- 1State Key Laboratory of Organic Geochemistry and Guangdong Key Laboratory of Environmental Protection and Resources Utilization, Guangzhou Institute of Geochemistry, Chinese Academy of Sciences, Guangzhou, China
- 2CAS Center for Excellence in Deep Earth Science, Guangzhou, China
- 3CSIC, Global Ecology Unit CREAF-CSIC-UAB, Barcelona, Spain
- 4CREAF, Barcelona, Spain
- 5College of Resources and Environment, University of Chinese Academy of Sciences, Beijing, China
- 6Fujian Provincial Key Laboratory of Soil Environmental Health and Regulation, College of Resources and Environment, Fujian Agriculture and Forestry University, Fuzhou, China
- 7Faculty of Science and Technology, Free University of Bozen-Bolzano, Bolzano, Italy
The exchange of isoprenoids, which includes isoprene, monoterpenes, and sesquiterpenes, between ecosystem soils and the atmosphere plays a significant role in soil ecology and atmospheric chemistry. However, research on flux exchange rates in subtropical ecosystems has been limited, as previous studies have mainly focused on temperate and boreal environments. In this study, we aimed to quantify the exchange of isoprenoids between the soil (with or without surface litter) and the atmosphere in a subtropical Eucalyptus urophylla plantation forest during the daytime in the wet season of subtropical China. Additionally, we investigated the influence of soil and litter variables on the fluxes of isoprenoids. Our results unveiled the exchange of isoprene and 17 terpenoid compounds, comprising 11 monoterpenes and 6 sesquiterpenes, between the studied soils and the atmosphere. Interestingly, regardless of the presence of surface litter, the studied soils acted as net sinks for isoprenoids, with isoprene being the most absorbed compound (−71.84 ± 8.26 μg m−2 h−1). The removal of surface litter had a significant impact on the exchange rates of two monoterpenes (α-pinene and β-pinene), resulting in decreased fluxes. Furthermore, the exchange rates of isoprene were positively correlated with litter dry weight and negatively correlated with soil temperature. The higher exchange rates of monoterpenes and sesquiterpenes were associated with increased levels of soil respiration and the abundance of leaf litter. These findings suggest that, in the context of projected global warming scenarios, the capacity of subtropical soils to act as sinks for isoprenoids is expected to increase in subtropical China. These changes in sink capacity may have implications for regional-scale atmospheric chemistry and ecosystem functioning.
Highlights
-First reported soil isoprene and sesquiterpenes in subtropics.
-Subtropical soils are a significant isoprene sink.
-Microclimate, microbes, and litter drive soil isoprenoid exchanges.
-Positive correlation of the sink with temperature: impacted by climate change.
1. Introduction
Forest soils can emit or absorb biogenic volatile organic compounds (BVOCs) due to a simultaneous occurrence of multiple biological and physico-chemical processes related to soil BVOC dynamics in complex soil environments (Asensio et al., 2007c; Leff and Fierer, 2008; Peñuelas et al., 2014). Isoprenoids and oxygenated BVOCs dominate the bidirectional fluxes between forest soils and the atmosphere (Hellén et al., 2006; Aaltonen et al., 2011, 2013; Mäki et al., 2017). Understanding the direction and magnitude of these fluxes, driven by factors like microclimate and litter, is critical for soil ecology (Wenke et al., 2010; Peñuelas et al., 2014; Svendsen et al., 2018) and atmospheric chemistry (Kramshøj et al., 2016; Nölscher et al., 2016; Tang et al., 2019). Soil BVOC emissions vary depending on ecosystem type, environmental conditions, and season (Tang et al., 2019; Mäki et al., 2019a; Mu et al., 2020). They can originate from litter, microorganisms, and roots, constituting a significant fraction of ecosystem emissions (Aaltonen et al., 2011, 2013; Kreuzwieser and Rennenberg, 2013). Soil can act as a sink or source of BVOCs, impacting their release from the ecosystem (Owen et al., 2007; Kramshøj et al., 2018). Biotic degradation and abiotic processes, such as physical diffusion or adsorption, and chemical reactions influence BVOC exchanges (Insam and Seewald, 2010; Ramirez et al., 2010; Albers et al., 2018; Mu et al., 2020).
Measuring soil BVOC fluxes across different climate zones is crucial. Previous studies focused on temperate and boreal regions (Asensio et al., 2007b,c; Mäki et al., 2019a,b), with limited research in tropical and subtropical forests. Soil BVOC fluxes in these regions remain inconsistent, requiring more data to improve modeling and ecosystem understanding (Bourtsoukidis et al., 2018; Huang et al., 2021; Llusià et al., 2022). Subtropical China, characterized by ideal tree growth conditions, has plantation forests lacking BVOC flux information. Eucalyptus urophylla, a significant emitter of isoprenoids, dominates these plantations (Zeng et al., 2022a). In this exploratory pilot study, we quantified isoprenoid exchange rates using a dynamic chamber and investigated their relationship with drivers like temperature, moisture, and respiration in an E. urophylla plantation. We aimed to address the knowledge gap regarding soil BVOC fluxes in subtropical forests. Forest soils in subtropical regions, known for their warm and humid climate, play a vital role in commercial forestry plantations (Ming et al., 2014; Wei and Blanco, 2014). These plantations cover a significant portion of the country’s forested landscape and are predominantly composed of monoculture coniferous and broadleaved species, including E. urophylla (He et al., 2013; Wei and Blanco, 2014; Bai et al., 2021).
Despite the vital role these ecosystems play, there is currently a dearth of information regarding soil BVOC fluxes, particularly from plantation forests. Remarkably, E. urophylla stands out as a significant emitter of isoprenoids in subtropical China, boasting the highest isoprene emission factor among dominant tree species (Zeng et al., 2022a). Consequently, our study aimed to address this knowledge gap by:
Quantifying the exchange rates of isoprenoids, encompassing individual and total monoterpenes (MTs) and sesquiterpenes (SQTs), in addition to isoprene, between the atmosphere and forest soils. We achieved this through the use of a dynamic chamber.
Exploring potential relationships between these exchange rates and various biotic and abiotic factors, such as soil temperature, moisture levels, and respiration, within the E. urophylla plantation.
2. Materials and methods
2.1. Study site and experimental design
The study was conducted in a E. urophylla plantation forest (22° 39′ N, 112° 54′ E) located in Heshan, Guangdong Province, southeastern China (see Supplementary Figure S1). The E. urophylla trees were artificially planted at the study site and had similar sizes (trunk circumference at breast height: 12–20 cm). The study site experiences a subtropical monsoon climate with an average annual temperature of approximately 21.7°C, and 80% of the mean annual precipitation (approximately 1700 mm) occurs during the wet season (April to September) (Wang et al., 2010). The soils in the study area are classified as Ultisols that developed from sand shales (Fang et al., 2023). The understory vegetation mainly comprised of low-statured species, such as Dicranopteris pedate, Pogonatherum crinitum, Mallotus apelta, Mikania micrantha, and Persicaria chinensis, which are commonly distributed throughout southeastern China.
A 50 × 50 m study plot was established in the central area of the plantation, and six sampling points were selected randomly within the central 40 × 40 m area, with a distance of more than 10 m between any two points. In each sampling point, two adjacent stainless steel soil collars were inserted into the soil (4 cm) for BVOCs and CO2 sampling, respectively, approximately 1.5 months before sampling began. The litter and understorey vegetation were not removed from the BVOC collars prior to sampling to ensure that the measurements reflected the real-world soil fluxes in field conditions, and to find out the role of litter in the soil exchanges of BVOCs by measuring the fluxes both with and without litter in the sampling campaign. The aboveground parts of the understorey vegetation were removed from the CO2 collars during installation, and litter was removed both during installation and prior to sampling to ensure that the measurements represented belowground soil respiration.
2.2. Isoprenoid sampling
Sampling was conducted in the middle of September 2022, during sunny or slightly cloudy days, between 10:00 and 15:00 h. One or two sampling points were sampled per day. Isoprenoids emitted from the soil were collected using a cylindrical semi-open dynamic chamber (internal diameter: 25 cm; height: 14 cm; volume: 6.8 L) placed over the BVOCs collar (see Supplementary Figure S2). The chamber and collar were fitted and water-sealed through the slot on the top of the collar. The chamber was constructed with polymethyl methacrylate and coated with fluorinated ethylene propylene (FEP) [Teflon film, (FEP 100, Type 200A; DuPont, United States)] on its inner surface. To promote uniform conditions and prevent condensation buildup inside the chamber, a Teflon fan (Shenzhen Shuangmu Plastic Material Co. Ltd., Shenzhen, China) was affixed to the top of the chamber, and small holes (5 mm I.D.) were drilled in the rim. The circulating air was generated by an air pump (MPU2134-N920-2.08, KNF, Freiburg, Germany) pumping the ambient air near the ground (approximately 18 cm above ground) and was controlled at a rate of 4.2 L min−1 by a mass flow controller (Alicat Scientific, Inc., Tucson, AZ, United States). The circulating air passed through two ozone scrubbers (Na2S2O3, Helmig et al., 2007; Zeng et al., 2022b) before being drawn into the lower position of the chamber through a random hole at the upper edge (see Supplementary Figure S3). A three-way valve was used to sample inlet air from circulating air just before entering the chamber, while the outlet air was sampled from the upper position of the chamber through another hole opposite to the previous one. Inlet and outlet air were sampled simultaneously using an automatic sampler (JEC921, Jectec Science and Technology, Co., Ltd., Beijing, China) equipped with two stainless style cartridges (see Supplementary Figure S4) filled with adsorbents (150 mg of Tenax TA and 150 mg of Carbograph 5TD, 9, Markes International Ltd., Bridgend, United Kingdom). The sampled air flow rate through the cartridges was 200 mL min−1 for 30 min. The flow rate of circulating air was 4 L min−1, and the residence time of circulating air was approximately 1.7 min during sampling. The sampled cartridges were detached from the automatic sampler, sealed with copper caps, and then stored in a portable refrigerator at 4°C in the field and at −20°C after being taken to the laboratory. Three samplings were conducted at each sampling point, with two of the three samples collected without removing the litter and the third sample collected after removing the litter with great care not to damage the superficial roots. The chamber was initially flushed with ambient air for 10–20 min before each sampling to avoid the influence of artificial disturbance on sampling. Air temperature was measured by a sensor (HC2A-S, Rotronic, Bathersdorf, Switzerland) placed over the chamber. The removed litter was collected and taken to the laboratory for litter composition and dry weight measurements.
2.3. Soil temperature, moisture, and respiration
The LI-6800 gas-exchange system (Li-COR Inc., Lincoln, NE, United States; see Supplementary Figure S3) was used to determine soil temperature (ST), moisture (v/v; SM), and respiration (SR), with 6–10 replicate measurements taken during each soil BVOC sampling. Soil respiration was measured using a soil CO2 collar in a closed system with a soil CO2 flux chamber (6800-09, Li-COR Inc., Lincoln, NE, United States) connected to the LI-6800. Soil temperature and moisture were measured around the soil BVOC chamber using a Stevens HydraProbe (900-17114, Li-COR Inc., Lincoln, NE, United States) equipped for the 6800-09.
2.4. Surface litter layer
The composition of the surface litter layer was assessed by sorting the collected litter into two fractions: leaf material and other material consisting of small branches, bark material, and understory vegetation. The dry-mass weight of the two fractions was measured after drying for 48 h in an oven at 70°C, resulting in the dry weight of total litter (TDW), leaf material (LDW), and other material (ODW).
2.5. Isoprenoid analysis
Isoprenoid analysis was performed using a gas chromatography-mass selective detector (GC-MSD) system (7890A GC interfaced with a 5975C VL MSD; Agilent Technologies, Inc., California, United States) coupled with an automatic thermal desorption system (TD-100, Markes International Ltd., United Kingdom). The sampled isoprenoids were thermally desorbed from cartridges by the TD-100 at 280°C for 10 min and then transferred by pure helium into a cryogenic trap (U-T11PGC-2S, Markes International Ltd., Bridgend, United Kingdom) at −10°C. The trap was rapidly heated to transfer the isoprenoids to the GC-MSD system with an HP-5MS capillary column (30 m × 0.25 mm × 0.25 μm, Agilent Technologies, CA, United Kingdom). Zeng et al. (2022a) provide a detailed description of the chromatographic method and MSD mode for isoprenoid analysis. The target compounds were identified by comparing their retention times with standards and quantified with standard calibration curves. Zeng et al. (2022b) provide a detailed description of the identification and quantification of isoprenoids.
The soil isoprenoids fluxes (E, μg m−2 h−1) were calculated as μg of isoprenoids per square meter and hour using the following formula:
Here, F (L min−1) represents the flow rate of circulating air, Cin (ng L−1) and Cout (ng L−1) represent the isoprenoid concentrations of inlet and outlet air, respectively, and A (m2) represents the area of soil surface enclosed by the sampling chamber.
The litter isoprenoids fluxes (Elitter, ng g−1 h−1) were calculated as ng of isoprenoids per gram and hour using the following formula:
Here, Cwith litter (ng L−1) is the difference between the concentrations of inlet and outlet air in samplings with litter, Cwithout litter (ng L−1) is the difference between the concentrations of inlet and outlet air in samplings without litter, and W (g) is the dry mass of the total litter collected in the sampling chamber.
2.6. Statistical analyses
To test for statistical differences between samplings with and without litter, a student’s t-test was conducted and significance was set at p < 0.05. The associations between soil or litter variables and isoprenoid exchanges, ambient isoprenoid concentrations and isoprenoid exchanges were tested using Pearson correlation analysis. Pearson correlation coefficient (r) between soil or litter variables and isoprenoid exchanges were represented in a heatmap using the reshape2 package in R (v. 4.1.1). A principal component analysis (PCA) was performed with the FactoMineR and factoextra packages in R to visualize the multivariate correlations between the previously standardized variables. Vector variables were marked as isoprenoid exchanges and soil or litter variables. The ggplot2 package was used for visualization in R.
3. Results
3.1. Soil and litter variables
The mean soil temperatures at the sampling points ranged between 30.34 ± 0.25°C and 33.38 ± 0.53°C, and the mean soil moisture (v/v) ranged between 6.88 ± 1.41% and 19.20 ± 1.05% (Supplementary Figures S5A,B). The mean soil respiration ranged between 2.07 ± 0.10 μmol m−2 s−1 and 3.05 ± 0.17 μmol m−2 s−1 (Supplementary Figure S5C). The mean values for soil temperature, moisture, and respiration across all sampling points were 32.02 ± 0.49°C, 11.89 ± 1.78%, and 2.53 ± 0.14% μmol m−2 s−1, respectively. The litter collected from the surface layer of the sampling points was measured for dry weight, which ranged between 9.39 g and 32.09 g with an average of 18.61 ± 3.33 g. The dry weight of leaf material ranged between 4.38 g and 16.73 g with an average of 9.35 ± 2.21 g (Supplementary Figure S6).
3.2. Soil isoprenoid fluxes
We observed net uptakes of isoprene (−71.84 ± 8.26 μg m−2 h−1), monoterpenes (−6.20 ± 13.98 μg m−2 h−1), and sesquiterpenes (−6.19 ± 3.23 μg m−2 h−1), with isoprene being the most absorbed compound (Figure 1A). The removal of the surface layer litter marginally increased total monoterpene uptakes (p = 0.09; Supplementary Table S1), coinciding with higher uptakes of α-pinene (p < 0.05; Figure 1B), β-pinene (p < 0.05; Figure 1B), and limonene (p = 0.06; Supplementary Table S1).
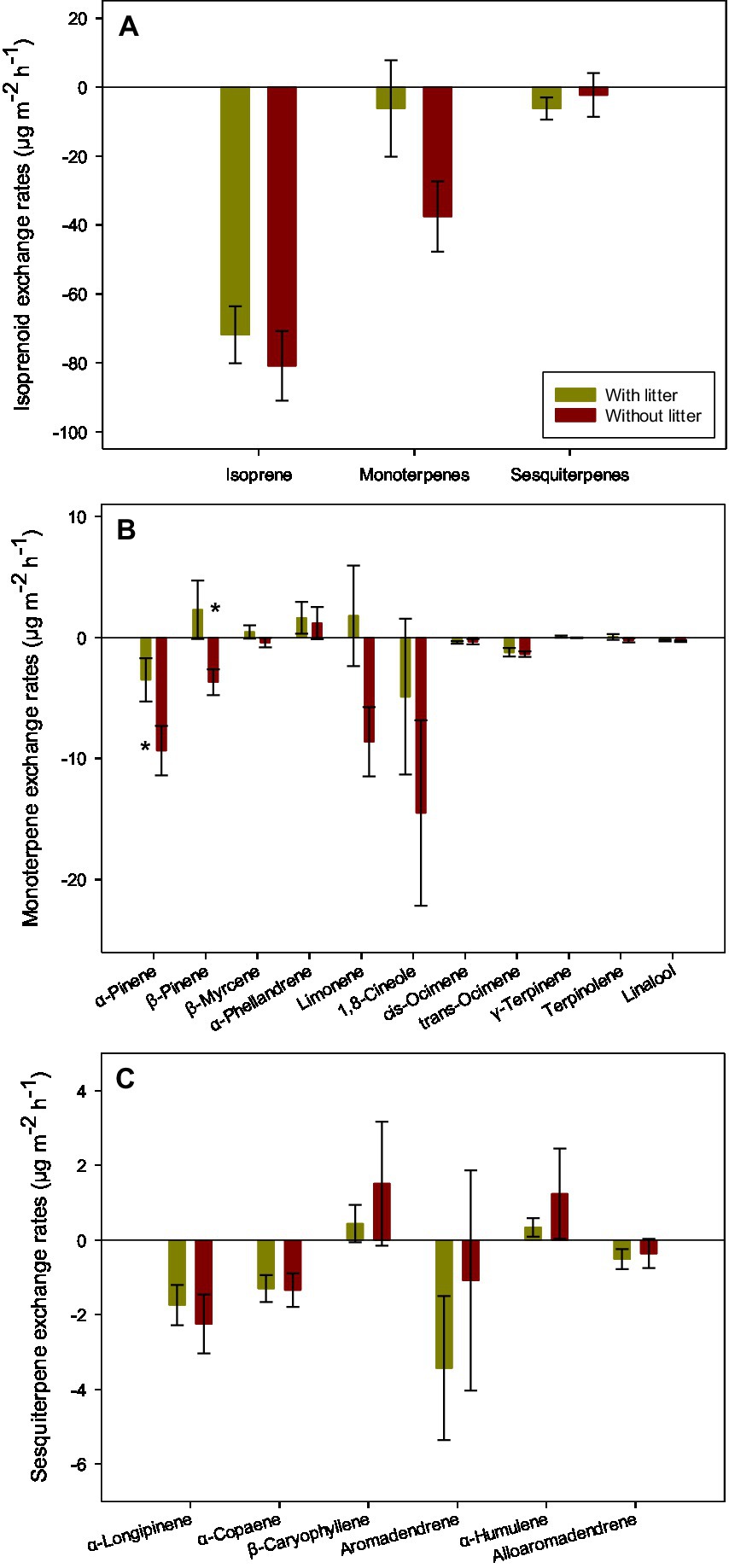
Figure 1. The soil exchange rates of isoprenoids (A), individual monoterpenes (B), and individual sesquiterpenes (C) in samplings with and without litter in the subtropical E. urophylla plantation forest. Vertical bars represent the standard errors of the means. Asterisks indicate a significant difference (*p < 0.05) identified by student’s t-tests between samplings with and without litter (n = 6).
We detected 17 terpenoid compounds, including 11 monoterpenes and 6 sesquiterpenes. The fluxes of monoterpenes were dominated by α-pinene, β-pinene, α-phellandrene, limonene, 1,8-cineole, and trans-ocimene, with mean values above 1 μg m−2 h−1 (Figures 1B, 2). Regardless of the presence of litter, α-pinene, 1,8-cineole, and trans-ocimene were net absorbed, and α-phellandrene was net emitted. β-pinene and limonene were only net absorbed after removing the surface layer litter. The fluxes of individual sesquiterpenes showed similar magnitudes, with α-longipinene, α-copaene, aromadendrene, and alloaromadendrene being absorbed and β-caryophyllene and α-humulene being emitted (Figures 1C, 2).
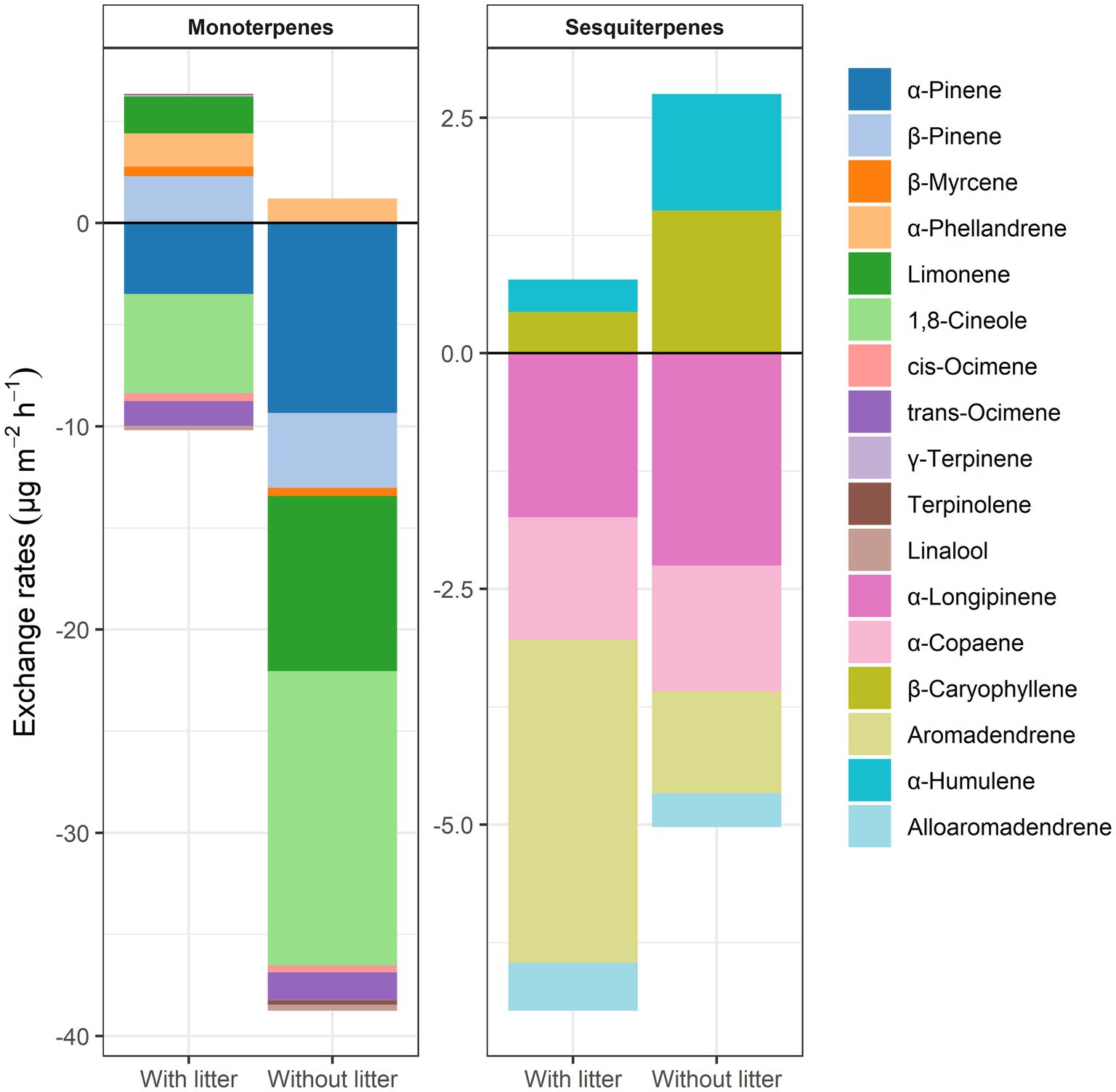
Figure 2. Distribution of soil monoterpene and sesquiterpene exchanges in samplings with and without litter in the subtropical E. urophylla plantation forest.
3.3. Litter isoprenoid fluxes
The litter in the surface layer emitted a net amount of isoprene (67.80 ± 16.36 ng g−1 h−1) and monoterpenes (109.09 ± 41.75 ng g−1 h−1) while also taking in sesquiterpenes (−12.64 ± 14.98 ng g−1 h−1; Figure 3A). The dominant contributors to the fluxes of monoterpenes were α-pinene, β-pinene, limonene, and 1,8-cineole, with mean values exceeding 10 ng g−1 h−1 (Figure 3B). The fluxes of sesquiterpenes were dominated by aromadendrene (8.27 ± 7.12 ng g−1 h−1; Figure 3B).
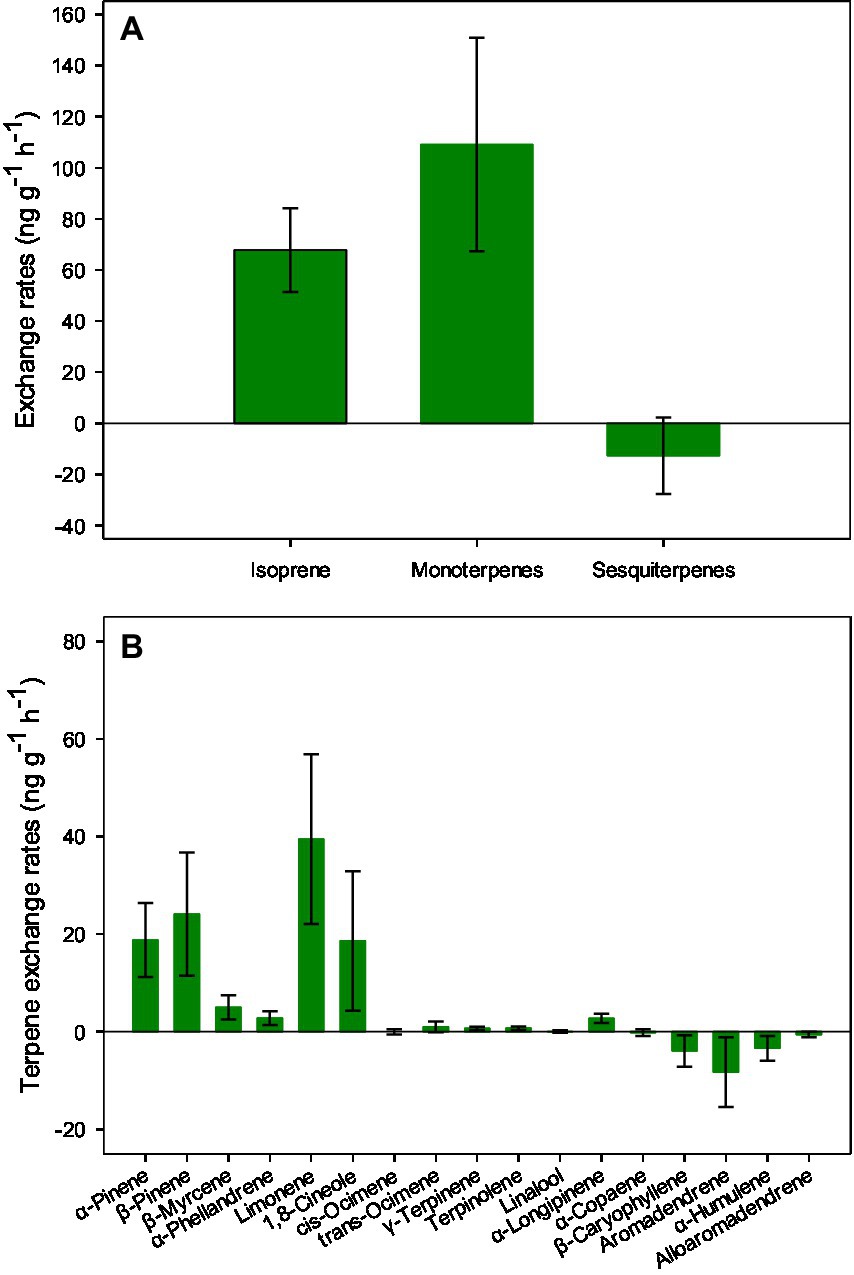
Figure 3. Litter exchange rates of isoprenoids (A) and individual terpenes (B) in the subtropical E. urophylla plantation forest. Vertical bars represent the standard errors of the means (n = 12).
3.4. Associations of soil isoprenoid fluxes with soil and litter variables
The Pearson correlation analysis identified strong correlations (r > 0.60 and p < 0.05) between isoprenoid fluxes and soil or litter variables (Figure 4 and Supplementary Table S2). The fluxes of isoprene, 1,8-cineole, cis-ocimene, and α-longipinene were negatively correlated with soil temperature, whereas the fluxes of α-pinene, α-phellandrene, 1,8-cineole, cis-ocimene, terpinolene, total monoterpenes, α-longipinene, α-copaene, alloaromadendrene, and total sesquiterpenes were positively correlated with soil respiration.
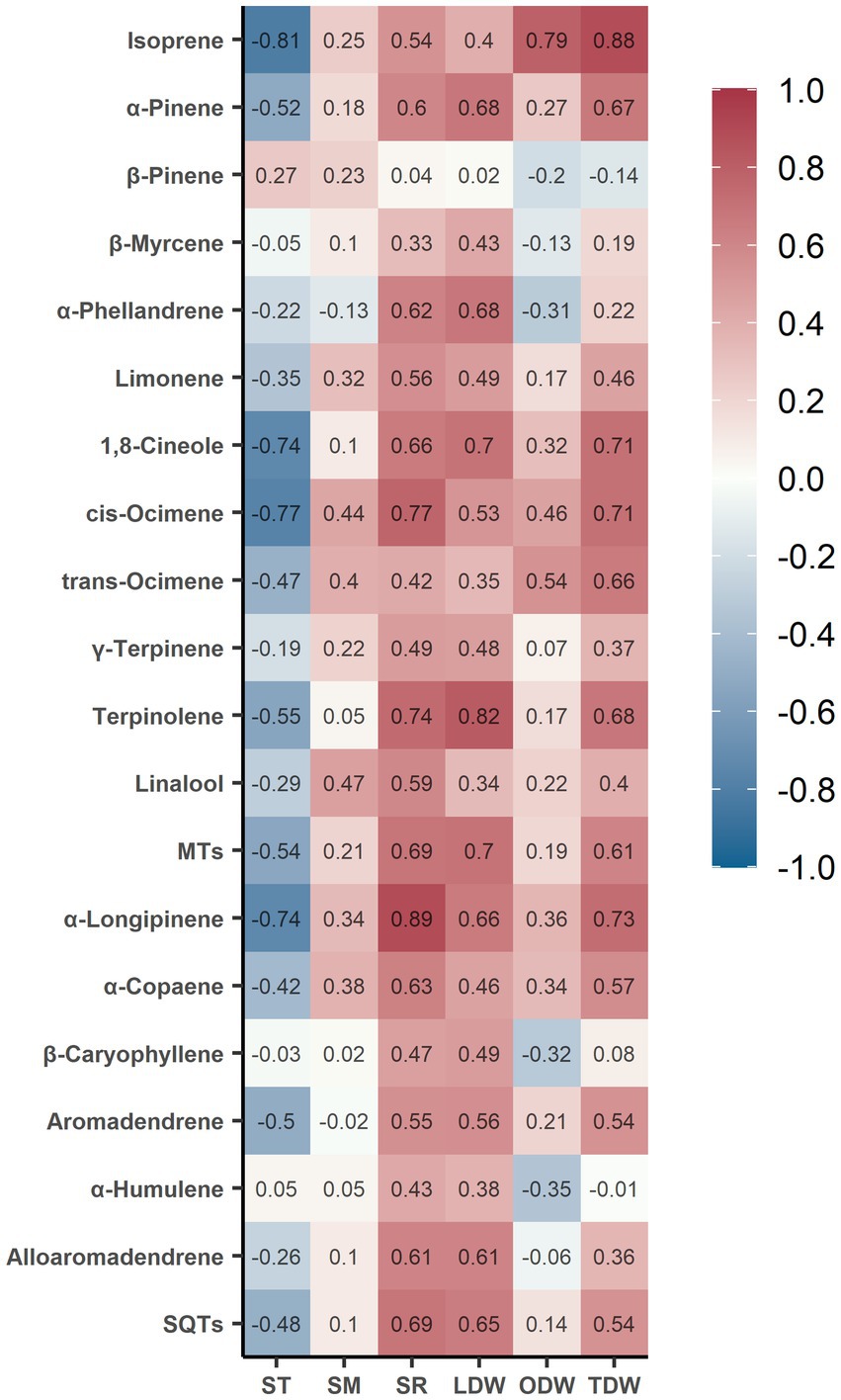
Figure 4. Heat map of Pearson correlation coefficient (r) between soil or litter variables and isoprenoid exchanges in the subtropical E. urophylla plantation forest. The colors represent the coefficient of correlation between each pair of variables, where red represents a positive correlation and blue represents a negative correlation. The r and p-values are shown in Supplementary Table S2. MTs, monoterpenes; SQTs, sesquiterpenes; ST, soil temperature; SM, soil moisture; SR, soil respiration; LDW, leaf material dry weight; ODW, other material dry weight; TDW, total litter dry weight.
Moreover, the fluxes of isoprene, α-pinene, 1,8-cineole, cis-ocimene, trans-ocimene, terpinolene, total monoterpenes, and α-longipinene were positively correlated with the dry weight of litter. Additionally, the fluxes of α-pinene, α-phellandrene, 1,8-cineole, terpinolene, total monoterpenes, α-longipinene, alloaromadendrene, and total sesquiterpenes increased with higher levels of leaf material mass, whereas the increases in fluxes of isoprene were correlated with higher levels of non-leaf materials.
The first (PC1) and second (PC2) principal components of the PCA explained 56% and 23% of the total variance, respectively, (Figure 5). The fluxes of isoprene and two terpene groups, along with soil respiration and temperature, total dry weight of litter mainly contributed to PC1, while the two dry weights of litter fraction and soil moisture mainly contributed to PC2. The fluxes of isoprene were positively correlated with the total dry weight of litter and soil respiration and negatively with soil temperature. The fluxes of the two terpene groups were positively correlated with soil respiration, the total dry weight of litter and the dry weight of leaf materials.
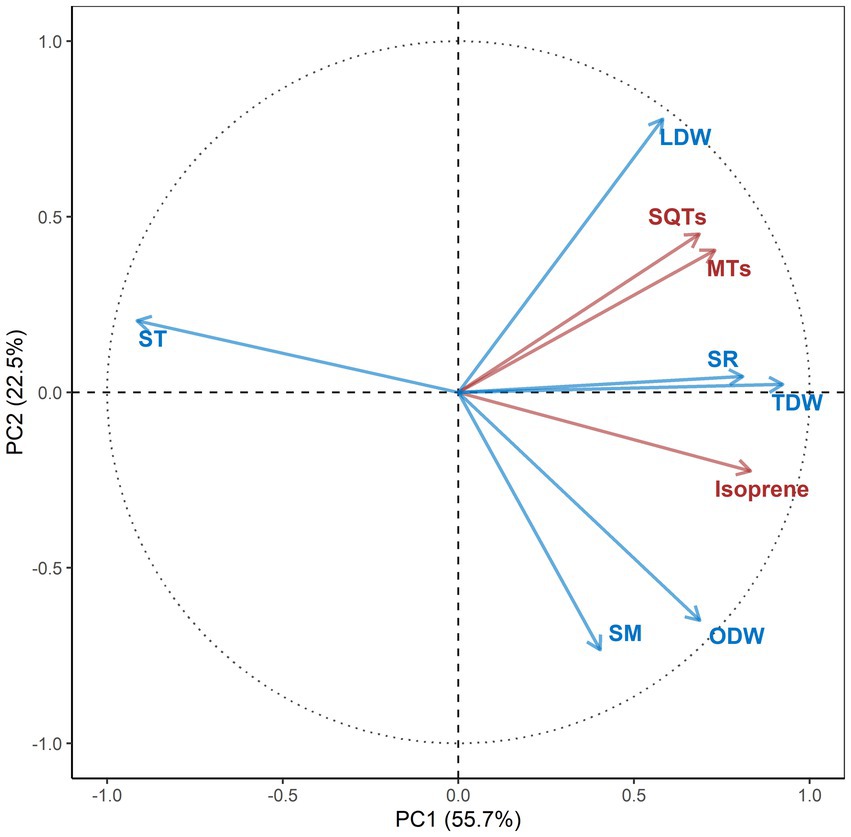
Figure 5. Two-dimensional representation defined by the first two principal components (PC1 and PC2) of the principal component analysis of soil or litter variables (blue) and isoprenoid exchanges (red) in the subtropical E. urophylla plantation forest. The percentages contributions to the total variability are in parentheses. For the definition of the acronyms, see Figure 4.
3.5. Ambient isoprenoid concentrations
Mean ground-level concentrations (Cin) of isoprene, monoterpenes, and sesquiterpenes were 17.47 ± 2.38, 14.07 ± 2.27, and 2.89 ± 0.55 ng L−1, respectively (Supplementary Figure S7A). The ambient concentrations of terpene compounds were dominated by α-pinene, β-pinene, limonene, 1,8-cineole, and aromadendrene, with mean values above 1 ng L−1 (Supplementary Figure S7B). The fluxes of isoprene, total monoterpenes, total sesquiterpenes, α-pinene, 1,8-cineole, cis-ocimene, trans-ocimene, γ-terpinene, terpinolene, α-longipinene, α-copaene, β-caryophyllene, aromadendrene, and alloaromadendrene were negatively correlated with their ambient concentrations, respectively (r > 0.60 and p < 0.05; Supplementary Table S3).
4. Discussion
4.1. Soil isoprenoid fluxes in a subtropical Eucalyptus urophylla plantation forest
The isoprenoids fluxes recorded in this study showed that forest soils acted as sinks for isoprenoids, with net adsorption of isoprene occurring at high rates. This net isoprene adsorption was observed regardless of the presence of surface litter, consistent with previous studies reporting that soils act as a sink for isoprene (Cleveland and Yavitt, 1997, 1998; Pegoraro et al., 2005; Gray et al., 2015; Trowbridge et al., 2020). The surface litter layer was found to play a minor role in soil isoprene fluxes (Figure 1A). While low net isoprene emissions have been reported in some forest soils (Purser et al., 2021), significant emissions are rare (Rinnan and Albers, 2020). Isoprene is thought to be produced in soils as a microbial metabolite (Veres et al., 2014), and understorey vegetation such as grasses and mosses can contribute to soil emissions, leading to a small net isoprene emission in some ecosystems (Purser et al., 2021). Isoprene can also be consumed as a carbon and energy source for isoprene-degrading microbes (Cleveland and Yavitt, 1997; Pegoraro et al., 2005; Lin et al., 2007). The large microbial consumption of isoprene that exceeds its production could cause net uptake of isoprene in soils. However, the high uptake of isoprene in this study could be partly due to a large isoprene concentration gradient between the atmosphere and the soil, as the high ambient isoprene concentrations (17.47 ± 2.38 ng L−1; Supplementary Figure S7A) observed in the study site and E. urophylla has a large emission factor of isoprene as reported in other studies in subtropical China (Zeng et al., 2022a).
In this study, forest soils acted as sinks for monoterpenes with large variations in fluxes that indicate the possibility of low emissions (Figure 1A). The surface litter layer served as a source of monoterpenes, with emissions (109.09 ± 41.75 ng g−1 h−1; Figure 3A) around one-tenth of the emission factor (1.02 ± 0.65 μg g−1 h−1; Zeng et al., 2022a) of E. urophylla reported in subtropical China. This contrasts with previous results from tropical and subtropical soils, where the presence of surface litter did not affect soil monoterpene exchanges (Huang et al., 2021; Llusià et al., 2022), although the emitted monoterpenes may hardly escape from the litter-soil layer due to higher soil uptakes of monoterpenes in this study. However, upward fluxes may occur when a large amount of litter (especially leaf litter, see below) covers the soil surface, leading to monoterpene production in the surface litter layer that exceeds soil uptakes of monoterpenes. The net monoterpene adsorption observed here contrasts with net emissions observed in other subtropical or tropical ecosystems, such as 10.4 ± 2.93 μg m−2 h−1 in two tropical forests of French Guiana (Llusià et al., 2022), and 15.0 ± 15.3 μg m−2 h−1in a Eucalyptus gunnii plantation in the United Kingdom (Purser et al., 2021). The emitted monoterpenes could originate from litter, whose emissions are associated with storage pools (Greenberg et al., 2012; Staudt et al., 2019) and microbial decomposition (Gray et al., 2010; Viros et al., 2021), and from soils where plant root systems (Nishida et al., 2005; Purser et al., 2021) and soil microbial communities (Asensio et al., 2008a; Ramirez et al., 2010) are major sources. Like isoprene, microbial uptake of monoterpenes was also widely reported and well understood (Insam and Seewald, 2010; Ramirez et al., 2010; Peñuelas et al., 2014; Tang et al., 2019). This consumption process may dominate monoterpene fluxes, leading to the small net adsorption observed here.
In this study, sesquiterpenes were found to be net adsorbed at rates similar to those of monoterpenes, with the surface litter layer and soil both acting as sinks for sesquiterpenes (Figures 1A, 3A). However, the adsorption rates (6.19 ± 3.23 μg m−2 h−1; Figure 1A) were much lower compared to those observed in tropical forests of French Guiana (65.97 ± 21.15 μg m−2 h−1; Llusià et al., 2022), and this contrasts with the strong sesquiterpene emissions that can be of comparable magnitude to canopy emissions recorded from Amazonian soils (Bourtsoukidis et al., 2018). Like monoterpenes, sesquiterpenes have been shown to be released from litter (Isidorov et al., 2003; Svendsen et al., 2018), microorganisms (Horváth et al., 2012; Mäki et al., 2017; Bourtsoukidis et al., 2018), and roots (Lin et al., 2007; Asensio et al., 2007a, 2008b). However, studies identifying soils as sinks for sesquiterpenes are scarce (Tang et al., 2019; Llusià et al., 2022). Llusià et al. (2022) attributed the net sinks of sesquiterpenes to the effects of biological factors, such as microbial consumption of soil-litter sesquiterpenes, and physical factors, such as high sesquiterpene concentration gradients between the atmosphere and soil air. These factors favor downward fluxes of sesquiterpenes and may also contribute to the small net adsorption observed in this study.
4.2. Abiotic and biotic controls of soil isoprenoid fluxes in the plantation forest
The fluxes of isoprene, total monoterpenes (i.e., 1,8-cineole and cis-ocimene), and total sesquiterpenes (i.e., α-longipinene and aromadendrene) were negatively correlated with soil temperature in our study (Figures 4, 5). Our results suggest that a linear relationship, as found in some temperate forest soils (Trowbridge et al., 2020), is sufficient to explain isoprene fluxes (r = −0.81 and p < 0.001; Figure 4 and Supplementary Table S2). This contrasts with the decrease in the observed rate of isoprene uptake from temperate forest soils at high temperature (>30°C, Cleveland and Yavitt, 1998). The significant negative linear correlation between monoterpene fluxes and soil temperature was consistent with results found in subtropical pine and broad-leaved forest soils (Huang et al., 2021) and temperate forest soils (Trowbridge et al., 2020). However, significant correlations of soil temperature with monoterpenes and sesquiterpenes contrast with the finding that neither terpene group correlated with soil temperature in tropical forest soils (Llusià et al., 2022). These results suggest that isoprenoid emission rates decreased and/or uptake rates increased with increasing temperature, indicating that the soil capacity of isoprenoid sink is expected to increase due to global warming in subtropical China (IPCC, 2014; Zhang et al., 2019).
The isoprenoid fluxes were generally not correlated with soil moisture in this study (Figures 4, 5), and the lack of association for soil monoterpene fluxes was also found in previous studies conducted in subtropical forests (Huang et al., 2021). Similarly, Llusià et al. (2022) reported weak associations between the exchanges of monoterpenes (r = 0.45, p < 0.01) and sesquiterpenes (r = −0.38, p < 0.01) and soil moisture in tropical forest soils. An increase in soil moisture can increase microbial activity at low soil moisture content, but also decrease the amount of gas accessible to soil microbes (Cleveland and Yavitt, 1997; Asensio et al., 2007a; Tang et al., 2019), leading to increased (Asensio et al., 2007a) or decreased (Trowbridge et al., 2020) uptake of isoprenoids, as observed in temperate and Mediterranean forest soils. However, changes in soil moisture may have less effect on warmer and wetter subtropical and tropical ecosystems than in temperate ecosystems. Nevertheless, isoprenoids are hydrophobic VOCs, and their soil fluxes may be less dependent on soil moisture than those of hydrophilic VOCs (e.g., acetic acid, acetaldehyde, acetone; Seco et al., 2007; Baggesen et al., 2022), which could also contribute to the low correlation with soil moisture.
The fluxes of isoprene, total monoterpenes (i.e., cis-ocimene and terpinolene), and total sesquiterpenes (i.e., α-longipinene and α-copaene) were all positively correlated with soil respiration (Figures 4, 5). The positive correlation between soil isoprenoid fluxes and respiration may indicate that increases in microbial activity could promote isoprenoid production to a greater degree than consumption and/or mineralization in ecosystem soils. This result contrasts with the negative or insignificant relationship between some monoterpenes of forest soils and soil respiration reported in previous studies (Leff and Fierer, 2008; Huang et al., 2021). It has been shown that soil isoprenoid flux depends on the metabolic activities of microbes (Cleveland and Yavitt, 1998; Albers et al., 2018), while both isoprenoid production and consumption associated with microbes usually occur simultaneously (Insam and Seewald, 2010; Peñuelas et al., 2014). Nevertheless, the CO2 and isoprenoid fluxes were also a result of root activity (Gray et al., 2014; Tang et al., 2019; Mu et al., 2022). Thus, the highly variable correlation between soil isoprenoid flux and respiration among ecosystem soils may be explained by differences in their microbial community composition.
The fluxes of isoprene, total monoterpenes (i.e., 1,8-cineole and cis-ocimene), and total sesquiterpenes (i.e., α-longipinene and α-copaene) were all positively correlated with total litter dry weight (Figures 4, 5), which points to an effect of the litter layer on soil isoprenoid fluxes. The fluxes of the two terpene groups showed a strong correlation with leaf litter, indicating that their emissions mainly originated from the evaporation of stored terpenes in leaf litter, as E. urophylla is a terpene-storing species (Külheim et al., 2015). Meanwhile, isoprene fluxes were more correlated with non-leaf litter (branches, barks, and understory vegetation), indicating that their fluxes may be more related to microbe-mediated decomposition of plant residues (Sivy et al., 2002; Mancuso et al., 2015) and/or adsorption–desorption processes in the litter layer. Thus, these results highlight the relevance of studying the effects of litter composition on soil isoprenoid fluxes (Purser et al., 2021).
4.3. Methodological considerations
The soil BVOCs were sampled using a soil BVOC dynamic chamber that evolved from a recent dynamic chamber (Zeng et al., 2022b) designed and well-characterized for measuring branch-scale BVOC emissions. The short residence time (approximately 1.7 min) and ozone-scrubbed circulating air could reduce the adsorptive and reactive loss in samplings. The inlet and outlet air were sampled simultaneously at a constant flow rate (200 mL min−1) to avoid environmental and instrumental disturbance. The isoprenoids were identified with authentic standards and quantified with compound-specific standard calibration curves to ensure high accuracy of results.
The field sampling was conducted exclusively during the hottest and wettest period of the year, spanning from June to September. This limited sampling period may not accurately represent the field conditions throughout the entire year, consequently constraining the scope of our study. For instance, our findings enable us to conclude that subtropical soils possess the capacity to absorb isoprenoids, with this absorption capacity being particularly robust during wet seasons. However, our study falls short of providing a comprehensive assessment of net soil isoprenoid fluxes across all seasons of the year. The soil variables (temperature, moisture, and respiration) were measured only during the BVOC sampling rather than throughout the month or season, which leads to uncertainties in the correlation analysis, as the effects of soil variables on BVOC fluxes may not be instantaneous. Moreover, it is important to consider that our results may have been affected by the ambient concentrations of isoprenoids. Elevated ambient concentrations tend to promote downward fluxes for most compounds examined in this study (Supplementary Table S3). This influence of ambient concentrations is particularly noteworthy in the case of isoprene, owing to its notably high ambient concentrations (17.47 ± 2.38 ng L−1; Supplementary Figure S7A). Additionally, there is a strong correlation between ambient concentrations and exchange rates (r = −0.96 and p < 0.001; Supplementary Table S3), which likely contributes to the substantial net absorption of isoprene observed in our study. Furthermore, we hypothesize that the soil’s ability to serve as a sink for isoprenoids may be regulated by soil microbes. To gain a more comprehensive understanding of soil BVOCs in subtropical ecosystems and to accurately characterize and project this sink capacity, it is advisable to incorporate additional microbial indicators, especially microbial biomass and community data. A deeper comprehension of the roles played by ambient concentrations and soil microbes in soil-atmosphere isoprenoid exchanges within subtropical ecosystems is essential. This understanding will enhance our capacity to unlock the full potential of soil BVOC carbon sinks, potentially contributing to climate change mitigation efforts. The method does have other limitations; however, through this exploratory pilot study, we have gained primary insight into the complex mechanisms controlling the variability of soil BVOC fluxes in subtropical ecosystems.
5. Conclusion
Our results demonstrate the capacity of subtropical soils to absorb isoprenoids, emphasizing the importance of incorporating this data into models for soil biogenic volatile organic compounds (BVOCs). Our study reiterates the crucial roles played by soil microclimate, microbes, and litter as drivers of isoprenoid exchanges between soils and the atmosphere. The direction and magnitude of these fluxes are often determined by the combined effects of these factors. However, to gain a deeper understanding of the complex mechanisms governing the variability of soil BVOC fluxes, further research involving long-term field measurements and laboratory incubation experiments is essential. Our investigation reveals that under current conditions, the subtropical soils in our study function as significant net sinks for isoprene. This phenomenon can be attributed to various biological and physical factors, including the microbial consumption of soil-litter volatiles and the presence of high atmospheric isoprene concentrations, which promote isoprene uptake by the soil. However, as temperatures increase in the future, along with adequate moisture levels, the consumption of soil-litter isoprenoids by soil microbial communities may intensify, leading to a decrease in soil isoprenoid exchange rates. Consequently, we anticipate that the soil’s capacity to act as a sink for isoprenoids will increase due to climate change in subtropical regions of China.
Data availability statement
The original contributions presented in the study are included in the article/Supplementary material, further inquiries can be directed to the corresponding author.
Author contributions
ZM: Conceptualization, Data curation, Formal analysis, Investigation, Methodology, Software, Visualization, Writing – original draft, Writing – review & editing. JZ: Data curation, Formal analysis, Investigation, Methodology, Writing – review & editing. YZ: Funding acquisition, Methodology, Validation, Writing – review & editing, Resources. WS: Data curation, Formal analysis, Investigation, Writing – review & editing. WP: Data curation, Formal analysis, Investigation, Writing – review & editing. ZY: Validation, Writing – review & editing. DA: Validation, Writing – review & editing. JL: Validation, Writing – review & editing. JP: Funding acquisition, Validation, Writing – review & editing. XW: Conceptualization, Funding acquisition, Methodology, Project administration, Resources, Supervision, Validation, Writing – review & editing.
Funding
The author(s) declare financial support was received for the research, authorship, and/or publication of this article. This work was supported by the National Natural Science Foundation of China (Nos. 42022023 and 42321003), the Hong Kong Research Grant Council (No. T24-504/17-N), the Department of Science and Technology of Guangdong (No. 2023B1212060049), the Guangzhou Municipal Science and Technology Bureau (No. 202206010057), the TED2021-132627B-I00 grant funded by the Spanish MCIN, AEI/10.13039/501100011033 and the European Union NextGenerationEU/PRTR, the Fundación Ramón Areces grant CIVP20A6621, and the Catalan Government grant SGR 2021-1333. ZM also acknowledges the financial support from the Guangdong Provincial Postdoctoral Talent-Introduction Program.
Conflict of interest
The authors declare that the research was conducted in the absence of any commercial or financial relationships that could be construed as a potential conflict of interest.
The author(s) declared that they were an editorial board member of Frontiers, at the time of submission. This had no impact on the peer review process and the final decision.
Publisher’s note
All claims expressed in this article are solely those of the authors and do not necessarily represent those of their affiliated organizations, or those of the publisher, the editors and the reviewers. Any product that may be evaluated in this article, or claim that may be made by its manufacturer, is not guaranteed or endorsed by the publisher.
Supplementary material
The Supplementary material for this article can be found online at: https://www.frontiersin.org/articles/10.3389/ffgc.2023.1260327/full#supplementary-material
References
Aaltonen, H., Aalto, J., Kolari, P., Pihlatie, M., Pumpanen, J., Kulmala, M., et al. (2013). Continuous VOC flux measurements on boreal forest floor. Plant Soil 369, 241–256. doi: 10.1007/s11104-012-1553-4
Aaltonen, H., Pumpanen, J., Pihlatie, M., Hakola, H., Hellén, H., Kulmala, L., et al. (2011). Boreal pine forest floor biogenic volatile organic compound emissions peak in early summer and autumn. Agric. For. Meteorol. 151, 682–691. doi: 10.1016/j.agrformet.2010.12.010
Albers, C. N., Kramshøj, M., and Rinnan, R. (2018). Rapid mineralization of biogenic volatile organic compounds in temperate and Arctic soils. Biogeosciences 15, 3591–3601. doi: 10.5194/bg-15-3591-2018
Asensio, D., Owen, S. M., Llusià, J., and Peñuelas, J. (2008a). The distribution of volatile isoprenoids in the soil horizons around Pinus halepensis trees. Soil Biol. Biochem. 40, 2937–2947. doi: 10.1016/j.soilbio.2008.08.008
Asensio, D., Peñuelas, J., Filella, I., and Llusià, J. (2007a). On-line screening of soil VOCs exchange responses to moisture, temperature and root presence. Plant Soil 291, 249–261. doi: 10.1007/s11104-006-9190-4
Asensio, D., Peñuelas, J., Llusià, J., Ogaya, R., and Filella, I. (2007b). Interannual and interseasonal soil CO2 efflux and VOC exchange rates in a Mediterranean holm oak forest in response to experimental drought. Soil Biol. Biochem. 39, 2471–2484. doi: 10.1016/j.soilbio.2007.04.019
Asensio, D., Peñuelas, J., Ogaya, R., and Llusià, J. (2007c). Seasonal soil VOC exchange rates in a Mediterranean holm oak forest and their responses to drought conditions. Atmos. Environ. 41, 2456–2466. doi: 10.1016/j.atmosenv.2006.05.007
Asensio, D., Peñuelas, J., Prieto, P., Estiarte, M., Filella, I., and Llusià, J. (2008b). Interannual and seasonal changes in the soil exchange rates of monoterpenes and other VOCs in a Mediterranean shrubland. Eur. J. Soil Sci. 59, 878–891. doi: 10.1111/j.1365-2389.2008.01057.x
Baggesen, N. S., Davie-Martin, C. L., Seco, R., Holst, T., and Rinnan, R. (2022). Bidirectional exchange of biogenic volatile organic compounds in subarctic heath mesocosms during autumn climate scenarios. J. Geophys. Res. Biogeosci. 127:e2021JG006688. doi: 10.1029/2021JG006688
Bai, Y., Zhou, Y., Du, J., Zhang, X., and Di, N. (2021). Effects of a broadleaf-oriented transformation of coniferous plantations on the hydrological characteristics of litter layers in subtropical China. Glob. Ecol. Conserv. 25:e01400. doi: 10.1016/j.gecco.2020.e01400
Bourtsoukidis, E., Behrendt, T., Yañez-Serrano, A. M., Hellén, H., Diamantopoulos, E., Catão, E., et al. (2018). Strong sesquiterpene emissions from Amazonian soils. Nat. Commun. 9:2226. doi: 10.1038/s41467-018-04658-y
Cleveland, C. C., and Yavitt, J. B. (1997). Consumption of atmospheric isoprene in soil. Geophys. Res. Lett. 24, 2379–2382. doi: 10.1029/97GL02451
Cleveland, C. C., and Yavitt, J. B. (1998). Microbial consumption of atmospheric isoprene in a temperate forest soil. Appl. Environ. Microbiol. 64, 172–177. doi: 10.1128/AEM.64.1.172-177.1998
Fang, J., Tan, X., Yang, Z., Shen, W., and Peñuelas, J. (2023). Contrasting terpene emissions from canopy and understory vegetation in response to increases in nitrogen deposition and seasonal changes in precipitation. Environ. Pollut. 317:120800. doi: 10.1016/j.envpol.2022.120800
Gray, C. M., Helmig, D., and Fierer, N. (2015). Bacteria and fungi associated with isoprene consumption in soil. Elem. Sci. Anth. 3:000053. doi: 10.12952/journal.elementa.000053
Gray, C. M., Monson, R. K., and Fierer, N. (2010). Emissions of volatile organic compounds during the decomposition of plant litter. J. Geophys. Res. Biogeosci. 115:G03015. doi: 10.1029/2010JG001291
Gray, C. M., Monson, R. K., and Fierer, N. (2014). Biotic and abiotic controls on biogenic volatile organic compound fluxes from a subalpine forest floor. J. Geophys. Res. Biogeosci. 119, 547–556. doi: 10.1002/2013JG002575
Greenberg, J. P., Asensio, D., Turnipseed, A., Guenther, A. B., Karl, T., and Gochis, D. (2012). Contribution of leaf and needle litter to whole ecosystem BVOC fluxes. Atmos. Environ. 59, 302–311. doi: 10.1016/j.atmosenv.2012.04.038
He, Y., Qin, L., Li, Z., Liang, X., Shao, M., and Tan, L. (2013). Carbon storage capacity of monoculture and mixed-species plantations in subtropical China. For. Ecol. Manag. 295, 193–198. doi: 10.1016/j.foreco.2013.01.020
Hellén, H., Hakola, H., Pystynen, K. H., Rinne, J., and Haapanala, S. (2006). C2–C10 hydrocarbon emissions from a boreal wetland and forest floor. Biogeosciences 3, 167–174. doi: 10.5194/bg-3-167-2006
Helmig, D., Ortega, J., Duhl, T., Tanner, D., Guenther, A., Harley, P., et al. (2007). Sesquiterpene emissions from pine trees—identifications, emission rates and flux estimates for the contiguous United States. Environ. Sci. Technol. 41, 1545–1553. doi: 10.1021/es0618907
Horváth, E., Hoffer, A., Sebők, F., Dobolyi, C., Szoboszlay, S., Kriszt, B., et al. (2012). Experimental evidence for direct sesquiterpene emission from soils. J. Geophys. Res. 117:D15304. doi: 10.1029/2012JD017781
Huang, X., Zheng, L., Guo, P., and Yi, Z. (2021). Nitrogen addition inhibits total monoterpene emissions in subtropical forest floor of South China. Soil Ecol. Lett. 3, 63–72. doi: 10.1007/s42832-020-0056-0
Insam, H., and Seewald, M. S. A. (2010). Volatile organic compounds (VOCs) in soils. Biol. Fertil. Soils 46, 199–213. doi: 10.1007/s00374-010-0442-3
IPCC (2014). “Climate change 2014: impacts, adaptation, and vulnerability. Part A: global and sectoral aspects” in Contribution of working group II to the fifth assessment report of the intergovernmental panel on climate change (New York: Oxford University Press)
Isidorov, V. A., Vinogorova, V. T., and Rafałowski, K. (2003). HS-SPME analysis of volatile organic compounds of coniferous needle litter. Atmos. Environ. 37, 4645–4650. doi: 10.1016/j.atmosenv.2003.07.005
Kramshøj, M., Albers, C. N., Holst, T., Holzinger, R., Elberling, B., and Rinnan, R. (2018). Biogenic volatile release from permafrost thaw is determined by the soil microbial sink. Nat. Commun. 9:3412. doi: 10.1038/s41467-018-05824-y
Kramshøj, M., Vedel-Petersen, I., Schollert, M., Rinnan, A., Nymand, J., Ro-Poulsen, H., et al. (2016). Large increases in Arctic biogenic volatile emissions are a direct effect of warming. Nat. Geosci. 9, 349–352. doi: 10.1038/ngeo2692
Kreuzwieser, J., and Rennenberg, H. (2013). “Flooding-driven emissions from trees” in Biology, controls and models oftree volatile organic compound emissions. eds. Ü. Niinemets and R. K. Monson (Dordrecht: Springer), 237–252.
Külheim, C., Padovan, A., Hefer, C., Krause, S. T., Köllner, T. G., Myburg, A. A., et al. (2015). The Eucalyptus terpene synthase gene family. BMC Genomics 16:450. doi: 10.1186/s12864-015-1598-x
Leff, J. W., and Fierer, N. (2008). Volatile organic compound (VOC) emissions from soil and litter samples. Soil Biol. Biochem. 40, 1629–1636. doi: 10.1016/j.soilbio.2008.01.018
Lin, C., Owen, S. M., and Peñuelas, J. (2007). Volatile organic compounds in the roots and rhizosphere of Pinus spp. Soil Biol. Biochem. 39, 951–960. doi: 10.1016/j.soilbio.2006.11.007
Llusià, J., Asensio, D., Sardans, J., Filella, I., Peguero, G., Grau, O., et al. (2022). Contrasting nitrogen and phosphorus fertilization effects on soil terpene exchanges in a tropical forest. Sci. Total Environ. 802:149769. doi: 10.1016/j.scitotenv.2021.149769
Mäki, M., Aalto, J., Hellén, H., Pihlatie, M., and Bäck, J. (2019a). Interannual and seasonal dynamics of volatile organic compound fluxes from the boreal forest floor. Front. Plant Sci. 10:191. doi: 10.3389/fpls.2019.00191
Mäki, M., Aaltonen, H., Heinonsalo, J., Hellén, H., Pumpanen, J., and Bäck, J. (2019b). Boreal forest soil is a significant and diverse source of volatile organic compounds. Plant Soil 441, 89–110. doi: 10.1007/s11104-019-04092-z
Mäki, M., Heinonsalo, J., Hellén, H., and Bäck, J. (2017). Contribution of understorey vegetation and soil processes to boreal forest isoprenoid exchange. Biogeosciences 14, 1055–1073. doi: 10.5194/bg-14-1055-2017
Mancuso, S., Taiti, C., Bazihizina, N., Costa, C., Menesatti, P., Giagnoni, L., et al. (2015). Soil volatile analysis by proton transfer reaction-time of flight mass spectrometry (PTR-TOF-MS). Appl. Soil Ecol. 86, 182–191. doi: 10.1016/j.apsoil.2014.10.018
Ming, A., Jia, H., Zhao, J., Tao, Y., and Li, Y. (2014). Above- and below-ground carbon stocks in an indigenous tree (Mytilaria laosensis) plantation chronosequence in subtropical China. PLoS One 9:e109730. doi: 10.1371/journal.pone.0109730
Mu, Z., Asensio, D., Llusià, J., Filella, I., Ogaya, R., Yi, Z., et al. (2022). Annual and seasonal variations in soil volatile organic compound concentrations in a Mediterranean shrubland and holm oak forest. Geoderma 405:115401. doi: 10.1016/j.geoderma.2021.115401
Mu, Z., Llusià, J., and Peñuelas, J. (2020). Ground level isoprenoid exchanges associated with Pinus pinea trees in a Mediterranean turf. Atmosphere 11:809. doi: 10.3390/atmos11080809
Nishida, N., Tamotsu, S., Nagata, N., Saito, C., and Sakai, A. (2005). Allelopathic effects of volatile monoterpenoids produced by Salvia leucophylla: inhibition of cell proliferation and DNA synthesis in the root apical meristem of Brassica campestris seedlings. J. Chem. Ecol. 31, 1187–1203. doi: 10.1007/s10886-005-4256-y
Nölscher, A. C., Yañez-Serrano, A. M., Wolff, S., de Araujo, A. C., Lavrič, J. V., Kesselmeier, J., et al. (2016). Unexpected seasonality in quantity and composition of Amazon rainforest air reactivity. Nat. Commun. 7:10383. doi: 10.1038/ncomms10383
Owen, S. M., Clark, S., Pompe, M., and Semple, K. T. (2007). Biogenic volatile organic compounds as potential carbon sources for microbial communities in soil from the rhizosphere of Populus tremula. FEMS Microbiol. Lett. 268, 34–39. doi: 10.1111/j.1574-6968.2006.00602.x
Pegoraro, E., Abrell, L., Haren, J. V., Barron-Gafford, G., Grieve, K. A., Malhi, Y., et al. (2005). The effect of elevated atmospheric CO2 and drought on sources and sinks of isoprene in a temperate and tropical rainforest mesocosm. Glob. Chang. Biol. 11, 1234–1246. doi: 10.1111/j.1365-2486.2005.00986.x
Peñuelas, J., Asensio, D., Tholl, D., Wenke, K., Rosenkranz, M., Piechulla, B., et al. (2014). Biogenic volatile emissions from the soil. Plant Cell Environ. 37, 1866–1891. doi: 10.1111/pce.12340
Purser, G., Drewer, J., Morison, J. I. L., and Heal, M. R. (2021). A first assessment of the sources of isoprene and monoterpene emissions from a short-rotation coppice Eucalyptus gunnii bioenergy plantation in the United Kingdom. Atmos. Environ. 262:118617. doi: 10.1016/j.atmosenv.2021.118617
Ramirez, K. S., Lauber, C. L., and Fierer, N. (2010). Microbial consumption and production of volatile organic compounds at the soil-litter interface. Biogeochemistry 99, 97–107. doi: 10.1007/s10533-009-9393-x
Rinnan, R., and Albers, C. N. (2020). Soil uptake of volatile organic compounds: ubiquitous and underestimated? J. Geophys. Res. Biogeosci. 125:e2020JG005773. doi: 10.1029/2020JG005773
Seco, R., Peñuelas, J., and Filella, I. (2007). Short-chain oxygenated VOCs: emission and uptake by plants and atmospheric sources, sinks, and concentrations. Atmos. Environ. 41, 2477–2499. doi: 10.1016/j.atmosenv.2006.11.029
Sivy, T. L., Shirk, M. C., and Fall, R. (2002). Isoprene synthase activity parallels fluctuations of isoprene release during growth of Bacillus subtilis. Biochem. Biophys. Res. Commun. 294, 71–75. doi: 10.1016/S0006-291X(02)00435-7
Staudt, M., Byron, J., Piquemal, K., and Williams, J. (2019). Compartment specific chiral pinene emissions identified in a maritime pine forest. Sci. Total Environ. 654, 1158–1166. doi: 10.1016/j.scitotenv.2018.11.146
Svendsen, S. H., Priemé, A., Voriskova, J., Kramshøj, M., Schostag, M., Jacobsen, C. S., et al. (2018). Emissions of biogenic volatile organic compounds from arctic shrub litter are coupled with changes in the bacterial community composition. Soil Biol. Biochem. 120, 80–90. doi: 10.1016/j.soilbio.2018.02.001
Tang, J., Schurgers, G., and Rinnan, R. (2019). Process understanding of soil BVOC fluxes in natural ecosystems: a review. Rev. Geophys. 57, 966–986. doi: 10.1029/2018RG000634
Trowbridge, A. M., Stoy, P. C., and Phillips, R. P. (2020). Soil biogenic volatile organic compound flux in a mixed hardwood forest: net uptake at warmer temperatures and the importance ofmycorrhizal associations. J. Geophys. Res. Biogeosci. 125:e2019JG005479. doi: 10.1029/2019JG005479
Veres, P. R., Behrendt, T., Klapthor, A., Meixner, F. X., and Williams, J. (2014). Volatile organic compound emissions from soil: using proton-transfer-reaction time-of-flight mass spectrometry (PTR-TOF-MS) for the real time observation of microbial processes. Biogeosci. Discuss. 11, 12009–12038. doi: 10.5194/bgd-11-12009-2014
Viros, J., Santonja, M., Temime-Roussel, B., Wortham, H., Fernandez, C., and Ormeño, E. (2021). Volatilome of Aleppo pine litter over decomposition process. Ecol. Evol. 11, 6862–6880. doi: 10.1002/ece3.7533
Wang, F., Li, Z., Xia, H., Zou, B., Li, N., Liu, J., et al. (2010). Effects of nitrogen-fixing and non-nitrogen-fixing tree species on soil properties and nitrogen transformation during forest restoration in southern China. Soil Sci. Plant Nutr. 56, 297–306. doi: 10.1111/j.1747-0765.2010.00454.x
Wei, X., and Blanco, J. A. (2014). Significant increase in ecosystem C can be achieved with sustainable forest management in subtropical plantation forests. PLoS One 9:e89688. doi: 10.1371/journal.pone.0089688
Wenke, K., Kai, M., and Piechulla, B. (2010). Belowground volatiles facilitate interactions between plant roots and soil organisms. Planta 231, 499–506. doi: 10.1007/s00425-009-1076-2
Zeng, J., Song, W., Zhang, Y., Mu, Z., Pang, W., Zhang, H., et al. (2022a). Emissions of isoprenoids from dominant tree species in subtropical China. Front. For. Glob. Change 5:1089676. doi: 10.3389/ffgc.2022.1089676
Zeng, J., Zhang, Y., Zhang, H., Song, W., Wu, Z., and Wang, X. (2022b). Design and characterization of a semi-open dynamic chamber for measuring biogenic volatile organic compound (BVOC) emissions from plants. Atmos. Meas. Tech. 15, 79–93. doi: 10.5194/amt-15-79-2022
Keywords: soil isoprenoid exchanges, isoprene, dynamic chamber, litter, soil temperature, Eucalyptus urophylla , plantation forest, subtropical China
Citation: Mu Z, Zeng J, Zhang Y, Song W, Pang W, Yi Z, Asensio D, Llusià J, Peñuelas J and Wang X (2023) Soil uptake of isoprenoids in a Eucalyptus urophylla plantation forest in subtropical China. Front. For. Glob. Change. 6:1260327. doi: 10.3389/ffgc.2023.1260327
Edited by:
Ling Zhang, Jiangxi Agricultural University, ChinaReviewed by:
Dianming Wu, East China Normal University, ChinaClément Stahl, Délégation Ile-de-France Sud (CNRS), France
Copyright © 2023 Mu, Zeng, Zhang, Song, Pang, Yi, Asensio, Llusià, Peñuelas and Wang. This is an open-access article distributed under the terms of the Creative Commons Attribution License (CC BY). The use, distribution or reproduction in other forums is permitted, provided the original author(s) and the copyright owner(s) are credited and that the original publication in this journal is cited, in accordance with accepted academic practice. No use, distribution or reproduction is permitted which does not comply with these terms.
*Correspondence: Xinming Wang, d2FuZ3htQGdpZy5hYy5jbg==