- 1Department of Plant Production and Forest Resources, Sustainable Forest Management Research Institute (iuFOR). Higher Technical School of Agricultural Engineering (ETSIIAA), University of Valladolid, Palencia, Spain
- 2Instituto de Recursos Naturales y Agrobiología, IRNASA-CSIC, Salamanca, Spain
- 3Grupo de Interacción Planta-Microorganismo, USAL, Unidad Asociada al CSIC por el IRNASA, Salamanca, Spain
Introduction: Forests in the Mediterranean basin are currently in decline. Their resilience has been eroded as a result of climate change and anthropogenic impacts, making them vulnerable to increasingly frequent episodes of drought, fire and the spread of pests and diseases. The impact of these natural and anthropogenic events on soil biodiversity is of particular concern, as the soil fungal community plays a key role in ecosystem homeostasis.
Objectives and methods: In order to analyse the relationship between soil health status and fungal diversity, soil samples were collected from declining Mediterranean forests of Castanea sativa (chestnut), Quercus ilex (holm oak), Quercus suber (cork oak) and Quercus pyrenaica (Pyrenean oak). A metabarcoding study was carried out by sequencing the ITS genomic region.
Results: A total of 674 fungal genera were found. It has not been possible to explain the differences in health status from the fungal genera found exclusively on declining forest soils, as none of them have been described as pathogenic. Healthy chestnut soils were characterized by a high alpha diversity and a higher abundance of the genus Metarhizium. No differentially abundant genera were found in any of the other forest species tested. Declining chestnut soils harbored more abundance of ectomycorrhizae and soil saprotrophs than healthy samples. Ectomycorrhizae were the dominant lifestyle in all oak species regardless of health status, whereas arbuscular mycorrhizae were preferentially found in declining cork oak soils.
Discussion: This work highlights the resilience of fungal communities of soil against decline and highlights the need to further investigate its relationship with the forest’s ability to cope with the challenges of climate change.
1. Introduction
Mediterranean forests are characteristic ecosystems of the Mediterranean Basin, but also present in California, South Africa and Chile. These forests are a hot spot of genetic diversity in the European continent, as they harbor 290 tree species, compared to 135 reported in the rest of Europe (Gauquelin et al., 2018). The organisms that make up the Mediterranean forests are usually adapted to very specific climatic factors, such as the existence of a marked seasonality characterized by long periods of summer drought, and great variability in the total annual rainfall. In addition, Mediterranean forests have been subjected for millennia to anthropogenic impacts, mainly fragmentation due to land use change toward agriculture and grazing, overexploitation to obtain goods and services and, more recently, abandonment of their use (Fady et al., 2022). These characteristics have confirmed that Mediterranean forests are as vulnerable to management policies as they are to climate change (Morán-Ordóñez et al., 2020).
Some of the main tree species found in Mediterranean forests are chestnut, cork oaks, holm oaks and Pyrenean oaks. Chestnut (Castanea sativa Mill.) covers more than 2.5 million hectares in the Mediterranean Basin, being the only species of the genus native to this region. Chestnut has been widely cultivated as a monoculture for centuries and as a source of timber and fruit, although in recent decades it has been progressively felled (Garfì et al., 2022). Cork oak (Quercus suber L.) spreads over between 1.7 and 2.7 million ha of the Mediterranean Basin and has been cultivated for 3,000 years for the production of bark, representing the second most important marketable non-timber forest product (Gauquelin et al., 2018). Holm oak (Quercus ilex L.) forests comprise 4% of the forests of the Mediterranean Basin, being fully adapted to a wide range of soil and climatic conditions. In spite of the continuous anthropic exploitation of holm oaks during several centuries, the reduction in the use of their wood as fuel in the last 50 years is turning holm oak forests into high forests (Camponi et al., 2023). Pyrenean oak (Quercus pyrenaica Willd.) is found only in France, Spain, Portugal and Morocco. Pyrenean oak forests have historically been exploited by man for timber, and still are to this day, and constitute the main ecosystem of the Mediterranean Basin in diversity of hoverfly species (Perez-Luque et al., 2020; Martín-Pinto et al., 2023).
At present, the Mediterranean forest is in a situation of regression and dieback in several areas of the Mediterranean Basin, due to increased periods of drought, overexploitation, fire, soil degradation and the spread of pests and diseases (Peñuelas and Sardans, 2021). The decline of Mediterranean forests is a complex phenomenon due to multiple and very different causes that can occur slowly and over several years, ending with tree death (Gentilesca et al., 2017). In the case of chestnuts, drought (Waldboth and Oberhuber, 2009), or pathogens such as Cryphonectria parasitica (Elliott and Swank, 2008; Waldboth and Oberhuber, 2009), or Phytophthora sp. (Vettraino et al., 2005; Akıllı et al., 2012) have been reported as causes related to decline. In cork oak, causes include drought (Zribi et al., 2016; Camilo-Alves et al., 2017; El Ahmadi et al., 2022) and fungi such as Phytophthora sp. (Smahi et al., 2017). Or in the case of holm oak, drought events (Limousin et al., 2009) or pathogens such as Phytophthora cinnamomi (Frisullo et al., 2018) have been associated with decline.
Soil fungi play fundamental roles in the functioning of ecosystems, as decomposers of organic matter and as beneficial and detrimental organisms in interaction with plants (Wardle and Lindahl, 2014; Fernandes et al., 2022). In the study of soil mycobiome diversity, the DNA metabarcoding methodology represents one of the most widely used tools in the last decade (Wardle and Lindahl, 2014; Tedersoo et al., 2021). Precisely, metabarcoding has identified how disturbance events of natural and anthropogenic origin have a significant effect on the soil mycobiome of forests (Bowd et al., 2022; Martín-Pinto et al., 2023). This methodology of mycobiota diversity analysis has already been used in Mediterranean forest, being described as an effective way for the real knowledge of microbial diversity and functionality (Adamo et al., 2021a,b), also in situations of disturbance, such as fire (García-Carmona et al., 2021) or climate change (Diez-Hermano et al., 2022).
In chestnut, the study of soil fungal diversity by metabarcoding has been confirmed as a more comprehensive strategy than the study of fruiting bodies (Baptista et al., 2015). In the presence of the pathogenic oomycete Phytophthora cambivora, it has been reported by metabarcoding how soil mycobiota show resilience to the pathogen, and may play a key role in the forest’s resistance to the disease (Venice et al., 2021). The use of metabarcoding in the study of rhizospheric mycobiota of cork oaks has revealed the influence of abiotic factors, such as climate, on its composition (Costa et al., 2022). In addition, the diversity of soil mycobiota is closely related to the decline of cork oaks (Maghnia et al., 2019). In this sense, the fungal diversity most studied by metabarcoding in relation to decline is that of the holm oaks of Spanish “dehesas.” In this way, it has been possible to identify how pathogens such as Phytophthora cinnamomi in combination with others (such as Fusarium spp., Alternaria spp. or Pythium spiculum) and not in isolation are involved in the development of decline, as well as how fungi such as Trichoderma spp. or ectomycorrhizae improve the response of trees affected by these pathogens (Ruiz-Gómez et al., 2019a,b). With respect to Pyrenean oak, to our knowledge, the study of its rhizospheric/soil mycobiota by metabarcoding has been little addressed so far. For example, it has been possible to relate how different management strategies have an impact in soil fungal diversity (Martín-Pinto et al., 2023).
Therefore, the aim of this work is to relate soil mycobiota with the health status of Mediterranean forests. To this end, chestnut, cork oak, holm oak and Pyrenean oak have been selected as key forest species, obtaining rhizospheric soil samples from healthy and declining trees in forests with known presence of pests and pathogens. Soil mycobiota was analyzed by metabarcoding using the ITS region of ribosomal DNA.
2. Materials and methods
2.1. Sampling sites and procedure
Soil samples were extracted from the rhizosphere of healthy and declining tree of Castanea sativa (chestnut), Quercus suber (cork oak), Quercus ilex (holm oak) and Quercus pyrenaica (pyrenean oak) from Salamanca (Castile and Leon, Spain) forests (Table 1). Each site sampled corresponded to a single tree species. Soil collection was conducted for all four sites in June and July 2020.
For all sampling sites, declining patches were defined as areas with high percentage of declining trees (presence of canker wounds or stem bleeds, >70% of trees with severe dieback and foliage wilting). Health condition was assessed visually from the ground following guidelines from the ICP Forests Manual Part IV “Visual Assessment of Crown Condition and Damaging Agents” (Eichhorn et al., 2016). Stands of healthy trees were primarily composed of asymptomatic trees free of dieback or crown transparency. Data on tree assessment as well as a map of sampling locations can be found in Supplementary Figures S1, S2.
Three circular plots of healthy trees and three with high degree of decline were selected in each site. Distance between sampled trees ranged between 10 and 50 m. Soil under the canopy of five live trees was sampled in and pooled per plot. Surface debris was removed and four cores (100 cm3 each at opposite N, S, W, E cardinal points) of topsoil from underneath the litter layer were collected, around one meter from each tree trunk. Coarse roots and stones were removed. All soil cores from each plot were pooled, resulting in a composite soil sample per plot and totalling 24 samples (4 sampling sites × 3 plots × 2 health conditions). Samples were stored at −20°C prior to processing.
Throughout this paper soil sampled from stands of healthy trees will be referred to as “healthy trees soil” whereas soil from stands of declining trees will be termed “declining trees soil.”
2.2. Sample processing and sequencing
Physicochemical analyses of soil samples were performed in triplicate, at the Analysis and Instrumentation Service of IRNASA-CSIC by standard methods. Analyzed soil properties can be found in Supplementary Table S1.
Samples were sent for molecular analysis to Base Clear B.V (Leiden, Netherlands). DNA extraction was performed using the DNeasy PowerLyzer PowerSoil kit (Qiagen). Region 2 of fungal Internal Transcribed Spacer (ITS2) gene was amplified using the following primers: ITS7-F: 5′-GTG ART CAT CGA RTC TTT G-3′, ITS4-R: 5’-TCC TCC GCT TAT TGA TAT GC-3′ (Fujita et al., 2001; Ihrmark et al., 2012). Paired-end sequence reads (2×300 bp) were generated using the Illumina MiSeq V2 system under accreditation according to the scope of BaseClear B.V (L457; NEN-EN-ISO/IEC 17025). FASTQ read sequence files were generated using bcl2fastq2 version 2.18. Initial quality assessment was based on data passing the Illumina Chastity filtering. Subsequently, reads containing PhiX control signal were removed using an in-house filtering protocol. In addition, reads containing (partial) adapters were clipped (up to a minimum read length of 50 bp). The second quality assessment was based on the remaining reads using the FASTQC quality control tool version 0.11.5. ITS2 sequences were processed following the DADA2 pipeline to generate amplicon sequence variants (ASV) (Callahan et al., 2016). Parameters’ values were as follows: filtering and trimming (maxN = 0, maxEE = 2, truncQ = 2, minLen = 50, rm.phix = TRUE, compress = TRUE), learning error rates (nbases = 1e+08, nreads = NULL, errorEstimationFunction = loessErrfun, MAX_CONSIST = 10, OMEGA_C = 0), merging paired reads (errorEstimationFunction = loessErrfun, selfConsist = FALSE, pool = FALSE) and removing chimeras (method = “consensus”). Taxonomy assignment and abundance estimation were performed comparing ASVs against UNITE database version 9.0 (Abarenkov et al., 2022). Rarefaction curves were used to evaluate the relationship between sequencing depth and the number of ASVs.
2.3. Statistical analysis
Prior to the analysis, taking into consideration that only ITS2 marker was used, ASVs’ raw reads were aggregated at genus level to have better confidence, and to avoid misidentification of closely-related species (Yang et al., 2018).
Differences between mycobiome communities were evaluated in terms of alpha and beta diversity. Alpha diversity was assessed using Hill diversity indexes according to the equation
where D is diversity, S is the number of taxa, pi is the proportion of all individuals that belong to taxa i, ri is the rarity of taxa i, defined as 1/pi, and l is the exponent that determines the rarity scale and corresponds to richness (l = 1) or equivalence-corrected versions of Shannon (l = 0) and Simpson indexes (l = −1) (Roswell et al., 2021). Differences between health conditions per tree species at l = [−1, 0, 1] were contrasted using Wilcoxon’s rank test.
Beta diversity was evaluated in terms of differential abundance by taking into account that high-throughput sequencing counts should be considered compositional data (Gloor et al., 2017). Analyses followed the ZicoSeq procedure, which has been shown to be overall more robust and powerful than other existing methods in recent benchmarks (Yang and Chen, 2022). Compositional effects were addressed by adopting a reference-based approach (selecting close-to-invariant taxa as baseline abundances) and association testing was conducted by linear model-based Smith permutation testing [LDM and DACOMP methods in Brill et al. (2022) and Hu and Satten (2020), respectively]. Reference taxa were adjusted for health status (factor with two levels: healthy, declining) as a covariate. Taxa were filtered if their prevalence was less than 20% and their mean relative abundance was less than 0.2%. Percentage of top outliers replaced by winsorization was 10%. Abundances were square root transformed. Multiple test correction of p-values was based on 500 permutation tests.
All fungal genera were included in the functional analyses. Each genus was assigned a functional guild according to the “primary lifestyle” column obtained from FungalTraits database V1.2 (Põlme et al., 2020). Raw read numbers of each guild were summed per tree species and health condition and expressed as log2(guild abundance/total abundance).
All analyses were performed in R environment 4.1.3 (R Core Team, 2022). Analysis of sequencing data and ASV identification were performed using the packages Biostrings (Pagès et al., 2022), dada2 (Callahan et al., 2016) and ShortRead (Morgan et al., 2009). Hill diversity analysis was carried out using the package MeanRarity (Roswell and Dushoff, 2022). For compositional analysis the package GUniFrac (Chen et al., 2022) was used. Taxonomic information was handled and plotted with the package metacoder (Foster et al., 2017).
3. Results
3.1. Soil description
Physico-chemical properties of soils were analyzed and summarized using Principal Component Analysis (Figure 1). Chestnut’s soils were characterized by clay and slit texture, higher content of assimilable and free Mg and K and a slight tendency to acidity with high concentration of ionized NH4+. Oak’s soils were characterized by sandy textures and a higher C/N ratio, except for soils from declining Pyrenean oaks, which were enriched in Ca, N, P and organic matter. No further associations between health status and soil properties were observed.
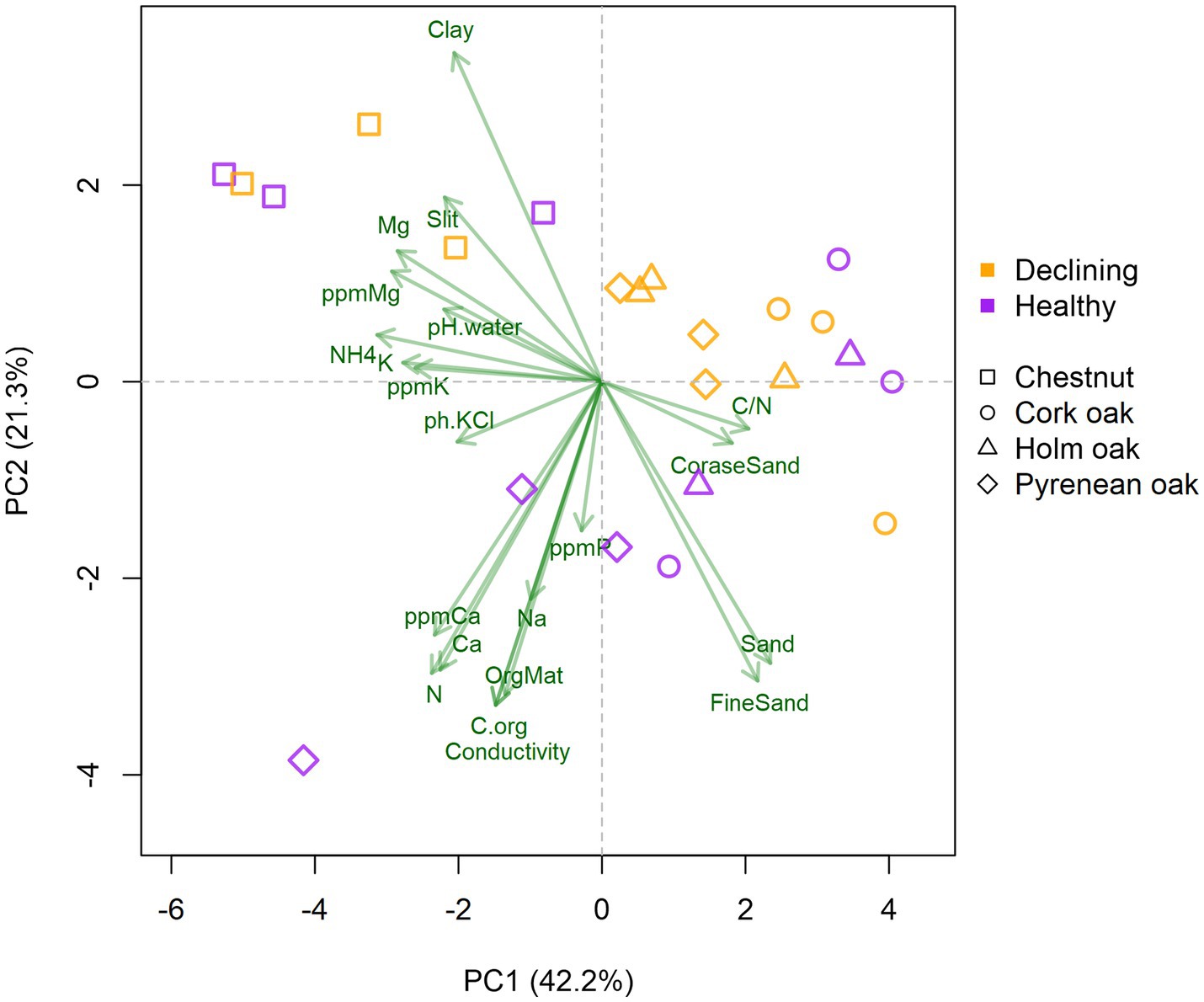
Figure 1. Biplot of soil properties, tree species and health status. Green arrows represent soil properties and colored symbols represent soil samples. Axis correspond to the first and second principal components, accounting for 63.5% of total variance. Variables were scaled prior to analysis.
3.2. Fungal community description
Sequencing yielded 32,042 (29590–33,305) reads on average (median and P25-75) following quality control (Supplementary Table S2). All samples showed plateauing rarefaction curves (Supplementary Figure S1). The analysis of the fungal communities reported 674 different genera distributed in 15 phyla (Supplementary Table S3). The majority of the phyla were Ascomycota (⁓66%) and Basidiomycota (⁓26%) (Figure 2).
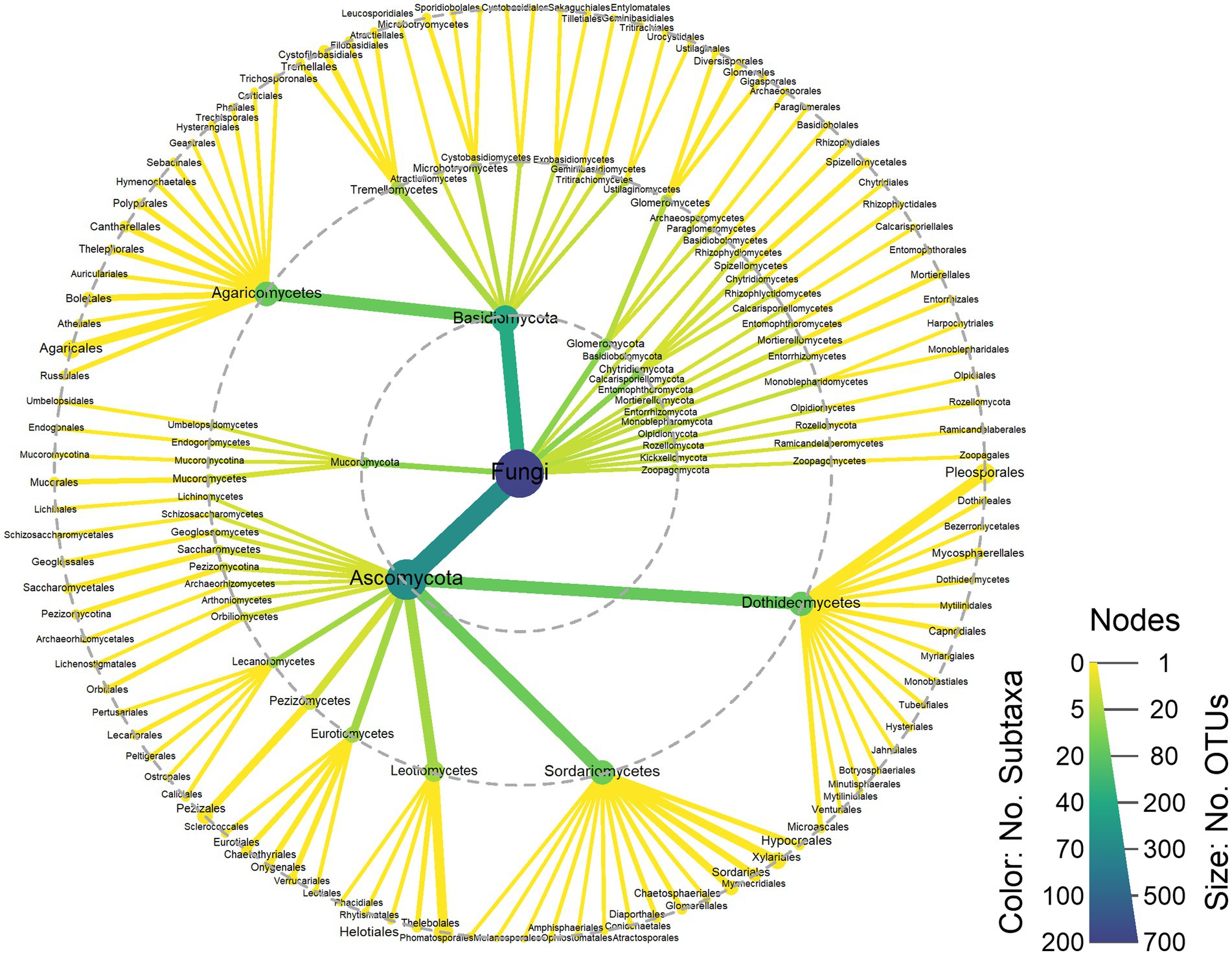
Figure 2. Taxonomic heat tree. Node size represents the number of OTUs and color scale represents the number of subtaxa. Dashed grey circles indicate the following taxonomic levels: phylum (inner), class (middle), order (outer). NA: not assigned.
To analyse the relationship between the presence/absence of certain fungal genera and forest health status, a comparison was made without considering the plant species. Only those genera that were present in at least 80% of the samples were taken into account. Twenty-two fungal genera (68.8%) were found common to healthy and declining trees soils, while six genera (18.8%) were only present in healthy trees soils and four genera (12.5%) were exclusive to declining forest soils. The genera found in healthy forest soils were Coleophoma, Geomyces, Podila, Sagenomella, Scytalidium, and Varicellaria. On the other hand, the fungal genera found in declining trees soils, which did not appear in samples of healthy trees, were Humicola, Meliniomyces, Pleurotus and Polyphilus (Figure 3).
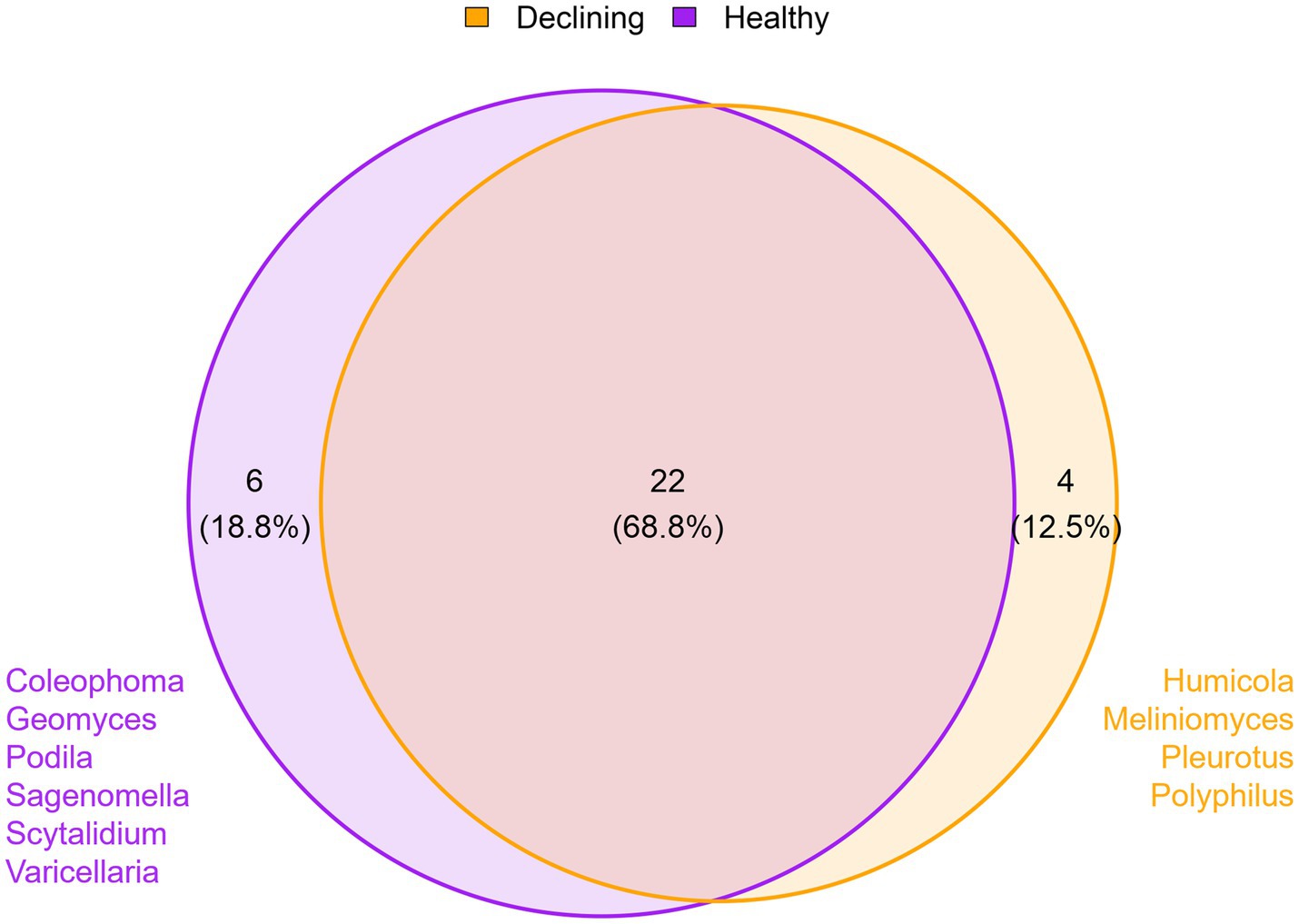
Figure 3. Overall effect of health status on mycobiota. Venn’s diagram showing the number of common and exclusive genera between samples from healthy (n = 12) and declining (n = 12) trees. Only genera present in 80% of samples per condition are included, regardless of tree species.
3.3. Diversity analysis
Diversity analysis was performed by comparing the effect of decline in the different tree species. In chestnut there was significantly higher alpha diversity in soil from healthy trees than in soil from declining trees. On the other hand, both cork oak and holm oak reported higher alpha diversity in soil from declining trees than in healthy trees; specifically, the diversity was significantly higher for dominant fungal genera in cork oak (Hill = −1) and for rare genera in holm oak (Hill = 1). In the case of Pyrenean oak, no significant differences were found between fungal genus diversity in soil from healthy and declining trees (Figure 4).
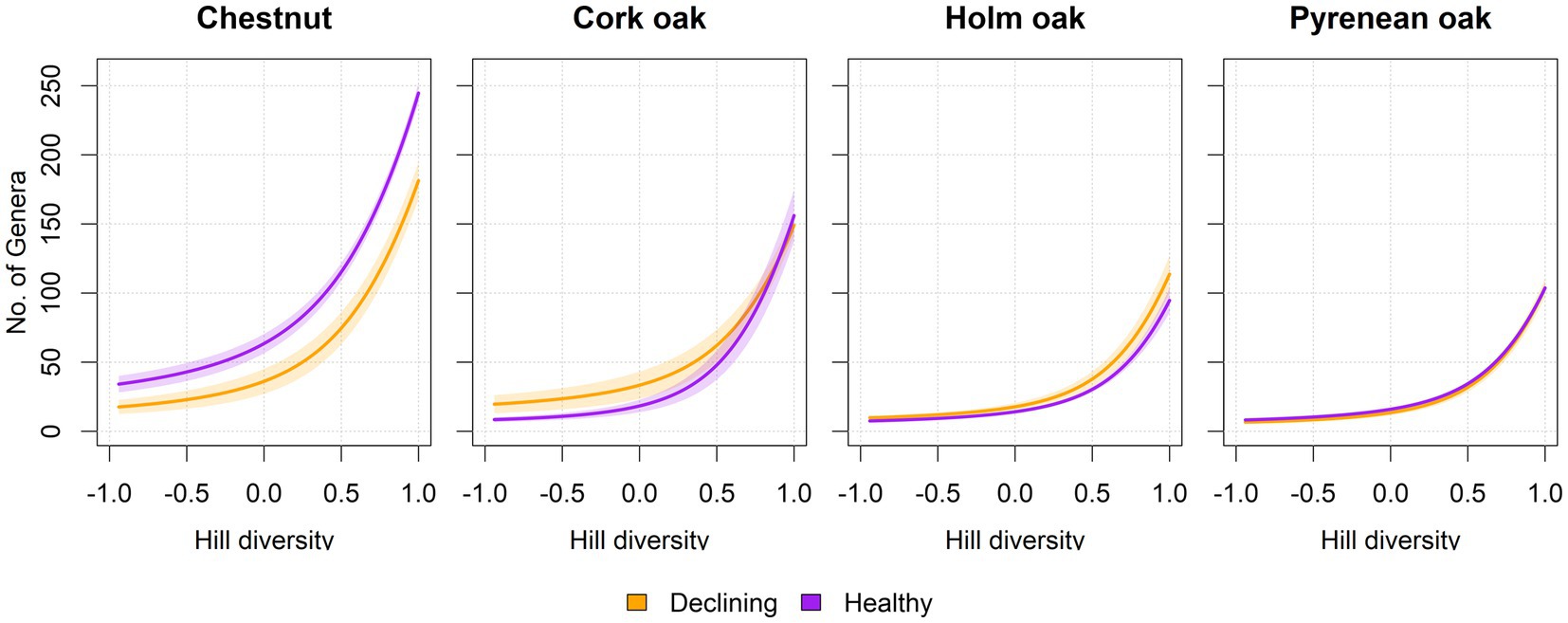
Figure 4. Diversity profiles per tree species and health status. Curves represent the average diversity in samples from healthy and declined trees in terms of an imaginary assemblage with that same diversity, but in which all species are equally abundant (Jost, 2006; Roswell et al., 2021). The horizontal axis represents the exponent l of Hill diversity, which can be interpreted as equivalence-corrected versions for richness (l = 1), Shannon (l = 0) and Simpson (l = −1) diversity estimators. Shadowed intervals correspond to standard error. Significant differences were found at l = [1, 0, −1] between declining and healthy chestnut soils (Wilcoxon test, n = 3 per tree species and health condition, p-val < 0.05). Raw curves prior to averaging can be found in Supplementary Figure S2.
Beta diversity was evaluated in terms of differential abundance (DA) of fungal compositions using square root-transformed values of raw reads. In cork oak, holm oak and Pyrenean oak, no fungal genus was found significantly more abundant in soils of declining trees, nor in healthy trees (Figure 5). Only the genus Metarhizium was found to be significantly more abundant in soils of healthy chestnuts (p-val = 0.1 and R2 = 0.2). However, no fungal genus was reported to be significantly more abundant in declining chestnut soils.
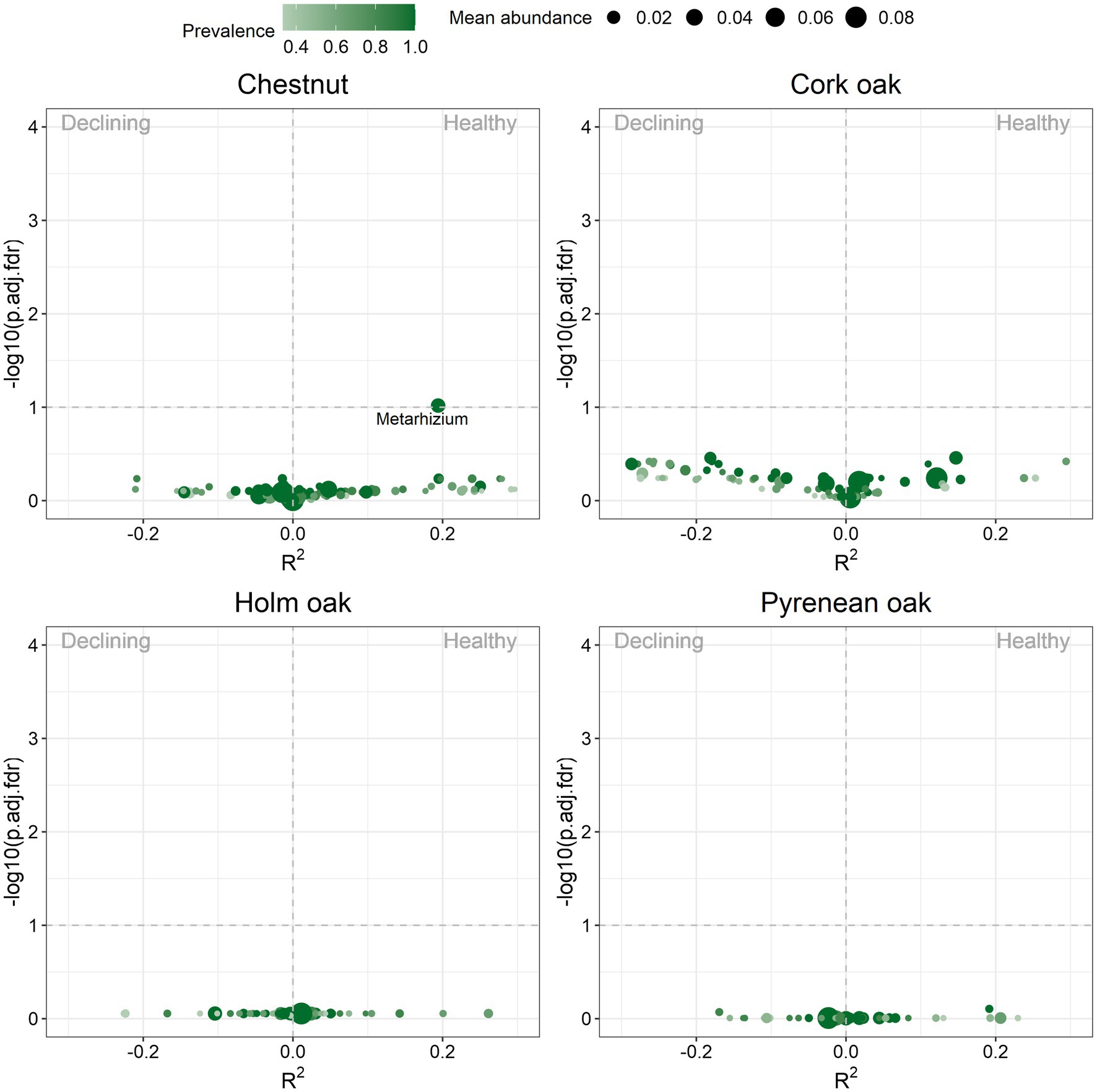
Figure 5. Volcano plot of differentially abundant genera detected by ZicoSeq analysis. Each genus is depicted by a dot. Dot size indicates the mean abundance across all samples and color indicates the proportion of samples in which the genus is present. Vertical axis represents value of p adjusted by false discovery rate (FDR) in logarithmic scale (1 = 0.1, 1.30 = 0.05, 2 = 0.01 and so on). Horizontal axis represents the strength of association between abundance and health condition, with the sign indicating the association direction (negative for declining, positive for healthy). Genera that surpass the horizontal dashed line are differentially abundant (p-val < 0.1).
3.4. Functional profiles
Primary lifestyle was assigned to each of the fungal genera reported in healthy and declining forest soils per tree species. Most prominent functional niches identified were ectomycorrhizae (EcM) and soil saprophytes, followed by litter/wood saprophytes and plant pathogens, regardless of tree species (Figure 6). Root endophytes were also particularly abundant in soil from holm oak and Pyrenean oak. When taking health status into account, the biggest differences were found in chestnuts and cork oaks, with holm oaks and Pyrenan oaks having similar functional profiles between healthy and declining trees’ soil. Fungal genera whose primary function is to form part of the lichen symbiosis were found to be more abundant in healthy chestnut and cork oak soils than in declining soils. Sooty mold fungi in chestnut soils followed the same trend. In cork oaks the largest differences corresponded to arbuscular mycorrhizae (AM), more present in declining than in healthy trees’ soil. Some lifestyles were absent from the majority of samples, such as moss symbiont, protistan parasites, epiphytes and algal parasites.
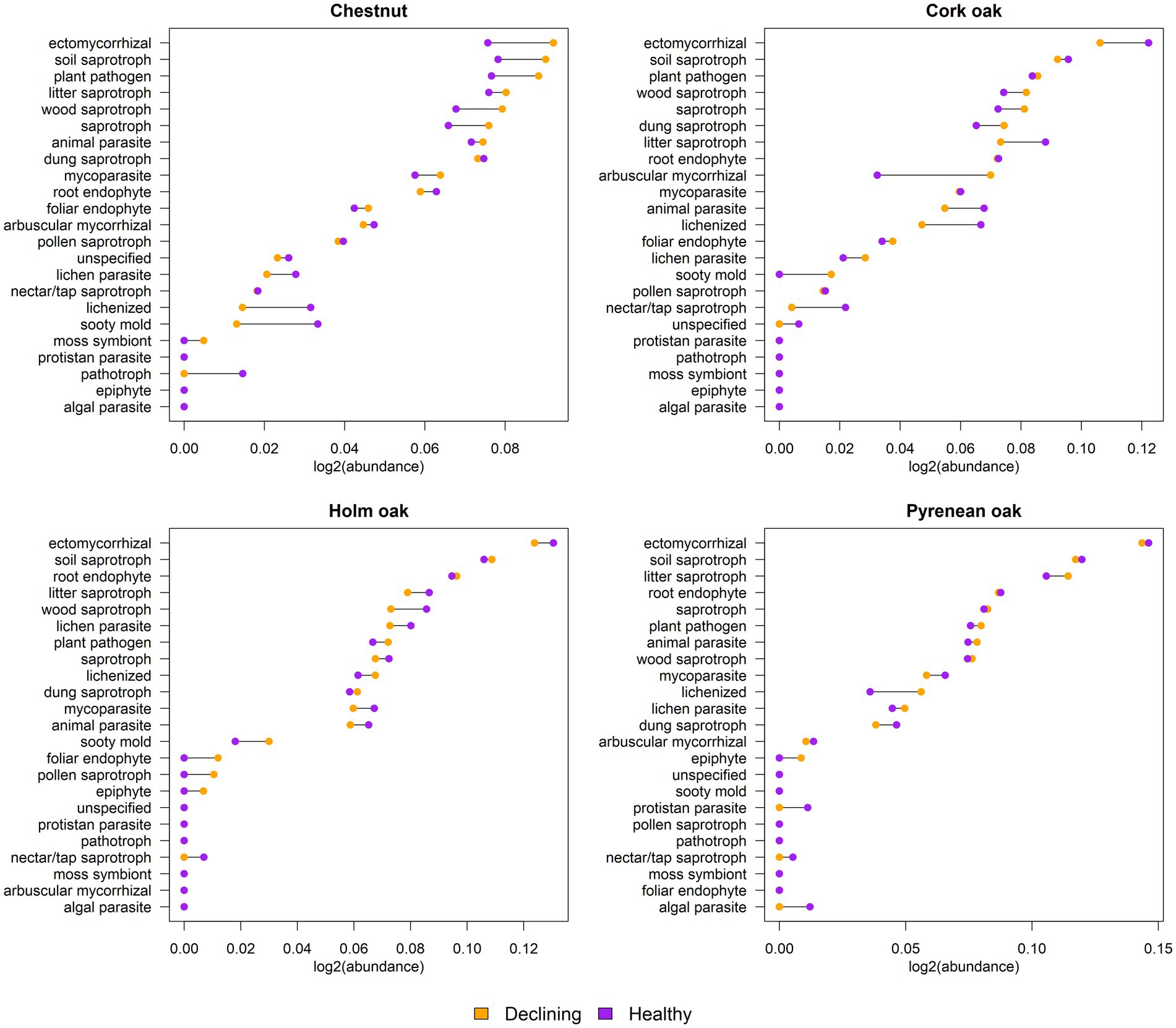
Figure 6. Comparison of functional guilds between declined and healthy samples. Horizontal axis represents sum of abundances expressed as log2 (guild abundance/total abundance). Connecting lines between two dots correspond to the difference between declined and healthy samples.
4. Discussion
Soil mycobiota play a key role in forest functioning and health (Fernandes et al., 2022). Therefore, knowing the fungal diversity of forest soils under different situations and health status is fundamental to understand the role that each component of this diversity plays in the sustainability of the ecosystem, being metabarcoding a proven tool for this purpose (Bowd et al., 2022). In our study, this methodology has allowed us to identify 674 different fungal genera as inhabitants of the rhizosphere of chestnuts, cork oaks, holm oaks and Pyrenean oaks.
Some fungal genera were only present in soils of declining trees. However, none of these genera has been previously described as a forest pathogen, which might be indicative of the soil conditions playing an important role as a predisposing or inciting factor of decline per se. Humicola is a genus of endophytic fungi of great biotechnological interest in recent years, mainly related to halophytic plants and production of chemical compounds for industrial use (Hosseyni-Moghaddam et al., 2020). Meliniomyces is a genus of endophytic fungi and ericoid mycorrhiza (Ohtaka and Narisawa, 2008; Vohnik et al., 2013). Pleorotus is a genus of widely known edible saprophytic fungus (Suwannarach et al., 2020; Doroški et al., 2022) which also includes biological control agents against nematodes (Singh et al., 2019), and its presence is most likely tied to clearings in declining areas. Polyphilus is a recently created fungal genus, which includes nematophagous fungi of eggs and cysts of plant-parasitic nematodes (Ashrafi et al., 2018). However, other important microbial communities with putative pathogenic species and capable of exerting direct and indirect effects on these forest species, such as bacteria, oomycetes or nematodes, have not been addressed here. Future work would be interesting in this regard, and could provide important information on their relationship with the health status of the trees as well as their impact on population dynamics of non-pathogenic species.
Similarly, we also found fungal genera exclusive to healthy forest soils, which could be related to control activity of different pathogens. Varicellaria is a fungal genus whose life form is algal symbiosis to form lichens (Schmitt et al., 2012) and could be related to the presence of more humidity due to better vegetative condition of the trees. A few species of Podila have been described as mycophilic fungi or mycoparasites (Telagathoti et al., 2022), but the absence of specific studies on their potential biocontrol effect prevents associating their presence with the absence of decline. Some genera found can act as animals’ pathogens, such as the genus Geomyces (Warnecke et al., 2012). The genus Sagenomella is widely known to cause systemic illness in animals (Gené et al., 2003), whereas the genus Scytalidium includes the causal agent of scytalidiosis and onychomycosis, human skin diseases (Elewski, 1996; Machouart et al., 2013). Interestingly, the genus Coleophoma, also absent from declining samples, has been widely described as a forest pathogen (Pehl and Wulf, 2003; Bianchinotti and Rajchenberg, 2004). Grazing activities that take place regularly in the sampling sites could offer an explanation to the presence of fungi pathogenic to animals, although their association with healthy trees soils would point to a preference of cattle for such areas that has not been explored.
Regarding biodiversity, a higher alpha diversity was observed in soils of healthy chestnuts than in declining trees. In this sense, other authors have previously described that the rhizosphere of chestnuts has a high diversity, even much higher than that of other forest ecosystems (Kelly et al., 2021). Moreover, this fungal diversity plays a key role in the resistance of trees against pathogens such as the oomycete Phytophthora cambivora (Venice et al., 2021). On the other hand, in both cork oak and holm oak, we found a higher fungal diversity in the rhizosphere of declining trees than in healthy ones. Therefore, a higher fungal diversity in these trees may not be related to the biological control of the decline, as has been described by other authors (Gómez-Aparicio et al., 2022). Finally, no differences in alpha diversity were reported between soils of healthy and declining Pyrenean oaks, a novel aspect that requires further studies. Differential abundance analysis indicated that the genus Metarhizium is more present in healthy chestnut soils than in declining soils. Metarhizium has been widely described as a biological control agent against plant pests and pathogens (Stone and Bidochka, 2020), which might be indicative of this genus playing a significant role in the absence of declining in the selected healthy chestnut stands. Influence of environmental drivers on biodiversity such as slope or elevation has not been evaluated and would be an important confusion factor to be considered in future works.
With respect to the assignment of primary lifestyles, most common functional niches found were EcM and soil saprophytes, very widespread and important functions in fungi that make up the mycobiota of all forest soils (Li et al., 2022). EcM fungi were dominated by some ubiquitous genera such as Cenococcum, Cortinarius, Inocybe, Laccaria, Russula and Tomenteria, with Russula being particularly more abundant in holm oak and Pyrenean oak soils regardless of health status (see complete list in Figure S5). In general, all oak species shared a predominance of EcM over other functional guilds. Inverse relationship between abundance of EcM and abundance of other guilds has been found before in oaks’ soils (Ruiz Gómez et al., 2019b). In contrast, chestnut showed evenness of EcM, saprotrophs and pathogens. We hypothesize that these differences in balance of functional guilds between oaks and chestnuts could be related to oaks’ soils being generally poorer and dryer and thus more prone to prevalence of EcM than chestnuts’ soils, where water is more readily available to the plant. The aforementioned guilds were also found to be more abundant in declining chestnut soils. Higher presence of opportunistic pathogens is to be expected in the rhizosphere of weakened trees, whereas saprobes might benefit from the excess of dead matter and leaf litter. Declining chestnuts could also have increased generation of roots as an attempt to compensate for compromised water transport, which in turn would allow for more colonization of EcM, however, this phenomenon was not observed in the oak species. Regarding other lifestyles, a significant proportion of AM belonging to 15 genera was found in chestnut and declining cork oak soils, whereas holm oak, Pyrenean oak and healthy cork oak soils showed a remarkable absence of AM (Supplementary Figure S5). In particular, presence of some genera such as Archaeospora, Entrophospora and Dominikia was tied to health status in cork oak soils, favoring declining samples. The persistence of AM has been reported in dying succulent trees in correlation with NO3− levels in soil (Vivas et al., 2018), although we found no differences in nitrogen compounds between healthy and declining cork oak soils in our samples.
This work describes the fungal soil mycobiota of four Mediterranean forest species and explores differences between healthy and declining stands. Healthy chestnut soils were characterized by higher alpha diversity and higher abundance of the genus Metarhizium, but such relationships were not found in the rest of the forest species included in the study. Finally, with respect to the functional profile, declining chestnut soils harbored more abundance of EcM, saprobic and pathogenic guilds than healthy samples. EcM were the dominant lifestyle in all oak species regardless of health status, whereas AM were preferentially found in healthy cork oak soils. Further experimental validations would be necessary to assess whether functional shifts in soil mycobiota are causally related to trees’ health status.
Data availability statement
The datasets presented in this study can be found in online repositories. The names of the repository/repositories and accession number(s) can be found at: https://www.ncbi.nlm.nih.gov/genbank/, PRJNA953856 https://github.com/serbiodh/2023_FrontiersForests_Soil, GitHub.
Author contributions
IB-A collected the data. SD-H analyzed the data. JP, SD-H, and JD wrote the manuscript. IB-A, JN-S, PM-P, AP, and JD designed the sampling scheme. All authors contributed to the article and approved the submitted version.
Funding
This work was supported by the LIFE project MYCORESTORE “Innovative use of mycological resources for resilient and productive mediterranean forests threatened by climate change, LIFE18 CCA/ES/001110,” and projects PID2019-110459RB-I00 and PLEC2021-008076 funded by the MICINN (Spain) as well as the project VA208P20 and the Strategic Research Program for Units of Excellence CLU-2019-05 (Unit of Excellence IRNASA/CSIC) both co-funded by the Junta de Castilla y León and European Union (ERDF “Europe drives our growth”).
Acknowledgments
The authors would like to thank Álvaro Benito Delgado, Cristina Zamora Ballesteros, and Mariano Rodríguez Rey for their valuable contributions to sampling, laboratory work and troubleshooting.
Conflict of interest
The authors declare that the research was conducted in the absence of any commercial or financial relationships that could be construed as a potential conflict of interest.
Publisher’s note
All claims expressed in this article are solely those of the authors and do not necessarily represent those of their affiliated organizations, or those of the publisher, the editors and the reviewers. Any product that may be evaluated in this article, or claim that may be made by its manufacturer, is not guaranteed or endorsed by the publisher.
Supplementary material
The Supplementary material for this article can be found online at: https://www.frontiersin.org/articles/10.3389/ffgc.2023.1215701/full#supplementary-material
References
Abarenkov, K., Zirk, A., Piirmann, T., Pöhönen, R., Ivanov, F., Nilsson, R. H., et al. (2022). UNITE general FASTA release for fungi. Version 16.10.2022. UNITE Commun. doi: 10.15156/BIO/2483911
Adamo, I., Castano, C., Bonet, J. A., Colinas, C., de Aragon, J. M., and Alday, J. G. (2021b). Soil physico-chemical properties have a greater effect on soil fungi than host species in Mediterranean pure and mixed pine forests. Soil Biol. Biochem. 160:108320. doi: 10.1016/j.soilbio.2021.108320
Adamo, I., Piñuela, Y., Bonet, J. A., Castaño, C., Martínez de Aragón, J., Parladé, J., et al. (2021a). Sampling forest soils to describe fungal diversity and composition. Which is the optimal sampling size in mediterranean pure and mixed pine oak forests? Fungal Biol. 125, 469–476. doi: 10.1016/j.funbio.2021.01.005
Akıllı, S., Serçe, Ç. U., Katırcıoğlu, Y. Z., and Maden, S. (2012). Involvement of phytophthora spp. in chestnut decline in the Black Sea region of Turkey. For. Pathol. 42, 377–386. doi: 10.1111/j.1439-0329.2012.00770.x
Ashrafi, S., Knapp, D. G., Blaudez, D., Chalot, M., Maciá-Vicente, J. G., Zagyva, I., et al. (2018). Inhabiting plant roots, nematodes, and truffles—Polyphilus, a new helotialean genus with two globally distributed species. Mycologia 110, 286–299. doi: 10.1080/00275514.2018.1448167
Baptista, P., Reis, F., Pereira, E., Tavares, R. M., Santos, P. M., Richard, F., et al. (2015). Soil DNA pyrosequencing and fruitbody surveys reveal contrasting diversity for various fungal ecological guilds in chestnut orchards. Environ. Microbiol. Rep. 7, 946–954. doi: 10.1111/1758-2229.12336
Bianchinotti, V., and Rajchenberg, M. (2004). Coleophoma gevuinae comb. nov., a foliar pathogen on Gevuina avellana (Proteaceae). Sydowia 56, 217–221.
Bowd, E. J., Banks, S. C., Bissett, A., May, T. W., and Lindenmayer, D. B. (2022). Disturbance alters the forest soil microbiome. Mol. Ecol. 31, 419–447. doi: 10.1111/mec.16242
Brill, B., Amir, A., and Heller, R. (2022). Testing for differential abundance in compositional counts data, with application to microbiome studies. Ann. Appl. Stat. 16, 2648–2671. doi: 10.1214/22-AOAS1607
Callahan, B. J., McMurdie, P. J., Rosen, M. J., Han, A. W., Johnson, A. J. A., and Holmes, S. P. (2016). DADA2: high-resolution sample inference from Illumina amplicon data. Nat. Methods 13, 581–583. doi: 10.1038/nmeth.3869
Camilo-Alves, C. S., Vaz, M., Da Clara, M. I. E., and Ribeiro, N. M. D. A. (2017). Chronic cork oak decline and water status: new insights. New For. 48, 753–772. doi: 10.1007/s11056-017-9595-3
Camponi, L., Cardelli, V., Cocco, S., Serrani, D., Salvucci, A., Cutini, A., et al. (2023). Holm oak (Quercus ilex L.) cover: a key soil-forming force in controlling C and nutrient stocks in long-time coppice-managed forests. J. Environ. Manag. 330:117181. doi: 10.1016/j.jenvman.2022.117181
Chen, J., Zhang, X., and Yang, L. (2022). GUniFrac: generalized UniFrac distances, distance-based multivariate methods and feature-based univariate methods for microbiome data analysis. R package version 1.7. Available at: https://CRAN.R-project.org/package=GUniFrac
Costa, D., Tavares, R. M., Baptista, P., and Lino-Neto, T. (2022). The influence of bioclimate on soil microbial communities of cork oak. BMC Microbiol. 22:163. doi: 10.1186/s12866-022-02574-2
Diez-Hermano, S., Ahmad, F., Niño-Sanchez, J., Benito, A., Hidalgo, E., Escudero, L. M., et al. (2022). Health condition and mycobiome diversity in Mediterranean tree species. Frontiers in Forests and Global Change 5:1056980. doi: 10.3389/ffgc.2022.1056980
Doroški, A., Klaus, A., Režek Jambrak, A., and Djekic, I. (2022). Food waste originated material as an alternative substrate used for the cultivation of oyster mushroom (Pleurotus ostreatus): a review. Sustainability 14:12509. doi: 10.3390/su141912509
Eichhorn, J., Roskams, P., Potočić, N., Timmermann, V., Ferretti, M., Mues, V., et al. (2016). “Part IV: visual assessment of crown condition and damaging agents. In: UNECE ICP forests programme coordinating Centre” in Manual on methods and criteria for harmonized sampling, assessment, monitoring and analysis of the effects of air pollution on forests (Eberswalde, Germany: Thünen Institute of Forest Ecosystems), 49.
El Ahmadi, S., Ramzi, H., Aafi, A., Jmii, N. E., and Aadel, T. (2022). Assessment of Cork oak decline using digital multispectral imagery in relation with in situ crown condition. Open Journal of Forestry 13, 145–160. doi: 10.4236/ojf.2023.131010
Elliott, K. J., and Swank, W. T. (2008). Long-term changes in forest composition and diversity following early logging (1919–1923) and the decline of American chestnut (Castanea dentata). Plant Ecol. 197, 155–172. doi: 10.1007/s11258-007-9352-3
Elewski, B. E. (1996). Onychomycosis caused by Scytalidium dimidiatum. J. Am. Acad. Dermatol. 35, 336–338. doi: 10.1016/S0190-9622(96)90664-7
Fady, B., Esposito, E., Abulaila, K., Aleksic, J. M., Alia, R., Alizoti, P., et al. (2022). Forest genetics research in the Mediterranean Basin: bibliometric analysis, knowledge gaps, and perspectives. Curr. Forest. Rep. 8, 277–298. doi: 10.1007/s40725-022-00169-8
Fernandes, M. L. P., Bastida, F., Jehmlich, N., Martinović, T., Větrovský, T., Baldrian, P., et al. (2022). Functional soil mycobiome across ecosystems. J. Proteome 252:104428. doi: 10.1016/j.jprot.2021.104428
Foster, Z., Sharpton, T., and Grünwald, N. (2017). Metacoder: an R package for visualization and manipulation of community taxonomic diversity data. PLoS Comput. Biol. 13, e1005404–e1005415. doi: 10.1371/journal.pcbi.1005404
Frisullo, S., Lima, G., Magnano di San Lio, G., Camele, I., Melissano, L., Puglisi, I., et al. (2018). Phytophthora cinnamomi involved in the decline of holm oak (Quercus ilex) stands in southern Italy. For. Sci. 64, 290–298. doi: 10.1093/forsci/fxx010
Fujita, S. I., Senda, Y., Nakaguchi, S., and Hashimoto, T. (2001). Multiplex PCR using internal transcribed spacer 1 and 2 regions for rapid detection and identification of yeast strains. J. Clin. Microbiol. 39, 3617–3622. doi: 10.1128/JCM.39.10.3617-3622.2001
García-Carmona, M., García-Orenes, F., Mataix-Solera, J., Roldán, A., Pereg, L., and Caravaca, F. (2021). Salvage logging alters microbial community structure and functioning after a wildfire in a Mediterranean forest. Appl. Soil Ecol. 168:104130. doi: 10.1016/j.apsoil.2021.104130
Garfì, V., Marziliano, P. A., Chirici, G., Nicolaci, A., and Iovino, F. (2022). Forest management scenarios to reduce the fire risk in chestnut coppices in the Mediterranean area. Ann. Silvicult. Res. 47, 63–77. doi: 10.12899/asr-2387
Gauquelin, T., Michon, G., Joffre, R., Duponnois, R., Génin, D., Fady, B., et al. (2018). Mediterranean forests, land use and climate change: a social-ecological perspective. Reg. Environ. Chang. 18, 623–636. doi: 10.1007/s10113-016-0994-3
Gené, J., Blanco, J. L., Cano, J., García, M. E., and Guarro, J. (2003). New filamentous fungus Sagenomella chlamydospora responsible for a disseminated infection in a dog. J. Clin. Microbiol. 41, 1722–1725. doi: 10.1128/JCM.41.4.1722-1725.2003
Gentilesca, T., Camarero, J. J., Colangelo, M., Nole, A., and Ripullone, F. (2017). Drought-induced oak decline in the western Mediterranean region: an overview on current evidences, mechanisms and management options to improve forest resilience. iForest-Biogeosci. Forest. 10, 796–806. doi: 10.3832/ifor2317-010
Gloor, G. B., Macklaim, J. M., Pawlowsky-Glahn, V., and Egozcue, J. J. (2017). Microbiome datasets are compositional: and this is not optional. Front. Microbiol. 8:2224. doi: 10.3389/fmicb.2017.02224
Gómez-Aparicio, L., Domínguez-Begines, J., Villa-Sanabria, E., García, L. V., and Muñoz-Pajares, A. J. (2022). Tree decline and mortality following pathogen invasion alters the diversity, composition and network structure of the soil microbiome. Soil Biol. Biochem. 166:108560. doi: 10.1016/j.soilbio.2022.108560
Hosseyni-Moghaddam, M. S., Safaie, N., Soltani, J., and Pasdaran, A. (2020). Endophytic association of bioactive and halotolerant Humicola fuscoatra with halophytic plants, and its capability of producing anthraquinone and anthranol derivatives. Antonie Van Leeuwenhoek 113, 279–291. doi: 10.1007/s10482-019-01336-x
Hu, Y. J., and Satten, G. A. (2020). Testing hypotheses about the microbiome using the linear decomposition model (LDM). Bioinformatics 36, 4106–4115. doi: 10.1093/bioinformatics/btaa260
Ihrmark, K., Bödeker, I. T., Cruz-Martinez, K., Friberg, H., Kubartova, A., Schenck, J., et al. (2012). New primers to amplify the fungal ITS2 region–evaluation by 454-sequencing of artificial and natural communities. FEMS Microbiol. Ecol. 82, 666–677. doi: 10.1111/j.1574-6941.2012.01437.x
Kelly, C. N., Schwaner, G. W., Cumming, J. R., and Driscoll, T. P. (2021). Metagenomic reconstruction of nitrogen and carbon cycling pathways in forest soil: influence of different hardwood tree species. Soil Biol. Biochem. 156:108226. doi: 10.1016/j.soilbio.2021.108226
Li, X., Qu, Z., Zhang, Y., Ge, Y., and Sun, H. (2022). Soil fungal community and potential function in different Forest ecosystems. Diversity 14:520. doi: 10.3390/d14070520
Limousin, J. M., Rambal, S., Ourcival, J. M., Rocheteau, A., Joffre, R., and Rodriguez-Cortina, R. (2009). Long-term transpiration change with rainfall decline in a Mediterranean Quercus ilex forest. Glob. Chang. Biol. 15, 2163–2175. doi: 10.1111/j.1365-2486.2009.01852.x
Machouart, M., Menir, P., Helenon, R., Quist, D., and Desbois, N. (2013). Scytalidium and scytalidiosis: what's new in 2012? J. de mycologie médicale 23, 40–46. doi: 10.1016/j.mycmed.2013.01.002
Maghnia, F. Z., Abbas, Y., Mahé, F., Prin, Y., El Ghachtouli, N., Duponnois, R., et al. (2019). The rhizosphere microbiome: a key component of sustainable cork oak forests in trouble. For. Ecol. Manag. 434, 29–39. doi: 10.1016/j.foreco.2018.12.002
Martin-Pinto, P., Sanz-Benito, I., Santos, M., Oria-de-Rueda, J. A., and Geml, J. (2021). Anthropological impacts determine the soil fungal distribution of Mediterranean oak stands. Ecol. Indic. 132:108343. doi: 10.1016/j.ecolind.2021.108343
Martín-Pinto, P., Dejene, T., Benucci, G. M. N., Mediavilla, O., Hernández-Rodríguez, M., Geml, J., et al. (2023). Co-responses of bacterial and fungal communities to fire management treatments in Mediterranean pyrophytic ecosystems. Sci. Total Environ. 875:162676. doi: 10.1016/j.scitotenv.2023.162676
Morán-Ordóñez, A., Ameztegui, A., De Cáceres, M., De-Miguel, S., Lefèvre, F., Brotons, L., et al. (2020). Future trade-offs and synergies among ecosystem services in Mediterranean forests under global change scenarios. Ecosyst. Serv. 45:101174. doi: 10.1016/j.ecoser.2020.101174
Morgan, M., Anders, S., Lawrence, M., Aboyoun, P., Pagès, H., and Gentleman, R. (2009). ShortRead: a bioconductor package for input, quality assessment and exploration of high-throughput sequence data. Bioinformatics 25, 2607–2608. doi: 10.1093/bioinformatics/btp450
Ohtaka, N., and Narisawa, K. (2008). Molecular characterization and endophytic nature of the root-associated fungus Meliniomyces variabilis (LtVB3). J. Gen. Plant Pathol. 74, 24–31. doi: 10.1007/s10327-007-0046-4
Pagès, H., Aboyoun, P., Gentleman, R., and DebRoy, S. (2022). Biostrings: efficient manipulation of biological strings. R package version 2.64.1. Available at: https://bioconductor.org/packages/Biostrings
Pehl, L., and Wulf, A. (2003). Coleophoma cylindrospora-a new needle fungus of Tsuga canadensis. Nachrichtenblatt des Deutschen Pflanzenschutzdienstes 55, 110–112.
Peñuelas, J., and Sardans, J. (2021). Global change and forest disturbances in the Mediterranean basin: breakthroughs, knowledge gaps, and recommendations. Forests 12:603. doi: 10.3390/f12050603
Perez-Luque, A. J., Benito, B. M., Bonet-Garcia, F. J., and Zamora, R. (2020). Ecological diversity within rear-edge: a case study from Mediterranean Quercus pyrenaica Willd. Forests 12:10. doi: 10.3390/f12010010
Põlme, S., Abarenkov, K., Henrik Nilsson, R., Lindahl, B. D., Clemmensen, K. E., Kauserud, H., et al. (2020). FungalTraits: a user-friendly traits database of fungi and fungus-like stramenopiles. Fung. Divers. 105, 1–16. doi: 10.1007/s13225-020-00466-2
R Core Team (2022). R: A Language and Environment for Statistical Computing. Vienna: R Foundation for Statistical Computing.
Roswell, M., and Dushoff, J. (2022). MeanRarity: hill diversity estimation and visualisation. R package version 0.0.1.0004. Available at: https://github.com/mikeroswell/MeanRarity
Roswell, M., Dushoff, J., and Winfree, R. (2021). A conceptual guide to measuring species diversity. Oikos 130, 321–338. doi: 10.1111/oik.07202
Ruiz-Gómez, F. J., Pérez-de-Luque, A., and Navarro-Cerrillo, R. M. (2019a). The involvement of phytophthora root rot and drought stress in holm oak decline: from ecophysiology to microbiome influence. Curr. Forest. Rep. 5, 251–266. doi: 10.1007/s40725-019-00105-3
Ruiz Gómez, F. J., Navarro-Cerrillo, R. M., Pérez-de-Luque, A., Oβwald, W., Vannini, A., and Morales-Rodríguez, C. (2019b). Assessment of functional and structural changes of soil fungal and oomycete communities in holm oak declined dehesas through metabarcoding analysis. Sci. Rep. 9, 5315–5316. doi: 10.1038/s41598-019-41804-y
Schmitt, I., Otte, J., Parnmen, S., Sadowska-Deś, A., Luecking, R., and Lumbsch, T. (2012). A new circumscription of the genus Varicellaria (Pertusariales, Ascomycota). MycoKeys 4, 23–36. doi: 10.3897/mycokeys.4.3545
Singh, R. K., Pandey, S. K., Singh, D., and Masurkar, P. (2019). First report of edible mushroom Pleurotus ostreatus from India with potential to kill plant parasitic nematodes. Indian Phytopathol. 72, 173–176. doi: 10.1007/s42360-018-0093-0
Smahi, H., Belhoucine-Guezouli, L., Franceschini, A., and Scanu, B. (2017). Phytophthora species associated with cork oak decline in a Mediterranean forest in western Algeria. Int. Protect. Oak Forest. IOBC-WPRS Bullet. 127, 123–129.
Stone, L. B., and Bidochka, M. J. (2020). The multifunctional lifestyles of Metarhizium: evolution and applications. Appl. Microbiol. Biotechnol. 104, 9935–9945. doi: 10.1007/s00253-020-10968-3
Suwannarach, N., Kumla, J., Satienperakul, K., Sungpalee, W., Sri-Ngernyuang, K., and Lumyong, S. (2020). Pleurotus sirindhorniae (Pleurotaceae, Agaricales), a new species from northern Thailand. Phytotaxa 460, 285–295. doi: 10.11646/phytotaxa.460.4.6
Tedersoo, L., Mikryukov, V., Anslan, S., Bahram, M., Khalid, A. N., Corrales, A., et al. (2021). The global soil mycobiome consortium dataset for boosting fungal diversity research. Fungal Divers. 111, 573–588. doi: 10.1007/s13225-021-00493-7
Telagathoti, A., Probst, M., Mandolini, E., and Peintner, U. (2022). Mortierellaceae from subalpine and alpine habitats: new species of Entomortierella, Linnemannia, Mortierella, Podila and Tyroliella gen. Nov. Stud. Mycol. 103, 25–58. doi: 10.3114/sim.2022.103.02
Venice, F., Vizzini, A., Frascella, A., Emiliani, G., Danti, R., Della Rocca, G., et al. (2021). Localized reshaping of the fungal community in response to a forest fungal pathogen reveals resilience of Mediterranean mycobiota. Sci. Total Environ. 800:149582. doi: 10.1016/j.scitotenv.2021.149582
Vettraino, A. M., Morel, O., Perlerou, C., Robin, C., Diamandis, S., and Vannini, A. (2005). Occurrence and distribution of phytophthora species in European chestnut stands, and their association with ink disease and crown decline. Eur. J. Plant Pathol. 111, 169–180. doi: 10.1007/s10658-004-1882-0
Vivas, M., Crous, C. J., Dames, J. F., van der Linde, J. A., Coetzee, M. P. A., and Roux, J. (2018). Arbuscular mycorrhizal fungi persist in dying Euphorbia ingens trees. S. Afr. J. Bot. 115, 12–17. doi: 10.1016/j.sajb.2017.12.009
Vohnik, M., Mrnka, L., Lukešová, T., Bruzone, M. C., Kohout, P., and Fehrer, J. (2013). The cultivable endophytic community of Norway spruce ectomycorrhizas from microhabitats lacking ericaceous hosts is dominated by ericoid mycorrhizal Meliniomyces variabilis. Fungal Ecol. 6, 281–292. doi: 10.1016/j.funeco.2013.03.006
Waldboth, M., and Oberhuber, W. (2009). Synergistic effect of drought and chestnut blight (Cryphonectria parasitica) on growth decline of European chestnut (Castanea sativa). For. Pathol. 39, 43–55. doi: 10.1111/j.1439-0329.2008.00562.x
Wardle, D. A., and Lindahl, B. D. (2014). Disentangling global soil fungal diversity. Science 346, 1052–1053. doi: 10.1126/science.aaa1185
Warnecke, L., Turner, J. M., Bollinger, T. K., Lorch, J. M., Misra, V., Cryan, P. M., et al. (2012). Inoculation of bats with European Geomyces destructans supports the novel pathogen hypothesis for the origin of white-nose syndrome. Proc. Natl. Acad. Sci. 109, 6999–7003. doi: 10.1073/pnas.1200374109
Yang, L., and Chen, J. (2022). A comprehensive evaluation of microbial differential abundance analysis methods: current status and potential solutions. Microbiome 10:130. doi: 10.1186/s40168-022-01320-0
Yang, R. H., Su, J. H., Shang, J. J., Wu, Y. Y., Li, Y., Bao, D. P., et al. (2018). Evaluation of the ribosomal DNA internal transcribed spacer (ITS), specifically ITS1 and ITS2, for the analysis of fungal diversity by deep sequencing. PLoS One 13:e0206428. doi: 10.1371/journal.pone.0206428
Keywords: global change, forest declines, metabarcoding, biodiversity, forest pathology, Quercus , Castanea
Citation: Diez-Hermano S, Poveda J, Niño-Sanchez J, Bocos-Asenjo IT, Peix &, Martín-Pinto P and Diez JJ (2023) Rhizosphere mycobiome diversity in four declining Mediterranean tree species. Front. For. Glob. Change. 6:1215701. doi: 10.3389/ffgc.2023.1215701
Edited by:
Salvatore Moricca, University of Florence, ItalyReviewed by:
Francisco J. Ruiz-Gomez, University of Cordoba, SpainFederico Sebastiani, National Research Council, Italy
Copyright © 2023 Diez-Hermano, Poveda, Niño-Sanchez, Bocos-Asenjo, Peix, Martín-Pinto and Diez. This is an open-access article distributed under the terms of the Creative Commons Attribution License (CC BY). The use, distribution or reproduction in other forums is permitted, provided the original author(s) and the copyright owner(s) are credited and that the original publication in this journal is cited, in accordance with accepted academic practice. No use, distribution or reproduction is permitted which does not comply with these terms.
*Correspondence: Sergio Diez-Hermano, sergio.diez.hermano@uva.es; Julio Javier Diez, JulioJavier.Diez@uva.es
†These authors have contributed equally to this work