- 1Bern University of Applied Sciences, BFH-HAFL, Zollikofen, Switzerland
- 2TUM School of Life Sciences, Department of Life Science Systems, Chair of Ecosystem Dynamics and Forest Management, Technical University of Munich, Freising, Germany
- 3Department of Natural Hazards, Austrian Research Centre for Forests (BFW), Innsbruck, Austria
- 4WSL Institute for Snow and Avalanche Research SLF, Davos, Switzerland
- 5Climate Change, Extremes and Natural Hazards in Alpine Regions Research Centre CERC, Davos, Switzerland
Forests in mountain areas provide an indispensable ecosystem service by protecting people and infrastructure against natural hazards. As forests are increasingly affected by global change, including climate change, more frequent and severe natural disturbances, and shifts in land use, open questions remain regarding the long-term and sustainable provision of this crucial protective service. To improve our understanding of the various effects of global change on protective forests, we summarized the current knowledge based on a systematic review. Focusing on changes in mountain forests’ protective effect against snow avalanches, landslides, rockfall, torrential floods and debris flow, we assessed 72 peer-reviewed, English publications. Overall, climate-induced changes are expected to increase forests’ protective effect at higher elevations but reduce it at lower elevations mainly due to increased drought. Natural disturbances usually decrease the protective effect of forests, and their impact is often further exacerbated by salvage logging. Different forest management strategies are often studied using forest simulation models, and their impacts on protective forests strongly depend on the local context and interactions with climate change. While clearcuts consistently reduce the protective effect, other forest management interventions such as thinning can have either positive or negative effects. Most of the reviewed studies were case studies based on forest simulation or process-based hazard models (but rarely combining the two), while empirical evidence was scarce. Forests’ protective effect is often assessed using (diverse) indicators of forest structure, but evaluations of resulting risks are less common. More consistent modeling approaches linking forest structure to hazard and risk, as well as consistent indicators across different case studies, are needed for a better understanding of changes in protective forests and the service they provide under global change.
1. Introduction
Forest ecosystems provide multiple goods and services to society, such as natural habitat for endangered plant and animal species, timber and non-wood forest products, freshwater, as well as key recreational and cultural values (Jenkins and Schaap, 2018; Winkel et al., 2022). In mountain areas, a key role of forests is the protection of people and infrastructure against the impacts of natural hazards, such as snow avalanches, landslides, rockfall or torrential floods and debris flows (Brang et al., 2001; Sudmeier-Rieux et al., 2021; Teich et al., 2022a). Numerous studies have shown the high effectiveness of forests in mitigating damages from these natural hazards (e.g., Olschewski et al., 2012; Moos et al., 2017; Bhattacharjee and Behera, 2018; Gehring et al., 2019; Grima et al., 2020). These so-called protective forests are a nature-based solution that can serve similar functions as technical (gray) infrastructure, such as avalanche barriers or rockfall nets, but usually at lower costs, while also providing a wide array of co-benefits (Getzner et al., 2017; Ruangpan et al., 2020). However, adverse impacts of global change on mountain forests are becoming increasingly evident (e.g., Mina et al., 2017; Albrich et al., 2020), raising questions about their long-term and sustainable capacity to provide protection from natural hazards (Makino and Rudolf-Miklau, 2021).
Protective forests are forests that have the primary function of reducing the risk of natural hazards for settlements and infrastructure by affecting one or several risk components, namely the hazard, exposure, and vulnerability (Brang et al., 2001; IPCC, 2012). The hazard refers to the occurrence probability of an event that can potentially cause damage (UNISDR, 2009). Forests can reduce the hazard by either preventing the release of a mass (e.g., snow avalanches, landslides) or hindering its downslope propagation (e.g., rockfall, snow avalanches), thus reducing its onset and/or propagation probability (Moos et al., 2018). Exposure is a measure of the possible loss that may result from the impacts of a damaging event on people and their properties (UNISDR, 2009). By dissipating energy and retaining material, forests can reduce the intensity of a natural hazard process and, consequently, the extent of damage (Poratelli et al., 2020). Vulnerability describes the degree of physical, economic, societal, or environmental loss caused by a natural hazard event (Fritzsche et al., 2014). It can be reduced by forests as they provide communities with resources to withstand and recover from damaging events (Sudmeier-Rieux et al., 2021). The degree to which a forest can mitigate risks emanating from natural hazards corresponds to its “protective effect” (Brang et al., 2001; Teich et al., 2022a), which largely depends on the forest extent, structure, and composition, but also on the magnitude of the natural hazard event (Schwarz et al., 2010; Moos et al., 2016; Costa et al., 2021; Perzl et al., 2021).
Generally, protective forests represent a sustainable and reliable protection system (Moos et al., 2019a). However, since forest ecosystems are dynamic, their protective effect is also subject to fluctuations. Direct anthropogenic impacts, such as land-use change or forest management interventions, can alter the extent and structure of protective forests (e.g., Bigot et al., 2009; Vergani et al., 2016). Natural forest dynamics and disturbances can result in temporary or irreversible loss of the protective effect, and these dynamics are being accelerated by climate change (e.g., Wohlgemuth et al., 2017; Teich et al., 2019; Oven et al., 2020; Caduff et al., 2022). At the same time, rising air temperatures and more frequent and severe drought periods will lead to shifts in tree species distribution and forest composition, which may impact their protective effect differently depending on the type of natural hazard. The uncertainties related to these changes pose great challenges for the sustainable management of protective forests and the key ecosystem services they provide in mountain areas (Thrippleton et al., 2023).
This review seeks to synthesize the various types of global change impacts on protective forests, as independent or interacting factors. We therefore conducted a state-of-the-art systematic analysis of the English-language and peer-reviewed literature, examining the influence of global change on protective forests. We focused on climate - or anthropogenic-driven changes, as well as (changing) natural disturbance regimes and their implications for the protective effect of forests. We constrained the assessment to protective forests situated in mountain areas that are characterized by changes in elevation over short distances. These forests directly protect against the gravity-driven natural hazards snow avalanches, rockfall, landslides, torrential floods, and debris flows. Our review provides an overview of the existing literature, including the geographical distribution of the studies, the considered hazard processes, and types of global change impacts, as well as the methods applied to detect the changes in forests’ protective effect. Based on our analysis, we discuss the interactions of different global change impacts and identify knowledge gaps and future research avenues, which may help shape future research programs and inform forest management.
2. Methods
2.1. Literature search
We conducted a systematic literature research following the PRISMA (Preferred Reporting Items for Systematic reviews and Meta-Analyses) method to ensure transparency (Page et al., 2021). In a first step, we identified potentially relevant publications based on searches with predefined search terms in the two databases Web of Science and CAB Abstracts (Figure 1). We therefore combined “protective forest” terms with terms concerning “global change” and the “protective service” (Table 1). We searched for the respective terms in the title, keywords and/or the abstract. This resulted in 797 papers from Web of Science and 247 from CAB Abstracts. They were retrieved on August 4, 2022. We expanded our search with results from GoogleScholar, by searching for the terms “protective forest” and “climate change” as well as “impact of global change on protective forests” (27 additional articles) and with publications we had known before and judged as relevant, but which were not included in the results of the initial search (6 additional publications). We then screened the publication’s titles and abstracts to assess whether they were thematically relevant or not. Only papers that specifically addressed changes in forests’ protective effect against natural hazards in mountain areas under global change were included in the subsequent detailed review. The detailed inclusion criteria are reported in Table 2. We further excluded “gray” literature (e.g., non-peer reviewed documents and reports) as well as non-English publications. This resulted in a total of 72 relevant publications (Supplementary Table S1).
2.2. Literature assessment and data analysis
In a next step, the author team reviewed the relevant publications following a systematic protocol (Supplementary Table S2). For each publication, basic information, such as the investigated natural hazard process(es), the country and location, the forest type, or the considered elevation range, were recorded. We further categorized the studies based on the addressed type(s) of global change impact, distinguishing three different main types:
• Climate-induced forest change: Change in forest extent, structure or composition due to the direct effect of changing climate (e.g., altering growth conditions / mortality due to changing air temperature and precipitation; treeline shift);
• (Changing) natural disturbances: Forest change following natural disturbance (e.g., forest fires, windthrow, insects and pathogens, mass movements);
• Anthropogenic forest change: Change in forest extent, structure, or composition due to direct human influence (e.g., land-use change, management interventions, de−/afforestation, introduction of alien species).
We assessed whether forests changed in their (i) composition, (ii) structure, and/or (iii) extent (see Supplementary Table S2). We recorded any changes in the protective effect of forests due to the global change impacts revealed by the studies, classifying them in five categories (increase, decrease, no change, scenario-dependent, inconclusive). In case different scenarios were considered in a study, we determined the forest changes and their impacts on the protective effect for each scenario. Additionally, the methodologies used by the studies to assess a change in the protective effect were characterized (field-based/empirical; statistical modeling; numerical/process-based modeling; experimental; expert estimation; participatory studies; review) and the variables used to characterize the change were assessed. It was then evaluated whether these were (i) qualitative or quantitative, and (ii) directly associated with the different effects of forests on natural hazards (i.e., effect on the frequency, magnitude/intensity, or risk of the hazard), or rather related to forest structure and thus only indirectly to the protective effect. The variables and methods used were analyzed per hazard type. When available, the quantitative changes in protective effect were extracted (see summary Supplementary Table S1 and full review table: https://doi.org/10.5281/zenodo.8232212).
In a final step, the different categories of global change impact were analyzed in more depth to detect differences, generalities, and potential interactions between the different global change impacts. In particular, we assessed how protective forests at different elevations were affected by climate change, and how different types of management and disturbances influenced their protective effect. Due to the wide range of different variables used to quantify the changes in the protective effect, as well as the different temporal and spatial scales analyzed by the studies, the quantitative results of different studies could not be summarized in a quantitative analysis, so we focused on the direction of changes reported in the reviewed studies.
3. Results
3.1. General overview
The first publications assessed in this review were from 2000. The number continuously increased with two peaks in 2017 (10 publications) and in 2021 (11 publications). Anthropogenic - and natural disturbance-related impacts on the protective effect of forests were addressed throughout the observation period (any time until August 4, 2022), while the first publication looking at climate-induced forest change was published in 2009, followed by more publications in 2013 (Figure 2). A clear increase was observed in studies about the impacts of natural disturbances, which may be related to the emerging research following unprecedented bark beetle outbreaks in high elevation forests in the United States since the early 2000s (Bentz et al., 2010; Logan et al., 2010) or the big storm events in Central Europe in the 1990s (Vivian and Lothar; Wohlgemuth and Brang, 2015). Overall, the majority of studies addressed anthropogenic impacts on protective forests (74%), followed by effects of natural disturbances (38%) and climate-induced forest change (28%), whereas about one third of the studies looked at coupled/interacting effects. The studies were predominantly carried out in Europe, followed by North America and Asia. Only two studies looked at global change impacts on forests’ protective effect in South America, and one in Africa (Figure 3).
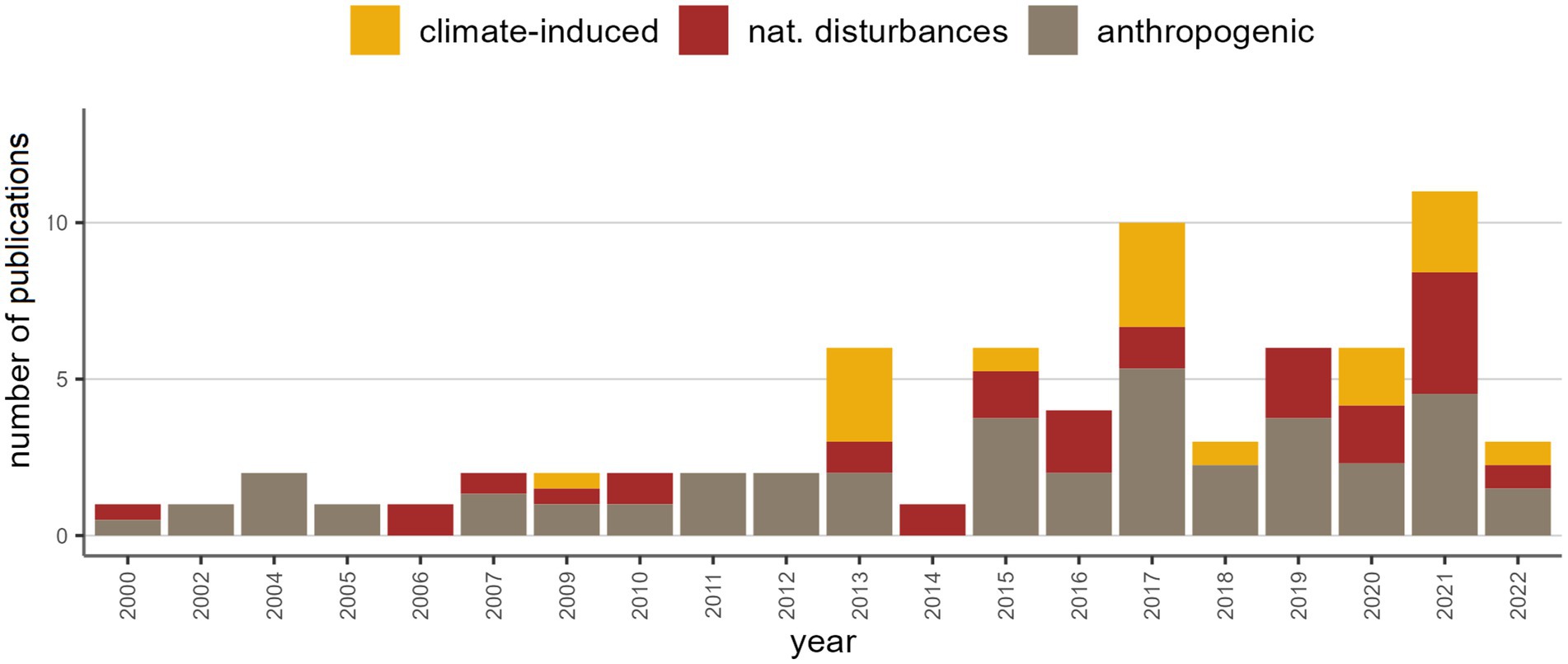
Figure 2. Proportion of publications (published by August 4, 2022) per year, divided by global change impact category. Climate-induced: change of forest extent, structure or composition due to direct impact of changing air temperature and/or precipitation; nat. disturbances: impact from (changing) natural disturbance regimes; anthropogenic: forest change due to direct anthropogenic influence such as management interventions or land-use changes.
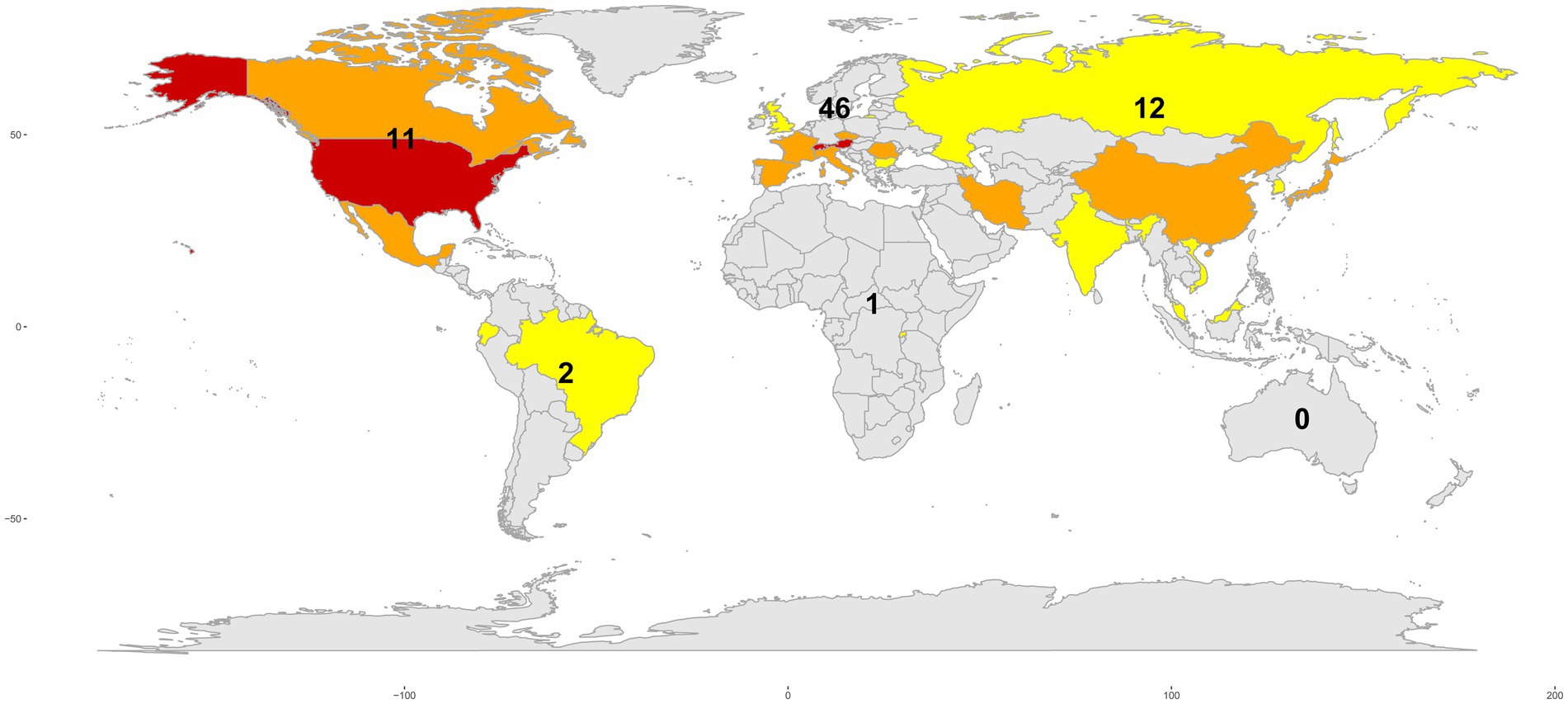
Figure 3. Number of reviewed publications per continent. The countries where the studies were conducted are shown in yellow (1 study only), orange (2–4 studies), and red (≥ 5 studies).
Approximately one third of the studies investigated the protective effect against rockfall, landslides and/or snow avalanches, while 43% addressed floods and only two studies addressed debris flow. Most studies were based on quantitative methods (82%), a large proportion of which used numerical or process-based modeling to assess global change impacts (56% of total studies; Figure 4). Among these, commonly used models include forest simulation models, such as iLand (Scheidl et al., 2020), PICUS (Maroschek et al., 2015; Pardos et al., 2017; Zlatanov et al., 2017; Irauschek et al., 2017a,b), MASSIMO (Mathys et al., 2021), TreeMig (Moos et al., 2021) and ForClim (Elkin et al., 2013; Mina et al., 2017; Thrippleton et al., 2020), as well as process-based hydrological models (e.g., Seibert et al., 2010; Versini et al., 2013; Surfleet et al., 2014). However, forest simulation models and process-based models of natural hazards are only rarely combined (but see, e.g., Scheidl et al., 2020; Moos et al., 2021). Field-based or empirical data were used in 32% of the reviewed studies, while the rest applied statistical modeling (20%) or was based on expert estimation, review, participatory methods, or experiments (23%). The field-based / empirical studies and those using statistical modeling predominantly assessed the protective effect against floods, while the studies using numerical models mainly focused on rockfall, followed by avalanches, landslides, and floods in equal proportion.
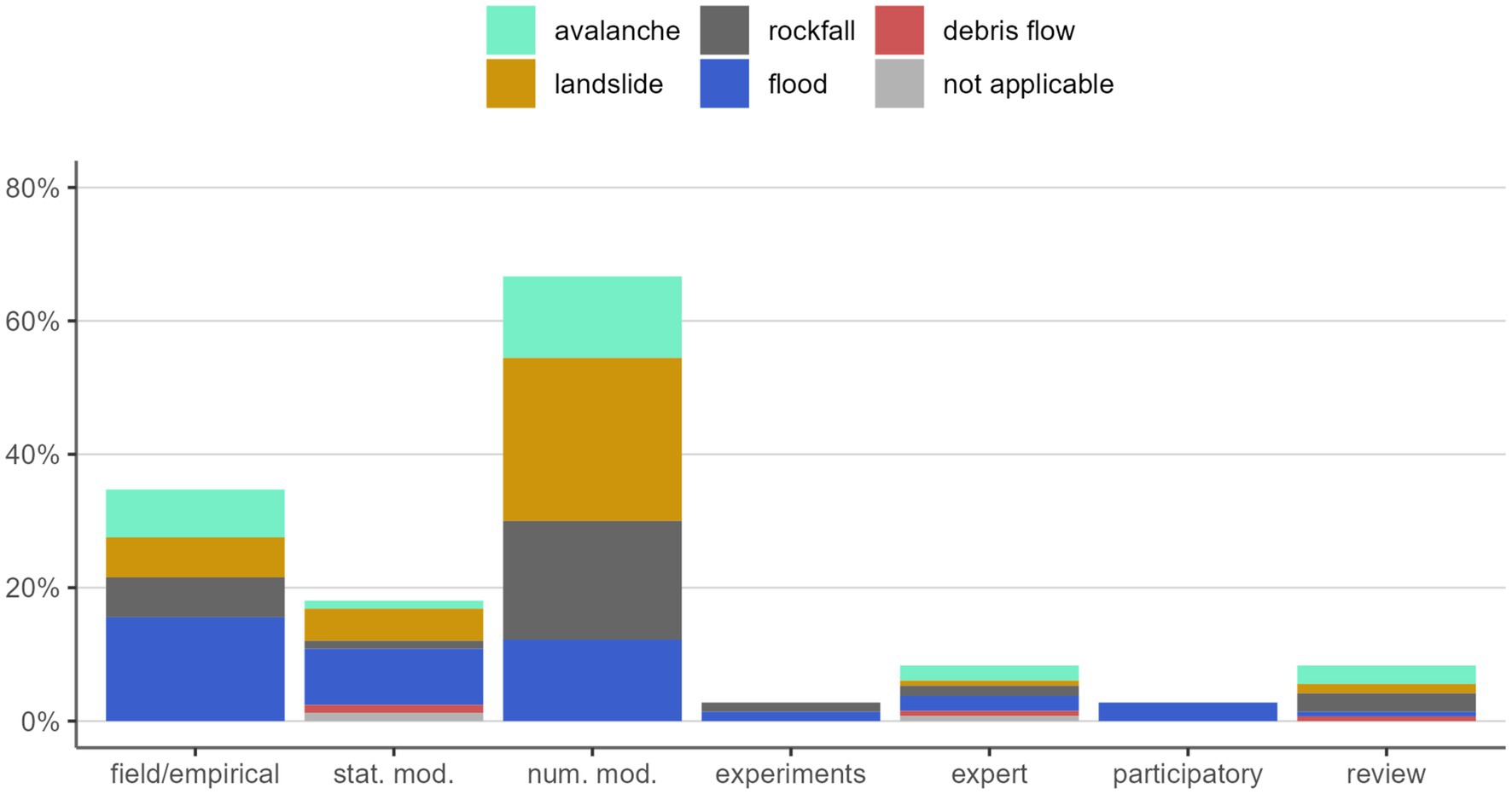
Figure 4. Methods used in the studies to assess the change in the protective effect of forests per natural hazard type (total number of studies = 72; multiple methods per study possible). Stat. mod. = statistical modeling; num. mod. = numerical/process-based modeling; expert = expert estimation.
A mere 15% of the studies took into account the risk posed by natural hazard processes (i.e., potential consequences). Among these, only about two thirds (n = 7) utilized a quantitative, or at least partly quantitative, measure of natural hazard risk (Figure 5, d). Four of these publications treated the protective effect of forests against rockfall (Farvacque et al., 2019; Moos et al., 2019a,b, 2021) two against avalanches (García-Hernández et al., 2017; Stritih et al., 2021) and one against floods (Bhattacharjee and Behera, 2018). Most of the publications addressing floods used a measure of process intensity/magnitude to assess the protective effect, the majority of which was “peak flow” (Figure 5, c). A large proportion of the studies dealing with rockfall, avalanches and landslides assessed the protective effect indirectly based on forest structure characteristics. Mostly, they used a protective forest index (Figure 5, a) based on different combinations of forest parameters such as stem density and species composition. Most landslide studies used a measure of hazard frequency (e.g., landslide susceptibility or event frequency; Figure 5, b).
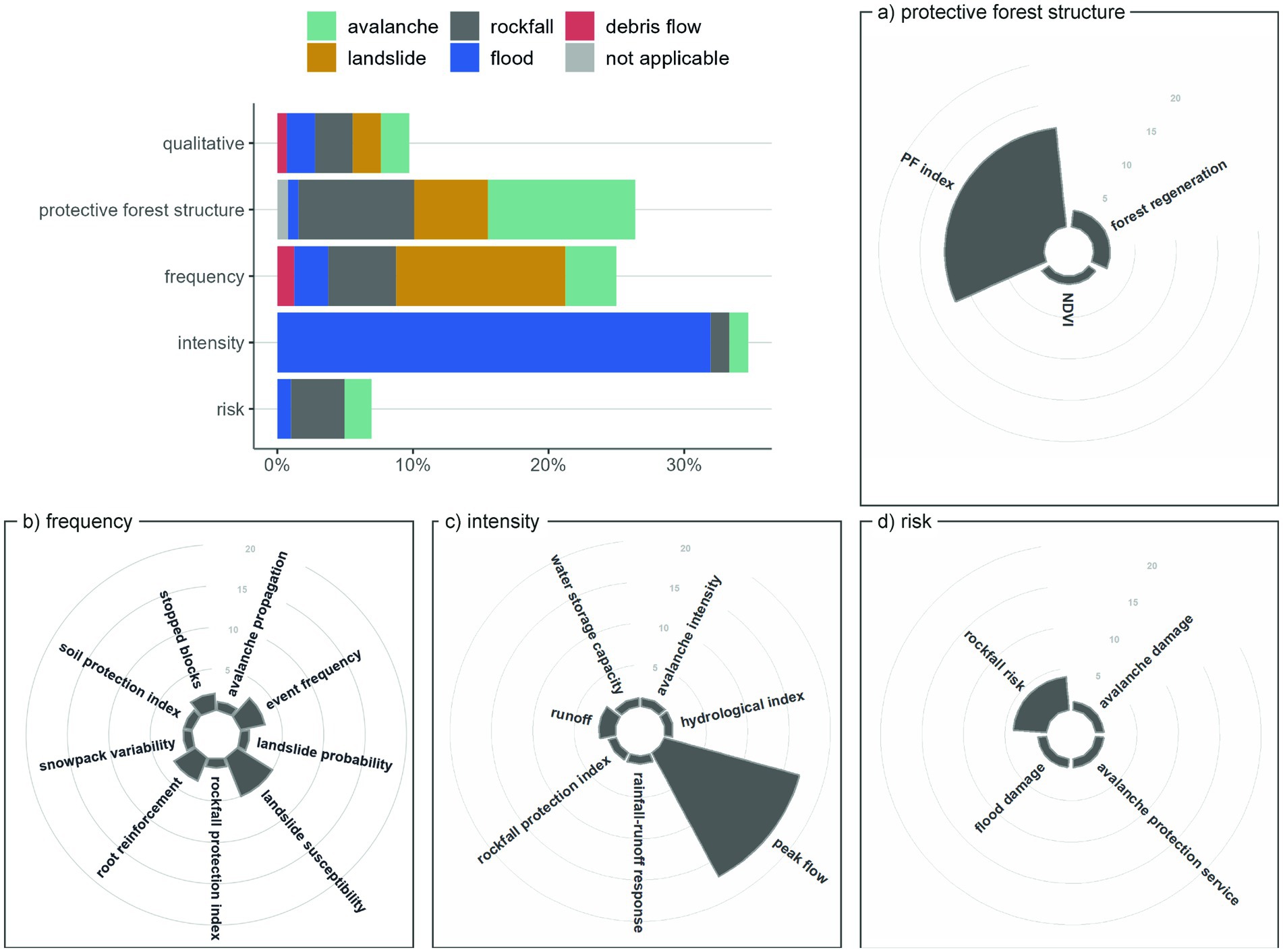
Figure 5. Upper left panel: proportion of all reviewed publications that assessed the impacts of global change on the protective effect qualitatively (“qualitative”) or quantitatively based on the protective forest structure or a component of natural hazard risk (i.e., frequency, intensity/magnitude, or risk). Panels a-d: number of publications per target variable that assessed global change impacts on forests’ protective effect with regard to protective forest structure, natural hazard frequency, intensity/magnitude or risk. PF index: “protective forest index” based on specific combinations of forest parameters; NDVI: Normalized Difference Vegetation Index; forest regeneration: combination of multiple parameters characterizing forest regeneration (e.g., regeneration density or height).
About half of the studies (52%) solely addressed the protective service of forests, while the others investigated multiple ecosystem services (13% wood production, 14% biodiversity, the remaining climate regulation, and water and soil services).
3.2. Climate-induced forest change
Almost one third of the publications (n = 19) considered the impacts of climate-induced forest changes on the protective effect. They all addressed changes in forest composition or structure, and only two addressed a change in forest extent (Bebi et al., 2009; Lingua et al., 2020). Most of these studies (n = 16; reviews excluded) were “predictive,” meaning that they assessed potential future forest changes. Observation-based studies examining the effect of past and current climate change on the protective effect were generally lacking. Borgniet et al. (2013) found a decrease in the protective effect of forests in the Aosta Valley (Italy) between 2000 and 2009 due to a dieback of Pinus sylvestris based on remote sensing data, while Lange et al. (2013) used a space-for-time approach to assess how climate-driven changes in species composition might affect flood-protective forests.
More than half of the “predictive” studies (n = 12) used dynamic forest simulation models to simulate future forest development under different climate scenarios (e.g., Mina et al., 2017; Irauschek et al., 2017b; Jandl et al., 2018; Moos et al., 2021). These modeling studies assessed the change in the protective effect mostly using protective forest indices of forest structure, rarely quantifying the forest’s effect on the hazard. However, Scheidl et al. (2020), for example, combined the dynamic forest model iLand (Seidl et al., 2012) with the hydrological model GEOtop (Rigon et al., 2006) and a slope stability model, while Moos et al. (2021) coupled the dynamic forest model TreeMig (Lischke et al., 2006) with a rockfall simulation model (RockyFor3D, Dorren, 2016) to predict changes in rockfall risk.
Numerous studies concur that rising air temperatures stimulate forest growth, thereby enhancing the protective role of forests at high elevations (Figure 6; e.g., Elkin et al., 2013; Mina et al., 2017; Pardos et al., 2017; Irauschek et al., 2017a,b). Furthermore, forest expansion at and above the current treeline will also likely contribute to an increased protective effect (Bebi et al., 2009). On the other hand, more frequent and severe droughts may lead to a decrease in the protective effect at lower elevations due to a decrease in growing stock caused by increased mortality and reduced growth (e.g., Elkin et al., 2013; Mina et al., 2017; Zlatanov et al., 2017; Moos et al., 2021; Figure 7). Based on the assessed studies, which predominantly originate from Europe, positive effects of increasing air temperature can generally be expected for elevations >1,500 m a.s.l., and negative effects for elevations <1,000 m a.s.l. In-between, climate change effects depend on local conditions and the climate scenario (Figure 6). Furthermore, Maroschek et al. (2015) and Irauschek et al. (2017b) reported counterbalancing effects of climate warming on tree growth (increase in protective effect) and increasing natural disturbances (decrease in protective effect). A few studies did not find a significant change in the protective effect due to climate-induced forest cover change (e.g., Irauschek et al., 2017a in the Alps and Mina et al., 2017 in the Iberian Mountains).
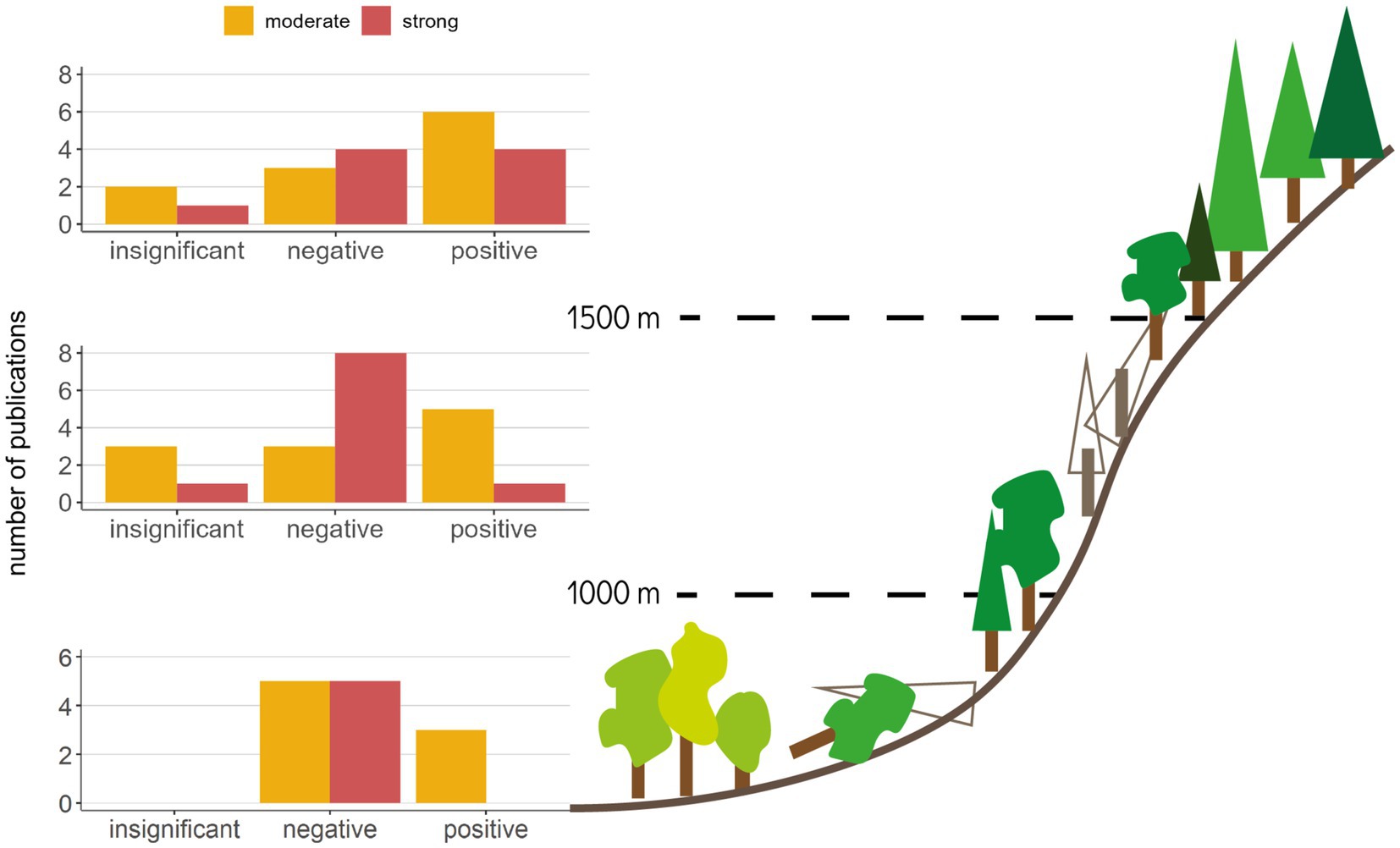
Figure 6. Number of publications reporting an overall positive, negative, or insignificant effect of climate-induced forest changes on the protective effect below 1,000 m a.s.l., between 1,000 and 1,500 m a.s.l. and above 1,500 m a.s.l. and for moderate (orange) and strong (red) climate change. Moderate climate change includes climate scenarios with a mean air temperature increase of ~1.5–3.5°C and no or a slight precipitation decrease of ~10–20%. Strong climate change scenarios foresee a mean air temperature increase of ~3.5–6°C and a significant precipitation decrease of ~20–40%. Overall, 14 studies reporting quantitative results were considered (reviews excluded). They originate mainly from Europe [Austria (n = 5), Bulgaria (n = 1), Italy (n = 1), Romania (n = 1), Spain (n = 2), Switzerland (n = 5)].
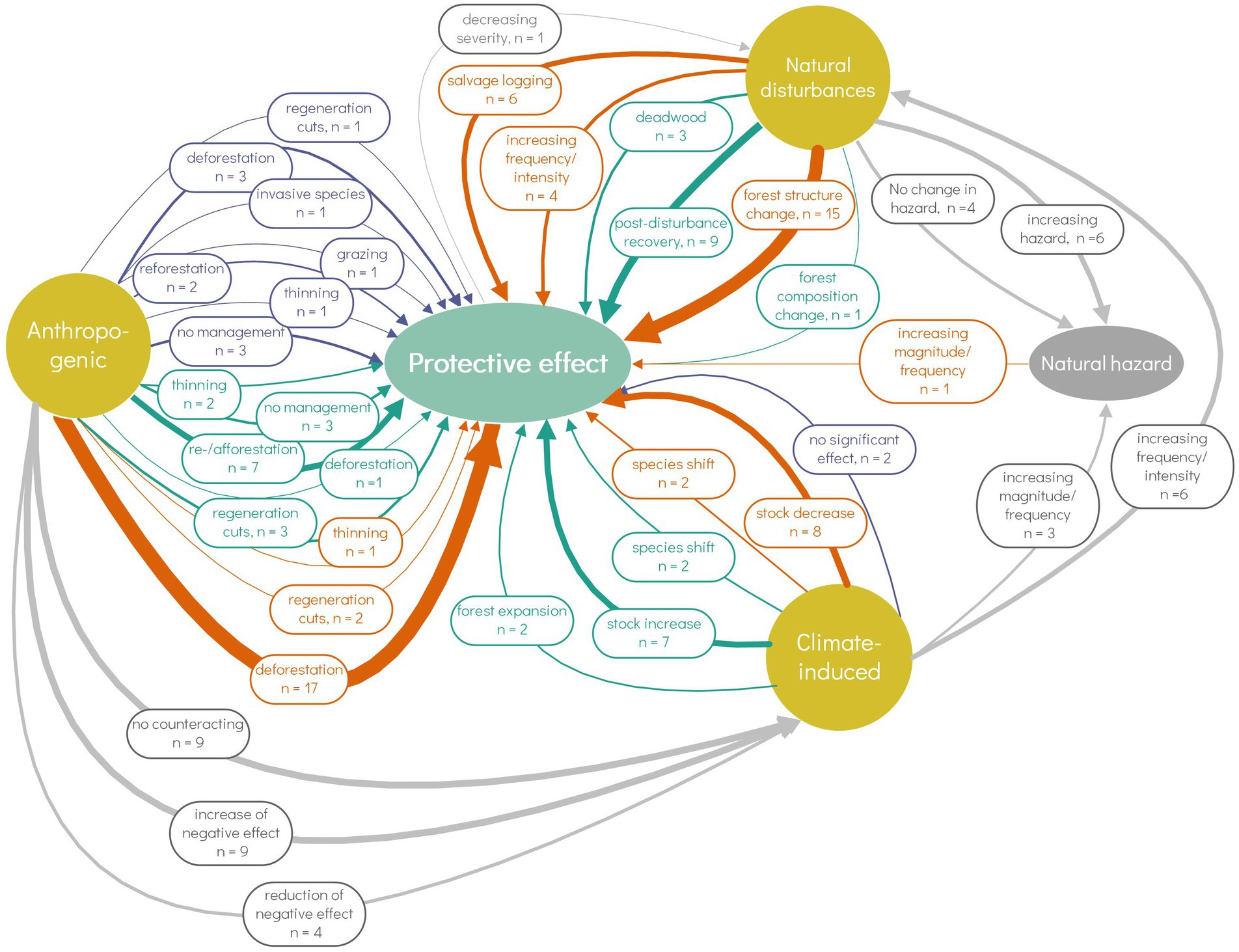
Figure 7. Summary of detected global change impacts on the protective effect of forests against natural hazards as well as relations/interactions between the three main impact categories “anthropogenic forest change,” “climate-induced forest change” and “natural disturbances” and/or the natural hazard. The “n” indicates the number of studies reporting a certain impact (publications are counted multiple times, if multiple scenarios were analyzed in one study). Orange arrows: “impact decreased protective effect”; green arrows: “impact increased protective effect”; purple arrows: “no or insignificant impact”; gray arrows: “relation between impact categories”.
3.3. (Changing) natural disturbances
Among the reviewed publications, 26 addressed how forests’ protective effect against natural hazards is affected by natural forest disturbances (such as forest fires, windthrows, and bark beetle outbreaks, see Figure 8). Natural disturbances often cause a decrease in the protective effect for years to decades after the disturbance. The impact depends on the severity of the disturbance (e.g., the level of tree mortality or the degree of change in forest structure), which was, however, often not explicitly quantified in the reviewed studies. Among different disturbance agents, forest fires were addressed most often (11 studies). Forest fires have been found to reduce forests’ flood protective effect, which can lead to increased peak flows. These effects were often studied using a combination of process-based hydrological models and observational data, with observed post-fire peak flows up to 120% higher compared to modeled pre-fire conditions (Seibert et al., 2010). However, the effects of fires on peak flows were highly variable, sometimes with no clear effects in large watersheds (Buttle and Metcalfe, 2000) or in case of low-to-moderate fire severity (Surfleet et al., 2014). Nonetheless, climate change and increased forest fire risk are expected to lead to increased flood risk, e.g., in Mediterranean watersheds (Versini et al., 2013).
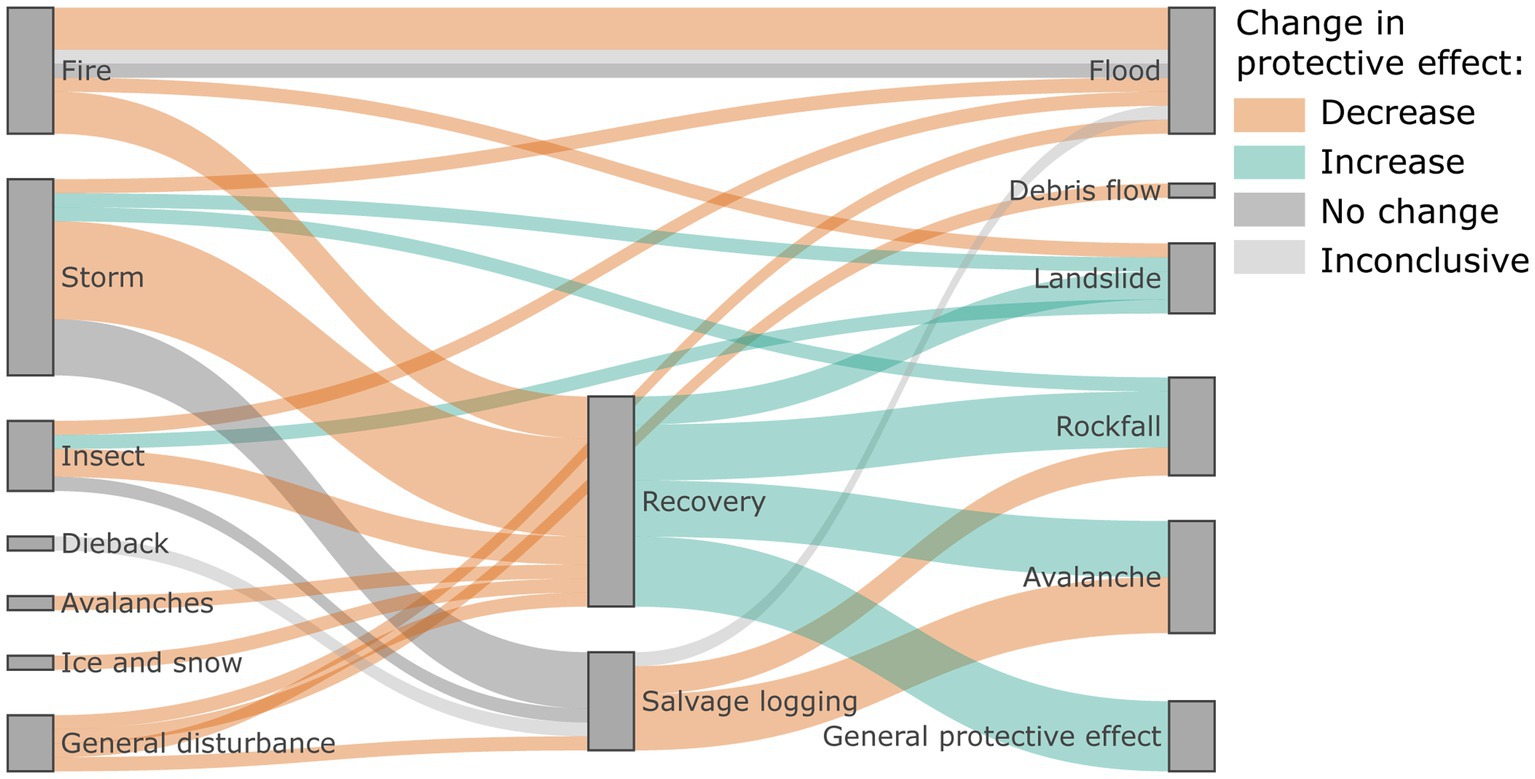
Figure 8. Impacts of natural disturbances (left) on the protective effect against different types of natural hazards (right), mediated by processes of salvage logging and forest recovery. The width of the connections corresponds to the number of studies that examined a specific link, where studies examining multiple effects or scenarios are represented multiple times in the figure.
After windthrow and bark beetle disturbances, management decisions had an important impact on the post-disturbance protective effect. If left in the stand, deadwood could maintain forests’ protective effect after a disturbance (Rammig et al., 2007; Wohlgemuth et al., 2017; Teich et al., 2019; Costa et al., 2021; Caduff et al., 2022), especially during the first 15 years (Caduff et al., 2022). Rockfall simulations have shown that lying deadwood even increased forests’ protective effect (Costa et al., 2021). In contrast, salvage logging after a disturbance could further impair forests’ protective effect (Leverkus et al., 2021).
Several studies addressed the development of forests’ protective effect over time after disturbances, especially in case of insect, wind, and fire disturbances in the European Alps. For example, Caduff et al. (2022) surveyed sites affected by windthrow and bark beetles using remote sensing data and used avalanche simulations to assess the disturbed forests’ protective effect, which was lowest after deadwood was removed or decayed, approximately 10–15 years after disturbance (Caduff et al., 2022). Approximately 25 years after windthrow events, the protective effect was still judged to be insufficient based on field surveys and expert assessment (Wohlgemuth et al., 2017), while both field observations combined with rockfall simulations (Maringer et al., 2016) and forest simulation models indicate that the protective effect will recover within 40–50 years after disturbance (Rammig et al., 2007; Moos et al., 2019a). On longer timescales, natural disturbances can have a positive influence on forests’ protective effect. For example, Scheidl et al. (2020) found that wind and bark beetle disturbances can contribute to slope stability over 50–200 years by facilitating a compositional shift from Picea abies toward species with deeper rooting systems (Figure 7).
Most studies on natural disturbances focused on effects at rather local scales, from single disturbed sites (especially in the case of windthrow and bark beetle) to watersheds (particularly for flood-related studies). At larger (national) scales, regularly occurring disturbances (i.e., press disturbances, including anthropogenic disturbances such as clearcutting) have been shown to have a stronger effect on the occurrence of natural hazards (flood, debris flow) than more sporadic pulses of natural disturbances (Sebald et al., 2019).
3.4. Anthropogenic forest change
Impacts of anthropogenic forest changes on the protective effect of forests were related to land-use changes (deforestation including clear-cuts, afforestation of previously non-forested areas, and reforestation often related to the abandonment of pastures), management interventions (thinning, regeneration cuts, coppicing, and afforestation) and other human-related activities (e.g., forest grazing, introduction of invasive species, or reduced/no management activities; see Figures 7, 9). Most of the studies on anthropogenic forest change centered around deforestation, including partial clear-cuts (n = 21). “No management” was the second most studied determinant of change (n = 11), followed by regeneration cuts (n = 9) and thinning (n = 7; including different thinning methods, such as thinning from above, from below or free thinning). Reforestation (n = 6), afforestation (n = 3), and coppicing (n = 1) were addressed only by a few studies. One paper examined the effect of forest grazing (Mayer and Stöckli, 2005), another one the spread of invasive species (Moos et al., 2019b), and one reported anthropogenic forest change of unknown nature (Lim and Kim, 2022). Several studies (e.g., Maroschek et al., 2015; Pardos et al., 2017; Irauschek et al., 2017b; Thrippleton et al., 2020; Mathys et al., 2021) used forest simulation modeling approaches (e.g., using models like PICUS, ForClim, iLand, MASSIMO) to investigate the effect of anthropogenic forest change on the protective effect against natural hazards, solely or in interaction with moderate or strong climate scenarios.
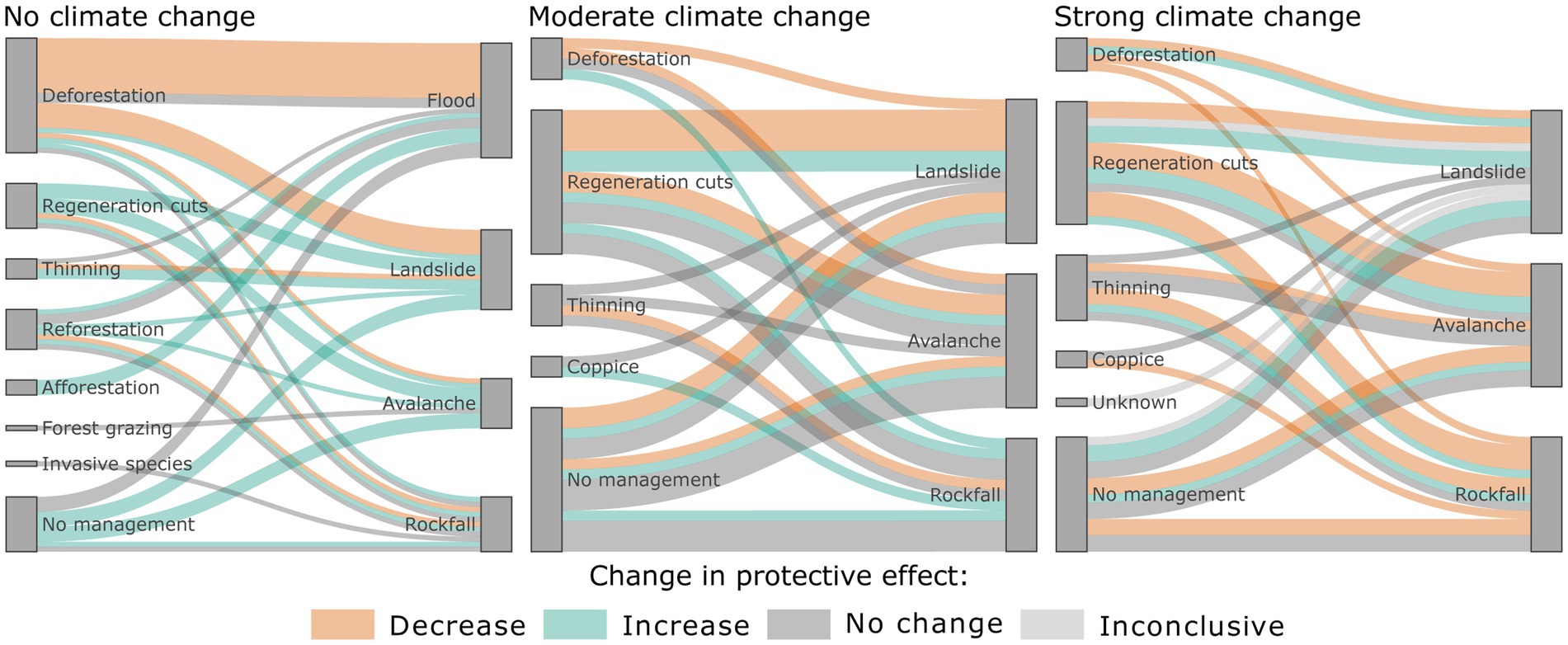
Figure 9. Impacts of anthropogenic forest change (left side of the panels) on the protective effect against different types of natural hazards (right side of the panels), under different climate change scenarios (no, moderate, and strong climate change). Specific management interventions were grouped into macro-categories, such as “thinning” or “regeneration cuts.” The width of the connections corresponds to the number of studies/scenarios that examined a specific link, where studies examining multiple natural hazards or scenarios are represented multiple times. Moderate climate change includes climate scenarios with a mean air temperature increase of ~1.5–3.5°C and a slight precipitation decrease of ~10–20%. Strong climate change scenarios foresee a mean air temperature increase of ~3.5–6°C and a significant precipitation decrease of ~20–40%.
Deforestation was mainly analyzed with respect to floods and landslides and had generally a negative impact on the protective effect provided by forests, independently of climate change (Figure 9). One study (Irauschek et al., 2017b), however, reported a positive effect of logging (in this case, clearcuts concentrated on 5,000 m2 strips) on the protective effect against landslides, avalanches, and rockfall under no climate change to moderate climate change scenarios, and especially when followed by artificial regeneration.
Other anthropogenic changes were mostly related to forest management and climate interactions, and their effects were strongly dependent on the considered climate change scenarios. Without climate change, “no management” mostly increased the protection against natural hazards. However, under moderate and strong climate change scenarios, negative effects of “no management” were found for landslides, avalanches, and rockfall (Irauschek et al., 2017b; Thrippleton et al., 2020). Regeneration cuts and thinning had a contrasting influence on the protective effect of forests against natural hazards under different climate scenarios (Figure 7). Positive effects of these management interventions were most often reported under no climate change, with negative effects becoming more predominant under moderate and strong climate change scenarios. However, even under strong climate change scenarios, several studies reported a relative positive influence of clearcuts (Irauschek et al., 2017b), regeneration cuts (Irauschek et al., 2017a,b; Mathys et al., 2021), thinning (Mathys et al., 2021), and the omission of management (Irauschek et al., 2017a,b) on forests’ protective effect (Figure 9), further highlighting the complexity of the interactions between climate change and direct anthropogenic influences on protective forests (Figure 7).
4. Discussion
The publications assessed in this review reported a wide range of global change impacts that were directly linked to potential changes in the protective effect of forests against natural hazards. On the one hand, a decline in forest growing stock due to more frequent and severe disturbances, drought-related mortality, or growth decrease, as well as due to land-use change or intensive management is expected to reduce the protective effect in the long term. On the other hand, climate change-related growth increase at high elevations, re−/afforestation as well as post-disturbance deadwood legacies and recovery can positively affect protective forests. Additionally, forest interventions aimed at promoting regeneration can in some cases increase the protective effect. Overall, most of the reviewed studies report at least partly negative effects of global change on the protective effect of forests against natural hazards, especially under exacerbating climate change scenarios.
Our review shows that evidence on global change impacts on the forests’ protective effect is relatively vague and associated with high uncertainties. In particular, impacts of changing air temperature and precipitation regimes were almost exclusively analyzed using simulation models, while empirical evidence is still largely missing. This is, however, to be expected, since the global air temperature increase is a rather recent phenomenon-at least in relation to the growth rate of trees-and forest ecosystems react to it in complex ways (e.g., Camarero et al., 2015). Likewise, studies on climate-induced changes have only been emerging in the past 10 years. Studies on the effects of natural disturbances and anthropogenic impacts on protective forests, on the other hand, date back somewhat longer and are characterized by a greater proportion of empirical analyses. These often include the combination of data-based analyses and reconstruction of the protective forest’s change with a simulation-based assessment of its protective effect, as for example in a case study on impacts of land-use change on rockfall risk conducted by Farvacque et al. (2019). Empirical evidence on the development of the protective effect is scarce, as direct field measurements of forests’ effect on, e.g., avalanches or rockfall are difficult to obtain (Ringenbach et al., 2022), and long data series on hazard events (e.g., García-Hernández et al., 2017) are rarely available (Perzl and Teich, 2022). Furthermore, the reviewed studies cover a large variety of different forest types and natural hazard dispositions, making general conclusions difficult.
Overall, the geographic distribution of the locations of the studies is very uneven, reflecting high research funds and data availability in Europe as well as the long tradition and institutional embedding of protective forest management mainly in Alpine countries. Similar geographic biases have also been shown by recent studies in the field of nature-based solutions (e.g., Sudmeier-Rieux et al., 2021; Nehren et al., 2023). A closer look at the network of the authors of the reviewed publications shows also a very strong connection of the authors of studies on avalanche, landslide and rockfall protective forests mainly in the European Alps, while flood-related studies are geographically more distributed and their authors less connected (Supplementary Figure S2).
Only a few studies assessed how treeline shifts affect the protective effect of forests, which is surprising considering the vast amount of literature available on treeline shifts in mountains (e.g., Smith et al., 2009; Cudlin et al., 2017; Zindros et al., 2020). This may point out a potential limitation of our search protocol or, more likely, suggest that treeline research has hardly been directly linked to the consequences of treeline shifts for the forest’s protective effect, which was one of the eligibility criteria for the inclusion of a publication in this review. The maintenance and recovery of high-elevation protective forests was a driver of recent treeline research (Holtmeier and Broll, 2019), but apparently without assessment of the direct impacts on their protective effect.
Many studies assessed potential changes in the protective effect based on protective forest indices (e.g., related to forest cover density or basal area). Only a few studies tried to quantify the effects of changes in the protective forests on the risk for people and infrastructure in terms of avoided costs (e.g., Farvacque et al., 2019; Stritih et al., 2019; Moos et al., 2021). However, a quantification of the monetary consequences of global change impacts on protective forests would facilitate decisions on financial and organizational resources required to ensure the durability of protective forests (Dorren and Moos, 2021). This is of particular importance when it comes to the question whether additional technical protection measures are required to compensate for potential losses in the protective effect, where a comparison based on cost–benefit analysis can support decision-making (Alves et al., 2016; Onuma and Tsuge, 2018). Besides changes in their capacity to provide protection, the value of the protective service provided by forests can also be affected by changes on the demand side. For example, land-use change in downstream areas can lead to an increase in population or infrastructure exposed to natural hazards (Tran et al., 2010), thus augmenting the value of protective forests. However, this component of risk is often not explicitly considered in studies on forests’ protective effect.
4.1. Implications for protective forest management
The reviewed studies on anthropogenic forest changes mostly focused on impacts at the opposite ends of the management and land-use spectrum-deforestation and no management. Deforestation mostly had a negative impact on the protective effect of forests, particularly against floods and landslides (e.g., Munoz-Villers and McDonnell, 2013; De Aguiar et al., 2018; Malek et al., 2018; Hajian et al., 2019). The process of deforestation can initiate complex, non-linear relationships between the biosphere and atmosphere by changing the hydrological conditions of a site (i.e., water storage capacity, interception, or infiltration; Ellison et al., 2012; Lima et al., 2014). This, in turn, can have a cascade of negative effects on ecosystem services, such as protection against floods and landslides (e.g., Du et al., 2016; Lopez-Ramirez et al., 2020).
Interventions such as thinning and regeneration cuts were also examined by the reviewed studies but showed contrasting impacts on the protective effect of forests against natural hazards, not allowing us to draw general conclusions for the future management of protective forests. On the one hand, this highlights the complexity of the effects of different management interventions, interacting with a changing climate, on the protective effect of forests. On the other hand, it also suggests a strong local context dependency that shapes these interactions. Environmental context dependency is a known phenomenon in ecological research, e.g., on drought stress, biodiversity, or ecosystem functioning, where site characteristics may modulate responses depending on the environmental context (e.g., Forrester et al., 2016; Ratcliffe et al., 2017). Reduced management intensity, for example, was found to improve the protective effect of forests by increasing stand basal area; however, the same management may lead to higher predisposition to disturbances and subsequent risks for the protective effect of forests (Mathys et al., 2021, Switzerland). Reduced harvest intensity improved landslide prevention in conifer plantations in Japan (Yamaura et al., 2021). Regeneration cuts were found to have contrasting results on the protective effect of forests in different sites (e.g., negative effect in Quercus and Pinus nigra mixed forests in the French Alps, Bigot et al., 2009; positive effect in Picea abies-dominated forests in western Austria, Irauschek et al., 2017a; negative to non-significant effects in key forest types and governance settings in the main mountain ranges of central and southern Europe, Mina et al., 2017) but also within the same study area (in mixed broadleaf and conifer forests in western Austria, Irauschek et al., 2017b). Although mountain landscapes have a high level of spatial heterogeneity, this variability is often not explicitly considered in the analyzed studies, making it difficult to draw definite conclusions on the impact of different local factors that may have contributed to variations in forests’ protective effect.
Additionally, besides being case-specific, most studies used modeling approaches to investigate the effect of anthropogenic forest changes and climate change scenarios on forests’ protective effect. The assumptions used by different models, as well as limitations related to the sometimes not thorough descriptions of the investigated processes, add uncertainties to an already complex system and make generalizations harder. The strong context dependency and uncertainty about changes in protective forests highlights the need for adaptive forest management, where forest managers need to monitor ongoing changes, learn from these observations, and adapt their management accordingly (Innes et al., 2009).
Among different types of management interventions, the reviewed studies were most consistent about the impact of salvage logging. Most studies that addressed salvage logging after natural disturbances identified a detrimental effect on the protective effect of forests against avalanches and rockfall (e.g., Rammig et al., 2007; Wohlgemuth et al., 2017). As natural disturbances become more frequent under climate change, it will be increasingly important to question the common practice of salvage logging, which has a negative effect on many ecosystem services (Leverkus et al., 2021).
In the face of climate change, management strategies such as thinning and the promotion of regeneration have been increasingly recommended to cope with drought, promote resilience and assure the continuity of forests and the ecosystem services they provide (Bottero et al., 2017; Albrich et al., 2020; Zhang et al., 2023). Our review highlights that understanding in which situations these management strategies may have a negative impact on the protective effect of forests is pivotal to improve the sustainable management of protective forests in mountain areas.
4.2. Implications for future research
The scarce empirical evidence of the effects of global change on protective forests demonstrates the need for increasing efforts in the monitoring of protective forests and their protective effect, as well as the collection and provision of high-quality data on global change impacts at different spatial and temporal resolutions (Ruiz-Benito et al., 2020). Understanding the highly context-specific ways in which different aspects of global change affect protective forests requires in-depth analyses at the local scale. This includes, for example, the long-term monitoring of the effects of management interventions and natural disturbances or the retrospective analysis of the development of the forests’ protective effect depending on the forest condition (e.g., Rickli et al., 2019).This could include, for example, long-term monitoring plots of forest recovery and the dynamics of the protective effect after natural disturbances and under different (controlled) local conditions (e.g., Winter et al., 2015; Vergani et al., 2017). Such monitoring efforts will help designing and further developing modeling approaches that explicitly address protective forests and the interacting effects of global change impacts, as for example proposed by Schmid et al. (2023). Local-scale studies currently use a wide range of indicators and methods to measure forests’ protective effect (see Figure 5), which limits their comparability and possibility to conduct meta-analyses across sites. Using consistent indicators (e.g., Blattert et al., 2017) to describe protective forest structure and its impacts on hazard frequency, intensity, and risk, would make it easier to identify potential general patterns in protective forest changes across sites.
The growing accessibility of regional and even global datasets will facilitate the investigation of these processes at a larger scale, an area that is currently clearly underrepresented in research. Most of the analyzed studies here were, in fact, restricted to the slope or watershed scale, while regional, national, or even global studies represented a minority or were even completely lacking for flood protective forests (Supplementary Figure S2). Recent advances in large-scale mapping of forest disturbances over time (e.g., Senf and Seidl, 2021) and their effects on forest structure (Stritih et al., 2023) will facilitate assessments of how changing disturbances may alter the protective effect of forests. However, measuring the protective effect of forests using remote sensing data remains a complex and challenging task. Most of the protective forest indices used to link forest structure to the protective effect (see, e.g., Elkin et al., 2013; Irauschek et al., 2017a,b; Pardos et al., 2017) are based on below-canopy structural characteristics, such as stem density and tree diameter, while remote sensing studies more often provide information on canopy cover (e.g., Vacchiano et al., 2016). Recent approaches linking remote sensing data on forest structure with natural hazard simulations (e.g., Bebi et al., 2021; Costa et al., 2021; Sidau et al., 2021) may help bridging the gap between large-scale observations of forest dynamics and local-scale studies on forests’ protective effect.
There is a need for scientific data not only on global change impacts on protective forests, but also on their implications for society. Carrying out comprehensive and long-term inventories of natural hazard events and related damages would enable the assessment of the actual effects of global change impacts on protective forests for communities. Additionally, approaches and tools allowing for a risk-based evaluation and prediction of the forest’s protective effect in future should be promoted and further developed. Furthermore, there should be a concerted effort to promote and further develop approaches and tools that allow a risk-based evaluation and prediction of the protective effect of forests in the future (e.g., Moos et al., 2018; Stritih et al., 2019; Chabba et al., 2022; Teich et al., 2022b).
A large knowledge gap prevails regarding the impacts of compound events on protective forests. These are a combination of multiple climate-related events, such as a drought event followed by a forest fire and a flood event, resulting in cascading, often very severe impacts (e.g., Kleinman et al., 2019; Bastos et al., 2021). To investigate the risks emerging from such compound events affecting protective forests, integrative approaches that combine different models, datasets, and expertise are necessary, along with applications to real cases (Leonard et al., 2014; Zscheischler and Seneviratne, 2017). Additionally, climate change is likely to affect the frequency and intensity of natural hazard events (Stoffel et al., 2014), which may in turn further influence the protective effect of forests.
5. Conclusion
Our review evinces that the impacts of climate change, increasing natural disturbances, and anthropogenic forest changes on the protective effect of mountain forests against natural hazards are manifold and complex. Climate-induced changes are expected to increase forests’ protective effect at higher elevations but reduce it at lower elevations, while the impacts of anthropogenic changes, including various management interventions, are highly dependent on the local context and on interactions with climate change. Natural disturbances can decrease the protective effect of forests and this impact is further exacerbated by salvage logging. However, forests are likely to recover their protective effect several years to decades following disturbance, depending on disturbance severity and local site conditions. Most research on protective forests under global change is case-study specific and based on simulation modeling, while empirical evidence remains scarce. We therefore conclude that we need (i) increasing monitoring efforts of global change impacts on protective forests at the large scale; (ii) detailed local-scale assessments of interacting and compound effects as well as (iii) risk-based evaluations of changes in the protective effect of forests to support a pro-active and sustainable management of these extremely valuable nature-based solutions.
Author contributions
CM: conceptualization, literature search, literature review, data analysis, visualization, and writing first draft. AS: conceptualization, literature review, visualization, and writing. MT and AB: conceptualization, literature review, and writing and editing. All authors contributed to the article and approved the submitted version.
Funding
This research was supported by the Interreg Alpine Space Programme project MOSAIC co-funded by the European Union (grant number ASP0100014). AS further received funding from the Bavarian State Ministry for Food, Agriculture and Forestry (StMELF) in the frame of the project “Risikoanalyse Gebirgswald Bayern”.
Conflict of interest
The authors declare that the research was conducted in the absence of any commercial or financial relationships that could be construed as a potential conflict of interest.
Publisher’s note
All claims expressed in this article are solely those of the authors and do not necessarily represent those of their affiliated organizations, or those of the publisher, the editors and the reviewers. Any product that may be evaluated in this article, or claim that may be made by its manufacturer, is not guaranteed or endorsed by the publisher.
Supplementary material
The Supplementary material for this article can be found online at: https://www.frontiersin.org/articles/10.3389/ffgc.2023.1223934/full#supplementary-material
References
Albrich, K., Rammer, W., and Seidl, R. (2020). Climate change causes critical transitions and irreversible alterations of mountain forests. Glob. Chang. Biol. 26, 4013–4027. doi: 10.1111/gcb.15118
Alves, A., Sanchez, A., Vojinovic, Z., Seyoum, S., Babel, M., and Brdjanovic, D. (2016). Evolutionary and holistic assessment of green-grey infrastructure for CSO reduction. Water 8:402. doi: 10.3390/w8090402
Bastos, A., Orth, R., Reichstein, M., Ciais, P., Viovy, N., Zaehle, S., et al. (2021). Vulnerability of European ecosystems to two compound dry and hot summers in 2018 and 2019. Earth Syst. Dynam. 12, 1015–1035. doi: 10.5194/esd-12-1015-2021
Bebi, P., Bast, A., Helzel, K. P., Schmucki, G., Brozova, N., and Bühler, Y. (2021). Avalanche protection forest maps: from process knowledge to interactive maps. In: protection forests as ecosystem-based solution for disaster risk reduction (eco-DRR).
Bebi, P., Kulakowski, D., and Rixen, C. (2009). Snow avalanche disturbances in forest ecosystems-state of research and implications for management. For. Ecol. Manag. 257, 1883–1892. doi: 10.1016/j.foreco.2009.01.050
Bentz, B. J., Régniére, J., Fettig, C. J., Hansen, E. M., Hayes, J. L., Hicke, J. A., et al. (2010). Climate change and bark beetles of the Western United States and Canada: direct and indirect effects. Bioscience 60, 602–613. doi: 10.1525/bio.2010.60.8.6
Bhattacharjee, K., and Behera, B. (2018). Does forest cover help prevent flood damage? Empirical evidence from India. Glob. Environ. Chang. 53, 78–89. doi: 10.1016/j.gloenvcha.2018.09.004
Bigot, C., Dorren, L. K. A., and Berger, F. (2009). Quantifying the protective function of a forest against rockfall for past, present and future scenarios using two modelling approaches. Nat. Hazards 49, 99–111. doi: 10.1007/s11069-008-9280-0
Blattert, C., Lemm, R., Thees, O., Lexer, M. J., and Hanewinkel, M. (2017). Management of ecosystem services in mountain forests: review of indicators and value functions for model based multi-criteria decision analysis. Ecol. Indic. 79, 391–409. doi: 10.1016/j.ecolind.2017.04.025
Borgniet, L., Toe, D., Berger, F., Galvagno, M., Panigada, C., Colombo, R., et al. (2013). “Monitoring climatic change impacts on protection forests in Aosta Valley (Italy) and in Drôme (France) using medium and high resolution remote sensing and Mateloscopes plots” in Management Strategies to Adapt Alpine Space Forests to Climate Change Risks. eds. G. Cerbu, M. Hanewinkel, G. Gerosa, and R. Jandl, vol. 261 (Paris: Books on Demand)
Bottero, A., D’Amato, A. W., Palik, B. J., Bradford, J. B., Fraver, S., Battaglia, M. A., et al. (2017). Density-dependent vulnerability of forest ecosystems to drought. J. Appl. Ecol. 54, 1605–1614. doi: 10.1111/1365-2664.12847
Brang, P., Schönenberger, W., Ott, E., and Gardner, B. (2001). “Forests as protection from natural hazards” in The forests handbook, volume 2: applying forest science for sustainable management. ed. J. Evans (Hoboken, NJ: Blackwell Science), 53–81.
Buttle, J. M., and Metcalfe, R. A. (2000). Boreal forest disturbance and streamflow response, northeastern Ontario. Can. J. Fish. Aquat. Sci. 57, 5–18. doi: 10.1139/cjfas-57-S2-5
Caduff, M. E., Brožová, N., Kupferschmid, A. D., Krumm, F., and Bebi, F. (2022). How large-scale bark beetle infestations influence the protective effects of forest stands against avalanches: a case study in the Swiss Alps. For. Ecol. Manag. 514:120201. doi: 10.1016/j.foreco.2022.120201
Camarero, J. J., Gazol, A., Sangüesa-Barreda, G., Oliva, J., and Vicente-Serrano, S. M. (2015). To die or not to die: early warnings of tree dieback in response to a severe drought. J. Ecol. 103, 44–57. doi: 10.1111/1365-2745.12295
Chabba, M., Bhat, M. G., and Sarmiento, J. P. (2022). Risk-based benefit-cost analysis of ecosystem-based disaster risk reduction with considerations of co-benefits, equity, and sustainability. Ecol. Econ. 198:107462. doi: 10.1016/j.ecolecon.2022.107462
Costa, M., Marchi, N., Bettella, F., Bolzon, P., Berger, F., and Lingua, E. (2021). Biological legacies and rockfall: the protective effect of a windthrown forest. Forests 12:1141. doi: 10.3390/f12091141
Cudlin, P., Klopčič, M., Tognetti, R., Máli, F., Alados, C. L., Bebi, P., et al. (2017). Drivers of treeline shift in different European mountains. Clim. Res. 73, 135–150. doi: 10.3354/cr01465
De Aguiar, W., Sampaio, S. C., Paisani, J. C., and Dos Reis, R. R. (2018). Implications for peak flows of the Marrecas river basin due to changes in the Brazilian forest code. Engenharia Agric. 38, 277–283. doi: 10.1590/1809-4430-Eng.Agric.v38n2p277-283/2018
*Depicker, A., Jacobs, L., Mboga, N., Smets, B., Van Rompaey, A., Lennert, M., et al. (2021). Historical dynamics of landslide risk from population and forest-cover changes in the Kivu rift. Nat. Sustain., 4, 965–974. doi: 10.1038/s41893-021-00757-9
Dorren, L. (2016). Rockyfor3D (v5.2) revealed–transparent description of the complete 3D Rockfall mode. Geneva: Eocrisq. Available at: https://www.ecorisq.org.
Dorren, L., and Moos, C. (2021). Towards quantitative evidence of eco-DRR in mountains: a concise review. Ecol. Eng. 175:106485. doi: 10.1016/j.ecoleng.2021.106485
Du, E., Link, T. E., Wei, L., and Marshall, J. D. (2016). Evaluating hydrologic effects of spatial and temporal patterns of forest canopy change using numerical modelling. Hydrol. Process. 30, 217–231. doi: 10.1002/hyp.10591
Elkin, C., Gutiérrez, A. G., Leuzinger, S., Manusch, C., Temperli, C., Rasche, L., et al. (2013). A 2 °C warmer world is not safe for ecosystem services in the European Alps. Glob. Chang. Biol. 19, 1827–1840. doi: 10.1111/gcb.12156
Ellison, D., Futter, M. N., and Bishop, K. (2012). On the forest cover–water yield debate: from demand-to supply-side thinking. Glob. Chang. Biol. 18, 806–820. doi: 10.1111/j.1365-2486.2011.02589.x
Farvacque, M., Lopez-Saez, J., Corona, C., Toe, D., Bourrier, F., and Eckert, N. (2019). How is rockfall risk impacted by land-use and land-cover changes? Insights from the French Alps. Glob. Planet. Chang. 174, 138–152. doi: 10.1016/j.gloplacha.2019.01.009
*Flepp, G., Robyr, R., Scotti, R., Giadrossich, F., Conedera, M., and Vacchiano, G. (2021). Temporal dynamics of root reinforcement in European spruce forests. Forests 12:815. doi: 10.3390/f12060815
Forrester, D. I., Bonal, D., Dawud, S., Gessler, A., Granier, A., Pollastrini, M., et al. (2016). Drought responses by individual tree species are not often correlated with tree species diversity in European forests. J. Appl. Ecol. 53, 1725–1734. doi: 10.1111/1365-2664.12745
Fritzsche, K., Schneiderbauer, S., Bubeck, P., Kienberger, S., Buth, M., Zebisch, M., et al. (2014). The vulnerability sourcebook: concept and guidelines for standardised vulnerability assessments. Deutsche Gesellschaft für Internationale Zusammenarbeit (GIZ) GmbH and European Academy Bozen (EURAC), Bonn, Germany.
García-Hernández, C., Ruiz-Fernández, J., Sánchez-Posada, C., Pereira, S., Oliva, M., and Vieira, G. (2017). Reforestation and land use change as drivers for a decrease of avalanche damage in mid-latitude mountains (NW Spain). Global and Planetary Change 153, 35–50. doi: 10.1016/j.gloplacha.2017.05.001
Gehring, E., Conedera, M., Maringer, J., Giadrossich, F., Guastini, E., and Schwarz, M. (2019). Shallow landslide disposition in burnt European beech (Fagus sylvatica L.) forests. Sci. Rep. 9:8638. doi: 10.1038/s41598-019-45073-7
Getzner, M., Gutheil-Knopp-Kirchwald, G., Kreimer, E., Kirchmeir, H., and Huber, M. (2017). Gravitational natural hazards: valuing the protective function of alpine forests. Forest Policy Econ. 80, 150–159. doi: 10.1016/j.forpol.2017.03.015
*Gholzom, E. H., and Gholami, V. (2012). A comparison between natural forests and reforested lands in terms of runoff generation potential and hydrologic response (case study: Kasilian watershed). Soil Water Res., 7, 166–173. doi: 10.17221/18/2012-SWR
Grima, N., Edwards, D., Edwards, F., Petley, D., and Fisher, B. (2020). Landslides in the Andes: forests can provide cost-effective landslide regulation services. Sci. Total Environ. 745:141128. doi: 10.1016/j.scitotenv.2020.141128
*Guns, M., and Vanacker, V. (2013). Forest cover change trajectories and their impact on landslide occurrence in the tropical Andes. Environ. Earth Sci., 70, 2941–2952. doi: 10.1007/s12665-013-2352-9
Hajian, F., Dykes, A. P., and Cavanagh, S. (2019). Assessment of the flood hazard arising from land use change in a forested catchment in northern Iran. J Flood Risk Manage 12:12481. doi: 10.1111/jfr3.12481
*Harder, P., Pomeroy, J. W., and Westbrook, C. J. (2015). Hydrological resilience of a Canadian Rockies headwaters basin subject to changing climate, extreme weather, and forest management. Hydrol. Process., 29, 3905–3924. doi: 10.1002/hyp.10596
Holtmeier, F.-K., and Broll, G. (2019). Treeline research - from the roots of the past to present time. A review. forests 11:38. doi: 10.3390/f11010038
Innes, J., Joyce, L. A., Kellomäki, S., Louman, B., Ogden, A., Parrotta, J., et al. (2009). “Management for adaptation” in Adaptation of forests and people to climate change: a global assessment report. eds. R. Seppälä, A. Buck, and P. Katila (Helsinki: IUFRO), 135–186.
IPCC. (2012). Managing the risks of extreme events and disasters to advance climate change adaptation. Cambridge University Press, Cambridge, p. 594.
Irauschek, F., Rammer, W., and Lexer, M. J. (2017a). Can current management maintain forest landscape multifunctionality in the eastern Alps in Austria under climate change? Reg. Environ. Chang. 17, 33–48. doi: 10.1007/s10113-015-0908-9
Irauschek, F., Rammer, W., and Lexer, M. J. (2017b). Evaluating multifunctionality and adaptive capacity of mountain forest management alternatives under climate change in the eastern Alps. Eur. J. For. Res. 136, 1051–1069. doi: 10.1007/s10342-017-1051-6
Jandl, N., Jandl, R., and Schindlbacher, A. (2018). Future management options for cembran pine forests close to the alpine timberline. Ann. For. Sci. 75:81. doi: 10.1007/s13595-018-0760-4
Jenkins, M., and Schaap, B. (2018). Forest ecosystem services. Background study prepared for the thirteenth session of the United Nations Forum on Forests. Available at: https://www.un.org/esa/forests/wp-content/uploads/2018/05/UNFF13_BkgdStudy_ForestsEcoServices.pdf.
Kleinman, J. S., Goode, J. D., Fries, A. C., and Hart, J. L. (2019). Ecological consequences of compound disturbances in forest ecosystems: a systematic review. Ecosphere 10:e02962. doi: 10.1002/ecs2.2962
*Komatsu, H., Shinohara, Y., Kume, T., and Otsuki, K. (2011). Changes in peak flow with decreased forestry practices: analysis using watershed runoff data. J. Environ. Manag., 92, 1528–1536. doi: 10.1016/j.jenvman.2011.01.010
*Krecek, J., Novakova, J., Palan, L., Pazourkova, E., and Stuchlik, E. (2021). Role of forests in headwater control with changing environment and society. Int. Soil Water Conserv. Res. 9, 143–157. doi: 10.1016/j.iswcr.2020.11.002
Lange, B., Germann, P. F., and Lüscher, P. (2013). Greater abundance of Fagus sylvatica in coniferous flood protection forests due to climate change: impact of modified root densities on infiltration. Eur. J. For. Res. 132, 151–163. doi: 10.1007/s10342-012-0664-z
*Langhammer, J., Su, Y., and Bernsteinova, J. (2015). Runoff response to climate warming and Forest disturbance in a Mid-Mountain basin. Water, 7, 3320–3342. doi: 10.3390/w7073320
Leonard, M., Westra, S., Phatak, A., Lambert, M., van den Hurk, B., McInnes, K., et al. (2014). A compound event framework for understanding extreme impacts. Wiley Interdiscip. Rev. Clim. Chang. 5, 113–128. doi: 10.1002/wcc.252
Leverkus, A. B., Buma, B., Wagenbrenner, J., Burton, P. J., Lingua, E., Marzano, R., et al. (2021). Tamm review: does salvage logging mitigate subsequent forest disturbances? For. Ecol. Manag. 481:118721. doi: 10.1016/j.foreco.2020.118721
Lima, L. S., Coe, M. T., Soares Filho, B. S., Cuadra, S. V., Dias, L. C. P., Costa, M. H., et al. (2014). Feedbacks between deforestation, climate, and hydrology in the southwestern Amazon: implications for the provision of ecosystem services. Landsc. Ecol. 29, 261–274. doi: 10.1007/s10980-013-9962-1
Lim, C. H., and Kim, H. J. (2022). Can Forest-related adaptive capacity reduce landslide risk attributable to climate change? Case of Republic of Korea. Forests 13:49. doi: 10.3390/f13010049
Lingua, E., Bettella, F., Pividori, M., Marzano, R., Garbarino, M., Piras, M., et al. (2020). “The protective role of forests to reduce Rockfall risks and impacts in the Alps under a climate change perspective” in Climate change, hazards and adaptation options. eds. W. L. Filho, G. J. Nagy, M. Borga, P. D. C. Muñoz, and A. Magnuszewski (Cham: Springer), 333–347.
Lischke, H., Zimmermann, N. E., Bolliger, J., Rickebusch, S., and Löffler, T. J. (2006). TreeMig: a forest-landscape model for simulating spatio-temporal patterns from stand to landscape scale. Ecol. Model. 199, 409–420. doi: 10.1016/j.ecolmodel.2005.11.046
Logan, J. A., Macfarlane, W. W., and Willcox, L. (2010). Whitebark pine vulnerability to climate-driven mountain pine beetle disturbance in the greater Yellowstone ecosystem. Ecol. Appl. 20, 895–902. doi: 10.1890/09-0655.1
*Lopez-Ramirez, S. M., Saenz, L., Mayer, A., Munoz-Villers, L. E., Asbjornsen, H., Berry, Z. C., et al. (2020). Land use change effects on catchment streamflow response in a humid tropical montane cloud forest region, Central Veracruz, Mexico. Hydrol. Process., 34, 3555–3570. doi: 10.1002/hyp.13800
Makino, Y., and Rudolf-Miklau, F. (2021). The protective functions of forests in a changing climate-European experience. FAO and Austrian Federal Ministry for Agriculture, regions and tourism. Rome, Italy.
*Malek, Z., Boerboom, L., and Glade, T. (2015). Future Forest cover change scenarios with implications for landslide risk: an example from Buzau Subcarpathians, Romania. Environ. Manag. 56, 1228–1243. doi: 10.1007/s00267-015-0577-y
Malek, Z., Zumpano, V., and Hussin, H. (2018). Forest management and future changes to ecosystem services in the Romanian Carpathians. Environ. Dev. Sustain. 20, 1275–1291. doi: 10.1007/s10668-017-9938-4
Maringer, J., Ascoli, D., Dorren, L., Bebi, P., and Conedera, M. (2016). Temporal trends in the protective capacity of burnt beech forests (Fagus sylvatica L.) against rockfall. Eur. J. For. Res. 135, 657–673. doi: 10.1007/s10342-016-0962-y
Maroschek, M., Rammer, W., and Lexer, M. J. (2015). Using a novel assessment framework to evaluate protective functions and timber production in Austrian mountain forests under climate change. Reg. Environ. Chang. 15, 1543–1555. doi: 10.1007/s10113-014-0691-z
*Masum, K. M., Mansor, A., Sah, S. A. M., and Lim, H. S. (2017). Effect of differential forest management on land-use change (LUC) in a tropical hill forest of Malaysia. J. Environ. Manag., 200, 468–474. doi: 10.1016/j.jenvman.2017.06.009
Mathys, A. S., Bottero, A., Stadelmann, G., Thürig, E., Ferretti, M., and Temperli, C. (2021). Presenting a climate-smart forestry evaluation framework based on national forest inventories. Ecol. Indic. 133:108459. doi: 10.1016/j.ecolind.2021.108459
Mayer, A. C., and Stöckli, V. (2005). Long-term impact of cattle grazing on subalpine forest development and efficiency of snow avalanche protection. Arct. Antarct. Alp. Res. 37, 521–526. doi: 10.1657/1523-0430(2005)037[0521:LIOCGO]2.0.CO;2
Mina, M., Bugmann, H., Cordonnier, T., Irauschek, F., Klopcic, M., Pardos, M., et al. (2017). Future ecosystem services from European mountain forests under climate change. J. Appl. Ecol. 54, 389–401. doi: 10.1111/1365-2664.12772
Moos, C., Bebi, P., Graf, F., Mattli, J., Rickli, C., and Schwarz, M. (2016). How does forest structure affect root reinforcement and susceptibility to shallow landslides? Earth Surf. Process. Landf. 41, 951–960. doi: 10.1002/esp.3887
Moos, C., Bebi, P., Schwarz, M., Stoffel, M., Sudmeier-Rieux, K., and Dorren, L. (2018). Ecosystem-based disaster risk reduction in mountains. Earth Sci. Rev. 177, 497–513. doi: 10.1016/j.earscirev.2017.12.011
Moos, C., Dorren, L., and Stoffel, M. (2017). Quantifying the effect of forests on frequency and intensity of rockfalls. Nat. Hazards Earth Syst. Sci. 17, 291–304. doi: 10.5194/nhess-17-291-2017
Moos, C., Guisan, A., Randin, C. F., and Lischke, H. (2021). Climate change impacts the protective effect of forests: a case study in Switzerland. Front. Forests Glob. Change 4:682923. doi: 10.3389/ffgc.2021.682923
Moos, C., Thomas, M., Pauli, B., Bergkamp, G., Stoffel, M., and Dorren, L. (2019a). Economic valuation of ecosystem-based rockfall risk reduction considering disturbances and comparison to structural measures. Sci. Total Environ. 697:134077. doi: 10.1016/j.scitotenv.2019.134077
Moos, C., Toe, D., Bourrier, F., Knüsel, S., Stoffel, M., and Dorren, L. (2019b). Assessing the effect of invasive tree species on rockfall risk–the case of Ailanthus altissima. Ecol. Eng. 131, 63–72. doi: 10.1016/j.ecoleng.2019.03.001
*Muma, M., Assani, A. A., Landry, R., Quessy, J.-F., and Mesfioui, M. (2011). Effects of the change from forest to agriculture land use on the spatial variability of summer extreme daily flow characteristics in southern Quebec (Canada). J. Hydrol. 407, 153–163. doi: 10.1016/j.jhydrol.2011.07.020
Munoz-Villers, L. E., and McDonnell, J. J. (2013). Land use change effects on runoff generation in a humid tropical montane cloud forest region. Hydrol. Earth Syst. Sci. 17, 3543–3560. doi: 10.5194/hess-17-3543-2013
Nehren, U., Arce-Mojica, T., Cara Barrett, A., Cueto, J., Doswald, N., Janzen, S., et al. (2023). Towards a typology of nature-based solutions for disaster risk reduction. Nat. Based Solutions 3:100057. doi: 10.1016/j.nbsj.2023.100057
Olschewski, R., Bebi, P., Teich, M., Hayek, U. W., and Grêt-Regamey, A. (2012). Avalanche protection by forests—a choice experiment in the Swiss Alps. Forest Policy Econ. 17, 19–24. doi: 10.1016/j.forpol.2012.02.016
Onuma, A., and Tsuge, T. (2018). Comparing green infrastructure as ecosystem-based disaster risk reduction with gray infrastructure in terms of costs and benefits under uncertainty: a theoretical approach. Int. J. Disaster Risk Reduct. 32, 22–28. doi: 10.1016/j.ijdrr.2018.01.025
Oven, D., Zabota, B., and Kobal, M. (2020). The influence of abiotic and biotic disturbances on the protective effect of alpine forests against avalanches and rockfalls. Acta Silvae Ligni 121, 1–18. doi: 10.20315/ASetL.121.1
Page, M. J., McKenzie, J. E., Bossuyt, P. M., Boutron, I., Hoffmann, T. C., Mulrow, C. D., et al. (2021). The PRISMA 2020 statement: an updated guideline for reporting systematic reviews. BMJ 372:n71. doi: 10.1136/bmj.n71
Pardos, M., Perez, S., Calama, R., Alonso, R., and Lexer, M. J. (2017). Ecosystem service provision, management systems and climate change in Valsain forest, Central Spain. Reg. Environ. Chang. 17, 17–32. doi: 10.1007/s10113-016-0985-4
Perzl, F., Bono, A., Garbarino, M., and Motta, R. (2021). “Protective effects of forests against gravitational natural hazards” in Protective forests as ecosystem-based solution for disaster risk reduction. (eco-DRR). eds. M. Teich, C. Accastello, F. Perzl, and K. Kleemayr (London: IntechOpen)
Perzl, F., and Teich, M. (2022). Geodata requirements for mapping protective functions and effects of forests. in Protective forests as ecosystem-based solution for disaster risk reduction (eco-DRR). eds. M. Teich, C. Accastello, F. Perzl, and K. Kleemayr London: IntechOpen.
Poratelli, F., Cocuccioni, S., Accastello, C., Steger, S., Schneiderbauer, S., and Brun, F. (2020). State-of-the-art on ecosystem-based solutions for disaster risk reduction: the case of gravity-driven natural hazards in the alpine region. Int. J. Disast. Risk Reduc. 51:101929. doi: 10.1016/j.ijdrr.2020.101929
Rammig, A., Fahse, L., Bebi, P., and Bugmann, H. (2007). Wind disturbance in mountain forests: simulating the impact of management strategies, seed supply, and ungulate browsing on forest succession. For. Ecol. Manag. 242, 142–154. doi: 10.1016/j.foreco.2007.01.036
Ratcliffe, S., Wirth, C., Jucker, T., Van der Plas, F., Scherer-Lorenzen, M., Verheyen, K., et al. (2017). Biodiversity and ecosystem functioning relations in European forests depend on environmental context. Ecol. Lett. 20, 1414–1426. doi: 10.1111/ele.12849
Rickli, C., Bebi, P., Graf, F., and Moos, C. (2019). “Shallow landslides: retrospective analysis of the protective effects of forest and conclusions for prediction” in Recent Advances in geotechnical research (Berlin: Springer), 175–185.
Rigon, R., Bertoldi, G., and Over, T. M. (2006). GEOtop: a distributed hydrological model with coupled water and energy budgets. J. Hydrometeorol. 7, 371–388. doi: 10.1175/JHM497.1
Ringenbach, A., Stihl, E., Bühler, Y., Bebi, P., Bartelt, P., Rigling, A., et al. (2022). Full-scale experiments to examine the role of deadwood in rockfall dynamics in forests. Nat. Hazards Earth Syst. Sci. 22, 2433–2443. doi: 10.5194/nhess-22-2433-2022
Ruangpan, L., Vojinovic, Z., Di Sabatino, S., Leo, L. S., Capobianco, V., Oen, A. M., et al. (2020). Nature-based solutions for hydro-meteorological risk reduction: a state-of-the-art review of the research area. Nat. Hazards Earth Syst. Sci. 20, 243–270. doi: 10.5194/nhess-20-243-2020
Ruiz-Benito, P., Vacchiano, G., Lines, E. R., Reyer, C. P., Ratcliffe, S., Morin, X., et al. (2020). Available and missing data to model impact of climate change on European forests. Ecol. Model. 416:108870. doi: 10.1016/j.ecolmodel.2019.108870
Scheidl, C., Heiser, M., Kamper, S., Thaler, T., Klebinder, K., Nagl, F., et al. (2020). The influence of climate change and canopy disturbances on landslide susceptibility in headwater catchments. Sci. Total Environ. 742:140588. doi: 10.1016/j.scitotenv.2020.140588
Schmid, U., Frehner, M., Glatthorn, J., and Bugmann, H. (2023). FroForM: A simulation model for the management of mountain protection forests. Ecological Moldelling 478:11029. doi: 10.1016/j.ecolmodel.2023.110297
Schwarz, M., Preti, F., Giadrossich, F., Lehmann, P., and Or, D. (2010). Quantifying the role of vegetation in slope stability: a case study in Tuscany (Italy). Ecol. Eng. 36, 285–291. doi: 10.1016/j.ecoleng.2009.06.014
Sebald, J., Senf, C., Heiser, M., Scheidl, C., Pflugmacher, D., and Seidl, R. (2019). The effects of forest cover and disturbance on torrential hazards: large-scale evidence from the eastern Alps. Environ. Res. Lett. 14:114032. doi: 10.1088/1748-9326/ab4937
Seibert, J., McDonnell, J. J., and Woodsmith, R. D. (2010). Effects of wildfire on catchment runoff response: a modelling approach to detect changes in snow-dominated forested catchments. Hydrol. Res. 41, 378–390. doi: 10.2166/nh.2010.036
Seidl, R., Rammer, W., Scheller, R. M., and Spies, T. A. (2012). An individual-based process model to simulate landscapescale forest ecosystem dynamics. Ecol. Model. 231, 87–100. doi: 10.1016/j.ecolmodel.2012.02.015
*Semenova, O. M., Lebedeva, L. S., Nesterova, N. V., and Vinogradova, T. A. (2015). Evaluation of short-term changes of hydrological response in mountainous basins of the Vitim plateau (Russia) after forest fires based on data analysis and hydrological modelling. Hydrologic non-stationarity and extrapolating models to predict the future. IAHS Symposium HS02, 26th General Assembly of the International Union of Geodesy and Geophysics, Prague, Czech Republic, pp. 157–162.
Senf, C., and Seidl, R. (2021). Mapping the forest disturbance regimes of Europe. Nat. Sustain. 4, 63–70. doi: 10.1038/s41893-020-00609-y
Sidau, M. R., Horváth, C., Cheveresan, M., Sandric, I., and Stoica, F. (2021). Assessing hydrological impact of forested area change: a remote sensing cas study. Atmosphere 12:817. doi: 10.3390/atmos12070817
Smith, W. K., Germino, M. J., Johnson, D. M., and Reinhardt, K. (2009). The altitude of alpine treeline: a bellwether of climate change effects. Bot. Rev. 75, 163–190. doi: 10.1007/s12229-009-9030-3
Stoffel, M., Tiranti, D., and Huggel, C. (2014). Climate change impacts on mass movements—case studies from the European Alps. Sci. Total Environ. 493, 1255–1266. doi: 10.1016/j.scitotenv.2014.02.102
Stritih, A., Bebi, P., and Grêt-Regamey, A. (2019). Quantifying uncertainties in earth observation-based ecosystem service assessments. Environ. Model Softw. 111, 300–310. doi: 10.1016/j.envsoft.2018.09.005
Stritih, A., Bebi, P., Rossi, C., and Grêt-Regamey, A. (2021). Addressing disturbance risk to mountain forest ecosystem services. J. Environ. Manag. 296:113188. doi: 10.1016/j.jenvman.2021.113188
Stritih, A., Seidl, R., and Senf, C. (2023). Alternative states in the structure of mountain forests across the Alps and the role of disturbance and recovery. Landsc. Ecol. 38, 933–947. doi: 10.1007/s10980-023-01597-y
Sudmeier-Rieux, K., Arce-Mojica, T., Boehmer, H. J., Doswald, N., Emerton, L., Friess, D. A., et al. (2021). Scientific evidence for ecosystem-based disaster risk reduction. Nat Sustain 4, 803–810. doi: 10.1038/s41893-021-00732-4
Surfleet, C. G., Dietterick, B., and Skaugset, A. (2014). Change detection of storm runoff and sediment yield using hydrologic models following wildfire in a coastal redwood forest, California. Can. J. For. Res. 44, 572–581. doi: 10.1139/cjfr-2013-0328
*Tani, M., Fujimoto, M., Katsuyama, M., Kojima, N., Hosoda, I., Kosugi, K., et al. (2012). Predicting the dependencies of rainfall-runoff responses on human forest disturbances with soil loss based on the runoff mechanisms in granite and sedimentary rock mountains. Hydrol. Process., 26, 809–826. doi: 10.1002/hyp.8295
Teich, M., Accastello, C., Perzl, F., and Berger, F. (2022a). “Protective forests for ecosystem-based disaster risk reduction (eco-DRR) in the alpine space” in Protective forests as ecosystem-based solution for disaster risk reduction (eco-DRR). eds. M. Teich, C. Accastello, F. Perzl, and K. Kleemayr (London: IntechOpen)
Teich, M., Accastello, C., Perzl, F., and Kleemayr, K.. (2022b). Protective forests as ecosystem-based solution for disaster risk reduction (eco-DRR). London: IntechOpen.
Teich, M., Giunta, A. D., Hagenmuller, P., Bebi, P., Schneebeli, M., and Jenkins, M. J. (2019). Effects of bark beetle attacks on forest snowpack and avalanche formation–implications for protection forest management. For. Ecol. Manag. 438, 186–203. doi: 10.1016/j.foreco.2019.01.052
*Tetzlaff, D., Malcolm, I. A., and Soulsby, C. (2007). Influence of forestry, environmental change and climatic variability on the hydrology, hydrochemistry and residence times of upland catchments. J. Hydrol. 346, 93–111. doi: 10.1016/j.jhydrol.2007.08.016
Thrippleton, T., Luscher, F., and Bugmann, H. (2020). Climate change impacts across a large forest enterprise in the northern pre-Alps: dynamic forest modelling as a tool for decision support. Eur. J. For. Res. 139, 483–498. doi: 10.1007/s10342-020-01263-x
Thrippleton, T., Temperli, C., Krumm, F., Mey, R., Zell, J., Stroheker, S., et al. (2023). Balancing disturbance risk and ecosystem service provisioning in Swiss mountain forests: an increasing challenge under climate change. Reg. Environ. Chang. 23:29. doi: 10.1007/s10113-022-02015-w
Tran, P., Marincioni, F., and Shaw, R. (2010). Catastrophic flood and forest cover change in the Huong river basin, Central Viet Nam: a gap between common perceptions and facts. J. Environ. Manag. 91, 2186–2200. doi: 10.1016/j.jenvman.2010.05.020
UNISDR. (2009). 2009 UNISDR terminology on disaster risk reduction. Geneva, Switzerland: UNISDR, p. 35.
*Vacchiano, G., Berretti, R., Mondino, E. B., Meloni, F., and Motta, R. (2016). Assessing the effect of disturbances on the functionality of direct protection forests. Mt. Res. Dev., 36, 41–55. doi: 10.1659/mrd-journal-d-15-00075.1
*Van Eck, N. J., and Waltman, L.. (2010). Software survey: VOSviewer, a computer program for bibliometric mapping. Scientometrics, 84, 523–538. doi: 10.1007/s11192-009-0146-3
*VanShaar, J. R., Haddeland, I., and Lettenmaier, D. P.. (2002). Effects of land-cover changes on the hydrological response of interior Columbia River basin forested catchments. Hydrol. Process. 16, 2499–2520. doi: 10.1002/hyp.1017
Vergani, C., Schwarz, M., Soldati, M., Corda, A., Giadrossich, F., Chiaradia, E. A., et al. (2016). Root reinforcement dynamics in subalpine spruce forests following timber harvest: a case study in Canton Schwyz, Switzerland. Catena 143, 275–288. doi: 10.1016/j.catena.2016.03.038
Vergani, C., Werlen, M., Conedera, M., Cohen, D., and Schwarz, M. (2017). Investigation of root reinforcement decay after a forest fire in a scots pine (Pinus sylvestris) protection forest. For. Ecol. Manag. 400, 339–352. doi: 10.1016/j.foreco.2017.06.005
Versini, P.-A., Velasco, M., Cabello, A., and Sempere-Torres, D. (2013). Hydrological impact of forest fires and climate change in a Mediterranean basin. Nat. Hazards 66, 609–628. doi: 10.1007/s11069-012-0503-z
Winkel, G., Lovrić, M., Muys, B., Katila, P., Lundhede, T., Pecurul, M., et al. (2022). Governing Europe's forests for multiple ecosystem services: opportunities, challenges, and policy options. Forest Policy Econ. 145:102849. doi: 10.1016/j.forpol.2022.102849
Winter, M. B., Baier, R., and Ammer, C. (2015). Regeneration dynamics and resilience of unmanaged mountain forests in the northern limestone Alps following bark beetle-induced spruce dieback. Eur. J. For. Res. 134, 949–968. doi: 10.1007/s10342-015-0901-3
*Wissmar, R. C., Timm, R. K., and Logsdon, M. G. (2004). Effects of changing forest and impervious land covers on discharge characteristics of watersheds. Environ. Manag. 34, 91–98. doi: 10.1007/s00267-004-0224-5
Wohlgemuth, T., and Brang, P. (2015). 25 Jahre Erfahrung mit Sturmschäden. 166. Switzerland: Editorial in the Swiss Forestry Journal, p. 117.
Wohlgemuth, T., Schwitter, R., Bebi, P., Sutter, F., and Brang, P. (2017). Post-windthrow management in protection forests of the Swiss Alps. Eur. J. For. Res. 136, 1029–1040. doi: 10.1007/s10342-017-1031-x
Yamaura, Y., Yamada, Y., Matsuura, T., Tamai, K., Taki, H., Sato, T., et al. (2021). Modeling impacts of broad-scale plantation forestry on ecosystem services in the past 60 years and for the future. Ecosyst. Serv. 49:101271. doi: 10.1016/j.ecoser.2021.101271
*Yu, P., Wang, Y., Coles, N., Xiong, W., and Xu, L. (2015). Simulation of runoff changes caused by cropland to Forest conversion in the upper Yangtze River region, SW China. PLoS One, 10:132395. doi: 10.1371/journal.pone.0132395
*Zhang, H., Cheng, J., and Chen, Z. (2004). Effects of forest change on runoff and sediment in three-gorge region of Yangtze river. Proceedings of the 9th International Symposium on River Sedimentation (Yichang, 2004).
Zhang, Z., Zhang, L., Xu, H., Creed, I. F., Blanco, J. A., Wei, X., et al. (2023). Forest water-use efficiency: effects of climate change and management on the coupling of carbon and water processes. For. Ecol. Manag. 534:120853. doi: 10.1016/j.foreco.2023.120853
Zindros, A., Radoglou, K., Milios, E., and Kitikidou, K. (2020). Tree line shift in the Olympus Mountain (Greece) and climate change. Forests 11:985. doi: 10.3390/f11090985
Zlatanov, T., Elkin, C., Irauschek, F., and Lexer, M. J. (2017). Impact of climate change on vulnerability of forests and ecosystem service supply in Western Rhodopes Mountains. Reg. Environ. Chang. 17, 79–91. doi: 10.1007/s10113-015-0869-z
Zscheischler, J., and Seneviratne, S. I. (2017). Dependence of drivers affects risks associated with compound events. Sci. Adv. 3:e1700263. doi: 10.1126/sciadv.1700263
Keywords: protective forest, nature-based solution, disturbance, avalanche, rockfall, landslide, torrential flood, climate change
Citation: Moos C, Stritih A, Teich M and Bottero A (2023) Mountain protective forests under threat? an in-depth review of global change impacts on their protective effect against natural hazards. Front. For. Glob. Change. 6:1223934. doi: 10.3389/ffgc.2023.1223934
Edited by:
Isabella De Meo, Council for Agricultural and Economics Research (CREA), ItalyReviewed by:
Marc Hanewinkel, University of Freiburg, GermanyJiří Schneider, Mendel University in Brno, Czechia
Zuzana Sitková, National Forest Centre, Slovakia
Copyright © 2023 Moos, Stritih, Teich and Bottero. This is an open-access article distributed under the terms of the Creative Commons Attribution License (CC BY). The use, distribution or reproduction in other forums is permitted, provided the original author(s) and the copyright owner(s) are credited and that the original publication in this journal is cited, in accordance with accepted academic practice. No use, distribution or reproduction is permitted which does not comply with these terms.
*Correspondence: Christine Moos, christine.moos@bfh.ch