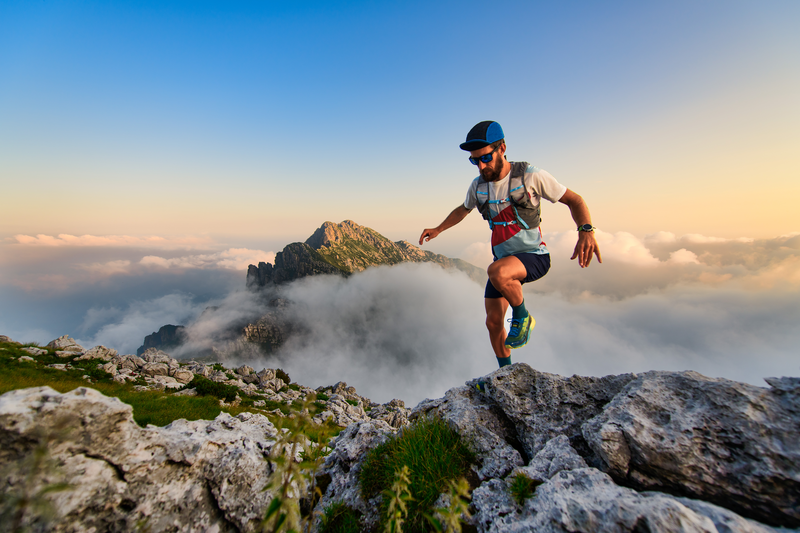
95% of researchers rate our articles as excellent or good
Learn more about the work of our research integrity team to safeguard the quality of each article we publish.
Find out more
ORIGINAL RESEARCH article
Front. For. Glob. Change , 28 September 2023
Sec. Forest Management
Volume 6 - 2023 | https://doi.org/10.3389/ffgc.2023.1246992
Introduction: Clear-cutting an even-aged pure forest is a conventional forest operation for wood production. However, this type of operation is unsuitable for sustainable management with multiple disadvantages. By contrast, mixed forests are a forestation strategy that accommodates diversity. This study aims to assess and compare the timber value and carbon credits of a pure forest and a mixed forest, which is transformed from a pure forest. Two alternative options in managing plantations of pure forest (with Cryptomeria japonica) and mixed forest (with part of C. japonica cut and Cinnamomum camphora replanted) are evaluated considering both timber value and carbon credits. Scenarios with various harvesting intensities and carbon payments were also considered.
Method: A theoretical model was applied, converting pure forest into mixed forest, then two species are cut or replanted in the second round. By contrast, in the pure forest situation, the setting for the second rotation period is a pure forest for 20 years. The model was applied in a simulation experiment and the study area is Taiwan. The selected tree species are representative and have been chosen for analysis.
Results: This study showed that even-aged pure forests had higher wood sales and lower carbon payments than uneven-aged mixed forests. The net present value from market value would be from −255,403 NTD ha−1 to −74,134 NTD ha−1 and that from carbon value will be from 156,076 NTD ha−1 to 208,937 NTD ha−1.
Discussion: This study showed strategies by which values could be increased during the transition from an even-aged pure forest to a mixed forest. Feasible methods included reducing the costs of reforestation, management, and cutting while increasing carbon prices to increase profits from wood and carbon income. A higher harvesting intensity could contribute to greater production and increase the area available for planting, resulting in greater profits from wood and carbon income.
Reforestation brings both direct and indirect benefits involving tangible and intangible values (Ihemezie et al., 2021). The tangible values have quantifiable benefits, such as income from harvested timber. The intangible value associates with ecological benefits, including carbon sequestration, water conservation, land conservation, and protecting wildlife habitats (Jaina et al., 2017). Reforestation also contributes to the stabilization of the microclimatic environment (National Taiwan University Biodiversity Research Center, 2006).
Even-aged stands are normal outcomes of wood production after clear-cutting, as they are usually applied to natural forests or plantations during a rotation period (Bettinger et al., 2016). Re-establishing an even-aged stand is simple and produces wood of similar size. Even-aged management is widespread throughout the world (Germany and Alps, Coppice in south Europe, Canada, United States, etc.). However, cleared land lacks forest protection, and soil erosion easily occurs at the beginning of forest stand renewal (Guo and Yang, 2014; Liu et al., 2018). Wildlife habitats in even-aged pure forests are also destroyed by clear-cutting, causing devastation among animals (Subasinghe et al., 2014; Chaudhary et al., 2016; Dislich et al., 2017). The monoculture of an even-aged pure forest involves low species diversity, which increases its vulnerability to meteorological factors and insect damage (Hartley, 2002; Nyland, 2002; Bowyer, 2006; Carnus et al., 2006; Brockerhoff et al., 2013; Chiu et al., 2014; Moghaddam, 2014). Therefore, most even-aged pure stands do not meet the requirements for sustainable forests (Guo and Yang, 2014). A sustainable forest is a managed ecosystem that balances environmental, economic, and social needs to maintain long-term health and productivity while minimizing negative impacts (Liu et al., 2023). In forest management, numerous strategies can be adopted to reduce the negative effects of large-scale clear-cutting, which is an extensive forestry method that involves completely harvesting vast areas of forest without selective tree removal (Liu and Chuang, 2023). These strategies include gradually removing the upper stories of trees, reducing the area of forest renewal, extending the rotation period, planting forests with more than two species (particularly coniferous and broad-leaved mixed forests), and adopting thinning approaches, which involve selective tree removal to reduce density, allowing remaining trees to grow better. It improves forest health by reducing competition and enhancing growth conditions (Nyland, 2002; Gagnon et al., 2003; Page and Cameron, 2006; Pothier and Marcel, 2008; Lin et al., 2010).
The goal of reforestation has gradually shifted from one of forest production to multiple objectives (O’Hara, 2014; Sharma et al., 2014, 2019), including forest maintenance, biodiversity enhancement, and increasing carbon storage (Pérez-Silos et al., 2021). Various forest-management strategies have been developed to achieve these objectives, including activities like selective harvesting, controlling invasive species, and maintaining ecological balance (Liu et al., 2017, 2021).
Forest maintenance” refers to the ongoing management of a forest to ensure its health and sustainability. It includes activities like selective harvesting, controlling invasive species, and maintaining ecological balance.
Of these strategies, mixed forest management naturally results in biological diversity. Mixed forests are more resistant to various biotic stressors, such as insect damage. They also have greater resistance to abiotic stressors, such as windstorms and droughts (Pardos et al., 2021). Additionally, mixed forests can enhance the esthetic value of landscapes and landscape restoration is the focus of interest in Europe (De Deyn et al., 2004; Jactel et al., 2005; Haas et al., 2011; Lin et al., 2014; Dawud et al., 2016; Coll et al., 2018).
Taiwanese forests are dominated by even-aged pure forests, such as Japanese Cedar (Cryptomeria japonica), Cedar (Cunninghamia lanceolata), Taiwan Acacia (Acacia confuse), Formosan Ash (Fraxinus griffithii), Camphor tree (Cinnamomum camphora), and Taiwan red pine (Pinus taiwanensis) in the early years. Since the 1940s, the reforestation policy has changed to select tree species suitable for the local environment (Chiu, 2010; Ekholm, 2016; Saraev et al., 2019). For example, Michelia compressa, C. camphora, and Calocedrus formosana were selected from northern Taiwan. The selection of reforestation tree species is mostly determined by the natural conditions, such as the growth rate. In addition, the selection of reforestation tree species also needs to consider the economic value of retrievable timber and non-timber products. Additionally, different tree species should be selected to mitigate the insect damage caused by single-species reforestation. Therefore, the five most common species of coniferous trees and similarly of broad-leaved trees in Taiwan were selected. However, as previously described, the specific species for reforestation should be selected according to the environmental conditions (National Taiwan University Biodiversity Research Center, 2006; Chiu, 2010; Ekholm, 2016; Saraev et al., 2019).
Previous studies focused on the transformation of even-aged pure forests into mixed forests in Taiwan (Lin et al., 2010; Chiu et al., 2014). For example, Lin et al. (2010) conducted a study and recommended that coniferous and broad-leaved trees are planted in the Chilanshan area after row thinning of the C. japonica pure forest. This strategy increased economic value and maintained income between the long rotation periods of high-priced cypress stands. Chiu et al. (2014) conducted an experiment using four thinning levels in a C. formosana plantation. This study proposed that if the underwood of the stand is well cultivated and appropriate re-thinning is applied to the upper story of C. formosana, then the growth and survival of the trees under the forest may be enhanced. This strategy also enhanced the carbon sequestration efficiency of C. formosana. Harvesting intensity and carbon payment in their economic analysis were conducted in Nölte et al. (2018), but it is unclear if the findings are consistent with those in Taiwan.
The aim of this study was to estimate the land expectation value of logging an even-aged pure forest replanted with native broadleaved tree species at a fixed harvesting intensity. This study also aims to analyze the land expectation value of cutting at different cutting times and with different harvesting intensities. Carbon payments, in addition to wood sales, were considered as the income of the forest owner. Costs associated with reforestation, management, and logging were included in the model. This study also analyzed critical variables such as logging time, harvesting intensity, and carbon price. Specifically, the effects of these variables on the land expectation value for transforming an even-aged pure forest into uneven-aged mixed forest were determined.
This study assesses an operation model that transforms from an even-aged pure forest into a mixed forest in Taiwan. Analysis in which an even-aged pure forest of C. japonica was cut under a fixed harvesting intensity and replanted with the native broad-leaved tree species C. camphora were conducted. The primary income for the forest owner was derived from wood sales and carbon payments. After deducting the costs of afforestation, management, and cutting, the land expectation value was estimated. The effects of critical variables, such as cutting time, harvesting intensity, and carbon price, were analyzed.
This study mainly focuses on a changed forest phase. The model was applied in a simulation experiment. Cryptomeria japonica are cut and C. camphora are representative so they were selected for analysis. In the model of converting pure forest into mixed forest, part of the C. japonica are cut and C. camphora are then replanted to achieve mixed forests in the second round. By contrast, in the pure forest situation, the setting for the second rotation period is “making it grow to a pure forest for 20 years” to ensure that a pure forest can be formed. The second timber income is not included before the next cutting is conducted. In the present study, an even-aged pure forest (aged T0) was set as the plantation forest. After T1, the forest age was T0 + T1 and was ready for clear-cutting. The costs incurred during T1 were due to its management and clear-cutting. The income sources during T1 were carbon payments from carbon sequestration and sales of harvested wood products (HWP). After clear-cutting, the same tree species was reforested until T2 year. The resulting planted forest was an even-aged, pure forest, aged T2 – T1. The costs incurred during (T2 – T1) years are from reforestation and its management. Sources of income are carbon payments from carbon sequestration during (T2 – T1) years. For a specific area, the land expected value (LEV) of an even-aged pure forest was evaluated as follows:
where A is the total area of the planted forest (ha); is the income from HWP at time point T, in which p is the per-unit wood price (NTD/m3) and is the per-unit volume of wood at T (m3/ha); is the total income from carbon payment, in which g is the per-unit carbon payment (NTD/ton CO2, 1 USD = 31.91 NTD on August 13, 2023) and is the amount of carbon dioxide sequestration per unit volume of wood at T (ton CO2/ha); is the total cost of reforestation and management for the forestland of total area A (NTD); is the logging cost for the total land area at T, in which l is the per-unit logging cost (m3/NTD); r is the discount rate that reflects the future values of income and costs to the present time.
In this study, the planted forest (aged T0 years) was a coniferous even-aged pure forest in the process of growing to a forest age of T0 + T1, at which point it may be cut after native broad-leaved species are replanted. During T1, the costs included its management and cutting expenses, and the sources of income were carbon payments and HWP sales from the coniferous forest.
After cutting, the forest was replanted and transformed into an uneven-aged coniferous and broad-leaved mixed forest, and the forestry operation continued for T2 – T1 years. The forest age of the planted native broadleaved trees was T2 – T1. In T2, the cutting and replanting were repeated. The costs during T2 – T1 included the reforestation and management of the replanted native broad-leaved forest, management of the remaining coniferous forest in T1; and cutting, reforestation, and replanting of the original broad-leaved forest in T2. Income was from carbon payments from mixed-forest carbon sequestration during T2 – T1 and from the sales of HWP from cutting the remaining coniferous population in T2. If the area of forestland is fixed, then the LEV from transforming the even-aged pure forest into a mixed forest is as follows:
where a is the cutting intensity (%); c1(T) and c2(T) are the amounts of carbon dioxide sequestration per unit volume of wood (ton CO2/ha) for the coniferous and native broad-leaved forests at T, respectively; G1 and G2 are the total carbon payment amounts for the coniferous and primitive broad-leaved forests, respectively; F1 and F2 are the reforestation and management costs for the coniferous and primitive broad-leaved forests, respectively.
Cryptomeria japonica is an economically important tree species in Taiwan. Its planted forest is distributed over 1,000–2,000 m above the sea level. The Fourth Forest Resources Survey Report (Forestry Bureau, Council of Agriculture, Executive Yuan, 2022a,b,c) indicated that C. japonica was distributed across a total area of approximately 41,390 ha, and the forest stock per unit area of planted C. japonica was 388.89 m3, which was the highest among planted coniferous forests in Taiwan. According to Forestry Statistics (Forestry Bureau, Council of Agriculture, Executive Yuan, 2022a,b,c), the yearly production of C. japonica timber was 9,252.39 m3, which was the highest among conifer species in Taiwan. It is noted that the forest stock per unit area and timber production are related to age.
C. camphora is a commercial tree species native to Taiwan. It is widely distributed across areas below an altitude of 1,200 m a.s.l. in northern Taiwan and below 1,800 m a.s.l. in southern Taiwan. The optimal growth altitude for this species is <1,500 m a.s.l. (Feng and Lee, 2009). Lin et al. (2016) reported that the Forestry Bureau personnel responsible for reforestation recommend both C. japonica and C. camphora for reforestation. Specifically, C. camphora is recommended because it has a high survival rate, can be planted in various environments, and has various applications. Cinnamomum camphora can thrive in diverse growth environments, including areas with varying levels of sunlight, well-drained soils, and different climatic conditions. Guo (2013) showed that C. camphora can tolerate limited light conditions in forests. Cinnamomum camphora has applications in timber, essential oil production, camphor extraction, ornamental planting, traditional medicine, insect repellents, wood carving, cultural rituals, agricultural practices, and landscape planting.
In this study of the transformation of an even-aged pure forest to a mixed forest, the replanting of trees at the lowest layers of the forest was assumed to enable the survival of trees in the canopy gaps of the cut coniferous forest. The species populating the planted coniferous forest in this study was C. japonica, and that in the native broad-leaved forest was C. camphora.
In this study, the plantation forest was set as a mature forest and the forest age was set at T0 = 20. According to the recommendations for reforestation tree species and the corresponding rotation periods from the Handbook of the Nationwide Reforestation Program (Forestry Bureau, Council of Agriculture, Executive Yuan, 1998), the rotation period of C. camphora was 30 years and that of C. japonica was 20 years. Considering that a few studies have proposed a 30-year rotation period for C. japonica (National Taiwan University Biodiversity Research Center, 2006), T1 was set to 10 years. In the LEV model of the even-aged pure forest, the planted forest land was set to grow into an even-aged pure forest for 20 years. In the LEV model for transforming the even-aged pure forest into a mixed forest, T2 was set to 30 years for the formation of the uneven-aged mixed broad-leaved forest. In this study, no specific silvicultural treatment was assumed. A typical rate of cutting of 30% is selected since only part of the forest can be cut.
This study also analyzed and compared the results for various time points of cutting C. japonica and then replanting C. camphora. Specifically, the results for cutting in the 10th, 15th, and 20th years under 30% harvesting intensity were calculated for all scenarios with cutting and replanting repeated in the 30th year. The forest owner obtained wood income from two cuttings of C. japonica. In addition, the forest owner obtained annual carbon payments from cutting at the initial time point up to various cutting times and during the growth period of the mixed forest for a total of 30 years of carbon payments.
Based on the C. japonica growth pattern proposed by Chang et al. (1987), the volume (of stem and branches) of C. japonica was estimated using the following formula:
(unit: m3 ha−1).
where T refers to forest age. Based on the growth pattern of C. camphora proposed by Lin et al. (2002), the estimated volume of C. camphora is given by the following formula:
(unit: m3 ha−1).
The total volume growth curves of C. japonica and C. camphora were obtained by calculating the total volume at each forest age based on their growth patterns, as shown in Figure 1. The volume in mixed stands is calculated by the product of volume, area at that age, and percentage of the species per area. We also assume the rate of growth rate in mixed stands is the same of that in pure ones.
Figure 1. Growth curve of the total volumes of Cinnamomum camphora (solid line) and Cryptomeria japonica (dashed line).
Based on current wood use in Taiwan, the harvested wood was sorted into categories of wood products to determine the HWP sale value. According to the Food and Agriculture Organization of the United Nations (FAO), HWP categories include roundwood, sawnwood, wood-based panels, pulpwood, and wood chips (FAO, 2020). According to Forestry Bureau, Council of Agriculture, Executive Yuan (2023), the demand for various wood materials was roundwood logs = 13%, sawnwood = 23%, wood-based panels = 27%, and pulpwood and wood chips = 37%. The selected species are able to produce these assortments in this proportion in Taiwan and these proportions were used for the breakdown of the HWP in this study.
To determine the price of HWP, this study calculated the average prices of roundwood, panels, and slabs of C. japonica for the past 10 years (Forestry Bureau, Council of Agriculture, Executive Yuan, 2022a,b,c), which were 4,341, 10,623, and 5,756 NTD/m3, respectively. The price of pulpwood and wood chips was set at 1,619 NTD/m3 based on the average price of debarked branches over the past 10 years (Forestry Bureau, Council of Agriculture, Executive Yuan, 2022c). According to the Wood Price Information System of the Forestry Bureau, the average price of C. camphora roundwood over the past decade has been 4,341 NTD/m3.
Under the operational scenario in the present study, reforestation was conducted after clear-cutting the even-aged pure forest. To transform an even-aged pure forest into a mixed forest, native broad-leaved trees were replanted after cutting a coniferous forest. It is noted that the clear-cut is not over the whole forest area, but only in portions or with partial canopy uncovering through thinnings. The cost of reforestation and management should also be considered. In a study by Liu et al. (2009), the cost of reforestation was reduced based on the costs of raising seedlings, outplanting, and weeding. Under the current regulations, the costs of raising seedlings can be estimated. For example, the forest owner must pay 30,000 NTD/ha in the first year of outplanting if certain conditions are met. Regarding outplanting and weeding, there are guidelines on the required manpower and wages according to the Forestry Bureau, Council of Agriculture, Executive Yuan (2002, 2022a,b,c). For example, a 1-year-old planted forest land should be weeded twice a year; each round of weeding requires eight labor days per hectare, and weeding workers need to pay 1,500 NTD each day.
This study examined the operational scenario of clear-cutting an even-aged purely planted forest. An even-aged, pure-planted forest must be clear-cut to facilitate its transformation into a mixed forest. Zheng and Shih (2006) applied a regression analysis to the cost of forest cutting and operational data of 46 leased national forestlands under the management of the Nantou Forest District Office. The average cutting cost per cubic meter of leased national forestland was 1,493 NTD/m3.
Increased greenhouse gas (GHG) emissions are the primary cause of climate change. Forests can absorb and store carbon dioxide, and this forest-based process is crucial for reducing greenhouse gas emissions. This study incorporated carbon payments into the operation scenarios for even-aged pure forests and for transforming them from even-aged pure forests to mixed forests. The effect of transforming the forest tree species composition on carbon sequestration benefits was analyzed. The carbon dioxide storage transformation formula proposed by the Intergovernmental Panel on Climate Change (IPCC) of the United Nations (Eggleston et al., 2006 and its refinement Shukla et al., 2019) is given by
where B is the biomass per hectare (ton/ha), V is the wood volume per hectare (m3/ha), VT is the transformation coefficient between the whole-tree volume and dry wood volume, WT is the transformation coefficient of weight and volume, is the carbon dioxide storage amount per hectare (ton CO2/ha), CT is the transformation coefficient for carbon content, and CO2/C is the transformation coefficient for carbon dioxide and carbon.
Table 1 presents the key parameters for carbon dioxide storage in C. japonica and C. camphora. Wood volume was transformed into the whole-tree volume, and the specific weight of wood was multiplied to obtain the forest biomass. Subsequently, forest biomass was multiplied by the transformation coefficients of carbon and carbon dioxide (3.67) to determine the amount of carbon dioxide that could be stored. The CT values of C. japonica and C. camphora are 0.4974 and 0.47, respectively. Figure 2 presents the carbon dioxide storage curves for C. japonica and C. camphora.
Table 1. Key parameters for the carbon dioxide storage (Lin et al., 2002).
Based on the Greenhouse Gas Reduction and Management Act of the Environmental Protection Administration, Executive Yuan (2022), this study assumed the carbon payment for carbon dioxide per ton to be 1,500 NTD. This amount is actually paid for plantations that are then cut and timber used.
This study used the LEV model to analyze operational scenarios for an even-aged pure forest and the transformation from an even-aged pure forest to a mixed forest. To analyze and compare each scenario at the same time point, the future income, and costs for a 20-year-old planted forest land were considered. The current preferential interest rate for reforestation loans (1.25%) was used as the discount rate in this study (Bureau of Agricultural Finance, Council of Agriculture, Executive Yuan, 2022).
In the case of converting an even-aged pure forest into a mixed forest, this simulation study gradually removes C. japonica, narrows the area of forest renewal, extends the rotation period, and builds a mixed forest of two species to reduce the negative impact caused by large-scale clear cutting. The scenario for an even-aged pure forest is shown in Figure 3. The scenario of transformation from an even-aged pure forest to a mixed forest is shown in Figure 4.
This study calculated the per-unit LEV model for the 20-year-old even-aged pure forests of C. japonica and C. camphora. The initial forest age was set at 20. Clear cutting and reforestation using the same tree species were implemented when the forest reached the age of 30, and the forest grew into an even-aged pure forest of age 20. The forest owner earned wood income when the forest was 30 years old. The forest owner obtained a total worth of 30 years in carbon payments, specifically for the years of forest age 20–30 and 20 years of reforestation. The individual net present value of income from unit wood, net present value per-unit carbon payment, and per-unit LEVs of the two pure forests are presented in Table 2. As can be seen, the unit LEV from planting C. camphora in the even-aged pure forest was 293,249 NTD higher than that from planting C. japonica. Specifically, the net present value of the unit wood income of C. camphora was 93,064 NTD higher than that of C. japonica, and the net present value of the per-unit carbon payment for C. camphora was 200,185 NTD higher than that of C. japonica.
The initial forest age was set to 20. The processes of cutting at 30% harvesting intensity and replanting of C. camphora were conducted in the 30th year to transform the C. japonica forest into a mixed forest. The mixed forest continued to grow for another 20 years, after which cutting and replanting procedures were repeated. The forest owner obtained wood income by cutting the C. japonica forest when C. japonica was 30 years old and when the mixed forest was 20 years old. The forest owner obtained carbon payments for the years when the C. japonica forest was aged 20–30 years, and for the 20 years of mixed forest growth, for a total worth of 30 years in carbon payments. Table 3 presents the results for the per-unit LEV in the basic scenario of transforming an even-aged pure forest into a mixed forest. The net present value of per-unit wood income in the basic scenario was calculated to be −124,259 NTD, the net present value of per-unit carbon payment was 191,317 NTD, and the per-unit LEV was 67,057 NTD.
The results for this scenario are presented in Table 4. The net present value of per-unit wood income, the net present value of per-unit carbon payments, and the per-unit LEV were lower at later cutting times. These later cutting times corresponded to increased reforestation and management costs of C. japonica during the cutting period. Moreover, the growth of C. japonica increased the cost of cutting; thus, the net present value of per-unit wood income decreased from −124,259 to −125,645 when the cutting time was delayed. The results for the even-aged pure forest indicated that C. camphora stored a greater amount of carbon dioxide than did C. japonica; thus, the carbon payment for C. camphora was higher. However, delayed cutting time reduced the growth time of the replanted C. camphora, and the carbon payment was slightly lower. Specifically, the net present value of per-unit carbon payments decreased from 191,317 NTD to 163,985 NTD. Therefore, the per-unit LEV decreased from 67,057 to 38,341 NTD when the cutting time was delayed.
In this study, we analyzed and compared LEVs harvested at various intensities. The initial C. japonica forest age was set at 20. This scenario involved the transformation of a pure forest of the same age into a mixed forest. Three harvesting intensities were analyzed and compared. Specifically, the results were examined for replanting with C. camphora after cutting at harvesting intensities of 10, 20, and 40%, where cutting and replanting were repeated in the 30th year for all cases. Based on the harvesting intensity, the forest owner obtained wood income when the C. japonica forest was 30 years old and when the mixed forest was 20 years old. Additionally, the owner obtained annual carbon payments for the C. japonica forest at forest ages 20–30 and for 20 years of mixed forest growth, for a total of 30 years of carbon payments.
Table 5 shows the results of cutting C. japonica and replanting with C. camphora under the aforementioned conditions. The net present value of per-unit wood income, net present value of per-unit carbon payment, and per-unit LEVs all increased with an increase in harvesting intensity. Increases in cutting intensity resulted in increased costs of cutting C. japonica and replanting with C. camphora, but increased cutting intensity also corresponded with greater income from greater sales of C. camphora HWP. Specifically, the net present value of per-unit wood income increased from −255,403 NTD to −74,134 NTD. The area of replanted C. camphora increased with increasing harvesting intensity, contributing to an increase in the net present value per unit carbon payment from 156,076 NTD to 208,937 NTD. Therefore, the per-unit LEV increased from −99,327 to 134,804 NTD with an increase in the harvesting intensity.
This study analyzed and compared the LEVs at various carbon prices. Carbon payments were calculated according to the Greenhouse Gas Reduction and Management Act of the Environmental Protection Administration, Executive Yuan (2022). Specifically, the carbon payment was set at 1,500 NTD per ton of carbon dioxide. The initial C. japonica forest age was set at 20. When the forest reached the age of 30 years, it was cut at 30% harvesting intensity, and replanting was conducted using C. camphora. Thus, a pure forest was transformed into a mixed forest. The operation continued for 20 years, after which the cutting and replanting procedures were repeated. The forest owner obtained wood income from C. japonica when it was 30 years old, and the mixed forest was 20 years old. Under various carbon prices, the income of the forest owner was the sum of the carbon payments for C. japonica at the forest age of 20–30 years and the carbon payments received during 20 years of mixed forest growth. Thus, 30 years of annual carbon payments from the production of C. japonica and C. camphora were received.
Table 6 presents the results of the study. The net present value of the per-unit carbon payment and per-unit LEV increased with an increase in carbon payment. The net present value per unit wood income was fixed at −124,259 NTD. Specifically, the net present value of per-unit carbon payments increased from 64,680 NTD to 637,723 NTD. Accordingly, the per-unit LEV gradually increased from −59,579 to 513,464 NTD.
This study explored an operational model for transforming an even-aged pure forest into a mixed forest. Analysis and comparison of the operation models of the even-aged pure forest indicated that the LEV of planted C. camphora was higher than that of planted C. japonica. Therefore, although the cost of cutting C. camphora was higher, the wood income from C. camphora was still higher than that from C. japonica. The amount of carbon dioxide stored in the growth model of C. camphora was also higher than that of C. japonica, indicating that the carbon payment from planting C. camphora was higher than that of C. japonica.
Although the average unit price for C. japonica HWP (5,161 NTD) was higher than that for C. camphora (4,023 NTD), the growth curves of C. japonica and C. camphora (Figure 1) illustrated that the unit stock of C. camphora was higher than that of C. japonica. Although planting C. camphora in the even-aged pure forest involved higher cutting-related costs, the income from C. camphora wood was higher than that from C. japonica wood. According to the curves of carbon dioxide storage for C. japonica and C. camphora (see Figure 2), the unit stock of C. camphora was higher than that of C. japonica. Carbon dioxide storage was calculated based on the growth of C. camphora, and the results indicated that the amount of carbon dioxide stored was higher than that stored in C. japonica. Additionally, during the 30 years of forest growth, the slope of the carbon dioxide storage curve for C. camphora was steeper than that for C. japonica. Thus, the carbon payment was higher in the model of planting C. camphora in the even-aged pure forest. Accordingly, the per-unit LEV of the operational model for planting C. camphora in the even-aged pure forest was higher than that for planting C. japonica.
An even-aged pure forest was transformed into a mixed forest in the 10th year and replanted with C. camphora after C. japonica was cut at 30% harvesting intensity. Growth into an unevenly aged coniferous, broad-leaved mixed forest continued until the 30th year, when the same cutting procedure was repeated, yielding a per-unit LEV of 67,057 NTD. Later cutting times increased the costs of reforestation, management, and cutting of C. japonica. After replanting with C. camphora, carbon payments decreased; thus, the per-unit LEV decreased. An increase in harvesting intensity resulted in higher income from selling the HWP of C. japonica as well as an increased area replanted with C. camphora. Carbon payments increased after cutting C. japonica and replanting it with C. camphora, resulting in an increase in per-unit LEV. The result was different from the study conducted by Nölte et al. (2018), which concluded that the carbon payments and LEV increased as the harvesting intensity decreased. It is speculated that because Taiwan is a subtropical region, trees grow faster and thinning actually leads to an increase in growth volume.
The operation model of the even-aged pure forest of C. camphora maximized the net present value of wood income, which was 97,697 NTD ha−1. For the model transforming an even-aged pure forest into a mixed forest, wood income was not derived from cutting C. japonica at 30% harvesting intensity. A total of 51% of the C. japonica forest was replanted with C. camphora. After deducting related expenses such as reforestation and cutting, the net present value of per unit wood income was only −124,259 NTD ha−1.
The even-aged pure forest of C. camphora exhibited the highest net present value of per-unit carbon payment because the unit stock of C. camphora was high, and thus correlated with a relatively high amount of carbon dioxide storage. Specifically, the per-unit carbon payment was 374,062 NTD ha−1. To transform an even-aged pure forest into a mixed forest, C. japonica was cut and replanted with C. camphora, which exhibited greater carbon sequestration efficiency. These measures increased the income from carbon payments for the original forestland. The net present value of the per-unit carbon payment was 191,317 NTD ha−1, which was 17,440 NTD ha−1 higher than that of the even-aged pure forest of C. japonica. In particular, the even-aged pure forest of C. camphora exhibited the highest LEV (471, 759 NTD ha−1). The net present values of per unit wood income and per unit carbon payments for the even-aged pure forest of C. camphora were much higher than those for the mixed forest transformed from an even-aged pure forest.
With respect to harvesting intensity, a greater harvesting intensity resulted in higher production for the C. japonica forest, as well as a larger area for planting C. camphora, in turn increasing income from wood and carbon payments. A greater harvesting intensity substantially affects forest ecology. Forestry strategies that focus only on increasing the output value of the forestland neglect the initial purpose of transforming an even-aged pure forest into a mixed forest.
In this study, C. japonica products were classified as roundwood/logs, sawnwood, wood-based panels, pulpwood, and wood chips, and prices were assigned based on the HPW classifications defined by the FAO, the results of Chen et al. (2012), the Wood Price Information System (Forestry Bureau, Council of Agriculture, Executive Yuan, 2022a,b,c), and the prices in the forestry statistics (Forestry Bureau, Council of Agriculture, Executive Yuan, 2022a,b,c). However, for C. camphora, only the log price was published in the wood market price information system, and forestry statistics (Forestry Bureau, Council of Agriculture, Executive Yuan, 2022a,b,c) did not provide species-specific production details. Therefore, the price setting for C. camphora products could be improved in subsequent studies. Should the government update the information system regarding the market prices of wood products such updated information may benefit decision–making in the forestry sector and serve as a reference for academic research and inquiries.
The simulation results of this study explore how to enhance the value of transforming even-aged pure forests into mixed forests. This can be achieved by reducing costs (for reforestation, management, and nurturing) and increasing carbon prices. Increasing the harvesting intensity can enhance the amount of C. japonica cutting and the area for planting C. camphora, thereby increasing income from timber and carbon payment. The study area is Taiwan, but the methodology can be applied to other areas with different tree species. Our model considers the transition of a mixed forest from a pure forest, incorporating dynamic benefits to align with the current situation. It is noted that an increased harvesting intensity could also inflict severe impacts on forest ecology. If only focused on elevating land productivity, the original intent of altering the even-aged pure forest and creating mixed forests could be lost.
To incentivize forest owners to invest in mixed forests, it is suggested the government establish a reliable carbon trading system and increase the standards and penalties of the Greenhouse Gas Reduction and Management Act. In addition to researching and developing green technologies and strategies for pollution prevention, the government should consider adopting forestry operations as the primary method of reducing carbon emissions. Forest owners can provide carbon storage, which can be sold to meet enterprise and factory demands for carbon emission reduction. These transactions could form a carbon trading market that would increase the incomes of forest owners and enable them to invest in mixed forests. This may encourage enterprises to invest in planting forests, thereby increasing forest coverage and the associated effects of carbon sinks in Taiwan.
In actual situations, the growth rate in mixed stands may not be the same of that in pure ones (Pretzsch et al., 2019). The values may also fluctuate with the prices of raw materials and wages, and forest growth would affect the costs of reforestation, management, and cutting. Additionally, only a 30-year operation period was considered to transform an even-aged pure forest into a mixed forest. In future research, an analysis based on other periods can be conducted to enhance the comprehensiveness of the operational model for a mixed forest. Finally, this study did not consider the proportion of carbon emissions in the scenarios or the use of HWPs. Cutting and wood use affect the carbon emissions from forests. Future studies should consider carbon emissions when determining the actual value of carbon payments.
The original contributions presented in the study are included in the article/supplementary material, further inquiries can be directed to the corresponding author.
Y-RL analyzed the data and drafted the manuscript. W-YL contributed to the investigation, data analysis, the results, conclusion, and as the corresponding author on their behalf throughout the review, editing, and submission process. All authors contributed to the article and approved the submitted version.
This work has been supported in part by the National Science and Technology Council, Taiwan, under Grants NSTC 112-2321-B-005-007 and NSTC 112-2628-H-005-003; and the Ministry of Education, Taiwan, under the Higher Education Sprout Project.
The authors declare that the research was conducted in the absence of any commercial or financial relationships that could be construed as a potential conflict of interest.
All claims expressed in this article are solely those of the authors and do not necessarily represent those of their affiliated organizations, or those of the publisher, the editors and the reviewers. Any product that may be evaluated in this article, or claim that may be made by its manufacturer, is not guaranteed or endorsed by the publisher.
Bettinger, P., Boston, K., Siry, J., and Grebner, D. L. (2016). Forest management and planning. United States: Academic press.
Bowyer, J. L. (2006). Forest plantations threatening or saving natural forests? Arborvitae (The IUCN/WWF Forest Conservation Newsletter) 31, 8–9. doi: 10.1088/1748-9326/abca64
Brockerhoff, E. G., Jactel, H., Parrotta, J. A., and Ferraz, S. F. B. (2013). Role of eucalypt and other planted forests in biodiversity conservation and the provision of biodiversity-related ecosystem services. For. Ecol. Manag. 301, 43–50. doi: 10.1016/j.foreco.2012.09.018
Bureau of Agricultural Finance, Council of Agriculture, Executive Yuan (2022) Regulations on afforestation loan, agricultural project loans. Available at: https://goo.gl/HkZW6u/ (Accessed April 1, 2022).
Carnus, J. M., Parrotta, J., Brockerhoff, E., Arbez, M., Jactel, H., Kremer, A., et al. (2006). Planted forests and biodiversity. J. For. 104, 65–77. doi: 10.1093/jof/104.2.65
Chang, J. S., Chen, L. C., and Jen, I. A. (1987). An analysis of the growth and yield of man-made forest in Taiwan. Bulletin Taiwan Forestry Res. Institute 2, 17–29.
Chaudhary, A., Burivalova, Z., Koh, L. P., and Hellweg, S. (2016). Impact of forest management on species richness: global meta-analysis and economic trade-offs. Sci. Rep. 6, 1–10. doi: 10.1038/srep23954
Chen, L. C., Lin, J. C., Wu, C. S., Huang, G. M., and Chen, Y.-H. (2012). The current status of the wood product demand in Taiwan. Quarterly J. Forest Res. 34, 287–296. doi: 10.29898/SHBQ.201212.0004
Chiu, C. M. (2010). New thinking on Taiwan plantation foreset management-learning from nature and close to natural forest management. Forestry Res. Newsletter 17, 7–12.
Chiu, C. M., Su, S. H., Tang, S. L., and Fuh, C. H. (2014). Assessing the benefits of thinning and underplanting of broadleaved trees in a Taiwan incense-cedar plantation. Quarterly J. Chinese Forestry 47, 137–154.
Coll, L., Ameztegui, A., Collet, C., Löf, M., Mason, B., Pach, M., et al. (2018). Knowledge gaps about mixed forests: what do european forest managers want to know and what answers can science provide? For. Ecol. Manag. 407, 106–115. doi: 10.1016/j.foreco.2017.10.055
Dawud, S. M., Raulund-Rasmussen, K., Domisch, T., Finér, L., Jaroszewicz, B., and Vesterdal, L. (2016). Is tree species diversity or species identity the more important driver of soil carbon stocks, C/N ratio, and pH? Ecosystems 19, 645–660. doi: 10.1007/s10021-016-9958-1
De Deyn, G. B., Raaijmakers, C. E., van Ruijven, J., Berendse, F., and van der Putten, W. H. (2004). Plant species identity and diversity effects on different trophic levels of nematodes in the soil food web. Oikos 106, 576–586. doi: 10.1111/j.0030-1299.2004.13265.x
Dislich, C., Keyel, A. C., Salecker, J., Kisel, Y., Meyer, K. M., Auliya, M., et al. (2017). A review of the ecosystem functions in oil palm plantations, using forests as a reference system. Biol. Rev. Camb. Philos. Soc. 92, 1539–1569. doi: 10.1111/brv.12295
Eggleston, H. S., Buendia, L., Miwa, K., Ngara, T., and Tanabe, K. (eds). (2006). 2006 IPCC guidelines for national greenhouse gas inventories. Japan: National Greenhouse Gas Inventories Programme, Institute for Global Environmental Strategies (IGES).
Ekholm, T. (2016). Optimal forest rotation age under efficient climate change mitigation. Forest Policy Econ. 62, 62–68. doi: 10.1016/j.forpol.2015.10.007
Environmental Protection Administration, Executive Yuan. (2022). Greenhouse gas reduction and management act. Available at: https://goo.gl/Gaijp3/ (Accessed April 1, 2022).
EU ETS Carbon Pulse. (2022). EU ETS carbon pulse. Available at: https://goo.gl/SJGuQ4/ (Accessed April 1, 2022).
FAO. (2020). Yearbook of Forest products 2018. Rome, Italy: Food and Agriculture Organization of the United Nations (FAO). 358pp.
Feng, F.-L., and Lee, H.-T. (2009). The status and perspectives of camphor tree in Taiwan. Biological Sci. 51, 37–51. doi: 10.29981/CB.200912.0004
Forestry Bureau, Council of Agriculture, Executive Yuan. (1998). Handbook of the Nationwide reforestation program. Taipei, Taiwan: Forestry Bureau, Council of Agriculture, Executive Yuan.
Forestry Bureau, Council of Agriculture, Executive Yuan. (2002). Forest rule no.0910000477, online inquiry system-forestry Bureau, Council of Agriculture, Executive Yuan. Available at: https://goo.gl/kX5Jg8/ (Accessed April 1, 2022).
Forestry Bureau, Council of Agriculture, Executive Yuan. (2022a). The 4th Forest resources survey report. Available at: https://goo.gl/75Xpd5/ (Accessed April 1, 2022).
Forestry Bureau, Council of Agriculture, Executive Yuan. (2022b). Forestry statistics. Available at: https://goo.gl/4LH2M6/ (Accessed April 1, 2022).
Forestry Bureau, Council of Agriculture, Executive Yuan. (2022c). Wood Price information system. Available at: https://goo.gl/L1TRgU/ (Accessed April 1, 2022).
Forestry Bureau, Council of Agriculture, Executive Yuan. (2023). Introduction of Taiwan wood. Available at: https://www.forest.gov.tw/taiwanwood (Accessed August 13, 2023)
Gagnon, J. L., Jokela, E. J., Moser, W. K., and Huber, D. A. (2003). Dynamic of artificial regeneration gaps within a longleaf pine Flatwoods ecosystem. For. Ecol. Manag. 172, 133–144. doi: 10.1016/S0378-1127(01)00808-8
Guo, Y.-L. (2013). Shade-tolerance of plants and the category of native shade-tolerant tree species in Taiwan. Forestry Res. Newsletter 20, 36–40.
Guo, X. R., and Yang, Z. C. (2014). Genetic characteristics of different shade-tolerant tree species and their application in forest regeneration. Taiwan Forestry J. 40, 40–49.
Haas, S. E., Hooten, M. B., Rizzo, D. M., and Meentemeyer, R. K. (2011). Forest species diversity reduces disease risk in a generalist plant pathogen invasion. Ecol. Lett. 14, 1108–1116. doi: 10.1111/j.1461-0248.2011.01679.x
Hartley, M. J. (2002). Rationale and methods for conserving biodiversity in plantation forests. For. Ecol. Manag. 155, 81–95. doi: 10.1016/S0378-1127(01)00549-7
Ihemezie, E. J., Nawrath, M., Strauß, L., Stringer, L. C., and Dallimer, M. (2021). The influence of human values on attitudes and behaviours towards forest conservation. J. Environ. Manag. 292:112857. doi: 10.1016/j.jenvman.2021.112857
Jactel, H., Brockerhoff, E. G., and Duelli, P. (2005). “A test of the biodiversity-stability theory: meta-analysis of tree species diversity effects in insect pest infestations, and re-examination of responsible factors” in Forest diversity and function: Temperate and boreal systems. Ecological studies. eds. M. Scherer Lorenzen, C. Korner, and E.-D. Schulze, vol. 176 (Berlin, Germany: Springer), 235–261.
Jaina, A., Chandrab, G., and Nautiyal, R. (2017). Valuating intangible benefits from afforested areas: a case study in India. Economía agraria y recursos naturales 17, 89–100. doi: 10.7201/earn.2017.01.04
Lin, J.-C., Cheng, M.-J., Liu, S.-F., and Lee, K.-C. (2002). Economic benefit evaluation of the potential CO2 sequestration by the national reforestation program. Taiwan J. Forest Sci. 17, 311–321. doi: 10.7075/TJFS.200209.0311
Lin, C.-Y., Chiu, C.-M., Lin, S.-T., Chung, C.-H., and Lin, C.-L. (2010). Regeneration development after row thinning in Cryptomeria Japonica plantations at chi-Lan-Shan area. Quarterly J. Chinese Forestry 43, 233–247.
Lin, J.-C., Hsu, Y.-R., Chan, W.-H., and Wang, P.-J. (2016). Survey and analysis of tree species used in National Leasehold Forest Afforestation. Quarterly J. Chinese Forestry 49, 261–278.
Lin, J.-C., Liu, I.-H., and Tang, S.-C. (2014). The growth performances and carbon stock changes of broadleaf mixed stands. Quarterly J. Forest Res. 36, 57–66.
Liu, W.-Y., and Chuang, Y.-L. (2023). Assessing the incentives and financial compensation of agroforestry considering the uncertainty of price and yield. Ecol. Indic. 146:109753. doi: 10.1016/j.ecolind.2022.109753
Liu, C. L. C., Kuchma, O., and Krutovsky, K. V. (2018). Mixed-species versus monocultures in plantation forestry: development, benefits, ecosystem services and perspectives for the future. Global Ecol. Conser. 15, e00419–e00413. doi: 10.1016/j.gecco.2018.e00419
Liu, W. -Y., Lin, C.-C., and Su, K.-H. (2017). Modelling the spatial forest-thinning planning problem considering carbon sequestration and emissions. Forest Policy Econ. 78, 51–66. doi: 10.1016/j.forpol.2017.01.002
Liu, W.-Y., Lu, Y.-M., and Lin, K.-C. (2009). Measuring the optimal rotation period under carbon price and timber price uncertainty. Taiwanese Agri. Econ. Rev. 15, 1–35. doi: 10.6196/TAER.2009.15.1.4
Liu, W.-Y., Lu, Y.-H., and Lin, C.-C. (2023). Assessing carbon abatement costs considering forest carbon sequestration and carbon offset mechanism: evidences from Taiwan. For. Sci. 69, 382–396. doi: 10.1093/forsci/fxad011
Liu, W.-Y., Wu, C.-C., and Wang, S.-Y. (2021). Forest management and adaptation strategies in response to climate change by the Taiwanese public. Atmosfera 12:1056. doi: 10.3390/atmos12081056
Moghaddam, E. R. (2014). Growth, development, and yield in pure and mixed forest 103 stands. Int. J. Adv. Biol. Biomed. Res. 2, 2725–2730.
National Taiwan University Biodiversity Research Center. (2006). The natural resources and ecology database of Taiwan III: Agricultural, forestry, fishery and husbandry. Taipei, Taiwan: Forestry Bureau, Council of Agriculture, Executive Yuan.
Nölte, A., Meilby, H., and Yousefpour, R. (2018). Multi-purpose forest management in the tropics: incorporating values of carbon, biodiversity and timber in managing Tectona grandis (teak) plantations in Costa Rica. For. Ecol. Manag. 422, 345–357. doi: 10.1016/j.foreco.2018.04.036
Nyland, R. D. (2002). Silviculture: Concepts and application, 2nd Edn. New York, NY: McGraw-Hill Higher Company. 704.
O’Hara, K. L. (2014). Multiaged Silviculture: Managing for complex Forest stand structures. Oxford University Press, USA.
Page, L. M., and Cameron, A. D. (2006). Regeneration dynamics of Sitka spruce in artificially created forest gaps. For. Ecol. Manag. 221, 260–266. doi: 10.1016/j.foreco.2005.10.006
Pérez-Silos, I., Álvarez-Martínez, J. M., and Barquín, J. (2021). Large-scale afforestation for ecosystem service provisioning: learning from the past to improve the future. Landsc. Ecol. 36, 3329–3343.
Pardos, M., Del Río, M., Pretzsch, H., Jactel, H., Bielak, K., Bravo, F., et al. (2021). The greater resilience of mixed forests to drought mainly depends on their composition: analysis along a climate gradient across Europe. For. Ecol. Manag. 481:118687. doi: 10.1016/j.foreco.2020.118687
Pothier, D., and Marcel, P. (2008). Regeneration development under shelter wood in a lowland red spruce-balsam fir stand. Canadian J. Forest Res. 38, 31–39. doi: 10.1139/X07-135
Pretzsch, H., del Río, M., Biber, P., Arcangeli, C., Bielak, K., Brang, P., et al. (2019). Maintenance of long-term experiments for unique insights into forest growth dynamics and trends: review and perspectives. Eur. J. For. Res. 138, 165–185. doi: 10.1007/s10342-018-1151-y
Saraev, V., Valatin, G., Peace, A., and Quine, C. (2019). How does a biodiversity value impact upon optimal rotation length? An investigation using species richness and forest stand age. Forest Policy Econ. 107:101927. doi: 10.1016/j.forpol.2019.05.013
Sharma, A., Bohn, K., Jose, S., and Cropper, W. P. (2014). Converting even-aged plantations to uneven-aged stand conditions: a simulation analysis of Silvicultural regimes with slash pine (Pinus elliottii Engelm.). For. Sci. 60, 893–906. doi: 10.5849/forsci.13-097
Sharma, A., Bohn, K. K., McKeithen, J., and Singh, A. (2019). Effects of conversion harvests on light regimes in a southern pine ecosystem in transition from intensively managed plantations to uneven-aged stands. For. Ecol. Manag. 432, 140–149. doi: 10.1016/j.foreco.2018.09.019
Shukla, P. R., Skea, J., Calvo Buendia, E., Masson-Delmotte, V., and Pörtner, H. O. (eds.)(2019). IPCC, 2019: Climate change and land: An IPCC special report on climate change, desertification, land degradation, sustainable land management, food security, and greenhouse gas fluxes in terrestrial ecosystems. Intergovernmental Panel on Climate Change (PCC).
Subasinghe, K., Sumanapala, A. P., and Weerawardhena, S. R. (2014). The impact of forest conversion on bird communities in the northern flank of the Knuckles Mountain Forest range. Sri Lanka. J. Asia-Pacific Biodiversity 7, 367–373. doi: 10.1016/j.japb.2014.07.004
Keywords: even-aged pure forest, uneven-aged mixed forest, carbon payment, harvesting intensity, non-timber value
Citation: Lin Y-R and Liu W-Y (2023) Assessment of timber value and carbon credits provided by pure and mixed forests in Taiwan. Front. For. Glob. Change. 6:1246992. doi: 10.3389/ffgc.2023.1246992
Received: 25 June 2023; Accepted: 01 September 2023;
Published: 28 September 2023.
Edited by:
Walter Mattioli, Council for Agricultural and Economics Research (CREA), ItalyReviewed by:
Antonio Tomao, University of Udine, ItalyCopyright © 2023 Lin and Liu. This is an open-access article distributed under the terms of the Creative Commons Attribution License (CC BY). The use, distribution or reproduction in other forums is permitted, provided the original author(s) and the copyright owner(s) are credited and that the original publication in this journal is cited, in accordance with accepted academic practice. No use, distribution or reproduction is permitted which does not comply with these terms.
*Correspondence: Wan-Yu Liu, d3lsaXVAbmNodS5lZHUudHc=
Disclaimer: All claims expressed in this article are solely those of the authors and do not necessarily represent those of their affiliated organizations, or those of the publisher, the editors and the reviewers. Any product that may be evaluated in this article or claim that may be made by its manufacturer is not guaranteed or endorsed by the publisher.
Research integrity at Frontiers
Learn more about the work of our research integrity team to safeguard the quality of each article we publish.