- 1Department of Biology, University of Oxford, Oxford, United Kingdom
- 2The Nature Conservancy, Arlington, VA, United States
- 3Smithsonian Conservation Biology Institute, Front Royal, VA, United States
- 4Department of Biological Sciences, Royal Holloway University of London, Egham, United Kingdom
- 5German Centre for Integrative Biodiversity Research (iDiv) Halle-Jena-Leipzig, Leipzig, Germany
- 6Institute of Biology, Leipzig University, Leipzig, Germany
- 7Département de biologie, Université de Sherbrooke, Sherbooke, QC, Canada
- 8ForestGEO, Smithsonian Tropical Research Institute, Panama City, Panama
- 9INRAE, Univ. Bordeaux, BIOGECO, Bordeaux, France
- 10Birmingham Institute of Forest Research, Birmingham, United Kingdom
- 11School of Biosciences, Edgbaston Campus, University of Birmingham, Birmingham, United Kingdom
- 12Département de sciences naturelles, Institut des sciences de la forêt tempérée (ISFORT), Université du Québec en Outaouais (UQO), Gatineau, QC, Canada
- 13Centre for Forest Research, Université du Québec à Montréal, Montréal, QC, Canada
- 14Ontario Forest Research Institute, Ontario Ministry of Natural Resources, and Forestry, Sault Ste. Marie, ON, Canada
- 15Department of Biology, McGill University, Montréal, QC, Canada
- 16Smithsonian Tropical Research Institute, Panama City, Panama
- 17Department of Forest Resources, University of Minnesota, St. Paul, MN, United States
- 18Hawkesbury Institute for the Environment, Western Sydney University, Penrith, NSW, Australia
- 19Institute for Global Change Biology, and School for the Environment and Sustainability, University of Michigan, Ann Arbor, MI, United States
Although decades of research suggest that higher species richness improves ecosystem functioning and stability, planted forests are predominantly monocultures. To determine whether diversification of plantations would enhance aboveground carbon storage, we systematically reviewed over 11,360 publications, and acquired data from a global network of tree diversity experiments. We compiled a maximum dataset of 79 monoculture to mixed comparisons from 21 sites with all variables needed for a meta-analysis. We assessed aboveground carbon stocks in mixed-species planted forests vs. (a) the average of monocultures, (b) the best monoculture, and (c) commercial species monocultures, and examined potential mechanisms driving differences in carbon stocks between mixtures and monocultures. On average, we found that aboveground carbon stocks in mixed planted forests were 70% higher than the average monoculture, 77% higher than commercial monocultures, and 25% higher than the best performing monocultures, although the latter was not statistically significant. Overyielding was highest in four-species mixtures (richness range 2–6 species), but otherwise none of the potential mechanisms we examined (nitrogen-fixer present vs. absent; native vs. non-native/mixed origin; tree diversity experiment vs. forestry plantation) consistently explained variation in the diversity effects. Our results, predominantly from young stands, thus suggest that diversification could be a very promising solution for increasing the carbon sequestration of planted forests and represent a call to action for more data to increase confidence in these results and elucidate methods to overcome any operational challenges and costs associated with diversification.
1. Introduction
There is growing momentum to restore forest cover to meet climate change mitigation, biodiversity conservation, and sustainable development goals (Griscom et al., 2017; Seddon et al., 2020; Di Sacco et al., 2021). Restoration of forest cover can store substantial carbon within the forest (Cook-Patton et al., 2020), as well as potentially in harvested wood products that are substituted for fossil fuels and/or carbon-intensive products such as concrete or steel (Forster et al., 2021). Although experimental and theoretical work demonstrates that diverse plant systems are more productive and stable through time compared to monocultures, as well as better able to support diverse animal assemblages and provide other critical ecosystem services (Cardinale et al., 2012; Tilman et al., 2014; Messier et al., 2022), many national forest restoration commitments focus on monoculture plantations. Indeed, 45% of forest restoration commitments from tropical countries involve establishing monoculture plantations, despite diversification of plantations being a key policy recommendation (Lewis et al., 2019; Pörtner et al., 2021).
In contrast, growing evidence suggests a positive relationship between diversity and productivity in natural (e.g., Liang et al., 2016; Xu et al., 2020) and planted forest systems (e.g., Ewel et al., 2015; Huang et al., 2018; Feng et al., 2022). Others found that primary production in mixed forests exceeds that in monocultures by about 15%–20% (Zhang et al., 2012; Jactel et al., 2018). There are multiple mechanisms that can explain why mixed plantations might outperform monocultures. Although monoculture species are often selected for their high yield and ease of management (Nabuurs et al., 2018), they may lack resilience to perturbation (Jactel et al., 2017, 2021), which could compromise their long-term carbon storage potential (Hutchison et al., 2018; Osuri et al., 2020; Messier et al., 2022), especially under future climate conditions. For example, in a tree planting experiment over 15 years in Panama, growth in mixed stands was more stable in response to climatic extremes, and tree mortality was lower, compared to monocultures (Hutchison et al., 2018). There is also evidence that carbon capture can be more stable through time and recover more rapidly after drought in species-rich natural forests, compared to species-poor plantations (Osuri et al., 2020; Pardos et al., 2021). The simple act of mixing species with different functional traits can also result in more reliable levels of establishment, compared to a mosaic of monocultures of different species, where some may perform poorly (Tuck et al., 2016). Finally, diversification of forests is expected to increase resistance to pests and disease (Jactel and Brockerhoff, 2007; Gamfeldt et al., 2013; van der Plas et al., 2016; Jactel et al., 2021), which could also improve carbon stability and storage.
Alongside increased resilience to perturbation, there are other mechanisms that may lead to greater carbon accumulation in mixed-species planted forests compared to monocultures. Species mixtures may demonstrate “complementarity,” whereby niche differentiation or facilitation among individual species enhances overall performance (Loreau and Hector, 2001; Feng et al., 2022). For example, variation in crown architecture complementarity can achieve overyielding in mixed-species planted forests (Jucker et al., 2015; Williams et al., 2017). Complementarity may also be higher among native species that have coevolved (Cook-Patton and Agrawal, 2014; Zuppinger-Dingley et al., 2014). However, non-native plantation species might be expected to outperform native plantation species; plantation species (e.g., Eucalyptus and Pinus species) are often planted outside their native range and selected for their fast growth rates (Heryati et al., 2011; Marron and Epron, 2019). Species with particular traits can also enhance the overall productivity of a mixture. For example the presence of nitrogen-fixing species can enhance nitrogen availability for species unable to fix nitrogen, boosting carbon accumulation potential (Loreau and Hector, 2001; Mayoral et al., 2017). However, species mixing could lead to lower productivity in diverse stands where a lower yielding species dilutes a monoculture of a fast-growing, commercially valuable species. Mixing lower yielding species with a very high yielding species could lead to lower stand-level carbon accumulation because the diverse stand would fail to demonstrate “transgressive overyielding,” where diverse systems outperform even the most productive monoculture.
Planted forests can provide timber and other forest products, support local communities and provide an economic income, while helping to alleviate pressure on primary and semi-natural forest (McEwan et al., 2020; Messier et al., 2022). Given the dominant practice of establishing plantations as monocultures and the anticipated benefits of mixed-species planting for carbon accumulation (Beugnon et al., 2021) and biodiversity (Ampoorter et al., 2020), we need a robust examination of how carbon stocks compare in mixed planted forests relative to monocultures. Here, we present results of a meta-analysis, combining data from the Tree Diversity Network (TreeDivNet) (Verheyen et al., 2016) with data from peer-reviewed publications identified via a comprehensive literature search, to assess the effect of diversification on carbon stocks in planted forests, addressing the following research questions:
1. Are carbon stocks in mixed-species planted forests higher than in monocultures? We compared carbon stocks in mixed planted forests to carbon stocks in (a) the average of monocultures, (b) the most productive monoculture, and (c) monocultures of commercial timber species, within an experiment.
2. Does the difference in carbon storage between mixtures and monocultures increase with species richness of the mixture?
3. What are potential mechanisms driving differences in carbon stocks between mixtures and monocultures? Specifically, we compared how responses changed in stands with and without nitrogen fixers and in native versus non-native stands. We also compared tree diversity experiments to forestry plantations, hypothesizing that experiments may better control confounding factors and thus may be more likely to demonstrate any positive effect of diversity.
2. Methods
2.1. Data collation
This meta-analysis used studies compiled in a previous systematic literature review, aiming to assess carbon or biomass accumulation in establishing forests. Cook-Patton et al. (2020) compiled 10,937 studies using a systematic keyword search in Web of Science (19 April 2017) for studies published since 1975: TOPIC: (biomass OR carbon OR agb OR recover* OR accumulat*) AND (forest) AND (restorat* OR reforest* OR afforest* OR plantation* OR agroforest* OR secondary*). This initial literature set was augmented to 11,360 studies by including additional papers referenced within the original studies and datasets from Oak Ridge National Laboratory, International Centre for Research in Agroforestry, and the Chinese Academy of Forestry. Abstracts were reviewed to identify studies describing reestablishment of forest cover; these were reduced to 1,400 studies which quantified carbon or biomass stocks. Sixty-two of these studies directly compared carbon in mixed and monoculture planted forests within one study and were assessed for inclusion in our meta-analysis.
We selected the subset of studies that met our criteria for inclusion: mixed and monoculture planted forests of the same age and containing a measure of aboveground carbon or biomass, monocultures composed of a constituent species of the mixed treatment, and a specific geographic location (to classify species as native or non-native and to classify commercial species). Studies also needed to contain necessary information (mean, standard deviation, sample size) to calculate the effect size for the meta-analysis, the standardised mean difference (SMD) or “Hedges’ g” (Hedges, 1981). A total of 9 of the 62 studies met these criteria (Supplementary Table S1). Common reasons for exclusion were confounding variables in the mixed and monoculture treatments (e.g., mixed and monoculture stands of different ages) or failure to provide the metrics needed to conduct a meta-analysis. We included two additional studies from a meta-analysis by Zhang et al. (2012). We then augmented this literature-based dataset with seven additional datasets from TreeDivNet,1 made available by TreeDivNet projects in response to a call for data, bringing the total dataset to 18 studies. Some studies included multiple experiments, producing 21 independent experiments from 18 studies. The studies often included multiple distinct mixed treatments (multiple species combinations or ratios between species), yielding a total of 79 monoculture-to-mixed comparisons. Each unique species combination (based on species composition or species ratios) was defined as a treatment. We extracted from each experiment: (1) mean aboveground carbon or biomass for each treatment (calculated in the original studies using allometric equations); (2) standard deviation of the carbon or biomass measure (in some cases calculated from standard error and sample size); (3) sample size for each treatment; (4) species composition of each treatment; (5) stand age and (6) geographic location. When data were presented graphically in the papers, we extracted estimates using WebPlotDigitizer.2
2.2. Data preparation
For research question 1a, where we were comparing carbon stocks in mixed planted forests to the average of carbon stocks in constituent species monocultures, this required us to calculate the mean and standard deviation across the monocultures relevant to each mixed treatment. In 9 of the 79 comparisons, only one of the mixed treatment constituent species was planted as a monoculture, so in these cases this monoculture was used in the comparison to the mixed treatment. Where two or more monoculture treatments were available, we calculated the average carbon/biomass and standard deviation. For the TreeDivNet data we had access to the full raw data, which was used to calculate the mean and standard deviation. For the studies resulting from the literature search, we used the extracted means and standard deviations for each monoculture treatment, to calculate a mean, standard deviation and sample size across monoculture treatments. The standard deviation was calculated based on the formula (Frey et al., 2006). The sample size of the average carbon/biomass measure was the aggregate sample size of all contributing monoculture treatments. The resulting means, standard deviations and sample sizes were used to calculate the SMDs.
For research questions 1b and 1c, the calculated (TreeDivNet studies) or extracted (studies from literature search) means, standard deviations and sample sizes from the mixed and monoculture treatments within each comparison were used to calculate SMDs. Question 1b compared carbon stocks in mixtures to the associated monoculture with the highest carbon/biomass stocks (n = 79 comparisons), again including the 9 comparisons where only a single monoculture was available. For research question 1c, we compared carbon stocks in mixtures to monocultures composed of commonly used commercial plantation species (n = 38 comparisons). We classified commercial species monocultures when there was evidence that these species were commonly used as commercial species in the study region (Supplementary Table S2). We hypothesised that, as commercially grown tree species are often selected for their high yields, they may outperform the mixed treatments. The calculated (TreeDivNet studies) or extracted (studies from literature search) means, standard deviations and sample sizes were used to calculate SMDs for the comparison to the best or commercial species monocultures.
To assess some potential mechanisms behind differences in carbon stocks between mixed and monoculture treatments we classified each mixed treatment based on its species richness, presence of a nitrogen-fixing species in the mixture (yes/no), origin of the species (all native vs. some/all species non-native) and study design (designed experiment vs. existing forestry plantations).
To also present our results in real terms we calculated the percentage of monoculture carbon in the mixed treatment for each comparison and the 95% confidence interval around this value, as SMD is on the scale of standard deviations. The carbon stocks in each treatment are presented in Supplementary Tables S3, S4. Where stocks were given as biomass in the original study, we converted these to carbon using the conversion factor of 0.47 (Aalde et al., 2006).
2.3. Meta-analysis
All data manipulation, calculations and analysis were carried out in R (R Core Team, 2020). We used the escalc function in the package metafor to calculate the SMD (Hedges’ g) in carbon or biomass for each monoculture-to-mixed comparison and the associated sampling variance (Viechtbauer, 2010). Although some studies reported biomass stocks and others carbon stocks, we assume that carbon is a constant proportion of biomass (Aalde et al., 2006). Given this assumption, the calculation of the SMD therefore converts biomass and carbon onto the same scale. We hereafter refer solely to aboveground carbon stocks in the Results and Discussion. We calculated SMDs of the mixture treatment relative to the average of the monoculture treatments (overyielding), the monoculture treatment with the highest carbon stocks in a study (transgressive overyielding) and commercial monoculture treatments (when available).
Multi-level random-effects models with restricted maximum likelihood estimation were fitted using the function rma.mv in the metafor package (Viechtbauer, 2010), model structures are summarised in Supplementary Table S3. Many of our studies contained multiple mixed treatments which were compared to the same monoculture controls (71 out of 79 comparisons shared a monoculture with at least one other comparison). We included a random effect for study site to account for this. For the comparison to the best monoculture, we also included a random effect for monoculture treatment nested within study site. For the comparison to commercial monocultures, in some cases the same mixed treatment was compared to different monocultures and there were also cases where different mixed treatments were compared to the same monoculture; we included a random effect grouping by either shared mixed or monoculture treatment, nested within study site. All comparisons within a study site were between planted forests of the same age, however study sites covered the age range 3.5 to 28 years, the random effect for study site therefore also accounts for this. A random effect for each comparison was also included, to allow heterogeneity this allows the true effect sizes to vary within study sites (Nakagawa et al., 2023). We assessed the significance of the SMDs by determining whether the 95% confidence intervals included zero.
To assess the effect of the level of diversity on SMD, we fitted a mixed-effects model with a moderator [or explanatory variable, which is assumed to modulate the magnitude of effect size (Nakagawa et al., 2023)] for species richness (2–6 species), as a discrete variable, and separately compared mixed vs. average of monoculture and mixed vs. best monoculture. For mixed vs. commercial species monoculture comparisons, there were insufficient data across the levels of species richness to fit it as a moderator. For the mixed vs. average of monocultures and mixed vs. best performing monoculture analyses we fitted separate models for two, four, and six species mixtures, to estimate the effect size for each level of species richness; mixtures with three and five species only had one monoculture-to-mixed comparison each. We also fitted a model to estimate the effect size for two species mixtures compared to commercial monocultures.
To test potential predictors of differences in carbon stocks, we fitted separate mixed-effects models with moderators for presence vs. absence of nitrogen-fixer in the mixed treatment, native vs. some or all non-native species in the mixed treatment and designed experiments vs. existing plantations. For these models we only used data from two-species mixtures, as this was the only species richness level with good representation of both factor levels (Figure 1). To estimate the overall effect size for each moderator level, we subset the data by each level of the moderator and fitted separate models for: nitrogen-fixer present, nitrogen-fixer absent, native species, non-native/mixed origin species, designed experiments, and existing plantations.
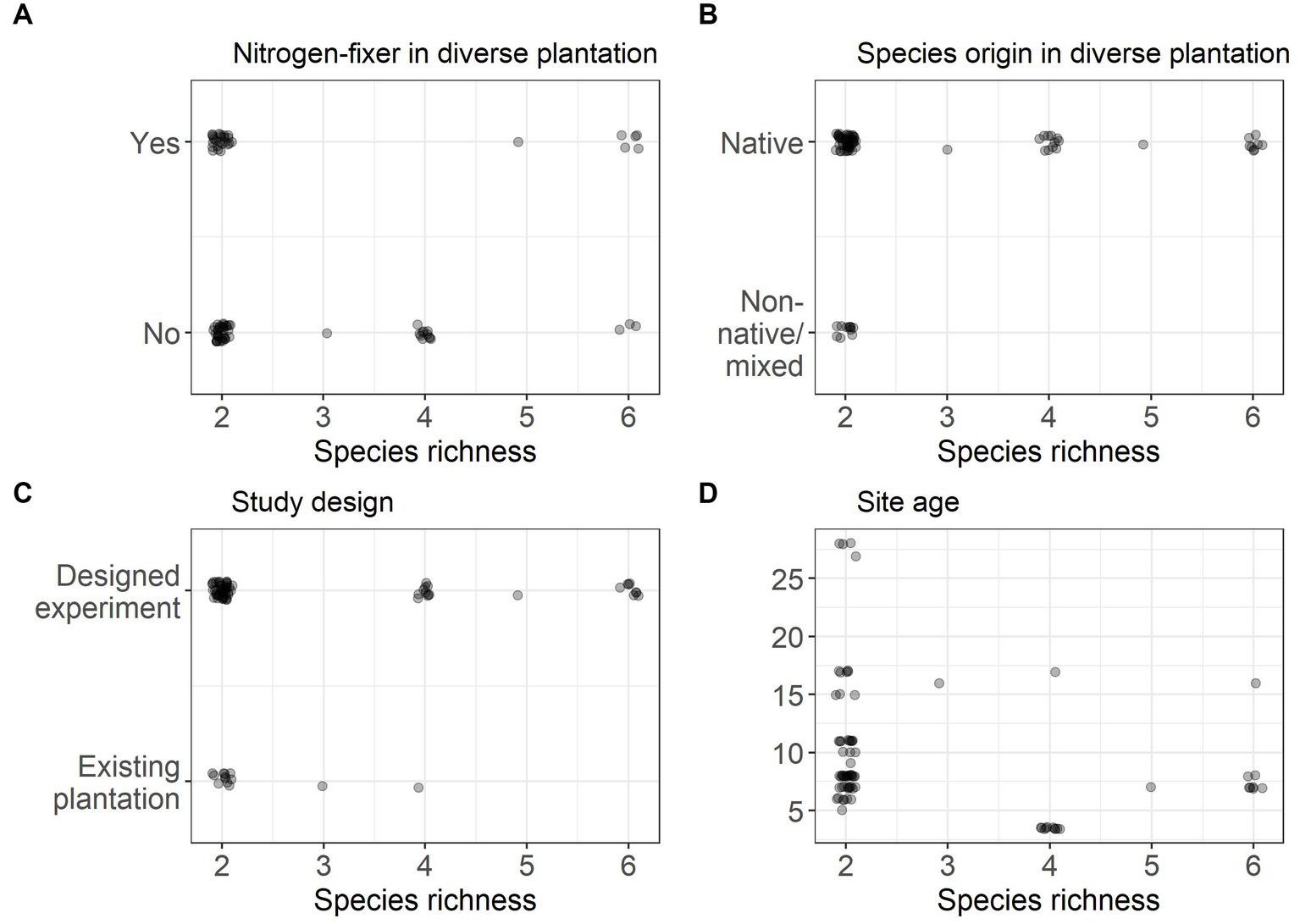
Figure 1. Data representation for each moderator, (A) presence of nitrogen-fixer in the mixed plantation, (B) species origin of the species in the mixed plantation, (C) study design, and (D) age of plantations, used to assess differences in carbon accumulation in mixed plantations compared to the average of monocultures/best associated monoculture.
Age may mediate the effect of diversity on carbon stocks, to assess this we fitted a mixed-effects model with a continuous moderator for stand age for the mixed vs. average, best and commercial monoculture comparisons. These models were fitted using data from two-species mixtures, as this was the only species richness level with good representation across the age range (Figure 1A). A linear and quadratic fit were compared using AIC; the quadratic relationship was fitted post-hoc, based on visual inspection of the data.
We checked for publication bias using funnel plots fitted for each analysis (Supplementary Material S2) and explored the removal of the most extreme positive and negative SMDs. The impact on the interpretation of our results is discussed in Supplementary Material S2.
3. Results
From a systematic review of over 11,360 publications and compilation of data from a global network of tree diversity experiments, we were only able to compile a maximum dataset of 79 monoculture to mixed comparisons, from 21 sites, highlighting the relatively small dataset on mixed vs. monoculture plantations within the forest carbon/biomass literature. Within the 79 comparisons there were 51 unique mixed-species combinations from a pool of 54 species (Supplementary Table S4). Carbon stocks in mixed planted forests were compared to carbon stocks in the average of constituent species monocultures, the best performing constituent monoculture (from a pool of 26 species) and, for a subset of comparisons, commercial species monocultures (from a pool of 11 species). Two species mixtures were the most well represented across the diversity range (species richness 2–6) (Supplementary Figure S1). Geographically the study sites used were biased to the northern hemisphere, with no sites in Africa or South America (Figure 2). The age of study sites ranged from 3.5 to 28 years, with most comparisons from sites less than 20 years old (Figure 1D).
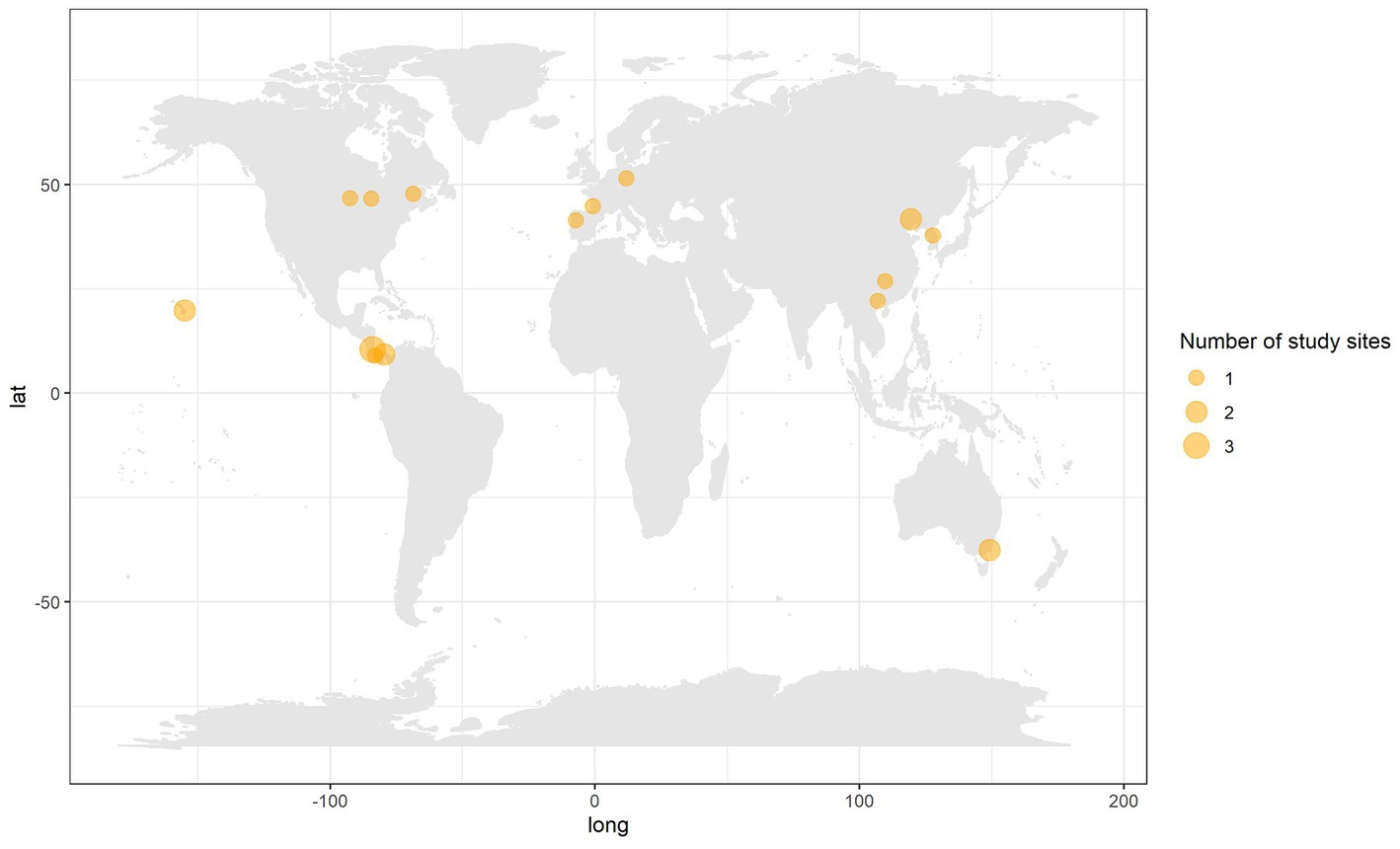
Figure 2. The geographic distribution of study sites. Points are proportional to the number of independent sites in that location (where sites are spatially separate at a distance that would not be discernible at this map scale).
Our overall meta-analyses showed that aboveground carbon stocks in mixed planted forests were higher than in the average of the monocultures (pooled SMD ± 95% CI: 1.41 ± 0.66, k = 79, Figure 3) and higher than in commercial monocultures (1.06 ± 0.59, k = 38, Figure 3). Carbon stocks in mixed planted forests were on average higher than the most productive monoculture, but the confidence interval overlapped zero slightly (0.62 ± 0.63, k = 79, Figure 3). We noted that for the comparison to the best monoculture, removal of the most extreme outliers (SMD >6 and <−3) altered the confidence interval for this result (0.75 ± 0.43, k = 73) (Supplementary Material S2). Although the statistical tests are based on SMDs (in units of standard deviations), the underlying data suggest that mean carbon ±95% CI in the mixed treatments was 171% ± 27 (Supplementary Table S4) of the carbon in the average of monocultures, 174% ± 53 of the carbon in commercial monocultures (Supplementary Table S5) and 127% ± 16 of the carbon in the most productive monocultures (Supplementary Table S4). The community with the greatest relative gain in aboveground carbon was a 3.5 years-old four-species mixture comprised of Betula pendula, Fagus sylvatica, Quercus petraea and Tilia platyphyllos in Saxony-Anhalt, Germany, which contained 43.9 Mg/ha of carbon compared to an average of 6.5 Mg/ha across the monocultures and 13.7 Mg/ha in the best monoculture (Betula pendula) (Supplementary Table S4).
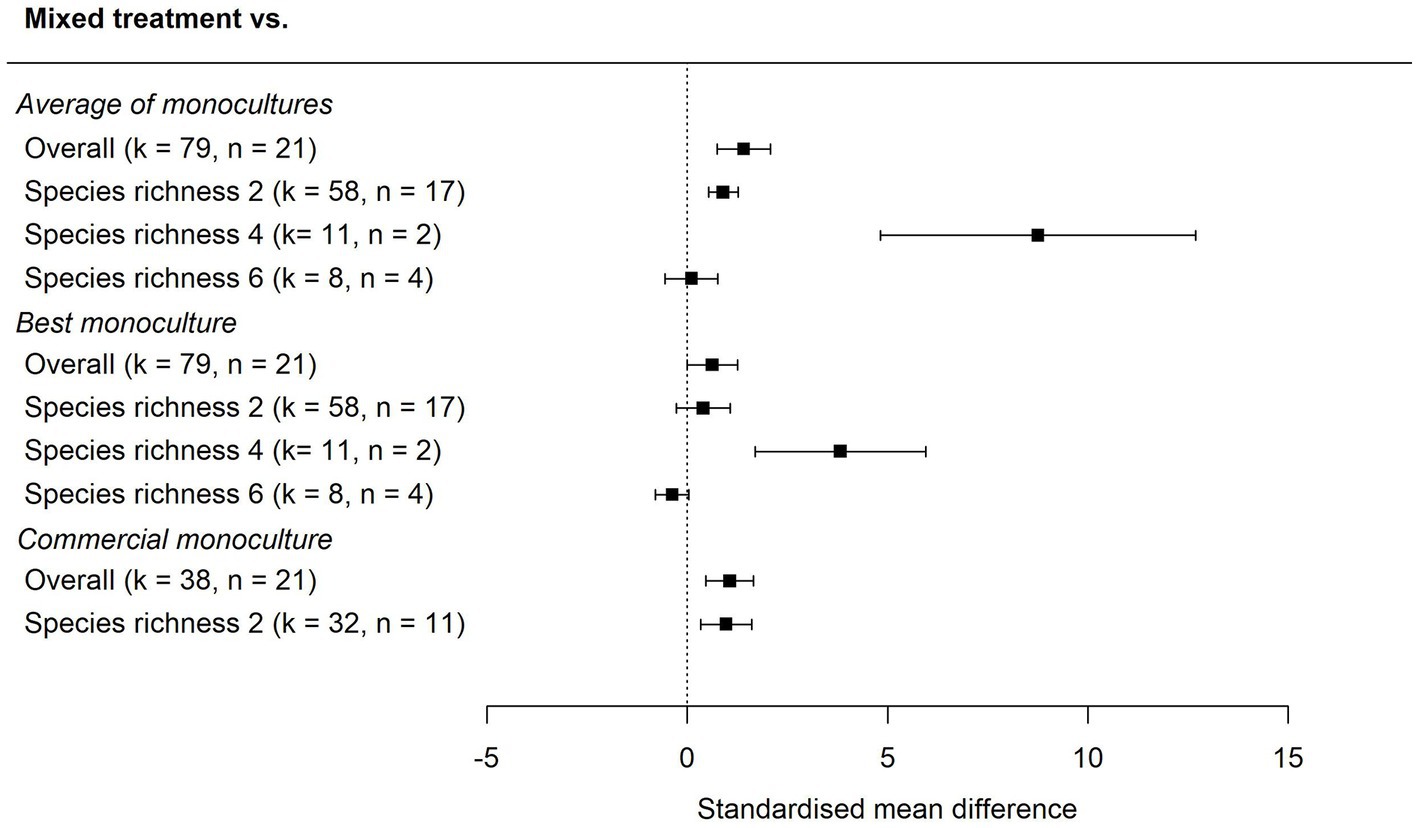
Figure 3. The effect of diversification of planted forests on aboveground carbon stocks relative to the average of associated monocultures, the best associated monoculture, and monocultures of commercial species, using all comparisons and subset by level of species richness. Effect sizes are standardised mean differences. 95% confidence intervals overlapping zero suggest no statistically detectible effect of diversification. Positive values indicate higher carbon stocks in mixtures than in monocultures. Number of comparisons (k) and number of study sites (n) are shown.
The greatest positive difference in carbon stocks was seen in mixed planted forests with four species, which had greater aboveground carbon stocks than the average of monocultures (8.76 ± 3.94, k = 11, Figure 3) and most productive monocultures (3.82 ± 2.13, k = 11, Figure 3). Using the raw data, we calculated that carbon (±95% CI) in the four-species mixtures was 411% ± 86 of the carbon in the average of monocultures and 232% ± 14 of the carbon in the best monoculture. Two-species mixtures also had greater aboveground carbon stocks than the average of monocultures (0.90 ± 0.37, k = 58, Figure 3) and commercial monocultures (0.97 ± 0.63, k = 32, Figure 3), but carbon stocks were not significantly greater than the most productive monocultures (0.40 ± 0.12, k = 58, Figure 3). Carbon in two-species mixtures was 135% ± 13 of carbon in the average of monocultures and 113% ± 6 of carbon in the most productive monoculture (Supplementary Table S4). There was no clear difference in aboveground carbon stocks in six-species mixtures compared to the average of monocultures (0.11 ± 0.66, k = 8, Figure 3) and potential under-yielding relative to the most productive monoculture, although with a wide interval (−0.38 ± 0.41, k = 8, Figure 3). When we fitted a moderator for species richness for the comparison to the average of monocultures and the best monoculture, we found that the moderator was significant for the comparison of mixtures to the average of monocultures (QM = 46.79, p < 0.01, k = 79, Supplementary Figure S2A), but not for the comparison to the best monoculture (QM 8.78, p = 0.067, k = 79, Supplementary Figure S2B).
Moderators for presence vs. absence of a nitrogen-fixer in mixtures (analyses possible for two-species mixtures only) were not significant for the comparison to the average of monocultures (QM = 0.37, p = 0.54, k = 58), best monoculture (QM = 1.35, p = 0.25, k = 58) or commercial species monoculture (QM = 1.33, p = 0.25, k = 32). We used data subset by presence or absence of a nitrogen-fixing species in the mixture to estimate the pooled effect sizes for aboveground carbon stocks in each group (Figure 4A). In mixtures with a nitrogen-fixer present carbon was 145% ± 9 of the carbon in the average of monocultures and in mixtures without a nitrogen-fixer carbon was 128% ± 8 of the carbon in the average of monocultures.
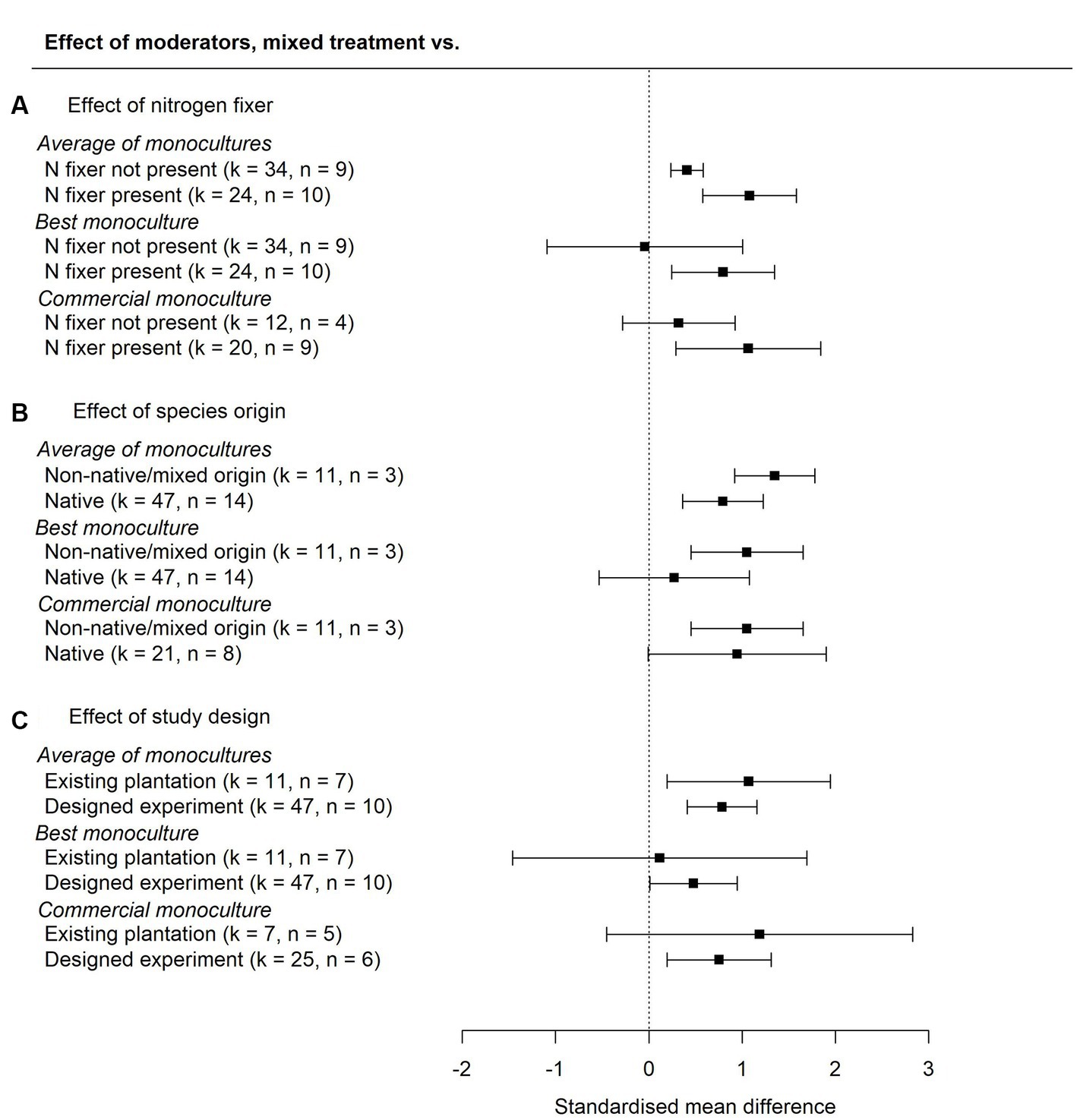
Figure 4. The effect of (A) diversification with/without a nitrogen-fixing species, (B) diversification with all native or non-native/mixed origin species, and (C) study design, on aboveground carbon stocks relative to the average of associated monocultures, the best associated monoculture, and monocultures of commercial species (analysis limited to two-species mixtures). Effect sizes are standardised mean differences, calculated using data subset by presence or absence of a nitrogen-fixing species in the mixture, species origin, and study design. 95% confidence intervals overlapping zero suggest no statistically detectible effect of diversification. Positive values indicate higher carbon stocks in mixtures than in monocultures. Number of comparisons (k) and number of study sites (n) are shown.
Species origins in the mixed treatment (analyses possible for two-species mixtures only) were not significant for any of the comparisons (average of monocultures, QM = 0.94, p = 0.16, k = 58; best performing monoculture QM = 0.75, p = 0.39, k = 58; commercial monoculture QM = 0.091, p = 0.76, k = 32). Effect sizes estimated for each level of the moderator using subsets of the data confirmed that there was no clear effect of diversification based on the geographic origin of the species used (Figure 4B). In mixtures of non-native species or a mixture of non-native and native species carbon was 156% ± 8 of the carbon in the average of monocultures and in mixtures with native species only carbon was 130% ± 8 of the carbon in the average of monocultures.
The effect of study type (analyses possible for two-species mixtures only) was not significant for any of the mixed to monoculture comparisons (average of monocultures QM = 0.24, p = 0.62, k = 58; best monoculture QM = 0.63, p = 0.43, k = 58; commercial species monoculture QM = 0.46, p = 0.50, k = 32). Subsequently, overall effect sizes were estimated using subsets of the data, confirming that there were no clear differences in the effect of diversification based on study type (Figure 4C). In studies using forestry plantations, carbon in the mixed treatment was 128% ± 8 of the carbon in the average of monocultures and in experimental studies carbon in mixtures was 136% ± 7 of the carbon in the average of monocultures.
Analyses of the effect of age were possible for two-species mixtures only. Age had a better fit as a quadratic predictor rather than linear predictor for the comparison of mixed to the average of monocultures (AIC 155.9 vs. 162.6), showing a peak in the positive effect of diversification at the middle of the age range (quadratic term ±95% CI: −0.013 ± 10) (Figure 5A). For the relationship between age and SMD relative to the best monoculture, a post-hoc quadratic predictor provided a better fit than a linear predictor (AIC 149.3 vs. 158.7), showing a peak in the positive effect of diversification before a downturn after age 17 years (quadratic term −0.019 ± 10) (Figure 5B). For the relationship between age and the difference in aboveground carbon stocks for mixed treatments compared to commercial monocultures a linear relationship provided the best fit but was not statistically distinguishable from zero (QM = 0.14, p = 0.71, k = 32, Figure 5C).
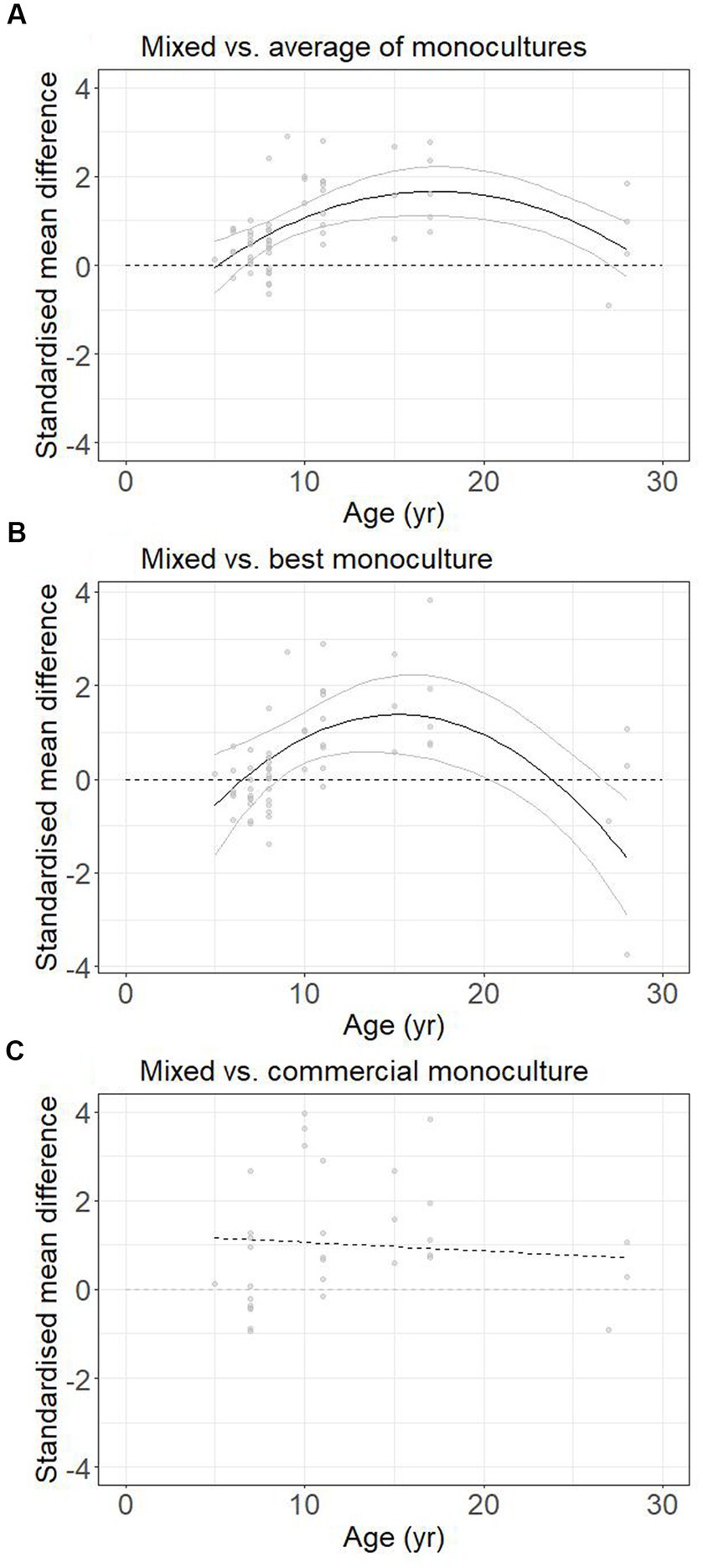
Figure 5. The relationship between stand age and the standardised mean difference in aboveground carbon stocks (analysis limited to two-species mixtures) relative to (A) the average of associated monocultures, k = 58; (B) the best associated monoculture, k = 58; (C) monocultures of commercial species, k = 32. Non-significant trends shown as dashed lines.
4. Discussion
Our results, which are predominantly from young stands, indicate that mixed planted forests clearly outperformed the average of monocultures and commercial monocultures, with no carbon stock penalty even relative to the best performing monoculture. Previous meta-analyses have shown similar trends, using a mixture of studies in planted and natural forests (Piotto, 2008; Zhang et al., 2012; Jactel et al., 2018; Feng et al., 2022); our work emphasises the opportunity to maximise carbon stocks through diversification of the increasing area of planted forests worldwide (FAO, 2020). We found a peak in the diversity benefit in four-species mixtures. However, our meta-analysis also highlights the lack of data from planted forests at higher levels of tree diversity, and more data across the range of species richness is needed to better characterise this relationship. We noted that removal of the most extreme outliers altered the results for the comparison to the best monoculture, such that mixtures clearly outperformed the monoculture (Supplementary Material S2). This further emphasises the need for more data to explore these trends. Given the young age of the planted forests used in our meta-analysis (3.5–28 years), and the expectation that diversity relationships will strengthen over time, further analysis as these forests age and from older planted forests would be informative (Guerrero-Ramírez et al., 2017). Alongside the expected benefits of increased provision of other ecosystem services, enhanced resilience, and resistance to pests and disease, our results further support diversifying planted forests (Aerts and Honnay, 2011; Messier et al., 2022). A critical next step is to integrate this information with analyses of the economics of diversifying plantations (Hildebrandt and Knoke, 2011).
The greatest positive effect of diversification was in mixtures with four species. Other studies have identified a hump-shaped (Xu et al., 2020), positive (Huang et al., 2018), and positive plateauing relationship (Zhang et al., 2012; Liang et al., 2016) between tree species richness and productivity in forest systems. However, in all these studies the relationship remained positive beyond a species richness of 20, except in Zhang et al. (2012), where the relationship plateaued at six species. Our data indicates an apparent peak in the positive effect of diversification in four species mixtures, however, all but one effect size for this species richness level is from one study, where the experimental plots were aged 3.5 years. Our dataset limited exploration of the diversity gradient, and the paucity of studies with higher levels of species richness could also explain the less clear effect of diversification in mixtures with >4 species.
The selection effect may explain the lack of transgressive overyielding found in our analysis, as when the monoculture species is particularly high yielding, it is harder for the mixture to outperform this. This can often be the case in commercial plantations, where species are usually selected for their fast growth rates, as well as attributes such as wood quality (Liu C. L. C. et al., 2018). Moreover, the benefits of diversification may become more apparent over the longer term and under future climate conditions, since mixed plantations may be more likely to maintain productivity even under perturbation (Osuri et al., 2020). As the study sites used in our analysis age, diversity effects may become more pronounced, and the relationship between diversity and function has been shown to become increasingly non-saturating over time (Reich et al., 2012; Guerrero-Ramírez et al., 2017).
When we included nitrogen-fixer as a moderator in our models, which was only possible for two-species mixtures, there was no clear effect. Presence or absence of species with particularly influential functional traits are expected to have a large effect on productivity (Tilman et al., 1997) and addition of a nitrogen-fixing tree species is a reasonably common method of forest diversification, aiming to reduce the need for fertiliser inputs (Temperton et al., 2007; Richards et al., 2010; Marron and Epron, 2019). However, other studies have also found that the presence of nitrogen-fixers in a mixture does not explain overyielding (Jactel et al., 2018; Feng et al., 2022; Quesada-Ávila et al., 2023), but there is evidence that they can increase the growth rates of non-fixing species (Piotto, 2008). It has been shown that different species of nitrogen-fixers fix nitrogen at different rates and that their contribution of nitrogen fixation to a forest stand can change as forests age (Batterman et al., 2013, 2018). This may complicate the mechanism by which nitrogen-fixers contribute to overall forest productivity and accumulation of carbon stocks, which may not be captured in an analysis of presence vs. absence of a nitrogen-fixer in the mixture.
We found that non-native/mixed origin planted forests did not clearly outperform native planted forests. We expected that mixtures of native species only could lead to lower aboveground carbon stocks than mixtures including non-native species, which are often selected for their fast growth rates (Heryati et al., 2011). Fast-growing species such as Eucalyptus and Pinus make up a large proportion of planted forests, collectively comprising 75% of the world’s commercial plantations, often in locations where these species are not native (Marron and Epron, 2019). However, in many of the studies used here, the fast growing species selected for plantations were native to the study location, for example Eucalyptus globulus in Australia (Bauhus et al., 2004).
We also found that the benefit of diversification held up in both highly controlled (e.g., experiments) and less controlled environments. It has often been questioned whether the influence of biodiversity on ecosystem function observed in controlled experiments is expressed in natural and managed ecosystems, where abiotic forcing and complex interactions occur (Duffy et al., 2017; Manning et al., 2019). Both operational forestry plantations and scientific tree diversity experiments are subject to some level of control relative to naturally established forests, with biodiversity experiments more often being more tightly controlled than forestry plantations. However, we found no clear influence of the study context on the effect of diversification on carbon stocks.
We have already highlighted the lack of representation across the diversity gradient and lack of higher diversity studies. Another limitation is that the study sites contributing to our meta-analysis are relatively young, aged between 3.5 and 28 years. This is particularly notable as forests are long-lived and, in the context of production, harvesting of forest products takes place beyond the timescale of our dataset. We accounted for the differing ages of the planted forests in our analysis by including a random effect for study site; however, we also assessed the relationship between forest age and the carbon accumulation relative to monocultures for two-species mixtures. Previous meta-analyses have found that diversity effects increase over time (Cardinale et al., 2011), which has also been observed in forest systems (Guerrero-Ramírez et al., 2017; Huang et al., 2018; Urgoiti et al., 2022). We found a peak in the effect of diversity at 17 years old, however, our a-priori expectation was for a linear or saturating relationship (e.g., Reich et al., 2012; Thakur et al., 2015) and these quadratic models were fitted post-hoc following visual inspection of the data. The apparent downturn after age 17 is driven by the three comparisons at age 28, from two study sites. The effects of complementarity between species are expected to strengthen over time (Fargione et al., 2007; Reich et al., 2012). But as forests age changes in competition can also strongly influence mortality and therefore productivity (Pretzsch et al., 2023), the lack of data from older forests limits our potential to explore the relative influence of these forces with diversification on timescales relevant to the forestry industry (Huang et al., 2018). A recent meta-analysis incorporating studies over longer timescales also found that benefits of diversification increased in young stands, before decreasing after 25 years (Feng et al., 2022). Other studies support this, for example showing increasing effects of species richness on stand volume over time (Bongers et al., 2021); however this study is also limited to 10 years. One of the longest running tree diversity experiments analysed tree growth over 16 years, finding that the effect of species diversity on productivity increased over time (Schnabel et al., 2019). Longer term data from the TreeDivNet experiments used here will be an important resource for further investigation of the effects of diversification on productivity and our results highlight the importance of maintaining tree experiments over the long-term to be able to assess carbon sequestration dynamics as the trees age (Verheyen et al., 2016).
Data limitations also restricted our ability to assess the mechanisms behind differences in carbon accumulation, we were only able to assess moderators individually and for two-species mixtures only. We therefore cannot rule out collinearity between our moderators, although none of them were significant. Furthermore, there are other potential drivers of carbon accumulation that we did not explore. There are multiple traits that might underpin complementarity between species in a mixture and enhancement of carbon stocks; differences in growth rates, and canopy structure and light acquisition all lead to enhanced productivity in mixed plantations (Morin et al., 2011; Jucker et al., 2015; Williams et al., 2021; Urgoiti et al., 2023). Studies designed to manipulate functional diversity alongside species richness allow exploration of these mechanisms and have shown that, for example spatial complementarity underpinned by crown differences can be an important mechanism for explaining productivity increases with diversity (Williams et al., 2017). Establishment of future plantations using species that are selected because they are expected to be functionally complementary will be more informative than some earlier biodiversity experiments where species were selected entirely at random (Ebeling et al., 2014; Tobner et al., 2016; Liu X. et al., 2018). Conifer and broadleaf species often have dissimilar traits and mixing these two groups could be a simple way of forest managers achieving functional diversity without detailed assessment of species traits. Conifer-broadleaf mixtures have been shown to outperform conifer-only or broadleaf-only mixtures in herbivore resistance (Jactel and Brockerhoff, 2007), resistance to windthrow (Jactel et al., 2017) and enhancing productivity (Urgoiti et al., 2023).
Beyond species choices, other management decisions may influence carbon stocks, such as tree density. At low densities, the positive effect of species mixing increases as planting density increases up to a threshold, after which negative effects can emerge at high densities (Feng et al., 2022). Moreover, there are different mechanisms that could be used to establish mixed plantations. On the larger scales at which plantations are typically established, diversification could be achieved through intermixing of species at the individual tree level or through compartments/zones comprising different species. The preferred option will depend on the scale at which diversity influences carbon accumulation, resilience and delivery of ecosystem services and trade-offs with practical constraints of planting, management and harvesting practices. If increased productivity depends on complementarity between individuals of different species then intermixing of species will be important, whereas resilience to extreme climatic events or delivery of certain services may occur at the landscape scale (Aquilué et al., 2020). These uncertainties call for studies exploring the potential scale-dependence of biodiversity effects on critical ecosystem services (Gonzalez et al., 2020) to inform the types of systems that can optimize carbon storage and other ecosystem services, while minimizing practical and financial constraints.
Our study focuses on aboveground carbon stocks only, which is typically the most easily measured forest carbon pool. However, belowground carbon stocks, in tree biomass and in the soil, can be important, with soil carbon stocks ranging from up to 90% of the total carbon stock in boreal forests to 50% in tropical forests (Malhi et al., 2002). Previous studies have found a neutral or negative influence of diversity on belowground biomass. A recent study showed that, while mixtures showed overyielding aboveground, the belowground response was neutral, although the overall impact on carbon stocks remained significantly positive (Martin-Guay et al., 2020). If this result is replicated in other tree diversity studies, this emphasises that, by focussing on aboveground biomass, we may overestimate the impact of tree diversification on forest carbon accumulation (Martin-Guay et al., 2020). Further data on the impact of tree diversity on total ecosystem carbon stocks and an assessment of the responses of different carbon pools would therefore be valuable.
Diversification of plantations is one of the key actions recommended in Messier et al. (2022) and in a recent IPBES-IPCCC report on biodiversity and climate change, which aims to identify synergies and trade-offs between biodiversity protection and climate change mitigation and adaptation (Pörtner et al., 2021). The justification for use of mixed species plantations is that they will store more carbon, be more resilient to perturbation and provide greater support for associated biodiversity (Pörtner et al., 2021; Messier et al., 2022). Our study further supports the use of mixed species plantations as a method to increase carbon stocks and hence climate change mitigation (Beugnon et al., 2021). Accumulating evidence that diversification can increase carbon storage, resistance to perturbation (Hutchison et al., 2018), resilience to pests and disease (Jactel and Brockerhoff, 2007) and delivery of other ecosystem services (Gamfeldt et al., 2013), provides a strong justification for wider implementation of diversification in plantations (Messier et al., 2022). Even where mixtures do not provide substantial increases in carbon storage over monocultures (and we re-emphasise that we find no clear yield losses in mixtures relative to the best monoculture), they may nevertheless be desirable to increase levels of diversity of both the trees and associated organisms (Schuldt et al., 2018, 2019), as well as providing other potential benefits.
Data availability statement
Summary data presented in the study are included in the Supplementary material. The full dataset can be found here: https://zenodo.org/records/8430799. Further inquiries can be directed to the corresponding author.
Author contributions
SC-P and AH conceived the study idea. EW shaped the research focus and conducted the data extraction and analysis. JK gave advice on the analysis. NE, OF, DG, JH, HJ, CaM, CeM, CnM, AP, WP, CP, and PR contributed data to the analysis. EW led the writing, alongside AH and SC-P. All authors contributed to the article and approved the submitted version.
Funding
EW’s PhD was funded by the Natural Environment Research Council NE/L002612/1, Oxford-NERC Doctoral Training Partnership in Environmental Research. The Bezos Earth Fund supported SC-P’s time on this work. NE and OF gratefully acknowledge the support by the German Centre for Integrative Biodiversity Research (iDiv) funded by the German Research Foundation (DFG–FZT 118, 202548816). The contribution of PR was supported by the U.S. NSF Biological Integration Institutes grant DBI-2021898.
Acknowledgments
The authors gratefully acknowledge the support of the Tree Diversity Network and all associated colleagues & institutions who have worked to establish, maintain, and collect data from this global network of Tree Diversity experiments. We also thank the reviewers and editor for their helpful comments.
Conflict of interest
The authors declare that the research was conducted in the absence of any commercial or financial relationships that could be construed as a potential conflict of interest.
Publisher’s note
All claims expressed in this article are solely those of the authors and do not necessarily represent those of their affiliated organizations, or those of the publisher, the editors and the reviewers. Any product that may be evaluated in this article, or claim that may be made by its manufacturer, is not guaranteed or endorsed by the publisher.
Supplementary material
The Supplementary material for this article can be found online at: https://www.frontiersin.org/articles/10.3389/ffgc.2023.1226514/full#supplementary-material
SUPPLEMENTARY MATERIAL S1 | Supplementary Figures and Tables.
SUPPLEMENTARY MATERIAL S2 | Checking for publication bias and the impact of the removal of outliers.
Footnotes
References
Aalde, H., Gonzalez, P., Gytarsky, M., Krug, T., Kurz, W. A., Ogle, S., et al. (2006). Chapter 4 forest land. Eds. H. S. Eggleston, L. Buendia, K. Miwa, T. Ngara and K. Tanabe (Japan: IPCC Guidelines for National Greenhouse Gas Inventories Volume 4 Agriculture, Forestry & Other Land Use, IGES).
Aerts, R., and Honnay, O. (2011). Forest restoration, biodiversity and ecosystem functioning. BMC Ecol. 11:29. doi: 10.1186/1472-6785-11-29
Ampoorter, E., Barbaro, L., Jactel, H., Baeten, L., Boberg, J., Carnol, M., et al. (2020). Tree diversity is key for promoting the diversity and abundance of forest-associated taxa in Europe. Oikos 129, 133–146. doi: 10.1111/oik.06290
Aquilué, N., Filotas, É., Craven, D., Fortin, M. J., Brotons, L., and Messier, C. (2020). Evaluating forest resilience to global threats using functional response traits and network properties. Ecol. Appl. 30, e02095–e02014. doi: 10.1002/eap.2095
Batterman, S. A., Hall, J. S., Turner, B. L., Hedin, L. O., LaHaela Walter, J. K., Sheldon, P., et al. (2018). Phosphatase activity and nitrogen fixation reflect species differences, not nutrient trading or nutrient balance, across tropical rainforest trees. Ecol. Lett. 21, 1486–1495. doi: 10.1111/ele.13129
Batterman, S. A., Hedin, L. O., van Breugel, M., Ransijn, J., Craven, D. J., and Hall, J. S. (2013). Key role of symbiotic dinitrogen fixation in tropical forest secondary succession. Nature 502, 224–227. doi: 10.1038/nature12525
Bauhus, J., van Winden, A. P., and Nicotra, A. B. (2004). Aboveground interactions and productivity in mixed-species plantations of Acacia mearnsii and Eucalyptus globulus. Can. J. For. Res. 34, 686–694. doi: 10.1139/x03-243
Beugnon, R., Ladouceur, E., Sünnemann, M., Cesarz, S., and Eisenhauer, N. (2021). Diverse forests are cool: promoting diverse forests to mitigate carbon emissions and climate change. J. Sustain. Agric. Environ. 1, 5–8. doi: 10.1002/sae2.12005
Bongers, F. J., Schmid, B., Bruelheide, H., Bongers, F., Li, S., von Oheimb, G., et al. (2021). Functional diversity effects on productivity increase with age in a forest biodiversity experiment. Nat. Ecol. Evol. 5, 1594–1603. doi: 10.1038/s41559-021-01564-3
Cardinale, B. J., Duffy, J. E., Gonzalez, A., Hooper, D. U., Perrings, C., Venail, P., et al. (2012). Biodiversity loss and its impact on humanity. Nature 486, 59–67. doi: 10.1038/nature11148
Cardinale, B. J., Matulich, K. L., Hooper, D. U., Byrnes, J., Duffy, J. E., Gamfeldt, L., et al. (2011). The functional role of producer diversity in ecosystems. Am. J. Bot. 98, 572–592. doi: 10.3732/ajb.1000364
Cook-Patton, S. C., and Agrawal, A. A. (2014). Exotic plants contribute positively to biodiversity functions but reduce native seed production and arthropod richness. Ecology 95, 1642–1650. doi: 10.1890/13-0782.1
Cook-Patton, S. C., Leavitt, S. M., Gibbs, D., Harris, N. L., Lister, K., Anderson-Teixeira, K. J., et al. (2020). Mapping carbon accumulation potential from global natural forest regrowth. Nature 585, 545–550. doi: 10.1038/s41586-020-2686-x
Di Sacco, A., Hardwick, K., Blakesley, D., Brancalion, P. H. S., Breman, E., Rebola, L. C., et al. (2021). Ten golden rules for reforestation to optimise carbon sequestration, biodiversity recovery and livelihood benefits. Glob. Chang. Biol. 27, 1328–1348. doi: 10.1111/gcb.15498
Duffy, J. E., Godwin, C. M., and Cardinale, B. J. (2017). Biodiversity effects in the wild are common and as strong as key drivers of productivity. Nature 549, 261–264. doi: 10.1038/nature23886
Ebeling, A., Pompe, S., Baade, J., Eisenhauer, N., Hillebrand, H., Proulx, R., et al. (2014). A trait-based experimental approach to understand the mechanisms underlying biodiversity-ecosystem functioning relationships. Basic Appl. Ecol. 15, 229–240. doi: 10.1016/j.baae.2014.02.003
Ewel, J. J., Celis, G., and Schreeg, L. (2015). Steeply increasing growth differential between mixture and monocultures of tropical trees. Biotropica 47, 162–171. doi: 10.1111/btp.12190
Fargione, J., Tilman, D., Dybzinski, R., Lambers, J. H. R., Clark, C., Harpole, W. S., et al. (2007). From selection to complementarity: shifts in the causes of biodiversity-productivity relationships in a long-term biodiversity experiment. Proc. R. Soc. B 274, 871–876. doi: 10.1098/rspb.2006.0351
Feng, Y., Schmid, B., Loreau, M., Forrester, D. I., Fei, S., Zhu, J., et al. (2022). Multispecies forest plantations outyield monocultures across a broad range of conditions. Science 376, 865–868. doi: 10.1126/science.abm6363
Forster, E. J., Healey, J. R., Dymond, C., and Styles, D. (2021). Commercial afforestation can deliver effective climate change mitigation under multiple decarbonisation pathways. Nat. Commun. 12:3831. doi: 10.1038/s41467-021-24084-x
Frey, C., Penman, J., Hanle, L., Monni, S., and Ogle, S. (2006). Chapter 3: uncertainties Eds. H.S Uncertainties in Eggleston, L. Buendia, K. Miwa T. Ngara, and K. Tanabe (Japan: IPCC Guidelines for National Greenhouse Gas Inventories Volume 1 General Guidance and Reporting, IGES).
Gamfeldt, L., Snäll, T., Bagchi, R., Jonsson, M., Gustafsson, L., Kjellander, P., et al. (2013). Higher levels of multiple ecosystem services are found in forests with more tree species. Nat. Commun. 4:1340. doi: 10.1038/ncomms2328
Gonzalez, A., Germain, R. M., Srivastava, D. S., Filotas, E., Dee, L. E., Gravel, D., et al. (2020). Scaling-up biodiversity-ecosystem functioning research. Ecol. Lett. 23, 757–776. doi: 10.1111/ele.13456
Griscom, B. W., Adams, J., Ellis, P. W., Houghton, R. A., Lomax, G., Miteva, D. A., et al. (2017). Natural climate solutions. Proc. Natl. Acad. Sci. U. S. A. 114, 11645–11650. doi: 10.1073/pnas.1710465114
Guerrero-Ramírez, N. R., Craven, D., Reich, P. B., Ewel, J. J., Isbell, F., Koricheva, J., et al. (2017). Diversity-dependent temporal divergence of ecosystem functioning in experimental ecosystems. Nat. Ecol. Evol. 1, 1639–1642. doi: 10.1038/s41559-017-0325-1
Hedges, L. V. (1981). Distribution theory for Glass’s estimator of effect size and related estimators. J. Educ. Stat. 6, 107–128. doi: 10.3102/10769986006002107
Heryati, Y., Abdu, A., Mahat, M. N., Abdul-Hamid, H., Jusop, S., Majid, N. M., et al. (2011). Assessing forest plantation productivity of exotic and indigenous species on degraded secondary forests. Am. J. Agric. Biol. Sci. 6, 201–208. doi: 10.3844/ajabssp.2011.201.208
Hildebrandt, P., and Knoke, T. (2011). Investment decisions under uncertainty-a methodological review on forest science studies. Forest Policy Econ. 13, 1–15. doi: 10.1016/j.forpol.2010.09.001
Huang, Y., Chen, Y., Castro-Izaguirre, N., Baruffol, M., Brezzi, M., Lang, A., et al. (2018). Impacts of species richness on productivity in a large-scale subtropical forest experiment. Science 362, 80–83. doi: 10.1126/science.aat6405
Hutchison, C., Gravel, D., Guichard, F., and Potvin, C. (2018). Effect of diversity on growth, mortality, and loss of resilience to extreme climate events in a tropical planted forest experiment. Sci. Rep. 8, 15443–15410. doi: 10.1038/s41598-018-33670-x
Jactel, H., Bauhus, J., Boberg, J., Bonal, D., Castagneyrol, B., Gardiner, B., et al. (2017). Tree diversity drives forest stand resistance to natural disturbances. Curr. For. Reports 3, 223–243. doi: 10.1007/s40725-017-0064-1
Jactel, H., and Brockerhoff, E. G. (2007). Tree diversity reduces herbivory by forest insects. Ecol. Lett. 10, 835–848. doi: 10.1111/j.1461-0248.2007.01073.x
Jactel, H., Gritti, E. S., Drössler, L., Forrester, D. I., Mason, W. L., Morin, X., et al. (2018). Positive biodiversity-productivity relationships in forests: climate matters. Biol. Lett. 14, 12–15. doi: 10.1098/rsbl.2017.0747
Jactel, H., Moreira, X., and Castagneyrol, B. (2021). Tree diversity and forest resistance to insect pests: patterns, mechanisms, and prospects. Annu. Rev. Entomol. 66, 277–296. doi: 10.1146/annurev-ento-041720-075234
Jucker, T., Bouriaud, O., and Coomes, D. A. (2015). Crown plasticity enables trees to optimize canopy packing in mixed-species forests. Funct. Ecol. 29, 1078–1086. doi: 10.1111/1365-2435.12428
Lewis, S. L., Wheeler, C. E., Mitchard, E. T. A., and Koch, A. (2019). Restoring natural forests is the best way to remove atmospheric carbon. Nature 568, 25–28. doi: 10.1038/d41586-019-01026-8
Liang, J., Crowther, T. W., Picard, N., Wiser, S., Zhou, M., Alberti, G., et al. (2016). Positive biodiversity-productivity relationship predominant in global forests. Science 354:aaf8957. doi: 10.1126/SCIENCE.AAF8957
Liu, C. L. C., Kuchma, O., and Krutovsky, K. V. (2018). Mixed-species versus monocultures in plantation forestry: development, benefits, ecosystem services and perspectives for the future. Glob. Ecol. Conserv. 15:e00419. doi: 10.1016/j.gecco.2018.e00419
Liu, X., Trogisch, S., He, J.-S., Niklaus, P. A., Bruelheide, H., Tang, Z., et al. (2018). Tree species richness increases ecosystem carbon storage in subtropical forests. Proc. R. Soc. B 285:20181240. doi: 10.1098/rspb.2018.1240
Loreau, M., and Hector, A. (2001). Partitioning selection and complementarity in biodiversity experiments. Nature 412, 72–76. doi: 10.1038/35083573
Malhi, Y., Meir, P., and Brown, S. (2002). Forests, carbon and global climate. Philos. Trans. R. Soc. A 360, 1567–1591. doi: 10.1098/rsta.2002.1020
Manning, P., Loos, J., Barnes, A. D., Batáry, P., Bianchi, F. J. J. A., Buchmann, N., et al. (2019). Transferring biodiversity-ecosystem function research to the management of ‘real-world’ ecosystems. Adv. Ecol. Res. 61, 323–356. doi: 10.1016/BS.AECR.2019.06.009
Marron, N., and Epron, D. (2019). Are mixed-tree plantations including a nitrogen-fixing species more productive than monocultures? For. Ecol. Manag. 441, 242–252. doi: 10.1016/j.foreco.2019.03.052
Martin-Guay, M. O., Paquette, A., Reich, P. B., and Messier, C. (2020). Implications of contrasted above- and below-ground biomass responses in a diversity experiment with trees. J. Ecol. 108, 405–414. doi: 10.1111/1365-2745.13265
Mayoral, C., van Breugel, M., Cerezo, A., and Hall, J. S. (2017). Survival and growth of five Neotropical timber species in monocultures and mixtures. For. Ecol. Manag. 403, 1–11. doi: 10.1016/J.FORECO.2017.08.002
McEwan, A., Marchi, E., Spinelli, R., and Brink, M. (2020). Past, present and future of industrial plantation forestry and implication on future timber harvesting technology. J. For. Res. 31, 339–351. doi: 10.1007/s11676-019-01019-3
Messier, C., Bauhus, J., Sousa-Silva, R., Auge, H., Baeten, L., Barsoum, N., et al. (2022). For the sake of resilience and multifunctionality, let’s diversify planted forests! Conserv. Lett. 15, 1–8. doi: 10.1111/conl.12829
Morin, X., Fahse, L., Scherer-Lorenzen, M., and Bugmann, H. (2011). Tree species richness promotes productivity in temperate forests through strong complementarity between species. Ecol. Lett. 14, 1211–1219. doi: 10.1111/j.1461-0248.2011.01691.x
Nabuurs, G.-J., Verkerk, P. J., Schelhaas, M.-J., González Olabarria, J. R., Trasobares, A., and Cienciala, E. (2018). Climate-smart forestry: mitigation impacts in three European regions. Available at: https://www.efi.int/sites/default/files/files/publication-bank/2018/efi_fstp_6_2018.pdf
Nakagawa, S., Yang, Y., Macartney, E. L., Spake, R., and Lagisz, M. (2023). Quantitative evidence synthesis: a practical guide on meta-analysis, meta-regression, and publication bias tests for environmental sciences. Environ. Evid. 12, 1–19. doi: 10.1186/s13750-023-00301-6
Osuri, A. M., Gopal, A., Raman, T. R. S., Defries, R., Cook-Patton, S. C., and Naeem, S. (2020). Greater stability of carbon capture in species-rich natural forests compared to species-poor plantations. Environ. Res. Lett. 15:034011. doi: 10.1088/1748-9326/ab5f75
Pardos, M., del Río, M., Pretzsch, H., Jactel, H., Bielak, K., Bravo, F., et al. (2021). The greater resilience of mixed forests to drought mainly depends on their composition: analysis along a climate gradient across Europe. For. Ecol. Manag. 481:118687. doi: 10.1016/j.foreco.2020.118687
Piotto, D. (2008). A meta-analysis comparing tree growth in monocultures and mixed plantations. For. Ecol. Manag. 255, 781–786. doi: 10.1016/J.FORECO.2007.09.065
Pörtner, H. O., Scholes, R. J., Agard, J., Archer, E., Arneth, A., Bai, X., et al. (2021). IPBES-IPCC co-sponsored workshop report on biodiversity and climate change. IPBES and IPCC.
Pretzsch, H., del Río, M., Arcangeli, C., Bielak, K., Dudzinska, M., Ian Forrester, D., et al. (2023). Competition-based mortality and tree losses. An essential component of net primary productivity. For. Ecol. Manag. 544:121204. doi: 10.1016/j.foreco.2023.121204
Quesada-Ávila, G., Turner, B. L., and Hall, J. S. (2023). No evidence that the valuable timber species, Dalbergia retusa, enhances nutrient cycling and uptake by neighboring timber species. J. Sustain. For. 42, 205–217. doi: 10.1080/10549811.2021.1989700
R Core Team (2020). R: A language and environment for statistical computing. Available at: https://www.r-project.org/
Reich, P. B., Tilman, D., Isbell, F., Mueller, K., Hobbie, S. E., Flynn, D. F. B., et al. (2012). Impacts of biodiversity loss escalate through time as redundancy fades. Science 336, 589–592. doi: 10.1126/science.1217909
Richards, A. E., Forrester, D. I., Bauhus, J., and Scherer-Lorenzen, M. (2010). The influence of mixed tree plantations on the nutrition of individual species: a review. Tree Physiol. 30, 1192–1208. doi: 10.1093/treephys/tpq035
Schnabel, F., Schwarz, J. A., Dănescu, A., Fichtner, A., Nock, C. A., Bauhus, J., et al. (2019). Drivers of productivity and its temporal stability in a tropical tree diversity experiment. Glob. Chang. Biol. 25, 4257–4272. doi: 10.1111/gcb.14792
Schuldt, A., Assmann, T., Brezzi, M., Buscot, F., Eichenberg, D., Gutknecht, J., et al. (2018). Biodiversity across trophic levels drives multifunctionality in highly diverse forests. Nat. Commun. 9:2989. doi: 10.1038/s41467-018-05421-z
Schuldt, A., Ebeling, A., Kunz, M., Staab, M., Guimarães-Steinicke, C., Bachmann, D., et al. (2019). Multiple plant diversity components drive consumer communities across ecosystems. Nat. Commun. 10:1460. doi: 10.1038/s41467-019-09448-8
Seddon, N., Chausson, A., Berry, P., Girardin, C. A. J., Smith, A., and Turner, B. (2020). Understanding the value and limits of nature-based solutions to climate change and other global challenges. Philos. Trans. R. Soc. B 375:20190120. doi: 10.1098/rstb.2019.0120
Temperton, V. M., Mwangi, P. N., Scherer-Lorenzen, M., Schmid, B., and Buchmann, N. (2007). Positive interactions between nitrogen-fixing legumes and four different neighbouring species in a biodiversity experiment. Oecologia 151, 190–205. doi: 10.1007/s00442-006-0576-z
Thakur, M. P., Milcu, A., Manning, P., Niklaus, P. A., Roscher, C., Power, S., et al. (2015). Plant diversity drives soil microbial biomass carbon in grasslands irrespective of global environmental change factors. Glob. Chang. Biol. 21, 4076–4085. doi: 10.1111/gcb.13011
Tilman, D., Isbell, F., and Cowles, J. M. (2014). Biodiversity and ecosystem functioning. Annu. Rev. Ecol. Evol. Syst. 45, 471–493. doi: 10.1146/annurev-ecolsys-120213-091917
Tilman, D., Knops, J., Wedin, D., Reich, P., Ritchie, M., and Siemann, E. (1997). The influence of functional diversity and composition on ecosystem processes. Science 277, 1300–1302. doi: 10.1126/science.277.5330.1300
Tobner, C. M., Paquette, A., Gravel, D., Reich, P. B., Williams, L. J., and Messier, C. (2016). Functional identity is the main driver of diversity effects in young tree communities. Ecol. Lett. 19, 638–647. doi: 10.1111/ele.12600
Tuck, S. L., O’Brien, M. J., Philipson, C. D., Saner, P., Tanadini, M., Dzulkifli, D., et al. (2016). The value of biodiversity for the functioning of tropical forests: insurance effects during the first decade of the Sabah biodiversity experiment. Proc. R. Soc. B 283:20161451. doi: 10.1098/rspb.2016.1451
Urgoiti, J., Messier, C., Keeton, W. S., and Paquette, A. (2023). Tree community overyielding during early stand development is explained by asymmetric species-specific responses to diversity. Funct. Ecol. 1–13. doi: 10.1111/1365-2435.14414
Urgoiti, J., Messier, C., Keeton, W. S., Reich, P. B., Gravel, D., and Paquette, A. (2022). No complementarity no gain—net diversity effects on tree productivity occur once complementarity emerges during early stand development. Ecol. Lett. 25, 851–862. doi: 10.1111/ele.13959
van der Plas, F., Manning, P., Allan, E., Scherer-Lorenzen, M., Verheyen, K., Wirth, C., et al. (2016). Jack-of-all-trades effects drive biodiversity–ecosystem multifunctionality relationships in European forests. Nat. Commun. 7:11109. doi: 10.1038/ncomms11109
Verheyen, K., Vanhellemont, M., Auge, H., Baeten, L., Baraloto, C., Barsoum, N., et al. (2016). Contributions of a global network of tree diversity experiments to sustainable forest plantations. Ambio 45, 29–41. doi: 10.1007/s13280-015-0685-1
Viechtbauer, W. (2010). Conducting meta-analyses in R with the metafor package. J. Stat. Softw. 36, 1–48. doi: 10.18637/jss.v036.i03
Williams, L. J., Butler, E. E., Cavender-Bares, J., Stefanski, A., Rice, K. E., Messier, C., et al. (2021). Enhanced light interception and light use efficiency explain overyielding in young tree communities. Ecol. Lett. 24, 996–1006. doi: 10.1111/ele.13717
Williams, L. J., Paquette, A., Cavender-Bares, J., Messier, C., and Reich, P. B. (2017). Spatial complementarity in tree crowns explains overyielding in species mixtures. Nat. Ecol. Evol. 1:0063. doi: 10.1038/s41559-016-0063
Xu, S., Eisenhauer, N., Ferlian, O., Zhang, J., Zhou, G., Lu, X., et al. (2020). Species richness promotes ecosystem carbon storage: evidence from biodiversity-ecosystem functioning experiments. Proc. R. Soc. B 287:20202063. doi: 10.1098/rspb.2020.2063rspb20202063
Zhang, Y., Chen, H. Y. H., and Reich, P. B. (2012). Forest productivity increases with evenness, species richness and trait variation: a global meta-analysis. J. Ecol. 100, 742–749. doi: 10.1111/j.1365-2745.2011.01944.x
Keywords: tree diversity, aboveground carbon stocks, biodiversity, ecosystem functioning, plantation, forest, climate mitigation, nature-based solution
Citation: Warner E, Cook-Patton SC, Lewis OT, Brown N, Koricheva J, Eisenhauer N, Ferlian O, Gravel D, Hall JS, Jactel H, Mayoral C, Meredieu C, Messier C, Paquette A, Parker WC, Potvin C, Reich PB and Hector A (2023) Young mixed planted forests store more carbon than monocultures—a meta-analysis. Front. For. Glob. Change. 6:1226514. doi: 10.3389/ffgc.2023.1226514
Edited by:
Lars Vesterdal, University of Copenhagen, DenmarkReviewed by:
Olivier Bouriaud, Ștefan cel Mare University of Suceava, RomaniaDietrich Hertel, University of Göttingen, Germany
Copyright © 2023 Warner, Cook-Patton, Lewis, Brown, Koricheva, Eisenhauer, Ferlian, Gravel, Hall, Jactel, Mayoral, Meredieu, Messier, Paquette, Parker, Potvin, Reich and Hector. This is an open-access article distributed under the terms of the Creative Commons Attribution License (CC BY). The use, distribution or reproduction in other forums is permitted, provided the original author(s) and the copyright owner(s) are credited and that the original publication in this journal is cited, in accordance with accepted academic practice. No use, distribution or reproduction is permitted which does not comply with these terms.
*Correspondence: Emily Warner, ZW1pbHkud2FybmVyQGJpb2xvZ3kub3guYWMudWs=; ZW0ud2FybmVyMjhAZ21haWwuY29t
†These authors have contributed equally to this work and share senior authorship