai, Q. (2015). Linkages of plant and soil C:N:P stoichiometry and their relationships to forest growth in subtropical plantations. Plant Soil 392, 127–138. doi: 10.1007/s11104-015-2444-2
Fang, Z., Li, D.-D., Jiao, F., Yao, J., and Du, H.-T. (2019). The latitudinal patterns of leaf and soil C:N:P stoichiometry in the Loess Plateau of China. Front. Plant Sci. 10:85. doi: 10.3389/fpls.2019.00085
Guan, F., Tang, X., Fan, S., Zhao, J., and Peng, C. (2015). Changes in soil carbon and nitrogen stocks followed the conversion from secondary forest to Chinese fir and Moso bamboo plantations. Catena 133, 455–460. doi: 10.1016/j.catena.2015.03.002
Han, W., Fang, J., Guo, D., and Zhang, Y. (2005). Leaf nitrogen and phosphorus stoichiometry across 753 terrestrial plant species in China. New Phytol. 168, 377–385. doi: 10.1111/j.1469-8137.2005.01530.x
Hobbie, S. E. (2015). Plant species effects on nutrient cycling: revisiting litter feedbacks. Trends Ecol. Evol. 30, 357–363. doi: 10.1016/j.tree.2015.03.015
Hou, L., Zhang, Y., Li, Z., Shao, G., Song, L., and Sun, Q. (2021). Comparison of soil properties, understory vegetation species diversities and soil microbial diversities between Chinese Fir plantation and close-to-natural forest. Forests 12:632. doi: 10.3390/f12050632
Hu, C., Li, F., Xie, Y. H., Deng, Z. M., Hou, Z. Y., and Li, X. (2019). Spatial distribution and stoichiometry of soil carbon, nitrogen and phosphorus along an elevation gradient in a wetland in China. Eur. J. Soil Sci. 70:12821. doi: 10.1111/ejss.12821
Jiang, L., He, Z., Liu, J., Xing, C., Gu, X., Wei, C., et al. (2019). Elevation gradient altered soil C, N, and P stoichiometry of Pinus taiwanensis Forest on Daiyun Mountain. Forests 10:1089. doi: 10.3390/f10121089
Jin, X., Liu, Y., Hu, W., Wang, G., Kong, Z., Wu, L., et al. (2019). Soil bacterial and fungal communities and the associated nutrient cycling responses to forest conversion after selective logging in a subtropical forest of China. For. Ecol. Manag. 444, 308–317. doi: 10.1016/j.foreco.2019.04.032
Ladanai, S., Ågren, G. I., and Olsson, B. A. (2010). Relationships between tree and soil properties in Picea Abies and Pinus Sylvestris forests in Sweden. Ecosystems 13, 302–316. doi: 10.1007/s10021-010-9319-4
Lai, J., Zou, Y., Zhang, J., and Peres-Neto, P. R. (2022). Generalizing hierarchical and variation partitioning in multiple regression and canonical analyses using the rdacca.hp R package. Methods Ecol. Evol. 13, 782–788. doi: 10.1111/2041-210X.13800
Lambers, H., Mougel, C., Jaillard, B., and Hinsinger, P. (2009). Plant-microbe-soil interactions in the rhizosphere: an evolutionary perspective. Plant Soil 321, 83–115. doi: 10.1007/s11104-009-0042-x
Legendre, P., Mi, X., Ren, H., Ma, K., Yu, M., Sun, I.-F., et al. (2009). Partitioning beta diversity in a subtropical broad-leaved forest of China. Ecology 90, 663–674. doi: 10.1890/07-1880.1
Li, R., Zhu, S., Lian, J., Zhang, H., Liu, H., Ye, W., et al. (2021). Functional traits are good predictors of tree species abundance across 101 aubtropical forest species in China. Front. Plant Sci. 12:541577. doi: 10.3389/fpls.2021.541577
Li, W., Shen, F., Liu, Y., Li, Z., Jiang, J., Li, Q., et al. (2022). Soil depth determine the ecological stoichiometry of soil aggregates after returning ancient rice terraces to forest. Catena 219:106587. doi: 10.1016/j.catena.2022.106587
Li, Y., Zhao, X., Zhang, F., Awada, T., Wang, S., Zhao, H., et al. (2015). Accumulation of soil organic carbon during natural restoration of desertified grassland in China’s Horqin Sandy Land. J. Arid. Land 7, 328–340. doi: 10.1007/s40333-014-0045-1
Liu, F., Liu, Y., Wang, G., Song, Y., Liu, Q., Li, D., et al. (2015). Seasonal variations of C: N: P stoichiometry and their trade-offs in different organs of suaeda salsa in coastal wetland of Yellow River Delta, China. PLoS One 10, e0138169. doi: 10.1371/journal.pone.0138169
Liu, J., Coomes, D. A., Gibson, L., Hu, G., Liu, J., Luo, Y., et al. (2019). Forest fragmentation in China and its effect on biodiversity. Biol. Rev. 94, 1636–1657. doi: 10.1111/brv.12519
Luo, W., Elser, J. J., Lü, X., Wang, Z., Bai, E., Yan, C., et al. (2015). Plant nutrients do not covary with soil nutrients under changing climatic conditions. Glob. Biogeochem. Cycles 29, 1298–1308. doi: 10.1002/2015GB005089
Ma, R., Hu, F., Liu, J., Wang, C., Wang, Z., Liu, G., et al. (2020). Shifts in soil nutrient concentrations and C:N:P stoichiometry during long-term natural vegetation restoration. PeerJ 8, e8382. doi: 10.7717/peerj.8382
Mooshammer, M., Hofhansl, F., Frank, A. H., Wanek, W., Hämmerle, I., Leitner, S., et al. (2017). Decoupling of microbial carbon, nitrogen, and phosphorus cycling in response to extreme temperature events. Sci. Adv. 3, e1602781. doi: 10.1126/sciadv.1602781
Qin, Y., Feng, Q., Adamowski, J. F., Zhu, M., and Zhang, X. (2021). Community level response of leaf stoichiometry to slope aspect in a montane environment: A case study from the Central Qilian Mountains, China. Glob. Ecol. Conserv. 28, e01703. doi: 10.1016/j.gecco.2021.e01703
R Core Team (2022). R: A language and environment for statistical computing. Vienna: R Foundation for Statistical Computing.
Reich, P. B., and Oleksyn, J. (2004). Global patterns of plant leaf N and P in relation to temperature and latitude. Proc. Natl. Acad. Sci. U.S.A. 101, 11001–11006. doi: 10.1073/pnas.0403588101
Ren, S., Yu, G., Tao, B., and Wang, S. (2007). [Leaf nitrogen and phosphorus stoichiometry across 654 terrestrial plant species in NSTEC]. Huan Jing Ke Xue 28, 2665–2673.
Sardans, J., Rivas-Ubach, A., and Peñuelas, J. (2012). The C:N:P stoichiometry of organisms and ecosystems in a changing world: A review and perspectives. Perspect. Plant Ecol. Evol. Syst. 14, 33–47. doi: 10.1016/j.ppees.2011.08.002
Schillereff, D. N., Chiverrell, R. C., Sjöström, J. K., Kylander, M. E., Boyle, J. F., Davies, J. A. C., et al. (2021). Phosphorus supply affects long-term carbon accumulation in mid-latitude ombrotrophic peatlands. Commun. Earth Environ. 2:241. doi: 10.1038/s43247-021-00316-2
Tang, J., Sun, B., Cheng, R., Shi, Z., Da, L., Liu, S., et al. (2019). Effects of soil nitrogen (N) deficiency on photosynthetic N-use efficiency in N-fixing and non-N-fixing tree seedlings in subtropical China. Sci. Rep. 9:4604. doi: 10.1038/s41598-019-41035-1
Tang, L., Han, W., Chen, Y., and Fang, J. (2013). Resorption proficiency and efficiency of leaf nutrients in woody plants in eastern China. J. Plant Ecol. 6, 408–417. doi: 10.1093/jpe/rtt013
Tian, H., Chen, G., Zhang, C., Melillo, J. M., and Hall, C. A. S. (2010). Pattern and variation of C:N:P ratios in China’s soils: a synthesis of observational data. Biogeochemistry 98, 139–151. doi: 10.1007/s10533-009-9382-0
Tong, R., Zhou, B., Jiang, L., Ge, X., Cao, Y., and Shi, J. (2021). Leaf litter carbon, nitrogen and phosphorus stoichiometry of Chinese fir (Cunninghamia lanceolata) across China. Glob. Ecol. Conserv. 27, e01542. doi: 10.1016/j.gecco.2021.e01542
Villéger, S., Mason, N. W. H., and Mouillot, D. (2008). New multidimensional functional diversity indices for a multifaceted frameworh in functional ecology. Ecology 89, 2290–2301. doi: 10.1890/07-1206.1
Wang, F., Ding, Y., Sayer, E. J., Li, Q., Zou, B., Mo, Q., et al. (2017). Tropical forest restoration: Fast resilience of plant biomass contrasts with slow recovery of stable soil C stocks. Funct. Ecol. 31, 2344–2355. doi: 10.1111/1365-2435.12925
Wang, L., Li, Y., Wu, J., An, Z., Suo, L., Ding, J., et al. (2023). Effects of the rainfall intensity and slope gradient on soil erosion and nitrogen loss on the sloping fields of Miyun Reservoir. Plants 12:423. doi: 10.3390/plants12030423
Wang, L., Zhang, G., Zhu, P., Xing, S., and Wang, C. (2022). Soil C, N and P contents and their stoichiometry as affected by typical plant communities on steep gully slopes of the Loess Plateau, China. Catena 208:105740. doi: 10.1016/j.catena.2021.105740
Wang, N., Fu, F., Wang, B., and Wang, R. (2018). Carbon, nitrogen and phosphorus stoichiometry in Pinus tabulaeformis forest ecosystems in warm temperate Shanxi Province, north China. J. For. Res. 29, 1665–1673. doi: 10.1007/s11676-017-0571-8
Wang, X., Dong, S., Yang, B., Li, Y., and Su, X. (2014). The effects of grassland degradation on plant diversity, primary productivity, and soil fertility in the alpine region of Asia’s headwaters. Environ. Monit. Assess. 186, 6903–6917. doi: 10.1007/s10661-014-3898-z
Wang, Z., and Zheng, F. (2021). Impact of vegetation succession on leaf-litter-soil C:N:P stoichiometry and their intrinsic relationship in the Ziwuling Area of China’s Loess Plateau. J. For. Res. 32, 697–711. doi: 10.1007/s11676-020-01149-z
Wu, T., Chen, B., Xiao, Y., Pan, Y., Chen, Y., and Xiao, J. (2010). Leaf stoichiometry of trees in three forest types in Pearl River Delta, South China. Chinese J. Plant Ecol. 34:58. doi: 10.3773/j.issn.1005-264x.2010.01.009
Yang, J., El-Kassaby, Y. A., and Guan, W. (2020). The effect of slope aspect on vegetation attributes in a mountainous dry valley, Southwest China. Sci. Rep. 10:16465. doi: 10.1038/s41598-020-73496-0
Yang, L., Yang, Z., Zhong, X., Xu, C., Lin, Y., Fan, Y., et al. (2021). Decreases in soil P availability are associated with soil organic P declines following forest conversion in subtropical China. Catena 205:105459. doi: 10.1016/j.catena.2021.105459
Yang, Y., Liu, B.-R., and An, S.-S. (2018). Ecological stoichiometry in leaves, roots, litters and soil among different plant communities in a desertified region of Northern China. Catena 166, 328–338. doi: 10.1016/j.catena.2018.04.018
Zeng, Q., Li, X., Dong, Y., An, S., and Darboux, F. (2016). Soil and plant components ecological stoichiometry in four steppe communities in the Loess Plateau of China. Catena 147, 481–488. doi: 10.1016/j.catena.2016.07.047
Zhang, J., Zhao, N., Liu, C., Yang, H., Li, M., Yu, G., et al. (2018). C:N:P stoichiometry in China’s forests: From organs to ecosystems. Funct. Ecol. 32, 50–60. doi: 10.1111/1365-2435.12979
Zhang, W., Zhao, J., Pan, F., Li, D., Chen, H., and Wang, K. (2015). Changes in nitrogen and phosphorus limitation during secondary succession in a karst region in southwest China. Plant Soil 391, 77–91. doi: 10.1007/s11104-015-2406-8
Zhang, X., Cao, Q. V., Wang, H., Duan, A., and Zhang, J. (2020). Projecting stand survival and basal area based on a self-thinning model for Chinese Fir plantations. For. Sci. 66, 361–370. doi: 10.1093/forsci/fxz086
Zhang, Y., Li, C., and Wang, M. (2019a). Linkages of C: N: P stoichiometry between soil and leaf and their response to climatic factors along altitudinal gradients. J. Soils Sediments 19, 1820–1829. doi: 10.1007/s11368-018-2173-2
Zhang, Y., Li, P., Liu, X., Xiao, L., Shi, P., and Zhao, B. (2019b). Effects of farmland conversion on the stoichiometry of carbon, nitrogen, and phosphorus in soil aggregates on the Loess Plateau of China. Geoderma 351, 188–196. doi: 10.1016/j.geoderma.2019.05.037
Zhang, Z.-S., Song, X.-L., Lu, X.-G., and Xue, Z.-S. (2013). Ecological stoichiometry of carbon, nitrogen, and phosphorus in estuarine wetland soils: influences of vegetation coverage, plant communities, geomorphology, and seawalls. J. Soils Sediments 13, 1043–1051. doi: 10.1007/s11368-013-0693-3
Zhao, N., Yu, G., He, N., Wang, Q., Guo, D., Zhang, X., et al. (2016). Coordinated pattern of multi-element variability in leaves and roots across Chinese forest biomes: Multi-element variability in leaves and roots. Glob. Ecol. Biogeogr. 25, 359–367. doi: 10.1111/geb.12427
Zhou, W., Li, C., Zhao, W., Stringer, L. C., and Fu, B. (2023). Spatial distributions of soil nutrients affected by land use, topography and their interactions, in the Loess Plateau of China. Int. Soil Water Conserv. Res. doi: 10.1016/j.iswcr.2023.02.005 [Epub ahead of print].
Zhou, Y., Boutton, T. W., and Wu, X. B. (2018). Soil C:N:P stoichiometry responds to vegetation change from grassland to woodland. Biogeochemistry 140, 341–357. doi: 10.1007/s10533-018-0495-1
Keywords: forest conversion, subtropical forests, topography, soil stoichiometry, leaf stoichiometry
Citation: Zhang C, Li Y, Li X, Chen F, Jin Z, Li Y, Ma Y, Zeng S, Yang S and Bu W (2023) Tight relationships between leaf and topsoil stoichiometries after 42 years of forest conversion from old-growth forests to Chinese fir plantations. Front. For. Glob. Change 6:1268262. doi: 10.3389/ffgc.2023.1268262
Received: 27 July 2023; Accepted: 27 November 2023;
Published: 15 December 2023.
Edited by:
Romà Ogaya, Ecological and Forestry Applications Research Center (CREAF), SpainReviewed by:
Jianfeng Liu, Chinese Academy of Forestry, ChinaXuehua Ye, Chinese Academy of Sciences (CAS), China
Copyright © 2023 Zhang, Li, Li, Chen, Jin, Li, Ma, Zeng, Yang and Bu. This is an open-access article distributed under the terms of the Creative Commons Attribution License (CC BY). The use, distribution or reproduction in other forums is permitted, provided the original author(s) and the copyright owner(s) are credited and that the original publication in this journal is cited, in accordance with accepted academic practice. No use, distribution or reproduction is permitted which does not comply with these terms.
*Correspondence: Wensheng Bu, YndzMjAwN0AxNjMuY29t
†These authors have contributed equally to this work and share first authorship