- 1FisioKlima-AgroSosT Research Group, Plant Biology and Ecology Department, Faculty of Pharmacy, University of the Basque Country, UPV-EHU, Vitoria-Gasteiz, Spain
- 2Department of Biodiversity, Ecology and Evolution, Faculty of Biological Sciences, Universidad Complutense de Madrid, Madrid, Spain
- 3Centro de Investigaciones sobre Desertificación (CIDE, CSIC-UV-GV), Valencia, Spain
- 4Grupo de Ecología y Restauración Forestal (FORECO), Departamento de Ciencias de la Vida, Universidad de Alcalá, Madrid, Spain
Introduction: Drought-associated tree mortality has been increasing worldwide since the last decades, impacting structure and functioning of forest ecosystems, with implications for energy, carbon and water fluxes. However, the understanding of the individual vulnerability to drought-induced mortality is still limited.
Methods: We aimed to identify the factors that triggered the mortality of the widely distributed Pinus sylvestris L. in an extensive forest area in central Spain. We compared radial growth patterns in pairs of alive and recently dead individuals that co-occur in close proximity and present similar age and size, thereby isolating the effects of size and environment from the mortality process. Temporal dynamics of growth, growth synchrony, and growth sensitivity to water availability (precipitation minus potential evapotranspiration) were compared between alive and recently dead trees.
Results and discussion: Over the last 50 years, although we did not detect significant differences in growth between alive and dead trees, an increase in the growth synchrony and sensitivity to water availability (i.e. slope of the climatic water balance in the growth model) was observed in all trees as drought intensity increased. 20 years before mortality, dead individuals showed lower growth synchrony and growth sensitivity to water availability than alive ones, without significant differences in growth. Recorded reduction in growth synchrony and growth sensitivity to water availability in dead trees suggests a decoupling between tree growth and climate, which could increase the risk of hydraulic failure and/or carbon starvation under increasingly arid conditions. Thus, the use of reduced growth sensitivity to water availability as potential early-warning signal of tree mortality, together with reduced growth synchrony, should be further explored, particularly in pine species in seasonally dry areas.
1. Introduction
The widespread increase in tree mortality has become one of the most serious threats to forest ecosystems worldwide over the past few decades (Allen et al., 2010; Millar and Stephenson, 2015; Greenwood et al., 2017). Increased aridity, along with extreme droughts and heat waves have been identified as the main drivers of tree mortality (Park Williams et al., 2013; Ruiz-Benito et al., 2013; Hammond et al., 2022). Moreover, both human management legacies and biotic agents can interact with climatic stressors, further increasing tree mortality (Linares et al., 2010; Anderegg et al., 2015; Sangüesa-Barreda et al., 2015; McDowell et al., 2022). The impact of increasing tree mortality, particularly in the form of massive mortality events, extends beyond individual trees and can have far-reaching effects on ecological communities, ecosystem functioning and services, and land-climate interactions (Anderegg et al., 2013). High tree mortality can alter species composition and distribution, and ultimately shifts forest communities towards higher dominance of shrub species (Allen and Breshears, 1998; Peñuelas et al., 2007; Herrero et al., 2013; Herrero and Zamora, 2014; Allen et al., 2015; Ruiz-Benito et al., 2017). The loss of tree cover can also have significant implications for energy, carbon and water fluxes, and nutrient cycling (Hughes et al., 2006; Allen, 2007; Royer et al., 2011; Xiong et al., 2011; Anderegg et al., 2013).
The current importance of tree mortality in forest functioning and resilience triggered the study of the tree mortality process (e.g., McDowell et al., 2022). However, the understanding of underlying mechanisms of tree mortality and the development of robust early-warning indicators remain limited due to complex interactions between drivers and involved processes (Camarero et al., 2015; Cailleret et al., 2017; Hartmann et al., 2018; McDowell et al., 2022). Tree mortality has been widely linked to hydraulic failure and carbon starvation, with evidence suggesting that they are not mutually exclusive but interdependent (McDowell et al., 2011). In this regard, dendroecology provides valuable information to unravel the physiological mechanisms behind tree mortality and to develop early warning indicators (Cailleret et al., 2017; Hartmann et al., 2018). Dendroecological studies allow for easy observation of growth rates, widely used as an indicator of tree vitality (Dobbertin, 2005), for almost the entire lifespan of sampled individuals across extensive areas, which is key for assessing climatic effects on tree functioning. Additionally, synchrony in radial growth, defined as coincident increases or decreases in radial growth over time across individuals in a single population, has been widely used as a sign of forest vulnerability to climate change. In this context, high coincidence in growth patterns between individuals (i.e., higher correlation in tree growth among individuals) reveals the strong impact of climatic stressors (Boden et al., 2014; Shestakova et al., 2016). Overall, the study of temporal changes in tree growth responses to drought and warming can provide insights into individual tree predisposition to mortality.
The comparison of growth patterns between co-occurring dead and surviving trees has become a common method for understanding and modelling tree mortality (Pedersen, 1998; Ogle et al., 2000; Cailleret et al., 2017). In many cases, a decline in growth prior to mortality has been observed, although the magnitude and duration of this decline varied depending on species’ functional characteristics and factors driving mortality (Gea-Izquierdo et al., 2014, 2019; Cailleret et al., 2017). For example, while drought-tolerant species and trees that died due to competition showed strong and long-lasting growth declines, trees that died from biotic attacks often showed small and short-term reductions (Cailleret et al., 2017). Growth patterns prior to mortality can provide valuable information on the physiological mechanisms underlying the mortality process, as changes in tree function (e.g., hydraulic conductivity) and structure (e.g., leaf area) affecting tree growth often precede mortality (McDowell et al., 2011; Seidl et al., 2011). Growth synchrony can also serve as an early warning indicator of mortality. For example, Cailleret et al. (2019) showed a decrease in the synchrony of gymnosperm species 20 years before mortality. However, to make comparisons between alive and dead trees more robust, it is essential to standardize variables that may affect radial growth and its variability, such as tree size and biotic and abiotic effects (Muñoz-Gálvez et al., 2021; Neycken et al., 2022). Studies that compare growth patterns between alive and dead trees while controlling for these key variables may provide new insights into possible growth-based early warning indicators. Given the wide variety of species and population responses, types of climatic stress, and combinations of mortality drivers (Cailleret et al., 2017), it is necessary to take advantage of as many tree die-off events as possible in order to refine and expand the existing knowledge regarding the tree mortality process and mortality risk indicators.
Tree species at the limits of their distribution, such as temperate or boreal species in drought-prone Mediterranean areas, often face particularly adverse ecological conditions (Hampe and Petit, 2005; Hampe and Jump, 2011). This could be aggravated by the increasing magnitude and frequency of extreme drought events in Mediterranean areas projected by climate change scenarios (IPCC, 2021), making temperate and boreal species growing in Mediterranean areas especially vulnerable to drought-induced mortality (Galiano et al., 2010; Herrero et al., 2013; Vila-Cabrera et al., 2013). Furthermore, reforestation and afforestation, and the abandonment of traditional forest management practices (e.g., tree harvest for wood fuel and forest clearing for cattle ranching) have increased the density of many Mediterranean forests, leading to increased inter-individual competition for water resources (Linares et al., 2010; Vila-Cabrera et al., 2013) and ultimately to forest decline and mortality events (Martínez-Vilalta and Pinol, 2002; Galiano et al., 2010; Vila-Cabrera et al., 2013; Gazol and Camarero, 2022). In this context, there is a critical need to study growth-based early-warning indicators of mortality in tree species’ rear-edge populations in Mediterranean areas, which can aid in implementing forest management and biodiversity conservation actions for the adaptation of these ecosystems to climate change.
In this study, we investigated growth-based mortality risk indicators in Scots pine (Pinus sylvestris L.) trees in a high-density forest in Central Spain. P. sylvestris is an economically important and widely distributed boreo-alpine species in Europe (Barbéro et al., 1998), with its southernmost limit in the Iberian Peninsula where it has experienced recent mortality events (Galiano et al., 2010; Vila-Cabrera et al., 2013). For this, we (i) evaluated the effects of intrinsic (tree size), biotic (competition) and abiotic (water availability) factors, and status (dead/alive) on the growth rates, (ii) analyzed the temporal pattern of growth sensitivity to water availability comparing dead and alive trees, and (iii) quantified growth synchrony comparing dead and alive trees. By doing so, we sought to gain insights into the mechanisms underlying tree mortality and help to develop a reliable growth-based mortality risk indicator for P. sylvestris in Mediterranean areas. In agreement with previous findings in gymnosperm species, we expect lower growth rates in dead than in surviving trees before mortality, likely due to their isohydric water regulation (Salmon et al., 2015; Cailleret et al., 2017). Furthermore, we anticipate that competition had a greater impact on growth in dead trees, which could intensify the adverse impact of drought (Linares et al., 2010). Finally, we also expect a decrease in growth synchrony in dead trees before mortality (Cailleret et al., 2019), suggesting a mismatch between growth and high-frequency climatic fluctuations.
2. Materials and methods
2.1. Study area
The study area is an extensive pine forest located in the Alto Tajo Natural Park of central Spain at an elevation of 1,450–1,600 m a.s.l. (40° 39′ 6.8″N, 1° 41′ 53.1″W). This forest experienced a widespread tree mortality event during 2016 and 2017. No sign of biotic agents was detected. The forest area is dominated by P. sylvestris. The soil type in the area is primarily calcium cambisols (Martín-Moreno et al., 2014). The study area is submitted to a continental Mediterranean climate with cold winters and mild and dry summers, with a mean annual temperature of 10.5°C and a mean annual total precipitation of 468 mm (period 1948–2017), according to data from a meteorological station of AEMET (Spanish Meteorological Agency) located at Molina de Aragón, which is situated 25 km from the study area.
2.2. Sampling design and dendrochronological methods
During October 2017, we sampled 39 pairs of trees within the study area. For each pair, one tree was observed to be in a healthy state (with no signs of decline), while the other was dead, but with dry needles still present in the crown indicating recent mortality. Tree mortality was confirmed in the following years. The sampled pairs had similar size and they were located no more than 5 meters apart from each other (with an average distance of 3 meters). Two cores were taken from each tree in opposite directions using a Pressler increment borer. The diameter at breast height (DBH) of each sampled tree was also recorded, along with the DBH of every neighboring adult tree (i.e., DBH > 7.5 cm, height > 130 cm) within a circular plot of 10 meters radius measured from the target trees.
Cores were processed following standard ecological methods (Fritts, 1976). They were air-dried for 48–72 h, mounted on wooden supports, and sanded with progressively finer grades of sandpaper to highlight ring-width patterns. All cores were visually cross-dated following the procedures described by Yamaguchi (1991). Cores were scanned at 1,200 dpi resolution (EPSON Perfection v800) and tree-ring width was measured using Image J software (Schneider et al., 2012). Trees can die during the growing season before ring formation is complete, which induces an incomplete outermost ring. As tree could have died at different times during the 2017 growing season, we did not consider the last ring of sampled trees (Cailleret et al., 2017). Ring width was converted to basal area increment (BAI, cm2 year−1) assuming a circular stem cross-section and using the following formula:
where rt and rt-1 are the radius of the tree at the end and at the beginning of a given annual ring, respectively. For each tree and year, the BAI was calculated as an average of the two extracted cores. BAI is a meaningful indicator of tree growth as it removes the variation in growth due to increasing circumference, making it more related to biomass increment than ring width (Biondi and Qeadan, 2008).
To quantify inter-individual competition in sampled trees, we calculated the Lorimer’s competition index (CI, Lorimer, 1983) using the following formula:
where BAvi is the basal area of each neighbor tree within the circular plot of 10 m radius and BAt is the basal area of the target tree. We employed the distance-independent Lorimer’s competition index, since it is appropriate to measure competition in monospecific forests (Kanta Bhandari et al., 2023 and references within) and has been previously used in Mediterranean areas (e.g., Muñoz-Gálvez et al., 2021).
2.3. Water availability
To quantify the annual water availability, we calculated the annual climatic water balance by subtracting the annual potential evapotranspiration (PET) from the total annual precipitation (P). The PET was determined following the method proposed by Thornthwaite (1948). To account for the influence of previous year conditions on the current growing season, we calculated annual climatic water balance from October of the previous year to September of the present year. Previous autumn and winter precipitation can play an important role recharging soil water reserves, and can boost current year radial growth in P. sylvestris (Sánchez-Salguero et al., 2012). We used climatic data from the AEMET meteorological station at Molina de Aragón.
2.4. Data analysis
We used a linear mixed-effect model to compare BAI series between alive and dead trees and assess the effect of tree size, competition and water availability on tree growth during the 1948–2017 period (a period selected to increase sample size). We included tree identity nested within tree-pairs as a random effect to account for the repeated measurements on the alive/dead paired individuals. We also used an autoregressive structure to consider the temporal autocorrelation of successive annual growth increments (Pinheiro et al., 2018). Tree size (DBH at the beginning of each year), competition (CI), water availability (P-PET), status (alive vs. dead), and the interactions status × competition and status × water availability were considered as fixed effects in the model. Size was included as a second-degree polynomial to allow for nonlinear responses of growth to variations in size (Sumida et al., 2013). All the variables were standardized by subtracting from its sample mean and by dividing by its standard deviation.
In order to assess the temporal variation of growth sensitivity to water availability in both alive and dead trees we used the mixed effect model described above. The model was fitted to 5-year lagged windows of 20 years (Díaz-Martínez et al., 2023; i.e., 11 time-windows of 20 years with a starting year from 1948 to 1998).
To assess growth synchrony within alive and dead trees we followed the procedure described by Alday et al. (2018). Briefly, we used variance–covariance mixed modelling using year as random term and calculated the mean inter-chronology correlation (i.e., growth synchrony) as the common inter-annual growth variance to all dead and alive trees. To do this, we tested different variance–covariance structures (see Alday et al., 2018 for a complete overview of variance–covariance structures) that allow to characterize synchrony for each level of the grouping variable (i.e., dead and alive trees). The best variance–covariance structure was selected using the Akaike Information Criterion (AIC, Zuur et al., 2009). The temporal changes in growth synchrony for alive and dead trees were also assessed using 20-year intervals with a 5-year moving window.
All statistical analyses were done in R 4.1.3 (R Core Team, 2022) using the nlme (Pinheiro et al., 2018), MuMIn (Barton, 2019) and R DendroSync (Alday et al., 2018) packages.
3. Results
3.1. Characteristics of sampled trees
Considering raw growth data (BAI) for the 1900–2017 period, alive and dead trees showed similar patterns along the 1900–2017 period, yet alive trees showed significantly lower mean BAI than dead trees (Table 1), especially between 1930 and 1980 (Figure 1). After 1980s, alive and dead trees showed similar growth rates, except in the last years when dead trees also showed greater growth than alive trees (Figure 1). No significant differences were found for age, size (DBH), competition index (CI) and surrounding basal area of sampled individuals (BA) between alive and dead trees (Table 1). All sampled trees presented an average age of 130 years and average DBH of 34 cm (Table 1).
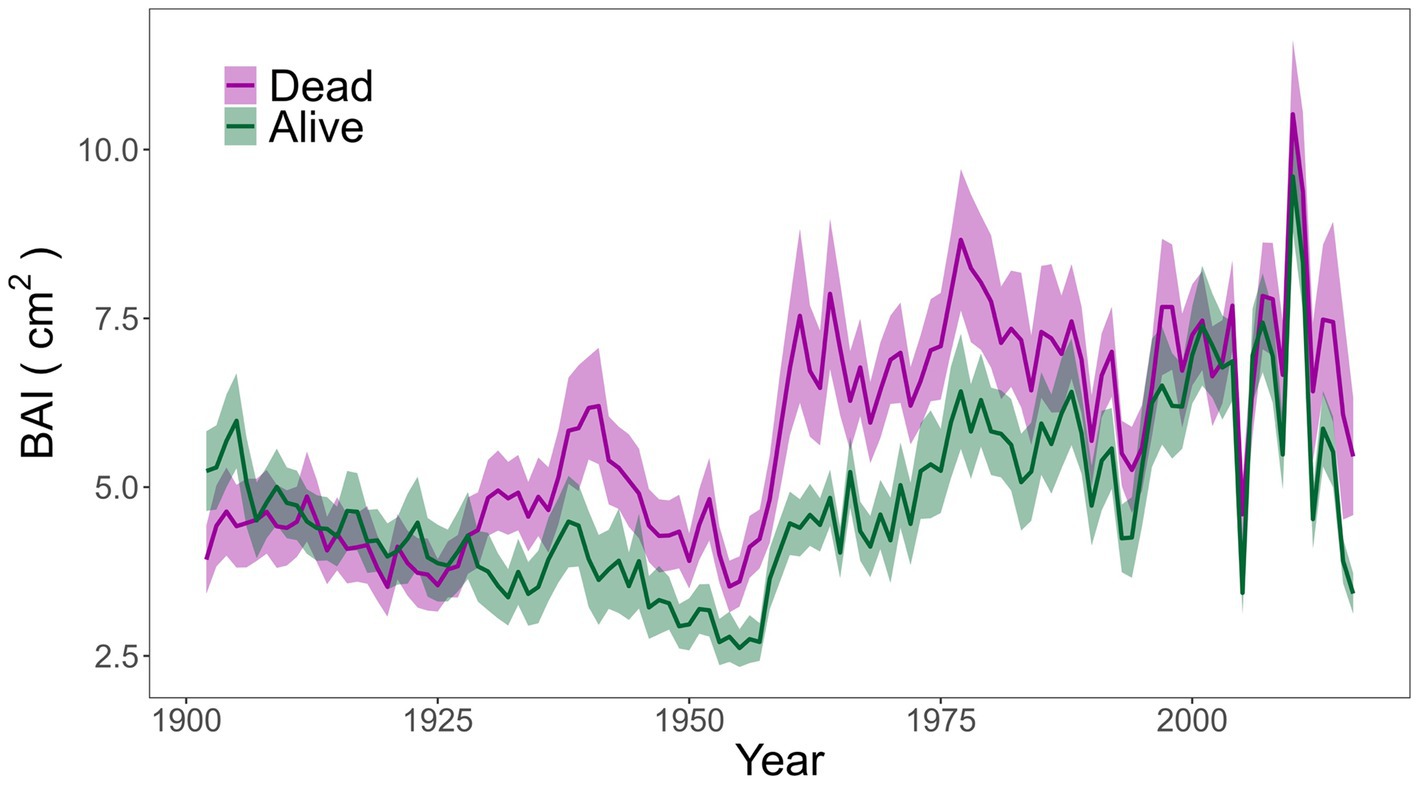
Figure 1. Basal Area Increment (BAI, cm2, ± SE) of alive and dead trees evaluated for the 1900–2017 period.
3.2. Effect of size, competition and climate on growth
Tree radial growth was significantly influenced by tree size (F = 86.74, p < 0.001), competition index (F = 7.35, p = 0.01), water availability (F = 207.11, p < 0.001) and its interaction with tree status (i.e., alive or dead; F = 8.97, p < 0.01). Overall, no significant differences were found on tree growth between alive and dead trees for the 1948–2017 period (F = 1.98, p = 0.17; Table 2). However, when water availability was low, dead trees showed greater growth than live trees (Figure 2). Competition had a negative effect on growth, whereas the effect of the water availability was positive (Table 2). Dead trees showed significantly less sensitivity to water availability (lower slope) than alive trees.
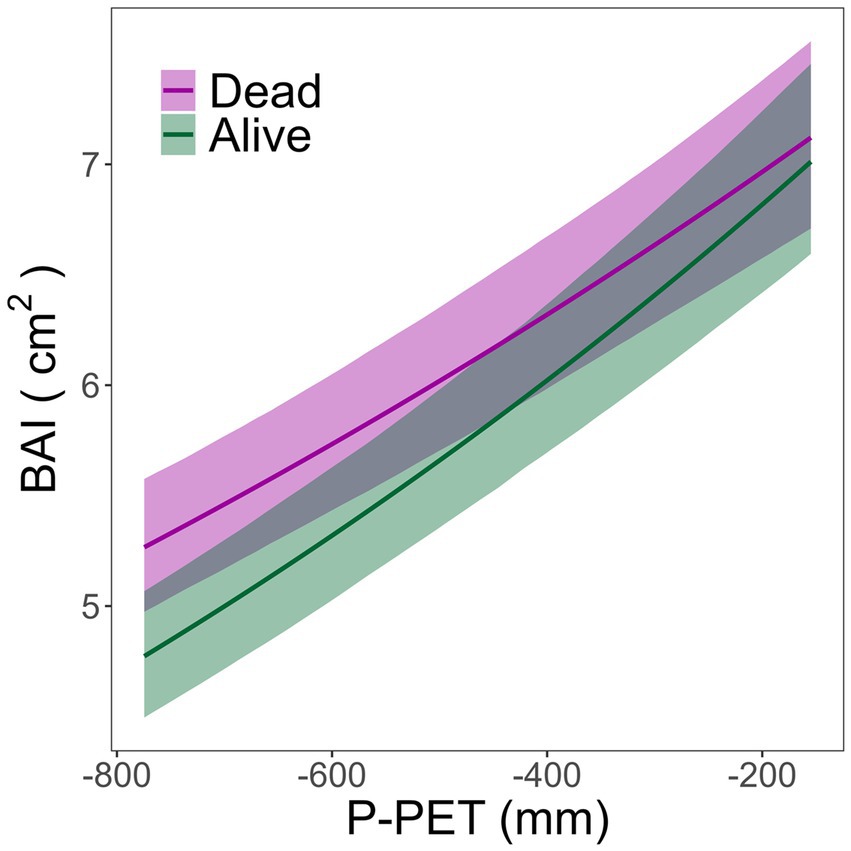
Figure 2. Predicted growth response (BAI, cm2, ± SE) to water availability (P-PET) for the period 1948–2017 in dead and alive trees.
3.3. Temporal changes in growth patterns
Time windows analysis showed similar growth patterns between live and dead trees for the studied period (Figure 3; Supplementary Table S1).
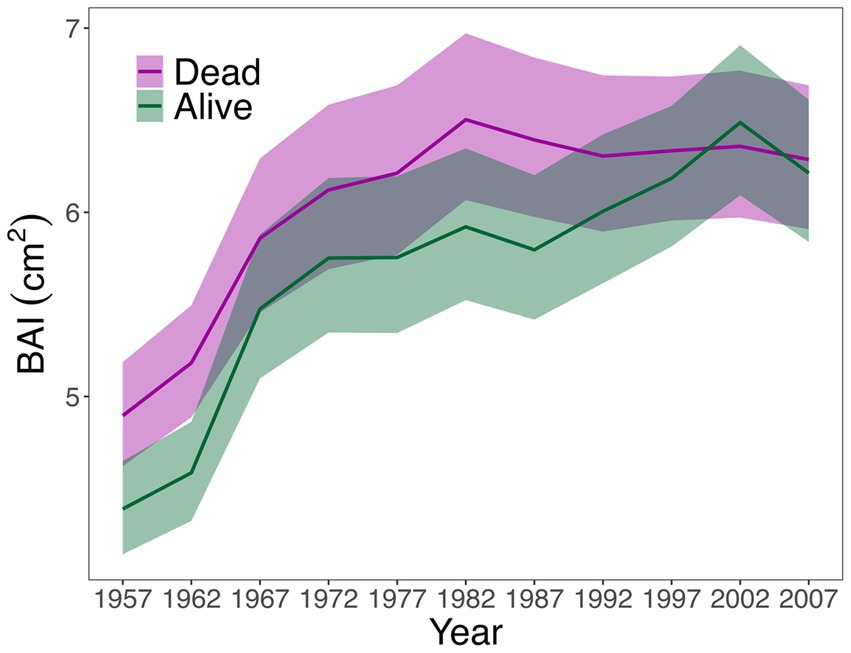
Figure 3. Predicted temporal growth evolution (BAI, cm2, ± SE) in alive and dead trees for the 1948–2017 period applying the model at 20-year intervals with a 5-year moving window. The years appearing on the x-axis correspond to the midpoints of each 20-year interval.
Growth sensitivity to water availability increased along the study period, coinciding with a decrease in water availability (Figure 4). Alive trees showed greater sensitivity than dead trees during the first half of the study period. However, this difference disappeared, as water availability decreased, until the last two 20-year intervals, when dead trees showed lower growth sensitivity to water availability than alive ones (Figure 4).
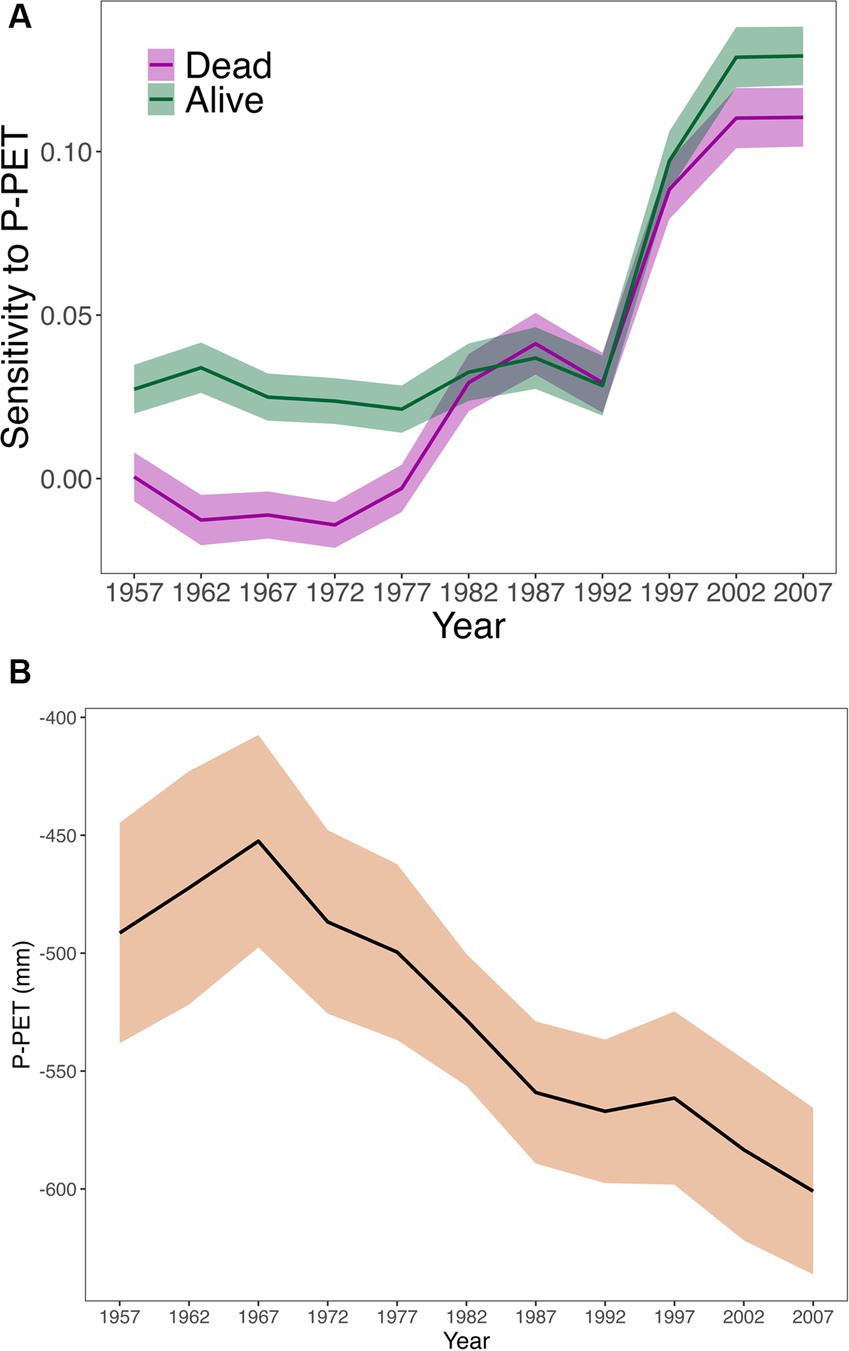
Figure 4. Temporal evolution of (A) predicted (± SE) growth sensitivity to water availability (i.e., slope of climatic water balance in the growth model) in dead and alive trees, and of (B) the water availability (P-PET) for the 1948–2017 period. Data are showed for 20-year intervals with a 5-years moving window. The years appearing on the x-axis correspond to the midpoints of each 20-year interval.
3.4. Growth synchrony
The best-fit variance–covariance structure for calculating synchrony was a heterocedastic matrix with a composite symmetry structure (mHeCS; Supplementary Table S2). Firstly, the selection of a heterocedastic matrix implies that the magnitude of the common growth signal (i.e., growth synchrony) varied significantly between live and dead trees. Secondly, a matrix with a symmetrical structure suggests the existence of fluctuations in growth that were common for both live and dead trees. Overall, alive trees exhibited significantly higher synchrony than dead ones (0.23 ± 0.03 and 0.16 ± 0.02, respectively).
Regarding temporal changes in synchrony, growth synchrony values were relatively low until the last 30 years, when a steep increase in synchrony was recorded (Figure 5). The synchrony values of alive and dead trees diverged markedly during two periods: in the second quarter of the study period and in the last interval analyzed (just before the mortality event; Figure 5).
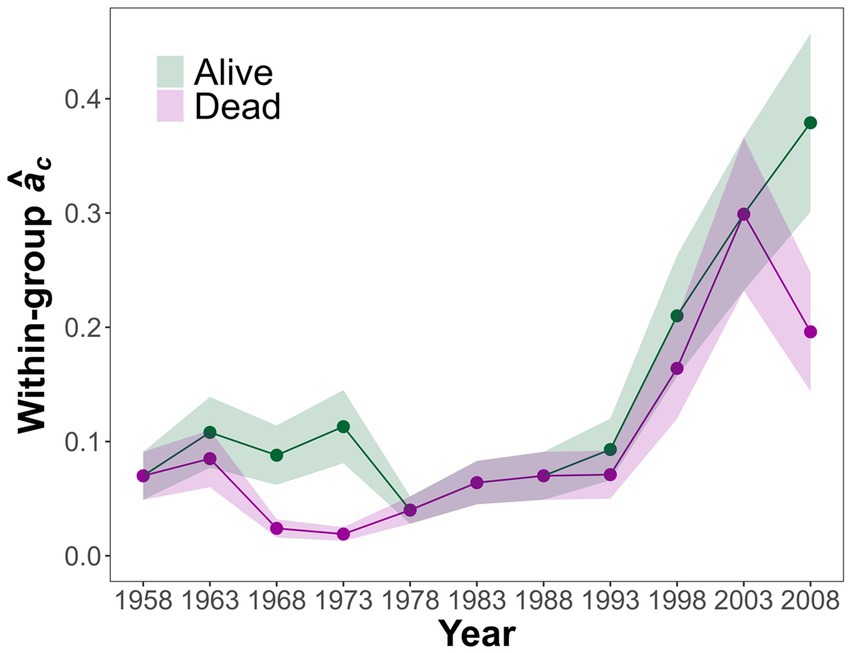
Figure 5. Temporal evolution of growth synchrony estimates (âc) for live and dead trees for the 1948–2017 study period. Data are showed for 20-year intervals with a 5-years moving window. The years appearing on the x-axis correspond to the midpoints of each 20-year interval.
4. Discussion
Our study revealed significant differences between alive and dead trees in growth-related parameters under a context of increasing aridity. Both, growth sensitivity to water availability and synchrony were lower in dead than in alive trees before mortality. This suggests a decoupling of tree growth from climatic fluctuations in dead trees that may have increased their risk of sudden death due to hydraulic failure and/or carbon starvation. Thus, reductions in growth sensitivity to water availability, together with decreased tree growth synchrony, should also be considered as an early-warning indicator of tree mortality in Mediterranean P. sylvestris populations located at the southern distribution limit of the species.
Contrary to our first hypothesis, our study did not find lower growth in dead than in alive trees prior to mortality, nor a sustained decrease in growth in dead trees or any other apparent signs of growth decline. These results contrast with those reported by Cailleret et al. (2017) for several gymnosperm species. Nevertheless, our results are consistent with other studies that have found no differences between dead and alive trees at stand and regional scales (Ferrenberg et al., 2014; Rowland et al., 2015; Berdanier and Clark, 2016; Herguido et al., 2016). When considering our design based on dead/alive tree pairs, there was no significant difference in growth between dead and alive trees during the first 50 years of life (data not shown), ruling out the possibility that mortality was due to a trade-off between early-growth and longevity (Bigler et al., 2007; Bigler and Veblen, 2009). Additionally, the size of alive and dead trees studied was similar. Therefore, we argue that dead trees could have prioritized radial growth (over other tree functions) until death regardless of environmental stress, or that mortality occurred abruptly due to an extreme environmental stress leading to tree death without a previous impact on its carbon balance (Cailleret et al., 2017).
We found that the effect of water availability on growth varied between alive and dead trees, with the later showing higher growth during extremely dry conditions (i.e., lower growth sensitivity to water availability). Thus, under severe drought conditions, dead trees may exhibit a less conservative water use than live trees, which could expose them to a higher risk of hydraulic failure due to the accumulation of enbolisms in the xylem (McDowell et al., 2022). Our results contrast with previous studies on P. sylvestris, which showed greater sensitivity to drought and hot conditions for dead trees and a sustained decline in growth (Hereş et al., 2012). However, our results are consistent with previous studies performed on saplings, where P. sylvetstris appears unable to decrease its height growth through more strict stomatal control under extreme drought conditions (Herrero et al., 2013). These differences in growth responses to drought conditions in dead trees between studies may be due to intraspecific variability and the intensity and recurrence of drought events (Cailleret et al., 2017).
We did not find support for a greater impact of competition on growth in dead than alive trees. Our results showed that negative effects of competition on tree growth were similar for alive and dead trees, as well as the inter-individual competition index (CI), which suggests a limited effect of competition on the mortality event. Nonetheless, the consistent negative effect of competition on radial growth of alive and dead trees suggests the key role of competition on exacerbating drought impacts on Mediterranean forests (Linares et al., 2010; Vila-Cabrera et al., 2013). However, it should be noted that we used a static competition index measured at sampling, so it can not reflect the effect of competition on the entire growth series. This represents an important limitation in clarifying the role that competition may play in drought-induced growth reductions over time (Marqués et al., 2021).
The study area experienced a progressive decrease in water availability over time. As drought conditions intensified, sampled trees increased their growth sensitivity to water availability. However, at the beginning and at the end of the study period, dead trees showed lower sensitivity to water availability, indicating potential differences in physiological responses to water stress between alive and dead individuals. Lower growth sensitivity to water availability preceding mortality implies lower stomatal responsiveness, which can lead to higher stomatal conductance and water use under dry conditions as confirmed by higher growth of dead trees under low water availability conditions. This exposes trees to higher risk of xylem embolism, increasing the probability of drought-induced hydraulic failure through accumulation of cavitation processes (McDowell et al., 2008, 2022). The gradual increase in aridity may have pushed individuals closer to threshold values where cavitation processes occur. However, the interdependence of water and carbon fluxes in tree functioning and vigor preclude us to exclude carbon starvation as the main mechanism involved in the mortality process (McDowell et al., 2022). Rising temperatures increase respiration costs, decreasing even more the carbon supply for tree functions during dry periods of low carbon assimilation (Adams et al., 2009; McDowell et al., 2011). Also, the less conservative water use associated to lower growth sensitivity to water availability could increase tree allocation to aboveground biomass (particularly leaf area) during favorable conditions, which cannot be hydraulically and metabolically sustained during extreme and hot droughts (i.e., structural overshoot, Jump et al., 2017). Therefore, in this context of increasing aridity, reduced sensitivity of growth to water availability may increase the risk of drought mortality and could be used as an early-warning signal of tree mortality.
The observed patterns of increased growth synchrony over time are similar to the increased sensitivity to water availability. In fact, higher growth sensitivity could explain, in part, the increase in growth synchrony, since tree growth would be more and more linked to water availability as conditions becomes progressively drier, homogenizing growth responses across the population. The observed increase in growth synchrony is consistent with previous studies at local and regional scales recording pervasive climatic effects on tree growth (Boden et al., 2014; Shestakova et al., 2016; Gazol et al., 2020). Actually, increased synchrony is considered an indicator of tree vulnerability to climate change, as it reduces individual variability and resilience capacity at population level (Clark et al., 2012; Tejedor et al., 2020). That is, increased growth synchrony to climatic stressors amplify the risk of extensive negative impacts on tree vigor at population level. In this context of increased aridity and vulnerability at individual and population levels, differences in growth responses between individuals could play a key role in mortality risk through the aforementioned susceptibility to xylem embolism and decreased carbon supply to tree functions. Therefore, under drier conditions trees would be closer to threshold values related to key tree functions (e.g., stomatal conductance) leading to permanent drought-induced damage, and ultimately to tree death. From this perspective, and in line with our third hypothesis, lower synchrony was observed in dead trees both prior to the mortality event and during the first half of the study period. The observed decrease in synchrony before mortality agrees with previous studies in gymnosperms (Cailleret et al., 2019) and is also consistent with the reduction in growth sensitivity to water availability. Both aspects indicate a decoupling between growth and climatic fluctuations, which could be linked to the aforementioned less conservative water use in dead than in alive individuals (Herrero et al., 2013; present study), and the subsequent increase in vulnerability to cavitation processes (McDowell et al., 2008). Thus, reductions in growth synchrony should be also considered as a potential indicator for tree mortality risk in seasonally water limited areas within P. sylvestris distribution.
Overall, our study, which takes advantage of a paired design that minimizes the effects of size and immediate tree environment on potential causes of mortality, highlights reduced sensitivity to water availability and growth synchrony as potential indicators of drought-mortality risk in southern populations of the widely distributed P. sylvestris. These indicators could also be relevant for other isohydric pine species (Salmon et al., 2015) in seasonally dry areas. Thus, we suggest the need for assessing changes in growth sensitivity to water availability in further studies (Suarez et al., 2004; Férriz et al., 2021; present study). We also recommend to further study the responses of growth sensitivity to water availability to elucidate the underlying tree mortality mechanisms at different spatial extents and standardize the assessment method of growth sensitivity to water availability, in order to make studies comparable. Despite our sampling protocol is aimed to reduce environmental differences between dead and alive trees, we cannot discard that intraspecific differences between trees in functional traits (e.g., rooting depth) and/or in soil properties might lead to different drought impacts in sampled trees. In this regard, the quantification of other variables, in addition to radial growth, related to tree vigor (e.g., branch and foliar biomass) and physiological responses to drought (e.g., water use efficiency) as well as a more robust evaluation of competition along tree lifespan, may also provide valuable insights into the mechanisms involved in the tree mortality process and inform forest management for the adaptation to climate change.
Data availability statement
The raw data supporting the conclusions of this article will be made available by the authors, without undue reservation.
Author contributions
EA, PR-B, and AH: sampling design and idea. EA, PG-D, and PR-B: field work. RG-G: sample processing. EA and AH: analyses. AH: manuscript writing. All authors contributed to the article and approved the submitted version.
Funding
AH was supported by the Basque Country Government funding support to FisioKlima-AgroSosT (IT1682-22) consolidated research group. EA acknowledges the support of grants PID2019-110470RA-100 (ADAPTAMIX) funded by MCIN/AEI/10.13039/501100011033, and REMEDINAL-TE (Community of Madrid, S2018/EMT-4338). PG-D was supported by a postdoctoral contract under the grant RYC2020-029226-I. PR-B and AH were supported by the Science and Innovation Ministry (subproject LARGE, N° _PID2021-123675OB-C41). PR-B was supported by the Community of Madrid Region under the framework of the multi-year Agreement with the University of Alcalá (Stimulus to Excellence for Permanent University Professors, EPU-INV/2020/010).
Acknowledgments
We thank Daniel Gómez and Pablo Quiles for field assistance.
Conflict of interest
The authors declare that the research was conducted in the absence of any commercial or financial relationships that could be construed as a potential conflict of interest.
Publisher’s note
All claims expressed in this article are solely those of the authors and do not necessarily represent those of their affiliated organizations, or those of the publisher, the editors and the reviewers. Any product that may be evaluated in this article, or claim that may be made by its manufacturer, is not guaranteed or endorsed by the publisher.
Supplementary material
The Supplementary material for this article can be found online at: https://www.frontiersin.org/articles/10.3389/ffgc.2023.1249246/full#supplementary-material
References
Adams, H. D., Guardiola-Claramonte, M., Barron-Gafford, G. A., Villegas, J. C., Breshears, D. D., Zou, C. B., et al. (2009). Temperature sensitivity of drought-induced tree mortality portends increased regional die-off under global-change-type drought. Proc. Natl. Acad. Sci. 106, 7063–7066. doi: 10.1073/pnas.0901438106
Alday, J. G., Shestakova, T. A., Resco de Dios, V., and Voltas, J. (2018). DendroSync: an R package to unravel synchrony patterns in tree-ring networks. Dendrochronologia 47, 17–22. doi: 10.1016/j.dendro.2017.12.003
Allen, C. D. (2007). Interactions across spatial scales among forest dieback, fire, and erosion in northern New Mexico landscapes. Ecosystems 10, 797–808. doi: 10.1007/s10021-007-9057-4
Allen, C. D., and Breshears, D. D. (1998). Drought-induced shift of a forest-woodland ecotone: rapid landscape response to climate variation. Proc. Natl. Acad. Sci. U. S. A. 95, 14839–14842. doi: 10.1073/pnas.95.25.14839
Allen, C. D., Breshears, D. D., and McDowell, N. G. (2015). On underestimation of global vulnerability to tree mortality and forest die-off from hotter drought in the Anthropocene. Ecosphere 6, 1–55. doi: 10.1890/es15-00203.1
Allen, C. D., Macalady, A. K., Chenchouni, H., Bachelet, D., McDowell, N., Vennetier, M., et al. (2010). A global overview of drought and heat-induced tree mortality reveals emerging climate change risks for forests. For. Ecol. Manag. 259, 660–684. doi: 10.1016/j.foreco.2009.09.001
Anderegg, W. R. L., Hicke, J. A., Fisher, R. A., Allen, C. D., Aukema, J., Bentz, B., et al. (2015). Tree mortality from drought, insects, and their interactions in a changing climate. New Phytol. 208, 674–683. doi: 10.1111/nph.13477
Anderegg, W. R. L., Kane, J. M., and Anderegg, L. D. L. (2013). Consequences of widespread tree mortality triggered by drought and temperature stress. Nat. Clim. Chang. 3, 30–36. doi: 10.1038/nclimate1635
Barbéro, M., Loisel, R., Quézel, P., Richardson, D. M., and Romane, F. (1998). “Pines of the Mediterranean Basin” in Ecology and biogeography of Pinus. ed. D. M. Richardson (Cambridge: Cambridge University Press), 153–170.
Barton, K. (2019). Mu-MIn: Multi-model inference. R Package Version1.43.6. Available at: http://R-Forge.R-project.org/projects/mumin/ (Accessed October 31, 2023).
Berdanier, A. B., and Clark, J. S. (2016). Multiyear drought-induced morbidity preceding tree death in southeastern U.S. forests. Ecol. Appl. 26, 17–23. doi: 10.1890/15-0274
Bigler, C., Gavin, D. G., Gunning, C., and Veblen, T. T. (2007). Drought induces lagged tree mortality in a subalpine forest in the Rocky Mountains. Oikos 116, 1983–1994. doi: 10.1111/j.2007.0030-1299.16034.x
Bigler, C., and Veblen, T. T. (2009). Increased early growth rates decrease longevities of conifers in subalpine forests. Oikos 118, 1130–1138. doi: 10.1111/j.1600-0706.2009.17592.x
Biondi, F., and Qeadan, F. (2008). A theory-driven approach to tree-ring standardization: defining the biological trend from expected basal area increment. Tree-Ring Res. 64, 81–96. doi: 10.3959/2008-6.1
Boden, S., Kahle, H.-P., Wilpert, K.von, and Spiecker, H. (2014). Resilience of Norway spruce (Picea abies (L.) karst) growth to changing climatic conditions in Southwest Germany. For. Ecol. Manag. 315, 12–21. doi: 10.1016/j.foreco.2013.12.015
Cailleret, M., Dakos, V., Jansen, S., Robert, E. M. R., Aakala, T., Amoroso, M. M., et al. (2019). Early-warning signals of individual tree mortality based on annual radial growth. Front. Plant Sci. 9:1964. doi: 10.3389/fpls.2018.01964
Cailleret, M., Jansen, S., Robert, E. M. R., Desoto, L., Aakala, T., Antos, J. A., et al. (2017). A synthesis of radial growth patterns preceding tree mortality. Glob. Chang. Biol. 23, 1675–1690. doi: 10.1111/gcb.13535
Camarero, J. J., Gazol, A., Sangueesa-Barreda, G., Oliva, J., and Vicente-Serrano, S. M. (2015). To die or not to die: early warnings of tree dieback in response to a severe drought. J. Ecol. 103, 44–57. doi: 10.1111/1365-2745.12295
Clark, J. S., Bell, D. M., Kwit, M., Stine, A., Vierra, B., and Zhu, K. (2012). Individual-scale inference to anticipate climate-change vulnerability of biodiversity. Philos. Trans. R. Soc. B Biol. Sci. 367, 236–246. doi: 10.1098/rstb.2011.0183
Díaz-Martínez, P., Ruiz-Benito, P., Madrigal-González, J., Gazol, A., and Andivia, E. (2023). Positive effects of warming do not compensate growth reduction due to increased aridity in Mediterranean mixed forests. Ecosphere 14:e4380. doi: 10.1002/ecs2.4380
Dobbertin, M. (2005). Tree growth as indicator of tree vitality and of tree reaction to environmental stress: a review. Eur. J. For. Res. 124, 319–333. doi: 10.1007/s10342-005-0085-3
Ferrenberg, S., Kane, J. M., and Mitton, J. B. (2014). Resin duct characteristics associated with tree resistance to bark beetles across lodgepole and limber pines. Oecologia 174, 1283–1292. doi: 10.1007/s00442-013-2841-2
Férriz, M., Martin-Benito, D., Cañellas, I., and Gea-Izquierdo, G. (2021). Sensitivity to water stress drives differential decline and mortality dynamics of three co-occurring conifers with different drought tolerance. For. Ecol. Manage. 486:118964. doi: 10.1016/j.foreco.2021.118964
Galiano, L., Martinez-Vilalta, J., and Lloret, F. (2010). Drought-induced multifactor decline of scots pine in the Pyrenees and potential vegetation change by the expansion of co-occurring oak species. Ecosystems 13, 978–991. doi: 10.1007/s10021-010-9368-8
Gazol, A., and Camarero, J. J. (2022). Compound climate events increase tree drought mortality across European forests. Sci. Total Environ. 816:151604. doi: 10.1016/j.scitotenv.2021.151604
Gazol, A., Camarero, J. J., Sangüesa-Barreda, G., Serra-Maluquer, X., Sánchez-Salguero, R., Coll, L., et al. (2020). Tree species are differently impacted by cumulative drought stress and present higher growth synchrony in dry places. Front. For. Glob. Chang. 3:573346. doi: 10.3389/ffgc.2020.573346
Gea-Izquierdo, G., Férriz, M., García-Garrido, S., Aguín, O., Elvira-Recuenco, M., Hernandez-Escribano, L., et al. (2019). Synergistic abiotic and biotic stressors explain widespread decline of Pinus pinaster in a mixed forest. Sci. Total Environ. 685, 963–975. doi: 10.1016/j.scitotenv.2019.05.378
Gea-Izquierdo, G., Viguera, B., Cabrera, M., and Cañellas, I. (2014). Drought induced decline could portend widespread pine mortality at the xeric ecotone in managed mediterranean pine-oak woodlands. For. Ecol. Manag. 320, 70–82. doi: 10.1016/j.foreco.2014.02.025
Greenwood, S., Ruiz-Benito, P., Martínez-Vilalta, J., Lloret, F., Kitzberger, T., Allen, C. D., et al. (2017). Tree mortality across biomes is promoted by drought intensity, lower wood density and higher specific leaf area. Ecol. Lett. 20, 539–553. doi: 10.1111/ele.12748
Hammond, W. M., Williams, A. P., Abatzoglou, J. T., Adams, H. D., Klein, T., López, R., et al. (2022). Global field observations of tree die-off reveal hotter-drought fingerprint for Earth’s forests. Nat. Commun. 13:1761. doi: 10.1038/s41467-022-29289-2
Hampe, A., and Jump, A. S. (2011). Climate relicts: past, present, future. Annu. Rev. Ecol. Evol. Syst. 42, 313–333. doi: 10.1146/annurev-ecolsys-102710-145015
Hampe, A., and Petit, R. J. (2005). Conserving biodiversity under climate change: the rear edge matters. Ecol. Lett. 8, 461–467. doi: 10.1111/j.1461-0248.2005.00739.x
Hartmann, H., Moura, C. F., Anderegg, W. R. L., Ruehr, N. K., Salmon, Y., Allen, C. D., et al. (2018). Research frontiers for improving our understanding of drought-induced tree and forest mortality. New Phytol. 218, 15–28. doi: 10.1111/nph.15048
Hereş, A. M., Martínez-Vilalta, J., and Claramunt López, B. (2012). Growth patterns in relation to drought-induced mortality at two scots pine (Pinus sylvestris L.) sites in NE Iberian Peninsula. Trees 26, 621–630. doi: 10.1007/s00468-011-0628-9
Herguido, E., Granda, E., Benavides, R., García-Cervigón, A. I., Camarero, J. J., and Valladares, F. (2016). Contrasting growth and mortality responses to climate warming of two pine species in a continental Mediterranean ecosystem. For. Ecol. Manag. 363, 149–158. doi: 10.1016/j.foreco.2015.12.038
Herrero, A., Castro, J., Zamora, R., Delgado-Huertas, A., and Querejeta, J. I. (2013). Growth and stable isotope signals associated with drought-related mortality in saplings of two coexisting pine species. Oecologia 173, 1613–1624. doi: 10.1007/s00442-013-2707-7
Herrero, A., and Zamora, R. (2014). Plant responses to extreme climatic events: A field test of resilience capacity at the southern range edge. PLoS One 9:e87842. doi: 10.1371/journal.pone.0087842
Hughes, R. F., Archer, S. R., Asner, G. P., Wessman, C. A., McMurtry, C., Nelson, J., et al. (2006). Changes in aboveground primary production and carbon and nitrogen pools accompanying woody plant encroachment in a temperate savanna. Glob. Chang. Biol. 12, 1733–1747. doi: 10.1111/j.1365-2486.2006.01210.x
IPCC (2021). “Climate change 2021: the physical science basis” in Contribution of working group I to the sixth assessment report of the intergovernmental panel on climate change. eds. V. Masson-Delmotte, P. Zhai, A. Pirani, S. L. Connors, C. Péan, and S. Berger, et al. (Cambridge, United Kingdom and New York, NY, USA: Cambridge University Press), 2391.
Jump, A. S., Ruiz-Benito, P., Greenwood, S., Allen, C. D., Kitzberger, T., Fensham, R., et al. (2017). Structural overshoot of tree growth with climate variability and the global spectrum of drought-induced forest dieback. Glob. Chang. Biol. 23, 3742–3757. doi: 10.1111/gcb.13636
Kanta Bhandari, S., Veneklaas, E. J., McCaw, L., Mazanec, R., Whitford, K., and Renton, M. (2023). Investigating above- vs. below-ground competition by accounting for azimuth of competitors in native eucalypt forests. For. Ecol. Manag. 542:121103. doi: 10.1016/j.foreco.2023.121103
Linares, J. C., Camarero, J. J., and Carreira, J. A. (2010). Competition modulates the adaptation capacity of forests to climatic stress: insights from recent growth decline and death in relict stands of the Mediterranean fir Abies pinsapo. J. Ecol. 98, 592–603. doi: 10.1111/j.1365-2745.2010.01645.x
Lorimer, C. G. (1983). Tests of age-independent competition indices for individual trees in natural hardwood stands. For. Ecol. Manag. 6, 343–360. doi: 10.1016/0378-1127(83)90042-7
Marqués, L., Camarero, J. J., Zavala, M. A., Stoffel, M., Ballesteros-Cánovas, J. A., Sancho-García, C., et al. (2021). Evaluating tree-to-tree competition during stand development in a relict scots pine forest: how much does climate matter? Trees 35, 1207–1219. doi: 10.1007/s00468-021-02109-8
Martínez-Vilalta, J., and Pinol, J. (2002). Drought-induced mortality and hydraulic architecture in pine populations of the NE Iberian Peninsula. For. Ecol. Manag. 161, 247–256. doi: 10.1016/S0378-1127(01)00495-9
Martín-Moreno, C., Fidalgo Hijano, C., Martín Duque, J. F., González Martín, J. A., Zapico Alonso, I., and Laronne, J. B. (2014). The Ribagorda sand gully (East-Central Spain): sediment yield and human-induced origin. Geomorphology 224, 122–138. doi: 10.1016/j.geomorph.2014.07.013
McDowell, N. G., Beerling, D. J., Breshears, D. D., Fisher, R. A., Raffa, K. F., and Stitt, M. (2011). The interdependence of mechanisms underlying climate-driven vegetation mortality. Trends Ecol. Evol. 26, 523–532. doi: 10.1016/j.tree.2011.06.003
McDowell, N., Pockman, W. T., Allen, C. D., Breshears, D. D., Cobb, N., Kolb, T., et al. (2008). Mechanisms of plant survival and mortality during drought: why do some plants survive while others succumb to drought? New Phytol. 178, 719–739. doi: 10.1111/j.1469-8137.2008.02436.x
McDowell, N. G., Sapes, G., Pivovaroff, A., Adams, H. D., Allen, C. D., Anderegg, W. R. L., et al. (2022). Mechanisms of woody-plant mortality under rising drought, CO2 and vapour pressure deficit. Nat. Rev. Earth Environ. 3, 294–308. doi: 10.1038/s43017-022-00272-1
Millar, C. I., and Stephenson, N. L. (2015). Temperate forest health in an era of emerging megadisturbance. Science 349, 823–826. doi: 10.1126/science.aaa9933
Muñoz-Gálvez, F. J., Herrero, A., Esther Pérez-Corona, M., and Andivia, E. (2021). Are pine-oak mixed stands in Mediterranean mountains more resilient to drought than their monospecific counterparts? For. Ecol. Manag. 484:118955. doi: 10.1016/j.foreco.2021.118955
Neycken, A., Scheggia, M., Bigler, C., and Lévesque, M. (2022). Long-term growth decline precedes sudden crown dieback of European beech. Agric. For. Meteorol. 324:109103. doi: 10.1016/j.agrformet.2022.109103
Ogle, K., Whitham, T. G., and Cobb, N. S. (2000). Tree-ring variation in pinyon predicts likelihood of death following severe drought. Ecology 81, 3237–3243. doi: 10.1890/0012-9658(2000)081[3237:TRVIPP]2.0.CO;2
Park Williams, A., Allen, C. D., Macalady, A. K., Griffin, D., Woodhouse, C. A., Meko, D. M., et al. (2013). Temperature as a potent driver of regional forest drought stress and tree mortality. Nat. Clim. Chang. 3, 292–297. doi: 10.1038/nclimate1693
Pedersen, B. S. (1998). Modeling tree mortality in response to short- and long-term environmental stresses. Ecol. Model. 105, 347–351. doi: 10.1016/S0304-3800(97)00162-2
Peñuelas, J., Ogaya, R., Boada, M., and Jump, S. (2007). Migration, invasion and decline: changes in recruitment and forest structure in a warming-linked shift of European beech forest in Catalonia (NE Spain). Ecography 30, 829–837. doi: 10.1111/j.2007.0906-7590.05247.x
Pinheiro, J., Bates, D., DebRoy, S., and Sarkar, D.R., (2018). Linear and nonlinear mixed effects models. R package version 3.1-131.1. Available at: http://CRAN.R-project.org/package=nlme (Accessed October 31, 2023).
R Core Team (2022). R: A language and environment for statistical computing. R Foundation for Statistical Computing, Vienna, Austria. Available at: https://www.R-project.org/ (Accessed October 31, 2023).
Rowland, L., da Costa, A. C. L., Galbraith, D. R., Oliveira, R. S., Binks, O. J., Oliveira, A. A. R., et al. (2015). Death from drought in tropical forests is triggered by hydraulics not carbon starvation. Nature 528, 119–122. doi: 10.1038/nature15539
Royer, P. D., Cobb, N. S., Clifford, M. J., Huang, C.-Y., Breshears, D. D., Adams, H. D., et al. (2011). Extreme climatic event-triggered overstorey vegetation loss increases understorey solar input regionally: primary and secondary ecological implications. J. Ecol. 99, 714–723. doi: 10.1111/j.1365-2745.2011.01804.x
Ruiz-Benito, P., Lines, E. R., Gómez-Aparicio, L., Zavala, M. A., and Coomes, D. A. (2013). Patterns and drivers of tree mortality in Iberian forests: climatic effects are modified by competition. PLoS One 8:e56843. doi: 10.1371/journal.pone.0056843
Ruiz-Benito, P., Ratcliffe, S., Zavala, M. A., Martínez-Vilalta, J., Vilà-Cabrera, A., Lloret, F., et al. (2017). Climate- and successional-related changes in functional composition of European forests are strongly driven by tree mortality. Glob. Chang. Biol. 23, 4162–4176. doi: 10.1111/gcb.13728
Salmon, Y., Torres-Ruiz, J. M., Poyatos, R., Martinez-Vilalta, J., Meir, P., Cochard, H., et al. (2015). Balancing the risks of hydraulic failure and carbon starvation: a twig scale analysis in declining scots pine. Plant Cell Environ. 38, 2575–2588. doi: 10.1111/pce.12572
Sánchez-Salguero, R., Navarro-Cerrillo, R. M., Julio Camarero, J., and Fernandez-Cancio, A. (2012). Selective drought-induced decline of pine species in southeastern Spain. Clim. Chang. 113, 767–785. doi: 10.1007/s10584-011-0372-6
Sangüesa-Barreda, G., Linares, J. C., and Camarero, J. J. (2015). Reduced growth sensitivity to climate in bark-beetle infested Aleppo pines: connecting climatic and biotic drivers of forest dieback. For. Ecol. Manag. 357, 126–137. doi: 10.1016/j.foreco.2015.08.017
Schneider, C. A., Rasband, W. S., and Eliceiri, K. W. (2012). NIH image to ImageJ: 25 years of image analysis. Nat. Methods 9, 671–675. doi: 10.1038/nmeth.2089
Seidl, R., Fernandes, P. M., Fonseca, T. F., Gillet, F., Jönsson, A. M., Merganičová, K., et al. (2011). Modelling natural disturbances in forest ecosystems: a review. Ecol. Model. 222, 903–924. doi: 10.1016/j.ecolmodel.2010.09.040
Shestakova, T. A., Gutiérrez, E., Kirdyanov, A. V., Camarero, J. J., Génova, M., Knorre, A. A., et al. (2016). Forests synchronize their growth in contrasting Eurasian regions in response to climate warming. Proc. Natl. Acad. Sci. 113, 662–667. doi: 10.1073/pnas.1514717113
Sumida, A., Miyaura, T., and Torii, H. (2013). Relationships of tree height and diameter at breast height revisited: analyses of stem growth using 20-year data of an even-aged Chamaecyparis obtusa stand. Tree Physiol. 33, 106–118. doi: 10.1093/treephys/tps127
Suarez, M. L., Ghermandi, L., and Kitzberger, T. (2004). Factors predisposing episodic drought-induced tree mortality in Nothofagus– site, climatic sensitivity and growth trends. J. Ecol. 92, 954–966. doi: 10.1111/j.1365-2745.2004.00941.x
Tejedor, E., Serrano-Notivoli, R., de Luis, M., Saz, M. A., Hartl, C., St George, S., et al. (2020). A global perspective on the climate-driven growth synchrony of neighbouring trees. Glob. Ecol. Biogeogr. 29, 1114–1125. doi: 10.1111/geb.13090
Thornthwaite, C. W. (1948). An approach toward a rational classification of climate. Geogr. Rev. 38, 55–94. doi: 10.2307/210739
Vila-Cabrera, A., Martinez-Vilalta, J., Galiano, L., and Retana, J. (2013). Patterns of Forest decline and regeneration across scots pine populations. Ecosystems 16, 323–335. doi: 10.1007/s10021-012-9615-2
Xiong, Y., D’Atri, J. J., Fu, S., Xia, H., and Seastedt, T. R. (2011). Rapid soil organic matter loss from forest dieback in a subalpine coniferous ecosystem. Soil Biol. Biochem. 43, 2450–2456. doi: 10.1016/j.soilbio.2011.08.013
Yamaguchi, D. K. (1991). A simple method for cross-dating increment cores from living trees. Can. J. For. Res. 21, 414–416. doi: 10.1139/x91-053
Keywords: climate change, dendroecology, synchrony, vulnerability, scots pine, tree die-off, climatic water balance
Citation: Herrero A, González-Gascueña R, González-Díaz P, Ruiz-Benito P and Andivia E (2023) Reduced growth sensitivity to water availability as potential indicator of drought-induced tree mortality risk in a Mediterranean Pinus sylvestris L. forest. Front. For. Glob. Change. 6:1249246. doi: 10.3389/ffgc.2023.1249246
Edited by:
Erwin Dreyer, INRA Centre Nancy-Lorraine, FranceReviewed by:
Mathieu Lévesque, ETH Zürich, SwitzerlandMaxime Cailleret, Institut National de recherche pour l’agriculture, l’alimentation et l’environnement (INRAE), France
Copyright © 2023 Herrero, González-Gascueña, González-Díaz, Ruiz-Benito and Andivia. This is an open-access article distributed under the terms of the Creative Commons Attribution License (CC BY). The use, distribution or reproduction in other forums is permitted, provided the original author(s) and the copyright owner(s) are credited and that the original publication in this journal is cited, in accordance with accepted academic practice. No use, distribution or reproduction is permitted which does not comply with these terms.
*Correspondence: Asier Herrero, YXNpZXIuaGVycmVyb0BlaHUuZXVz